- 1Key Laboratory of Ecological Restoration and Conservation of Coastal Wetlands in Universities of Shandong (Ludong University), The Institute for Advanced Study of Coastal Ecology, Ludong University, Yantai, China
- 2School of Ecology, Resources and Environment, Dezhou University, Dezhou, China
Metal distribution in different wetlands under the complex influence of natural and anthropogenic activities in the Liaohe Delta was analyzed to better protect and manage delta areas. Ninety-six soil samples were collected from six wetland types, and the levels, depth distributions, risk, and influencing factors of metals (i.e., As, Cd, Cr, Ni, Cu, Pb, Zn, Mn, Al, and Fe) were analyzed. The results showed that the concentrations of As, Cd, Cr, Ni, Zn, and Al in more than 70% of the soil samples were higher than the background value of Liaoning Province. The concentration of As decreased with distance from the estuary, while that of Cr was higher downstream of Liaohe. The spatial distributions of Cd, Ni, Cu, Pb, and Zn were similar. The concentrations of most metals in the topsoil were higher than those in the subsoil. According to the Nemerow pollution index, higher pollution was observed in the area far away from the Liaohe River and Bohai Sea for the topsoil layer, while for the subsoil layers, higher pollution was observed in the area near the Liaohe estuary. The correlation analysis showed that the wetland type was clearly correlated with Cd, Cu, Al, and S. The concentrations of Cd, Pb, Zn, C, N, P, and S and the electrical conductivity obviously decreased with soil depth, while the concentration of Mn was positively correlated with soil depth. Mn was negatively correlated with total organic carbon (TOC), total nitrogen (TN), and total phosphorus (TP), whereas Cd, Pb, and Zn showed positive correlation with TOC, TN, and total sulfur (TS). The general linear model indicated that wetland type had a significant effect on As, Cd, Ni, Cu, Pb, Zn, Al, and Fe (p< 0.01), while depth had a significant effect on Cd, Cr, and Pb (p< 0.01). The findings of this work will contribute to pollution control and ecosystem health conservation of the Liaohe Delta.
Introduction
With rapid industrialization and intensified anthropogenic activities, high metal concentrations have frequently been detected in the delta area (Mandour et al., 2021; Yu et al., 2022), a fluvial plain formed by deposited sediment originating from the river. For example, the soil in the Nile Delta is polluted by vanadium (V), chromium (Cr), nickel (Ni), cobalt (Co), and cadmium (Cd), with a temporal increase in 1995–2015 (Mandour et al., 2021). Yin et al. (2021) found that the concentrations of lead (Pb), Cd, mercury (Hg), and arsenic (As) in the Pearl River Delta were at a high level. Zhao et al. (2019) analyzed 217 surface sediment samples collected across 14 typical delta areas in China and illustrated that the concentrations of Cd, Cr, copper (Cu), Pb, and zinc (Zn) ranged from 0 to 0.51, from 0.82 to 146.77, from 0.25 to 133.23, from 1.02 to 176.42, and from 1.92 to 208.12 mg/kg, respectively. Among the 14 typical delta areas, 11 were exposed to moderate ecological risk in the soil with potential adverse biotic effects. Metals are hazardous to humans and ecosystems due to their toxicity, persistence, and transitivity (Xu et al., 2020). Metals in delta areas threaten the ecological environment of both the ocean and land because delta is the result of the interaction of ocean and land (Mandour et al., 2021). Therefore, investigating the spatial distribution of metals and identifying their influencing factors are imperative to the ecological environment of delta areas.
The spatial distribution of metals generally has obvious spatial heterogeneity in estuary delta areas (Delavar and Safari, 2016), which could help define high-risk areas and to explore the influencing factors of metals (Wu et al., 2020; Zeng et al., 2022). Yu et al. (2022) explored the spatial distribution of metals in 106 surface soil samples from the Yellow River Delta and clarified that pH and salinity are important influencing factors. Three hotspots of metal accumulation in the top 20 cm soil were observed in the spatial distributions of heavy metals in the Yellow River Delta, and the trend in 40–50 cm soil was in accordance with the variations in hydrological conditions (Zhao et al., 2021). Nguyen et al. (2022) drew a spatial distribution map of the presence of As in the Red River Delta. This spatial distribution figure showed that the coastal area appeared as a hotpot of As, with saltwater intrusion as the main influencing factor.
The concentrations and spatial contribution of metals in delta soils are influenced by various factors, including soil properties, wetland types, hydrological condition, and anthropogenic activities, among others (Zhong et al., 2020; Xu et al., 2021; Mei et al., 2022). For example, a high correlation between total organic carbon (TOC) and metals was observed in soil given that TOC acts as the main contributor to exchanged or dissolved metals in the sediment (Zhang et al., 2022). Moreover, an increase in salinity has been shown to be associated with a declining trend of As and other metal concentrations (Bai et al., 2019). Li et al. (2016) reported that the presence of wetland plants increased the redox potential and redistributed metals in the rhizosphere, with variations observed across different plants. In the delta area, wetland types are mainly determined by hydrological conditions such as tidal inundation, precipitation runoff, groundwater flow, evapotranspiration, artificial inflow, and drainage (White and Madsen, 2016). For example, Suaeda salsa wetland and mudflat were mainly influenced by tidal inundation. Thus, the hydrological conditions have a powerful effect on the concentrations and distributions of metals.
The Liaohe Delta is the second largest permanent Phragmites australis in the world (Yan et al., 2018). Owing to different hydrological conditions and human activities, various wetland types have formed in the Liaohe Delta, with different marshes including P. australis, S. salsa, mudflat, paddy field, etc. Additionally, the third largest oilfield in China, the Liao River oilfield, is located in the delta. Tian et al. (2017) clarified that multiple human activities occupied large areas of various wetlands. The total area of coastal wetlands in the Liaohe Delta has decreased by 14.8% in 1986–2018, with 45.7% of mudflats eroded (Cong, 2021). Approximately 22.1% of S. salsa marshes decreased in 2012–2018, while P. australis marsh areas increased (Cong, 2021).
Knowledge of the metal distribution and influencing factors directly affects how delta areas are protected and managed (Wang et al., 2022). However, only a few studies have focused on metal distributions in different wetlands under the complex influences of natural and anthropogenic activities in the Liaohe Delta. The effect and the extent of wetland type, depth, electrical conductivity (EC), and nutrient elements on the metal concentration in soil also require extensive investigation. Therefore, in this study, six types of wetlands were selected to study the characteristics and influencing factors of metal distribution. Our aims were: 1) to clarify the spatial distribution of metals; 2) to reveal the correlations among metals, wetland types, and soil properties; and 3) to assess the metal pollution at different soil depths.
