- 1Sorbonne Université, UMR 7619 Metis, Paris, France
- 2Global Ecology Unit, CREAF-UAB, Bellaterra, Spain
- 3CNRS, UMR 7619 Metis, Paris, France
- 4IFREMER, Département Océanographie et Dynamique Ecosystème, Plouzané, France
- 5IFREMER, UMR 9220 ENTROPIE (IRD, Univ. Réunion, IFREMER, Univ. Nouvelle-Calédonie, CNRS), Nouméa, New Caledonia
Three scenarios for the future of the French agro-food system were specified for the territory draining into the Seine Bight, comprising Paris megacity and the Seine watershed. The first scenario assumes the pursuit of the current trend of opening and specialization of agriculture, as well as of concentration of population within the Paris agglomeration. The second scenario assesses the generalization of agro-ecological practices and a healthier human diet. A third, hybrid, scenario was elaborated assuming that agro-ecological practices were implemented in some protected areas (namely Regional Natural Parks and drinking water protection areas, making up about one-third of the total watershed area), while the rest of the territory will follow the trends of the first scenario. We use a unique modelling chain (GRAFS-Riverstrahler-ECOMARS3D) that couples the biogeochemical functioning of the river basin, the estuary and the adjacent bay. It allows exploring the implications of changes in terrestrial activities throughout the aquatic continuum in a consistent way and assessing to what extent changes in population, agricultural practices, food consumption, and sanitation may sustain the current trend of recovery in the Seine River or either increase stress on the system. Results show that only the full agro-ecological scenario would be able to restore good water quality everywhere in the river network, as well as decrease significantly the risks of toxic algal blooms in the coastal zone. Intermediate situations, such as the protection of specific areas, however attractive as a solution, are not enough to offset the impacts of intensive human activities unless the protected areas dedicated to compensating for damage are sufficiently large.
Introduction
With the recognition of ICEP (Indicator of coastal water eutrophication, Billen and Garnier, 2007; Garnier et al., 2010a) as an indicator for Sustainable Development Goal 14 (https://sdg.data.gov/14-1-1/), the issue of linking changes in watershed management to nutrient inputs at the river mouth deserves increasing attention.
The Seine River is one of the best examples of heavily populated rivers where the imprint of human activities on the biogeochemistry of the water can be tracked throughout many centuries. Billen et al. (2007) provided a historical approach to many of the changes in the river since the 16th century, and pointed out the importance of past transformations in understanding the dynamics of the system. The input of nutrients in the basin peaked between the 1950s and the 1990s, first linked to the rapid increase of population and then to the expansion of modern agricultural and farming practices (Billen et al., 2007). Thereafter, population stabilized, and improvements in sanitation, together with a progressive implementation of good agricultural practices (e.g., optimisation of mineral fertiliser inputs, enhanced use of organic fertilisers, longer rotations with N-fixing crops, etc.) have resulted in a remarkable decrease of P contamination and a levelling off of N, resulting in a general improvement of the ecological status of the river (Romero et al., 2016; Garnier et al., 2020).
The downstream sector of the river opens out into a large macrotidal estuary, which, like the rest of the watershed, is subject to great human pressure. Urban and industrial activities abound: the estuarine basin hosts ~1 million inhabitants, including the cities of Rouen and Le Havre. It is also a major trade hub in France: about 60% of all national exchanges in agricultural goods take place in the NW region of France (Le Noë et al., 2016), and a large fraction of such commodities enter the country via the Seine estuary. This thriving industry has indeed led to many morphological changes over the past century (Lafite and Romaña, 2001; Lafite et al., 2007; Foussard et al., 2010). A number of dykes and floodgates were constructed to facilitate navigation and allow large vessels to reach the inland port of Rouen, and downstream Tancarville (ca. 25 km from the coast) the estuary has become a quasi-linear cemented channel that is regularly dredged.
In parallel to the evolution described for the river, both the water quality and the biogeochemical functioning of the estuary have changed throughout the years driven by human transformations. Romero et al. (2016) reported an amelioration of the water conditions in the estuary after the second half of the 1990s. Episodes of hypoxia, which were very frequent decades ago, have practically disappeared and the algal biomass has decreased substantially –both in relative and absolute terms– within the estuary and in the Seine Bight (Romero et al., 2013; Passy et al., 2016). Despite such improvements, the buffer role of the estuary –i.e., the capacity of estuarine waters to retain, transform and remove nutrients before they reach the sea– remains limited, and the risk of harmful algal blooms (HABs) in the adjacent marine waters is still high (Romero et al., 2019).
Over the past 20 years, a series of restoration measures have been implemented to recover part of the intertidal mudflats and reclaim habitats for flora and fauna (increased connectedness between streams and salt marshes; removal of seawalls and dykes; creation of protected areas for nesting and breeding of aquatic species; Ducrotoy, 2010 and references therein). These measures could eventually enhance the buffer role of the estuary and contribute to improve water quality, but the increase in human pressure may outpace the rate of recovery. Future socio-economic projections foresee an increase in population in the basin, and some regional plans include an expansion of the commercial port activities in the estuary. To what extent the future changes in population, agricultural practices, food consumption, and sanitation may sustain the current trend of recovery or either increase stress on the system, pushing it towards situations similar to those observed in the past, is highly uncertain.
We here use a deterministic biogeochemical representation of the land-to-sea continuum (Romero et al., 2019) that couples the GRAFS-Riverstrahler model and an extended configuration of the ECO-MARS3D model to project different future scenarios, placing special emphasis on the changes of the agro-food system. Agricultural land represents about half of the watershed area, and any changes in agricultural practices may typically have a significant impact on the water quality of the river –not immediate, however, taking into account the long residence times and the inertia of the aquifer (Flipo et al., 2020).
Moving on a common axis that describes the openness/closeness of the agro-food system, we propose three scenarios: two opposite extreme scenarios (already discussed in Billen et al., 2018), and an intermediate one, newly developed here. The first scenario assumes the pursuit of the current trend of opening and hyper specialisation of agriculture, accompanied by the concentration of population within the Paris agglomeration. The second scenario assumes quite the opposite: the generalization of agro-ecological practices and reduction of animal protein in the human diet. The third, hybrid, scenario assumes that agro-ecological practices are implemented in some protected areas (namely Regional Natural Parks and drinking water protection areas, making up about one-third of the total watershed area), while the rest of the territory follows the lines of the first scenario.
For each projection, we analyse the impact in terms of (1) the riverine water quality, (2) the filtering capacity of the estuary, and (3) the risk of algal blooms in the estuarine reaches and the Seine Bight. We point out those factors that can tip the balance to one side or the other regarding the good environmental status of the river and the estuary, and discuss whether the current situation can be a turning point for the worst and the best: recovering an essential ecosystem for its buffering role at the riverine-coastal interface, and making it less vulnerable to climate change, or risking that the set of environmental stresses will reverse the efforts and improvements achieved in recent decades.
The seine land-to-sea continuum
The Seine River extends for over 760 km and drains a watershed of 76,260 km2 (Fig. 1). The basin is densely populated (>17 million inhabitants and, on average, 200 inhabitants·km-2). Cities and industrial states are mainly located in the lower part of the river basin, while agricultural landscapes occupy the upper part of the catchment, upstream of the city of Paris (Passy et al., 2016). Arable land (primarily devoted to cereals and industrial crops) covers 52.7% of the catchment, forests account for 25.8%, and grasslands and meadows, many of them for cattle breeding, represent ca. 10% of the basin surface (Garnier et al., 2013).
At its lower end, the river becomes a large estuary. The Seine estuary is a macrotidal temperate estuary of about 50 km2, with an intra-estuarine basin that drains 4515 km2, ca. 6% of the total surface of the catchment (Garnier et al., 2013). Two sections can be clearly distinguished: a freshwater tidal sector –from Caudebec up to Poses, where a lock prevents further tidal propagation–, and a lower part affected by a salinity gradient (Garnier et al., 2010b; Fisson et al., 2014). The mean residence time in the estuary spans from 5–7 days to ~18 days (for a flow of 1000 m3 s-1 or 200 m3 s-1 at Poses, respectively; Le Hir, 2001; Even et al., 2007). The estuary is also characterised by the formation of a turbidity maximum zone (TMZ), mostly located between Honfleur and Tancarville (Etcheber et al., 2007; Garnier et al., 2008). The location of the Seine basin within France, the estuary, and the coastal area included in the present study are shown in Figure 1.
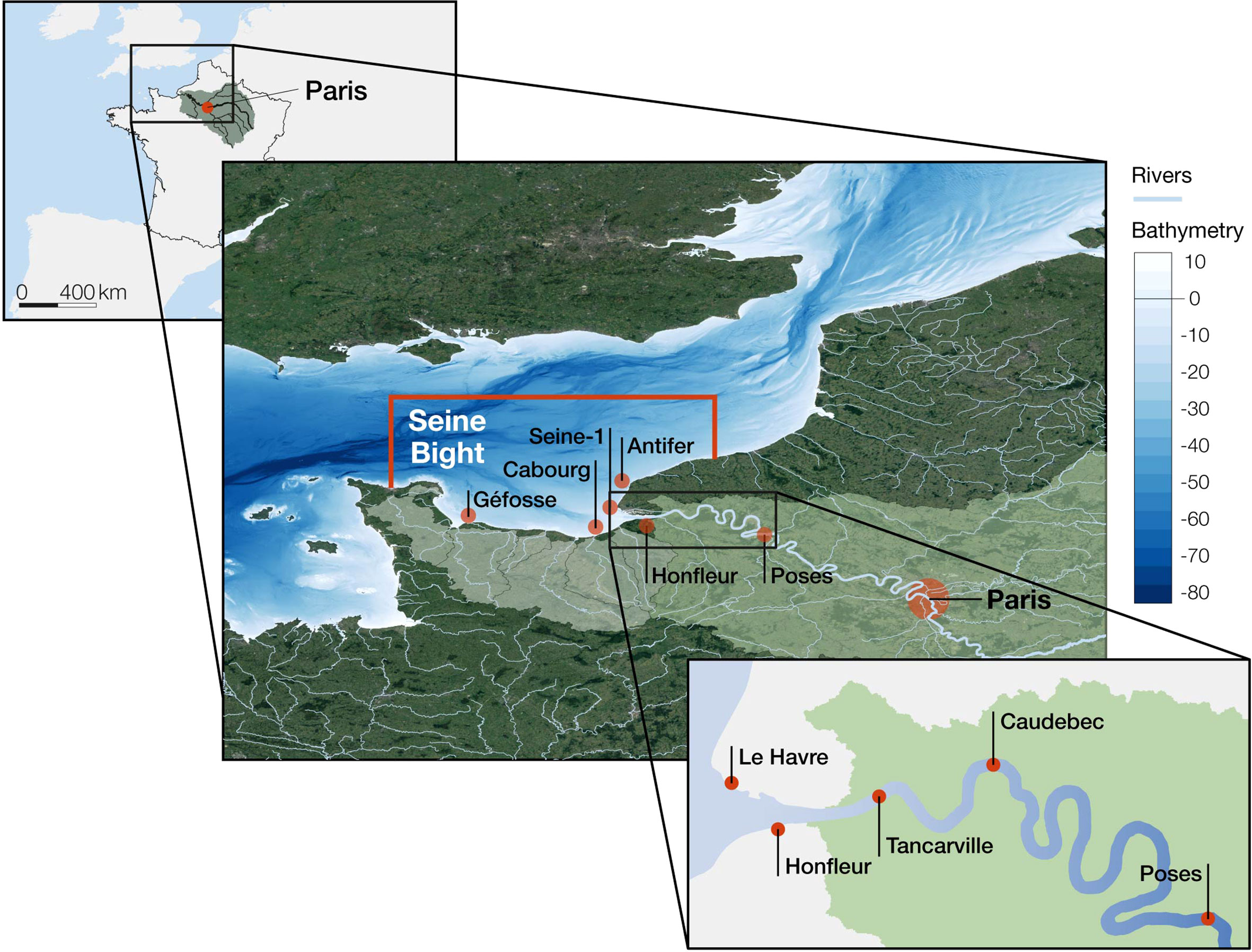
Figure 1 (left) The Seine basin in France; (middle) The lower part of the basin and the Seine Bight, showing the marine limits of the ECO-MARS3D model (red lines), the marine stations and the coastal river basins that flow into the Seine Bight and that are also considered in the study (W to E, Saire, Douve, Taute, Vire, Aure, Seulles, Orne, Dives et Touques). (right) A zoom on the estuary.
