- 1College of Marine and Environmental Sciences, Tianjin University of Science and Technology, Tianjin, China
- 2Research Centre for Indian Ocean Ecosystem, Tianjin University of Science and Technology, Tianjin, China
- 3Department of Biology and Chemistry, City University of Hong Kong, Hongkong, Hong Kong SAR, China
- 4College of Marine Science and Technology, China University of Geosciences (Wuhan), Wuhan, China
- 5Institute for Advanced Marine Research, China University of Geosciences, Guangzhou, China
Bottom trawling has widespread impact on the structure and composition of benthic communities. In this study, soft-bottom macrofauna in a heavily trawled area and an adjacent marine park that has been closed to bottom trawling for more than ten years were sampled, allowing to investigate the impact of and recovery from trawling. Both areas were equally affected by seasonal summer hypoxia. Polychaetes were the most abundant at trawled (78-86%) and non-trawled (85-94%) sites in both summer and winter. The non-trawled sites inside the marine park area had a lower level of species composition and abundance as compared to the trawled sites in summer, whereas in winter the benthic communities at these trawled and non-trawled sites appeared much similar with the majority of the identified taxa being dominated by new recruiting juveniles in both sampling areas. Results of permutational multivariate analysis of variance (PERMANOVA) on transformed infaunal abundance and biomass data revealed significant differences (p<0.05) between trawled and non-trawled treatments and seasons, as well as their interactions. Pair-wise a posteriori PERMANOVA further indicated significant differences for the abundance and biomass data in summer but insignificant differences in winter. PERMANOVA of the biological traits of infauna weighted with transformed biomass also confirmed significant difference for seasons only. Such findings imply that the effect of season was more important than that of trawling especially on the biological trait structure of the benthic communities. In addition, bottom trawling may contribute to a higher level of dissolved oxygen when the area suffered from hypoxia, resulting a higher biodiversity in the trawled area in summer. By comparing data taken prior to closure of the marine park from trawling, the recovery of infaunal community has been slow over the 12 years as a trawl-free area. Apart from natural disturbance of summer hypoxia, such recovery could also be complicated with the presence of artificial reefs deployed within the marine park, which may affect the hydrodynamics of the benthic environment and modify the community structure and function.
Introduction
Bottom trawling is the most widespread anthropogenic disturbance to the seabed globally (Halpern et al., 2008). When heavy trawling gear is dragged along the seabed, sediments are resuspended (O’Neill and Ivanović, 2016; Hiddink et al., 2017), seafloor habitats are damaged (Cook et al., 2013), and the benthic community structure is disrupted (Kaiser et al., 2000). Effects of bottom trawling, including reduced ecosystem functioning, decreased biomass and lowered diversity of benthic organisms, have been well documented in marine ecosystems, which eventually lead to an overall reduction in productivity in the long term (Queirós et al., 2006; Hinz et al., 2009; Sköld et al., 2018; Ramalho et al., 2020; McLaverty et al., 2021).
The degree of such impact depends on the characteristics of the trawling gear (Steele et al., 2002; Rijnsdorp et al., 2015), type of benthic habitat (Koslow et al., 2001; Kenchington et al., 2006; Queirós et al., 2006), trawling frequencies (Enticknap, 2002), size of the trawled area (Watling and Norse, 1998), and natural disturbances such as seasonal changes in water quality and hydrodynamics in the local environment (Ortega et al., 2018). Bottom trawling in vulnerable habitats, e.g., coral reefs, can be particularly damaging and cause substantial declines in associated benthic communities (Althaus et al., 2009; Cook et al., 2013; Williams et al., 2020). However, effects of trawling in coastal soft-bottom areas under frequent natural disturbances may not be so obvious or even negligible as community response to both anthropogenic (e.g., trawling) and natural disturbance (e.g., hypoxic events) could be similar (Moritz et al., 2015; Szostek et al., 2016). For example, frequent natural disturbance resulting from seasonal storm events has led to an increase in the abundance of small-bodied, opportunistic and short-lived (r-selected) species with a concomitant loss of larger-bodied, longer-lived and slower growing (K-selected) species (Anderlini and Wear, 1992). Similarly, bottom trawling can also cause a shift of trait composition of benthic species with the dominance of r-selected characters (Mangano et al., 2013; Kaiser et al., 2018). Hence the impact of trawling on the benthic communities may not be easily observed in areas where natural disturbance frequently occurs (van Denderen et al., 2015; Ortega et al., 2018).
Although the adverse consequences of trawling on the seabed fauna are widely documented, relative few studies have been conducted on the recovery of benthic communities from cessation of trawling operations (Hiddink et al., 2006b; Hiddink et al., 2017). The difficulty of following such a recovery process is that trawling has usually operated for a long time and there are little or no data prior to trawling for comparison (Thrush and Dayton, 2002). By collating available data from 24 comparative and 46 experimental studies of trawling impacts on communities of soft-bottom macroinvertebrates, Hiddink et al. (2017) estimated that the recovery rates of benthic communities after trawling is stopped would range between 1.9 and 6.4 years. A meta-analysis of 122 experiments on the effects of bottom trawling to evaluate rates of recovery following disturbance also revealed that sessile and low mobility biota with longer life-spans, such as sponges, soft corals and bivalves, would take longer (>3 years) to recover from bottom trawling than mobile biota (<1 year) with shorter life-spans, such as polychaetes and most crustaceans (Sciberras et al., 2018). However, these estimations were based on the assumption that the observed state of biota is in equilibrium with the reported trawling effort. Other environmental drivers which may exist and mask the impact caused by trawling, such as natural disturbance resulting from seasonal hypoxic events, were not explicitly considered. Hence, empirical studies are needed to determine the trawling impacts and recovery of benthic ecosystems in areas where natural disturbance phenomena, such as seasonal hypoxia, are frequent.
Hong Kong lies within the subtropical region in the northern part of the South China Sea and is influenced by the estuary of the Pearl River from the west and seasonal currents and monsoons from the east. The territory is known to have a long fishing history with heavy trawling in most areas around the coast. A port survey, conducted by the Agriculture, Fisheries and Conservation Department (AFCD) of the Government of the Hong Kong Special Administrative Region (HKSAR), China, has shown that nearly all Hong Kong’s territorial waters have been trawled, except for a few small protected areas and shipping channels (Agriculture, Fisheries and Conservation Department, 2006). In 2010, up to 400 trawlers operated partly or wholly within the Hong Kong waters accounting for 80% of the total fishing efforts estimated to be 93% higher than the maximum sustainable fishing yield (Wang et al., 2021). In order to rehabilitate the damaged habitats and depletion of marine resources, the HKSAR Government imposed a permanent territory-wide ban on all types of trawling operations since the end of 2012.
Hoi Ha Wan Marine Park is a semi-enclosed bay within Tolo Channel on the northeast of Hong Kong (Figure 1). The park, covering an area of 260 hectares, is a sheltered bay with pristine water quality and provides a good marine environment for nurturing a great variety of organisms and habitats, including coral reef, hard rock, mangroves, sandy and soft muddy bottom. It is also a trawl-free area since the designation of the marine park in 1996. In contrast, outside the park boundary, the seabed of Tolo Channel has been intensively trawled until the ban started at the end of 2012. Both the park and channel, however, have been affected by seasonal changes of water quality due to heavy rainfall and limited water exchanges with frequent occurrences of hypoxia in the summer (Wu, 1988; Fleddum et al., 2011). These two sites thus provided an opportunity to investigate the potential changes in structure and function of soft-bottom benthic communities through comparison of data obtained from the park (i.e., non-trawled area) and channel (i.e., trawled area), so as to decipher possible community recovery from cessation of trawling under seasonal disturbance. Such studies are relatively lacking in tropical and subtropical waters (Wang et al., 2021).
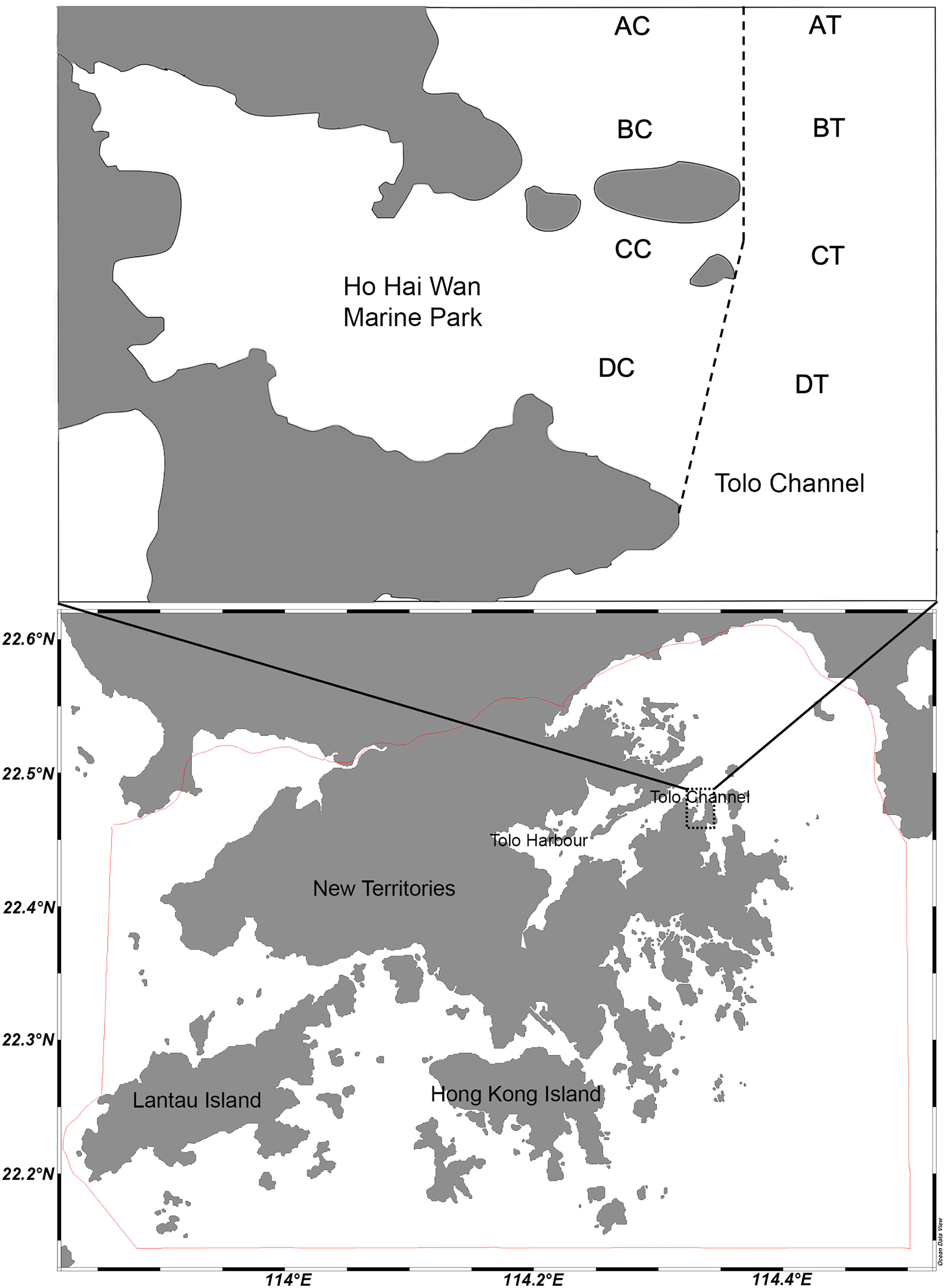
Figure 1 Map showing sampling sites AC-DC in the non-trawled area inside the Hoi Ha Wan Marine Park and AT-DT in the trawled area along the Tolo Channel. The dotted line indicates the boundary of the marine park and the red line shows the boundary of territorial waters of Hong Kong.
