- 1Marine Mammal Laboratory, Alaska Fisheries Science Center, National Oceanic and Atmospheric Administration, Seattle, WA, United States
- 2Cooperative Institute for Climate, Ocean, & Ecosystem Studies, University of Washington, Seattle, WA, United States
- 3Pacific Marine Environmental Laboratory, National Oceanic and Atmospheric Administration, Seattle, WA, United States
A key aspect of foraging ecology research is understanding how predator foraging behavior and success are influenced by variation in prey resources. For northern fur seals (Callorhinus ursinus), an understanding of predator-prey relationships is critical to help identify potential causes for the unexplained population decline in Alaska. To examine how foraging behavior differs based on prey size selection, we equipped northern fur seals on St. Paul and St. George islands (Alaska, USA) in September (2017, n=6) and August (2018, n=4, and 2019, n=3) with satellite-linked dive recorders and animal-borne video cameras. We categorized prey capture attempts based on relative prey size (small vs. large) and examined differences in capture depth, time of day, water temperature, and depth relative to the mixed-layer. Successful prey captures (n= 2224) primarily occurred at night (89.7 ± 3.1%) and small prey accounted for the majority of captures (70.5 ± 13.2%), but there was significant variation among individuals. Large prey were captured at nearly twice the depth of small prey (42.9 ± 3.7 m and 23.1 ± 1.8 m, respectively) and the proportion of large prey caught during the day was 3 times higher than at night (0.77 ± 0.1 vs. 0.25 ± 0.1). There was no relationship between prey size and water temperature after we accounted for temperature changes with depth. The highest proportion of prey captures occurred below the mixed-layer depth regardless of prey size, but the proportion of small prey captures above mixed-layer depth was double that of large prey. This enhanced understanding of northern fur seal prey capture behavior will be pivotal for better interpretation of decades of historical dive and diet data and can provide insight into how northern fur seals may respond to future variation in prey resources, which is essential to develop ecosystem-based approaches for northern fur seal conservation.
Introduction
Advances in biologging technology, such as dive recorders and satellite tracking instruments, have greatly expanded our ability to understand the at-sea behavior of marine predators (Kooyman, 2004; Rutz and Hays, 2009; Cooke et al., 2012). These instruments, which can be used to track habitat use and underwater movements, have provided insight into the foraging behavior of numerous species including the ability to identify how physiology, environmental conditions, and prey availability shape behavior (Butler et al., 1995; Block, 2005; Evans et al., 2013). Yet, for many species, collecting data on when and where successful foraging occurs has been challenging. Recent improvements to animal-borne video technology, specifically a reduction in size and increased recording duration, have made it possible to address this challenge and get a direct view into the foraging behavior of marine predators (e.g., Kernaléguen et al., 2016; Handley et al., 2018; Sutton et al., 2020; Yoshino et al., 2020). In addition, unlike other techniques used to quantify prey capture, such as jaw sensors or stomach temperature recorders (Austin et al., 2006; Liebsch et al., 2007; Iwata et al., 2011), video cameras can often provide more detail about the specific prey being targeted (e.g., species, age-class, or size) (Watanabe and Takahashi, 2013; Heaslip et al., 2014; Volpov et al., 2015; Yoshino et al., 2020). This detailed information about predator-prey interactions and foraging success obtained from animal-borne video can be used to link fine-scale behavior and habitat use with prey availability, which is essential for developing ecosystem-based conservation approaches for marine predators.
Understanding predator-prey relationships for the northern fur seal (Callorhinus ursinus) is critical to help identify potential causes for the unexplained population decline. The Eastern Pacific stock of northern fur seals, which includes animals that breed on St. Paul, St. George, and Bogoslof islands (Alaska, USA), once comprised over three-quarters of the world’s population (Loughlin et al., 1994; Gentry, 1998; NMFS, 2007). The Eastern Pacific stock was designated as depleted under the Marine Mammal Protection Act in 1988 and continued declines since the 1970s have resulted in pup production on St. Paul Island surpassing a 100-year low in 2018 (Towell et al., 2006; NMFS, 2007; Towell et al., 2018). The northern fur seal conservation plan identifies possible reasons for the decline including natural factors (e.g., predation, disease, and environmental change), human-related factors (e.g., effects of commercial fishing, pollution, and poaching), or a combination of multiple factors (NMFS, 2007). Obtaining more information about relationships between fur seals and fish resources in the Bering Sea has been identified as an important research priority to recover and protect this stock (NMFS, 2007).
On the Pribilof Islands (St. Paul and St. George), variability in northern fur seal diet during the summer reproductive season is correlated with rookery location and the use of distinct foraging habitats within the Bering Sea (Zeppelin and Ream, 2006). For adult female fur seals, a long-term diet study found twenty-three fish and four squid species are consumed, with the frequency of occurrence (FO) varying based on rookery location and whether an animal forages in the deep Bering Sea basin (> 200 m) or on the Bering shelf (Zeppelin and Ream, 2006). Nevertheless, regardless of rookery location, the fur seals’ primary prey species is walleye pollock (Gadus chalcogrammus, hereafter “pollock”, FO 42.7% - 91.4%, Zeppelin and Ream, 2006; Jeanniard-du-Dot et al., 2017), which is also the target of the largest commercial fishery by volume in the United States (NMFS, 2020). Patterns of annual variation in fur seal diet at the population level suggest that although both young and adult pollock are consumed, pollock age-classes are differentially targeted each year (Sinclair and Loughlin, 1994; Gudmundson et al., 2006; McHuron et al., 2020). These age-related consumption patterns coincide with annual variations in pollock recruitment with a high prevalence of age-0 pollock in the fur seal diet during years of strong recruitment and larger pollock identified in years with lower age-0 availability (Sinclair et al., 1996; McHuron et al., 2020). Studies of fur seal dive behavior suggest that there may also be variation among individuals within a year for the age-class of pollock being consumed (Kooyman and Goebel, 1986; Kuhn et al., 2010; Nordstrom et al., 2012).
To understand how foraging behavior varies when fur seals target different sizes or age-classes of prey, it is necessary to identify feeding events and the prey being consumed. By equipping northern fur seals with video cameras, we were able to characterize prey capture behavior, specifically examining the size of the targeted prey in relation to dive depth, time of day, and water temperature. Based on the biology and behavior of pollock and historic data on northern fur seal dive behavior, we hypothesized that 1) large fish will be captured at deeper depths and closer to the sea floor (Bailey, 1989; Sogard and Olla, 1993; Boldt et al., 2012); 2) large fish will be captured more often during the day (Kooyman and Goebel, 1986; Goebel et al., 1991; Kuhn et al., 2010); and 3) large fish will be captured at colder water temperatures, below the mixed-layer depth (Francis and Bailey, 1983; Olla and Davis, 1990; Boldt et al., 2012).
Materials and methods
Fur seal instrumentation
This research was conducted as part of a larger study examining the summer foraging behavior of northern fur seals on St. Paul and St. George islands, Alaska (Figure 1). We tracked adult female fur seals from July to September (2017) and August to September (2018 and 2019). Females observed with a dependent pup were captured, weighed using a digital scale (± 0.2 kg), and physically restrained to attach tracking instruments. Fur seals were equipped with a SPLASH10-X instrument (105 × 56 × 34 mm, 218 g, Wildlife Computers) and VHF tag (18 × 44 × 8 mm, 17 g, Advanced Telemetry Solutions) for the duration of the tracking period (July/August-September), and a CATS cam instrument (110 × 80 × 40 mm, 390 g, Customized Animal Tracking Solutions) for 1 to 3 foraging trips (2017: n=6, 2018: n=4, and 2019: n=3 fur seals). Instruments were attached directly to the dorsal fur using a combination of Loctite 422 and Devcon quick-set epoxy. The total mass of instruments was on average 1.7 ± 0.05% of body mass (max < 2.1%). The results presented only include data collected when the fur seals were equipped with all tracking equipment (SPLASH10-X, VHF tag, and CATS cam).
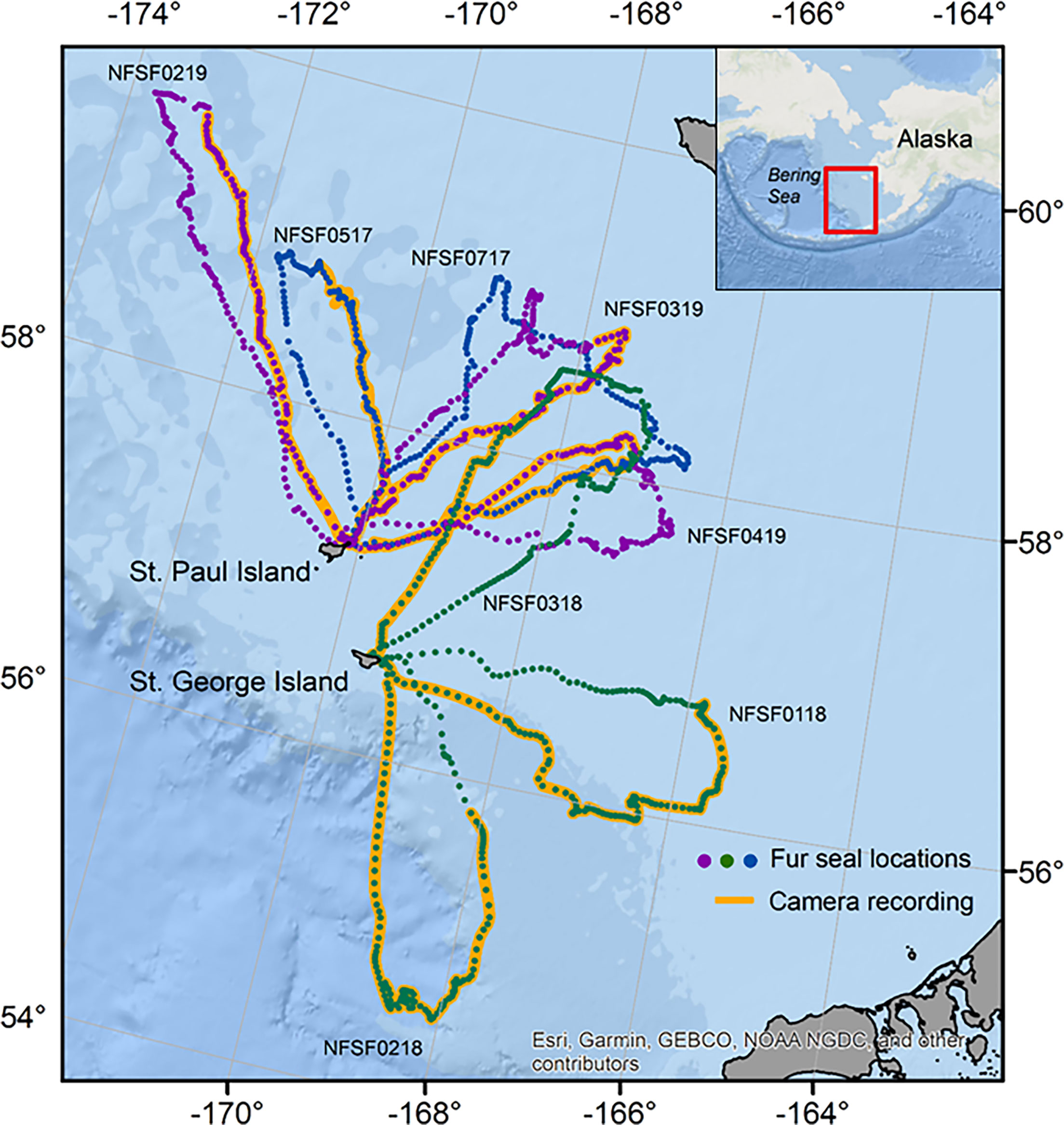
Figure 1 Hourly locations for eight northern fur seals (2017: blue, 2018: green, and 2019: purple points) equipped with animal-borne video cameras. The portion of the foraging trip with video data is highlighted in orange. Northern fur seals were instrumented on St. Paul Island and St. George Islands in the Bering Sea, Alaska (inset).
