- 1College of Fisheries, Guangdong Ocean University, Zhanjiang, China
- 2Aquatic Animals Precision Nutrition and High-Efficiency Feed Engineering Research Centre of Guangdong Province, Zhanjiang, China
- 3Key Laboratory of Aquatic, Livestock and Poultry Feed Science and Technology in South China, Ministry of Agriculture, Zhanjiang, China
A 56-day trial investigated the impact of the dietary inclusion of cellulose with different viscosities on the growth, nutrient digestibility, serum biochemical indices, and the hepatic and gut morphology of largemouth bass juveniles. Four practical diets (42.50% protein and 13.70% lipid) were designed containing 8% microcrystalline cellulose (MC) and carboxymethyl cellulose (CMC) of 2,500, 5,000, and 6,500 mPa s dynamic viscosity [named MC, low-viscosity CMC (Lvs-CMC), medium-viscosity CMC (Mvs-CMC), and high-viscosity CMC (Hvs-CMC) groups, respectively]. Fish of a uniform size (6.0 g) were randomly assigned into 16 cages, with 40 fish per cage. The results showed that the protein and lipid deposition rates, specific growth rate, protein efficiency ratio, and the weight gain rate decreased significantly in the CMC groups compared to the MC group, whereas the feed intake and feed coefficient rate exhibited the opposite trend. Moreover, the intestinal Na+/K+-ATPase, alkaline phosphatase, and lipase activities significantly decreased in the Mvs-CMC and Hvs-CMC groups compared to the MC group, as well as the serum triglyceride, total cholesterol, and high-/low-density lipoprotein contents. The nutrient apparent digestibility significantly decreased in the CMC groups compared to the MC group. The viscerosomatic and intestinal length indices in the CMC groups and the villus height in the Hvs-CMC group were significantly lower than those in the MC group, whereas the number of gut goblet cells and muscular thickness in the Mvs-CMC and Hvs-CMC groups exhibited opposing results. The results also showed that dietary CMC damaged the hepatic and gut morphology and decreased the digestive enzyme activity, nutrient apparent digestibility, and growth of largemouth bass. In summary, viscosity is the main anti-nutritional effect of dietary CMC and soluble non-starch polysaccharides.
Introduction
Non-starch polysaccharides (NSPs) mainly consist of hemicellulose, pectin, and cellulose, which compose the plant cell wall (Ma et al., 2017). Hence, plant feed ingredients usually contain high concentrations of NSPs (Choct, 2015; Cai et al., 2019). Recently, the increasing price of fishmeal has forced the addition of more plant-based feed ingredients in aquafeed to reduce production costs (Steinberg, 2022). In addition, some binders and fillers have also been used in feed formulations to improve the physical quality of the feed, such as wheat bran and rice bran. These strategies ultimately increased the contents of NSPs in the aquafeed (Deng et al., 2021). However, dietary NSPs cannot be directly digested by fish. They are trapped in the intestine, they inhibit nutrient digestion and absorption, and they reduce fish growth (Cai et al., 2019; Ren et al., 2020; Deng et al., 2021; Liu et al., 2022a; Liu et al., 2022b).
The physiological influences of dietary NSPs on aquatic animals have recently gained increasing attention. Commonly thought to be a class of anti-nutritional factors, these biomolecules have been shown to interfere with the absorption process, reduce the nutrient apparent digestibility, and induce metabolic disorders and metabolic organ damage in fish (Glencross et al., 2012; Gao et al., 2018; Cai et al., 2019; Deng et al., 2021). Based on the solubility of NSPs in natural buffers, they can be classified into insoluble and soluble types (INSP and SNSP, respectively), and differences in solubility lead to the varied viscosities of these two NSP types (Sinha et al., 2011). To date, many studies have found that dietary INSPs and SNSPs exhibit inconsistent physiological influence on aquatic animals, with dietary SNSPs typically exhibiting stronger anti-nutritional effects than dietary INSPs (Glencross et al., 2012; Deng et al., 2021; Jiang et al., 2022; Liu et al., 2022a). Recent studies have shown that the inclusion of 16.8% SNSPs extremely impaired gut health in rainbow trout (Oncorhynchus mykiss) compared to supplementation with 24.8% NSPs (Deng et al., 2021); moreover, supplementation with 30% SNSP (pectin) extremely decreased nutrient digestibility and induced intestine and liver impairments in yellow catfish (Pelteobagrus fulvidraco) compared to supplementation with 30% INSP (cellulose) (Cai et al., 2019). Thus, it can be speculated that the inconsistent physiological effects of dietary INSPs and SNSPs on fish may be associated with the differences in their physicochemical properties, including solubility and viscosity. However, there is limited information related to this issue in fish.
It is worth noting that dietary INSPs and SNSPs exert different effects on the physicochemical properties of the digesta. For example, dietary INSPs swelled with water have been shown to increase chyme volume, while dietary SNSPs tend to increase chyme viscosity (Sinha et al., 2011). The intestine is the main digestive organ for fish; therefore, dietary INSPs and SNSPs will inevitably affect the morphology and the development of the intestine. Although scholars have confirmed that dietary NSPs affect the intestinal development and morphology in fish (Leigh et al., 2018; Cai et al., 2019; Lin et al., 2020), the relationship between the viscosity of dietary NSPs and the digestive organ’s morphology remains unclear.
Carnivorous fish have high dietary protein requirements, and fishmeal is usually added to their commercial feeds at more than 30% (Ma et al., 2020). For instance, the commercial feed of largemouth bass (Micropterus salmoides) contains 35%–50% fishmeal (Yang et al., 2022), while that of hybrid grouper (Epinephelus fuscoguttatus♀ × E. lanceolatus♂) is supplemented with 50% fishmeal, indicating that the commercial feed of carnivorous fish has broad potential for fishmeal substitution. Carnivorous fish are not equipped with the digestive physiology to cope with NSPs because their natural diet does not contain NSPs. Hence, dietary NSPs may have extreme impacts on carnivorous fish. However, there is limited knowledge on the physiological influences of dietary NSPs on carnivorous fish, and the correlation between the viscosity of dietary NSPs and their physiological effects is poorly understood. Therefore, it is necessary to investigate the correlation between the viscosity of dietary NSPs and their anti-nutritional effects in order to design feasible strategies for carnivorous fish to cope with the challenges of dietary NSPs. Toward this goal, the present trial investigated the influences of the physicochemical properties of dietary NSPs on the digestive enzyme activity, nutrient apparent digestibility, hepatic and gut morphology, and the growth of largemouth bass.
Materials and methods
Feed preparation
Four practical diets containing 8% microcrystalline cellulose (MC) and carboxymethyl cellulose (CMC) of 2,500, 5,000, and 6,500 mPa s [hereinafter MC, low-viscosity CMC (Lvs-CMC), medium-viscosity CMC (Mvs-CMC), and high-viscosity CMC (Hvs-CMC) groups, respectively] were designed (values in millipascal second denote the dynamic viscosity, which represents the internal friction force generated by the interaction of fluids between two 1-m2 flat plates with a distance of 1 m when they move relative to each other at a speed of 1 m/s). The control group data have been published in a previous study (Liu et al., 2022b). All materials were first finely milled into powder, mixed thoroughly after being screened using a 0.30-mm diameter mesh, and then accurately weighed. Subsequently, the mixture was combined with the oil source following diet formulation (Table 1) and then 30% of pure water added to make a dough. Finally, using a double screw extruder (F-75; South China University of Technology, China), the dough was extruded into a moist feed (2.0 mm) and then stored at −20°C after air drying.
Fish and farming
The juvenile largemouth bass used in this trial were supplied by the Freshwater Aquaculture Base of Guangdong Ocean University. A total of 640 fish of similar size (6.00 ± 0.01 g) were randomly assigned to 16 net cages after being fasted for 24 h. The cages with dimensions of 1.2 m × 0.8 m × 1.0 m were set in a pool. For farmed water quality: temperature, average of 29.31°C; pH, average 7.02; ammonia nitrogen, <0.02 mg/L; nitrite, <0.05 mg/L; and dissolved oxygen, >6.00 mg/L. Fish were fed to satiation twice a day (0700 and 1700 hours), and fish mortality and feeding amount were accurately recorded during the feeding trial (56 days).
Digestibility test
The digestibility test was carried out in the feeding period using yttrium trioxide (Y2O3, 99.9% purity) as the indicator. Fecal collection was initiated 2 weeks after the fish had adapted to the diet. Feces at the bottom of the cages were collected daily using a 200-mesh brail net, with intact feces selected for subsequent analysis.
