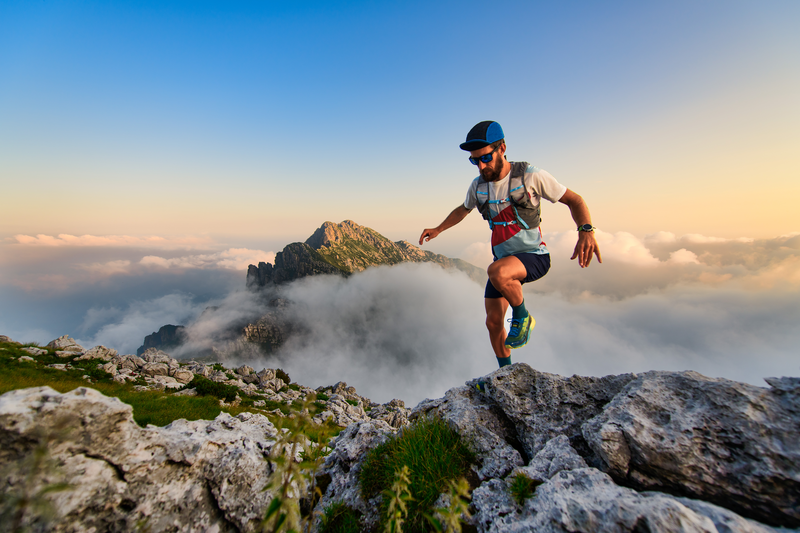
94% of researchers rate our articles as excellent or good
Learn more about the work of our research integrity team to safeguard the quality of each article we publish.
Find out more
ORIGINAL RESEARCH article
Front. Mar. Sci. , 17 November 2022
Sec. Coastal Ocean Processes
Volume 9 - 2022 | https://doi.org/10.3389/fmars.2022.1034876
This article is part of the Research Topic Macroecology of Coastal Zone under Global Changes View all 15 articles
The invasion or aggregation of marine biota in coastal waters has become a common problem, threatening the safety of cooling water systems in the context of environmental and climate changes. However, studies on issues of coastal nuclear power plants from the view of marine ecology have mostly focused on the thermal effluent, entrainment effect on organisms, residual chlorine, radioactivity, etc. There is a lack of information on the safety of cooling water systems in nuclear power plants blocked by marine biota. Based on field and historical datasets, this study screened phytoplankton species that may potentially block the cooling water system of the Fuqing nuclear power plant on the Southeast China coast, identified the phytoplankton risk and a risk calendar, and established a catalog of risk phytoplankton species for the cooling water system. The screening criteria were simultaneously required as follows: (1) particle size of cells or group >2000 μm; (2) phytoplankton bloom or aggregation events occurred in surrounding areas; (3) and species abundance maintained at >0.5×105 cells/L in the surrounding area. Nine high-risk species were screened out that can easily form colonies, clusters, and groups, including Thalassiosira diporocyclus, Thalassiosira subtilis, Noctiluca scintillans, Phaeocystis globosa, Pseudo-nitzschia pungens, Pseudo-nitzschia delicatissima, Skeletonema costatum, Skeletonema dohrnii, Paralia sulcata. Due to small cells or the formation of short chains, some dominant phytoplankton species, even those with advantages in abundance and occurrence, had difficulty threatening the reliability of cooling water systems in nuclear power plants. The outbreak of different phytoplankton species varied daily, and the risk of blockage was highest from April to July. The biological characteristics, ecological mechanisms underlying outbreaks of high-risk phytoplankton species are reviewed and discussed, suggesting a high degree of temporal and spatial variability of blockage risk. Finally, main prevention and control measures used against high-risk phytoplankton species are provided to imply new ideas and methods for nuclear power plants. These strategies have very important practical and scientific significance.
1. Nuclear power plants face a high risk of blockage by marine biota outbreaks in cooling water systems.
2. Blockages risk from phytoplankton was concentrated from April to July.
3. Nine high-risk species of phytoplankton that can easily form colonies, clusters, and groups were screened out.
4. The biological characteristics, ecological mechanisms underlying outbreaks of high-risk phytoplankton species suggested a huge temporal and spatial variability of blockage.
5. Main prevention and control measures used against high-risk phytoplankton species are provided.
With the depletion of traditional power sources, such as coal and oil, nuclear energy has been developed and utilized by more countries as a clean and efficient alternative (Dagnall, 2005; Yang et al., 2020). Nuclear power plants are mainly located on the coast, and water supply systems using seawater as the final cooling water source are widely used. The main cooling water system, also known as the circulating water system, is the cold end system for operating the power plant (Dagnall, 2005; Liu, 2019). The operational state of the cooling water system directly affects the safety of the power plant (Alsaffar and Zheng, 1999; Ruan, 2015).
The reliability of the cooling water system in nuclear power plants is crucial for their safe operation. The intrusion or aggregation of marine biota into cooling water systems seriously threatens nuclear safety in the context of environmental and climate changes (Alsaffar and Zheng, 1999; ERPI, 2015; Fu et al., 2020). The increasing amount of waste, marine biota, sediment, sea ice, etc. can block cooling water systems (Chen, 2009; Ruan, 2015; Arefiev et al., 2015; Yan and Lu, 2016). If a large number of blockages are pumped into the system, clogging the water intake components, it will seriously threaten the normal operation and cause huge hidden dangers to the safe operation of nuclear power plants (Mark, 2012; Kumar et al., 2017; Fu et al., 2020). The World Association of Nuclear Operators (WANO) announced that 38% of the 60 blocking events from 2008 to 2015 were caused by marine biota; 38% were sand, silt, and debris; and 24% were other sources (sediment, plastic). Among the blockage events caused by marine biota since 1980, jellyfish account for 59%, phytoplankton for 11%, fish and shrimp for 10%, and microalgae and aquatic plants for 7% (ERPI, 2015; Han et al., 2018). Therefore, the aggregation and outbreak of marine biota is an important threat to cooling water systems of coastal nuclear power plants (Table 1). Table 1 indicates the most common issue occurs when jellyfish block the water intake filter screen and even cause the reactor to shut down (Matsumura et al., 2005; Mark, 2012; Florin et al., 2013). In addition to jellyfish, other marine biota have caused blockages, such as that caused by an outbreak of pelagic tunicates (Salpa fusiformis) and Euphausiids (Chae et al., 2008). In recent years, coastal nuclear power plants in China have also experienced incidents that threaten their cooling water systems, due to outbreaks of marine biota (Table 1). In July 2014, the water intake of the Hongyanhe nuclear power plant in northern China was blocked by A. aurita, resulting in a 10 day shutdown (Zhang et al., 2019). From December 2014 to February 2015, a bloom of Phaeocystis globosa erupted in the area of the Fangchenggang nuclear power plant, blocking some filtration equipment to varying degrees (He et al., 2019b; Kang et al., 2020). In January 2015, Acetes chinensis, Acetes erythraeus, and Acetes japonicus tripped the single circulating pump in Daya Bay nuclear power plant (Meng et al., 2018). In August 2015, the blocking of the Ningde nuclear power plant in Fujian by benthos, sea cucumbers Acaudina molpadioidea, was related to typhoons (Chen, 2018; Tang, 2018). Therefore, it can be seen that for phytoplankton or algae species, the blockage risk is relatively low, with relatively short time during blockage.
Table 1 List of shutdown cases or threats to nuclear power plants caused by substances and invasion or aggregation of marine biota.
The monitoring and prevention of organisms that typically block coastal nuclear power plants aims to provide an analysis of contributing factors and prevention methods for outbreaks or aggregations (Chae et al., 2008; Moreau et al., 2013; Florin et al., 2013; Arefiev et al., 2015; Fu et al., 2020). The ecological mechanisms of outbreaks of some marine biota in areas near nuclear power plants have been preliminarily analyzed. Chae et al. (2008) found that the warm surface current that occasionally extends to the nearshore area, makes Salpa fusiformis enter the cooling waters of Uljin nuclear power plant in South Korea. Prakash et al. (2012) used hydrological and seaweed migration models and found that the Salem power plant was invaded by seaweed from the intertidal swamp 3 miles away. Florin et al. (2013) traced the mussels, Mytilus edulis, that first appeared around the Bothnian Sea nuclear power plant in Sweden. Chen (2018) speculated that the block of the Ningde nuclear power plant may have been caused by the combinded effect of ocean currents caused by typhoons, the disturbance of the bottom habitat, and the self reproduction and growth of A. molpadioidea. Consequently, the biggest threat to the cooling water system of nuclear power plants is the uncertainty of outbreaks of marine biota in the context of environmental and climate changes (Deretz, 2007; King et al., 2010; Prakash et al., 2012; Kumar et al., 2017; Fu et al., 2020). In general, these outbreaks have no obvious signs or specific times, which causes problems for studying bio-blocking in cooling water systems. Changes in the marine environment and climate, the impact of thermal effluent, and species distribution are all potential factors for the aggregation or outbreak of marine biota (Kumar et al., 2017; Wu et al., 2017; Chen, 2018; Tang, 2018; Fu et al., 2020; Yu et al., 2021).