The aims of this study were to (1) investigate the trawling impact on the structure and function of infaunal communities in subtropical waters that experience significant seasonal changes in dissolved oxygen, and (2) address the possibility of recovery inside the marine park by comparing with the heavily-trawled area nearby. Since benthic organisms generally have a life cycle time between 40 days to 10 years (Watling and Norse, 1998), it is expected that the seabed environment has been stabilized from previous historical trawling operations and has undergone various successional stages after the area has been protected as a marine park since 1996. It is hypothesized that biodiversity, with higher biomass and more rare species (i.e., species with one individual recorded), would have increased in the park during the protected time and larger, longer-lived species would dominate. Our findings could provide new evidence regarding the recovery of benthic communities from bottom trawling in subtropical coastal waters where natural disturbance such as seasonal hypoxia is frequent.
Materials and methods
Design of the case study
Figure 1 and Table S1 show the location and information of the eight sampling sites for macrobenthic infauna in Hoi Ha Wan Marine Park and nearby Tolo Channel, with four non-trawled sites AC to DC inside the park boundary and four trawled sites AT to DT along the channel. During the study period, two paired Chinese traditional shrimp trawlers with six connected bottom nets (each of about 2 m width) were observed in the morning and in the afternoon, covering the whole area of the Tolo Channel during the day. This fishing pattern was regular in this particular area except at AT where some large stones are located at the bottom and trawling frequency was lower. The trawling intensity was, on average, five trawls a week for the trawled sites BT, CT and DT and one time a week for site AT (WWF Hong Kong, pers. comm.). Artificial reefs and coral communities are located in the marine park area. Hence, the location of sampling sites AC to DC was chosen carefully to minimize interference with these structures.
Field sampling and environmental measurements
Five replicates of 0.1 m2 van Veen grab were used to collect infaunal samples at each of the trawled (AT, BT, CT and DT) and non-trawled sites (AC, BC, CC and DC), respectively, at the beginning of October 2008 (end of summer, wet season) and at the beginning of March 2009 (end of winter, dry season) before the implementation of territory-wide trawl ban at the end of 2012. Additionally three replicate samples were taken for measurement of total organic carbon (TOC) and for particle size analysis in the laboratory. Prior to field sampling, a diver went down to the seabed at each site and took photographs of the sediment surface to make sure that those designated trawled sites along the channel had actually been trawled with evidence of track marks while those non-trawled sites within the park were not disturbed. Water depth, temperature and dissolved oxygen (DO) were also measured at the surface of the bottom sediment at each site by a diver using a hand-held digital depth gauge, thermometer and optical dissolved oxygen sensor (model D-Opto, Zebra-Tech Ltd., USA) respectively. Each replicate of grab sediments collected for faunal analysis was washed over a sieve with a 0.5 mm mesh opening on board. All residues were preserved in 70% ethanol and stained with Rose Bengal.
Laboratory analyses
Upon arrival to the laboratory, the sediment samples for TOC were frozen at 20°C for 24 h followed by freeze-drying. The freeze-dried sediments were homogenized to<0.25 mm with a mechanical homogenizer and stored in a desiccator for further analyses. TOC in sediment was then measured following the Walkley–Black wet titration method (Walkley and Black, 1934). For particle size analysis, the wet-sieving method was performed according to Buchanan (1984). A geometric grade scale modified by Wentworth in Φ values (Buchanan, 1984) was used to classify the grain size, in which higher Φ values represent finer sediments (Table S2).
The residues collected from the infaunal samples were sorted under a dissecting microscope and animals were picked, and later identified to lowest possible taxa and counted. The biomass (wet weight) was measured using an electronic balance for each species, after blotting the animals on filter paper for one minute before weighing to the nearest 0.01 g, since most of the animals were small and juvenile. Larger animals were blotted on filter paper for 2-3 minutes according to Jennings et al. (2002). Tube-forming polychaetes were removed from tubes before weighing. For damaged specimens, the following procedures, according to Jennings et al. (2002), were applied: (1) ‘complete’ animals were assembled from fragments in the sample as far as possible and recorded as single individuals and (2) if fragments of an animal constituted less than 30% of the expected mass of a complete animal, they were discarded.
Classification of biological traits
Macrofaunal genera and species were classified into seven biological traits according to the body size, larval type, mobility, body form, degree of attachment, living habit and feeding habit. These traits were subdivided into 36 categories (Table S3) and each category was scored according to the affinity of each individual taxon for each trait category, ranging from 0 - 3, with 0 being no affinity and 3 being total affinity, which is referred as fuzzy-coding (Chevene et al., 1994). Information of biological traits was referenced from Fleddum (2010), based on various literature sources including scientific publications, theses, web databases and expert knowledge. When there was no trait information for a species, with the exception of the body size, the trait scores from the same genus were used since it is generally accepted that there are similar traits between species of the same genus (Fleddum, 2010). In this study, changes in functional composition on biomass were adopted in the biological trait analysis as many important ecological processes are strongly affected by the biomass of important functional groups within the benthic community (Tillin et al., 2006).
Data treatment and statistical analysis
Six environmental parameters including depth, DO, temperature, trawling intensity, TOC and sediment median diameter (MD Φ) were examined using normalized principal component analysis (PCA) with Euclidean distance to investigate the variance within the sampling sites and between the trawled and non-trawled treatments for each season (Pielou, 1984). Percentage of TOC was arcsine transformed before data being normalized. Permutational multivariate analysis of variance (PERMANOVA) was also performed to test for significant differences in environmental variables between trawled and non-trawled treatments and between summer and winter seasons (Anderson et al., 2008).
The infaunal data of five grab replicates at each site were pooled as one sample. Both abundance and biomass data were square-root transformed to reduce the effect of species with high abundance or biomass, and the dominant taxa groups were calculated as percentages, Shannon diversity (H’) and Pielou evenness (J’) of the infauna for each site were determined based on the transformed pooled abundance data (Schratzberger and Jennings, 2002). Principal coordinate analysis (PCoA) of the transformed, pooled biomass was applied to elucidate community structure between treatments and seasons (Anderson et al., 2008). In the present study, biomass data were adopted for PCoA, as many important ecological processes are strongly affected by the biomass of important functional groups within the benthic community (Tillin et al., 2006). PERMANOVA was also conducted to test for differences in infaunal abundance and biomass between treatments and seasons, with “treatments” (fixed factor) crossed with “seasons” (fixed factor). Where differences or interactions were significant, pair-wise permutation tests were used to further explore these differences and the contribution of species to the average Bray-Curtis dissimilarity between treatments and seasons were further examined with similarity percentage (SIMPER) analysis (Clarke and Gorley, 2006). The species abundance data were also categorized in log2 classes to depict the distribution of commonly and rarely occurring species for both treatments and seasons.
Similarly, to evaluate the biological trait relationship from the infaunal samples, PCoA of the weighted biological traits with square-root transformed biomass was conducted between trawled and non-trawled treatments and seasons. PERMANOVA was also applied to test differences for weighted biological traits between treatments and seasons, followed by pair-wise permutation tests if significant differences or interactions were found. The contribution of biological trait categories to the average Bray-Curtis dissimilarity was further examined with SIMPER analysis and the categories in each biological trait were compared using the Mann-Whitney U test, if significant differences were noted between treatments and seasons from PERMANOVA. The biological trait contribution for rare species from summer and winter (trawled and non-trawled treatments) was also presented as percent of the whole trait pool. Although such rare species occur in less abundance, they collectively contribute to, and exert a greater effect, on ecosystem functioning (Ellingsen et al., 2007).
All the above multivariate analyses were performed with PRIMER-E v.6 and its add-on package PERMANOVA+ (PRIMER-E, Plymouth Marine Laboratory, UK), whereas the determination of Mann-Whitney U test was conducted through STATISTICA v.8 (StatSoft, Oklahoma, USA).
Results
Environmental variables
The entire study area was considered to be fairly uniform with respect to bottom depth, temperature, DO, TOC and sediment median diameter (MD Φ) during the sampling period within each season (Table 1). Water depth at the trawled sites was between 18 and 23 m, whereas at the non-trawled sites between 14 and 16 m. The temperature ranged from 26.66 to 27.18°C in summer and 19.01 to 19.86°C in winter across all the sampling sites. The DO at the seabed was higher in winter (>8 ppm) than summer (~5-6 pm) for both trawled and non-trawled sites, and slightly higher DO was registered for the non-trawled sites (summer 6.29-6.56, winter 8.85-9.05 ppm) than the trawled sites (summer 5.31-6.50 ppm, winter 8.38-8.91 ppm). The percentage of TOC was higher for both trawled and non-trawled sites in summer (2.03-2.54%) than winter (1.06-1.94%). Across all the sampling sites, the sediment was classified as silt (Table S2) with sediment median diameter between 5.72 and 6.21 Φ, with exception at the trawled site AT (4.65-5.56 Φ). Figure 2 depicts the relationship between the sampling sites and environmental variables from the PCA results. The first two axes from the PCA plot contributed to 85% (eigenvalue = 2.64 and 2.46) of the variance in summer (Figure 2A) and 87.3% (eigenvalue = 3.1 and 2.14) in winter (Figure 2B). The grouping of the sampling sites was quite similar for both seasons, with the trawled sites being positioned to the right and non-trawled sites at the upper left. Except for AT, the grouping of the other three trawled sites was correlated with water depth, trawling intensity and, to a lesser extent, TOC, whereas for the four non-trawled sites, they were correlated with DO and median sediment diameter. The trawled site AT showed a different pattern than the other trawled sites with lower trawling intensity and coarser sediment (Table 1).
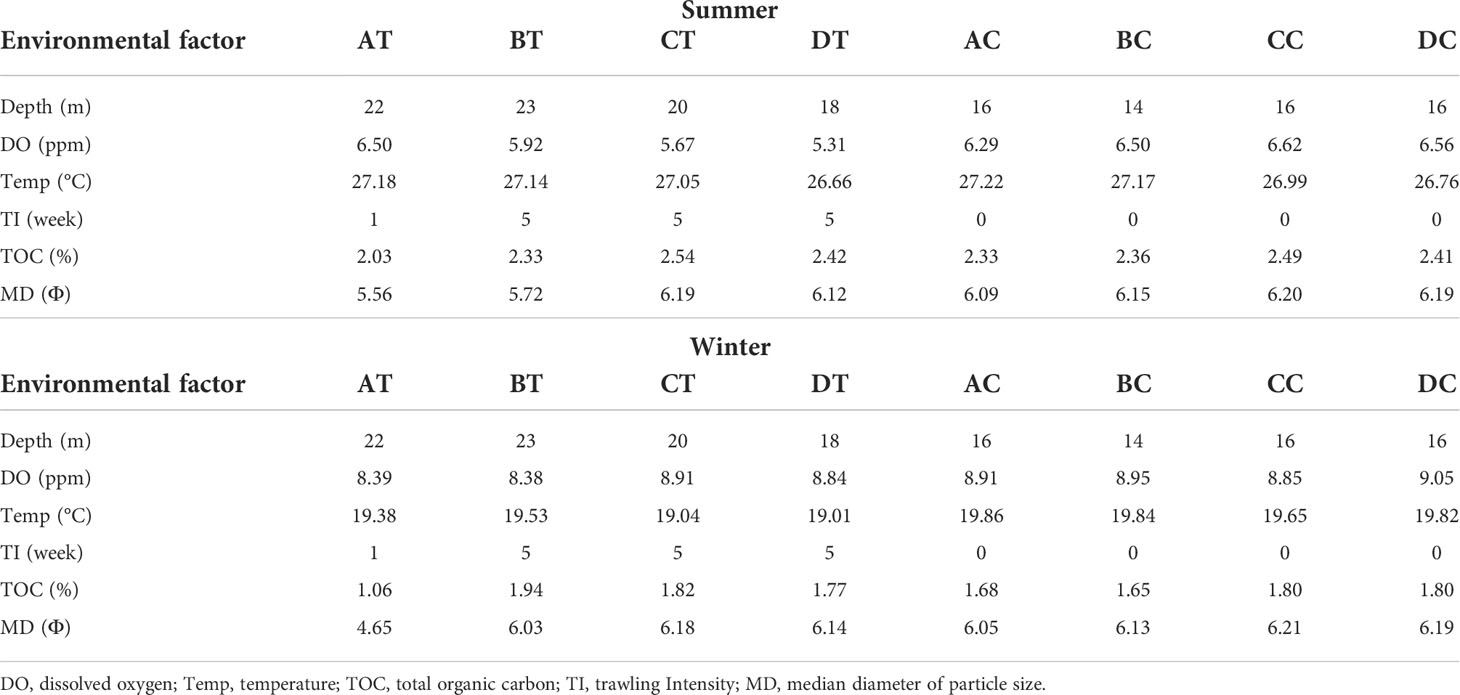
Table 1 Summary of the environmental characteristics and trawling intensity per week of all the sampling sites in trawled and non-trawled treatments and seasons.
