- 1Guangxi Key Laboratory of Beibu Gulf Marine Resources, Environment and Sustainable Development, Fourth Institute of Oceanography, Ministry of Natural Resources, Beihai, China
- 2Department of Environmental Biology and Fisheries Science, National Taiwan Ocean University, Keelung, Taiwan
- 3Department of Marine Science, Faculty of Mathematics and Natural Science, Sriwijaya University, Indralaya, Indonesia
- 4College of Ocean and Earth Sciences, Xiamen University, Xiamen, China
- 5Key Laboratory of Tropical Marine Ecosystem and Bioresource, Fourth Institute of Oceanography, Ministry of Natural Resources, Beihai, China
- 6Department of Biological Technology, Xiamen Ocean Vocational College, Xiamen, China
Microplastics and heavy metals are the two main contaminants that are often found in aquatic environments and can lead to major issues for aquatic organisms. Polystyrene (PS) is a type of microplastic that is commonly found in aquatic environments. Hard clams are one of the organisms that are often used as a bioindicator of water pollution, and they can live in a certain salinity range. The objective of this study is to investigate the potential of PS particles as heavy metal vectors in M. lusoria influenced by differences in salinity. The result showed that the highest increase in concentrations of all heavy metals in hard clams was found in those placed at higher salinities. Hard clams that were placed at higher salinity required more water, allowing more PS particles to enter the clams’ bodies. Hard clams placed at salinity 30‰ always gathered significantly more PS particles (p<0.05) than hard clams placed at the other two salinities (20 and 25‰). This is also corroborated by water depletion at a salinity of 30‰, which is significantly higher (p<0.05) than the other two salinities. Our findings indicate that PS particles have the potential as vectors for heavy metal pollutants in hard clams in environments of varying salinity.
1 Introduction
Microplastics of the polystyrene (PS) kind are frequently observed in aquatic environments (Andrady, 2017; Gambardella et al., 2017). Vinyl benzene, a styrene plastic monomer derived from petroleum and used to make PS, is renowned for its low weight and insulating properties. In the last two decades, the number of PS applications has continued to increase. According to Lithner et al. (2011), 32.7 million tonnes of PS plastic were manufactured worldwide in 2011. This is one of the factors contributing to the highest level of plastic pollution in the ocean (above 60%) (Hahladakis, 2020; Galloway and Lewis, 2016). PS particles that accumulate can cause serious threats to aquatic organisms because they contain carcinogenic substances, namely styrene monomer (Wright et al., 2013).
One of the causes of the ocean’s pollution, which makes up 8% of all pollutants, is heavy metals (Hahladakis, 2020). Heavy metals are difficult to eliminate, so it is easy to accumulate in the environment and their existence is naturally difficult to degrade. Heavy metals are absorbed by microplastics in both seawater (Brennecke et al., 2016; Gao et al., 2019) and freshwater (Holmes et al., 2014). Heavy metals and MPs can interact in two different ways: first, heavy metals dissolved in water can be absorbed by MPs, and second, heavy metals that have been absorbed on the surface of MPs can be released back into the water and pollute it. Our earlier research demonstrated that the two phases of interaction might be influenced by the salinity level of the water (Barus et al., 2021). These interactions can cause harmful effects to aquatic organisms. MPs particles that have absorbed heavy metals will be eaten by organisms and cause heavy metal contamination as well. The concentration of heavy metals in the bodies of organisms contained in MPs was higher than in organisms that were not found in microplastics (Vedolin et al., 2018; Mohsen et al., 2019). Besides, heavy metals that have been absorbed by MP particles can be released back into the water and cause the water to become polluted by heavy metals (Barus et al., 2021), which has an impact on the organisms that live in these waters.
Hard clams (M. lusoria) are the favored species of edible clam and one of the most economically important fisheries in Asia (Huber, 2010; Ju et al., 2020). M. lusoria is a sizeable component of the infaunal bivalve mollusk community that lives on the seafloor of fine sand, silt, or sandy silt and forms extensive and dense layers (Chen et al., 2007; Satheeswaran et al., 2019; Ju et al., 2020). These organisms are used as bioindicators of water quality because their lives settle in sediments (Satheeswaran et al., 2019). Hard clams are filter feeders that employ chiffon to draw in water, filter organic materials, and sift water and debris from one chiffon (Chien and Hsu, 2006). Hard clams are osmoconformers, meaning that the osmolality of their bodily fluids varies in the same direction as changes in the salinity of their surroundings. To deal with either hypo- or hyperosmotic stress, marine mollusks display a variety of osmoregulatory mechanisms (Lin et al., 2016). Heavy metals in hard clams can be transferred to humans through the consumption of these clams, causing harmful effects to human health and causing public health problems.
Studies have documented the buildup of heavy metals and microplastics in hard clams as well as the negative consequences. However, very few studies have examined the role of microplastics as carriers of heavy metals into the hard clam bodies, and as far as we are aware, no research has been done in various salinity levels. The purpose of this study was to investigate the effect of salinity on heavy metal contamination in hard clam using polystyrene particles as a carrier.
2 Materials and methods
2.1 Materials
The virgin PS particles with diameters of 50 and 250 µm used in this study were purchased from Tersulan Chemical Co., Ltd. (Guangdong, China). Lead (Pb), zinc (Zn), copper (Cu), and cadmium (Cd), four heavy metals used in this investigation, were bought from Merck (Darmstadt, Germany). The heavy metal stock solution was prepared at 1000 mg L-1 using 1 liter of distilled water. The stock solution was diluted to 1, 2, 5, and 10 mg L-1. These heavy metal solutions with different concentrations were used for heavy metal adsorption on PS particles before exposure to biota.
2.2 Experimental animals
Adult hard clams (M. lusoria) with an average weight of 19.62 ± 0.04 g was bought from a local market (Keelung Fish Market, Taiwan). Hard clams were maintained at 1000 L of seawater with a salinity of 30‰ for 5 days. The seawater is pumped and filtered from the coast offshore by the National Taiwan Ocean University. After this time, some of the hard clams were maintained and adapted to salinities of 25 and 20‰. Salinities between 20 and 30‰ are thought to be hard clams’ ideal physiological range (Malouf and Bricelj, 1989). The reduction in salinity was carried out by 1‰ per day to ensure that the clam could adapt. This group division aims to perform tests in environments with different salinities. Organisms were kept and acclimated at a constant temperature (22 ± 0.5°C) and with continuous aeration. During maintenance, M. lusoria was fed with green algae. After acclimatization and prior to PS particle exposure, the meat of the five hard clams was sampled to verify background contamination by heavy metals.
2.3 Experimental design
Before conducting the experiment, analysis of the heavy metal content of virnin particles was also carried out. The results show that all particles are metal free (undetectable). One gram of PS particles was introduced to a beaker glass along with 100 mL of a heavy metal solution, which was then agitated for 24 hours at 180 rpm using a magnetic stirrer. The concentrations of PS particles of size 50 and 150 µm with a weight of 1 g were 3 x 105 and 4 x 104 particles L-1, respectively. Because the equilibrium point for the absorption of heavy metals into PS particles had been reached within that time frame, the duration of exposure to PS particles and heavy metals was chosen (Barus et al., 2021). Tests were carried out using different concentrations of heavy metals (1, 2, 5, and 10 mg L-1). After 24 hours, filter the particles using filter paper of 0.45 µm. The clams M. lusoria that have been maintained and adapted to different salinities are put into a glass bath containing 1 L of seawater with a different salinity (20, 25, and 30‰) and then added PS particles that have been filtered from a heavy metal solution. Each glass tube contains 10 hard clams and is supported by continuous aeration to keep the clams alive and also to ensure that the particles in the tubes keep stirring. M. lusoria were collected after a contact duration of 6, 12, 18, and 24 hours, respectively. Each test was repeated 3 times.
The amount of heavy metal absorbed in PS particles of various sizes (50 and 250 µm) following interaction with heavy metals at various concentrations for 24 hours was also tested. Three times were run through the test. After 24 hours, the particles were filtered using 0.45 µm filter paper. Each sample’s particles underwent sonication extraction (120 W, 10 min.) in nitric acid at a 2% concentration. A flame atomic adsorption spectrophotometer was used to measure the concentrations of heavy metals in the extraction solution (SpeactAA 240-FS, VARIAN, Palo Alto, CA, USA).
Five hard clams M. lusoria that have been in prolonged contact with the solution are taken, then the meat is taken and frozen at -20°C. The samples were freeze-dried (FD-20A2D, H.C.S., Taipei, Taiwan) at-60 °C. After 3 days, concentrated nitric acid (70%) was added to the samples, and they were digested in a microwave-accelerated reactor (MARS Xpress, CEM, Matthews, NC, USA). A flame atomic absorption spectrophotometer was used to measure the levels of heavy metals in clam bodies.