Materials and methods
Soil sampling and determination
The Liaohe Delta, adjacent to the Bohai Sea, is located in Liaoning Province, northeastern China. The Liaohe Delta has a warm, semi-humid continental climate with four distinct seasons. The temperature ranges from −20.4°C to 36.4°C, with an annual temperature of 8.3°C. The annual precipitation is 611.6 mm, and annual evaporation is 1,705 mm. The water supply mainly comes from precipitation, runoff, and periodic tide. The preponderant vegetation in the Liaohe Delta are P. australis and S. salsa. Saline–alkaline soil areas account for the majority of the soil in the Liaohe Delta.
The following six types of wetlands were selected as the sampling sites: natural P. australis marsh (NP, n = 6); P. australis marsh in oilfield (PO, n = 6); degraded P. australis marsh, where water was drained and P. australis grew poorly (DP, n = 5); S. salsa marsh (SS, n = 2); mudflat (MF, n = 3); and rice paddy (RP, n = 2) (Figure 1). In April 2018, soil cores were randomly collected at depths of 0–40 cm and broke four soil layers at 10-cm intervals at each site. Subsequently, all soil samples were sealed in polyethylene bags and brought to the laboratory. The soil samples were air dried at room temperature and sieved through a 2-mm sieve to remove extraneous matter, such as leaves and large stones. The dried soil was then ground into particles with diameters of<200 μm and stored at 4°C before analysis.
Soil EC was measured in the supernatant of 1:5 (w/v) soil–water mixtures using a pH meter (PHBJ-260, INESA, Shanghai, China). TOC was detected using a dichromate oxidation–colorimetric method (Zhao et al., 2020). The Kjeldahl nitrogen method was used to determine the total nitrogen (TN). Soil was digested with perchloric acid–sulfuric acid, and then total phosphorus (TP) was detected using a segmented flow analyzer (SFA, AMS Alliance, France).
The soil samples were digested using a mixture of hydrofluoric–nitric–perchloric acid according to the national standard method for China (GB, 1995). The concentrations of manganese (Mn), aluminum (Al), iron (Fe), and total sulfur (TS) in the digestion solution were determined with inductively coupled plasma atomic emission spectroscopy (ICP-AES; ICPS-7500, Agilent Technologies, Tokyo, Japan). The concentrations of As, Cd, Cr, Ni, Cu, Pb, and Zn were determined with inductively coupled plasma mass spectrometry (ICP-MS; NexION 350D, Perkin-Elmer, Shelton, CT, America). Duplicate samples were measured for data quality assurance. The recovery rate was obtained by adding geochemical reference materials (e.g., GSS23), one every 10 samples, and was calculated as the ratio of the determined value and the reference value of the reference material. The recovery rate was within the permissible range.
Pollution index calculation
The Nemerow pollution index (NPI) was employed to assess the degree of metal pollution in soil, which was calculated using Eq. (1) and Eq. (2) for each sampling site (Yang et al., 2011; Kowalska et al., 2016).
where Px is the single pollution index of metal x; Cx is the concentration of metal x; Sx is the background value of the study area, which refers to the background value of Liaoning Province (BL; China National Environmental Monitoring Center, 1990); Px,max is the maximum pollution index of metal x; and Px,mean is the average pollution index of metal x. Five contamination categories were recognized according to the value of the NPI: non-pollution (NIPI ≤ 0.7), warning line of pollution (0.7< NIPI ≤ 1), low-level pollution (1< NIPI ≤ 2), moderate-level pollution (2< NIPI ≤ 3), and high-level pollution (NIPI > 3) (Memoli et al., 2019).
Data analysis
Descriptive statistical analyses were performed using Microsoft Excel 2010, IBM SPSS Statistics 22, and Origin 2017. ArcGIS 10.0 (Esri, Redlands, CA, USA) was used for spatial calculations and for drawing maps. Interpolated figures were graphed based on the kriging method through ArcGIS 10.0. Correlation analysis was performed to evaluate the degree of correlation between metals, nutrient elements, and wetland types. Considering that the wetland type is a non-numerical variable, Spearman’s correlation analysis was chosen for this study. The freely available “R” statistical software package (R Core Team; https://www.r-project.org/) was employed to conduct correlation analysis.
To display the degree of differences in the spatial distribution of metals, the coefficient of variability (CV) was introduced to analyze the dispersion degree of the data. Normally, if the CV is higher than 0.3, the spatial distribution of the metals is considered to be very different. The general linear model (GLM) was used to determine the main effect of wetland type and depth and the interactive effect on the concentrations of metals and nutrients.
Results
Contents of metals and nutrients in the Liaohe Delta wetland soils
The concentrations of metals showed a large range from 0.04 mg/kg (Cd in site G, DP) to 1,529 mg/kg (Mn in site W, RP) owing to the various levels of enrichment in the natural environment and the different emission intensities from human activities (Table 1). With the exception of Cu, the mean concentrations of the metals were higher than the BL (China National Environmental Monitoring Center, 1990). The concentrations of As, Cd, Cr, Ni, Zn, and Al in more than 70% of the soil samples were higher than the BL, indicating that anthropogenic activities had a direct effect on their concentrations.
The concentration ranges of TOC, TN, TP, and TS were 1.61–167.83, 0.16–4.45, 0.02–0.09, and 0.38–6.15 g/kg, respectively. The EC values ranged from 111.60 to 5,512.00 μs/cm, with a mean value of 1,510.04 μs/cm.
In this study, the CVs for Cd, Cr, Zn, and Mn were 0.76, 0.34, 0.41, 0.31, respectively, which showed obvious discrepancies for the different sites. Compared to metals, nutrient elements showed greater differences within sites, with higher CVs of 1.22 (TOC), 1.00 (TN), 0.95 (TS), and 0.26 (TP).
Spatial distribution of metals in wetland soils
As shown in Figure 2 (interpolated figures), the concentration of As decreased with distance from the estuary; that is, the shorter the distance from the estuary, the higher the As concentration. These results demonstrated a relationship between As and the intrusion of seawater. Higher Cr mainly occurred downstream of Liaohe. The spatial distributions of Cd, Ni, Cu, Pb, and Zn were similar, with lower concentrations in the soil along Liaohe. The concentration of Zn at site W (rice paddy) was higher than those in other areas. The spatial distribution trend of Mn, Al, and Fe was consistent, indicating common sources and influencing factors. The higher concentrations mainly occurred in the north and south parts of the study area.
