- College of Animal Science and Technology, Anhui Agricultural University, Hefei, China
Aquaporins (Aqp)1 and Aqp3 are the representatives of two types of aquaporins (classical aquaporin and aquaglyceroporin) and have been proven to potentially facilitate ammonia detoxification in air-breathing fishes. Paramisgurnus dabryanus is one of East Asia’s most important commercially farmed species and can breathe air directly through its hindgut. Besides, excellent ammonia tolerance was reported in this species. However, the mechanisms of its tolerance of high ammonia are still unclear. To explore the physiological roles of Aqp1 and Aqp3 in Paramisgurnus dabryanus, we detected the expression pattern of these two Aqps in several tissues and their transcriptional changes in response to different levels (30, and 70 mmol L-1 NH4Cl) and periods (0, 12, 48, and 96 h) of ammonia exposure and different periods (0, 12, 48, and 96 h) of drought stress. The aqp1 mRNA showed a high abundance in the brain and the osmoregulatory tissues, such as the intestine and kidney. While aqp3 was mainly expressed in the epithelium of skin and gills that were directly exposed to the environment. The expression level of aqp3 in gills was more responsive than aqp1 to ammonia challenges, although both of them were up-regulated. Considering the wide difference between aqp1 and aqp3 in the skin, aqp3 increased significantly after 12 h of air exposure. The changes of the two aqps in the intestine of P. dabryanus were relatively stable after ammonia stress. After drought stress, intestinal aqp1 increased significantly, while aqp3 decreased. Combined with the effects of tissue location and environmental stress on Aqps, it was suggested that Aqp1 was mainly involved in osmotic regulation, providing water as raw material for body metabolism. Accordingly, our results suggest that Aqp3 has the positive function of assisting ammonia transportation and epidermal moisturizing.
Introduction
Aquaporins (Aqp) are a superfamily member of integral membrane proteins and mainly function as membranal transport channels for water and glycerol (Cerdà and Finn, 2010). At present, 17 Aqps (Aqp0-16) have been identified in animals (Finn and Cerdà, 2015), while the classifications and physiological functions of Aqp13-16 are still unclear (Finn and Cerdà, 2015; Madsen et al., 2015). The studies of the physiological functions of Aqps were mostly found in mammals, including the transport of water, glycerol, urea, and several gases (e.g., CO2, NO, NH3, and O2). The previous results indicated that Aqp1, 3, 8, and 9 played an important role in regulating membranal NH3 permeability and consequently affected the ammonia transport in mammals (Saparov et al., 2007; Musa-Aziz et al., 2009; Herrera and Garvin, 2011; Geyer et al., 2013). For aquatic organisms, the most relevant studies focused on the effects of Aqps on regulating osmotic pressure (Deane et al., 2011; Engelund and Madsen, 2011). Only a few studies reported that Aqp1 and 3 were very related to ammonia tolerance in fish species (Chen et al., 2010; Kolarevic et al., 2012; Ip et al., 2013; Talbot et al., 2015). However, the effects and regulation mechanisms of Aqp1 and 3 on teleosts adapted to high environmental and/or endogenous ammonia are still unclear. Therefore, there is a great need to reveal the effects of Aqp1 and 3 on regulating ammonia tolerance in fish species because the results will help to analyze the mechanisms of fish adapted to deteriorating or drought environments.
Compared with mammals, the information on the physiological function of Aqps in teleosts is very poor. Whole-genome duplication in zebrafish (Danio rerio) obtained 20 Aqps (including isoforms) and the function of these Aqps was shown to be similar to mammals (Tingaud-Sequeira et al., 2010). Subsequently, functional Aqps were identified in several teleost species, such as Dicentrarchus labrax (Giffard-Mena et al., 2011), Sparus sarba (Deane et al., 2011), salmons (Kolarevic et al., 2012; Macqueen and Johnston, 2014), Oryzias dancena (Kim et al., 2014), Anabas testudineus (Ip et al., 2013), Protopterus annectens (Chng et al., 2016), Misgurnus mizolepis (Lee et al., 2017), Lateolabrax maculatus (Shen et al., 2019), and Trachidermus fasciatus (Ma et al., 2020). It follows that Aqps are widely distributed in teleosts. However, research on the physiological function of Aqps in teleosts is still limited. Recent reports have shown that Aqp1aa and Aqp1a1 located in the gill of zebrafish played an important role in gas transport and significantly increased the membranal NH3 permeability (Chen et al., 2010; Talbot et al., 2015). For Salmo salar, 22 days of ammonia exposure induced a reduction of aqp3a mRNA expression quantity, while up-regulation of aqp3a mRNA expression quantity was observed when the exposure time was prolonged to 105 days (Kolarevic et al., 2012), suggesting that Aqp3a could facilitate ammonia transport in fish species. Similarly, aqp1aa mRNA quantity in the gill and skin of A. testudineus decreased remarkably during ammonia exposure. This obvious down-regulation could be used to inhibit external ammonia uptake (Ip et al., 2013). Although the reports about the effects of Aqps on ammonia transport in fish are very poor, all the results of previous studies reveal that several Aqps, especially Aqp1 and 3, are closely related to the adaptation to internal and/or external ammonia loading in teleosts.
P. dabryanus is an important economic aquaculture variety in East Asia (Liu et al., 2018) and has the characteristics of low oxygen tolerance, fast growth, high nutrition, and delicious taste (Dong et al., 2018). Compared with other fishes, P. dabryanus has great advantages in aquaculture management and long-distance transportation (Zaccone et al., 2018). As a typical air-breathing fish (Zhang et al., 2016), the gills, skin, and intestines of P. dabryanus are the main sites that contribute to the alleviation of osmotic stress and harbor mechanisms to respire. Moreover, P. dabryanus is an excellent model for the study of the mechanisms controlling the acquisition, retention, and metabolic waste excretion due to its characteristics of high tolerance to the harsh environment (Zhang et al., 2017; Zhang et al., 2019; Zhang et al., 2021; Huang et al., 2022). However, the regulation mechanism of air-breathing fish facing a harsh environment is not clear. Further study of the function and molecular regulation mechanisms of Aqps in the process of environmental stress is not only the theoretical basis of fish physiology and ecology but also an important issue to improve the utilization rate of water in the aquaculture industry. Therefore, the present study aims to determine the tissue distribution of Aqp1 and Aqp3 and their transcriptional changes in response to high levels of exogenous and endogenous ammonia loading in P. dabryanus. These results provide theoretical support for a comprehensive understanding of the potential function of Aqps in biological processes and the coordination role of Aqps in maintaining physiological homeostasis. An understanding of the involvement of Aqps in ammonia defense in teleosts, especially in air-breathing species, leads to improved information on the air-breathing fish adapted to high ammonia environments. The present paper will provide a broader perspective on ammonia defensive strategies in teleosts and is helpful for molecular-assisted breeding of ammonia-resistant varieties.
