- 1Key Laboratory of Integrated Rice-Fish Farming, Ministry of Agriculture and Rural Affairs, Shanghai Ocean University, Shanghai, China
- 2Key Laboratory of Exploration and Utilization of Aquatic Genetic Resources, Ministry of Education, Shanghai Ocean University, Shanghai, China
- 3Southern Marine Science and Engineering Guangdong Laboratory, Guangzhou, China
Mytilus coruscus is a significant economic species in China’s eastern coastal areas. As a dioecious species, it lacks secondary sexual characteristics, which makes it difficult for selective breeding. However, limited research is carried out on the genetic data regarding reproductive development and gender differentiation. In the current study, de novo transcriptome sequencing analyses were used to detect gonad-specific genes and miRNAs in M. coruscus. By comparing testis and ovary, 159,970 unigenes and 300 miRNAs were obtained totally, of which differentially expressed genes and miRNAs were 9,566 and 25, respectively. Analysis of qRT-PCR showed that cyp26a, dmrt4, foxl2, gdf9, 17β-hsd14, sc6a9, zar1, and zp4 were highly expressed in the ovary as compared to the testis, while sox2 showed lower expression in the ovary. Expression of miR-750-3p, novel 1, and miR-193 was higher in the ovary than that in the testis, whereas the expression of miR-9-5p, miR-9-3p, miR-317, novel 124, miR-2d and miR-263b were lower in the ovary. Furthermore, analysis of miR-317 by Targetscan and MiRanda predicted to target dmrt4 and the luciferase reporter was performed to confirm it. Our research provides a molecular basis for understanding sexual development and reproductive regulation. Further research is needed on the mechanism of gonadal maturation and differentiation in M. coruscus.
Highlights:
● Transcriptome sequencing was used to analyze the mRNA and miRNA in gonads of Mytilus coruscus.
● In total, 9,566 DEGs and 25 DEMs were identified from the gonad of Mytilus coruscus.
● Dual-luciferase reporter assay proved that a testis biased expressed miRNA-133 targeting 5-HT1.
Introduction
In the field of life science, the concept of sex is among the most intriguing. In most animals, once the sex is established, it is typically retained for life. The gonad, which includes the testis and ovary, is a necessary reproductive organ. Gonadal development and sex determination are regulated by several genes that express themselves differently in testes and ovaries (Li et al., 2022a). The process of sex determination involves different sex-determining genes, and several conserved sex-determining genes have been identified and systematically studied in vertebrates. Previous study showed that sex-related genes DMY (Matsuda et al., 2002), HMG box (SOX) (Sutton et al., 2011), and forkhead box L3 (FOXL3) (Nishimura et al., 2015) are identified. These genes were also found as sex-determining genes in invertebrates, especially in economically important bivalve mollusks (Zhang et al., 2014; Ning et al., 2021). Recent studies have identified vg as a gonadal-associated gene and highly expressed in the ovary in the Pacific White Shrimp Litopenaeus vannamei (Li et al., 2022b). The genes foxl2, dmrt, soxE, soxH, and wnt4 all have been recently reported in shellfish (Naimi et al., 2009; Shi et al., 2015; Li et al., 2016; Shi et al., 2018), which are identified to be associated with gonadal development and gender differentiation.
MicroRNA is a non-coding single-stranded RNA molecule in eukaryotes, consisting of approximately 22 nucleotides. It is generally believed that miRNA binds to specific sequences of a target gene, the so-called “seed sequences”, which can cause degradation of target mRNA or inhibit its translation (Ambros, 2004; Bartel, 2007). Previous studies found that miRNAs participated in regulating many biogenesis processes, such as cell growth, cell proliferation, cell apoptosis, tissue differentiation, and embryo development (Mitchell et al., 2008; Miao et al., 2017; Saliminejad et al., 2019). The research on the expression of miRNA has been intensively studied in gonads of different aquatic species such as yellow catfish (Jing et al., 2014), Eriocheir sinensis (He et al., 2015) and Oryzias latipes (Qiu et al., 2018), Macrobrachium rosenbergii (Xia et al., 2022).
As a dioecious species, Mytilus coruscus (M. coruscus) is one of the most popular economic shellfish in China’s eastern coastal areas (Ye et al., 2012; Yang et al., 2021), which has the advantage of fast growth, immense reproductive ability, strong disease resistance, strong adaptability and easy artificial breeding. So far, studies have been conducted on pharmacological (Yang et al., 2013a) and molecular microbiology levels (Yang et al., 2013b; Yang et al., 2014). However, little research is conducted on genes and miRNAs related to gonadal development and differentiation. A comprehensive study of the mussel developmental mechanisms, including gonad-related genes and miRNAs, is currently required.
This study aims to identify important differentially expressed genes and miRNAs controlling sex determination and gonadal development in mussels and their potential biological functions. This research might help us to understand the mechanism of sex differentiation of mussels at the molecular level and provide data for the follow-up study of sexual dimorphism of economic shellfish.
Materials and methods
Sample collection
Three healthy male mussels and three healthy female mussels were collected from Gouqi Island in Zhejiang province. At the time of sampling, M. coruscus were one year old and had reached the stage of sexual differentiation. After dissection, samples of the ovary, testis, mantle, muscle, labial flap, and foot from mussels were obtained and frozen in liquid nitrogen at once and stored at −80°C. All the experiments about M. coruscus were conducted under the Declaration of Helsinki and approved by the Shanghai Ocean University Animal Care and Use Committee with approval number SHOU-2021-118.2.2.
Gonadal histology
To investigate the gonadal developmental stages of mussels, histological analysis was performed. The tissues were fixed in 4% polyformaldehyde (PFA) for 24 hours, and the fixed tissues were dehydrated in a gradient of 70-100% ethanol gradient, embedded in paraffin, sectioned, and stained with hematoxylin-eosin (H.E). Gender and period of gonadal development were determined by mounting sections of each sample on glass slides and examining them under a microscope.
Construction of mRNA and small RNA library
By using Trizol® (Invitrogen, Carlsbad, CA), the total gonadal RNA was extracted. The RNA integrity was evaluated by Agilent 2100 Bioanalyzer (Agilent Technologies, Santa Clara, CA, USA). To obtain cDNA, 1 μg of the above RNA was taken, and then reversed transcribed. The cDNA was further amplified by the PCR method. After that, cDNA constructs were purified. Following the instructions of the TruSeq Small RNA Sample Preparation Kit (Illumina, San Diego, USA), the libraries were conducted using 1 μg total RNA. Then the RNA-seq was conducted on the Illumina sequencing platform (HiSeq 2500) at OE Biotech. Co., Ltd. (Shanghai, China).
Bioinformatics analysis of mRNA and miRNA
Trimmomatic was used to process raw data (raw reads). To get clean reads, the ploy-N-containing reads and the poor-quality sequences were removed. Then the clean reads were assembled into expressed sequence tag clusters (contigs). And de novo assembled into the transcript via the usage of Trinity (version 2.4) within the paired-end strategy. Based on the size and similarity of sequences, the longest transcript was selected as a unigene for subsequent investigation.
For the miRNA transcriptome, cutadapt was used to remove the linker sequence. The sequence was subjected to Q20 quality control using a fast toolkit (version 0.0.13), and those sequences with Q20 of at least 80% were retained. Afterward, using NGSQCToolkit to filter out reads containing N bases (version 2.3.2) Finally, high-quality clean reads were evaluated to analyze the subsequent study. Novel miRNAs were predicted by analyzing the unannotated small RNAs using mirdeep2. The corresponding miRNA star sequences were also determined by analyzing the hairpin structure of pre-miRNAs and aligning with the miRBase database.
