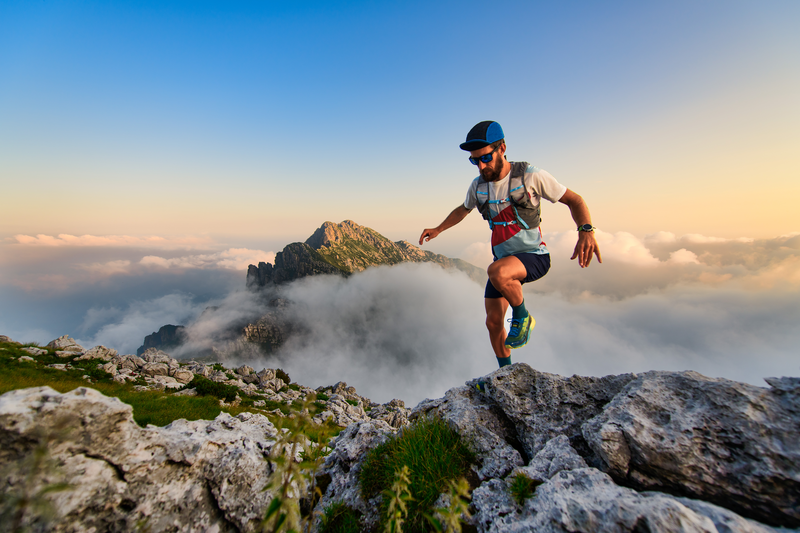
95% of researchers rate our articles as excellent or good
Learn more about the work of our research integrity team to safeguard the quality of each article we publish.
Find out more
ORIGINAL RESEARCH article
Front. Mar. Sci. , 17 October 2022
Sec. Marine Pollution
Volume 9 - 2022 | https://doi.org/10.3389/fmars.2022.1021170
This article is part of the Research Topic Plastic Pollution in a Changing Marine Environment: Effects and Risk View all 7 articles
Eighty-four wild seahorses, including three of China’s dominant species, were collected from seven coastal provinces to evaluate their common contaminant contents. Heavy metals (including Cu, Pb, Cd, Cr, and Hg), Benzo(a)pyrene (B[a]P), and microplastics were detected in the collected seahorses. The results indicate that considerable contamination variations are present in seahorses from different regions and species. Elevated concentrations of Cu and Cr, compared to other heavy metals, were observed in most sampled locations. In addition, B[a]P concentrations were far greater in Hippocampus mohnikei and H.trimaculatus compared with H.kelloggi (P < 0.05). Substantial changes in microplastic concentrations were also observed in seahorses from different locations, and significant differences were observed between sampled species (P < 0.05). Further, a correlation analysis obtained strong correlations among four heavy metals (Cu, Pb, Cd, and Cr), except Hg. A significant correlation was identified between B[a]P and microplastics (P < 0.01), suggesting potential synergistic effects between these two contaminants. In brief, this study indicates that wild seahorse populations are experiencing environmental pollution.
With rapid marine economic development, the pollution of coastal water environments has become severe. Common environmental pollutants include heavy metals, persistent organic compounds such as polycyclic aromatic hydrocarbons (PAHs) and polychlorinated biphenyls (PCBs), and microplastics, which have recently attracted significant attention (Storelli, 2008). Heavy metals are highly toxic substances that can continuously accumulate in the bodies of living organisms, thereby causing both acute and chronic toxic effects. Many studies have reported that different degrees of heavy metal pollution have been detected within seafood in the coastal areas of China (Liu et al., 2018; Milenkovic et al., 2019; Hossain, 2020; Liu et al., 2020a; Ngoc et al., 2020; Pandion et al., 2022). Benzo[a]pyrene (B[a]P) is a common persistent organic pollutant (POP) that is widely found in aquaculture environments and has stable properties, strong toxicity, and carcinogenic effects (Quintanilla-Mena et al., 2021; Ju et al., 2022). Other studies have revealed that shellfish biomagnifies B[a]P; and high B[a]P content has been detected in various shellfish samples (Qu et al., 2019). Microplastics are a new type of environmental pollutant, and they are widely prevalent in the marine environment. Their potential to cause harm to organisms has been identified, including physical damage and the release of certain biological and chemical toxicants that result in further toxic effects, which explains why they have been extensively studied. Moreover, microplastics have a strong adsorption capacity for heavy metals, B[a]P, and other environmental pollutants, and as the particle size of microplastics is similar that of many types of fish food, it is easy to be mistakenly consumed by the aquatic animals (Chen et al., 2022). Honryo et al. (2021) reported that tuna accidentally ingested polystyrene flakes, which would accumulate in the body and affect the swimming speed and feeding of tuna. Consequently, microplastics may pose a greater safety risk than metals and organic pollutants (Sun et al., 2019). Once these environmental pollutants enter the food chain and accumulate in aquatic organisms, they will cause adverse effects on organisms and damage human health (Gao et al., 2022). Therefore, there is an urgent need for contaminant analysis of Chinese coastal marine organisms.
