- 1Key Laboratory of Mariculture & Stock Enhancement in North China’s Sea, Ministry of Agriculture, Dalian Ocean University, Dalian, China
- 2Modern Agricultural Production Development Service Center of Dalian, Dalian, China
- 3Dalian Agriculture and Fishery Industry Group, Dalian, China
- 4Changhai County Economic Development Service Center, Dalian, China
Neptunea cumingii is a carnivorous snail with a very high market value, but it cannot be cultivated on a large scale at present due to the lack of an appropriate artificial feed. In this study, we fed N. cumingii four kinds of diets (Cristaria plicata meat, Chlamys farreri mantle, Mytilus edulis meat, and artificial feed) for 90 days and then measured growth indexes and digestive and immune enzyme indexes. We also subjected liver samples to transcriptome sequencing to detect significant differences among the four groups at the transcriptome level. The survival rate of N. cumingii fed with each type of feed was > 90%. The weight, shell length, shell height, and weight gain of the N. cumingii in the C. plicata meat group were significantly higher than those of the other treatment groups, while the values in the artificial feed group were significantly lower than those of the other treatment groups. The superoxide dismutase, catalase, amylase, protease, and lipase activities were higher in the stomach, intestine, and liver of snails in the C. plicata meat feeding group than in those of the other treatment groups, and they were significantly higher than those of the artificial feed group (p < 0.05); the opposite was true for malondialdehyde content. A total of 3407 differentially expressed genes (DEGs) were found in the M. edulis meat group vs. C. plicata meat group comparison. A total of 3422 DEGs genes were identified in the artificial feed group vs. C. plicata meat group comparison. A total of 3,929 DEGs were found in the C. plicata meat group vs. C. farreri mantle group comparison. Through Gene Ontology (GO) and Kyoto Encyclopedia of Genes and Genomes (KEGG) enrichment analysis, we found the DEGs in the liver of N. cumingii fed with the different diets were mainly enriched in the energy metabolism, growth, and immunity pathways. Our results indicated that among the four diets tested, C. plicata meat had the best effect on N. cumingii, and they provided an important reference for identification of suitable diets for the development of N. cumingii artificial breeding.
1 Introduction
Neptunea cumingii (Gastropoda, Probranchia, Neptunea, Mothidae) is a carnivorous snail, and it is the main economically important shellfish along the northern coast of China (Qi, 1979). Its meat is delicious, nutritious, and an ideal source of dietary protein and fat (Hao et al., 2016), and it is deeply loved by consumers. At present, the demand for N. cumingii is increasing, and natural sources can no longer meet the demand. Since the middle of the twentieth century, the world’s aquaculture industry has developed rapidly (Jiang, 2015), which has opened up new ways to produce aquatic species. Factory farming of N. cumingii has become necessary (Sun and Yang, 2011), but developing appropriate feed to optimize production remains an obstacle to large scale culture of this species.
Feed composition is very important for aquaculture animals, as the feed affects body growth as well as the activities of digestive and immune enzymes (Liao et al., 2018). The level of digestive enzyme activity affects the absorption of major nutrients such as fat, starch, and protein by the digestive tissue of aquatic animals, thereby affecting the growth of cultured organisms (Xu, 2006; Zhou et al., 2018). To a certain extent, the activity of immune enzymes can more accurately reflect the impact of different feeds on the immune ability of cultured organisms (Wang et al., 2012), which in turn affects their growth. In recent years, the development of high-throughput sequencing technology enables researchers to study the effect of food on animal growth and enzyme activities from a genetic perspective. In particular, transcriptome sequencing plays an important role in biological studies of gene expression and in differential expression analysis when the whole genome of the target species is unknown (Morozova et al., 2009). It is an efficient method to obtain massive amounts of genetic data in a short time and at low cost (Wang et al., 2018).
At present, the effect of feed on N. cumingii is poorly understood. Therefore, we studied the effects of four low-cost feed substitutes (Cristaria plicata meat, Chlamys farreri mantle, Mytilus edulis meat, and an artificial diet) on the growth and related digestive and immune enzyme activities of N. cumingii. We also used transcriptome sequencing technology to study the differences in the liver tissue of N. cumingii in the different diet groups. Our results can be used to develop a suitable feed for N. cumingii breeding and for industrialized marine aquaculture of this species.
2. Materials and methods
2.1 Ethics approval statement
The animals used in this study are unregulated invertebrates. Animal welfare and experimental procedures were carried out in accordance with the Laboratory animals-General requirements for animal experiment (National Standards of P. R. China, GB/T 35823-2018).
2.2 Experimental materials
The 480 N. cumingii used in the experiment were taken from the sea area of Zhangzidao, Dalian City, Liaoning Province, China. They were transported back to the North Key Laboratory of Marine Aquaculture, Ministry of Agriculture and Rural Affairs, Dalian Ocean University in a damp state. The samples were kept for 2 weeks in a 1,000 L culture tank with water temperature 19 ± 1°C, salinity 30 ± 1%, continuous aeration without feeding, and 50% water change every day (tank clean every 7 days). The specimens had a wet weight of 10.5 ± 2.0 g, shell length of 4.25 ± 0.2 cm, and shell width 2.35 ± 0.1 cm.
The artificial feed raw materials were fish meal (33%), soybean meal (33%), alpha starch (18%), dextrin (4%), fish oil (5%), calcium dihydrogen phosphate (3%), choline chloride (0.5%), multi-vitamin (1%), and multiple minerals (1.5%). Before preparing the feed, we fully crushed the raw materials, passed them through an 80-mesh sieve, and mixed them. After confluence, we poured the material into a mixer to concentrate and mix it thoroughly. After mixing, we poured the mixture into a basin, added fish oil, food attractant, and a little water, continued mixing, and then waited until the raw materials could be squeezed and kneaded into blocks. We poured the mixed raw materials into an extruder, heated and shaped it, and placed the formed feed in a cool place to dry. The natural feeds consisted of C. plicata, C. farreri mantle, and M. edulis.
2.3 Experimental method
2.3.1. Experimental setup and culture methods
The experiment contained four groups, each with three replicates of 40 snails, and each group was fed only one kind of feed. Each replicate group was cultured in a 300 L water tank for 90 days. The feed was provided every day at 9:00 and 17:00. Two hours after feeding, 50% of the water was changed and the residual feed was collected, dried, and weighed. Wash the water tank every 7 days. The growth indexes of N. cumingii in each group were measured and recorded every 15 days. After 90 days, three N. cumingii were randomly selected from each group. After dissection, their liver tissues were removed. Mixed samples were placed in liquid nitrogen and stored in a -80°C freezer for future analysis.
2.3.2. Determination of four kinds of feed nutrients
Proximate analysis of four kinds of feed were measured according to standard Association of Official Agricultural Chemists methods (AOAC, 1995). Crude protein content was determined by measuring nitrogen (N × 6.25) levels using the Kjeldahl method following acid digestion with an auto Kjeldahl System (Kjelflex K-360; BUCHI Labortechnik AG, Flawil, Switzerland). Moisture content was measured by freeze-drying samples for 48 h in a vacuum freeze dryer (Christ Beta 2–4 LD plus LT, Marin Christ Corporation, Osterode, Ger-many). Ash level was examined through incineration at 550 °C for 24 h in a muffle furnace. Content of amino acid were carried by an automatic amino acid analyzer (Agilent-1100, Agilent Technologies Co., Ltd., Santa Clara, CA, USA) as described in previous study (Ren et al., 2017). The fatty acid composition of experimental diets was determined by series gas chromatography (Agilent 7820a, Agilent Technologies Co., Ltd., Santa Clara, CA, USA) according to Li et al. (2016). Three technical replicates were designed in this experiment.
2.3.3 Digestive enzymes and immune enzyme
Three individuals per replicate were selected for enzyme activity assays. Intestine, stomach and liver tissues were removed from N. cumingii and immediately frozen in liquid nitrogen.
Intestine, stomach and liver were shredded and weighed (accurate to 0.01 g), diluted 10 times with distilled water, centrifuged at 4°C for 10 min (5000 r/min), after which each supernatant was placed in a centrifuge tube, and stored at 4°C. The results were determined within 24 h. Amylase, lipase, protease activity (Long et al., 2015), superoxide dismutase (SOD) (Xu and Pan, 2014), catalase activity (CAT) (Kim et al., 2015), and malondialdehyde (MDA) (Yin et al., 2018) were determined using commercial assay kits (Nanjing Jiancheng Bioengineering Institute, Nanjing, China).