Sampling strategy
The fish were counted and weighed accurately after a 24-h fast after the fish had eaten their last meal, and then they were anesthetized using 100 mg/L of an MS-222 solution. From each cage, four fish were randomly chosen for the measurement of body length and weight, and then the fish were dissected on an ice plate. The visceral mass, gut, and liver were weighed accurately and the intestinal length measured. Another group of fish (n = 4 from each cage) was randomly selected for the collection of blood samples according to the method described by Liu et al. (2022b). The proximal and distal intestines of two fish from each cage were collected into separate Eppendorf (EP) tubes and stored at −80°C for subsequent analysis. Thereafter, another batch of fish (n = 3 from each cage) was randomly collected and stored at −20°C for whole-body chemical composition analysis.
Gut and hepatic morphological observation
One hindgut (1 cm) and liver sample per cage was collected into separate EP tubes and then fixed using 4% formaldehyde solution to prepare hematoxylin–eosin (HE) staining sections according to the method described by Liu et al. (2022b). HE-stained sections were observed using a Nikon Ni-U microscope imaging system (Nikon Ni-U, Tokyo, Japan) following the method described by Huang et al. (2022).
Furthermore, another hindgut tissue was collected per cage in the MC, Lvs-CMC, and Hvs-CMC groups and then fixed with 2.5% glutaraldehyde to prepare ultrathin sections according to the method described by Liu et al. (2022b). Finally, the ultrathin sections were examined using a transmission electron microscope (HT7600; Hitachi, Tokyo, Japan) according to the method of Huang et al. (2022).
Chemical analysis
Feces, whole-body, and the diet’s approximate composition were measured using a laboratory method (AOAC, 2005), as follows: moisture, drying samples at 105°C until obtaining a constant weight; crude protein, using the Kjeldahl method; crude lipid, using the Soxhlet extraction method; and crude ash, burning the samples in a muffle furnace. Dietary viscosity was detected using a viscometer (LV-SSR type) with reference to the methods described in Liu et al. (2022b). The content of yttrium in the feed and fecal samples was measured using inductively coupled plasma mass spectrometry. Firstly, 100–200 mg sample was digested with a digestion solution (1 ml hydrogen peroxide and 6 ml nitric acid) in a microwave digestion apparatus (Multiwave PRO 41HVT56; Anton Paar, Graz, Austria). Thereafter, the digested solution of each sample was used to determine the yttrium content using mass spectrometry (7500cx; Agilent, Santa Clara, CA, USA).
Intestinal digestive enzyme activity analysis
Moist intestinal samples were first precisely weighed and then homogenized (IKA Works Asia, Bhd., Rawang, Malaysia) by adding 9x phosphate buffer (ice-cold, v/w) to obtain the supernatant for the analysis of enzyme activity. The activities of intestinal creatine kinase (CK), lipase, Na+/K+-ATPase, protease, alkaline phosphatase (AKP), and amylase and the concentration of protein were determined using commercial kits following the instructions of the manufacturer (ELISA; Shanghai Enzyme Link Biotechnology Co., Ltd., Shanghai, China).
Serum biochemical index analysis
The contents of serum low-/high-density lipoprotein cholesterol (LDL-C/HDL-C, respectively), malondialdehyde (MDA), blood urea nitrogen (BUN), triglyceride (TG), total amino acid (TAA), and total cholesterol (T-CHO) and the activities of serum superoxide dismutase (SOD), peroxidase (POD), catalase (CAT), and alanine and aspartate aminotransferase (ALT and AST, respectively) were examined using commercial kits according to the manufacturer’s instructions (Nanjing Jiancheng Bioengineering Institute, Nanjing, China).
Calculation and statistical analysis
The formulas used in the present study were as follows:
Experimental data were presented as the mean ± standard error of the mean (SEM). The percentage data were arcsine-transformed before analysis, and all data were subjected to one-way analysis of variance with SPSS software (version 22.0; Chicago, IL, USA). Tukey’s multiple range test was performed when there was a significant difference between data (p < 0.05).
Results
Growth indices
The survival rate (SR) of largemouth bass was not significantly affected by the experimental diets (p > 0.05; Table 2). The protein efficiency ratio (PER), protein deposition rate (PDR), specific growth rate (SGR), and the weight gain rate (WGR) in the CMC groups were significantly lower than those in the MC group, whereas the feed intake (FI) and feed conversion ratio (FCR) in the CMC groups exhibited the opposite results (p < 0.05). Moreover, the lipid deposition rate (LDR) decreased significantly in the CMC groups compared to that in the MC group, and this parameter also decreased significantly with increasing CMC viscosity (p < 0.05).
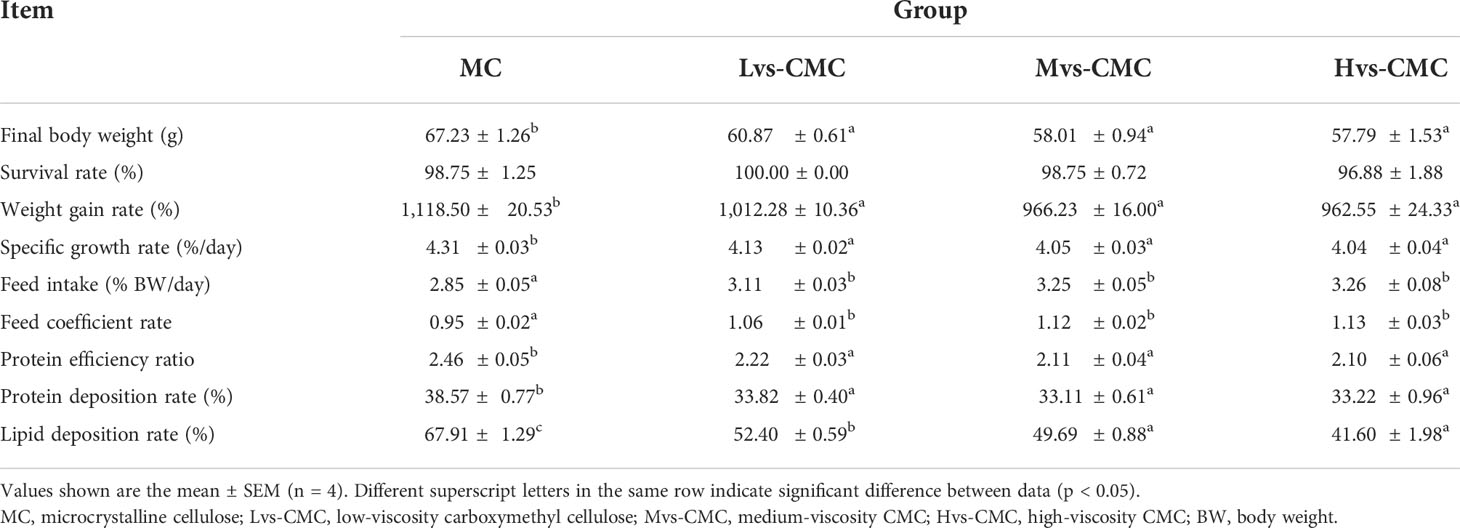
Table 2 Effects of increasing dietary viscosity on the growth and feed utilization of juvenile largemouth bass.
Chemical composition and morphological parameters
The organ index (OI) and the whole-body crude protein and moisture contents were not significantly affected by the experimental diets (p > 0.05; Table 3). The condition factor (CF) in the Hvs-CMC group was significantly lower than that in the other groups. The hepatosomatic index (HSI) in the CMC groups was significantly lower than that in the MC group; moreover, this parameter significantly decreased with increased CMC viscosity (p < 0.05). The viscerosomatic index (VSI) and intestinal length index (ILI) in the CMC groups were significantly higher than those in the MC group, with the VSI showing an increasing trend with increased CMC viscosity (p < 0.05). Moreover, the whole-body crude lipid content decreased significantly in the CMC groups compared to that in the MC group, and this parameter decreased significantly in the Hvs-CMC group compared to the Lvs-CMC and Mvs-CMC groups (p < 0.05).