Narratives and quantification of the scenarios
In a recent paper (Billen et al., 2018), two contrasted scenarios for the future of the French agro-food system were described at the scale of 33 agricultural French regions, among which 15 concern the Seine Basin. These scenarios are based on the extension to their logical end of opposite trends currently acting on the dynamics of the system, namely opening to global markets and crop/livestock farming disconnection on the one hand; and search for autonomy, local reconnection, and sobriety on the other hand. Both scenarios have been shown to be able to feed the French population and even export a significant amount of cereals to the international market, with however much lower environmental impact for the latter than for the former.
Here we specify these scenarios for the area corresponding to the NW quarter of France, including the watershed of the Seine River and a number of smaller coastal rivers draining into the Seine Bight. This area is the more active region of France in terms of commercial exchanges of agricultural commodities, concentrating 60% of their total flux values (Le Noë et al., 2016). Paris megacity is by far the dominant urban pole, deeply structuring the economic activity of the whole area.
Indeed, the first scenario, called Grand Paris, often referred to in official discourses and prospective studies (e.g., Attali, 2010) involves the extreme development of the Paris conurbation (Grand Paris). The population increases around Paris and downstream along the Seine River to the detriment of the cities further upstream in the basin. However, the scenario also assumes that the most advanced technologies of centralized urban wastewater purification are implemented. The Seine ports (Le Havre, Rouen, Paris) are carrying out an ambitious development project around the Seine Gateway® (Haropa, 2014; Duszyński et al., 2015), with the agro-food and agro-chemical export industries as two of the main strategic priorities. A vast port hinterland, extending over the whole NW quarter of France, is made accessible by new transport infrastructures, including the deepening of the current estuarine channel to improve ship connections, and a new rail link. Field crop regions intensify their specialisation and focus on the production of cereals and oilseeds, using the most rigorous techniques of chemical agriculture (green chemistry, agrofuels) in accordance with the current regulations regarding fertilization practices. Livestock farming is excluded from the central part of the area, specialised in stockless crop farming, while animal production is concentrated in peripheral regions, according to an intensive industrial mode of production largely based on feed imports.
The Autonomous-Reconnected-Demitarian (A-R-D) or Agro-Ecological scenario, at the opposite of this Grand Paris scenario, depicts a situation where proactive actions were put in place at the regional and the national level to speed up the ecological transition (Billen et al., 2018). These implicitly include the development of renewable energies and an explicit land policy aimed at limiting metropolitan urban expansion, in addition to deep changes in the agro-food system –in line with the Afterres2050 (Couturier et al., 2017) or TYFA (Poux and Aubert, 2018) scenarios–, while preventing the population decline of the existing small and medium-sized towns in the upstream part of the basin.
Citizenship awareness of the environmental impacts of the food industry leads to a desire to re-establish social links with local producers. Local supply is therefore favoured, as well as organic food products. Consumption of meat and dairy products is halved in favour of vegetal proteins, including legumes, as recommended for public health and environmental reasons by the EAT- Lancet Commission (Willett et al., 2019) and the Nitrogen on the Table report (Westhoek et al., 2015). There is also a significant reduction in food waste.
Organic farming practices are widespread. In terms of nitrogen, the extensive use of legumes and diversification of the rotations makes it possible to avoid chemical fertilizers and pesticides, and this is accompanied by a reconnection with livestock farming, which is being reintroduced in currently stockless cropping regions. At the same time, livestock density decreases in regions currently specialized in livestock.
A third scenario, called Dual Agriculture, sets up a situation characterized by the coexistence of the two above-mentioned antagonistic trajectories: one promoting the A-R-D conditions, and the other in favour of the Grand Paris premises. The population is extremely polarized between (i) a liberal trend that favours growth and competitiveness in the context of globalization, and (ii) a trend of ecological awareness advocating the defence of the environment and local circular autonomy. Their confrontation leads to a division of the territory between areas of specialized economic development and areas of protection, in which various alternative modes of production and consumption are tested on a territorial scale. These protected areas include protected catchment areas for drinking water supply (PCA) (https://www.data.gouv.fr/fr/datasets/aire-dalimentation-de-captages-aac-destines-a-laep/) and Regional Natural Parks (RNP) (https://www.parcs-naturels-regionaux.fr/). The former are areas delimited by law where specific regulations are issued in order to prevent nitrate and pesticides contamination of groundwater. The latter are territories where local stakeholders agree on a series of sustainable development objectives; their motto –Another life is being invented here!– clearly refers to social innovation and structural changes. Together, PCA and RNP cover more than one third of the territory of the Seine basin (Figure 2), thus potentially representing a significant impact on the functioning of the entire basin.
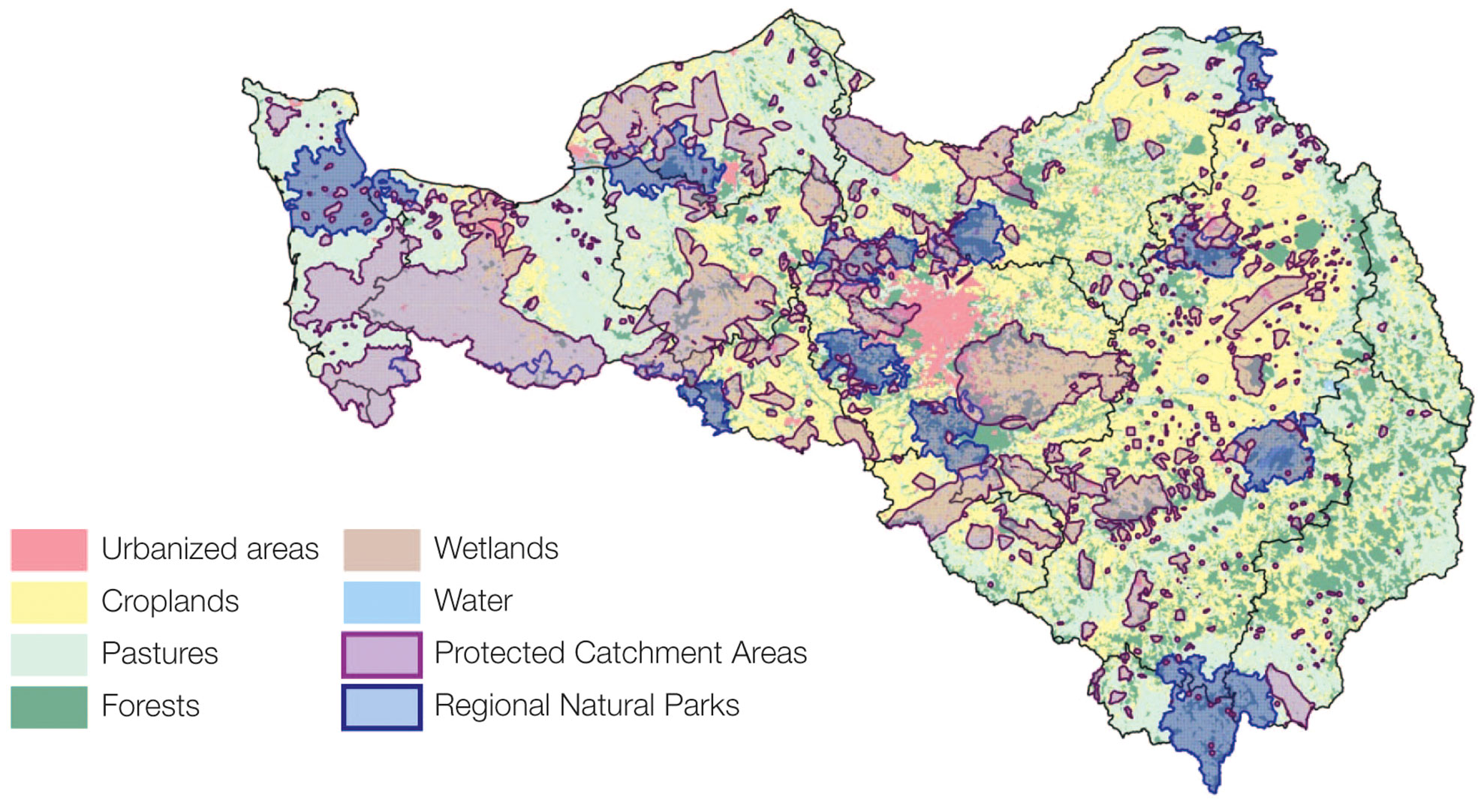
Figure 2 Land use in the Seine River catchment, showing the location of Protected Catchment Areas (PCA) and Regional Natural Parks (RNP). [Sources: AESN, Corine Land Cover 2006, IGN-GEOFLA, INPN].
This last scenario, which can be viewed as a compromise where two antagonistic visions of the agro-food system coexist in separate territories, can also represent a transitory step toward the advent of the scenario A-R-D prone to extend beyond the protected areas, where it is developed first. Note, in any case, that the local agro-food system in the protected areas remains closely embedded in the global “Food Regime”, so that some of its characteristics, including the human diet, remain those of the still dominant globalized agro-food system.
Table 1 summarizes the main features of each scenario, as well as some quantitative indicators of their performances.
Models
We coupled two different models to take into account changes in the terrestrial sources of nutrients and study their biogeochemical processing along the entire aquatic continuum: the GRAFS-Riverstrahler model and the ECO-MARS3D model. For the reference situation, the modelling results (in terms of N, P, Si) showed good performance (N-RMSE, % Bias) compared to in-situ observations (Romero et al., 2019, see Figure 3), which allowed here to use the model chain to explore scenarios.
