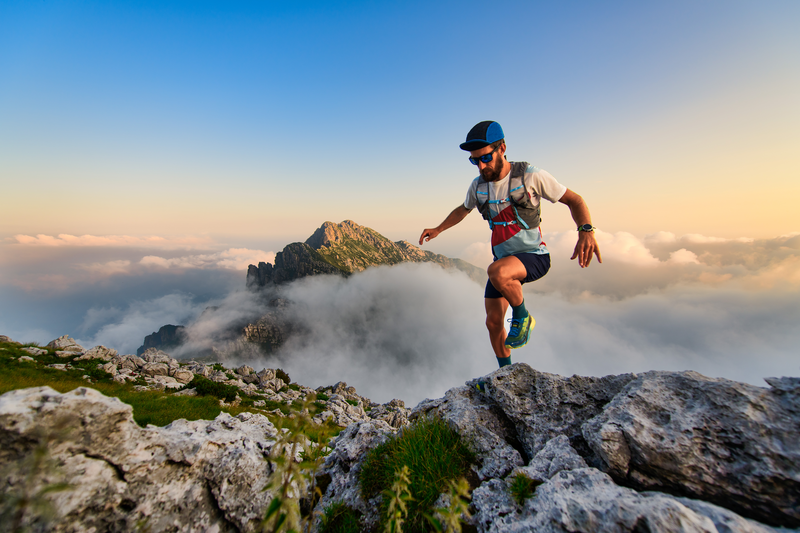
95% of researchers rate our articles as excellent or good
Learn more about the work of our research integrity team to safeguard the quality of each article we publish.
Find out more
MINI REVIEW article
Front. Mar. Sci. , 07 October 2022
Sec. Marine Biotechnology and Bioproducts
Volume 9 - 2022 | https://doi.org/10.3389/fmars.2022.1015419
Photosynthetic organisms such as eukaryotic microalgae and prokaryotic cyanobacteria synthesize a wide range of valuable chemicals. They are predicted to become efficient and renewable sources for valuable products in the future due to their high biomass synthesis using CO2 and solar energy. Microalgae are producers of several carotenoids including lutein, which is a xanthophyll carotenoid with several health advantages, including the prevention of age-related macular degeneration. Currently, it is extracted on commercial scale from marigold flower petals, however, production from plant sources is highly affected by seasonal variations, requires arable land, and has high production cost. Microalgae, on the other hand, are an ideal alternative for lutein synthesis due to their rapid growth and high biomass and lutein yield. It is, however, necessary to further improve lutein productivity, for a successful transition to commercial production. This article describes lutein biosynthesis in microalgae by using their native biochemical pathways, as well as possible target genes for genetic engineering to enhance lutein production. Understanding the processes behind lipid droplet synthesis in chloroplasts, as well as carotenoid transport across chloroplast membranes and carotenoid esterification, might lead to novel ways to boost lutein levels in microalgae.
Carotenoids are a group of terpenoid pigments having a C40 backbone that is beneficial to human health. Over 1100 carotenoids have been identified so far, and are found in a variety of animals, fruits, and green vegetables (Mussagy et al., 2021). Terpenoids are one of the most diverse classes of compounds found in nature, and their biological and pharmacological actions are making them essential for the food and pharmaceutical industries (Ma et al., 2021). Microalgae efficiently synthesize various bio-pigments intracellularly such as β-carotene, lutein, prasinoxanthin, siphonaxanthin, fucoxanthin, zeaxanthin, diadinoxanthin, lycopene, astaxanthin, chlorophyll, phycoerythrin and phycobiliproteins etc. (Begum et al., 2016). Since some carotenoids offer protection from reactive oxygen species and intense light, they are crucial for the survival of microalgae (Praveen et al., 2022). These pigments are synthesized by various microalgal species but the concentration in each microalga is dependent on the particular strain and their cultivation condition e.g. Dunaliella salina preferentially synthesize β-carotene and Hematococcus pluvialis predominantly synthesize astaxanthin (Khoo et al., 2019; Wolf et al., 2021).
Among them, lutein is categorised in the xanthophyll family which is the 2nd most prominent carotenoid having its valuable health benefits including prevention of acute and chronic coronary syndrome, and age-related macular degeneration, as well as assisting in maintaining normal vision (Zheng et al., 2022). Lutein, widely known as the “eye vitamin,” protects the eye tissues from UV and oxidative damage and age-related macular degeneration (AMD) due to having antioxidants properties (Roberts et al., 2009). Oxidation or clouding of natural lens responsible of focusing of light on retina is major cause of cataracts. Lutein is one of the most significant carotenoids present in human milk responsible for infant visual and cognitive development (Fitzpatrick and Dhawan, 2014; Eggersdorfer and Wyss, 2018). It takes almost 66 to 77% of total carotenoids responsible for brain development in the infant (Vishwanathan et al., 2014).
Commercial extraction of lutein is carried out from marigold flower petals and its concentration varies from 17 to 570 mg/100g between the different species (Piccaglia et al., 1998). Lutein is found in its esterified form in the marigold flower, where half of its weight consists of fatty acids that need to be removed by saponification to obtain pure lutein (Zhao et al., 2021). However, commercial production of lutein from plant sources is hindered by several factors such as the limitation of production in certain periods of the year that the plant can be harvested and tedious petal separation (Lin et al., 2015). Other sources such as corn, leafy green vegetables and egg yolk have also drawbacks, owing to the low lutein concentration and bioavailability (Zhao et al., 2021). Those challenges can hinder the viable commercial production of lutein, making photosynthetic eukaryotic and prokaryotic microorganisms a promising alternative source. Under optimized cultivation conditions, microalgae can accumulate significant amounts of biomass and lutein (Ren et al., 2021). Lutein is an antenna pigment in the light-harvesting complex (LHC) of microalgal photosynthetic apparatus that can prevent oxidative damage of cells by non-photochemical quenching under high light intensity (Fernández-Sevilla et al., 2010).