We examined the residual concentrations in PS particles and in seawater at each observation interval to determine the contribution of heavy metals adsorbed in PS particles on the rise in heavy metals in hard clams. After 24 hours, the particles were filtered using filter paper with a 0.45 µm particle size. Each sample’s particles underwent sonication extraction (120 W, 10 min.) in nitric acid at a 2% concentration. A flame atomic adsorption spectrophotometer (SpeactAA 240-FS, VARIAN, Palo Alto, CA, USA) was used to measure the concentrations of heavy metals in the extraction solution. We also measured whether there was a change in water volume before and after exposure (water depletion) to determine the effect of salinity through the osmoregulation process.
2.4 The number of PS particles in M. lusoria
After the contact period, three hard clams were removed, and the flesh and clams were divided. 30% H2O2 was used to digest the flesh in accordance with the methods outlined by Galgani et al. (2013) and Li et al. (2015). Samples were treated with 250 mL of 30% H2O2 for 48 hours at 65 0C in an oscillation incubator running at 80 rpm. In order to keep the PS particles floating while the tissues settled to the bottom of each digestion bottle, 800 mL of filtered sodium chloride solution was added once the digestion process was complete. This concentrated saline solution (NaCl: 120 g L-1) was created. The solution was then filtered with a Millipore vacuum pump onto Whatman® GF/C filters (0.45 µm pore size) to recover floating PS particles. In glass Petri plates with covers, each filter was put before being counted after it had dried at room temperature. The 40x stereo microscope was used to analyze the filtered microplastic particles. The primary characteristics used to count and distinguish PS particles from other particles were size, color, form, and surface roughness.
2.5 Data analysis
One- and two-way ANOVA were used to analyze all of the data in this study. Using Duncan’s multiple range test, the significant differences between the treatments were determined. We tested for significant differences between the increasing heavy metal in the clam body (%) with respect to different water salinities. At the 0.05 level, statistical significance was recognized for all tests. The linear relationships among increasing heavy metal in the clam body with heavy metal concentration, salinity, and the number of accumulated PS particles were tested using a general linear model.
3 Results and discussion
3.1 PS particle adsorption capacity
The amount of heavy metal adsorbed on the PS particles was measured after a 24-hour period of contact. The results revealed that the adsorption amount varied depending on particle size. PS particles with a larger size absorb more heavy metals. Figure 1 shows that in all the initial heavy metal concentrations, PS particles of size 250 µm absorb more heavy metals than PS particles of size 50 µm. These results are in line with previous studies (Holmes et al., 2014; Fred-Ahmadu et al., 2019; Barus et al., 2021). Larger-diameter particles have a larger surface area, which serves as an adsorption site for heavy metals.
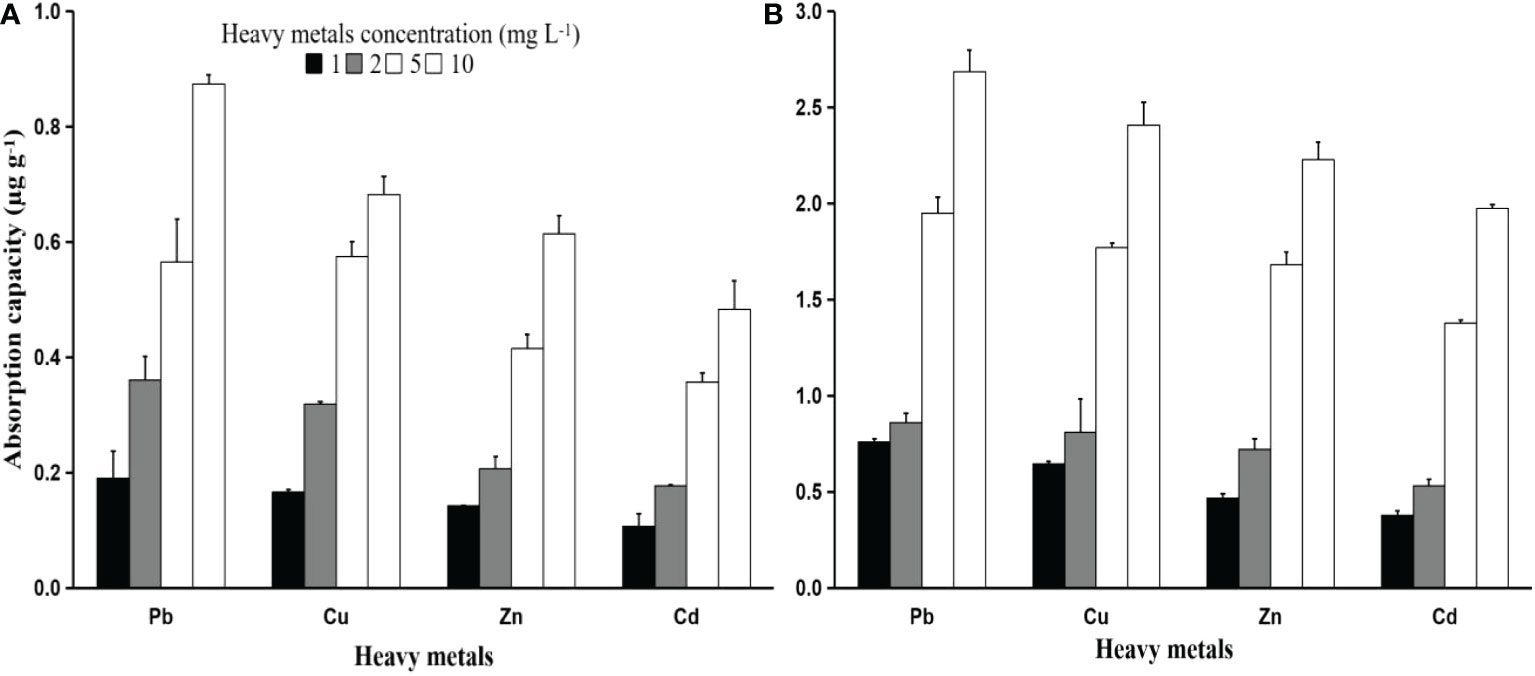
Figure 1 Heavy metals (Pb, Zn, Cu, and Cd) absorption capacity (µg g-1) of PS of different sizes 50 µm (A) and 250 µm (B) after 24 hours of exposure to different heavy metal concentrations (1, 2, 5, and 10 mg L-1).
According to Figure 1, Pb has the highest rate of heavy metal absorption among PS particles, with Cu, Zn, and Cd having the lowest rates. These results were found for both PS particle sizes. Previous studies reported similar results (Turner and Holmes, 2015; Gao et al., 2019). Gao et al. (2019) reported that the heavy metal Pb was absorbed the highest in microplastic particles compared to other heavy metals, both in fresh water and sea water. This indicates that Pb has a higher potential to be a pollutant combined with MPs because Pb has a greater affinity for particle size and type compared to Zn, Cu, and Cd. Factors such as ionic radii, hydrolysis value, and softness cause differences in absorption rates for each type of heavy metal (Covelo et al., 2011; Hu et al., 2017). The absorption rate of heavy metals is inversely proportional to the ionic radius of the heavy metal. The smaller the ionic radius of a heavy metal, the greater the potential for adsorption (Covelo et al., 2011; Saha et al., 2002). The radii of the hydrated ions of Pb2+, Cu2+, and Cd2+ were 0.401, 0.419, and 0.426 nm, respectively. The relationship between the hydrated ionic radius and electrostatic interactions influences the degree to which metals adsorb on MPs. In comparison to other heavy metals, the Pb2+ ion has the lowest hydrated ionic radius and hence, the strongest electrostatic contact with MP.
The concentrations of heavy metals Pb, Cu, Zn, and Cd that were absorbed by PS particles of size 50 µm after 24 hours of contact duration with a heavy metal 1 mg L-1 were 0.257 ± 0.012, 0.236 ± 0.004, 0.165 ± 0.006, and 0.135 ± 0.006 µg g-1, respectively. Meanwhile, the adsorption concentrations of 250 µm PS particles were 0.767 ± 0.021, 0.648 ± 0.008, 0.468 ± 0.007, and 0.375 ± 0.009 µg g-1, respectively (Figure 1). The absorption of heavy metals in PS particles increases with the increase in the concentration of heavy metals in the solution. The highest adsorption capacity of PS particles was found in contact with heavy metals with a concentration of 10 mg L-1, followed by 5, 2, and 1 mg L-1. These results were found for both PS particle sizes (Figure 1). According to Hodson et al. (2017), the adsorption capacities reached 236-7171 mg g-1 at relatively high starting concentrations of Zn (0.1-100 mg L-1), which was significantly more than what was seen at low beginning concentrations (Holmes et al., 2012; Turner and Holmes, 2015).
