- Laboratory of Biological Oceanography, Graduate School of Agricultural Science, Tohoku University, Sendai, Japan
The population dynamics of the capitellid polychaete Capitella aff. teleta were studied in Gamo Lagoon, located in northeast Japan, for the subsequent 2 years from 2016, when a series of restoration works was conducted following the 2011 Great East Japan Earthquake and tsunami. Capitella aff. teleta was found to be widely distributed from the estuary side, where the levee was located, to the innermost part but was more abundant in the innermost part, which is rich in organic matter. In the lagoon, the daily maximum water level dropped from 2017 to 2018 during the reconstruction of a flow-conducting levee, which blocked water flow and isolated the inner part of the lagoon. Although the density decreased drastically for approximately 11 months under diurnal hypoxia and strongly reducing conditions, small-sized new recruits were observed and the population recovered quickly after the daily maximum water level increased. In Gamo Lagoon, C. aff. teleta inhabiting the innermost part and estuary side of the lagoon contributed to maintaining the population by dispersing planktonic larvae between them. Thus, the maximum water level had a significant effect on the maintenance of the C. aff. teleta population in the lagoon, and sufficiently high water levels enable the dispersion of planktonic larvae to help recover the population quickly, suggesting that it is important to keep the water area connected.
Introduction
The annelid species belonging to the genus Capitella (family Capitellidae) are opportunistic species in marine eutrophic sediments and are widely distributed globally (Rouse and Pleijel, 2001). Capitella aff. teleta found only in Gamo Lagoon (Tomioka et al., 2016) predominantly appeared in Gamo Lagoon immediately after the tsunami that followed the 2011 Great East Japan Earthquake and tsunami (Kondoh, 2017; Kondoh et al., 2020).
Of the 17 Capitella species known (Warren, 1991; Blake et al., 2009; Magalhães and Bailey-Brock, 2012; Silva et al., 2016), Capitella capitata (Fabricius, 1780) is best known as an opportunistic (Kanaya and Kikuchi, 2011) species that is predominant in eutrophic sediments and widely distributed worldwide (Blake, 2009). However, studies on the anatomy, growth, and physiology of C. capitata (Grassle and Grassle, 1976; Eckelbarger and Grassle, 1983; Chareonpanich et al., 1993; Gamenick et al., 1996; Gamenick et al., 1998; Linke-Gamenick et al., 2000; Méndez, 2002; Méndez, 2006) have revealed that C. capitata comprises at least 10 different species (Blake et al., 2009). One of these species, Capitella sp. I, which was thought to be distributed in North America and Japan, was determined and described as C. teleta (Blake et al., 2009).
In Japan, five species or subspecies of the genus Capitella have been described: C. capitata, C. capitata japonica (Kitamori, 1960), Capitella jonesi (Hartman, 1959), Capitella minima (Langerhans, 1880), and C. teleta (Kitamori, 1960; Imajima and Hartman, 1964; Imajima, 2015; Kanaya et al., 2015). Before C. teleta was described in 2009, most Capitella species in Japan were considered C. capitata (Kitamori, 1960; Imajima and Hartman, 1964; Honma et al., 1974; Ueno and Yamamoto, 1982; Tsutsumi and Kikuchi, 1984; Tsutsumi, 1987; Tsutsumi et al., 1991). However, Tsutsumi and Montani (1993) reported that the species from Tomoe Bay, Amakusa, and Kyushu are identical to Capitella sp. I (Grassle and Grassle, 1976) from Massachusetts, USA, in terms of the karyotype, mating ability, and life history. Subsequent ecological studies after 2009 have revealed that this species is identical to Capitella sp. I (Tsutsumi, 2005; Tsutsumi et al., 2005; Kinoshita et al., 2008) or C. teleta (Nishi et al., 2010; Kanaya, 2014; Kanaya et al., 2015).
Gamo Lagoon is a eutrophic lagoon located at the mouth of the Nanakita River in Miyagi Prefecture, northeastern Japan. Gamo Lagoon was catastrophically damaged by a megathrust earthquake—the 2011 Great East Japan Earthquake—and huge tsunamis that occurred in March 2011 (Ide et al., 2011; Ozawa et al., 2011), which have caused drastic topographic changes (Kondoh et al., 2020). Just after the disturbance, a sand dune completely disappeared but was rapidly reformed, the river mouth was completely closed, a seasonal typhoon-induced flood hit the lagoon, and an intense deluge occurred in 2015. The second tsunami followed by the Fukushima Earthquake hit this estuary again in 2016; therefore, the balance of sand sedimentation dynamics remained unstable (Kondoh et al., 2020). Restoration and reconstruction work are underway, although many years have passed since the 2011 earthquake and tsunami.
Many coastal benthic species have a planktonic larval stage in their early life history. During this period, the larvae are passively transported and may settle and grow far from their original habitats. Thus, having a pelagic period leads to habitat expansion. The extinction of local populations caused by small-scale disturbances can be recovered by transferring from neighboring local populations. In other words, the dispersal of planktonic larvae leads to not only the expansion of the habitat of the species but also its sustainable survival in the wider region. Usually, geographically distant local communities interact in this complex way through the dispersal of organisms. Such regional aggregations of local communities are called metacommunities (Wilson, 1992). The local populations of each benthic species with planktonic larvae maintain and establish their populations through larval dispersal from other water sites.
The life history and population dynamics of C. teleta were studied on the coast of Kyushu in Japan (Tsutsumi and Kikuchi, 1984; Tsutsumi, 1987; Tsutsumi, 1990; Tsutsumi, 2005). Genetic analysis revealed that the species in Gamo Lagoon is a sister species of C. teleta and was reported as C. aff. teleta by Tomioka et al. (2016). This species has only been found in Gamo Lagoon, predominantly after the 2011 earthquake. The levee that was constructed at the border between Gamo Lagoon and the Nanakita River was destroyed by tsunamis but is being reconstructed since 2017. These events have caused major topographic changes within Gamo Lagoon and allowed us to analyze the change in the population dynamics of C. aff. teleta.
In recent years, changes in the environment have become evident in the form of torrential rains and frequent typhoons. Natural disturbances, such as earthquakes and tsunamis, followed by restoration work are common. Coastal areas have been directly affected by these natural and anthropogenic disturbances, resulting in significant changes in the topography and the communities that inhabit them. In this study, to clarify the population maintenance mechanism of C. aff. teleta, which first appeared immediately after the disturbance and is one of the key species in understanding the environmental changes and community dynamics in Gamo Lagoon, we examined the environmental changes in the lagoon and the interdependence between individuals at multiple stations in different environments in the lagoon, as well as the factors affecting population dynamics at different stations.
Materials and methods
Study area
Gamo Lagoon, located at the mouth of the Nanakita River in Miyagi Prefecture, northeastern Japan, is a eutrophic shallow tidal flat with a water surface area of 13 ha: 860 m long and 250 m wide, with a mean water depth of 0.8 m (Kondoh, 2017: data before March 2011). The lagoon was separated from the estuary by a stone levee with three water gates, a flow-conducting levee, and, from Sendai Bay, by a 150 m wide dune before March 2011 (Kanaya et al., 2014). The bottom sediment of the riverside and the inner part of the lagoon are sandy and muddy, respectively, and high reduction conditions were observed in the innermost part of the lagoon. Gamo Lagoon was designated as the Gamo Special Protection Area in the nationally designated Sendai Coastal Wildlife Protection Area in 1973. Many conservation efforts have been made to protect the biological communities and brackish water environment.
The 2011 Great East Japan Earthquake, with a magnitude of 9.0, occurred in March 2011 followed by 7.2-m-high tsunamis (data from the Japan Meteorological Agency) at the nearby Sendai Port and 3–4.5 m tsunamis (data from the Sendai Science Museum) in the Gamo area. In addition to the initial disturbance, heavy rainfall occurred in the Kanto and Tohoku regions in September 2015 (Tanaka et al., 2016), and another tsunami was generated following the Fukushima Earthquake in November 2016. Thus, topographic changes have occurred continuously at Gamo Lagoon: the topography around the mouth of the Nanakita River was significantly altered by the heavy rain, and sand deposition was observed in the middle part of Gamo Lagoon by the second tsunami. In the area around the estuary, including Gamo Lagoon, a series of restoration works began in 2016. The reconstructions of the levee at the entrance to Gamo Lagoon and the embankment on the landward side have been ongoing since November and February 2017, respectively. An artificial deep channel was excavated in the middle part of the lagoon by Miyagi Prefecture in August 2018.
Environmental data
The survey was conducted at Gamo Lagoon during low tide once a month from September 2016 to October 2018. The survey was included just after the tsunami on 28 November 2016. Three measuring points (Stns A, C, and D; Figure l) were set up in Gamo Lagoon from the estuary side to the innermost part of the lagoon to cover the entire tidal flat area. At Stn C, the water level became so low that the sediments dried out during low tide after April 2018; therefore, Stn C′ was set up nearby where the water level was higher.
