- 1East China Sea Fisheries Research Institute, Chinese Academy of Fishery Sciences, Shanghai, China
- 2Key Laboratory of East China Sea Fishery Resources Exploitation and Utilization, Ministry of Agriculture and Rural Affairs, Shanghai, China
In the year of 2016 to 2017, record-breaking marine heatwave (MHW) events occurred along the Chinese coast, exerting severe impacts on coastal fishes and fisheries. However, the response of the nekton community to MHW events still remains poorly understanding. Thus, to investigate the influences of the MHW events on species composition dynamics, this study conducted a survey on nekton communities in the northern East China Sea in the summers during 2014 to 2017. The recently-developed “heatwaveR” package was adopted to describe the variation characteristics of heatwaves within the study area, based on high-resolution remote-sensing sea surface temperature products. We also compared variations in the community structures of different biological groups before (2014–2015) and during the occurrence of the MHW events (2016–2017). During the MHW events, temperature increased by approximately 2.3°C in both 2016 and 2017. Compared with those before the MHW events, the biomass and dominance of several important crustaceans decreased, and the biomass of warm water affinity species increased significantly, while their richness and diversity decreased; the biomass of warm temperature affinity species declined significantly, while their diversity increased slightly. These results show the difference in the response rates of species with different thermal preferences and different species compositions to the extreme climate change. Beta diversity analysis revealed that the inter-group variation before and during the MHW event exceeded the intra-group variation, proving that MHWs changed the community structure to a certain extent. PERMANOVA test showed that the species compositions of planktivorous fish (FG1) and small-omnivorous fish (FG2) in the feeding-functional groups were significantly different before and during the MHW event, which may be attributed to the impact of MHWs on oligotrophic fishes through thermal preference and the trophic cascade effect. There was no significant change in the species compositions of other functional groups, indicating that medium-trophic and eutrophic fishes possessed certain adaptability to MHWs. Regarding fisheries management under extreme warming events, it would be advisable to diagnose temperature anomalies in the early stage, monitor changes in species abundance in the early life cycle, and then rapidly develop suitable fishing strategies.
Introduction
Under global climate change, extreme weather and climate events, as major causes of disasters and disaster risk sources, have attracted increasing attention (Parmesan et al., 2000; Ummenhofer and Meehl, 2017; Harris et al., 2020). Marine heatwaves (MHWs) are extreme climate events caused by the coupling of the atmosphere and sea water. They are characterized by prolonged periods of anomalously warm ocean temperatures, and they affect coastal marine ecosystems worldwide. The frequency, duration, and intensity of MHWs have been reported to be increasing over the past decades in the most parts of the world (Frölicher et al., 2018; Oliver, 2019; Guo et al., 2022). Moreover, future projections indicate the number of MHW days will increase by several orders of magnitude by the end of the century even under scenarios of strong greenhouse gas emissions mitigation (Gupta et al., 2020; Plecha and Soares, 2020).
Some cases of recently observed MHWs revealed the high vulnerability of marine ecosystems and fisheries to such extreme climate events (Garrabou et al., 2009; Marbà and Duarte, 2010; Mills et al., 2013; Caputi et al., 2016; Wernberg et al., 2016). MHWs can alter the structure and functions of entire ecosystems by inducing widespread mortality, species range shifts, and community reconfiguration (Garrabou et al., 2009; Smale and Wernberg, 2013; Wernberg et al., 2013; Smale et al., 2019). Some patterns of response during the MHWs emerged were detected across taxa and food webs of various biological communities covered nearshore intertidal to offshore oceanic domains (Smale et al., 2019). Many ecologically and commercially important marine species respond to environmental disruptions by relocating to favourable habitats, and dramatic range shifts of mobile marine species are among the conspicuous impacts of MHWs (Jacox et al., 2020), for example, a 2012 marine heatwave in the northwest Atlantic pushed commercial species such as squid and flounder hundreds of miles northward (Mills et al., 2013). Given anticipated increases in marine heatwaves under current climate projections, it remains uncertain when or if the ecosystem will return to a pre-MHW state (Suryan et al., 2021).
Research shows that sea surface temperature (SST) exhibits a significant warming trend in the waters of China, and its rate of increase is far higher than the global average (Wu et al., 2012; Wang et al., 2021a). In July and August 2017, exceptionally warm SST anomalies (SSTAs) were observed in the northern Yellow Sea, which lasted for 60 days with a maximum daily SSTA of 2.93°C on the date of the peak. An MHW event was considered as one of the most important factors leading to the mass emaciation and mortality of scallops in Zhangzi Island (Li et al., 2019, Wang et al., 2021b). The East China Sea (ECS) is a marginal sea in the Western Pacific Ocean. As the most important current affecting ECS, the Kuroshio changes SST by weakening the East Asian monsoon and strengthening warm water intrusion. In the past few years, the frequency, duration, and intensity of MHWs showed a significant upward trend in ECS (Tan and Cai, 2018; Yao et al., 2020). Wu et al. (2012) reported that the rate of SST increase associated with western boundary currents (such as the Kuroshio) is 2–3 times that of the global average. At a local scale, during 1958–2014, SST in ECS increased by ~0.86°C at a rate of 0.15°C per decade during summer (Cai et al., 2017; Yao et al., 2020). In August 2016, a record-breaking MHW event occurred in ECS, which had a serious impact on China’s fisheries and aquaculture industry (Tan and Cai, 2018). Schlegel et al. (2017) revealed that MHWs in coastal waters could be even more harmful to shallow water ecosystems than to marine ecosystems in open oceans. Moreover, shallow water ecosystems are also the most seriously disturbed by human activities (Lopez et al., 2006; Halpern et al., 2008; Johnston et al., 2022). Under the present warming trend influenced by anthropogenic forcing, the frequency and duration of MHWs are predicted to increase in the next few years. Therefore, it is important to explore the community and ecosystem response to MHW events in order to improve adaptive strategies for fisheries management.
Thus far, fisheries research has mainly focused on the trends of species response to long-term climate change; however, discrete extreme events are emerging as pivotal factors shaping ecosystems, by driving sudden and dramatic shifts in ecological structure and functioning (Smale et al., 2019). To address this shortcoming, this study investigated the responses of species composition to the 2016–2017 MHW events in the ECS. Considering different ecological types and functional groups, the following objectives were set: (1) Estimate changes in the compositions and structures of offshore nekton in ECS in response to the MHW event in 2016 and 2017. (2) Determine the characteristics of these possible changes. (3) Identify differences in the responses of different ecological types and functional groups, and their potential influences. Using scientific survey data of independent fisheries from 2014 to 2017, variations in the community structure of nekton during periods of high and low SST were comparatively analyzed, focusing on the change patterns and local differences of alpha and beta diversities. This study expounds hot scientific issues not only in the domain of ecosystem stability research, but also reveal potential risk between extreme climate and fishery output capacity. The findings are expected to provide essential scientific basis for offshore fisheries management in response to sudden climate change.
Materials and methods
Study area and in-situ survey
The study area is located in the offshore waters of the northern ECS (30–33°N) (Figure 1), which are shallow marginal seas adjacent to the northwest Pacific Ocean (Liu et al., 2017). The boundary, roughly a line from Cheju Island to the mouth of the Yangtze River (Chiang Jiang) on China’s eastern coast, separates ECS from the Yellow Sea. The shallow shelf areas in the southern Yellow sea are covered by sediments from the bordering landmasses deposited mainly by the Yangtze and other rivers near the northern part of the Yangtze Estuary. There are many islands in the south of the Yangtze River Estuary. The Zhoushan Archipelago, the largest archipelago in China, is located in the northwestern part of ECS. The study area is one of the most productive marine areas in the world (Tian et al., 1993; Chen et al., 1999; Matsuzaki et al., 2019). The enhanced SST warming in this area is attributable to the weakening of the East Asian Monsoon and intensification of the Kuroshio intrusion (Cai et al., 2011; Sasaki and Umeda, 2021).
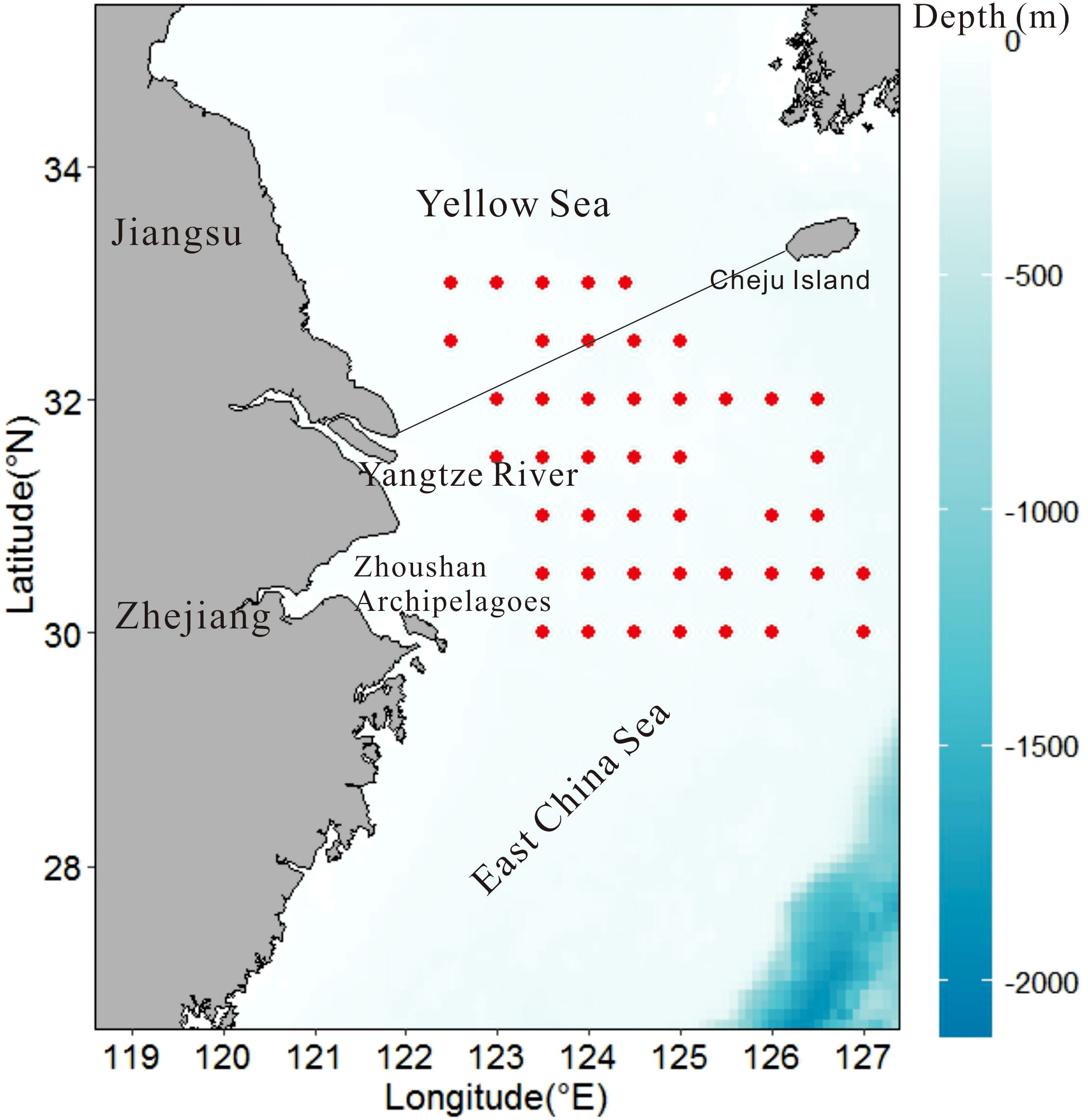
Figure 1 Sample locations for demersal trawl surveys for fish stocks in the northern East China Sea. Color bar indicates water depth.
We collected data from on-going scientific survey programs over wide areas of ECS. The surveys provide the most spatially intensive and yearly representative marine dataset. Since August 2000, the East China Sea Fisheries Research Institute has been continuously carrying out scientific otter-trawl surveys over a broad area of ECS (26°30’N–33°00’N) from the coast to the continental shelf (20–150 m depth) using fisheries vessels (220 kw). A standard bottom trawling gear (4 m × 100 mesh, cod-end mesh size 20 mm) and sampling protocol were used. Trawling speed was approximately 3 knots and the duration of each tow was approximately 60 min. During these surveys, on-board researchers recorded the survey date, location, catch weights, and sample weights. In the laboratory, all marine organisms were classified into species, and the abundance and biomass for each species were standardized to the number (individuals/h) and weight (kg/h) (catch per unit effort, CPUE) as a measure of relative fish abundance. We limited our data to four consecutive years from 2014 to 2017, and selected 45 sites with available data for each year. Considering the occurrence of MHWs events in 2016 and 2017, 2014 and 2015 were taken as normal years (NY). The availability of continuous data for consecutive years facilitated the comparison and analysis of changes in community structure before and during the MHWs.
Ecological types
According to taxonomic affiliation, nektons were divided into fish, crustaceans, and cephalopods. Fish included bony and cartilaginous fish (such as ray, shark and skate). Cephalopods included cuttlefish, octopus, and squid. Crustaceans included shrimps, crabs, and others.
Thermal conditions are expected to directly constrain species distribution (Freitas et al., 2021). Changes in thermal conditions may promote fish migration for habitat selection, thereby changing the community structure. According to their thermal preference for habitats, the fish were then divided into warm water (WW) species, warm temperature (WT) species, cold temperature (CT) species, and eurythermic (EU) species. Cold water species were not observed during the investigation.