3.2 Heavy metal concentration in M. lusoria before exposure
Prior to contact with PS particles that had absorbed heavy metals, the value of heavy metals in hard clam meat was evaluated. The results show that the hard clam contains heavy metals with relatively low values. The heavy metal concentrations of Pb, Zn, Cu, and Cd in the hard clam body were 0.033 0.005, 8.572 0.892, 0.117 0.011, and 0.049 0.008 g g-1, respectively. This indicates that the hard clam (M. lusoria) has been contaminated by heavy metals from the ocean. Previous studies have shown that Cd, Cr, Cu, Ni, Zn, and Pb metals can accumulate in hard clams (Satheeswaran et al., 2019). Hard clams are filter feeders that live on the bottom of the water, which use chiffon to attract water filtering organic matter (Chien and Hsu, 2006) and accumulate pollutants in their bodies (Satheeswaran et al., 2019).
3.3 Heavy metal concentration in M. lusoria after exposure
The concentration of heavy metals in the hard clam body has increased following contact with PS particles that have absorbed heavy metals. Larger particles (250 µm) increased the quantity of heavy metals in hard clam bodies in contact with PS more than small particles (50 µm) did (Figures 2 and 3). This was because the particles with a larger size have a higher capacity to absorb heavy metals based on Figure 1, so when these particles were eaten by clams, the heavy metals that were carried into the clams became higher. Additionally, heavy metals in the water become higher when they are released into the water (Figure 4). Extreme increases started to appear after the first six hours of observation. Additionally, the rise in heavy metals starts at a modest level and didn’t change much until the observation’s end (24 hours). This was most likely caused by the hard clams eating PS particles at the beginning up to 6 hours later, which still absorbed high levels of heavy metals before dispersing into the water. The concentration of heavy metals remaining in PS particles decreased dramatically at 6 hours of exposure and continued until the end of the observation, while the concentration in seawater increased even though in very low concentrations. This was found in tests using both PS particle sizes (Figures 4 and 5). This shows that the role of PS particles as heavy metal vectors in hard clams can occur through two pathways. First, PS particles can absorb heavy metals (Brennecke et al., 2016; Gao et al., 2019; Galloway et al., 2017) and enter the clams, causing the clams to be contaminated with heavy metals. Second, the heavy metals that have been adsorbed on the PS particles can be released back into the solution and cause the solution where the clam lives to be polluted by heavy metals (Costa, 2017; Barus et al., 2021).
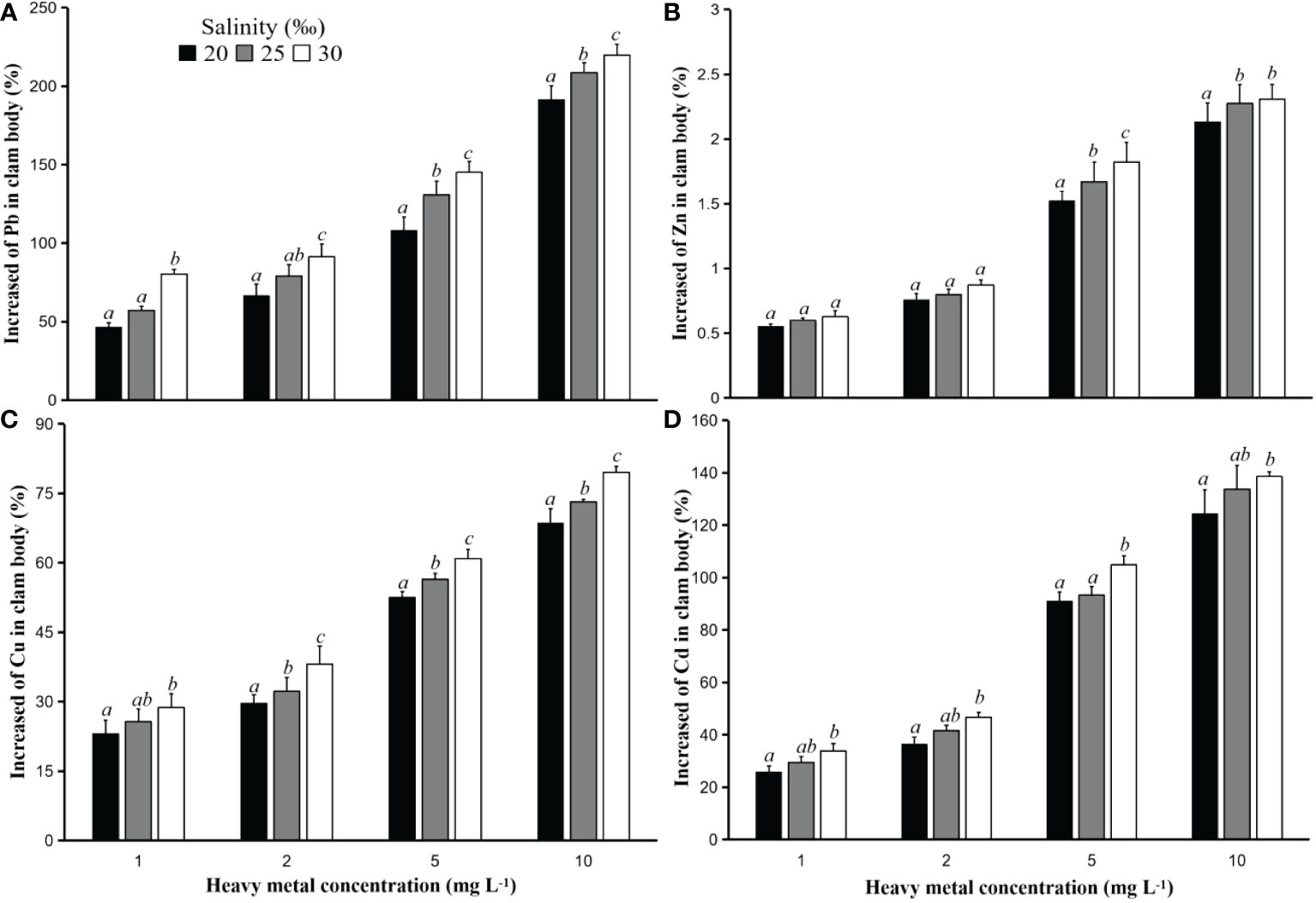
Figure 2 Increased heavy metal concentrations (%) Pb (A), Zn (B), Cu (C), and Cd (D) in the hard clam (M. lusoria) body after 24 hours of exposure to PS particles with a size of 50 µm that had contact with heavy metals at various concentrations (1, 2, 5, and 10 mg L-1) and salinities (20, 25, and 30‰). The data for increased heavy metal concentrations in clam body with different letters (a, b, and c) differed significantly (p<0.05) between salinities.