Microalgae display a variety of metabolic pathways resulting in the accumulation of various metabolites. The role of these metabolites in carbon partitioning in microalgae having variable cell compositions depending on various cultivation modes (autotrophic, heterotrophic and mixotrophic) including light intensity, pH of the medium, dissolved O2 and nutrient-limited conditions (Vuppaladadiyam et al., 2018). Primary metabolites are produced when microalgae are cultivated in sufficient nutrient and light conditions, while adverse conditions, including the nutrient deficit, high light intensity, and salt stress are critical elements which activate defence systems in microalga cells that lead to the accumulation of secondary metabolites (Vuppaladadiyam et al., 2018). Algae-derived lutein is in free, non-esterified form, and has long been thought to be a good alternative to traditional plant sources. Harvesting costs, extraction of crude lutein, purification and bioavailability analyses have all been studied concerning microalgae to replace marigold plants in the production of lutein. Many microalgal genera, such as Scenedesmus, Chlorella, Coccomyxa, Parachlorella, and Tetraselmis, have been studied for lutein synthesis thus far (Xie et al., 2021). In this review article, we provide an overview of recent developments in the production of lutein from microalgae by employing high yield strains and genetic modification techniques to boost the lutein content.
Several genera of microalgae have been studied for lutein production in the literature. The production yield varies based on the selected strains, the medium composition used for their growth and other cultivation conditions. As a result, optimizing cultivation conditions has been intensively studied with the objective of boosting growth performance and high lutein productivity. Microalgae can be cultivated under different mode of nutrition such as photoautotrophic, heterotrophic or mixotrophic for lutein production. Pigments are usually synthesized under photoautotrophic growth, but the cell density is usually low under these conditions. On the other hand, heterotrophic growth results in high biomass formation but with lower amount of pigments. The low growth rate in photoautotrophic conditions and low pigment concentration in heterotrophic conditions can be resolved by using mixotrophic cultivation. For example, C. sorokiniana MB-1-M12 synthesized lutein up to 50.6 mg/L under mixotrophic cultivation, by using 6 g/L of sodium acetate as organic carbon with replacement of medium under semi-continuous mode (Chen et al., 2019). In addition to this, other researchers used two-stage cultivation strategies to enhance lutein content. For example, Chlorella sorokiniana FZU60 was initially cultivated under mixotrophic conditions in fed-batch mode and then shifted to photoautotrophic mode for enhanced lutein production (Xie et al., 2020). Similarly, Scenedesmus incrassatulus was firstly cultivated under heterotrophic mode by using glucose as a carbon source for high cell density, and thereafter the shifted to a photobioreactor for lutein production (Flórez-Miranda et al., 2017).
Chlorella sp. is the most studied genus for lutein production. The cold-tolerant microalga Chlorella vulgaris UTEX 265 was used in a coiled tubular tree photobioreactor where light intensity and period and temperature were optimized toward high lutein productivity. Under optimal conditions of lighting for 14 h per day with 25 μmol photons m−2 s−1 and growth at 22 °C, it synthesized 9.82 mg/g of lutein (Gong and Bassi, 2017). Chen et al. (2016) investigated the extraction of lutein from Chlorella sorokiniana MB-1 under semi-batch mixotrophic conditions, by studying various factors such as biomass pretreatment, extraction pressure, temperature and duration, and solvent type. They found that by using a French press for biomass pretreatment and tetrahydrofuran (THF) as solvent resulted as the extraction solvent, nearly 100% of lutein recovery was achieved in 40 minutes at 850 mbar and 25°C (Chen et al., 2016). In another study C. sorokiniana MB-1 was cultivated under a semi-batch-integrated two-stage cultivation mode with 80% of medium replacement, achieving the lutein productivity of 7.62 mg/L/d (Chen and Liu, 2018). Chen et al. (2021) cultivated C. sorokiniana AK-1 on untreated piggery wastewater where it synthesized 4.56 mg/g of lutein, while efficiently removing COD, BOD, TN and TP content from the wastewater (Chen et al., 2021). In another study, 0-200 mM sodium bicarbonate was used as an inorganic carbon source to cultivate Chlorella pyrenoidosa (Sampathkumar and Gothandam, 2019). When 100 mM of sodium bicarbonate was used, under photoautotrophic conditions with a light intensity of 42 μmol m−2 s−1 (16:8 light/dark cycles) at 27 °C, lutein content reached 4.84 mg/g (Sampathkumar and Gothandam, 2019). Asker and Awad (2019) isolated the novel marine strain Auxenochlorella sp. LEU27A, which is capable of accumulating up to 996.6 ± 98 μg/g of lutein under heterotrophic cultivation on marine broth 2216 (contains per L: yeast extract, 8 g; peptone, 5 g) (Asker and Awad, 2019). Lutein synthesis in C. vulgaris was optimized with the low light intensity of 160 μmolm−2 s−1 and the addition of nitrate in 5 L photobioreactor, resulting in 10.4 ± 5.5 mg/g which was more than double compared to the medium without nitrate addition (4.3 ± 2.9 mg/g) (McClure et al., 2019). The lutein concentration was further enhanced to up to 15 - 20 mg/L when the cultivation was performed in 50 L bubble column photobioreactor under semi-continuous mode (McClure et al., 2019).
Scenedesmus incrassatulus CLHE-S01 was cultivated in a fed-batch airlift photobioreactor under photoautotrophic conditions with three different nitrogen concentrations, synthesizing 1.88 ± 0.08 mgL−1 day−1 of lutein under high nitrogen concentration (García-Cañedo et al., 2016). In another study S. incrassatulus CLHE-Si01 was cultivated under a sequential two-stage, where initially it was cultivated heterotrophically in stirred tank bioreactor with glucose, followed by transfer to an airlift photobioreactor for light induction of lutein, which accumulates up to 1.49 ± 0.03 mg/g when supplemented with urea + vitamins (Flórez-Miranda et al., 2017). Scenedesmus sp. CAS-173 was cultivated under the mixotrophic conditions in an airlift photobioreactor using glycerol obtained from a biodiesel production unit for lutein production, resulting in maximum productivity of lutein of 3.59 mg/L/d with 6 g/L of crude glycerol (Rajendran et al., 2020). In another study, light intensity was optimized for lutein production from Scenedesmus obliquus FSP-3, showing maximum lutein productivity of 4.08 mg/L/d when cultivated under a light intensity of 300 μmol m−2 s−1 by using a TL5 fluorescent lamp (Ho et al., 2014).