2.3.4 Liver transcriptome
One gram of the liver tissue frozen in liquid nitrogen were removed for transcriptome sequencing.
Total RNA was extracted using the mirVana miRNA Isolation Kit (Ambion) following the manufacturer’s protocol. RNA integrity was evaluated using the Agilent 2100 Bioanalyzer (Agilent Technologies, Santa Clara, CA, USA). The samples with RNA Integrity Number (RIN) ≥ 7 were subjected to the subsequent analysis. The libraries were constructed using TruSeq Stranded mRNA LTSample Prep Kit (Illumina, San Diego, CA, USA) according to the manufacturer’s instructions. Then these libraries were sequenced on the Illumina sequencing platform (HiSeqTM 2500 or Illumina HiSeq X Ten) and 125bp/150bp paired-end reads were generated.
The transcriptome sequencing and analysis were conducted by OE biotech Co., Ltd. (Shanghai, China). Raw data (raw reads) were processed using Trimmomatic. The reads containing ploy-N and the low quality reads were removed to obtain the clean reads. After removing adaptor and low quality sequences, the clean reads were assembled into expressed sequence tag clusters (contigs) and de novo assembled into transcript by using Trinity (version: 2.4) in paired-end method. The longest transcript was chosen as a unigene based the similarity and length of a sequence for subsequent analysis.
The function of the unigenes was annotated by alignment of the unigenes with non-Redundant Protein Sequence Database (NR), SwissProt, and Clusters of orthologous groups for eukaryotic complete genomes (KOG) databases using Blastx with a threshold E-value of 10−5. The proteins with the highest hits to the unigenes were used to assign functional annotations thereto. Based on the SwissProt annotation, Gene ontology (GO) classification was performed by the mapping relation between SwissProt and GO term. The unigenes were mapped to the Kyoto Encyclopedia of Genes and Genomes (KEGG) database to annotate their potential metabolic pathways.
FPKM and read counts value of each unigene was calculated using bowtie2 and eXpress. DEGs were identified using the DESeq (2012) functions estimateSizeFactors and nbinomTest. P value < 0.05 and foldChange >2 was set as the threshold for significantly differential expression. Hierarchical cluster analysis of DEGs was performed to explore transcripts expression pattern. GO enrichment and KEGG pathway enrichment analysis of DEGs were respectively performed using R based on the hypergeometric distribution
2.3.5 Data analysis
We used SPSS 17.0 software (Chicago, IL, USA) for statistical analysis of experimental data and univariate analysis (analysis of variance, Tukey’s test) to assess significant differences among groups (p < 0.05). The results are expressed as the mean ± standard deviation.
3. Results
3.1 Nutrient composition of different feeds
Figure 1 shows the nutrient composition of the four different feeds tested in this study. The water content of M. edulis meat was the highest (71.32%), and that of the artificial feed was the lowest (10.58%). The crude protein (dry weight; 55.16%) and crude fat (6.08%) contents of C. plicata meat were the highest. The ash content of the C. farreri mantle was the highest (13.74%) and that of M. edulis meat was the lowest (1.46%).
Supplementary Table 1 shows the amino acid contents of the four different feeds. Eighteen types of amino acids were detected in the C. farreri mantle, M. edulis meat, and artificial feed, including eight essential amino acids, eight nonessential amino acids, and five flavor amino acids. Seventeen kinds of amino acids were detected in C. plicata meat, as cysteine was absent.
The fatty acid content of the four different feeds is shown in Supplementary Table 2. We detected 23 fatty acids in C. plicata meat, including nine saturated fatty acids (SFAs), six monounsaturated fatty acids (MUFAs), and eight polyunsaturated fatty acids (PUFAs), whereas we found 15 fatty acids in C. farreri mantle, including five SFAs, three MUFAs, and seven PUFAs. M. edulis meat contained 17 fatty acids, including seven SFAs, four MUFAs, and six PUFAs. We detected 23 fatty acids in the artificial feed, including eight SFAs, 7 MUFAs, and eight PUFAs. The fatty acid content of C. plicata meat and the artificial feed group was relatively rich. Among the four groups of SFAs, palmitic acid (C16:0) and stearic acid (C18:0) were the most abundant.
3.2 Growth and survival rate differences for N. cumingii cultured using different feeds
Table 1 shows the weight changes of N. cumingii fed with different diets. After 90 days of feeding, the N. cumingii fed with C. plicata meat showed the best growth, with wet weight, shell width, and shell height increases of 4.95 g, 0.48 cm, and 0.95 cm, respectively. The artificial feed group had the worst growth, with wet weight, shell width, and shell increases of 3.06 g, 0.31 cm, and 0.64 cm, respectively. Significant differences in the growth of N. cumingii fed with different diets were detected (p < 0.05). The survival rates of N. cumingii fed with different diets were all > 90%, and differences among groups were not statistically significant.
3.3 Effects of different feeds on immunoenzyme activities of related tissues of N. cumingii
The immunoenzyme activity of N. cumingii differed significantly among different groups in the same tissue (Table 2). The highest SOD activity was found in the intestine tissue of M. edulis meat group (p < 0.05). The lowest MDA activity was found in the intestine tissue of C. plicata meat group (p < 0.05). The M. edulis meat group had the highest catalase CAT activity (0.44 U/mg protein) in the intestine tissue, and values for the other two groups were significantly higher than that of the artificial feed group (p < 0.05). The highest SOD activity was found in the stomach tissue of C. plicata meat group (p < 0.05). The highest MDA activity was found in the stomach tissue of M. edulis meat group (p < 0.05). The lowest SOD activity was found in the liver tissue of artificial feed group (p < 0.05). The highest MDA activity was found in the liver tissue of C. plicata meat group (p < 0.05). The lowest CAT activity was found in the liver tissue of artificial feed group (p < 0.05).
There were also some differences in the immunoenzyme activity of N. cumingii in different tissues in the same group. In the C. plicata meat group and the artificial feed group, SOD enzyme activity in stomach tissue was higher than that in intestine and liver tissue. In the C. farreri mantle group and M. edulis meat group, SOD enzyme activity in intestine tissue was higher than that in stomach and liver tissue. In the C. plicata meat group, C. farreri mantle group, and artificial feed group, the MDA level in liver tissue was higher than that in intestine and stomach tissue. In the M. edulis meat group, the MDA in the stomach was higher than that in intestine and liver tissue. In the C. plicata meat group, C. farreri mantle group, and M. edulis meat group, the CAT enzyme activity in liver tissue was higher than that in intestine and stomach tissue. In the artificial feed group, the CAT activity in intestine tissue was lower than that in stomach and liver tissue.
3.4 Effects of different feeds on digestive enzyme activities of related tissues of N. cumingii
The digestive enzyme activities of N. cumingii differed significantly among the different feed groups (Table 3). The highest amylase activity was found in the intestine tissue of M. edulis meat group (p < 0.05). The C. plicata meat group had the highest lipase activity (22.48 U/mg protein) in the intestine tissue, and the values for the C. farreri mantle group and artificial feed group were significantly lower (p < 0.05). However, the values for the C. plicata meat group and M. edulis meat group did not differ significantly (p > 0.05). In the stomach tissue, the M. edulis meat group had the highest amylase activity (0.80 U/mg protein), followed by slightly lower values for the artificial feed group and C. plicata meat group (p < 0.05). The lowest amylase activity was found in the stomach tissue of C. farreri meat group (p < 0.05). The lowest lipase activity was found in the stomach tissue of artificial feed group (p < 0.05). In the liver tissue, the amylase activity of the artificial feed group was highest (0.56 U/mg protein. It was significantly higher than that of the C. plicata meat group and M. edulis meat group (p < 0.05), but it did not differ significantly from that of the C. farreri mantle group (p < 0.05). The lowest lipase activity was found in the liver tissue of artificial feed group (p < 0.05).
In all three tissues, the protease activity of the artificial feed group was significantly lower than that of the other three groups (p < 0.05). In intestine, stomach, and liver tissue, the C. plicata meat group had the highest protease activity (44.30, 45.72, and 42.42 U/mg protein, respectively). The protease activities in the liver tissue of the C. plicata meat group and M. edulis group did not differ significantly from each other but were significantly higher than that of the other two groups.
There were also some differences in N. cumingii digestive enzyme activities in different tissues in the same group. In the C. plicata meat group and M. edulis meat group, the amylase activity in stomach and intestine tissue was higher than that in liver tissue. In the C. farreri mantle group, the amylase activity in intestine tissue was higher than that in stomach tissue and liver tissue. In the artificial feed group, the amylase activity in stomach tissue was higher than that in intestine tissue and liver tissue. In all four groups, the lipase activity in liver tissue was higher than that in stomach and intestine tissue. In the three bivalve groups, the protease activity in different tissues was similar. In the artificial feed group, the protease activity in the intestine tissue was the highest.