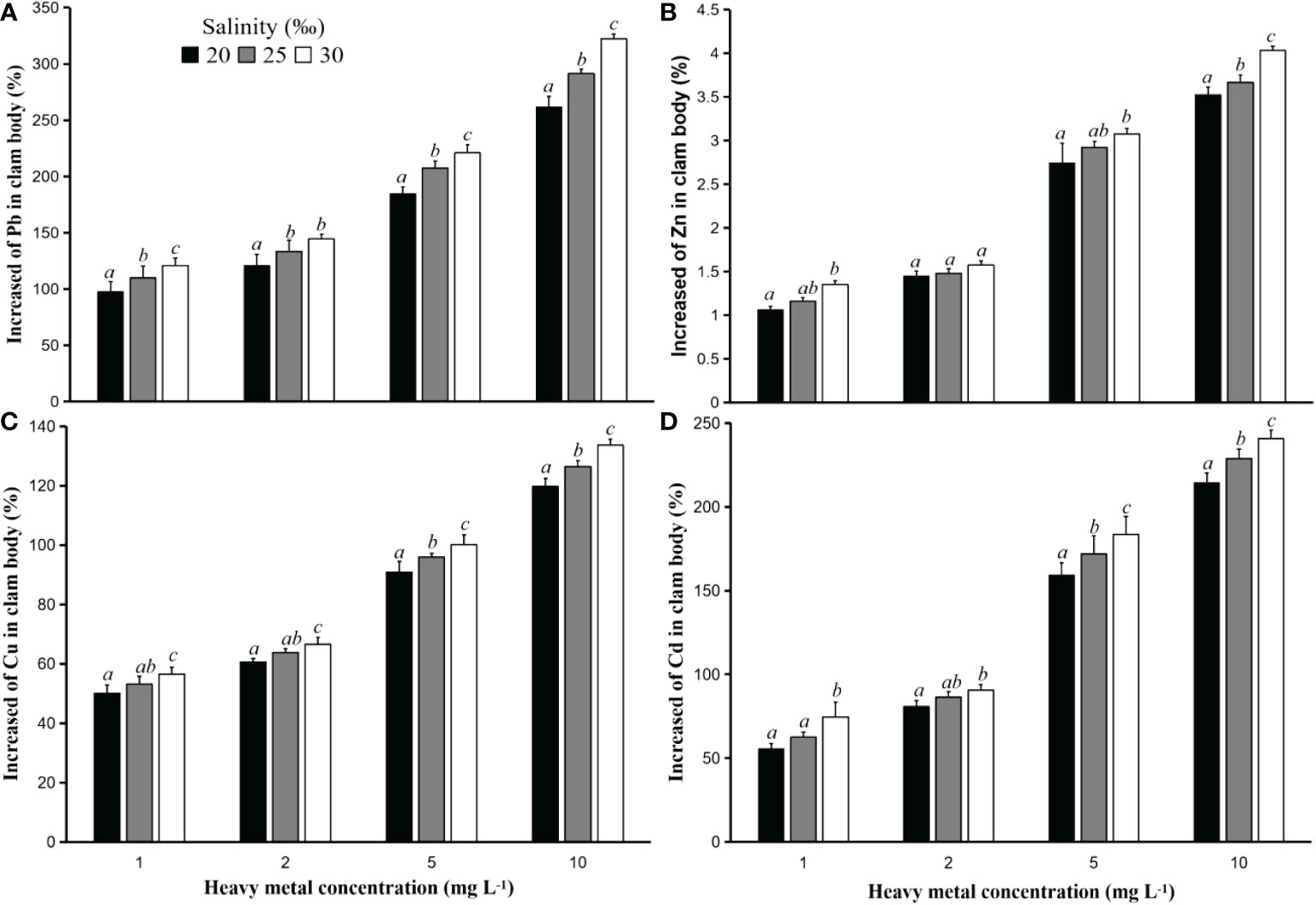
Figure 3 Increased heavy metal concentrations (%) Pb (A), Zn (B), Cu (C), and Cd (D) in the hard clam (M. lusoria) body after 24 hours of exposure to PS particles with a size of 250 m that had contact with heavy metals at different concentrations (1, 2, 5, and 10 mg L-1) and salinities (20, 25, and 30‰). See Figure 2 for statistical information.
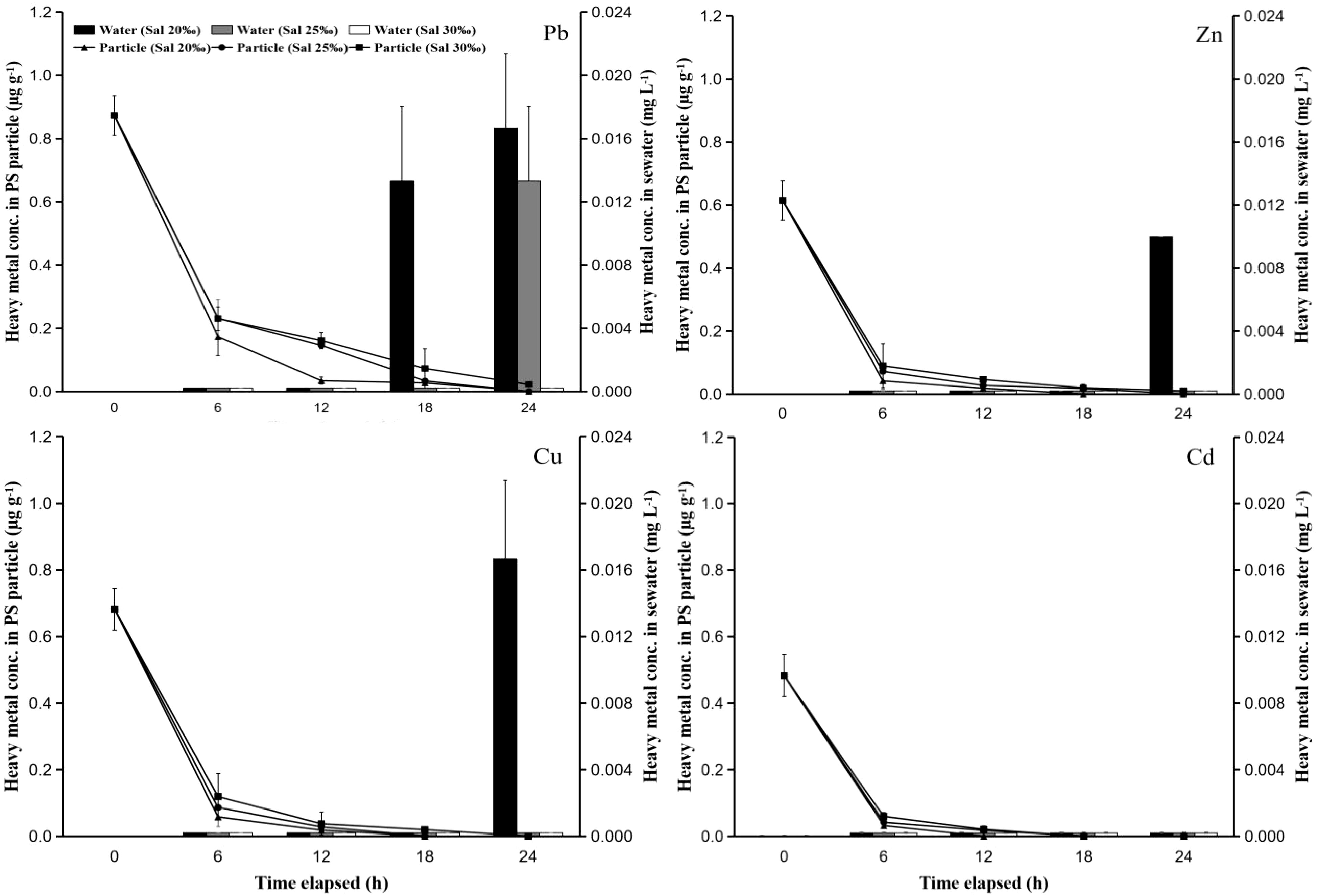
Figure 4 The time-course changes of heavy metal concentrations remaining in PS particles and water at the test used PS particles with a size of 50 µm that had contact with heavy metals at concentrations of 10 mg L-1 and different salinities (20, 25, and 30‰).