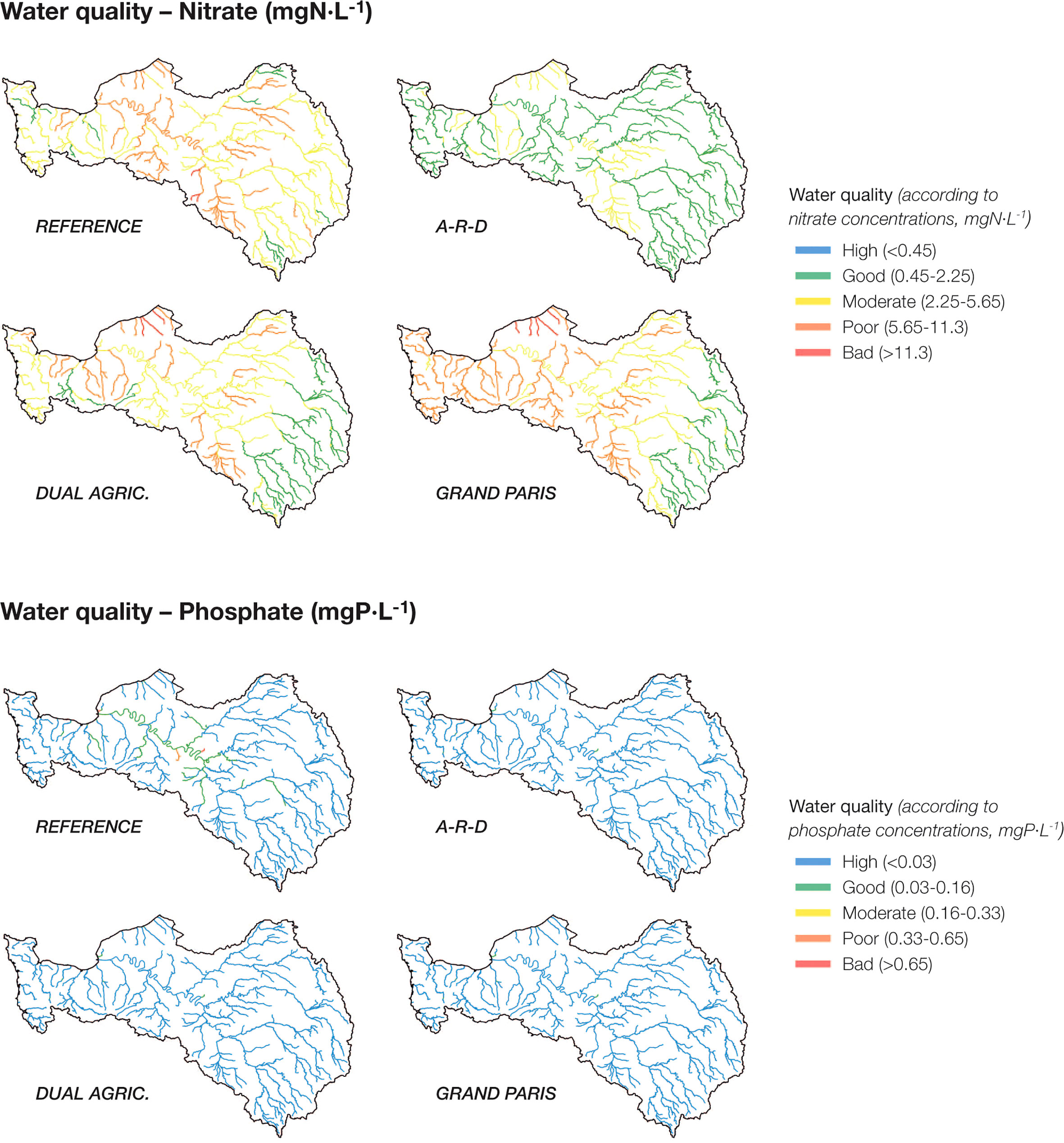
Figure 3 (top) Nitrate concentrations (mg N·l-1, average for the 10-year period) and (bottom) phosphate concentrations (mg P·l-1, average for the 10-year period) along the entire hydrographic network of the Seine basin, for the reference conditions and the three scenarios projected in the study. The nutrient scale and the colour codes are consistent with the nutrient thresholds established in European and French regulations to describe High/Good/Moderate/Poor/Bad environmental status in freshwater streams. Note that 0.45, 2.25, 5.65 and 11.3 mg N/L correspond to 32, 161, 404 and 807 µmol/L; and 0.03, 0.16 0.33 and 0.65 mg P/L correspond to 1, 5.2, 10.6 and 21 µmol/L.
Simulations for all scenarios were carried out over a 10-year period (2003-2013) to smooth the effect of different hydrological years, with an additional year of spin-up (2002). For the reference conditions, average socio-economical features of the year 2013 were considered –i.e., 17 million inhabitants in the basin; a protein ingestion of 7.3 kgN/yr per person, 65% of animal origin; wastewater treatments and agricultural practices in accordance with current environmental regulations.
The GRAFS-Riverstrahler model
The Riverstrahler modelling approach addresses the biogeochemical functioning of the Seine hydrographic network (RIVE model; Garnier et al., 2002) in connection with point and non-point pressures (Billen et al., 1994; Billen et al., 2001; Billen et al., 2007; Passy et al., 2013; Passy et al., 2016). The model takes into account the flow as the sum of surface/sub-surface runoff and groundwater base flow (Garnier et al., 2018). In-stream nutrient and organic matter processing (biological and physicochemical reactions), as well as riparian retention processes, are considered.
Data on industrial and urban wastewater facilities (point sources), their current capacity and the type of treatment come from the Seine water authority (AESN, Agence de l’Eau Seine-Normandie) and the French national database IREP (http://www.irep.ecologie.gouv.fr/IREP/index.php), and were informed on the entire basin. To account for non-point sources coming from agriculture we used the GRAFS tool (Billen et al., 2014; Lassaletta et al., 2014; Le Noë et al., 2017). GRAFS is based on a detailed assessment of the flows involved in the production, processing and consumption of animal and plant products in a territory, as well as the associated nutrient leakage to the environment. It allows calculating how many nutrients are left in the soil depending on several characteristics of the agro-food system (see Billen et al., 2014; Le Noë et al., 2017 for details) and therefore what fraction is liable to be leached and enter the different compartments of the aquatic continuum. The concentration of nutrients in infiltrated water from each land use class in the catchment is transferred as inputs to the Riverstrahler model.
The current version of the Riverstrahler model includes the discharge of the Seine (the entire basin down to Poses), but also the Eure, the Risle, and three other estuarine tributaries (Aubette, Cailly, and Austreberthe), plus the direct outputs from nine coastal rivers (W to E, Saire, Douve, Taute, Vire, Aure, Seulles, Orne, Dives et Touques, Figure 1) discharging along the Normandy coast.
The ECO-MARS3D model
The outputs from the GRAFS-Riverstrahler model are coupled to the ECO-MARS3D model. We applied the most recent configuration of ECO-MARS3D, thoroughly described in Romero et al. (2019). This latest version of the model uses an extended spatial scale, embracing the Seine Bay and the Seine estuary up to Poses, at the limit between the river and the estuary. Updated bathymetric data come from the French Naval Hydrographic and Oceanographic Service (SHOM, 2015). The hydrodynamics and the sedimentary dynamics in the turbidity maximum zone (TMZ) have been intensively calibrated, and the model includes additional biogeochemical processes –e.g., those related to the oxygen dynamics and the freshwater organisms– to adequately simulate the biogeochemical functioning of the estuarine reaches. The explicit details of this extended configuration are given in Romero et al. (2019), including the specific equations, the set of parameters used, and the model validation. The geographical domain of the model is shown in Figure 1.
Results
River water quality
The effects of the different scenarios in terms of the concentration of nutrients (nitrate and phosphate) along the hydrographic network are shown in Figure 3. In the reference conditions, nitrate values >2.25 mg N/L (>160.7 µmol/L) are widespread in the catchment, and concentrations exceed 5.6 mg N/L (400 µmol/L) in the main branch. These values correspond to the Moderate and Poor environmental status as set in European regulations (European Directives 2000/60/EC and 2008/105/EC). All three future scenarios show some improvement in water quality with respect to the reference conditions. For Grand Paris, water quality improves in the upper part of the basin but gets worse in the lower part, where most of the tributaries show nitrate concentrations >5.6 mg N/L. Nitrate values in the main branch remain between 2-6 mg N/L, mainly on account of continuous technological upgrades in wastewater treatment infrastructures.
The situation is similar for the Dual Agriculture scenario: lower nitrate values in the upper basin, but few changes downstream, where nitrate concentrations are never below 2 mg N/L (143 µmol/L). In contrast, major improvements in water quality are observed for the A-R-D scenario, where nitrate concentrations decrease drastically over the entire catchment and most streams fall within the Good environmental status category. The situation for phosphate is less complex: phosphate inputs have decreased greatly in recent decades, so even in the reference conditions the majority of streams show phosphate concentrations <0.03 mg P/L (<0.97 µmol/L), and they remain low under the three projected scenarios, consistently within the EU High environmental status thresholds. Silica concentrations (not shown) remain almost the same for the three scenarios.
Beyond the spatial patterns, we analysed the temporal evolution of nutrients at the outlet of the Seine and two large tributaries (Eure, Risle) (Figure 4). Differences are remarkable between scenarios for all three rivers, notably for nitrate. In the Seine, nitrate concentrations decrease from the 6-7 mg N/L of the reference state to 3-5 mg N/L for Grand Paris and Dual Agriculture, and drop to <3 mg N/L for the A-R-D scenario. However, the general improvement in water quality observed in the main branch of the Seine is not apparent for the quality of the tributaries, especially those draining the southwestern territories. In the Eure River, only the Dual Agriculture (-28%) and especially the A-R-D scenario (-75%) entail significant nitrate reductions; for Grand Paris, nitrate concentrations increase by +17% with respect to reference values. The results are even more sensitive in the Risle River, where nitrate concentrations drop by over half (-63%) in the A-R-D scenario, but increase in all other projections. Indeed, it is worth noting the disparity: in the Grand Paris scenario, for instance, nitrate concentrations could be higher in the Eure and the Risle than in the Seine (+45% and +74%, respectively), these two main tributaries of the downstream axis of the Seine being necessarily impacted by the Grand Paris population increase.
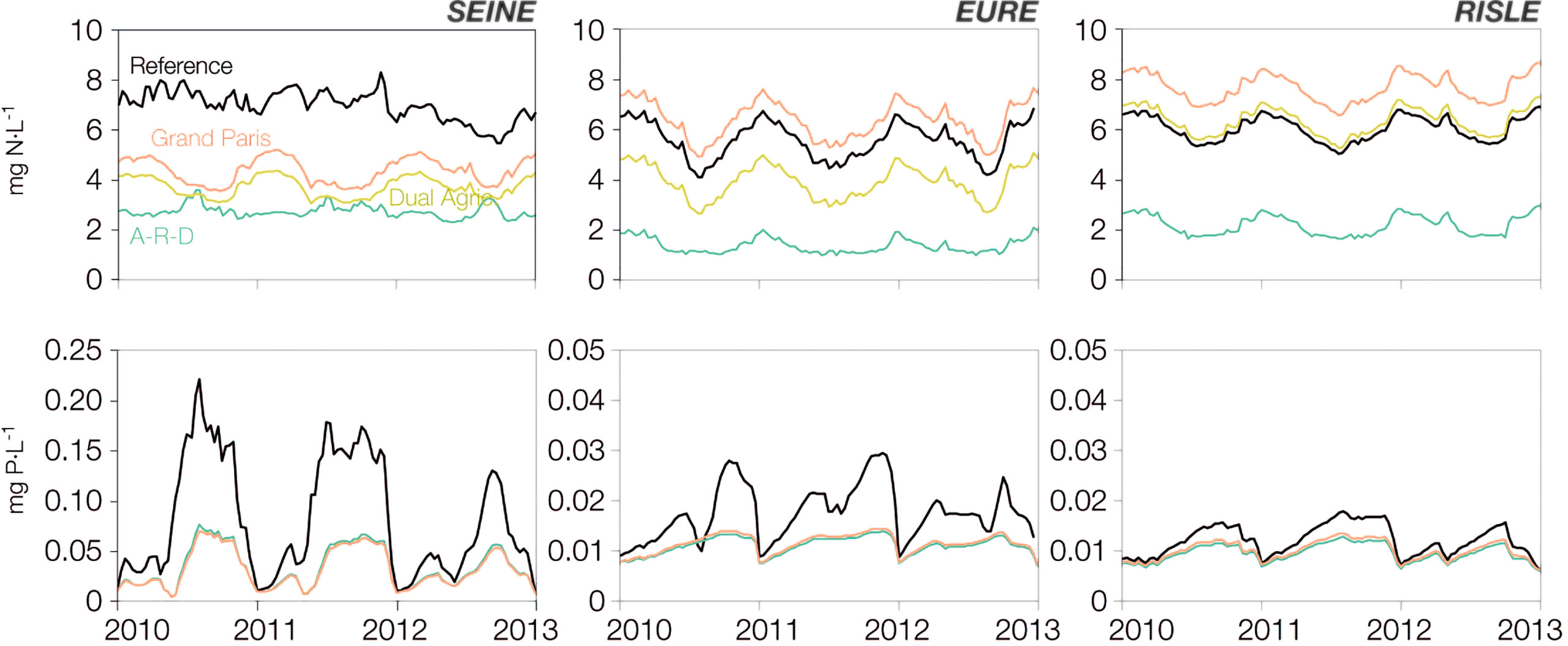
Figure 4 Interannual and seasonal dynamics of nitrate (top) and phosphate (bottom) concentrations for the reference conditions and for the different scenarios at the outlet of the Seine (Poses), the Eure and the Risle rivers. The scenarios were run over a 10-year period, but in order to emphasise the seasonal cycles and the resulting concentrations at the end of the period, only the last three years are shown here. Note that 10 mg N/L correspond to 714 µmol/L, and 0.05 mg P/L correspond to 1.6 µmol/L.