Materials and methods
Ethics statement
This study was carried out by the principles of the Basel Declaration and Recommendations of the Guide for the Care and Use of Laboratory Animals published by the Animal Care Committee of Anhui Agricultural University (Hefei, China). The protocol was approved by the Animal Care Committee of Anhui Agricultural University.
Animals
Fish specimens of P. dabryanus (18-22 g) were obtained from a local Hefei aquatic market. Fish were kept in dechlorinated tap water at 22-24 °C in fiberglass tanks for at least 2 weeks regime before the experiments. P. dabryanus with similar specifications was selected from the tank (70 cm × 40 cm × 40 cm) and placed in a square plastic basin (30 cm × 20 cm × 20 cm).
Experimental design and sample collection
Seven somatic tissues (gill, intestine, skin, muscle, liver, kidney, and brain) were derived from healthy individuals for the tissue distribution assay. The ammonia loading group was configured with NH4Cl and set into two concentration groups of 30 mmol L-1 and 70 mmol L-1, using NaOH (4 mol L-1) to adjust the pH to 7.2-7.5. During the exposure test, the NH4Cl solution was totally exchanged every 24 hours to maintain the ammonia concentration. In the drought stress group, the selected P. dabryanus were directly placed in the same temporary incubator and added 100 ml of distilled water to keep the body moist during exposure, and the temperature was kept at (25.0 ± 1.0) °C. The 100 ml of distilled water was totally exchanged every 8 hours to maintain the humidity. Fish reared in temporary tanks were used as a control group. There were 3 parallel groups at each exposure time (12, 48, and 96 h) for each group, and 4 fish in each parallel group. After the temporary cultivation, the experimental fish were anesthetized with MS-222 (200 mg L-1). The fish were dissected and the tissues were taken, and the fish was frozen and stored at -80 °C for subsequent use.
RNA extraction and cDNA synthesis
The total RNA of the sample was extracted using the Ultrapure RNA Kit (DNase I), and the concentration and purity of RNA were measured using a spectrophotometer. The extracted RNA was checked using 1% agarose electrophoretically and a NanoDrop 2000 spectrophotometer (Thermo Fisher Scientific, USA) to verify integrity. RNA samples were kept at -20 °C. The HiScriptIII RT SuperMix for qPCR (+gDNA wiper) kit was used to reverse transcribe the RNA into cDNA. DNase treatment was performed using template RNA (2 µl), 4× gDNA Wiper Mix (4 µl), and RNAse-free ddH2O (10 µl) which were configured into a 16 µl mixture at 42 °C for 2 min. The reverse transcription reaction was carried out with 5 HiScriptII qRT SuperMix (4 µl) directly added to the reaction solution of 16 µl, which was kept at 37°C for 15 minutes and then at 85°C for 5 seconds.
Real-time quantitative PCR
The gene sequence was obtained using primers designed from the highly conserved regions of the transcriptome sequencing sequence in our laboratory. All primers in this experiment were synthesized by Sangon Biotech (Shanghai, China) (Table 1). The cDNA of each tissue was used as a template, and the mixed solution was configured as 2×AceQ qPCR SYBR Green Master Mix (10 µl), forward primes (0.4 µl), reverse primer (0.4 µl), template cDNA (2 µl), and ddH2O (7.2 µl). The following qPCR conditions were used: 5 minutes of pre-denaturation at 95°C, 10 seconds of denaturation at 95°C, 30 seconds of annealing at 60°C, and 40 cycles. Relative mRNA levels were calculated using the 2-△△Ct method as described by Livak and Schmittgen (2001), with the reference gene β-actin being used as an internal standard to normalize the results.
Data analysis
The variance homogeneity of the data was examined using Levene’s test. One-way (exposure time, different groups) and two-way (exposure time × NH4Cl concentration) analysis of variance (ANOVA) was used to compare mRNA expression levels. Tukey’s (homogeneity of variance) and Games-Howell’s (inhomogeneity of variance) multiple tests were performed when significant differences were found at the 0.05 level. The t-test was performed to compare the differences between the 30 mmol L-1 and 70 mmol L-1 NH4Cl groups at the same exposure time. Statistical analyses were performed using SPSS software (SPSS Inc. Chicago, IL, USA).
Results
The distribution of aqp1 and aqp3 in the tissues of P. dabryanus
The expression levels of aqp1 and aqp3 mRNA of P. dabryanus are shown in Figure 1. The aqp1 expression level in the brain was the richest, up to 1448-fold of the skin, whereas the expression levels in the intestine and kidney were 166 and 129-fold of the control, respectively (P<0.05, Figure 1A). The aqp1 expression was relatively low in the liver, muscle, gills, and skin (P>0.05, Figure 1A). The transcript of aqp3 in the skin was 7503-fold higher than that in the liver (P<0.05, Figure 1B). Aside from the skin, aqp3 mRNA was primarily expressed in the gills, which was 2027-fold higher than the control (P<0.05, Figure 1B). aqp3 was relatively lower in muscle and intestinal tissues, but still 279-fold and 178-fold higher than that in control tissue (P<0.05, Figure 1B). The aqp3 mRNA expression level was the lowest in the kidney, brain, and liver (P>0.05, Figure 1B).
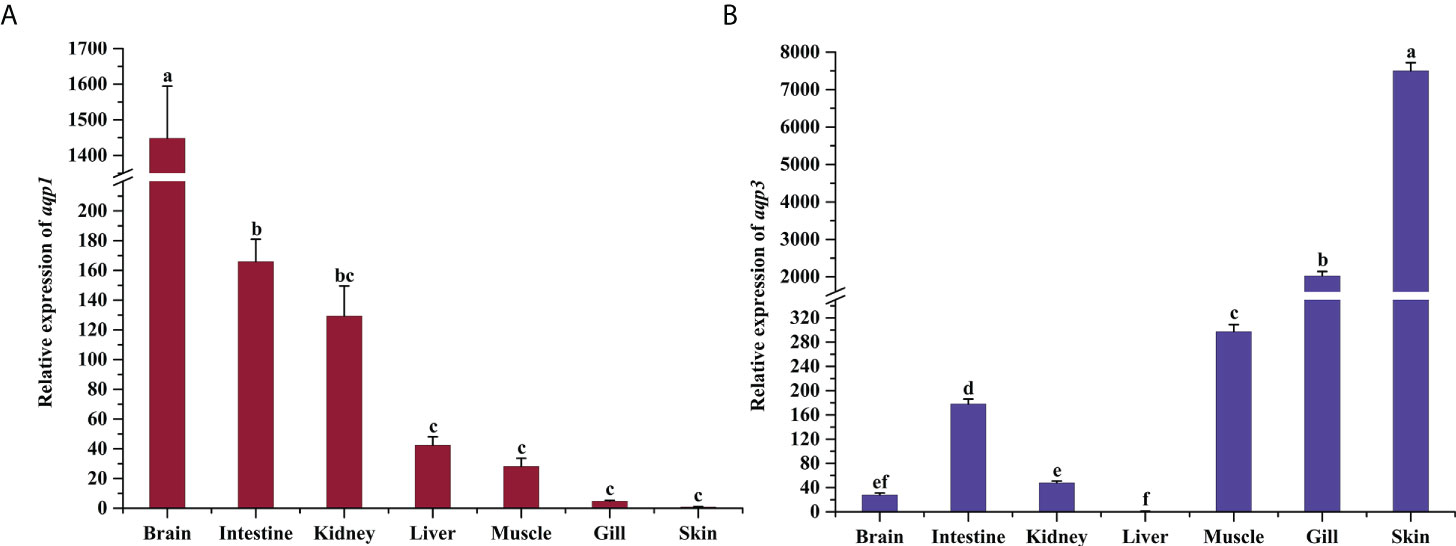
Figure 1 Tissue distribution and basal expression analysis of aqp1 (A) and aqp3 (B) in P. dabryanus. The different lowercase letters indicate significant differences among the different tissues. The bars represent the mean ± S.E. (n=3).