The seahorse is an important environmental indicator organism (Alves et al., 2013; Delunardo et al., 2015; Chipari-Gomes et al., 2017), and has an important place in ornamental aquarium (Vincent et al., 2011; Foster et al., 2016; Koning and Hoeksema, 2021). Seahorses primarily inhabit shallow coastal areas and are often found in seagrass beds, benthic algae, coral reefs, and mangroves (Barboza et al., 2020). Foster and Vincent (2004) reported that the unique life history of seahorses, such as male-pregnancy reproduction and limited moving range, increase their vulnerability to environmental change, including habitat destruction, overfishing, and marine pollution. In particular, the shallow coastal areas inhabited by seahorses are usually more susceptible to different marine contaminants (Pojana et al., 2007). Until now, little research has been reported on the presence of environmental contaminants in wild seahorses along the Chinese coast. The aim of this study was to investigate the presence and accumulation of heavy metals, B[a]P and microplastics in wild seahorse populations at different locations along the Chinese coast, and the overall results will help to improve our understanding of the accumulation of contaminants in wild seahorses along the Chinese coast.
Seahorses were collected from nine locations along the Chinese coastline, and different species were collected at each location due to the different geographical distribution of different species of seahorses (Figure 1A). Hippocampus mohnikei came from Qinhuangdao (QHD), Dongying (DY), and Weihai (WH); H. kelloggi from Xiangshan (XS), Nanao (NA), and Sanya (SY); and H. trimaculatus from Dongshan (DS), Beihai (BH), and Lingshui (LS), were approximately 7.61 ± 1.97 cm, 15.39 ± 2.51 cm, and 9.89 ± 1.11 cm, respectively (Figure 1B). Seahorses were caught by trawling using fishing boats, and 10 seahorses were collected from each location. They were anesthetized using eugenol (100 mg/L), packed in cryogenic vials (10 ml), and transported back to the laboratory on dry ice. Collection was all samples were washed 3 times with Mill-Q water (Millipore 18.2 M). The intestine and liver were removed by dissection using a dissection tool and the intestine was stored in glass vials at -20°C for microplastic analysis. The liver was stored in cryogenic vials (2 ml) at -20°C for determination of heavy metals and B[a]P content.
Figure 1 (A) Sampling locations along the Chinese coast. (B) Seahorse body lengths of the different populations. The numbers in the circles represent the number of seahorses collected at locations.
For the analysis, homogenization of each seahorse tissue (other than intestines) was performed using a grinder. Next, the homogenate was digested with 0.6 ml 65% HNO3 and 0.25 ml 70% HClO4 in a Teflon vessel for 15 min at 190°C (Song et al., 2015). After allowing at least 1 h for cooling, the digested sample was transferred to a graduated plastic test tube, and the volume was made up to 100 mL with Milli-Q water for instrumental analysis (Yi et al., 2011). Cu, Pb, Cr, Cd, and Hg in all samples were tested using a NexION 300 ICP-MS (Perkin Elmer, Inc., Shelton, CT, USA). Each test sample was determined three times. Quality control on the reliability of the preparation methods was conducted through blank experiments and blank spike experiments. Heavy metal concentrations were expressed as the weight of heavy metals per seahorse wet weight (mg/kg).
The analysis of B[a]P was carried out according to the procedure described by Serpe et al. (2010). The homogenate samples were weighed and mixed with 10 mL n-hexane using ultrasonic treatment at 40°C for 10 min. Then, the mixture was centrifuged at 7000 rpm for 5 min, and the supernatant was collected. An additional 10 mL n-hexane was added, and the same process was repeated. Both supernatants were combined and reduced to a small volume by rotary evaporation at 40°C. Then, 1 mL of acetonitrile was added and filtered through a microporous membrane. The filtered liquids were used for determining B[a]P concentrations using the HPLC method. B[a]P concentrations were expressed as the weight of B[a]P per seahorse wet weight (μg/kg).
The HNO3 solution digestion method was used to digest the seahorse digestive tracts (Desforges et al., 2015). Briefly, 65% HNO3 was added to each vial, after which the vials were covered and heated in a water bath at approximately 80°C for 1-3 h until the digestive tract was completely digested. The digested samples were then passed through 1-μm mixed cellulose ester filter papers, Immerse the filter paper in an ethanol solution for ultrasonic treatment to disperse any substance on it into the ethanol solution. Remove the filter paper in ethanol solution and rinse with ethanol several times. The solution is then placed in an oven and its concentration is increased by evaporation. The concentrated ethanol solution was added dropwise to the highly reflective glass for MPs analysis after complete evaporation of ethanol (Tian et al., 2022). MPs (particle size range: 0.5-5mm) were observed and measured using a stereomicroscope (Stemi SV11, ZEISS, Shanghai, China), and their abundance, color, and size were recorded. The concentrated ethanol solution of seahorse intestine was dropped on a highly reflective glass and subjected to laser infrared imaging LDIR (Agilent 8700 LDIR, Shanghai, WEIPU Testing Technology Group Co., LTD.) after complete evaporation of ethanol. In LDIR analysis, particle analysis mode and MPs spectral library construction were selected for automatic detection. LDIR imaging can be used to directly calculate the number of test points (particle size range: 20-500μm) and determine the polymer type, abundance and size distribution. According to the definition of MPs (particles with a diameter of less than 5 mm) and the results of the survey, the particle sizes of MPs in the hippocampus were classified into four grades: 20-200μm, 200-500μm, 500-1000μm and 1000-2000μm. Finally, three blanks with only HNO3 were run for each batch of samples to correct for potential air-borne MP deposition in the laboratory. During the microplastic composition analysis, all glass containers, instruments, and dissecting tools were washed three times with distilled water to reduce the contamination of exogenous microplastics. Microplastic concentrations were expressed as the number of microplastic pieces per seahorse (particles per individual).