For phosphate, consistent with the spatial results shown in Figure 3, concentrations decrease in all cases with respect to the reference state, with minor differences between scenarios.
Water quality in the estuary and the adjacent coast
The changes between scenarios in riverine nutrient concentrations propagate to the estuary and the nearby coastal waters. In Caudebec –at the intersection between the freshwater estuary and the brackish estuary– and Tancarville –downstream, within the brackish estuary– there are large decreases of phosphate, ammonium and nitrate in all three scenarios with respect to the reference conditions (Figure 5). The nutrient decrease entails a decline in chlorophyll: summer peaks of 15-25 µg·L-1 drop to maxima <10 µg·L-1. Moreover, there is little variation between scenarios, except for nitrate, for which the concentration gradient observed at Poses (Grand Paris > Dual Agriculture > A-R-D scenario) persists. The differences in nutrients and chlorophyll are somewhat smoothed out, but still apparent at Honfleur, at the outlet of the estuary.
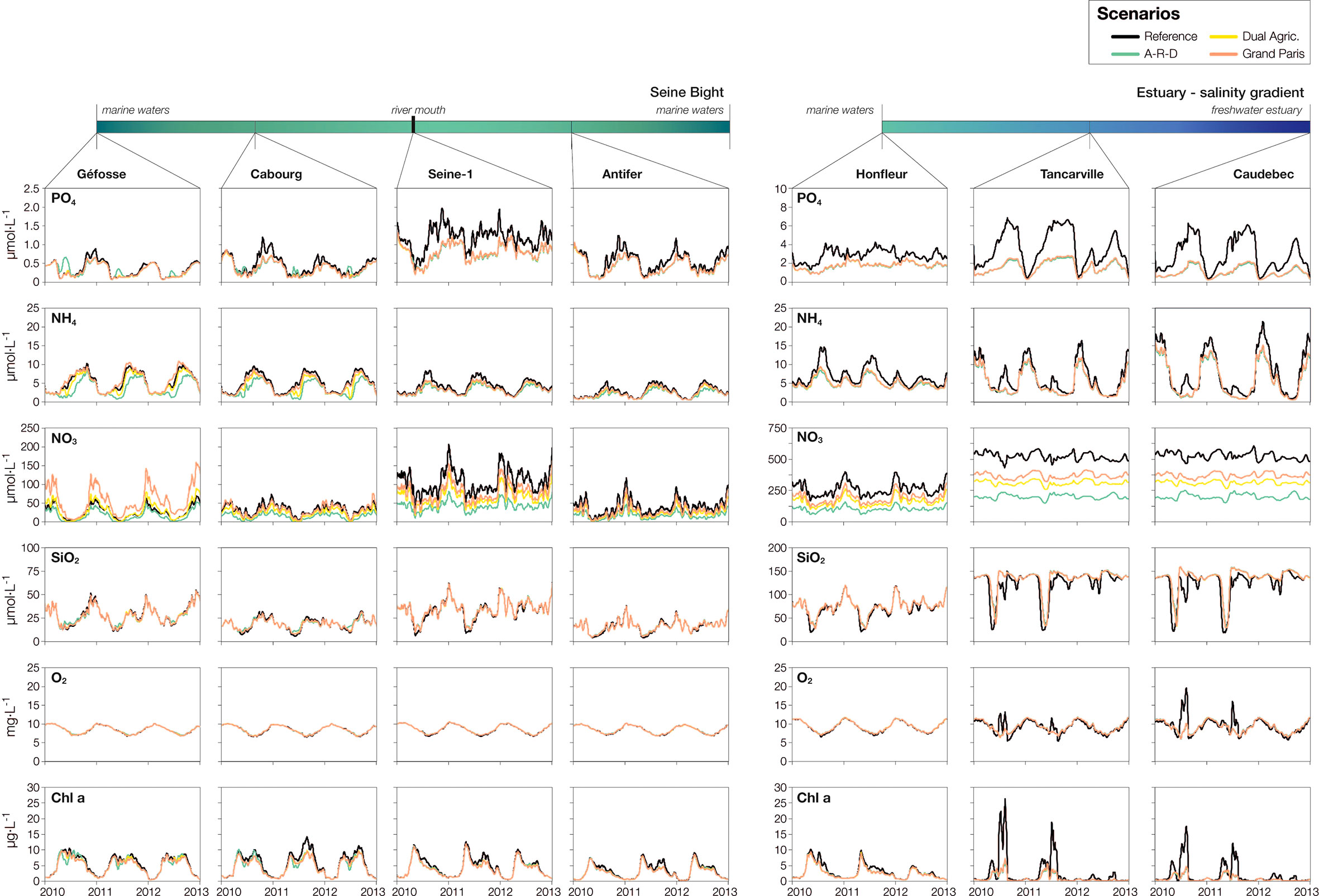
Figure 5 Nutrients, dissolved oxygen and chlorophyll concentration in three estuarine stations (upstream to downstream: Caudebec, Tancarville, Honfleur) and four marine stations (Géfosse, Cabourg, Seine1, Antifer). The location of the stations is shown in Figure 1. The period matches the one in Figure 4 (2010-2013) to allow comparisons between the riverine, estuarine and marine stations.
Interestingly, the divergence between scenarios in terms of nutrients and chlorophyll stands out in marine waters, probably related to the additional outflow of Normandy’s coastal rivers. In Seine-1, Cabourg and Antifer, all located near the mouth of the Seine estuary, the variations echo those of the Seine: the largest decreases in nutrients and chlorophyll correspond to the A-R-D scenario, while the lowest changes correspond to the Grand Paris projection. In contrast, in Géfosse –located in the western part of the Seine Bight– ammonium and nitrate increase in the Grand Paris and Dual Agriculture scenarios, although these nutrient increases do not drive changes in the concentration of chlorophyll, which remains consistently below 10 µg·L-1.
The retention capacity of the estuary and the fluxes delivered to the sea
The shifts in the agro-food system put forward in our scenarios not only have profound effects in terms of water quality, they also cause large variations in the total nutrient fluxes that reach the estuary and are exported to the sea. Results for the Reference scenario are in accordance with the estimates by Romero et al. (2019). On average for the 10-year period, about 126 kt N yr-1, 62 kt Si yr-1 and 2.6 kt P yr-1 enter the estuary annually at Poses, and 112 kt N yr-1, 57 kt Si yr-1, and 1.9 kt P yr-1 are exported from Le Havre to the adjacent sea. This means that the filtering capacity is ~10% for N and Si, and 28% for P (Figure 6). The fluxes of silicate –which does not come from anthropogenic sources– barely change between the three scenarios and the reference (<6% difference in the fluxes, the filter effect remains around 10%), but the scenarios decrease significantly the loads of P and N entering and leaving the estuary. In the A-R-D projection, inputs are 48 kt N yr-1 and 1.3 kt P yr-1, and outputs are 42 kt N yr-1 and 0.8 kt P yr-1 (less than half the reference values). The N retention capacity is only slightly higher (-11%) than in the reference conditions, but it increases to -41% in the case of P, against the 28% above. Intermediate retention values are found for the other two scenarios: -11% N (9-10 kt N yr-1), and -38% P (0.4 kt P yr-1) in the Grand Paris and Dual Agriculture projections.
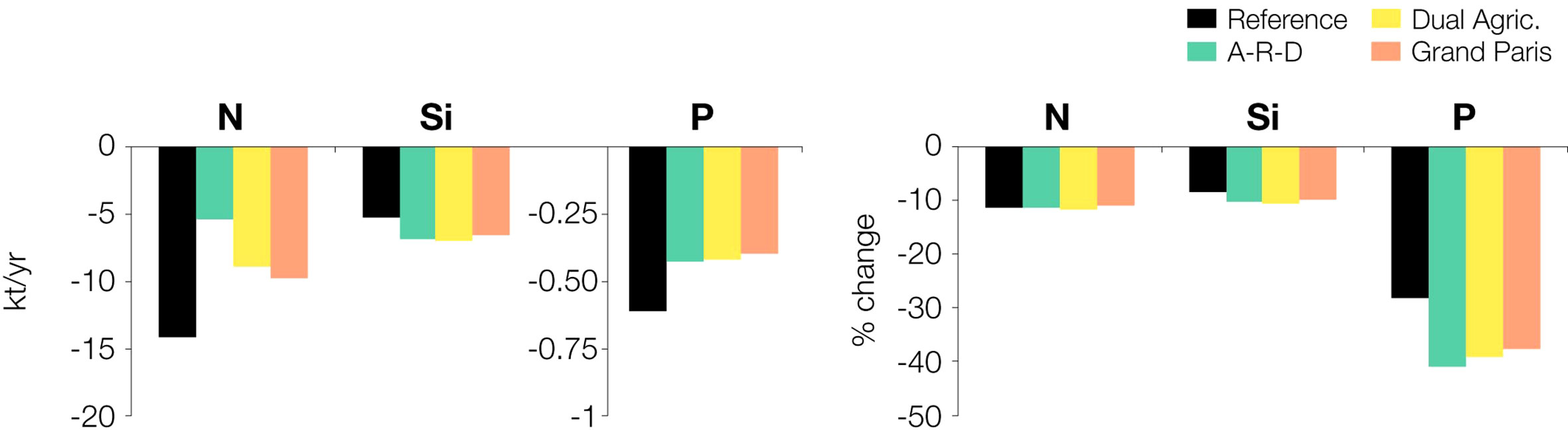
Figure 6 Difference in the fluxes of nitrogen (N), silica (Si) and phosphorus (P) between the inlet (Poses) and the outlet (Le Havre) of the Seine estuary (left, in Kt/year and right, in percentage).
Provided that scenarios affect differently the fluxes of N, P and Si entering and leaving estuarine waters, we aimed at assessing to what extent these differences have consequences in terms of the stoichiometric ratios. Imbalanced ratios between elements (i.e., biased with respect to the canonical Redfield ratio C:N:P = 106:16:1, Redfield et al., 1963) have been described for the Seine River and the Seine Bight (Billen and Garnier, 2007; Garnier et al., 2010a; Romero et al., 2013; Romero et al., 2016). Importantly, when there are large anthropogenic inputs of N and/or P into the system, completely decoupled from silica inputs –which mainly come from natural weathering of rocks– silica can be rapidly depleted, causing a shift from diatoms to non-diatoms, and posing a risk of eutrophication and food web dysfunction. Therefore, in densely populated river basins with intensive agriculture, as is the case in the Seine basin, the N and P loads in relation to Si determine a deviation from the Redfield ratio which can ultimately lead to poor conditions.