Changes in aqp1 and aqp3 expression after ammonia stress
Two-way ANOVA results showed there were significant interactions between different NH4Cl concentrations and exposure times on aqp1 and aqp3 expression in the gills, skin, and intestine (except aqp3 in gills) of P. dabryanus (P < 0.05, Table 2). The expression of aqp1 showed an opposite pattern in different groups in gill. It was significantly increased and reached 3.34-fold at 96 h in the 30 mmol L-1 group, while it decreased in the 70 mmol L-1 group (P<0.05, Figure 2A). aqp1 was significantly induced in the skin and increased to 3.78-fold in the 70 mmol L-1 group at 12 h (P<0.05, Figure 2A). Intestinal aqp1 only changed significantly at 12 h (P<0.05). It was increased in the low concentration group while decreased in the high concentration group (P>0.05, Figure 2A). It is worth noting that the changes in the expression levels of AQP1 and 3 in the skin were only related to the concentration of NH4CL, and were not significantly affected by exposure time(P>0.05, Table 2). aqp3 was significantly influenced by NH4Cl (P<0.05, Table 2) in the gills, and significantly up-regulated to 4.74 and 5.22-fold at 96 h of 30 mmol L-1 and 70 mmol L-1 groups, respectively (P<0.05, Figure 2B). The transcription of aqp3 in skin reached a maximum value of 4.11-fold at 48 h in the 30 mmol L-1 group (P<0.05, Figure 2B). Intestinal aqp3 increased significantly in the early period (P<0.05, Figure 2B). However, in the high concentration group, it was down-regulated in the early period (P<0.05, Figure 2B).
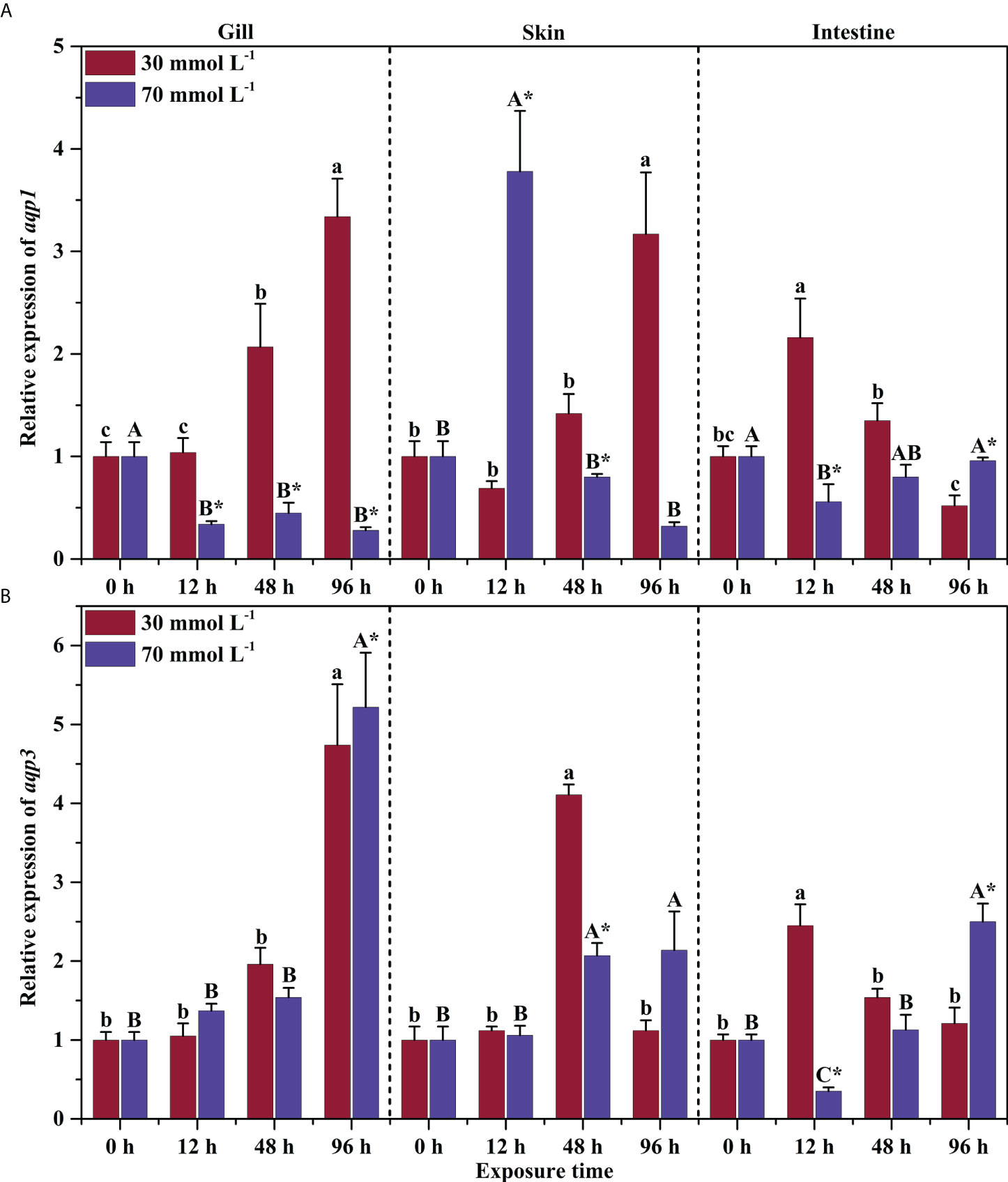
Figure 2 Effects of ammonia exposure on the relative expression of aqp1 (A) and aqp3 (B) in different tissues of P. dabryanus. The different lowercase letters in the same tissue are significant differences among the various periods of 30 mmol L-1 NH4Cl exposure. The different capital letters in the same tissue are significant differences among the various periods of 70 mmol L-1 NH4Cl exposure. *Significant difference between 30 mmol L-1 and 70 mmol L-1 NH4Cl exposure groups. The bars represent the mean ± S.E. (n=3).