The results presented herein are expressed as the mean ± standard deviation (mean ± SDM). One-way analysis of variance (ANOVA) and t-tests were used in the analyses. A significant difference between the two groups was defined when p < 0.05, while an extremely significant difference was defined when p < 0.01. Pearson correlation coefficient analysis among microplastic particles, heavy metals, and B[α]P content in wild seahorses was performed using the SPSS software (SPSS Statistics 25).
All experiments in this study were approved by the Ethics Committee of Tianjin Agricultural University and were performed in accordance with the regulations and guidelines established by the committee.
The heavy metal contents in the wild seahorse samples from different sites along the Chinese coastline are shown in Figure 2. Heavy metal contents in seahorses from different regions varied significantly. Cu, Pb, Cd, and Cr concentrations also varied significantly within the same enclosed sea area (e.g., the Bohai Sea, including WH, DY, and QHD). The highest cumulative heavy metal contents were recorded in WH. The Cd content in the seahorses was particularly high at the Beihai and Weihai sites (5.684 and 8.786 mg/kg, respectively), while low Cd contents were detected at nearby Sanya (0.096 mg/kg) and Nanao (0.134 mg/kg) sites in the northern South China Sea. The Hg contents of the seahorses were low at all sites (< 0.2 mg/kg). Further, significant differences in heavy metal contents were observed among the species, with the highest heavy metal bioaccumulation recorded in H. mohnikei (Figure 3).
Figure 2 Sampling locations along the China coast (Dongying, Qinghuangdao, Weihai, Lingshui, Beihai, Dongshan, Sanya, Nanao, Xiangshan). Different colors represent different heavy metals. Letters indicate significant difference(P < 0.05).
Figure 3 Heavy metal concentrations (Cu, Pb, Cd, Cr, and Hg) (mg/kg) in seahorse samples (H.mohnikei、H. kelloggi、H. trimaculatus). The letters (a–c) in the Figure represent significant variations between species (P < 0.05).
The B[a]P contents of the wild seahorses from various regions along the Chinese coast are shown in Figure 4. The B[a]P contents varied widely among the samples collected from different locations, ranging from 1.629 to 8.746 ug/kg (Figure 4A). The accumulated B[a]P in H. mohnikei was higher at WH than at QHD and DY, with significant differences between the two populations. Similar results were obtained for H. trimaculatus, which contained higher B[a]P contents in LS than in BH and DS. However, the accumulated B[a]P in H. kelloggi was relatively similar between the three regions. Among the different populations of seahorses, H. trimaculatus contained more accumulated B[a]P than H. mohnikei, and significantly more than H. kelloggi (P < 0.05) (Figure 4B).
Figure 4 Benzo(A)pyrene (B[a]P) contents of wild seahorses from different coastal regions (A) and in different species (B). The same color represents the same species of seahorse. The letters in the Figure represent significant variations between species (P < 0.05).
Large microplastic accumulations were observed in the intestines of wild seahorses from all sites sampled in this study, ranging from 92 to 322 particles per individual (Figure 5A). Relatively large differences in the accumulated microplastic particles were observed among seahorses of the same population at different locations. For example, the population of H. mohnikei in Weihai contained more particles than those in QHD and DY. H. trimaculatus samples from LS contained more particles than the samples from BH, and samples from both locations contained more particles than samples than those derived from DS. H. kelloggi samples from XS contained significantly higher numbers of particles than samples from SY. Further, among the seahorse populations, the number of microplastics observed in H. mohnikei and H. trimaculatus were significantly higher than in H. kelloggi (P < 0.05) (Figure 5B). Comparisons between samples collected from each region indicated that the number of microplastic particles was higher in samples collected from four regions (QHD, WH, BH, and LS). The heavy metal and B[a]P contents were also higher in samples from these regions. Therefore, the number of microplastic particles in seahorse intestines is likely related to the pollution levels of the region inhabited by the seahorses.
Figure 5 Microplastic contents of wild seahorses from different regions (A) and in different species (B). The same color represents the same species of seahorse. The letters in the Figure represent significant variations between species (P < 0.05).
At all collection sites, the most common colors observed for MPs in the seahorse were blue and green, accounting for 26% -33% and 20% -26% respectively, followed by black and white, while less MPs were transparent and other colors (Figure 6A). Seahorse collected at all locations were detected to have MPs ranging in size from 20-2000μm in their intestines (Figure 6B). The proportion of MPs with a size of less than 200μm is the highest, 37% -75%, respectively. Followed by 200-500μm and 500-1000μm, MPs with a size of more than 1000μm are less. In this study, no MPs larger than 2000μm were found in the seahorse. The main polymer types of MPs detected in the seahorse collected from all sites were polyethylene (PE), polyamide (PA), polyurethane (PU), polycarbonate (PC), polyethylene terephthalate (PET), etc (Figure 6C). The proportion of different polymer types in different collection sites is different. PE and PA polymers accounted for the highest proportion in all collection sites, followed by PU.
Correlation analysis was conducted between the content of heavy metal components, B[α]P content and the amount of microplastics in the intestinal tract of wild seahorses collected from various regions (Figure 7). The results showed that there was no significant correlation between the content of heavy metals and B[α]P in the intestine of seahorses (P > 0.05), indicating that there was no synergistic absorption of heavy metals and B[α]P in seahorses. There was no significant correlation between the amount of microplastics and the content of Cu and Hg (P > 0.05), but there was a highly significant correlation with B[α]P (P < 0.01). There were also significant correlations between microplastic particles and heavy metals Pb, Cd and Cr (P < 0.05). There was also a significant positive correlation (P < 0.01) between the content of various heavy metals in the wild seahorse.