Harmful algae blooms (HABs), mainly composed of phytoplankton, are an ecological anomaly caused by the proliferation of tiny unicellular organisms under specific environmental conditions (Berdalet et al., 2017). Fujian in Southeast China has a long coastline with numerous bays. As a result of global change and human activities, marine environment pollution is increasing in this area, making Fujian prone to HABs, with the occurrence of toxic HABs increasing rapidly (Li, 2012). Specifically, in 2012, a toxic Karenia mikimotoi bloom in Fujian resulted in the death of numerous more than 70% of cultured abalones and a direct economic loss of 2.1 billion yuan, which had a serious impact on fishery production, coastal tourism, and human health. This was the most serious economic loss caused by HABs reported in China (Lin et al., 2016). Once a large number of HABs organisms gather, the rotating filter screen (usually 3 or 5 mm) of the circulating water filtration system can be blocked, tripping the seawater circulating pump of nuclear power plants (Deretz, 2007; Mark, 2012; Fu et al., 2019; He et al., 2019b; Zhang et al., 2019; Kang et al., 2020). Therefore, as a potential risk organism, phytoplankton may accumulate, with a high number of cells that then deposit on the filter screen surface. Based on field monitoring data of phytoplankton in the 15 km around Fuqing nuclear power plant, combined with historical HABs datasets from the Marine Environmental Quality Bulletin in Fujian that covered 16 years from 2006 to 2021, phytoplankton species were screened and evaluated that may potentially block the cooling water system, a three principle of screening risk phytoplankton specie was established, a catalog of risk phytoplankton species and monthly risk calendar were determined. Finally, the ecological mechanisms of high-risk phytoplankton outbreak were reviewed and discussed, and an effective solution for prevention was provided. These strategies have very important practical and scientific significance.
Field monitoring data of phytoplankton species were obtained by water sampling quantitative method that followed the UNESCO Manuals and Guides (Karlson et al., 2010). Twelve stations (Figure 1) were set within 15 km around Fuqing nuclear power plant area according to technical guidelines for sea area utilization demonstration in China (General Administration of Quality Supervision, Inspection and Quarantine of the People's Republic of China 2010). Water samples (500 mL) from the surface (0.5 m) and bottom (0.5 m above the seabed) were taken in December 2020, February 2021, April 2021, May 2021, June 2021, August 2021, and November 2021. The samples were fixed with 1% neutral formalin and brought back to the laboratory for analysis. Data on phytoplankton species included species composition, abundance, and dominant species. Dominant species were defined as the dominance ≥0.02 (Xu, 2006). HABs organisms and outbreak events were screened out from historical datasets of the Marine Environmental Quality Bulletin in Fujian on Southeast China coast between 2006 and 2021. Based on the field monitoring and historical datasets, the potential phytoplankton species of risk in the target area were screened, and the risk of blockage was assessed.
Figure 1 (A) Field monitoring stations within 15 km around Fuqing nuclear power plant area on southesast China coast. (B) Distribution map of nuclear power plants in China mainland cited from China Nuclear Energy Association (http://www.china-nea.cn/).
The treatment and analysis of phytoplankton species of risk were carried out in accordance with the relevant provisions of the Survey of Marine Biology (General Administration of Quality Supervision, Inspection and Quarantine of the People's Republic of China, 2008), Technical Guidelines for Treatment with Red Tide Disaster (General Administration of Quality Supervision, Inspection and Quarantine of the People's Republic of China, 2014). Phytoplankton species were identified and counted at 200× to 400× magnification under an inverted optical microscope (Zeiss Z1, Germany). Phytoplankton cell or group or chain length was measured using AxioVision Rel. 4.8 lnk software connected with microscopy. Phytoplankton abundance was calculated as cells/L. HABs events in Fujian coastal waters over 16 years, including phytoplankton causing species, occurrence, and duration days, were classified. According to the ecological status of phytoplankton around Fuqing nuclear power plant and the HABs events previously recorded, combined with the quantitative assignment risk level assessment method suggested by Tang (2018); Zhang et al. (2019) and Yu et al. (2021), the screening criteria for blockage organisms, catalog of potential phytoplankton species of risk, and the monthly risk calendar were obtained. Furthermore, ecological mechanisms of high-risk species outbreaks and preventive measures are discussed in the context of a comprehensive literature review. This study provides a scientific basis to carry out purposeful monitoring to control marine biota of risk for cooling water system, and also provides a reference for further research.
A total of 147 phytoplankton species were recorded in the waters 15 km around Fuqing nuclear power plant, including 117 species of diatoms that dominated the community, 25 species of dinoflagellates, two species of Cyanobacteria, and two species of Chrysophyta (Figure 2A). In terms of monthly variation, the maximum species richness was 74 in June 2021, which was the same as in October 2021. The minimum species richness was 43 in February 2021. The monthly variation of diatom species richness was basically the same as that of the total phytoplankton. However, dinoflagellates had the maximum species richness in May 2021 (Figure 2B). The abundance of phytoplankton was the highest in June 2021, followed by May 2021, and the lowest in October 2021 (Figure 2C).
Figure 2 Monthly variation of phytoplankton composition (A), species richness (B) and abundance (C) around Fuqing nuclear power plant on southeast China coast.
The monthly variation of dominant species was evident (Table 2). The dominant species taking a greater advantage in months were chain-forming, mainly including Paralia sulcata, Skeletonema costatum, Skeletonema dohrnii, Pseudo-nitzschia pungens, Pseudo-nitzschia delicatissima, Eucampia zodiacus, Chaetoceros curvisetus, Leptocylindrus danicus, and Lauderia annulata. The major dominant species were diatoms. From December 2020 to April 2021, the major dominant species remained as chain-forming P. sulcata and S. costatum. From May 2021 to July 2021, chain-forming diatoms maintained high abundance and occurrence in the surrounding waters. These species are an important component of potential blocking organisms.
Table 2 Monthly variation of phytoplankton dominant species and its dominance around Fuqing nuclear power plant on southesast China coast.
The spatial distribution of major dominant species varied significantly (Figure 3). The most highly abundant species found in waters close to the nuclear power plant were S. dohrnii in December, L. annulata, E. zodiacus, C. curvisetus in June, and P. delicatissima and Bacillaria paradoxa in July. While the most highly abundant species found offshore from the nuclear power plant was Thalassiosira diporocyclus in May, P. pungens in June, P. sulcata, and S. costatum in February (Figure 3). Specifically, the maximum abundance of S. dohrnii in December was 1.10×105 cells/L with an average of 0.57×104 cells/L; the maximum abundance of L. annulata, E. zodiacus, C. curvisetus in June were 0.54×105 cells/L, 0.90×105 cells/L, 1.10×105 cells/L, respectively; and P. delicatissima and B. paradoxa in July had the maximum abundance of 1.07×105 cells/L and 0.97×105 cells/L, respectively. Subsequently, T. diporocyclus in May had the maximum abundance of 1.13×105 cells/L with an average of 3.01×104 cells/L, while P. pungens in June had 1.11×105 cells/L in maximum. The maximum abundance of P. sulcata, and S. costatum in February were 1.03×105 cells/L and 1.11×105 cells/L, respectively.
Figure 3 Horizontal distribution of 10 major phytoplankton dominant species around Fuqing nuclear power plant on southesast China coast.
Over 16 years, HABs outbreaks along the coast of Fujian in Southeast China were seasonal (Figure 4B), with 90.5% occurring from April to July. The highest incidence period of HABs was in May and June, with dinoflagellates as the majority of HABs causing species. The occurrence of HABs in May and June accounted for 60.0% of the total number, while the duration days accounted for 46.4% during the whole year. HABs showed a downward trend from 2014 to 2021, and there was a small increase by 2021 (Figure 4A). There were 13 HABs causing species from 2006 to 2021 in common, including S. costatum, Chaetoceros sp., Thalassiosira sp., E. zodiacus, P. pungens, Prorocentrum micans, Karenia mikimotoi, Scrippsiella trochoidea, Noctiluca scintillans, Gymnodinium catenatum, Chattonella marina, Akashiwo sanguine, and Phaeocystis globosa. The phytoplankton species that repeatedly cause HABs were P. micans, S. costatum, Thalassiosira sp., N. scintillans, and A. sanguine. Specifically, P. micans caused outbreaks from April to July each year along the coast of Fujian in Southeast China (Figure 4B).