The DEG difference algorithm in the R package was used to evaluate the p-value, and the miRNA and mRNA were screened out. TargetScan and MiRanda were used for targeting the prediction of differentially expressed mRNAs (DEGs) and miRNAs (DEMs). TargetScan and MiRanda were used for targeting the prediction of differentially expressed mRNAs and miRNAs.
mRNA/miRNA extraction and QRT-PCR
To validate the DEGs and DEMs in mussels, 9 mRNAs and 9 miRNAs were randomly selected. Total RNA extracted from 7 different tissues of mussel (ovary, testis, mantle, muscle, labial flap, foot) were collected and extracted into total RNA using TRizol® reagent. Then 1 μg total RNA was taken out and reverse transcribed into cDNA with M-MLV reverse transcriptase (Takara, Japan). miRNAs were obtained using TruSeq Small RNA Sample Preparation Kit (Illumina, San Diego, USA). miRNA primers were designed by the stem-loop method and verified by qRT-PCR. miRNA extraction kit was used to extract miRNA from the above 7 tissues. Then miRNAs were transcribed into cDNA using PrimerScript® RT reagent kit with gDNA Eraser kit (Takara, Japan). qRT-PCR was conducted using ABI 7500 real-time fluorescent PCR system and SYBR® premix Ex Taq™ II (Tli RNaseH Plus), and ROX plus (Takara, Japan). The procedure followed the guidelines described in the previous study (Zhang et al., 2021). Using the 2−ΔΔCt method to determine the relative expression of genes, the samples were normalized with 18S rRNA. Three parallel experiments were performed for each reaction. Using Student’s t-test to perform the comparative analysis of ovary and testis. P < 0.05 was considered significant difference. The specific primers for each mRNA and miRNA are shown in Table S1.
Molecular cloning of Dmrt4 gene and plasmid construction
Using the above cDNA, according to the database sequence obtained by sequencing, The primer dmrt4-F/R amplified the dmrt4 coding region by PCR, and the amino acid sequence was compared with the Vector NTI. Bioinformatics analysis showed that miR-317 has a binding site on dmrt4. To validate the targeting relationship between miR-317 and the 3’-UTR of dmrt4, the 3’-UTR of dmrt4 consisting of the miR-317 binding site was cloned into the pmirGLO vector to construct a non-mutant plasmid (WT-pGLO). The seed region where miR-317 binds to the 3’-UTR of dmrt4 was mutated by the primer mutation method and inserted into the pmirGLO vector to complete the mutant plasmid construction (MT-pGLO). For MT-pGLO, the binding sequence (TATACATCACAAAATGTGTGTTCC) was replaced by TATACATCACAAAATGACCAGAGC using the primers, which were conducted into the pmirGLO vector through XbaI and SalI sites. All recombinant plasmids have been sequenced by Sanger to confirm their accuracy. All primers mentioned in this part can be found in Table S1.
Dual-luciferase reporter assay
The culture and transfection methods of human embryonic kidney 293 (HEK293) cells used in the experiment were as previously described (Sun et al., 2020). Using FuGENE@HD (Promega, USA), WT-pGLO and MT-pGLO were co-transfected with miR-317 mimics to HEK293 cells respectively. After 36 hours of transfection, a luciferase activity reporter was conducted using the Dual-Glo® Luciferase Assay System, Promega, USA. To ensure the credibility of the results, each of the experiments was conducted at least three times.
Results
M. coruscus sex and gonad developmental stages
After dissection, gonadal sections of M. coruscus were examined under the microscope. The structure of the ovary and testis could be easily distinguished (Figure 1).oogonia and mature oocytes can be visible in the ovary section of M. coruscus (Figures 1A, B). In the mussel nest sections, spermatogonia and spermatocytes could be seen (Figures 1C, D). According to the histological results, the testicles and ovaries of M. coruscus were at stage III of gonadal maturation. These tissues were then used for RNA sequencing and qRT-PCR.
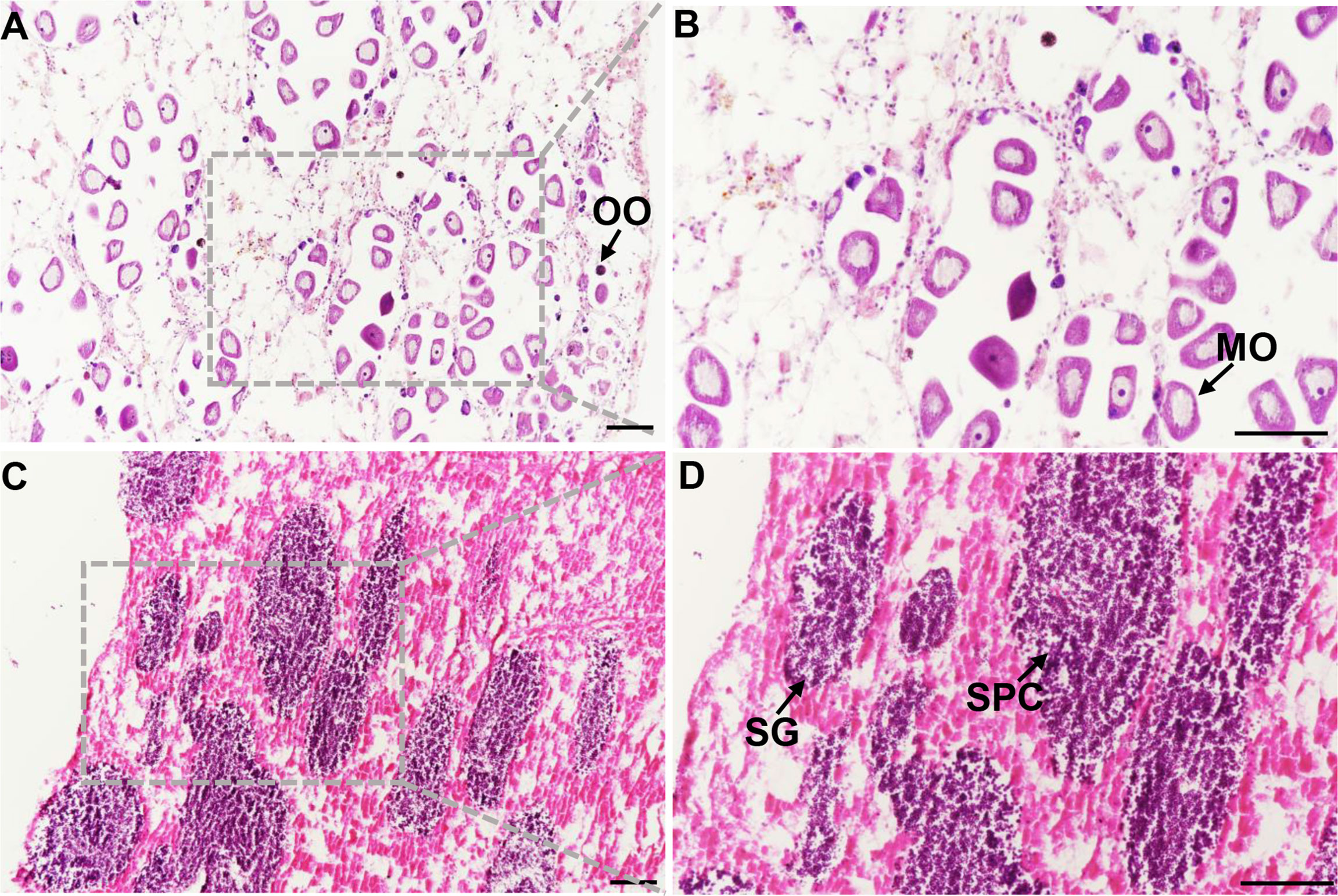
Figure 1 Mytilus coruscus gonad sections. (A) and (B) ovary tissue at stage III, (C) and (D) testis tissue at stage III. OO, oogonia; MO, mature oocyte; SG, spermatogonia; SPC, spermatocytes. Scale bars, 100 µm.
Assembly, annotation, and bioinformatical analysis
Six gonadal tissues (3 ovaries and 3 testes) were sequenced by Illumina HiSeq 2500. In this analysis, 159,970 unigenes were assembled with a length of 150,935,287 bp totally and an average length of 943 bp. Annotating the Unigene database, there were 32,460 (20.29%) annotated genes in the NR library, 17,903 (11.19%) annotated genes in the Swissprot library, and 14,631 (9.15%) annotated genes in the KOG library, 20,651 (12.91%) annotated genes in the eggNOG library, 11293 (7.06%) annotated genes in the KEGG library, and 16,359 (10.23%) annotated genes in the GO library. These reads were aligned to unigenes with an alignment rate of 79.11-81.46% (Table 1).
DEGs and DEMs in gonad
In this research, 9,566 DEGs were found when comparing the transcriptional data of the ovary and testis. As compared to the ovary, 2,575 DEGs were in higher expression and 6991 DEGs were in lower expression (Figure 2A). A total of 300 miRNAs between 15-26 nt in length were screened from miRNA sequencing data of 6 gonad tissues (3 ovaries and 3 testes), of which 206 were novel and 94 were known miRNAs. After further comparing the miRNA expression of the ovary and testis, 25 DEMs were obtained. As compared to the ovary, 11 DEMs were expressed highly and 14 DEMs were expressed lowly (Figure 2C). Clear differences in miRNAs expression between the male and female were shown in the volcano plot (Figures 2B, D).
