- 1Institut de Ciències del Mar (ICM-CSIC), Department of Renewable Marine Resources, Passeig Maritim de la Barceloneta, Barcelona, Spain
- 2Department of Biological Sciences, University of Cape Town, Cape Town, South Africa
- 3Fisheries Management, National Department of Forestry, Fisheries and the Environment, Cape Town, South Africa
- 4Ecopath International Initiative (EII) Research Association, Barcelona, Spain
Climate and fisheries interact, often synergistically, and may challenge marine ecosystem functioning and, ultimately, seafood provision and human wellbeing that depend on them. Holistic and integrative approaches aiming at evaluating the spatial overlap between these major stressors are crucial for identifying marine regions and key fish species that require conservation priority to prevent possible future collapses. Based on highly resolved information on environmental conditions and fishing pressure from the Southern Benguela and the Agulhas Bank ecosystems off South Africa, we identified the main areas where small pelagic fish species (European anchovy Engraulis encrasicolus, Pacific sardine Sardinops sagax, and West Coast round herring Etrumeus whiteheadi) have been highly impacted in terms of unfavourable environmental conditions and fishing pressure over the period 1993-2018. We termed these areas cumulative “hotspots” of climate change and fishing impact. We also identified fishing grounds where environmental conditions are now more favourable for these pelagic fish species, terming these “bright spots” of climate change. Environmental conditions and fishing intensity show contrasting patterns between the Southern Benguela and the Agulhas Bank ecosystems, with the Southern Benguela region accumulating most of the cumulative hotspots and showing the most negative trends in CPUE (a proxy for local fish abundance). Contrastingly, bright spots, identified on the south coast but also south of Cape Town, showed more positive trends in CPUE, suggesting that they may support sustainable growth of the small pelagic fishery in the medium term. Focussing future fishing effort on these bright spots may serve to alleviate pressure on the doubly and highly impacted cumulative hotspots from the western side of the southernmost tip of Africa.
Introduction
The oceans are one of the Earth’s biomes most impacted by human activities and are showing rates of environmental change similar to mass extinction events and ecosystem collapses captured in the Earth’s geological record (Halpern et al., 2007; Burrows et al., 2011; Halpern et al., 2015). Climate change is impacting the oceans at multiple levels (from genes to communities; Scheffers et al., 2016) and in different ways (from species’ physiological or demographic responses to distribution shifts and ecological interactions; Poloczanska et al., 2013; Poloczanska et al., 2016; Coll et al., 2020). Concurrently, fishing pressure has increased over the last decades, with recent assessments showing that ca. 60% and 34% of fish stocks worldwide are completely exploited and overexploited, respectively (Rousseau et al., 2019; FAO, 2020). Combined, climate and fishing threats can produce changes in physical and biological properties of the oceans that have far-reaching consequences for marine species, food webs, and, ultimately, provisioning services and human wellbeing (Steinacher et al., 2010; McClanahan et al., 2015; du Pontavice et al., 2020; Lenoir et al., 2020).
From a global perspective, the combined effect of climate change and fishing impacts will likely reshape the patterns of catch potential between regions and different fishery sectors, potentially leading to substantial conflict and increasing impacts on marine resources (Pecl et al., 2017; Pinsky et al., 2018; Boyce et al., 2020; Mendenhall, 2020). At national and regional levels, these impacts may jeopardize food security and economic well-being for local communities engaged in these activities (e.g., Kalikoski et al., 2010; Ramírez et al., 2022). Understanding how marine ecosystems and associated provisioning services respond to the combined impacts of climate change and fishing pressure is, therefore, a major societal challenge (García Molinos et al., 2016; Pecl et al., 2017; Free et al., 2020; Ojea et al., 2020). Accordingly, there is an urgent need for assessments providing a holistic vision of the combined impacts of fishing and climate change effects on marine ecosystems and associated supporting services.
Both climate and fishing impacts are unevenly distributed in space and time (Halpern et al., 2015; Ramírez et al., 2017; Ramírez et al., 2022), which makes the implementation of spatially-explicit approximations of impact distribution a priority for identifying those areas or marine ecosystems whose states might surpass their functional thresholds (Houk et al. 2018; Ramírez et al., 2022). Overall, this represents a major challenge for the vast and sometimes remote global ocean, where biological observations and integrated measures on the spatial distribution of these impacts are scarce and difficult to obtain. This is particularly challenging for the South-eastern Atlantic Ocean, where present knowledge on the spatial-temporal distribution of climate and fishing impacts is likely insufficient to identify highly impacted areas where fisheries should be more strongly monitored and regulated to enhance ecosystem resilience in the face of climate change (Ramírez et al., 2022).
This study targets the southernmost tip of Africa and used highly resolved spatial data to identify highly impacted areas in this region, termed “hotspots”, where the combined impact of climate change and fishing intensity may threaten important small pelagic fish species. Simultaneously, we aimed to identify alternative and more ‘resilient’ areas (or “bright spots” sensu, Queirós et al., 2021) that may support sustainable growth of the small pelagic fishery in the medium term. To achieve these aims, we combined spatially-explicit information on fish densities and fish catches with multiple environmental parameters, including bottom depth, sea surface temperature (SST), sea surface salinity (SSS), and net primary production of biomass expressed as carbon per unit volume in sea water (NPP). We then performed highly-resolved spatial assessments on the overlap between climate and fishing impacts, identifying potential hotspots and bright spots, and evaluating associated trends in fish catches per unit of effort (CPUE) as a proxy for local fish abundance (Brochier et al., 2018; Butterworth et al., 2021). Management and conservation actions aimed at allocating fisheries to bright spots for alleviating pressure on hotspots could potentially contribute to the present and future environmental status of South African marine ecosystems and the sustainable exploitation of marine resources (Ramírez et al., 2021; Ramírez et al., 2022).
Material and methods
Study area
Our study focuses on South Africa (Figure 1). The study area can be divided into two subsystems: namely the true upwelling area of the Southern Benguela ecosystem (hereafter, Southern Benguela), and the Agulhas Bank System (Agulhas), which is often included in the broader consideration of the Southern Benguela ecosystem to account for biological and oceanographic interlinkages between the west and south coasts of South Africa. In these subsystems, the combined effects of climate forcing and fishing pressure have been identified as major drivers of the dynamics of the ecosystem components (Shannon et al., 2004; Jarre et al., 2015).