Figure 4 (A) Yearly variation and (B) monthly variation of HABs numbers and the duration days causing by major phytoplankton species in Fujian coastal waters during the last 16 years.
According to the HABs events previously recorded within 16 years along the coast of Fujian, 87 phytoplankton species belonging to 39 genera of four phyla were selected as HABs organisms around Fuqing nuclear power plant (Figure 5A). Of these, 68 species of 27 genera belonging to diatoms accounted for 78% of the total species; 15 species of 10 genera from dinoflagellate accounted for 17%; two species of two genera from Chrysophyta accounted for 2%; and two species of one genus from cyanobacteria accounted for 3% (Figure 5A).
Figure 5 Monthly variation of HABs composition (A) and causing species richness (B) around Fuqing nuclear power plant on southeast China coast.
Based on the diameter of the drum net of the circulating water system (usually 3 or 5 mm) in nuclear power plants, combined with blockage events in cooling water system documented by marine biota, the screening criteria for potential risk phytoplankton species were as follows: (1) particle size of cells or groups >2000 μm; (2) phytoplankton bloom or aggregation events recorded in surrounding areas; and (3) high species abundance of >0.5×105 cells/L in the surrounding sea area. Notably, the entire three principles should be simultaneously required instead of optional selection. Consequently, 38 potential risk species among the above 87 HABs organisms and a monthly risk calendar were established for Fuqing nuclear power plant (Table 3). This includes species with high dominance over multiple months, such as S. costaum, S. dohrnii, P. sulcata, P. pungens, and L. danicus, as well as other non-dominant species with high abundance in the adjacent waters close to the nuclear power plant. Based on field monitoring and historical datasets, the phytoplankton of risk in the sea area around Fuqing nuclear power plant could be roughly determined (Table 3). Furthermore, the HABs events recorded in the adjacent waters or offshore from the nuclear power plant were referred to (Figure 5B). The potential risk from phytoplankton species may exist in a certain area in real time. Blockages in cooling water systems may occur when phytoplankton species abundance reaches a high level. Phytoplankton species that can easily form colonies, clusters, filaments, and groups were selected as high-risk organisms (Table 3).
Table 3 Totally 38 phytoplankton species as potential risk species and a monthly risk calendar for Fuqing nuclear power plant based on screening criteria.
In terms of the relationship between nuclear power plants and the marine environment, the impact of thermal effluent on marine biology is the most well studied (Kaplan et al., 2016; Dvorniko et al., 2017; Wei et al., 2018). However, with changes in the marine environment and climate, the safety of cooling water systems has become a new research area. The safe operation of cooling water systems requires establishing a catalog of risk organisms in the surrounding area, and developing monitoring systems, early warning technologies, and preventive countermeasures (Moreau et al., 2013; Tang et al., 2017; Zhang et al., 2018; Kirsti et al., 2018; Lu et al., 2018; Yu et al., 2021). It is a complex problem, and factors such as population composition, distribution, abundance of marine biota, trends and intensity into water intake components are important issues for evaluating the threat or risk. Tang (2018) used the Analytic Hierarchy Process, which initially proposed the principle of Ningde nuclear blockage causing organisms screening and determined the possibility blockage in accordance with the criteria: 0.000 to 0.249 for low risk, 0.250 to 0.499 for medium risk, 0.500 to 0.749 for high risk, 0.750 to 1.000 for very high risk. Some studies have established a catalog of risk organisms and a biological screening index system for jellyfish. For example, Zhang et al. (2019) according to relative biomass, i.e. the number of jellyfish caught per net per hour, suggested the risk level was “High” when relative density of jellyfish was ≥ 500 ind./(net.h), called the red level; level “Medium” was when the relative density was > 300 but < 500 ind./(net.h), called the yellow level; level “Low” was > 100 and < 300 ind./(net.h), called the blue level. Subsenquently, over the past 5 years, several monitoring plans, early warning technologies, and decision support systems have been developed for marine biota that block cooling water systems (Tang et al., 2017; Zhang et al., 2018; Kirsti et al., 2018; Lu et al., 2018; Meng et al., 2018).
In present study, utilizing the quantitative risk level evaluation method suggested by Tang (2018); Zhang et al. (2019) and Yu et al. (2021), the risk level of 38 potential risk phytoplankton species preliminarily screened were assigned to quantitative values of 1, 2, 3, and 4, with corresponding levels of low, medium, high, and extremely high. Consequently, a comprehensive quantitative analysis showed that the organisms of potential risk to Fuqing nuclear power plant mainly appeared between April and July, with occasional high abundance aggregation events occurring in May (Figure 6). At the species level, there were nine major high-risk species, including T. diporocyclus, T. subtilis, N. scintillans, Phaeocystis globosa, P. pungens, P. delicatissima, S. costatum, S. dohrnii, and P. sulcata (Figure 6A). There were seven species of medium risk, 22 species of low risk, and no species of extremely high risk. Temporally, the high-risk species were most abundant in June and July, and the risk of blockage was highest. In the other months, there was a low to medium risk of blockage (Figure 6B). Notably, the nine high-risk species in this study were only treated as a risk for the cooling water systems in nuclear power plants. For the entire ecosystem, these species had corresponding niches, and there was no distinction between mentions of high, medium, and low risk. The investigation and evaluation of marine biota should be guided by the data requirements of the calendar of marine organism outbreak risk (Mark, 2012; Florin et al., 2013; Tang, 2018; Zhang et al., 2019; He et al., 2019b; Yu et al., 2021).
Figure 6 (A) The quantitative risk evaluation of 38 potential risk phytoplankton species and (B) monthly quantitative risk variation of 21 top potential risk phytoplankton species for Fuqing nuclear power plant.
The increasing severity of HABs in the coastal waters of China has a close relationship with the intensified eutrophication, especially in the estuaries and bays (GEOHAB, 2010; Yu and Chen, 2019). Besides, the alteration of natural habitat by mariculture industry and other anthropogenic activities also contributes to the occurrence of HABs events (Figure 7), such as the green tides in the southern Yellow Sea. Other factors like climate change also influence the distribution, dynamics, and impacts of HABs in the coastal waters of China directly or indirectly, through the effects on water temperature, stratification, and currents (Figure 7) (Anderson et al., 2012; Glibert et al., 2018; Yu and Chen, 2019).
Figure 7 Ecological mechanism for HABs formation modified from GEOHAB (2010) suggests main environmental conditions and anthropogenic activities in coastal areas.
The presence of phytoplankton species was the main cause of HABs. Appropriate external conditions, including physical, chemical, biological, hydrological, meteorological, and other factors were the environmental cause of HABs. The formation of HABs requires certain species and specific environmental factors (Figure 7). However, predicting and understanding the ecological mechanism of HABs formations remains difficult because it has a high degree of temporal and spatial variability (GEOHAB, 2010; Anderson et al., 2012; Glibert et al., 2018; Yu and Chen, 2019). In this study, the biological characteristics and ecological mechanism of outbreaks for major high-risk phytoplankton species are discussed below.
Phaeocystis globosa belongs to the genus Phaeocystis of Haptophyta. It is a major causative agent of HABs in oceans globally, featuring a complex polymorphic life cycle, alternating between free-living solitary cells (3–8 μm) and colonial cells (maximum 3 cm) (Shen and Qi, 2021). A colony is the dominant morphotype during P. globosa blooms (Figures 8A-C). The sticky and elastic carbohydrate skins of colonies can block the cooling water system of nuclear power plants (He et al., 2019b; Wang et al., 2021). Colony skin reportedly blocked the entrance of the cooling water filtration system of the Fangchenggang Nuclear Power Plant in China (Kang et al., 2020). According to the historical datasets and field investigation of this study, Phaeocystis blooms were not found in Fuqing nuclear power plant but were detected in July and October in lower abundance (0.07×105 cells/L, 0.11×105 cells/L), so may become a germplasm source of Phaeocystis blooms (Table 3, Figure 6). In general, P. globosa is prone to bloom in coastal waters with abundant or eutrophic nutrients. The restriction of nitrogen and phosphate will inhibit the growth and life cycle transformation of P. globosa. P. globosa colonies are more susceptible to phosphate restriction than free single cells, so colonies cannot form at low phosphate concentrations (Wang, 2006). When nitrate is the nitrogen source, colonies can easily develop, while other forms of nitrogen lead to the dissipation of colonies (Tungaraza et al., 2003). The colonies can be formed in a wide range of salinities, but temperature plays a key role in the colony size (He et al., 2019a). Higher external temperatures will accelerate colony rupture, and make single cells rapidly form large colonies. Specifically, Wang and Tang (2010) found that the maximum size of the colonies can be obtained at a salinity of 28 and a temperature of 25°C or at a salinity of 25 and temperature of 20°C. In addition, the formation of P. globosa colonies also requires light intensity of more than 40 μmol/(m2s) (Deng, 2013). Water disturbance can promote the proliferation of P. globosa cells and prolong the growth time (Li et al., 2010; Cai et al., 2011). Therefore, water disturbance caused by monsoons may be one of the main factors of P. globosa blooms (Hai et al., 2010). Diatoms are often associated with the occurrence and dissipation of P. globosa blooms, especially chain diatoms, including Chaetoceros sp., Skeletonema costatum, and Pseudo-nitzschia sp. (Peperzak et al., 1998; Hamm and Rousseau, 2003). The formation and increase of P. globosa colonies causes a discrepancy in particle size between the food and the feeders, inhibits the feeding efficiency, and protects the colonies (Nejstgaard et al., 2007; Wang et al., 2011). Moreover, P. globosa can improve the inner space of colony formation when facing the feeding of mesozooplankton. In summary, the frequent occurrence of P. globosa blooms may be attributed to the competitive advantage of its life cycle transformation and colony formation.