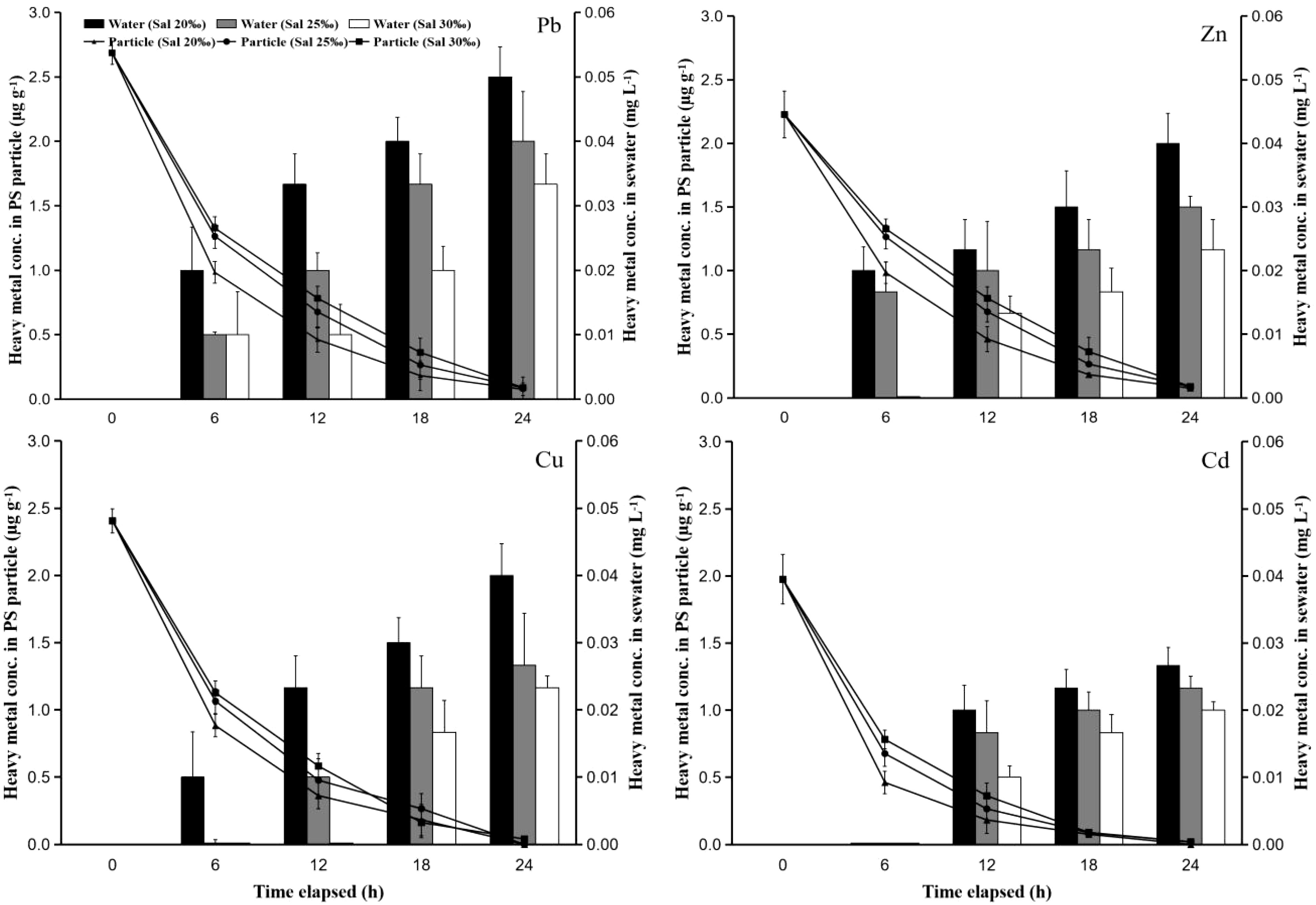
Figure 5 The time-course changes of heavy metal concentrations remaining in PS particles and water at the test used PS particles with a size of 250 µm that had contact with heavy metals at concentrations of 10 mg L-1 and different salinities (20, 25, and 30‰).
Additionally, it was discovered that when hard clams came into touch with PS particles that had been exposed to higher concentration of heavy metals, their bodies’ heavy metal concentrations increased. The value of the metal’s adsorption into the PS particles increases with the concentration of heavy metal in contact with the particles. Oz et al. (2019) discovered that the value of heavy metal adsorption in microplastic particles was higher at an initial heavy metal concentration of 4 mg L-1 in comparison to concentrations of 1 and 2 mg L-1. The highest increase in heavy metals in clam bodies in this study was found in tests using PS particles in contact with heavy metals with a concentration of 10 mg L-1, followed by 5, 2, and 1 mg L-1. These results were found both in tests with particles of size 50 and 250 µm, and in all types of heavy metals (Figures 2 and 3).
The concentration of heavy metals that build up in hard clam bodies is affected by the rise in water salinity. The higher the water salinity value, the greater the increased value of heavy metals in clam bodies. In addition, the number of particles accumulating in the hard clam bodies also has an important role in this case. The more PS particles that accumulate in the clams, the higher the increase in heavy metals.
The value of the increase in heavy metal accumulation in the hard clam (M. lusoria) in this study was determined by statistical analysis, which revealed that salinity, the concentration of heavy metals used in PS particle adsorption, and the quantity of accumulated PS particles all had a significant impact. According to this study, there is the following relationship between the increased rate (%) of each heavy metal and its concentration (C), salinity (S), and number of collected particles (P):
PS size 50 µm:
Increased Pb rate (%)= 69.693 + 13.094 C + 5.919 S + 0.357 P + 0.693 SP (R2 = 0.764)
Increased Zn rate (%)= 7.765 + 2.408 C + 1.472 S + 0.135 P + 0.848 SP (R2 = 0.854)
Increased Cu rate (%)= 20.774 + 6.266 C + 3.991 S + 0.433 P + 0.794 SP (R2 = 0.960)
Increased Cd rate (%)= 56.324 + 13.724 C + 5.399 S + 1.710 P + 0.887 SP (R2 = 0.851)
PS size 250 µm:
Increased Pb rate (%)= 37.159 + 22.681 C + 18.901 S + 0.702 P + 0.968 SP (R2 = 0.889)
Increased Zn rate (%)= 41.503 + 4.495 C + 3.606 S + 1.252 P + 0.855 SP (R2 = 0.746)
Increased Cu rate (%)= 52.995 + 9.203 C + 2.786 S + 0.698 P + 0.834 SP (R2 = 0.798)
Increased Cd rate (%)= 48.482 + 20.528 C + 13.472 S + 0.480 P + 0.819 SP (R2 = 0.964)
Figures 2, 3 demonstrate that, in tests employing particle sizes of 50 and 250 µm, the rise in heavy metal concentrations in the hard clam body varied according to the salt level of the water in which the clams were placed. All of the heavy metals utilized in this investigation produced results that were comparable. The concentration of heavy metals in the bodies of the hard clams increased as a result of being kept at a greater salinity.
In the test utilizing particle size of 250 µm, it was discovered that the difference in the increase in the concentration of heavy metals in hard clam bodies at changes in salinity was more evident. For example, the increase in heavy metal Pb in the test using particle size 250 µm in contact with heavy metal 1 mg L-1 and salinity 20‰ was less significant (p<0.05) compared to that at the other two salinities. The increase in heavy metals in clam bodies in the test with salinity 25‰ was also significantly lower (p<0.05) than that at salinity 30‰ (Figure 3). These results were found at all times of observation and at all initial Pb concentrations in this study. Meanwhile, the same test using particle size 50 µm showed that the increase in heavy metals in clam body placed at salinity 20‰ was not significantly different (p>0.05) from that at salinity 25‰, but significantly different (p<0.05) from that in hard clam placed at salinity 30‰ (Figure 2). These results were found at all times of observation. This is related to the concentration of heavy metals adsorbed on the PS particles. The larger the particle size, the higher the concentration of heavy metals that are absorbed, which causes the effect of the increase in heavy metals to be greater in the body of the hard clam.
In studies with a higher initial concentration of heavy metals in contact with PS particles, this impact was also observed. The increase in the concentration of heavy metal Pb in the body of hard clam placed at 20‰ salinity after 24 hours of exposure to PS particles in contact with heavy metal Pb with a concentration of 1 and 2 mg L-1 was not significantly different (p>0.05) with a salinity of 25‰, but significantly different (p<0.05) from that at 30‰ salinity. Furthermore, the test using concentrations of 5 and 10 mg L-1 in contact with PS particles showed that the increase in heavy metal Pb in hard clam bodies placed at different salinities was significantly different (p<0.05) from one another (Figure 2). This shows that the higher the concentration of heavy metals carried by the PS particles, the difference between the three salinities increases more clearly.
For other heavy metals, results with a nearly same trend were also discovered. The size of the particles used as carriers of heavy metals into the clam body had a strong influence on the increase in heavy metals Zn, Cu, and Cd in hard clam bodies placed at different salinities. The difference in the increase in heavy metals between different salinities is more pronounced the greater the particle size. The large value of heavy metal concentration used for contact with PS particles in the absorption process also has the same impact, namely the clearer difference in the increase in heavy metals in hard clam bodies with different salinities.
In this study, Zn showed the lowest rise in heavy metal content of any heavy metal in hard clam bodies. This was due to the fact that the clam also had the highest background heavy metal content. The effect of metal intake through particles and water is quite weak because to the substantial starting amount of heavy metals. According to Jaffar and Pervaiz (1989), the significant zinc accumulation in fish can be attributed to certain metabolic pathways and zinc-containing coenzyme catalytic reactions. In metal biomolecules with amino acid side chains having illustrious N, O, and/or sulfur donors, zinc additionally functions as a catalyst (Singh and Steinnes, 1994).