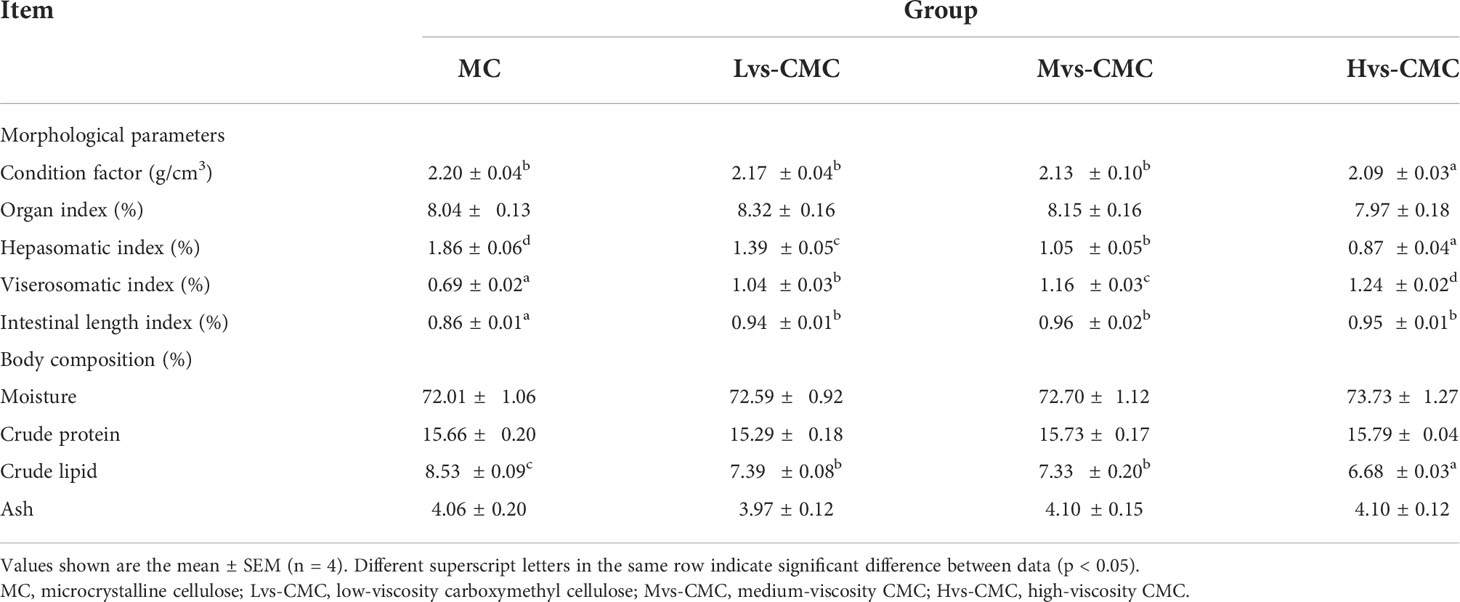
Table 3 Effects of increasing dietary viscosity on the morphological parameters and body composition of juvenile largemouth bass.
Dietary nutrient digestibility
Dietary crude lipid, crude protein, and the dry matter apparent digestibility coefficient in the CMC groups were significantly lower than those in the MC group (p < 0.05; Table 4). Additionally, the dietary dry matter apparent digestibility in the Lvs-CMC group was significantly higher than that in the Hvs-CMC group (p < 0.05).
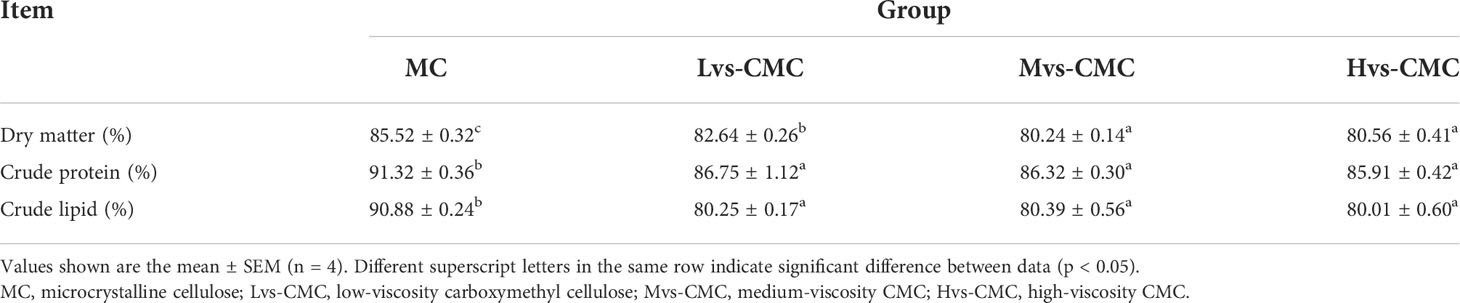
Table 4 Effects of increasing dietary viscosity on the dietary apparent digestibility of juvenile largemouth bass.
Digestive and absorption enzyme activity
The activities of intestinal amylase and CK were not significantly affected by the experimental diets (p > 0.05; Table 5). The activities of intestinal AKP and lipase in the CMC groups were significantly lower than those in the MC group (p < 0.05). Additionally, the activities of intestinal Na+/K+-ATPase and protease in the Mvs-CMC and Hvs-CMC groups were significantly lower than those in the Lvs-CMC group (p < 0.05).
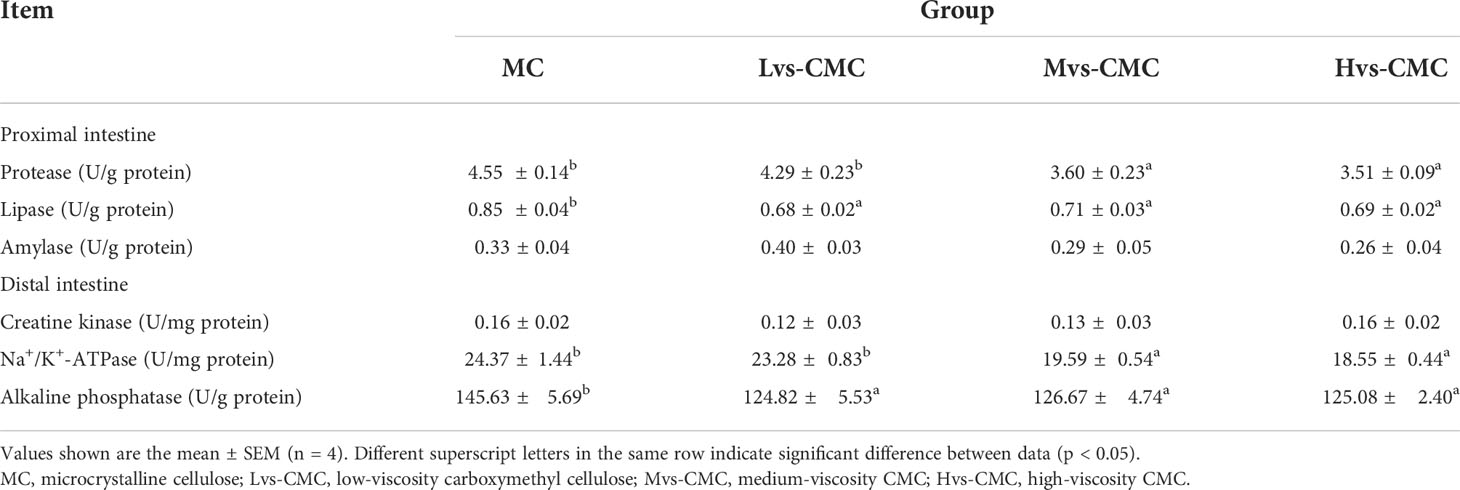
Table 5 Effects of increasing dietary viscosity on the intestinal digestive and absorptive enzyme activities of juvenile largemouth bass.
Serum biochemical indices
The concentrations of TAA and MDA and the activities of POD, SOD, and CAT in the serum were not significantly affected by the experimental diets (p > 0.05; Table 6). The concentrations of serum TG, HDL-C, LDL-C, and T-CHO in the CMC groups were significantly lower than those in the MC group (p < 0.05). Moreover, the serum HDL-C concentration in the Mvs-CMC and Hvs-CMC groups was significantly lower than that in the Lvs-CMC group (p < 0.05). Conversely, the activities of serum ALT and AST in the CMC groups were significantly higher than those in the MC group; the serum ALT activity increased significantly with increasing CMC viscosity (p < 0.05). The serum BUN content in the Hvs-CMC group was significantly higher than that in the other groups (p < 0.05).