SPLASH10-X tags sampled depth and external temperature (fast-response thermistor) at 1-s intervals, triaxial acceleration at 1/16-s intervals, and provided satellite locations via the Argos satellite system. To conserve battery power, satellite transmissions were shut off between 0:00 and 01:59 GMT and 10:00 and 11:59 GMT, which are periods of reduced satellite coverage. The CATS cams were programmed to record depth and temperature at 1-s intervals and HD video when the fur seals’ depth exceeded 10 m. A depth trigger of 10 m was chosen to extend video recording duration over a longer proportion of the foraging trip by reducing the chance video was recorded during shallow dives used during transit periods. The cameras were equipped with two IR LEDs on either side of the lens that turned on in low light conditions, such as during deep dives or at night. Video was recorded at 30 frames per second and 1280 × 720 p resolution. The CATS cams recorded for a minimum duration of 1 min once triggered by depth and stopped recording when the fur seal was < 10 m deep or at a maximum duration of 5 min. The VHF tag was used to locate the fur seals on land for recapture. Fur seals were physically restrained for instrument removal and reweighed prior to release.
Data analysis
SPLASH10-X and CATS cam dive data were processed using Instrument Helper (Wildlife Computers) after converting the CATS file to a format that could be read by the software. Dives were defined as a minimum depth of 3 m. Videos were analyzed using BORIS (Behavioral Observation Research Interactive Software) (Friard and Gamba, 2016). For successful prey captures, we attempted to identify the prey to class or family using distinguishing characteristics and a dichotomous key created specifically for fish encountered in the Northeast Pacific groundfish fishery (Alaska Fisheries Science Center, North Pacific Observer Program, unpublished). Due to rapid movements during prey capture events and because most captures occurred in low light, we were unable to identify many preys except when large fish were targeted (Figure 2). Based on historic northern fur seal food habits information, if a prey item was identified as a fish, we assumed it belonged to the most abundant class in the diet, Actinopterygii (Sinclair and Loughlin, 1994; Antonelis et al., 1997; Zeppelin and Ream, 2006).
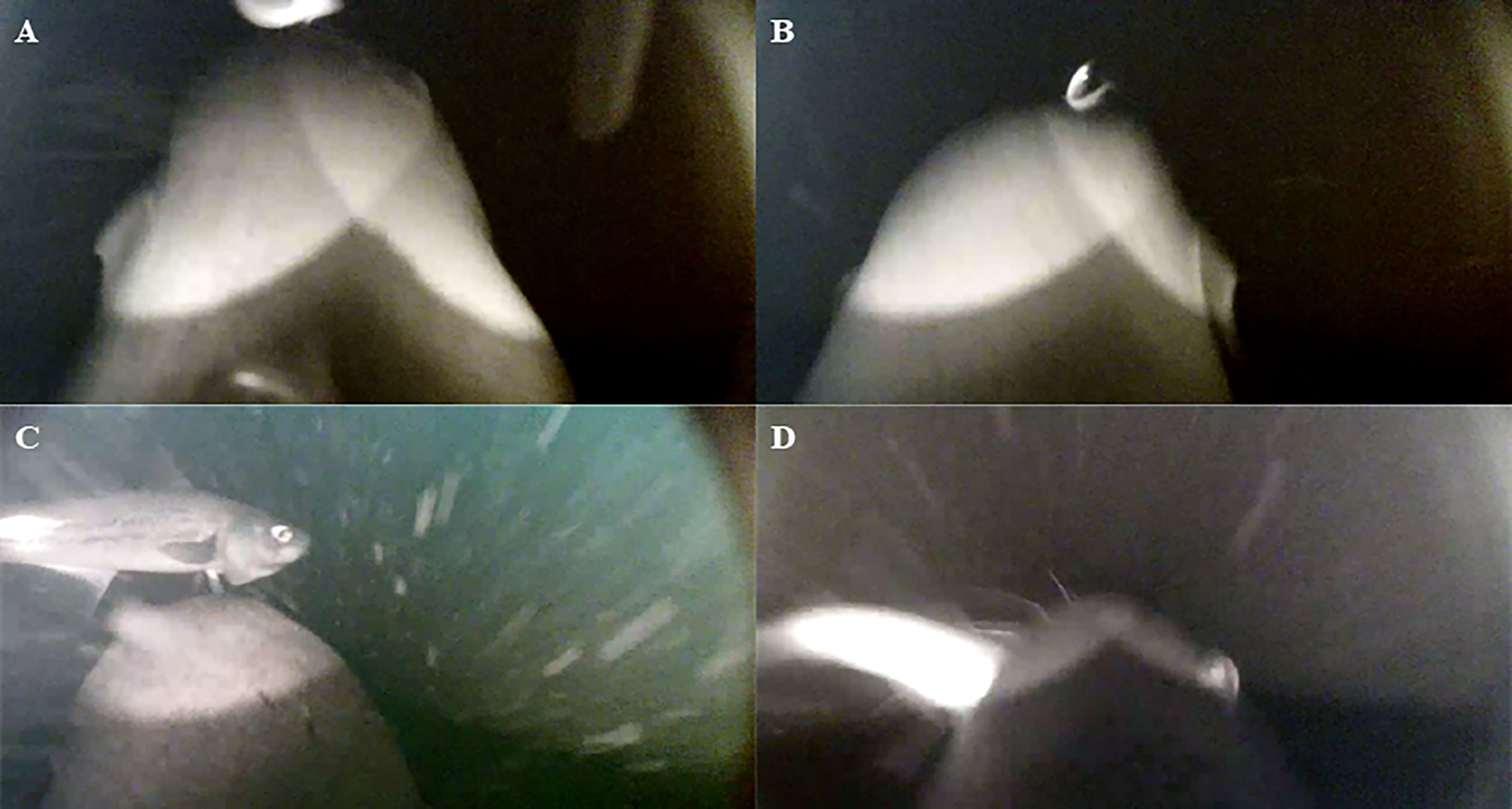
Figure 2 Still images collected from video files recorded from cameras mounted on northern fur seals. Cameras were placed in the upper back/shoulder region, so the fur seal’s head is visible. Images show examples of small prey prior to capture (A, B), and large prey prior to capture (C) and during handing (D). Illumination from the two IR LEDs is visible in night images (A, B, D) and at depth (C).
Northern fur seal diet in this region is dominated by a single species, walleye pollock, which suggests that prey size differences were related to fur seals targeting different pollock age classes. When fur seals foraged on the Bering Sea shelf (< 200 m), we are confident that in most cases the species being targeted was pollock (66.2% FO vs. the next highest prey species < 18% at all study locations) (Zeppelin and Ream, 2006). For fur seals that forage off the Bering Sea shelf, frequently targeted prey includes pollock (FO > 45%), northern smoothtongue (Leuroglossus schmidti) (maximum FO < 16%), and numerous squid species (FO up to 38%) (Zeppelin and Ream, 2006).
Each video was reviewed by two people to identify prey capture attempts (PCAs) and discrepancies were re-examined when the results were compiled. PCAs were characterized by a striking behavior and distinguished from other head movements by the presence of prey. For each PCA, reviewers determined if the prey was successfully captured (categories: captured or missed) and the size of the prey relative to the width of the fur seal’s head just prior to capture or during handling. Prey which were equal to or smaller than the fur seal’s head were classified as small and estimated to be approximately 150 mm or less, which would include age-0 and age-1 pollock (Figures 2A, B; Smith, 1979; Ianelli et al., 2019; McCarthy et al., 2020). Large fish, estimated to be larger than 150 mm, were often seen hanging out of the fur seal’s mouth when caught (Figures 2C, D). This cutoff was determined based on the pollock age-class distribution within the Bering Sea during the three study years, which generally followed a bimodal distribution with fewer pollock within the 200 to 350 mm length range (Ianelli et al., 2019; McCarthy et al., 2020).
In a few cases, fur seals displayed a stereotypical behavior that appeared to be chewing or repositioning prey after a clear head strike when no prey were visible; for example, when a strike occurred off camera or the fur seal’s head blocked the view. These were classified as successful PCAs but the prey size was marked as unknown. Given the lack of visible prey and the short duration of the handling movements, it is highly likely that these were small prey. As a result, they were included in the small prey category for analysis. We chose to not include missed PCAs in our analysis because we were unable to determine if subsequent capture attempts within a single dive were targeting the same or a different prey and did not want to overestimate prey interactions.
Factors related to prey capture
We examined prey size category in relation to three factors: capture depth, water temperature, and time of day. The SPLASH10-X and CATS cam data were synced by time using the dive analysis files, and capture depth and temperature were extracted from the raw SPLASH10-X data. Satellite locations were filtered based on a maximum transit rate of 3 m s-1 (R package “argosfilter”) after class Z locations were removed (Freitas et al., 2008). Foraging tracks were reconstructed at the time of each dive by modeling the filtered location data using a continuous-time correlated random walk model (Johnson et al., 2008). Time of day was a categorical variable (day or night) calculated using the start time of each dive with night being classified as a sun elevation < 0 (R package “maptools”).
We were also interested in how prey size category related to the mixed-layer depth (MLD) as it is likely driving prey capture relationships for both depth and temperature. Data from the annual Alaska Fisheries Science Center’s eastern Bering Sea bottom-trawl survey (available at https://www.fisheries.noaa.gov/alaska/commercial-fishing/alaska-groundfish-bottom-trawl-survey-data, Acuna and Lauth, 2008; Lauth and Acuna, 2009; Lauth, 2010) were used to estimate MLD, defined in this study as the depth at which temperature deviated by 0.2° C from a near surface (5.5 m) reference depth. MLD was estimated at each survey haul location and interpolated to dive locations using an inverse distance weighting method. MLD data from the annual surveys was used instead of temperature data from the tracking instruments because it has higher accuracy and it provided information throughout the fur seals’ habitat regardless of the depth of individual dives. For one fur seal (NFSF2018) that spent some time foraging off the Bering Sea shelf, the MLD was calculated using profile data from the closest Argo float (#4902930, June-August 2018, mldthermocline function in R by K. Evans, Roemmich et al., 2009). The average MLD over seven profiles was 27.5 ± 1.5 m (range: 22 to 32 m), which is similar to historical values reported in July and August for the Bering Sea basin (Roden, 1995; Johnson and Stabeno, 2017). The PCA depth in relation to the MLD was a categorical variable (above MLD, at MLD, below MLD). Above MLD included depths from the surface to 5 m above the MLD, at MLD included depths within ± 5 m of the MLD, and below MLD included depths > 5 m below the MLD to the seafloor. Two-minute gridded elevation data for the North Pacific derived from ETOPO 2 were used to estimate the seafloor depth at each dive position to calculate the relative depth of prey captures (capture depth/bottom depth).
Statistical analysis
We used generalized linear mixed-effects models (R package “lme4”, R 3.6.0) (R Core Team, 2019) to examine prey size category (large and small) in relation to capture depth, time of day, and water temperature. MLD was not included in the models because of its collinearity with both depth and temperature. Models were also run with PCAs of unknown prey size excluded from the small size category for comparison. Temperature residuals were used to account for the negative relationship between depth and water temperature. “Animal Id” was included as a random factor in all models and year was included as a fixed factor. We examined relationships between study year and fur seal mass, study year and daily mass change over the tracking period (mass change), mass change and the proportion of recorded dives with prey capture, and mass change and average prey captured per recorded dive using a linear regression after validating normality and homogeneity of variances. Summary statistics, including those presented in Tables 1 and 2, were calculated for each individual to account for large differences in the number of PCAs and presented as mean ± se. Factors were considered significant at P < 0.05.