The content of heavy metals in the bodies of hard clams increased according to the salt level of the water to which they adapted. This was closely tied to the hard clams’ osmoregulation mechanism. Clams that live in more salinized settings need and consume more water to keep their bodies in balance (Baker et al., 2005; Lin et al., 2016). If the water has been contaminated with heavy metals, this is consistent with the rise in the concentration of heavy metals in their bodies. In contrast, organisms that thrive in less salinity-saturated habitats need less water and release a lot of water to maintain their internal equilibrium. The effect of salinity on the osmoregulation process is increasingly visible from the results of measurements of water volume before and after exposure. The volume of water at higher salinity decreased (water depletion) more than that at lower salinity. In general, water depletion in each salinity is significantly different for almost the entire exposure time. The water depletion in the experiment with salinity 30‰ was significantly higher (p<0.05) than that in the other two salinities (Figure 6). The hard clams allow their blood salinity to vary. They need to keep the ion concentration in their cells relatively constant to maintain the function of important metabolic enzymes. In this study, clams living at a higher salinity drank more water than those living at a lower salinity. This caused PS particles mixed in the solution to have a higher probability of entering the hard clam body. This is indicated by the interaction between salinity and the number of particles accumulated in the clams in all the equations of the relationship above. The more PS particles caused an increase in the concentration of heavy metals in the hard clam bodies. However, maintaining a consistent salinity in cells presents a number of challenges. The salinity of the blood will be lower than the salinity of the cells when the salinity of seawater declines. Osmosis is the process by which water (but not ions) passes from the blood’s high concentration of water into the cell’s low concentration of ions (Berger and Kharazova, 1997; Baker et al., 2005). According to Jones (1975), Idotea baltica, Idotea neglecta, Idotea emarginata, and Jaera albifrons plants exposed to greater salinity saltwater showed higher amounts of Cd and Zn metals than those exposed to lower salinity seawater. The same results were found for the mercury content in the body of Jaera albifrons.
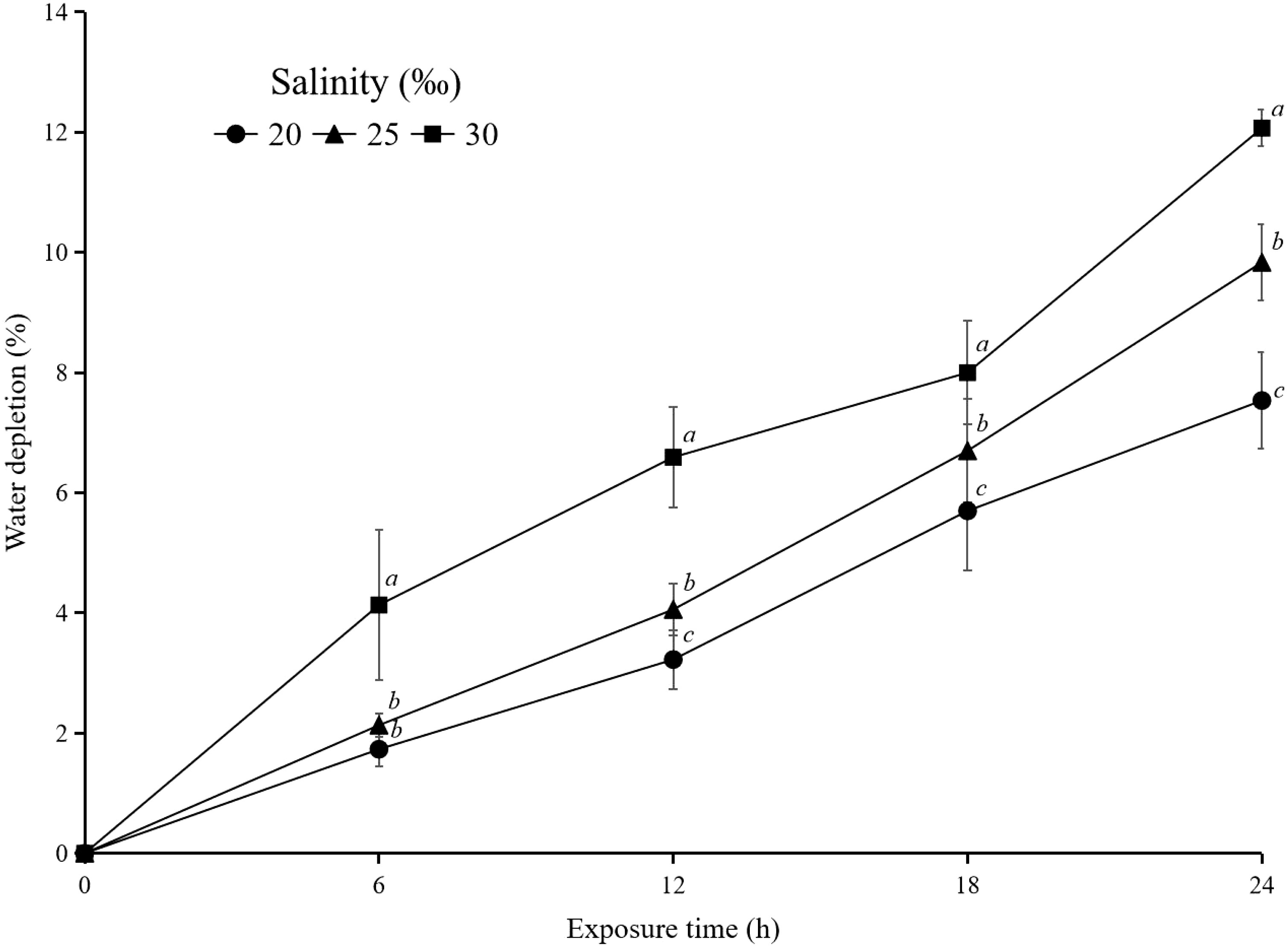
Figure 6 Water depletion (%) after exposure with hard clam (M. lusoria) (n=10) at different times dan salinities (20, 25, and 30‰). The data for water depletion (%) with different letters (a, b, and c) differ significantly (p<0.05) between salinities.
The rise in the number of accumulated particles in the first observation (6 h) did not differ significantly from the end of the observation (24 h). This was due to the limited body size of the clam to accumulate these particles. PS particles with a size of 50 µm accumulated more than those of 250 µm. This result was found in all tests. For example, the number of PS particles of size 50 µm that accumulated in the test with heavy metal Pb with a concentration of 1 mg L-1 and a salinity of 20‰ was 63 ± 5 particles (Figure 7A), while the size of 250 µm was 32 ± 4 particles (Figure 7B). The number of accumulated PS particles was also different in each test with a different salinity. The higher the salinity value, the more accumulated particles there are. These results were found in tests with both PS particle sizes and all tests with different heavy metals. In general, the number of particles accumulated in clam bodies placed at a salinity of 20‰ was significantly lower (p<0.05) than that at a salinity of 25‰. The number of particles accumulated in the clam body placed at a salinity of 25‰ was significantly lower (p<0.05) than that at a salinity of 30‰ (Figure 7). For example, after 24 hours of exposure to metal Zn at 1 mg L-1 concentrations and salinities of 20, 25, and 30‰, the number of PS particles of size 50 µm accumulated in the clam body was 62 ± 6, 70 ± 6, and 98 ± 6 particles, respectively (Figure 7A) whereas there were 37 ± 4, 55 ± 4, 71 ± 5 PS particles with a diameter of 250 µm (Figure 7B).These results indicate that salinity affects the number of particles that enter the hard clam bodies, causing the concentrations of heavy metals in their bodies to also increase. According to Viera et al. (2021), there may be a connection between the concentration of heavy metals and the particle MPs in oyster bodies. Oysters polluted with microplastics were shown to have increased heavy metal contents.