Predation is a key process through which energy and nutrients are transported between organisms of different trophic levels (Mihalitsis and Bellwood, 2021). To distinguish the response of different trophic species to MHW events, we introduced the concept of resource function group (FG), which helps in focusing on the same domain competitive species and functional specialization groups. FG is a collection of species that overlap significantly in their niche requirements but are not taxonomically related (Root, 1967; Muñoz and Ojeda, 1997). In this study, the fish species in the community were divided into 6 feeding functional groups based on the results of previous studies on feeding preferences (Zhang et al., 2007; Jiang et al., 2008), namely planktivores (FG1), small omnivores (FG2), piscivores (FG3), benthivores/piscivores (FG4), benthivores (FG5), and predatory fish (FG6).
Heatwaves event detection
MHW events were detected according to the definition provided by HobdayaLis et al. (2016), where an MHW is an anomalous warming event lasting for at least 5 days with daily temperatures higher than the 90th percentile over a 30‐year historical baseline period (HobdayaLis et al., 2016; Yao et al., 2020). The “heatwaveR” package in R software has been made available for the automatic detection and statistical descriptions of MHW events. In this paper, surface temperature during 1982–2011 is defined as the baseline temperature climatology, using all data within an 11-day window centered on the time of year. The extracted area, ranged from 30 to 33°N latitude and from coastline to 127°E longitude. MHW events were detected using high-resolution (1/4), global, daily SSTs from the Advanced Very High Resolution Radiometer satellite data (NOAA OISST V2) (Reynolds et al., 2007). A set of metrics can be derived for each MHW, including maximum intensity, mean intensity, cumulative intensity, and duration. However, monthly satellite data with higher resolution (0.083° × 0.083°) from CMEMS are used to quantify and map annual variability of sea surface temperature (Liu et al., 2022).
Analysis methods
The index of relative importance (IRI) was used to determine the relative importance of each species in the community (Hart et al., 2002). IRI is a composite measure that simultaneously combines individual number (N), weight (W), and frequency of occurrence (F) to evaluate ecological importance. It can be computed as follows:
where N% is the numerical proportion of each species in the community; W% is the weight proportion of each species in the community; F% is the relative frequency of occurrence of each species. The dominant species were determined according to IRI values of the top species (Li and Kang, 2020). In this study, species with IRI >500 were grouped into dominant groups and species with values of 100–500 into common groups.
We quantified the alpha diversity at each site with the Shannon diversity index and Chao1 richness index to explore the community pattern between NY and MHW years at the ecological group levels. The Shannon diversity metric is commonly used in ecological studies (Morris et al., 2014), and it accounts for both the abundance and evenness of species present in an area (Inyang and Wang, 2020). The higher the value of H, the higher the diversity of species in a particular community. Chao1 is a measure of species richness based on abundances (counts) at a single sample site, but it is sensitive to rare species (Oksanen et al., 2022). The Shannon diversity and Chao1 richness are calculated as follows:
where pi is the proportional biomass of species i; ln is the natural log; S0 is the observed number of species in the collection; a1 and a2 are the number of species occurring only at one or only at two sites in the collection; N is the number of sites in the collection.
We conducted an analysis of variance (ANOVA) on biomass to test possible differences between the two nekton communities (before and during MHWs) for each ecological group. CPUE between NY and MHW years was compared using the non-parametric Kruskal–Wallis Test. Species richness and diversity indices were applied with the Tukey test. We tested the dissimilarity among groups (NY-MHWs) using a permutational multivariate analysis of variance (PERMANOVA) in the vegan package (Oksanen et al., 2022). The distance of the Bray–Curtis similarity coefficient was calculated on the basis of the relative abundance matrices. The null hypothesis of this test is that the metric centroid does not differ between groups. As this test is sensitive to data dispersion and may therefore have difficulty distinguishing between within-group variation and among-group variation (Anderson, 2001; Lemieux-Labonté et al., 2016), the “betadisper” procedure was performed for the analysis of the multivariate homogeneity of group dispersions (variances), which was used to identify potential significant differences caused by different within-group variations (dispersion) (Anderson, 2001). For beta-dispersion with non-significant differences, visualization was performed by applying classical multidimensional scaling (MDS). Subsequently, a similarity of percentage analysis (SIMPER) was applied to estimate the contribution of a particular species to similarities and dissimilarities in the overall nekton community between NY and MHW years (Clarke and Warwick, 2001). To examine whether temporal beta-diversity was greater for NY than for MHWs, the beta-diversity between each site was compared using both pairwise and multiple-time temporal beta-diversity frameworks; the overall change was indicated using the Sørensen dissimilarity with the “betapart” package in R (Baselga et al., 2022).
We performed all analyses using R 4.1.3 (R Core Team, 2022). ANOVA was performed using the emmeans (Lenth, 2022) and multcomp (Hothorn et al., 2008) packages. Visualization was performed using the ggplot2 (Wickham, 2016), ggpubr (Kassambara, 2020), and heatwaveR packages (Schlegel and Smit, 2018).
Results
The situations of marine environment
The East China Sea experienced a significant warming event in the summer and autumn of 2016–2017. During this anomaly period, SST reached more than 2.5°C higher than the climatological values. The mean monthly SST was 29.2°C in the August of 2016–2017. Compared with the preceding 2 years, SST increased by 2.3°C (Figure 2). The warming event was driven by the strengthening of the Taiwan warm current and the transfer of warm tropical water northwestwards.
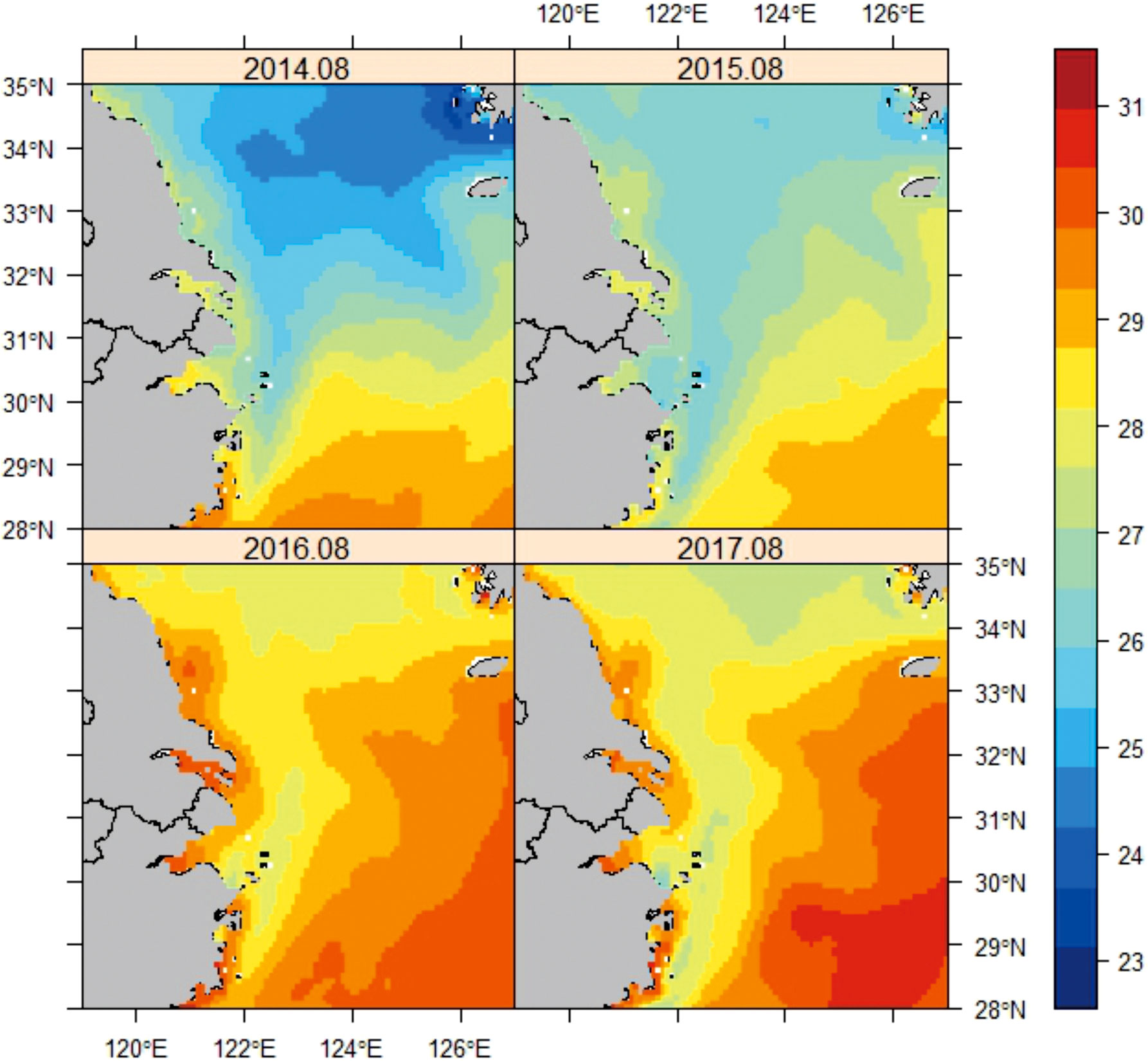
Figure 2 Mean monthly sea-surface temperature (SST) based on the 0.083° resolution extracted from the CMEMS dataset for August.
The statistics of MHWs in the study area are presented for 2014 to 2017. Figure 3 shows the duration (Figure 3A), maximum intensities (Figure 3B), and cumulative intensities (Figure 3C) of MHWs. Only two MHW events occurred during 2014–2015 (Figure 3, Figure 4A), with considerably short durations (less than 7 days) and low maximum intensity (1.7°C). In contrast, the frequency of MHWs increased to over 10 events between 2016 and 2017 (Figure 3, Figure 4B). Most of the detected MHW events were found to occur in summer and autumn (Figure 4B). In particular, the MHW frequency was the highest in 2017, but the MHW events in 2016 showed the maximum duration, maximum intensity, and maximum cumulative intensity. In the summer, the first two MHW events that lasted over 35 days occurred from July–August, with the maximum intensity reaching 2.8°C higher than the climatological values.
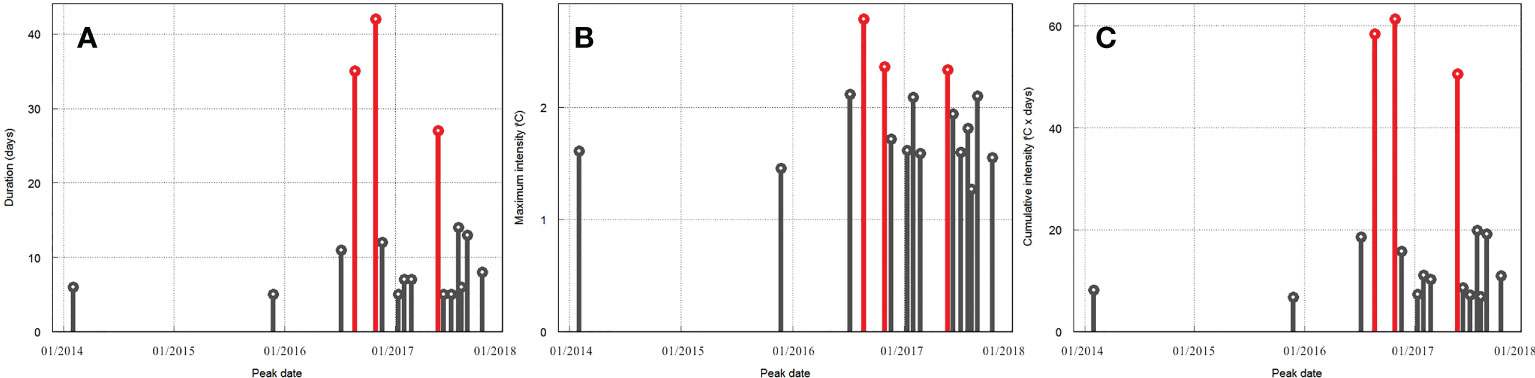
Figure 3 Mean MHW (A) duration, (B) maximum intensity, and (C) cumulative intensity in the study area from 2014 to 2017. The top three events were highlighted in red colour.
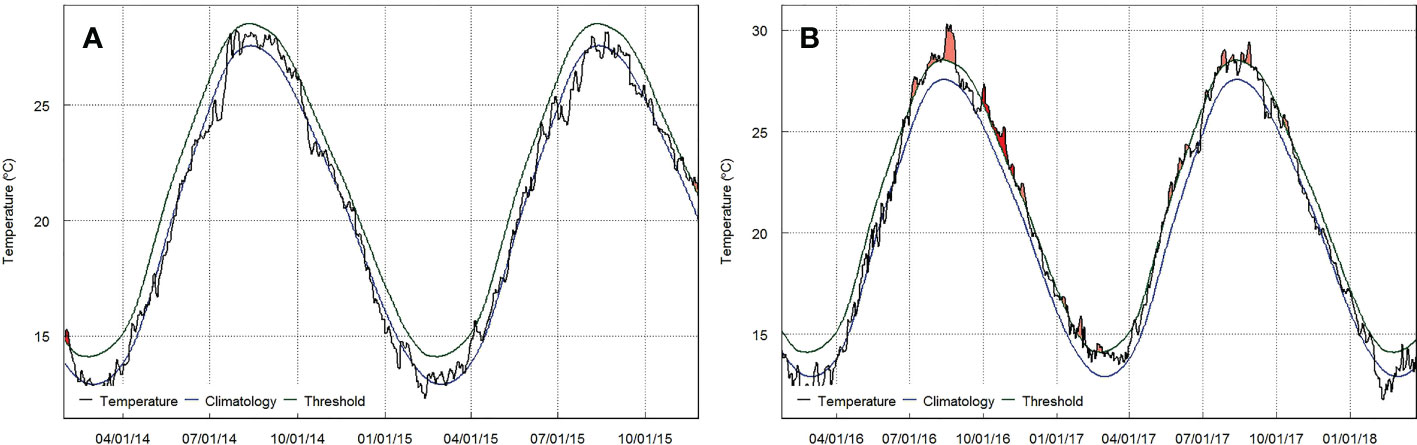
Figure 4 Event line for detected marine heatwaves at the study area during (A) 2014–2015 and (B) 2016–2017 (red filled area is the marine heatwaves evens of interest in this study). The blue line, green line, and black line represent SST climatology, 90th percentile MHW threshold, and SST time series.