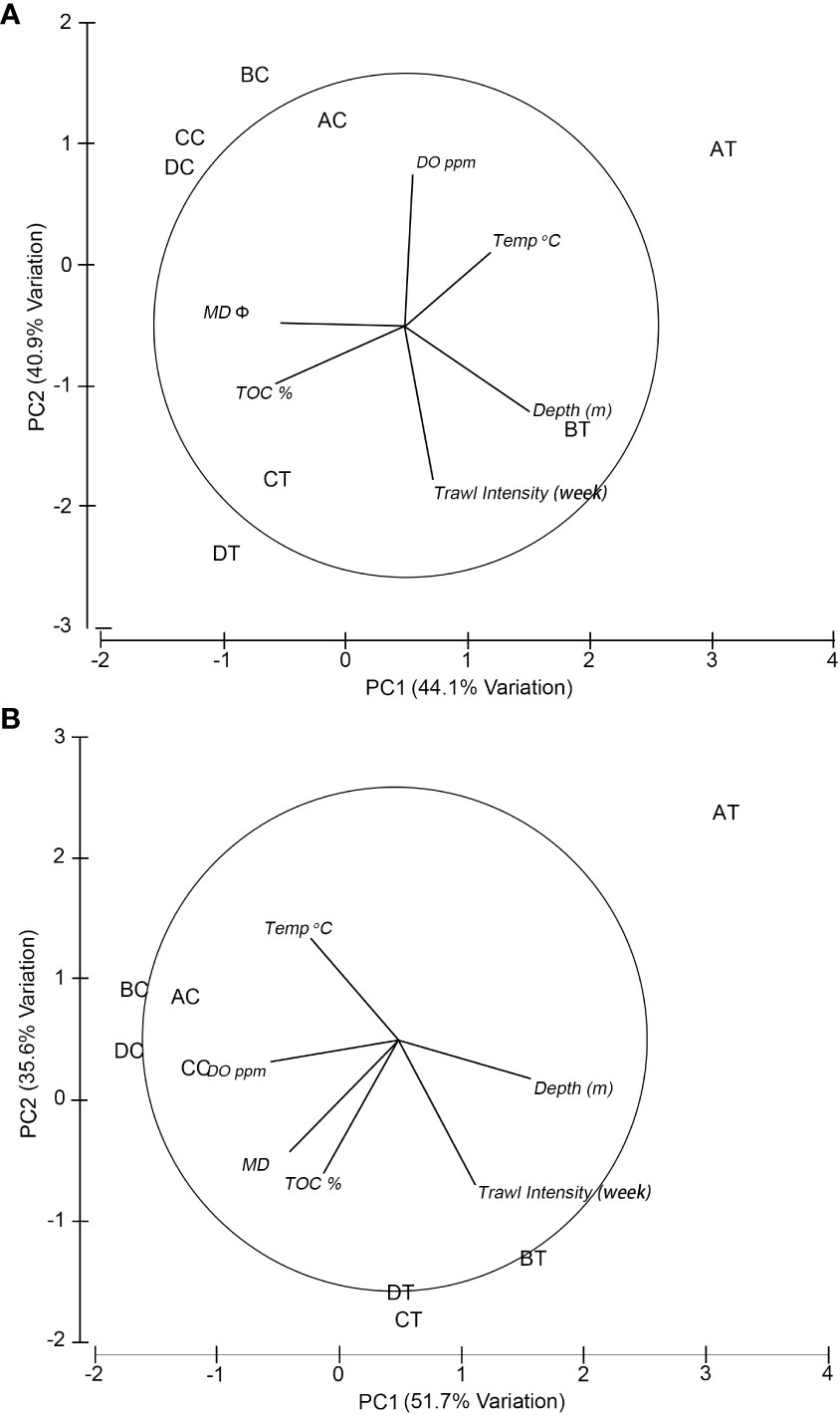
Figure 2 Principal component analysis (PCA) of normalised environmental factors using Euclidean distance for (A) summer and (B) winter. The lines show the direction of the environmental factors that are related to the sampling sites. AT-DT, trawling sites; AC-DC, non-trawled sites; Temp, temperature; DO, dissolved oxygen; TOC, total organic carbon; MD Φ, median sediment diameter in Φ scale (see Table S2).
A PERMANOVA test showed significant differences in environmental factors between trawled and non-trawled treatments (pseudo-F = 7.900, df = 1, p = 0.001) and seasons (pseudo-F = 12.610, df = 1, p = 0.001) but not significant for the interaction between treatments and seasons (pseudo-F = 0.110, df = 1, p = 0.927).
Macrobenthic community structure
A total of 77 species were identified for both seasons and treatments (41 in summer and 57 in winter, sharing 20 of the same taxa). Polychaetes were the most dominant taxa for all the sampling sites (non-trawled area: summer 94%; winter 85%; trawled area: summer 78%; winter 86% of total species) (Table S4). The highest percentage composition of crustaceans was found at the trawled sites in summer (14%), whereas the highest percentage composition of molluscs was recorded at these same sites in winter (7%).
In summer, the non-trawled sites inside the protected marine park area had a low level of species composition and abundance (Table 2). Among these four sites, BC recorded the highest species number (11/0.5 m2), total biomass (4.79 g/0.5 m2), number of individuals (17/0.5 m2), J’ (0.95) and loge H’ (2.28). In comparison with the non-trawled sites, except for J’, higher species richness, abundance, biomass and diversity index were recorded at the trawled sites with BT recording the highest species composition (23/0.5 m2), total biomass (3.87 g/0.5 m2) and loge H’ (2.84). Amongst these four trawled sites, AT also had the highest number of individuals (63/0.5 m2) and CT the highest J’ value (0.93). In contrast to summer, the macrobenthic community structure showed changes in winter (Table 2), in which the most of the identified taxa were dominated by new recruiting juveniles in both sampling areas. All the diversity indices increased at all sites with the exception of BT, which had a smaller species composition but more individuals. The infauna recorded from both trawled and non-trawled sites also revealed more similarities in winter for all other measurements, except for AT, which had lower trawling intensity but highest species number (31/0.5 m2), total biomass (21.53 g/0.5 m2) and number of individuals (353/0.5 m2).
The results from PCoA showed that the first axis contributed 25.4% of the total data variation and the second axis 13.3% (Figure 3). The PCoA plot of infaunal samples depicted distinct separation between seasons with winter samples located at the left side, closer to PCo2, while the summer samples located at the right side of the plot. Although the non-trawled site CC in summer had only one species with the largest contribution to the data variance, its position on the PCoA plot was still closer to other non-trawled sites than those trawled sites in summer.
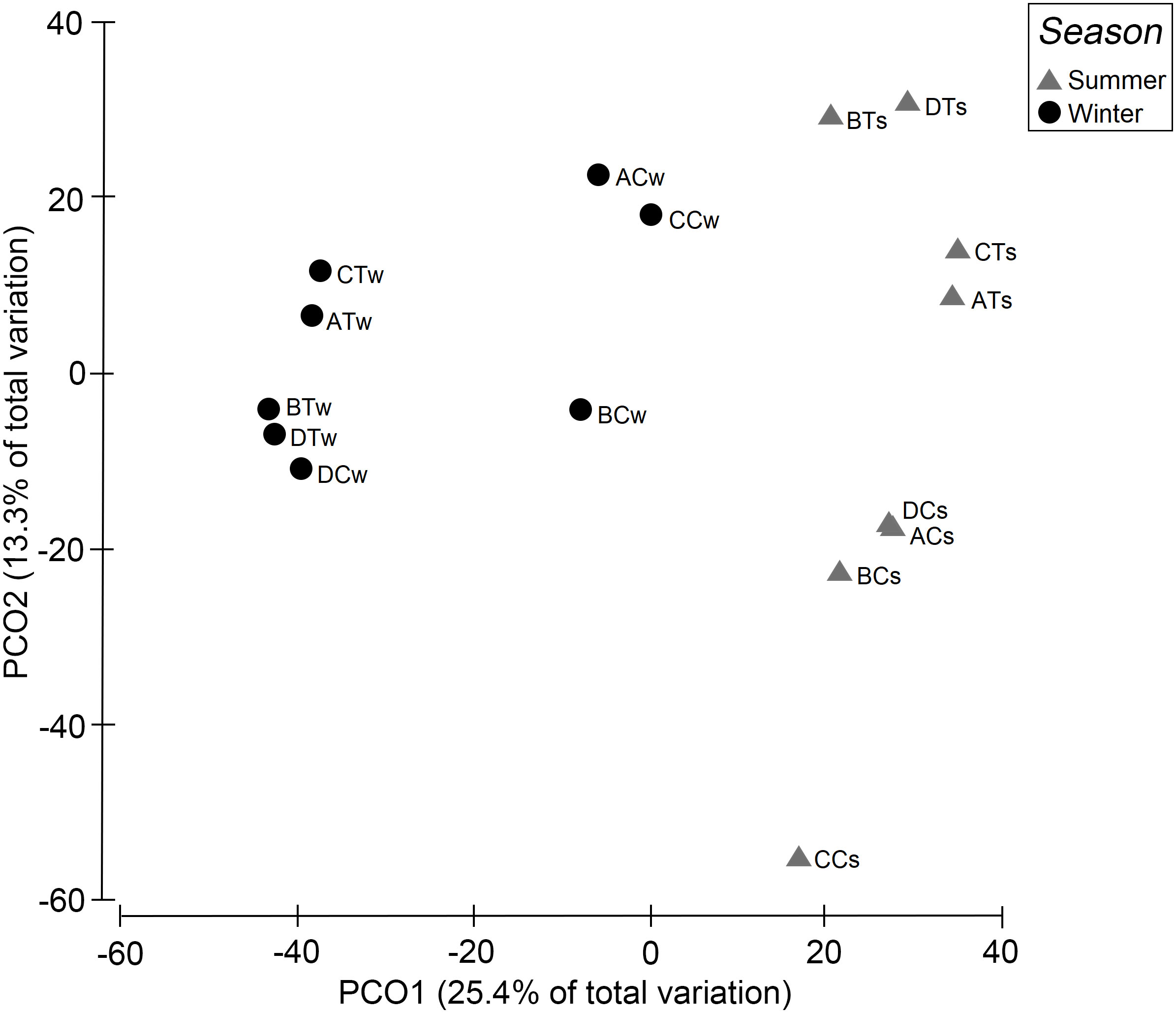
Figure 3 Principal coordinate analysis (PCoA) of the transformed pooled biomass (g/0.5 m2) for treatments (AT-DT = trawled sites, AC-DC = non-trawled sites) and seasons (s, summer; w, winter).
PERMANOVA on the transformed abundance data identified significant differences between trawled and non-trawled treatments (pseudo-F = 2.899, df = 1, p = 0.002), seasons (pseudo-F = 6.354, df = 1, p = 0.001) and the interaction between treatments and seasons (pseudo-F = 2.438, df = 1, p = 0.004). Similarly, PERMANOVA of the transformed biomass data also indicated significant differences between treatment (pseudo-F = 2.024, df = 1, p = 0.007), seasons (pseudo-F = 4.598, df = 1, p = 0.001) and interaction between treatments and seasons (pseudo-F = 1.950, df = 1, p = 0.016). Pair-wise a posteriori PERMANOVA between trawled and non-trawled sites for abundance and biomass data revealed significant differences for the biomass and abundance in summer but insignificant differences in winter (Table S5).