aqp1 and aqp3 expression changes after air exposure
The expression levels of aqp1 and aqp3 in the gills of P. dabryanus showed a similar pattern under drought conditions, and gradually decreased with the exposure time (P<0.05, Figure 3A). Transcripts of aqp1 and aqp3 showed opposite changes in the skin. During the early exposure time, aqp1 had no significant change, but higher mRNA levels were observed as the time went by (P<0.05, Figure 3A). Transcription of aqp3 increased significantly to 1.82-fold in a short period and then began to decline significantly (P<0.05, Figure 3B). The changes in intestinal aqp1 were more rapid than those of aqp3. The mRNA level of aqp1 increased significantly (4.8-fold) in the early time and remained significant at 96 h (P<0.05, Figure 3A), whereas the mRNA level of aqp3 decreased and was significantly lower than the control group (P<0.05, Figure 3B).
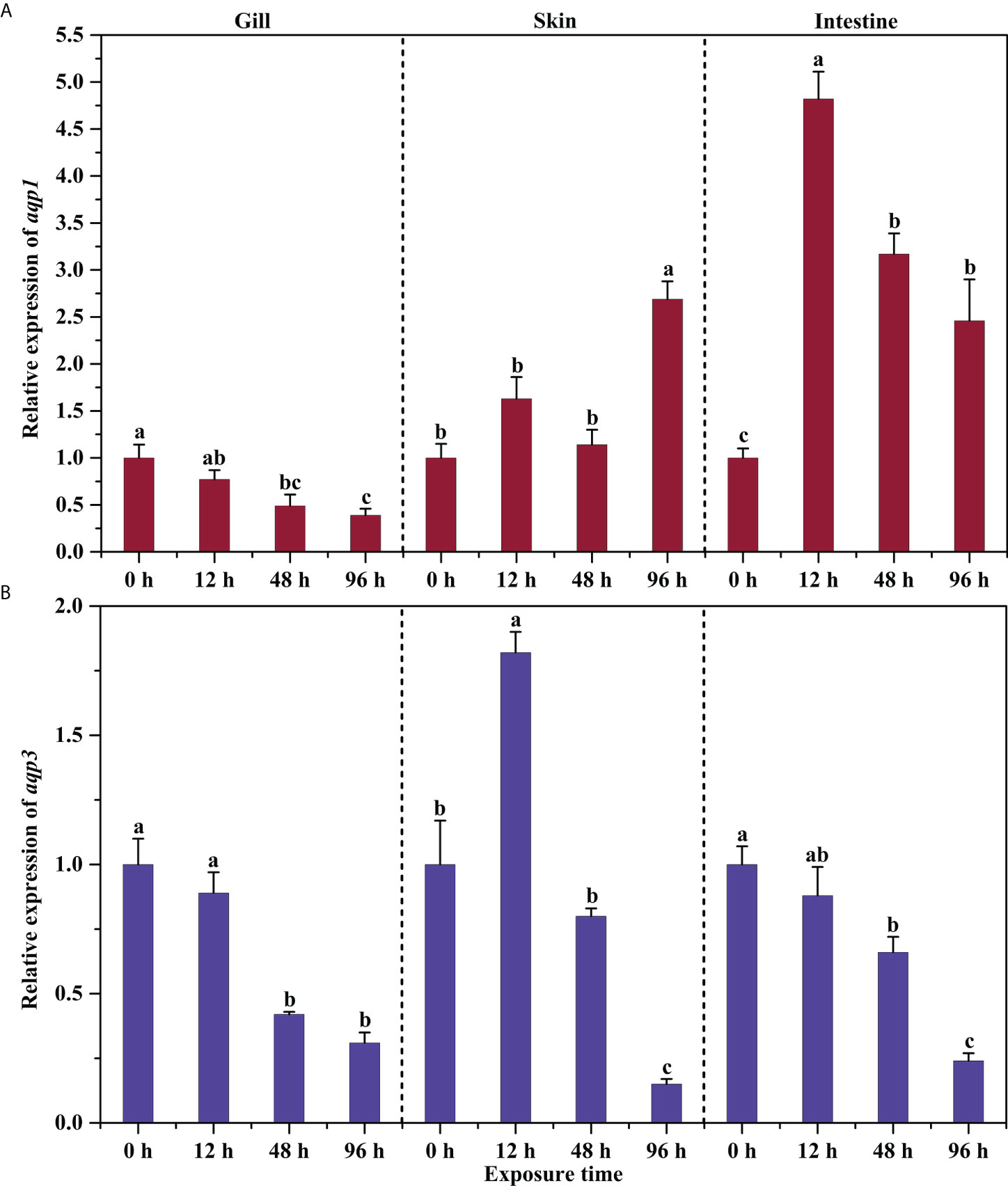
Figure 3 Effects of aerial exposure on the relative expression of aqp1 (A) and aqp3 (B) in different tissues of P. dabryanus. The different lowercase letters in the same tissue are significant differences among the various periods of aerial exposure. The bars represent the mean ± S.E. (n=3).
Discussion
Tissue expression assay of P. dabryanus aqp1 and aqp3
In the present study, transcripts encoding aqp1 were detectable in the brain, intestine, gills, kidney, and other tissues of P. dabryanus, which play an important role in osmotic regulation, while aqp3 was present in high levels in the skin, gills, and intestine that regulate respiration and osmotic pressure. The two distinct Aqps in the European eel (Anguilla anguilla) also have a similar distribution pattern. aqp1 was highly expressed in the brain, eye, and heart, but much lower in the esophagus, stomach, intestine, skeletal muscles, gills, and kidney. Aquaglyceroporin was mainly expressed in the intestine and kidney, but rarely in the brain (Martinez et al., 2005a).