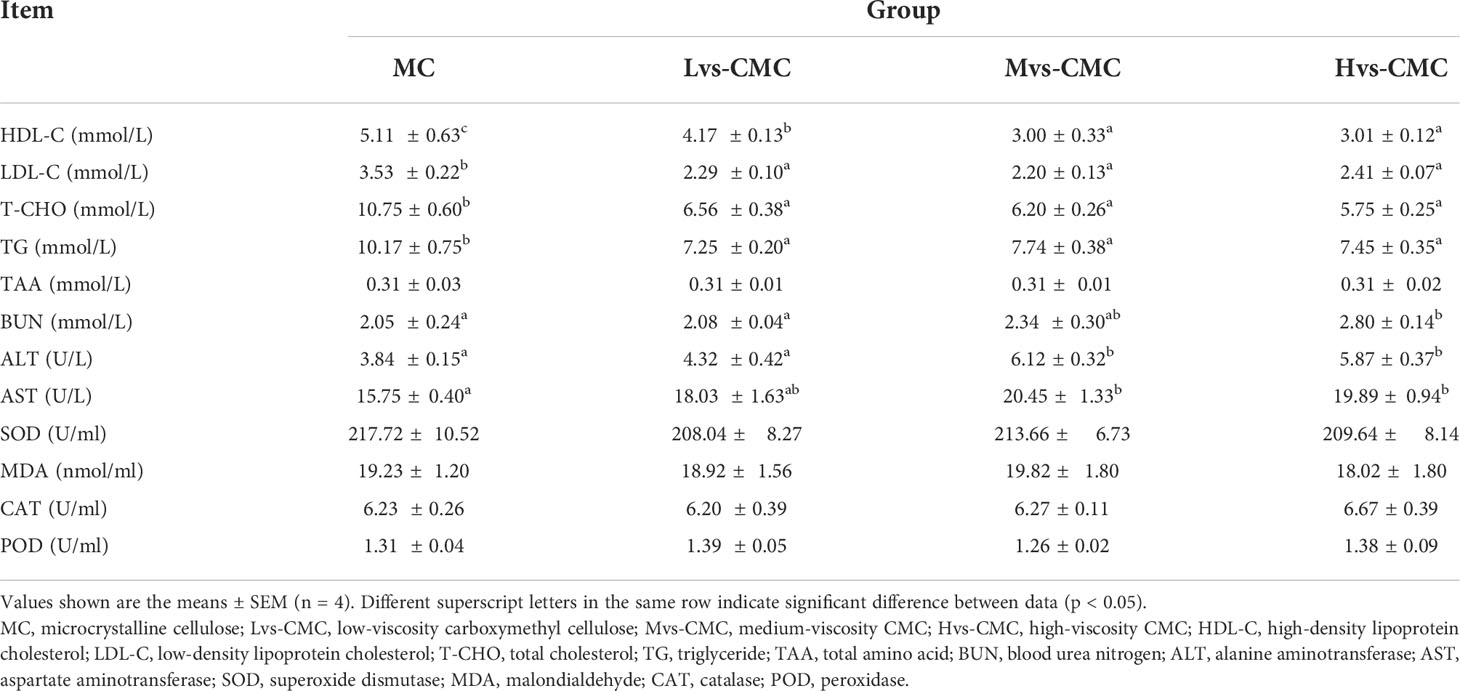
Table 6 Effects of increasing dietary viscosity on the serum biochemical indices of juvenile largemouth bass.
Hindgut and liver morphology observation
Morphological observations of the gut and liver are presented in Figures 1–3. The measurement strategy is also indicated in the figures. The gut crypt depth and villus width were not significantly affected by the experimental diets (p > 0.05; Table 7). The gut microvillus height in the CMC groups was significantly lower than that in the MC group, and this parameter decreased significantly with increasing CMC viscosity (p < 0.05). Moreover, the gut villus height in the Hvs-CMC group was significantly lower than that in the other groups (p < 0.05). The gut muscular thickness and goblet cell number in the Mvs-CMC and Hvs-CMC groups were significantly lower than those in the MC and Lvs-CMC groups (p < 0.05).
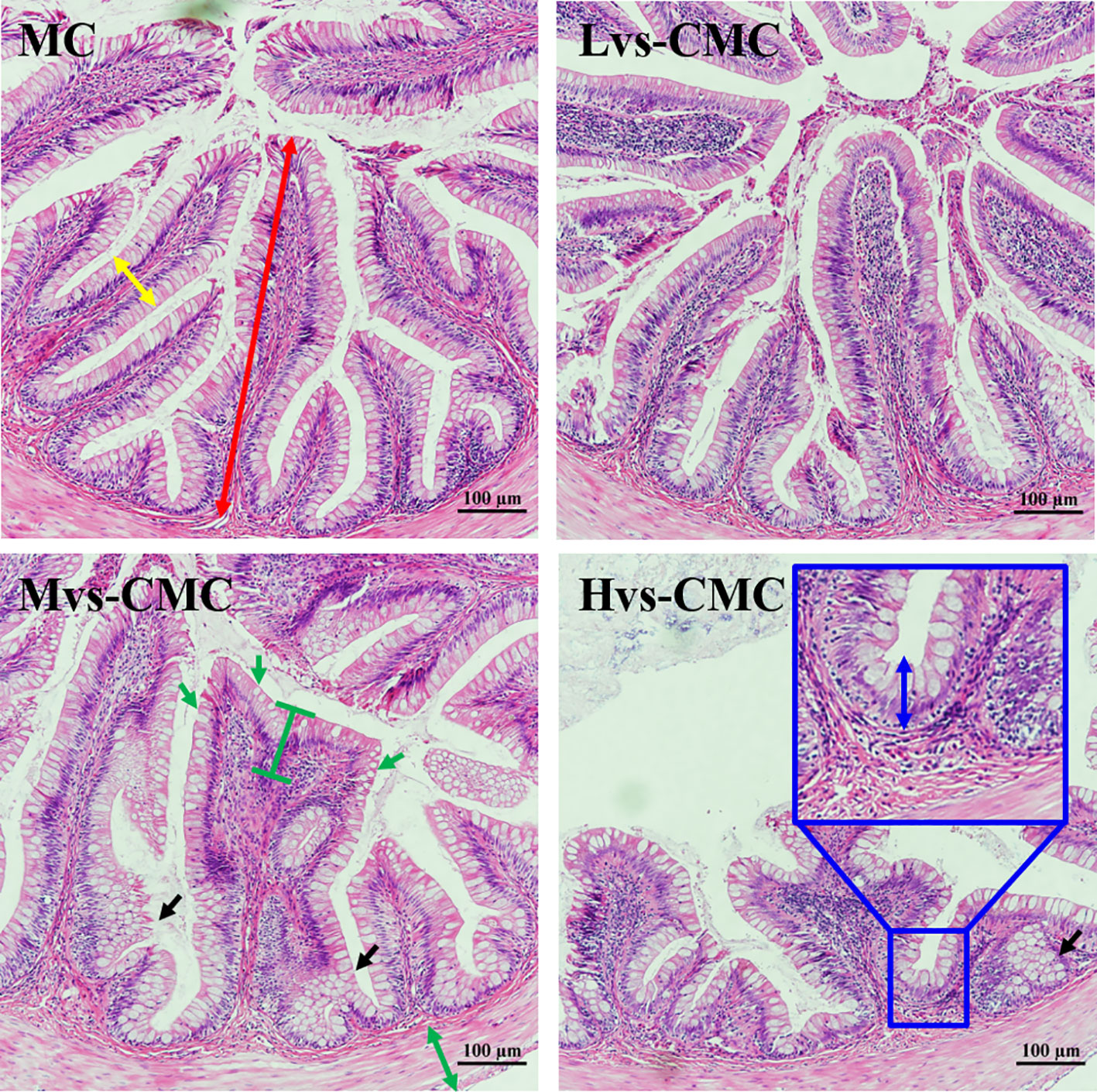
Figure 1 Hindgut hematoxylin–eosin (HE) staining of largemouth bass fed with the test diets (magnification, ×200). Yellow double-sided arrow, villus width; black arrow, crypt cell proliferation; red double-sided arrow, villus height; green double-sided arrow, muscular thickness; green arrow, goblet cell; blue double-sided arrow, crypt depth.