We calculated the relative ratios of N:P and N:Si at several stations along the estuary (Figure 7). For the reference, both N:P and N:Si values are remarkably above the Redfield values (N:P = 16; N:Si = 1). N:Si ratios decrease from 5.3 to 3.7 as waters flow downstream into the estuary, while N:P peaks at Caudebec (N:P = 143), at the furthest upstream point where we have the influence of marine waters. The A-R-D scenario is the only one that brings both ratios down towards the Redfield standard; N:Si falls to 1.5 (-66%), and the decrease in N:P averages -23% (-11% at Caudebec, where values remain high (127); -33% at Le Havre, where the ratio drops to 41). N:P increases swiftly in the Dual Agriculture and the Grand Paris scenarios, reaching values of 184 and 218 at Caudebec, respectively, and reinforcing the current imbalance.
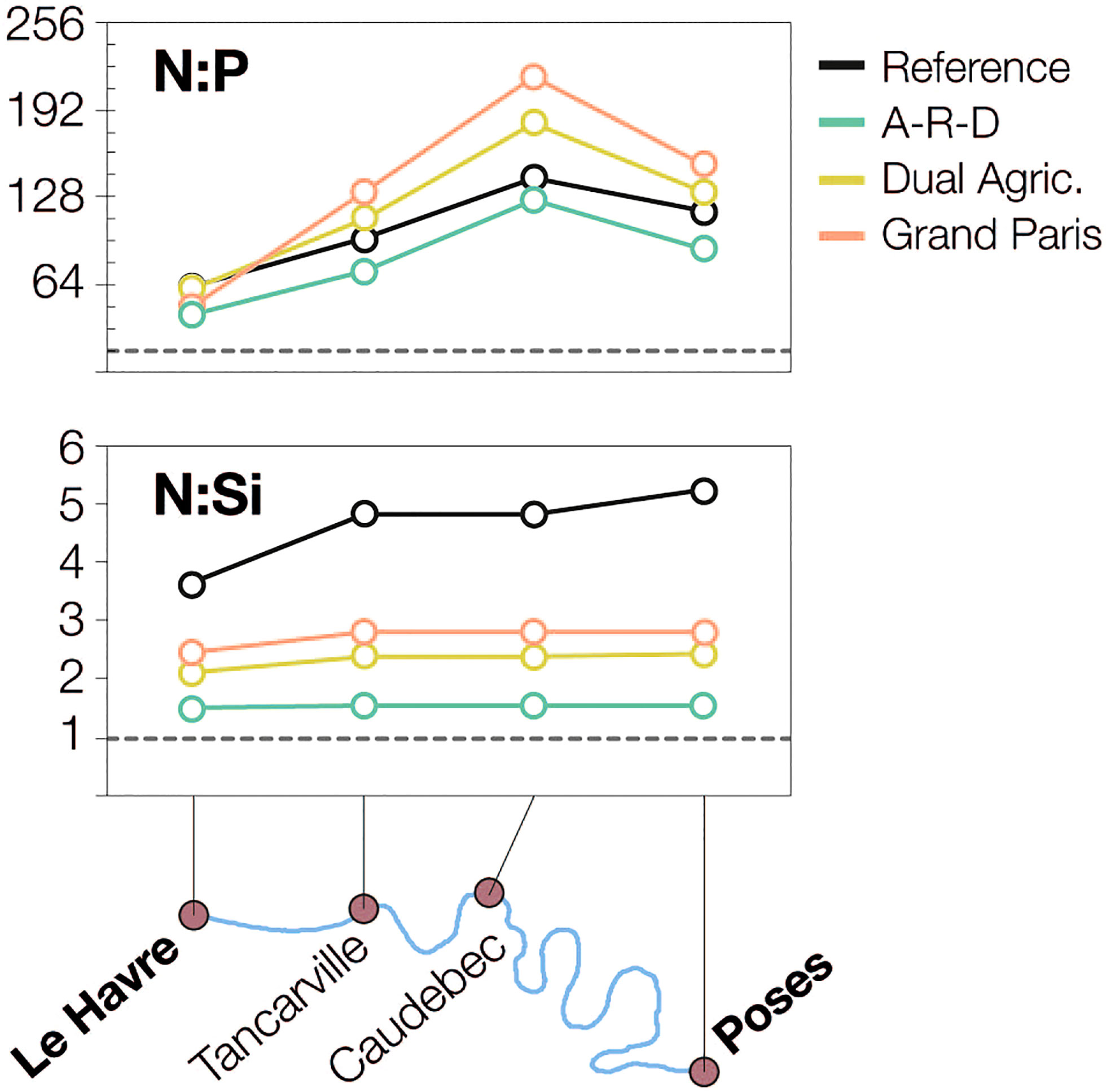
Figure 7 Stoichiometry (molar ratios) of nutrients in four stations along the estuary. The grey dotted lines mark the Redfield ratio (N:P = 16, N:Si = 1).
Coastal eutrophication issues
We assessed the impact of our scenarios on eutrophication issues in two different ways: first, we calculated the coastal eutrophication potential associated to the riverine nutrient fluxes entering the bay; second, we used the ECO-MARS3D to analyse the growth of phytoplankton groups in the Bay. Figure 8 shows the results of the Index of Coastal Eutrophication Potential (ICEP) as described by Billen and Garnier (2007). The ICEP measures the algal biomass (in terms of C) that can be built up considering the N (ICEP-N) or P (ICEP-P) available in excess over Si, assuming that the surplus of N and P may foster the growth of non-siliceous phytoplankton –most often groups of dinoflagellates that are associated to the nuisance blooms that occur in many coastal locations of the bay during the summer period–. Si deficiency may also cause shifts in diatom species, as discussed below.
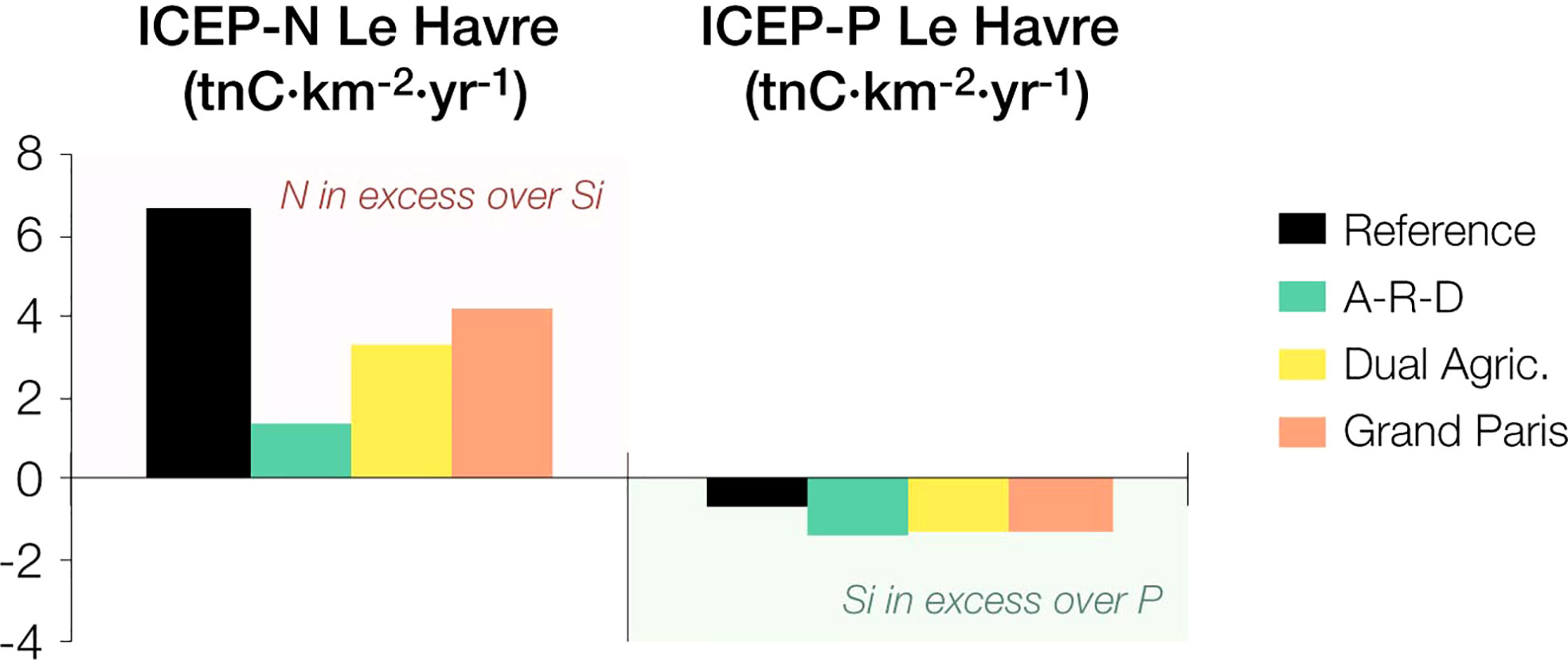
Figure 8 Index of Coastal Eutrophication Potential (ICEP) for nitrogen and phosphorus at the outlet of the Seine estuary.
The results show positive ICEP-N values at Le Havre for the reference and the three scenarios, which means that once Si is depleted, there are N leftovers that can be used by non-siliceous algae. The lowest ICEP-N and thus the lowest potential eutrophication risk corresponds to the A-R-D scenario. In contrast, the ICEP-P is negative in all cases, implying that P may be limiting primary production and acting as a natural firewall for algal blooms.
The maps in Figure 9 represent the chlorophyll concentration and the abundance of diatoms and dinoflagellates in the bay (on average for the entire 10-year period). We focus on these two algal groups because they are related to most eutrophication phenomena in the bay. The highest chlorophyll values occur along the coastline (ca. 7 µg·l-1), close to the mouths of the Seine estuary and the coastal rivers. Chlorophyll values decrease in all three scenarios with respect to the Reference, but reductions are widespread for the A-R-D while they concentrate near the mouth of the Seine estuary in the Grand Paris scenario. Consistent with this general decline in phytoplankton, the three scenarios show also lower values of diatoms than the Reference condition. The diatom decrease concentrates in the western part of the bay and is much more pronounced for the A-R-D scenario than for the other two, while the three scenarios show very similar results for dinoflagellates (Figure 9).