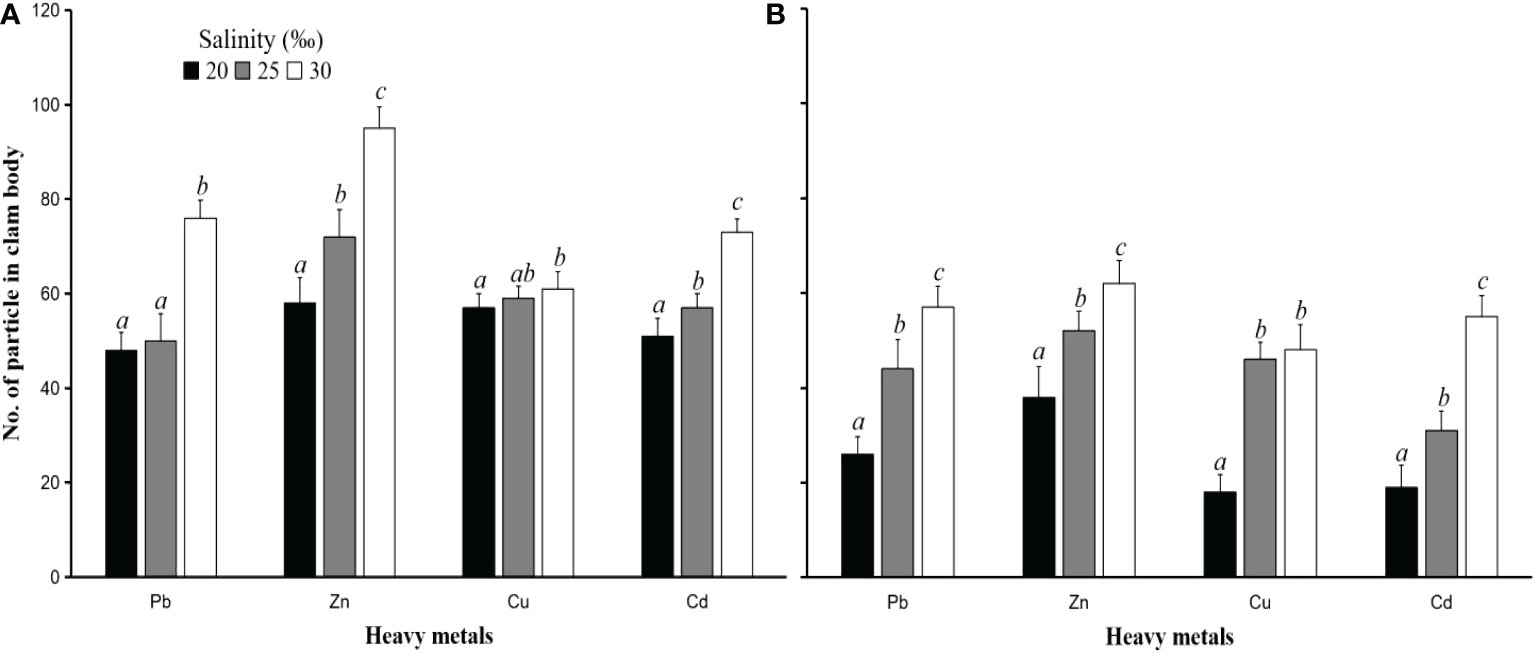
Figure 7 The number of PS particle sizes 50 µm (A) and 250 µm (B) accumulated in the hard clam body (M. lusoria) after contact with heavy metals (Pb, Zn, Cu, and Cd) with an initial concentration 10 mg L-1 at various salinities of 20, 25, and 30‰ after 24 hours of exposure time. The number of particles in the clam body with different letters (a, b, and c) differs significantly (p<0.05) between salinities.
The manner in which heavy metals are released into the water varies depending on the salinity of the water. The release of heavy metals at higher salinity would be slower than that at lower salinity (Barus et al., 2021). Figures 4 and 5 shows that the concentration of heavy metals remaining in the PS particles at the lower salinity test was lower than that at the higher concentration. This indicates that more heavy metals in the particles are released into the water. This is supported by the results of observations of heavy metals remaining in seawater at each observation time. Heavy metal concentrations in seawater with higher salinity have lower values. This was due to ion competition in the water. Competition and higher ion density are found in solutions with higher salt content (Holmes et al., 2014; Liu et al., 2018), which causes heavy metal ions that are absorbed on the surface of the PS particles to become harder to release. The increase in heavy metal concentrations in seawater was found to be higher in the test using PS particles with a size of 250 µm compared to the test with PS particles of 50 µm. This was due to the particles with a larger size absorbing more heavy metals, so that more heavy metals were released into the seawater (Holmes et al., 2014; Fred-Ahmadu et al., 2019). The higher concentration of heavy metals in the test with lower seawater salinity caused the increase in heavy metals in the clam body after 6 hours of observation to be also higher than that in the test with higher salinity.
Heavy metal levels in hard clam will be elevated due to the ongoing accumulation of heavy metals in hard clam. This will cause the concentration value in the body of the clam to exceed the tolerable standard limit. This situation will be even more dangerous when the clam is consumed by humans. Hard clam is a food ingredient that contains nutrients needed by humans. Hard clams are a protein-rich food that has nutritional significance since they are rich in minerals and amino acids (Karnjanapratum et al., 2013). Heavy metals that accumulate in the clam would be transferred to the human body and cause the human body to become contaminated with heavy metals.
4 Conclusion
Our finding shows that salinity has an effect on the rate of accumulation of heavy metals in hard clams (M. lusoria) carried by PS particles. The results of this study also demonstrate that heavy metals that collect in clam bodies can be transported by PS particles. These findings point to a process that has never been explored in other studies—a continuation of the interaction between heavy metals and microplastics that contaminates organisms. All the variables employed in this study, such as PS particle size, the initial concentration of heavy metals used in the absorption process by PS particles, and water salinity, have a significant role in determining the increasing value of heavy metals in hard bodies. Hard clam bodies from higher salinity environments were observed to accumulate more heavy metals. In order to demonstrate the role of microplastics as heavy metal vectors in the real environment, further research is advised. However, this study is restricted to a laboratory size, and other environmental elements are advised to be added.
Data availability statement
The original contributions presented in the study are included in the article/supplementary material. Further inquiries can be directed to the corresponding authors.
Author contributions
KC, MC, and S-YC contributed to conception and design of the study. BB and ZZ wrote the first draft of the manuscript. C-YC, KC, and JW wrote the sections of the manuscript. BB and HW organized the database. All authors contributed to the article and approved the submitted version.
Acknowledgments
This study was funded by Ministry of Science and Technology (Grant No. 106-2611-M-019-009), Taiwan. This research was also funded by the National Natural Science Foundation of China (U2005207, 41976216), the Fujian Provincial Natural Science Foundation of China (2014J06014); and Special Project of Marine Biological Application Technology Cooperative Innovation Center, Fujian Province (XTZX-HYSW-1802), and Guangxi Talent and Innovation Base Project (2018AD19280). We thank to Mr. Z. Y. Cyue, Mr. P. H. Chou and Ms. H. R. Hsieh for their help with the analyses.
Conflict of interest
The authors declare that the research was conducted in the absence of any commercial or financial relationships that could be construed as a potential conflict of interest.
Publisher’s note
All claims expressed in this article are solely those of the authors and do not necessarily represent those of their affiliated organizations, or those of the publisher, the editors and the reviewers. Any product that may be evaluated in this article, or claim that may be made by its manufacturer, is not guaranteed or endorsed by the publisher.
References
Andrady A. L. (2017). The plastic in microplastics: a review. Mar. pollut. Bull. 119 (1), 12–22. doi: 10.1016/j.marpolbul.2017.01.082
Baker S. M., Baker P., Heuberger D., Sturmer L. (2005). Short-term effects of rapid salinity reduction on seed clams (Mercenaria mercenaria). J.clamfish Res. 24, 29–33. doi: 10.2983/0730-8000(2005)24[29:SEORSR]2.0.CO;2
Barus B. S., Chen K., Cai M., Li R., Chen H., Li C., et al. (2021). Heavy metal adsorption and release on polystyrene particles at various salinities. Front. Mar. Sci. 8, 671802. doi: 10.3389/fmars.2021.671802
Berger V. J., Kharazova A. D. (1997). Mechanisms of salinity adaptations in marine molluscs. Hydrobiologia 355, 115–126. doi: 10.1023/A:1003023322263
Brennecke D., Duarte B., Paiva F., Caçador I., Clode J. (2016). Microplastics as vector for heavy metal contamination from the marine environment. Estuarine Coast. Shelf Sci. 178, 189–195. doi: 10.1016/j.ecss.2015.12.003
Chen W. L., Ching P. L., Kao H. L., Cheng S. J., Sheng W. W., Yung K. H., et al. (2007). Bioaccumulation of arsenic compounds in aquacultural clams (Meretrix lusoria) and assessment of potential carcinogenic risks to human health by ingestion. Chemosphere 69 (1), 128–134. doi: 10.1016/j.chemosphere.2007.04.038
Chien Y. H., Hsu W. H. (2006). Effects of diets, their concentrations and clam size on filtration rate of hard clams Meretrix lusoria. J. clamfish Res. 25 (1), 15–22. doi: 10.2983/0730-8000(2006)25[15:EODTCA]2.0.CO;2
Costa D. P. M. B. (2017). The potential of microplastic pellets as a vector to metal contamination in two sympatric marine species (Portugal: Universidade Nova de Lisboa), 60pp.