Figure 8 Morphological observation and mensuration of four high-risk phytoplankton species for Fuqing nuclear power plant. P. globosa: (A–C), N. scintillans: (D–F), T. diporocyclus: (G–I), P. pungens: (J, K).
N. scintillans, belonging to the genus Noctiluca of Pyrrophyta, is a unicellular species with a nearly spherical body and a cell diameter of 150–2000 μm, visible to the naked eye, especially giving off blue light at night (Figures 8D-F). It has both plant attributes and some animal functions; therefore, its floristic classification includes red heterotrophic species and green amphotropic species (Mikaelyan et al., 2014; Song et al., 2016). N. scintillans blooms frequently occur on the China coast, and its occurrence can reach about 50% of the total HABs (Tang et al., 2006). During the blooms, N. scintillans are concentrated on the surface or near the surface (Turkoglu, 2013), distributed in strips or blocks of dark pink and orange, with bloom abundance of >104 cells/L (Qian et al., 1994). The N. scintillans cell is large and has colloidal substances on its surface and so it may block the cooling water system and cause potential safety hazards during outbreaks. This species has frequently caused HABs in Fujian since 2007, and near the Yangtze River Estuary in 2021, which can easily block Fuqing nuclear power plant on Southeast China coast (Table 3, Figure 6).
The population dynamics of N. scintillans are affected by many factors, such as the environment, biological characteristics, and population structure. Water temperature is the main factor affecting the seasonal variation of N. scintillans (Harrison et al., 2011). A study in Jangmok Bay, South Korea, showed that N. scintillans abundance decreased sharply when the water temperature was >28°C (Jang et al., 2010). Le Fèvre and Grall (1970) reported that, as N. scintillans is a cold-water species, high temperatures would inhibit population growth (e.g. Huang et al., 1997; Tada et al., 2004). N. scintillans is a heterotrophic dinoflagellate, and its population dynamics will also be affected by food availability. Harrison et al. (2011) reported that the abundance of zooplankton also controlled N. scintillans abundance due to competition. Miyaguchi et al. (2006) found that the large number of sexual-multiplication cells is one of the necessary conditions for N. scintillans blooms. Excessive nutrients or eutrophication are important prerequisites and foundations for N. scintillans outbreaks (Turkoglu, 2013; Baliarsingh et al., 2016). Eutrophication provides a rich nutritional basis for the phytoplankton, which is beneficial for the proliferation of N. scintillans (Xu, 2009). Furthermore, Miyaguchi et al. (2006) documented that wind speed and precipitation can significantly affect the abundance of N. scintillans. Several recent studies have demonstrated the potential of satellite remote sensing in assessing N. scintillans blooms (Van Mol et al., 2007; Qi et al., 2019). N. scintillans cells have strong absorption in the blue–green wavelengths and strong scattering in all wavelengths (Astoreca et al., 2005), leading to distinguishable spectral reflectance shapes, for example, a sharp reflectance increases from 520 to 600 nm (Van Mol et al., 2007), where the enhanced reflectance continues to near-infrared wavelengths.
Thalassiosira diporocyclus is a diatom with a rounding, convex, or flat, rarely concave, cell shell, and a circle of small or long thorns arranged radially around the shell surface (Hasle and Vertsen, 1997). In the center of the shell surface, there is a hole for secreting colloid, from which thick or thin colloidal filaments are generated, connecting the cells into a straight or slightly curved chain group (Figures 8G-I). Many T. diporocyclus cells live together in a colloidal colony (1–2 cm), while few single cells live. The colloidal colony of T. diporocyclus is solid spherical or ellipsoidal in shape and tawny or milky white. The tawny group is a normal healthy colony, while the milky white group is a colony in decline. Each colloidal colony is generally composed of T. diporocyclus cells distributed in the outer layer and pennatae diatoms embedded in the colloid (Figure 8G). This species must be identified by electron microscope, so previous historical datasets are often missed or misjudged. In this study, T. diporocyclus was listed as a high-risk species for blockages (Table 3, Figure 6). T. diporocyclus often developed into HABs along the coast of Fujian in recent years (Figure 3). Therefore, it was classified as a high-risk organism for Fuqing nuclear power plant on Southeast China coast.
In January 2002, a T. diporocyclus bloom occurred in the waters around Nanao Island on the southeast coast of China, which was the first record in China of an outbreak of T. diporocyclus (Chen et al., 2004). T. diporocyclus has a low bloom occurrence, originating from the open sea. The ecological mechanism of T. diporocyclus outbreaks may be related to the abnormal increase of water temperature caused by El Niño (Nagai et al., 1995; Miyahara et al., 1996; Chen et al., 2004). In normal years, the wind in autumn and winter seasons along the coast of China is generally northwesterly, forcing strong coastal currents from north to south. If the weather is abnormal and the northwest wind weakens while the southeast wind prevails, the southward coastal current may lessen accordingly, providing an opportunity for T. diporocyclus from the open sea to invade the coast of China (Yin et al., 1999). The occurrence of T. diporocyclus blooms is closely related to low salinity (23–28). Consequently, the sudden decrease in salinity during El Niño may also be one of the factors in the T. diporocyclus outbreak (Nagai et al., 1995; Miyahara et al., 1996; Chen et al., 2004).
Pseudo-nitzschia pungens is a typical species of the genus Pseudonitzschia, a chain-forming cosmopolitan marine pennate diatom (Casteleyn et al., 2008; Kimon et al., 2012). Cells of P. pungens are 74–142 μm long and 2.9–4.5 μm wide, with 9–15 striae in 10 μm and 9–15 fibulae in 10 μm, mainly lanceolate in both valve and girdle views, forming a long stepped colony (Hasle and Vertsen, 1997; Li, 2005) (Figures 8J, K). Blooms have been reported in Dalian, Qingdao, the Yangtze River estuary, Xiamen Port, and many harbors in the northern South China Sea (Lu and Qi, 1992; Zou et al., 1993; Qi et al., 1994; Yang and Hodgkiss, 2002). In addition, P. pungens is also one of the important toxigenic species, and reports from New Zealand (Rhodes et al., 1996), Washington (Trainer et al., 1998), Montreal Bay, and California (Bates et al., 1998) found that it produces toxicants and is poisonous to aquatic organisms and humans. Chinese scholars have also tested the toxins of P. pungens in the East China Sea, but no domoic acid has been detected (Li, 2005).
Bates et al. (1998) identified that P. pungens blooms usually occur in summer, in the form of a chain-like multicellular population, while Dortch et al. (1997) reported that P. pungens is euryhaline, with a tolerance range of 0–36, which confers an advantage in salinities of 15–20. P. pungens has a strong ability to absorb inorganic nitrogen, which may make it competitive over other species for nitrogen to form blooms. However, its ability to absorb phosphate was weak, which may be a factor limiting its proliferation in the natural environment (Zhang and Zou, 1997). Experiments suggested that the nitrogen and phosphate demand for P. pungens growth did not show a proportional increase, but a certain range was required, with a relatively rapid growth in the range of 10–32 for c(N)/c(P). When c(N)/c(P) =5 or c(N)/c(P) =100, P. pungens growth was significantly inhibited (Lu et al., 2006). A long-term field observation on the south China coast showed that P. pungens blooms were positively correlated with nitrite and total nitrogen (Chen et al., 2002). Overall in this study, P. pungens was a high-risk, potential blocking organism in the sea area around the Fuqing nuclear power plant on Southeast China coast (Table 3, Figure 6).