The expression level of aqp1 in the brain of P. dabryanus was the highest, coinciding with the mammalian species, which plays an important role in cerebral edema, hydrocephalus, and the migration of brain tumors (Li et al., 2020). As the regulatory center, brain cells rich in Aqp1 can maintain cell matter exchange and energy production through rapid water transportation. M. mizolepis Aqp1a is also mainly distributed in heart and brain tissues, requiring high energy consumption (Lee et al., 2017). P. dabryanus brain aqp3 mRNA abundance was low, which might be due to its substrate permeability to NH3 and CO2 (Geyer et al., 2013). NH3 is neurotoxic to brain cells and can trigger severe blood-brain barrier breakdown, neuroinflammation, mitochondrial dysfunction, and affect the concentration of NO and Ca+ in cells, thereby leading to the excessive production of reactive oxygen species (ROS) (Jo et al., 2021). Excessive CO2 can cause increased cerebral blood volume, resulting in cerebral edema and increased intracranial pressure (Deng et al., 2020). A low level of Aqp3 in the brain can avoid the toxic damage of NH3 and CO2. The expression of aqp3 in P. dabryanus skin was very surprising. Similarly, it also has obvious advantages in external exposed organs such as the fins, gills, and eyes of M. mizolepis (Lee et al., 2017). Different from the first two species, the content of Aqp3 in the spleen and gills of Mozambique tilapia (Oreochromis mossambicus) was higher than that in the skin (Watanabe et al., 2005). For air-breathing fish like P. dabryanus, the skin acts as a transporting epithelium for gas, ions, and nutrients. Thus, the abundance of Aqp3 in the skin enables the epidermis to play a more active physiological role (Glover et al., 2013).
Gills are the primary water transport pathway, accounting for approximately 90% of total body water influx (Foguesatto et al., 2017). The expression level of branchial aqp1 in P. dabryanus was relatively low, which may be due to its role in concert with other homologs (e.g., Aqp3 in this study). Immunolocalization of the gill epithelium of Coris julis showed that Aqp1 was located in chlorine cells, while Aqp3 was in chlorine cells and accessory cells (Brunelli et al., 2010). Cutler and Cramb (2002) believed that Aqp3 may involve the discharge of ammonia and urea in eel gills, in addition to discharging excess water to prevent cell swelling and bursting.
P. dabryanus aqp3 mRNA exists sporadically in the kidney, as well as in spiny dogfish, Squalus acanthias, and these findings are consistent with our results (Cutler et al., 2022). After seawater domestication, aqp3 expression levels in the kidneys of European eel, Atlantic salmon (Salmo salar), and European bass (Dicentrarchus labrax) were significantly increased (Engelund and Madsen, 2011). In contrast to the European yellow eel, European silver eel Aqp1 is mainly distributed in the apical brush border of renal epithelial cells, with a small amount in vascular endothelium (Martinez et al., 2005a). Taken together, Aqp3 has potential functional roles in water retention, glycerol supplementation, and urea transportation in the teleost kidney. The expression level of aqp1 in the intestine of European eels in seawater was significantly increased by 10-25-fold compared to that in freshwater (Martinez et al., 2005b). European eel intestine Aqp3 was distributed in macrophage-like cells and goblet cells, and the number of goblet cells increased greatly and became wider and more round after seawater acclimatization (Lignot et al., 2002). The distribution characteristics of Aqp3 in the rectum indicated the potential role of Aqp3 in mucus secretion for keeping the surface of the intestine moist. The piscine liver performs basic metabolic functions, including bile formation and excretion, and is also known as the main detoxification organ, participating in the contribution of macrophages (Mokhtar, 2018). It has been reported that hepatic Aqp3 may be involved in both mammalian Kuffer cell migration and secretion of pro-inflammatory cytokines, reflecting its involvement in immune response (Rodríguez et al., 2011). The expression level of aqp3 in the liver of P. dabryanus was relatively lower compared with aqp1. Injection of LPS or Poly (I:C) can significantly increase aqp1 and aqp3 transcript levels in mud loach liver (Lee et al., 2017). Immune challenge-induced changes indicate that the two types of aquaporins have an important role in responding to inflammatory diseases or conditions. Transcripts encoding Aqp1 and Aqp3 were low in the muscle of P. dabryanus, which might be due to the presence of other homologous. Aqp4 was found to be abundant in the skeletal muscle of mammals using double immunofluorescence (Vizzaccaro et al., 2018). Abundant Aqp1 exists in tissues with high energy consumption, such as the human heart and skeletal muscle, but little Aqp3 was observed (Abdel-Sater, 2018). The co-expression of aqp4 and aqp3 in skeletal muscle may represent the need for water and non-ionic solute transportation.
Expression changes of aqp1 and aqp3 after ammonia and drought stress
The abundance of aqp3 mRNA in the gills of yellow and silver eels decreased by 76% and 97%, respectively, after 3 weeks of domestication in seawater (Cutler and Cramb, 2002). The expression of P. dabryanus gill aqp3 was always up-regulated under ammonia stress even when aqp1 was inhibited in high ammonia groups (70 mmol L-1). These findings suggest that aqp3 can not only regulate water flux but also facilitate ammonia excretion. The transcription levels and protein abundance of African lungfish (Protopterus annectens) gill aqp1 and aqp3 were both significantly down-regulated during the aestivation stage (Chng et al., 2016). This changing profile was also represented in P. dabryanus gill when exposed to air. This may be due to the closure of gill function during drought and aestivation, and the down-regulation of Aqp can reduce water loss of the gill epithelium.
Aqp3 and the homolog Aqp3l were detected and mainly expressed in the gills, skin, and eyes of zebrafish (Hamdi et al., 2009). Our ammonia loading study of P. dabryanus showed that skin aqp1 expression level was significantly up-regulated, whereas the aqp1aa mRNA of climbing perch (Anabas testudineus) under 100 mmol L-1 NH4Cl reached the opposite conclusion (Ip et al., 2013). The different results may be caused by the different concentrations of NH4Cl. The excessive concentration of NH4Cl has a toxic effect on climbing perch, leading to the direct down-regulation of the aqp1 transcription level.
Air-breathing fish excrete nitrogen-containing waste through their skin when their gills are no longer effective under drought stress (Glover et al., 2013). Both the gills and skin aqp1a abundance of climbing perch were increased after drought stress (Ip et al., 2013). The results of P. dabryanus in common with climbing perch, considering air exposure, could lead to water loss. Thus, Aqp1 might not be the main aquaporin for osmotic regulation. Hamdi et al. (2009) found that aquaglyceroporins (Aqp9a, Aqp9b, Aqp3, Aqp31, and Aqp10) have more effective trafficking of water or glycerin to the plasma membrane when heterologously expressed in Xenopus oocytes. The decrease of aqp3 transcription in the skin may be a feedback regulation mechanism of P. dabryanus loach to alleviate water loss and skin dryness caused by drought exposure. In addition, the inhibition of aqp1 and aqp3 transcription can reduce metabolizable energy requirements to a certain extent.
