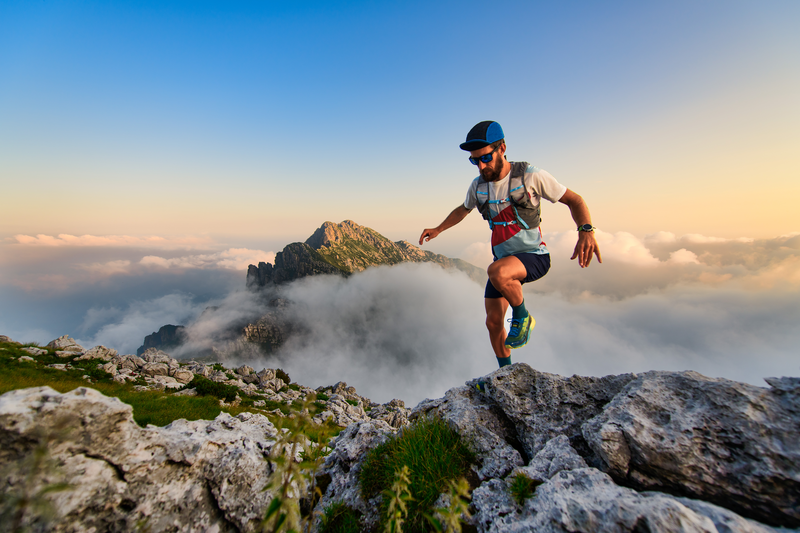
95% of researchers rate our articles as excellent or good
Learn more about the work of our research integrity team to safeguard the quality of each article we publish.
Find out more
EDITORIAL article
Front. Mar. Sci. , 18 August 2022
Sec. Marine Ecosystem Ecology
Volume 9 - 2022 | https://doi.org/10.3389/fmars.2022.1010651
This article is part of the Research Topic Ecophysiology and Biogeochemistry of Marine Plants in the Anthropocene View all 15 articles
Editorial on the Research Topic
Ecophysiology and biogeochemistry of marine plants in the anthropocene
Climate change in the Anthropocene detrimentally effects the livelihood of marine plants and their coastal habitats (Halpern et al., 2008; Waycott et al., 2009; Larkum et al., 2018a; Larkum et al., 2018b), due to increased frequency and severity of extreme environmental events such as: ocean deoxygenation, heatwaves, heavy rain and storm events (Easterling et al., 2000). Hypoxic conditions in the water column, e.g., induced by eutrophication or the shoaling of deoxygenated water (Diaz and Rosenberg, 2008), markedly affect the oxygen availability and internal aeration of marine plants (Colmer, 2003; Greve et al., 2003; Brodersen et al., 2017a), which increases their susceptibility to intrusion of reduced toxic compounds (e.g., hydrogen sulphide) from the sediment (Pedersen et al., 2004; Borum et al., 2005; Holmer and Hasler-Sheetal, 2014; Brodersen et al., 2015a). Coastal eutrophication-driven blooms of epiphytes on seagrass and other marine plant leaves (Borum, 1985) can shade the leaves for light and limit essential gas and nutrient exchange with the water column (Brodersen et al., 2015b; Brodersen et al., 2020; Noisette et al., 2020). Elevated temperature can weaken the blue carbon sink capacity of vegetated coastal ecosystems (Trevathan-Tackett et al., 2020) and can lead to oxygen deficiency in plants living close to their thermal stress threshold (Raun & Borum, 2013; Pedersen et al., 2016). Increased temperature can also lead to decreased seagrass productivity and biomass (George et al., 2018) and elevated microbial activity in the sediment increasing the sulphide pressure on the plants (Blaabjerg et al., 1998; Lamers et al., 2013) and elevated emissions of methane from seagrass sediments (George et al., 2020), further weakening their climate mitigation capacities. Finally, drops in salinity and high ammonium availability can interact synergistically in a negative impact on marine plant fitness (Villazán et al., 2015; Sola et al., 2020).