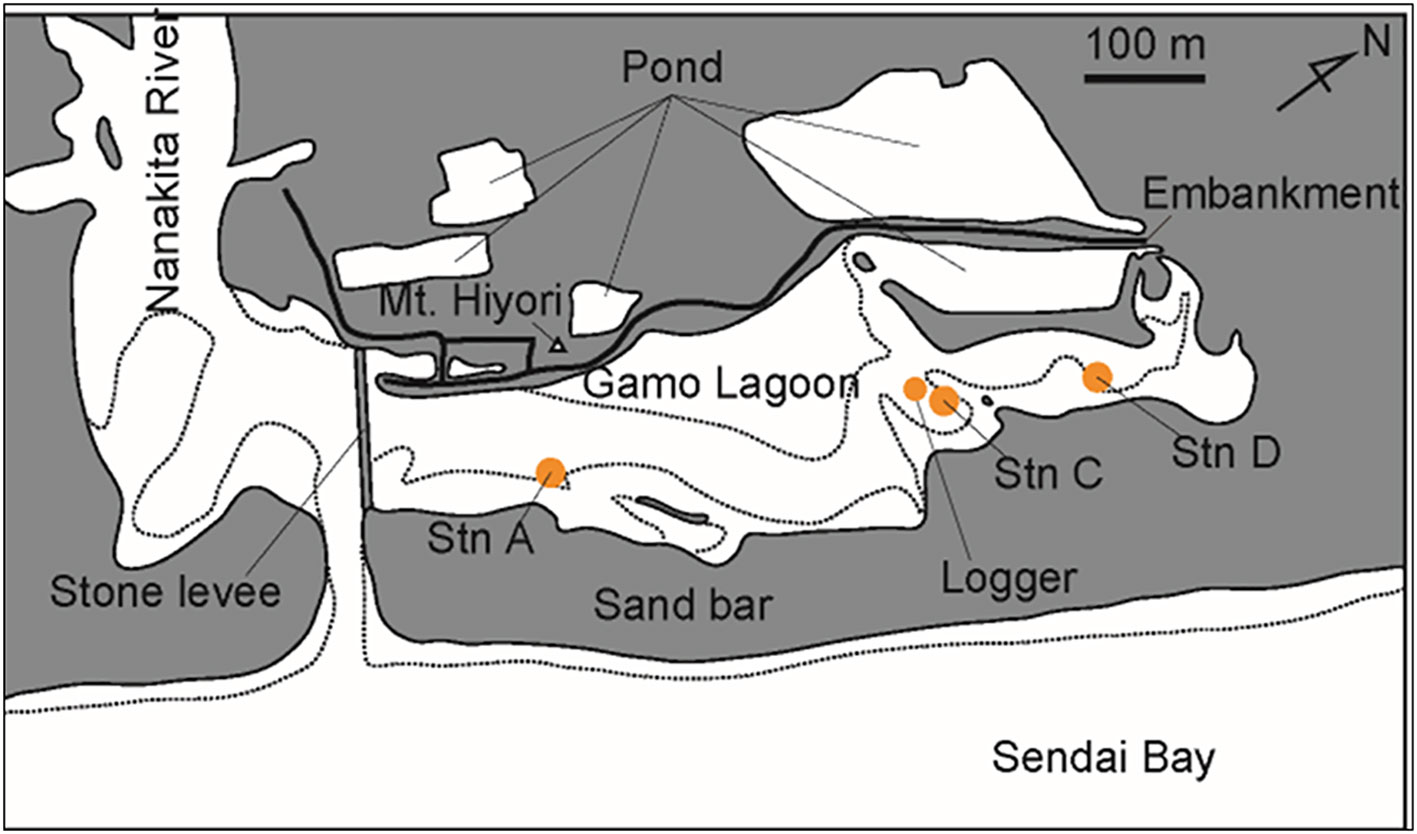
Figure 1 Gamo Lagoon. The bold line shows the location of Gamo Lagoon. Dashed lines show shorelines at low tide.
Environmental measurements were taken each month at each station for water temperature, mud temperature, salinity, the dissolved oxygen (DO) concentration, and the redox potential from the bottom layer. Water temperature and DO concentration were measured using a DO meter (HACH, HQ40d). Salinity was measured using a salinity meter (DKK-TOA, CM-21P). The oxidation–reduction potential and mud temperature were measured using an ORP meter (DKK-TOA, RM-30P). A data logger (YSI, 6000MS) was also installed near Stn C to measure water temperature, salinity, DO, and depth once every 30 min.
Changes in the density and size frequency distributions
Samples were collected from September 2016 to October 2018, once for each month and at each station using the Ekman–Birge grab sampler [240 mm (W) × 210 mm (D) × 350 mm (H)]. An extra sample was added just after the tsunami on 28 November 2016. Mud samples were sieved through a 500 µm mesh, and all organisms remaining on the sieve were fixed and preserved with 10% neutralized formalin.
The individuals of C. aff. teleta were sorted from bottom mud samples and counted under a stereomicroscope (OLYMPUS SZX12). Thoracic maximal body segment width was used as an index of body size (Tsutsumi and Kikuchi, 1984) and measured under a stereomicroscope (OLYMPUS MZX12). Individuals with reproductive bristles on chaetigers 8 and 9 were identified as males and those with eggs in the ventral side of the abdominal chaetigers as females. Individuals lacking the characteristics of males and females were identified as immature individuals.
Results
Environmental observations at the time of the survey
Changes in water and mud temperatures at the time of the survey are shown in Figures 2A, B. The differences between water and mud temperatures were small, with a minimum water temperature of 2.4°C at Stn C in March 2018 and a maximum water temperature of 31.1°C at Stn C′ in August 2018, showing a seasonal variation with the lowest water temperature in December–March and the highest water temperature in July–August. The differences between the stations were small.
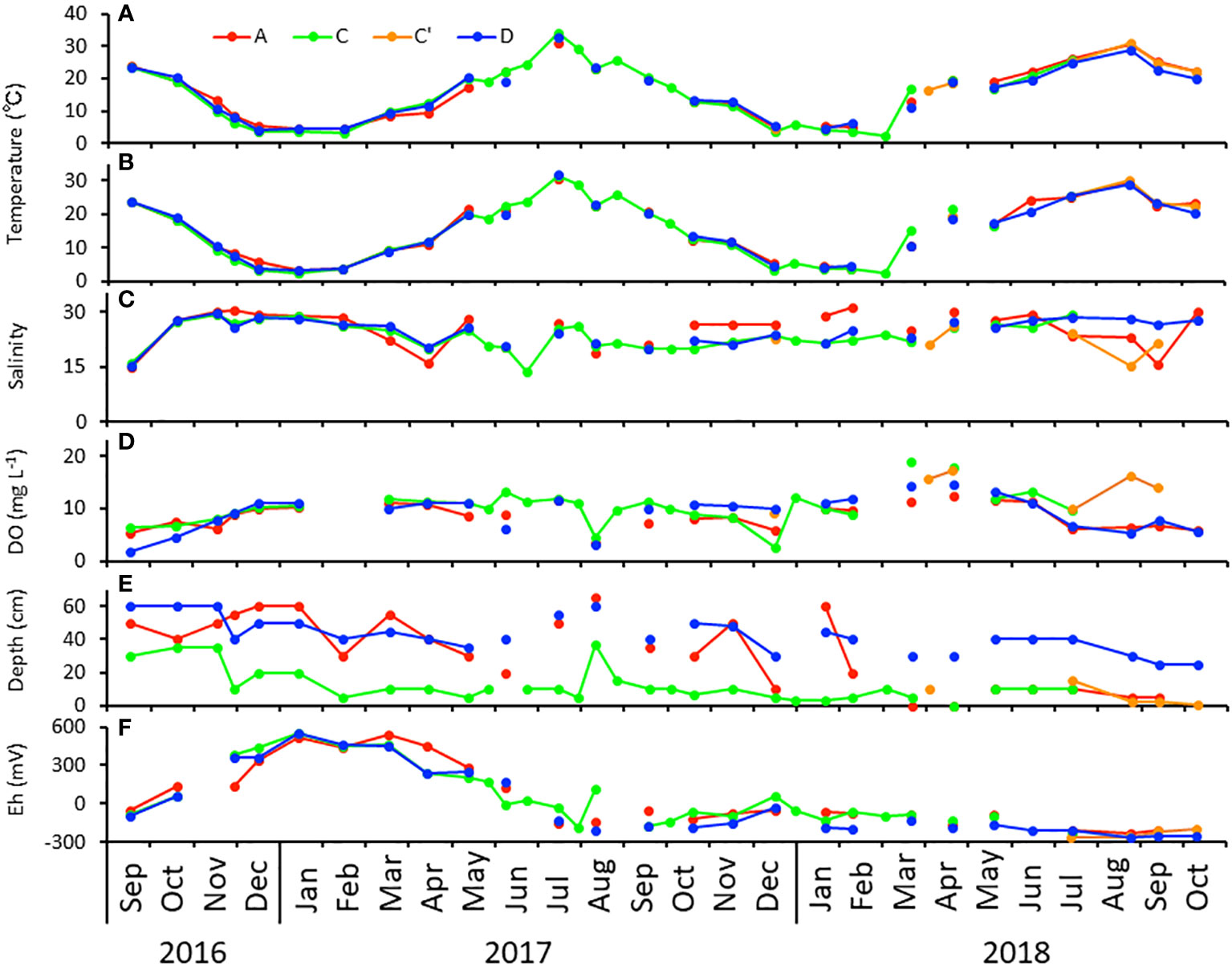
Figure 2 Seasonal changes in (A) water temperature, (B) sediment temperature, (C) salinity, (D) dissolved oxygen (DO) concentration, (E) water depth, and (F) the redox potential at Stns A, C (and C’), and D in Gamo Lagoon from September 2016 to October 2018. For missing data, no line connects before and after the missing data.