3.5 Transcriptome results
3.5.1 Transcriptome sequencing
We used Illumina HiSeq X Ten to generate the liver tissue transcriptome of N. cumingii fed with different feeds. Four sequencing libraries were prepared: C. plicata meat group (CP, 53,252,704 raw reads), artificial feed group (AF, 56,626,668), C. farreri mantle group (CF, 516,06,304), and M. edulis meat group (ME, 50,252,186). Filtering the raw data to remove low-quality sequences with linkers yielded 518,782,60, 55,222,734, 49,875,376, and 48,580,748 valid reads, respectively. The Q30 of each sample was greater than 90%, which indicated that subsequent analysis and research could be conducted. Table 4 shows the data quality and comparison results of the sequencing samples.
3.5.2 Common function database notes
The unigene gene sequences were aligned with the Nr, egg NOG, KOG, PFAM, SwissProt, GO, and KEGG databases to obtain the corresponding annotation information. Supplementary Table 3 shows the genes and percentages annotated in each database.
3.5.3 Analysis of DEGs
Comparative analysis of DEGs in liver tissue of N. cumingii fed with four different diets was conducted by screening for DEGs (P-value < 0.05 and |log2FC| > 1) in the following comparison groups: ME vs. CP, AF vs. CP, and CP vs. CF. The most DEGs were found in the CP vs. CF comparison, with 1260 up-regulated and 2669 down-regulated genes. The ME vs. CP comparison yielded the least DEGs, with 1811 up-regulated and 1685 down-regulated genes. Differential genes in each group are shown in the Table 5. Figure 2 shows the volcano plot of up-regulated and down-regulated DEG expression in each group.
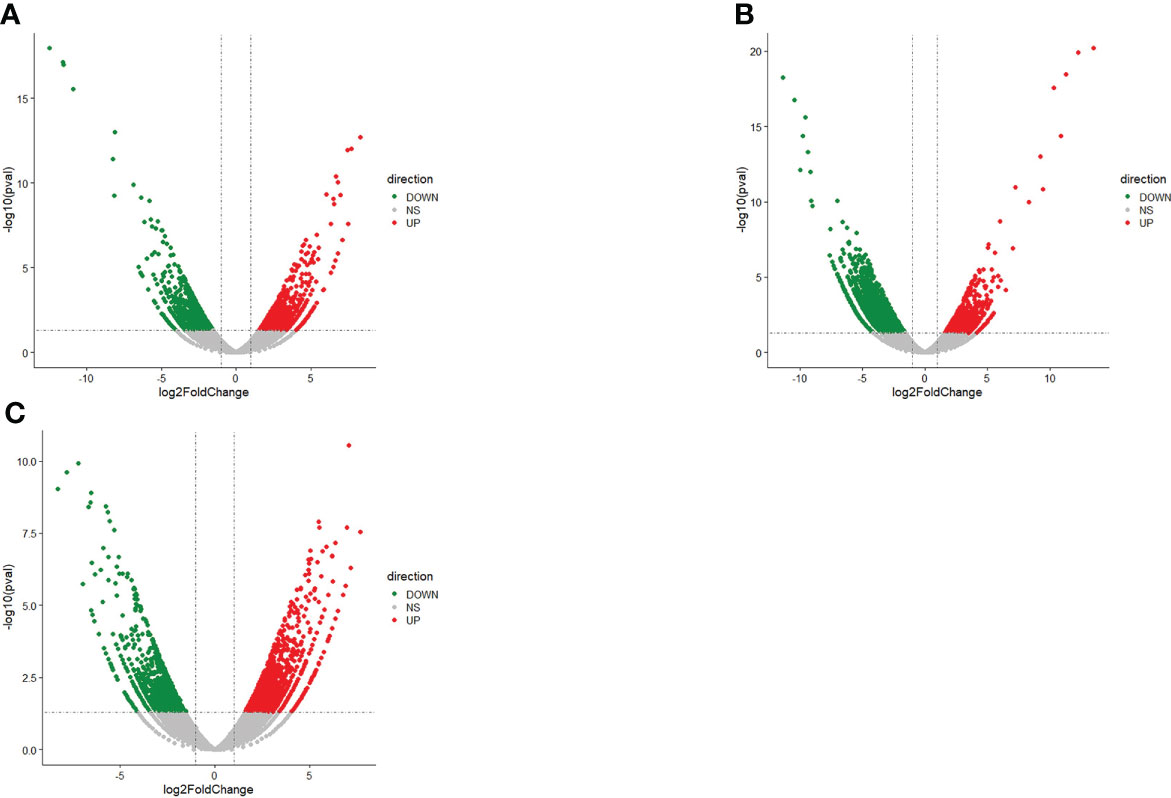
Figure 2 Volcano plot of DEGs. (A): AF vs CP: Volcano plot of DEGs. (B): CP vs CF: Volcano plot of DEGs. (C): ME vs CP: Volcano plot of DEGs.
3.5.4 GO functional enrichment analysis of DEGs
To evaluate the physiological regulation involved in differentially expressing genes in the liver of N. cumingii during adaptation to different feeds, we performed GO enrichment analysis on the DEGs to describe their functions. Unigenes were annotated into three categories (biological process (BP), cellular component (CC), and molecular function (MF)) in the GO classification system. Figure 3 is a schematic diagram showing the top 30 terms in the GO enrichment analysis for the three comparisons.
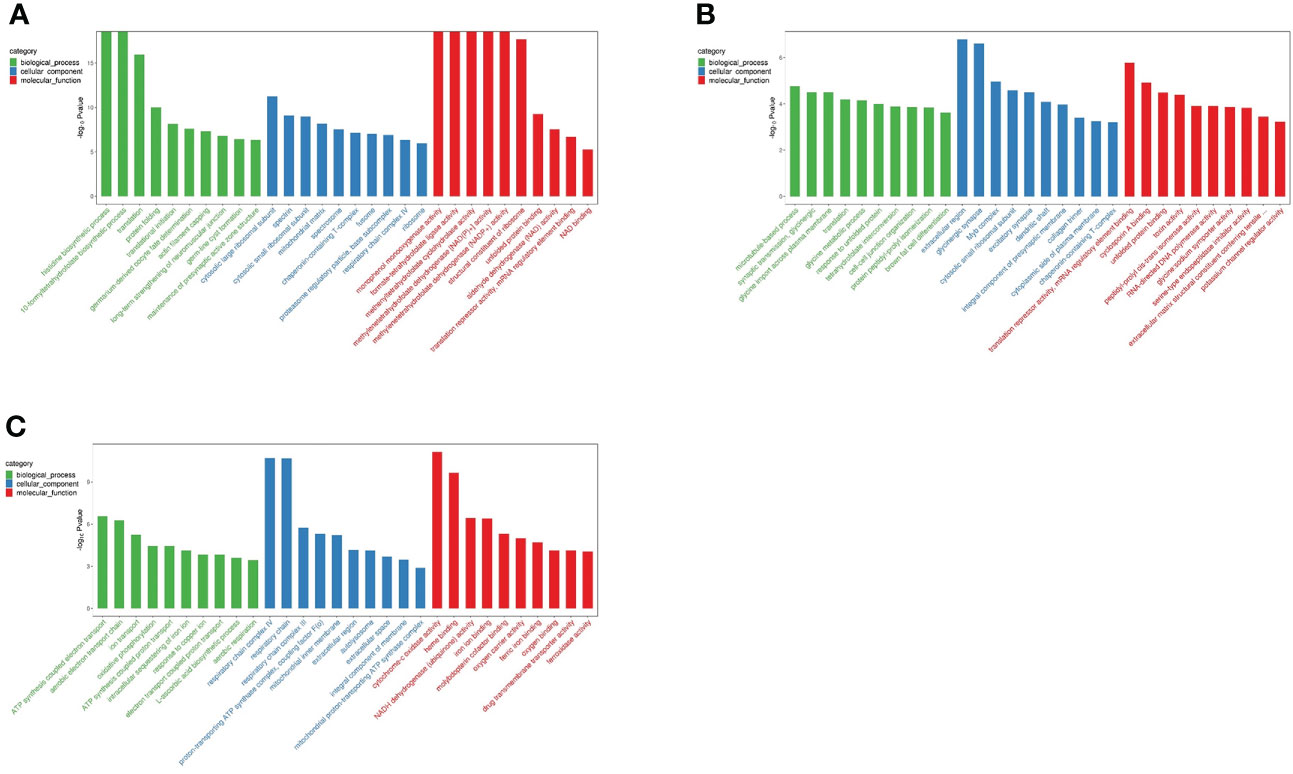
Figure 3 Top 30 GO enrichment terms. (A): CP vs CF: Top 30 GO Terms (B): AF vs CP: Top 30 GO Terms (C): ME vs CP: Top 30 GO Terms.