Profile distributions of metals in wetland soils
The mean concentrations of the metals were significantly different with soil depth in the wetlands (Figure 3). Higher As concentrations occurred in the SS and MF, while lower concentrations occurred in RP. Significant differences were also observed between the topsoil (0–10 cm) and subsoil (20–40 cm) layers for Cd in the NP, PO, and DP. For the RP, the concentrations of Cd were higher in the 0-30 cm layers (0.29, 0.31, and 0.45 mg/kg) than those in the 30-40 cm layer (0.11 mg/kg). However, the values for Cd were lower in the topsoil in the MF compared with those in the other soil layers. The mean concentration of Cr was higher in 0–10 and 20–30 cm soil and was lower in 10–20 and 30–40 cm soil in the SS, MF, and RP. The mean concentration of Cr in the NP increased with depth. In the 0–30 cm soil, the concentration of Cr increased with depth for PO and DP. The mean concentration of Cu was highest in the RP (21.02, 20.87, 21.38, and 21.13 mg/kg for 0–40 cm, all greater than the BL) in the study area, followed by the PO, NP, DP, MF, and SS. The distribution of Ni in the six wetland types was consistent with that of Cu. The mean concentrations of Pb and Zn in the topsoil of the NP, PO, and DP were higher than those in the subsoil. However, for the RP, Zn was much higher in 0–30 cm soil, suggesting an influence of agricultural activity. Mn was lower in the topsoil in the NP, PO, DP, which confirmed that it was easily lost with water (Huang et al., 2017). As shown in Figure 3, there were significant distinctions between the six wetland types for Al and Fe. Higher levels of Al and Fe occurred in the NP, PO, and RP.
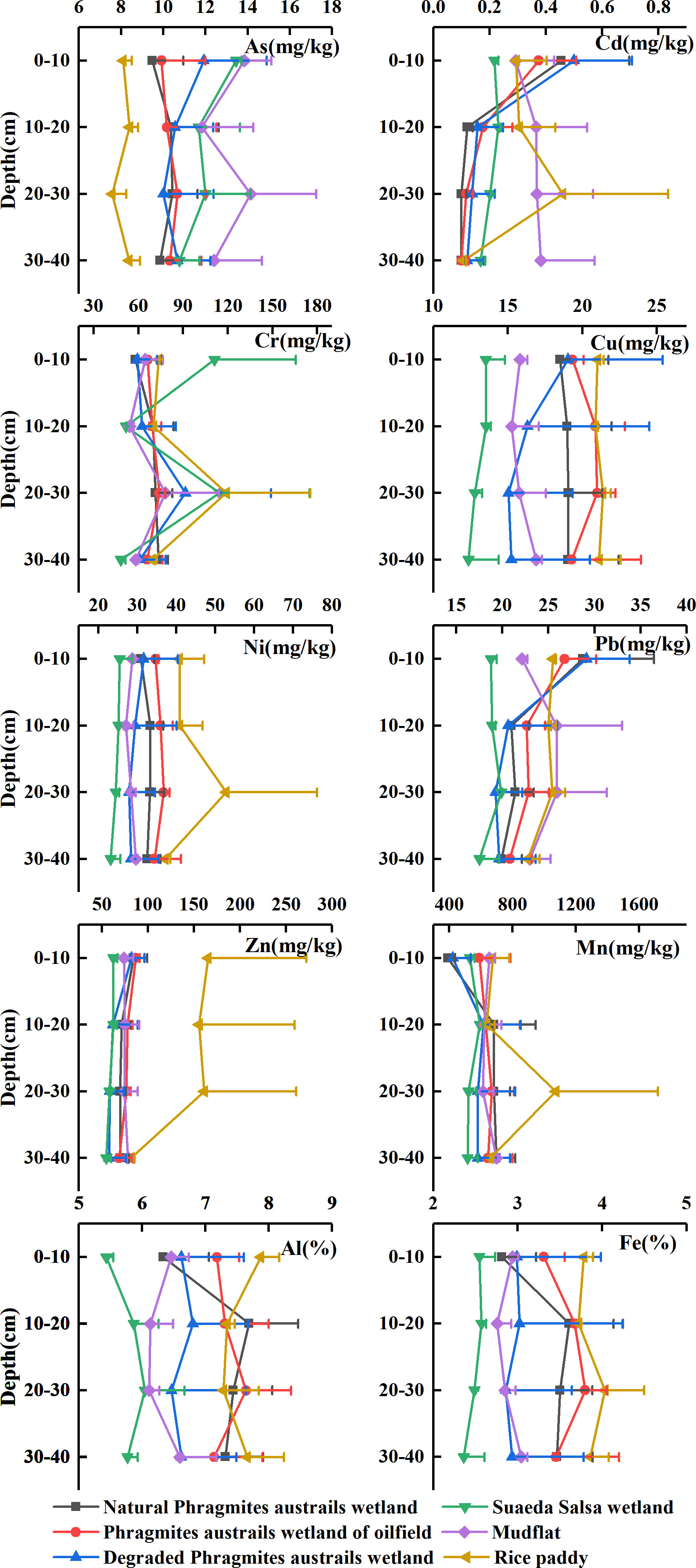
Figure 3 Metal distributions in different depths under the six wetland types for Liaohe Delta. Bars indicate the standard deviation.
Ecological risk of metals in the Liaohe Delta wetlands
The NPI values of approximately 96% of the samples were higher than 1, indicating metal pollution in the Liaohe Delta wetland soils (Figure 4). For 0–10 cm soil, the NPI values for all samples were higher than 1, indicating that metal pollution existed in the study area. Ten sites (approximately 42% of all sites) had an NPI >3, and seven sites had moderate-level pollution (2< NIPI ≤ 3). The highest NPI value was determined at site G, with an NPI of 5.20, showing the highest pollution level. In 10–20 cm samples, the NPI values of 96% of the sites were higher than 1, showing different metal pollution levels. The highest NPI value was calculated at site P, with an NPI of 3.78, showing the highest pollution level in 10–20 cm soil. The NPI values of all 20–30 cm samples were higher than 1. Additionally, 21 sites (approximately 88% of all sites) had an NPI >1 in 30–40 cm samples. It was notable that the NPI values of site P for all four depths were all higher than 3, showing a high level of metal pollution. Generally, the distribution of the NPI values showed obvious differences among the soil layers, with the higher values in the areas further away from the Liaohe River and Bohai Sea for the topsoil layer and higher values in the areas near the Liaohe estuary for the subsoil layers.