In light of the above-mentioned threats, future climate conditions will arguably have major impacts on the function and fitness of vegetated coastal ecosystems worldwide and thus on their ecological roles. Gaining a better understanding of the environmental regulation of marine plants and their ecophysiology is thus important for the planning of climate adaptation strategies and development of innovative and sustainable protection strategies for marine plants to better protect and secure vital ecosystem services and functions of vegetated coastal ecosystems in the Anthropocene. The present Research Topic is a compilation of 14 articles that aim to advance our understanding of how changing environmental conditions in the Anthropocene affects the ecophysiology and biogeochemistry of marine plants. The Research Topic is divided into 3 subtopics: (i) articles that discuss effects on the phyllosphere and rhizosphere conditions, (ii) articles that focus on impacts of ocean warming on marine plants and ecosystems, and (iii) articles that studied effects on seagrass metabolism and biogeochemical cycling.
Anthropogenic and climate change-related effects on seagrass phyllosphere and rhizosphere dynamics are discussed in the articles by Brodersen and Kühl, Koop-Jakobsen et al., Martin et al., Scholz et al., and Zhang et al. (subtopic 1). Brodersen and Kühl review how epiphytes affect the seagrass phyllosphere. They describe how the phyllosphere micro-habitat is affected by eutrophication-induced epiphytic biofilm communities and changing environmental conditions. Epiphytes impede leaf photosynthesis in light, owing to shading and a combination of induced hyperoxia and reduced CO2 availability due to phyllosphere basification and epiphytic carbon fixation. Also, absorbed light energy in the epiphyte cover can lead to leaf surface warming, potentially aggravating negative effects of climate change. In darkness, anoxia within the epiphytic biofilm can result in toxic nitric oxide production via denitrification. Such phyllosphere stress conditions at night-time may be exacerbated by global warming. Koop-Jakobsen et al. employed planar O2 optodes to describe rhizosphere oxygenation of high- and low-marsh ecotypes of Elymus athericus, a fast-spreading marsh plant. Results revealed oxygen release along the roots and oxic root zones in both marsh ecotypes in light and dark conditions. However, no differences were found in the belowground tissue oxygenation capacity between ecotypes, making E. athericus a highly competitive marsh plant in areas affected by fast sea-level rise. Martin et al. used 16S rRNA gene sequencing combined with analysis of seagrass nutrient (e.g., isotopic signatures of C and N) and heavy metal concentrations in tissue to investigate potential links between environmental metal and nutrient pollution to the microbial community associated with seagrass (Halophila ovalis) roots collected in an anthropogenically influenced estuary. Results indicate that changes in the composition of the seagrass root microbial community can potentially be used as a bioindicator for environmental conditions, such as pollution and contamination. Scholz et al. review experimental approaches to investigate the seagrass rhizosphere. They describe and discuss microenvironmental analysis techniques such as microsensors, optical sensors (e.g., planar optodes and sensor nanoparticles), and gel sampling methods (e.g., DETs and DGTs). Furthermore, they identify knowledge gaps and potential methodological advances in seagrass research. Studies of geochemical gradients in the seagrass rhizosphere are important as the plant-driven chemical gradients, protect the plants against reduced, anoxic sediment conditions (Brodersen et al., 2018) and can enable nutrient uptake (Brodersen et al., 2017b)while changes in the natural chemical gradients can cause plant stress and die-off (Borum et al., 2005; Brodersen et al., 2015a). Zhang et al. investigated the impact of epiphytic biofilms on the chemical microenvironment of the seagrass (Z. marina L.) leaf surface, as measured with microsensors. They demonstrated that the phyllosphere chemistry was mainly affected in the light by the metabolic activity of epiphytes leading to high O2 concentrations and pH in the seagrass leaf microenvironment, which reduced the CO2 availability for the seagrass leaf. The study thus advances our understanding of the effects of eutrophication-induced epiphytes on seagrass performance and the leaf surface microenvironment.