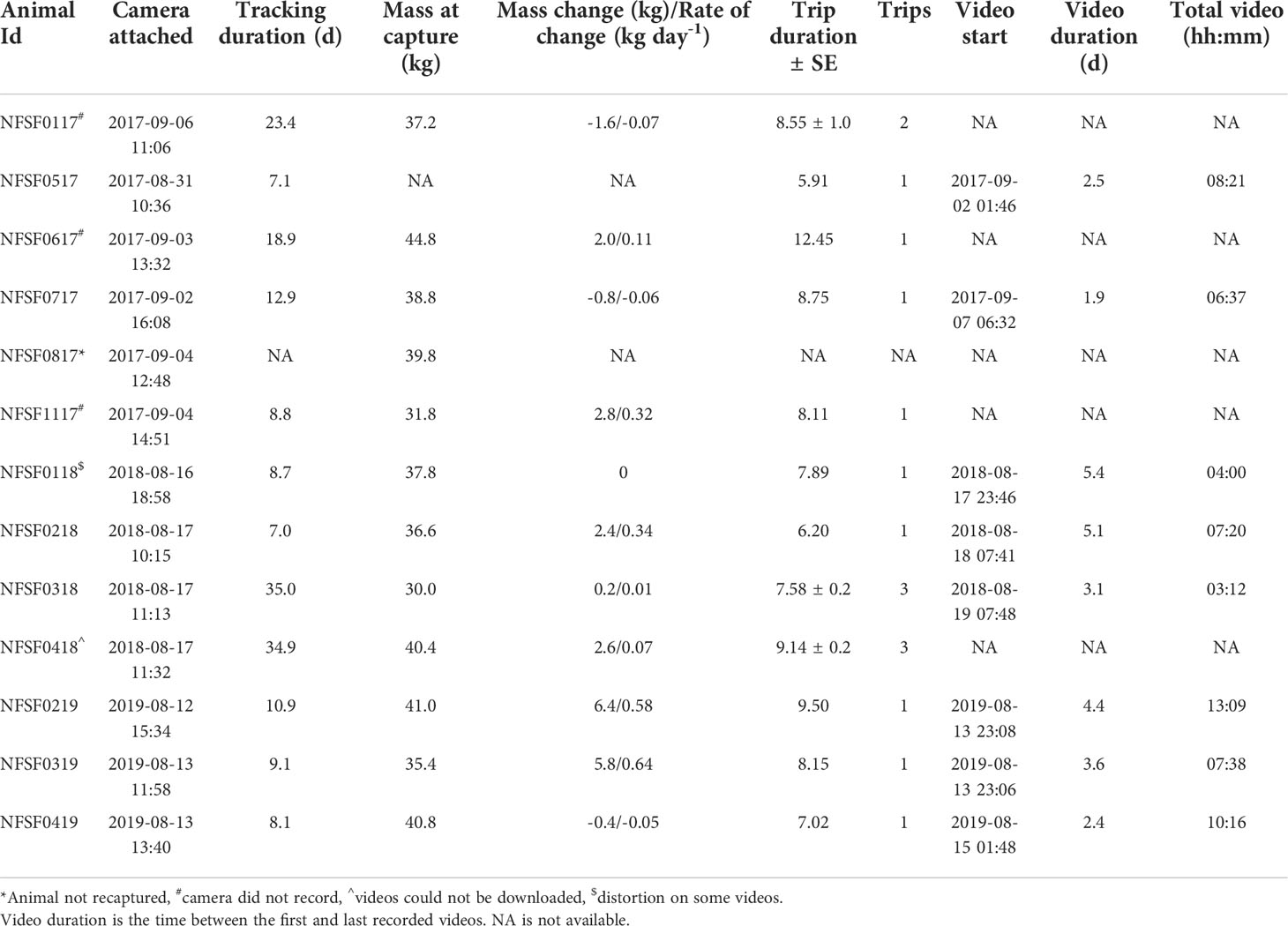
Table 1 Summary of tracking information and mass characteristics for 13 northern fur seals equipped with video cameras in 2017, 2018, and 2019.
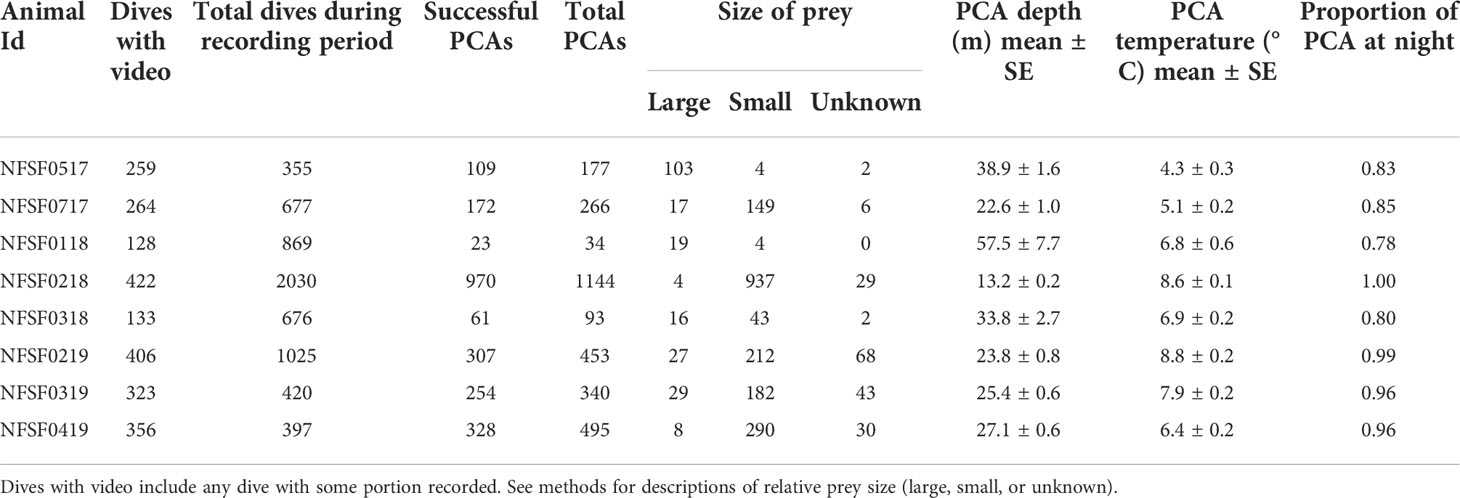
Table 2 Dive and prey capture attempt (PCA) metrics during the video recording period for eight northern fur seals tracked in 2017, 2018, and 2019.
Results
Fur seal behavior
At instrumentation, fur seals ranged in mass from 30.0 to 44.8 kg (average: 37.9 ± 1.2 kg, n=12) with no differences in mass among years (F2,9 = 0.47, p = 0.64, Table 1). Tracking durations ranged from 7.0 to 35.0 d (15.4 ± 3.0 d) covering 1 to 3 foraging trips that lasted 8.3 ± 0.5 d (range: 5.9 to 12.4 d, Table 1, Figure 1). In 2017, one fur seal was not recaptured after her satellite locations stopped while at-sea and her VHF tag was not heard again on the rookery. Mass change over the tracking period was 1.8 ± 0.8 kg (range: -1.6 to 6.4 kg, n=11, Table 1). The rate of mass change per day of tracking was 0.2 ± 0.08 kg d-1 and there were no differences among years (F2,8 = 1.72, p = 0.24, Table 1).
In 2017, software errors prevented three of the CATS cams from turning on and the two remaining CATS cams stopped after 255 videos were recorded. In 2018, one CATS cam didn’t function as programmed (NFSF0118) and the video recovered displayed intermittent color distortion which impacted our ability to analyze prey capture attempts. We ranked the video quality from 1 (no distortion) to 5 (can’t visualize any behavior) and removed videos classified as quality 4 (mostly distorted but can visualize some behavior) and 5. Because of the video quality, it is highly likely that some small prey captures were missed since small prey are harder to see. The inability to see small prey captures could impact some of the summary metrics calculated (e.g., PCA per hour or per dive) but would not impact the relationships between prey size and environmental covariates (e.g., depth and temperature). As a result, NFSF0118 was excluded from some summary metrics related to video data and prey capture where noted. Finally, in 2018, we were unable to download videos from one CATS cam.
Videos were recorded over 3.4 ± 0.5 d of each foraging trip (Table 1, Figure 1), with 60.3 total hours of video (hours per animal: 7.6 ± 1.0 h, Table 1). Videos were recorded for 51.2 ± 0.1% of the dives during the recording period (range: 19.7 to 89.7%, Table 2, NFSF0118 excluded). Out of 3002 recorded prey capture attempts, 2224 were successful (Table 2). Capture success varied among individuals (Table 2) and was not different between prey size classes (p=0.87, Figure 3). Successful PCAs were recorded on 976 dives, which meant prey capture occurred on 41.2 ± 5.1% of the recorded dives (range: 29.3 – 68.4%, NFSF0118 excluded). The average number of PCAs per recorded dive ranged from 0.4 to 2.2 (average: 0.87 ± 0.2, NFSF0118 excluded). If only recorded dives with prey capture are considered, fur seals captured 1.9 ± 0.04 prey per feeding dive (range: 1.2 to 3.4). There were no relationships between mass change over the tracking period and the proportion of recorded dives with prey capture (F1,5 = 0.11, p = 0.75) or the average number of prey captures per recorded dive (F1,5 < 0.001, p = 0.99).
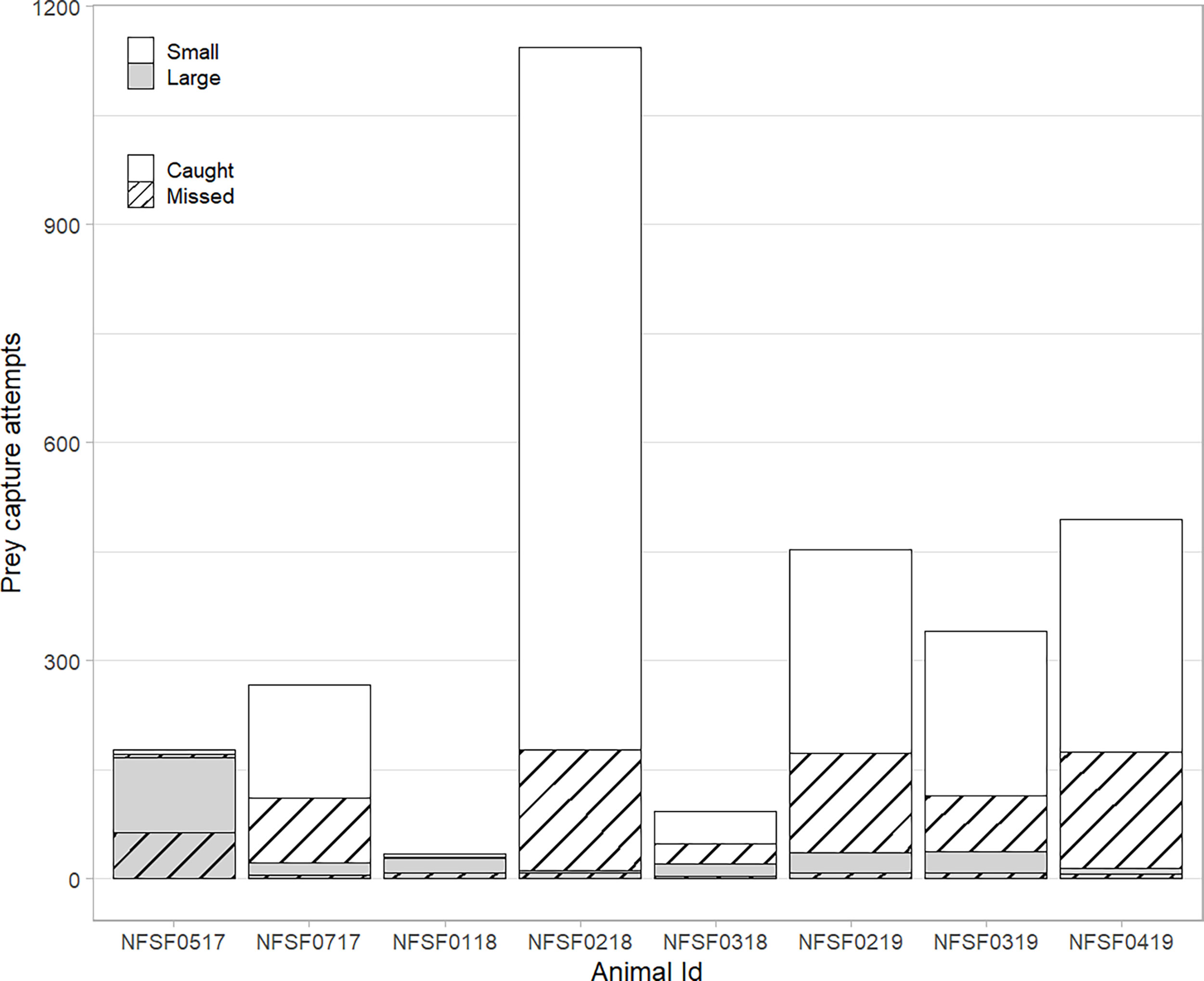
Figure 3 Prey capture success (caught= solid, missed= hatched) for eight northern fur seals by relative prey size (white= small, grey= large). Differences in total prey capture are primarily related to the duration of video recording (Table 1).