Species composition and dominance of nekton communities
Figure 5 shows the species composition among the taxonomic classification, thermal preference, and functional groups. In total, 194 species were collected from the 45 sampling sites; the number of species in 2014–2017 was 110, 117, 102, and 119, respectively. The most species-rich taxonomic group was fish, followed by shrimps, cephalopods, and crabs. Of these, most fish, shrimps, cephalopods, and crabs belong to WW species in terms of their thermal preference, followed by WT species. According to the classification of feeding functional groups, FG1 and FG2 were dominated by WW species, while the proportion of WT species in FG3, FG4, and FG5 showed a small increase trend compared with FG1 and FG2.
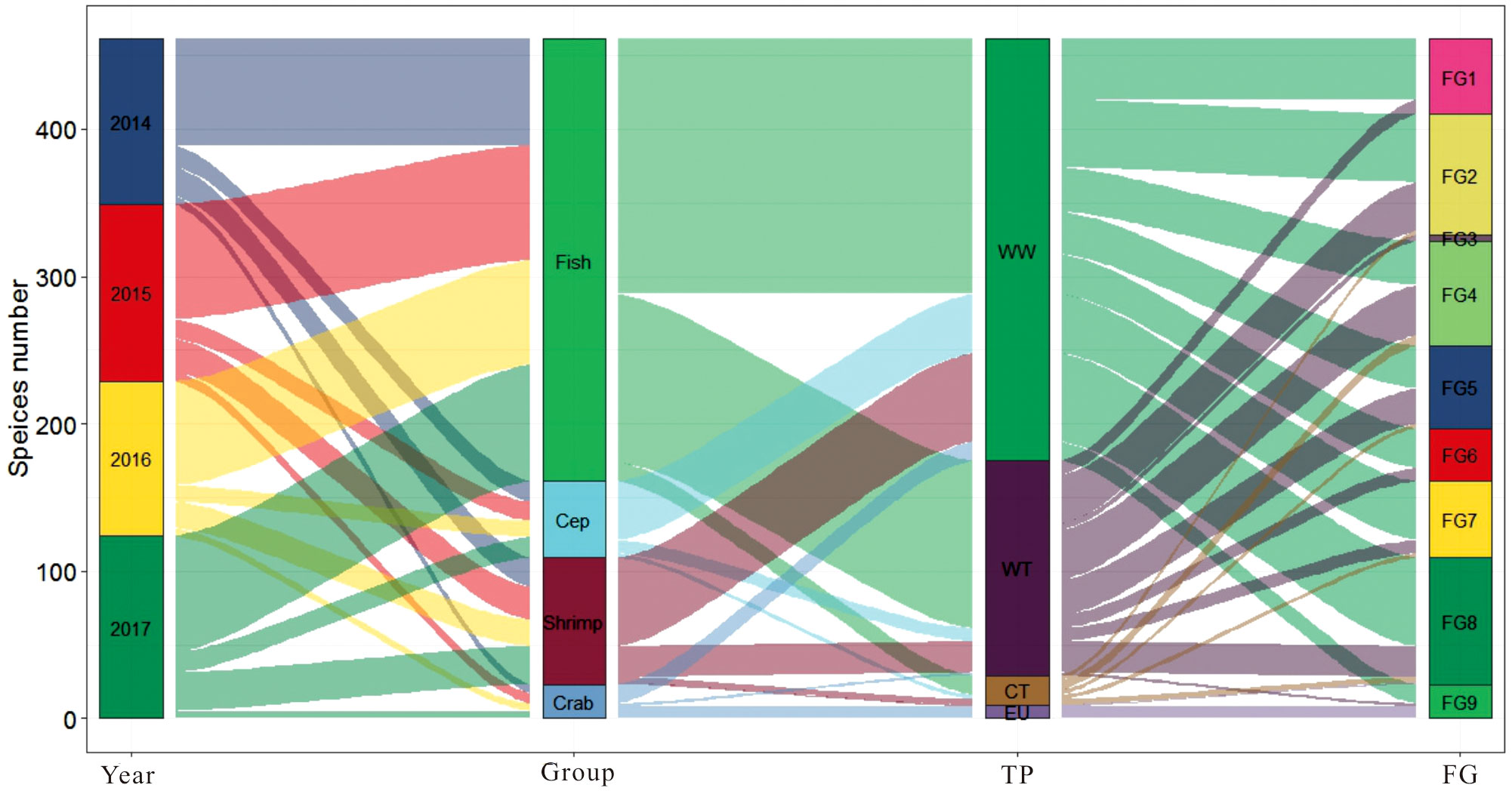
Figure 5 Diagram depicting taxonomic affiliation, thermal preference, and feeding function of the species number associated with each group. TP, thermal preference; FG, function group; Cep, cephalopods; WW, warm water; WT, warm temperature; CT, cold temperature; EU, eurythermic; FG1, planktivores; FG2, small omnivores; FG3, piscivores; FG4, benthivores/piscivores; FG5, benthivores; FG6, predatory fish; FG7, cephalopods; FG8, shrimp; FG9, crab.
Both Larimichthys polyactis and Trichiurus japonicas remained the dominant species in NY and MHW years, with their biomass and abundance accounting for 76.5–87.9% of the total biomass and 78.8–93.3% of the total individuals (Table 1). Furthermore, Portunus trituberculatus (IRI, 564) also appeared as a dominant species in 2014, while Solenocera melantho (IRI, 436) and Ovalipes punctatus (IRI, 267) appeared as important common species. However, both Solenocera m. and Ovalipes p. disappeared from the common species group in 2016 and 2017. In contrast, Pampus argenteus (IRI, 571) exhibited increasing IRI values and became the dominant species in 2016.
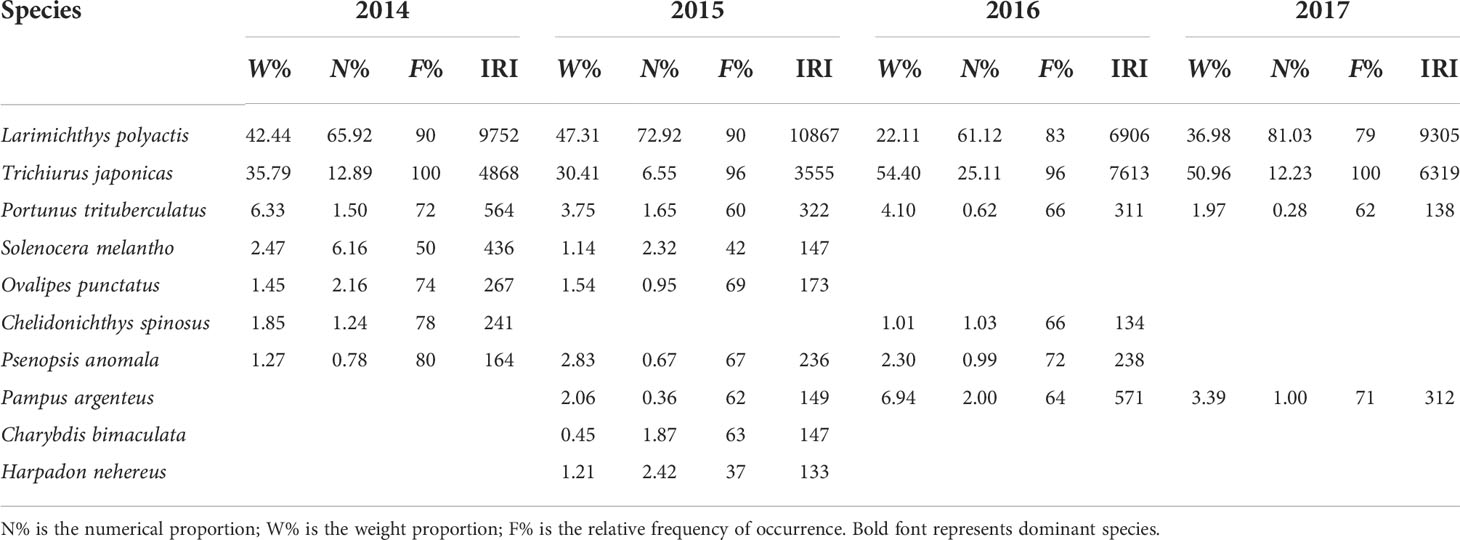
Table 1 Annual variation of dominant species and common species in the nekton community in the northern East China Sea.
Group level changes
The results of ANOVA tests of CPUE in different years for taxonomic affiliation are displayed in Figure 6. Significant differences were observed in the densities of fish and shrimps among different years (P<0.05). Furthermore, the Kruskal–Wallis Test revealed significant differences between NY and MHW years. Significant differences were observed in fish (P<0.05) and shrimps (P<0.01) between 2015 and 2016 and shrimps (P<0.05) between 2014 and 2016. Although average density values did not show any difference at the significance of α = 0.05 among the four years, statistically significant differences were observed between NY and MHW years.
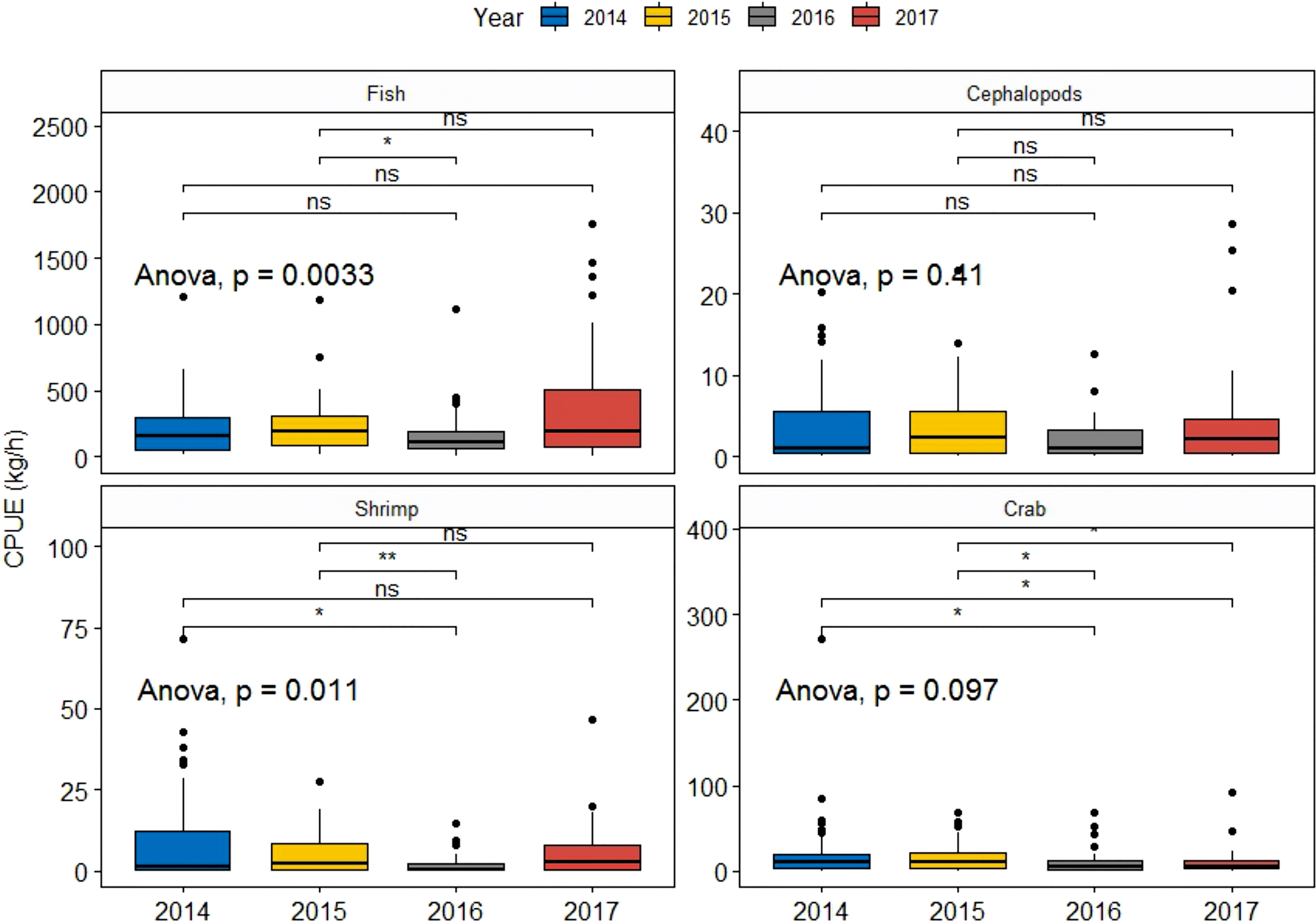
Figure 6 CPUE of fish, cephalopods, and crustaceans (shrimps, crabs) in the years before (2013–2014) and during (2016–2017) the MHW events. All pairs of groups were compared using the Kruskal–Wallis test for one-way ANOVA. * indicates significance at p<=0.05; ** indicates significance at p<=0.01; ns indicates no statistical significance.
