- 1School of Resource and Environmental Engineering, Ludong University, Yantai, China
- 2State Key Laboratory for Estuarine and Coastal Research, East China Normal University, Shanghai, China
- 3School of Geography, Geomatics and Planning, Jiangsu Normal University, Xuzhou, China
In this study, we collected surface sediments from the mainstream and major tributaries of the Yangtze River to systematically analyse their magnetic properties, and discuss the implications for tracing source–sink process of fluvio-clastic materials. Our results showed that the surface sediments from tributaries in the upper basin had a significantly higher content of ferromagnetic minerals and coarser grain sizes than that in the mid-lower basin. The magnetic susceptibility (χlf) of surface sediments from the mainstream was 34.16*10-8 m3/kg at Shigu, rapidly rose to 230.56*10-8 m3/kg at Yibin, and then dropped to 68.92*10-8 m3/kg at the river mouth. The χlf of Yalong River sediments in the upper basin was 276.86*10-8m3/kg, considerably higher than that of the sediments from other tributaries, and greatly contribute to the χlf of the sediments in the mainstream, reflecting the dominant constraining role of the Emeishan basalts. The distinctive magnetic properties of the upper core sediments from Jianghan basin and the Yangtze delta closely match those of surficial river sediments of the upper Yangtze basin. This may indicate the addition of clastic materials eroded from the upper basin, resulting from the cut-through of the Three Gorges during the evolution of the Yangtze River that occurred in the late Cenozoic. Investigating the magnetic properties of the surface sediments in the Yangtze River basin can provide insights into the source–sink process of clastic materials and environmental changes.
1. Introduction
Rivers, as the link between land and sea, play a key role in exchanging of global energy and materials. About 15–19*109 t of clastic materials are transported by rivers from basin to estuaries and marginal seas yearly (Milliman and Meade, 1983; Syvitski et al., 2003; Milliman and Farnsworth, 2011). This source–sink process makes a significant impact on earth surface processes such as soil erosion, shoreline change, and even global biogechemical cycle. (Wang et al., 2011; Wittmann et al., 2016; Li et al., 2017; Jiang et al., 2018; Nel et al., 2018; Park et al., 2021; Ji et al., 2022).
The Yangtze River, with a drainage area up to 1.8 × 106 km2, linking the Tibetan Plateau and the western Pacific marginal seas, is the longest river in Asia. It annually discharges 490×106 tons sediments into the delta and East China Sea, and dropped sharply to 130×106 tons due to the construction of the world’s largest reservoir– the Three Gorges Dam (Dai and Liu, 2013, Yang et al., 2014; Yang et al., 2015; Dai et al, 2018);. The source to sink transport processes of the Yangtze River sediments have recently attracted great interest on two aspects in terms of time scale (Fan et al., 2012). One is the attempt to decode the impact of human activities in river basin on spatio-temperal variation in sediment delivery processes, especially the industrial and agricultural development, dam construction (Yang et al., 2014; Yang et al., 2015; Dai et al., 2016; Luan et al., 2016; Dai et al., 2018; Yang et al., 2018; Guo et al., 2019; Mei et al., 2021; Yang et al., 2021). The other is to set up the provenance distinguishing models, to achieve robust understanding the evolution of Yangtze River in geological time and its relationship with the gigantic geomorphological changes in East Asia (uplift of the Tibet Plateau, the regional extension throughout eastern China) during the Late Cenozoic, and evolving east Asian monsoon (Yang et al., 2006; Gu et al., 2014; Yue et al., 2016; Liu et al., 2018; Yue et al., 2019; Zhang et al., 2019; Zheng et al., 2019; Yang et al., 2021).
A number of approaches including isotopic geochronology, geochemical compositions, mineral assemblages have been used to trace the source-sink processes of the Yangtze River sediment (Chappell et al., 2006; Yang et al., 2007; He et al., 2013; Zheng et al., 2013; Luo et al., 2014; Li et al., 2016; Yue et al., 2016; Yue et al., 2019; Yang et al., 2021). It is still difficult to fingerprint the discrimination of individual tributaries accurately for huge drainage area, complex tectonics, and various climatic environments (Fan et al., 2012; Yang et al., 2021). Therefore, it still needs to refine the sediment fingerprinting methodology and promote comparison studies between the surface sediments and sedimentary strata.
The magnetic properties of sediments have documented rich information on the environmental evolution (Liu et al., 2012). Previous studies have explored the magnetic properties of the Yangtze River sediments. However, they have primarily focused on local areas in the basin (Zhang et al., 2002; Niu et al., 2008; Zhou et al., 2008; Zhang et al., 2009; Luo et al., 2011; Luo et al., 2013; Pan et al., 2015; Chu et al., 2016). Systematic studies targeting the entire Yangtze River basin are limited. Here, we collected surface sediments from the mainstream and major tributaries of the Yangtze River basin, and systematically analysed their magnetic properties, and further discussed their implications for source-sink progress of sediment transport.
2. Background of study area
The Yangtze River basin lies between 90°33′–122°25′ E, 24°30′–35°45′ N. Geographically, the Yangtze River is approximately 6300 km long, and is divided into the upper, middle, and lower reaches with divisions at Yichang and Hukou. The upper Yangtze River is about 4500 km long, with an elevation above 2000 m and a basin area of roughly 100*104 km2. The major tributaries joining the mainstream in the upper reaches include the Yalong River, Dadu River, Min River, Tuo River, Jialing River, and Wu River. The middle Yangtze River is about 955 km long, with an elevation of 500–2000 m and a basin area of roughly 63*104 km2. The main tributaries joining the mainstream in the middle reaches include the Han River, Yuan River, Xiang River, and Gan River. Poyang Lake and Dongting Lake, two major freshwater lakes in China, are also distributed in the middle reaches. The lower Yangtze River is about 938 km long, with an elevation below 500 m and a basin area of roughly 12*104 km2. No large rivers join the mainstream of the Yangtze River in the lower reaches.
The Yangtze River basin is generally controlled by subtropical monsoon climate. The annual average temperature decreases from east to west. Due to the large area, complex landform, and monsoon climate, the mean annual precipitation varies dramatically throughout the Yangtze River basin. The mean annual precipitation is generally less than 400 mm in the source region and typically 800–1600 mm in the central and eastern parts of the basin.
Multiple types of source rocks are distributed in the upper reaches of the Yangtze River, including large areas of the Paleozoic carbonate rocks, Mesozoic sedimentary and igneous rocks, and scattered metamorphic rocks (Figure 1, Changjiang Water Resources Commission, 1999). The Emeishan basalts are distributed in the upper basin, and their formation is closely related to the volcanic eruption triggered by the intense mantle plume activities in the Permian. The middle and lower Yangtze River basin is mainly distributed with the Paleozoic marine, Mesozoic terrestrial sedimentary rocks, and Quaternary loose sediments of fluvial and lacustrine facies.