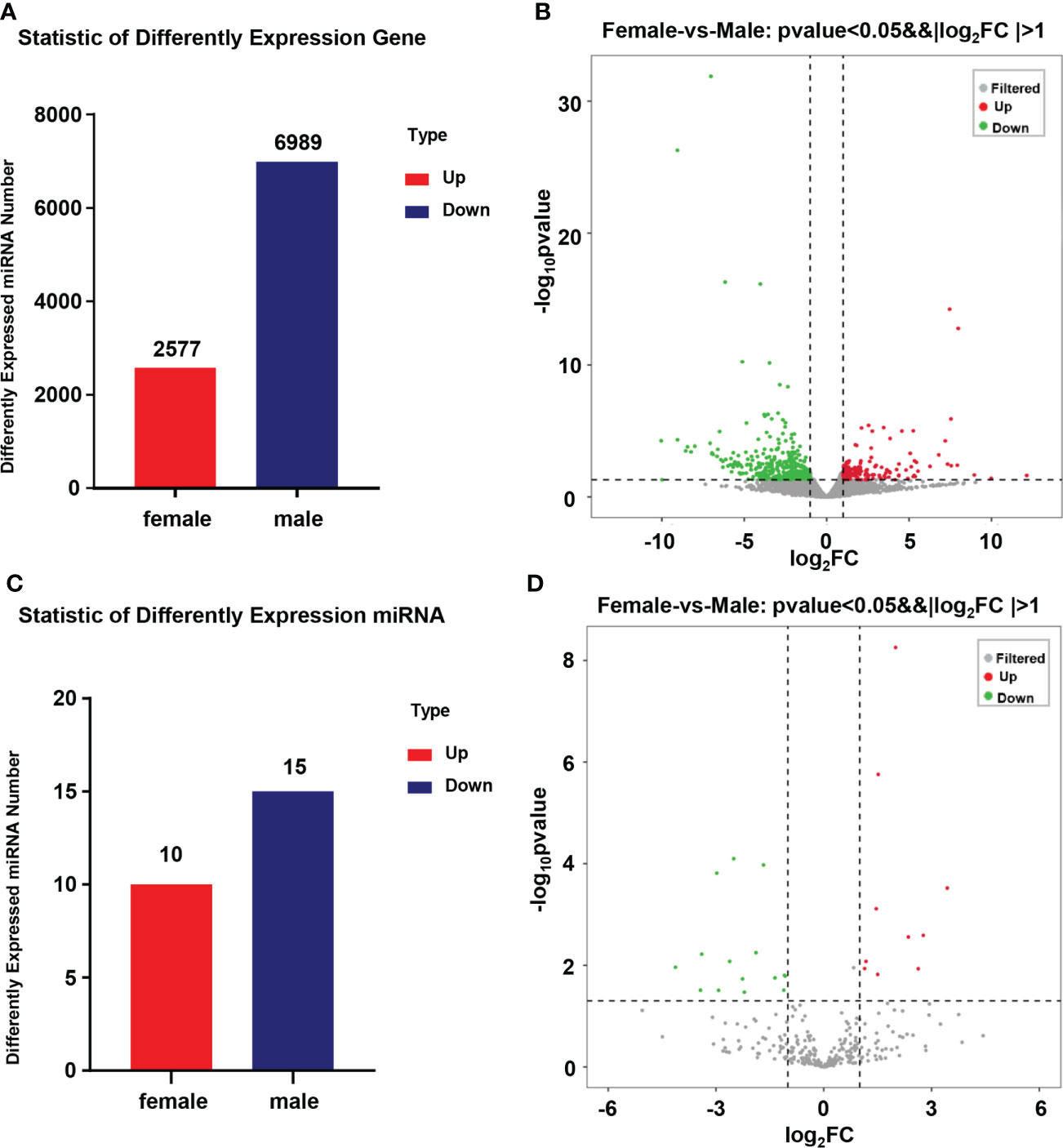
Figure 2 Statistics of DEGs and DEMs in the gonad of Mytilus coruscus. (A) and (C) Statistic of differently expressed mRNA and miRNA. (B) and (D) Volcanic map of male and female Mytilus coruscus differently expressed mRNA and miRNA in the gonad.
GO and KEGG analysis of DEMs
According to Gene Ontology (Mitchell et al., 2008) analysis, the functions of DEGs were described, and a total of 3,138 terms were enriched, including 2,076 biological processes, 390 cellular components, and 672 molecular functions. The most prevalent ones in the three comparison groups were the innate immune response, defense response to the virus, collagen trimer, and nuclear RNA-directed RNA polymerase complex. cysteine-type endopeptidase inhibitor activity (Figure 3A). In the KEGG database, all controls had 262 enriched pathways. The bubble chart stated the top 20 enrichment pathways of the KEGG analysis (Figure 3B). The main enrichment pathways of these differentially expressed genes were Apoptosis−multiple species, Toxoplasmosis, TNF signaling pathway, NF−kappa B signaling pathway, and Ubiquitin mediated proteolysis.
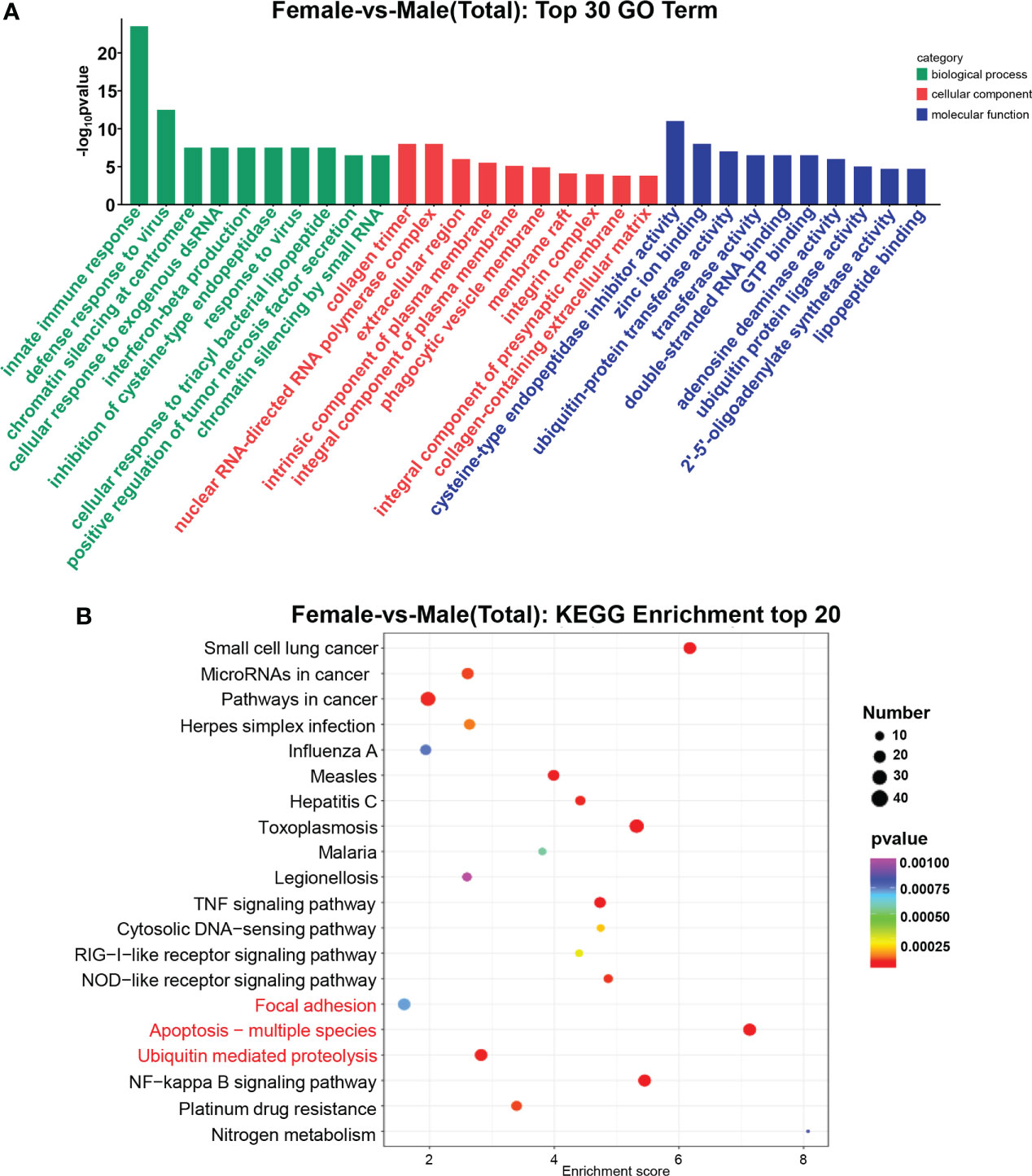
Figure 3 GO terms and KEGG pathway enrichments in DEGs. (A) Distribution of DE miRNAs among the top 30 GO terms. The GO terms are related to sex determination. (B) The top 20 enriched pathways in the gonad. Gonadal-related pathways are marked in red.