Impacts of increasing temperature on seagrass performance, health and abundance are described in articles by Graham et al., Hansen et al., Jueterbock et al., Plaisted et al., and Yucharoen et al. (subtopic 2). Graham et al. addressed the effects of seagrass wasting disease infection on eelgrass (Z. marina L.) growth and sugar production in a natural meadow in relation to ocean warming under climate change. Seagrass wasting disease impaired eelgrass growth and accumulation of belowground rhizome sugar, suggesting a disease-induced reduction of below-ground carbon accumulation. This study highlights the need to consider pathogen infection effects co-occurring with warmer conditions. Hansen et al. used gas exchange measurements to determine effects of global warming on the photosynthesis, respiration and diel O2 budget of eelgrass (Z. marina L.) leaves with and without epiphytes. Leaves with epiphytes were more affected by elevated seawater temperatures than bare seagrass leaves, as indicated by a lower light use efficiency, higher light requirements and a more negative diel O2 balance at high seawater temperatures. Epiphytic growth on seagrass leaves can thus aggravate other detrimental effects of climate change on seagrass performance.
Jueterbock et al. utilised a combination of Pulse Amplitude Modulated (PAM) fluorometry and RNA-sequencing to describe the photosynthetic characteristics of eelgrass (Z. marina L.) under strong annual fluctuations in day length to investigate adaptations to polar light conditions and the possibility of poleward range expansion into the Arctic region in response to global warming. Results suggested that Norwegian eelgrass populations are able to repress respiration and up-regulate genes related to carbon fixation and chlorophyll synthesis, to tolerate winter dim light conditions via metabolic dormancy. Plaisted et al. utilised long-term monitoring data to investigate regional changes in the presence and abundance of eelgrass (Z. marina L.) in relation to local sea surface temperatures. They demonstrated that the summer water temperature of the previous year is an important predictor of eelgrass presence in the region (Northeastern USA), where above average summer temperatures are associated with an increased probability of eelgrass absence, and below average summer temperatures are linked with the highest probability of eelgrass presence in the following year. The study thus delivers new insights on climate change related impacts on seagrass abundance. Yucharoen et al. utilised PAM fluorometry to assess the thermotolerance of tropical shallow-water corals, seagrasses and macroalgae. The study compared the physiological responses to elevated temperature and interspecific sensitivity of species from the different organism groups and furthermore examined the utility of an integrated biomarker response (IBR) approach to evaluate and integrate the candidate responses. Results showed that the IBR index provides a useful tool for assessing the vulnerability of marine organisms to ocean warming and demonstrated that species and organism groups differ in their sensitivity to elevated temperatures. This study underscore that extreme heating events and global warming can have consequences for ecosystem structure and functions in future oceans.