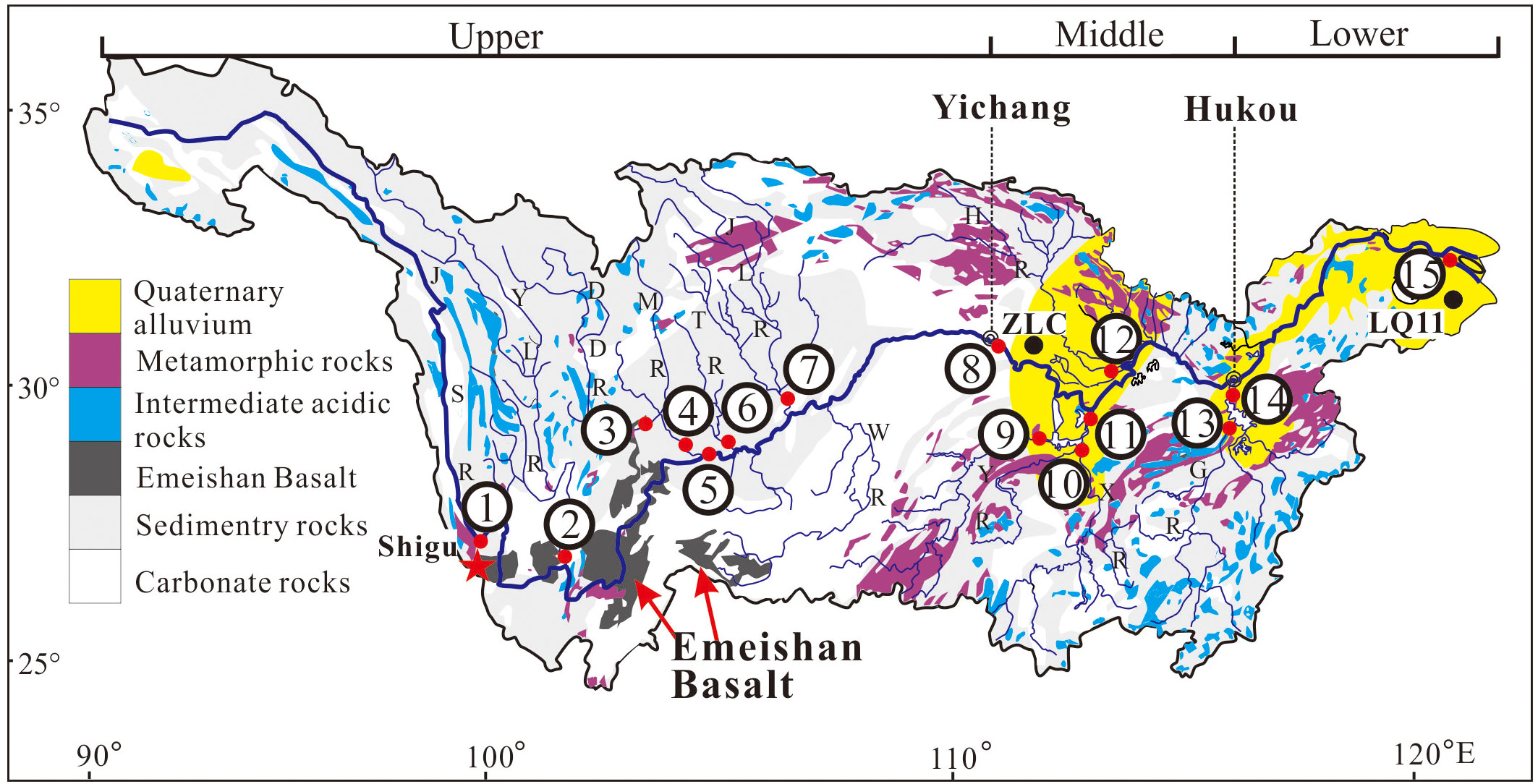
Figure 1 Distribution of source rocks in the Yangtze River basin and the sampling sites. 1) the large-scale Emeishan basalt block in the upper Yangtze basin; 2) sampling sites: ① Shigu (26°52′52″N, 99°57′31″E) ② Yalong River (YLR, 26°36′42″N, 101°48′00″E); ③ Dadu River (DDR, 29°33′19″N, 104°43′50″E); ④ Min River (MR, 29°35′28″N, 103°45′54″E); ⑤ Jinsha River (JSR, 26°36′42″N, 101°47′32″E); ⑥ Tuo River (TR, 29°35′39″N, 105°03′25″E); ⑦ Jialing River (JLR, 29°43′30″N, 106°32′08″E); ⑧ Three Gorges (Yichang, 30°40′47″N, 111°16′07″E); ⑨ Yuan River (YR, 29°01′50″N, 111°41′08″E); ⑩ Xiang River (XR, 27°49′27″N, 113°08′43″E); ⑪ Han River (HR, 30°22′53″N, 113°26′55″E); ⑫ Dongting Lake (DTL, 29°22′45″N, 113°05′10″E); ⑬ Gan River (GR, 28°32′28″N, 115°48′43″E); ⑭ Poyang Lake (PYL, 29°43′41″N, 116°11′51″E); ⑮ River Mouth(31°36′19″N, 121°25′26″E); the Emeishan Basalt site (star, 26°35′01″N, 101°38′36″E); 4) Zhoulao Core (ZLC), from the middle Yangtze basin, below the Three Gorges valley (Zhang et al., 2008; 30°02′09″N, 112°59′07″E); the Core LQ11 on the delta (Liu et al., 2018; 31°2′30″N, 121°23′54″E).
3. Methodology
Our research team collected mainstream samples in dry season (January 2013) on the floodplain at Shigu, Yibin, Yichang, and the river mouth (Figure 1). The tributary sediments were sampled at the confluences of tributaries and mainstream. To reduce random errors, we collected 2–7 surface sediment samples within a certain area around in each sampling site. A total of 65 samples were obtained from the mainstream and tributaries of the Yangtze River. Since Emeishan basalts are widely exposed in the upper Yangtze River basin, with an area of 30*104 km2, and their magnetic properties are distinct from those of other rock types, we collected one representative sample from the Jianchuan area in the upper basin (Figure 1).
The collected samples were dried in an oven at a low temperature of 30°C for 72 hours in the laboratory, avoiding any mineral changes (Maher, 1986). After the samples were completely dry, any plant roots or other impurities were removed from the sediments by plastic tweezers. Then, the sediments was packed into a non-magnetic plastic sample box (8 cm3 capacity) for further magnetic testing. Low and high frequency susceptibility (χlf and χlf) was measured at 0.47 kHz and 4.7 kHz, respectively, using a Bartington MS2 magnetometer. Each measurement consists of two replications in order to avoid measurement error. The frequency-dependent susceptibility (χfd%) was calculated as χfd% = (χlf – χhf)/χlf*100. The anhysteretic remanent magnetization (ARM) was determined using a Dtech2000 alternating field demagnetizer (with a maximum AC magnetic field of 100 mT and a DC magnetic field of 0.04 mT). The remanent magnetization was then measured by a Minispin spinner magnetometer. After obtaining the isothermal remanent magnetization under a 1 T magnetic field using an MMPM10 pulsed-field magnetometer, the samples were rapidly placed into the Minispin spinner magnetometer to measure their remanent magnetization. In this study, the isothermal remanent magnetization obtained under a 1 T magnetic field (IRM1T) is defined as saturation isothermal remanent magnetization (SIRM). After obtaining the SIRM, the samples were demagnetized under a 300 mT magnetic field in the opposite direction, and their remanent magnetization was measured again to derive the demagnetization parameters. S-ratio = – IRM– 300 mT/SIRM; HIRM (the “hard” IRM) = (SIRM – IRM– 300 mT)/2. The representative samples collected were examined using a variable field translational balance (VFTB) to obtain their hysteresis loops and thermomagnetic curves. The magnetic analyses were performed at the State Key Laboratory of Estuarine and Coastal Research, East China Normal University.
4. Results
4.1. Magnetic properties of surface sediments from the mainstream
The results showed that the χlf value of the surface sediments from the mainstream firstly increased and then decreased. The minimum value was 34.16*10-8 m3/kg at Shigu. Then it rapidly rose to 230.56*10-8 m3/kg at Yibin and slightly decreased to 210.50*10-8 m3/kg at Yichang. The value dropped to 68.92*10-8 m3/kg at the river mouth (Figure 2). The χfd% of all surface sediment samples from the mainstream was lower than 3%, indicating magnetic mineral grains in the surface sediments were basically free of superparamagnetic (SP) particles (Thompson and Oldfield, 1986). The variation trend of χARM was similar to those of χlf and SIRM. The χARM/χlf ratio gradually increased from Shigu through Yibin downstream, reaching the maximum at Yichang. Then, the value slightly decreased at the Yangtze River estuary. In comparison, the χARM/SIRM ratio exhibited a continuously increasing trend downstream, implying a gradually decreasing grain size of the magnetic minerals. The S-ratio was above 0.9, indicating the predominance of the low-coercivity ferromagnetic minerals. The HIRM value varied significantly among the sampling sites, the lowest at Shigu and the highest at Yibin (Thompson and Oldfield, 1986; Figure 2).