Chlamydomonas reinhardtii is considered a model microalga for carotenoid production with fast growth rate but limited biomass production. Light intensity is a major factor for lutein synthesis in this microalga. For example, when C. reinhardtii was cultivated under constant lighting of 149 μmol m−2 s−1 intensity for 10 days, it produced 0.87 mg/g lutein (El-Mekkawi et al., 2019). In another study, Chlamydomonas acidophila was illuminated with PAR light of 240 μmol m−2 s−1 and synthesized 20.2 ± 0.5 mg/L of lutein while increment of light intensity to 1000 μmol m−2 s−1 did not enhance lutein production (Garbayo et al., 2008). On the other hand, lutein content was enhanced under UV-A radiation or heated at 40 °C (Garbayo et al., 2008). The cultivation temperature was optimized for lutein production in Chlamydomonas sp. JSC4, achieved a maximum lutein productivity of 3.27 mg/L/d at 35°C while the maximum lutein content was achieved at 20 °C and reached 3.82 mg/g (Ma et al., 2020).
Carotenoids have a C40 methyl branched hydrophobic backbone with conjugated double bonds, which is necessary for pigmentation and visible wavelength photon absorption (Velmurugan and Kodiveri Muthukaliannan, 2022). Based on their distribution in the intracellular compartments and their role in cellular metabolisms, they can be divided into carotenes and xanthophylls, which can be primary or secondary. Primary carotenoids are mainly structural and functional photosystem metabolites and are synthesized in chloroplasts, whereas secondary carotenoids are synthesized in cytoplasmic lipid vesicles, and become more abundant in response to stress (Huang et al., 2017). Lutein is confined to several taxa of microalgae, but it is mainly found in Chlorophyta classes.
Elucidation of carotenoids production in bacteria, algae, protist (Patel et al., 2022) and higher plants has progressed significantly, and it is expected that primary carotenoids biosynthesis in these species follows a similar process. Figure 1 depicts the lutein synthesis route in green algae (Chlorophyta), which is subdivided into the different stages (Huang et al., 2021; Kato et al., 2021; Lou et al., 2021; Xie et al., 2021; Song et al., 2022; Velmurugan and Kodiveri Muthukaliannan, 2022). The major metabolic pathway includes the synthesis of isopentenyl diphosphate (IPP), geranylgeranyl pyrophosphate, phytoene, and desaturation of phytoene into lycopene, followed by cyclisation and hydroxylation of lycopene to lutein.
Figure 1 Biosynthetic pathway of lutein in microalgae; source references (Huang et al., 2021; Kato et al., 2021; Lou et al., 2021; Xie et al., 2021; Song et al., 2022; Velmurugan and Kodiveri Muthukaliannan, 2022).
Lutein synthesis starts with the formation of 1-deoxy-D-xylulose-5-phosphate (DXP) from glyceraldehyde-3-phosphate (GAP) and pyruvate. MEP (2-C-methyl-d-erythritol 4-phosphate) pathway plays a major role to convert DXP into IIP through a series of reactions which is found in plants, microalgae, cyanobacteria, and a few bacterial species (Rohmer, 1999). Geranyl pyrophosphate (GPP) is synthesized by the condensation of IIP and its isomer dimethylallyl diphosphate (DMAPP) and is considered as the first intermediate precursor in the lutein biosynthetic route. GPP is converted into farnesyl pyrophosphate (FPP) through the condensation with IIP, followed by another IIP unit addition which is catalyzed by the GGPP synthase and results in the synthesis of Geranylgeranyl pyrophosphate (GGPP) (Fraser et al., 2000). Then a rate-limiting membrane-bound, phytoene synthase (PSY) enzyme catalyzes the condensation of two GGPP molecules to form Phytoene (Shaker et al., 2021). PSY is highly conserved in prokaryotes and eukaryotes. Phytoene is subsequently converted into lycopene by two distinct desaturases, namely phytoene desaturase (PDS) and zeta-carotene desaturase (ZDS), through various steps, in which PDS initially converts phytoene to phytofluene and thereafter to ζ-carotene, followed by its conversion to neurosporene by ζ-carotene desaturase (ZDS). PDS is membrane-bound oxidoreductase enzyme that resides in plastids where it performs photosynthesis and carotenoid synthesis (Narang et al., 2022). Finally, neurosporene is converted to lycopene by ζ-carotene desaturase (ZDS), ζ-carotene isomerase (Z-iso), and carotenoid isomerase (CRTISO) (Cunningham and Gantt, 1998; Liang et al., 2019). This process differs in cyanobacteria, where a single phytoene desaturase (CrtI) enzyme catalyses the phytoene into lycopene (Molina-Márquez et al., 2019). Lycopene is usually the bright color carotenoid found in vegetables such as tomatoes. In photosynthetic microorganisms, lycopene is further converted into carotene by cyclization of one or both lycopene ends which is a crucial branching point for carotenoids synthesis. Finally, α-carotene is formed by β-cyclase (LCY-b) and ϵ-cyclase (LCY-e) that is responsible for β and ϵ rings formation at both ends of lycopene and is mainly found in plants and microalgae (Molina-Márquez et al., 2019). On the other hand, β-carotene is formed by the action of LCY-b alone, which catalyzed the synthesis of two β-rings at both ends of lycopene. Xanthophylls are formed by the oxidation of α- and β-carotene in higher plants and microalgae. Lutein is formed by hydroxylation of α- carotene at C-3 and C-3′ positions by the action of β- ring and ϵ-ring carotene hydroxylase encoded by CrtR-b and CrtR-e, respectively (Quinlan et al., 2012).