Validation of DEGs and DEMs by qRT-PCR
To evaluate the accuracy of transcriptomics data, qRT-PCR was further used to validate the expression levels of the randomly selected 9 mRNAs (cyp26a, dmrt4, foxl2, gdf9,17β-hsd14, sc6a9, sox2, zar1, zp4) and 9 miRNAs (miR-193, miR-317, miR-750-3p, miR-2d, miR-9-5p, miR-9-3p, miR-263b, novel 1, novel 124). The qRT-PCR results of the above genes and miRNAs were consistent with the results of RNA-seq. Meanwhile, their expressions in different tissues were detected.
Among the differentially expressed genes selected above, the expression levels of cyp26a, dmrt4, foxl2, gdf9, 17β-hsd14, sc6a9, zar1, and zp4 in the ovary were remarkably higher than those in the testis. However, sox2 was in high expression in the testis (Figure 4). Among the above-mentioned DEMs, the expression level of miR-193, novel 1, and miR-750-3p in the ovary was significantly higher than in the testis, whereas miR-9-5p, miR-9-3p, miR-317, novel 124, miR-2d, and miR-263b expressed lowly in the ovary. (Figure 5).
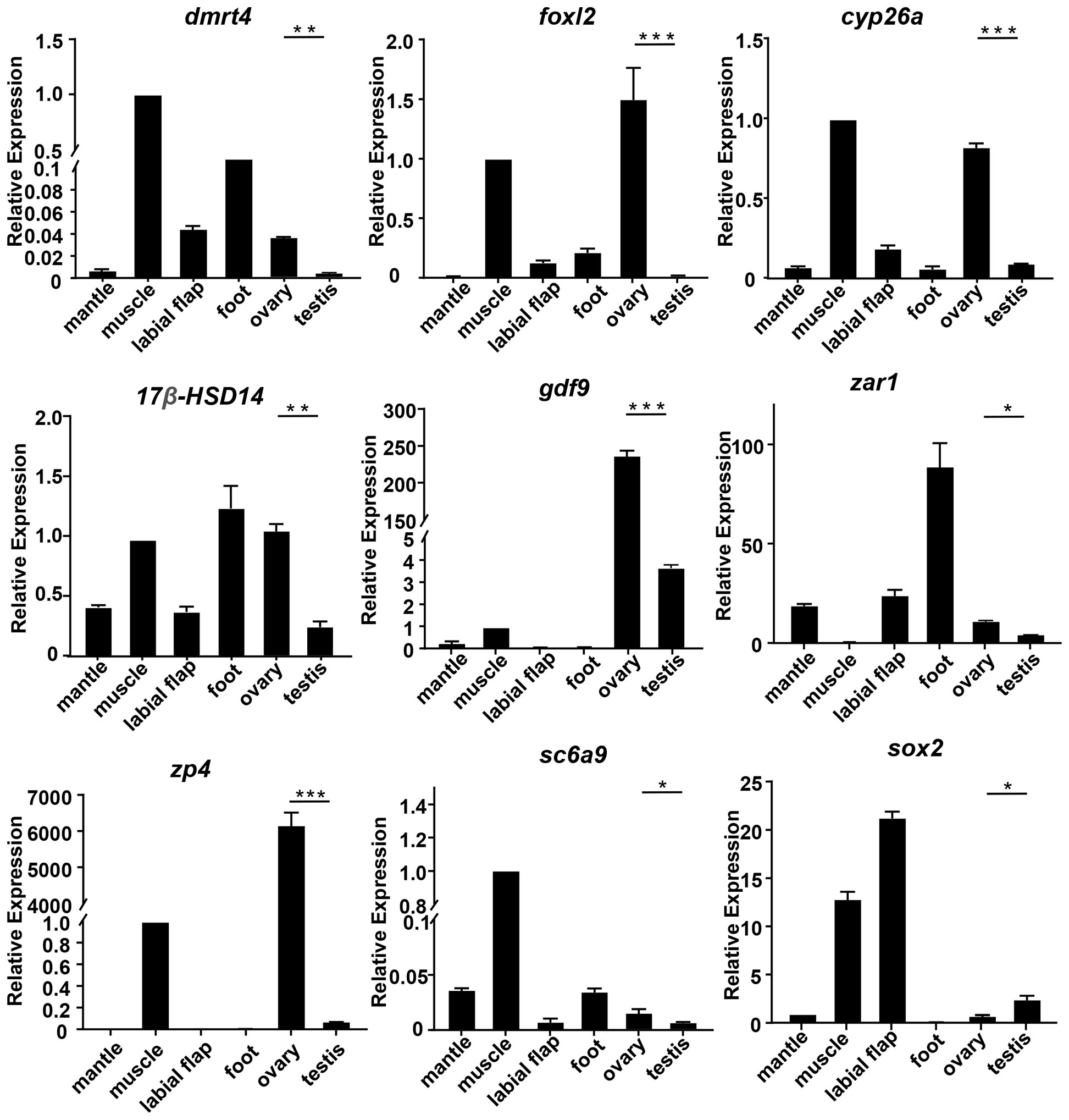
Figure 4 The relative expression of DEGs in mussels. The relative expression of each DEGs was determined by comparative CT (ΔΔCT) methods using 18s rRNA as the internal reference gene. *0.01 < p < 0.05; **0.001 < p < 0.01; ***p < 0.001.
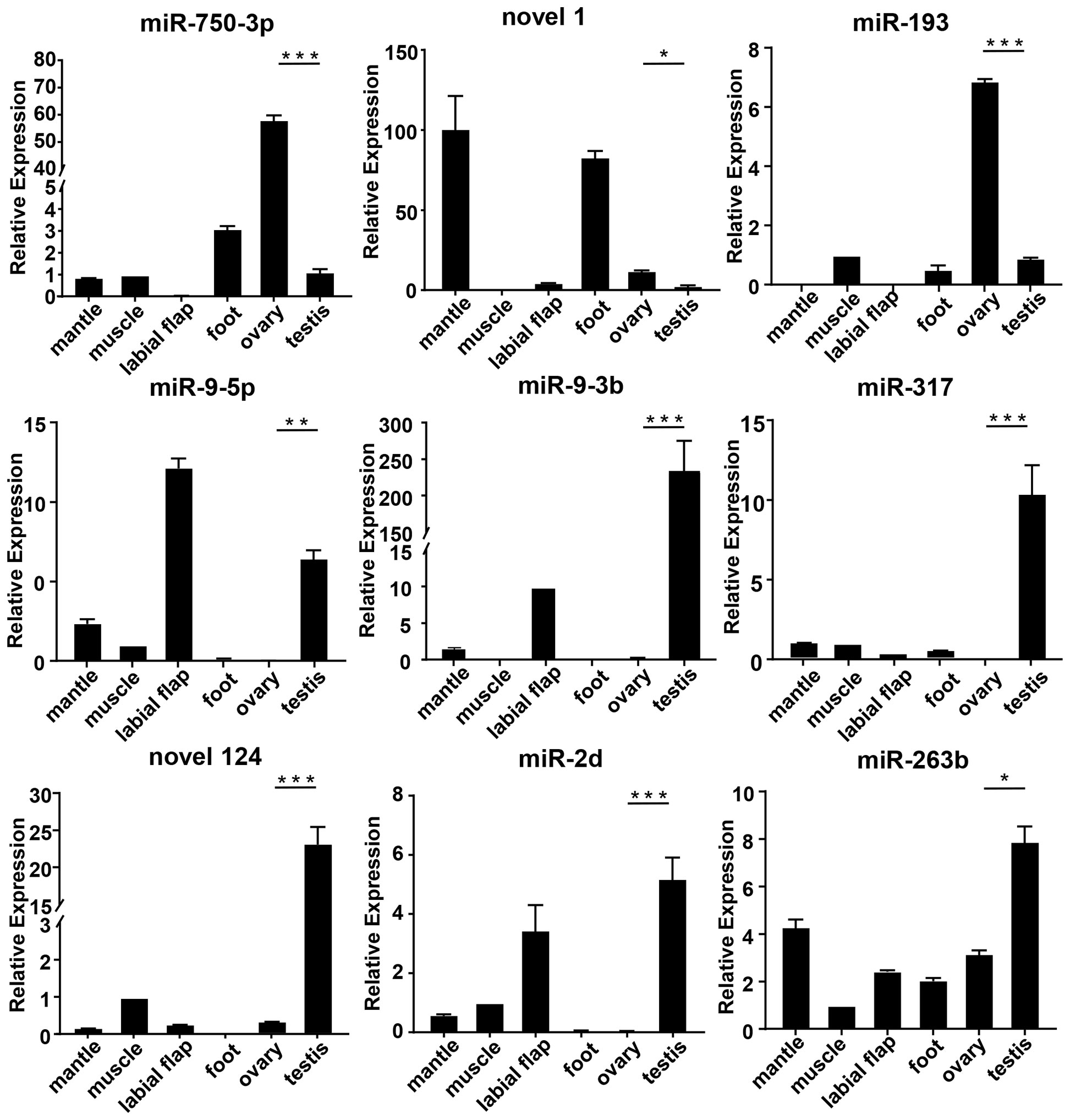
Figure 5 The relative expression of DEMs in mussels. The relative expression of each DEMs was determined by comparative CT (ΔΔCT) methods using 18s rRNA as the internal reference gene. *0.01 < p < 0.05; **0.001 < p < 0.01; ***p < 0.001.