Small prey accounted for 70.5 ± 13.2% of all prey captures, but the percent of small prey captured varied between 5.5 and 99.6% per individual (Table 2). Successful PCAs occurred at depths ranging from the surface to 136.5 m (average: 30.3 ± 1.9 m, Table 2, Figures 4A, B) and temperatures ranging from 1.5 to 13.0 °C (average: 6.8 ± 0.2 °C, Table 2, Figure 4C). The average depth for video recorded dives was significantly deeper than average depth for all dives during the recording period (F1,14 = 8.15, p=0.01) due to the depth trigger of the CATS cam being set at 10 m (Figure 5). On numerous occasions, the videos continued to record during the ascent until the fur seals reached the surface which allowed us to observe prey captures between 0 and 10 m (Figure 4). In addition, due to the CATS cams having a minimum recording time, some dives less than 10 m were also recorded if they followed a short dive (i.e., less than 1 minute). There was a significant negative relationship between depth and water temperature for PCAs (R2 = 0.35, F1, 2222 = 1214, p< 0.001). Most PCAs occurred at night (89.7 ± 3.1%), which was relatively consistent among fur seals (Table 2). All fur seals but one had successful PCAs in each of the MLD categories (above, at, and below, Table 2, Figure 6) and the majority of individuals had the largest proportion of their PCAs occur below the MLD (Figure 6).
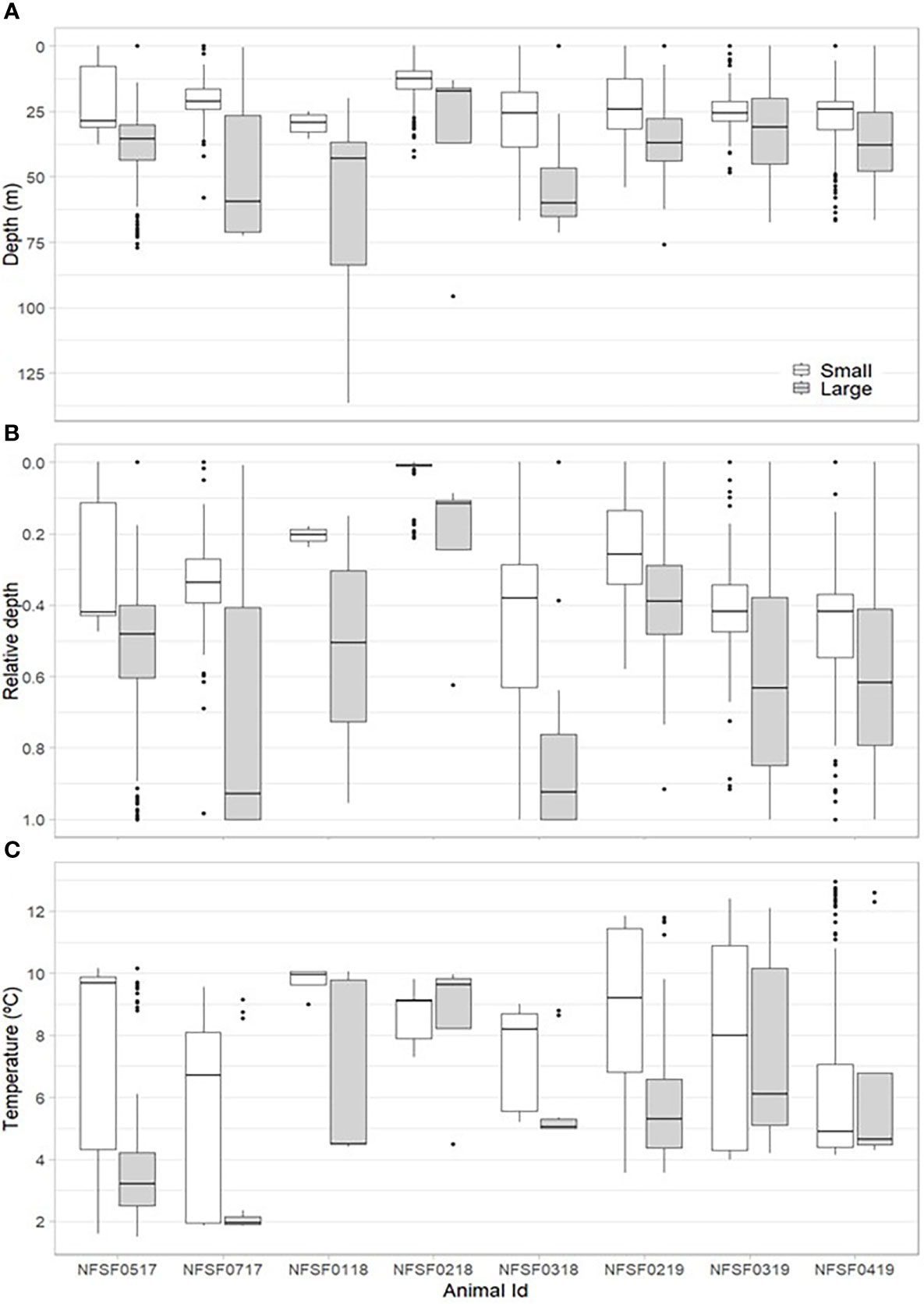
Figure 4 Boxplots depicting the depth (m, A), relative depth (depth/seafloor depth, B), and temperature (°C, C) where successful prey captures occurred for each fur seal by prey size (white= small, grey= large). Lines denote the median values, boxes encompass the first and third quartiles, points are outliers (> 1.5 * the inter-quartile range), and whiskers display the smallest or largest values beyond the inter-quartile range, excluding outliers.
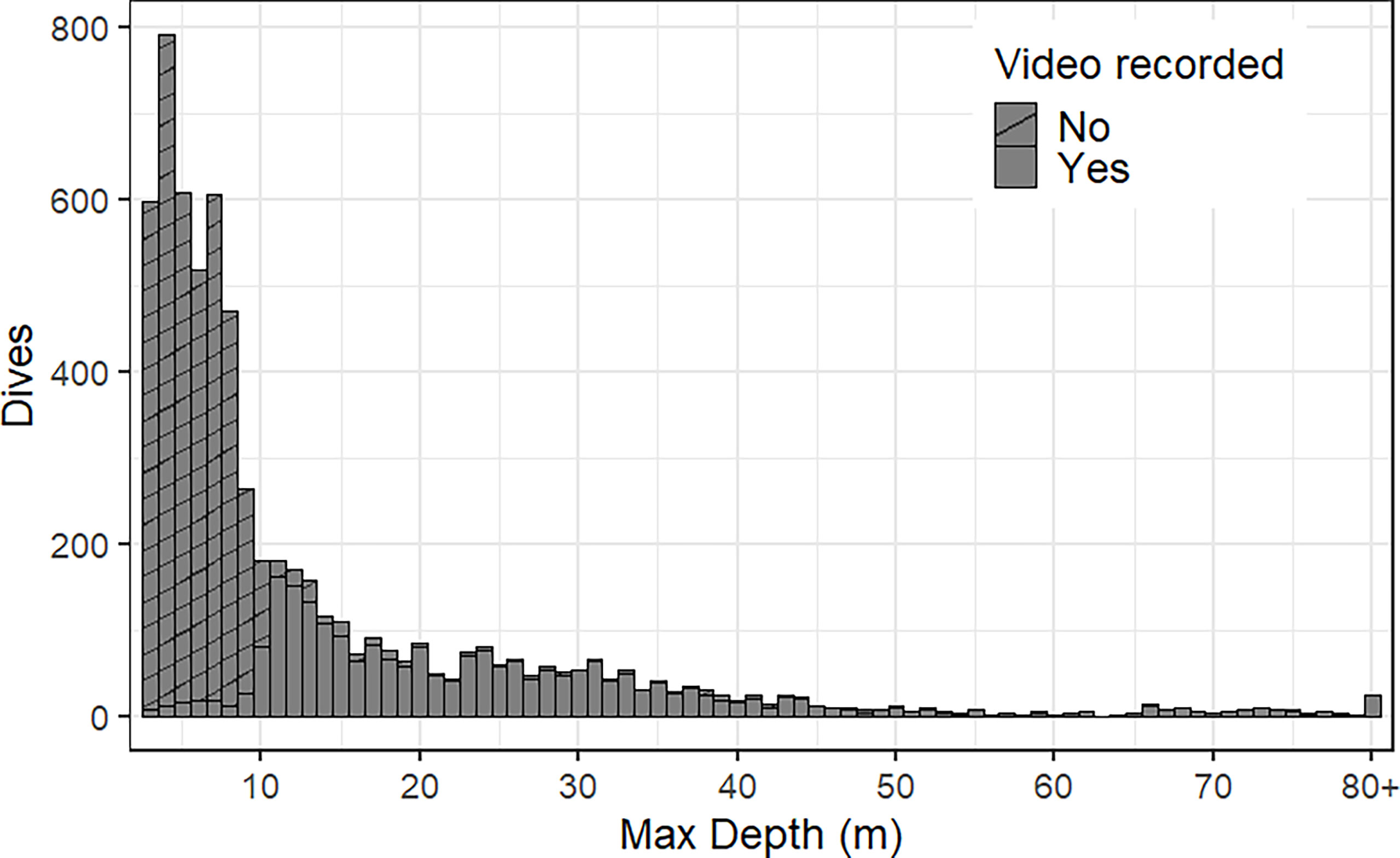
Figure 5 Distribution of northern fur seal maximum dive depths during the video recording period. Most dives under 10 m did not have video recordings (stripe filled) due to the preset depth trigger. However, as a result of the CATS cam minimum recording duration (1 min), some shallower dives (< 10 m) were recorded if they immediately followed a recorded dive (solid filled) with a short duration. Dives from NFSF0118 were excluded because of issues with the CATS cam and recorded videos (see Results for details).
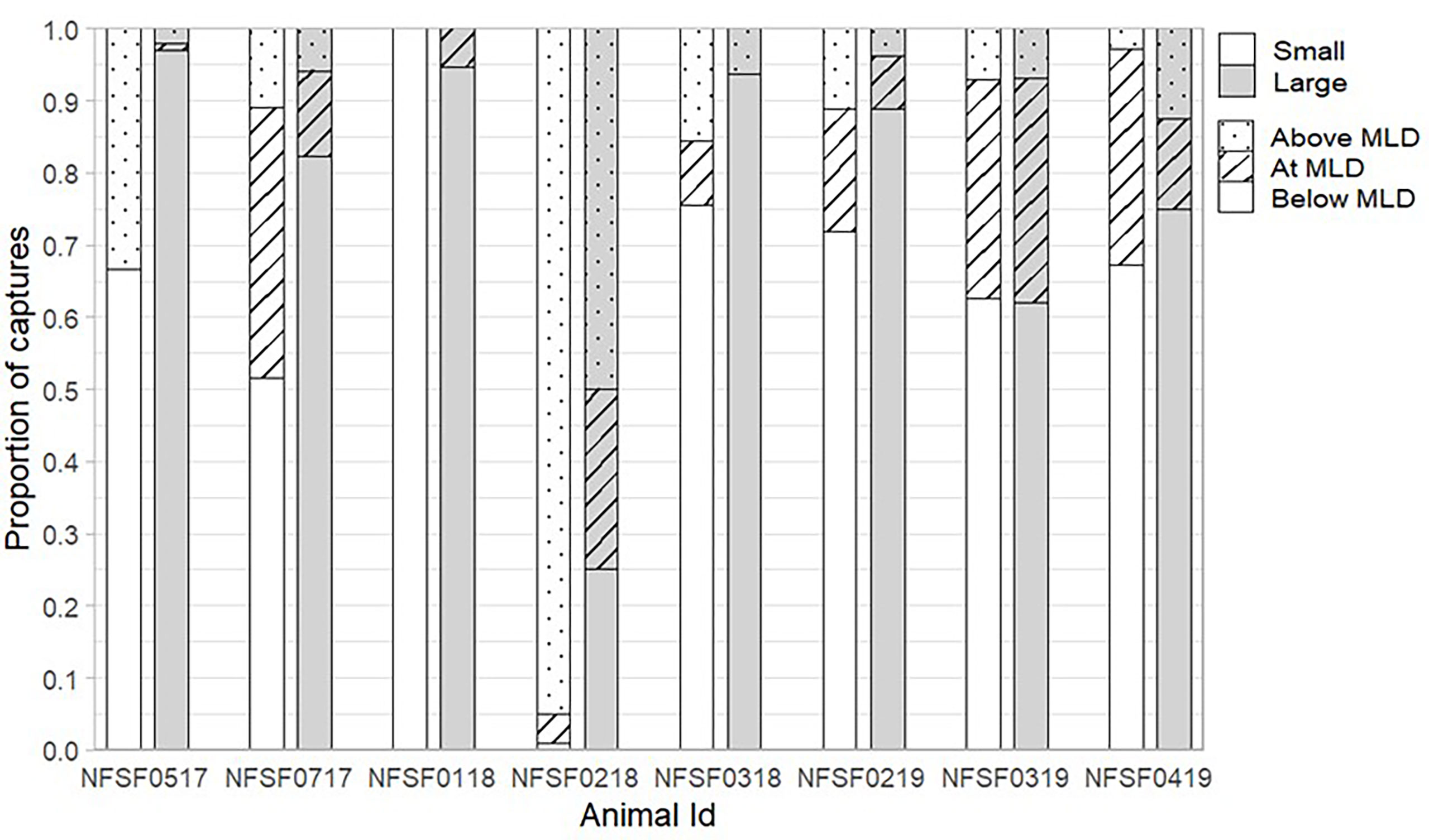
Figure 6 Proportion of prey captures in relation to the mixed-layer depth (MLD) for each fur seal by prey size (white= small, grey= large). Total captures for each female by prey size are found in Table 2. A description of each MLD category can be found in the methods.