SIMPER analysis revealed that some of the same species, including the polychaetes Chaetozone setosa, Aglaophamus dibranchis and Sigambra hanaokai, and the ribbon worm (Nemertea) Cerebratulidae sp., occurred in both treatments and/or in both seasons with slight differences in cumulative percentages in abundance (Table 3). Other most numerically dominant species recorded in summer were the polychaete Heteromastus filiformis at the non-trawled sites and the polychaete Euryothoe sp. and the tube-building amphipod Jassa marmorata from the trawled sites; whereas in winter, these comprised the polychaetes Prionospio ehlersi, P. malmgreni and Schistomeringos rudolphi at the non-trawled sites and the bivalves Tellina sp. and the peanut worm (Sipuncula) Apionsoma trichocephalus from the trawled areas.
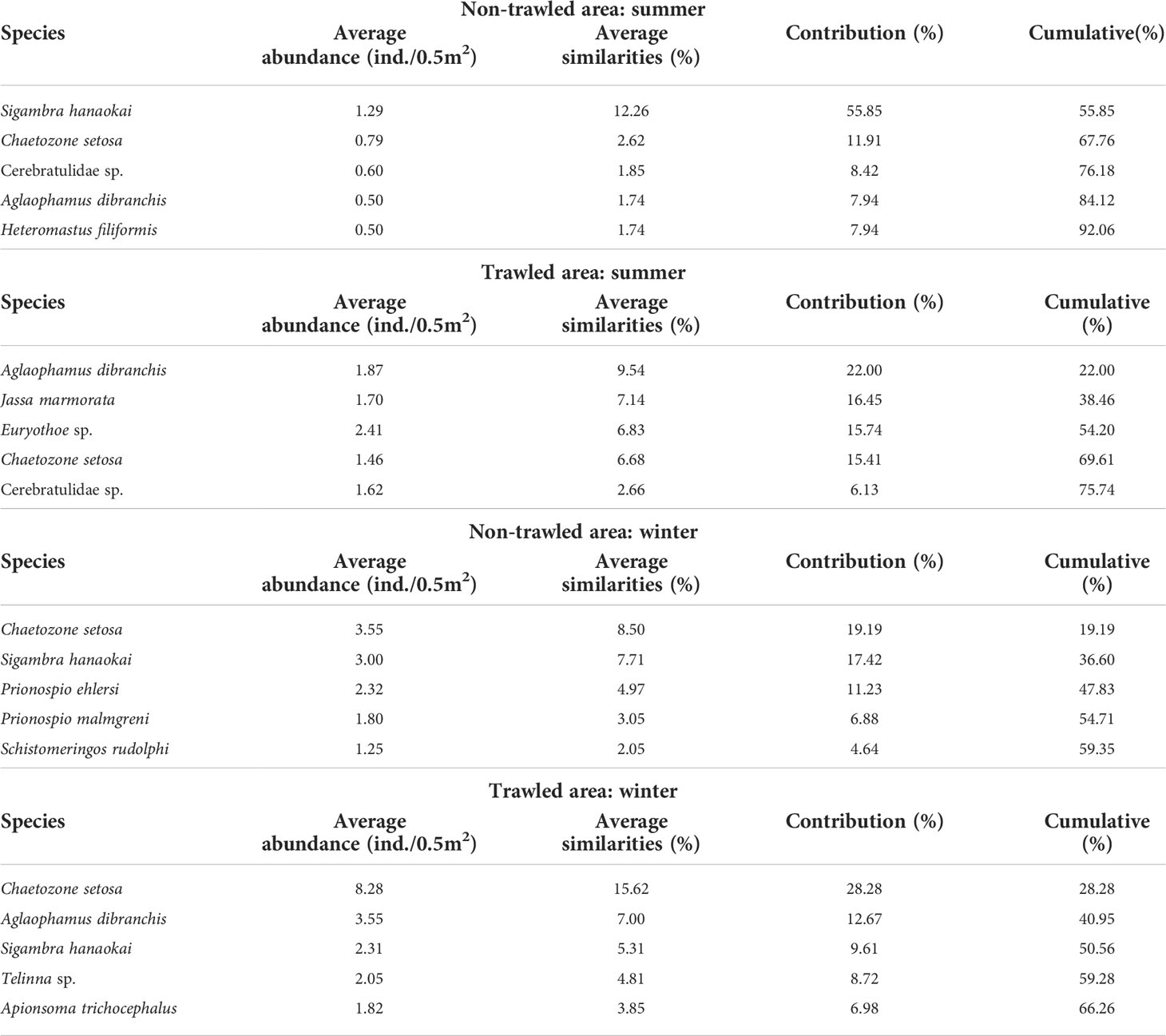
Table 3 The top five species contribution from SIMPER (Similarity Percentages) for summer and winter sampling.
The infauna from the summer sampling consisted more rare species (i.e., represented only by one individual) in the pooled samples than that from winter for the non-trawled sites (13/2 m2 versus 7/2 m2), whereas an opposite trend was noted for the trawled sites (13/2 m2 versus 19/2 m2) (Figure 4). The distributions of the abundance classes were overall similar at both the trawled and non-trawled sites in winter compared to summer, as there were fewer species and individuals recorded at the non-trawled sites in summer.
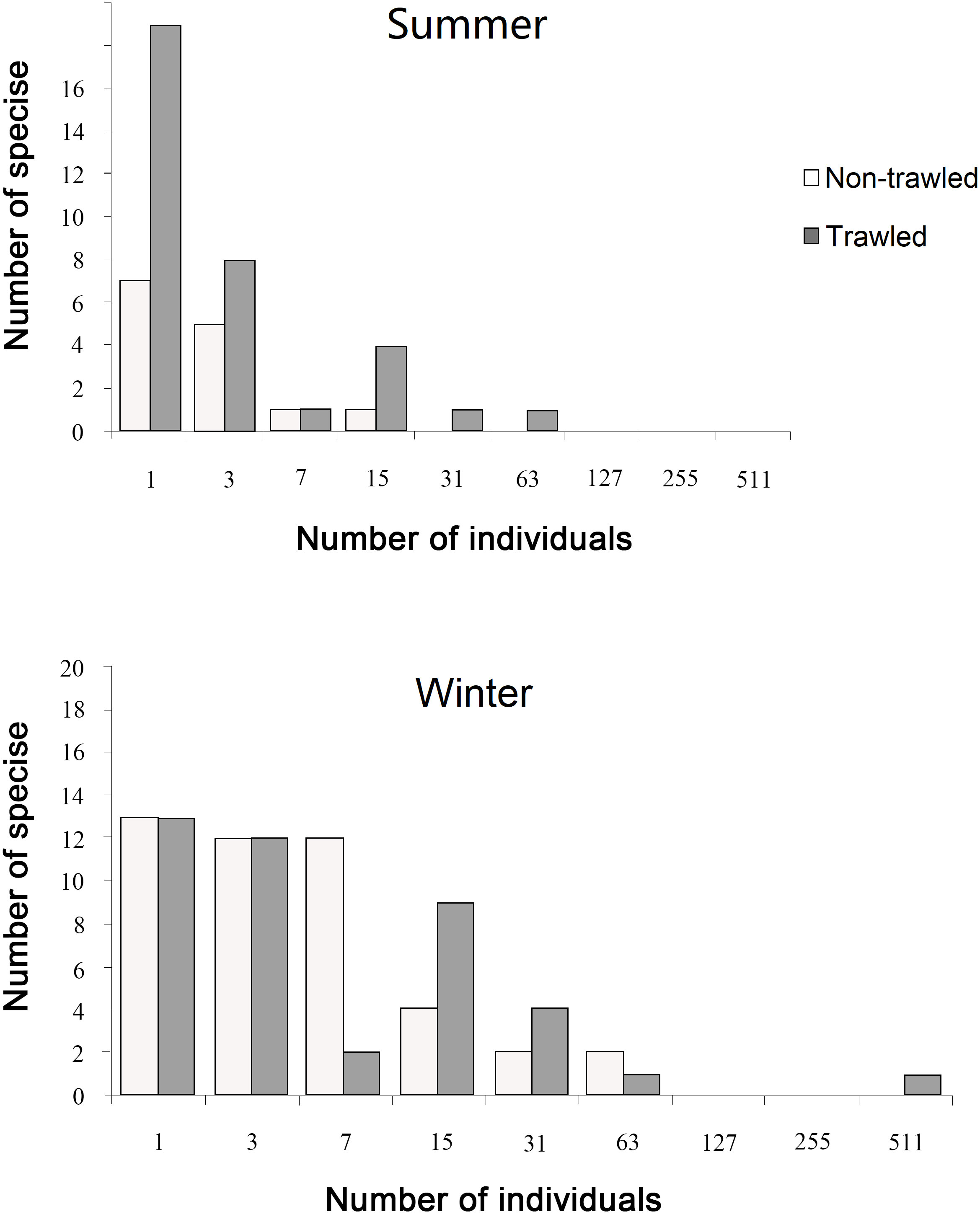
Figure 4 Species abundance distribution for each season and each treatment (trawled and non-trawled). The x-axis is in logarithmic scale (modified log2 classes). Class 1 represents 1 individual, 3 represents between 2-3 individuals, 7 represents 4-7 individuals, etc.
Biological trait analysis
The results from PCoA of biological traits showed that the first axis contributed to 44.5% of the total variation and the second axis 27% (Figure 5). The distance between seasons in the plot decreased after traits were weighed in with the biomass. With only one species, and low trait contribution, the non-trawled site CC was positioned closest to PCo1 and had the largest contribution to data variance. There was no clear grouping between the trawled and non-trawled treatments in summer or winter.
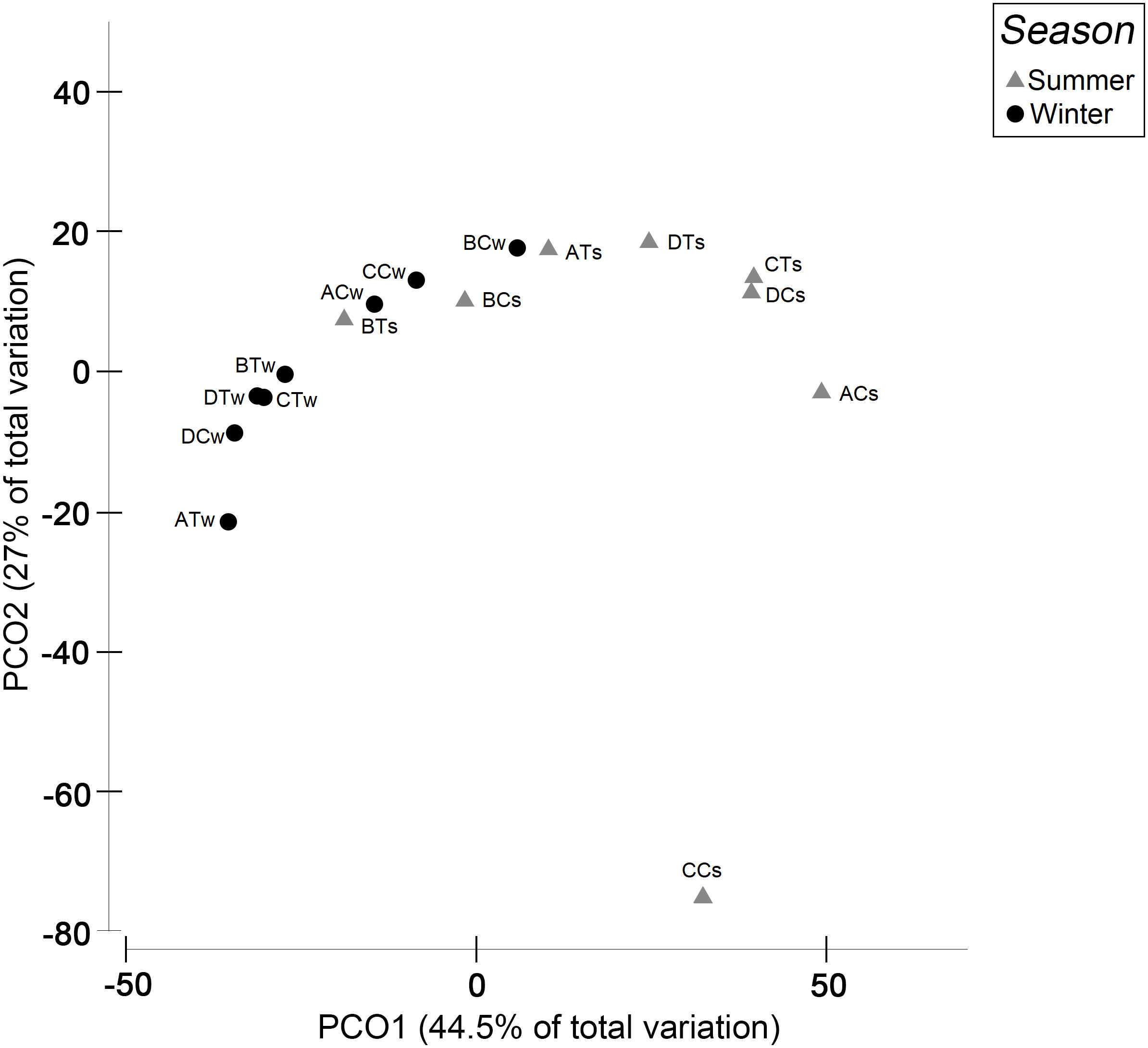
Figure 5 Principal coordinate analysis (PCoA) of weighted biological traits with square-root transformed biomass for treatments (AT-DT = trawled sites, AC-DC = non-trawled sites) and seasons (s, summer; w, winter).