The intestine acts as the organ for nutrient absorption and osmotic regulation in the teleost. The water absorption rate of the hindgut is usually higher than in the prosogaster. The aqp1 gene expression in both freshwater and seawater eel intestines tends to be higher in the prosogaster than in the hindgut, and the aqp1 in the intestines of sea eel is significantly higher than in freshwater eel (Aoki et al., 2003). At least 8 aquaporin isoforms have been identified in the small intestine of mammals (Aqp1, Aqp2, Aqp3, Aqp4, Aqp7, Aqp8, Aqp10, and Aqp11) (Zhu et al., 2016). Although the expression level of Sockeye salmon (Oncorhynchus nerka) aqp8 and seawater eel aqp1 in the intestine were significantly up-regulated after seawater domestication, there was no significant change in aqp3 in the intestine and esophagus (Cutler et al., 2007; Choi et al., 2013). A concentration of 100 mmol L-1 NH4Cl had no significant effect on aqp1aa transcripts in the intestinal tract of climbing perch (Ip et al., 2013). However, in high ammonia conditions, P. dabryanus intestinal aqp3 was significantly increased. Therefore, it can be inferred that aqp3 is associated with mucus secretion and ammonia excretion of intestinal cells, while aqp1 plays a greater role in water absorption of the intestinal surface.
After 1 day of exposure to air, aqp1 mRNA expression in the prosogaster and hindgut of climbing perch increased sharply to 4.95-fold and 2.03-fold, respectively (Ip et al., 2013). This was consistent with our results, where P. dabryanus intestinal aqp1 was up-regulated to 4.82-fold after 12 h of drought stress, while aqp3 was relatively down-regulated. It is speculated that the significant up-regulation of aqp1 in the intestine was due to the absorption of water from intestinal food to compensate for the dehydration caused by drought, while the down-regulation of aqp3 may be due to the reduced secretion of intestinal mucus.
Conclusion
P. dabryanus aqp1 and aqp3 mRNA showed broad distribution in multiple tissues, including breath-responsive tissues as well as osmoregulatory tissues. Hence, the diversity of Aqps distribution and expression possibly suggests potential functional roles in response to environmental stimuli. The transcription of aqp1 and aqp3 genes was differentially modulated by high ammonia challenges. In particular, the aqp3 expression level in the gills was more responsive to ammonia loading than that of aqp1. Taken together, environmental deterioration resulted in significant modulation of P. dabryanus Aqp genes, and the expression profiles during ammonia loading and drought challenge indicated that, in addition to their well-established osmoregulatory functions, aqp1 and aqp3 appear to play important roles in the ammonia defensive process.
Data availability statement
The original contributions presented in the study are included in the article/supplementary material. Further inquiries can be directed to the corresponding author.
Ethics statement
The animal study was reviewed and approved by The Animal Care Committee of Anhui Agricultural University.
Author contributions
MH wrote the paper. MH, L-JZ, M-XW, and G-FP performed the experiments and analyzed the data. Y-LZ, reviewed and edited the manuscript. All authors have read and approved the final manuscript.
Funding
This work was funded by the National Natural Science Foundation of China (No. 32101248) and the Provincial Natural Science Research Project of Anhui Provincial Higher University Education (No. YJS20210227).
Conflict of interest
The authors declare that the research was conducted in the absence of any commercial or financial relationships that could be construed as a potential conflict of interest.
Publisher’s note
All claims expressed in this article are solely those of the authors and do not necessarily represent those of their affiliated organizations, or those of the publisher, the editors and the reviewers. Any product that may be evaluated in this article, or claim that may be made by its manufacturer, is not guaranteed or endorsed by the publisher.
References
Abdel-Sater K. A. (2018). Physiology of the aquaporins. Am. J. Biomed. Sci. 10, 167–183. doi: 10.5099/aj180300167
Aoki M., Kaneko T., Katoh F., Hasegawa S., Tsutsui N., Aida K. (2003). Intestinal water absorption through aquaporin 1 expressed in the apical membrane of mucosal epithelial cells in seawater-adapted Japanese eel. J. Exp. Biol. 206, 3495–3505. doi: 10.1242/jeb.00579
Brunelli E., Mauceri A., Salvatore F., Giannetto A., Maisano M., Tripepi S. (2010). Localization of aquaporin 1 and 3 in the gills of the rainbow wrasse Coris julis. Acta Histochem. 112, 251–258. doi: 10.1016/j.acthis.2008.11.030
Cerdà J., Finn R. N. (2010). Piscine aquaporins: an overview of recent advances. J. Exp. Zool. A. Ecol. Integr. Physiol. 313, 623–650. doi: 10.1002/jez.634
Chen L.-M., Zhao J., Musa-Aziz R., Pelletier M. F., Drummond I. A., Boron W. F. (2010). Cloning and characterization of a zebrafish homologue of human AQP1: a bifunctional water and gas channel. Am. J. Physiol. Regul. Integr. Comp. Physiol. 299, R1163–R1174. doi: 10.1152/ajpregu.00319.2010
Chng Y. R., Ong J. L. Y., Ching B., Chen X. L., Hiong K. C., Wong W. P., et al. (2016). Molecular characterization of aquaporin 1 and aquaporin 3 from the gills of the African lungfish, Protopterus annectens, and changes in their branchial mRNA expression levels and protein abundance during three phases of aestivation. Front. Physiol. 7. doi: 10.3389/fphys.2016.00532