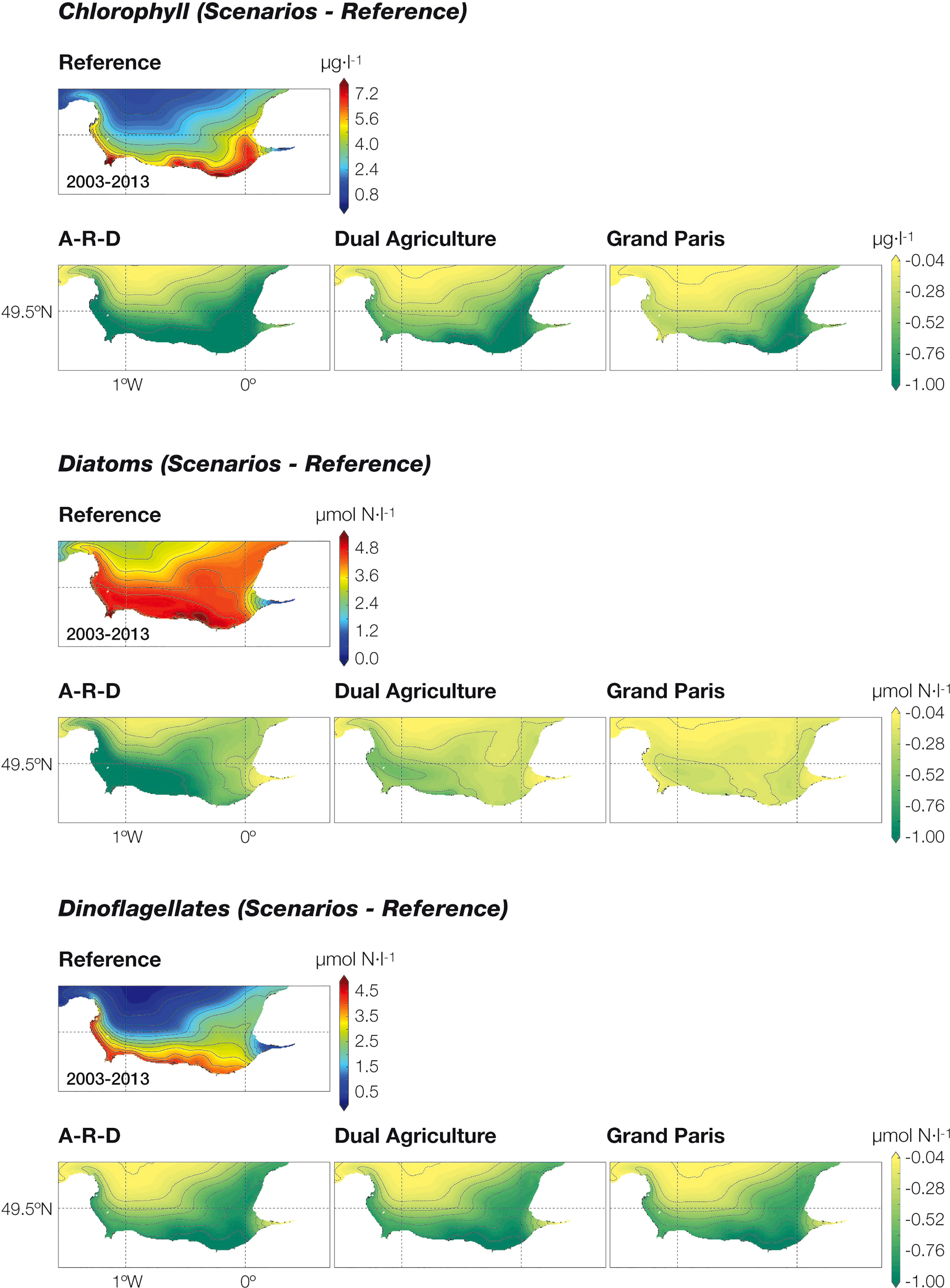
Figure 9 Concentration of chlorophyll, diatoms and dinoflagellates (expressed in µmol N·l-1) in the Seine Bight (average values for the 2003-2013 period). Note that, in order to highlight the divergences, we have plotted the difference between the reference situation and the outcome of each scenario.
Discussion
Previous work has paved the way for understanding how human activity in the Seine basin has modified the river’s biogeochemistry in the past (Billen et al., 2007; Romero et al., 2016; Garnier et al., 2020; Garnier et al., 2022). Agriculture, which occupies a considerable part of the entire basin, plays a major role in the N and P cycles and is also a decisive factor in terms of water availability [water scarcity due to change in hydrological regimes with climate change (Raimonet et al., 2018; Garnier et al., 2018) and use, e.g. for irrigation purposes (Isidoro and Aragüés, 2007; Quemada et al., 2013)]. In addition, trade of agricultural commodities is an integral element of the logistics and transport sector, whose economic weight and environmental impact are also very relevant in the region (Le Noë et al., 2016; Billen et al., 2022).
At the global level, agriculture is also recognised as one of the fundamental levers in the fight against global change. Drastic transformations in the agro-food system are required to ensure water and food security without compromising the health of terrestrial and marine ecosystems (Tilman et al., 2002; Howden et al., 2007; de Vries et al., 2013; Lynch et al., 2021).
However, in this respect, there is no general political consensus on the measures to be taken, and opposing trends have emerged. On the one hand, there are those who plead for a conservative continuation of the current model based on the extreme specialisation of agriculture and long-distance commercial markets. A certain tightening of environmental legislation and continuous technological developments are the countermeasures proposed to minimise environmental burdens (Klasen et al., 2016; Le Noë et al., 2018; Cadillo-Benalcazar et al., 2020). On the other hand, a shift towards circular systems involving changes in all sectors related to the food industry: from more sustainable agricultural practices to overhauling supply chains and international trade, including changes in food consumption patterns and a reduction in the share of animal protein in developed countries. Interestingly, both approaches have been tested at the scale of France (Billen et al., 2018) and at the scale of Europe (Billen et al., 2021), and both have proved to be feasible, productive enough to reach food and feed self-sufficiency for the French and European populations, respectively. Results also suggested large differences in their environmental performance in terms of cropland N soil surplus and N leaching.
On the basis of our understanding of the Seine basin, and taking advantage of the available modelling tools, we here test to what extent what was suggested at the national and European scale is observable at the basin scale, by further analysing the effects of the different approaches on the entire aquatic continuum, from headwater streams to the ocean (i.e. an integrated view of the water-agro-food system as defined in Garnier et al., 2015 and Billen et al., 2021).
The fundamental difference in the environmental footprint of the three scenarios is largely driven by the drastic decrease in N inputs from land-based sources in the A-R-D scenario. In fact, the N soil surplus potentially leached is much lower in agro-ecological farming systems (Anglade et al., 2015), as demonstrated in several studies (e.g., N surplus decreases from 63 to 30 kg N ha-1 yr-1 on average for countries in Europe, Billen et al., 2021; from over 80 to 30-50 kg N ha-1 yr-1 in several NUTS3 regions in France, Billen et al., 2018). The decrease in terrestrial N inputs has a direct impact on nitrate concentrations in surface and ground waters, and the improvement is transferred to the estuary and the coast. In the estuary, the current imbalance between N, P and Si decreases considerably, as does the risk of eutrophication in the adjacent bay.
The fact that major differences between the scenarios are particularly evident for N is indeed consistent with the assumptions made, as the changes in the agro-food system that are at the core of our projections largely affect diffuse sources and thus nitrate leaching to the aquatic compartment. Shortcutting diffuse sources is particularly important in light of future climate change impacts such as changes in precipitation, increased storminess and sea level rise. Changes in rainfall and sea level rise, for example, may alter the water balance of the estuary, affecting the time required to flush nutrients from the system, and storms can increase freshwater runoff and enhance diffuse nutrient fluxes.
For P, P inputs from synthetic fertilisers would also decrease significantly in the A-R-D scenario, but excess P in croplands is mostly bound to soil particles and remains in the soil, with very low P leaching (Le Noë et al., 2020). P diffuse sources are therefore mainly caused by erosion of arable soils. We considered that the erosion rate remains the same in all scenarios, as well as the P content of the arable soil, mostly determined by the long-term P legacy resulting from historical over-fertilization of agricultural soils rather than by current fertilization practices (Le Noë et al., 2017; Le Noë et al., 2020). With respect to P point sources, in all three scenarios the total population of the basin is the same (22.5M inhabitants), slightly higher than the reference (17M inhab.). Only the distribution of the additional population between the Paris agglomeration and the upstream basin differs. In all scenarios, however, the improvement of wastewater treatment is the same and results in a very low contribution of point sources to the total P load. Diffuse sources of P thus dominate in the scenarios, contrarily to what is the case in the current situation. This explains why the model predicts very small differences between scenarios in terms of P river fluxes.
It is noteworthy that the environmental impacts of the Dual Agriculture projection fall somewhere in between the Grand Paris and A-R-D scenarios, which is to be expected, but interestingly, in terms of spatial distribution, the scenario fails to reduce impacts across the whole basin, as does A-R-D. The comparative gap between the Paris conurbation –where most impacts are reduced– and the rest –where water quality worsens and the risk of eutrophication persists– is almost as large as in the Grand Paris scenario. The results suggest that protection of specific areas (e.g., as proposed in the EU Nitrates Directive, Council Directive 91/676/EEC), however attractive as a solution, is not enough to offset the impacts of intensive human activities elsewhere, unless the protected areas dedicated to compensating for damage are sufficiently large. In our simulation, these zones cover more than a third of the catchment and are still insufficient to restore water quality everywhere.
We were also particularly concerned to assess the effect of the scenarios on estuarine and coastal waters, because there are few studies in the literature that specifically address the outcome of agro-food changes in marine systems (see Lancelot et al., 2011; Desmit et al., 2018; Garnier et al., 2021), let alone those that describe the cascade of effects along the aquatic continuum. In this sense, the GRAFS-Riverstrahler-ECOMARS modelling chain we use is unique in providing insights on the connections between land and sea.
Estuaries are known to be powerful biogeochemical reactors. In the Seine, Romero et al. (2019) calculated that transformations, including consumption by the microbial community, retention in sediments, photo- and physicochemical reactions could lower the flux of nutrients entering the sea by 19 kt N yr-1 (-13%), 10 kt Si yr-1 (-11%), and 0.7 kt P yr-1 (-27%). We here performed similar calculations to test whether changes in the incoming load of nutrients linked to the different scenarios could improve or, on the contrary, deteriorate, the natural buffering function of the estuary. Our simulations clearly show that upstream nutrient reductions also improve estuarine conditions, lowering the fluxes of nutrients and reducing chlorophyll peaks, although only the A-R-D scenario is able to significantly decrease N fluxes and at least partly rebalance the N:P ratio and significantly reduce eutrophication risk (ICEP-N).
The ICEP is based on the excess of P or N over silica taking into account the classical algal requirements (Redfield ratio), and highlights the fact that once silica is limited or depleted, diatoms are replaced by non-diatom algae. These processes are not straightforward (e.g., Turner et al., 1998), and prior to diatom replacement, silica deficiency may also favour the emergence of toxic diatom species, such as Pseudo-nitzschia (Tatters et al., 2012).
Moreover, the imbalance between N and P, which is currently very high, increases in the Dual Agriculture and Grand Paris projections. This is not trivial, because although P may limit phytoplankton growth in the estuary and the bay, it is well known that P can be rapidly recycled by the planktonic community depending on the P fraction and the enzymatic activity (Kobori and Taga, 1979; Labry et al., 2016); its high turnover thus allows blooms to occur as long as sufficient N is available in the water. In addition, the hydro-morphology of the receiving marine bay (e.g., residence time) clearly influences algal development (Garnier et al., 2021).
Highly imbalanced N:P ratios are common in river systems around the world (Smayda, 1990; Justić et al., 1995; Glibert and Burkholder, 2006; Glibert and Burford, 2017; Grosse et al., 2017; Garnier et al., 2021; Li et al., 2022); they have been linked to a number of environmental problems (toxic algae, anoxia, prohibition of fishing, bathing, etc.) and are proving very difficult to reverse.
The fact that phytoplankton decrease more in the A-R-D is a positive issue for coastal eutrophication. However, because diatoms are more reduced than dinoflagellates means that P limitation may be particularly affecting this group, which blooms first in the spring time. Nitrogen leftovers after reduced (P-limited) diatom blooms may be large, and could allow for higher build up of biomass by dinoflagellates. Dinoflagellates can thrive under conditions of nutrient limitation –switching to mixotrophy, for example–, and would benefit from P recycling by the microbial loop and some P desorption from the sediments under low flow conditions (Romero et al., 2019). Having large blooms of dinoflagellates, toxic or not, can lead to the accumulation of organic matter and, eventually, to episodes of hypoxia (Diaz and Rosenberg, 2008).