PERMANOVA of the weighted trait data showed no significant differences between treatments (pseudo-F = 1.419, df = 1, p = 0.179) and interaction between treatments and seasons (pseudo-F = 1.939, df = 1, p = 0.066), but significant difference for seasons (pseudo-F = 5.793, df = 1, p = 0.003). Further exploration of the results from SIMPER and significant biological traits with Mann-Whitney U test between seasons is listed in Table 4. Nineteen of the 36 biological trait categories analyzed had up to 90% contribution to Bray-Curtis dissimilarity of 64.6% between seasons. The total rare species contributions to the biological trait pool were also lower for the non-trawled compared to the trawled sites in summer (Figure 6). However, in winter, the total rare species contribution at the non-trawled sites increased in several biological trait categories and was higher than that at the trawled sites, including species with smaller body size (<5 mm to 1 cm), planktotrophic or direct larval development, less motility, laterally flat or ball shape, temporary or permanent attachment, sessile life or surface crawling, and suspension or scavenger feeding (Figure 6; Table S3).
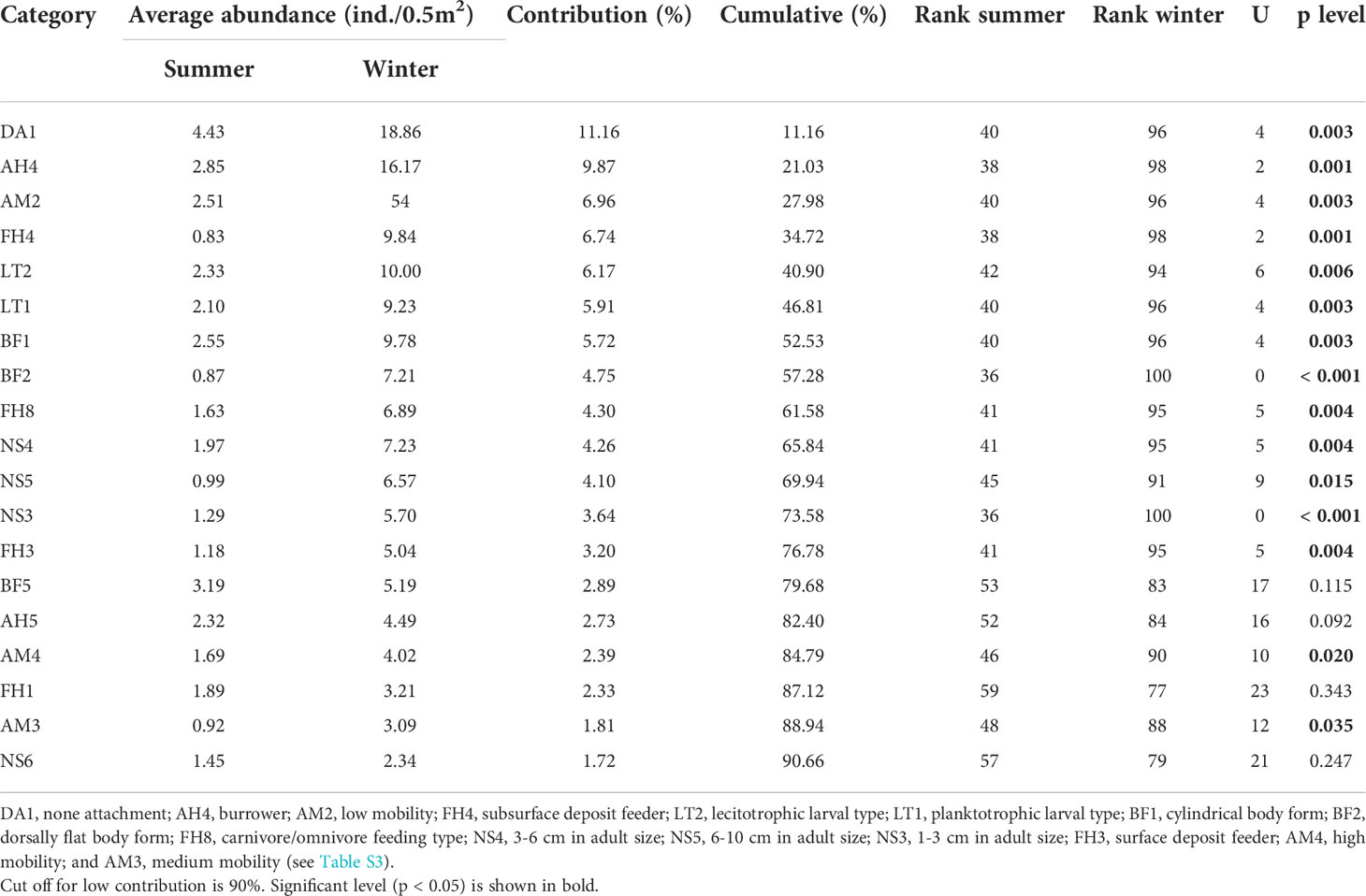
Table 4 Results of contribution of biological trait categories from SIMPER analysis and Mann-Whitney U test between seasons.
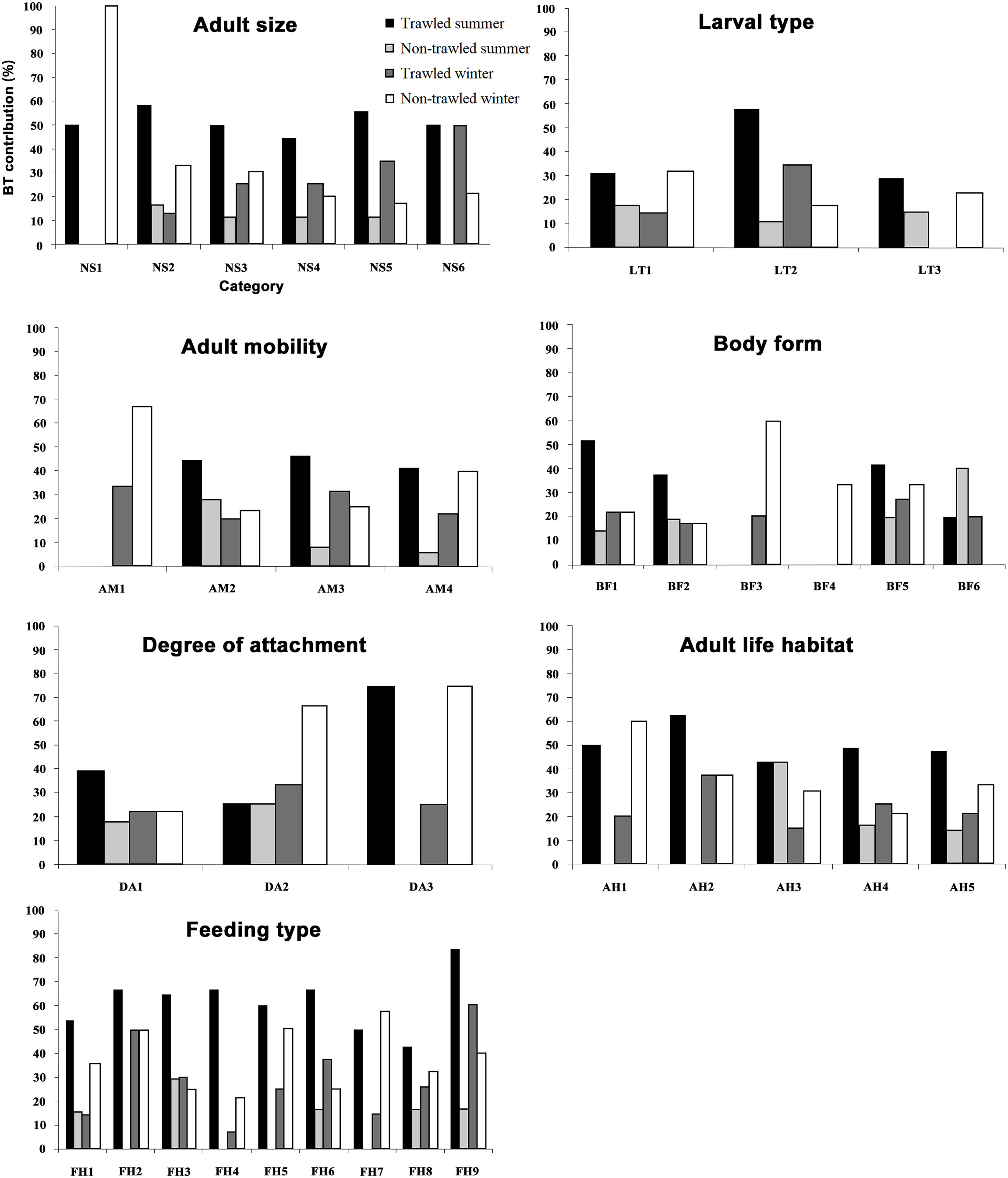
Figure 6 Biological trait (BT) contribution for rare species from summer and winter (trawled and non-trawled treatments) measured as percent of the whole trait pool. The biological trait is ranked as presence/absence. (Size, NS1:< 5 mm; NS2: 5 mm-1 cm; NS3: 1-3 cm; NS4: 3-6 cm; NS5: 6-10 cm; NS6: > 10 cm; Larval type, LT1: Planktotroph; LT2: Lecitotroph; LT3: Direct development; Mobility, AM1: None; AM2: Low; AM3: Medium; AM4: High; Body form, BF1: Short/cylindrical; BF2: Dorsally flat; BF3: Laterally flat; BF4: Ball shape; BF5: Long thin; BF6: Irregular; Attachment, DA1: None; DA2: Temporary; DA3: Permanent; Life Habitat, AH1: Sessile; AH2: Tube (permanent); AH3: Tube (semi-permanent); AH4: Burrower; AH5: Surface crawler; Feeding, FH1: Suspension/filter; FH2: Scraper/grazer; FH3: Surface deposit feeder; FH4: Subsurface deposit feeder; FH5: Dissolved matter/symbiont; FH6: Large detritus/sand licker; FH7: Scavenger; FH8: Carnivore/omnivore;FH9: Parasitic/commensal).