Choi Y. J., Shin H. S., Kim N. N., Cho S. H., Yamamoto Y., Ueda H., et al. (2013). Expression of aquaporin-3 and -8 mRNAs in the parr and smolt stages of sockeye salmon, Oncorhynchus nerka: effects of cortisol treatment and seawater acclimation. Comp. Biochem. Physiol. A. Mol. Integr. Physiol. 165, 228–236. doi: 10.1016/j.cbpa.2013.03.013
Cutler C. P., Cramb G. (2002). Branchial expression of an aquaporin 3 (AQP-3) homologue is downregulated in the European eel Anguilla anguilla following seawater acclimation. J. Exp. Biol. 205, 2643–2651. doi: 10.1242/jeb.205.17.2643
Cutler C. P., Murray D., Ojo T., Harmon S., Maciver B., Cramb G., et al. (2022). Aquaporin (AQP) channels in the spiny dogfish, Squalus acanthias i: Characterization of AQP3 and AQP15 function and expression, and localization of the proteins in gill and spiral valve intestine. Comp. Biochem. Physiol. B. Biochem. Mol. Biol. 258, 110702. doi: 10.1016/j.cbpb.2021.110702
Cutler C. P., Phillips C., Hazon N., Cramb G. (2007). Cortisol regulates eel (Anguilla anguilla) aquaporin 3 (AQP3) mRNA expression levels in gill. Gen. Comp. Endocrinol. 152, 310–313. doi: 10.1016/j.ygcen.2007.01.031
Deane E. E., Luk J. C., Woo N. Y. S. (2011). Aquaporin 1a expression in gill, intestine, and kidney of the euryhaline silver sea bream. Front. Physiol. 2. doi: 10.3389/fphys.2011.00039
Deng R.-M., Liu Y.-C., Li J.-Q., Xu J.-G., Chen G. (2020). The role of carbon dioxide in acute brain injury. Med. Gas Res. 10, 81–84. doi: 10.4103/2045-9912.285561
Dong Z. G., Zhang M., Wei S. F., Ge H. X., Li L. T., Ni Q. G., et al. (2018). Effect of farming patterns on the nutrient composition and farming environment of loach, Paramisgurnus dabryanus. Aquaculture 497, 214–219. doi: 10.1016/j.aquaculture.2018.07.061
Engelund M. B., Madsen S. S. (2011). The role of aquaporins in the kidney of euryhaline teleosts. Front. Physiol. 2. doi: 10.3389/fphys.2011.00051
Finn R. N., Cerdà J. (2015). Evolution and functional diversity of aquaporins. Biol. Bull. 229, 6–23. doi: 10.1086/BBLv229n1p6
Foguesatto K., Boyle R. T., Rovani M. T., Freire C. A., Souza M. M. (2017). Aquaporin in different moult stages of a freshwater decapod crustacean: Expression and participation in muscle hydration control. Comp. Biochem. Physiol. A. Mol. Integr. Physiol. 208, 61–69. doi: 10.1016/j.cbpa.2017.03.003
Geyer R. R., Musa-Aziz R., Qin X., Boron W. F. (2013). Relative CO2/NH3 selectivities of mammalian aquaporins 0-9. Am. J. Physiol. Cell Physiol. 304, C985–C994. doi: 10.1152/ajpcell.00033.2013
Giffard-Mena I., Boulo V., Abed C., Cramb G., Charmantier G. (2011). Expression and localization of aquaporin 1a in the sea-bass (Dicentrarchus labrax) during ontogeny. Front. Physiol. 2. doi: 10.3389/fphys.2011.00034
Glover C. N., Bucking C., Wood C. M. (2013). The skin of fish as a transport epithelium: a review. J. Comp. Physiol. B. 183, 877–891. doi: 10.1007/s00360-013-0761-4
Hamdi M., Sanchez M. A., Beene L. C., Liu Q., Landfear S. M., Rosen B. P., et al. (2009). Arsenic transport by zebrafish aquaglyceroporins. BMC Mol. Biol. 10, 104. doi: 10.1186/1471-2199-10-104
Herrera M., Garvin J. L. (2011). Aquaporins as gas channels. Pflügers Arch. Eur. J. Physiol. 462, 623–630. doi: 10.1007/s00424-011-1002-x
Huang M., Wu M.-X., Zhang L.-J., Mi D., Zhang Y.-L. (2022). Effects of carbonate alkalinity on branchial gene expression in the large-scale loach (Paramisgurnus dabryanus). Front. Mar. Sci. 9. doi: 10.3389/fmars.2022.983615
Ip Y. K., Soh M. M. L., Chen X. L., Ong J. L. Y., Chng Y. R., Ching B., et al. (2013). Molecular characterization of branchial aquaporin 1aa and effects of seawater acclimation, emersion or ammonia exposure on its mRNA expression in the gills, gut, kidney and skin of the freshwater climbing perch, Anabas testudineus. PloS One 8, e61163. doi: 10.1371/journal.pone.0061163
Jo D., Kim B. C., Cho K. A., Song J. (2021). The cerebral effect of ammonia in brain aging: Blood-brain barrier breakdown, mitochondrial dysfunction, and neuroinflammation. J. Clin. Med. 10, 2773. doi: 10.3390/jcm10132773
Kim Y. K., Lee S. Y., Kim B. S., Kim D. S., Kim Y. K. N. (2014). Isolation and mRNA expression analysis of aquaporin isoforms in marine medaka Oryzias dancena, a euryhaline teleost. Comp. Biochem. Physiol. A. Mol. Integr. 171, 1–8. doi: 10.1016/j.cbpa.2014.01.012
Kolarevic J., Takle H., Felip O., Ytteborg E., Selset R., Good C. M., et al. (2012). Molecular and physiological responses to long-term sublethal ammonia exposure in Atlantic salmon (Salmo salar). Aquat. Toxicol. 124-125, 48–57. doi: 10.1016/j.aquatox.2012.07.003
Lee S. Y., Nam Y. K., Kim Y. K. (2017). Characterization and expression profiles of aquaporins (AQPs) 1a and 3a in mud loach Misgurnus mizolepis after experimental challenges. Fish. Aquat. Sci. 20, 23. doi: 10.1186/s41240-017-0068-6
Li Q., Aalling N. N., Förstera B., Ertürk A., Nedergaard M., Møllgård K., et al. (2020). Aquaporin 1 and the Na+/K+/2Cl- cotransporter 1 are present in the leptomeningeal vasculature of the adult rodent central nervous system. Fluids Barriers CNS 17, 15. doi: 10.1186/s12987-020-0176-z
Lignot J.-H., Cutler C. P., Hazon N., Cramb G. (2002). Immunolocalisation of aquaporin 3 in the gill and the gastrointestinal tract of the European eel Anguilla anguilla (L.). J. Exp. Biol. 205, 2653–2663. doi: 10.1242/jeb.205.17.2653
Liu J., Wang Q., Yuan J., Zhang T., Ye S., Li W., et al. (2018). “Integrated rice-field aquaculture in China, a long-standing practice, with recent leapfrog developments,” in Aquaculture in China: Success stories and modern trends, p. 180. Eds. Gui J.-F., Tang Q., Li Z., Liu J., Silva S. S. D. (Hoboken: John Wiley & Sons Ltd).