The uncertainty in the response of planktonic organisms in estuarine and marine waters –and therefore the risk of not decreasing nutrient inputs– could be exacerbated in the future if we further consider the effects of climate change. Increased rainfall and storms can lead to higher nutrient inputs, while sustained periods of low precipitation can favour saltwater intrusions and affect the residence time in the estuary, altering the food web and allowing for higher algal growth. Sea level rise may also alter the location of the estuarine turbidity maximum, and warmer conditions would affect stratification and oxygen concentrations, all affecting phytoplankton growth.
Conclusions
The chain of models (GRAFS-Riverstrahler-ECOMARS) shows that a generalised decline in phytoplankton in the Seine Bight is only possible in the A-R-D scenario, assuming profound changes to the current agro-food system. With a scenario that simulates present agricultural trends, or even a mixture of these two, the decline is restricted to the influence area of the Seine. This is consistent with the fact that the Dual Agriculture and Grand Paris scenarios create a large difference in terms of nutrient fluxes between the Seine and the coastal streams of the Normandy coast. The latter not only do not improve their water quality but would continue being a risk for coastal eutrophication.
Overall, total phytoplankton biomass reduction (in terms of chlorophyll a) follows the scenarios in this order: A-R-D > Dual > Grand Paris, and the same pattern applies to diatoms (reductions A-R-D > Dual > Grand Paris). Dinoflagellates (in µmol N/L), however, are similarly reduced in all scenarios, suggesting that both the amount of nutrients and their balance need to be taken into account. Finally, it should be noted that the Dual Agriculture scenario, which includes deep agricultural changes for one third of the Seine basin, would maintain a high risk of coastal eutrophication, i.e. ICEP-N values three times higher than those of the A-R-D scenario.
Data availability statement
The raw data supporting the conclusions of this article will be made available by the authors, without undue reservation.
Author contributions
ER, JG, GB, PR and RG conceived and designed the analysis. ER, AR and RG collected the data. ER, JG, GB, AR and RG contributed data or analysis tools. ER, JG, GB and RG performed the analysis. ER, JG and GB wrote the paper. All authors contributed to the article and approved the submitted version.
Funding
This work was undertaken within the framework of the Scientific Program Seine-Aval, (French project RESET: Josette Garnier, principal investigator) sponsored by the consortium GIP-Seine-Aval (http://seine-aval.crihan.fr/web/). The authors acknowledge the GIP-Seine-Aval and the Federation Ile-de-France for Research on the Environment (FIRE) for funding the work of Dr. E. Romero. ER is also grateful to the Severo Ochoa Excellence Program (CEX2018-000828-S, Spanish Research Agency) for additional financial aid. The study benefited from the partnership with the Emosem Project (SeasEra), and the FLAM Project (Ministère de l’Ecologie, du Développement durable et de l’Energie-Agence de l’Eau Seine Normandie).
Conflict of interest
The authors declare that the research was conducted in the absence of any commercial or financial relationships that could be construed as a potential conflict of interest.
Publisher’s note
All claims expressed in this article are solely those of the authors and do not necessarily represent those of their affiliated organizations, or those of the publisher, the editors and the reviewers. Any product that may be evaluated in this article, or claim that may be made by its manufacturer, is not guaranteed or endorsed by the publisher.
References
Anglade J., Billen G., Garnier. J., Makridis T., Puech T., Tittel C. (2015). Nitrogen soil surface balance of organic vs conventional cash crop farming in the seine watershed. Agric. Syst. 139, 82–92. doi: 10.1016/j.agsy.2015.06.006
Attali J. (2010). Paris Et la mer. la seine est capitale (Fayard, Paris et la mer. La Seine est Capitale), 200.
Billen G., Aguilera E., Einarsson R., Garnier J., Gingrich S., Grizzetti B., et al. (2021). Reshaping the European agro-food system and closing its nitrogen cycle: The potential of combining dietary change, agroecology, and circularity. One Earth 4, 839–850. doi: 10.1016/j.oneear.2021.05.008
Billen G., Garnier J. (2007). River basin nutrient delivery to the coastal sea: Assessing its potential to sustain new production of non-siliceous algae. Mar. Chem. 106, 148–160. doi: 10.1016/j.marchem.2006.12.017
Billen G., Garnier J., Ficht A., Cun C. (2001). Modeling the response of water quality in the seine river estuary to human activity in its watershed over the last 50 years. Estuaries 24, 977–993. doi: 10.2307/1353011
Billen G., Garnier J., Hanset P. (1994). Modelling phytoplankton development in whole drainage networks: The RIVERSTRAHLER model applied to the seine river system. Hydrobiologia 289, 119–137. doi: 10.1007/BF00007414
Billen G., Garnier J., Nemery J., Sebilo M., Sferratore A., Barles S., et al. (2007). A long-term view of nutrient transfers through the seine river continuum. Sci. Total Environ. 375, 80–97. doi: 10.1016/j.scitotenv.2006.12.005
Billen G., Lassaletta L., Garnier J. (2014). A biogeochemical view of the global agro-food system: Nitrogen flows associated with protein production, consumption and trade. Glob. Food Sec. 3, 209–219. doi: 10.1016/j.gfs.2014.08.003
Billen G., Le Noë J., Garnier J. (2018). Two contrasted future scenarios for the French agro-food system. Sci. Total Environ. 637-638, 695–705. doi: 10.1016/j.scitotenv.2018.05.043
Billen G., Le Noë J., Noûs C., Garnier J. (2022). “Trajectoires socio-écologiques passées et futures de l’Estuaire de la seine,” in Changements globaux: Quelle gouvernance anticipative pour les métropoles estuariennes? Eds. Salles D., Mainguy G., De Godoy Leski C. (Paris: ISTE Éditions). Collection Environnement.
Cadillo-Benalcazar J. J., Renner A., Giampietro M. (2020). A multiscale integrated analysis of the factors characterizing the sustainability of food systems in Europe. J. Environ. Manage. 271, 110944. doi: 10.1016/j.jenvman.2020.110944
Couturier C., Charru M., Doublet S., Pointereau P. (2017). The Afterres2050 scenario 2016 version (Toulouse, France: Solagro Association). Available at: www.afterres2050.solagro.org.
Desmit X., Thieu V., Billen G., Campuzano F., Dulière V., Garnier J., et al. (2018). Reducing marine eutrophication may require a paradigmatic change. Sci. Total Environ. 635, 1444–1466. doi: 10.1016/j.scitotenv.2018.04.181
de Vries W., Kros J., Kroeze C., Seitzinger S. P. (2013). Assessing planetary and regional nitrogen boundaries related to food security and adverse environmental impacts. Curr. Opin. Environ. Sustainability. 5, 392–402. doi: 10.1016/j.cosust.2013.07.004
Diaz R. J., Rosenberg R. (2008). Spreading dead zones and consequences for marine ecosystems. Science 321, 926–929. doi: 10.1126/science.11564
Ducrotoy J.-P. (2010). Ecological restoration of tidal estuaries in north Western Europe: An adaptive strategy to multi-scale changes. Plankton Benthos Res. 5, 174–184. doi: 10.3800/pbr.5.174
Duszyński J., Audievre L., Mus M., Mary. J.-F. (2015). Seine gateway® at the heart of north-Western European freight flows: Regional dynamics and current challenges. Cent. Eur. Rev. Economics Manage. 15, 5–23. doi: 10.29015/cerem.71
Etcheber H., Taillez A., Abril G., Garnier J., Servais P., Moatar F., et al. (2007). Particulate organic carbon in the estuarine turbidity maxima of the gironde, Loire and seine estuaries: Origin and lability. Hydrobiologia 588, 245–259. doi: 10.1007/s10750-007-0667-9
Even S., Thouvenin B., Bacq N., Billen G., Garnier J., Guezennec L., et al. (2007). An integrated modelling approach to forecast the impact of human pressure in the seine estuary. Hydrobiologia 588, 13–29. doi: 10.1007/s10750-007-0649-y
Fisson C., Leboulenger F., Lecarpentier T., Moussard S., Ranvier. G. (2014). L’Estuaire de la seine: état de santé et évolution . Seine-Aval Scientific Report 3.1, Paris, France.
Flipo N., Gallois N., Labarthe B., Baratelli F., Viennot P., Schuite J., et al. (2020). “Pluri-annual water budget on the seine basin: Past, current and future trends,” in The seine river basin, vol. 90 . Eds. Flipo N., Labadie P., Lestel L. (Cham: Springer), 59–90. doi: 10.1007/698_2019_392
Foussard V., Cuvilliez A., Fajon P., Fisson C., Lesueur P., Macur O. (2010). Évolution morphologique d’un estuaire anthropisé de 1800 à nos jours. Sci. Programme Seine-Aval Scientific Report 2.3. Paris, France, 43. doi: 10.1371/journal.pone.0012257
Garnier J., Beusen A., Thieu V., Billen G., Bouwman L. (2010a). N:P:Si nutrient export ratios and ecological consequences in coastal seas evaluated by the ICEP approach. special issue “Past and future trends in nutrient export from global watersheds and impacts on water quality and eutrophication”. Global Biogeochem. Cycles 24, GB0A05. doi: 10.1029/2009GB003583
Garnier J., Billen G., Even S., Etcheber H., Servais P. (2008). Organic matter dynamics and budgets in the maximum turbidity zone of the seine estuary (France). Estuarine Coast. Schelf Sci. 77, 150–162. doi: 10.1016/j.ecss.2007.09.019
Garnier J., Billen G., Hannon E., Fonbonne S., Videnina Y., Soulie M. (2002). Modeling transfer and retention of nutrients in the drainage network of the Danube river. Estuar. Coast. Shelf Sci. 54, 285–308. doi: 10.1006/ecss.2000.0648
Garnier J., Billen G., Lassaletta L., Vigiak O., Nikolaidis N. P., Grizzetti B. (2021). Hydromorphology of coastal zone and structure of watershed agro-food system are main determinants of coastal eutrophication. Environ. Res. Lett. 16, 023005. doi: 10.1088/1748-9326/abc77
Garnier J., Billen G., Némery J., Sebilo M. (2010b). Transformations of nutrients (N, p, Si) in the turbidity maximum zone of the seine estuary and export to the sea. Estuar. Coast. Shelf Sci. 90, 129–141. doi: 10.1016/j.ecss.2010.07.012
Garnier J., Lassaletta L., Billen G., Romero E., Grizzetti B., Némery J., et al. (2015). Phosphorus budget in the water-agro-food system at nested scales in two contrasted regions of the world (ASEAN-8 and EU-27). Global Biog. Cycle 29, 21. doi: 10.1002/2015GB005147
Garnier J., Marescaux A., Guillon S., Vilmin L., Rocher V., Billen G., et al. (2020). “Ecological functioning of the seine river: From long-term modelling approaches to high-frequency data analysis,” in The seine river basin, vol. 90 . Eds. Flipo N., Labadie P., Lestel L. (Cham: Springer) 90, 189–216. doi: 10.1007/698_2019_379
Garnier J., Meybeck M., Ayrault S., Billen G., Blanchoud H., Carré C., et al. (2022). “Continental Atlantic rivers: The seine basin,” in Rivers of Europe, 2nd edition. Eds. Tockner K., Zarfl C., Robinson C. (Amsterdam: Elsevier), 291–330.
Garnier J., Passy P., Thieu V., Callens J., Silvestre M., Billen G. (2013). “Fate of nutrients in the aquatic continuum of the seine river and its estuary: Modelling the impacts of human activity changes,” in The watershed biogeochemical dynamics at Large river-coastal interfaces: Linkages with global climate change. Eds. Bianchi T. S., Allison M. A., Cai W.-J. (Cambridge: Cambridge University Press).