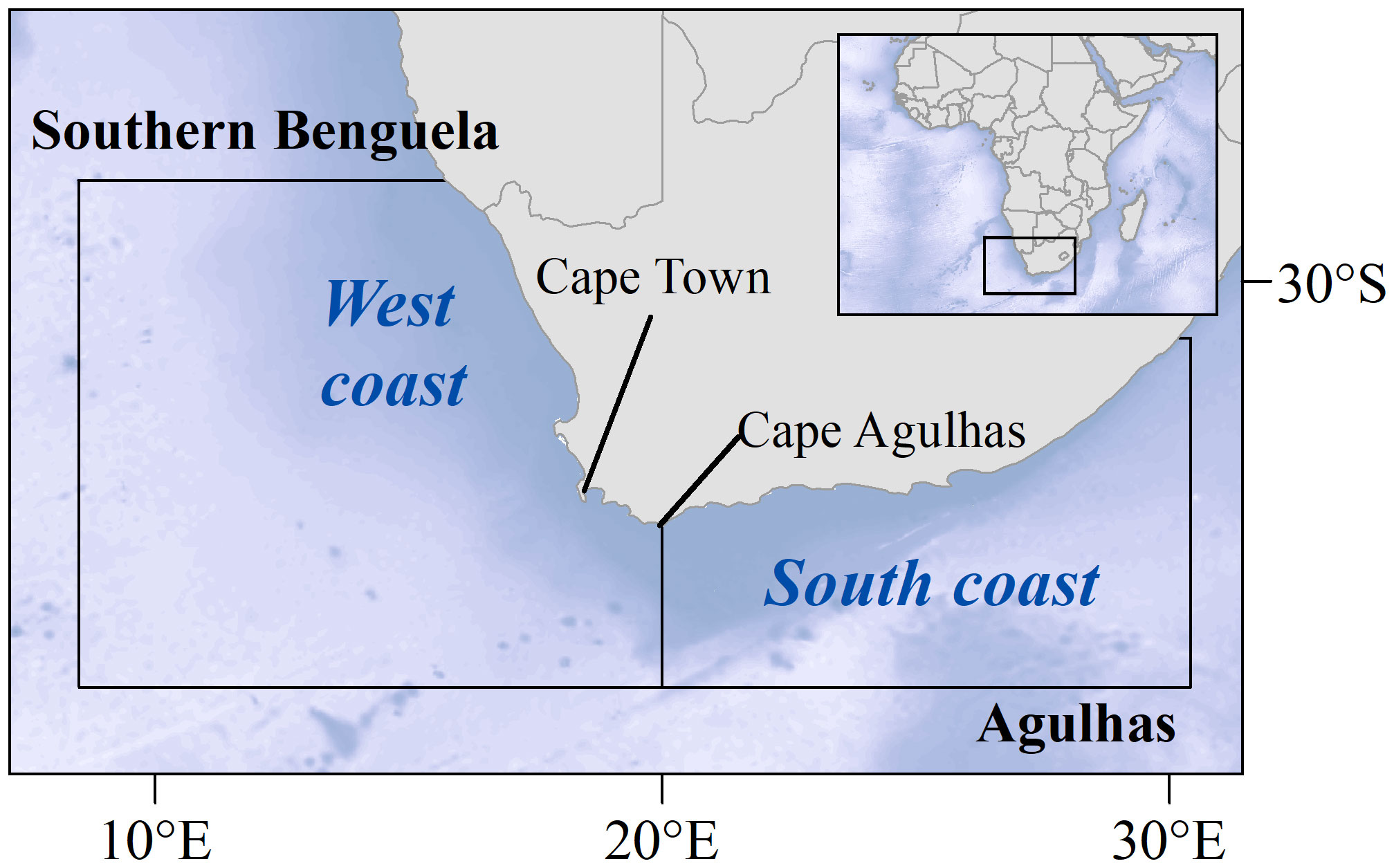
Figure 1 The study area focuses on the two oceanographic subsystems off South Africa that have different environmental regimes: namely the Southern Benguela upwelling ecosystem (hereafter, Southern Benguela) off the west coast, and the Agulhas Bank System (Agulhas) off the south coast.
Species optimal environmental ranges
We combined long-term (1993-2018) spatially explicit information on densities (g.m-2) for the ecologically and commercially important European anchovy Engraulis encrasicolus (hereafter anchovy), Pacific sardine Sardinops sagax (sardine), and West Coast round herring Etrumeus whiteheadi (herring) from annual research surveys with information on the various environmental parameters. Fish density data were collected during hydro-acoustic research surveys conducted annually (Oct-Dec; austral spring/summer) in the Southern Benguela and Agulhas systems to estimate the abundance of the three small pelagic species (see de Moor et al., 2008). The average density within ca. 10 n.mile acoustic intervals along cross-shelf survey transects of each species was provided as the mid-point (latitude and longitude) of that transect for each survey during the whole time period (1993-2018), which summed to 12 171 acoustic transects; Fig S1).
Information on bottom depth, SST, SSS and NPP were extracted to the particular coordinates for each interval transect. Because we aimed at evaluating long-term changes in yearly averaged environmental conditions driving optimal environmental habitats for target species, we considered year-specific environmental information, i.e., mean values for the whole annual period. Spatially explicit bottom depth information was obtained from the ETOPO1 Global Relief Model (NOAA, https://www.ngdc.noaa.gov/mgg/global/). Spatio-temporal information on ocean temperature was taken by deriving annual averages for the specified regions from the NOAA Optimum interpolation (OI) SST (°C) V2 high resolution dataset (0.25° horizontal resolution; sourced at https://psl.noaa.gov/data/gridded/data.noaa.oisst.v2.highres.html; accessed September 2019). SSS (PSU) and NPP (mg·m-3·day-1) were sourced from the Global Ocean Physics and Biogeochemistry Reanalyses (GLOBAL_REANALYSIS_PHY_001_030 -0.083° horizontal resolution- and GLOBAL_REANALYSIS_BIO_001_029 -0.25° horizontal resolution- for SSS and NPP, respectively; sourced at EU Copernicus Monitoring Environment Marine Service; https://marine.copernicus.eu/). Environmental time series were restricted to the 1993-2018 period; the time period for which information on SST, SSS and NPP is available.
We used generalized additive models (GAMs) implemented in ggplot R package (Wickham, 2016) to evaluate the response functions (relating biomass densities per species to environmental variables) and to identify the minimum and maximum thresholds delimiting species-specific and region-specific optimal parameter ranges (Table 1; Figures 2, S2). Our approach may fail in capturing actual environmental conditions associated to particular coordinates for each interval transect from research surveys. In addition, we did not consider the interactive effect of these multiple parameters on defining the optimal environmental ranges, as this is largely unknown, it may change across environmental ranges, and was out of the scope of this study. Thus, absolute values for the optimal parameter ranges provided in this study should be taken with caution. However, we argue that they are still useful for capturing the large-scale spatial and temporal trends in optimal environmental conditions likely driving species densities and distributions.
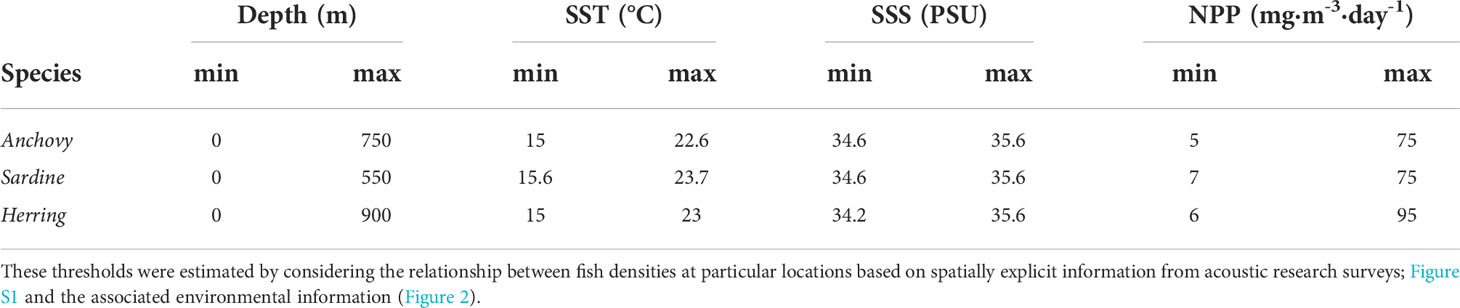
Table 1 Considered optimal ranges of depth, sea surface temperature (SST), sea surface salinity (SSS) and net primary productivity (NPP) for European anchovy (Anchovy), Pacific sardine (Sardine), and West Coast round herring (Herring) off South Africa.