Certain chemical inhibitors such as imidazole, pyridine, triethylamine, piperidine, nicotinic acid and nicotine, modify the carotenoids pathway to form specific products in microalgae. Yildirim et al., 2017 suggested that lutein biosynthesis in microalga can be enhanced by adding native biosynthetic pathways inhibitors. In this regard, they used 2-methylimidazole (2MI) during the cultivation of Dunaliella salina to inhibit the cyclization reactions in the biosynthesis pathway carotenoids (Yildirim et al., 2017). The addition of 2MI at a concentration of 1 mM enhanced the lutein content by 1.7-folds with a simultaneous reduction in β-carotene content, hence proving that 2MI affects the activity of lycopene β-cyclase (LCY-b) that is responsible for α-carotene synthesis (Yildirim et al., 2017). They suggested that inhibitor 2 MI-1 mM might be more effective on lycopene β-cyclase than lycopene ϵ-cyclase, and it directs the pathway to the α-carotene branch followed by lutein synthesis (Yildirim et al., 2017). Similarly, DCMU [3–(3′,4′-dichlophenyl)-1,1-dimethylurea] was used as an inhibitor of lycopene β-cyclase in the culture of Dunaliella bardawil strain V-101 that enhanced the lutein content by 2 fold (Mysore Doddaiah et al., 2013). They suggested that reducing β-carotene content in DCMU-treated cells was due to inhibition of lycopene β-cyclase resulting in enhancing lutein content through lycopene ϵ-cyclase (CrtR-e) and β-and ϵ-carotene hydroxylase activities (Mysore Doddaiah et al., 2013). In a study it has been showed that the overexpression of lycopene ϵ-cyclase (LCY-e) gene in C. reinhardtii resulted into enhancing the conversion of lycopene to α-carotene and finally the lutein concentration (Tokunaga et al., 2021). Likewise, lutein content in C. sorokiniana was improved by random mutagenesis with N-methyl-N′-nitro-nitrosoguanidine (MNNG), resulting in a 2-fold increase in the concentration of lutein compared (42 mg/L) to the wild strain (Cordero et al., 2011b).
Some examples of genetically modified microalgal strains for overproduction of lutein are listed in Table 1. In a study, the lutein content in C. reinhardtii was boosted by improving the biomass production after heterologous expression of carbonic anhydrase from Mesorhizobium loti (MlCA) and Sulfurihydrogenibium yellowstonense (SyCA) which increased CO2 capture (Lin et al., 2022). This microalga synthesized maximum lutein of 21.32 mg/L (a 4-fold increase from the wild type strain) under the mixotrophic condition in a photobioreactor by using 5% CO2 (Lin et al., 2022). When this microalga was genetically modified through heterologous expression of phytoene synthase gene (CzPSY) from Chlorella zofingiensis, it synthesized 2.2 folds higher lutein content than the one observed in untransformed cells (Cordero et al., 2011a). When another phytoene synthase gene isolated from D. salina was heterologously expressed, lutein content increased by 2.6 folds compared to the wild type cells (Couso et al., 2011). In the lutein synthesis pathway, lycopene epsilon-cyclase (LCY-e) is an important enzyme for the conversion of lycopene into δ-carotene, α-carotene and lutein. In this regard, CvLCYE gene from C. vulgaris was heterologously expressed in C. reinhardtii where it showed an enhanced lutein content by 2.3 folds compared to the wild type (Lou et al., 2021). Tokunaga et al. (2021) overexpressed the same LCY-e gene endogenously in C. reinhardtii resulting in an enhanced lutein production without affecting the total cell yields (Tokunaga et al., 2021). Rathod et al. (2020) further improved the lutein production in C. reinhardtii by heterologous expression of phytoene-β-carotene synthase gene (CrtYB) from the yeast Xanthophyllomyces dendrorhous (Rathod et al., 2020). This gene has a dual functional role in phytoene synthase (psy) and lycopene cyclization (Lcyb) in the carotenoid synthetic pathway (Rathod et al., 2020). Heterologous expression of this gene in C. reinhardtii boosted lutein content up to 8.9 mg/g which was 60% higher than the wild type under low light intensities of 75 μmol photons m−2 s−1 (Rathod et al., 2020). Recently, Song et al. (2022), used CRISPR-Cas9 RNP mediated knock out strategies to delete the zeaxanthin epoxidase (ZEP) gene along with the ADP−glucose pyrophosphorylase (AGP) gene in the C. reinhardtii that is responsible for xanthophyll and starch biosynthesis in this microalga (Song et al., 2022). This double knock-out mutant enabled to accumulate 2.93 mg/g of lutein under optimal cultivation conditions (Song et al., 2022).
Baek et al. (2018) suggested that genetically modified C. reinhardtii CC-4349 through knocking out the zeaxanthin epoxidase gene by preassembled DNA-free CRISPR-Cas9 ribonucleoproteins, showed a 56-fold increment in zeaxanthin content without affecting the lutein content significantly, which increased from 2.71 ± 0.04 mg/g to 3.08 ± 0.07 (Baek et al., 2018).
The majority of lutein that is now available on the market is produced from marigold petals. Microbial fermentation employing engineered cell factories is an appealing new production path to fulfil the expanding market demand, which is estimated to reach 360 million USD in 2022 (Lutein Market - Global Forecast to 2022, 2018). The majority of microalgal studies are limited to certain metabolites with the most notable to be lipids, however, other secondary metabolites are explored in a few microalgae strains. Among several genera, Chlorella is widely employed for lutein production using various fermentation strategies while C. reinhardtii is extensively explored for genetic modifications through traditional engineering, due to its GRAS status (generally recognized as safe). As a result of the advancement of efficient gene-editing tools, target specific gene editing has grown increasingly popular in recent years. In order to increase lutein content and productivity in microalgal strains, genetic engineering approaches are utilized to change the rate-limiting stage of the metabolic pathway (Saini et al., 2020). Even though the area of microalgal genetic engineering is still in its infancy, progress has been made in recent years utilizing this technique to boost the lutein content of microalgae. Our knowledge of the regulation of lutein metabolism in microalgal species has expanded with the introduction of novel molecular techniques like CRISPR/Cas9 genome editing and genome sequencing. (Baek et al., 2018; Patel et al., 2019).