The metabolism of seagrass and their contribution to biogeochemical processes and element cycling in the Anthropocene is described in articles by Apostolaki et al., Asplund et al., Léger-Daigle et al., and Olive et al. (subtopic 3). Apostolaki et al. investigated the potential of dead seagrass Posidonia oceanica mattes to act as biogeochemical sinks and archives in coastal areas of the Mediterranean. Results indicate that dead P. oceanica mattes are sinks for carbon and contaminants and thus contain signals of past environmental change and contamination. The study thus adds important new knowledge of paleoecology to blue carbon research of seagrass sediments. Asplund et al. utilized benthic chambers to determine methane (CH4) emissions from Nordic seagrasses (Z. marina L.) during summer. The authors provide new fundamental insights on the effects of temperature, salinity and soil carbon on net methane emissions from seagrass sediments. Results showed relatively weak methane emissions from Nordic Z. marina meadows, where the net methane release was positively affected by the sedimentary carbon content. Thus, the emission of methane from seagrass meadows might increase with elevated temperatures and eutrophication driven increases in sedimentation. Léger-Daigle et al. used PAM fluorometry, pigment content and metabolic measurements to assess photoacclimation and light thresholds of cold temperate seagrasses (Z. marina L.). The study provides novel insights into the photoacclimation response of eelgrass to a wide range of light conditions via photosynthetic and physiological changes. The authors show that eelgrass is capable of photosynthetic adjustments at irradiances below the threshold between limiting and saturating irradiances, as well as, sustained maximal photosynthesis rates from saturating light down to a photon irradiance of 74 µmol photons m-2 s-1. These results highlight the capacity of eelgrass to acclimate to decreasing light conditions in coastal environments, often considered one of the main limiting factors for eelgrass growth and persistence. Finally, Olive et al. assessed the metabolism and nutrient cycling contribution of tropical seagrass (Syringodium isoetifolium) in the sediment and benthic community of a tropical reef lagoon. They show that the S. isoetifolium meadow was net autotrophic across the lagoon and reduced the heterotrophy of the whole benthic community. However, there was a large variability in the metabolic balance of the benthic components among sites, mainly related to the environmental variability found in the lagoon. This study highlights the need to investigate how community functioning can vary according to environmental variability driven by human activities in changing oceans.
In summary, the articles in this Research Topic elucidate the diverse range of potential negative effects of human activity and climate change related impacts on marine plants in the Anthropocene. Such effects include epiphyte-induced extremes in the seagrass leaf surface microenvironment, as well as ocean warming-induced reduction in seagrass abundance and potential positive correlation with increased seagrass wasting disease, which may reduce belowground carbon accumulation in infected meadows. However, the contributions to this research topic also provide insights into other important aspects such as seagrass paleoecology, factors affecting the microbial community of the rhizosphere, methane emissions, and photoacclimatory responses.
The editorial was written by KEB with contributions from all remaining co-authors.
KB was supported by a grant from the Villum Foundation (00028156). ST was supported by the Australian Research Council’s DECRA Fellowship (DE210101029). MK was supported by grants from the Independent Research Fund Denmark (DFF-8021-00308B) and the Gordon and Betty Moore Foundation through grant no. GBMF9206 to MK (https://doi.org/10.37807/GBMF9206).
We thank the Editorial staff of the Frontiers in Marine Science and Frontiers in Plant Science for their invitation and support in producing this Research Topic.
The authors declare that the research was conducted in the absence of any commercial or financial relationships that could be construed as a potential conflict of interest.