In our classification scheme, WW species and WT species outnumber the other species. Therefore, we analyzed differences only for WW species and WT species (Figure 7). Both groups responded strongly to the MHW events (P<0.05). Post-hoc comparisons show that the median density during the MHW events in 2017 changed significantly compared with NY in 2014 or 2015, with increases in WW species (P<0.05) and decreases in WT species (P<0.05).
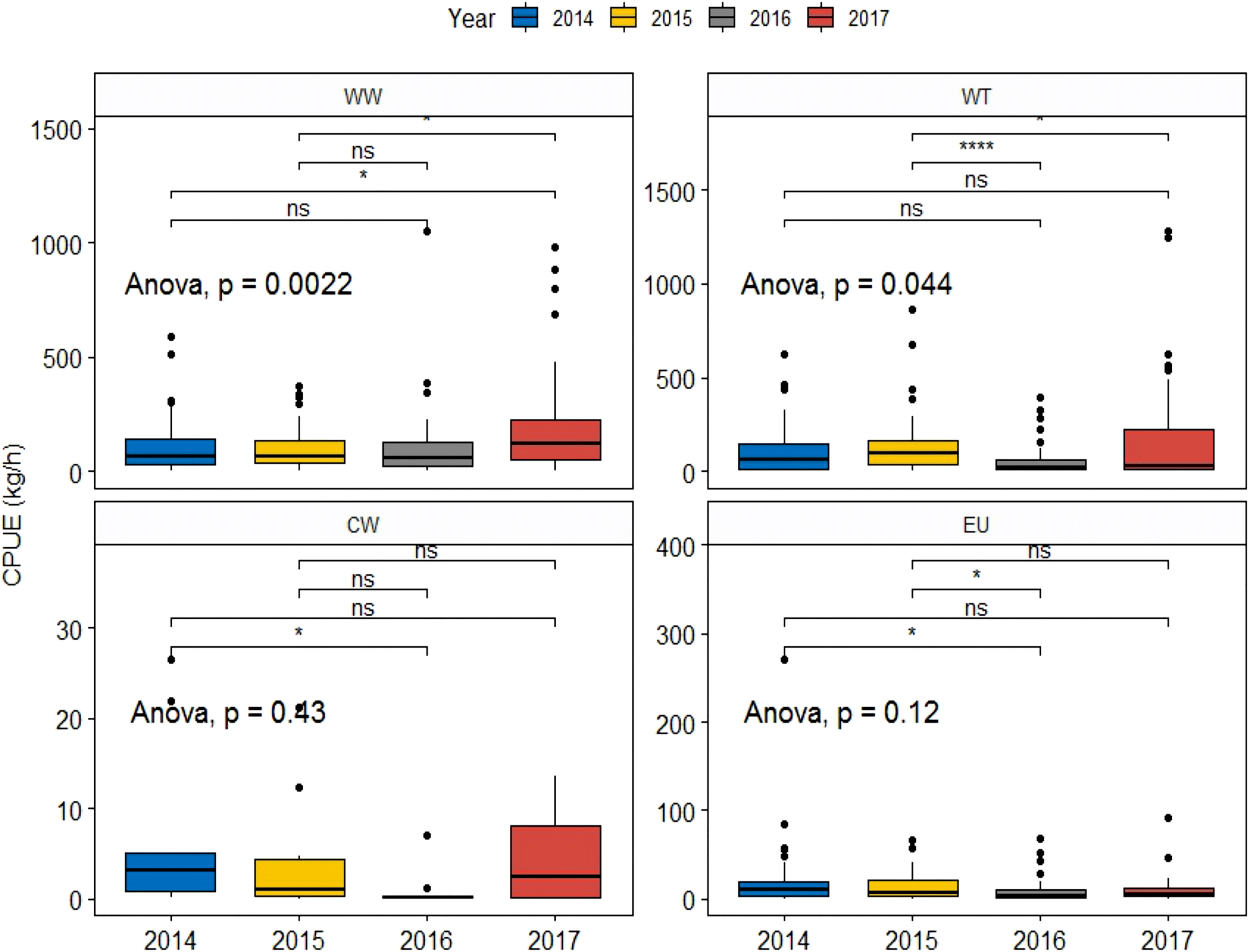
Figure 7 CPUE of WW, WT, CT, and EU species in the years before (2014–2015) and during (2016–2017) the MHW events. All pairs of groups were compared using the Kruskal–Wallis test for one-way ANOVA. * indicates significance at p<=0.05; **** indicates significance at p<=0.0001; ns indicates no statistical significance.
Yearly biomass for fish feeding functional groups from 2014 to 2017 is presented in Figure 8. FG2 and FG6 showed interannual differences. Except for 2014 and 2017, significant differences in FG2 were observed between NY and MHWs. The median density of FG2 showed a decreasing trend during MHWs compared with NY. In contrast to FG2, the densities of FG6 slightly increased in 2016, but it increased significantly in 2017.
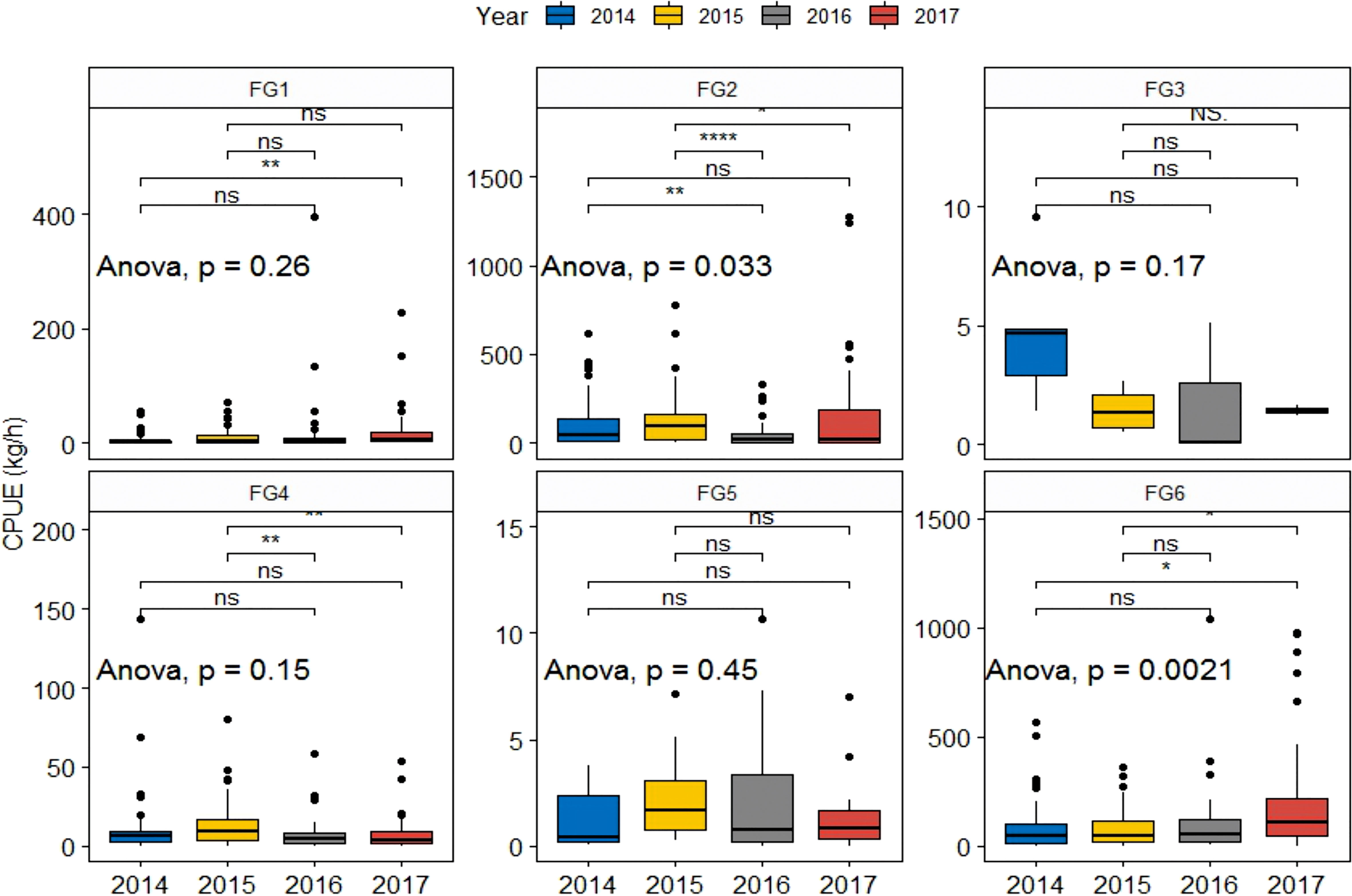
Figure 8 CPUE feeding functional groups for fish in the years before (2014–2014) and during (2016–2017) the MHWs. All pairs of groups were compared using the Kruskal–Wallis test for one-way ANOVA. * indicates significance at p<=0.05; ** indicates significance at p<=0.01; **** indicates significance at p<=0.0001; ns indicates no statistical significance.
Community level changes
Alpha diversity
To estimate the level of variation in terms of community population dynamics among years, alpha diversity was determined through Tukey’s test using the Chao1 species richness and Shannon diversity index. The Shannon diversity index and Chao1 richness are presented in Figure 9. Alpha diversity patterns differed depending on the facet (taxonomic, thermal, or feeding function) and component (composition or structure) considered. In terms of the taxonomic groups (Figures 9A, B), the Shannon diversity of fish varied prominently across the entire MHW events, with significant difference between 2016 and 2017. In case of cephalopods and crustaceans, no significant differences (P>0.05) were observed, but both Chao1 richness and Shannon diversity showed decreasing trends during the MHW events, indicating that these events can decrease the species richness and diversity of communities.
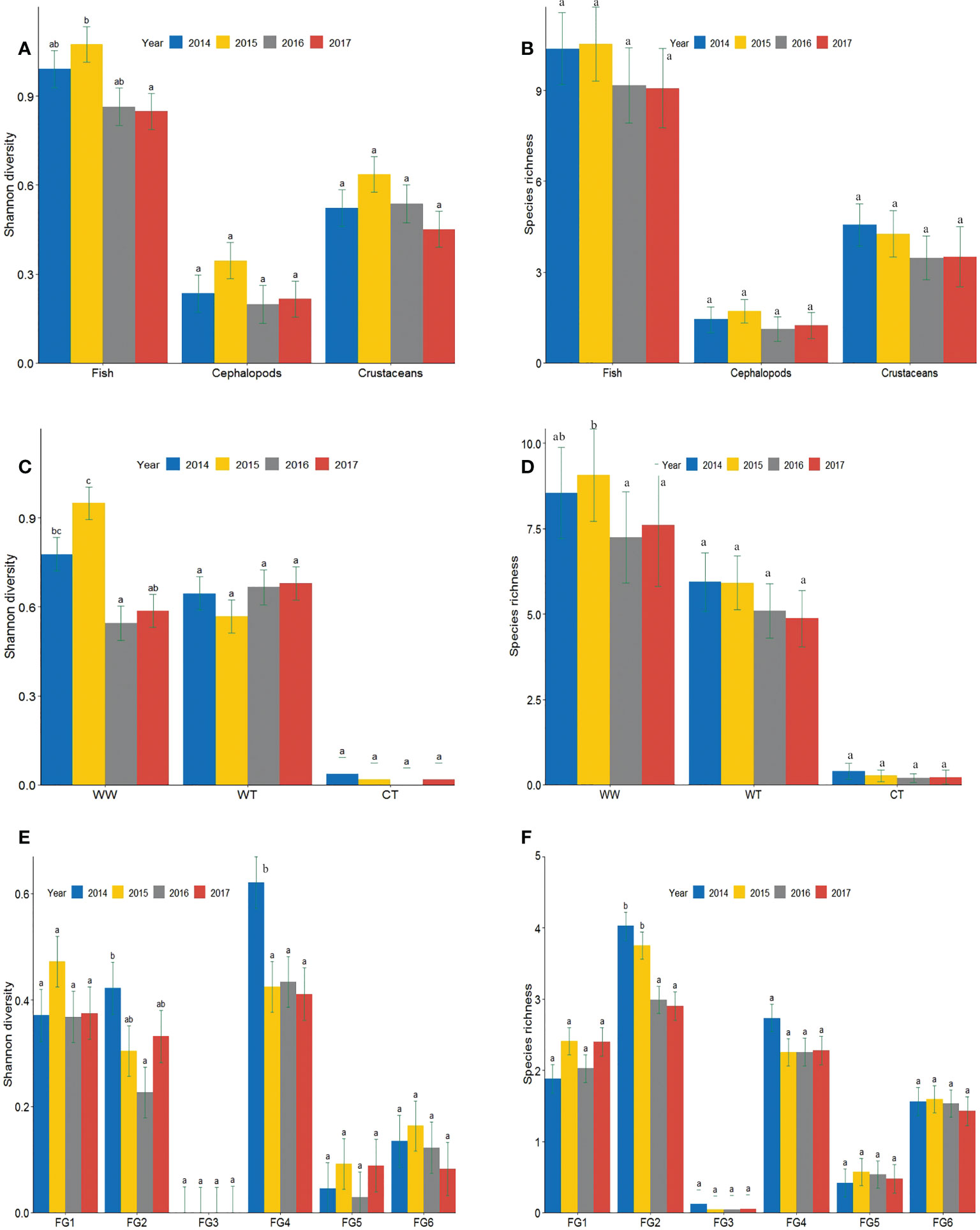
Figure 9 Group comparisons of (a-b) taxa, (c-d) thermal preference, and (e-f) feeding functions in terms of the Shannon diversity and Chao1 richness indices between the years before (2014–2015) and during (2016–2017) the MHW events. The bar column represents the mean ± standard error of richness and diversity among the different study groups. Letters indicate significance using Tukey’s post-hoc multi-comparison test for one-way ANOVA of mean richness and diversity values.