Changes in salinity, which varied between 13.9 and 31.2, at the time of the survey are shown in Figure 2C. Changes in the DO concentration during the survey are shown in Figure 2D. The lowest value during the study was 2.66 mg/L at Stn C in December 2017, and the highest value was 19.08 mg/L at Stn C in March 2018, with a weak tendency to drop in summer under 5 mg/L. In addition, the fluctuations were drastic from June 2017 both between and within stations.
Changes in the water level at the time of the survey are shown in Figure 2E. The water levels at Stns C and C′ were lower than those at Stns A and D throughout the study.
Changes in the redox potential at the time of the survey are shown in Figure 2F. The redox potential increased from September 2016 to January 2017, with a maximum value of 555 mV at Stn C in January 2017, and then remained high until March before decreasing again. The redox potential had negative values in many months after June 2017, and these low levels continued. The differences between stations were relatively small, but Stn D tended to show the lowest values throughout.
Environmental observations by data logger
Figure 3 shows the results of observations made by a data logger installed near Stn C. Water temperatures varied seasonally from a minimum of −1.7°C to a maximum of 37.9°C. Compared to 2016–2017, 2017–2018 had longer periods below 0°C from December to March and higher maximum water temperatures from June to September. Salinity varied more between August and October, from approximately 10 to 30. DO concentrations during high water temperature in summer were hypoxic (see Diaz and Rosenberg, 1995), with the DO levels of 0–2.8 mg/L at night and supersaturation during the day, showing diurnal hypoxia. In addition, frequent diurnal hypoxia occurred from November 2017 to March 2018 during low-water-temperature periods. Depths showed a decrease in minimum water levels from February to March 2017, from December 2017 to June 2018, and since August 2018. Moreover, maximum water levels decreased from February to March 2017 and from December 2017 to June 2018. Daily water levels fluctuated highly from August 2018 after the channel was excavated in the middle of the lagoon.
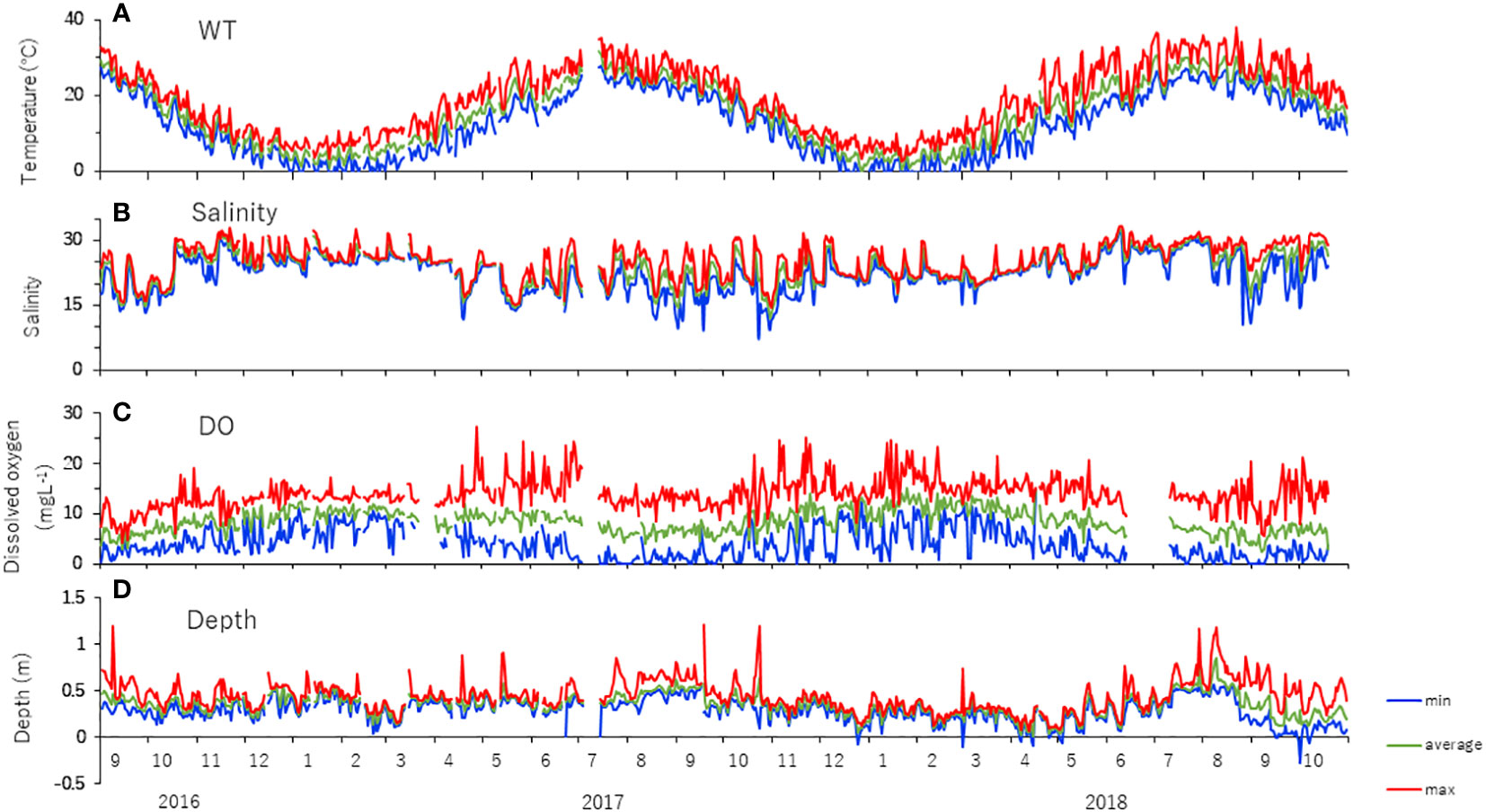
Figure 3 Seasonal changes in the daily minimum, average, and maximum (A) water temperature, (B) salinity, (C) DO, and (D) water depth recorded by the data logger at Stn C in Gamo Lagoon from September 2016 to October 2018.
Population dynamics of C. aff. teleta in Gamo Lagoon
Density
The population densities of C. aff. teleta at the three stations are shown in Figure 4. Although each station showed a large fluctuation in abundance, the two stations at the middle and innermost parts of the lagoon (Stns C, C’, and D) showed rather similar changes in density, whereas Stn A at the estuary side showed a different change.
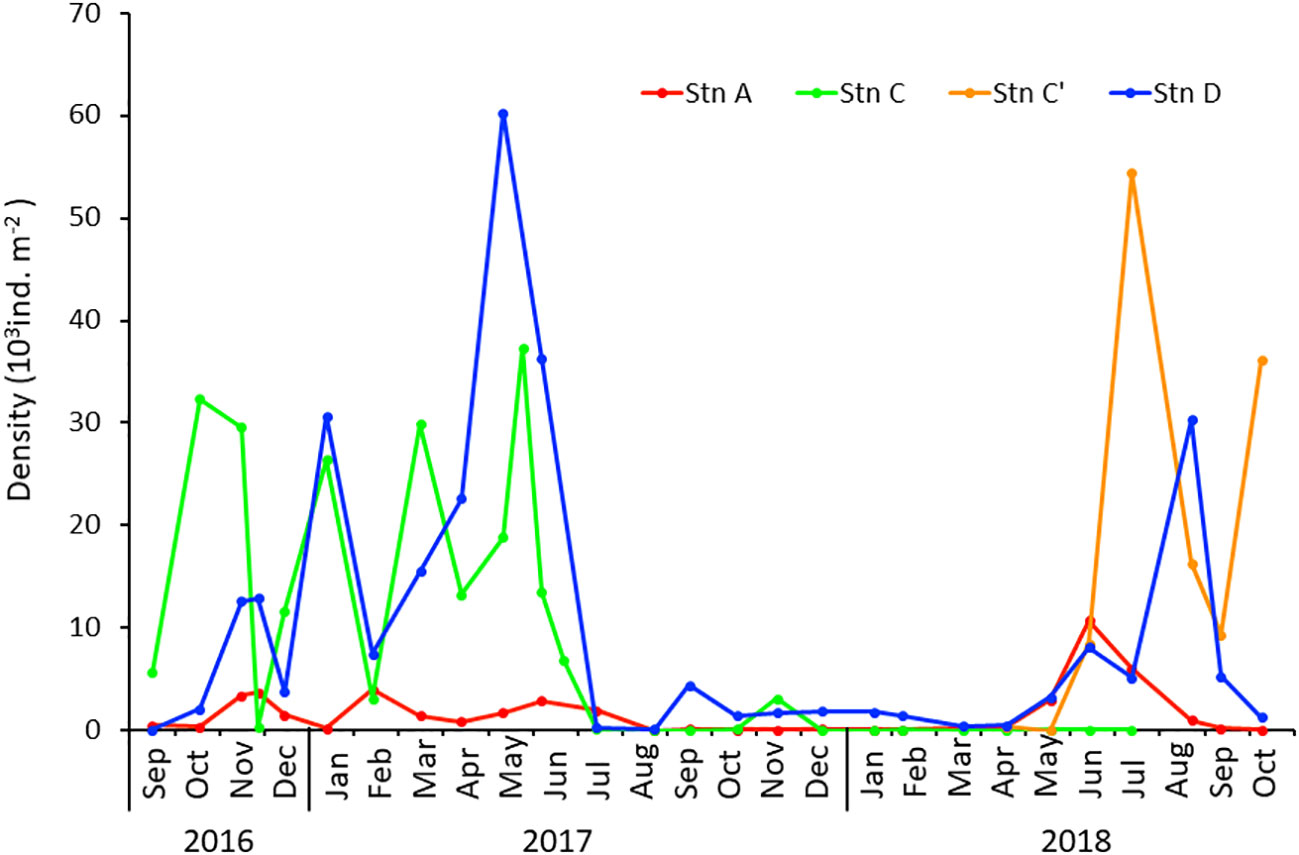
Figure 4 Seasonal changes in the density of Capitella aff. teleta at Stns A, C (and C′), and D in Gamo Lagoon from September 2016 to October 2018.