Discussion
Environmental parameters in trawled and non-trawled areas
The entire study area was considered uniform with respect to the measured environmental variables during the sampling period within summer and winter. The DO at the bottom was slightly higher in winter and the in-situ DO measurements in summer at the sampling time showed no signs of hypoxia. However, the study area is well-known for hypoxic periods during summer. Earlier DO measurements in the same sampling area taken during summer confirmed low oxygen (DO<2 ppm) at certain times of the year (Wu and Richards, 1979; Horikoshi and Thompson, 1980; Wu, 1982; Wu, 1988; Fleddum et al., 2011). Seasonal changes of other water quality parameters such as , and in the study area were minimal based on the online EPD monitoring database (2022). To better detect the effects of changes in macrobenthic community structure for each season, the end of summer and the end of winter were chosen for this study. This could be the reason for relatively higher DO recorded at the bottom during the end of summer, despite possibly lower DO occurrences during the mid-summer period, prior to the present field sampling. There were significant differences in environmental factors between trawled and non-trawled treatments and seasons but not for the interaction between treatments and seasons. This suggested that seasons are important in environmental changes for the whole sampling area but are not the only cause of the differences between trawled and non-trawled treatments. The trawled site AT, which had lower trawling intensity and coarser sediment, showed a different pattern than the other trawled sites in macrobenthic composition. The largest difference between the trawled and non-trawled areas was the trawling intensity. Trawling is known to change environmental factors, such as grain size moving towards finer fractions (Pilskaln et al., 1998; Reiss et al., 2009), TOC towards higher percentage levels (Tuck et al., 1998; Reiss et al., 2009) and DO towards higher levels (De Borger et al., 2021). However, the trend of decrease in median sediment diameter and increase in TOC in the trawled area as compared to the non-trawled area was not clear in the present study (Table 1). In addition, as the present field sampling was conducted at the end of summer instead of mid-summer when hypoxic events can be more frequent, the increase in DO caused by bottom trawling was not clearly demonstrated.
Effects of trawling on macrobenthic community
The largest differences between the trawled and non-trawled treatments were found during summer, with significantly higher abundance, biomass, biodiversity and numbers of rare species in the trawled area. The non-trawled sites inside the marine park had a lower level of species composition and abundance, and several of the grab samples were void of macrofauna. In summer, the structure and biological trait composition of macrobenthic communities in the trawled area were also significantly more diverse than that in the non-trawled area. Such differences in benthic community in favour of trawling during summer in the present study were unexpected. This finding was not in line with the hypothesis that intensive trawling activity results in a shift from K-selected to r-selected species, with a decrease in species diversity and rare species (de Juan and Demestre, 2012; Kaiser et al., 2018). It appeared that in Tolo Channel, summer may be favourable to trawling disturbances, relative to the environment in the protected, non-trawled area. Trawling can modify macrobenthic community structure and a higher abundance of opportunistic species is known to occur (Thrush and Dayton, 2002; McLaverty et al., 2020). However, higher abundance does not necessarily mean that the community is not disturbed. Watling and Norse (1998) compared trawling activities to forest cutting. Forest cutting is a change in the habitat from one type of community to another. The forest goes through different successional stages until, after several years, it reaches a similar habitat and community to what it was before, if the disturbance ceases. In that process, the biodiversity of opportunistic species increases until the strongest organisms inhabit and displace the weaker ones. This can be compared to trawling, in which the disturbed habitat may change its environmental factors and, in turn, affects the structure of the community. Intermediate disturbance hypothesis proposed by Connell (1978) has often been associated with some level of the successional stages and can be the reason for the highest biodiversity at the trawled site AT, where trawling occurred, but at a lower intensity. The succession that the benthic marine environment goes through will depend on the type of bottom and how it has been changed (Lu and Wu, 2000). The coral community may take decades to be repaired (MacNeil et al., 2015). Communities at the continental shelves and slopes, which have fewer natural disturbances, also have a slower recovery time, compared to areas with natural disturbances (Watling and Norse, 1998; Dernie et al., 2003). For communities in muddy environments, a few years should be enough to restore it back to what it was pre-trawled (Allen and Clarke, 2007; Sciberras et al., 2018). In the present study, repeated low summer DO events, coupled with heavy trawling activities, may have modified the macrobenthic community structure in Tolo Channel. Soft-bottom benthic communities with natural disturbances, such as hypoxia, could benefit from trawling in harsh times (in this case, wet, summer in Hong Kong) to some extent. One can consider that there is a trade-off between trawling and hypoxia. In winter, when DO was high and there was no occurrence of hypoxia, there were no significant differences in community structure between the trawled and non-trawled areas. Hypoxia is a natural event in the area and it is known to reduce the biodiversity drastically in the summer for both epi- and infauna; however, a rapid recovery of community structure to normal conditions has been noted in winter (Wu, 1988; Fleddum et al., 2011). It is thus suggested that trawling could contribute to a higher level of DO in a time when the area suffers from hypoxia (De Borger et al., 2021), thus increasing the chance of survival for the benthic animals. This might be an explanation of the higher biodiversity in summer in the trawled area as compared to the non-trawled sites. In a study of benthic communities in the southern Baltic Sea, van Denderen et al. (2022) reported that hypoxia may impact the community structure more significantly than trawling. Another explanation for the present findings could be that trawling may enhance the conditions of the seabed by removing large sessile organisms, such as sponges and soft corals, disturb the sea bottom and make food more available for some fish species (Rijnsdorp and Vingerhoed, 2001), which prey on invertebrates, such as polychaetes and crustaceans. However, it is difficult to estimate the exact impact of trawling in any study. Pitcher et al. (2000) removed seven tonnes of epifaunal biomass from the seabed in north-eastern Australia and still did not find any significant differences in the benthic communities in the trawled areas, which is an example of what is obvious but is not detectable (Thrush and Dayton, 2002).
It is complicated to evaluate the effects in areas with high natural disturbances (Drabsch et al., 2001; Kaiser et al., 2006). Natural disturbances can be factors such as wave forces, severe storms, earthquakes, volcanic activities and seasonal changes including hypoxia and heavy rainfall or large temperature differences during summer or winter. The marine fauna are affected by these natural factors and, therefore, it may be difficult to distinguish trawling from the effects of natural disturbances (Rijnsdorp et al., 2015). van Denderen et al. (2015) examined the effects of trawling and natural disturbance on benthic communities over gradients of commercial bottom trawling effort in the North and Irish Seas. They found that the impact of trawling was greatest in areas with low levels of natural disturbance, while similar impact was small in areas with high level of natural disturbance.
Recovery from trawling in the marine park
Recovery is a return of environmental variables and species composition to a state that existed before the disturbance occurred. Recovery from trawling depends on types of habitat (Collie et al., 2000; Eno et al., 2013), frequency of disturbance compared with natural changes (Hiddink et al., 2006a; van Denderen et al., 2015), species and life history characteristics (Hiddink et al., 2018) and the size of the area disturbed (Preciado et al., 2019). There are hardly any data on the macrobenthic community inside the Hoi Ha Wan Marine Park before the Government closed the area to trawling in 1996. However, Mackie et al. (1993) performed a survey in Hoi Ha Wan and Tolo Channel in April 1989 using duplicate 0.1 m2 van Veen grab (pool of two replicates) to investigate the macrofaunal community. This is the only set of data that can be used to compare with those obtained from the present study, since the four non-trawled sampling sites were located in the same survey area. In general, the abundance, number of species and Shannon diversity H´ were higher in 1989, while the evenness J´ was higher in this study, indicating a stronger similarity in abundance among the species and fewer numerically dominant species were found in the present case. Mackie et al. (1993) recorded the dominance of the polychaete Minuspio sp. in Hoi Ha Wan and Tolo Channel, which can serve as an indicator of organic pollution. In this study, there were only two individuals of Minuspio cirrifera found inside the protected marine park in summer and none in the trawled area along the Tolo Channel. The polychaetes Sigambra sp. and Aglaophamus sp. in the 1989 survey were among the top five abundant species, similar to this study, but the abundance was quite different. Among top five species, the abundance ranged from 11-260/0.2 m2 in April 1989, while 0-4/0.5 m2 were recorded in summer and 11-25/0.5 m2 in winter in the present study. Only three individuals of Chaetozone sp. were observed in the same area in 1989, while in this study the species C. setosa were among the most numerically dominant. Polychaetes were found to dominate in both studies. The trend in the infaunal communities of subtropical Hong Kong is known to exhibit low species richness and biodiversity, but high abundance and evenness, in undisturbed localities when compared to temperate regions (Mackie et al., 1993). This trend is not fully understood, but some factors, such as reduced salinity, large annual temperature fluctuations, intense biological interactions, high sediment silt-clay content, sediment instability and negative redox potential could be contributing to this state (Shin and Thompson, 1982; Shin, 1989).
Through a review of trawling data of experimental and comparative studies mainly from temperate waters, Hiddink et al. (2017) estimated the recovery rates of biota after trawling would range between 1.9 and 6.4 years. Using a similar review approach, Sciberras et al. (2018) further indicated that for motile and short life-span species, such as polychaetes and most crustaceans, the recovery rate from trawling could be less than 1 year. Hence, the implication from such estimations is that biota within Hoi Ha Wan Marine Park should have had enough time to recover from trawling after 12 years as a trawl-free area. However this was not the case, according to the data obtained in the present study. The low biomass of infauna recorded in the marine park was surprising and contradicted to our hypothesis that a higher biodiversity with larger body-sized and longer-lived species would be found in the non-trawled marine park area, as compared to the heavily trawled area in Tolo Channel. The marine park covers 260 hectares; however, there is no prior knowledge on what extent of seabed within the park was affected by bottom trawling in the past as recovery from trawling would depend on the size of the area that has been disturbed (Thrush et al., 1998; Sciberras et al., 2013). A recent study, conducted in subtropical Hong Kong by comparing of surveys conducted in June 2012 (before the territory-wide trawl ban in Hong Kong) and June 2015 (2.5 years after the ban), revealed a significant increase in site-based abundance, species richness, functional diversity and among-site similarity of macrobenthos after the trawl ban, except in Tolo Harbour and Tolo Channel which is influenced by seasonal hypoxic events (Wang et al., 2021). With natural disturbances, such as an annual hypoxic season, the non-trawled sites in Ho Hai Wan Marine Park would need several more years in order to achieve a recovery back to its former state. However, the recruitment rate in the non-trawled area appeared to be rapid since the biodiversity managed to restore itself during winter.
The hypoxic situation in the marine park area is a natural phenomenon, which is likely not the reason for the decreasing trend in biodiversity. Another important consideration is that the marine park itself may have contributed to the changes through time. It is now widespread that marine protected areas are effective tools for conserving marine ecosystems (Kelleher, 1999; Lubchenco et al., 2003; Palumbi, 2003). Studies have observed ranges of responses to such protected waters, such as increases in total biodiversity (Edgar and Barrett, 1999), abundance or biomass (Russ et al., 2004; Abesamis and Russ, 2005), frequency of aggressive interactions between adults within the same species (Abesamis and Russ, 2005), and mean body size (Edgar and Barrett, 1999; Tuya et al., 2000; Abesamis and Russ, 2005). However, there could be short- and long-term consequences to marine protected areas that have been exposed to impact, in varying degrees. After the Government closed the area for trawling, several artificial reefs have been deployed inside the marine park, with the intention to increase fish biodiversity (Wilson et al., 2002). These reefs are in a distance of at least 15 m from the four non-trawled sampling sites; however, whether or not the reef structures would affect the infuanl community around through changes in hydrodynamics of the benthic environment (Shin et al., 2014) is not clear. Hence in the present study, apart from seasonal hypoxic events, the infaunal community within the marine park may also be influenced by the presence of the artificial reefs over these years.