Relationship between metals and soil properties
According to the correlation analysis (Figure 5), there was a clear relationship between wetland type and Cd, Cu, Al, and S. The values of Cd, Pb, Zn, C, N, P, S, and EC decreased with soil depth. The concentration of Mn was positively correlated with soil depth (p< 0.01), and those of Cr, Ni, Cu, Pb, Zn, Mn, Al, and Fe were significantly positively correlated with each other. The concentration of As was positively correlated with Mn and EC, but was negatively correlated with TP. Mn was negatively correlated with TOC, TN, and TP, while Cd, Pb, and Zn were positively correlated with TOC, TN, and TS. EC demonstrated a positive correlation with As and Cd and a significant negative correlation with Cr, Ni, Cu, Al, and Fe (p< 0.01). Additionally, TOC, TN, TP, and TS were closely linked to each other.
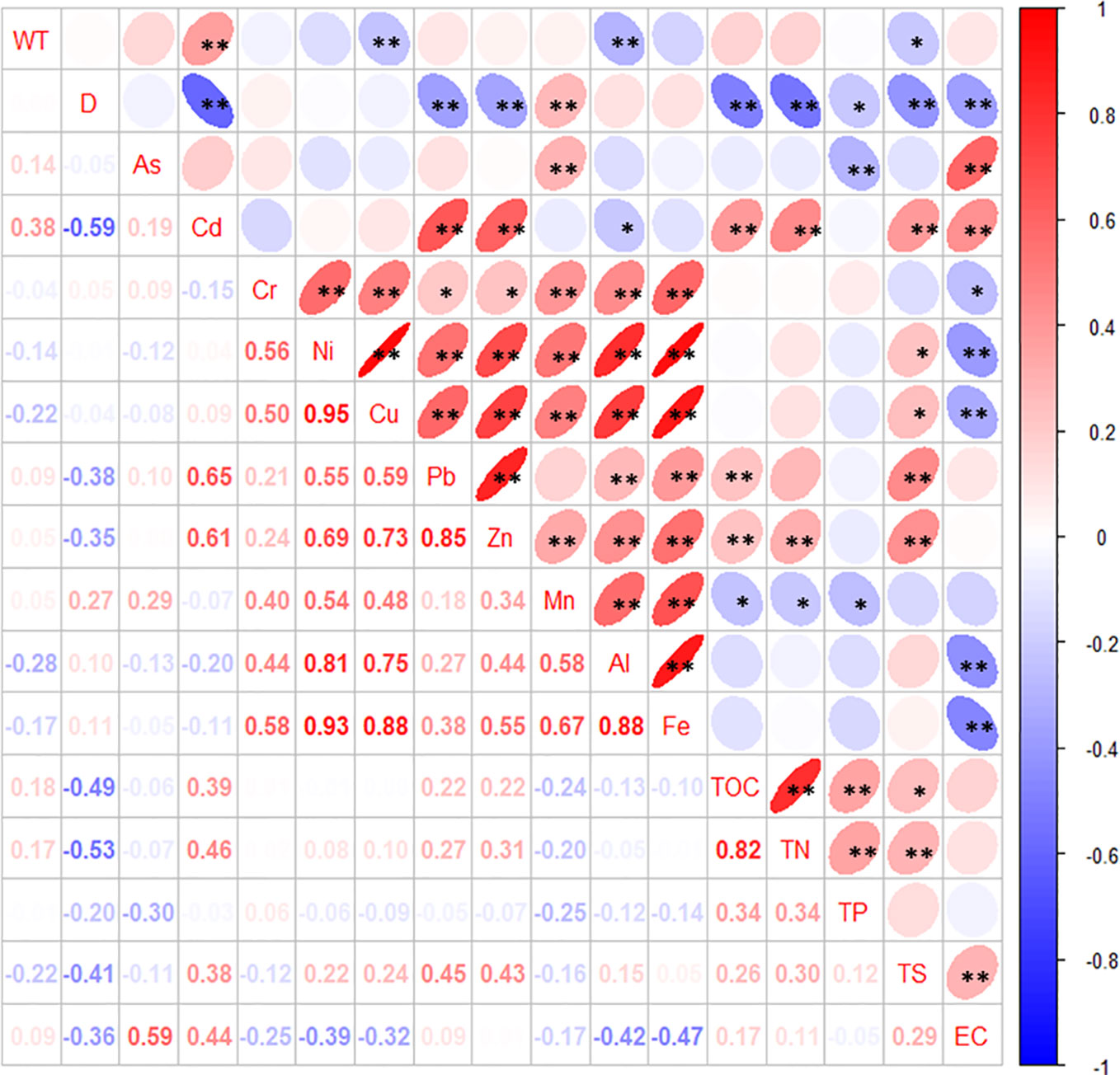
Figure 5 Spearman’s correlation analysis between metals and soil characteristics. WT, wetland type; D, depth. Red denotes a positive interaction and blue represents negative interaction. Lighter color indicates weaker correlation. *Correlation is significant at the 0.05 level (two-tailed). **Correlation is significant at the 0.01 level (two-tailed).
The GLM was further applied to examine the effects of wetland type and depth on the metal concentration. The results are shown in Table 2. Wetland type had a significant effect on As, Cd, Ni, Cu, Pb, Zn, Al, and Fe (p< 0.01), while depth had a significant effect on Cd, Cr, and Pb (p< 0.01). A significant influence (p< 0.01) was also found between Cd and the interaction effect of wetland type and depth.
Discussion
Effect of wetland type on the concentrations of metals in soil
Wetland type exerted a profound influence on the metal concentration mainly due to their unique condition of hydrological conditions, soil properties, plant growth, and human activity, among others. In this study, the concentration of As was higher in the SS and MF, where the EC and the salinity were high. Bai et al. (2016) observed that the concentrations of As increased with increasing distance from the river bank, with higher As concentrations observed in salt marshes in the Yellow River Delta. A significantly positive correlation was observed between As and EC, Na+, K+, Cl−, and in the Yellow River Estuary (Zhao et al., 2021). One possible explanation for this was that the seawater affected by tide provided a constant source of As and sulfur (S) for the soil in the SS and MF. The abundance of S from seawater can actually lead to As immobilization through the precipitation of As–S phases (thiolation) or the adsorption of As onto the FeS surface of Fe minerals (e.g., pyrite) in soil (Yan et al., 2022). Furthermore, the concentration of As was shown to be lower in the RP and the P. australis wetland, largely due to the lower salinity being able to enhance the plant uptake of metals in freshwater wetlands (Fritioff et al., 2005; Bai et al., 2016). As shown by Nguyen et al. (2022), rice is an As-accumulating plant species that can inadvertently assimilate As when it takes up phosphorus and silicon. In contrast to other food crops, the uptake of As in rice has been found to be substantially higher because of the prevalence of highly mobile and toxic arsenite [As(III)] under paddy soil conditions (Hussain et al., 2021).