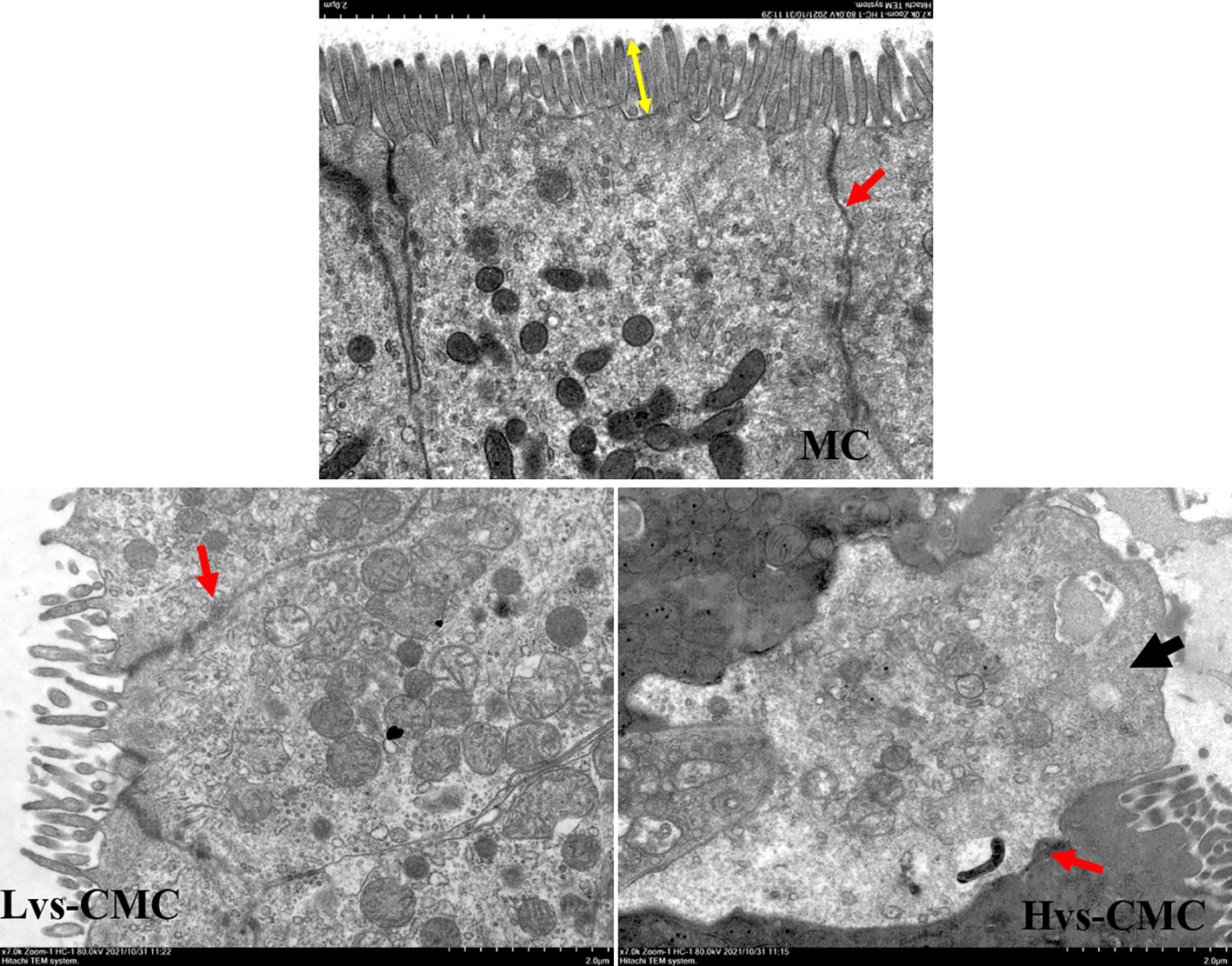
Figure 2 Hindgut transmission electron microscopy observation of juvenile largemouth bass fed with the test diets (magnification, ×7,000). Black arrow, epithelial cell death; red arrow, epithelial cell space; yellow double-sided arrow, microvillus height.
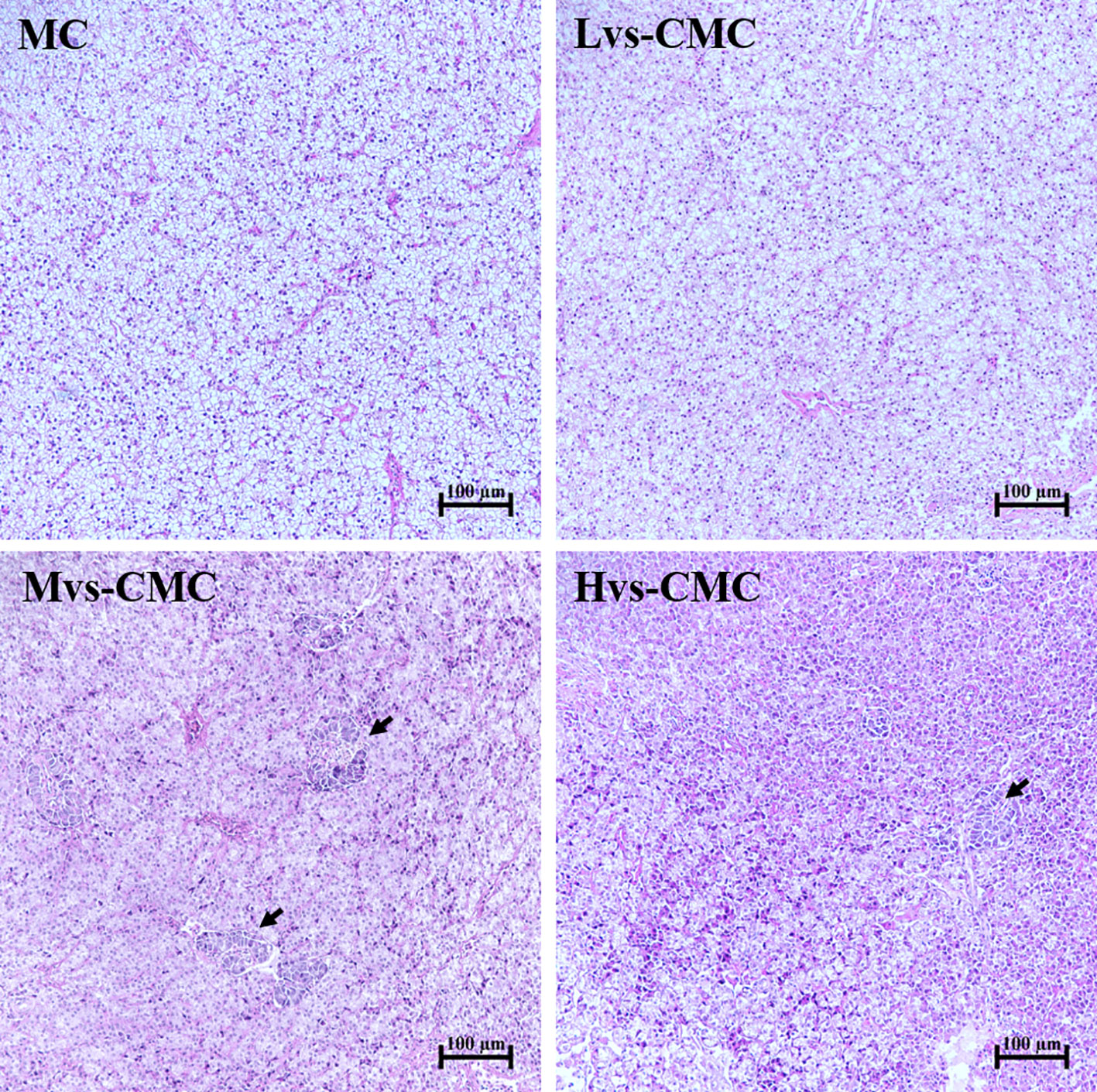
Figure 3 Hepatic hematoxylin–eosin (HE) staining of largemouth bass fed with the test diets (magnification, ×200). Black arrow, fibrosis of liver cells.
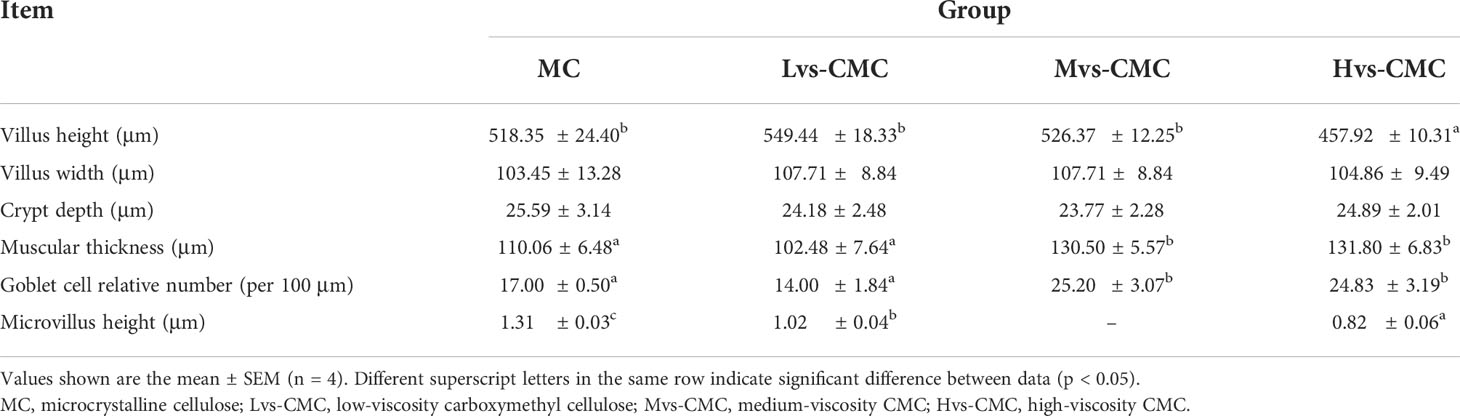
Table 7 Effects of increasing dietary viscosity on the hindgut morphology of juvenile largemouth bass.