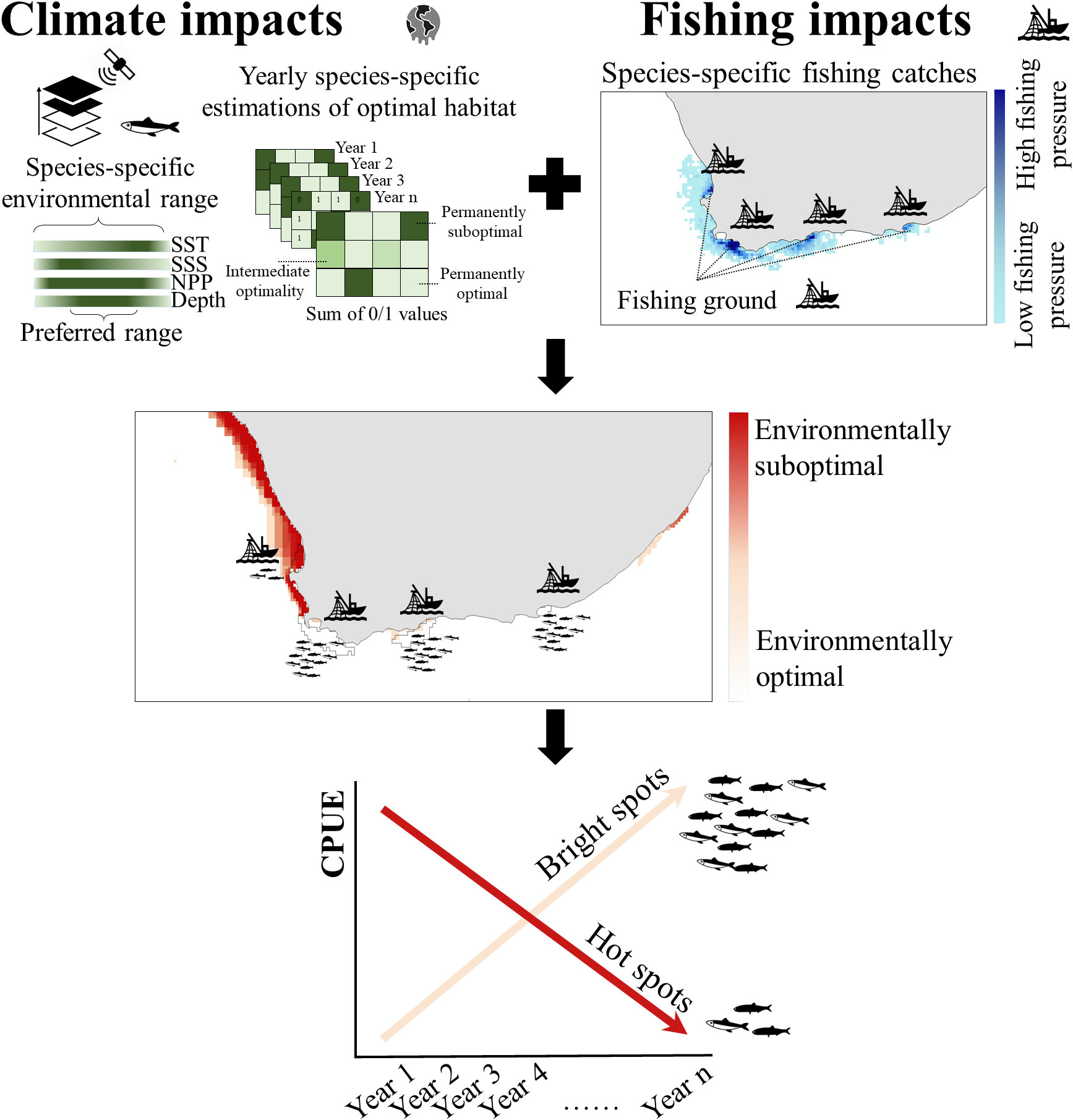
Figure 2 Workflow and work concept. Spatially-explicit assessments of climate impacts for small pelagic fish species combined with available data on industrial fish landings were used to identify highly impacted fishing grounds (cumulative hotspots); along with those with more favorable environmental conditions (bright spots). Bright spots may provide alternative areas on which to focus fishing activities and alleviate pressure on hotspots.
Spatial-temporal trends in optimal habitat availability
Based on water depth profiles and SST, SSS and NPP data, we evaluated how areas encompassing optimal parameter ranges for selected species (i.e., optimal habitats) varied temporally and spatially within the Southern Benguela and Agulhas ecosystems (Figure 2). We first estimated the total area of species-specific optimal habitats yearly within each system; i.e., optimal habitat availability: the spatial intersect between areas encompassing optimal ranges of depth, SST, SSS, and NPP, expressed in km2. As a proxy for changes in optimal habitat availability, we evaluated trends in the extent (km2) of optimal habitats during the 1993-2018 period. Our approach for estimating optimal habitat availability was restrictive as it considers as suboptimal those regions where any of these single parameters fall out of their optimal range. In addition, our approach considers that all parameters equally contribute to species’ optimal environmental conditions. This approach can be revisited, updated and refined by incorporating information on the relative weight these multiple drivers might have in shaping species-specific distributions; and by accounting for the spatial correlation among these multiple drivers. Although our approach can fail in capturing the actual extent of species-specific optimal habitats, we argue that it is still useful for evaluating the spatial-temporal trends in optimal habitat availability.
The visual inspection of estimated optimal habitat availabilities revealed a regime shift (or inflection point) in 2002 for all three species (Figure 3). As a proxy for the current trends in optimal habitat availability, we then used the slopes (and significances; α-value) of linear regressions with Gaussian distributions for the post-shift/inflection period (2003-2018) as estimates for the magnitudes and directions of observed changes in this latter period.
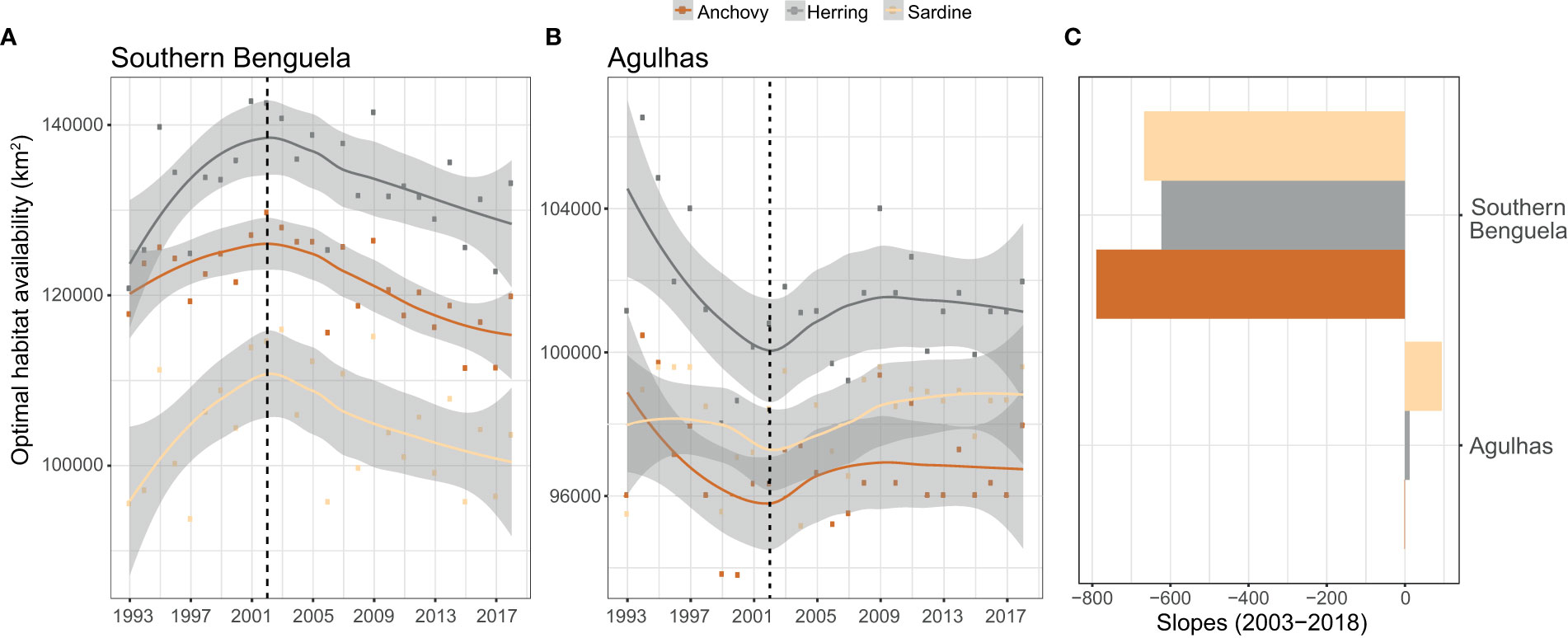
Figure 3 (A, B) Long term (1993-2018) trends in the extent (km2) of marine areas encompassing optimal ranges of depth, SST, SSS and NPP (i.e., optimal habitat availability) for three small pelagic fish species in (A) the Southern Benguela (west coast) and (B) Agulhas (south coast) systems. A regime shift was observed in 2002. Bar plots in (C) represent the slopes (magnitude) for the linear regressions on the long-term trends in the absolute values of optimal habitat availabilities for the post-inflection period (2003-2018).