Figure 7 Pearson correlation analysis results of the different pollutant types accumulated in seahorses. The colors indicate the correlation values (r). The asterisks represent a significant correlation at the 0.05 level (*) and 0.01 level (**), respectively.
Recent studies have shown that heavy metals, persistent organic pollutants, and microplastic pollution produce increasingly severe impacts on the marine environment. These pollutants also migrate upwards through the food chain, bioaccumulating in organisms and ultimately posing threats to human health and safety (Tang et al., 2021; Du et al., 2022). Seahorses have been labeled as a flagship species for marine conservation. However, their sensitivity to marine pollution and ability to bioaccumulate heavy metals at low levels, inhibiting growth and causing death, are of growing concern (Sun et al., 2019). Previous studies have determined that heavy metal pollution is common in most coastal areas around China (Han et al., 2021; Wang et al., 2022). Interestingly, in this study, we found that the heavy metal bioaccumulation in seahorses collected from different locations along the coastal waters of China was lower than that in majority of other marine species previously reported. We infer that this is because the smaller seahorse feeds primarily on plankton, which occupy a lower trophic niche than most carnivorous fish (Tipton and Bell, 1988), contributing to a lower cumulative efficiency. As adults, seahorses feed mainly on small crustaceans, including mysids shrimp, amphibians and other benthic crustaceans. The different seahorse populations were contaminated with Cu and Cr, which corresponds with previous studies on zooplankton (El-Metwally et al., 2022) and phytoplankton (Szymańska-Walkiewicz et al., 2022). Consistent with the experiments of Sun et al. (2019) in which the seahorse ingested heavy metals and MPs adsorbed by mysid shrimp, resulting in the accumulation of heavy metals and MPs in the seahorse and inhibition of growth. Implying that the heavy metals accumulated in the seahorses were most likely derived from food chain transmission. In addition, seahorses collected from different locations varied remarkably in their heavy metal bioaccumulation. Our results suggest that different seahorse species, which represented different life histories and micro-habitats, could contribute towards these regional variations. For example, the H. mohnikei populations prefer to live with seagrass or seaweed in shallower water, where more heavy metal pollution was observed (Khoei, 2022). The maximum differences between heavy metals (Cu and Cr) were identified between the QHD and WH populations for H. mohnikei, suggesting that regional variations in marine pollution, in addition to species, might also play a critical role in seahorse heavy metal bioaccumulation.
Our results demonstrate that microplastics accumulated in all wild seahorse populations along China’s coast; however, there were variations among different locations. Further, the significant variation of microplastic contents among three seahorse species suggests that different seahorse species are impacted differently by microplastic pollution. The surface color of MPs was mainly blue and green in seahorse intestines collected in nine cities. The reason may be that the seahorse feeds mainly on plankton and small arthropods. MPs suspended in water cause seahorses to ingest. In a recent study, the color of MPs in shrimp was determined to be predominantly blue (Yan et al., 2021). This indicates that MPs float with water and are similar in color and shape to prey. Aquatic animals may confuse blue debris or particles with prey, Ory et al. (2017) also confirmed this view. According to Bermúdez and Swarzenski (2021) micro-size plastics’ size range between 20–200μm, thus size equivalent to diatoms, dinoflagellates, ciliates, and daphnids and meso-size plastics 200–2000μm, equivalent to Amphipods, appendicularians, chetognatos, copepods, and thaliaceans. The seahorse mainly feeds on small arthropods and plankton, and MPs can accumulate in the form of food chains. This makes smaller MPs accumulate in the seahorse. In this study, the highest proportion of MPs smaller than 200μm was found in the seahorse, followed by 200-500μm and 500-1000μm. This suggests that MPs accumulate in organisms more through ingestion and food chain accumulation. In this study, there were few MPs with a size of more than 1000μm in the intestinal tract of seahorse, and no MPs with a size of more than 2000μm were found, indicating that MPs with larger particle size were not easily ingested by seahorse. We speculate that the remarkable variation in body size and depth preference might contribute towards the final differentiation among species (Choo and Liew, 2003). For example, H. mohnikei and H. trimaculatus are relatively small and prefer benthic habitats in Shallow water (Lourie et al., 2005), yet relatively high levels of MPs pollution in offshore waters greatly increase their risk of ingesting microplastics (Vroom et al., 2017). In contrast, H. kelloggi prefers to live in deeper offshore water (depths exceeding 65 m) (Choo and Liew, 2003), where fewer microplastics occur, and this species has the largest body size in the Hippocampus genus and prefers to feed on larger shrimps (Botterell et al., 2019). This suggests that aquatic organisms may directly or indirectly increase the accumulation of microplastics in organisms through food chains or food webs (Tang et al., 2021). In this study, the main polymer types detected in all seahorse intestines were PE and PA. PE is one of the main polymers in the ocean and is widely used in everyday products such as plastic packaging bags, plastic bottles and films. High strength polyethylene is widely used in the production of fishing nets and ropes (Hung et al., 2022). PA mainly used in industrial manufacturing and textiles, and is also a difficult contaminant to remove in sewage treatment (Liu and Nowack, 2022). In this experiment, the wild seahorse came from nine coastal cities, and the bay received sewage discharge from inland rivers and coastal cities. The pollution level of MPs was relatively high, and the results confirmed this finding.