All claims expressed in this article are solely those of the authors and do not necessarily represent those of their affiliated organizations, or those of the publisher, the editors and the reviewers. Any product that may be evaluated in this article, or claim that may be made by its manufacturer, is not guaranteed or endorsed by the publisher.
Blaabjerg V., Mouritsen K. N., Finster K. (1998). Diel cycles of sulphate reduction rates in sediments of a Zostera marina bed (Denmark). Aquat. Microbial. Ecol. 15 (1), 97–102. doi: 10.3354/ame015097
Borum J. (1985). Development of epiphytic communities on eelgrass (Zostera marina) along a nutrient gradient in a Danish estuary. Mar. Biol. 87 (2), 211–218. doi: 10.1007/BF00539431
Borum J., Pedersen O., Greve T. M., Frankovich T. A., Zieman J. C., Fourqurean J. W., et al. (2005). The potential role of plant oxygen and sulphide dynamics in die-off events of the tropical seagrass, Thalassia testudinum. J. Ecol. 93, 148–158. doi: 10.1111/j.1365-2745.2004.00943.x
Brodersen K. E., Hammer K. J., Schrameyer V., Floytrup A., Rasheed M. A., Ralph P. J., et al. (2017a). Sediment resuspension and deposition on seagrass leaves impedes internal plant aeration and promotes phytotoxic H2S intrusion. Front. Plant Sci. 8, 657. doi: 10.3389/fpls.2017.00657
Brodersen K. E., Koren K., Moßhammer M., Ralph P. J., Kühl M., Santner J. (2017b). Seagrass-mediated phosphorus and iron solubilization in tropical sediments. Environ. Sci. Technol. 51 (24), 14155–14163. doi: 10.1021/acs.est.7b03878
Brodersen K. E., Koren K., Revsbech N. P., Kühl M. (2020). Strong leaf surface basification and CO2 limitation of seagrass induced by epiphytic biofilm microenvironments. Plant Cell Environ. 43 (1), 174–187. doi: 10.1111/pce.13645
Brodersen K. E., Lichtenberg M., Paz L.-C., Kühl M. (2015b). Epiphyte-cover on seagrass (Zostera marina l.) leaves impedes plant performance and radial O2 loss from the below-ground tissue. Front. Mar. Sci. 2, 58. doi: 10.3389/fmars.2015.00058
Brodersen K. E., Nielsen D. A., Ralph P. J., Kühl M. (2015a). Oxic microshield and local pH enhancement protects Zostera muelleri from sediment derived hydrogen sulphide. New Phytol. 205, 1264–1276. doi: 10.1111/nph.13124
Brodersen K. E., Siboni N., Nielsen D. A., Pernice M., Ralph P. J., Seymour J., et al. (2018). Seagrass rhizosphere microenvironment alters plant-associated microbial community composition. Environ. Microbiol. 20 (8), 2854–2864. doi: 10.1111/1462-2920.14245
Colmer T. D. (2003). Long-distance transport of gases in plants: a perspective on internal aeration and radial oxygen loss from roots. Plant Cell Environ. 26, 17–36. doi: 10.1046/j.1365-3040.2003.00846.x
Diaz R. J., Rosenberg R. (2008). Spreading dead zones and consequences for marine ecosystems. Science 321 (5891), 926–929. doi: 10.1126/science.1156401
Easterling D. R., Meehl G. A., Parmesan C., Changnon S. A., Karl T. R., Mearns L. O. (2000). Climate extremes: observations, modeling, and impacts. Science 289 (5487), 2068–2074. doi: 10.1126/science.289.5487.2068
George R., Gullström M., Mangora M. M., Mtolera M. S. P., Björk M. (2018). High midday temperature stress has stronger effects on biomass than on photosynthesis: A mesocosm experiment on four tropical seagrass species. Ecol. Evol. 8, 4508–4517. doi: 10.1002/ece3.3952
George R., Gullström M., Mtolera M. S., Lyimo T. J., Björk M. (2020). Methane emission and sulfide levels increase in tropical seagrass sediments during temperature stress: A mesocosm experiment. Ecol. Evol. 10 (4), 1917–1928. doi: 10.1002/ece3.6009
Greve T. M., Borum J., Pedersen O. (2003). Meristematic oxygen variability in eelgrass (Zostera marina). Limnol. Oceanogr. 48 (1), 210–216. doi: 10.4319/lo.2003.48.1.0210
Halpern B. S., Walbridge S., Selkoe K. A., Kappel CV, Micheli F, d'Agrosa C, et al. (2008). A global map of human impact on marine ecosystems. Science 319(5865), 948–952. doi: 10.1126/science.1149345
Holmer M., Hasler-Sheetal H. (2014). Sulfide intrusion in seagrasses assessed by stable sulfur isotopes–a synthesis of current results. Front. Mar. Sci. 1, 64. doi: 10.3389/fmars.2014.00064
Lamers L. P., Govers L. L., Janssen I. C., Geurts J. J., van der Welle M. E., Van Katwijk M. M., et al. (2013). Sulfide as a soil phytotoxin–a review. Front. Plant Sci. 4. doi: 10.3389/fpls.2013.00268
Larkum A. W., Kendrick G. A., Ralph P. J. (2018a). Seagrasses of Australia: Structure, ecology and conservation (Cham, Switzerland: Springer). (Book).