The resolution of our spatial assessment on climate-driven environmental effects on small pelagic fish species was enhanced by quantifying the persistence of species-specific optimal habitats on a per-cell basis (Figure 2). We first categorized cells as optimal (with values within the optimal parameter ranges for all environmental parameters; i.e., depth, SST, SSS and NPP) or suboptimal (with values of one or more environmental parameters outside optimal ranges) for each year within the post-inflection period (2003-2018; 16 years). For each year, cells were assigned a value of 1 or 0 depending on whether they were considered as optimal or suboptimal, respectively. Previously, environmental data were resampled to match the 0.083° horizontal resolution. As a proxy for the persistency of optimal habitats or environmental conditions, we then added yearly layers to obtain a final product with cell values ranging from 0 to 16, with 0 denoting those cells that were always categorized as suboptimal (persistently suboptimal areas) and 16 indicating those that were always categorized as optimal (persistently optimal areas).
The overlapping impact of fisheries
Spatially-explicit catches (tons per haul) of anchovy, sardine and round herring for 2013-2020 (64,099 records; DFFE unpublished data) were used to evaluate fishing intensity in the study area. These data were used to estimate species-specific cumulative catches on a per-cell basis (0.083° horizontal resolution) for the whole period, to provide an overview of the distribution of fishing intensity over recent years (Figure 2).
By multiplying the species-specific cumulative catch (2013-2020) per cell by our spatial assessment of optimal environmental persistence for that cell, we obtained a proxy for the cumulative impact of climate effects and fishing pressure on small pelagic fish (Figure 2). Here, we interpret the cumulative impact as the spatial overlap between climate-driven environmental changes and fishing pressure. By combining both layers using the multiplication, we exclusively considered those marine areas where both impacts co-occur spatially (i.e., excluding those areas that were impacted only by climate forcing or by fishing pressure). The spatial output ranged from 0 (i.e., no fishing and climate impacts) to maximum values depicting those areas impacted the most by both stressors (i.e., cumulative hotspots).
The main fishing grounds for the three pelagic fish species were identified by categorizing cells according to quartiles (Q1 to Q4) covering the magnitude of their cumulative catches within the study area (Figure S3). Those regions with cumulative fishing catches within the Q4 were categorized as the main fishing grounds.
Spatially explicit information was also used for evaluating the temporal trends (2013-2020) in the yearly catches and CPUE (total catches relative to the number of fishing vessels operating) as a proxy for local fish abundance within the previously identified main fishing grounds. Trends in CPUE per fishing ground were evaluated through linear regressions including the year as a covariate, the species-specific fishing grounds as a multilevel fixed factor, along with their interaction to evaluate for contrasting trends in CPUE among fishing grounds. Model outputs were represented using the ‘lm’ method implemented in ggplot R package (Wickham, 2016).
Results
Climate change hotspots
Spatially explicit fish density information from acoustic research surveys revealed that species concentrated within particular environmental ranges of depth, SST, SSS and NPP (Table 1 and Figure S2). Optimal parameter ranges were rather similar for anchovy and sardine except for depth, with anchovy distribution reaching slightly deeper waters. Herring showed wider optimal parameter rangespt?>Based on optimal parameter ranges for small pelagic fish species, we evaluated trends in optimal habitat availability (spatial intersect between areas encompassing optimal parameter ranges, in km2) over the 1993-2018 period. The absolute values of optimal habitat differed between the three species, being highest for herring in both the Southern Benguela and Agulhas systems, and lowest for sardine in the Southern Benguela and for anchovy in the Agulhas. However, trends were similar among species but contrasted between the Southern Benguela and Agulhas regions although a general regime shift was observed in 2002 in both systems (Figure 3). In the Southern Benguela on the west coast, the extent of optimal habitat increased until 2002, and then decreased during the post-inflection period (2003-2018). An opposite trend was observed for the Agulhas system on the south coast, with optimal habitat availabilities decreasing until 2002, and then becoming slightly positive and then steady thereafter (Figure 3).
Cumulative hotspots
Overall, fish catches (in tons) were one order of magnitude higher in the Southern Benguela compared to the Agulhas system east of Cape Agulhas, where fisheries exclusively target sardine (Figure 4). Accordingly, the main fishing grounds for anchovy and herring occurred west of Cape Agulhas, whereas those for sardine also extended into Agulhas Bank waters (Figure S3).
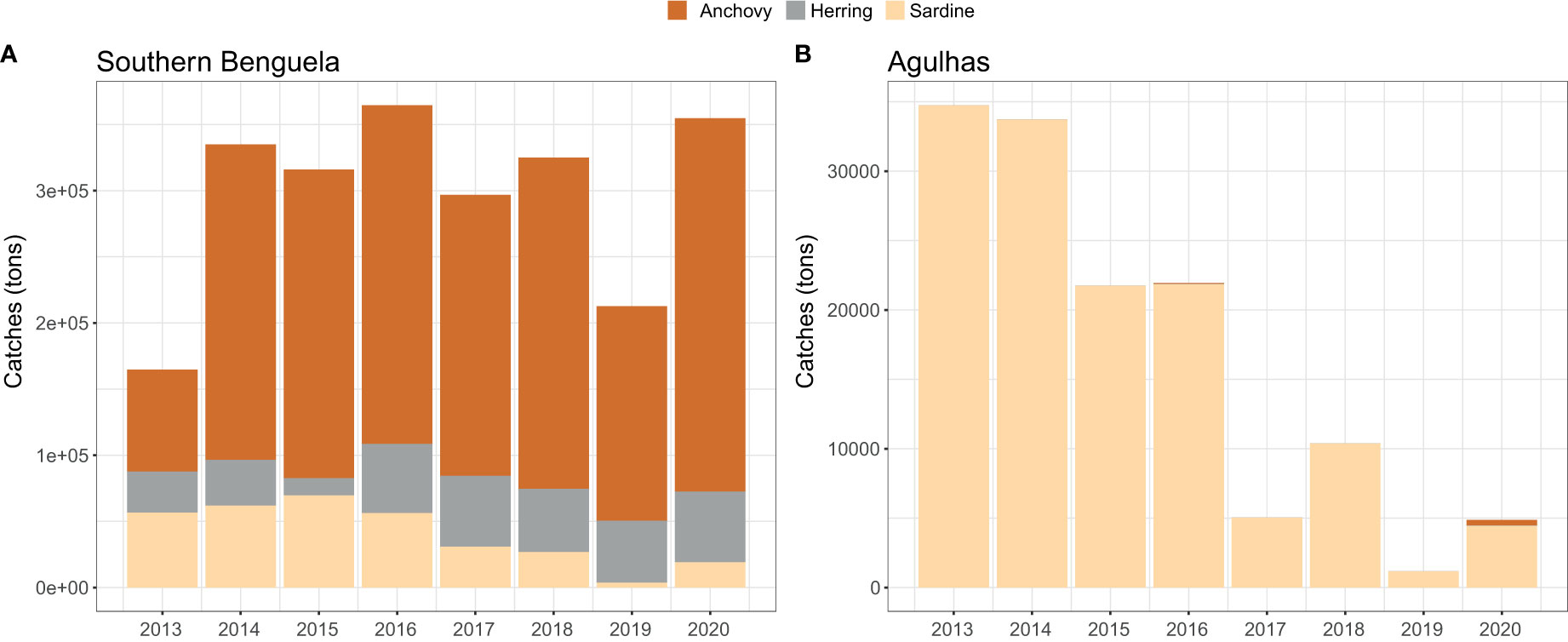
Figure 4 Yearly catches (tons) of three small pelagic fish species in (A) the Southern Benguela (west coast); and (B) the Agulhas (south coast) regions at the southernmost tip of Africa.