It is very difficult to produce lutein in non-photosynthetic microorganisms through heterologous expression due to challenges involved in lycopene asymmetric cyclization. However, some studies have succeeded in the heterologous biosynthesis in non-photosynthetic microorganisms such as Escherichia coli (Takemura et al., 2021) and Saccharomyces cerevisiae (Bian et al., 2021). Integration of several genes including isopentenyl diphosphate isomerase (IDI), lycopene ϵ-cyclase (LCY-e), lycopene β-cyclase (LCY-b) and cytochrome P450 97C (CYP97C) in E. coli leads to the synthesis of 11 mg/L of lutein (Takemura et al., 2021). In the other approach, combinatorial engineering in S. cerevisiae provides lutein content up to 438 μg/gCDW (Bian et al., 2021). The strategy used for the efficient heterologous lutein biosynthesis in yeast revealed that temporospatial pathway control may be used to solve intra-pathway competitions in both photosynthetic and non-photosynthetic strains as well, and it could also be used to promote the biosynthesis of other natural products.
In conclusion, lutein production from microalgae has been carried out significantly at the lab scale and is slowly approaching towards commercialization of microalgal-based lutein. However, the level of lutein in microalgal candidates is still very low in comparison with other carotenoids such as β-carotene (up to 14%) and astaxanthin (up to 5%) in Dunaliella spp. (Pourkarimi et al., 2020) and Haematococcus spp., respectively (Shah et al., 2016). It is already discussed by Xie et al. (2021), that lutein sequestration is a critical issue for its high content production in the intracellular compartment in microalgae. Lutein is synthesized in the inner membrane of the chloroplast that accumulates over there and can’t float in a hydrophilic environment of chloroplast due to lipophilic unsaturated polyene chain and the hydrophobic hydroxyl group of rings. This results in feedback inhibition of lutein synthesis or degradation by reactive oxygen species (ROS) or carotenoid cleaveage dioxygenases (CCDs) if appropriate sequestration is not achieved. As a result, lutein sequestration is required for lutein accumulation, and lutein storage capacity may influence the maximum lutein level in microorganisms (Xie et al., 2021).
Other challenges of lutein production from microalgae are its costly and time-consuming downstream processing that requires harvesting of microalgal biomass, extraction and purification of lutein. Due to the low cell density of large-scale microalgal cultures, energy-intensive harvesting and dewatering techniques are needed. To produce lutein of optimum quality, the microalgal slurry must be concentrated at least ten times before being processed for lutein extraction. Harvesting of lutein-rich microalgal biomass is usually carried out by several physical and chemical methods. Physical methods include gravimetric sedimentation, centrifugation, and filtration of biomass whereas chemical methods involve floatation and flocculation. Some effective techniques such as centrifugation can be costly, time-consuming or there is a chance of lutein degradation. Extraction is usually carried out after the proper disruption of biomass by physical, non-mechanical or enzymatic methods, which can be energy intensive due to the hard cell wall of microalgae that require significant pressures in order to rupture. Therefore, the implementation of microalgal lutein production depends on the advance of technology for processing microalgal biomass that is affordable, energy-efficient, and eco-friendly.
AP drafted the manuscript. UR, LM and PC, conceived the study, and discussed the content of the manuscript. All authors contributed to the article and approved the submitted version.
Authors would like to thank the Swedish Research Council (FORMAS) Sweden for supporting this work as part of the projects ‘Green and sustainable approach to valorise high saline and oily fish processing effluents for the production of nutraceuticals’ (INVENTION; 2020-01028).
The authors declare that the research was conducted in the absence of any commercial or financial relationships that could be construed as a potential conflict of interest.
All claims expressed in this article are solely those of the authors and do not necessarily represent those of their affiliated organizations, or those of the publisher, the editors and the reviewers. Any product that may be evaluated in this article, or claim that may be made by its manufacturer, is not guaranteed or endorsed by the publisher.
Asker D., Awad T. S. (2019). Isolation and characterization of a novel lutein-producing marine microalga using high throughput screening. Food Res. Int. 116, 660–667. doi: 10.1016/j.foodres.2018.08.093
Baek K., Yu J., Jeong J., Sim S. J., Bae S., Jin E. S. (2018). Photoautotrophic production of macular pigment in a chlamydomonas reinhardtii strain generated by using DNA-free CRISPR-Cas9 RNP-mediated mutagenesis. Biotechnol. Bioeng. 115, 719–728. doi: 10.1002/bit.26499
Begum H., Yusoff F. M., Banerjee S., Khatoon H., Shariff M. (2016). Availability and utilization of pigments from microalgae. Crit. Rev. Food Sci. Nutr. 56, 2209–2222. doi: 10.1080/10408398.2013.764841
Bian Q., Zhou P., Yao Z., Li M., Yu H., Ye L. (2021). Heterologous biosynthesis of lutein in s. cerevisiae enabled by temporospatial pathway control. Metab. Eng. 67, 19–28. doi: 10.1016/j.ymben.2021.05.008
Chen J. H., Chen C. Y., Hasunuma T., Kondo A., Chang C. H., Ng I. S., et al. (2019). Enhancing lutein production with mixotrophic cultivation of chlorella sorokiniana MB-1-M12 using different bioprocess operation strategies. Bioresour. Technol. 278, 17–25. doi: 10.1016/j.biortech.2019.01.041
Chen C. Y., Jesisca, Hsieh C., Lee D. J., Chang C. H., Chang J. S. (2016). Production, extraction and stabilization of lutein from microalga chlorella sorokiniana MB-1. Bioresour. Technol. 200, 500–505. doi: 10.1016/j.biortech.2015.10.071
Chen C. Y., Kuo E. W., Nagarajan D., Dong C. D., Lee D. J., Varjani S., et al. (2021). Semi-batch cultivation of chlorella sorokiniana AK-1 with dual carriers for the effective treatment of full strength piggery wastewater treatment. Bioresour. Technol. 326, 124773. doi: 10.1016/j.biortech.2021.124773