Discussion
An increasing amount of reports confirmed that the physiological impacts of dietary NSPs on aquatic animals are associated with the type of dietary NSPs (either insoluble or soluble) (Sinha et al., 2011; Ren et al., 2020; Deng et al., 2021; Jiang et al., 2022). Several studies have shown that the anti-nutritional impacts of dietary NSPs are mainly caused by the SNSP component (Cai et al., 2019; Ren et al., 2020; Deng et al., 2021; Liu et al., 2022b). However, there is limited information on the correlation between the physicochemical characteristics of NSPs and their anti-nutritional effects. Our data demonstrated that dietary CMC exerts a greater anti-nutritional influence compared to dietary MC, suggesting that solubility and viscosity are the major anti-nutritional features of dietary NSPs. Similarly, dietary SNSPs negatively affected the growth of yellow catfish and rainbow trout compared to dietary INSPs (Cai et al., 2019; Deng et al., 2021), and dietary supplementation exceeding SNSP (guar gum) negatively affected the growth performance of mullet (Mugil liza) and striped catfish (Pangasianodon hypophthalmus) (Ramos et al., 2015; Tran-Tu et al., 2018).
Dietary SNSPs increase the viscosity of the digesta and slow down the passage of gastrointestinal emptying (Tran-Tu et al., 2019), which may, in turn, reduce the intake of fish feed. Additionally, dietary SNSPs can induce the production of glucagon-like peptides and peptide YY through bacterial fermentation, thereby enhancing satiety in fish (Lattimer and Haub, 2010). Therefore, the increase of dietary SNSP levels is usually accompanied by a decrease in the FI of fish (Sinha et al., 2011). In previous studies, an increase in dietary viscosity has been shown to decrease the FI of M. liza (Ramos et al., 2015), but increased the FI of rainbow trout (Deng et al., 2021). In this study, dietary CMC supplementation significantly increased the FI of largemouth bass. The differences in these results suggest that the effect of dietary viscosity on the feeding rate of fish may be related to fish species.
Intestinal digestive enzymes play a crucial role in the absorption process of feed nutrients in fish, and their activity determines the nutrient absorption efficiency and growth rate of fish (Willora et al., 2022). On the other hand, digestive enzyme activity is inevitably influenced by the quantities and characteristics of feed ingredients (Zhang et al., 2021). Our data showed that dietary CMC extremely reduced the activities of the intestinal digestive enzymes compared to dietary MC, suggesting that soluble SNSPs are detrimental to dietary nutrient uptake. Moreover, the activities of intestinal protease and Na+/K+-ATPase exhibited a decreasing trend with increasing CMC viscosity, indicating that high-viscosity diets are more detrimental to nutrient digestion and absorption. A previous study indicated that dietary SNSPs bind to the enzymes in the gut, decrease the intestinal enzyme activities (Sinha et al., 2011), and may form some sticky granules that adhere to the intestinal villus, thereby interfering with the digestion and absorption processes (Nie et al., 2007). This evidence suggests that CMC diets may reduce the digestive enzyme activity through adhesion. Furthermore, AKP is also considered to be an important immune enzyme in fish, and a decrease in its activity represents a decreased immune status in fish (Yin et al., 2018; Yu et al., 2021). Combined with the poor gut morphology (epithelial cell death and increased cell intervals) (Figure 2) observed in the CMC groups, our results suggest that high dietary viscosity disrupts gut health.
Dietary NSPs have a large number of carboxyl and hydroxyl units that can interact with mineral elements (Ma et al., 2017), thereby accelerating the efflux of mineral components and reducing their absorption efficiency, especially for Na and K (Leenhouwers et al., 2006; Kraugerud et al., 2007; Leenhouwers et al., 2007). It is worth noting that the activity of Na+/K+-ATPase is affected by osmotic pressure (He et al., 2021) and is closely associated with the concentration of substrate ion (Gal-Garber et al., 2003). This evidence possibly explains the dramatic decrease in intestinal Na+/K+-ATPase activity in this study since a high-viscosity diet accelerates the excretion of Na, K, and other minerals.
Dietary proteins and lipids need to be broken down by protease and lipase before they can be absorbed and utilized by fish. Therefore, it can be hypothesized that the reduced apparent protein and lipid digestibility in the CMC groups is closely associated with the decreased activities of protease and lipase. In addition, endogenous nitrogen loss may also contribute to the decrease in apparent protein digestibility (Rgensen et al., 2003). Furthermore, the poor apparent lipid digestibility ultimately reduced the serum TG concentration and whole-body crude protein content in the CMC groups. Similarly, increasing dietary viscosity significantly decreased the dietary dry matter and crude protein digestibility in catfish (Clarias gariepinus) and striped catfish (Leenhouwers et al., 2006; Tran-Tu et al., 2018; Tran-Tu et al., 2019).
ALT and AST are amino acid metabolizing enzymes that are mainly located in hepatocytes and enter the blood when liver damage occurs (Chaklader et al., 2021). Hence, the activities of serum ALT and AST can reveal the hepatic function status (Hanim et al., 2015). In this study, dietary CMC increased the activities of serum ALT and AST, with both ALT and AST activities in the Hvs-CMC group being significantly lower than those in the MC group; in contrast, a worse hepatic morphology was observed in the CMC groups (Figure 3). Our results suggest that dietary CMC disrupts hepatic health, with a highly viscous CMC exhibiting a stronger destructive impact than the low-viscosity CMC. Similarly, dietary SNSPs lead to hepatic damage in yellow catfish (Cai et al., 2019).
Fish gut morphology is inevitably affected by dietary components; hence, gut morphology is a widely used measure to evaluate the potential physiological impacts of dietary components on fish (Hartviksen et al., 2014; Huang et al., 2022). Furthermore, gut morphology is closely associated with its physiological functions (e.g., digestion and absorption) (Fang et al., 2019). For example, variations in the height of the intestinal villus and the number of folds and goblet cells may affect intestinal digestion and absorption (Sang and Fotedar, 2010). Generally, factors that can increase the digestive area promote intestinal digestion and absorption function. In this study, fish fed with Hvs-CMC diets had the shortest intestinal villus height, suggesting that a high-viscosity diet is unfavorable for gut digestive function. Muscular thickness can efficiently reveal the intestinal peristaltic capacity since it is closely related to intestinal motility (Huang et al., 2022). As aforementioned, dietary SNSPs increased the digesta viscosity and prolonged the digesta transit time in the intestine (Sinha et al., 2011). Therefore, it can be hypothesized that the increase in muscular thickness was intended to enhance intestinal motility, as an adaptive change to highly viscous diets. The mucin secreted by goblet cells is a crucial part of the intestinal mucosal immune barrier, which participates in maintaining the intestinal health of fish (Zheng et al., 2015; Martín et al., 2019; Tan and Sun, 2020). Thus, an increase in the number of goblet cells is beneficial for promoting intestinal health. Moreover, Sinha et al. (2011) suggested that increasing the digesta viscosity decreased intestinal oxygen tension, thereby promoting the proliferation of anaerobic microbiota. Moreover, anaerobic microbiota is generally detrimental to host health and even induces infections by producing toxic metabolites such as endotoxins, histamine, and trimethylamine N-oxide (Santos et al., 2014; Subramaniam and Fletcher, 2018; Cobo, 2021). This evidence suggests that the increased number of intestinal goblet cells in largemouth bass may be a response to the adverse effects of the high-viscosity diet, thereby maintaining intestinal health. Overall, combined with the decrease in digestive enzyme activity, feed utilization, and growth, as well as the unfavorable dietary nutrient digestibility and worse intestinal morphology aforementioned, our results demonstrated that the anti-nutritional effect of dietary SNSPs is mainly associated with their viscosity.