Analysis of the miRNA–mRNA interaction
The interaction between miRNA and mRNA is one of the important regulatory roles of miRNA in biological processes. To understand the miRNA-mRNA interaction, we explored differentially expressed genes and their miRNAs with targeting relationships. A DEM could target one or more DEGs, which could also be predicted by one or more DEMs. This means that a complex regulatory network exists in miRNAs and mRNAs (Figure 6). For example, lgi-miR-92 and mle-miR-92b-3p can target dmrt4 and vasa.
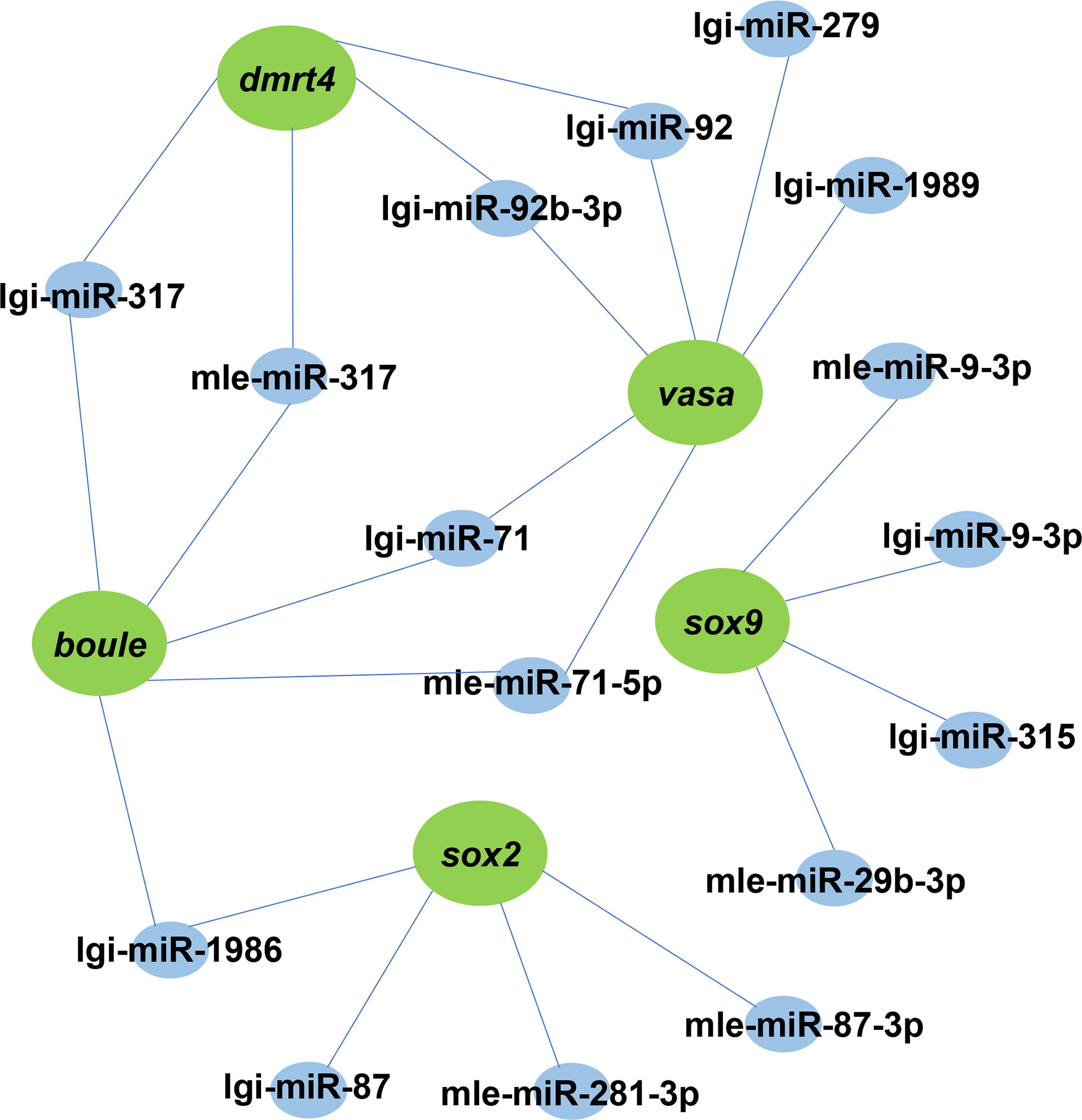
Figure 6 A proposed network of interactions between miRNAs and mRNAs. DEMs and DEGs regulatory networks are illustrated by Cytoscape. Blue indicates miRNA, green represents its target gene.
Cloning and characterization of Dmrt4
The dmrt4 sequence contains a 1050 bp open reading frame (ORF) encoding 349 amino acids (Figure S1). The results of multiple sequence alignment validated that dmrt4 had high homology with other organisms, among which Crassostrea gigas was 63.9%, Pinctada fucata was 62.1%, and Azumapecten farreri was 60.1% (Figure S2).
Dmrt4 is regulated by miR-317
By screening the target genes with Targetscgan threshold > 50 and Miranda energy value < -10, it was found that the miR-317 of the differentially expressed gene completely matched the 3’UTR of dmrt4. Sequence analysis revealed that the binding site miR-317 predicted on 3’UTR of dmrt4 was highly conserved in mussels (Figure 7A). To verify whether miR-317 and dmrt4 had a targeting relationship, two vectors (miR-dmrt4 3’-UTR WT and miR-dmrt4 3’-UTR MT) were co-transfected with miR-317 mimics into HEK293 cells respectively. The results indicated that miR-317 significantly reduced luciferase activity reported by dmrt4-3’-UTR-WT while there have no significance in the negative control (pmirGLO+ miR-317 mimics) and blank control (pmirGLO+ NC). Furthermore, there has no inhibitory effect on the luciferase activity reported by miR-dmrt4 RT 3’UTR MT (Figure 7B). The results strongly suggest that dmrt4 is regulated by miR-317 in the gonad and potentially involves in the gonadal development of mussel.