Chen C. Y., Liu C. C. (2018). Optimization of lutein production with a two-stage mixotrophic cultivation system with chlorella sorokiniana MB-1. Bioresour. Technol. 262, 74–79. doi: 10.1016/j.biortech.2018.04.024
Cordero B. F., Couso I., León R., Rodríguez H., Vargas M. Á. (2011a). Enhancement of carotenoids biosynthesis in chlamydomonas reinhardtii by nuclear transformation using a phytoene synthase gene isolated from chlorella zofingiensis. Appl. Microbiol. Biotechnol. 91, 341–351. doi: 10.1007/s00253-011-3262-y
Cordero B. F., Obraztsova I., Couso I., Leon R., Vargas M. A., Rodriguez H. (2011b). Enhancement of lutein production in chlorella sorokiniana (chorophyta) by improvement of culture conditions and random mutagenesis. Mar. Drugs 9, 1607–1624. doi: 10.3390/md9091607
Couso I., Vila M., Rodriguez H., Vargas M. A., León R. (2011). Overexpression of an exogenous phytoene synthase gene in the unicellular alga chlamydomonas reinhardtii leads to an increase in the content of carotenoids. Biotechnol. Prog. 27, 54–60. doi: 10.1002/btpr.527
Cunningham F. X., Gantt E. (1998). Genes and enzymes of carotenoid biosynthesis in plants. Annu. Rev. Plant Biol. 49, 557–583. doi: 10.1146/annurev.arplant.49.1.557
Eggersdorfer M., Wyss A. (2018). Carotenoids in human nutrition and health. Arch. Biochem. Biophys. 652, 18–26. doi: 10.1016/j.abb.2018.06.001
El-Mekkawi S. A., Hussein H. S., El-Enin S. A. A., El-Ibiari N. N. (2019). Assessment of stress conditions for carotenoids accumulation in chlamydomonas reinhardtii as added-value algal products. Bull. Natl. Res. Cent. 43:130. doi: 10.1186/s42269-019-0169-8
Fernández-Sevilla J. M., Acién Fernández F. G., Molina Grima E. (2010). Biotechnological production of lutein and its applications. Appl. Microbiol. Biotechnol. 86, 27–40. doi: 10.1007/s00253-009-2420-y
Fitzpatrick E., Dhawan A. (2014). Scanning the scars: The utility of transient elastography in young children. J. Pediatr. Gastroenterol. Nutr. 59, 551. doi: 10.1097/MPG.0000000000000522
Flórez-Miranda L., Cañizares-Villanueva R. O., Melchy-Antonio O., Martínez-Jerónimo F., Flores-Ortíz C. M. (2017). Two stage heterotrophy/photoinduction culture of scenedesmus incrassatulus: potential for lutein production. J. Biotechnol. 262, 67–74. doi: 10.1016/j.jbiotec.2017.09.002
Fraser P. D., Schuch W., Bramley P. M. (2000). Phytoene synthase from tomato (Lycopersicon esculentum) chloroplasts - partial purification and biochemical properties. Planta 211, 361–369. doi: 10.1007/s004250000293
Garbayo I., Cuaresma M., Vílchez C., Vega J. M. (2008). Effect of abiotic stress on the production of lutein and β-carotene by chlamydomonas acidophila. Process Biochem. 43, 1158–1161. doi: 10.1016/j.procbio.2008.06.012
García-Cañedo J. C., Cristiani-Urbina E., Flores-Ortiz C. M., Ponce-Noyola T., Esparza-García F., Cañizares-Villanueva R. O. (2016). Batch and fed-batch culture of scenedesmus incrassatulus: Effect over biomass, carotenoid profile and concentration, photosynthetic efficiency and non-photochemical quenching. Algal Res. 13, 41–52. doi: 10.1016/j.algal.2015.11.013
Gong M., Bassi A. (2017). Investigation of chlorella vulgaris UTEX 265 cultivation under light and low temperature stressed conditions for lutein production in flasks and the coiled tree photo-bioreactor (CTPBR). Appl. Biochem. Biotechnol. 183, 652–671. doi: 10.1007/s12010-017-2537-x
Guardini Z., Dall’osto L., Barera S., Jaberi M., Cazzaniga S., Vitulo N., et al. (2021). High carotenoid mutants of chlorella vulgaris show enhanced biomass yield under high irradiance. Plants 10:911. doi: 10.3390/plants10050911
Ho S. H., Chan M. C., Liu C. C., Chen C. Y., Lee W. L., Lee D. J., et al. (2014). Enhancing lutein productivity of an indigenous microalga scenedesmus obliquus FSP-3 using light-related strategies. Bioresour. Technol. 152, 275–282. doi: 10.1016/j.biortech.2013.11.031
Huang W., Lin Y., He M., Gong Y., Huang J. (2018). Induced high-yield production of zeaxanthin, lutein, and β-carotene by a mutant of chlorella zofingiensis. J. Agric. Food Chem. 66, 891–897. doi: 10.1021/acs.jafc.7b05400
Huang J. J., Lin S., Xu W., Cheung P. C. K. (2017). Occurrence and biosynthesis of carotenoids in phytoplankton. Biotechnol. Adv. 35, 597–618. doi: 10.1016/j.biotechadv.2017.05.001
Huang P. W., Wang L. R., Geng S. S., Ye C., Sun X. M., Huang H. (2021). Strategies for enhancing terpenoids accumulation in microalgae. Appl. Microbiol. Biotechnol. 105, 4919–4930. doi: 10.1007/s00253-021-11368-x
Kato Y., Oyama T., Inokuma K., Vavricka C. J., Matsuda M., Hidese R., et al. (2021). Enhancing carbohydrate repartitioning into lipid and carotenoid by disruption of microalgae starch debranching enzyme. Commun. Biol. 4:450. doi: 10.1038/s42003-021-01976-8
Khoo K. S., Lee S. Y., Ooi C. W., Fu X., Miao X., Ling T. C., et al. (2019). Recent advances in biorefinery of astaxanthin from haematococcus pluvialis. Bioresour. Technol. 288, 121606. doi: 10.1016/j.biortech.2019.121606
Liang M. H., Liang Z. C., Chen H. H., Jiang J. G. (2019). The bifunctional identification of both lycopene β- and ϵ-cyclases from the lutein-rich dunaliella bardawil. Enzyme Microb. Technol. 131, 109426. doi: 10.1016/j.enzmictec.2019.109426
Lin J., Lee D., Chang J. (2015). Bioresource technology lutein production from biomass : Marigold flowers versus microalgae. Bioresour. Technol. 184, 421–428. doi: 10.1016/j.biortech.2014.09.099
Lin J. Y., Sri Wahyu Effendi S., Ng I. S. (2022). Enhanced carbon capture and utilization (CCU) using heterologous carbonic anhydrase in chlamydomonas reinhardtii for lutein and lipid production. Bioresour. Technol. 351, 127009. doi: 10.1016/j.biortech.2022.127009
Lou S., Lin X., Liu C., Anwar M., Li H., Hu Z. (2021). Molecular cloning and functional characterization of CvLCYE, a key enzyme in lutein synthesis pathway in chlorella vulgaris. Algal Res. 55, 102246. doi: 10.1016/j.algal.2021.102246
Lutein Market - Global Forecast to 2022 (2018) (Globenewswire). Available at: https://www.globenewswire.com/news-release/2018/06/01/1515530/0/en/Lutein-Market-Global-Forecast-to-2022.html (Accessed April 24, 2022).