In total, 1,182 DEGs were annotated in the GO classification for the CP vs. CF comparison, and the significantly enriched gene functions in the BP category included histidine biosynthesis, 10-formyltetrahydrofolate biosynthesis, and translation. The significantly enriched gene functions in the CC category included cytoplasmic large ribosomal subunit, spectrin, and cytoplasmic small ribosomal subunit. Those significantly enriched in the MF category included monophenol mono oxygenase activity, formate-tetrahydrofolate ligase activity, methyltetrahydrofolate cyclohydrolase activity, and methylenetetrahydrofolate dehydrogenase [NAD(P)+] activity.
For the AF vs. CP comparison, 416 DEGs were annotated in the GO classification, and significantly enriched gene functions in the BP category included microtubule-based processes, synaptic transmission, and glycine trans-plasma membrane import. The enriched gene functions in the CC category included extracellular domain and glycinergic synapses, and those in the MF category included translation inhibitory activity, mRNA regulatory element binding, and cyclosporine A binding.
In total, 422 DEGs were annotated in the GO classification for the ME vs. CP comparison. Significantly enriched gene functions in the BP category included ATP synthesis coupled electron transport and aerobic electron transport chain, and those in the CC category included respiratory chain complex IV and respiratory chain. In the MF category, the most significantly enriched gene functions were cytochrome-c oxidase activity and heme binding.
3.5.5 KEGG enrichment analysis of DEGs
To further explore the biological functions of DEGs in N. cumingii fed with different diets, we used the KEGG database and conducted pathway analysis of DEGs in different groups. The DEGs were annotated into the following six metabolic pathways: Organismal Systems, Metabolism, Human Diseases, Genetic Information Processing, and Environmental Information Processing, Cellular Processes. Figure 4 shows the 20 most significantly enriched pathways for the three comparisons.
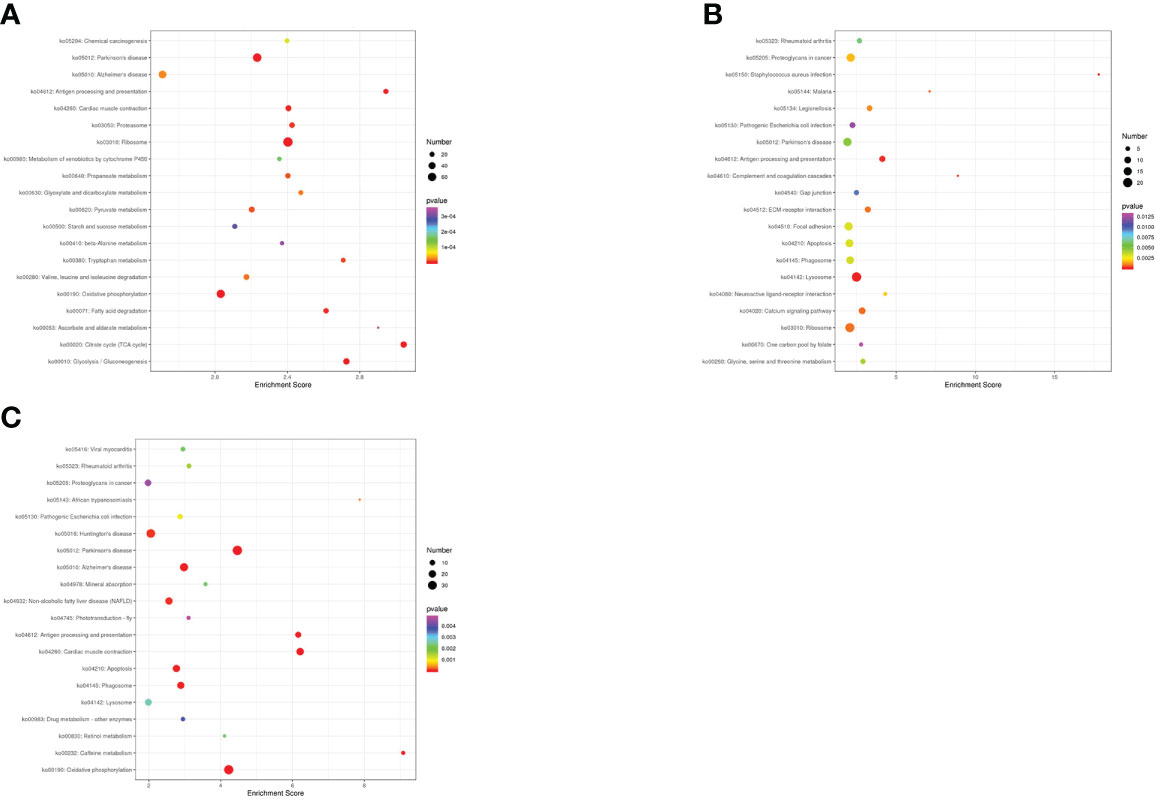
Figure 4 Top 20 KEGG enrichment terms. (A): CP vs CF: KEGG enrichment top 20 (B): AF vs CP: KEGG enrichment top 20. (C): ME vs CP: KEGG enrichment top 20.
For the CP vs. CF comparison, DEGs were enriched in 256 KEGG metabolic pathways and significantly enriched in the Citrate cycle (TCA cycle), Glycolysis/Gluconeogenesis, and Oxidative phosphorylation. For the AF vs. CP comparison, DEGs were enriched in 236 KEGG metabolic pathways and significantly enriched in Antigen processing and presentation, Lysosome, and Apoptosis. For the ME vs. CP comparison, DEGs were enriched in 210 KEGG metabolic pathways and significantly enriched in Antigen processing and presentation, Apoptosis, Phagosome, and Lysosome.
4. Discussion
4.1 Effects of different feeds on the growth of N. cumingii
High quality feed can promote the growth and development of the digestive organs of aquatic animals, thereby improving their digestive capacity (Chen et al., 2017). Studies have shown that the preference of aquatic animals for food is related to the type of feed, and it also affects their growth rate (Xu, 2006; Zhang et al., 2009; Liao et al., 2018; Zhou et al., 2018). Therefore, the preference of snails for feed affects their growth performance and mortality (Zheng et al., 2013). In this study, there were significant differences in the growth and development of N. cumingii among different feeds (p < 0.05). We found that N. cumingii fed with C. plicata meat had the fastest growth in terms of body weight, shell length, and shell height, and the snail’s feeding ability was also best with this food type. In contrast, the growth of snails in the artificial feed group was slowest. It is generally believed that different growth patterns of shellfish cultured under different feed conditions are related to the main nutrients, such as protein and fat, in the feed (Zhong et al., 2012). The nutrients contained in the four kinds of feeds tested in our study differed. C. plicata had the highest protein and fatty acid content, making it a high-quality high-protein feed (Li, 1993), whereas the artificial feed had the lowest levels. Cys was not detected in Cristaria plicata meat, probably because the amount of Cys in the body was very low (Ge et al., 2006). Among the four feeds, the price of C. plicata is relatively low. Moreover, N. cumingii fed C. plicata meat grew the fastest, which would translate to a shortened breeding time to market and greater higher economic benefits. Further research is needed to better understand the effect of the feed factor and price on the breeding efficiency of N. cumingii. Among the four kinds of feeds tested in our study, C. plicata meat was the most suitable feed for N. cumingii.
Protein is the material basis for the survival of aquatic animals. Enzymes that play an important role in the body’s metabolism, hormones that coordinate functions, as well as immune cytokines and antibodies are mainly composed of proteins, thus they play an important role in the growth and metabolism of animals (Xu, 2006). Studies have shown that different fat and protein sources in the feed had significant effects on the growth and development of snails (Luo et al., 2014; Du et al., 2014). For example, Luo et al. studied the effect of different protein contents in compound feed on the growth of Babylonia areolata juveniles and found that the suitable protein level was 42.78% (Luo et al., 2014). Xu et al. found that the suitable protein content in B. areolata feed was 36.47–43.10% (Xu et al., 2006). However, little is known about the protein requirements of N. cumingii. In our study, the protein content of the four feed types differed, and the protein content of C. plicata meat was much higher than 43.10%. We found that N. cumingii fed with C. plicata meat had the highest protease activity in the intestine, stomach, and liver compared to the other three feed types. The high protein content of C. plicata meat promoted N. cumingii to produce more protease, which in turn affected the feeding and growth of N. cumingii. Our results indicated that the higher protein content resulted in a faster growth rate.