According to the Important Operation Event Report of WANO and relevant datasets, nuclear power plants usually adopt the “prevention first and control combined” method for blockages of marine biota in the cooling water system (Zhan and Zhao, 2005; ERPI, 2015; Tang et al., 2017; He et al., 2019b). The specific methods are mainly divided into physical, biological, and chemical prevention, according to the relevant mechanism (Mark, 2012; Wu et al., 2017; Tang, 2018; Fu et al., 2020; Yu et al., 2021). In view of the characteristics of phytoplankton outbreaks, in terms of early warning technology, focus should be on investigating phytoplankton distribution and dynamic monitoring, long-term water temperature monitoring in the thermal effluent mixed area, and satellite remote sensing monitoring (Kaplan et al., 2016; Dvorniko et al., 2017; Tang et al., 2017; Wei et al., 2018; Lu et al., 2018; Yu et al., 2021).
In terms of algae disinfection and sterilization, measures and methods with mature technology application, reasonable economic cost control, and low damage to biological resources and the marine environment are preferred (Cao et al., 2017; Yu et al., 2017; He et al., 2019b; Kang et al., 2020). Specifically, the modified clay method is a HABs emergency treatment technology that can be applied on a large scale in the field (GEOHAB, 2010; Yu et al., 2017; Getchis and Shumway, 2017). There have been many successful applications of modified clay, such as for the treatment of P. globosa in Fangchenggang nuclear power plant, in which it has been very effective in removing microalgae cells, with a removal rate of >90%. P. globosa sank into the seabed with the modified clay, where it decomposed and was inactivated (Yu et al., 2017; Cao et al., 2017). Based on the results of long-term dynamic monitoring and analyses of historical datasets, modified clay removal research should be carried out in the laboratory for potential phytoplankton outbreaks, and the modified clay formula and sterilization strategy should be optimized to provide technical support for the safety of cooling water systems in nuclear power plants (He et al., 2019b; Kang et al., 2020).
Chemical reagents are also a common method for algae bloom sterilization. These reagents include thiazolidinedione derivative TD49, suitable for semi enclosed bays, which has the highest inhibition rate of HABs at 2.0 μM (Baek et al., 2014); Sophorolipid, which significantly inhibits the activity and growth of algal cells between 20 and 5 mg/L, with no recurrence at higher concentrations (Lee et al., 2008); Hydrogen peroxide (Burson et al., 2014); Chitosan (Divakaran and Pillai, 2002), and NaOCl (Jeong et al., 2008). However, attention should be paid to the toxic effects of these chemical reagents on another marine biota (Cao et al., 2017; Yu et al., 2017).
A number of marine biota cause outbreaks, blocking cooling water systems, leading to the shutdown of nuclear power plants, while the invasion of marine biota seriously affects their safe operation. Based on the field monitoring data of phytoplankton in the sea area within 15 km of the Fuqing nuclear power plant, in combination with HABs datasets in Fujian on Southeast China coast from 2006 to 2021, this study determined the risk from phytoplankton species that may potentially block the cooling water system. Three principles of screening blocking organisms were determined, and a catalog of risk organisms and a monthly risk calendar were established. Nine high-risk species of phytoplankton that can easily form colonies, clusters, and groups in case of outbreaks were screened out in this study. The outbreak of various phytoplankton species varied between days, and the risk of blockages from phytoplankton was concentrated from April to July. In addition, a preliminary discussion on the biological characteristics and ecological mechanism of four high-risk species is given, including Phaeocystis globosa, Noctiluca scintillans, Thalassiosira diporocyclus, and Pseudo-nitzschia pungens, suggesting that the ecological mechanism of HABs had a high degree of temporal and spatial variability. As for preventive measures against high-risk phytoplankton species, the modified clay method was an effective technology, while use of chemical reagents should be aware of their toxic effects on marine biota. The results of this study show that nuclear power plants face a high risk of blockage by marine biota in cooling water systems. It is recommended that the administration of nuclear power plants establish a regular monitoring mechanism for cooling water intake safety.
The data that support the findings of this study are available from the corresponding author or the first author upon reasonable request.
YW: conceptualization, data curation, investigation, methodology, writing–original draft, and funding acquisition. XC and YL: conceptualization, funding acquisition, and validation. SZ, LC, and XT: investigation, formal analysis, and data analysis. PX and HL: project administration and supervision. All authors contributed to the article and approved the submitted version.
This study was supported by the National Natural Science Foundation of China (Nos. 41506136 and 41306115) and the Project of Ministry of Science and Technology (No. GASI-01-02-04).
The authors acknowledge Dr. Senming Tang from Hongkong University for fruitful discussion and for helping to revise the manuscript. Thanks to the team workers from Third Institute of Oceanography for HABs analysis. We would like to thank MogoEdit (https://www.mogoedit.com) for its English editing during the preparation of this manuscript. We sincerely appreciate the constructive comments and suggestions of the reviewers.
YL was employed by Fujian Fuqing Nuclear Power Company Limited.
The remaining authors declare that the research was conducted in the absence of any commercial or financial relationships that could be construed as a potential conflict of interest.
All claims expressed in this article are solely those of the authors and do not necessarily represent those of their affiliated organizations, or those of the publisher, the editors and the reviewers. Any product that may be evaluated in this article, or claim that may be made by its manufacturer, is not guaranteed or endorsed by the publisher.
Alsaffar A. M., Zheng Y. F. (1999). Water intakes - Siting and design approaches. Congress of the International Association for Hydraulics Research (Maryland, USA: Biennial congress of the International Association for Hydraulics Research), 1–7.
Anderson D. M., Cembella A. D., Hallegraeff G. M. (2012). Progress in understanding harmful algal blooms: paradigm shifts and new technologies for research, monitoring, and management. Annu. Rev. Mar. Sci. 4, 143–176. doi: 10.1146/annurev-marine-120308-081121
Arefiev N., Mikhalev M., Zotov D., Zotov K., Vatin N., Nikonova O., et al. (2015). Physical modeling of suspended sediment deposition in marine intakes of nuclear power plants. Proc. Eng. 117, 32–38. doi: 10.1016/j.proeng.2015.08.120
Astoreca R., Rousseau V., Ruddick K., Van Mol B., Parent J. Y., Lancelot C. (2005). “Optical properties of algal blooms in an eutrophicated coastal area and its relevance to remote sensing,” in Remote sensing of the coastal oceanic environment. Eds. Frouin R. J., Babin M., Sathyendranath S. (San Diego, California, USA: Proc. SPIE), 245–255.
Baek S. H., Son M., Jung S. W., Na D. H., Cho H., Yamaguchi M., et al. (2014). Enhanced species-specific chemical control of harmful and non-harmful algal bloom species by the thiazolidinedione derivative TD49. J. Appl. Phycol 26, 311–321. doi: 10.1007/s10811-013-0046-z
Baliarsingh S. K., Lotliker A. A., Trainer V. L., Wells M. L., Parida C., Sahu B. K., et al. (2016). Environmental dynamics of red Noctiluca scintillans bloom in tropical coastal waters. Mar. pollut. Bull. 111, 277–286. doi: 10.1016/j.marpolbul.2016.06.103
Bates S. S., Garrison D. L., Horner R. A. (1998). “Bloom dynamics and physiology of domoic-acid- producing pseudo-nitzschia species,” in Physiological ecology of harmful algal blooms. Eds. Anderson D. M., Cembella A. D., Hallegraeff G. M. (Heidelberg: Springer-Verlag), 267–292.
Berdalet E., Kudela R., Urban E., Enevoldsen H., Banas N. S., Bresnan E., et al. (2017). Global HAB: A new program to promote international research, observations, and modeling of harmful algal blooms in aquatic systems. Oceanography 30, 70–81. doi: 10.5670/oceanog.2017.111
Burson A., Matthijs H. C., de Bruijne W., Talens R., Hoogenboom R., Gerssen A., et al. (2014). Termination of a toxic alexandrium bloom with hydrogen peroxide. Harmful Algae 31, 125–135. doi: 10.1016/j.hal.2013.10.017
Cai Z. P., Duan L. Y., Xiao Q., Duan S. S. (2011). Simulation study on promoted proliferation of Skeletonema costatum and Phaeocystis globosa disturbed by wave. Mar. Environ. Sci. 50, 630–643. doi: 10.1631/jzus.B1000171
Cao X. H., Yu Z. M., Qiu L. X. (2017). Field experiment and emergent application of modified clays for Phaeocystis globosa blooms mitigation. Oceanologia Limnologia Sin. 48, 753–759. doi: 10.11693/hyhz20170200026
Casteleyn G., Chepurnov V. A., Leliaert F., Mann D. G., Bates S. S., Lundholm N., et al. (2008). Pseudo-nitzschia pungens (Bacillariophyceae): a cosmopolitan diatom species? Harmful Algae 7, 241–257. doi: 10.1016/j.hal.2007.08.004
Chae J., Choi H. W., Lee W. J., Kim D., Lee J. H. (2008). Distribution of a pelagic tunicate, salpa fusiformis in warm surface current of the eastern Korean waters and its impingement on cooling water intakes of uljin nuclear power plant. J. Environ. Biol. 29, 585–590.