Conclusion
In conclusion, dietary CMC increases the dietary viscosity, decreases the digestive enzyme activities, and disrupts the intestinal morphology, thereby inhibiting dietary nutrient digestibility and reducing the growth of largemouth bass juveniles. Moreover, our data showed that solubility and viscosity are the dominant anti-nutritional features of NSPs and that the anti-nutritional effect of dietary SNSPs comes mainly from their viscosity.
Data availability statement
The raw data supporting the conclusions of this article will be made available by the authors, without undue reservation.
Ethics statement
The animal study was reviewed and approved by the Animal Research and Ethics Committee of Guangdong Ocean University.
Author contributions
YL: Conceptualization, formal analysis, data curation, and writing—original draft, review, and editing. JF, HZ, YZ, and HH: Methodology, project administration, and data curation. YC: Conceptualization, formal analysis, and data curation. WZ: Project administration and supervision. JD: Investigation, methodology, and resources. BT: Investigation, methodology, and resources. All authors contributed to the article and approved the submitted version.
Funding
This work was supported by the Program for Scientific Research Startup Funds of Guangdong Ocean University (grant no: 060302022007); National Key R&D Program of China (grant no: 2019YFD0900200); National Natural Science Foundation of China (grant no: 31760761); Foundation of Tongwei Co., Ltd. (grant no: TA2019A003); and Excellent Dissertation Training Program (grant no: 202246).
Acknowledgments
The authors would like to thank Professor Zhang Shuang and Dong Xiaohui, Dr. Yin Bin, Long Shuisheng, Huangyang, Xu jia, Xiao Yousang, Lu Bingneng, Sun Mengya, Liu Yongyin, Wei Zhangbo, and She Xiaoqi for their assistance in this study.
Conflict of interest
This study received funding from the Foundation of Tongwei Co., Ltd. The funder was not involved in the study design, sample collection and analysis, data analysis, paper writing, and paper publication decision.
The authors declare that the research was conducted in the absence of any commercial or financial relationships that could be construed as a potential conflict of interest.
Publisher’s note
All claims expressed in this article are solely those of the authors and do not necessarily represent those of their affiliated organizations, or those of the publisher, the editors and the reviewers. Any product that may be evaluated in this article, or claim that may be made by its manufacturer, is not guaranteed or endorsed by the publisher.
References
AOAC (2005). Official methods of analysis of AOAC international. 18th Ed (Gaithersburg, MD, USA: Association of Official Analytical Chemists).
Cai C., Ren S., Cui G., Ni Q., Cao X. (2019). Short-term stress due to dietary pectin induces cholestasis, and chronic stress induces hepatic steatosis and fibrosis in yellow catfish, Pelteobagrus fulvidraco. Aquaculture 516, 734607. doi: 10.1016/j.aquaculture.2019.734607
Chaklader M., Howieson J., Fotedar ,. R. (2021). Growth, hepatic health, mucosal barrier status and immunity of juvenile barramundi, lates calcarifer fed poultry by-product meal supplemented with full-fat or defatted hermetia illucens larval meal. Aquaculture 543, 737026. doi: 10.1016/j.aquaculture.2021.737026
Choct M. (2015). Feed non-starch polysaccharides for monogastric animals: classification and function. Anim. Produ. Sci. 55, 1360–1366. doi: 10.1071/AN15276
Cobo F. (2021). Infections caused by anaerobic microorganisms. Encycl. Infect. Immu. 1, 614–627. doi: 10.1016/B978-0-12-818731-9.00065-3
Deng J., Zhang X., Sun Y., Mi H., Zhang L. (2021). Effects of different types of non-starch polysaccharides on growth, digestive enzyme activity, intestinal barrier function and antioxidant activity of rainbow trout (Oncorhynchus mykiss). Aquac. Rep. 21, 100864. doi: 10.1016/j.aqrep.2021.100864
Fang H., Xie J., Liao S., Tian Y., Guo S., Yong J. (2019). Effects of dietary inclusion of shrimp paste on growth performance, digestive enzymes activities, antioxidant and immunological status and intestinal morphology of hybrid snakehead (Channa maculata ♀ × Channa argus ♂). Front. Physiol. 10. doi: 10.3389/fphys.2019.01027
Gal-Garber O., Mabjeesh S., Sklan D., Uni Z. (2003). Nutrient transport in the small intestine: Na+, k+-ATPase expression and activity in the small intestine of the chicken as influenced by dietary sodium. Poultry Sci. 82, 1127–1133. doi: 10.1093/ps/82.7.1127
Gao Y., Li X., Dong Y., Zhu X., Li W., Wu X. (2018). Replacement of fishmeal by mixed animal and plant protein sources in diets for juvenile mangrove red snapper (Lutjanus argentimaculatus) (Forsskal 1775). Israeli J. Aquac.- Bamidgeh 1462, 8. doi: 10.46989/001c.20950
Glencross B., Rutherford N., Bourne N. (2012). The influence of various starch and non-starch polysaccharides on the digestibility of diets fed to rainbow trout (Oncorhynchus mykiss). Aquacultures 356–357, 141–146. doi: 10.1016/j.aquaculture.2012.05.023
Hanim S. F., Azrina A., Khoo H. E., Amin I. (2015). Protective effects of pulp and kernel oils from Canarium odontophyllum fruit in normal and hypercholesterolemic rabbits. Int. Food Res. J. 22, 1318–1326.
Hartviksen M., Vecino J. L. G., Ringø E., Bakke A. M., Wadsworth S., Krogdahl Å., et al. (2014). Alternative dietary protein sources for Atlantic salmon (Salmo salar l.) effect on intestinal microbiota, intestinal and liver histology and growth. Aquac. Nutr. 20, 318–398. doi: 10.1111/anu.12087
He Y., Guo X., Tan B., Dong X., Yang Q., Liu H., et al. (2021). Replacing fish meal with fermented rice protein in diets for hybrid groupers (Epinephelus fuscoguttatus♀× Epinephelus lanceolatus♂): Effects on growth, digestive and absorption capacities, inflammatory-related gene expression, and intestinal microbiota. Aquac. Rep 19, 100603. doi: 10.1016/j.aqrep.2021.100603
Huang B., Zhang S., Dong X., Chi S., Yang Q., Liu H., et al. (2022). Effects of fishmeal replacement by black soldier fly on growth performance, digestive enzyme activity, intestine morphology, intestinal flora and immune response of pearl gentian grouper (Epinephelus fuscoguttatus ♀ × Epinephelus lanceolatus ♂). Fish Shellfish Immunol. 120, 497–506. doi: 10.1016/j.fsi.2021.12.027
Jiang W., Zhang Y., Yuan M., Liu Y., Deng J., Tan B. (2022). Effects of different types of non-starch polysaccharides on growth, digestive enzyme activity, intestinal barrier function and antioxidant activity of tilapia (Oreochromis niloticus). Aquac. Rep. 25, 101198. doi: 10.1016/j.aqrep.2022.101198
Kraugerud O. F., Penn M., Storebakken T., Refstie S., Krogdahl Å., Svihus B. (2007). Nutrient digestibilities and gut function in Atlantic salmon (Salmo salar) fed diets with cellulose or non-starch polysaccharides from soy. Aquaculture 273, 96–107. doi: 10.1016/j.aquaculture.2007.09.013
Lattimer J. M., Haub M. D. (2010). Effects of dietary fiber and its components on metabolic health. Nut 2, 1266–1289. doi: 10.3390/nu2121266
Leenhouwers J. I., Adjei-Boateng D., Verreth J. A. J., Schrama J. W. (2006). Digesta viscosity, nutrient digestibility and organ weights in African catfish (Clarias gariepinus) fed diets supplemented with different levels of a soluble non-starch polysaccharide. Aquac. Nutr. 12, 111–116. doi: 10.1111/j.1365-2095.2006.00389.x
Leenhouwers J. I., Ortega R. C., Verreth J. A. J., Schrama J. W. (2007). Digesta characteristics in relation to nutrient digestibility and mineral absorption in Nile tilapia (Oreochromis niloticus l.) fed cereal grains of increasing viscosity. Aquaculture 273, 556–565. doi: 10.1016/j.aquaculture.2007.10.044