Ma C., Zhang K., Zhang X., Liu G., Zhu T., Che Q., et al. (2021). Heterologous expression and metabolic engineering tools for improving terpenoids production. Curr. Opin. Biotechnol. 69, 281–289. doi: 10.1016/j.copbio.2021.02.008
Ma R., Zhao X., Ho S. H., Shi X., Liu L., Xie Y., et al. (2020). Co-Production of lutein and fatty acid in microalga chlamydomonas sp. JSC4 in response to different temperatures with gene expression profiles. Algal Res. 47, 101821. doi: 10.1016/j.algal.2020.101821
McClure D. D., Nightingale J. K., Luiz A., Black S., Zhu J., Kavanagh J. M. (2019). Pilot-scale production of lutein using chlorella vulgaris. Algal Res. 44, 101707. doi: 10.1016/j.algal.2019.101707
Molina-Márquez A., Vila M., Vigara J., Borrero A., León R. (2019). The bacterial phytoene desaturase-encoding gene (CRTI) is an efficient selectable marker for the genetic transformation of eukaryotic microalgae. Metabolites 9:49. doi: 10.3390/metabo9030049
Mussagy C. U., Khan S., Kot A. M. (2021). Current developments on the application of microbial carotenoids as an alternative to synthetic pigments. Crit. Rev. Food Sci. Nutr. 0, 1–15. doi: 10.1080/10408398.2021.1908222
Mysore Doddaiah K., Narayan A., Gokare Aswathanarayana R., Ravi S. (2013). Effect of metabolic inhibitors on growth and carotenoid production in dunaliella bardawil. J. Food Sci. Technol. 50, 1130–1136. doi: 10.1007/s13197-011-0429-6
Narang P. K., Dey J., Mahapatra S. R., Roy R., Kushwaha G. S., Misra N., et al. (2022). Genome-based identification and comparative analysis of enzymes for carotenoid biosynthesis in microalgae. World J. Microbiol. Biotechnol. 38, 1–22. doi: 10.1007/s11274-021-03188-y
Patel A., Bettiga M., Rova U., Christakopoulos P., Matsakas L. (2022). Microbial genetic engineering approach to replace shark livering for squalene. Trends Biotechnol. 40, 1261–1273. doi: 10.1016/j.tibtech.2022.03.008
Patel V. K., Soni N., Prasad V., Sapre A., Dasgupta S., Bhadra B. (2019). CRISPR–Cas9 system for genome engineering of photosynthetic microalgae. Mol. Biotechnol. 61, 541–561. doi: 10.1007/s12033-019-00185-3
Piccaglia R., Marotti M., Grandi S. (1998). Lutein and lutein ester content in different types of tagetes patula and t. erecta. Ind. Crops Prod. 8, 45–51. doi: 10.1016/S0926-6690(97)10005-X
Pourkarimi S., Hallajisani A., Nouralishahi A., Alizadehdakhel A., Golzary A. (2020). Factors affecting production of beta-carotene from dunaliella salina microalgae. Biocatal. Agric. Biotechnol. 29, 101771. doi: 10.1016/j.bcab.2020.101771
Praveen A., Kumar V., Gautam P., Kurbatova A. (2022). Microalgal Carotenoids : Biosynthesis and green extraction. Octa J. Biosci. 10, 6–12. Available at: http://sciencebeingjournal.com/search.html?keys=microalgal+carotenoids%3A+biosynthesis+and+green+extraction
Quinlan R. F., Shumskaya M., Bradbury L. M. T., Beltrán J., Ma C., Kennelly E. J., et al. (2012). Synergistic interactions between carotene ring hydroxylases drive lutein formation in plant carotenoid biosynthesis. Plant Physiol. 160, 204–214. doi: 10.1104/pp.112.198556
Rajendran L., Nagarajan N. G., Karuppan M. (2020). Enhanced biomass and lutein production by mixotrophic cultivation of scenedesmus sp. using crude glycerol in an airlift photobioreactor. Biochem. Eng. J. 161:107684. doi: 10.1016/j.bej.2020.107684
Rathod J. P., Vira C., Lali A. M., Prakash G. (2020). Metabolic engineering of chlamydomonas reinhardtii for enhanced β-carotene and lutein production. Appl. Biochem. Biotechnol. 190, 1457–1469. doi: 10.1007/s12010-019-03194-9
Ren Y., Sun H., Deng J., Huang J., Chen F. (2021). Carotenoid production from microalgae: Biosynthesis, salinity responses and novel biotechnologies. Mar. Drugs 19:713. doi: 10.3390/md19120713
Roberts R. L., Green J., Lewis B. (2009). Lutein and zeaxanthin in eye and skin health. Clin. Dermatol. 27, 195–201. doi: 10.1016/j.clindermatol.2008.01.011
Rohmer M. (1999). The discovery of a mevalonate-independent pathway for isoprenoid biosynthesis in bacteria, algae and higher plants. Nat. Prod. Rep. 16, 565–574. doi: 10.1039/a709175c
Saini D. K., Chakdar H., Pabbi S., Shukla P. (2020). Enhancing production of microalgal biopigments through metabolic and genetic engineering. Crit. Rev. Food Sci. Nutr. 60, 391–405. doi: 10.1080/10408398.2018.1533518
Sampathkumar S. J., Gothandam K. M. (2019). Sodium bicarbonate augmentation enhances lutein biosynthesis in green microalgae chlorella pyrenoidosa. Biocatal. Agric. Biotechnol. 22, 101406. doi: 10.1016/j.bcab.2019.101406