B[a]P content is one of the important environmental monitoring parameters (Cirillo et al., 2006; Gaspare et al., 2009). The toxic effects of B[a]P include tissue and organ damage and reduction of immune function in fish (Banaei et al., 2008). In the present study, the B[a]P content of the three seahorse species differed considerably. The B[a]P concentrations ranged from 1.6 to 8.7 μg/kg in all samples. In general, B[a]P contamination remains at relatively low levels among the wild seahorse species inhabiting the coast of China. The results were in accordance with the findings of studies on mussels originating from the Italian Adriatic and Campania coasts (Serpe et al., 2010), while the average B[a]P levels of farmed and wild mussels inhabiting the Gulf of Naples were found much higher at 15.4 ± 2.75 μg/kg and 29.5 ± 5.83 μg/kg, respectively (Mercogliano et al., 2016). B[a]P may be released to the aquatic environment through river runoff and industrial wastewater, and the aquaculture sites in the Bay of Naples are mainly located near local commercial and industrial activities, increasing the B[a]P content in mussels. The investigation of this experiment found that the B[a]P content in the seahorse collected in LS was much higher than that in other cities. It may be speculated that the junction of LS Port and the outer sea has a narrow diameter, insufficient seawater exchange, and the pollutants have a relatively large impact on the breeding environment, which increases the accumulation of B[a]P in the seahorse. In addition, we found a highly significant positive correlation between the B[α]P content in the seahorse population and the amount of MPs in the intestinal tract in this study. This may be related to the stronger adsorption of microplastics to heavy metals and organic pollutants (Fu et al., 2019). The adsorption of microplastics to pollutants in the environment and their re-release through the food chain into the organism may produce stronger toxicological effects (Liu et al., 2020b).
Microplastics are small in volume, with a large specific surface area and a porous and rough surface, easing their co-transport with other pollutants, such as polycyclic aromatic hydrocarbons (PAHs) and heavy metals (Wright et al., 2013; Fu et al., 2019). Thus, microplastics might disturb the bioaccumulation processes of other pollutants by altering their environmental behavior, toxicity, and bioavailability (Yang et al., 2020). Interestingly, we identified a strong correlation between microplastics and B[a]P, suggesting that the accumulation of microplastics might be associated with the accumulation of B[a]P in seahorses. A previous ex situ study proved that microplastics could carry B[a]P into the digestive system of zebrafish during a 24 h feeding experiment (Batel et al., 2018). In addition, we also found significant correlations between heavy metals (Pb, Cd, Cr) and microplastics, implying that microplastics might also increase the bioaccumulation of heavy metals, which corresponds with prior seahorse feeding studies (Sun et al., 2019). In addition, the correlation between microplastics and B[a]P may be due to the fact that the seahorse living environment is influenced by multiple pollutants and there may be a synergistic accumulation effect between pollutants. Therefore, the potential effect of microplastics on wild seahorse populations might be magnified, especially when multiple contaminant types co-exist in their habitat and environment.
Our study determined that wild seahorse populations in China commonly experience environmental pollutant exposure. Species and environmental pollution concentration are critical factors affecting the bioaccumulation in seahorses from different regions. Further, the remarkable correlation between microplastics and other pollutant types suggests that the synergistic effects of these toxic substances might threaten wild seahorse populations more than previously expected.
The original contributions presented in the study are included in the article/supplementary material. Further inquiries can be directed to the corresponding author.
The animal study was reviewed and approved by Ethics Committee of Tianjin Agricultural University.
JS: conceptualization, supervision, writing - review and editing, funding acquisition. YL: formal analysis, visualization, conceptualization, software, data curation, writing - original draft. DS: investigation, methodology, writing - review and editing. YY and PC: resources, visualization. All authors contributed to the article and approved the submitted version.
This work was supported by the National Key Research and Development Program of China (Grant No. 2018YFD0900206) and the Tianjin Science and Technology Program Project (21YDTPJC00340).
Author DS was employed by Tanjin Nongken Bohai Agricultural Group Co. Ltd.
The remaining authors declare that the research was conducted in the absence of any commercial or financial relationships that could be construed as a potential conflict of interest.
All claims expressed in this article are solely those of the authors and do not necessarily represent those of their affiliated organizations, or those of the publisher, the editors and the reviewers. Any product that may be evaluated in this article, or claim that may be made by its manufacturer, is not guaranteed or endorsed by the publisher.