According to the results of thermal preference groups, the species diversity of WW showed significant decreases in MHW years, but the species diversity of WT was not significantly affected, although it showed a slight increase (Figure 9C). In contrast to species diversity, the species richness of both WW and WT species showed a downward trend, but the decrease was not significant (Figure 9D).
The inter-annual variations in the diversity and richness of the feeding functional groups varied across the years. In terms of species diversity (Figure 9E), FG1, FG2, and FG3 showed extremely high values in one NY. Although FG2 exhibited a significant decrease in the first year (2016) of MHWs, it returned to the 2015 level in 2017. However, the species diversity of FG6 decreased during MHW years, compared to NY. In terms of species richness, FG2 dominated in the feeding functional groups and showed a decrease over time (Figure 9F).
Beta diversity
Beta diversity was calculated by temporal dissimilarity, which revealed different variations among samples within or between NY and MHW years. The median values of overall temporal dissimilarity within NY (2014 to 2015) and MHW years (2016 to 2017) were 0.478 and 0.476, respectively. These values showed little change, but in the regime shift from NY to MHW years (2015 to 2016), the overall dissimilarity increased to 0.519. Moreover, the median value of the overall temporal beta-diversity was larger for any combination of communities between NY and MHW years than that of communities within NY or MHW years (Figure 10).
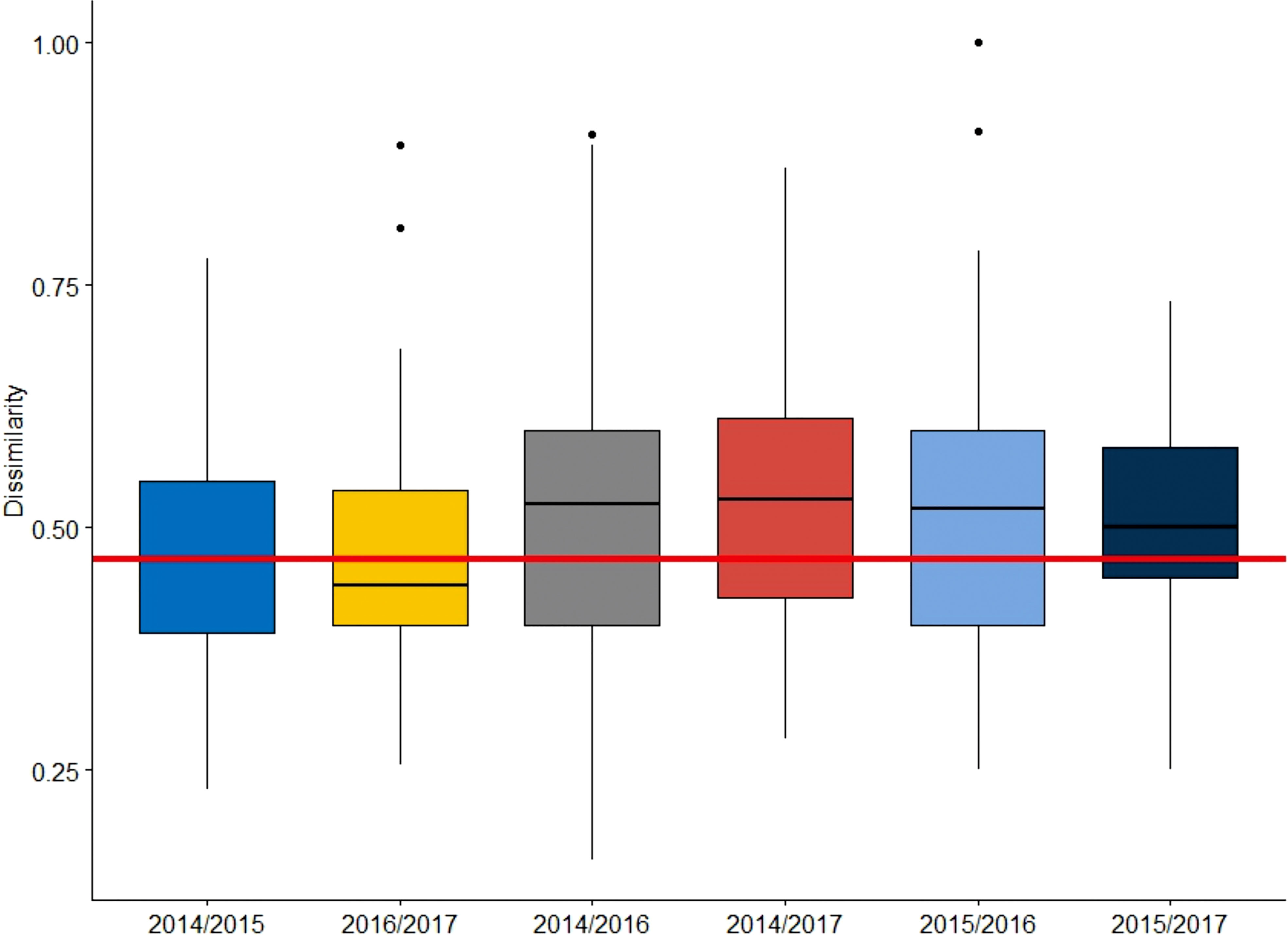
Figure 10 Pairwise temporal beta-diversity values for specific years before (2014–2015) and during (2016–2017) the MHW events across 45 sites in the northern East China Sea. The horizontal line indicates the smallest dissimilarity observed between 2016 and 2017.
The ANOVA of beta-dispersion revealed no significant differences in group dispersion (NY-MHWs in this paper) in terms of taxonomic classification (Table 2). This means that group dispersion, or variance, does not significantly differ and is likely homogeneous between groups. Beta diversity analysis was further performed to estimate the effects of the MHW events in shaping the composition of the overall, fish, cephalopod, and crustacean species. Analysis results of the main effect for the location and interaction term are not included in the Table 2. The complete results are provided in the Supplementary Material Table S1.
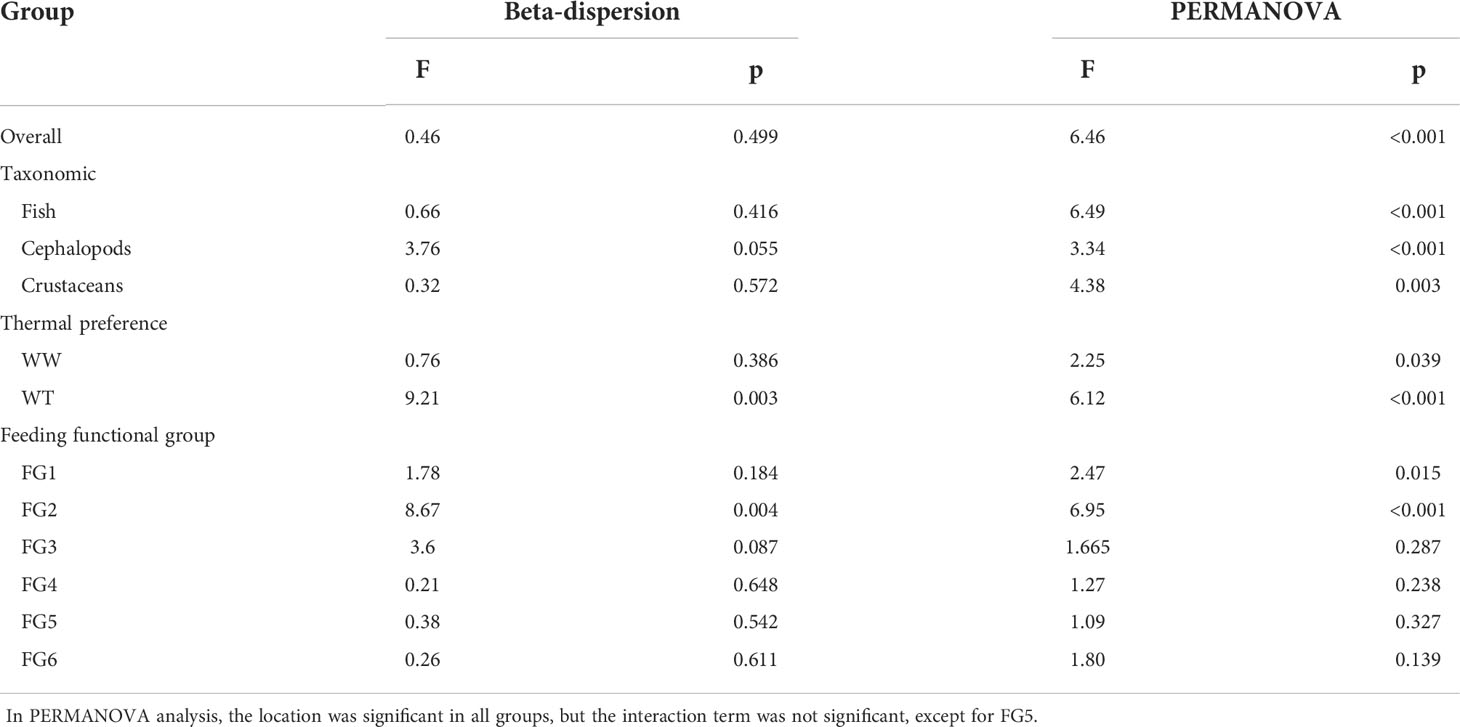
Table 2 Results of two-way ANOVA for dispersion and centroid differences in the community dissimilarity of different groups between sampling years and locations.
PERMANOVA revealed significant differences between mean group centroids for the overall community and taxonomic classification, including fish, cephalopods, and crustaceans. In terms of thermal preference (Table 2), WT species (F=6.12, p<0.001) appeared to exhibit more significant dissimilarity than WW species (F=2.25, p=0.039). The MDS ordination plot also strongly supported the results of PERMANOVA. We only provided MDS of communities exhibiting significant results in the PERMANOVA test (Figure 11). The MDS results showed that the MHW events induced partial clustering of the nekton community. Nevertheless, a few individuals at locations adjacent to a different group appeared to be more closely clustered than individuals at locations far from the same group, which indicates that spatial factors play an important role in shaping species composition.
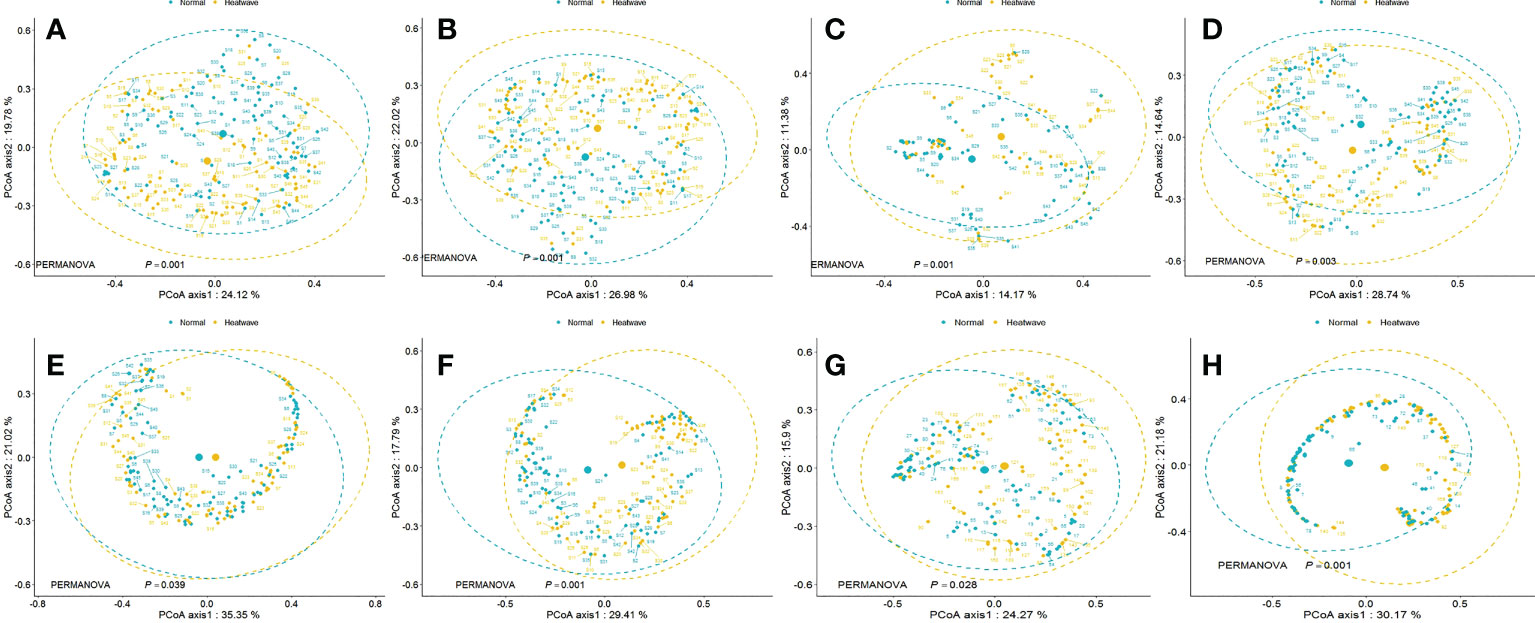
Figure 11 Principal coordinate analyses of Bray-Curtis distances on nekton community composition, calculated between NY (before MHWs) and MHWs for the (A) Overall community, (B) Fish community, (C) Cephalopod community, (D) Crustacean community, (E) WW community, (F) WT community, (G) FG1 community, and (H) FG2 community. Each dot represents a site.