Fat is a very important energy source for aquatic animals such as carnivorous marine fish, and the optimal requirement for fat is 8–16% (Wang et al., 2008). Xu et al. found that the fat content of 7.78-10.74% is the appropriate demand for B. areolate (Xu et al., 2006). Wang et al. fed juvenile B. areolata with five feeds containing different fat contents for 60 days and found that the optimal fat content was 10.01% (Wang et al., 2008). In our experiment, the fat content of C. plicata meat was 6.08%, which was significantly higher than that of the other feeds (p < 0.05), and the snails in this group grew the fastest. The C. plicata meat also contained the most types of fatty acids among the four kinds of feeds, and the snails in this group had the highest lipase activity in the intestine, stomach, and liver. Fat is an important nutritional element for carnivorous aquatic animals, and it has important physiological functions. In addition to being an energy source for animals, it provides fatty acids, especially essential fatty acids, needed for growth (Du et al., 2014). Among the four kinds of feeds tested, C. plicata meat was richest in fat content. We speculate that the higher fat content of this feed induced N. cumingii to produce more lipase, promoted digestion and absorption, and affected growth.
N. cumingii was not good at ingesting artificial feed. Snails only ingested artificial feed 2 hours after feeding, and snails in this group grew slowly. Similar to a study of B. areolata fed with compound feed (Xian et al., 2016), we found that our artificial feed had low water content. When the feed was soaked in water for a long time, it loosened and dissolved, which resulted in nutrient loss and polluted water. As a result, the utilization of artificial feed was not ideal. N. cumingii mainly relies on smell and touch for feeding, and it prefers benthic shellfish (mainly bivalves) or dead fish in nature (Wang et al., 2007). C. plicata and M. edulis are natural feeds that are suitable for N. cumingii in both shape and smell, and N. cumingii fed better on them than on artificial feed and C. farreri mantle.
Different feeds have different impacts on the growth and survival of snails (Fan et al., 2020). Xue et al. studied the morphological parameters of B. areolata under starvation and found that only one snail died after starvation for 120 days (Xue et al., 2010), indicating that the biological adaptability of these snails is very strong. In our experiment, the survival rate of N. cumingii was > 90% in all four experimental groups. This result suggests that N. cumingii is also very adaptable to the environment.
4.2 Effects of different feeds on digestive enzymes and antioxidant indexes of N. cumingii
The antioxidant level of the animal body reflects its health status to a certain extent (Li et al., 2008). Studies have shown that reactive oxygen species (ROS) may cause oxidative damage to lipids and proteins in animal cells (Li et al., 2008). SOD and CAT can catalyze the elimination of excess ROS in organisms (Qiao et al., 2011). The two are interrelated and work to maintain the balance of oxidation and anti-oxidation, thereby improving the body’s immunity and disease resistance (Ko et al., 2004). Their activities also indicate the strength of the body’s ability to scavenge reactive oxygen free radicals (Hong et al., 2007), with higher activities indicating greater scavenging ability (Muñoz et al., 2000). MDA is the product of the peroxidation reaction between ROS and lipids, and it has cytotoxicity (Morales et al., 2004). A higher MDA content indicates greater cytotoxicity (R., 1990). In this study, the activities of CAT and SOD in the body of N. cumingii were higher in C. plicata meat group and M. edulis meat group than in the other two groups, indicating that the antioxidant capacity of N. cumingii was enhanced after ingesting these feeds. The antioxidant capacity of the digestive system of N. cumingii was greater in these two groups, allowing better decomposition of the peroxides, protection of internal organs, and promotion of immunity. In contrast, the activities of CAT and SOD in the artificial feed group were significantly lower than those in the other three groups, indicating weaker antioxidant capacity of snails in this group. The results of this experiment showed that different feeds had different effects on the activities of SOD and CAT in the body, and the observed changes in these two antioxidant enzymes were similar. This result indicated that ingestion of the different diets unbalanced the metabolism of ROS in the body, which led to changes in the activities of CAT and SOD. C. plicata meat and M. edulis meat have high protein content, and N. cumingii had high CAT and SOD activities after ingesting these two feeds. This result is consistent with that reported by Jiang et al. (Jiang et al., 2013), who found that increasing the protein content in the feed improved the antioxidant capacity of crustaceans.
Studies have also shown that animals could reduce the content of MDA in tissues by increasing the enzyme activities of SOD and CAT (Li et al., 2008). We found that the MDA content of N. cumingii in the C. plicata meat group was lowest among the diets tested. This result suggests that snails ingesting C. plicata meat increased the activities of SOD and CAT in tissues, thereby inhibiting the peroxidation of lipid components, which resulted in a decreased MDA concentration in tissues. However, the artificial feed group showed the opposite trend. In our experiment, the C. plicata meat and M. edulis meat contained high amounts of isoleucine, and N. cumingii had high SOD activity and low MDA content after ingesting these two feeds. Huang et al. (Huang et al., 2017) reported that appropriate branched-chain amino acid content effectively improved the serum SOD activity of Trachinotus ovatus, thereby removing free radicals in the fish. Thus, free radical-induced damage to unsaturated fatty acids and lipid peroxidation were reduced, and the production of MDA, a lipid peroxidation product, was also reduced.
The ability of aquatic animals to digest food mainly depends on the presence of appropriate enzymes in the body, and the activity of digestive enzymes reflects the strength of animal metabolism and digestion (Cahu et al., 1998). Studies have shown that amylase, protease, and lipase digest starch, protein, and fat, respectively, and their activities are related to the nutrient content of the feed they eat (Yang et al., 2003). In our experiment, the results show that the amylase activity of intestine and stomach is higher than that of liver when under the same feeding condition. The amylase activity in intestinal and stomach of M. edulis meat group was higher than that in the other treatment groups. This is consistent with the results obtained by Fan et al. (2011) in the study of Trachinotus ovatus, which may be caused by the different functions of different organs in the digestive system of aquatic animals. The high amylase activity in the intestine and stomach of N. cumingii indicates that the intestine and stomach are the main organs for starch absorption and digestion. Appropriate carbohydrate levels in the feed can reduce the chance of protein being used as an energy source (LUO et al., 2005), and they also can promote the growth of snails to a certain extent (Zheng et al., 2013). Liu et al. (Liu et al., 2018) found that the protease content of the organism was closely related to the protein content and type of food, which to a certain extent showed that different types of food significantly affected the protease activity in the organism. In our study, the protease contents in the intestine, stomach, and liver of N. cumingii were significantly lower in the artificial feed group than in the other three feed groups, which was due to the relatively high content of animal protein in the biological feeds. This indicated that N. cumingii promoted the secretion of protease after ingesting high amounts of animal protein. Yang et al. (Yang et al, 2003) found that after Monopterus albus ingested some trace active substances present in live food, secretion of protease was promoted, resulting in higher protease activity in the live food group. In our study, the C. plicata meat group had the highest lipase activity in all three tissues tested. The fat content of C. plicata meat was significantly higher than that of the other three feeds, which indicated that the lipase activity of N. cumingii was positively correlated with the fat content of the feed. Similarly, Chen reported that Babylonia lutosa lipase activity was positively correlated with the fat content of the food eaten (Chen, 2015). Although the artificial feed had high fat and protein content, the activities of the three enzymes in N. cumingii were low. This may be because N. cumingii is a carnivorous snail and the intake of artificial feed is poor (Shen et al., 2009), resulting in relatively weak enzyme activities in the body. Further in-depth studies are needed to determine how feed affects the secretion of relevant digestive enzymes.
4.3 Transcriptome analysis
Transcriptome sequencing can be used to detect gene expression levels of species under arbitrary conditions, screen and analyze DEGs, and explore related metabolic pathways to understand their physiological regulatory mechanisms (Mu et al., 2020). We used RNA-Seq technology to assess the transcriptome trends in the liver of N. cumingii fed different diets. Numerous studies have shown that the liver is involved in important physiological functions. The liver is the largest digestive gland in mollusks, and it is generally believed that their digestion and absorption occurs in the liver (Owen, 1970). Transcriptomic data provide a resource for further studies of the molecular mechanisms underlying the metabolism and immune response of N. cumingii to different types of feed. In this study, DEGs were mainly identified as genes related to energy metabolism, immune response, and molecular function, and the results suggested that significant changes in tissue structure and function may have occurred in snails after they ingested different feeds. The KEGG database contains abundant pathway information, which helps explain the biological function of genes at the system level (Chen et al., 2011). In our study, KEGG pathway enrichment analysis showed that DEGs were mostly concentrated in Metabolism and Organic System processes, which are very important for normal life activities of the body.