The fine-scale analyses revealed that marine areas where climate change hotspots and fish catches were particularly high (cumulative hotspots) largely occurred along the west coast of South Africa, north of Cape Town (Figure 5). Trends in CPUE over the 2013-2020 period contrasted among the main fishing grounds, particularly for sardine (F7, 24 = 13.48, p < 0.001) and herring (F3, 12 = 8.29, p = 0.03), but with non-significant effects for anchovy (F9, 30 = 0.55, p < 0.83). Overall, those main fishing grounds occurring near cumulative hotspots showed lower and even negative trends in CPUE over time compared to those further away from cumulative hotspots (Table 2 and Figure 6).
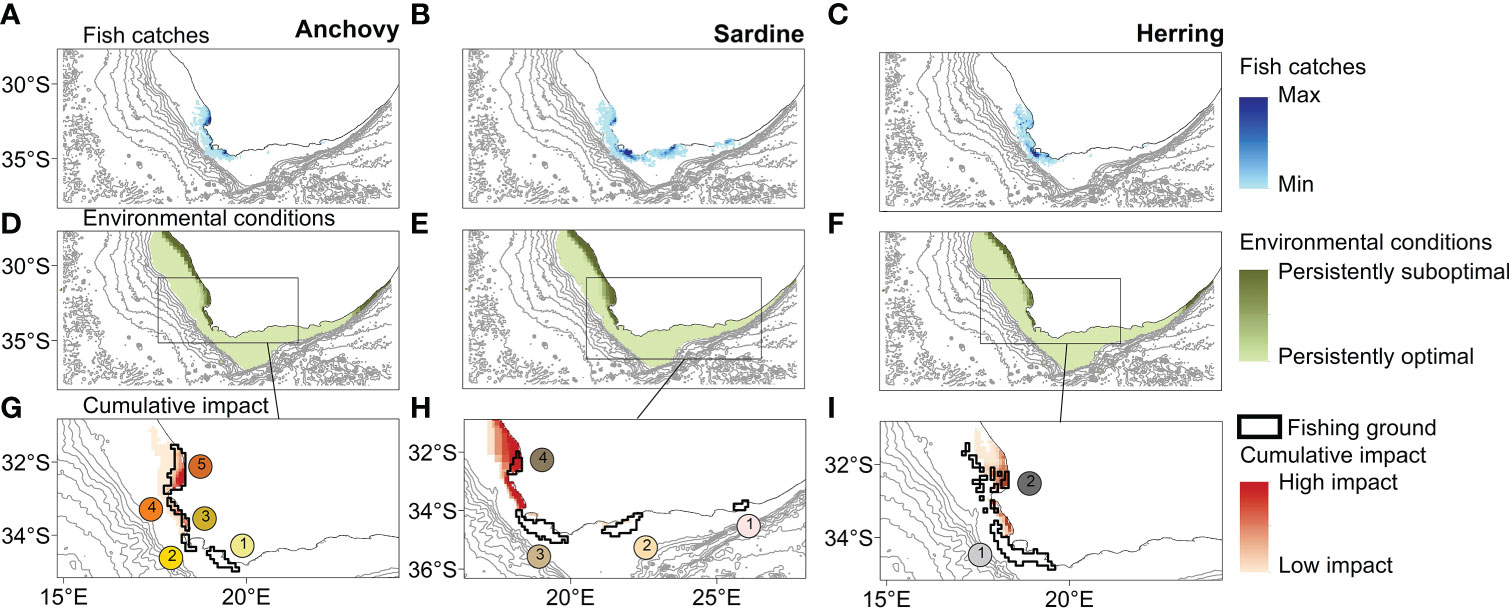
Figure 5 Spatially explicit information on catches of anchovy, sardine and herring (A-C) was combined with our spatial assessment on the persistency of optimal environmental conditions for the three species during the 2003-2018 period (D-F) to obtain a proxy for the cumulative impact of climate effects and fishing pressure on small pelagic fish (G-I). The numbers in g-i indicate the main fishing grounds for each species (see also Figure S3).
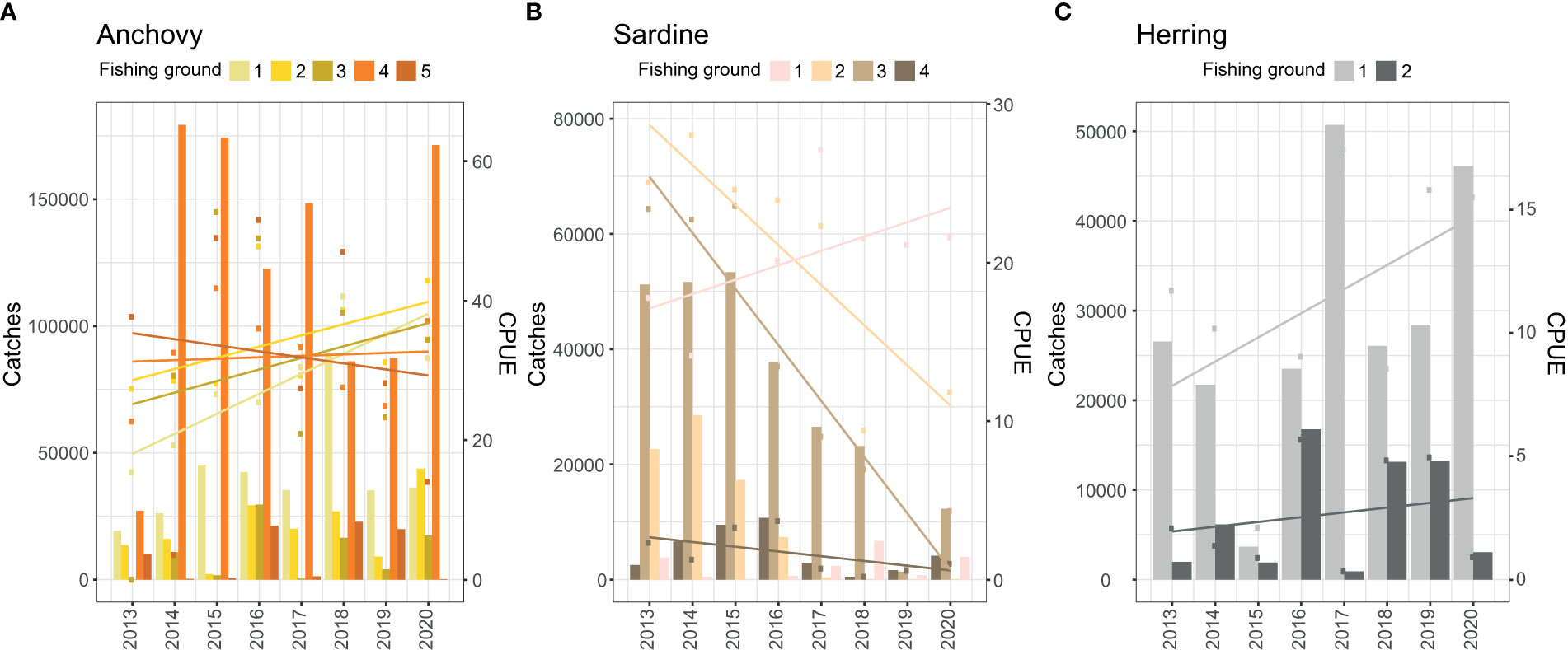
Figure 6 Trends in fish catches (bars, in tons) and CPUE (data points and regression lines; see Table 2 for linear regression slopes) against year (for the period 2013-2020) per main fishing ground (see Figs. 5 and S3 for locations) for anchovy (A), sardine (B), and herring (C), revealed that areas further from species-specific hot spots are those showing the most ‘positive’ trends in CPUE.