The PERMANOVA results could be affected by the non-homogeneous dispersion of data. Indeed, the WW species community appeared to be less dispersed in the MDS ordination results (Figure 11). Moreover, FG2 showed significant dispersion (F=8.67, p=0.004), for which the PERMANOVA results also showed significant dissimilarity (F=6.95, p<0.001) between NY and MHW years. However, these significant differences may be caused by different within-group dispersion instead of different mean values of the groups. The MDS results show that the distance between the center points of the WW species community is larger than that of the WT species community, which supports the speculation that the difference detected is attributable to the different center points of each group, rather than data dispersion.
Indicator species of community dissimilarity
SIMPER revealed dissimilarities in the nekton community structure and indicated the species with the largest contributions to the variations between NY and MHW years (Table 3). The average similarity was 41% for NY, and 44% for MHW years. The average dissimilarity was 72% between NY and MHWs. The species that had the largest contributions to the dissimilarity between NY and MHW years were Larimichthys polyactis, Trichiurus japonicas, Portunus trituberculatus, and others at the 90% dissimilarity cut-off. As the contribution of each species to the overall dissimilarity is primarily attributable to the variation in species abundances and only partially to differences among groups, the permutation test was conducted to determine the species for which the difference among groups is an important component of their contribution to dissimilarities. The results showed that Larimichthys polyactis, Solenocera melantho, Ovalipes punctatus, Harpadon nehereus, and Lophius Litulon contributed the most significantly to between-group dissimilarities.
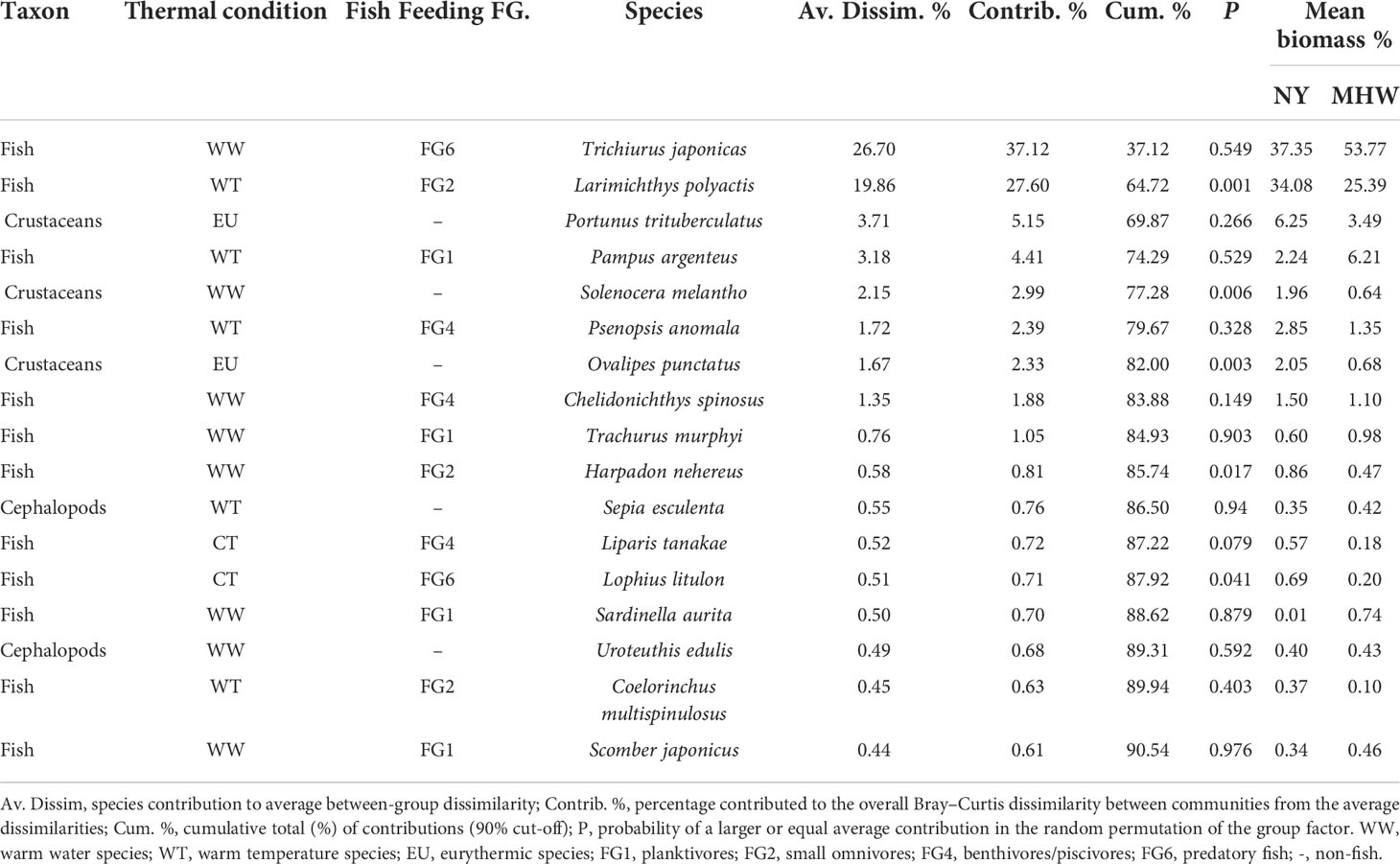
Table 3 Similarity of percentage analysis of community dissimilarity (Bray–Curtis) between NY and MHW samples.
Discussion
The diversity of life-history strategies, including different tolerances to water temperatures and seasonality of life stages, usually promotes the stability of biological communities (Arimitsu et al., 2021). However, MHW events fundamentally changed the nekton community structure, which was mainly caused by dominant species alternation, along with biomass declines of forage fish and crustaceans with short life cycles. This influence also depends on species taxonomies, thermal conditions, and feeding functional groups.
Response of different species to MHW events
The sensitivity and vulnerability of invertebrates to MHW events have also been reported for many marine species, such as the Roei abalone, scallops, tiger prawns, and blue swimmer crabs (Caputi et al., 2016). In terms of species biomass, we found crustaceans negatively responded to MHW, especially crabs with significantly decreased biomass, which altered the position of Portunus trituberculatus as the dominant species. The maximum thermal limit of Portunus trituberculatus can reach 35°C, but the optimal temperature for breeding is 24–27°C (Liao et al., 2008), heatwaves with a 2–3°C spike in SST during 2016–2017 would affect the physiological activities of crabs, such as neuromuscular activity, respiration, blood circulation, and lipid metabolism (Dai et al., 2014; Azra et al., 2020), resulting in poor growth and even death (Kim and Kim, 2017). Moreover, numerous crustaceans, including crabs, have been reported to respond to variations in water temperature by modifying the time and frequency of their shelling, growth rate, size at maturity, larval development. When these changes reach their temperature threshold, the survival rate will eventually be limited (Chandrapavan et al., 2019).
The similarity analysis revealed that Solenocera melantho and Ovalipes punctatus are also indicator species for distinguishing the dissimilarity in community structure between NY and MHW years. The relative biomass of both species decreased significantly by 64.4% and 65.2%, respectively, in the MHW years. Consequently, their status as common species in the community was altered, indicating that MHW events might pose more severe threats to invertebrates. Previous studies have shown that the heatwaves on the west coast of Australia in 2011 caused an SST rise of 5°C, resulting in massive deaths of blue swimmer crab (Portunus armatus) in Shark Bay and a sharp decline in fishery production (Chandrapavan et al., 2019; Caputi et al., 2019). A warm event in 1999 also contributed to a massive die-off of lobsters in Long Island Sound (Pearce and Balcom, 2009), and a warning was issued regarding the spread of lobster shell disease, which decimated their populations (Wahle et al., 2009).
Nevertheless, heatwaves also have positive effects on the invertebrates. Some species have also been reported to undergo geographical migration or phenological changes, which even had favorable results. The record temperatures in 2012 in the Gulf of Maine decreased the molting rates and enhanced the number of legal-sized lobsters, extending the fishing season and supporting the record landings (Mills et al., 2013).
Response of different thermal preference species to MHW events
The total biomass of WW species increased significantly during the MHW events, while that of WT species decreased significantly, which reflected a common phenomenon that WW species are expected to increase in a warming ocean (Poloczanska et al., 2016). The inconsistent change trends of the biomass and diversity of WW species may be attributed to the rapidly respond to the temperature variation by changing their habitats for small pelagic fish (Maureaud et al., 2019). However, as the hairtail with higher thermal safety margin and large biomass dominated the community, the total biomass was not affected by the MHWs. Maureaud et al. (2019) also reported that in the over-fished marine ecosystem, rare and sensitive species will decline locally, consequently, the biomass was driven by the dominance of species rather than niche complementarity.
Similar to the response of WW species to MHWs, the response of the biomass of WT species to heatwaves is mainly determined by dominant species. For example, the WT species Pseudosciaena crocea is the dominant species in the Yellow Sea. The northward movement of the distribution center indicates that the peak value of SST exceeded their thermal tolerance. A similar change pattern of Pseudosciaena crocea in the Yellow Sea has been reported (Han et al., 2021). In addition, extreme marine events may cause the death of small yellow croaker. For example, a large amount of dead small yellow croaker floated on the sea surface near 30.2°N, 125.3°E in the East China Sea on 19 February 2017, and more than 9,000 kilograms were caught by fishermen (Wei et al., 2022). Scholars believe that ‘cold shock’ caused by continuous ocean front and sharp temperature drop jointly affected the physiological regulation and survival of small yellow croaker (Wei et al., 2022). We found that a six-day marine heatwaves occurred from February 20 to 25, and the peak SST occurred on February 20. The possibility of this event being related to the death of small yellow croaker on the previous day is a matter of concern.
The diversity of WT species does not show any distinct response to MHWs, indicating that the WT species in this region may not be vulnerable to disturbances of extreme climate events. In terms of their habitat, WT species, such as flatfish, filefish, sharks, and rays, mainly dwell near the bottom or along reefs. These species are characterized by large body size and long life span, due to which they have a slow response to acute climate events (Rijnsdorp et al., 2009; Pörtner and Peck, 2010; Freedman et al., 2020). Therefore, the diversity did not change significantly.
Response of different trophic species to MHW events
The PERMANOVA results revealed that the species compositions of oligotrophic forage fish (FG1 and FG2) significantly differed between NY and MHW years. Changes in FG1 and FG2 communities may have driven the bottom-up regulation as the zooplankton community structure shifted. According to Zuo (2003) climate warming had driven the increase of small copepods in the east of the Yellow Sea, but krill, the prey of fish, would migrate to the north. Gao et al. (2019) also found the shrinking of the body size of zooplankton with elevated temperature, and the dynamics of zooplankton will affect the species abundance of lower trophic levels of fish through the trophic cascade effect. There is evidence of MHWs having a wide range of impacts on multiple trophic levels in northern Goa, leading to extensive changes in ecosystem functions (Suryan et al., 2021). The decline in the quality of zooplankton has led to a decline in the abundance of forage fish and young-of-the-year pollock. Previous studies on the oligotrophic level in the southern and central continental shelf of Alaska and the northern Gulf of Alaska also revealed a bottom-up driven system to a large extent. Changes in the physical environment led to changes in the composition and abundance of phytoplankton and zooplankton communities, which further reduced the abundance of forage fish (Batten et al., 2022).
We also found that different feeding function groups have different responses to temperature, which may be related to different temperature preferences, food adaptability, and competitiveness. For example, predatory fish have high temperature tolerance, and they can adjust their body functions to adapt to the habitat, or obtain bait organisms by changing the niche range through opportunistic survival strategies. In addition, under the conditions of high temperature and limited food, predatory fish usually have stronger feeding ability and bait selection range than forage fish. The abundance and size of different feeding functional groups may change the balance of fish communities. However, the mechanisms and consequences of the observed changes have not yet been fully understood.
Significance of heatwaves events to fishery management
Exploring the characteristics of changes induced by MHWs, a key environmental factor, is an important prerequisite for analyzing and evaluating the ecological vulnerability of marine organisms in China, comprehensive risk assessment, and developing response strategies (adaptation and mitigation). In the 21st century, climate change is expected to remain one of the main threats to biological systems (Pecl et al., 2017). One of the critical climate change processes is the intensification of MHW frequency and duration (Pecl et al., 2017; Oliver et al., 2018). The impact of MHWs on fisheries and protected species can be reduced by implementing preventive measures, which can be designed by summarizing corresponding research conclusions on different taxonomic groups and geographical regions in the world and identifying and monitoring the responses of climate-sentinel species to ocean warming and extreme climate events (Arafeh-Dalmau et al., 2019). Understanding the species compositions and their responses to MHWs is the first step toward predicting ecological changes and incorporating them in decision-making.