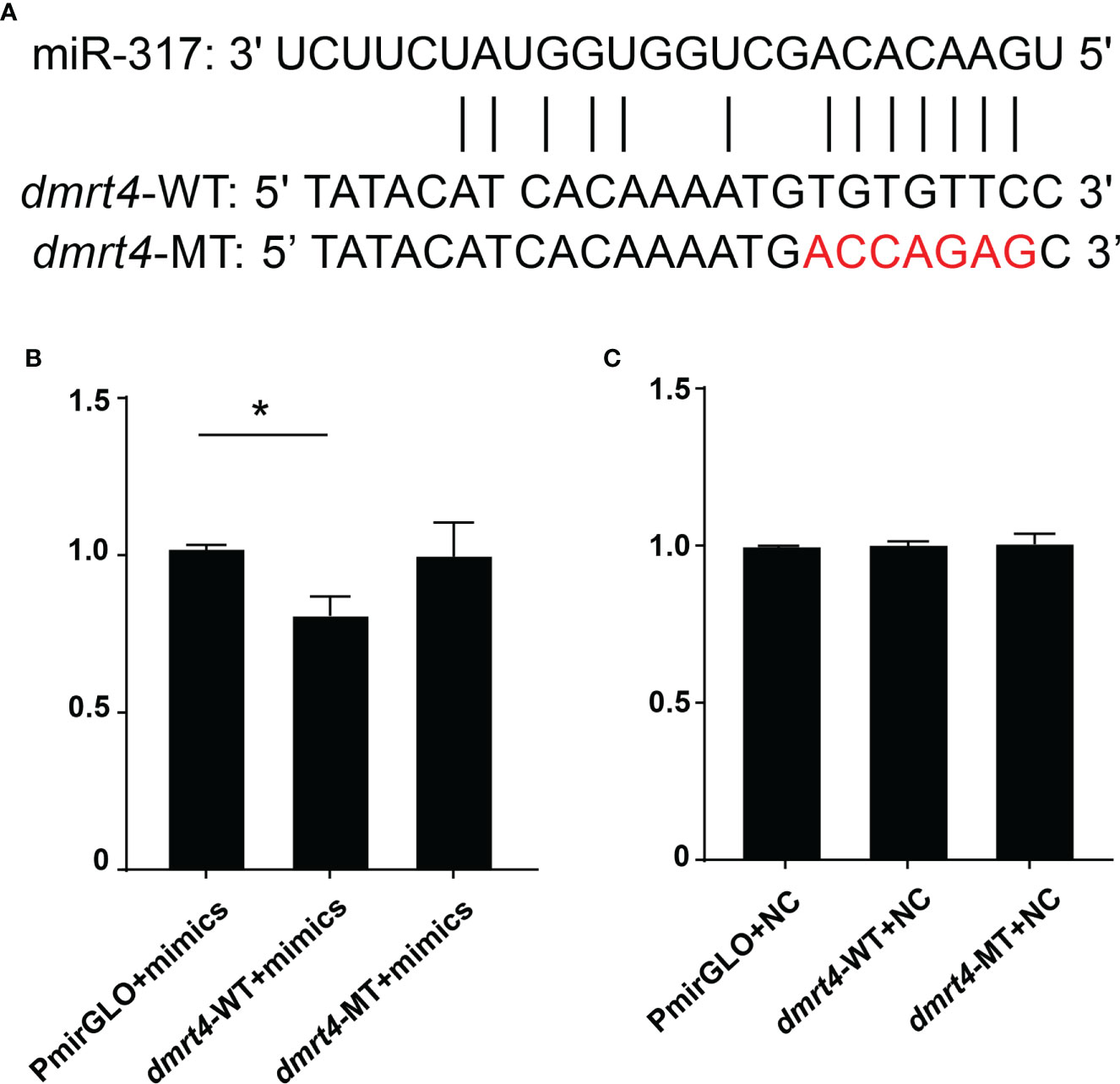
Figure 7 Dual-luciferase reporter assay of miR-317 and dmrt4. (A) Targeted sites of miR-317 and dmrt4 3’UTR, and mutated bases. (B) and (C) The relative luciferase activity in HEK293 cells. GraphPad Prism 7 draws a histogram. The means ± standard deviations of the three independent experiments are shown in the data. *p < 0.05.
Discussion
In the current investigation, high-throughput sequencing was performed in the gonad of mussels. After data was analyzed, the DEGs and DEMs were investigated in the ovary and testis of mussels. Additionally, the cloned dmrt4, which is differentially expressed in the gonad was predicted to be the target of miRNA-317. By luciferase reporter gene analysis, it was confirmed that miRNA-317 indeed inhibited the expression of dmrt4.
In this study, specific genes and miRNAs of mussel gonad were identified by transcriptome sequencing analysis. 159,970 unigenes were identified, of which 9,566 were expressed differently in the gonad. Compare to these DEGs in the ovary, of which 2,575 DEGs were highly expressed, while 6,991 DEGs were in low expression. Cyp26a is an important gene in the cytochrome P450 family, which is expressed highly in the ovary of Yellow catfish (Xiong et al., 2015). Foxl2, a central player in the ovary, elaborates on the functional divergence in gibel carp. Furthermore, Foxl2a-b deficiency led to ovary development arrest or complete sex reversal, while complete disruption of foxl2b-A and foxl2b-B result in the depletion of germ cells in gibel carp (Gan et al., 2021). Gdf9, as the transforming growth factor beta (TGF-β) superfamily, regulates steroidogenesis in granulosa cells (Yu et al., 2020). Sc6a9b is a glycine transporter gene whose expression is higher in the ovary and affects ovarian development by affecting neurotransmitters (Li et al., 2016). Using de novo transcriptome analysis, it is revealed that zp4 was differentially expressed in Opsariichthys bidens (Tang et al., 2022). Zar1 plays a role in the early zebrafish ovary by inhibiting ZP gene translation (Miao et al., 2017). The above DEGs have similar biological roles during sex gonadal development in diverse species, implying that these DEGs might involve in the gonadal development of mussels.
The method of studying the mechanism of sex determination by DEMs has been widely used as the high-throughput sequencing technology develops. In this research, compared with the ovary and testis, a total of 25 DEMs were found, of which 11 DEMs were expressed highly and 14 DEMs were in low expression. A great number of miRNAs have been studied that involve gonadal development and other biological processes. Studies have shown that miR-9 is expressed highly in the ovary of Mud Crab (Scylla paramamosain) (Meng et al., 2018) and swimming crab (Portunus trituberculatus) (Waiho et al., 2019). Furthermore, miR-9 can negatively regulate the expression of the ERK pathway genes Rap-1b in the ovary of Mud Crab (Scylla paramamosain) (Zhou et al., 2020). In Monopterus albus, the relationship between Foxl3 and miR-9 has been found, which could promote ovarian apoptosis and spermatogenesis (Gao et al., 2016). In Drosophila, miR-317 is one of the DEMs which are involved in early embryonic development and larval ovarian morphogenesis (Pushpavalli et al., 2014; Yang et al., 2016; Li et al., 2019). However, in our research, the results of qRT-PCR indicated that miR-9-3p, miR-9-5p, and miR-317 expressed highly in the testis. miR-193 involves the downregulation of c-kit protein, which regulates the development of ovarian follicles in Wistar rats. This is consistent with the expression pattern of miR-193 studied in M. coruscus.
By analyzing multiple amino acid sequences, we identified the dmrt4 and identify the DM domain of this gene. It is a cysteine-rich DNA-binding domain shared by the dmrt gene family, characterized by the intertwining of CCHC and HCCC which is the binding site for Zn2+ (Naimi et al., 2009). In the dmrt gene family, genes with different names have different expression patterns in different species (Hong et al., 2007). Currently, members of the dmrt gene family dmrt1 to dmrt5 have been cloned in a variety of fish particularly zebrafish, medaka, and tilapia (Winkler et al., 2004; Cao et al., 2007; Webster et al., 2017). A member of the dmrt gene family, specifically dmrt4, has expressed differently in various species, and these variations may be related to gonad developmental and other processes. In tilapia, the expression level of dmo (dmrt4 in other species) is substantially higher in the ovary than in the testes, thus suggesting that dmo may be a key regulator of gonadal development (Cao et al., 2007).In Oryzias latipes, dmrt4 is identified as maternal transcripts and expressed in gonads until the early larval stages (Kondo et al., 2002; Winkler et al., 2004). By conducting the qRT-PCR experiment, the pattern of the dmrt4 was verified in different tissues of mussels. The result showed that dmrt4 may participate in mussels’ gonadal differentiation. Furthermore, the high expression of dmrt4 in adductor and foot suggests that the gene has other biological functions besides gonadal differentiation. With the in-depth study of miRNAs, more and more miRNAs have been found to participate in regulation through the miRNA-mRNA regulatory network (Jing et al., 2014; Zhang et al., 2016). In this study, we focused on the relationship between miR-317 and dmrt4. The results of qRT-PCR indicated the opposite expression pattern of miR-317 and dmrt4 in the ovary and testis and the results of bioinformatics analysis indicated that dmrt4 may be the target gene of miR-317. Then, the targeting relationship between dmrt4 and miR-317 was confirmed by a luciferase reporter assay. The above experimental results indicate that miR-317-dmrt4 is essential for the gonadal development of mussels.