Leigh S., Nguyen-Phuc B., German and D. P. (2018). The effects of protein and fiber content on gut structure and function in zebrafish (Danio rerio). J. Comp. Physiol. B 188, 237–253. doi: 10.1007/s00360-017-1122-5
Lin S., Zhou X., Zhou Y., Kuang W., Chen Y., Luo L., et al. (2020). Intestinal morphology, immunity and microbiota response to dietary fibers in largemouth bass Micropterus salmoide. Fish Shellfish Immunol. 103, 135–142. doi: 10.1016/j.fsi.2020.04.070
Liu Y., Deng J., Tan B., Xie S., Zhang W. (2022a). Effects of soluble and insoluble non-starch polysaccharides on growth performance, digestive enzyme activity, antioxidant capacity, and intestinal flora of juvenile genetic of improvement of farmed tilapia (Oreochromis niloticus). Front. Mar. Sci. 9. doi: 10.3389/fmars.2022.872577
Liu Y., Huang H., Fan J., Zhou H., Zhang Y., Cao Y., et al. (2022b). Effects of different viscous guar gums on growth, apparent nutrient digestibility, intestinal development and morphology in juvenile largemouth bass, Micropterus salmoides. Front. Physiol. 13. doi: 10.3389/fphys.2022.927819
Ma J., Adler L., Srzednicki G., Arcot J. (2017). Quantitative determination of non-starch polysaccharides in foods using gas chromatography with flame ionization detection. Food Chem. 220, 100–107. doi: 10.1016/j.foodchem.2016.09.206
Martín R., Chamignon C., Mhedbi-Hajri N., Chain F., Derrien M., Escribano-Vázquez U., et al. (2019). The potential probiotic lactobacillus rhamnosus CNCM I-3690 strain protects the intestinal barrier by stimulating both mucus production and cytoprotective response. Sci. Rep. 9, 5398. doi: 10.1038/s41598-019-41738-5
Ma Y., Xu C., Li M., Chen H., Ye R., Zhang G., et al. (2020). Diet with a high proportion replacement of fishmeal by terrestrial compound protein displayed better farming income and environmental benefits in the carnivorous marine teleost (Trachinotus ovatus). Aquac. Rep. 18, 100449. doi: 10.1016/j.aqrep.2020.100449
Nie G., Wang J., Zhu M., Zhou H. (2007). The influences of xylanase added in wheat basal diet on intestine chyme viscosity and the development of villi and microvilli of tilapia. nilotica. J. Fish. China 31, 54–61. doi: 10.1360/yc-007-1071
Ramos L. R. V., Romano L. A., Monserrat J. M., Abreu P. C., Verde P. E., Tesser M. B. (2015). Biological responses in mullet mugil liza juveniles fed with guar gum supplemented diets. Anim. Feed Sci. Technol. 205, 98–106. doi: 10.1016/j.anifeedsci.2015.04.004
Ren S., Cai C., Cui G., Ni Q., Ye Y. (2020). High dosages of pectin and cellulose cause different degrees of damage to the livers and intestines of Pelteobagrus fulvidraco. Aquaculture 514, 734445. doi: 10.1016/j.aquaculture.2019.734445
Rgensen J., Zhao X. Q., Theil P. K., Gabert V. M., Knudsen K. (2003). Energy metabolism and protein balance in growing rats fed different levels of dietary fibre and protein. Arch. Für Tierernhrung 57, 83–98. doi: 10.1080/0003942031000107280
Sang H. M., Fotedar R. (2010). Effects of mannan oligosaccharide dietary supplementation on performances of the tropical spiny lobsters juvenile (Panulirus ornatus, fabricius 1798). Fish Shellfish Immunol. 28, 483–489. doi: 10.1016/j.fsi.2009.12.011
Santos J. A., Rodríguez-Calleja J. M., Otero A., García-López M. L. (2014). Plesiomonas in encyclopedia of food microbiology (Second edition. Eds. Batt C. A., Tortorello M. L. (Oxford: Academic Press), 47–52.
Sinha A. K., Kumar V., Makkar H., Boeck G. D., Becker K. (2011). Non-starch polysaccharides and their role in fish nutrition – a review. Food Chem. 127, 1409–1426. doi: 10.1016/j.foodchem.2011.02.042
Steinberg C. (2022). Nonstarch polysaccharides— ‘Neither sweet nor gluey–adverse? Aquat. Anim. Nutr., 509–529. doi: 10.1007/978-3-030-87227-4_22
Subramaniam S., Fletcher C. (2018). Trimethylamine n-oxide: breathe new life. Br. J. Pharmacol. 175, 1344–1353. doi: 10.1111/bph.13959
Tan X., Sun Z. (2020). Dietary dandelion extract improved growth performance, immunity, intestinal morphology and microbiota composition of golden pompano. Trachinotus ovatus. Aquac. Rep. 18, 100491. doi: 10.1016/j.aqrep.2020.100491
Tran-Tu L. C., Bosma R. H., Verstegen M. W. A., Schrama J. W. (2019). Effect of dietary viscosity on digesta characteristics and progression of digestion in different segments of the gastrointestinal tract of striped catfish (Pangasionodon hypophthalmus). Aquaculture 504, 114–120. doi: 10.1016/j.aquaculture.2019.01.047
Tran-Tu L. C., Hien T. T. T., Bosma R. H., Heinsbroek L. T. N., Verreth J. A. J., Schrama J. W. (2018). Effect of ingredient particle sizes and dietary viscosity on digestion and faecal waste of striped catfish (Pangasianodon hypophthalmus). Aquac. Nutr. 24, 961–969. doi: 10.1111/anu.12632
Willora F. P., Vatsos I. N., Mallioris P., Bordignon F., Keizer S., Martınez-Llorens S., et al. (2022). Replacement of fishmeal with plant protein in the diets of juvenile lumpfish (Cyclopterus lumpus, l. 1758): Effects on digestive enzymes and microscopic structure of the digestive tract. Aquaculture 561, 738601. doi: 10.1016/j.aquaculture.2022.738601
Yang P., Yao W., Wang Y., Li M., Li X., Leng X. (2022). Dietary effects of fish meal substitution with clostridium autoethanogenum on flesh quality and metabolomics of largemouth bass (Micropterus salmoides). Aquac. Rep. 23, 101012. doi: 10.1016/j.aqrep.2022.101012
Yin Y., Zhang P., Yue X., Du X., Li W., Yin Y., et al. (2018). Effect of sub-chronic exposure to lead (Pb) and bacillus subtilis on carassius auratus gibelio: Bioaccumulation, antioxidant responses and immune responses. Ecotoxicol. Environ. Saf. 161, 755–762. doi: 10.1016/j.ecoenv.2018.06.056
Yu Z., Zhao Y., Jiang N., Zhang A., Li M. (2021). Bioflocs attenuates lipopolysaccharide-induced inflammation, immunosuppression and oxidative stress in channa argus. Fish Shellfish Immunol. 114, 218–228. doi: 10.1016/j.fsi.2021.05.006
Zhang M., Pan L., Fan D., He J., Su C., Gao S., et al. (2021). Study of fermented feed by mixed strains and their effects on the survival, growth, digestive enzyme activity and intestinal flora of Penaeus vannamei. Aquaculture 530, 735703. doi: 10.1016/j.aquaculture.2020.735703
Keywords: carboxymethyl cellulose, viscous, growth performance, gut morphology, largemouth bass
Citation: Liu Y, Fan J, Zhou H, Zhang Y, Huang H, Cao Y, Zhang W, Deng J and Tan B (2022) Feeding juvenile largemouth bass (Micropterus salmoides) with carboxymethyl cellulose with different viscous: Impacts on nutrient digestibility, growth, and hepatic and gut morphology. Front. Mar. Sci. 9:1023872. doi: 10.3389/fmars.2022.1023872
Received: 20 August 2022; Accepted: 18 October 2022;
Published: 10 November 2022.
Edited by:
Zhendong Qin, Zhongkai University of Agriculture and Engineering, ChinaReviewed by:
Mohamed Hassaan, Benha University, EgyptZhenhua Ma, South China Sea Fisheries Research Institute (CAFS), China
Copyright © 2022 Liu, Fan, Zhou, Zhang, Huang, Cao, Zhang, Deng and Tan. This is an open-access article distributed under the terms of the Creative Commons Attribution License (CC BY). The use, distribution or reproduction in other forums is permitted, provided the original author(s) and the copyright owner(s) are credited and that the original publication in this journal is cited, in accordance with accepted academic practice. No use, distribution or reproduction is permitted which does not comply with these terms.
*Correspondence: Junming Deng, djunming@163.com; Beiping Tan, bptan@126.com