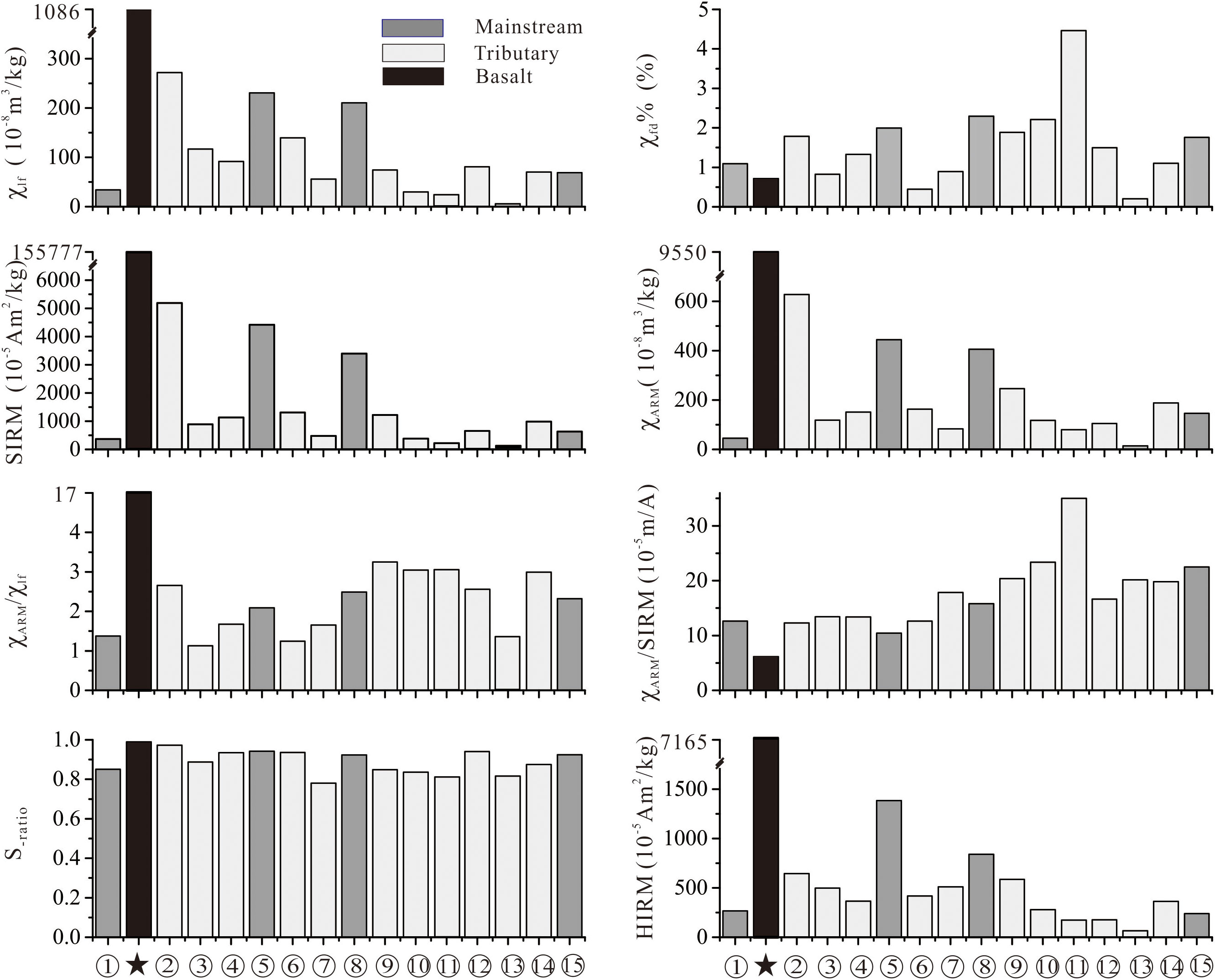
Figure 2 Magnetic parameters of the surface sediments from the mainstream and tributaries of the Yangtze River and those of the Emeishan basalts.
The variation patterns of the thermomagnetic curves for samples from different sites along the mainstream were relatively similar (Figure 3). The magnetization intensity sharply declined near 585°C and then decreased slightly, indicating a high content of magnetite and small amount of hematite in the samples. The hysteresis loops before and after paramagnetic correction were both narrow, and the closure point of the hysteresis loop after paramagnetic correction occurred at 300 mT, indicating a high abundance of low-coercivity ferromagnetic minerals (Thompson and Oldfield, 1986; Figure 4).
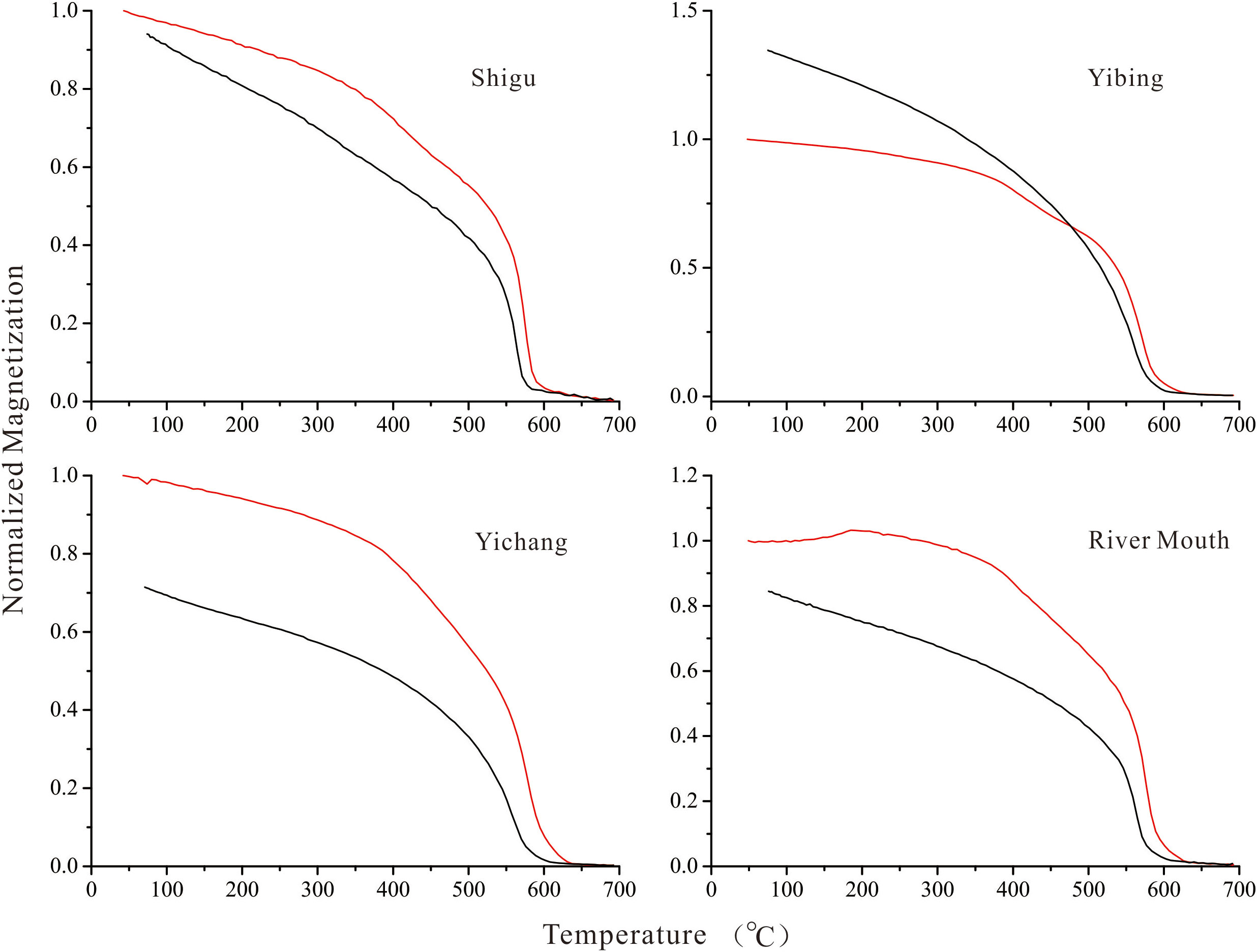
Figure 3 Thermomagnetic curves of the surface sediments from the Yangtze River basin (red: heating; black: cooling).
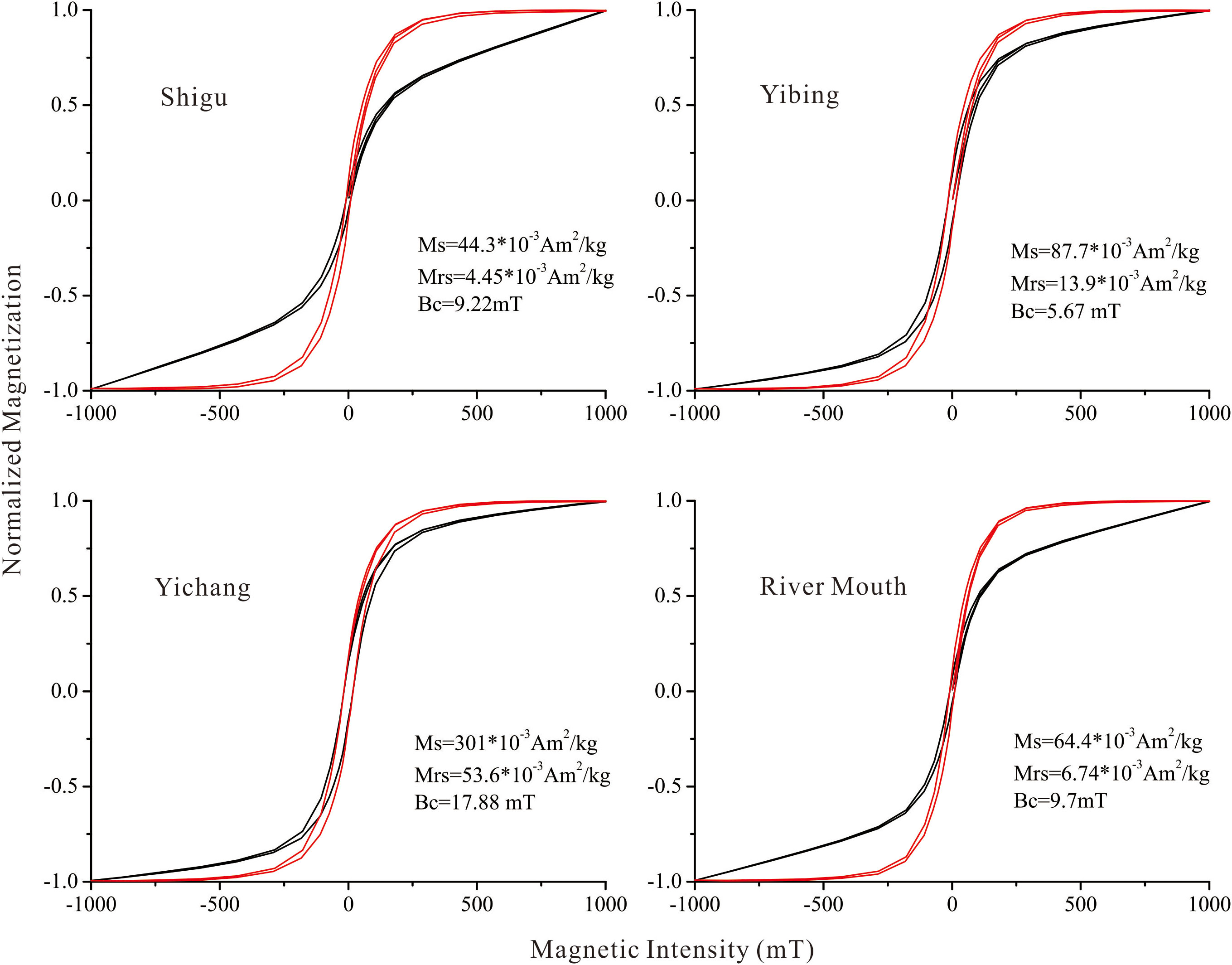
Figure 4 Hysteresis loops of the surface sediments from the Yangtze River basin (black: before paramagnetic correction; red: after the correction; Ms, Mrs and Bc in the figures means saturation magnetization, saturation remanent magnetization, and saturation coercivity respectively).