Under the influences of climate change, overfishing, habitat destruction, and other factors, the global fishery resources including China’s fishery are facing a serious decline. In terms of single species, the impact of climate change on marine fish is lagging behind. Many fisheries rely on commercial catch rates to assess the status of fish, resulting in an inherent delay between the decline in fish stocks and management actions, which may lead to overfishing during periods of low replenishment, thus delaying its recovery (Walters and Maguire, 1996; Cook et al., 1997; Xu and Liu, 2007; Dickey-Collas et al., 2010; Arafeh-Dalmau et al., 2019). In 2017, China started the pilot of a new mode of fishery output management based on quota fishing. Portunus trituberculatus is set as a pilot species in the East China Sea. Management efficacy determines whether the quota fishing policy can be completely implemented. According to our research, the dominance of Portunus trituberculatus decreased significantly in the MHW years, showing its vulnerability to heatwaves stress. Therefore, it is necessary to focus on the risk assessment of extreme climate events on the effectiveness and feasibility of quota fishing. The research, management, and industry experience of the invertebrate species aquaculture in handling MHW events provide valuable information, which is suitable for handling extreme environmental events (Azra et al., 2020). For instance, studies on the appropriate temperature, growth rate, and shelling time of Portunus trituberculatus at different life cycle stages can improve our knowledge of the response of wild marine crabs to extreme events. In the determination of total allowable catch, multiple factors, such as replenishment, parent size, and fishing capacity, need to be integrated. As Portunus trituberculatus larvae are sensitive to temperature changes (Dai, 2013), future catch can be predicted by monitoring MHW events and preliminary supplementary population survey of this species, such that decision-makers can implement preventive regulation and intervention fishing policies according to changes in abundance. For example, during outbreaks of MHWs, the risk of extreme climate events on the species and related fishing activities can be rapidly minimized by reducing the amount of catches and efforts, and even closing the fishery regularly. Studies have proved that the monitoring and investigation of early life history environment and amount of supplementation are very valuable in diagnosing the impact of MHWs on species population (Caputi et al., 2016).
At the local geographical scale, only few studies have investigated the effect of MHWs on community composition, which is helpful for understanding the patterns of biodiversity, ecosystem functions, and resilience to disturbances. As species have different response patterns to extreme climate events, different taxonomic groups or functional groups may suffer different fishing pressures under the same fishing intensity. Therefore, it is necessary to preliminarily identify the fitness of target species under different climate backgrounds before determining the fishing pressure and the replacement of dominant species. In this manner, more suitable adaptive and preventive fishery management strategies can be formulated and implemented in response to climate change. In the future, research on the mechanism of interactions between fishery ecosystems and local and global climate change should be strengthened, the dynamic change law of fishery ecosystems driven by natural and human forces should be explored, and risk monitoring of climate change on fishery should be improved. Moreover, means of improving the ability of fishery ecosystems to cope with extreme climate change should be developed.
Natural systems are complex in nature. It may be uncertain to attribute the change of nekton community only to the direct impact of heat wave, during the occurrence of MHWs, temperature rise affects not only a specific process, but all related processes in the system. For example, temperature rise may cause a series of linked changes, including environmental (acidification, hypoxia, reduction of primary productivity, etc.), biological, and interactive processes. Nevertheless, different processes may be affected to different degrees. The purpose of biological community analysis is to explore the direct and indirect relationship between variables considering complex phenomena. Although, we found that MHWs induced changes in the community structure, we did not study the driving mechanisms of biological communities. In future research, we should pay more attention to the interaction between different processes in the system, especially in the context of global change, focus on the study of biological complexity, analyze the impact factors and change trends of multiple processes, and clarify the assembly process and mechanism of biological communities, so as to improve the understanding of marine ecosystems.
Data availability statement
The raw data supporting the conclusions of this article will be made available by the authors, without undue reservation.
Ethics statement
Ethical review and approval was not required for the animal study because all the fish samples were collected by scientific investigation and no specific licenses for the studied populations were needed.
Author contributions
YJ and JC designed the study. LLY, MX, YZ and HZ collected the data. ZL and YJ performed modeling and statistical analyses. ZL wrote the original draft. ZL, YJ, and LPY contributed significantly to the drafting of the manuscript. JC was involved in the funding acquisition. All authors contributed to editing and approved the final manuscript. All authors contributed to the article and approved the submitted version.
Funding
This work was supported by the National Key Research and Development Project (2020YFD0900804), Shanghai Sailing Program (18YF1429800), Special funds for survey of offshore fishery resources by the Ministry of Agriculture and Rural Affairs (125C0505).
Conflict of interest
The authors declare that the research was conducted in the absence of any commercial or financial relationships that could be construed as a potential conflict of interest.
Publisher’s note
All claims expressed in this article are solely those of the authors and do not necessarily represent those of their affiliated organizations, or those of the publisher, the editors and the reviewers. Any product that may be evaluated in this article, or claim that may be made by its manufacturer, is not guaranteed or endorsed by the publisher.
Supplementary material
The Supplementary Material for this article can be found online at: https://www.frontiersin.org/articles/10.3389/fmars.2022.1036047/full#supplementary-material
References
Anderson M. J. (2001). A new method for non-parametric multivariate analysis of variance. Austral Ecol. 26, 32–46. doi: 10.1111/j.1442-9993.2001.01070.pp.x
Arafeh-Dalmau N., Montaño-Moctezuma G., Martínez J. A., Beas-Luna R., Schoeman D. S., Torres-Moye G. (2019). Extreme marine heatwaves alter kelp forest community near its equatorward distribution limit. Front. Mar. Sci. 6. doi: 10.3389/fmars.2019.00499
Arimitsu M. L., Piatt J. F., Hatch S., Suryan R. M., Batten S., Bishop M. A., et al. (2021). Heatwave-induced synchrony within forage fish portfolio disrupts energy flow to top pelagic predators. Glob. Change Biol. 27 (9), 1859–1878. doi: 10.1111/gcb.15556
Azra M. N., Aaqillah-Amr M. A., Ikhwanuddin M., Ma H., Waiho K., Ostrensky A., et al. (2020). Effects of climate-induced water temperature changes on the life history of brachyuran crabs. Rev. Aquacult. 12 (2), 1211–1216. doi: 10.1111/raq.12380
Baselga A., Orme D., Villeger S., De Bortoli J., Leprieur F., Logez M. (2022) Betapart: Partitioning beta diversity into turnover and nestedness components. r package version 1.5.6 (Accessed July 20, 2022).
Batten S. D., Ostle C., Hélaouët P., Walne A. (2022). Responses of gulf of Alaska plankton communities to a marine heat wave. Deep-Sea Res. Pt. Ii. 195, 105002. doi: 10.1016/j.dsr2.2021.105002
Cai R., Chen J., Tan H. (2011). Variations of the Sea surface temperature in the offshore area of China and their relationship with the East Asian monsoon under the global warming. Climatic Environ. Res. 16 (1), 94–104. doi: 10.3878/j.issn.1006-9585.2011.01.09
Cai R. S., Tan H. J., Kontoyiannis H. (2017). Robust surface warming in offshore China seas and its relationship to the East Asian monsoon wind field and ocean forcing on interdecadal timescales. J. Climate 30 (22), 8987–9005. doi: 10.1175/JCLID16-0016.1
Caputi N., Kangas M., Chandrapavan A., Hart A., Feng M., Marin M., et al. (2019). Factors affecting the recovery of invertebrate stocks from the 2011 Western Australian extreme marine heatwave. Front. Mar. Sci. 6. doi: 10.3389/fmars.2019.00484
Caputi N., Kangas M., Denham A., Feng M., Pearce A., Hetzel Y., et al. (2016). Management adaptation of invertebrate fisheries to an extreme marine heat wave event at a global warming hot spot. Ecol. Evol. 6 (11), 3583–3593. doi: 10.1002/ece3.2137
Chandrapavan A., Caputi N., Kangas M. (2019). The decline and recovery of a crab population from an extreme marine heatwave and aChanging climate. Front. Mar. Sci. 6. doi: 10.3389/fmars.2019.00510
Chen Y. L. L., Lu H. B., Shiah F. K., Gong G. C., Liu K. K., Kanda J. (1999). New production and f-ratio on the continental shelf of the East China Sea: comparisons between nitrate inputs from the subsurface kuroshio current and the changjiang river. Estuar. Coast. Shelf Sci. 48 (1), 59–75. doi: 10.1006/ecss.1999.0404
Clarke K. R., Warwick R. M. (2001). Change in marine communities: an approach to statistical analysis and interpretation. 2nd edn (Plymouth, UK: PRIMER-E Ltd).
Cook R. M., Sinclair A., Stefánsson G. (1997). Potential collapse of north Sea cod stocks. Nautre 385 (6616), 521–522. doi: 10.1038/385521a0
Dai C. (2013). Effect of temperature on molting, physiological character and respiratory metabolism of the swimming crab portunus trituberculatus (Qingdao: Ocean University of China).
Dai C., Wang F., Fang Z., Dong S. (2014). Effects of temperature on the respiratory metabolism and activities of related enzymes of swimming crab Portunus trituberculatus. Prog. Fish. Sci. 35 (2), 90–96. doi: 10.3969/j.issn.1000-7075.2014.02.013
Dickey-Collas M., Nash R. M. D., Brunel T., van Damme C. J. G., Marshall C. T., Payne M. R., et al. (2010). Lessons learned from stock collapse and recovery of north Sea herring: a review. ICES J. Mar. Sci. 67 (9), 1875–1886. doi: 10.1093/icesjms/fsq033
Freedman R. M., Brown J. A., Caldow C., Caselle J. E. (2020). Marine protected areas do not prevent marine heatwave−induced fish community structure changes in a temperate transition zone. Sci.rep. 10, 21081. doi: 10.1038/s41598-020-77885-3
Freitas C., Villegas-Ríos D., Moland E., Olsen E. M. (2021). Sea Temperature effects on depth use and habitat selection in a marine fish community. J. Anim. Ecol. 90 (7), 1787–1800. doi: 10.1111/1365-2656.13497
Frölicher T. L., Fischer E. M., Gruber N. (2018). Marine heatwaves under global warming. Nature 560, 360–364. doi: 10.1038/s41586-018-0383-9
Gao X., Chen H., Govaert L., Wang W., Yang J. (2019). Responses of zooplankton body size and community trophic structure to temperature change in a subtropical reservoir. Ecol. Evol. 9 (22), 12544–12555. doi: 10.1002/ece3.5718
Garrabou J., Coma R., Bensoussan N., Bally M., Chevaldonné P., Cigliano M., et al. (2009). Mass mortality in northwestern Mediterranean rocky benthic communities: effects of the 2003 heat wave. Glob. Change Biol. 15, 1090–1103. doi: 10.1111/j.1365-2486.2008.01823.x
Guo X., Gao Y., Zhang S., Wu L., Chang P., Cai W., et al. (2022). Threat by marine heatwaves to adaptive large marine ecosystems in an eddy-resolving model. Nat. Clim. Change 12, 179–186. doi: 10.1038/s41558-021-01266-5
Gupta A. S., Thomsen M., Benthuysen J. A., Hobday A. J., Oliver E., Alexander L. V., et al. (2020). Drivers and impacts of the most extreme marine heatwave events. Sci. Rep. 10, 19359. doi: 10.1038/s41598-020-75445-3
Halpern B. S., Walbridge S., Selkoe K. A., Kappel C. V., Micheli F., D'Agrosa C., et al. (2008). A global map of human impact on marine. Science 319 (948), 948–952. doi: 10.1126/science.1149345
Han Q., Grüss A., Shan X., Jin X., Thorson J. T. (2021). Understanding patterns of distribution shifts and range expansion/contraction for small yellow croaker (Larimichthys polyactis) in the yellow Sea. Fish. Oceanogr. 30 (1), 69–84. doi: 10.1111/fog.12503
Harris R. M. B., Loeffler F., Rumm A., Fischer C., Horchler P., Scholz M., et al. (2020). Biological responses to extreme weather events are detectable but difficult to formally attribute to anthropogenic climate change. Sci. Rep. 10, 14067. doi: 10.1038/s41598-020-70901-6
Hart R. K., Calver M. C., Dickman C. R. (2002). The index of relative importance: an alternative approach to reducing bias in descriptive studies of animal diets. Wildlife Res. 29 (5), 415–421. doi: 10.1071/WR02009
HobdayaLis A. J., Alexander L. V., Perkins S. E., Smale D. A., Straub S. C., Oliver E. C. J., et al. (2016). A hierarchical approach to defining marine heatwave. Progr. Oceanogr. 141, 227–238. doi: 10.1016/j.pocean.2015.12.014
Hothorn T., Bretz F., Westfall P. (2008). Simultaneous inference in general parametric models. Biometrical J. 50 (3), 346–363. doi: 10.1002/bimj.200810425
Inyang A. I., Wang Y. (2020). Phytoplankton diversity and community responses to physicochemical variables in mangrove zones of guangzhou province, China. Ecotoxicology 29, 650–668. doi: 10.1007/s10646-020-02209-0
Jacox M. G., Alexander M. A., Bograd S. J., Scott J. D. (2020). Thermal displacement by marine heatwaves. Nature 584, 82–86. doi: 10.1038/s41586-020-2534-z
Jiang Y., Cheng J., Li S. (2008). Variation in fish community structure and biodiversity in the north of the East China Sea between two periods. J. Fish. Sci. China 15 (3), 453–458. doi: 10.3321/j.issn:1005-8737.2008.03.011
Johnston E. L., Clark G. F., Bruno J. F. (2022). The speeding up of marine ecosystems. Clim. Change Ecol. 3, 100055. doi: 10.1016/j.ecochg.2022.100055
Kassambara A. (2020) Ggpubr: 'ggplot2' based publication ready plots. r package version 0.4.0 (Accessed July 20, 2022).