Chen F. (2009). The principle of choosing tunnel gate location for nuclear power plants in the coastal sites in China. Nucl. Saf. 2, 25–29. doi: 10.3969/j.issn.1672-5360.2009.02.005
Chen H. (2018). Research on the causes, prevention and control measures of bio-blogging on nuclear power cold source by acaudina molpadioidea. master thesis (Shanghai: Shanghai Ocean University).
Chen S. W., Gao Y. H., Du H., Dong Q. X., Huang C. J. (2004). First recording of Thalassiosira diporocyclus bloom in the southeast China Sea. Oceanologia Limnologia Sin. 35, 130–137. CNKI:SUN:HYFZ.0.2004-02-003
Chen J. F., Xu N., Wang Z. H., Huang W. J., Qi Y. Z. (2002). Dynamics of Pseudo-nitzschia spp. and environmental factors in daya bay, the south china sea. Acta Scientiae Circumstantiae 22, 743–748. CNKI:SUN:HJXX.0.2002-06-010
Dagnall S. (2005). Nuclear fission: continuing evolution of a future generation. Proc. Institution Civil Engineers-Civil Eng. 158, 12–19. doi: 10.1680/cien.2005.158.6.12
Deng K. (2013). The life cycle of phaeocystis globosa and the effects of light, nutrient and co-existing diatom, dinoflagellates (Guangzhou: Jinan University).
Deretz D. (2007). Manual reactor scram due to blocked circulating water intake screens (Washington D.C, USA: United States Nuclear Regulatory Commission).
Divakaran R., Pillai V. N. S. (2002). Flocculation of algae using chitosan. J. Appl. Phycology 14, 419–422. doi: 10.1023/A:1022137023257
Dortch Q., Robichaux R., Pool S., Milsted D., Parsons M. L. (1997). Abundance and vertical flux of Pseudo-nitzschia in the northern gulf of mexico. Mar. Ecol. Prog. 146, 249–264. doi: 10.3354/meps146249
Dvorniko A. Y., Martyanov S. D., Ryabchenko V. A., Eremina T. R., Isaev A. V., Sein D. V. (2017). Assessment of extreme hydrological conditions in the bothnian bay, Baltic Sea, and the impact of the nuclear power plant “Hanhikivi-1” on the local thermal regime. Earth System Dynamics 8, 265–282. doi: 10.5194/esd-8-265-2017
ERPI (2015). Service water intake blockages event forecasting for minimizing impacts (Palo Alto: EPRI).
Florin A. B., Mo K., Svensson F., Schagerström E., Kautsky L., Bergström L. (2013). First records of conrad’s false mussel, mytilopsis leucophaeata (Conrad 1831) in the southern bothnian Sea, Sweden, near a nuclear power plant. Ann-Britt Florin Kerstin Mo Filip Svensson BioInvasions Records 4, 1–7. doi: 10.3391/bir.2013.2.4.07
Fu X. C., Du F. L., Pu X., Wang X., Han F. Z. (2020). Analysis on critical factors of marine organism impacts on water intake safety at nuclear power plants. J. Nucl. Eng. Radiat. Sci. 6, 1–6. doi: 10.1115/1.4048112
Fu Y. Z., Han C. W., Xu S. G. (2019). Review on mechanisms and mitigation measures of harmful algal blooms in coastal regions. Mar. Environ. Sci. 7, 146–152. doi: 10.13634/j.cnki.mes.2019.01.045
General Administration of Quality Supervision, Inspection and Quarantine of the People's Republic of China (2008). Specifications for oceanographic survey-part 5: Survey of marine biology (Beijing, China: China Standard Press), 1–40.
General Administration of Quality Supervision, Inspection and Quarantine of the People's Republic of China (2010). Technical guidelines for sea area utilization demonstration in China (Beijing, China: China Standard Press), 1–23.
General Administration of Quality Supervision, Inspection and Quarantine of the People's Republic of China (2014). Technical guidelines for treatment with red tide disaster (Beijing, China: China Standard Press), 1–70.
GEOHAB (2010). Global ecology and oceanography of harmful algal blooms: Harmful algal blooms in Asia (Paris and Newark, Delaware: IOC and SCOR), 68.
Getchis T. L., Shumway S. E. (2017). Harmful algae: An executive summary. Connecticut Sea grant college program. CTSG-17-08, 1–16.
Glibert P. M., Al-Azri A., Allen J. I., Bouwman A. F., Beusen A. H. W., Burford M. A., et al. (2018). “Key questions and recent research advances on harmful algal blooms in relation to nutrients and eutrophication,” in Global ecology and oceanography of harmful algal blooms. Eds. Glibert P. M., Berdalet E., Burford M. A., Pitcher G., Zhou M. J. (Cham: Springer), 229–259.
Hai D. N., Lam N. N., Dippner J. W. (2010). Development of Phaeocystis globosa blooms in the upwelling waters of the south central coast of Viet nam. J. Mar. Syst. 83, 253–261. doi: 10.1016/j.jmarsys.2010.04.015
Hamm C. E., Rousseau V. (2003). Composition, assimilation and degradation of Phaeocystis globosa-derived fatty acids in the north Sea. J. Sea Res. 50, 271–283. doi: 10.1016/S1385-1101(03)00044-3
Han D., Ji P., Zhao Y. J., Liu Y., Kang Z. S. (2018). Investigation and analysis of water intake blockage in binhai nuclear power plant. Water Wastewater Eng. 44, 75–79. doi: 10.13789/j.cnki.wwe1964.2018.0265
Harrison P. J., Furuya K., Glibert P. M., Xu J., Liu H. B., Yin K., et al. (2011). Geographical distribution of red and green noctiluca scintillans. J. Oceanology Limnology 29, 807–831. doi: 10.1007/s00343-011-0510-z
Hasle G. R., Vertsen E. E. (1997). “Marine diatoms,” in Identifying marine phytoplankton. Ed. Tomas C. R. (San Diego: Academic Press), 5–385.
He C., Song S. Q., Li C. W. (2019a). The spatial-temperal distribution of phaeocystis globosa colonies and related affecting factors in guangxi beibu gulf. Oceanologia Limnologia Sin. 50, 630–643. doi: 10.11693/hyhz20180800192
He L. Y., Song X. X., Yu F., Wang K., Song S. Q., Yu Z. M. (2019b). Potential risk and prevention of phytoplankton outbreak to water-cooling system in nuclear power plant in fangchenggang, guangxi. Oceanologia Limnologia Sin. 50, 700–706. doi: 10.11693/hyhz20190100004
Huang C. J., Qi S., Qi Y. Z., Lin X. (1997). The position and function of Noctiluca scintillans in its ecological community in dapeng bay, the south China Sea. Oceanologia Limnologia Sin. 28, 348–355. doi: 10.1007/BF02951625
Jang M. C., Shin K. S., Jang P. G., Lee W. J. (2010). Relationship between environmental factors and short-term variations of mesozooplankton during summer in jangmok bay, south coast of Korea. Ocean Polar Res. 32, 41–52. doi: 10.4217/OPR.2010.32.1.041
Jeong H. J., Kim J. S., Yoo Y. D., Kim S. T., Song J. Y., Kim T. H., et al. (2008). Control of the harmful alga Cochlodinium polykrikoides by the naked ciliate strombidinopsis jeokjo in mesocosm enclosures. Harmful Algae 7, 368–377. doi: 10.1016/j.hal.2007.12.004
Kang Z., Yang B., Lai J., Ning Y., Zhong Q., Lu D. L., et al. (2020). Phaeocystis globosa bloom monitoring: Based on P. globosa induced seawater viscosity modification adjacent to a nuclear power plant in qinzhou bay, china. J. Ocean Uni. China 19, 1207–1220. doi: 10.1007/s11802-020-4481-6
Kaplan E. M., Shvarts A. A., Luneva E. V., Makushenko M. E., Rumynin V. G. (2016). Transboundary aspect of assessing the impact of NPPs under construction on aquatic ecosystems: Case study of the baltiiskaya NPP. Water Resour 43, 911–922. doi: 10.1134/S0097807816070046
Karlson B., Cusack C., Bresnan E. (2010). Microscopic and molecular methods for quantitative phytoplankton analysis. IOC manuals and guides, 55 (Paris: UNESCO), 114.