The mean concentration of Zn in the RP ranged from 155.75 to 165.33 mg/kg for the 0-30 cm soil layers, which was more than twice that of the BL of Zn in Liaoning Province (China National Environmental Monitoring Center, 1990). These concentrations were higher than the hazardous concentrations affecting 5% of the species in alkaline calcareous soils (155 mg/kg) and lower than that for alkaline soils in China (217 mg/kg) (Zhao et al., 2022). Agricultural activities may be the main reason for the accumulation of Zn (Karstens et al., 2016). Zinc deficiency in rice would cause a range of symptoms including leaf bronzing, stunted growth, reduced yield, and, often, high mortality (Weiss et al., 2021), which has been a limiting factor for rice production on millions of hectares of arable land (Pilbeam, 2015). During the rice growing process, Zn fertilizer is commonly applied to generate a higher yield, especially in saline–alkali areas (Impa et al., 2013). Ali et al. (2014) conducted field experiments by adding Zn at different levels (0, 8, 10, 12, and 14 kg/ha) and clarified that 14 Zn/ha−1 produced the maximum rice yield. However, most of the Zn applied as fertilizer was adsorbed in soil and only a small proportion was recovered by rice crops during the growth period due to the high clay concentrations and high pH in alkaline soils (Ali et al., 2014). Additionally, Cu and Ni are essential for rice growth, but can be toxic to rice at high concentrations (Karstens et al., 2016). Agricultural production and fertilizer were identified as the major causes of the highest Cu and Ni concentrations occurring in the RP.
Under the RP, NP, and PO, the concentrations of Al and Fe were much higher than those in other wetlands. Previous studies have shown that the roots of P. australis and paddy would transfer oxygen via aerenchyma for root respiration in anaerobic soils. The loss of oxygen in roots could stimulate Fe(II) oxidization and the deposition of a reddish Fe plaque that coats the root surface (Khan et al., 2016). Fe plaques can inhibit the Al absorbed by plants (Chen et al., 2006), which could explain why the concentrations of Al and Fe were much higher in the RP, NP, and PO.
The concentrations of Ni, Cu, Pb, and Zn were lower in the SS of this study. This is likely because S. salsa could enrich metals and was suitable for the phytoremediation of Ni, Cu, and Pb (Song and Sun, 2014).
Effect of depth on the metal concentration in soil
The metals showed different tendencies with soil depth. Generally, the metal concentrations in the topsoil are higher than those in the subsoil (Bai et al., 2019). As shown by the NPI values, the risk from topsoil was much higher than that from subsoil. Moreover, the concentrations of Cd, Pb, and Zn decreased with buried depth in this study area, according to the results of the correlation analysis. As discussed by Liu et al. (2022), the concentrations of Cd and Pb decreased with depth. Additionally, Pb easily migrates into the deep soil (Liu et al., 2022). Consistently, the horizon distribution trend of metals can be used to reveal their sources. A high metal concentration in the topsoil indicates that there exist new metal inputs, such as atmospheric deposition and agricultural activities (Zhang et al., 2012; Wu et al., 2022). However, the concentration of Mn was negatively correlated with depth in the Liaohe Delta. Additionally, there were obvious discrepancies among the four depths for Cd (p< 0.01), Cr (p< 0.01), Pb (p< 0.01), and Zn (p< 0.05).
As mentioned above, the EC, TOC, TN, TP, and TS were significantly negatively correlated with depth, in that the EC and nutrient elements decreased with increasing depth. In line with this, soil properties, such as EC and nutrient element, play a crucial role in the distribution of metals (Bai et al., 2019; Xu et al., 2021). Indeed, Liu et al. (2022) reported that the concentrations of As, Cr, and Hg increased with increasing soil depth, whereas those of Cd, Cu, and Pb decreased with increasing depth.
Effect of EC and nutrient elements
Previous studies have provided proof that EC is significantly correlated with metals in both soils (Zhong et al., 2020) and plant tissues (Yuan et al., 2021). Higher EC values usually accompany a higher ionic strength and a higher metal release capacity (Friedman, 2005). In the estuary region, the metals were easily desorbed from the soil to the water due to the higher EC, which may explain why Cr, Ni, Cu, Al, and Fe were significantly negatively correlated with EC in this study. Bai et al. (2019) reported higher metal levels observed at sites with lower EC values, whereas lower metal levels were observed at sites with higher EC values. The positive correlation of As and Cd with EC might have resulted from these two metals being easily deposited in the soil as sulfide (Du Laing et al., 2008; Yan et al., 2022). Moreover, Bai et al. (2016) reported that, in the Yellow River Delta, As and Cr were significantly positively correlated with soil salinity, which was proportional to EC.
Nutrient elements, mainly TOC, TN, TP, and TS, play a dominant role in the metal concentration in soil (Xu et al., 2021) and represent important factors for the mobility and the binding of metals (Sundaramanickam et al., 2016). Du Laing et al. (2008) reported that metals accumulate according to the soil element characteristics in intertidal marshes, while Xu et al. (2021) elucidated that low soil nutrient elements result in the lowest soil metal concentration. In this research, the concentrations of TOC, TN, TP, and TS were significantly and positively correlated with each other in the Liaohe Delta, except for TP and TS, demonstrating that the nutrient element in this study exhibited consistency in either source or characteristics.