Conclusion
The present study was conducted in a heavily trawled area and a nearby marine park which has been closed to bottom trawling for more than 10 years, enabling the examination of the impact of and recovery from trawling on benthic communities. As the study sites were influenced by summer hypoxia, the results obtained imply that natural disturbance may exert a greater influence on the community structure and function than from trawling. Moreover, bottom trawling may contribute to a higher level of DO in a time when the area suffers from hypoxia, thus favouring the survival of benthic animals. By comparing data taken prior to closure of the marine park from trawling, the recovery of infaunal community appeared to be slow over the 12 years as a trawl-free area. Such recovery could also be complicated with the presence of artificial reefs deployed within the marine park, which may affect the hydrodynamics of the benthic environment and modify the community structure and function. Further studies on the structure and function of benthic communities, both on spatial changes around the artificial reefs and temporal changes over time, are thus suggested within the marine park area and in adjacent waters, so as to investigate the longer-term recovery progress of benthic ecosystems after the trawl ban.
Data availability statement
The raw data supporting the conclusions of this article will be made available by the authors, without undue reservation.
Author contributions
PS designed the study and finalized the manuscript. WX assisted in the study and revised the manuscript. AF conducted the field and laboratory work and wrote the manuscript. JS provided comments on the drafts of the manuscript. All authors contributed to the article and approved the submitted version.
Funding
This research was supported by a postgraduate studentship awarded to AF at City University of Hong Kong, Hong Kong SAR, China.
Acknowledgments
Thanks for Paul Hodgson and his diving team for in-situ measurements of water depth, temperature and DO and checking the seabed condition prior to field sampling over the study period.
Conflict of interest
The authors declare that the research was conducted in the absence of any commercial or financial relationships that could be construed as a potential conflict of interest.
Publisher’s note
All claims expressed in this article are solely those of the authors and do not necessarily represent those of their affiliated organizations, or those of the publisher, the editors and the reviewers. Any product that may be evaluated in this article, or claim that may be made by its manufacturer, is not guaranteed or endorsed by the publisher.
Supplementary material
The Supplementary Material for this article can be found online at: https://www.frontiersin.org/articles/10.3389/fmars.2022.1010909/full#supplementary-material
References
Abesamis R. A., Russ G. R. (2005). Density-dependent spillover from a marine reserve: Long-term evidence. Ecol. Appl. 15 (5), 1798–1812. doi: 10.1890/05-0174
Agriculture, Fisheries and Conservation Department (2006) Agriculture, fisheries and conservation department port survey. Available at: https://www.afcd.gov.hk/english/fisheries/fish_cap/fish_cap_latest/fish_cap_latest.html.
Allen J. I., Clarke K. R. (2007). Effects of demersal trawling on ecosystem functioning in the north Sea: A modelling study. Mar. Ecol. Prog. Ser. 336, 63–75. doi: 10.3354/meps336063
Althaus F., Williams A., Schlacher T. A., Kloser R. J., Green M. A., Barker B. A., et al. (2009). Impacts of bottom trawling on deep-coral ecosystems of seamounts are long-lasting. Mar. Ecol. Prog. Ser. 397, 279–294. doi: 10.3354/meps08248
Anderlini V. C., Wear R. G. (1992). The effect of sewage and natural seasonal disturbances on benthic macrofaunal communities in Fitzroy bay, Wellington, new Zealand. Mar. pollut. Bull. 24 (1), 21–26. doi: 10.1016/0025-326X(92)90312-T
Anderson M. J., Gorley R. N., Clarke K. R. (2008). PERMANOVA+ for PRIMER: Guide to software and statistical methods (Plymouth, UK: PRIMER-E).
Buchanan J. B. (1984). “Sediment analysis,” in Methods for the study of marine benthos. IBP handbook second ed, 2nd ed., vol. vol. 16 . Eds. Holme N. A., McIntyre A. D. (Oxford, UK: Blackwell Scientific Publications), 41–65.
Chevene F., Doledec S., Chessel D. (1994). A fuzzy coding approach for the analysis of long-term ecological data. Freshw. Biol. 31, 295–309. doi: 10.1111/j.1365-2427.1994.tb01742.x
Collie J. S., Hall S. J., Kaiser M. J., Poiner I. R. (2000). A quantitative analysis of fishing impacts on shelf-sea benthos. J. Anim. Ecol. 69, 785–798. doi: 10.1046/j.1365-2656.2000.00434.x
Connell J. H. (1978). Diversity in tropical rain forests and coral reefs: high diversity of trees and corals is maintained only in a nonequilibrium state. Science 199, 1302–1310. doi: 10.1126/science.199.4335.1302
Cook R., Fariñas-Franco J. M., Gell F. R., Holt R. H. F., Holt T., Lindenbaum C., et al. (2013). The substantial first impact of bottom fishing on rare biodiversity hotspots: A dilemma for evidence-based conservation. PloS One 8, e69904. doi: 10.1371/journal.pone.0069904
De Borger E., Tiano J., Braeckman U., Rijnsdorp A. D., Soetaert K. (2021). Impact of bottom trawling on sediment biogeochemistry: a modelling approach. Biogeosciences 18, 2539–2557. doi: 10.5194/bg-18-2539-2021
de Juan S., Demestre M. (2012). A trawl disturbance indicator to quantify large scale fishing impact on benthic ecosystems. Ecol. Indic. 18, 183–190. doi: 10.1016/j.ecolind.2011.11.020
Dernie K. M., Kaiser M. J., Warwick R. M. (2003). Recovery rates of benthic communities following physical disturbance. J. Anim. Ecol. 72 (6), 1043–1056. doi: 10.1046/j.1365-2656.2003.00775.x
Drabsch S. L., Tanner J. E., Connell S. D. (2001). Limited infaunal response to experimental trawling in previously untrawled areas. Ices. J. Mar. Sci. 58 (6), 1261–1271. doi: 10.1006/jmsc.2001.1105
Edgar G. J., Barrett N. S. (1999). Effects of the declaration of marine reserves on Tasmanian reef fishes, invertebrates and plants. J. Exp. Mar. Biol. Ecol. 242 (1), 107–144. doi: 10.1016/S0022-0981(99)00098-2
Ellingsen K. E., Hewitt J. E., Thrush S. F. (2007). Rare species, habitat diversity and functional redundancy in marine benthos. J. Sea. Res. 58 (4), 291–301. doi: 10.1016/j.seares.2007.10.001
Eno N. C., Frid C. L. J., Hall K., Ramsay K., Sharp R. A. M., Brazier D. P., et al. (2013). Assessing the sensitivity of habitats to fishing: from seabed maps to sensitivity maps. J. Fish. Biol. 83 (4), 826–846. doi: 10.1111/jfb.12132
Enticknap B. (2002). Trawling the North Pacific. Understanding the effects of bottom trawl. Fisheries on alaska’s living seafloor. (Anchorage, AK:Alaska marine conservation council, fisheries project coordinator). 22 pp.
EPD (2022) Marine water quality data (Hong Kong S.A.R: Environmental Protection Department). Available at: https://cd.epic.epd.gov.hk/EPICRIVER/marine/ (Accessed 1 September 2022).
Fleddum A. L. (2010). Effects of human disturbance on biological traits and structure of macrobenthic communities. PhD thesis (Hong Kong: City University of Hong Kong), 325 pp.
Fleddum A. L., Cheung S. G., Hodgson P., Shin P. K. S. (2011). Impact of hypoxia on the structure and function of benthic epifauna in tolo harbour, Hong Kong. Mar. pollut. Bull. 63, 221–229. doi: 10.1016/j.marpolbul.2011.03.019
Halpern B. S., Walbridge S., Selkoe K. A., Kappel C. V., Micheli F., D’Agrosa C., et al. (2008). A global map of human impact on marine ecosystems. Science 319, 948–952. doi: 10.1126/science.1149345
Hiddink J. G., Jennings S., Kaiser M. J. (2006a). Indicators of the ecological impact of bottom-trawl disturbance on seabed communities. Ecosystems 9, 1190–1199. doi: 10.1007/s10021-005-0164-9
Hiddink J. G., Jennings S., Kaiser M. J., Queirós A. M., Duplisea D. E., Piet G. J. (2006b). Cumulative impacts of seabed trawl disturbance on benthic biomass, production, and species richness in different habitats. Can. J. Fish. Aquat. Sci. 63 (4), 721–736. doi: 10.1139/f05-266
Hiddink J. G., Jennings S., Sciberras M., Bolam S. G., Cambiè G., McConnaughey R. A., et al. (2018). Assessing bottom trawling impacts based on the longevity of benthic invertebrates. J. Appl. Ecol. 56 (5), 1075–1084. doi: 10.1111/1365-2664.13278
Hiddink J. G., Jennings S., Sciberras M., Szostek C. L., Hughes K. M., Ellis N., et al. (2017). Global analysis of depletion and recovery of seabed biota after bottom trawling disturbance. Proc. Natl. Acad. Sci. U.S.A. 114, 8301–8306. doi: 10.1073/pnas.1618858114
Hinz H., Prieto V., Kaiser M. J. (2009). Trawl disturbance on benthic communities: chronic effects and experimental predictions. Ecol. Appl. 19 (3), 761–773. doi: 10.1890/08-0351.1
Horikoshi L. B., Thompson G. B. (1980). “Distribution of subtidal molluscs collected by trawling in tolo harbour and tolo channel, Hong Kong, with special reference to habitat segregation in two venerid bivalves,” in The malacofauna of Hong Kong and southern China. Ed. Morton B. (Hongkong, China:Hong Kong University Press), 149–162.
Jennings S., Nicholson M. D., Dinmore T. A., Lancaster J. E. (2002). Effects of chronic trawling disturbance on the production of infaunal communities. Mar. Ecol. Prog. Ser. 243, 251–260. doi: 10.3354/meps243251
Kaiser M. J., Clarke K. R., Hinz H., Austen M. C. V., Somerfield P. J., Karakassis I. (2006). Global analysis of response and recovery of benthic biota to fishing. Mar. Ecol. Prog. Ser. 311, 1–14. doi: 10.3354/meps311001
Kaiser M. J., Hormbrey S., Booth J. R., Hinz H., Hiddink J. G. (2018). Recovery linked to life history of sessile epifauna following exclusion of towed mobile fishing gear. J. Appl. Ecol. 55, 1060–1070. doi: 10.1111/1365-2664.13087
Kaiser M. J., Ramsay K., Richardson C. A., Spence F. E., Brand A. R. (2000). Chronic fishing disturbance has changed shelf sea benthic community structure. J. Anim. Ecol. 69, 494–503. doi: 10.1046/j.1365-2656.2000.00412.x
Kelleher G. (1999). Guidelines for marine protected areas (Switzerland and Cambridge UK: IUCN, Gland), 107 pp.
Kenchington E. L. R., Gilkinson K. D., Kevin G., MacIsaac K. G., Bourbonnais-Boyce C., Kenchington T. J., et al. (2006). Effects of experimental otter trawling on benthic assemblages on Western bank, northwest Atlantic ocean. J. Sea. Res. 56, 249–270. doi: 10.1016/j.seares.2006.03.010
Koslow J. A., Gowlett-Holmes K., Lowry J. K., O’Hara T., Poore G. C. B., Williams A. (2001). Seamount benthic macrofauna off southern Tasmania: community structure and impacts of trawling. Mar. Ecol. Prog. Ser. 213, 111–125. doi: 10.3354/meps213111
Lubchenco J., Palumbi S. R., Gaines S. D., Andelman S. (2003). Plugging a hole in the ocean: The emerging science of marine reserves. Ecol. Appl. 13, 3–7. doi: 10.1890/1051-0761(2003)013[0003:PAHITO]2.0.CO;2
Lu L., Wu R. S. S. (2000). An experimental study on recolonization and succession of marine macrobenthos in defaunated sediment. Mar. Biol. 136, 291–302. doi: 10.1007/s002270050687
Mackie A. S. Y., Oliver P. G., Kingston P. F. (1993). “The macrobenthic infauna of hoi ha wan and tolo channel, Hong Kong,” in Proceedings of the first international conference on the marine biology of Hong Kong and the south China Sea, Hong Kong. Ed. Morton B. (Hong Kong: Hong Kong University Press), 657–674. 28 October - 3 November 1990.