In the KEEG pathway enrichment analysis of the CP vs. CF groups, the DEGs were mainly enriched in glycolysis and pyruvate metabolism. Glycolysis is an important glucose metabolism pathway, and pyruvate kinase is an important rate-limiting and regulating enzyme in the process of glycolysis (Dai et al., 2013). Phosphoenolpyruvate carboxykinase (PEPCK) gene was found in glycolysis and pyruvate metabolism pathways. In the CP vs. CF group, PEPCK gene was significantly down-regulated in the glycolysis and pyruvate metabolism pathways. PEPCK catalyzes the conversion of oxaloacetate to phosphoenolpyruvate to generate glucose, which is the first and most important reaction link in the process of gluconeogenesis (Jianqi et al., 2009). PEPCK plays an important role in the regulation of energy metabolism in aquatic animals. For example, a high sugar diet can significantly reduce the mRNA expression and enzymatic activity of PEPCK in Sparus aurata and Cyprinus carpio, while a low sugar diet can enhance the mRNA expression of PEPCK (Panserat et al., 2002). Under adverse conditions, PEPCK gene expression in Pinctada fucata martensii is increased to produce more glycogen so that the organism can survive (Chen et al., 2022). When glucose content in the body is insufficient, the PEPCK activity increases, whereas it decreases when the content is sufficient (Wang et al., 2002). In our study, the amylase activity in the liver of N. cumingii in the CP group was lower than that in the CF group. Amylase catalyzes the hydrolysis of starch and glycogen to generate glucose and maltose. Our result indicated that the glucose content in the liver of N. cumingii in the CP group was high, which in turn affected the expression of glycolysis genes. However, N. cumingii in the CF group increased gluconeogenesis and the body’s glycogen content to adapt to energy consumption. Thus, the expression of the PEPCK gene in N. cumingii was significantly correlated with its feed intake. However, whether the PEPCK gene in N. cumingii has functions similar to those of other species requires further study.
In the KEEG pathway enrichment analysis of the AF vs. CP and ME vs. CP comparisons, the DEGs were mainly enriched in lysosomes. Lysosomes play an important role in digestion and are an important common intersection of signal transduction pathways, including cell autolysis, defense, and utilization of certain substances (Lamming and Bar-Peled, 2019). Cathepsin L(CTSL) gene was found in lysosomal pathway. It was significantly down-regulated in lysosomal pathways in AF vs. CP and ME vs. CP comparisons. Cathepsins are a class of proteolytic enzymes that mainly exist in lysosomes and participate in lysosome-mediated protein digestion and transformation together with other proteases (Mellman, 1996). CTSL is widely distributed in the lysosome or cytoplasm of various organisms and plays an important role in the process of proteolysis (Turk et al., 2000). In Argopecten irradians, CTSL is likely to be functionally similar to its homologous protein and has the ability to hydrolyze a variety of muscle-related proteins (Li et al., 2011). In a metabolic study of the growth efficiency of M. edulis, researchers found that CTSL has higher activity in the tissues that store nutrients, indicating that it functions to degrade proteins to provide energy (Hawkins and Day, 1996). In our study, CTSL gene in the AF vs. CP and ME vs. CP groups was significantly down-regulated, while the protease activity of the N. cumingii liver in the CP group was significantly higher than that in the AF and CF groups. The protein content of C. plicata meat was significantly higher than that of C. farreri mantle and artificial feed, thus CTSL may be involved in protein degradation as in the liver of N. cumingii to provide energy for growth and development.
Venier et al. studied Mytilus galloprovincalis in a stress breeding environment containing heavy metals and found that the mRNA expression of CTSL was significantly higher than that of the control group, suggesting that it may be involved in the immune stress response (Venier et al., 2006). After P. fucata was infected by Vibrio alginolyticus, the expression of the CTSL gene in hemolymph was significantly up-regulated, indicating that it is involved in the body’s immune defense against the invasion of external pathogens (Wang et al., 2013). When P. fucata was stimulated by V. alginolyticus or lipopolysaccharide, the expression of CTSL1 and CTSL2 gene mRNAs in digestive glands was significantly up-regulated, indicating that these genes are involved in the innate immune response of P. fucata (Ma et al., 2010). Thus, CTSL may play an important role in the defense response of invertebrates to clear pathogens. The liver of shellfish secretes a variety of different enzymes to hydrolyze pathogenic microorganisms and participate in digestive and defense functions (Peng et al., 2016). We found that the activities of liver immune enzymes SOD and CAT in the CP group were significantly higher than those in the ME and AF groups. The changes in liver physicochemical factors caused by different diets likely altered the activities of enzymes related to disease resistance. CTSL gene in the AF vs. CP and ME vs. CP groups was significantly down-regulated. It may indicate that when N. cumingii ingests C. plicata meat, it activates the lysosomal pathway, and CTSL participates in immune regulation and plays a role in eliminating bacteria. However, the specific roles and related mechanisms of the CTSL gene in N. cumingii require further study.
5. Conclusions
In summary, we found that different feeds had different effects on the growth performance and activities of digestive and immune enzymes of N. cumingii. C. plicata meat had a better effect on growth than the other feeds, and the effect of artificial feed was slightly worse. We successfully constructed and sequenced the liver transcriptome of N. cumingii, which provided transcriptomic data for further study of the related signaling pathways at work after N. cumingii ingested the different feeds. Our results provided a basis for elucidating the gene expression levels of N. cumingii cultured using different feeds, and they could be applied to artificial breeding studies and to formulating an effective compound feed for use when culturing this species.
Data availability statement
The datasets presented in this study can be found in online repositories. The names of the repository/repositories and accession number(s) can be found below: https://www.ncbi.nlm.nih.gov/, PRJNA866477.
Author contributions
ZH and YC designed the study. JZ, ZL, and QC performed the study. JM, XW, and XG analyzed the data. XG wrote the manuscript. All authors contributed to the article and approved the submitted version.
Funding
This study was supported by funds from National Natural Science Foundation of China (42076101), General project of Education Department of Liaoning Province (LJKZZZ0700), Project of Liaoning Provincial Natural Resources Department and Dalian key Research and Development Project (2021YF16SN015).
Acknowledgments
The authors wish to express thanks to the staffs of Key Laboratory of Mariculture & Stock Enhancement in North China’s Sea, Ministry of Agriculture, P.R. China for their help with the experiment. The authors are also grateful to the reviewers for the great elaboration of the manuscript through their critical reviewing and comments. In addition, the author would like to thank the International Science Editing Company for helping to improve the language ability of this article.
Conflict of interest
The authors declare that the research was conducted in the absence of any commercial or financial relationships that could be construed as a potential conflict of interest.
Publisher’s note
All claims expressed in this article are solely those of the authors and do not necessarily represent those of their affiliated organizations, or those of the publisher, the editors and the reviewers. Any product that may be evaluated in this article, or claim that may be made by its manufacturer, is not guaranteed or endorsed by the publisher.
Supplementary material
The Supplementary Material for this article can be found online at: https://www.frontiersin.org/articles/10.3389/fmars.2022.1013180/full#supplementary-material
References
AOAC (1995). Official methods of analysis of AOAC International. In Cunniff P. (Ed.), Ofcial analytical chemists (16th ed., p. 1141). Arlington, VA, USA: AOAC International.