In this study, TOC and TN were positively correlated with Cd, Pb, and Zn, which is in agreement with the results of previous studies (Liu et al., 2016; Islam et al., 2022; Mei et al., 2022). This could be explained by the fact that TOC, which is associated with wetland plants, may decrease the mobility of metals through exchange, chelation, and complexation (María-Cervantes et al., 2010). Under the influence of TN, the adsorption of TOC onto metals would be enhanced, but its desorption from metals would be reduced (Li, 2019). Generally, TN is composed of organic (proteins, amino acids, etc.) and inorganic (mainly –N and –N) nitrogen. On the one hand, organic nitrogen could transform metals from a dissolved fraction to an organically bound fraction, thus increasing metal accumulation in soils. On the other hand, inorganic nitrogen could improve the transfer of soil metals and reduce the sorption of metals by soil particles through increasing the metal solubility in soil (Xu et al., 2021), resulting in a lower accumulation of Mn in the Liaohe Delta.
No significant correlations were observed between TP and most of the metals in this study. In previous wetland studies, the close correlation between TP and metals was the result of the application of phosphate fertilizer in agricultural activities (Karstens et al., 2016). In the Liaohe Delta, the concentration of TP was highest in the RP. However, among the 24 sampling sites, only two were RPs. Considering the reduction in agricultural area and the external input of phosphorous, the correlation between TP and the metals in this study was weak.
In delta areas, S plays a vital role in the concentrations of metals (Du Laing et al., 2008; Nguyen et al., 2022). Metals easily settle as metal sulfide, especially with frequent flooding and drying (Yan et al., 2021). The abundance of S (TS, 0.38–6.15 g/kg) in the coastal area provided favorable conditions for metal accumulation in the soil, which explains the positive correlation between TS and Cd, Ni, Cu, Pb, and Zn in the Liaohe Delta.
Conclusions
In the Liaohe Delta, pollution with As, Cd, Cr, Ni, Zn, and Al was observed in more than 70% of the soil samples, with obvious discrepancies across sites for Cd, Cr, Zn, and Mn. The periodic tide and the abundance of S in seawater led to the deposition of As in the SS and MF through the precipitation of As–S phases (thiolation) or the adsorption of As onto the FeS surface of Fe minerals. Agricultural production and fertilizer application in the RP were the main causes of the high Zn, Cu, and Ni accumulation in soil. The concentrations of Al and Fe were much higher in the RP, NP, and PO. The concentrations of Cr, Ni, Cu, Al, and Fe were significantly negatively correlated with EC. TOC and TN were positively correlated with Cd, Pb, and Zn. TS was positively correlated with Cd, Ni, Cu, Pb, and Zn in the Liaohe Delta, largely due to metals easily settling as metal sulfide under the influence of frequent flooding and drying. As shown by the NPI values, the risk from topsoil was much higher than that from subsoil, with metal pollution present in approximately 96% of the topsoil samples.
Data availability statement
The original contributions presented in the study are included in the article/supplementary material. Further inquiries can be directed to the corresponding authors.
Author contributions
YY: Investigation, conceptualization, writing the original draft, and editing. JZ and JQ: Data curation. JSY: Investigation, supervision, and review. QZ: Review. ZW: Resources and formal analysis. NS: Review. JBY: Supervision and review. All authors contributed to the article and approved the submitted version.
Acknowledgments
This research was supported by the National Natural Science Foundation of China (U1806218 and 41871087) and the Project of the Cultivation Plan of Superior Discipline Talent Teams of Universities in Shandong Province: “the Coastal Resources and Environment team for Blue-Yellow Area”. We are grateful for the support. We also wish to express our gratitude to the editors and reviewers for their suggestions and advice pertaining to this manuscript.
Conflict of interest
The authors declare that the research was conducted in the absence of any commercial or financial relationships that could be construed as a potential conflict of interest.
Publisher’s note
All claims expressed in this article are solely those of the authors and do not necessarily represent those of their affiliated organizations, or those of the publisher, the editors and the reviewers. Any product that may be evaluated in this article, or claim that may be made by its manufacturer, is not guaranteed or endorsed by the publisher.
References
Ali H., Hasnain Z., Shahzad A. N., Sarwar N., Qureshi M. K., Khaliq S., et al. (2014). Nitrogen and zinc interaction improves yield and quality of submerged basmati rice (Oryza sativa l.). Not. Bot. Horti. Agrobo. 42 (2), 372–379. doi: 10.15835/nbha4229469
Bai J., Jia J., Zhang G., Zhao Q., Lu Q., Cui B., et al. (2016). Spatial and temporal dynamics of heavy metal pollution and source identification in sediment cores from the short-term flooding riparian wetlands in a Chinese delta. Environ. pollut. 219, 379–388. doi: 10.1016/j.envpol.2016.05.016
Bai J., Zhao Q., Wang W., Wang X., Jia J., Cui B., et al. (2019). Arsenic and heavy metals pollution along a salinity gradient in drained coastal wetland soils: Depth distributions, sources and toxic risks. Ecol. Indic. 96, 91–98. doi: 10.1016/j.ecolind.2018.08.026
Chen R., Shen R., Gu P., Dong X., Du C., Ma J. (2006). Response of rice (Oryza sativa) with root surface iron plaque under aluminium stress. Ann. Bot.-London. 98 (2), 389–395. doi: 10.1093/aob/mcl110
China National Environmental Monitoring Center (1990). “The background concentrations of soil elements of China,” in China Environmental science (Press. Beijing (in chinese).