Capitella aff. teleta showed unstable density at Stn A, which varied from approximately 0–4,000 ind/m2 from September 2016 to August 2017. During this period, a significant decline in the density was seen from December 2016 to January 2017 and March–May 2017. In addition, density did not increase from August 2017 to April 2018, continuing at almost zero level. However, after that period, density suddenly increased from May and reached 10,000 ind/m2 in June but again began to decrease from July and dropped to almost zero in October.
At Stn C, density was higher than that at Stn A (Figure 4). It apparently plummeted just after the 2016 Fukushima Earthquake and tsunami in November. Thereafter, density fluctuated largely as it continued to increase in December 2016 and January 2017, decreased in February 2017, increased again in March, and decreased in April to one-half of the previous month’s level. Thus, density was not stable after the tsunami from November 2016 to May 2017, with significant changes occurring every few months. Moreover, C. aff. teleta disappeared for approximately a year after July 2017. At nearby Stn C’, an alternative station to dry up Stn C, density increased after June 2018 and reached up to >50,000 ind/m2, decreased drastically in August and September, and increased again in October 2018.
The density at Stn D had the highest value among the three stations. Large fluctuations were observed from almost 0 (September 2016 and July 2017) to 60,000 ind/m2 (May 2017) during September 2016–July 2017 (Figure 4). Density showed a rapid decrease in December 2016 and February 2017. The highest density of 60,000 ind/m2 was recorded in May 2017. Density did not increase from July 2017 to April 2018. After May 2018, density showed an increasing trend and reached a maximum at approximately 30,000 ind/m2 in August 2018.
Size frequency distribution
The size frequency distributions of the maximum width of the thoracic segment at Stn A are shown in Figure 5. At Stn A, smaller individuals with body width < 0.5 mm appeared each month, except December 2016, August and November 2017, and February and March 2018. Body width increased gradually from September to December in both 2016 and 2017 and decreased gradually from April to August in both 2017 and 2018. Larger individuals with body width >1 mm appeared from November 2016 to April 2017, except January 2017. Mature individuals appeared from November 2016 to April 2017, except January, and April–June 2018. Large mature individuals with body width approximately >1 mm appeared in November and December 2016, February–April 2017, and April and May 2018. Smaller mature individuals with body width <0.5 mm appeared in November 2016 and April and June 2018. Both the minimum and maximum body widths of mature individuals increased from November 2016 to February 2017 and decreased again from February. The presence of females and males revealed that no females appeared since April 2017.
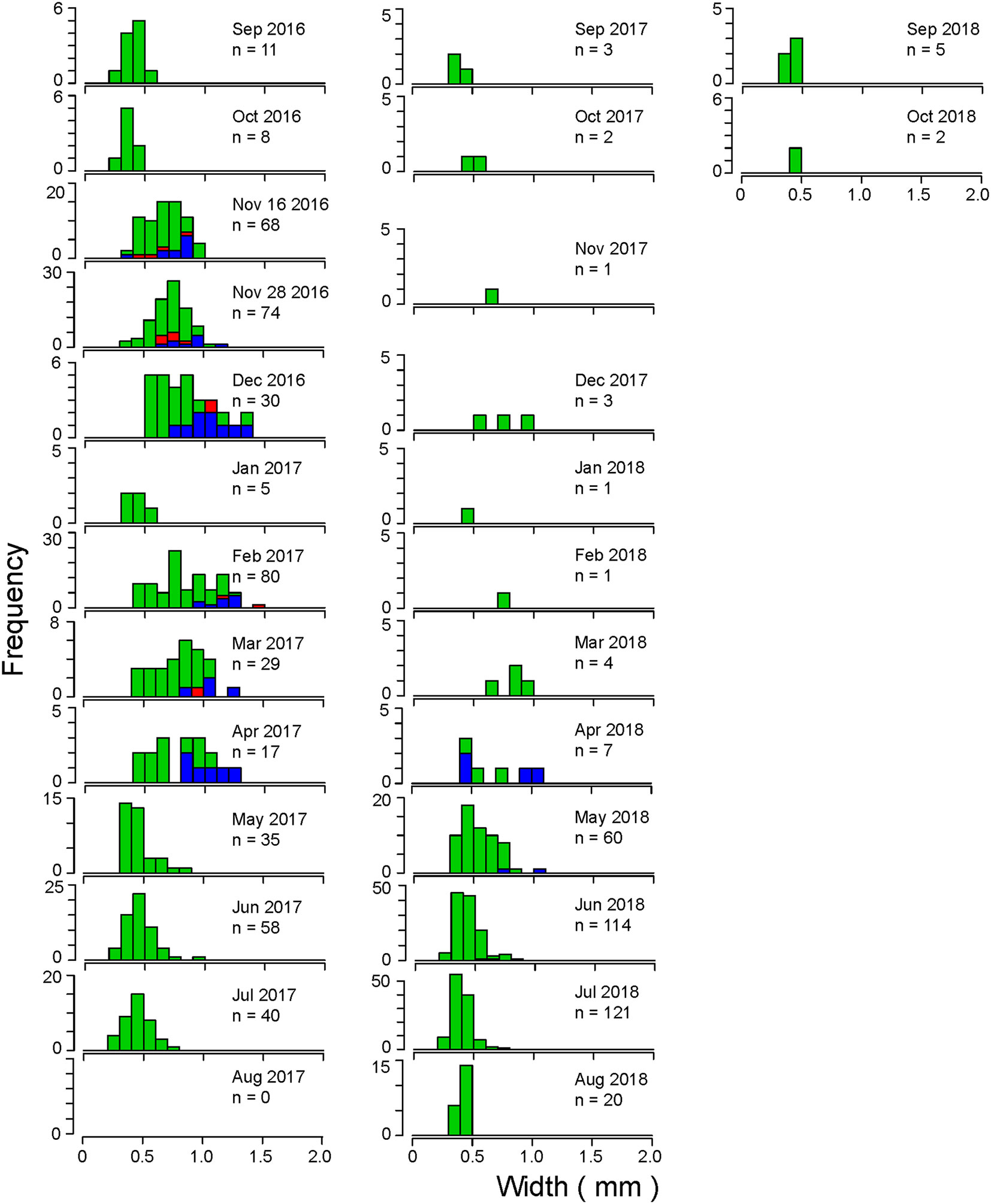
Figure 5 Size frequency distributions of the maximum width of the thoracic segment of C. aff. teleta at Stn A in Gamo Lagoon from September 2016 to October 2018. Red: females, blue: males, green: immature individuals.
A similar trend in change in body width was observed in Stn C, showing a gradual increase from September to December 2016 and a gradual decrease from April to June 2017 (Figure 6). In January 2017, many individuals with body width >1 mm were observed in Stn C, although very few were observed at Stn A. Small individuals (<0.5 mm) appeared, except from July to October and December 2017 and January, February, and May 2018. In November and December 2016; January, March–May, and November 2017; and April 2018, larger mature individuals (≥1 mm body width) appeared. In November 2016 and from June 2018, smaller mature individuals (<0.5 mm body width) were observed. The occurrence of males and females at both Stns C and C’ showed that males tended to occur more frequently than females.