Kim Y. H., Kim S. H. (2017). Molting and growth of the larval swimming crab, Portunus trituberculatus (Miers 1876), at different water temperature. J. Fish. Mar. Sci. Educ. 29 (2), 2049–2288. doi: 10.13000/JFMSE.2017.29.2.422
Lemieux-Labonté V., Tromas N., Shapiro B. J., Lapointe F. J. (2016). Environment and host species shape the skin microbiome of captive neotropical bats. PeerJ 4, e2430. doi: 10.7717/peerj.2430
Lenth R. V. (2022) Emmeans: Estimated marginal means, aka least-squares means. r package version 1.7.5 (Accessed July 20, 2019).
Liao Y., Xiao Z., Yuan Y. (2008). Temperature tolerance of larva and juvenile of Portunus trituberculatus. Acta Hydrobiol. Sin. 32 (4), 534–543. doi: 10.3724/SP.J.1035.2008.00534
Li J., Kang B. (2020). Dominant species drive seasonal dynamics of the fish community in the Min estuary, China. Oceanol. Hydrobiol. St. 49 (1), 34–48. doi: 10.1515/ohs-2020-0004
Li Y., Ren G., Wang Q., You Q. (2019). More extreme marine heatwaves in the China seas during the global warming hiatus. Environ. Res. Lett. 14, 104010. doi: 10.1088/1748-9326/ab28bc
Liu Z., Jin Y., Yan L., Zhang Y., Zhang H., Shen C., et al. (2022). Identifying priority conservation areas of largehead hairtail (Trichiurus japonicus) nursery grounds in the East China Sea. Front. Mar. Sci. 8. doi: 10.3389/fmars.2021.779144
Liu J., Su N., Wang X., Du J. (2017). The sea annually receives an immense quantity of sediments, mostly from the Huang he (via the bo hai) and the Yangtze river, both of which have formed large deltas. J. Geophys. Res. Oceans 122, 139–152. doi: 10.1002/2016JC012282
Lopez C. B., Cloern J. E., Schraga T. S., Little A. J., Lucas L. V., Thompson J. K., et al. (2006). Ecological values of shallow-water habitats: Implications for the restoration of disturbed ecosystems. Ecosystems 9, 422–440. doi: 10.1007/s10021-005-0113-7
Marbà N., Duarte C. M. (2010). Mediterranean Warming triggers seagrass (Posidonia oceanica) shoot mortality. Glob. Change Biol. 16, 2366–2375. doi: 10.1111/j.1365-2486.2009.02130.x
Matsuzaki M. M., Itaki T., Tada R. (2019). Paleoceanographic changes in the northern East China Sea during the last 400 kyr as inferred from radiolarian assemblages (IODP site U1429). Prog. Earth Planet Sci. 6 (22), 10–1186. doi: 10.1186/s40645-019-0256-3
Maureaud A., Hodapp D., van Denderen P. D., Hillebrand H., Gislason H., Dencker T. S., et al. (2019). Biodiversity–ecosystem functioning relationships in fish communities: biomass is related to evenness and the environment, not to species richness. Proc. R. Soc B 286, 20191189. doi: 10.1098/rspb.2019.1189
Mihalitsis M., Bellwood D. R. (2021). Functional groups in piscivorous fishes. Ecol. Evol. 11 (18), 12765–12778. doi: 10.1002/ece3.8020
Mills K. E., Pershing A. J., Brown C. J., Chen Y., Chiang F. S., Holland D. S., et al. (2013). Fisheries management in a changing climate: lessons from the 2012 ocean heat wave in the Northwest Atlantic. Oceanography 26, 191–195. doi: 10.5670/oceanog.2013.27
Morris E. K., Caruso T., Buscot F., Fischer M., Hancock C., Maier T. S., et al. (2014). Choosing and using diversity indices: insights for ecological applications from the German biodiversity exploratories. Ecol.Evol. 4 (18), 3514–3524. doi: 10.1002/ece3.1155
Muñoz A. A., Ojeda F. P. (1997). Feeding guild structure of a rocky intertidal fish assemblage in central Chile. Environ.Biol. Fish. 49, 471–479. doi: 10.1007/s004420050481
Oksanen J., Simpson G. L., Blanchet F. G., Kindt R., Legendre P., Minchin P. R., et al. (2022) Vegan: Community ecology package. r package version 2.6-2 (Accessed July 12, 2022).
Oliver E. C. J. (2019). Mean warming not variability drives marine heatwave trends. Clim. Dyn. 53, 1653–1659. doi: 10.1007/s00382-019-04707-2
Oliver E. C., Donat M. G., Burrows M. T., Moore P. J., Smale D. A., Alexander L. V., et al. (2018). Longer and more frequent marine heatwaves over the past century. Nat. Commun. 9, 1324. doi: 10.1038/s41467-018-03732-9
Parmesan C., Root T. L., Willig M. R. (2000). Impacts of extreme weather and climate on terrestrial biota. Bull. Am. Meteorol. Soc 81, 443–450. doi: 10.1175/1520-0477(2000)081%3c0443:ioewac%3e2.3.co;2
Pearce J., Balcom N. (2009). The 1999 long island sound lobster mortality event: Findings of the comprehensive research initiative. J. Shellfish Res. 24, 691–697. doi: 10.2983/0730-8000(2005)24[691:TLISLM]2.0.CO;2
Pecl G. T., Araújo M. B., Bell J. D., Blanchard J., Bonebrake T. C., Chen I. C., et al. (2017). Biodiversity redistribution under climate change: impacts on ecosystems and human well-being. Science 355 (6332), i9214. doi: 10.1126/science.aai9214
Plecha S. M., Soares P. M. M. (2020). Global marine heatwave events using the new CMIP6 multi-model ensemble: from shortcomings in present climate to future projections. Environ. Res. Lett. 15, 124058. doi: 10.1088/1748-9326/abc847
Poloczanska E. S., Burrows M. T., Brown C. J., García Molinos J., Halpern B. S., Hoegh-Guldberg O., et al. (2016). Responses of marine organisms to climate change across oceans. Front. Mar. Sci. 3. doi: 10.3389/fmars.2016.00062
Pörtner H. O., Peck M. A. (2010). Climate change effects on fishes and fisheries: towards a cause-and-effect understanding. Fish Biol. 77 (8), 1745–1779. doi: 10.1111/j.1095-8649.2010.02783.x
R Core Team (2022). R: A language and environment for statistical computing (Vienna, Austria: R Foundation for Statistical Computing).
Reynolds R. W., Smith T. M., Liu C., Chelton D. B., Casey K. S., Schlax M. G. (2007). Daily high-resolution-blended analyses for sea surface temperature. J. Climate 20, 5473–5496. doi: 10.1175/2007JCLI1824.1
Rijnsdorp A. D., Peck M. A., Engelhard G. H., Möllmann C., Pinnegar J. K. (2009). Resolving the effect of climate change on fish populations. ICES J. Mar. Sci. 66 (7), 1570–1583. doi: 10.1093/icesjms/fsp056
Root R. B. (1967). The niche exploitation pattern of the blue-gray gnatcatcher. Ecol. Monogr. 37, 317–350. doi: 10.2307/1942327
Sasaki Y. N., Umeda C. (2021). Rapid warming of Sea surface temperature along the kuroshio and the China coast in the East China Sea during the twentieth century. Cover J. Clim. 34 (12), 4803–4815. doi: 10.1175/JCLI-D-20-0421.1
Schlegel R. W., Oliver E. C., Wernberg T., Smit A. J. (2017). Nearshore and offshore cooccurrence of marine heatwaves and cold-spells. Progr. Oceanogr. 151, 189–205. doi: 10.1016/j.pocean.2017.01.004
Schlegel R. W., Smit A. J. (2018). heatwaveR: A central algorithm for the detection of heatwaves and cold-spells. J. Open Sour. Soft. 3 (27), 821. doi: 10.21105/joss.00821
Smale D. A., Wernberg T. (2013). Extreme climatic event drives range contraction of a habitat-forming species. Proc. R. Soc B 280, 20122829. doi: 10.1098/rspb.2012.2829
Smale D. A., Wernberg T., Oliver E. C. J., Thomsen M., Harvey B. P., Straub S. C., et al. (2019). Marine heatwaves threaten global biodiversity and the provision of ecosystem services. Nat. Clim. Change 9, 306–312. doi: 10.1038/s41558-019-0412-1
Suryan R. M., Arimitsu M. L., Coletti H. A., Hopcroft R. R., Lindeberg M. R., Barbeaux S. J., et al. (2021). Ecosystem response persists after a prolonged marine heatwave. Sci. Rep. 11, 6235. doi: 10.1038/s41598-021-83818-5
Tan H., Cai R. (2018). What caused the record-breaking warming in East China seas during august 2016? Atmos. Sci. Lett. 19 (10), e853. doi: 10.1002/asl.853
Tian R. C., Hu F. X., Martin J. M. (1993). Summer nutrient fronts in the changjiang (Yantze river) estuary. Estuar. Coast. Shelf Sci. 37 (1), 27–41. doi: 10.1006/ecss.1993.1039
Ummenhofer C. C., Meehl G. A. (2017). Extreme weather and climate events with ecological relevance: a review. Phil. Trans. R. Soc B 372 (1723), 20160135. doi: 10.1098/rstb.2016.0135
Wahle R. A., Gibson M., Fogarty M. J. (2009). Distinguishing disease impacts from larval supply effects in a lobster fishery collapse. Mar. Ecol. Progr. Ser. 376, 185–192. doi: 10.3354/meps07803
Walters C., Maguire J. J. (1996). Lessons for stock assessment from the northern cod collapse. Rev. Fish Biol. Fish. 6, 125–137. doi: 10.1007/bf00182340
Wang Q., Li Q., Li Y., Liu Y., Wang Y. (2021a). Temporal and spatial characteristics of marine heat waves in the bohai Sea and yellow Sea during 1982–2019. HaiyangXuebao 43 (12), 38–49. doi: 10.12284/hyxb2021179
Wang A., Wang H., Fan W., Luo J., Li W., Xu S. (2021b). Study on characteristics of marine heatwave in the China offshore in 2019. HaiyangXuebao 43 (6), 35–44. doi: 10.12284/hyxb2021076
Wei Z., Jiao X., Zhang J., Du Y., Rong Z., Wu H., et al. (2022). Dynamic cause of the sudden death event of small yellow croaker (Larimichthys polyactis) in the East China Sea in winter 2017. Front. Mar. Sci. 9. doi: 10.3389/fmars.2022.908195
Wernberg T., Bennett S., Babcock R. C., De Bettignies T., Cure K., Depczynski M., et al. (2016). Climate-driven regime shift of a temperate marine ecosystem. Science 377 (6605), 169–172. doi: 10.1126/science.aad874
Wernberg T., Smale D. A., Tuya F., Thomsen M. S., Langlois T. J., De Bettignies T., et al. (2013). An extreme climatic event alters marine ecosystem structure in a global biodiversity hotspot. Nat. Clim. Change 3 (1), 78–82. doi: 10.1038/nclimate1627
Wu L., Cai W., Zhang L., Nakamura H., Timmermann A., Joyce T., et al. (2012). Enhanced warming over the global subtropical western boundary currents. Nat. Clim. Change 2, 161–166. doi: 10.1038/nclimate1353
Xu K., Liu Z. (2007). The current stock of large yellow croaker Pseudosciaena crocea in the East China Sea with respects of its stock decline. J. Dalian Fish. Univ. 22 (5), 392–396. doi: 10.3969/j.issn.1000-9957.2007.05.015
Yao Y., Wang J., Yin J., Zou X. (2020). Marine heatwaves in china's marginal seas and adjacent offshore waters: Past, present, and future. J. Geophys. Res-Oceans 125, e2019J–e15801J. doi: 10.1029/2019JC015801
Zhang B., Tang Q., Jin X. (2007). Functional groups of fish assemblages and their mayor species at high trophic level in the East China Sea. J. Fish. Sci. China 14 (6), 939–949. doi: 10.3321/j.issn:1005-8737.2007.06.009
Keywords: climate change, marine heat wave, East China Sea, feeding functional group, diversity, thermal preference
Citation: Liu Z, Yang L, Yan L, Zhang H, Zhang Y, Xu M, Jin Y and Cheng J (2022) Alteration of alpha and beta diversity in nekton community by extreme marine heatwave events: An example from the East China Sea. Front. Mar. Sci. 9:1036047. doi: 10.3389/fmars.2022.1036047
Received: 03 September 2022; Accepted: 24 October 2022;
Published: 03 November 2022.
Edited by:
Kui Zhang, South China Sea Fisheries Research Institute, Chinese Academy of Fishery Sciences (CAFS), ChinaCopyright © 2022 Liu, Yang, Yan, Zhang, Zhang, Xu, Jin and Cheng. This is an open-access article distributed under the terms of the Creative Commons Attribution License (CC BY). The use, distribution or reproduction in other forums is permitted, provided the original author(s) and the copyright owner(s) are credited and that the original publication in this journal is cited, in accordance with accepted academic practice. No use, distribution or reproduction is permitted which does not comply with these terms.
*Correspondence: Yan Jin, jenniferyanjin@163.com; Jiahua Cheng, dhsziyuan@163.com