Cong P. (2021). Continuous change mapping to understand wetland quantity and quality evolution and driving forces: a case study in the liao river estuary from 1986 to 2018. Remote Sens.-Basel. 13, 4900. doi: 10.3390/rs13234900
Delavar M. A., Safari Y. (2016). Spatial distribution of heavy metals in soils and plants in zinc town, northwest Iran. Int. J. Environ. Sci. Technol. 13 (1), 297–306. doi: 10.1007/s13762-015-0868-0
Du Laing G., De Vos R., Vandecasteele B., Lesage E., Tack F. M. G., Verloo M. G. (2008). Effect of salinity on heavy metal mobility and availability in intertidal sediments of the scheldt estuary. Estuar. Coast. Shelf S. 77 (4), 589–602. doi: 10.1016/j.ecss.2007.10.017
Friedman S. P. (2005). Soil properties influencing apparent electrical conductivity: a review. Comput. Electron. Agr. 46 (1-3), 45–70. doi: 10.1016/j.compag.2004.11.001
Fritioff Å., Kautsky L., Greger M. (2005). Influence of temperature and salinity on heavy metal uptake by submersed plants. Environ. pollut. 133 (2), 265–274. doi: 10.1016/j.envpol.2004.05.036
Huang Q., Tang S., Huang X., Zhang F., Yi Q., Li P., et al. (2017). Influence of rice cultivation on the abundance and fractionation of fe, Mn, zn, Cu, and Al in acid sulfate paddy soils in the pearl river delta. Chem. Geol. 448, 93–99. doi: 10.1016/j.chemgeo.2016.11.012
Hussain M. M., Bibi I., Niazi N. K., Shahid M., Iqbal J., Shakoor M. B., et al. (2021). Arsenic biogeochemical cycling in paddy soil-rice system: Interaction with various factors, amendments and mineral nutrients. Sci. Total Environ. 773, 145040. doi: 10.1016/j.scitotenv.2021.145040
Impa S. M., Morete M. J., Ismail A. M., Schulin R., Johnson-Beebout S. E. (2013). Zn uptake, translocation and grain zn loading in rice (Oryza sativa l.) genotypes selected for zn deficiency tolerance and high grain zn. J. Exp. Bot. 64 (10), 2739–2751. doi: 10.1093/jxb/ert118
Islam M. M., Akther S. M., Hossain M. F., Parveen Z. (2022). Spatial distribution and ecological risk assessment of potentially toxic metals in the sundarbans mangrove soils of Bangladesh. Sci. Rep. 12 (1), 10422. doi: 10.1038/s41598-022-13609-z
Karstens S., Buczko U., Jurasinski G., Peticzka R., Glatzel S. (2016). Impact of adjacent land use on coastal wetland sediments. Sci. Total Environ. 550, 337–348. doi: 10.1016/j.scitotenv.2016.01.079
Khan N., Seshadri B., Bolan N., Saint C. P., Kirkham M. B., Chowdhury S., et al. (2016). Root iron plaque on wetland plants as a dynamic pool of nutrients and contaminants. Adv. Agron. 138, 1–96. doi: 10.1016/bs.agron.2016.04.002
Kowalska J., Mazurek R., Gąsiorek M., Setlak M., Zaleski T., Waroszewski J. (2016). Soil pollution indices conditioned by medieval metallurgical activity – a case study from Krakow (Poland). Environ. pollut. 218, 1023–1036. doi: 10.1016/j.envpol.2016.08.053
Li R. (2019). “Effect of wastewater irrigation and nitrogen fertilizer application on the bioavailablity of heavy metals in farmland soil,” (Lanzhou: Lanzhou Jiaotong University), 65.
Li W. C., Deng H., Wong M. H. (2016). Metal solubility and speciation under the influence of waterlogged condition and the presence of wetland plants. Geoderma. 270, 98–108. doi: 10.1016/j.geoderma.2015.10.012
Liu H., Xiong Z., Jiang X., Liu G., Liu W. (2016). Heavy metal concentrations in riparian soils along the han river, China: The importance of soil properties, topography and upland land use. Ecol. Eng. 97, 545–552. doi: 10.1016/j.ecoleng.2016.10.060
Liu F., Yang Z., Yang R., Wang R., Zhou J. (2022). Migration characteristics and potential ecological environment evaluation of metal elements in surface soil. KSCE J. Civ. Eng. 26 (5), 2068–2076. doi: 10.1007/s12205-022-1422-5
Mandour A., El-Sayed M. K., El-Gamal A. A., Khadr A. M., Elshazly A. (2021). Temporal distribution of trace metals pollution load index in the Nile delta coastal surface sediments. Mar. pollut. Bull. 167, 112290. doi: 10.1016/j.marpolbul.2021.112290
María-Cervantes A., Conesa H. M., González-Alcaraz M. N. (2010). Rhizosphere and flooding regime as key factors for the mobilisation of arsenic and potentially harmful metals in basic, mining-polluted salt marsh soils. Appl. Geochem. 25 (11), 1722–1733. doi: 10.1016/j.apgeochem.2010.08.019
Mei J., Jia J., Bi C., Liu J., Zeng Y., Chen Z. (2022). Effects of human enclosure and farming activities on heavy metals in sediments/soils of the coastal reclamation areas in the Yangtze estuary J. Soil. Sediment. 22, 2435–47. doi: 10.1007/s11368-022-03248-2
Memoli V., Esposito F., Panico S. C., De Marco A., Barile R., Maisto G. (2019). Evaluation of tourism impact on soil metal accumulation through single and integrated indices. Sci. Total Environ. 682, 685–691. doi: 10.1016/j.scitotenv.2019.05.211
Nguyen M. N., Tran T. M., Dang Q. T., Dinh V. M. (2022). Coastal paddies could emerge as hotspots of arsenic accumulation in rice: A perspective from the red river delta. Appl. Geochem. 142, 105330. doi: 10.1016/j.apgeochem.2022.105330
Pilbeam D. J. (2015). Breeding crops for improved mineral nutrition under climate change conditions. J. Exp. Bot. 66 (12), 3511–3521. doi: 10.1093/jxb/eru539
Song H., Sun Z. (2014). Temporal variations and bioaccumulation of heavy metals in different Suaeda salsa marshes of the yellow river estuary, china. environ. Sci. pollut. R. 21 (24), 14174–14187. doi: 10.1007/s11356-014-3296-7
Sundaramanickam A., Shanmugam N., Cholan S., Kumaresan S., Madeswaran P., Balasubramanian T. (2016). Spatial variability of heavy metals in estuarine, mangrove and coastal ecosystems along parangipettai, southeast coast of India. Environ. pollut. 218, 186–195. doi: 10.1016/j.envpol.2016.07.048
Tian Y., Luo L., Mao D., Wang Z., Li L., Liang J. (2017). Using landsat images to quantify different human threats to the shuangtai estuary ramsar site, China. Ocean Coast. Manage. 135, 56–64. doi: 10.1016/j.ocecoaman.2016.11.011
Wang G. D., Wang M., Xue Z. S., Ren Z. B., Jiang M., Otte M. (2022). Vegetation regulates element composition of soils by enhancing organic matter accumulation in the salt marshes of liao river delta, China. Front. Mar. Sci. 9. doi: 10.3389/fmars.2022.888605