Alves R., Oliveira T., Rosa I. L. (2013). “Seahorses in traditional medicines: A global overview,” (Springer Berlin Heidelberg), 207–240. doi: 10.1007/978-3-642-29026-8
Banaei M., Mir Vagefei A. R., Rafei G. R., Majazi Amiri B. (2008). Effect of sub-lethal diazinon concentrations on blood plasma biochemistry. Int. J. Environ. Res. 2, 189–198. Available at: https://pesquisa.bvsalud.org/portal/resource/pt/emr-86894
Barboza L. G. A., Cunha S. C., Monteiro C., Fernandes J. O., Guilhermino L.. (2020). Bisphenol a and its analogs in muscle and liver of fish from the north East Atlantic ocean in relation to microplastic contamination. exposure and risk to human consumers. J. Hazard Mater. 393, 122419. doi: 10.1016/j.jhazmat.2020.122419
Batel A., Borchert F., Reinwald H., Erdinger L., Braunbeck T.. (2018). Microplastic accumulation patterns and transfer of benzo[a]pyrene to adult zebrafish (Danio rerio) gills and zebrafish embryos. Environ. pollut. 235, 918–930. doi: 10.1016/j.envpol.2018.01.028
Bermúdez J. R., Swarzenski P. W. (2021). A microplastic size classification scheme aligned with universal plankton survey methods. MethodsX 8, 101516. doi: 10.1016/j.mex.2021.101516
Botterell Z. L. R., Beaumont N., Dorrington T., Steinke M., Thompson R. C., Lindeque P. K., et al. (2019). Bioavailability and effects of microplastics on marine zooplankton: A review. Environ. pollut. 245, 98–110. doi: 10.1016/j.envpol.2018.10.065
Chen Y., Li W., Xiang L., Mi X., Duan M., Wu C., et al. (2022). Fish personality affects their exposure to microplastics. Ecotox Environ. Safe 233, 113301. doi: 10.1016/j.ecoenv.2022.113301
Chipari-Gomes A. R., Delunardo F. A. C., Sadauskas-Henrique H., Mota S. B., de Almeida-Val V. (2017). Genotoxic and biochemical responses triggered by polycyclic aromatic hydrocarbons in freshwater and marine fish: Tambaqui and seahorse as bioindicatorsIn Ecotoxicology and Genotoxicology (pp. 278–304).
Choo C. K., Liew H. C. (2003). Spatial distribution, substrate assemblages and size composition of sea horses (Family syngnathidae) in the coastal waters of peninsular Malaysia. J. Mar. Biol. Assoc. UK 83, 271–276. doi: 10.1017/S0025315403007069h
Cirillo T., Montuori P., Mainardi P., Russo I., Triassi M., Amodio-Cocchieri R., et al. (2006). Multipathway polycyclic aromatic hydrocarbon and pyrene exposure among children living in campania (Italy). J. Environ. Sci. Health A Tox Hazard Subst Environ. Eng. 41, 2089–2107. doi: 10.1080/10934520600867854
Delunardo F., Carvalho L., Silva B., Galão M., Val A. L., Chippari-Gomes A. R. (2015). Seahorse (Hippocampus reidi) as a bioindicator of crude oil exposure. Ecotox Environ. Safe. 117, 28–33. doi: 10.1016/j.ecoenv.2015.03.016
Desforges J.-P. W., Galbraith. M., Ross. P. S. (2015). Ingestion of microplastics by zooplankton in the northeast pacific ocean. Arch. Environ. Con Tox. 69, 320–330. doi: 10.1007/s00244-015-0172-5
Du H., Xie Y., Wang J. (2022). Environmental impacts of microplastics on fishery products: An overview. Gondwana Res. 108, 213–220. doi: 10.1016/j.gr.2021.08.013
El-Metwally M. E. A., Abu El-Regal M. A., Abdelkader A. I., Sanad E. F.. (2022). Heavy metal accumulation in zooplankton and impact of water quality on its community structure. Arab J. Geosci. 15, 1–14. doi: 10.1007/s12517-021-09424-x
Foster S. I., Vincent A. V. (2004). Life history and ecology of seahorse: Implication of conservation and management. J. Fish Biol. 65, 1–61. doi: 10.1111/j.0022-1112.2004.00429.x
Foster S., Wiswedel S., Vincent A. (2016). Opportunities and challenges for analysis of wildlife trade using CITES data – seahorses as a case study. Aquat. Conservation: Aquat Conserv. 26, 154–172. doi: 10.1002/aqc.2493
Fu D., Zhang Q., Fan Z., Qi H., Peng L. (2019). Aged microplastics polyvinyl chloride interact with copper and cause oxidative stress towards microalgae chlorella vulgaris. Aquat Toxicol. 216, 105319. doi: 10.1016/j.aquatox.2019.105319
Gao S., Yan K., Liang B., Shu R., Wang N., Zhang S., et al. (2022). The different ways microplastics from the water column and sediment accumulate in fish in haizhou bay. Sci. Total Environ. 158575. doi: 10.1016/j.scitotenv.2022.15857510.1016/j.scitotenv.2022.158575
Gaspare L., Machiwa J. F., Mdachi S. J., Streck G., Brack W. (2009). Polycyclic aromatic hydrocarbon (PAH) contamination of surface sediments and oysters from the inter-tidal areas of dar es salaam, Tanzania. Environ. pollut. 157, 24–34. doi: 10.1016/j.envpol.2008.08.002
Han X., Wang J., Cai W., Xu X., Sun M. (2021). The pollution status of heavy metals in the surface seawater and sediments of the tianjin coastal area, north China. Int. J. Env. Res. Pub He. 18, 11243. doi: 10.3390/ijerph182111243