Shah M. M. R., Liang Y., Cheng J. J., Daroch M. (2016). Astaxanthin-producing green microalga haematococcus pluvialis: From single cell to high value commercial products. Front. Plant Sci. 7. doi: 10.3389/fpls.2016.00531
Shaker S., Morowvat M. H., Ghasemi Y. (2021). Bioinformatics analysis and identification of phytoene synthase gene in microalgae. Recent Pat. Biotechnol. 15, 216–226. doi: 10.2174/1872208315666210712121951
Song I., Kim S., Kim J., Oh H., Jang J., Jeong S. J., et al. (2022). Macular pigment-enriched oil production from genome-edited microalgae. Microb. Cell Fact. 21, 1–12. doi: 10.1186/s12934-021-01736-7
Steinbrenner J., Sandmann G. (2006). Transformation of the green alga haematococcus pluvialis with a phytoene desaturase for accelerated astaxanthin biosynthesis. Appl. Environ. Microbiol. 72, 7477–7484. doi: 10.1128/AEM.01461-06
Takemura M., Kubo A., Watanabe A., Sakuno H., Minobe Y., Sahara T., et al. (2021). Pathway engineering for high-yield production of lutein in escherichia coli. Synth. Biol. 6, 1–9. doi: 10.1093/synbio/ysab012
Tokunaga S., Morimoto D., Koyama T., Kubo Y., Shiroi M., Ohara K., et al. (2021). Enhanced lutein production in chlamydomonas reinhardtii by overexpression of the lycopene epsilon cyclase gene. Appl. Biochem. Biotechnol. 193, 1967–1978. doi: 10.1007/s12010-021-03524-w
Velmurugan A., Kodiveri Muthukaliannan G. (2022). Genetic manipulation for carotenoid production in microalgae an overview. Curr. Res. Biotechnol. 4, 221–228. doi: 10.1016/j.crbiot.2022.03.005
Vishwanathan R., Kuchan M. J., Sen S., Johnson E. J. (2014). Lutein and preterm infants with decreased concentrations of brain carotenoids. J. Pediatr. Gastroenterol. Nutr. 59, 659–665. doi: 10.1097/MPG.0000000000000389
Vuppaladadiyam A. K., Prinsen P., Raheem A., Luque R., Zhao M. (2018). Microalgae cultivation and metabolites production: a comprehensive review. Biofuels Bioprod. Biorefining 12, 304–324. doi: 10.1002/bbb.1864
Wolf L., Cummings T., Müller K., Reppke M., Volkmar M., Weuster-Botz D. (2021). Production of β-carotene with dunaliella salina CCAP19/18 at physically simulated outdoor conditions. Eng. Life Sci. 21, 115–125. doi: 10.1002/elsc.202000044
Xie Y., Li J., Ho S. H., Ma R., Shi X., Liu L., et al. (2020). Pilot-scale cultivation of chlorella sorokiniana FZU60 with a mixotrophy/photoautotrophy two-stage strategy for efficient lutein production. Bioresour. Technol. 314, 123767. doi: 10.1016/j.biortech.2020.123767
Xie Y., Xiong X., Chen S. (2021). Challenges and potential in increasing lutein content in microalgae. Microorganisms 9:1068. doi: 10.3390/microorganisms9051068
Yildirim A., Akgün İ. H., Conk Dalay M. (2017). Converted carotenoid production in dunaliella salina by using cyclization inhibitors 2-methylimidazole and 3-amino-1,2,4-triazole. Turkish J. Biol. 41, 213–219. doi: 10.3906/biy-1606-10
Zhao Z., Ci F., Pang H., Yu Q., Dong R., Xing A. (2021). Advances in research on natural distribution, biosynthesis, detection, bioactivity, and application of lutein. ACS Agric. Sci. Technol. 2, 258−269. doi: 10.1021/acsagscitech.1c00284
Keywords: microalgae, carotenoids, lutein, genetic engineering, fermentation
Citation: Patel A, Rova U, Christakopoulos P and Matsakas L (2022) Microalgal lutein biosynthesis: Recent trends and challenges to enhance the lutein content in microalgal cell factories. Front. Mar. Sci. 9:1015419. doi: 10.3389/fmars.2022.1015419
Received: 02 September 2022; Accepted: 27 September 2022;
Published: 07 October 2022.
Edited by:
Neha Arora, University of South Florida, United StatesReviewed by:
Ambarish Nag, National Renewable Energy Laboratory (DOE), United StatesCopyright © 2022 Patel, Rova, Christakopoulos and Matsakas. This is an open-access article distributed under the terms of the Creative Commons Attribution License (CC BY). The use, distribution or reproduction in other forums is permitted, provided the original author(s) and the copyright owner(s) are credited and that the original publication in this journal is cited, in accordance with accepted academic practice. No use, distribution or reproduction is permitted which does not comply with these terms.
*Correspondence: Alok Patel, YWxvay5rdW1hci5wYXRlbEBsdHUuc2U=
Disclaimer: All claims expressed in this article are solely those of the authors and do not necessarily represent those of their affiliated organizations, or those of the publisher, the editors and the reviewers. Any product that may be evaluated in this article or claim that may be made by its manufacturer is not guaranteed or endorsed by the publisher.
Research integrity at Frontiers
Learn more about the work of our research integrity team to safeguard the quality of each article we publish.