Cahu C. L., Infante J., Péres A., Quazuguel P., Gall M. (1998). Algal addition in sea bass (Dicentrarchus labrax) larvae rearing: effect on digestive enzymes. Aquaculture 161 (1–4), 479–489. doi: 10.1016/S0044-8486(97)00295-0
Chen X. (2015). Effects of different feed additives on the growth and digestive enzyme activity of Babylonia lutosa. J. Fisher. Res. 37 (04), 301–307. doi: 10.14012/j.cnki.fjsc.2015.04.006
Chen C., Chen J., Wang S., You C., Li Y. (2017). Effects of different dietary ratios of linolenic to linoleic acids or docosahexaenoic to eicosapentaenoic acids on the growth and immune indices in grouper, epinephelus coioides. Aquaculture 473, 153–160. doi: 10.1016/j.aquaculture.2017.02.010
Chen K., Lai Z., Liu Y., Hao R., Wang Q. (2022). Sequence characteristics of PEPCK gene of pinctada fucata martensii and its selection in low temperature-resistant line. J. Fisher. Sci. China. 29 (01), 58–69. doi: 10.12264/JFSC2021-0323
Chen X., Mao X., Huang J., Yang D., Wu J., Dong S., et al. (2011). KOBAS 2.0: a web server for annotation and identification of enriched pathways and diseases. Nucleic Acids Res. 39, 316–322. doi: 10.1093/nar/gkr483
Dai C., Wang F., Fang Z., Dong S. (2013). Effects of temperature on energy metabolic enzymes of swimming crab (Portunus trituberculatus)in the post-molt stage. J. Fisher. China. 37 (09), 1334–1341. doi: 10.3724/SP.J.1231.2013.38676
Du X., Jiang X., Jiang X., Ye L. (2014). Study on the optimal lipid level in formulated feed of hemifusus tuba (Gmelin). Ecol. Science. 33 (03), 540–544+552. doi: 10.CNKI:SUN:STKX.0.2014-03-026
Fan H., Du X., Wen Y., Huang J., Luo F., Chen L., et al. (2020). Effects of different feeds on the growth and survival of larval cipangopaludina cahayensis. China Feed. 07), 86–89. doi: 10.15906/j.cnki.cn11-2975/s.20200719
Fan C., Qu Y., Li J., Yu N. (2011). Studies on digestive enzyme activity of trachinotus ovatus V the effects of salinity on digestive enzyme activity in different digestive organs of large-size young fish. Mar. Fisher. 33 (04), 423–428. doi: 10.13233/j.cnki.mar.fish.2011.04.010
Ge Z., Li B., Shen A., Deng X., Jiang Y, et al. (2006). Comparative analysis of nutritional value between corbicula fluminea and two mussels in taihu lake. J. Hydroecol. 01), 1–2+4. doi: 10.3969/j.issn.1003-1278.2006.01.001
Hao Z., Wang Y., Yu Y., Zhan Y., Tian Y., Wang L., et al. (2016). Evaluation of nutritive composition in the muscle of neptunea arthritica cumingii crosse (Gastropoda: Buccinidae). J. Dalia. Univ. 37 (06), 66–70. doi: 10.3969/j.issn.1008-2395.2016.06.016
Hawkins A. J. S., Day A. J. (1996). The metabolic basis of genetic differences in growth efficiency among marine animals. J. Exp. Mar. Biol. Ecol. 203 (1), 93–115. doi: 10.1016/0022-0981(96)02572-5
Hong M., Chen L., Gu S., Liu C., Zhang L., Li E. (2007). Effect of temperature change on lmmunochemical lndexes of eriocheir sinensis. Chin. J. Appl. Environ. Biol. 06), 818–822. doi: 10.3321/j.issn:1006-687x.2007.06.014
Huang Z., Zhou C., Lin H., Tan X., Peng J., Zhou W., et al. (2017). Effects of dietary isoleucine levels on activities of digestive enzymes and immune index of trachinotus ovatus. South China Fisher. Science. 13 (01), 50–57. doi: 10.3969/j.issn.2095-0780.2017.01.007
Jiang T. (2015). ). the study on the comprehensive benefit evalution of pond culture (Qingdao China: Ocean University of China).
Jiang Q., Feng X., Zhang C., Tang Y., Pan D., Li F., et al. (2013). Effects of dietary protein level on growth,activities of digestive enzyme and antioxidant system of cherax quadricarinatus. Freshw. Fisher. 43 (02), 60–65. doi: 10.3969/j.issn.1000-6907.2013.02.011
Jianqi Y., Kalhan. S. C., Hanson R. W. (2009). What is the metabolic role of phosphoenolpyruvate carboxykinase? J. Biol. Chem. 284 (40), 227025–9. doi: 10.1074/jbc.R109.040543
Kim M. S., Min E. Y., Kim J. H., Koo J. K., Kang J. C. (2015). Growth performance and immunological and antioxidant status of chinese shrimp, fennerpenaeus chinensis reared in bio-floc culture system using probiotics. Fi. Shellf. Immunol. 47 (1), 141–146. doi: 10.1016/j.fsi.2015.08.027
Ko Y. H., Yang H. Y., Jang I. S. (2004). Effect of conjugated linoleic acid on intestinal and hepatic antioxidant enzyme activity and lipid peroxidation in broiler chickens. Asian Australas. J. Anim. Sci. 17 (8), 1162–1166. doi: 10.5713/ajas.2004.1162
Lamming D. W., Bar-Peled L. (2019). Lysosome: The metabolic signaling hub. Traffic. 20 (1), 27–38. doi: 10.1111/tra.12617
Liao Y., Yang C., Wang Q., Sun R., Deng Y., Du X. (2018). Effects of different artificial diets on digestive enzymes, immunity and growth-related gene expression of pinctada fucata martensii. Genomics and Applied Biology 37, 12, 5251–5257. doi: 10.13417/j.gab.037.005251
Li S., Ji H., Zhang B., Tian J., Zhou J., Yu H. (2016). Influence of black soldier fly (Hermetia illucens) larvae oil on growth performance, body composition, tissue fatty acid composition and lipid deposition in juvenile jian carp (Cyprinus carpio var. jian). Aquaculture 465, 43–52. doi: 10.1016/j.aquaculture.2016.08.020
Li J., Li L., Zhang G. (2011). Cloning and sequence analysis of cathepsin l gene from bay scallop argopecten irradians. Mar. Sci. Bulletin. 30 (03), 338–343. doi: 10.3969/j.issn.1001-6392.2011.03.017
Liu F., Lv X., Liu Y., Lou B., Chen R. (2018). Effect of fast on digestion enzyme activity of juvenile Large yellow croaker (Larimichthys crocea). Periodical. Ocean. Univ. China. 48 (S1), 16–22. doi: 10.16441/j.cnki.hdxb.20170436
Li C., Xu Q., Xu H., Zhang T. (2008). Effects of different feed additives on immunity and antioxidation on rainbow trout (Oncrhynchus mykiss walbaum). J. Anhui. Agric. Univ. 03), 456–461. doi: 10.13610/j.cnki.1672-352x.2008.03.031
Long L., Yang J., Li Y., Guan C., Wu F. (2015). Effect of biofloc technology on growth, digestive enzyme activity, hematology, and immune response of genetically improved farmed tilapia (oreochromis niloticus). Aquaculture. 448, 135–141. doi: 10.1016/j.aquaculture.2015.05.017
LUO Z., Liu Y.-J., Mai K.-S., Tian L.-X., Liu D.-H., Tan X.-Y., et al. (2005). Effect of dietary lipid level on growth performance, feed utilization and body composition of grouper epinephelus coioides juveniles fed isonitrogenous diets in floating netcages. Aquacult. Int. 13 (3), 257–269. doi: 10.1007/s10499-004-2478-6
Luo J., Luo M., Li Y., Guo J. (2014). The effect of diet protein levels on growth performance and body composition of Babylonia areolata. J. Aquacult. 35 (01), 11–16. doi: 10.3969/j.issn.1004-2091.2014.01.003
Ma J., Zhang D., Jiang J., Cui S., Pu H., Jiang S. (2010). Molecular characterization and expression analysis of cathepsin L1 cysteine protease from pearl oyster pinctada fucata. Fi. Shellf. Immunol. 29 (3), 501–507. doi: 10.1016/j.fsi.2010.05.006
Mellman I. (1996). Endocytosis and molecular sorting. Annu. Rev. Cell Dev. Biol. 12 (1), 575–625. doi: 10.1146/annurev.cellbio.12.1.575
Morales A. E., Pérez-Jiménez A., Hidalgo M. C., Abellán E., Cardenete G. (2004). Oxidative stress and antioxidant defenses after prolonged starvation in dentex dentex liver. Comp. Biochem. Physiol. Part C. 139 (1), 153–161. doi: 10.1016/j.cca.2004.10.008
Morozova O., Hirst M., Marra M. A. (2009). Applications of new sequencing technologies for transcriptome analysis. annual review of genomics and human genetics 10 (1), 135–151. doi: 10.1146/annurev-genom-082908-145957