4.2. Magnetic properties of surface sediments from the tributaries
The χlf values of the surface sediments from the tributaries in the upper Yangtze River basin were generally high. The Yalong River sediments had the highest values, with a mean of 276.86*10-8 m3/kg. The Jialing River sediments had the lowest values, with a mean of 55.86*10-8 m3/kg (Figure 2). The χlf values of the surface sediments from the tributaries in the middle and lower Yangtze River basin were generally low. In which, the Han River sediments had the highest values, with a mean of 80.35*10-8 m3/kg. The Gan River sediments had the lowest values, with a mean value of 5.86*10-8 m3/kg. The SIRM of the surface sediments from the tributaries exhibited similar variation trends to the χlf results. The mean SIRM of the Yalong River sediments was 51908.04*10-5 Am2/kg, significantly higher than that of the sediments from the other tributaries. The SIRM of the Gan River sediments was the lowest, with a mean value of only 669.22*10-5 Am2/kg. Except for the surface samples from Dongting Lake, the χfd% of each tributary sample was less than 3%, indicating that the magnetic mineral grains in the surface sediments from most tributaries were essentially free of SP particles. Except for the Yalong River sediments, the χARM of the surface sediments from each tributary in the upper Yangtze River basin was 83.23–162.79*10-8 m3/kg, with minor fluctuations. The χARM/χlf and χARM/SIRM of most samples from the upper reaches were significantly smaller than those from the middle and lower reaches, indicating that the grain size of magnetic minerals from the tributaries in the upper Yangtze River basin was generally coarser than those from the tributaries in the middle and lower basins. The S-ratio and HIRM of most samples from the tributaries in the upper Yangtze River basin were slightly higher than those from the tributaries in the middle and lower basins.
The thermomagnetic curves of the representative samples generally exhibit similar patterns. The magnetization intensity sharply declined near 585°C and then decreased slightly, indicating a high content of magnetite and small amount of hematite in the samples (Figure 5). The hysteresis loops before and after paramagnetic correction were both narrow, and the closure point of the hysteresis loop after paramagnetic correction occurred at 300 mT, indicating a high abundance of low-coercivity ferromagnetic minerals, such as magnetite (Figure 6). Compared to the hysteresis loops of the other samples, those of the Dongting Lake samples, before and after paramagnetic correction, were distinct, indicating a higher content of paramagnetic minerals in the Dongting Lake sample. Moreover, the hysteresis loop after paramagnetic correction was still not completely closed at 500 mT, implying that the sample also contained certain amounts of high-coercivity magnetic minerals. The magnetization intensity of the samples from the Gan River and Xiang River decreased to a certain extent at 750 mT, indicating a certain amount of diamagnetic minerals in samples.
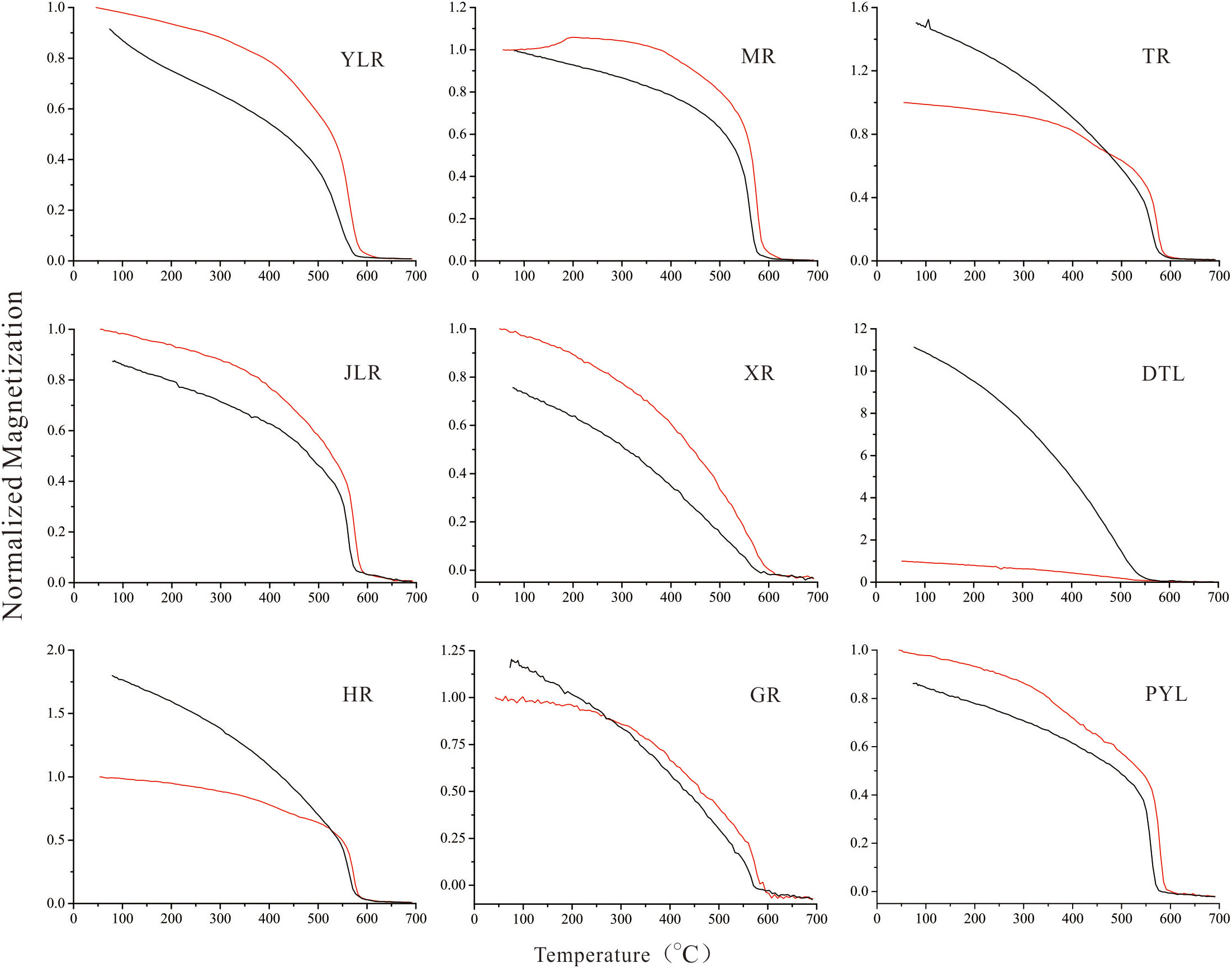
Figure 5 Thermomagnetic curves of the surface sediments from the mainstream of the Yangtze River (red: heating; black: cooling).