MacNeil M. A., Graham N. A., Cinner J. E., Wilson S. K., Williams I. D., Maina J., et al. (2015). Recovery potential of the world’s coral reef fishes. Nature 520, 341–344. doi: 10.1038/nature14358
Mangano M. C., Kaiser M. J., Porporato E. M., Spanò N. (2013). Evidence of trawl disturbance on mega-epibenthic communities in the southern tyrrhenian Sea. Mar. Ecol. Prog. Ser. 475, 101–117. doi: 10.3354/meps10115
McLaverty C., Dinesen G. E., Gislason H., Brooks M. E., Eigaard O. R. (2021). Biological traits of benthic macrofauna show size-based differences in response to bottom trawling intensity. Mar. Ecol. Prog. Ser. 671, 1–19. doi: 10.3354/meps13790
McLaverty C., Eigaard O. R., Dinesen G. E., Gislason H., Kokkalis A., Erichsen A. C., et al. (2020). High-resolution fisheries data reveal effects of bivalve dredging on benthic communities in stressed coastal systems. Mar. Ecol. Prog. Ser. 642, 21–38. doi: 10.3354/meps13330
Moritz C., Gravel D., Savard L., McKindsey C. W., Brêthes J. C., Archambault P. (2015). No more detectable fishing effect on northern gulf of St Lawrence benthic invertebrates. ICES. J. Mar. Sci. 72, 2457–2466. doi: 10.1093/icesjms/fsv124
O’Neill F. G., Ivanović A. (2016). The physical impact of towed demersal fishing gears on soft sediments. ICES. J. Mar. Sci. 73, i5–i14. doi: 10.1093/icesjms/fsv125
Ortega I., Colling L. A., Dumont L. F. C. (2018). Response of soft-bottom macrobenthic assemblages to artisanal trawling fisheries in a subtropical estuary. Estuar. Coast. Shelf. Sci. 207, 142–153. doi: 10.1016/j.ecss.2018.04.007
Palumbi S. R. (2003). Population genetics, demographic connectivity, and the design of marine reserves. Ecol. Appl. 13, 146–158. doi: 10.1890/1051-0761(2003)013[0146:PGDCAT]2.0.CO;2
Pielou E. C. (1984). The interpretation of ecological data: A primer on classification and ordination (New York, USA: John Wiely & Sons), 263 pp.
Pilskaln C. H., Churchill J. H., Mayer L. M. (1998). Resuspension of sediment by bottom trawling in the gulf of Maine and potential geochemical consequences. Conserv. Biol. 12 (6), 1223–1229. doi: 10.1046/j.1523-1739.1998.0120061223.x
Pitcher C. R., Poiner I. R., Hill B. J., Burridge C. Y. (2000). Implications of the effects of trawling on sessile megazoobenthos on a tropical shelf in northeastern Australia. ICES. J. Mar. Sci. 57, 1359–1368. doi: 10.1006/jmsc.2000.0911
Preciado I., Arroyo N. L., González-Irusta J. M., López-López L., Punzón A., Muñoz I., et al. (2019). Small-scale spatial variations of trawling impact on food web structure. Ecol. Indic. 98, 442–452. doi: 10.1016/j.ecolind.2018.11.024
Queirós A. M., Hiddink J. G., Kaiser M. J., Hinz H. (2006). Effects of chronic bottom trawling disturbance on benthic biomass, production and size spectra in different habitats. J. Exp. Mar. Bio. Ecol. 335, 91–103. doi: 10.1016/j.jembe.2006.03.001
Ramalho S. P., Lins L., Soetaert K., Lampadariou N., Cunha M. R., Vanreusel A., et al. (2020). Ecosystem functioning under the influence of bottom-trawling disturbance: An experimental approach and field observations from a continental slope area in the West Iberian margin. Front. Mar. Sci. 7. doi: 10.3389/fmars.2020.00457
Reiss H., Simon P. R., Greenstreet S. P. R., Sieben K., Ehrich S., Piet G. J., et al. (2009). Effects of fishing disturbance on benthic communities and secondary production within an intensively fished area. Mar. Ecol. Prog. Ser. 394, 201–213. doi: 10.3354/meps08243
Rijnsdorp A. D., Bastardie F., Bolam S. G., Buhl-Mortensen L., Eigaard O. R., Hamon K. G., et al. (2015). Towards a framework for the quantitative assessment of trawling impacts on the sea bed and benthic ecosystems. ICES. J. Mar. Sci. 73 (1), 27–38. doi: 10.1093/icesjms/fsv207
Rijnsdorp A. D., Vingerhoed B. (2001). Feeding of plaice, Pleuronectes platessa l. and sole Solea solea (L.) in relation to the effects of bottom trawling. J. Sea. Res. 45 (3-4), 219–229. doi: 10.1016/S1385-1101(01)00047-8
Russ G. R., Alcala A. C., Maypa A. P., Calumpong H. P., White A. T. (2004). Marine reserve benefits local fisheries. Ecol. Appl. 14 (2), 597–606. doi: 10.1890/03-5076
Schratzberger M., Jennings S. (2002). Impacts of chronic trawling disturbance on meiofaunal communities. Mar. Biol. 141, 991–1000. doi: 10.1007/s00227-002-0895-5
Sciberras M., Hiddink J. G., Jennings S., Szostek C. L., Hughes K. M., Kneafsey B., et al. (2018). Response of benthic fauna to experimental bottom fishing: A global meta-analysis. Fish. Fish. (Oxf.) 19 (4), 698–715. doi: 10.1111/faf.12283
Sciberras M., Jenkins S. R., Kaiser M. J., Hawkins S. J., Pullin A. S. (2013). Evaluating the biological effectiveness of fully and partially protected marine areas. Environ. Evid. 2 (1), 1–31. doi: 10.1186/2047-2382-2-4
Shin P. K. S. (1989). Natural disturbance of benthic infauna in the offshore waters of Hong Kong. Asian Mar. Biol. 6, 193–207.
Shin P. K. S., Cheung S. G., Tsang T. Y., Wai H. Y. (2014). “Ecology of artificial reefs in the subtropics,” in Advances in marine biology 68. Ed. Lesser M. P. (Oxford, UK: Academic Press), 1–63, ISBN: ISBN 978-0-12-800169-1.
Shin P. K. S., Thompson G. B. (1982). Spatial distribution of the infaunal benthos of Hong Kong. Mar. Ecol. Prog. Ser. 10, 37–47. doi: 10.3354/meps010037
Sköld M., Göransson P., Jonsson P., Bastardie F., Blomqvist M., Agrenius S., et al. (2018). Effects of chronic bottom trawling on soft-seafloor macrofauna in the kattegat. Mar. Ecol. Prog. Ser. 586, 41–55. doi: 10.3354/meps12434
Steele J., Alverson D. L., Auster P., Collie J., DeAlteris J. T., Deegan L., et al. (2002). Effects of trawling and dredging on seafloor habitat (Washington, DC.: National Academy Press), 126 pp.
Szostek C. L., Murray L. G., Bell E., Rayner G., Kaiser M. J. (2016). Natural vs. fishing disturbance: drivers of community composition on traditional king scallop, Pecten maximus, fishing grounds. ICES. J. Mar. Sci. 73 (supplement 1), i70–i83. doi: 10.1093/icesjms/fsv152
Thrush S. F., Dayton P. K. (2002). Disturbance to marine benthic habitats by trawling and dredging: implications for marine biodiversity. Annu. Rev. Ecol. Evol. Syst. 33, 449–473. doi: 10.1146/annurev.ecolsys.33.010802.150515
Thrush S. F., Hewitt J. E., Cummings V. J., Dayton P. K., Cryer M. (1998). Disturbance of the marine benthic habitat by commercial fishing: impacts at the scale of the fishery. Ecol. Appl. 8, 866–879. doi: 10.1890/1051-0761(1998)008[0866:DOTMBH]2.0.CO;2
Tillin H. M., Hiddink J. G., Jennings S., Kaiser M. J. (2006). Chronic bottom trawling alters the functional composition of benthic invertebrate communities on a sea-basin scale. Mar. Ecol. Prog. Ser. 318, 31–45. doi: 10.3354/meps318031
Tuck I. D., Hall S. J., Robertson M. R., Armstrong E., Basford D. J. (1998). Effects of physical trawling disturbance in a previously unfished sheltered Scottish sea loch. Mar. Ecol. Prog. Ser. 162, 227–242. doi: 10.3354/meps162227
Tuya F. C., Soboil M. L., Kido J. (2000). An assessment of the effectiveness of marine protected areas in the San Juan islands, Washington, USA. ICES. J. Mar. Sci. 57 (4), 1218–1226. doi: 10.1006/jmsc.2000.0808
van Denderen P. D., Bolam S. G., Hiddink J. G., Jennings S., Kenny A., Rijnsdorp A. D., et al. (2015). Similar effects of bottom trawling and natural disturbance on composition and function of benthic communities across habitats. Mar. Ecol. Prog. Ser. 541, 31–43. doi: 10.3354/meps11550
van Denderen P. D., Törnroos A., Sciberras M., Hinz H., Friedland R., Lasota R., et al. (2022). Effects of bottom trawling and hypoxia on benthic invertebrate communities. Mar. Ecol. Prog. Ser. 694, 13–27. doi: 10.3354/meps14094
Walkley A., Black I. A. (1934). An examination of the degtjareff method for determining soil organic matter, and a proposed modification of the chromic acid titration method. Soil Sci. 37, 29–38. doi: 10.1097/00010694-193401000-00003
Wang Z., Leung K. M., Sung Y. H., Dudgeon D., Qiu J. W. (2021). Recovery of tropical marine benthos after a trawl ban demonstrates linkage between abiotic and biotic changes. Commun. Biol. 4 (1), 1–8. doi: 10.1038/s42003-021-01732-y
Watling L., Norse E. A. (1998). Disturbance of the seabed by mobile fishing gear: A comparison to forest clearcutting. Conserv. Biol. 12, 1180–1197. doi: 10.1046/j.1523-1739.1998.0120061180.x
Williams A., Althaus F., Maguire K., Green M., Untiedt C., Alderslade P., et al. (2020). The fate of deep-sea coral reefs on seamounts in a fishery-seascape: What are the impacts, what remains, and what is protected? Front. Mar. Sci. 7. doi: 10.3389/fmars.2020.567002
Wilson K. D., Leung A. W., Kennish R. (2002). Restoration of Hong Kong fisheries through deployment of artificial reefs in marine protected areas. ICES. J. Mar. Sci. 59, 157–163. doi: 10.1006/jmsc.2002.1186
Wu R. S. S. (1982). Periodic defaunation and recovery in a sub-tropical epibenthic community, in relation to organic pollution. J. Exp. Mar. Biol. Ecol. 64, 253–269. doi: 10.1016/0022-0981(82)90013-2
Keywords: bottom trawling, benthic community, biological trait analysis, hypoxia, recovery
Citation: Xu W, Fleddum AL, Shin PKS and Sun J (2022) Impact of and recovery from seabed trawling in soft-bottom benthic communities under natural disturbance of summer hypoxia: A case study in subtropical Hong Kong. Front. Mar. Sci. 9:1010909. doi: 10.3389/fmars.2022.1010909
Received: 03 August 2022; Accepted: 12 September 2022;
Published: 03 October 2022.
Edited by:
Meilin Wu, South China Sea Institute of Oceanology, Chinese Academy of Sciences (CAS), ChinaReviewed by:
Juan Chen, Hohai University, ChinaYafen Wang, China University of Geosciences Wuhan, China
Copyright © 2022 Xu, Fleddum, Shin and Sun. This is an open-access article distributed under the terms of the Creative Commons Attribution License (CC BY). The use, distribution or reproduction in other forums is permitted, provided the original author(s) and the copyright owner(s) are credited and that the original publication in this journal is cited, in accordance with accepted academic practice. No use, distribution or reproduction is permitted which does not comply with these terms.
*Correspondence: Paul K. S. Shin, cGF1bGtzc2hpbkBnbWFpbC5jb20=
†These authors have contributed equally to this work and share first authorship