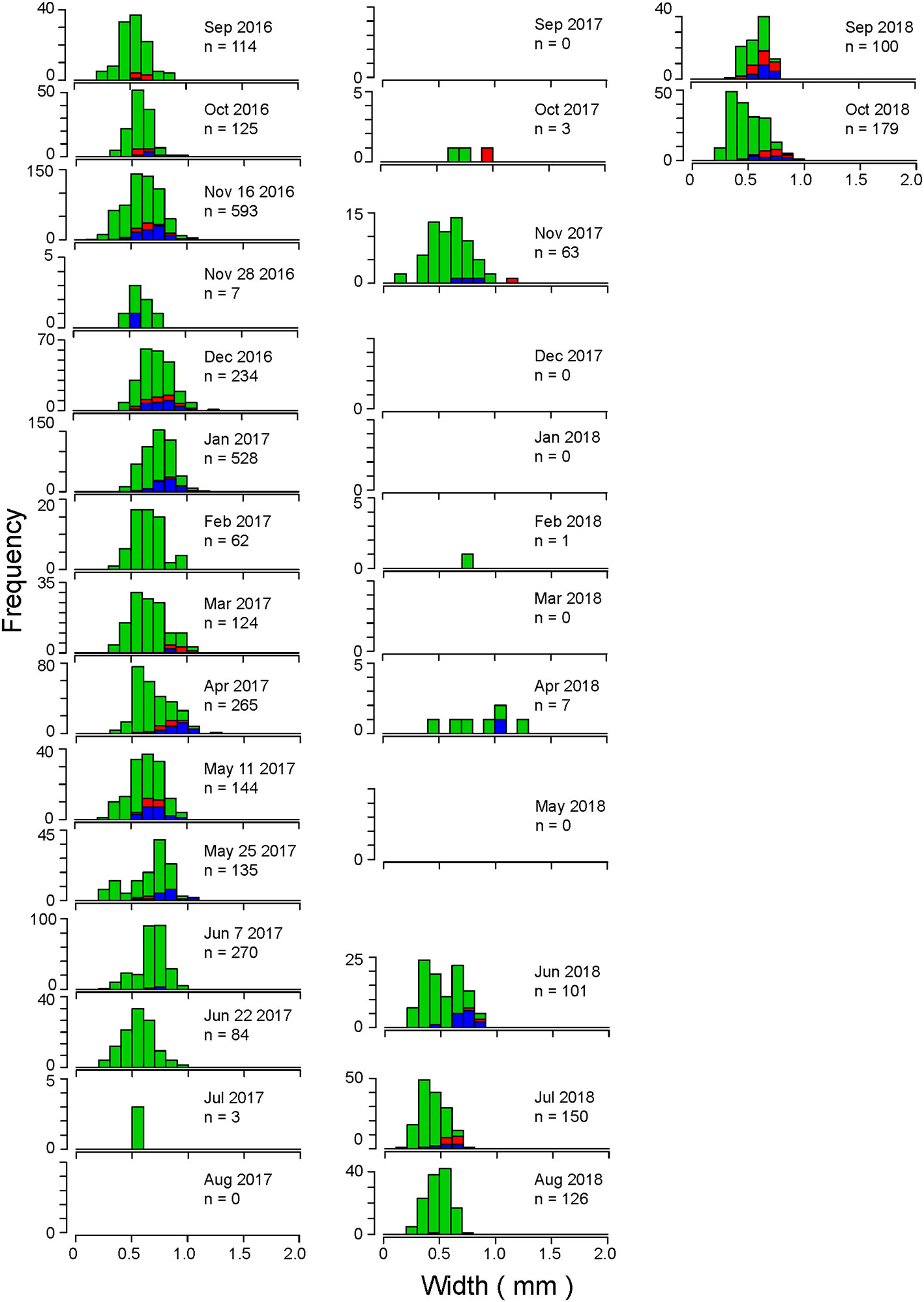
Figure 6 Size frequency distributions of the maximum width of the thoracic segment of C. aff. teleta at Stn C from September 2016 to March 2018 and at Stn C′ from April 2018 to October 2018 in Gamo Lagoon. Red: females, blue: males, green: immature individuals.
At Stn D, individuals with body width <0.5 mm appeared every month, except September 2016 and April 2018 (Figure 7). Large individuals (≥1 mm) emerged from October 2016 to April 2017 and from December 2017 to February and May 2018. Larger mature individuals (≥1 mm) emerged from October 2016 to April 2017 and January 2018. Smaller mature individuals (<0.5 mm) emerged in November 2016; January, April, and September 2017; and August and September 2018. The occurrence of males tended to be more abundant than females in Stn D.
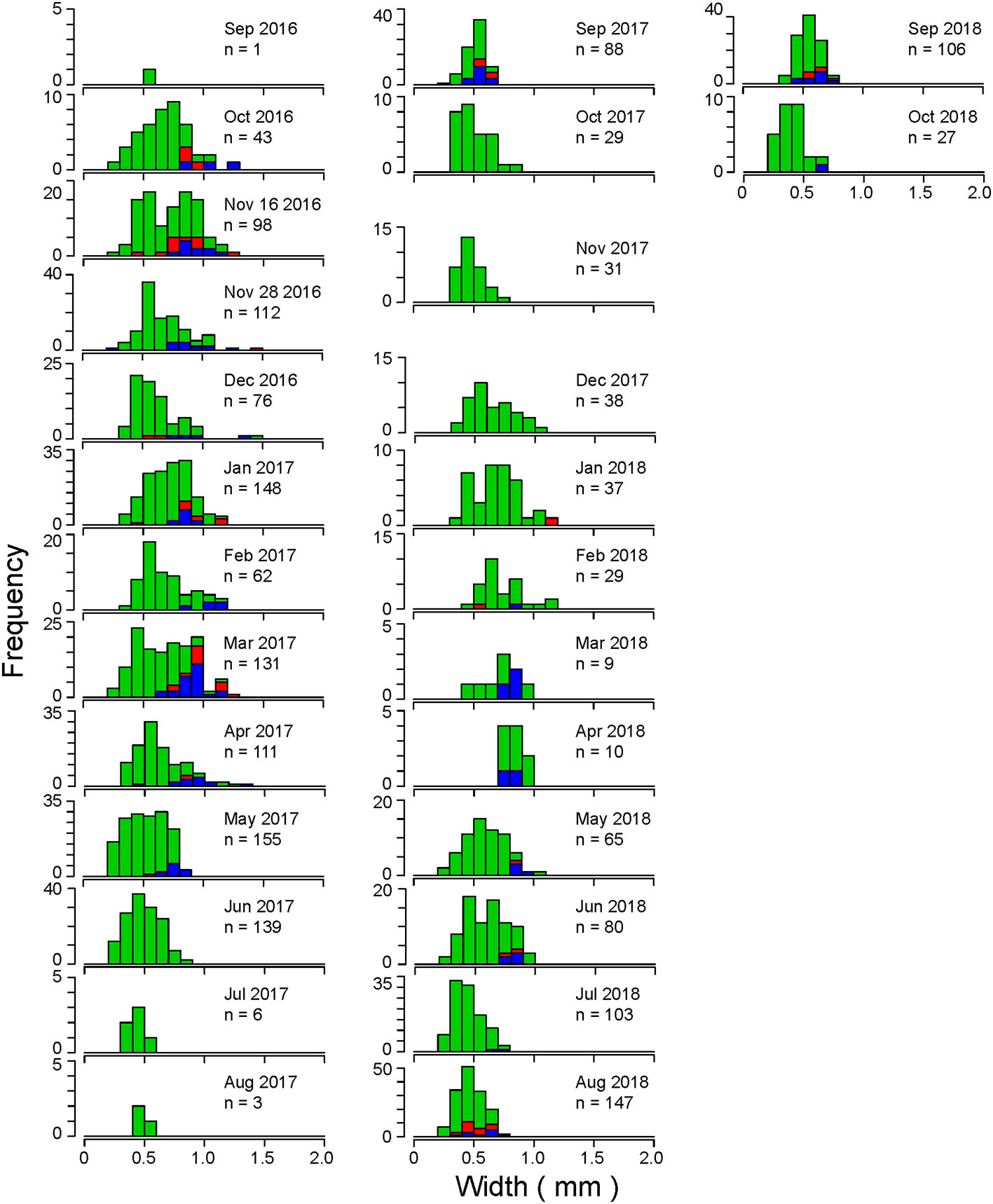
Figure 7 Size frequency distributions of the maximum width of the thoracic segment of C. aff. teleta at Stn D in Gamo Lagoon from September 2016 to October 2018. Red: females, blue: males, green: immature individuals.
Discussion
Environmental changes in Gamo Lagoon
The environment of Gamo Lagoon can be divided into low- and high-water-temperature periods, with a water temperature of 15°C. Diurnal hypoxia was observed mainly during the high-water-temperature period, but diurnal hypoxia was also observed from November 2017 to March 2018. Therefore, factors other than high water temperature can be related to a decrease in the levels of the DO concentration in Gamo Lagoon. Salinity fluctuated greatly from August to October, suggesting an increase in water runoff from the Nanakita River.
Seasonal fluctuations were observed in redox potential values, which tended to be higher during the low-water-temperature period and lower during the high-water-temperature period. During the low-water-temperature period from 2017 to 2018, the redox potential did not increase as much as it did from 2016 to 2017. From July 2017 onward, the redox potential continued to show negative values. This suggests that although water temperature or DO levels seemed to recover, the redox potential did not change and had low values since July 2017. Various factors, such as water temperature, DO, hydrogen sulfide, and pH, are thought to affect redox potential values—with hydrogen sulfide having a particularly strong effect—an increase in hydrogen sulfide results in a decrease in the redox potential (Muto and Kim, 1986).