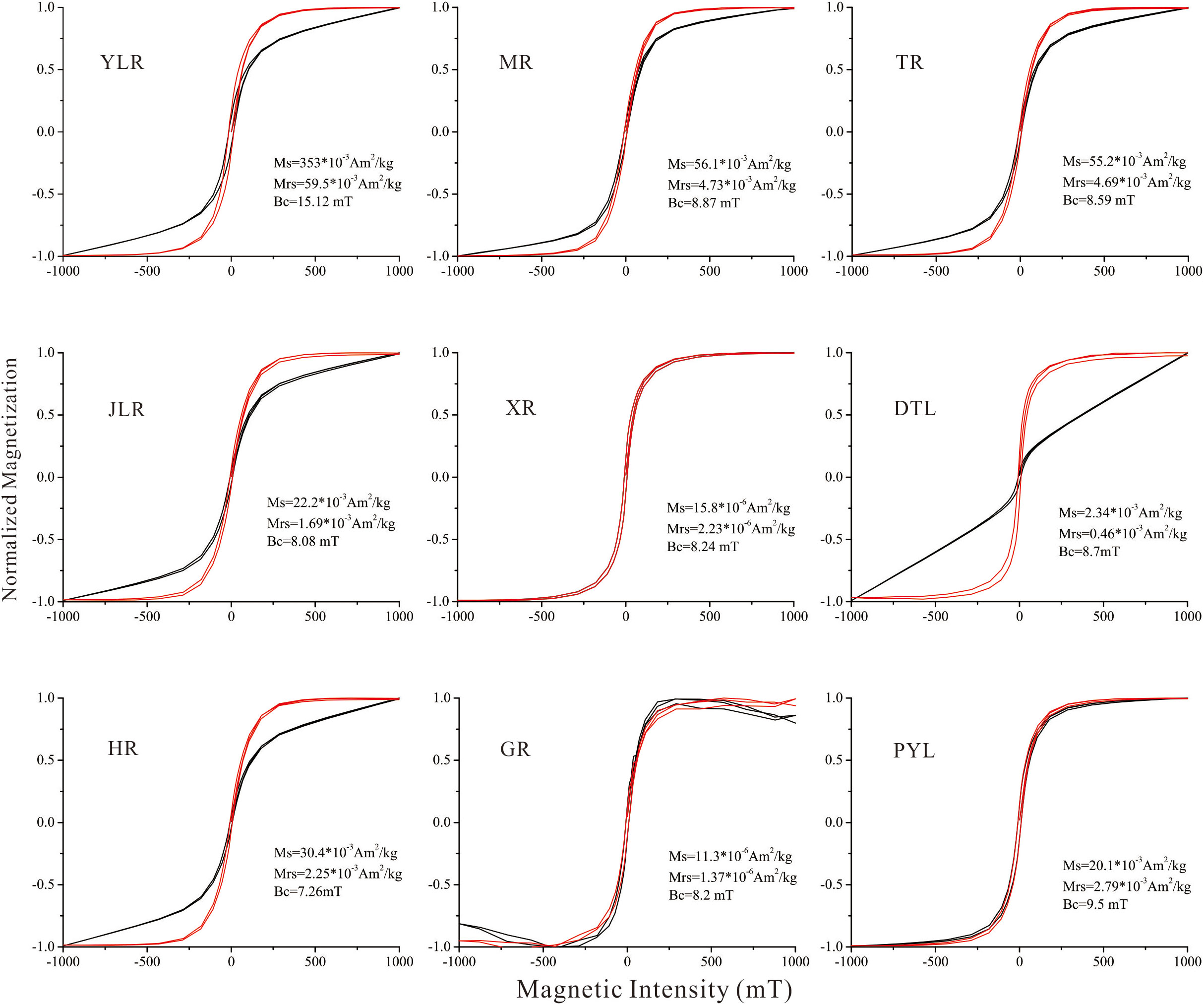
Figure 6 Hysteresis loops of the surface sediments from the mainstream of the Yangtze River (black: before paramagnetic correction; red: after the correction).
4.3. Magnetic properties of the Emeishan basalt
The magnetic parameters of the Emeishan basalt sample from Jianchuan (Figure 2) are as follows. The χlf and SIRM exhibited significantly high values of 1086.73*10-8 m3/kg and 155776.63*10-5 Am2/kg, respectively. The χARM/χlf and χARM/SIRM ratios were 9.59 and 6.69*10-5 A/m, respectively. The S-ratio of the sample was 0.99, showing the predominance of the low-coercivity ferromagnetic minerals in the magnetic particles.
The thermomagnetic curves of the Emeishan basalt sample (Figure 7) showed the following properties. The magnetization intensity remained roughly constant before reaching 585°C and then dropped sharply near 585°C, indicating a high abundance of representative coarse-grained magnetite. The hysteresis loops before and after paramagnetic correction (Figure 7) almost completely overlapped, and the loop of magnetization intensity was completely closed under a magnetic field of 100 mT, implying that low-coercivity magnetic minerals dominate the magnetic particles.
5. Discussion
5.1. Relationship between magnetic properties of the surface sediments and source rocks in the basin
The fluvial clastic deposits may be subjected to hydrodynamic sorting during the transportation process. The statistical results show that the sediment flux into the sea before the closure of the Three Gorges Dam primarily came from the upper Yangtze River basin (Chen et al., 2001). The heavy mineral assemblages and trace element properties of magnetite in the sediments from the estuary were highly consistent with those in the sediments from the upper basin, which means that the hydrodynamic sorting effect on the minerals was overall not significant (Yue et al., 2016).
Significant differences exist in the magnetic properties of various rocks. The magnetic susceptibility (χlf) primarily depends on the content of ferromagnetic minerals (Thompson and Oldfield, 1986). The χlf of basic and ultrabasic rocks is significantly high, typically greater than 1000*10-8 m3/kg, and the average of basalts can reach 1800*10-8 m3/kg. The average χlf of granite is 20*10-8 m3/kg. The χlf of metamorphic rocks is generally low, with an average of 5*10-8 m3/kg for common gneisses and 1*10-8 m3/kg for slates. Sedimentary rocks have the lowest susceptibility overall, generally less than 10*10-8 m3/kg (Thompson and Oldfield, 1986).
In the lower Yalong River basin, the outcrops were dominated by Paleozoic carbonate rocks, low-grade metamorphic rocks, and Emeishan basalts (Changjiang Water Resources Commission, 1999). Among the tributaries in the basin, the mean χlf of the surface sediments from the Yalong River was 276.86*10-8 m3/kg, and significantly higher than that of the samples from other tributaries. The χlf of the Emeishan basalt sample from Jianchuan was 1086.73*10-8 m3/kg, indicating that the widely exposed Emeishan basalts have a significant contribution to the χlf of the surface sediments in the Yalong River.
The χlf of the samples from the tributaries in the middle and lower basin was typically low except Han River. The χlf of the Han River sediments was relatively high, with an average of 80.35*10-8 m3/kg. Quaternary loess deposits are widely distributed in the upper Han River basin. The χlf of the loess on the Loess Plateau deposited during glacial periods was primarily 40−60*10-8 m3/kg, and the paleosols developed during interglacial periods had a susceptibility in the range of 100−200*10-8 m3/kg (Evans and Heller, 2001). The widely distributed Quaternary loess deposits within the upper Han River basin may cause the relatively high χlf of the surface sediments in the Han River.
The χlf of the surface sediments from the major tributaries in the middle and lower Yangtze River basins, such as the Xiang River, Yuan River, and Gan River, is generally low and roughly related to the types of strata exposed in these tributary basins. The surface sediments in the Dongting Lake were mainly from the Xiangjiang River and Yuanjiang River. The strata exposed in the upper Yuan River basin are primarily sandstones, slates, and the Cretaceous red beds. The Cretaceous red beds, along with a few sandy conglomerates, are widely distributed in the middle and lower basin (Changjiang Water Resources Commission, 1999). The strata exposed in the Xiang River basin are predominantly Carboniferous limestones and Cretaceous red beds. The Gan River basin is widely distributed with pre-Sinian and pre-Devonian metamorphic rocks.
The outcrops in the area from the source of Yangtze River to Shigu were mainly carbonate and clastic rocks, scattered with a few intermediate-acid intrusive and metamorphic rocks, whose χlf was generally low, thus leading to the low χlf (34.16*10-8 m3/kg) of the surface sediments at Shigu. The χlf of the surface sediments in the mainstream of the Yangtze River rapidly increased to 230.56*10-8 m3/kg at Yibin and slightly decreased to 210.50*10-8 m3/kg at Yichang. The χlf of the surface sediments from the mainstream at Yibin and Yichang was slightly lower than that of the Yalong River samples but significantly higher than the samples from other tributaries in the upper Yangtze River basin. This means that the Yalong River sediments greatly contribute to the susceptibility value of the sediments in the mainstream. With the addition of the low-susceptibility clastic materials in the middle and lower reaches, the susceptibility of the sediments in the mainstream gradually decreased to 68.92*10-8 m3/kg at the river mouth.
5.2. The source-sink progress of sediment transport
The Yangtze River originates in the northeast region of the Tibetan Plateau, and flows through three major topographic regions of China before injecting into the East China Sea. A series of previous studies have demonstrated that the Yangtze River was formed under drastic macro-geomorphological changes in Asia during the Cenozoic such as the uplift of the Tibetan Plateau, continuous subsidence of eastern China (Clark et al., 2004; Fan et al., 2012; Yue et al., 2019). After a series of geomorphological processes such as river capture and headward erosion, a large river roughly 6300 km long was finally shaped.