Livak K. J., Schmittgen T. D. (2001). Analysis of relative gene expression data using realtime quantitative PCR and the 2-ΔΔCT method. Methods 25, 402–408. doi: 10.1006/meth.2001.1262
Macqueen D. J., Johnston I. A. (2014). A well-constrained estimate for the timing of the salmonid whole genome duplication reveals major decoupling from species diversification. Proc. R. Soc B. Biol. Sci. 281, 20132881. doi: 10.1098/rspb.2013.2881
Madsen S. S., Engelund M. B., Cutler C. P. (2015). Water transport and functional dynamics of aquaporins in osmoregulatory organs of fishes. Biol. Bull. 229, 70–92. doi: 10.1086/BBLv229n1p70
Ma Q., Liu X., Li A., Liu S., Zhuang Z. (2020). Effects of osmotic stress on the expression profiling of aquaporin genes in the roughskin sculpin (Trachidermus fasciatus). Acta Oceanol. Sin. 39, 19–25. doi: 10.1007/s13131-020-1594-0
Martinez A.-S., Cutler C. P., Wilson G. D., Phillips C., Hazon N., Cramb G. (2005a). Cloning and expression of three aquaporin homologues from the European eel (Anguilla anguilla): effects of seawater acclimation and cortisol treatment on renal expression. Biol. Cell. 97, 615–627. doi: 10.1042/bc20040111
Martinez A.-S., Cutler C. P., Wilson G. D., Phillips C., Hazon N., Cramb G. (2005b). Regulation of expression of two aquaporin homologs in the intestine of the European eel: effects of seawater acclimation and cortisol treatment. Am. J. Physiol. Regul. Integr. Comp. Physiol. 288, R1733–R1743. doi: 10.1152/ajpregu.00747.2004
Mokhtar D. M. (2018). Cellular and stromal elements organization in the liver of grass carp, ctenopharyngodon idella (Cypriniformes: Cyprinidae). Micron 112, 1–14. doi: 10.1016/j.micron.2018.06.006
Musa-Aziz R., Chen L. M., Pelletier M. F., Boron W. F. (2009). Relative CO2/NH3 selectivities of AQP1, AQP4, AQP5, AmtB, and RhAG. Proc. Natl. Acad. Sci. U. S. A. 106, 5406–5411. doi: 10.1073/pnas.0813231106
Rodríguez A., Catalán V., Gómez-Ambrosi J., García-Navarro S., Rotellar F., Valentí V., et al. (2011). Insulin-and leptin-mediated control of aquaglyceroporins in human adipocytes and hepatocytes is mediated via the PI3K/Akt/mTOR signaling cascade. J. Clin. Endocrinol. Metab. 96, E586–E597. doi: 10.1210/jc.2010-1408
Saparov S. M., Liu K., Agre P., Pohl P. (2007). Fast and selective ammonia transport by aquaporin-8. J. Biol. Chem. 282, 5296–5301. doi: 10.1074/jbc.M609343200
Shang Z.-H., Huang M., Wu M.-X., Mi D., You K., Zhang Y.-L. (2021). Transcriptomic analyses of the acute aerial and ammonia stress response in the gill and liver of large-scale loach (Paramisgurnus dabryanus). Comp. Biochem. Physiol. C. Toxicol. Pharmacol. 250, 109185. doi: 10.1016/j.cbpc.2021.109185
Shen Y., He Y., Bi Y., Chen J., Zhao Z., Li J., et al. (2019). Transcriptome analysis of gill from Lateolabrax maculatus and aqp3 gene expression. Aquacult. Fish. 4, 247–254. doi: 10.1016/j.aaf.2019.03.004
Talbot K., Kwong R. W. M., Gilmour K. M., Perry S. F. (2015). The water channel aquaporin-1a1 facilitates movement of CO2 and ammonia in zebrafish (Danio rerio) larvae. J. Exp. Biol. 218, 3931–3940. doi: 10.1242/jeb.129759
Tingaud-Sequeira A., Calusinska M., Finn R. N., Chauvigné F., Lozano J., Cerdà J. (2010). The zebrafish genome encodes the largest vertebrate repertoire of functional aquaporins with dual paralogy and substrate specificities similar to mammals. BMC Evol. Biol. 10, 38. doi: 10.1186/1471-2148-10-38
Vizzaccaro E., Terracciano C., Rastelli E., Massa R. (2018). Aquaporin 4 expression in human skeletal muscle fiber types. Muscle Nerve 57, 856–858. doi: 10.1002/mus.26024
Watanabe S., Kaneko T., Aida K. (2005). Aquaporin-3 expressed in the basolateral membrane of gill chloride cells in Mozambique tilapia Oreochromis mossambicus adapted to freshwater and seawater. J. Exp. Biol. 208, 3673–3682. doi: 10.1242/jeb.01684
Zaccone G., Lauriano E. R., Capillo G., Kuciel M. (2018). Air- breathing in fish: Air- breathing organs and control of respiration: Nerves and neurotransmitters in the air-breathing organs and the skin. Acta Histochem. 120, 630–641. doi: 10.1016/j.acthis.2018.08.009
Zhang Y.-L., Shang Z.-H., Wang G.-Y., You K., Mi D. (2021). High concentrations of environmental ammonia induced changes in large-scale loach (Paramisgurnus dabryanus) immunity. Ecol. Evol. 11, 8614–9622. doi: 10.1002/ece3.7675
Zhang Y.-L., Wang G.-Y., Zhang Z.-H., Xie Y.-Y., Jin H., Dong Z.-R. (2019). Partial amino acid metabolism and glutamine synthesis as the ammonia defensive strategies during aerial exposure in Chinese loach Paramisgurnus dabryanus. front. Physiol 10. doi: 10.3389/fphys.2019.00014
Zhang Y., Wu Q., Hu W., Wang F., Shao W., Zhang C., et al. (2016). Morphological changes and allometric growth in hatchery-reared Chinese loach Paramisgurnus dabryanus (Dabry de thiersan). Chin. J. Oceanol. Limnol. 34, 757–762. doi: 10.1007/s00343-016-5079-0
Zhang Y.-L., Zhang H.-L., Wang L.-Y., Gu B.-Y., Fan Q.-X. (2017). Changes of ammonia, urea contents and transaminase activity in the body during aerial exposure and ammonia loading in Chinese loach Paramisgurnus dabryanus. Fish. Physiol. Biochem. 43, 631–640. doi: 10.1007/s10695-016-0317-0
Keywords: aquaporins, air-breathing fish, ammonia loading, drought stress, gene expression
Citation: Huang M, Zhang L-J, Wu M-X, Peng G-F and Zhang Y-L (2022) Aquaporins1 and 3 in the tissues of Paramisgurnus dabryanus and their expression profiles in response to ammonia and drought. Front. Mar. Sci. 9:1009679. doi: 10.3389/fmars.2022.1009679
Received: 02 August 2022; Accepted: 24 August 2022;
Published: 12 September 2022.
Edited by:
Mohamed Ashour, National Institute of Oceanography and Fisheries (NIOF), EgyptReviewed by:
Gamal Ammar, City of Scientific Research and Technological Applications, EgyptEman Abbas, National Institute of Oceanography and Fisheries (NIOF), Egypt
Copyright © 2022 Huang, Zhang, Wu, Peng and Zhang. This is an open-access article distributed under the terms of the Creative Commons Attribution License (CC BY). The use, distribution or reproduction in other forums is permitted, provided the original author(s) and the copyright owner(s) are credited and that the original publication in this journal is cited, in accordance with accepted academic practice. No use, distribution or reproduction is permitted which does not comply with these terms.
*Correspondence: Yun-Long Zhang, zhangyunlong@ahau.edu.cn