Relationship between prey size and depth, water temperature, and time of day
Large prey was captured in deeper water and more often during the day than small prey (p< 0.001 for depth and time of day). There was no relationship between prey size and the temperature residuals and no differences among years. These relationships were similar when the unknown prey size class was excluded from the small prey group and when NFSF0218 was excluded due to some foraging occurring in the Bering Sea basin (depth: p<0.001, time of day: p< 0.001). On average, large prey were captured at nearly twice the depth of small prey (42.9 ± 3.7 m and 23.1 ± 1.8 m, respectively, Figure 4A) and at temperatures over 1.5 °C colder (6.0 ± 0.6 °C and 7.7 ± 0.5 °C, respectively, Figure 4C). The proportion of large fish caught during the day was 3 times higher than at night (0.77 ± 0.1 vs. 0.25 ± 0.1). For all fur seals combined, the highest proportion of PCAs occurred below the MLD regardless of size (large: 0.77 ± 0.9, small: 0.62 ± 0.1, Figure 6), but the proportion of PCAs for small prey above the MLD was double that of large prey (small: 0.25 ± 0.1, large: 0.12 ± 0.1, Figure 6). Only one fur seal, NFSF0218, had a higher proportion of PCAs above the MLD, which was consistent between prey sizes.
Prey identification
Over 40,000 images that contained visible prey during a successful capture event were examined to identify the prey being consumed (Figure 2). Prey were identified to class for 841 successful PCAs (41% of successful captures) using at least one identifiable feature or movement patterns (fish: n= 836, cephalopod: n= 5) (Supplemental Material, Table S1). For the fish identified, 129 could be narrowed down to family (Supplemental Material, Table S1), with 93.8% being identified as Gadidae. All of the Gadidae were categorized as large fish. Five Salmonidae and three Clupeidae (likely Pacific herring, Clupea pallasii) were also identified. An additional three fish had identifiable characteristics not consistent with family Gadidae, such as a highly forked tail (Supplemental Material Table S1). All Cephalopoda (n=5) were characterized as likely squid which could include several species from the family Gonatidae based on northern fur seal diet studies (Zeppelin and Ream, 2006).
Discussion
An understanding of fine-scale predator-prey relationships can provide insight into factors that influence an individual’s foraging decisions and success. By combining animal-borne video cameras with tracking instruments that record diving patterns and environmental characteristics, we were able to investigate the influence of prey size on northern fur seal foraging behavior. The incorporation of animal-borne video into marine predator tracking studies has exploded in recent years with videos helping to quantify prey encounter rates (e.g., Watanabe and Takahashi, 2013; Thiebot et al., 2016; Oliver et al., 2022), prey avoidance behavior (Handley et al., 2018; Mattern et al., 2018), location and timing of foraging (e.g., Yoshino et al., 2020; Sutton et al., 2021), and impacts of multi-species foraging groups (Sutton et al., 2020; Michel et al., 2022). Studies examining the foraging success of individuals are critical for understanding how changes to habitat and prey resources could impact overall population dynamics (e.g., Lewis et al., 2001; Pettorelli et al., 2001).
Although great strides have been made to increase capabilities while simultaneously reducing the size of animal-borne video cameras (Moll et al., 2007; Holton et al., 2021), most studies still require cameras to go through cycles of on/off periods to extend recording duration (e.g., Kernaléguen et al., 2016; Volpov et al., 2016). This can leave data gaps and result in missing rare behaviors (Sutton et al., 2020; Hinke et al., 2021) or rare prey captures (Pistorius et al., 2020; Hinke et al., 2021). Further, the additional animal handling requirements for video studies, which predominantly require that the animal is recaptured for instrument recovery, along with the increased financial cost of video cameras means that study sample sizes are generally small. These issues were factors in our study where only a small number of individuals could be tracked each year and video recordings did not cover the entire fur seal foraging trip (Table 1). In addition, other camera issues including failure to turn on, distorted video recordings, and shorter than expected recording durations further limited our sample size (Table 1). These challenges with small sample size also likely limited our ability to find relationships between foraging success and mass change over the tracking period. Regardless, our study provides greater insight into northern fur seal prey size selection, prey encounter rates, and foraging success, data which are not available for many large marine predators.
All fur seals foraged on a mix of small and large prey, although relative proportions varied among individuals. Past diet studies, which primarily sampled scat, have highlighted the importance of small pollock in the northern fur seal diet (Zeppelin and Ream, 2006; Sinclair et al., 2008; Call and Ream, 2012), but fewer studies have been able to assess the prominence of larger, adult pollock because enumerable hard parts (bones and otoliths) from larger fish rarely pass through the digestive system to be recovered in scat (Sinclair et al., 1996; Gudmundson et al., 2006; McHuron et al., 2020). Interestingly, we found nearly equal capture success for both small and large prey. Based on the schooling behavior of juvenile pollock, which can act as a mechanism to reduce predation risk (Landeau and Terbough, 1986; Magurran, 1990), we expected capture success to be lower for small prey. This was the case for Australian fur seals (Arctocephalus pusillus doriferus), where the highest success rates (up to 100%) occurred when fur seals targeted large, solitary prey in comparison to smaller, schooling baitfish (Meyers et al., 2021). In contrast, Sutton et al. (2021) found that all capture attempts by Macaroni penguins feeding on patches of krill were successful. Given that the majority of the fur seals’ foraging occurred at night, we may be underestimating unsuccessful prey capture attempts due to the inability to visualize prey at a distance. This would be more pronounced for capture attempts on smaller prey. In addition, various head movements, including rapid head strikes that could have been prey capture attempts, were noted throughout the videos, but these could also be associated with other non-feeding behaviors. As a result, our estimates of the differences in capture success by size should be interpreted cautiously.
Foraging occurred predominantly at night for all individuals with little variation (Table 2). Juvenile and adult pollock (to a lesser extent) perform a diel vertical migration, (Smith, 1979; Adams et al., 2009), which could lead to a higher nighttime foraging efficiency as fur seals reduce underwater commuting time (Croxall et al., 1985; Trillmich, 1990; Kooyman et al., 1992). The complete lack of daytime diving by one fur seal (NFSF0218) was likely related to her spending some time foraging off the Bering Sea shelf (Figure 1). Fur seals that feed in the Bering Sea basin are known to have a higher proportion of other prey species in their diet, with squid reaching up to 36% FO (Zeppelin and Ream, 2006). Squid, and other off-shelf prey species including myctophids and smoothtongue, migrate into deeper water during the day which means that fur seals are only able to reach these species at night (Roper and Young, 1975; Zeppelin and Ream, 2006; Sterling et al., 2014). For all fur seals, when foraging did occur during the day, there was a higher proportion of large prey being consumed. We also observed fur seals targeting both large and small fish aggregated among jellyfish found in shallow water (Supplemental Material, Figure S1). A similar foraging tactic was described for thick-billed murres (Uria lomvia) and researchers found the probability of foraging was positively related to fish densities around the jellyfish (Sato et al., 2015). This behavior could also be occurring at night as we observed fur seals move through jellyfish tentacles during capture events, but it was harder to document due to the limited sighting distance.
Prey were captured over a large range of depths and the clear relationship between depth and prey size reflects the age-depth stratification of the fur seal’s primary prey (Traynor, 1986; Bailey, 1989). As adults, pollock are strongly associated with the seafloor, whereas juveniles can be found throughout the water column with higher densities above the thermocline (Smith, 1979; Swartzman et al., 1994; Olla et al., 1996). This pattern is also present in the prey captures as large prey were captured closer to the seafloor than small prey (Figure 4B). This size-based segregation has been suggested as a mechanism for age-0 pollock to reduce cannibalism by adults (Francis and Bailey, 1983). When diving deeper, such as during the day (Kooyman and Goebel, 1986; Goebel et al., 1991; Kuhn, 2011), fur seals may balance the trade-off of increased effort by selectively targeting larger fish (Costa, 1991; Blakeway et al., 2021). Costa, 1991 suggested this shift to consuming larger prey is similar to the central place foraging theory where the surface is the place of return and the increased travel to reach deeper depths favors selection of larger prey items. Gentoo penguins (Pygoscelis papua) showed a similar pattern, switching from foraging on krill in shallow waters to targeting fish during deeper dives (Croxall et al., 1988). One notable exception to our pattern of larger fish being caught at deeper depths could be when fur seals target salmon which spend a significant proportion of their time in shallow water (< 40 m, Supplemental Material, Video S1, Ogura and Ishida, 1995; Walker et al., 2007). Both our video results and diet studies, however, show salmon appear to be rare in the northern fur seal diet (3-15% FO) with prevalence varying annually and among breeding sites (Sinclair et al., 2008).
Larger prey were captured at lower temperatures than small prey but this was expected due to the relationship between depth and temperature in the Bering Sea. In the summer months, a stable thermocline can be found over much of the Bering Sea shelf (Stabeno et al., 2001; Stabeno et al., 2007). In addition, a subsurface ‘‘cold pool’’, which is defined as an isolated water mass less than 2.0° C, can form in the middle region of the shelf (50 – 100 m) due to the presence of winter sea ice (Stabeno et al., 2001; Stabeno et al., 2007; Grüss et al., 2021). These subsurface oceanographic features can play a role in the depth and geographic distribution of pollock (Wyllie-Echeverria and Wooster, 1998; Stabeno et al., 2001). During years with an extensive cold pool, the distribution of adult pollock is compressed and pushed to the outer shelf region as adult pollock tend to avoid the colder waters (Wyllie-Echeverria and Wooster, 1998; Eisner et al., 2020). If fur seals were targeting these larger fish away from the cold pool, then we would expect to see a relationship between prey size and temperature residuals (i.e., larger fish would be found in warmer than expected temperatures based on depth alone). This was not the case, but it’s important to note that both 2018 and 2019 were considered warm years and the cold pool was not found within most of the fur seals foraging range (Ianelli et al., 2019; Eisner et al., 2020). Only in 2017 were prey captured at or near 2°C (Figure 4C) and this was the case for both large and small prey.