Most scholars believe that the Huangling anticline in the Three Gorges area was once the drainage divide of the paleo-Yangtze River system and the paleo-Jinsha River system (Clark et al., 2004; Zheng et al., 2013). The paleo-Jinsha river flowed westward through the Sichuan Basin and eventually into the South China Sea. The paleo-Yangtze River was the prototype of the modern Yangtze River, flowing eastward into the sea. After multiple phases of river capture, the flow direction of the paleo-Jinsha River was reversed, thus forming the modern Yangtze River.
After the incision of the Three Gorges, clastic materials from the upper Yangtze River basin first arrived in the Jianghan Basin. Thick sedimentary layers in the Jianghan Basin are ideal research objects for investigating the tectonic movements in the Three Gorges area and the evolution of the Yangtze River. Magnetic analysis of the sediment from the Zhoulao core in the Jianghan Basin has shown that the χlf of the sediments significantly increases at the depth of 110 m (Figure 8, Zhang et al., 2008). At the horizons with abrupt changes in magnetic properties, the sediment grains also become coarser, indicating sharply increased hydrodynamic forces during the deposition period. These results are related to the addition of the Emeishan basalt fragments and the clastic sediments from the source region of the modern Yangtze River, which may imply a major reconstruction of the Yangtze River system.
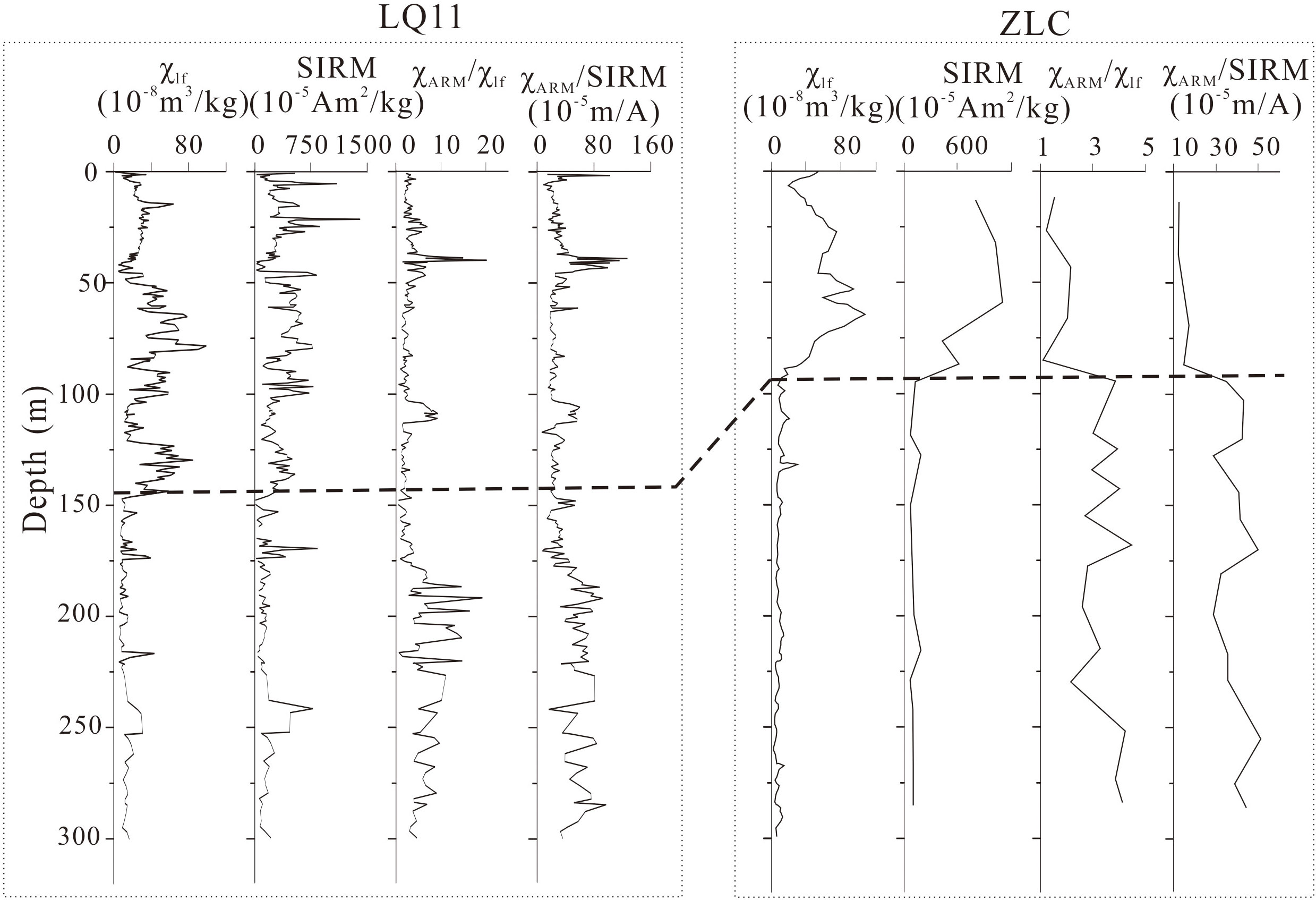
Figure 8 Comparison of magnetic parameters of the sediments from the LQ11 borehole at the Yangtze River delta (Liu et al., 2018) and the Zhoulao borehole in the Jianghan Basin (Zhang et al., 2008).
The sediments in the upper Yangtze River basin have a significantly higher content of ferromagnetic minerals than those in the middle and lower basin (Figure 9). During the source–sink transportation process, the materials from the upper basin are mixed with the low χlf clastic materials from the middle and lower basin, thus the sediments reaching the estuary are a complex of the mixed clastic materials. The content and grain size magnetic materials in the strata at the Yangtze River delta have consistent variation trends with those in the strata in the Jianghan Basin (Figure 8). The content of the ferromagnetic minerals of the core LQ11 significantly increases at the depth of 110 m (1.2 Ma), and the magnetic mineral grains become coarser at the same time (Liu et al., 2018). The high χlf value of the strata at the Yangtze River delta implies that the clastic materials from the upper reaches of the Yangtze River, especially those eroded from the Emeishan basalts, might have reached the estuary, which also means the incision of the Three Gorges and the formation of the modern Yangtze River.
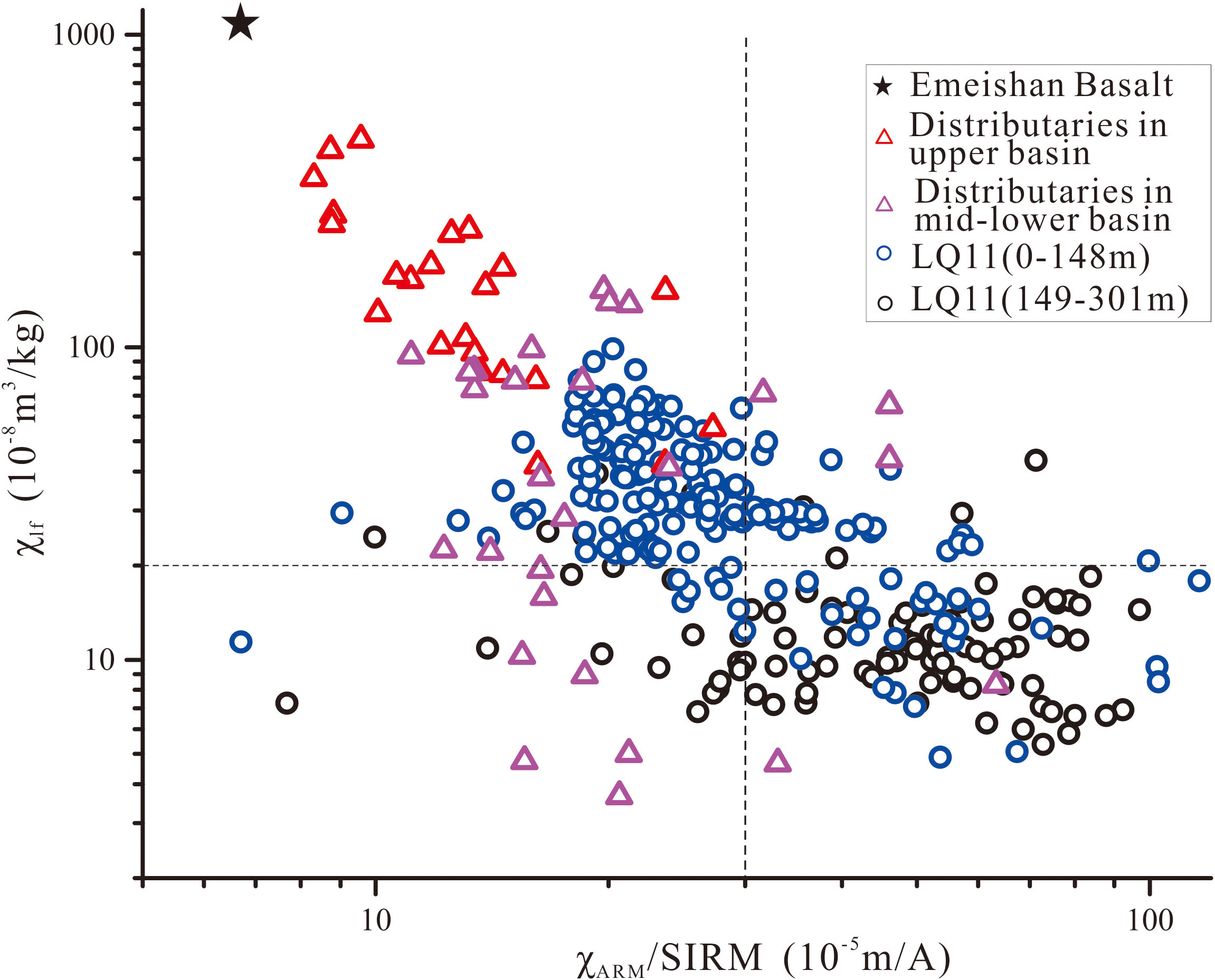
Figure 9 Relationship between the magnetic parameters of the sediments from the LQ11 borehole at the Yangtze River delta and the surface sediments from the Yangtze River basin.