Topographic changes in Gamo Lagoon
Water levels, both the daily minimum water depth and daily maximum water depth, declined from February to March 2017 and from December 2017 to May 2018. Moreover, sand sedimentation became noticeable in the beginning of summer 2017. Following these periods, the innermost part of the lagoon was found to be isolated in March 2017 and December 2017–April 2018 during low tide. During this period, a series of restoration works occurred at the estuarine side of the lagoon, which includes the reconstruction of the levee that was damaged by the 2011 tsunami (Figure 8) (Public Works Department, Miyagi Prefecture, 2021) and may have affected the water level of Gamo Lagoon. As the inner part of the lagoon began to dry and water flow continued to stagnate, artificial channel excavation was conducted in August 2018 to allow water to pass through the estuarine side and the innermost part of the lagoon (Figure 9) (Public Works Department, Miyagi Prefecture, 2021).
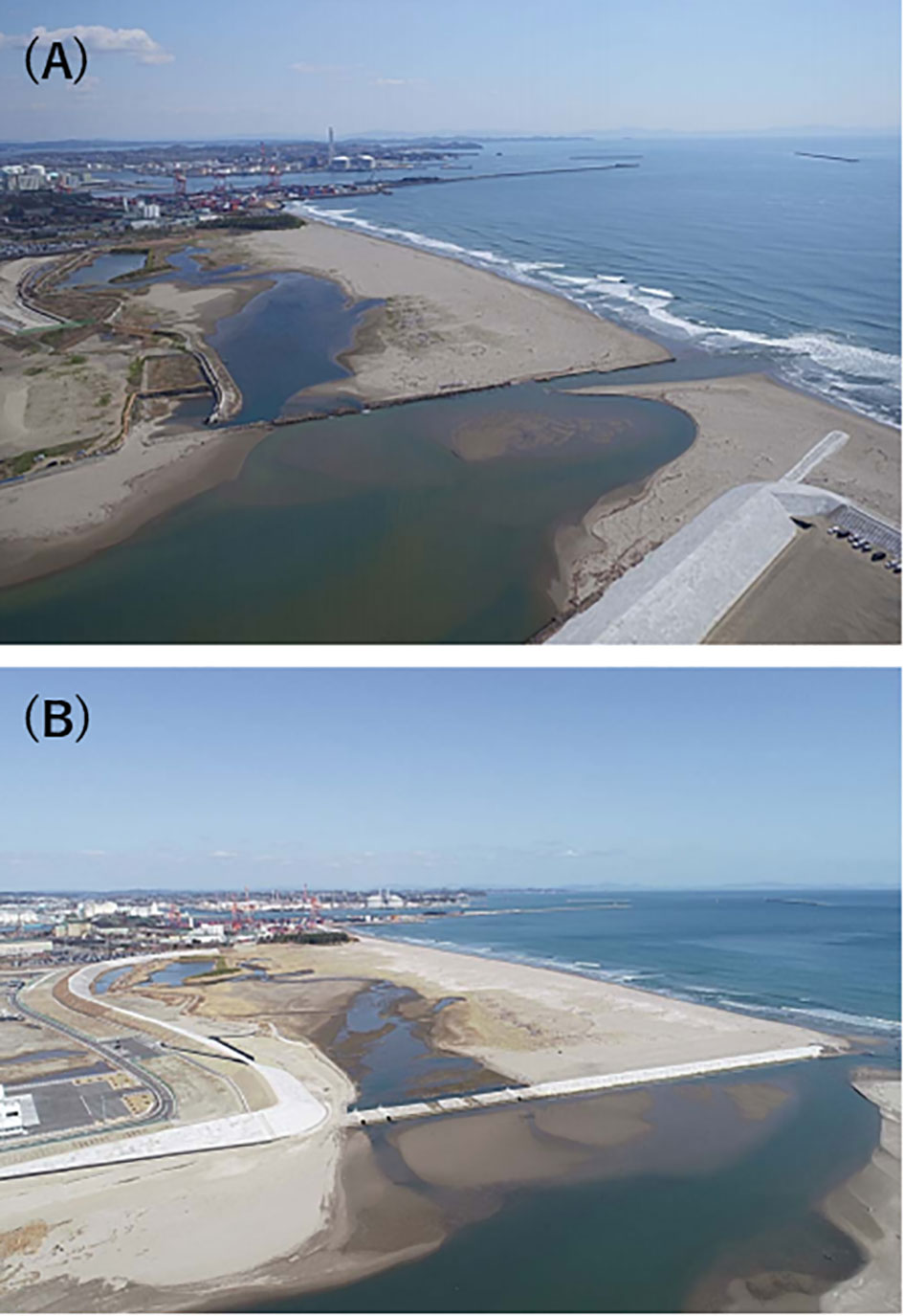
Figure 8 Photographs (A) before and (B) after the reconstruction of the levee in Gamo Lagoon. (A) Photographed in April 2017 and (B) March 2021 (from Public Works Department, Miyagi Prefecture, March 2021).
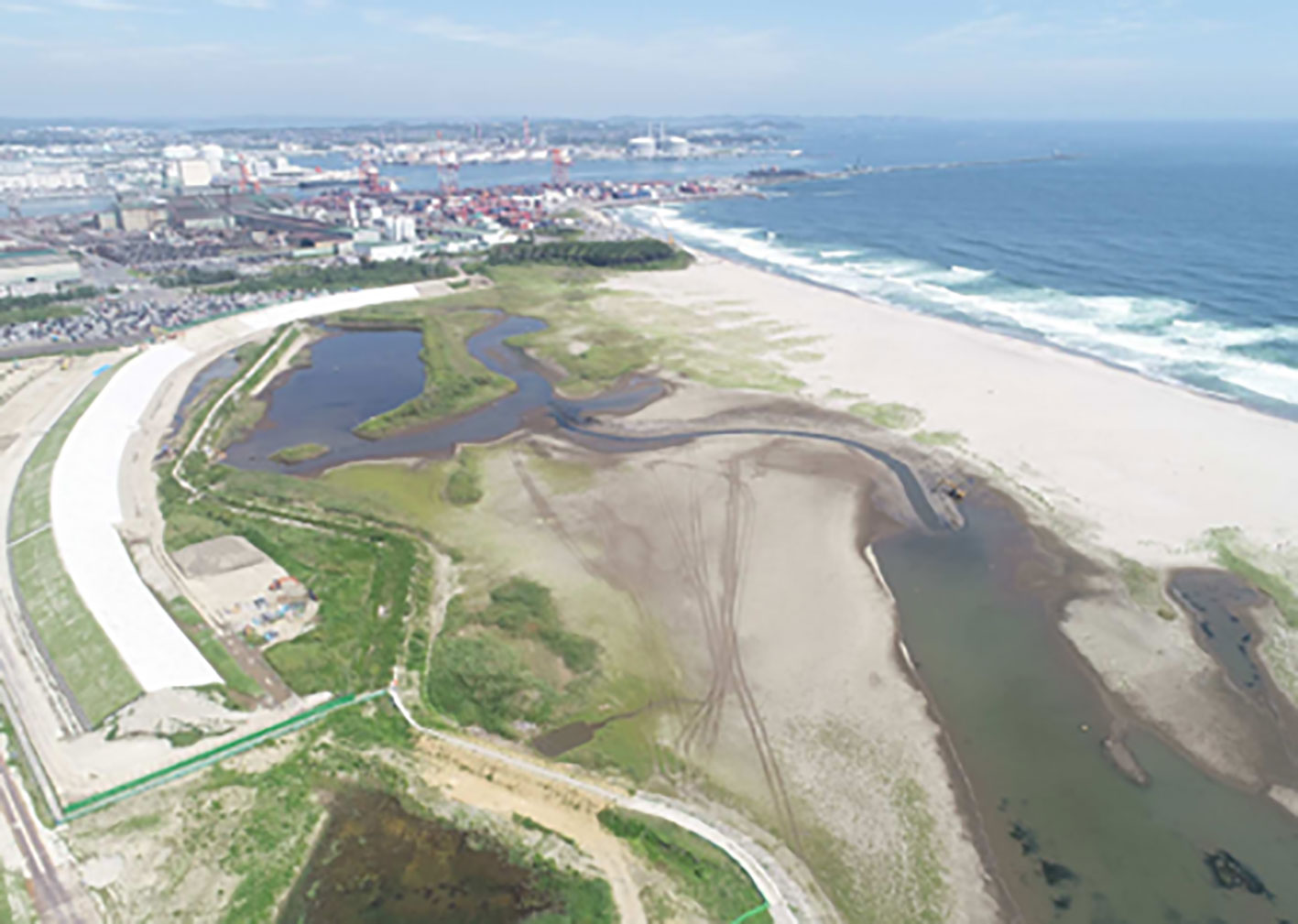
Figure 9 Photograph after channel excavation was conducted in August 2018 (from Public Works Department, Miyagi Prefecture, March 2021).
Although many periods, when the minimum water level decreased, showed a simultaneous decrease in the maximum water level, the maximum water level did not decrease after August 2018, resulting in more fluctuating water levels (Figure 3D). A possible reason for this is that a channel was excavated artificially in August (Figure 9) (Public Works Department, Miyagi Prefecture, 2021). The construction of the channel is thought to have increased the amount of flowing water, and the large fluctuations in water levels suggest that the amount of water conversion increased significantly since August 2018.