Covelo E. F., Couce M. L. A., Vega F. A. (2011). Competitive adsorption and desorption of cadmium, chromium, copper, nickel, lead, and zinc by humic umbrisols. Commun. Soil Sci. Plant Anal. 35, 2709–2729. doi: 10.1081/CSS-200036421
Fred-Ahmadu O., Bhagwat G., Idowu O., Benson N. (2019). Interaction of chemical contaminants with microplastics: principles and perspectives. Sci. Total Environ. 706, 135978. doi: 10.1016/j.scitotenv.2019.135978
Galgani F., Hanke G., Werner S., Oosterbaan L., Nilsson P., Fleet D., et al. (2013). Guidance on monitoring for marine litter in European seas. MSFD technical subgroup on marine litter (TSG-ML). European commission. Joint Res. Centre Sci. 128. doi: 10.2788/99475
Galloway T. S., Cole M., Lewis C. (2017). Interactions of microplastic debris throughout the marine ecosystem. Nat. Ecol. Evol. 1, 0116. doi: 10.1038/s41559-017-0116
Galloway T. S., Lewis C. (2016). Marine microplastics spell big problems for future generations. Proc. Natl. Acad. Sci. U. S. A. 113, 2331–2333. doi: 10.1073/pnas.1600715113
Gambardella C., Morgana S., Ferrando S., Bramini M., Piazza V., Costa E., et al. (2017). Effects of polystyrene microbeads in marine planktonic crustaceans. Ecotoxicol. Environ. Saf. 145, 250–257. doi: 10.1016/j.ecoenv.2017.07.036
Gao F., Li J., Sun C., Zhang L., Jiang F., Cao W. (2019). Study on the capability and characteristics of heavy metals enriched on microplastics in marine environment. Mar. pollut. Bull. 144, 61–67. doi: 10.1016/j.marpolbul.2019.04.039
Hahladakis J. N. (2020). Delineating the global plastic marine litter challenge: clarifying the misconceptions. Environ. Monit. Assess. 192, 267. doi: 10.1007/s10661-020-8202-9
Hodson M. E., Duffus-Hodson C. A., Clark A., Prendergast-Miller M. T., Thorpe K. L. (2017). Plastic bag derived-microplastics as a vector for metal exposure in terrestrial invertebrates. Environ. Sci. Technol. 51, 4714–4721. doi: 10.1021/acs.est.7b00635
Holmes L. A., Turner A., Thompson R. C. (2012). Adsorption of trace metals to plastic resin pellets in the marine environment. Environmental Pollution 160, 42–48.
Holmes L. A., Turner A., Thompson R. C. (2014). Interactions between trace metals and plastic production pellets under estuarine conditions. Mar. Chem. 167, 25–32. doi: 10.1016/j.marchem.2014.06.001
Huber M. (2010). “Compendium of bivalves. a full-color guide to 3,300 of the world's marine bivalves,” in A status on bivalvia after 250 years of research (Hackenheim: ConchBooks), 901.
Hu J. Q., Yang S. Z., Guo L., Xu X., Yao T., Xie F. (2017). Microscopic investigation on the adsorption of lubrication oil on microplastics. J. Mol. Liq 227, 351–355. doi: 10.1016/j.molliq.2016.12.043
Jaffar J., Pervaiz S. (1989). Investigation of multiorgan heavy trace metal content of meat of selected dairy, poultry, fowl and fish species Pakistan. J. Sci. Indust Res. 32, 175–177.
Jones M. B. (1975). Synergistic effects of salinity, temperature and heavy metals on mortality and osmoregulation in marine and estuarine isopods (Crustacea). Mar. Biol. 30, 13–20. doi: 10.1007/BF00393748
Ju Y. R., Chen C. F., Chuang X. Y., Lim Y. C., Chen C. W. (2020). Biometry-dependent metal bioaccumulation in aquaculture shellfishes in southwest Taiwan and consumption risk. Chemosphere 253, 126685. doi: 10.1016/j.chemosphere.2020.126685
Karnjanapratum S., Benjakul S., Kishimura H., Tsai Y. H. (2013). Chemical compositions and nutritional value of Asian hard clam (Meretrix lusoria) from the coast of Andaman Sea. Food Chem. 141, 4138–4145. doi: 10.1016/j.foodchem.2013.07.001
Lin C. H., Yeh P. L., Lee T. H. (2016). Ionic and amino acid regulation in hard clam (Meretrix lusoria) in response to salinity challenges. Front. Physiol. 7, 368. doi: 10.3389/fphys.2016.00368
Lithner D., Larsson A Fau - Dave G., Dave G. (2011). Environmental and health hazard ranking and assessment of plastic polymers based on chemical composition. Sci. Total Environ. 409 (18), 3309–3324. doi: 10.1016/j.scitotenv.2011.04.038
Liu G., Zhu Z., Yang Y., Sun Y., Yu F., Ma J. (2018). Sorption behavior and mechanism of hydrophilic organic chemicals to virgin and agedmicroplastics in freshwater and seawater. Environ. pollut. 246, 26–33. doi: 10.1016/j.envpol.2018.11.100
Li J., Yang D., Li L., Shi H. (2015). Microplastics in commercial bivalves from China. Environ. pollut. 207, 190–195. doi: 10.1016/j.envpol.2015.09.018
Malouf R. E., Bricelj V. M. (1989). “Comparative biology of clams: environmental tolerances, feeding, and growth,” in Clam mariculture in north America. Eds. Manzi J. J., Castagna M. (Amsterdam: Elsevier), 23–71.
Mohsen M., Sun L., Zhang L., Lin C. (2019). Microplastic fibers transfer from the water to the internal fluid of the sea cucumber Apostichopus japonicus. Environ. pollut. 27, 113606. doi: 10.1016/j.envpol.2019.113606
Oz N., Kadizade G., Yurtsever M. (2019). Investigation of heavy metal adsorption on microplastics. Appl. Ecol. Environ. Res. 17 (4), 7301–7310. doi: 10.15666/aeer/1704_73017310
Saha U. K., Taniguchi S., Sakurai K. (2002). Simultaneous adsorption of cadmium, zinc, and lead on hydroxyaluminum-and hydroxyaluminosilicatemontmorillonite complexes. Soil Sci. Soc Am. J. 66, 117–128. doi: 10.2136/sssaj2002.1170
Satheeswaran T., Yuvaraj P., Damotharan P., Karthikeyan V., Jha D. K., Dharani G., et al. (2019). Assessment of trace metal contamination in the marine sediment, seawater, and bivalves of parangipettai, southeast coast of India. Mar. pollut. Bull. 149, 110499. doi: 10.1016/j.marpolbul.2019.110499
Singh B. R., Steinnes E. (1994). Soil and water contamination by heavy metals, in r. Lal B. Stewart (eds) Soil Processes Water Quality Lewis Publishers/CRC Press, pp. 233–279.
Turner A., Holmes L. A. (2015). Adsorption of trace metals by microplastic pellets in fresh water. Environ. Chem. 12, 600–610. doi: 10.1071/EN14143
Vedolin M. C., Teophilo C. Y. S., Turra A., Figueira R. C. L. (2018). Spatial variability in the concentrations of metals in beached microplastics. Mar. pollut. Bull. 129, 487–493. doi: 10.1016/j.marpolbul.2017.10.019
Vieira K. S., Neto J. A. B., Crapez M. A. C., Gaylarde C., Pierri B. S., Saldana-Serrano M., et al. (2021). Occurrence of microplastics and heavy metals accumulation in native oysters crassostrea gasar in the paranagua ´ estuarine system, Brazil. Mar. pollut. Bull. 166, 112225. doi: 10.1016/j.marpolbul.2021.112225
Keywords: microplastic, polystyrene, heavy metal, salinity, hard clam
Citation: Barus BS, Zhu Z, Cheuch C-Y, Chen K, Wang J, Cai M, Cheng S-Y and Wei H (2023) Polystyrene as a vector of heavy metals in hard clam Meretrix lusoria under various salinities. Front. Mar. Sci. 9:1014103. doi: 10.3389/fmars.2022.1014103
Received: 08 August 2022; Accepted: 26 December 2022;
Published: 11 January 2023.
Edited by:
Xuetao Guo, Northwest A&F University, ChinaReviewed by:
Abolfazl Naji, Leibniz Centre for Tropical Marine Research (LG), GermanyKe Pan, Shenzhen University, China
Copyright © 2023 Barus, Zhu, Cheuch, Chen, Wang, Cai, Cheng and Wei. This is an open-access article distributed under the terms of the Creative Commons Attribution License (CC BY). The use, distribution or reproduction in other forums is permitted, provided the original author(s) and the copyright owner(s) are credited and that the original publication in this journal is cited, in accordance with accepted academic practice. No use, distribution or reproduction is permitted which does not comply with these terms.
*Correspondence: Kai Chen, kchen@xmu.edu.cn; Minggang Cai, mgcai@xmu.edu.cn; Sha-Yen Cheng, eric@mail.ntou.edu.tw
†These authors have contributed equally to this work