6. Conclusions
Our study systematically analyzed the magnetic properties of surface sediments from the mainstream and major tributaries of the Yangtze River basin, tracing the source–sink process of clastic materials. The surface sediments from tributaries in the upper basin had a significantly higher content of ferromagnetic minerals and coarser grain sizes than that in the mid-lower basin. The χlf of the Yalong River sediments in the upper basin was 276.86*10-8m3/kg, greatly contribute to the χlf of the sediments in the mainstream, reflecting the dominant constraining role of the Emeishan basalts. The distinctive magnetic properties of the upper core sediments from major depocenters including Jianghan basin and the Yangtze delta closely match those of surficial river sediments of the upper Yangtze basin. This may indicate the addition of clastic materials eroded from the upper basin, resulting from the cut-through of the Three Gorges during the evolution of the Yangtze River. Investigating the magnetic properties of the surface sediments in the Yangtze River basin can provide insights into the source–sink process of clastic materials and environmental changes.
Data availability statement
The original contributions presented in the study are included in the article/Supplementary Material, further inquiries can be directed to the corresponding author.
Author contributions
XL, JC, WY, and QW designed the research. LZ and WY wrote the manuscript, which was edited by all of the co-authors. WY did the fieldwork. XL, LZ, and LW analyzed the magnetic data. QW, CZ, JS, and BC refined the interpretations. All authors contributed to the article and approved the submitted version.
Funding
This work was supported financially by the National Natural Science Foundation of China (No. 41901102), the National Science Foundation of China-Shandong United (No. U1706220), and the Natural Science Foundation of Shandong Province (No. ZR2019PD013 and ZR2019MD009).
Conflict of interest
The authors declare that the research was conducted in the absence of any commercial or financial relationships that could be construed as a potential conflict of interest.
Publisher’s note
All claims expressed in this article are solely those of the authors and do not necessarily represent those of their affiliated organizations, or those of the publisher, the editors and the reviewers. Any product that may be evaluated in this article, or claim that may be made by its manufacturer, is not guaranteed or endorsed by the publisher.
References
Changjiang Water Resources Commission (1999). Atlas of changjiang river basin (Beijing: Geological Publishing House), 32–33.
Chappell J., Zheng H. B., Fifield K. (2006). Yangtse river sediments and erosion rates from source to sink traced with cosmogenic 10Be, sediments from major rivers[J]. Palaeogeogr. Palaeoclimatol. Palaeocol. 241, 79–94. doi: 10.1016/j.palaeo.2006.06.010
Chen Z. Y., Li J. F., Shen H. T., Wang Z. H. (2001). Yangtze River of china, historical analysis of discharge variability and sediment flux. Geomorphology 41, 77–91. doi: 10.1016/S0169-555X(01)00106-4
Chu H. M., Zhou L. M., Huang J., Gao B. J., Liu F., Zheng X.M. (2016). Rock magnetic properties of the point bar deposits in the upper mainstream of minjiang river and their origin. Mar. Geol. Quat. Geol. 36, 57–66. doi: 10.16562/i.cnki.0256-1492.2016.04.007
Clark M. K., Schoenbohm L. M., Royden L. H., Whipple K. X., Burchfiel B. C., Zhang X., et al. (2004). Surface uplift, tectonics, and erosion of eastern Tibet from large-scale drainage patterns. Tectonics 23, TC1006. doi: 10.1029/2002TC001402
Dai Z. J., Fagherazzi S., Mei X. F., Chen J. Y., Meng Y. (2016). Linking the infilling of the north branch in the changjiang (Yangtze) estuary to anthropogenic activities from 1958 to 2013. Mar. Geol. 379, 1–12. doi: 10.1016/j.margeo.2016.05.006
Dai Z. J., Liu J. T. (2013). Impacts of large dams on downstream fluvial sedimentation, an example of the three gorges dam (TGD) on the changjiang (Yangtze river). J. Hydrol. 480, 10–18. doi: 10.1016/j.jhydrol.2012.12.003
Dai Z. J., Mei X. F., Stephen. E., Lou Y., Li W. (2018). Fluvial sediment transfer in the changjiang (Yangtze) river-estuary depositional system. J. Hydrol. 566, 719–734. doi: 10.1016/j.jhydrol.2018.09.019
Evans M. E., Heller F. (2001). Magnetism of loess/palaeosol sequences, recent developments. Earth Sci. Rev. 54, 129–144. doi: 10.1016/S0012-8252(01)00044-7
Fan D. D., Wang Y. Y., Wu Y. J. (2012). Advances in provenance studies of changjiang riverine sediments. Adv. Earth Sci. 27, 515–552. doi: 10.11867/j.issn.1001-8166.2012.05.0515
Gu J. W., Chen J., Sun Q. L., Wang Z. H., Wei Z. X., Chen Z. Y. (2014). China's Yangtze delta, geochemical fingerprints reflecting river connection to the sea. Geomorphology 227, 166–173. doi: 10.1016/j.geomorph.2014.05.015
Guo L. C., Su N., Townend I., Wang Z. B., Zhu C. Y., Zhang Y. N., et al. (2019). From the headwater to the delta, a synthesis of the basin-scale sediment load regime in the changjiang river. Earth Sci. Rev. 197, 102900. doi: 10.1016/j.earscirev.2019.102900
He M. Y., Zheng H. B., Huang X. T., Jia J. T., Li L. (2013). Yangtze River sediments from source to sink traced with clay mineralogy. J. Asian Earth Sci. 69, 60–69. doi: 10.1016/j.jseaes.2012.10.001
Jiang C., Chen S. L., Pan S., Fan Y. S., Ji H. Y. (2018). Geomorphic evolution of the yellow river delta, quantification of basin-scale natural and anthropogenic impacts. Catena 163, 361–377. doi: 10.1016/j.catena.2017.12.041
Ji H. Y., Chen S. L., Pan S., Xu C. L., Tian Y. Y., Li P., et al. (2022). Fluvial sediment source to sink transfer at the yellow river delta, quantifications, causes, and environmental impacts. J. Hydrol. 608, 127622. doi: 10.1016/j.jhydrol.2022.127622
Li X., Liu J. P., Saito Y., Nguyen V. L. (2017). Recent evolution of the Mekong delta and the impacts of dams. Earth Sci. Rev. 175, 1–17. doi: 10.1016/j.earscirev.2017.10.008
Liu X. B., Chen J., Maher B. A., Zhao B. C., Yue W., Sun Q. L., et al. (2018). Connection of the proto-Yangtze river to the East China Sea traced by sediment magnetic properties. Geomorphology 303, 162–171. doi: 10.1016/j.geomorph.2017.11.023
Liu Q. S., Roberts A. P., Larrasoaña J. C., Banerjee S. K., Guyodo Y., Tauxe L., et al. (2012). Environmental magnetism, principles and applications. Rev. Geophys. 50, 1–144. doi: 10.1029/2012RG000393
Li C., Yang S., Zhao J. X., Dosseto A., Bi L., Clark T. R. (2016). The time scale of river sediment source-to-sink processes in East Asia. Chem. Geolo. 446, 138–146. doi: 10.1016/j.chemgeo.2016.06.012
Luan H. L., Ding P. X., Wang Z. B., Ge J. Z., Yang S. L. (2016). Decadal morphological evolution of the Yangtze estuary in response to river input changes and estuarine engineering projects. Geomorphology 265, 12–23. doi: 10.1016/j.geomorph.2016.04.022
Luo C., Zheng H. B., Tada R., Wu W. H., Irino T., Yang S. Y., et al. (2014). Tracing sr isotopic composition in space and time across the yangtze river basin - sciencedirect. Chem. Geol. 388, 59–70. doi: 10.1016/j.chemgeo.2014.09.007
Luo Y., Zhang W. G., Liu Y., Liu Y. (2011). Magnetic properties of sediments from the major tributaries of jialing river and their implications for provenance identification. J. Ocean Univ. China 2, 99–107. doi: 10.3969/j.issn.1000-5641.2011.02.013
Luo C., Zheng Y., Zheng H. B., Wang P., He M. Y., Wu W. H. (2013). Magnetic properties of suspended sediment in the Yangtze river and its provenance implications. Quat. Sci. 33, 684–696. doi: 10.3969/j.issn.1001-7410.2013.04.06
Maher B. A. (1986). Characterisation of soils by mineral magnetic measurements, phys. Earth Planet. Inter. 42, 76–92. doi: 10.1016/S0031-9201(86)80010-3
Mei X. F., Dai Z. J., Stephen E., Zhang M., Cai H. Y., Wang J., et al. (2021). Landward shifts of the maximum accretion zone in the tidal reach of the changjiang estuary following construction of the three gorges dam. J. Hydrol., 592. doi: 10.1016/j.jhydrol.2020.125789
Milliman J. D., Farnsworth K. L. (2011). River discharge to the coastal ocean – a global synthesis (Cambridge: Cambridge University Press).