In conclusion, mRNA and miRNA from the ovary and testis of mussels were sequenced by transcriptome sequencing and then analyzed using bioinformatics. A total of 159,970 unigenes and 300 miRNAs were identified. qRT-PCR technology was performed to validate the accuracy of RNA-seq results, and also explore the expression patterns of DEGs and DEMs. Then, the structural network of mRNA-miRNA interaction was constructed. Moreover, Bioinformatics analysis and luciferase reporter analysis confirmed a targeting relationship between miR-317 and dmrt4. These results are of great significance to our further study of the gonad-related mechanism of mussels and provide valuable data for future research on mussel gonadal development.
Data availability statement
The datasets presented in this study can be found in online repositories. The names of the repository/repositories and accession number(s) can be found below: https://figshare.com/, https://doi.org/10.6084/m9.figshare.20424369.v2.
Ethics statement
The animal study was reviewed and approved by Shanghai Ocean University Animal Care and Use Committee.
Author contributions
Methodology, Investigation Data Curation, Analysis, Writing: MW and JX. Visualization: LG and XL. Review and Editing: MJ and WW. Funding, Writing - Review and Editing: ML and JY. All authors have read and agreed to the published version of the manuscript.
Funding
This work was supported by the National Key R&D Program of China (2018YFD0901205), Program of Shanghai Academic Research Leader (20XD1421800), Key Special Project for Introduced Talents Team of Southern Marine Science and Engineering Guangdong Laboratory (Guangzhou) (GML2019ZD0402) and Program for study on genetic resources, environment and strategy of mussel culture in coast of Gouqi Island offshore.
Conflict of interest
The authors declare that the research was conducted in the absence of any commercial or financial relationships that could be construed as a potential conflict of interest.
Publisher’s note
All claims expressed in this article are solely those of the authors and do not necessarily represent those of their affiliated organizations, or those of the publisher, the editors and the reviewers. Any product that may be evaluated in this article, or claim that may be made by its manufacturer, is not guaranteed or endorsed by the publisher.
Supplementary material
The Supplementary Material for this article can be found online at: https://www.frontiersin.org/articles/10.3389/fmars.2022.1013857/full#supplementary-material
References
Ambros V. (2004). The functions of animal microRNAs. Nature. 431(7006), 350–355. doi: 10.1038/nature02871
Bartel D. P. (2007). MicroRNAs: Genomics, biogenesis, mechanism, and function. Cell 131 (4), 11–29. doi: 10.1016/s0092-8674(04)00045-5
Cao J., Cao Z., Wu T. (2007). Generation of antibodies against DMRT1 and DMRT4 of oreochromis aurea and analysis of their expression profile in Oreochromis aurea tissues. J. Genet. Genomics 34 (6), 497–509. doi: 10.1016/S1673-8527(07)60055-1
Gan R. H., Wang Y., Li Z., Yu Z. X., Li X. Y., Tong J. F., et al. (2021). Functional divergence of multiple duplicated Foxl2 homeologs and alleles in a recurrent polyploid fish. Mol. Biol. Evol. 38 (5), 1995–2013. doi: 10.1093/molbev/msab002
Gao Y., Jia D., Hu Q., Li D. (2016). Foxl3, a target of miR-9, stimulates spermatogenesis in spermatogonia during natural sex change in Monopterus albus. Endocrinology 157 (11), 4388–4399. doi: 10.1210/en.2016-1256
He L., Wang Y. L., Li Q., Yang H. D., Duan Z. L., Wang Q. (2015). Profiling microRNAs in the testis during sexual maturation stages in Eriocheir sinensis. Anim. Reprod. Sci. 162, 52–61. doi: 10.1016/j.anireprosci.2015.09.008
Hong C.-S., Park B.-Y., Saint-Jeannet J.-P. (2007). The function of dmrt genes in vertebrate development: It is not just about sex. Dev. Biol. 310 (1), 1–9. doi: 10.1016/j.ydbio.2007.07.035
Jing J., Wu J., Liu W., Xiong S., Ma W., Zhang J., et al. (2014). Sex-biased miRNAs in gonad and their potential roles for testis development in yellow catfish. PloS One 9 (9), e107946. doi: 10.1371/journal.pone.0107946
Kondo M., Froschauer A., Kitano A., Nanda I., Hornung U., Volff J. N., et al. (2002). Molecular cloning and characterization of DMRT genes from the medaka oryzias latipes and the platyfish xiphophorus maculatus. Gene 295 (2), 213–222. doi: 10.1016/S0378-1119(02)00692-3
Li R., Huang Y., Zhang Q., Zhou H., Jin P., Ma F. (2019). The miR-317 functions as a negative regulator of toll immune response and influences drosophila survival. Dev. Comp. Immunol. 95, 19–27. doi: 10.1016/j.dci.2019.01.012
Li X. Y., Mei J., Ge C. T., Liu X. L., Gui J. F. (2022a). Sex determination mechanisms and sex control approaches in aquaculture animals. Sci. China Life Sci. 65 (6), 1091–1122. doi: 10.1007/s11427-021-2075-x
Li Y., Zhang L., Sun Y., Ma X., Wang J., Li R., et al. (2016). Transcriptome sequencing and comparative analysis of ovary and testis identifies potential key sex-related genes and pathways in scallop Patinopecten yessoensis. Mar. Biotechnol. (NY) 18 (4), 453–465. doi: 10.1007/s10126-016-9706-8
Li Z., Zhou M., Ruan Y., Chen X., Ren C., Yang H., et al. (2022b). Transcriptomic analysis reveals yolk accumulation mechanism from the hepatopancreas to ovary in the pacific white shrimp Litopenaeus vannamei. Front. Mar. Sci. 9. doi: 10.3389/fmars.2022.948105
Matsuda M., Nagahama Y., Shinomiya A., Sato T., Matsuda C., Kobayashi T., et al. (2002). DMY is a y-specific DM-domain gene required for male development in the medaka fish. Nature 417 (6888), 559–563. doi: 10.1038/nature751
Meng X., Zhang X., Li J., Liu P. (2018). Identification and comparative profiling of ovarian and testicular microRNAs in the swimming crab Portunus trituberculatus. Gene 640, 6–13. doi: 10.1016/j.gene.2017.10.026
Miao L., Yuan Y., Cheng F., Fang J., Zhou F., Ma W., et al. (2017). Translation repression by maternal RNA binding protein Zar1 is essential for early oogenesis in zebrafish. Development 144 (1), 128–138. doi: 10.1242/dev.144642
Mitchell P. S., Parkin R. K., Kroh E. M., Fritz B. R., Wyman S. K., Pogosova-Agadjanyan E. L., et al. (2008). Circulating microRNAs as stable blood-based markers for cancer detection. Proc. Natl. Acad. Sci. United States America 105 (30), 10513–10518. doi: 10.1073/pnas.0804549105
Naimi A., Martinez A. S., Specq M. L., Mrac A., Diss B., Mathieu M., et al. (2009). Identification and expression of a factor of the DM family in the oyster Crassostrea gigas. Comp. Biochem. Physiol. A Mol. Integr. Physiol. 152 (2), 189–196. doi: 10.1016/j.cbpa.2008.09.019
Ning J., Cao W., Lu X., Chen M., Liu B., Wang C. (2021). Identification and functional analysis of a sex-biased transcriptional factor Foxl2 in the bay scallop argopecten irradians irradians. Comp. Biochem. Physiol. B Biochem. Mol. Biol. 256, 110638. doi: 10.1016/j.cbpb.2021.110638
Nishimura T., Sato T., Yamamoto Y., Watakabe I., Ohkawa Y., Suyama M., et al. (2015). Sex determination. foxl3 is a germ cell-intrinsic factor involved in sperm-egg fate decision in medaka. Science 349 (6245), 328–331. doi: 10.1126/science.aaa2657