Bright spots
Contrasting trends in CPUE were observed for those fishing grounds where environmental conditions have become more favourable for small pelagic fish species (Table 2 and Figure 6). These areas or fishing grounds showing little or no overlap with our proxy for cumulative impact (bright spots) occur mainly on the south coast, but also south of Cape Town (Figure 5).
Discussion
Changing environmental conditions
Empirical data as to how climate impacts marine species is scarce and is largely biased towards the Northern hemisphere (Comte et al., 2020; Lenoir et al., 2020; Morato et al., 2020; Pinsky et al., 2020). In this study, we provide spatially explicit assessments of changing environmental conditions that are likely driving the dynamics and distributions of the main ecologically and economically relevant small pelagic species in South African marine ecosystems. In this area, several large-scale changes in the environment including regime shifts have occurred over recent decades, causing changes in stock sizes, relative abundance and geographic distributions of small pelagic fish species (Shannon et al., 2004; van der Lingen et al., 2006; Shannon et al., 2010; Jarre et al., 2015). Similarly, our environmental analyses revealed that a regime shift or inflection point in the extent of optimal habitat for small pelagic fish occurred in the Southern Benguela and Agulhas ecosystems in 2002. This shift differently affected the areas west and east of Cape Agulhas. Optimal habitats for anchovy, sardine and round herring (i.e., the spatial intersect between areas encompassing species-specific optimal ranges) decreased in extent during the post-inflection period (2003-2018) on the west coast, whereas optimal habitat availabilities showed slightly positive trends east of Cape Agulhas. This eastward shift in optimal habitat availability corresponds with the widely reported eastward shifts in the distribution of these pelagic species in recent decades (van der Lingen et al., 2006; Roy et al., 2007; Coetzee et al., 2008; Watermeyer et al., 2016) and is in agreement with previously identified regime shifts in demersal fish assemblages (Atkinson et al., 2012), and in mean wind and upwelling (Blamey et al., 2012) off South Africa.
Spatial distribution of fishing
There is evidence that high fishing pressures may make marine systems and fish stocks more vulnerable to unfavourable environmental conditions (Planque et al., 2010; Coll et al., 2019). Overall, fishing intensity in our study area is largely concentrated west of Cape Agulhas, where all three species are caught, compared to east of Cape Agulhas, where only sardine is caught, and catches have declined over the last decade. These spatial distributions of catch contrast with the observed spatial distribution of fish densities based on acoustic research surveys (Figure S1), which show that all three species also occur in high densities east of Cape Agulhas. Catch data reflect changing fish biomasses, and varying fishing efforts and efficiencies (Pauly et al., 2013). The contrasting spatial distributions of catches and fish densities likely reflect the historical development and investment in infrastructure in the small pelagic fishery on the west coast (Hutchings et al., 2012).
Considering the combined effects of climate and fishing
Fishing heavily in areas where climate change may result in unfavourable environmental conditions might be detrimental, not only to fished stocks but to the state of the underlying ecosystems as a whole, with far-reaching, socio-economic consequences (Jarre et al., 2015; Ramírez et al., 2021; Ramírez et al., 2022). Based on high spatially resolved observations of environmental conditions, fish densities and fishing intensity, this study adds to the evidence that the combined effects of climate and fishing impacts may jeopardize fish stocks and local fisheries in African ecosystems.
The uneven distribution of environmental trends and conditions, combined with high fishing intensity, results in doubly and highly impacted fishing grounds, our cumulative hotspots, in the Southern Benguela, north of Cape Town. Interestingly, these areas showed lower or even negative trends in CPUE in comparison to those fishing grounds south of Cape Town and in the Agulhas system, where environmental conditions were more favourable in the time period examined (1993-2018). This might be a reflection of the combined effect of climate and fishing as drivers of the changing dynamics in ecosystems (Jarre et al., 2015), which underscores the importance of considering both drivers simultaneously within an holistic, integrative and spatially-explicit framework, such as the one we provide in this study.
Regulating and/or redistributing fisheries to alleviate pressure on cumulative hotspots off the west coast of South Africa, north of Cape Town, may contribute to the necessary shifts towards more sustainable fisheries. Neighbouring marine areas in Southern Benguela, south of Cape Town, along with those occurring east of Cape Agulhas where environmental conditions seem to become more favourable to small pelagic fish, may support sustainable expansion of the small pelagic fisheries and partially absorb fishing pressure presently exerted in cumulative hotspots, thus minimizing the socio-economic impacts associated with resource depletion and stricter fishing regulations.
Reducing fishing pressure in marine areas where environmental conditions are deteriorating may support long-term sustainable fisheries by enhancing the resilience of fish stocks in the face of climate change (Ramírez et al., 2017; Ramírez et al., 2018; Ramírez et al., 2021). However, fisheries constitute an important socio-economic sector that provides a major source of food, employment and other economic benefits (Pinello et al., 2017). To ameliorate socio-economic impacts of resource depletion at a national level, part of the fishing effort could be redistributed towards neighbouring marine areas where environmental conditions are more favourable for small pelagic fish, such as the Agulhas Bank off the south coast. These bright spots could partially absorb fishing pressure, thus minimizing the socio-economic impacts associated with stricter fishing regulations that would be needed to ensure ecological sustainability in hot spots. However, shifting fishing areas comes with complex socio-economic implications as it would necessitate longer steaming distances and fishing trips for the large purse seiners operating from fishing ports currently located off South Africa’s west coast. Fisher communities on the west coast of South Africa are dependent on pelagic fishery-related jobs, with little flexibility for alternative livelihoods (Cochrane et al., 2020). Nevertheless, ecologically, minimizing small pelagic fishing intensity and catches in hot spots should benefit pelagic-feeding, seabirds including South Africa’s African Penguin, Cape Gannet and Cape Cormorant, all of which are classified as Endangered. Their foraging ranges are confined during breeding and their sustainability as species is dependent on prey availability near to breeding colonies (Crawford et al., 2019; Crawford et al., 2022).
Spatially-explicit approaches, such as the one applied in this study, may contribute to adaptive and effective management of fisheries. However, the uneven distribution of environmental drivers and fishing pressures reported in this study might not be indicative of future trends and patterns (Rilov et al., 2019). Accordingly, these analyses must be periodically revisited and updated to remain relevant. They could also incorporate model-driven projections of plausible future scenarios of fishing and climate change to anticipate future depletions or changed distributions of marine resources, and to explore management alternatives. Last, we acknowledge that many other ecological, biological, and physical variables may play roles as drivers of ecosystem dynamics. Our approach is not limited to the environmental drivers considered in this study, and can be expanded to use any set of drivers of change. For instance, the further consideration of subsurface environmental conditions may add to these environmental risk assessments by considering alternative drivers of the dynamics of other key components of these marine ecosystems. The addition of the time scale may also add further complexity to our assessments as the multiple impacts are not necessarily temporally coincident.
Data availability statement
The original contributions presented in the study are included in the article/Supplementary Material. Further inquiries can be directed to the corresponding author/s.
Author contributions
All authors conceived the work. FR and LJ extracted and analysed the data. FR drafted the paper. All authors contributed to the article and approved the submitted version.
Funding
The study was carried out within the European Union’s Horizon 2020 research and innovation programme under grant agreement No 817578 (TRIATLAS project) and the Spanish Ministry of Science and Innovation grants agreements N° PID2020-118097RB-I00 (ProOceans) and PID2021-124831OA-I00 (SOSPEN). The authors acknowledge financial support from the Spanish government through the ‘Severo Ochoa Centre of Excellence’ accreditation (CEX2019-000928-S, hereafter SO). FR, LS, JS and MC were supported by TRIATLAS. FR also received support from, SO, and the Ramón y Cajal programme (Spanish Ministerio de Ciencia e Innovación, RYC2020-030078-I).
Acknowledgments
We thank members of the MC research group (https://martacollmarine.science/) and partners from TRIATLAS for their helpful comments regarding data analyses and interpretation of results.
Conflict of interest
The authors declare that the research was conducted in the absence of any commercial or financial relationships that could be construed as a potential conflict of interest.