Honryo T., Sakurai Y., Yamao S., Okada T., Agawa Y., Sawada Y., et al. (2021). The problem of marine litters for cultured teleost. Mar. pollut. Bull. 170, 112679. doi: 10.1016/j.marpolbul.2021.112679
Hossain M. (2020). 1. heavy metal contamination in tilapia, oreochromis niloticus collected from different fish markets of mymensingh district. J. Agriculture Food Environ. (JAFE)| ISSN (Online Version): 2708-5694 1 (4), 1–5. doi: 10.47440/JAFE.2020.1401
Hung C.-M., Huang C.-P., Hsieh S.-L., Chen Y. T., Ding D. S., Hsieh S., et al. (2022). Exposure of goniopora columna to polyethylene microplastics (PE-MPs): Effects of PE-MP concentration on extracellular polymeric substances and microbial community. Chemosphere 297, 134113. doi: 10.1016/j.chemosphere.2022.134113
Ju Y.-R., Chen C.-F., Wang M.-H., Chen C.-W., Dong C.-D. (2022). Assessment of polycyclic aromatic hydrocarbons in seafood collected from coastal aquaculture ponds in Taiwan and human health risk assessment. J. Hazard Mater 421, 126708. doi: 10.1016/j.jhazmat.2021.126708
Khoei A. J. (2022). A comparative study on the accumulation of toxic heavy metals in fish of the Oman Sea: effects of fish size, spatial distribution and trophic level. Toxin Rev., 1–8. doi: 10.1080/15569543.2022.2040033
Koning S., Hoeksema B. W. (2021). Diversity of seahorse species (Hippocampus spp.) in the international aquarium trade. Diversity 13 (5), 187. doi: 10.3390/d13050187
Liu Y., Feng Y., Li J., Zhou D., Guo R., Ji R., et al. (2020b). The bioaccumulation, elimination, and trophic transfer of BDE-47 in the aquatic food chain of chlorella pyrenoidosa-daphnia magna. Environ. pollut. 258, 113720. doi: 10.1016/j.envpol.2019.113720
Liu Q., Liao Y., Shou L. (2018). Concentration and potential health risk of heavy metals in seafoods collected from sanmen bay and its adjacent areas, China. Mar. pollut. Bull. 131, 356–364. doi: 10.1016/j.marpolbul.2018.04.041
Liu Q., Liao Y., Xu X., Shi X., Zeng J., Chen Q., et al. (2020a). Heavy metal concentrations in tissues of marine fish and crab collected from the middle coast of zhejiang province, China. Environ. Monit Assess. 192 (5), 1–12. doi: 10.1007/s10661-020-8234-1
Liu Z., Nowack B. (2022). Probabilistic material flow analysis and emissions modeling for five commodity plastics (PUR, ABS, PA, PC, and PMMA) as macroplastics and microplastics. Resour Conserv. Recy 179, 106071. doi: 10.1016/j.resconrec.2021.106071
Lourie S. A., Green D. M., Vincent A. C. (2005). Dispersal, habitat differences, and comparative phylogeography of southeast Asian seahorses (Syngnathidae: Hippocampus). Mol. Ecol. 14, 1073–1094. doi: 10.1111/j.1365-294X.2005.02464.x
Mercogliano R., Santonicola S., Felice A. D., Anastasio A., Murru N., Ferrante M. C., et al. (2016). Occurrence and distribution of polycyclic aromatic hydrocarbons in mussels from the gulf of Naples, tyrrhenian Sea, Italy. Mar. pollut. Bull. 104, 386–390. doi: 10.1016/j.marpolbul.2016.01.015
Milenkovic B., Stajic J. M., Stojic N., Pucarevic M., Strbac S. (2019). Evaluation of heavy metals and radionuclides in fish and seafood products. Chemosphere 229, 324–331. doi: 10.1016/j.chemosphere.2019.04.189
Ngoc N. T. M., Chuyen N. V., Thao N. T. T., Duc N. Q., Trang N. T. T., Binh N. T. T., et al. (2020). Chromium, cadmium, lead, and arsenic concentrations in water, vegetables, and seafood consumed in a coastal area in northern Vietnam. Environ. Health Insig 14, 1178630220921410. doi: 10.1177/1178630220921410
Ory N. C., Sobral P., Ferreira J. L., Thiel M. (2017) Amberstripe scad decapterus muroadsi (Carangidae) fish ingest blue microplastics resembling their copepod prey along the coast of rapa nui (Easter island) in the south pacific subtropical gyre. Sci. Total Environ 586, 430–437. doi: 10.1016/j.scitotenv.2017.01.175
Pandion K., Khalith S. M., Ravindran B., Chandrasekaran M., Rajagopal R., Alfarhan A., et al. (2022). Potential health risk caused by heavy metal associated with seafood consumption around coastal area. Environ. pollut. 294, 118553. doi: 10.1016/j.envpol.2021.118553
Pojana G., Gomiero A., Jonkers N., Marcomini A. (2007). Natural and synthetic endocrine disrupting compounds (EDCs) in water, sediment and biota of a coastal lagoon. Environ. Int. 33, 929–936. doi: 10.1016/j.envint.2007.05.003
Quintanilla-Mena M., Vega-Arreguin J., Río-García D., Patiño-Suárez V., Peraza-Echeverria S., Puch-Hau C. (2021). The effect of benzo [a] pyrene on the gut microbiota of Nile tilapia (Oreochromis niloticus). Appl. Microbiol. Biot 105 (20), 7935–7947. doi: 10.1007/s00253-021-11592-5
Qu C., Liu S., Tang Z., Li J., Liao Z., Qi P. (2019). Response of a novel selenium-dependent glutathione peroxidase from thick shell mussel mytilus coruscus exposed to lipopolysaccharide, copper and benzo [α] pyrene. Fish Shellfish Immun. 89, 595–602. doi: 10.1016/j.fsi.2019.04.028