Mu Y., Li W., Wu B., Chen J., Chen X. (2020). Transcriptome analysis reveals new insights into immune response to hypoxia challenge of large yellow croaker (Larimichthys crocea). Fi. Shellf. Immunol. 98 (C), 738–747. doi: 10.1016/j.fsi.2019.11.021
Muñoz M., Cedeño R., Rodríguez J., van der Knaap W. P. W., Mialhe E. L., Bachère E. (2000). Measurement of reactive oxygen intermediate production in haemocytes of the penaeid shrimp, penaeus vannamei. Aquaculture 191 (1–3), 89–107. doi: 10.1016/S0044-8486(00)00420-8
Owen G. (1970). The fine structure of the digestive tubules of the marine bivalve cardium edule. philosophical transactions of the royal society of London. Ser. B. Biol. Sci. 258 (822), 245–60. doi: 10.1098/rstb.1970.0035
Panserat S., Plagnes-Juan E., Kaushik S. (2002). Gluconeogenic enzyme gene expression is decreased by dietary carbohydrates in common carp (Cyprinus carpio) and gilthead seabream (Sparus aurata). BBA. -. Gene Structure. Expression. 1579 (1), 35–42. doi: 10.1016/S0167-4781(02)00501-8
Peng K., Hu B., Zhao D., Wang J., Sheng J., Wu D., et al. (2016). Molecular characterization and expression analysis of cathepsin L1-like from hyriopsis schlegeli. J. Fisher. China. 40 (10), 1556–1566. doi: 10.11964/jfc.20150509890
Qiao Q., Xu W., Zhu H., Liu Z., Liu W. (2011). Effects of starvation-and-refeeding on the growth,body composition and intestinal digestive enzyme of megalobrama amblycephala yih. Freshw. Fisher. 41 (02), 63–68. doi: 10.3969/j.issn.1000-6907.2011.02.010
R. J. D. (1990). Malondialdehyde and thiobarbituric acid-reactivity as diagnostic indices of lipid peroxidation and peroxidative tissue injury. Free Radical Biol. Med. 9 (6), 515–540. doi: 10.1016/0891-5849(90)90131-2
Ren M., Liang H., He J., Masagounder K., Yue Y., Yang H., et al. (2017). Effects of DL-methionine supplementation on the success of fish meal replacement by plant proteins in practical diets for juvenile gibel carp (Carassius auratus gibelio). Aquacult. Nutr. 23 (5), 934–941. doi: 10.1111/anu.12461
Shen W., Yu D., Li W., Luo W., Zhu Y. (2009). Effects of bacillus lichenif ormis on activities of digestive enzymes, immunological and antioxidant indices of hyriopsis cumingii. Chin. J. Anim. Nutr. 21 (01), 95–100. doi: 10.3969/j.issn.1006-267x.2009.01.016
Sun J., Yang D. (2011). Study on sustainable development of Sea aquaculture in China. J. Shanxi. Agric. Sci. 39 (07), 733–735+746. doi: 10.3969/j.issn.1002-2481.2011.07.30
Turk B., Turk D., Turk. V. (2000). Lysosomal cysteine proteases: more than scavengers. Biochim. Biophys. Acta 1477 (1-2), 98–111. doi: 10.1016/S0167-4838(99)00263-0
Venier P., Pittà C. D., Pallavicini A., Marsano F., Varotto L., Romualdi C., et al. (2006). Development of mussel mRNA profiling: Can gene expression trends reveal coastal water pollution? Mutat. Res. -. Fundam. Mol. Mech. Mutagen. 602 (1), 121–134. doi: 10.1016/j.mrfmmm.2006.08.007
Wang Z., Jian J., Lu Y., Ding J., Wang B., Chen G., et al. (2013). Cloning expression analysis of the cathepsin l gene from pearl oyster pinctada fucata. Oceanol. Limnol. Sinica. 44 (06), 1604–1611. doi: 10.11693/hyhz201306029
Wang C., Lu W., Lin Y., Luo J. (2018). Development and application of transcriptome sequencing. Eucalypt Sci. Technol. 35 (04), 20–26. doi: 10.13987/j.cnki.askj.2018.04.005
Wang D., Tang D., Wang W., Zhou P. (2008). Study on the fat requirement of Babylonia areolata. Fe. Res. 04), 59–61. doi: CNKI:SUN:SLYJ.0.2008-04-021
Wang J., Zhang G., Yu C, Wang T, Wang L, Wang G, et al. (2007). Liaoning marine fishery administration manual (Shenyang: Liaoning People’s Publishing House).
Wang T., Zhou X., Zhao C. (2012). Effect of three kinds of feed on growth, immune enzyme, amino acid content and digestive enzymes activity of procambarus clarkii. J. Shanghai. Ocean. Univ. 21 (06), 1011–1101. doi: CNKI:SUN:SSDB.0.2012-06-012
Xian J., Zhang X., Li J., Wang D. (2016). Research progress in nutritional requirement and compound feed for spotted babylon Babylonia areolata. Fe. Industry. 37 (24), 24–27. doi: 10.13302/j.cnki.fi.2016.24.006
Xu Y. (2006). Studies on protein and lipid requirement of Babylonia areolate link (Xiamen China: Xiamen University).
Xue M., Ke C., Di G. (2010). Effects of fasting on the morphology and biochemical composition of tissues of juvenile spotted babylon, Babylonia areolata. J. Appl. Oceanogr. 29 (02), 205–211. doi: 10.3969/J.ISSN.1000-8160.2010.02.009
Xu Y., Ke C., Wang D., Wei Y., lv J. (2006). Studies on protein requirement of Babylonia areolata link. J. Xiamen. Univ(Natural. Science). S1), 216–220. doi: 10.3321/j.issn:0438-0479.2006.z1.053
Xu W. J., Pan L. Q. (2014). Evaluation of dietary protein level on selected parameters of immune and antioxidant systems, and growth performance of juvenile litopenaeus vannamei reared in zero-water exchange biofloc-based culture tanks. Aquaculture. 426-427, 181–188. doi: 10.1016/j.aquaculture.2014.02.003
Yang D., Yan A., Chen F., Ruan G., Fang C. (2003). Effects of different diets on activities ofdigestive enzymes of monopterus albus. J. Fisher. China. 06), 558–563. doi: 10.3321/j.issn:1000-0615.2003.06.009
Yin Y., Zhang P., Yue X., Du X., Li W., Yin Y., et al. (2018). Effect of sub-chronic exposure to lead (pb) and bacillus subtilis on carassius auratus gibelio: bioaccumulation, antioxidant responses and immune responses. Ecotoxicol. Environ. Safety. 161, 755–762. doi: 10.1016/j.ecoenv.2018.06.056
Zhang L., Zhou Q., Cheng Y., Wei X., Zhang S. (2009). Effect of dietary carbohydrate sources on Growth,Feed utilization and digestive enzymes activities of juvenile lvory shell Babylonia areolate. J. Guangdong. Ocean. Univ. 29 (04), 14–18. doi: 10.3969/j.issn.1673-9159.2009.04.004
Zheng Y., Zeng Z., Liu B., Lin G., Li Z., Li L., et al. (2013). Influence on growth and survival of Babylonia lutosa juveniles fed with several natural diets. J. Fisher. Res. 35 (04), 301–306. doi: 10.14012/j.cnki.fjsc.2013.04.012
Zhong H., Wang D., Wang G. (2012). Research progress on nutrition and bait of Babylonia. Hebei. Fisher. 09), 50–53. doi: 10.3969/j.issn.1004-6755.2012.09.018
Keywords: Neptunea cumingii, diets, grow, enzyme activity, transcriptome
Citation: Ge X, Zhao J, Liang Z, Chi Q, Mao J, Wang X, Chang Y and Hao Z (2022) Comparative analysis of Neptunea cumingii growth, related digestive and immune enzyme indicators, and liver transcriptome under different feeding conditions. Front. Mar. Sci. 9:1013180. doi: 10.3389/fmars.2022.1013180
Received: 06 August 2022; Accepted: 02 September 2022;
Published: 20 September 2022.
Edited by:
Jinghui Fang, Yellow Sea Fisheries Research Institute, (CAFS), ChinaReviewed by:
H-Michael Habte-Tsion, University of Maine, United StatesFei Xu, Institute of Oceanology, (CAS), China
Copyright © 2022 Ge, Zhao, Liang, Chi, Mao, Wang, Chang and Hao. This is an open-access article distributed under the terms of the Creative Commons Attribution License (CC BY). The use, distribution or reproduction in other forums is permitted, provided the original author(s) and the copyright owner(s) are credited and that the original publication in this journal is cited, in accordance with accepted academic practice. No use, distribution or reproduction is permitted which does not comply with these terms.
*Correspondence: Yaqing Chang, yaqingchang@hotmail.com; Zhenlin Hao, haozhenlin@126.com
†These authors have contributed equally to this work