The reviewer TL declared a past co-authorship/collaboration with the authors CvdL, LS, MC to the handling editor.
Publisher’s note
All claims expressed in this article are solely those of the authors and do not necessarily represent those of their affiliated organizations, or those of the publisher, the editors and the reviewers. Any product that may be evaluated in this article, or claim that may be made by its manufacturer, is not guaranteed or endorsed by the publisher.
Supplementary material
The Supplementary Material for this article can be found online at: https://www.frontiersin.org/articles/10.3389/fmars.2022.1031784/full#supplementary-material
References
Atkinson L. J., Jarre A., Shannon L. J., Field J. G. (2012). “Evidence for shifts in demersal fish populations on the west coast of South Africa: 1986 to 2009,” in Global progress in ecosystem-based fisheries management (Fairbanks, USA: Alaska Sea Grant, University of Alaska Fairbanks), 45–64. doi: 10.4027/gpebfm.2012.03
Blamey L. K., Howard J. A. E., Agenbag J., Jarre A. (2012). Regime-shifts in the southern Benguela shelf and inshore region. Prog. Oceanography 106, 80–95. doi: 10.1016/j.pocean.2012.07.001
Boyce D. G., Lotze H. K., Tittensor D. P., Carozza D. A., Worm B. (2020). Future ocean biomass losses may widen socioeconomic equity gaps. Nat. Commun. 11, 2235. doi: 10.1038/s41467-020-15708-9
Brochier T., Auger P.-A., Pecquerie L., Machu E., Capet X., Thiaw M., et al. (2018). Complex small pelagic fish population patterns arising from individual behavioral responses to their environment. Prog. Oceanography 164, 12–27. doi: 10.1016/j.pocean.2018.03.011
Burrows M. T., Schoeman D. S., Buckley L. B., Moore P., Poloczanska E. S., Brander K. M., et al. (2011). The pace of shifting climate in marine and terrestrial ecosystems. Science 334, 652–655. doi: 10.1126/science.1210288
Butterworth D., Ross-Gillespie A., Coetzee J. (2020). Can CPUE inform on sardine and anchovy recruitment strength? Unpublished Scientific Working Group document FISHERIES/2020/JUL/SWG-PEL/59. (Cape Town, South Africa: Department of Environment, Forestry and Fisheries). doi: 10.25375/uct.13726003.v1
Cochrane K., Ortega-Cisneros K., Iitembu J., dos Santos C., Sauer W. (2020). Application of a general methodology to understand vulnerability and adaptability of the fisheries for small pelagic species in the Benguela countries: Angola, Namibia and South Africa. Afr. J. Mar. Sci. 42, 473–493. doi: 10.2989/1814232X.2020.1844798
Coetzee J. C., van der Lingen C. D., Hutchings L., Fairweather T. P. (2008). Has the fishery contributed to a major shift in the distribution of South African sardine? ICES J. Mar. Sci. 65, 1676–1688. doi: 10.1093/icesjms/fsn184
Coll M., Albo-Puigserver M., Navarro J., Palomera I., Dambacher J. (2019). Who is to blame? plausible pressures on small pelagic fish population changes in the northwestern Mediterranean Sea. Mar. Ecol. Prog. Ser. 617–618, 277–294. doi: 10.3354/meps12591
Coll M., Steenbeek J., Pennino M. G., Buszowski J., Kaschner K., Lotze H. K., et al. (2020). Advancing global ecological modelling capabilities to simulate future trajectories of change in marine ecosystems. Front. Mar. Science Mar. Fisheries Aquaculture Living Resour. 7. doi: 10.3389/fmars.2020.567877
Comte L., Grenouillet G., Bertrand R., Murienne J., Bourgeaud L., Hattab T., et al. (2020). BioShifts: A global geodatabase of climate-induced species redistribution over land and sea. figshare. Dataset doi: 10.6084/m9.figshare.7413365.v1
Crawford R. J. M., Sydeman W. J., Thompson S. A., Sherley R. B., Makhado A. B. (2019). Food habits of an endangered seabird indicate recent poor forage fish availability off western South Africa. ICES J. Mar. Sci 76 (5), 1344–1352. doi: 10.1093/icesjms/fsz081
Crawford R. J. M., Sydeman W. J., Tom D. B., Thayer J. A., Sherley R. B., Shannon L. J., et al. (2022). Food limitation of seabirds in the benguela ecosystem and management of their prey base. Namibian J. Environ. 6, A–13.
de Moor C., Butterworth D., Coetzee J. (2008). Revised estimates of abundance of South African sardine and anchovy from acoustic surveys adjusting for echosounder saturation in earlier surveys and attenuation effects for sardine. Afr. J. Mar. Sci. 30, 219–232. doi: 10.2989/AJMS.2008.30.2.2.552
du Pontavice H., Gascuel D., Reygondeau G., Maureaud A., Cheung W. W. L. (2020). Climate change undermines the global functioning of marine food webs. Global Change Biol. 26, 1306–1318. doi: 10.1111/gcb.14944
FAO (2020). The state of world fisheries and aquaculture. Sustainability in action (Rome, Italy). doi: 10.4060/ca9229en
Free C. M., Mangin T., Molinos J. G., Ojea E., Burden M., Costello C., et al. (2020). Realistic fisheries management reforms could mitigate the impacts of climate change in most countries. PloS One 15, e0224347. doi: 10.1371/journal.pone.0224347
García Molinos J., Halpern B. S., Schoeman D. S., Brown C. J., Kiessling W., Moore P. J., et al. (2016). Climate velocity and the future global redistribution of marine biodiversity. Nat. Clim. Change 6, 83–88. doi: 10.1038/nclimate2769
Halpern B. S., Frazier M., Potapenko J., Casey K. S., Koenig K., Longo C., et al. (2015). Spatial and temporal changes in cumulative human impacts on the world’s ocean. Nat. Commun. 6, 7615. doi: 10.1038/ncomms8615
Halpern B. S., Selkoe K. A., Micheli F., Kappel C. V. (2007). Evaluating and ranking the vulnerability of global marine ecosystems to anthropogenic threats. Conserv. Biol. 21, 1301–1315. doi: 10.1111/j.1523-1739.2007.00752.x
Houk P., Cuetos-Bueno J., Kerr A. M., McCann K. (2018). Linking fishing pressure with ecosystem thresholds and food web stability on coral reefs. Ecological Monographs 88, 109–119. doi: 10.1002/ecm.1278
Hutchings L., Jarre A., Lamont T., van den Berg M., Kirkman S. P. (2012). St Helena Bay (southern Benguela) then and now: Muted climate signals, large human impact. African Journal of Marine Science 34 (4), 559–583. doi: 10.2989/1814232X.2012.689672
Jarre A., Hutchings L., Kirkman S. P., Kreiner A., Tchipalanga P. C. M., Kainge P., et al. (2015). Synthesis: Climate effects on biodiversity, abundance and distribution of marine organisms in the Benguela. Fisheries Oceanography 24, 122–149. doi: 10.1111/fog.12086
Kalikoski D. C., Quevedo Neto P., Almudi T. (2010). Building adaptive capacity to climate variability: The case of artisanal fisheries in the estuary of the patos lagoon, Brazil. Mar. Policy 34, 742–751. doi: 10.1016/j.marpol.2010.02.003
Lenoir J., Bertrand R., Comte L., Bourgeaud L., Hattab T., Murienne J., et al. (2020). Species better track climate warming in the oceans than on land. Nat Ecol Evol 4, 1044–1059. doi: 10.1038/s41559-020-1198-2
McClanahan T., Allison E. H., Cinner J. E. (2015). Managing fisheries for human and food security. Fish Fisheries 16, 78–103. doi: 10.1111/faf.12045
Mendenhall E. (2020). Climate change increases the risk of fisheries conflict. Mar. Policy 117, 103954. doi: 10.1016/j.marpol.2020.103954
Morato T., González-Irusta J.-M., Dominguez-Carrió C., Wei C.-L., Davies A., Sweetman A. K., et al. (2020). Climate-induced changes in the suitable habitat of cold-water corals and commercially important deep-sea fishes in the north Atlantic. Global Change Biol. 26, 2181–2202. doi: 10.1111/gcb.14996
Ojea E., Lester S. E., Salgueiro-Otero D. (2020). Adaptation of fishing communities to climate-driven shifts in target species. One Earth 2, 544–556. doi: 10.1016/j.oneear.2020.05.012
Pauly D., Hilborn R., Branch T. (2013). Fisheries: Does catch reflect abundance? Nature 494, 303–306. doi: 10.1038/494303a
Pecl G. T., Araújo M. B., Bell J. D., Blanchard J., Bonebrake T. C., Chen I.-C., et al. (2017). Biodiversity redistribution under climate change: Impacts on ecosystems and human well-being. Science 355, eaai9214. doi: 10.1126/science.aai9214
Pinello D., Gee J., Dimech M. (2017). Handbook for fisheries socio-economic sample survey - principles and practice (Rome: FAO Fisheries and Aquaculture Technical Paper).