Serpe F. P., Esposito M., Gallo P., Serpe L. (2010). Optimisation and validation of an HPLC method for determination of polycyclic aromatic hydrocarbons (PAHs) in mussels. Food Chem. 122, 920–925. doi: 10.1016/j.foodchem.2010.03.062
Song D., Zhuang D., Jiang D., Fu J., Wang Q. (2015). Integrated health risk assessment of heavy metals in suxian county, south China. Int. J. Env. Res. Pub He. 12, 7100–7117. doi: 10.3390/ijerph120707100
Storelli M. M. (2008). Potential human health risks from metals (Hg, cd, and Pb) and polychlorinated biphenyls (PCBs) via seafood consumption: Estimation of target hazard quotients (THQs) and toxic equivalents (TEQs). Food Chem. Toxicol. 46 (8), 2782–2788. doi: 10.1016/j.fct.2008.05.011
Sun J., Xia S., Ning Y., Pan X., Qu J., Xu Y., et al. (2019). Effects of microplastics and attached heavy metals on growth, immunity, and heavy metal accumulation in the yellow seahorse, Hippocampus kuda bleeker. Mar. pollut. Bull. 149, 110510.110511–110510.110517. doi: 10.1016/j.marpolbul.2019.110510
Szymańska-Walkiewicz M., Glińska-Lewczuk K., Burandt P., Obolewski K. (2022). Phytoplankton sensitivity to heavy metals in Baltic coastal lakes. Int. J. Env. Res. Pub He. 19, 4131. doi: 10.3390/ijerph19074131
Tang Y., Liu Y., Chen Y., Zhang W., Zhao J., He S., et al. (2021). A review: Research progress on microplastic pollutants in aquatic environments. Sci. Total Environ. 766, 142572. doi: 10.1016/j.scitotenv.2020.142572
Tian X., Beén F., Bäuerlein P. S. (2022). Quantum cascade laser imaging (LDIR) and machine learning for the identification of environmentally exposed microplastics and polymers. Environ. Res. 212, 113569. doi: 10.1016/j.envres.2022.113569
Tipton K., Bell S. S. (1988). Foraging patterns of two syngnathid fishes: Importance of harpacticoid copepods. Mar. Ecol. Prog. Ser. 47, 31–13. doi: 10.3354/meps047031
Vincent A. C., Foster S., Koldewey H. (2011). Conservation and management of seahorses and other syngnathidae. J. Fish Biol. 78, 1681–1724. doi: 10.1111/j.1095-8649.2011.03003.x
Vroom R., Koelmans A. A., Besseling E., Halsband C. (2017). Aging of microplastics promotes their ingestion by marine zooplankton. Environ. pollut. 231, 987–996. doi: 10.1016/j.envpol.2017.08.088
Wang L., Wang X., Chen H., Wang Z., Jia X. (2022). Oyster arsenic, cadmium, copper, mercury, lead and zinc levels in the northern south China Sea: Long-term spatiotemporal distributions, combined effects, and risk assessment to human health. Environ. Sci. pollut. R. 29, 12706–12719. doi: 10.1007/s11356-021-18150-6
Wright S. L., Thompson R. C., Galloway T. S. (2013). The physical impacts of microplastics on marine organisms: A review. Environ. pollut. 178, 483–492. doi: 10.1016/j.envpol.2013.02.031
Yang W. F., Gao X. X., Wu Y. X., Wan L., Tan L. C., Yuan S. M., et al. (2020). The combined toxicity influence of microplastics and nonylphenol on microalgae Chlorella pyrenoidosa. Ecotox Environ. Safe. 195, 110484. doi: 10.1016/j.ecoenv.2020.110484
Yan M., Li W., Chen X., He Y., Zhang X., Gong H. (2021). A preliminary study of the association between colonization of microorganism on microplastics and intestinal microbiota in shrimp under natural conditions. J. Hazard Mater 408, 124882. doi: 10.1016/j.jhazmat.2020.124882
Keywords: seahorse, bioaccumulation, microplastics, heavy metal, benzo(α)pyrene 1
Citation: Liu Y, Shang D, Yang Y, Cui P and Sun J (2022) Bioaccumulation of contaminants in wild seahorses collected from coastal China. Front. Mar. Sci. 9:1021170. doi: 10.3389/fmars.2022.1021170
Received: 17 August 2022; Accepted: 03 October 2022;
Published: 17 October 2022.
Edited by:
Jana Asselman, Ghent University, BelgiumReviewed by:
Jorge Palma, University of Algarve, PortugalCopyright © 2022 Liu, Shang, Yang, Cui and Sun. This is an open-access article distributed under the terms of the Creative Commons Attribution License (CC BY). The use, distribution or reproduction in other forums is permitted, provided the original author(s) and the copyright owner(s) are credited and that the original publication in this journal is cited, in accordance with accepted academic practice. No use, distribution or reproduction is permitted which does not comply with these terms.
*Correspondence: Jinhui Sun, amhzdW4xMDA4QDE2My5jb20=
Disclaimer: All claims expressed in this article are solely those of the authors and do not necessarily represent those of their affiliated organizations, or those of the publisher, the editors and the reviewers. Any product that may be evaluated in this article or claim that may be made by its manufacturer is not guaranteed or endorsed by the publisher.
Research integrity at Frontiers
Learn more about the work of our research integrity team to safeguard the quality of each article we publish.