Population dynamics of C. aff. teleta
Density
Differences in the size density were observed between the three stations during September 2016–October 2018. Stn A, on the estuary side, had the lowest density throughout the period, whereas Stns C (and C′) and D, located in the inner part, had one order of magnitude higher density than Stn A. Densities at the three stations were unstable and fluctuated under 4,000 ind/m2 at Stn A, 40,000 ind/m2 at Stn C, and 60,000 ind/m2 at Stn D from September 2016 to July 2017. Although each station had density peaks at different times and the pattern of increase and decrease was different and not identical during the fluctuation period, C. aff. teleta almost disappeared in all three stations beginning from July or August 2017 to April or May 2018. Although the number of individuals was very low, such that the density indicated almost zero, it was never absent for that period. Environmental observations revealed that the period coincided with the period under diurnal hypoxia and low-redox-potential conditions during high water temperatures and followed a decrease in the water level below approximately 0.5 m (Figure 3D). However, after that period, individuals suddenly started to increase from May or June, and the peak densities at Stns A, C′, and D were observed to shift by 1 month each to June (reached up to 10,000 ind/m2), July (55,000 ind/m2), and August (30,000 ind/m2), respectively, resulting in the recovery of abundance. The increase in daily maximum water level coincided with the timing of abundance increase.
In the three stations in Gamo Lagoon, population density decreased drastically after the tsunami that occurred following the Fukushima Earthquake in late November 2016. However, by January 2017, density rapidly recovered to almost above pre-tsunami levels in Stns C and D. Notably, the tsunami that occurred after the 2016 Fukushima Earthquake seemed to be have a partial impact; only the density at Stn C declined immediately and temporarily, whereas no immediate decrease was observed at Stns A and D. However, in February 2017, density again declined dramatically to below that of the immediate post-tsunami period in Stns C and D. This period coincides with a period of decreased water depth. The population density then increased through May and June but then decreased toward July. Density did not increase even after the high-water-temperature period ended, probably because diurnal hypoxia frequently occurred and the redox potential remained severely low under low water levels because of the start of the levee reconstruction work. However, densities increased from June to August 2018, even under hypoxia and high redox conditions, but the daily maximum water level increased. It appeared that the daily maximum water level had a large impact on the population density of C. aff. teleta in the entire lagoon (Figure 10). This suggests that after the water level recovered and circulated normally, C. aff. teleta would interact with other stations through water flow.
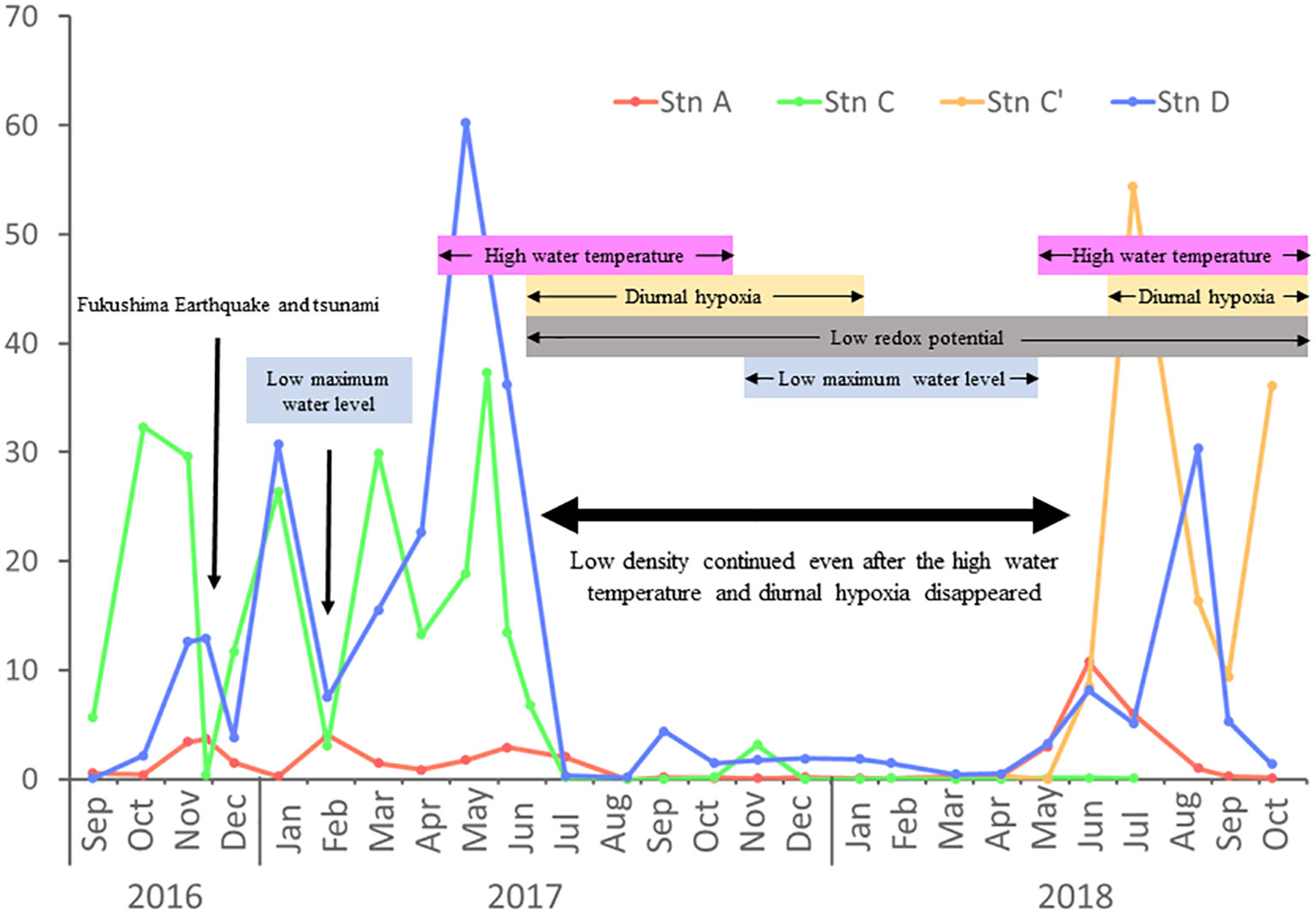
Figure 10 Summarizing C. aff. teleta density and environmental factors associated with water stagnation and circulation due to levee construction and channel excavation.
Size frequency distribution
The size histogram data from three stations (A, C and C′, and D) were combined to analyze changes in the size frequency distribution of C. aff. teleta in Gamo Lagoon as a single water area (Figure 11). Smaller individuals with body width <0.5 mm appeared each month. Even during the periods of very low density from July 2017 to April 2018, the recruitment of a small number of juveniles and smaller individuals, with body width <0.5 mm, was observed at least in one station and in most months in all stations (Figures 5–7). Body width increased gradually from September to March or April 2016–2018 and gradually decreased from May to August in both 2017 and 2018 (Figure 11). From October 2016 to April 2017 and November 2017 to May 2018, individuals with >1 mm body width were observed. No individual with >1 mm body width was observed in July–September in all stations during the 3 years. More males than females appeared in many months in Gamo Lagoon. Although females with eggs in their coeloms were few during the entire period and at all stations, females were always present in at least one station in all months, except July, August, and December 2017 and March and April 2018. From November 2016 to February 2017, the minimum body width of mature individuals increased. Mature individuals with >1 mm body width appeared from November 2016 to April 2017; November 2017; and January, April, and May 2018. Smaller mature individuals with body width ≤0.5 mm also appeared in November 2016, September 2017, April 2018, and after June 2018, mainly during the high-water-temperature period. These results did not contradict a preliminary study of the life history and lifespan of C. aff. teleta in Gamo Lagoon by Kondoh (2017) based on laboratory breeding experiments. The previous study reported that at <15°C water temperature, C. aff. teleta spawn 55 d after settling, with multiple spawning cycles and a lifespan of approximately 3 months. Body size increased in this case. Mature females spawned eggs in 10 d, and spawning to planktonic larval hatching took 2 weeks. During the high-water-temperature period, the size of the mature females was smaller and the lifespan seemed to be approximately 2 months after one spawning (Kondoh, 2017). Thus, during high water temperatures, they matured and spawned faster, and, during low water temperatures, their body size increased and they spawned many times.