The stable thermocline, which sets up in May and begins to break down in fall (Sept-Nov), can also act as a barrier to separate small and larger pollock within the water column (Traynor, 1986; Stabeno et al., 2001; Ladd and Stabeno, 2012). Juvenile pollock, which can be found in a range of temperatures and depths, primarily congregate in the mixed-layer above the thermocline, but become more demersal as they age (Bailey, 1989; Swartzman et al., 1994; Olla et al., 1996). Although we did find that large prey were captured almost exclusively below the mixed-layer depth, we were surprised that smaller prey were also often captured within or below the mixed-layer depth. The lack of small prey captures above the mixed-layer depth may be related to the programming of our cameras, as there was limited video recording from the surface to 10 m. This could, however, also be a result of a predator response behavior by the fish or a specific hunting tactic used by the fur seals. For example, the pollock could be responding to the presence of a predator by moving to deeper depths, below the mixed-layer (Sogard and Olla, 1993; Olla et al., 1996). During laboratory experiments, when an adult pollock was introduced, juvenile pollock responded by diving to the bottom even when a thermocline was present (Sogard and Olla, 1993). A similar response in the natural environment could explain the high proportion of small fish captured at or below the mixed-layer depth. Juvenile pollock have also been shown to form schools that can span over 10 m in depth (Stienessen et al., 2019). As a visual predator, fur seals could be using the backlight from the surface to target fish near the bottom of a school, at its deepest depth, which would result in the capture of juvenile prey deeper in the water column than expected based on average school depths. Capture attempts from below are well documented in other species including penguins, whales, and sharks (Klimley et al., 1992; Watanabe et al., 2004; Sutton et al., 2021). During the daytime when the surface could be identified we regularly observed fur seals targeting prey from below (Supplemental Material, Figure S1, Video S2).
Although most of the fur seals tracked in this study foraged on the Bering Sea shelf, a significant proportion of the population also feeds over deep water in the Bering Sea basin (Kooyman and Goebel, 1986; Goebel et al., 1991). The use of these different habitats has been shown to influence both diet and dive behavior (Zeppelin and Ream, 2006, Kuhn et al., 2014; Jeanniard-du-Dot et al., 2017) and we predict that this would influence prey encounter rates and foraging success as well. The single fur seal in this study that foraged in the Bering Sea basin did have a markedly higher number of prey captures per recorded dive than all of the others (2.2 vs. < 1 successful captures/recorded dive, Table 2). Future northern fur seal foraging studies using video cameras should aim to track more individuals that forage over the basin to investigate these differences. In addition, until further developments lead to more reliable animal-borne video cameras that are able to record for longer durations, future studies could also use the video data as a tool to validate other methods for identifying foraging behavior or prey capture attempts (e.g., Volpov et al., 2016; McGovern et al., 2019; Sutton et al., 2020).
Identifying foraging behavior using data from dive recorders is not new, but studies have found varying success and the metrics used to identify foraging are not consistent. For example, using just depth data, foraging dives by Australian fur seals were identified with 77% accuracy based on descent rate alone (Volpov et al., 2016). By incorporating accelerometers and magnetometers, McGovern et al. (2019) were able to not only identify foraging dives, but also individual prey encounters based on 3D dive patterns with 95% accuracy. The ability to transition away from video recorders, would result in both a reduction in the size and cost of instruments, and potentially make it possible to use instruments that do not need to be recovered (Cox et al., 2017; Heerah et al., 2019; Robinson et al., 2021). This could result in larger sample sizes and the tracking of sex or age-classes that do not predictably return to the rookery. However, this would also result in the loss of the other beneficial data collected from animal-borne videos (e.g., visualizing prey species and determining capture success).
Conclusions
The enhanced understanding of predator-prey relationships provided by animal-borne video cameras will be invaluable to better interpret years of historical northern fur seal biologging data and to provide baseline data for modeling future impacts of variations in prey resources. Previous northern fur seal studies have suggested that more frequent deep or daytime dives was related to increased consumption of adult pollock, but direct evidence wasn’t available (Sinclair and Loughlin, 1994; Sterling, 2009). Sterling (2009) found that variations in fur seal dive depths correlated with changes in pollock age-class recruitment, as fur seals shifted from shallow diving in 1992 to deeper diving in 1995, possibly following the dominant 1992 pollock cohort. McHuron et al. (2020) showed that during years of low pollock recruitment, the increased consumption of larger pollock may be necessary for fur seals to meet their energetic demands. The reduced abundance of age-0 and age-1 pollock in the fur seals foraging area in 2017 (Ianelli et al., 2019; Eisner et al., 2020) could have been a factor driving NFSF0517 to primarily target adult pollock during her foraging trip. Our results provide a direct link between dive behavior and prey consumption and demonstrate that changes in dive depth and the timing of dives can be used as an indicator of shifts in diet between small and large prey for northern fur seals.
As marine environments continue to change, either from climate-related or anthropogenic threats, it becomes crucial to understand relationships between marine predators and their prey. Walleye pollock distribution and recruitment are fundamentally linked to temperature variability in the Bering Sea (Kotwicki et al., 2005; Mueter et al., 2011; Eisner et al., 2020). With climate models predicting future warming in the Bering Sea, the predicted shift in pollock distribution and declines in abundance may have adverse impacts on not only northern fur seal populations, but other marine mammals and seabirds that rely on this prey resource (Springer et al., 1986; Mueter et al., 2011; Spencer et al., 2016; Ianelli et al., 2019; Eisner et al., 2020). Using a range of IPCC climate projections, Mueter et al. (2011) predicted pollock recruitment could decline by 32-58% in the Bering Sea by 2040-2050. Having the ability to collect data to quantify foraging behavior, visualize the prey being consumed, and identify factors affecting foraging success, will help inform models to predict the future impacts of climate-related changes to the northern fur seals’ habitat and prey resources.
Data availability statement
The raw data supporting the conclusions of this article and associated R code used for analysis will be made available on request to the corresponding author, without undue reservation.
Ethics statement
Research was conducted under Marine Mammal Protection Act permit #14327 and approved by the National Marine Fisheries Service IACUC A/NW 2013-3/2016-2.
Author contributions
CK, JS, and CM study conception. CK and CM funding. CK and JS conducted data collection. CK, JS, MM, BB, AS, and AF analyzed data. CK wrote with input from JS, MM, BB, AS, AF, and CM. All authors contributed to the article and approved the submitted version.
Funding
This project was funded by the National Oceanic and Atmospheric Administration’s (NOAA) Alaska Fisheries Science Center, the U.S. Integrated Ocean Observing System through the Animal Tracking Network, the NOAA Office of Oceanic and Atmospheric Research’s Pacific Marine Environmental Laboratory (PMEL), and by the University of Washington’s Cooperative Institute for Climate, Ocean, and Ecosystem Sciences (CIOCES) under NOAA Cooperative Agreement NA20OAR4320271. CATS video cameras were funded by the Innovative Technology for Arctic Exploration (ITAE) program. ITAE is a collaborative research effort by University of Washington (CICOES) and NOAA engineers and scientists at PMEL and is funded by NOAA Research and PMEL.
Acknowledgments
We’d like to thank the dedicated staff of the Alaska Ecosystems Program of the Alaska Fisheries Science Center who assisted with data collection, Amanda Warlick and Victoria Peterson for all their efforts reviewing videos, Katie Luxa and Brian Mason for providing guidance on prey identification, and Brian Brost for his support with data analysis. Finally, we’d like to thank William Walker for sharing his vast knowledge of Bering Sea marine life and his time to assist with prey identification. This is PMEL contribution number 5392 and CICOES contribution number 2022-1213. Reference to trade names does not imply endorsement by the National Marine Fisheries Service, NOAA.
Conflict of interest
The authors declare that the research was conducted in the absence of any commercial or financial relationships that could be construed as a potential conflict of interest.
Publisher’s note
All claims expressed in this article are solely those of the authors and do not necessarily represent those of their affiliated organizations, or those of the publisher, the editors and the reviewers. Any product that may be evaluated in this article, or claim that may be made by its manufacturer, is not guaranteed or endorsed by the publisher.
Supplementary material
The Supplementary Material for this article can be found online at: https://www.frontiersin.org/articles/10.3389/fmars.2022.1015594/full#supplementary-material
References
Acuna E., Lauth R. R. (2008). Results of the 2007 Eastern Bering Sea continental shelf bottom trawl survey of groundfish and invertebrate resources (Seattle, WA: U.S. Dep. Commer., NOAA Tech. Memo.NMFS-AFSC-181), 195 p.
Adams C. F., Foy R. J., Kelley J. J., Coyle K. O. (2009). Seasonal changes in the diel vertical migration of walleye pollock (Theragra chalcogramma) in the northern gulf of Alaska. Environ. Biol. Fish. 86 (2), 297–305. doi: 10.1007/s10641-009-9519-y
Antonelis G. A., Sinclair E. H., Ream R. R., Robson B. W. (1997). Inter-island variation in the diet of female northern fur seals (Callorhinus ursinus) in the Bering Sea. J. Zool. (London) 242, 435–451. doi: 10.1111/j.1469-7998.1997.tb03847.x
Austin D., Bowen W. D., McMillan J. I., Boness D. J. (2006). Stomach temperature telemetry reveals temporal patterns of foraging success in a free-ranging marine mammal. J. Anim. Ecol. 75 (2), 408–420. doi: 10.1111/j.1365-2656.2006.01057.x
Bailey K. M. (1989). Interaction between the vertical distribution of juvenile walleye pollock theragra chalcogramma in the eastern Bering Sea, and cannibalism. Mar. Ecol. Prog. Ser. 53, 205–213. doi: 10.3354/meps053205
Blakeway J.-A., Arnould J. P. Y., Hoskins A. J., Martin-Cabrera P., Sutton G. J., Hückstädt L. A., et al. (2021). Influence of hunting strategy on foraging efficiency in Galapagos sea lions. PeerJ 9, e11206. doi: 10.7717/peerj.11206
Block B. A. (2005). Physiological ecology in the 21st century: Advancements in biologging sciences. Integr. Comp. Biol. 45, 305–320. doi: 10.1093/icb/45.2.305
Boldt J. L., Buckley T. W., Rooper C. N., Aydin K. (2012). Factors influencing cannibalism and abundance of walleye pollock (Theragra chalcogramma) on the eastern Bering Sea shel-2006. Fish. Bulletin U.S. (Washington D.C.) 110, 293–306.
Butler P. J., Bevan R. M., Woakes A. J., Croxall J. P., Boyd I. L. (1995). The use of data loggers to determine the energetics and physiology of aquatic birds and mammals. Braz. J. Med. Biol. Res. 28, 1307–1317.
Call K. A., Ream R. (2012). Prey selection of subadult male northern fur seals (Callorhinus ursinus) and evidence of dietary niche overlap with adult females during the breeding season. Mar. Mammal Sci. 28, 1–15. doi: 10.1111/j.1748-7692.2011.00463.x
Cooke S. J., Hinch S., Lucas M. C., Lutcavage M. (2012). “Biotelemetry and biologing,” in Fisheries techniques 3rd ed. Eds. Zale A., Parrish D., Sutton T. (Bethesda, Maryland: American Fisheries Society).
Costa D. P. (1991). Reproductive and foraging energetics of high-latitude penguins, albatrosses and pinnipeds: implications for life-history patterns. Am. Zool. 31 (1), 111–130. doi: 10.1093/icb/31.1.111
Cox S. L., Orgeret F., Gesta M., C. R., Heizer I., Weimerskirch H., et al. (2017). Processing of acceleration and dive data on-board satellite relay tags to investigate diving and foraging behaviour in free-ranging marine predators. Methods Ecol. Evol. 9, 64–77. doi: 10.1111/2041-210X.12845
Croxall J. P., Davis R. W., O'Connell M. J. (1988). Diving patterns in relation to diet of gentoo and macaroni penguins at south Georgia. Condor 90, 157–167. doi: 10.2307/1368444
Croxall J., Everson G., Kooyman G. L., Ricketts C., Davis R. W. (1985). Fur seal diving behaviour in relation to vertical distribution of krill. J. Anim. Ecol. 54, 1–8. doi: 10.2307/4616
Eisner L. B., Zuenko Y. I., Basyuk E. O., Britt L. L., Duffy-Anderson J. T., Kotwicki S., et al. (2020). Environmental impacts on walleye pollock (Gadus chalcogrammus) distribution across the Bering Sea shelf. Deep Sea Res. Part II: Topical Stud. Oceanogr. 181, 104881. doi: 10.1016/j.dsr2.2020.104881
Evans K., Lea M. A., Patterson T. A. (2013). Recent advances in bio-logging science: Technologies and methods for understanding animal behaviour and physiology and their environments. Deep-Sea Res. Part II: Topical Stud. Oceanogr. 88-89, 1–6. doi: 10.1016/j.dsr2.2012.10.005
Francis R. C., Bailey K. M. (1983). “Factors affecting recruitment of selected gadoids in the northeast pacific and east Bering Sea,” in From year to year. Ed. Wooster W. S. (Seattle, WA: Washington Sea Grant, University of Washington), 35–60.