Kimon K. M., Athanasios D. B., Panagiota K., Dimitra P., Georgios N., Theodore J. A. (2012). Inter- and intra-specific diversity of Pseudo-nitzschia (Bacillariophyceae) in the northeastern mediterranean. Eur. J. Phycology 47, 321–339. doi: 10.1080/09670262.2012.713998
King R. G., Seegert G., Vondruska J., Perry E. S., Dixon D. A. (2010). Factors influencing impingement at 15 Ohio river power plants. N. Am. J. Fish Manage 30, 1149–1175. doi: 10.1577/M09-121.1
Kirsti J., Matti K., Carl F., Hilppa G., Juho H., Otto H., et al. (2018). Recent meteorological and marine studies to support nuclear power plant safety in finland. Energy 165, 1102–1118. doi: 10.1016/j.energy.2018.09.033
Kumar S. B., Mohanty A. K., Das N. P. I., Satpathy K. K., Sarkar S. K. (2017). Impingement of marine organisms in a tropical atomic power plant cooling water system. Mar. pollut. Bull. 124, 555–562. doi: 10.1016/j.marpolbul.2017.07.067
Lee Y. J., Choi J. K., Kim E. K., Youn S. H., Yang E. J. (2008). Field experiments on mitigation of harmful algal blooms using a sophorolipid–yellow clay mixture and effects on marine plankton. Harmful Algae 7, 154–162. doi: 10.1016/j.hal.2007.06.004
Le Fèvre J., Grall J. R. (1970). On the relationships of noctiluca swarming off the western coast of Brittany with hydrological features and plankton characteristics of the environment. J. Exp. Mar. Biol. Ecol. 4, 287–306. doi: 10.1016/0022-0981(70)90040-7
Li A. F. (2005). Studies on the analytical methods of marine biotoxins with liquid chromatography mass spectrometry (LC-MS) (Qingdao: Institute of Oceanology, Chinese Academy of Sciences).
Li X. D. (2012). Analysis on characteristics of red tide in fujian coastal waters during the last 10 years. Environ. Sci. 33, 2210–2216. CNKI:SUN:HJKZ.0.2012-07-011
Li D. M., Gao Y. L., Tian T., Cyrus L., Yin K. D. (2010). Effects of turbulence on phytoplankton: species differences. J. Trop. Oceanography 29, 65–70. doi: 10.3969/j.issn.1009-5470.2010.06.010
Lin J. N., Tian Y., Zhang Q. C., Wang Y. F., Liu Q., Zhou M. J. (2016). The detrimental impacts of Karenia mikimotoi blooms on the abalone Haliotis discus hannai in fujian province. Mar. Environ. Sci. 35, 27–34. doi: 10.13634/j.cnki.mes.2016.01.005
Liu H. Y. (2019). The importance of cold source in nuclear power plant. Ind. Sci. Tribune 18, 69–70. CNKI:SUN:CYYT.0.2019-09-036
Lu S. H., Chen H. L., He Z. Q. (2006). The effects of different c(N)/c(P) ratios on the growth of Pseudo-nitzschia pungens. Ecol. Environ. 15, 697–701. doi: 10.3969/j.issn.1674-5906.2006.04.008
Lu H. R., Meng Y. H., Zhang X. C., Duan Y. B. (2018). Research on monitoring and early-warning system of marine organisms for the intake of nuclear power plants. Anim. Husbandry Feed Sci. 10, 236–240. CNKI:SUN:AHFS.0.2018-04-006
Lu S. H., Qi Y. Z. (1992). Main red tide causative species of da peng bay, south China Sea. J. Jinan Univ. (Natural Science) 13, 130–133.
Matsumura K., Kamiya K., Yamashita K., Hayashi F., Watanabe I., Murao Y., et al. (2005). Genetic polymorphism of the adult medusae invading an electric power station and wild polyps of aurelia aurita in wakasa bay, Japan. J. Mar. Biol. Assoc. Uk 85, 563–568. doi: 10.1017/S0025315405011483
Meng Y. H., Liu L., Guo X. J., Liu N., Liu Y. (2018). An early warning and decision support system of marine organisms in a water cooling system in a nuclear power plant. J. Dalian Ocean Univ. 33, 108–112. doi: 10.16535/j.cnki.dlhyxb.2018-020
Mikaelyan A. S., Malej A., Shiganova T. A., Turk V., Sivkovitch A. E., Musaeva E. I., et al. (2014). Populations of the red tide forming dinoflagellate Noctiluca scintillans (Macartney): A comparison between the black Sea and the northern Adriatic Sea. Harmful Algae 33, 29–40. doi: 10.1016/j.hal.2014.01.004
Miyaguchi H., Fujiki T., Kikuchi T., Kuwahara V. S., Toda A. T. (2006). Relationship between the bloom of Noctiluca scintillans and environmental factors in the coastal waters of sagami bay, Japan. J. Plankton Res. 28, 313–324. doi: 10.1093/plankt/fbi127
Miyahara K., Nagai S., Itakura S., Yamamoto K., Fujisawa K., Iwamoto T., et al. (1996). First record of a bloom of thalassiosira diporocyclus in the eastern seto inland Sea. Fisheries Sci. 62, 878–882. doi: 10.2331/fishsci.62.878
Moreau O., Beddek K., Clénet S., Le Menach Y. (2013). Stochastic nondestructive testing simulation: sensitivity analysis applied to material properties in clogging of nuclear power plant steam generators. IEEE Trans. Magnetics 49, 1873–1876. doi: 10.1109/TMAG.2013.2243409
Nagai S., Miyahara K., Hori Y. (1995). Blooming of Thalassiosira sp. in harima-nada, the eastern seto inland Sea, in the winters of 1994 and 1995. Bull. Hyogo Prefect 32, 9–17.
Nejstgaard J. C., Tang K. W., Steinke M., Dutz J., Koski M., Long A. (2007). Phaeocystis, major link in the biogeochemical cycling of climate-relevant elements || zooplankton grazing on phaeocystis: A quantitative review and future challenges. Biogeochemistry 83, 147–172. doi: 10.1007/s10533-007-9098-y
Peperzak L., Colijn F., Gieskes W., Peeters J. (1998). Development of the diatom-phaeocystis spring bloom in the Dutch coastal zone of the north Sea: The silicon depletion versus the daily irradiance threshold hypothesis. J. Plankton Res. 20, 517–537. doi: 10.1093/plankt/20.3.517
Prakash S., Kolluru V. S., Tutton P. (2012). “Semi-Lagrangian approach to studying grassing issue on a nuclear power plant cooling water intake,” in Proceedings of 10th International Conference on Hydroscience and Engineering (ICHE 2012). (Orlando, Florida, USA)., 1–26.
Qian H. L., Liang S., Qi Y. Z., Deng A. Q., Chen J. H. (1994). Study on ecology model of Noctiluca scintillans red tide in north coastal waters of south China Sea. Ecol. Sci. 40, 39–46.
Qi L., Tsai S. F., Chen Y., Le C., Hu C. (2019). In search of red Noctiluca scintillans blooms in the East China Sea. Geophys. Res. Lett. 46, 5997–6004. doi: 10.1029/2019GL082667
Qi Y. Z., Wang J., Zheng L. (1994). “The taxonomy and bloom ecology of pseudo-nitzschia on the coasts of China,” in Proceedings IOC-WESTPAC third international scientific symposium (Bali, Indonesia: Indonesia Institute of Sciences), 88–95.
Rhodes L. L., White D., Syhre M., Atkinson M. (1996). “Pseudo-nitzschia species isolated from new Zealand coastal waters: Domoic acid production in vitro and links with shellfish toxicity,”. Eds. Yasumoto T., Oshima Y., Fukuyo Y.. Harmful and Toxic Algal Blooms (Paris: IOC UNESCO), 155–158.
Ruan G. P. (2015). Reason analysis and corresponding strategy for cooling water intake blockage at nuclear power plants. Nucl. Power Eng. 36, 151–154. doi: 10.13832/j.jnpe.2015.S1.0151
Shen P. P., Qi Y. Z. (2021). Research progress on species diversity and distribution of the genus phaeocystis. Oceanol Et Limnol Sin. 52, 1–15. doi: 10.11693/hyhz20200300085
Song S. Q., Li C., Sun J. (2016). Progress on studies of sexual reproduction in Noctiluca scintillans. Acta Ecologica Sin. 36, 2451–2459. doi: 10.5846/stxb201312212994
Tada K., Pithakpol S., Montani S. (2004). Seasonal variation in the abundance of Noctiluca scintillans in the seto inland Sea, Japan. Plankton Biol. Ecol. 51, 7–14.