Weiss D., Northover G., Hanif M., García-España E., Vilar R., Arnold T., et al. (2021). Isotope fractionation of zinc in the paddy rice soil-water environment and the role of 2’deoxymugineic acid (DMA) as zincophore under zn limiting conditions. Chem. Geol. 577, 120271. doi: 10.1016/j.chemgeo.2021.120271
White S. M., Madsen E. A. (2016). Tracking tidal inundation in a coastal salt marsh with helikite airphotos: Influence of hydrology on ecological zonation at crab haul creek, south Carolina. Remote Sens. Environ. 184, 605–614. doi: 10.1016/j.rse.2016.08.005
Wu Q., Bian F., Eller F., Wu M., Han G., Yu J., et al. (2022). Pollution levels and toxicity risks of heavy metals in different reed wetland soils following channel diversion in the yellow river delta. Wetlands 42 (4), 31. doi: 10.1007/s13157-022-01547-5
Wu Z., Chen Y., Han Y., Ke T., Liu Y. (2020). Identifying the influencing factors controlling the spatial variation of heavy metals in suburban soil using spatial regression models. Sci. Total Environ. 717, 137212. doi: 10.1016/j.scitotenv.2020.137212
Xu J., Wang X., Wang J., Xu L., Zheng X., Zhang Y., et al. (2021). Dominant environmental factors influencing soil metal concentrations of poyang lake wetland, China: Soil property, topography, plant species and wetland type. Catena 207, 105601. doi: 10.1016/j.catena.2021.105601
Xu J., Zheng L., Xu L., Wang X. (2020). Uptake and allocation of selected metals by dominant vegetation in poyang lake wetland: From rhizosphere to plant tissues. Catena (Giessen) 189, 104477. doi: 10.1016/j.catena.2020.104477
Yan J., Fischel M., Chen H., Siebecker M. G., Wang P., Zhao F., et al. (2021). Cadmium speciation and release kinetics in a paddy soil as affected by soil amendments and flooding-draining cycle. Environ. pollut. 268, 115944. doi: 10.1016/j.envpol.2020.115944
Yang Z., Lu W., Long Y., Bao X., Yang Q. (2011). Assessment of heavy metals contamination in urban topsoil from changchun city, China. J. Geochem. Explor. 108 (1), 27–38. doi: 10.1016/j.gexplo.2010.09.006
Yan X., Liu M., Zhong J., Guo J., Wu W. (2018). How human activities affect heavy metal contamination of soil and sediment in a long-term reclaimed area of the liaohe river delta, north China. Sustainability-Basel 10 (2), 338. doi: 10.3390/su10020338
Yan K., Planer-Friedrich B., Knobloch P. V. T., Guo Q., Wang L., Zhao Q. (2022). Effects of thiolation and methylation on arsenic sorption to geothermal sediments. Sci. Total Environ. 827, 154016. doi: 10.1016/j.scitotenv.2022.154016
Yin G., Zhu H., Chen Z., Su C., He Z., Chen X., et al. (2021). Spatial distribution and source apportionment of soil heavy metals in pearl river delta, China. Sustainability-Basel 13 (17), 9651. doi: 10.3390/su13179651
Yuan L., Guo P., Guo S., Wang J., Huang Y. (2021). Influence of electrical fields enhanced phytoremediation of multi-metal contaminated soil on soil parameters and plants uptake in different soil sections. Environ. Res. 198, 111290. doi: 10.1016/j.envres.2021.111290
Yu Y., Ling Y., Li Y. Z., Lv Z. B., Du Z. H., Guan B., et al. (2022). Distribution and influencing factors of metals in surface soil from the yellow river delta, China. Land 11 (4), 523. doi: 10.3390/land11040523
Zeng J., Luo X., Cheng Y., Ke W., Hartley W., Li C., et al. (2022). Spatial distribution of toxic metal(loid)s at an abandoned zinc smelting site, southern China. J. Hazard. Mater. 425, 127970. doi: 10.1016/j.jhazmat.2021.127970
Zhang H., Cui B., Zhang K. (2012). Surficial and vertical distribution of heavy metals in different estuary wetlands in the pearl river, south China. CLEAN-Soil Air Water. 40 (10), 1174–1184. doi: 10.1002/clen.201100730
Zhang Y., Mao W., Li R., Liu Y., Wang P., Zheng Z., et al. (2022). Distribution characteristics, risk assessment, and quantitative source apportionment of typical contaminants (HMs, n, p, and TOC) in river sediment under rapid urbanization: A study case of shenzhen river, pearl river delta, China. Process Saf. Environ. 162, 155–168. doi: 10.1016/j.psep.2022.03.032
Zhao Q., Bai J., Gao Y., Zhang G., Lu Q., Jia J. (2021). Heavy metal contamination in soils from freshwater wetlands to salt marshes in the yellow river estuary, China. Sci. Total Environ. 774, 145072. doi: 10.1016/j.scitotenv.2021.145072
Zhao Q., Bai J., Wang X., Zhang W., Huang Y., Wang L., et al. (2020). Soil organic carbon content and stock in wetlands with different hydrologic conditions in the yellow river delta, China. Ecohydrol. Hydrobiol. 20 (4), 537–547. doi: 10.1016/j.ecohyd.2019.10.008
Zhao S., Qin L., Wang L., Sun X., Yu L., Wang M., et al. (2022). Ecological risk thresholds for zn in Chinese soils. Sci. Total Environ. 833, 155182. doi: 10.1016/j.scitotenv.2022.155182
Zhao M., Wang E., Xia P., Feng A., Chi Y., Sun Y. (2019). Distribution and pollution assessment of heavy metals in the intertidal zone environments of typical sea areas in China. Mar. pollut. Bull. 138, 397–406. doi: 10.1016/j.marpolbul.2018.11.050
Keywords: metal, wetland type, depth distribution, risk assessment, Liaohe Delta
Citation: Yu Y, Zhang J, Qin J, Yang J, Wang Z, Song N and Yu J (2022) Characteristics and influencing factors of metal distribution in different wetlands of the Liaohe Delta, Northeastern China. Front. Mar. Sci. 9:1011457. doi: 10.3389/fmars.2022.1011457
Received: 04 August 2022; Accepted: 20 September 2022;
Published: 14 October 2022.
Edited by:
Tian Xie, Beijing Normal University, ChinaReviewed by:
Jia Jia, Yellow River Institute of Hydraulic Research, ChinaWangming Zhou, Key Laboratory of Forest Ecology and Management (CAS), China
Copyright © 2022 Yu, Zhang, Qin, Yang, Wang, Song and Yu. This is an open-access article distributed under the terms of the Creative Commons Attribution License (CC BY). The use, distribution or reproduction in other forums is permitted, provided the original author(s) and the copyright owner(s) are credited and that the original publication in this journal is cited, in accordance with accepted academic practice. No use, distribution or reproduction is permitted which does not comply with these terms.
*Correspondence: Jisong Yang, yangjisong@ldu.edu.cn; Junbao Yu, junbao.yu@gmail.com