Freitas C., Lydersen C., Ims R. A., Fedak M. A., Kovacs K. M. (2008). A simple new algorithm to filter marine mammal Argos locations. Mar. Mammal Sci. 24, 315–325. doi: 10.1111/j.1748-7692.2007.00180.x
Friard O., Gamba M. (2016). BORIS: a free, versatile open-source event-logging software for video/audio coding and live observation. Methods Ecol. Evol. 7, 1325–1330. doi: 10.1111/2041-210X.12584
Gentry R. L. (1998). Behavior and ecology of the northern fur seal (Princeton, New Jersey: Princeton University Press).
Goebel M. E., Bengtson J. L., DeLong R. L., Gentry R. L., Loughlin T. R. (1991). Diving patterns and foraging locations of female northern fur seals. Fish. Bulletin U.S. 89 (2), 171–179.
Grüss A., Thorson J. T., Stawitz C. C., Reum J. C., Rohan S. K., Barnes C. L. (2021). Synthesis of interannual variability in spatial demographic processes supports the strong influence of cold-pool extent on eastern Bering Sea walleye pollock (Gadus chalcogrammus). Prog. Oceanogr. 194, 102569. doi: 10.1016/j.pocean.2021.102569
Gudmundson C. J., Zeppelin T. K., Ream R. R. (2006). Application of two methods for determining diet of northern fur seals (Callorhinus ursinus). Fish. Bulletin U.S. 104, 445–455.
Handley J. M., Thiebault A., Stanworth A., Schutt D., Pistorius P. (2018). Behaviourally mediated predation avoidance in penguin prey: in situ evidence from animal-borne camera loggers. R. Soc. Open Sci. 5, 171449. doi: 10.1098/rsos.171449
Heaslip S. G., Bowen W. D., Iverson S. J. (2014). Testing predictions of optimal diving theory using animal-borne video from harbour seals (Phoca vitulina concolor). Can. J. Zool. 92 (4), 309–318. doi: 10.1139/cjz-2013-0137
Heerah K., Cox S. L., Blevin P., Guinet C., Charrassin J.-B. (2019). Validation of dive foraging indices using archived and transmitted acceleration data: the case of the weddell seal. Front. Ecol. Evol. 7, 30. doi: 10.3389/fevo.2019.00030
Hinke J. T., Russell T. M., Hermanson V. R., Brazier L., Walden S. L. (2021). Serendipitous observations from animal−borne video loggers reveal synchronous diving and equivalent simultaneous prey capture rates in chinstrap penguins. Mar. Biol. 168, 135. doi: 10.1007/s00227-021-03937-5
Holton M. D., Wilson R. P., Teilmann J., Siebert U. (2021). Animal tag technology keeps coming of age: an engineering perspective. Philos. Trans. R. Soc. B 376 (1831), 20200229. doi: 10.1098/rstb.2020.0229
Ianelli J., Fissel B., Holsman K., Honkalehto T., Kotwicki S., Monnahan C., et al. (2019)Assessment of the walleye pollock stock in the Eastern Bering Sea. In: Stock assessment and fishery evaluation report for the groundfish resources of the Bering Sea/Aleutian islands region (Anchorage, AK: North Pacific Fishery Management Council). Available at: https://archive.afsc.noaa.gov/refm/docs/2019/EBSPollock.pdf (Accessed 09 December 2020).
Iwata T., Sakamoto K. Q., Takahashi A., Edwards E. W., Staniland I. J., Trathan P. N., et al. (2011). Using a mandible accelerometer to study fine-scale foraging behavior of free-ranging Antarctic fur seals. Mar. Mammal Sci. 28 (2), 345–357. doi: 10.1111/j.1748-7692.2011.00482.x
Jeanniard-du-Dot T., Thomas A. C., Cherel Y., Trites A. W., Guinet C. (2017). Combining hard-part and DNA analyses of scats with biologging and stable isotopes can reveal different diet compositions and feeding strategies within a fur seal population. Mar. Ecol. Prog. Ser. 584, 1–16. doi: 10.3354/meps12381
Johnson D. S., London J. M., Lea M. A., Durban J. W. (2008). Continuous-time correlated random walk model for animal telemetry data. Ecology 89 (5), 1208–1215.
Johnson G. C., Stabeno P. J. (2017). Deep Bering Sea circulation and variability 2001-2016, from argo data. J. Geophys. Res.: Oceans 122, 9765–9779. doi: 10.1002/2017JC013425
Kernaléguen L., Dorville N., Ierodiaconou D., Hoskins A. J., Baylis A. M. M., Hindell M. A., et al. (2016). From video recordings to whisker stable isotopes: a critical evaluation of timescale in assessing individual foraging specialisation in Australian fur seals. Oecologia 180, 657–670. doi: 10.1007/s00442-015-3407-2
Klimley A. P., Anderson S. D., Pyle P., Henderson R. P. (1992). Spatiotemporal patterns of white shark (Carcharodon carcharias) predation at the south farallon islands, California. Copeia 680–690. doi: 10.2307/1446143
Kooyman G. L. (2004). Genesis and evolution of bio-logging devices: 1963-2002. Memoirs Natl. Institute Polar Res. 58, 15–22.
Kooyman G. L., Cherel Y., Le Maho Y., Croxall J. P., Thorson P. H., Ridoux V., et al. (1992). Diving behavior and energetics during foraging cycles in king penguins. Ecol. Monogr. 62, 143–163. doi: 10.2307/2937173
Kooyman G. L., Goebel M. E. (1986). “Feeding and diving behavior of northern fur seals,” in Fur seals: Maternal strategies on land and at Sea. Eds. Gentry R. L., Kooyman G. L. (Princeton, New Jersey: Princeton University Press), 61–78.
Kotwicki S., Buckley T. W., Honkalehto T., Walters G. (2005). Variation in the distribution of walleye pollock (Theragra chalcogramma) with temperature and implications for seasonal migration. Fish. Bulletin U.S. (Washington D.C.) 103, 574–587.
Kuhn C. E. (2011). The influence of subsurface thermal structure on the diving behavior of northern fur seals (Callorhinus ursinus) during the breeding season. Mar. Biol. 158, 649–663. doi: 10.1007/s00227-010-1589-z
Kuhn C. E., Ream R. R., Sterling J. T., Thomason J. R., Towell R. T. (2014). Spatial segregation and the influence of habitat on the foraging behavior of northern fur seals (Callorhinus ursinus). Can. J. Zool. 92, 861–873. doi: 10.1139/cjz-2014-0087
Kuhn C. E., Tremblay Y., Ream R. R., Gelatt T. S. (2010). Coupling GPS tracking with dive behavior to examine the relationship between foraging strategy and fine-scale movements in northern fur seals (Callorhinus ursinus). Endangered Species Res. 12, 125–139. doi: 10.3354/esr00297
Ladd C., Stabeno P. J. (2012). Stratification on the Eastern Bering Sea shelf revisited. Deep Sea Res. Part II: Topical Stud. Oceanogr. 65, 72–83. doi: 10.1016/j.dsr2.2012.02.009
Landeau L., Terbough J. (1986). Oddity and the ‘confusion effect’ in predation. Anim. Behav. 34 (5), 1372–1380. doi: 10.1016/S0003-3472(86)80208-1
Lauth R. R. (2010). Results of the 2009 eastern Bering Sea continental shelf bottom trawl survey of groundfish and invertebrate resources (Seattle, WA: U.S. Dep. Commer., NOAA Tech. Memo. NMFS-AFSC-204), 228 p.
Lauth R. R., Acuna E. (2009). Results of the 2008 eastern Bering Sea continental shelf bottom trawl survey of groundfish and invertebrate resources (Seattle, WA: U.S. Dep. Commer., NOAA Tech. Memo.NMFS-AFSC-195), 229 p.
Lewis S., Sherratt T. N., Hamer K. C., Wanless S. (2001). Evidence of intra-specific competition for food in a pelagic seabird. Nature 412, 816–819. doi: 10.1038/35090566
Liebsch N., Wilson R. P., Bornemann H., Adelung D., Plötz J. (2007). Mouthing off about fish capture: Jaw movement in pinnipeds reveals the real secrets of ingestion. Deep-Sea Res. Part II: Topical Stud. Oceanogr. 54, 256–269. doi: 10.1016/j.dsr2.2006.11.014
Loughlin T. R., Antonelis G. A., Baker J. D., York A. E., Fowler C. W., DeLong R., et al. (1994). “Status of the northern fur seal population in the united states during 1992,” in Fur seal investigations 1992 (Seattle, WA: U.S. Dep. Commer., NOAA Tech. Memo. NMFS-AFSC-45), 9–28.
Magurran A. E. (1990). The adaptive significance of schooling as an anti-predator defence in fish. Annales. Zool. 27 (2), 51–66.
Mattern T., McPherson M. D., Ellenberg U., van Heezik Y., Seddon P. J. (2018). High definition video loggers provide new insights into behaviour, physiology, and the oceanic habitat of a marine predator, the yellow-eyed penguin. PeerJ 6, e5459. doi: 10.7717/peerj.5459
McCarthy A., Honkalehto T., Lauffenburger N., De Robertis A. (2020). Results of the acoustic-trawl survey of walleye pollock (Gadus chalcogrammus) on the U.S. Bering Sea shelf in June - august 2018 (DY1807) (7600 Sand Point Way NE, Seattle WA 98115: Alaska Fish. Sci. Cent., NOAA, Natl. Mar. Fish. Serv.), 83 p.
McGovern K. A., Rodríguez D. H., Lewis M. N., Davis R. W. (2019). Diving classification and behavior of free-ranging female southern elephant seals based on three-dimensional movements and video-recorded observations. Mar. Ecol. Prog. Ser. 620, 215–232. doi: 10.3354/meps12936
McHuron E. A., Luxa K., Pellend N. A., Holsman K., Ream R., Zeppelin T., et al. (2020). Practical application of a bioenergetic model to inform management of a declining fur seal population and their commercially important prey. Front. Mar. Sci. 7, 597973. doi: 10.3389/fmars.2020.597973
Meyers N., Speakman C. N., Dorville N.A.S.-Y., Hindell M. A., Semmens J. M., Monk J., et al. (2021). The cost of a meal: factors influencing prey profitability in Australian fur seals. PeerJ 9, e12608. doi: 10.7717/peerj.12608
Michel L., Cianchetti-Benedetti M., Catoni C., Dell'Omo G. (2022). How shearwaters prey. new insights in foraging behaviour and marine foraging associations using bird-borne video cameras. Mar. Biol. 169, 7. doi: 10.1007/s00227-021-03994-w
Moll R. J., Millspaugh J. J., Beringer J., Sartwell H., He Z. (2007). A new ‘view’ of ecology and conservation through animal-borne video systems. Trends Ecol. Evol. 22 (12), 660–668. doi: 10.1016/j.tree.2007.09.007
Mueter F. J., Bond N. A., Ianelli J. N., Hollowed A. B. (2011). Expected declines in recruitment of walleye pollock (Theragra chalcogramma) in the eastern Bering Sea under future climate change. ICES J. Mar. Sci. 68 (6), 1284–1296. doi: 10.1093/icesjms/fsr022
NMFS (2007). Conservation plan for the Eastern pacific stock of northern fur seal (Callorhinus ursinus) (Juneau, AK: National Marine Fisheries Service, NOAA, Silver Spring, MD).
NMFS (2020). “Fisheries of the united state,” in US. department of commerce, NOAA current fishery statistics no. 2018. (Silver Spring, MD: National Marine Fisheries Service, NOAA). fmars.2022.1015594 Available at: https://www.fisheries.noaa.gov/national/commercial-fishing/fisheries-united-states-2018.