Tang Y. F. (2018). Study on typical disaster-causing organisms of coastal nuclear power operation safety:Taking ningde nuclear power as an example (Shanghai: Shanghai Ocean University).
Tang Z., Cheng F., Jin X., Sun L., Bao R. Y., Liu Y. (2017). An automatic marine-organism monitoring system for the intake water of the nuclear power plant. Ann. Nucl. Energy 109, 208–211. doi: 10.1016/j.anucene.2017.05.040
Tang D. L., Di B. P., Wei G. F., Ni I. H., Oh I. S., Wang S. F. (2006). Spatial, seasonal and species variations of harmful algal blooms in the south yellow Sea and East China Sea. Hydrobiologia 568, 245–253. doi: 10.1007/s10750-006-0108-1
Trainer V. L., Adams N. G., Bill B. D., Anulacion B. F., Wekell J. C. (1998). Concentration and dispersal of a Pseudo-nitzschia bloom in Penn Cove, Washington, USA. Nat. Toxins 6, 1–13. doi: 10.1002/(SICI)1522-7189(199805/08)6:3/4<113::AID-NT14>3.0.CO;2-B
Tungaraza C., Rousseau V., Brion N., Lancelot C., Gichuki J., Baeyens W., et al. (2003). Contrasting nitrogen uptake by diatom and phaeocystis dominated phytoplankton assemblages in the north Sea. J. Exp. Mar. Biol. Ecol. 292, 19–41. doi: 10.1016/S0022-0981(03)00145-X
Turkoglu M. (2013). Red tides of the dinoflagellate Noctiluca scintillans associated with eutrophication in the Sea of marmara (the dardanelles, Turkey). Oceanologia 55, 709–732. doi: 10.5697/oc.55-3.709
Van Mol B., Ruddick K., Astoreca R., Park Y. J., Nechad B. (2007). Optical detection of a Noctiluca scintillans bloom. EARSeL eProceedings 6, 130–137. doi: 10.3354/meps132299
Wang M. (2006). Study on determination of biomass and the effects of salinity, nitrogen, phosphorus, iron on the growth of phaeocystis globosa (Guangzhou: Jinan University).
Wang K., Chen B. H., Gao Y. H., Lin H. (2021). Harmful algal blooms caused by phaeocystis globosa from 1997 to 2018 in Chinese coastal waters. Mar. pollut. Bull. 173, 112949. doi: 10.1016/j.marpolbul.2021.112949
Wang X. D., Tang K. W. (2010). Buoyancy regulation in Phaeocystis globosa scherffel colonies. Open Mar. Biol. J. 4, 115–121. doi: 10.2174/1874450801004010115
Wang X. D., Wang Y., Smith J. R. W. O. (2011). The role of nitrogen on the growth and colony development of Phaeocystis globosa (Prymnesiophyceae). Eur. J. Phycology 46, 305–314. doi: 10.1080/09670262.2011.602430
Wei X. Y., Wang Y. C., Zhang K., Dang Y. Q., Xiong X. W., Shang Z. R. (2018). Review of impact assessments of thermal discharges from power plants on aquatic biota. J. Hydroecology 39, 1–10. doi^ 978-1-5386-1243-9/18/$31.00_c2018IEEE
Wu Y. N., Wang Y. Q., Hou Q. M., Jiao F., Sun G. C. (2017). Experience feedbacks on events of nuclear power plants cold source systems blocked by oceanic foreign matter. Nucl. Saf. 16, 26–32. doi: 10.16432/j.cnki.1672-5360.2017.01.006
Xu Z. (2006). Ecological characters of the Eucalanus subcrassus population in the East China Sea. Acta Ecologica Sin. 26, 1151–1158. doi: 10.3321/j.issn:1000-0933.2006.04.024
Xu Z. L. (2009). The inter annual variations in Noctiluca scintillans abundance and eutrophication in changjiang estuary. Oceanologia Limnologia Sin. 40, 743–748. doi: 10.11693/hyhz200906019019
Yang Z. B., Hodgkiss I. J. (2002). “Potentially harmful pseudo-nitzschia species in Hong Kong coastal waters,” in Prevention and management of harmful algal blooms in the south China Sea. Eds. Ho K. C., Lin H. Y., Yu Z. X. (Hong Kong: Environmental Publication House), 123–128.
Yang J., Zhang E. H., Guo Z. H., Wu A. G., Wang B. Q., Shi L. H., et al. (2020). Recent progress of frontier nuclear energy science and technology. Sci. Technol. Rev. 38, 35–49. doi: 10.3981/j.issn.1000-7857
Yan G. C., Lu W. T. (2016). Prevention and management of invasions blockage in water intake of power plant. Ind. Technol. 14, 119–119. CNKI:SUN:CXYY.0.2016-14-091
Yin K. D., Harrison P. J., Chen J., Huang W., Qian P. Y. (1999). Red tides during spring 1998 in Hong Kong:Is El niño responsible? Mar. Ecol. Prog. Ser. 187, 289–294. doi: 10.3354/meps187289
Yu Z. M., Chen N. S. (2019). Emerging trends in red tide and major research progresses. Oceanologia Limnologia Sin. 050, 474–486. CNKI:SUN:HYFZ.0.2019-03-003
Yu Z. M., Kai W., Cao X. H., Wang B. Z., Xin X. (2017). “Feasibility and implementation of the modified clay technique in control of Phaeocytstis globosa blooms in the water-intake area of the fangchenggang nuclear power plant,” in 2017 25th International Conference on Nuclear Engineering, American Society of Mechanical Engineers Digital Collection. (Shanghai, China) 1–7. doi: 10.13634/j.cnki.mes.2021.01.019
Yu F., Xu B. T., Wu X., Bai J., Li Y. (2021). Study on the method of marine organisms investigation, screening and evaluation based on nuclear power plant cold source safety. Mar. Environ. Sci. 40, 139–143.
Zhang C. W., Guan C. J., Peng X. U., Liu G. Z., Xu Q. M., Ye J., et al. (2019). Analysis on risk organisms for the cold source water of nuclear power plant in the eastern waters of liaodong bay. Mar. Environ. Sci. 38, 41–45. doi: 10.13634/j.cnki.mes.2019.01.030
Zhang Q., Zhou S., Wang X. H., Zhang J. Q. (2018). Design and implementation of marine biological preparedness monitoring system of nuclear power plant. DEStech Trans. Comput. Sci. Eng. (wcne) 453–457. doi: 10.12783/dtcse/wcne2017/19897
Zhang C., Zou J. Z. (1997). Nutrient uptake kinetics and growth under nutrient limitation of Pseudo-nitzschia. Oceanologia Limnologia Sin. 28, 599–603. doi: 10.1007/BF02951625
Zhan L., Zhao M. (2005). Statistics and analysis of WANO human factor events. Nucl. Power Eng. 26, 291–296. doi: 10.3969/j.issn.0258-0926.2005.03.019
Keywords: phytoplankton outbreak, cooling water system, blockage risk, nuclear power plants, ecological mechanism, preventive measures
Citation: Wang Y, Chen X, Lin Y, Zhang S, Chang L, Tang X, Xiang P and Lin H (2022) Potential risk from and prevention of phytoplankton outbreaks in blocking the cooling water system in a nuclear power plant on the Southeast China coast. Front. Mar. Sci. 9:1034876. doi: 10.3389/fmars.2022.1034876
Received: 02 September 2022; Accepted: 25 October 2022;
Published: 17 November 2022.
Edited by:
Meilin Wu, South China Sea Institute of Oceanology (CAS), ChinaReviewed by:
Kaizhi Li, South China Sea Institute of Oceanology (CAS), ChinaCopyright © 2022 Wang, Chen, Lin, Zhang, Chang, Tang, Xiang and Lin. This is an open-access article distributed under the terms of the Creative Commons Attribution License (CC BY). The use, distribution or reproduction in other forums is permitted, provided the original author(s) and the copyright owner(s) are credited and that the original publication in this journal is cited, in accordance with accepted academic practice. No use, distribution or reproduction is permitted which does not comply with these terms.
*Correspondence: Peng Xiang, eGlhbmdwZW5nQHRpby5vcmcuY24=; Heshan Lin, bGluaGVzaGFuQHRpby5vcmcuY24=
†These authors share first authorship
Disclaimer: All claims expressed in this article are solely those of the authors and do not necessarily represent those of their affiliated organizations, or those of the publisher, the editors and the reviewers. Any product that may be evaluated in this article or claim that may be made by its manufacturer is not guaranteed or endorsed by the publisher.
Research integrity at Frontiers
Learn more about the work of our research integrity team to safeguard the quality of each article we publish.