Larkum A. W., Pernice M., Schliep M., Davey P., Szabo M., Raven J. A., et al. (2018b). Photosynthesis and metabolism of seagrasses. In: Seagrasses Australia Springer (Book) pp, 315–342. doi: 10.1007/978-3-319-71354-0_11
Noisette F., Depetris A., Kühl M., Brodersen K. E. (2020). Flow and epiphyte growth effects on the thermal, optical and chemical microenvironment in the leaf phyllosphere of seagrass (Zostera marina). J. R. Soc. Interface 17 (171), 20200485. doi: 10.1098/rsif.2020.0485
Pedersen O., Binzer T., Borum J. (2004). Sulphide intrusion in eelgrass (Zostera marina l.). Plant Cell Environ. 27, 595–602. doi: 10.1111/j.1365-3040.2004.01173.x
Pedersen O., Colmer T. D., Borum J., Zavala-Perez A., Kendrick G. A. (2016). Heat stress of two tropical seagrass species during low tides–impact on underwater net photosynthesis, dark respiration and diel in situ internal aeration. New Phytol. 210 (4), 1207–1218. doi: 10.1111/nph.13900
Raun A. L., Borum J. (2013). Combined impact of water column oxygen and temperature on internal oxygen status and growth of Zostera marina seedlings and adult shoots. J. Exp. Mar. Biol. Ecol. 441, 16–22. doi: 10.1016/j.jembe.2013.01.014
Sola J., Sorrell B. K., Olesen B., Søndergaard Jørgensen M., Lund-Hansen L. C. (2020). Acute and prolonged effects of variable salinity on growth, gas exchange and photobiology of eelgrass (Zostera marina l.). Aquat. Bot. 165, 103236. doi: 10.1016/j.aquabot.2020.103236
Trevathan-Tackett S. M., Brodersen K. E., Macreadie P. I. (2020). Effects of elevated temperature on microbial breakdown of seagrass leaf and tea litter biomass. Biogeochemistry 151 (2), 171–185. doi: 10.1007/s10533-020-00715-1
Villazán B., Salo T., Brun F. G., Vergara J. J., Pedersen M. F. (2015). High ammonium availability amplifies the adverse effect of low salinity on eelgrass Zostera marina. Mar. Ecol. Prog. Ser. 536, 149–162. doi: 10.3354/meps11435
Keywords: seagrass, macroalgae, ecology, photosynthesis, climate change
Citation: Brodersen KE, Trevathan-Tackett SM, Noisette F, Björk M, Larkum AWD, Holmer M and Kühl M (2022) Editorial: Ecophysiology and biogeochemistry of marine plants in the anthropocene. Front. Mar. Sci. 9:1010651. doi: 10.3389/fmars.2022.1010651
Received: 03 August 2022; Accepted: 05 August 2022;
Published: 18 August 2022.
Edited and Reviewed by:
Angel Borja, Technological Center Expert in Marine and Food Innovation (AZTI), SpainCopyright © 2022 Brodersen, Trevathan-Tackett, Noisette, Björk, Larkum, Holmer and Kühl. This is an open-access article distributed under the terms of the Creative Commons Attribution License (CC BY). The use, distribution or reproduction in other forums is permitted, provided the original author(s) and the copyright owner(s) are credited and that the original publication in this journal is cited, in accordance with accepted academic practice. No use, distribution or reproduction is permitted which does not comply with these terms.
*Correspondence: Kasper Elgetti Brodersen, a2FzcGVyLmVsZ2V0dGkuYnJvZGVyc2VuQGJpby5rdS5kaw==
Disclaimer: All claims expressed in this article are solely those of the authors and do not necessarily represent those of their affiliated organizations, or those of the publisher, the editors and the reviewers. Any product that may be evaluated in this article or claim that may be made by its manufacturer is not guaranteed or endorsed by the publisher.
Research integrity at Frontiers
Learn more about the work of our research integrity team to safeguard the quality of each article we publish.