Nordstrom C. A., Battaile B. C., Cotté C., Trites A. W. (2012). Foraging habitats of lactating northern fur seals are structured by thermocline depths and submesoscale fronts in the eastern Bering Sea. Deep-Sea Res. Part II. 88–89, 78–96. doi: 10.1016/j.dsr2.2012.07.010
Ogura M., Ishida Y. (1995). Homing behavior and vertical movements of four species of pacific salmon (Oncorhynchus spp.) in the central Bering Sea. Can. J. Fish. Aquat. Sci. 52 (3), 532–540. doi: 10.1139/f95-054
Oliver P. A., Andrews R., Burkanov V., Davis R. W. (2022). Diving behavior, foraging strategies, and energetics of female steller sea lions during early lactation. J. Exp. Mar. Biol. Ecol. 550, 151707. doi: 10.1016/j.jembe.2022.151707
Olla B. L., Davis M. W. (1990). Behavioral responses of juvenile walleye pollock theragra chalcogramma pallas to light, thermoclines and food: possible role in vertical distribution. J. Exp. Mar. Biol. Ecol. 135, 59–68. doi: 10.1016/0022-0981(90)90198-L
Olla B. L., Davis M. W., Ryer C. H., Sograd S. M. (1996). Behavioural determinants of distribution and survival in early stages of walleye pollock, theragra chalcogrammai a synthesis of experimental studies. Fish. Oceanogr. 5, 167–178. doi: 10.1111/j.1365-2419.1996.tb00090.x
Pettorelli N., Coulson T., Durant S. M., Gaillard J.-M. (2001). Predation, individual variability and vertebrate population dynamics. Oecologia 167, 305–314. doi: 10.1007/s00442-011-2069-y
Pistorius P., Green D. B., Seddon P. J., Thiebault A. (2020). In situ observation of a record−sized squid prey consumed by a gentoo penguin. Polar Biol. 43, 279–283. doi: 10.1007/s00300-020-02630-5
R Core Team (2019). R: A language and environment for statistical computing [Online] (Vienna, Austria: R Foundation for Statistical Computing). Available at: https://www.R-project.org/www.R-project.org.
Robinson S., Battaile B., Hale J., Wilson K. (2021). Classifying Hawaiian monk seal foraging behaviors using metrics based on triaxial accelerometry: pilot data, evaluation, and future research recommendations Vol. H-21-05 (Honolulu, HI: NOAA Admin Rep), 41 p. doi: 10.25923/fadf-cx48
Roden G. I. (1995). Aleutian Basin of the Bering Sea: Thermohaline, oxygen, nutrient, and current structure in July 1993. J. Geophys. Res. 100, 13539–13554. doi: 10.1029/95JC01291
Roemmich D., Johnson G. C., Riser S., Davis R., Gilson J., Owens W. B., et al. (2009). The argo program: Observing the global ocean with profiling floats. Oceanography 22.2, 34–43. doi: 10.5670/oceanog.2009.36
Roper C. F. E., Young R. E. (1975). “Vertical distribution of pelagic cephalopods,” in Smithsonian Contributions to zoology. (City of Washington: Smithsonian Institution Press)
Rutz C., Hays G. C. (2009). New frontiers in biologging science. Biol. Lett. 5, 289–292. doi: 10.1098/rsbl.2009.0089
Sato N. N., Kokubun N., Yamamoto T., Watanabe Y., Kitaysky A. S., Takahashi A. (2015). The jellyfish buffet: jellyfish enhance seabird foraging opportunities by concentrating prey. Biol. Lett. 11 (8), 20150358. doi: 10.1098/rsbl.2015.0358
Sinclair E. H., Antonelis G. A., Robson B. W., Ream R. R., Loughlin T. R. (1996). Northern fur seal, callorhinus ursinus, predation on juvenile walleye pollock, theragra chalcogramma (Seattle, WA: U.S. Dep. Commer. NOAA Tech. Rep. NMFS), 126.
Sinclair E., Loughlin T. (1994). Prey selection by northern fur seals (Callorhinus ursinus) in the eastern Bering Sea. Fish. Bulletin U.S. (Washington D.C.) 92, 144–156.
Sinclair E. H., Vlietstra L. S., Johnson D. S., Zeppelin T. K., Byrd G. V., Springer A. M., et al. (2008). Patterns in prey use among fur seals and seabirds in the pribilof islands. Deep-Sea Res. II 55, 1897–1918. doi: 10.1016/j.dsr2.2008.04.031
Smith G. B. (1979). “The biology of walleye pollock,” in Fisheries oceanography - Eastern Bering Sea shelf, vol. 79-20 . Ed. Favorite F. (Seattle, WA: Northwest and Alaska Fisheries Center Processed Rep.), 213–279.
Sogard S. M., Olla B. L. (1993). Effects of light, thermoclines and predator presence on vertical distribution and behavioral interactions of juvenile walleye pollock, theragra chalcogramma pallas. J. Exp. Mar. Biol. Ecol. 167 (2), 179–195. doi: 10.1016/0022-0981(93)90030-R
Spencer P. D., Holsman K. K., Zador S., Bond N. A., Mueter F. J., A.B. H., et al. (2016). Modelling spatially dependent predation mortality of eastern Bering Sea walleye pollock, and its implications for stock dynamics under future climate scenarios. ICES J. Mar. Sci. 73.5, 1330–1342. doi: 10.1093/icesjms/fsw040
Springer A. M., Roseneau D. G., Lloyd D. S., McRoy C. P., Murphy E. C. (1986). Seabird responses to fluctuating prey availability in the eastern Bering Sea. Mar. Ecol. Prog. Ser. 32, 1–12. doi: 10.3354/meps032001
Stabeno P. J., Bond N. A., Kachel N. B., Salo S. A., Schumacher J. D. (2001). On the temporal variability of the physical environment over the south-eastern Bering Sea. Fish. Oceanogr. 10 (1), 81–98. doi: 10.1046/j.1365-2419.2001.00157.x
Stabeno P. J., Bond N. A., Salo S. A. (2007). On the recent warming of the southeastern Bering Sea shelf. Deep-Sea Res. Part II: Topical Stud. Oceanogr. 54, 2599–2618. doi: 10.1016/j.dsr2.2007.08.023
Sterling J. T. (2009). Northern fur seal foraging behaviors, food webs, and interactions with oceanographic features in the eastern Bering Sea (Seattle WA: University of Washington).
Sterling J. T., Springer A. M., Iverson S. J., Johnson S. P., Pelland N. A., Johns D. J., et al. (2014). The sun, moon, wind, and biological imperative–shaping contrasting wintertime migration and foraging strategies of adult male and female northern fur seals (Callorhinus ursinus). PloS One 9 (4), e93068. doi: 10.1371/journal.pone.0093068
Stienessen S. C., Wilson C. D., Weber T. C., Parrish J. K. (2019). External and internal grouping characteristics of juvenile walleye pollock in the Eastern Bering Sea. Aquat. Living Resour. 32, 19. doi: 10.1051/alr/2019016
Sutton G. J., Bost C. A., Kouzani A. Z., Adams S. D., Mitchell K., Arnould J. P. Y. (2021). Fine-scale foraging effort and efficiency of macaroni penguins is influenced by prey type, patch density and temporal dynamics. Mar. Biol. 168, 3. doi: 10.1007/s00227-020-03811-w
Sutton G., Pichegru L., Botha J. A., Kouzani A. Z., Adams S., Bost C. A., et al. (2020). Multi-predator assemblages, dive type, bathymetry and sex influence foraging success and efficiency in African penguins. Peer J. 8, e9380. doi: 10.7717/peerj.9380
Swartzman G., Stuetzle W., Kulman K., Powojowski M. (1994). Relating the distribution of pollock schools in the Bering Sea to environmental factors. J. Mar. Sci. 51 (4), 481–492. doi: 10.1006/jmsc.1994.1049
Thiebot J.-B., Ito K., Raclot T., Poupart T., Kato A., Ropert-Coudert Y., et al. (2016). On the significance of Antarctic jellyfish as food for adélie penguins, as revealed by video loggers. Mar. Biol. 163, 108. doi: 10.1007/s00227-016-2890-2
Towell R. G., Ream R. R., Sterling J. T., Bengtson J. L., Williams M. (2018). “Population assessment of northern fur seals on the pribilof islands, alask-2016,” in Fur seal investigations 2015-2016. Ed. Testa J. W. (Seattle, WA: U.S. Dep. Commer., NOAA Tech. Memo. NMFS-AFSC-375), 8–25.
Towell R. G., Ream R. R., York A. E. (2006). Decline in northern fur seal (Callorhinus ursinus) pup production on the pribilof islands. Mar. Mammal Sci. 22 (2), 486–491. doi: 10.1111/j.1748-7692.2006.00026.x
Traynor J. J. (1986). “Midwater abundance of walleye pollock in the eastern Bering Se And 1982,” in International north pacific fisheries communication bulletin. (Anchorage, AK, International North Fisheries Commission).
Trillmich F. (1990). The behavioral ecology of maternal effort in fur seals and sea lions. Behavior 114, 3–20. doi: 10.1163/156853990X00022
Volpov B. L., Hoskins A. J., Battaile B. C., Viviant M., Wheatley K. E., Marshall G. J., et al. (2015). Identification of prey captures in Australian fur seals (Arctocephalus pusillus doriferus) using head-mounted accelerometers: field validation with animal-borne video cameras. PloS One 10 (6), e0128789. doi: 10.1371/journal.pone.0128789
Volpov B. L., Rosen D. A. S., Hoskins A. J., Lourie H. J., Dorville N., Baylis A. M. M., et al. (2016). Dive characteristics can predict foraging success in Australian fur seals (Arctocephalus pusillus doriferus) as validated by animal-borne video. Biol. Open 5, 262–271. doi: 10.1242/bio.016659
Walker R. V., Sviridov V. V., Urawa S., Azumaya T. (2007). Spatio-temporal variation in vertical distributions of pacific salmon in the ocean. N. Pac. Anadr. Fish. Commun. Bull. 4, 193–201.
Watanabe Y., Baranov E. A., Sato K., Naito Y., Miyazaki N. (2004). Foraging tactics of baikal seals differ between day and night. Mar. Ecol. Prog. Ser. 279, 283–289. doi: 10.3354/meps279283
Watanabe Y. Y., Takahashi A. (2013). Linking animal-borne video to accelerometers reveals prey capture variability. Proc. Natl. Acad. Sci. 110 (6), 2199–2204. doi: 10.1073/pnas.1216244110
Wyllie-Echeverria T., Wooster W. S. (1998). Year-to-year variations in Bering Sea ice cover and some consequences for fish distributions. Fish. Oceanogr. 72 (2), 159–170. doi: 10.1046/j.1365-2419.1998.00058.x
Yoshino K., Takahashi A., Adachi T., Costa D. P., Robinson P. W., Peterson S. H., et al. (2020). Acceleration-triggered animal-borne videos show a dominance of fish in the diet of female northern elephant seals. J. Exp. Biol. 223, jeb212936. doi: 10.1242/jeb.212936
Keywords: northern fur seal, Callorhinus ursinus, Bering Sea, foraging behavior, video, prey capture, walleye pollock, dive behavior
Citation: Kuhn CE, Sterling JT, McCormley M, Birkemeier B, Sar A, Flock A and Mordy CW (2022) Animal-borne video cameras reveal differences in northern fur seal foraging behavior related to prey size selection. Front. Mar. Sci. 9:1015594. doi: 10.3389/fmars.2022.1015594
Received: 09 August 2022; Accepted: 02 September 2022;
Published: 27 September 2022.
Edited by:
Rob Harcourt, Macquarie University, AustraliaReviewed by:
Randall William Davis, Texas A&M University at Galveston, United StatesBrian Battaile, University of California, Santa Cruz, United States
Copyright © 2022 Kuhn, Sterling, McCormley, Birkemeier, Sar, Flock and Mordy. This is an open-access article distributed under the terms of the Creative Commons Attribution License (CC BY). The use, distribution or reproduction in other forums is permitted, provided the original author(s) and the copyright owner(s) are credited and that the original publication in this journal is cited, in accordance with accepted academic practice. No use, distribution or reproduction is permitted which does not comply with these terms.
*Correspondence: Carey E. Kuhn, Q2FyZXkuS3VobkBub2FhLmdvdg==