Milliman J. D., Meade R. H. (1983). World-wide delivery of river sediment to the oceans. J. Geol. 91, 1–21. doi: 10.1086/628741
Nel H. A., Dalu T., Wasserman R. J. (2018). Sinks and sources, assessing microplastic abundance in river sediment and deposit feeders in an austral temperate urban river system. Sci. Total Environ. 612, 950–956. doi: 10.1016/j.scitotenv.2017.08.298
Niu J. L., Yang Z. S., Li Y. H., Qiao. S. Q. (2008). The characteristics of the environmental magnetism in sediment from the river mouth of the changjiang river and the huanghe river and their comparison study. Mar. Sci. 32, 23–30. doi: 10.5194/npg-8-1-2001
Pan D. D., Wang Z. H., Chen T., Gao X. Q., Li X., Zhan Q. (2015). Mineral magnetic characteristics of surficial sediments and their implications for identifying sedimentary environments at the changjiang river mouth. Acta Oceanol. Sinic. 37, 101–111. doi: 10.3969/j.issn.0253-4193.2015.05.010
Park E., Loc H. H., Lim J., Herrin J., Chitwatkulsiri D. (2021). Source-to-sink sediment fluxes and budget in the chao phraya river, Thailand, a multi-scale analysis based on the national dataset. J. Hydrol. 594, 1–14. doi: 10.1016/j.jhydrol.2020.125643
Syvitski J., Peckham. S. D., Hilberman. R., Mulder T. (2003). Predicting the terrestrial flux of sediment to the global ocean, a planetary perspective. Sediment. Geol. 162, 5–24. doi: 10.1016/S0037-0738(03)00232-X
Wang H. J., Saito Y., Bi N. S., Sun X., Yang Z. S. (2011). Recent changes of sediment flux to the western pacific ocean from major rivers in East and southeast Asia. Earth Sci. Rev. 108, 80–100. doi: 10.1016/j.earscirev.2011.06.003
Wittmann H., Malusà M. G., Resentini A., Garzanti E., Niedermann S. (2016). The cosmogenic record of mountain erosion transmitted across a foreland basin, source-to-sink analysis of in situ 10Be, 26Al and 21Ne in sediment of the po river catchment[. Earth. Planet. Sci. Lett., 452, 258–271. doi: 10.1016/j.epsl.2016.07.017
Yang S. Y., Jiang S. Y., Ling H. F., Xia X. P., Sun M., Wang D. J. (2007). Sr-Nd Isotopic compositions of the changjiang sediments, implications for tracing sediment sources. Sci. China 50, 1556–1565. doi: 10.1007/s11430-007-0052-6
Yang S. Y., Li C. X., Yokoyama K. (2006). Elemental compositions and monazite age patterns of core sediments in the changjiang delta, implications for sediment provenance and development history of the changjiang river. Earth. Planet. Sci. Lett. 245, 762–776. doi: 10.1016/j.epsl.2006.03.042
Yang S. L., Milliman J. D., Xu K. H., Deng B., Zhang X. Y., Luo X. X. (2014). Downstream sedimentary and geomorphic impacts of the three gorges dam on the Yangtze river. Earth Sci. Rev. 138, 469–486. doi: 10.1016/j.earscirev.2014.07.006
Yang C. F., Vigier N., Yang S. Y., Revel M., Bi. L. (2021). Clay Li and Nd isotopes response to hydroclimate changes in the changjiang (Yangtze) basin over the past 14,000 years. Earth. Planet. Sci. Lett. 561, 116793. doi: 10.1016/j.epsl.2021.116793
Yang S. L., Xu K. H., Milliman J. D., Yang H. F., Wu C. S. (2015). Decline of Yangtze river water and sediment discharge, impact from natural and anthropogenic changes. Sci. Rep. 5, 1–14. doi: 10.1038/srep12581
Yang H. F., Yang S. L., Meng Y., Xu K. H., Luo.X.X. Wu C. S., et al. (2018). Recent coarsening of sediments on the southern Yangtze subaqueous delta front, a response to river damming. Continent. Shelf Res. 155, 45–51. doi: 10.1016/j.csr.2018.01.012
Yang H. F., Yang S. L., Xu K. H., Milliman J. D., Wang H., Yang Z., et al. (2018). Human impacts on sediment in the Yangtze river, a review and new perspectives. Glob. Planet. Change 162, 8–17. doi: 10.1016/j.gloplacha.2018.01.001
Yue W., Liu J. T., Zhang D., Wang Z. H., Zhao B. C., Chen Z. Y., et al. (2016). Magnetite with anomalously high Cr2O3 as a fingerprint to trace upper Yangtze sediments to the sea. Geomorphology 268, 4–20. doi: 10.1016/j.geomorph.2016.05.032
Yue W., Yang S. Y., Zhao B. C., Chen Z. Y., Chen J. (2019). Changes in environment and provenance within the changjiang (Yangtze river) delta during pliocene to pleistocene transition. Mar. Geol. 416, 105976. doi: 10.1016/j.margeo.2019.105976
Zhang Y.F., Li C.A., Wang Q.L., Chen L., Ma Y.F., Kang C.G. (2008). Magnetism parameters characteristics of drilling deposits in Jianghan Plain and indication for forming of the Yangtze River Three Gorges. China. Sci. Bull. 53, 584–590. doi: 10.1007/s11434-008-0111-1
Zhang J., Wan S., Clift P. D., Huang J., Zhang X. (2019). History of yellow river and yangtze river delivering sediment to the yellow sea since 3.5ma, tectonic or climate forcing? Quat.Sci. Rev. 216, 74–88. doi: 10.1016/j.quascirev.2019.06.002
Zhang W. G., Yu L. Z., Lu M. (2002). Relationship between magnetic properties and grain sizes in intertidal sediments of the Yangtze estuary, China. Sci. China 32, 783–792. doi: 10.3321/j.issn,1006-9267.2002.09.010
Zheng H. B., Clift P. D., Wang P., Tada R., Jia J. T., He M. Y., et al. (2013). Pre-Miocene birth of the Yangtze river. Proc. Natl. Acad. Sci. U.S.A. 110, 7556–7561. doi: 10.1073/pnas.1216241110
Zheng Y., Yang S., Deng C. (2019). Provenance and climate changes inferred from magnetic properties of the sediments from the lower yangtze river (china) during the last 130 years. J. Asian Earth Sci. 175, 128–137. doi: 10.1016/j.jseaes.2019.01.036
Keywords: the Yangtze River, magnetic properties, source-sink process, the surface sediments, Emeishan basalts
Citation: Liu X, Chen J, Yue W, Wang Q, Zhan C, Zeng L, Song J, Wang L and Cui B (2022) Tracing the source–sink process of fluvio-clastic materials: Magnetic records of surface sediments in the Yangtze River basin. Front. Mar. Sci. 9:1002335. doi: 10.3389/fmars.2022.1002335
Received: 25 July 2022; Accepted: 06 December 2022;
Published: 19 December 2022.
Edited by:
Guan-hong Lee, Inha University, South KoreaReviewed by:
Abd Mujahid Hamdan, Ar-Raniry State Islamic University Banda Aceh, IndonesiaJinhai Zheng, Hohai University, China
Copyright © 2022 Liu, Chen, Yue, Wang, Zhan, Zeng, Song, Wang and Cui. This is an open-access article distributed under the terms of the Creative Commons Attribution License (CC BY). The use, distribution or reproduction in other forums is permitted, provided the original author(s) and the copyright owner(s) are credited and that the original publication in this journal is cited, in accordance with accepted academic practice. No use, distribution or reproduction is permitted which does not comply with these terms.
*Correspondence: Xianbin Liu, bGl1eGJfODAxQDE2My5jb20=