Pinsky M. L., Reygondeau G., Caddell R., Palacios-Abrantes J., Spijkers J., Cheung W. W. L. (2018). Preparing ocean governance for species on the move. Science 360, 1189–1191. doi: 10.1126/science.aat2360
Pinsky M. L., Rogers L. A., Morley J. W., Frölicher T. L. (2020). Ocean planning for species on the move provides substantial benefits and requires few trade-offs. Sci. Adv. 6, eabb8428. doi: 10.1126/sciadv.abb8428
Planque B., Fromentin J.-M., Cury P., Drinkwater K. F., Jennings S., Perry R. I., et al. (2010). How does fishing alter marine populations and ecosystems sensitivity to climate? J. Mar. Syst. 79, 403–417. doi: 10.1016/j.jmarsys.2008.12.018
Poloczanska E. S., Brown C. J., Sydeman W. J., Kiessling W., Schoeman D. S., Moore P. J., et al. (2013). Global imprint of climate change on marine life. Nat. Clim Change 3, 919–925. doi: 10.1038/nclimate1958
Poloczanska E. S., Burrows M. T., Brown C. J., García Molinos J., Halpern B. S., Hoegh-Guldberg O., et al. (2016). Responses of marine organisms to climate change across oceans. Front. Mar. Sci. 3. doi: 10.3389/fmars.2016.00062
Queirós A. M., Talbot E., Beaumont N. J., Somerfield P. J., Kay S., Pascoe C., et al. (2021). Bright spots as climate-smart marine spatial planning tools for conservation and blue growth. Global Change Biol. 27, 5514–5531. doi: 10.1111/gcb.15827
Ramírez F., Afán I., Davis L. S., Chiaradia A. (2017). Climate impacts on global hot spots of marine biodiversity. Sci. Adv. 3, e1601198. doi: 10.1126/sciadv.1601198
Ramírez F., Coll M., Navarro J., Bustamante J., Green A. J. (2018). Spatial congruence between multiple stressors in the Mediterranean Sea may reduce its resilience to climate impacts. Sci. Rep. 8, 14871. doi: 10.1038/s41598-018-33237-w
Ramírez F., Pennino M. G., Albo-Puigserver M., Steenbeek J., Bellido J. M., Coll M. (2021). SOS Small pelagics: A safe operating space for small pelagic fish in the Western Mediterranean Sea. Sci. Total Environ. 756, 144002. doi: 10.1016/j.scitotenv.2020.144002
Ramírez F., Shannon L. J., Angelini R., Steenbeek J., Coll M. (2022). Overfishing species on the move may burden seafood provision in the low-latitude Atlantic ocean. Sci. Total Environ. 836, 155480. doi: 10.1016/j.scitotenv.2022.155480
Rilov G., Mazaris A. D., Stelzenmüller V., Helmuth B., Wahl M., Guy-Haim T., et al. (2019). Adaptive marine conservation planning in the face of climate change: What can we learn from physiological, ecological and genetic studies? Global Ecol. Conserv. 17, e00566. doi: 10.1016/j.gecco.2019.e00566
Rousseau Y., Watson R. A., Blanchard J. L., Fulton E. A. (2019). Evolution of global marine fishing fleets and the response of fished resources. Proc. Natl. Acad. Sci. U.S.A. 116, 12238–12243. doi: 10.1073/pnas.1820344116
Roy C., van der Lingen C., Coetzee J., Lutjeharms J. (2007). Abrupt environmental shift associated with changes in the distribution of cape anchovy Engraulis encrasicolus spawners in the southern Benguela. Afr. J. Mar. Sci. 29, 309–319. doi: 10.2989/AJMS.2007.29.3.1.331
Scheffers B. R., De Meester L., Bridge T. C. L., Hoffmann A. A., Pandolfi J. M., Corlett R. T., et al. (2016). The broad footprint of climate change from genes to biomes to people. Science 354, aaf7671. doi: 10.1126/science.aaf7671
Shannon L. J., Coll M., Yemane D., Jouffre D., Neira S., Bertrand A., et al. (2010). Comparing data-based indicators across upwelling and comparable systems for communicating ecosystem states and trends. ICES J. Mar. Sci. 67, 807–832. doi: 10.1093/icesjms/fsp270
Shannon L. J., Field J. G., Moloney C. L. (2004). Simulating anchovy–sardine regime shifts in the southern Benguela ecosystem. Ecol. Model. 172, 269–281. doi: 10.1016/j.ecolmodel.2003.09.011
Steinacher M., Joos F., Frölicher T. L., Bopp L., Cadule P., Cocco V., et al. (2010). Projected 21st century decrease in marine productivity: A multi-model analysis. Biogeosciences 7, 979–1005. doi: 10.5194/bg-7-979-2010
van der Lingen C. D., Shannon L. J., Cury P., Kreiner A., Moloney C. L., Roux J.-P., et al. (2006). “Resource and ecosystem variability, including regime shifts, in the Benguela Current system,” in Benguela: Predicting a Large Marine Ecosystem 14. Eds. Shannon V., Hempel G., Malanotte-Rizzoli P., Moloney C., Woods J. (Amsterdam, Netherlands: Elsevier), 147–184. doi: 10.1016/S1570-0461(06)80013-3
Watermeyer K. E., Hutchings L., Jarre A., Shannon L. J. (2016). Patterns of distribution and spatial indicators of ecosystem change based on key species in the southern Benguela. PloS One 11, e0158734. doi: 10.1371/journal.pone.0158734
Wickham H. (2016) ggplot2: Elegant graphics for data analysis (New York: Springer-Verlag). Available at: https://ggplot2.tidyverse.org (Accessed July 7, 2019).
Keywords: bright spots, climate change, cumulative impacts, fishing, hotspots, marine ecosystem, small pelagic fish, southern Benguela ecosystem
Citation: Ramírez F, Shannon LJ, van der Lingen CD, Julià L, Steenbeek J and Coll M (2022) Climate and fishing simultaneously impact small pelagic fish in the oceans around the southernmost tip of Africa. Front. Mar. Sci. 9:1031784. doi: 10.3389/fmars.2022.1031784
Received: 30 August 2022; Accepted: 12 October 2022;
Published: 02 November 2022.
Edited by:
Vitor H. Paiva, University of Coimbra, PortugalReviewed by:
Lida Teneva, Independent Researcher, Sacramento, United StatesTarron Lamont, Forestry, Fisheries and the Environment (DFFE), South Africa
Copyright © 2022 Ramírez, Shannon, van der Lingen, Julià, Steenbeek and Coll. This is an open-access article distributed under the terms of the Creative Commons Attribution License (CC BY). The use, distribution or reproduction in other forums is permitted, provided the original author(s) and the copyright owner(s) are credited and that the original publication in this journal is cited, in accordance with accepted academic practice. No use, distribution or reproduction is permitted which does not comply with these terms.
*Correspondence: Francisco Ramírez, cmFtaXJlekBpY20uY3NpYy5lcw==