Garnier J., Ramarson A., Billen G., Théry S., Thiéry D., Thieu V., et al. (2018). Nutrient inputs and hydrology together determine biogeochemical status of the Loire river (France): Current situation and possible future scenarios. Sci. Total Environ. 637–638, 609–624. doi: 10.1016/j.scitotenv.2018.05.045
Glibert P., Burford M. (2017). Globally changing nutrient loads and harmful algal blooms: Recent advances, new paradigms, and continuing challenges. Oceanography 30, 58–69. doi: 10.5670/oceanog.2017.110
Glibert P. M., Burkholder J. M. (2006). “The complex relationships between increasing fertilization of the earth, coastal eutrophication and proliferation of harmful algal blooms,” in Ecology of harmful algae. Eds. Granéli E., Turner J. (Heidelberg: Springer), 341–354.
Grosse J., Burson A., Stomp M., Huisman J., Boschker H. T. S. (2017). From ecological stoichiometry to biochemical composition: Variation in n and p supply alters key biosynthetic rates in marine Phytoplankton.Front. Microbiol. 8. doi: 10.3389/fmicb.2017.01299
Haropa (2014). Projet stratégique 2015–2020 (Paris, France: Ports de Paris). Available at: https://fr.calameo.com/read/00134416550222bc5417e.
Howden S. M., Soussana J.-F., Tubiello F. N., Chhetri N., Dunlop M., Meinke. H. (2007). Adapting agriculture to climate change. Proc. Natl. Acad. Sci. 104, 19691–19696. doi: 10.1073/pnas.0701890104
INSEE (2022) Projections de population 2013-2050 pour les départements et les régions. Available at: https://www.insee.fr/fr/statistiques/2859843.
Isidoro D., Aragüés R. (2007). River water quality and irrigated agriculture in the ebro basin: An overview. Int. J. Water Resour. Dev. 23, 91–106. doi: 10.1080/07900620601159743
Justić D., Rabalais N. N., Turner R. E. (1995). Stoichiometric nutrient balance and origin of coastal eutrophication. Mar. Pollut. Bull. 30, 41–46. doi: 10.1016/0025-326X(94)00105-I
Klasen S., Meyer K. M., Dislich C., Euler M., Faust H., Gatto M., et al. (2016). Economic and ecological trade-offs of agricultural specialization at different spatial scales. Ecol. Economics 122, 111–120. doi: 10.1016/j.ecolecon.2016.01.001
Kobori H., Taga N. (1979). Phosphatase activity and its role in the mineralization of organic phosphorus in coastal sea water. J. Exp. Mar. Bio. Ecol. 36, 23–39. doi: 10.1016/0022-0981(79)90098-4
Labry C., Delmas D., Youenou A., Quere J., Leynaert A., Fraisse S., et al. (2016). High alkaline phosphatase activity in phosphate replete waters. Case two macrotidal estuaries Limnol. Oceanogr. 61, 1513–1529. doi: 10.1002/lno.10315
Lafite R., Garnier J., De Jonge V. (Ed.) (2007). Consequences of estuarine management on hydrodynamics and ecological functioning: ECSA 38th Symposium - Rouen 2004 Co-organisation Seine-Aval Programme and ECSA. Hydrobiologia (The Netherlands: Springer) 588, 302
Lafite R., Romaña L. A. (2001). A man-altered macrotidal estuary: The seine estuary (France): Introduction to the special issue. Estuaries 24, 939–939. doi: 10.1007/bf02691262
Lancelot C., Thieu V., Polard A., Garnier J., Billen G., Hecq W., et al. (2011). Ecological and economic effectiveness of nutrient reduction policies on coastal phaeocystis colony blooms in the southern north Sea: An integrated modeling approach. Sci. Total Environ. 409, 2179–2191. doi: 10.1016/j.scitotenv.2011.02.023
Lassaletta L., Billen G., Grizzetti B., Anglade J., Garnier. J. (2014). 50 year trends in nitrogen use efficiency of world cropping systems: The relationship between yield and nitrogen input to cropland. Environ. Res. Lett. 9, 105011. doi: 10.1088/1748-9326/9/10/105011
Le Hir P.. (2001). Courants, vagues et marées: les mouvements de l’eau. Seine-Aval Scientific Report 1.2, Paris, France, 32. doi: 10.2214/ajr.173.6.10584819
Le Noë J., Billen G., Esculier F., Garnier J. (2018). Long term socio-ecological trajectories of agro-food systems revealed by n and p flows: The case of French regions from 1852 to 2014. Agriculture Ecosyst. Environ. 265, 132–143. doi: 10.1016/j.agee.2018.06.006
Le Noë J., Billen G., Garnier J. (2017). How the structure of agro-food systems shapes nitrogen, phosphorus, and carbon fluxes: The generalized representation of agro-food system applied at the regional scale in France. Sci. Total Environ. 586, 42–55. doi: 10.1016/j.scitotenv.2017.02.040
Le Noë J., Billen G., Lassaletta L., Silvestre M., Garnier J. (2016). La place du transport de denrées agricoles dans le cycle biogéochimique de l’azote en France: un aspect de la spécialisation des territoires. Cahiers Agricultures 25, 15004. doi: 10.1051/cagri/2016002
Le Noë J., Roux N., Billen G., Gingrich S., Erb K., Krausmann F., et al. (2020). The phosphorus legacy offers opportunities for agro ecological transition (France 1850-2075). Environ. Res. Lett. 15. doi: 10.1088/1748-9326/ab82cc
Li H., Li X., Xu Z., Liang S., Ding Y., Song D., et al. (2022). Nutrient budgets for the bohai Sea: Implication for ratio imbalance of nitrogen to phosphorus input under intense human activities. Mar. Pollut. Bull. 179, 113665. doi: 10.1016/j.marpolbul.2022.113665
Lynch J., Cain M., Frame D., Pierrehumbert R. (2021). Agriculture's contribution to climate change and role in mitigation is distinct from predominantly fossil CO2-emitting sectors. Front. Sustain. Food Syst. 4. doi: 10.3389/fsufs.2020.518039
Passy P., Gendre R. L., Garnier J., Cugier P., Callens J., Paris F., et al. (2016). Eutrophication modelling chain for improved management strategies to prevent algal blooms in the bay of seine. Mar. Ecol. Prog. Ser. 543, 107–125. doi: 10.3354/meps11533
Passy P., Gypens N., Billen G., Garnier J., Thieu V., Rousseau V., et al. (2013). A-model reconstruction of riverine nutrient fluxes and eutrophication in the Belgian coastal zone since 1984. J. Mar. Syst. 128, 106–122. doi: 10.1016/j.jmarsys.2013.05.005
Poux X., Aubert P.-M. (2018). An agro-ecological Europe in 2050: multifunctional agriculture for healthy eating. findings from the ten years for agroecology (TYFA) modelling exercise Study N°09/18, (Paris, France: Iddri-AScA) 74.
Quemada M., Baranski M., Nobel-de Lange M. N. J., Vallejo A., Cooper J. M. (2013). Meta-analysis of strategies to control nitrate leaching in irrigated agricultural systems and their effects on crop yield. Agriculture Ecosyst. Environ. 174, 1–10. doi: 10.1016/j.agee.2013.04.018
Raimonet M., Thieu V., Silvestre M., Oudin L., Rabouille C., Vautard R., et al. (2018). Coastal eutrophication potential under future climate change: a landward perspective. Front. Mar. Science section Mar. Biogeochemistry 5. doi: 10.3389/fmars.2018.00136
Redfield A. C., Ketchum B. H., Richards F. A. (1963). “The influence of organisms on the composition of sea-water,” in The Sea. Ed. Hill M. N. (New York, NY, USA: JohnWiley), 12–37.
Romero E., Garnier J., Billen G., Ramarson A., Riou P., Le Gendre R. (2019). Modeling the biogeochemical functioning of the seine estuary and its coastal zone: export, retention and transformations. Limnology Oceanography. 64, 895–912. doi: 10.1002/lno.11082
Romero E., Garnier J., Lassaletta L., Billen G., Gendre R. L., Riou P., et al. (2013). Large-Scale patterns of river inputs in southwestern Europe: Seasonal and interannual variations and potential eutrophication effects at the coastal zone. Biogeochemistry 113, 481–505. doi: 10.1007/s10533-012-9778-0
Romero E., Le Gendre R., Garnier J., Billen G., Fisson C., Silvestre M., et al. (2016). Long-term water quality in the lower seine: Lessons learned over 4 decades of monitoring. Environ. Sci. Policy 58, 141–154. doi: 10.1016/j.envsci.2016.01.016
SHOM (2015). MNT bathymétrique de façade atlantique (Projet homonim) (Brest, France: SHOM). doi: 10.17183/MNT_ATL100m_HOMONIM_WGS84
Smayda T. J. (1990). “Novel and nuisance phytoplankton blooms in the sea: evidence for a global epidemic,” in Toxic marine phytoplankton. Eds. Granéli E., Sundström B., Edler R., Anderson D. M. (New York: Elsevier), 29–40.
Tatters A. O., Fu F. X., Hutchins D. A. (2012). High CO2 and silicate limitation synergistically increase the toxicity of Pseudo-nitzschia fraudulenta. PloS One 7 (2), e32116. doi: 10.1371/journal.pone.0032116
Tilman D., Cassman K. G., Matson P. A., Naylor R., Polasky. S. (2002). Agricultural sustainability and intensive production practices. Nature 418, 671–677. doi: 10.1038/nature01014
Turner R. E., Qureshi N. A., Rabalais N. N., Dortch Q., Justic D., Shaw R., et al. (1998). Fluctuating silicate: Nitrate ratios and coastal plankton food webs. Proc. Natl. Acad. Sci. U.S.A. 95, 13048–13051. doi: 10.1073/pnas.95.22.13048
Westhoek H., Lesschen J. P., Leip A., Rood T., Wagner S., De Marco A., et al. (2015). Nitrogen on the table: The influence of food choices on nitrogen emissions and the European environment (Edinburgh, UK: Centre for Ecology & Hydrology).
WFD (2000) EU Water framework directive (WFD), directive 2000/60/EC of the European parliament and of the council establishing a framework for the community action in the field of water policy. Available at: https://ec.europa.eu/environment/water/water-framework/index_en.html.
Keywords: scenarios, biogeochemical processes, estuary, eutrophication, agro-food system
Citation: Romero E, Garnier J, Billen G, Ramarson A, Riou P and Le Gendre R (2022) Assessing the water quality of the Seine land-to-sea continuum for three agro-food system scenarios. Front. Mar. Sci. 9:1010887. doi: 10.3389/fmars.2022.1010887
Received: 03 August 2022; Accepted: 14 October 2022;
Published: 28 October 2022.
Edited by:
Wei Cheng, Center, TaiwanReviewed by:
Maximilian Berthold, Mount Allison University, CanadaQinghua Ye, Deltares, Netherlands
Alfredo L. Aretxabaleta, United States Geological Survey (USGS), United States
Copyright © 2022 Romero, Garnier, Billen, Ramarson, Riou and Le Gendre. This is an open-access article distributed under the terms of the Creative Commons Attribution License (CC BY). The use, distribution or reproduction in other forums is permitted, provided the original author(s) and the copyright owner(s) are credited and that the original publication in this journal is cited, in accordance with accepted academic practice. No use, distribution or reproduction is permitted which does not comply with these terms.
*Correspondence: Estela Romero, estela.romero@creaf.uab.es; Romain Le Gendre, romain.le.gendre@ifremer.fr