Pushpavalli S. N., Sarkar A., Bag I., Hunt C. R., Ramaiah M. J., Pandita T. K., et al. (2014). Argonaute-1 functions as a mitotic regulator by controlling cyclin b during drosophila early embryogenesis. FASEB J. 28 (2), 655–666. doi: 10.1096/fj.13-231167
Qiu W., Zhu Y., Wu Y., Yuan C., Chen K., Li M. (2018). Identification and expression analysis of microRNAs in medaka gonads. Gene 646, 210–216. doi: 10.1016/j.gene.2017.12.062
Saliminejad K., Khorram Khorshid H. R., Soleymani Fard S., Ghaffari S. H. (2019). An overview of microRNAs: Biology, functions, therapeutics, and analysis methods. J. Cell Physiol. 234 (5), 5451–5465. doi: 10.1002/jcp.27486
Shi J., Hong Y., Sheng J., Peng K., Wang J. (2015). De novo transcriptome sequencing to identify the sex-determination genes in Hyriopsis schlegelii. Biosci. Biotechnol. Biochem. 79 (8), 1257–1265. doi: 10.1080/09168451.2015.1025690
Shi Y., Liu W., He M. (2018). Proteome and transcriptome analysis of ovary, intersex gonads, and testis reveals potential key sex Reversal/Differentiation genes and mechanism in scallop chlamys nobilis. Mar. Biotechnol. (NY) 20 (2), 220–245. doi: 10.1007/s10126-018-9800-1
Sun L. L., Zhong Y., Qiu W. W., Guo J., Gui L., Li M. Y. (2020). MiR-26 regulates ddx3x expression in medaka (Oryzias latipes) gonads. Comp. Biochem. Physiol. B-Biochemistry Mol. Biol. 246-247, 110456. doi: 10.1016/j.cbpb.2020.110456
Sutton E., Hughes J., White S., Sekido R., Tan J., Arboleda V., et al. (2011). Identification of SOX3 as an XX male sex reversal gene in mice and humans. J. Clin. Invest. 121 (1), 328–341. doi: 10.1172/JCI42580
Tang R., Zhu Y., Gan W., Zhang Y., Yao Z., Ren J., et al. (2022). De novo transcriptome analysis of gonads reveals the sex-associated genes in Chinese hook snout carp Opsariichthys bidens. Aquaculture Rep. 23, 101068. doi: 10.1016/j.aqrep.2022.101068
Waiho K., Fazhan H., Zhang Y., Zhang Y., Li S., Zheng H., et al. (2019). Gonadal microRNA expression profiles and their potential role in sex differentiation and gonadal maturation of mud crab Scylla paramamosain. Mar. Biotechnol. (NY) 21 (3), 320–334. doi: 10.1007/s10126-019-09882-1
Webster K. A., Schach U., Ordaz A., Steinfeld J. S., Draper B. W., Siegfried K. R. (2017). Dmrt1 is necessary for male sexual development in zebrafish. Dev. Biol. 422 (1), 33–46. doi: 10.1016/j.ydbio.2016.12.008
Winkler C., Hornung U., Kondo M., Neuner C., Duschl J., Shima A., et al. (2004). Developmentally regulated and non-sex-specific expression of autosomal dmrt genes in embryos of the medaka fish (Oryzias latipes). Mech. Dev. 121 (7-8), 997–1005. doi: 10.1016/j.mod.2004.03.018
Xia J., Liu D., Zhou W. Z., Yi S. K., Wang X. H., Li B. L., et al. (2022). Comparative transcriptome analysis of brain and gonad reveals reproduction-related miRNAs in the giant prawn, Macrobrachium rosenbergii. Front. Genet. 2331. doi: 10.3389/fgene.2022.990677
Xiong S., Jing J., Wu J., Ma W., Dawar F. U., Mei J., et al. (2015). Characterization and sexual dimorphic expression of cytochrome P450 genes in the hypothalamic-pituitary-gonad axis of yellow catfish. Gen. Comp. Endocrinol. 216, 90–97. doi: 10.1016/j.ygcen.2015.04.015
Yang J. L., Feng D. D., Liu J., Xu J. K., Chen K., Li Y. F., et al. (2021). Chromosome-level genome assembly of the hard-shelled mussel Mytilus coruscus, a widely distributed species from the temperate areas of East Asia. Gigascience 10 (4), giab024. doi: 10.1093/gigascience/giab024
Yang H., Li M., Hu X., Xin T., Zhang S., Zhao G., et al. (2016). MicroRNA-dependent roles of drosha and pasha in the drosophila larval ovary morphogenesis. Dev. Biol. 416 (2), 312–323. doi: 10.1016/j.ydbio.2016.06.026
Yang J. L., Li X., Liang X., Bao W. Y., Shen H. D., Li J. L. (2014). Effects of natural biofilms on settlement of plantigrades of the mussel Mytilus coruscus. Aquaculture 424, 228–233. doi: 10.1016/j.aquaculture.2014.01.007
Yang J. L., Li S. H., Li Y. F., Liu Z. W., Liang X., Bao W. Y., et al. (2013a). Effects of neuroactive compounds, ions and organic solvents on larval metamorphosis of the mussel Mytilus coruscus. Aquaculture 396, 106–112. doi: 10.1016/j.aquaculture.2013.02.039
Yang J. L., Shen P. J., Liang X., Li Y. F., Bao W. Y., Li J. L. (2013b). Larval settlement and metamorphosis of the mussel Mytilus coruscus in response to monospecific bacterial biofilms. Biofouling 29 (3), 247–259. doi: 10.1080/08927014.2013.764412
Ye Y. Y., Li J. J., Wu C. W., Xu M. Y., Guo B. Y. (2012). Genetic analysis of mussel (Mytilus coruscus) populations on the coast of East China Sea revealed by ISSR-PCR markers. Biochem. Systematics Ecol. 45, 1–6. doi: 10.1016/j.bse.2012.07.022
Yu H., Wang Y., Wang M., Liu Y., Cheng J., Zhang Q. (2020). Growth differentiation factor 9 (gdf9) and bone morphogenetic protein 15 (bmp15) are potential intraovarian regulators of steroidogenesis in Japanese flounder (Paralichthys olivaceus). Gen. Comp. Endocrinol. 297, 113547. doi: 10.1016/j.ygcen.2020.113547
Zhang N., Xu F., Guo X. (2014). Genomic analysis of the pacific oyster (Crassostrea gigas) reveals possible conservation of vertebrate sex determination in a mollusc. G3 (Bethesda) 4 (11), 2207–2217. doi: 10.1534/g3.114.013904
Zhang X., Yuan L., Li L., Jiang H., Chen J. (2016). Conservation, sex-biased expression and functional annotation of microRNAs in the gonad of amur sturgeon (Acipenser schrenckii). Comp. Biochem. Physiol. Part D Genomics Proteomics 18, 54–61. doi: 10.1016/j.cbd.2016.04.001
Zhang Y., Zhong Y., Guo S. Y., Zhu Y. F., Guo J., Fu Y. S., et al. (2021). CircRNA profiling reveals circ880 functions as miR-375-3p sponge in medaka gonads. Comp. Biochem. Physiol. D-Genomics Proteomics 38, 100797. doi: 10.1016/j.cbd.2021.100797
Keywords: mytilus coruscus, transcriptome, gonad, mRNA, miRNA, miRNA-mRNA interaction, DMRT4, miR-317
Citation: Wang M, Xia J, Jawad M, Wei W, Gui L, Liang X, Yang J-L and Li M (2022) Transcriptome sequencing analysis of sex-related genes and miRNAs in the gonads of Mytilus coruscus. Front. Mar. Sci. 9:1013857. doi: 10.3389/fmars.2022.1013857
Received: 07 August 2022; Accepted: 05 September 2022;
Published: 20 September 2022.
Edited by:
Zhiguo Dong, Jiangsu Ocean Universiity, ChinaReviewed by:
Yuehuan Zhang, Chinese Academy of Sciences (CAS), ChinaKaida Xu, Zhejiang Ocean University, China
Baoying Guo, Zhejiang Ocean University, China
Copyright © 2022 Wang, Xia, Jawad, Wei, Gui, Liang, Yang and Li. This is an open-access article distributed under the terms of the Creative Commons Attribution License (CC BY). The use, distribution or reproduction in other forums is permitted, provided the original author(s) and the copyright owner(s) are credited and that the original publication in this journal is cited, in accordance with accepted academic practice. No use, distribution or reproduction is permitted which does not comply with these terms.
*Correspondence: Jin-Long Yang, jlyang@shou.edu.cn; Mingyou Li, myli@shou.edu.cn
†These authors have contributed equally to this work and share first authorship