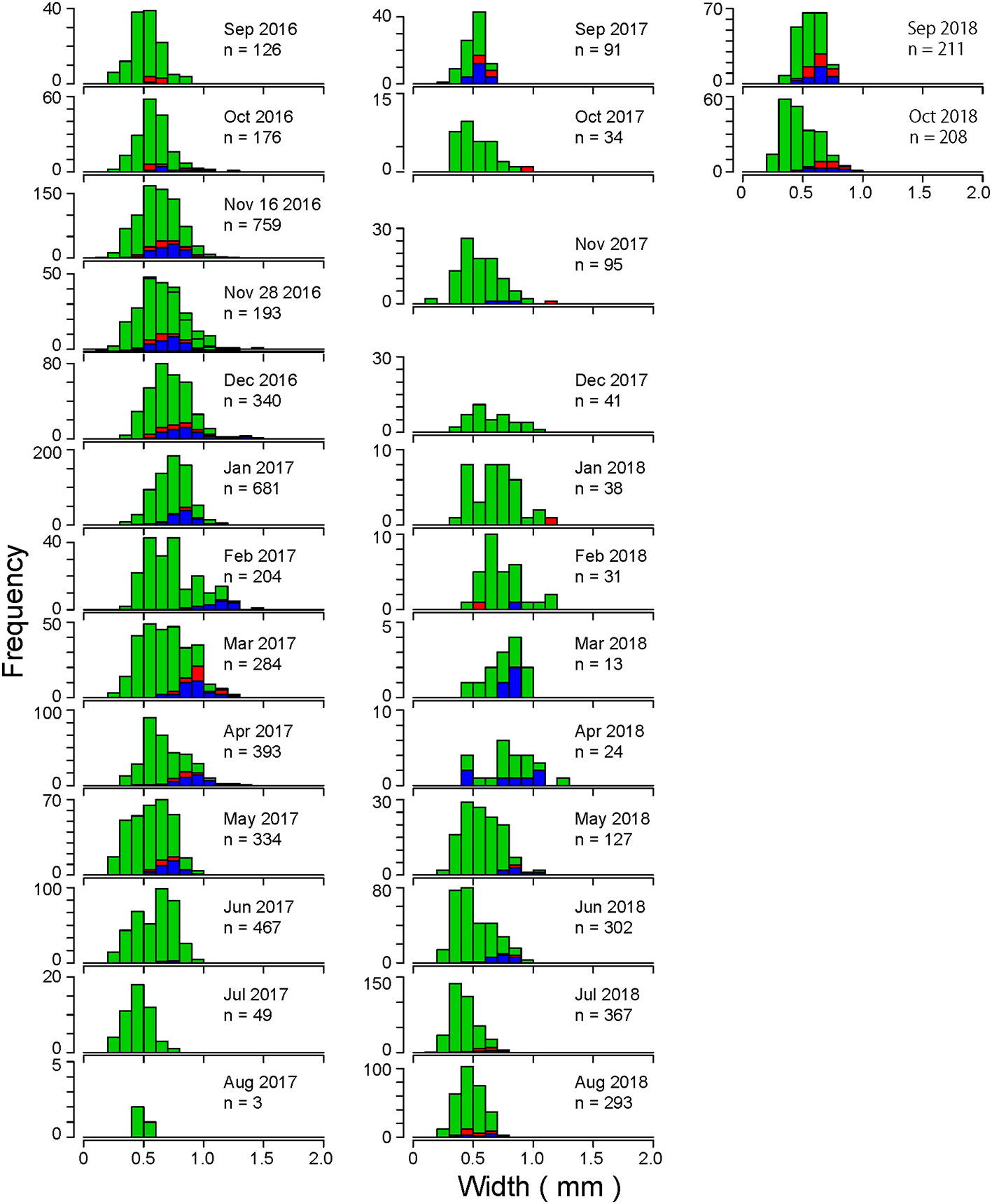
Figure 11 Size frequency distributions of the maximum width of the thoracic segment of total C. aff. teleta at the three stations in Gamo Lagoon from September 2016 to October 2018. Red: females, blue: males, green: immature individuals.
A detailed body-width composition analysis was performed for each station. At Stn A, new recruitment was observed and abundance was maintained thereafter, although no females had appeared since April 2017. During this period, females were observed at Stns C (and C′) and D. In addition, despite the presence of females in the previous month, no new recruitment was seen in December 2016. This may indicate that the new recruitment at Stn A was largely dependent on planktonic larva recruitment from other stations. By contrast, at Stns C and D, new recruitment was observed in March 2017 although females were absent in the previous month. In February, females were observed only in Stn A, suggesting that planktonic larvae from Stn A were transferred to Stns C and D. At Stn D, although females were absent in July 2018, new recruitment was observed in August and density increased rapidly. No females were observed in Stns A, C, and D in July; however, females were observed in Stn C′. The new recruitment to Stn D may have been transferred by planktonic larvae from Stn C′. These results suggest that each station was supported by the transfer of planktonic larvae from other stations inside Gamo Lagoon.
At Stn D, from October 2016 to April 2017 and December 2017 to May 2018, individuals with body size >1 mm in width appeared for a long period of time. We believe that the presence of larger-sized individuals in Stn D during the low-temperature period contributed to the continuous increase in population.
Population density decreased dramatically in February 2017 despite the presence of mature individuals in January 2017 and new recruitment in February 2017. Similarly, although mature individuals were seen in September 2017 and many new recruitments were seen in October 2017, population density did not increase in October. The decrease in density was not likely due to the lack of new recruitment but rather other factors, such as environmental factors.
How to maintain a population in Gamo Lagoon
By comparing the change in the density and size frequency distribution of C. aff. teleta at multiple sampling stations inside the lagoon, we could examine how C. aff. teleta maintains its population in Gamo Lagoon, where the environment has been fluctuating and unstable due to restoration work.
The Fukushima Earthquake and tsunami in November 2016 added to the initial disturbances following the 2011 Great East Japan Earthquake and tsunami in the lagoon. The reconstruction of the destroyed levee began in 2017. This resulted in destabilizing the sand sedimentation dynamics and changes in the water level. Although these changes were not as large as the disturbance caused by the 2011 Great East Japan Earthquake and tsunami (Kanaya et al., 2012; Kondoh et al., 2020), they likely had a significant impact on the population dynamics of C. aff. teleta.
We have discussed the significance of each station in Gamo Lagoon and how C. aff. teleta maintains its population at these stations by showing the environmental changes at each station and comparing and summarizing the characteristics of C. aff. teleta dynamics at each station. Stn A is located closer to the river, whereas Stns C and D are in the middle and inner parts of the lagoon, respectively. In Gamo Lagoon, only one levee exists at the riverside, which tends to stagnate topographically; the amount of organic matter increases as one moves into the inner part (Kanaya and Kikuchi, 2004). Population growth increases in organically rich muddy sediment (Kanaya et al., 2016), and, as shown in this study, densities at the inner parts of Stns C and D were higher than those at Stn A. Thus, Stns C and D—located in the inner part of the lagoon, with higher organic matter—are more suitable for increasing the density of C. aff. teleta than Stn A.
As eutrophication progresses, the bottom sediment becomes hypoxic and anaerobic metabolism becomes dominant (Hargrave, 2008), leading to the accumulation of toxic substances, such as free hydrogen sulfide (Gray et al., 2002). Microbial sulfate reduction, to release dissolved sulfides, is predominant in the anaerobic sediment layers of marine environments (Hargrave, 2008). Sulfides usually do not accumulate in the form of free hydrogen sulfide because they react quickly with metals, such as iron, and precipitate as insoluble sulfides; however, under excessive organic loading, sulfide formation exceeds the buffering capacity of iron in the sediment (Heijs et al., 1999), leading to the accumulation of free hydrogen sulfide (Marvin-DiPasquale and Capone, 1998). High hydrogen sulfide concentrations (up to 6.6 mmol/L of sediment) have been detected in Gamo Lagoon, especially in the inner part of the lagoon, with high water temperatures. These values are similar to or considerably higher than those of other eutrophic estuarine sediments (Gamenick et al., 1996; Marvin-DiPasquale and Capone, 1998; Sakai et al., 2012; Kanaya et al., 2015).
In Gamo Lagoon, the population growth of many opportunistic polychaetes, including C. aff. teleta, was shown to be inhibited by the excessive accumulation of sulfides under hypoxic conditions (Kanaya, 2014). During winter and spring, when sediment sulfide concentrations decrease, the number of opportunistic polychaetes increases rapidly (Kanaya et al., 2005; Kanaya and Kikuchi, 2011). Our findings revealed that in addition to hypoxia and high reduction conditions during the high-water-temperature period, hypoxia occurred frequently from November 2017 to March 2018 (Figure 3C). During this period, very small numbers of C. aff. teleta were observed from July 2017 to April 2018. Simultaneously, large numbers were observed after June 2018, when hypoxic conditions were also frequent. The daily maximum water level was higher after May 2018, and water level fluctuations increased in August. This may have led to a large increase in water conversion. Although sulfides in the sediments have been reported to a decrease in concentration as they are washed away after water conversion increases (Nielsen et al., 2003; Volkenborn, 2007), the reduction potential values remained low from June 2017 in this study.
We hypothesized that the formation of a large population only after June 2018, despite the same frequency of hypoxic and negative redox potential conditions, was due to differences in the water level. Therefore, the maximum water level must be maintained at a high level for enough water change so that the C. aff. teleta population can be maintained in the inner part. Moreover, our findings revealed that C. aff. teleta can maintain its population under low DO and redox conditions if water conversion is enough.
When the highest water level decreased in February 2017, a decrease in density was observed in Stns C and D, which are close to the water level observation point, whereas the opposite trend was observed in Stn A, which is close to the riverside. This suggests that the decrease in the water level has a direct impact on the population in the inner part of the lagoon. However, when the highest water level dropped again from November 2017 to May 2018, the density decreased at Stn A as well. Considering that Stn A maintains its population through larval transfers from other stations, the failure to increase densities at Stns C and D due to the drop in the water level for a long period may have affected population density at Stn A. In addition, since June 2018, C. aff. teleta could increase in abundance throughout Gamo Lagoon, although the minimum water level was lower and the maximum water level was higher. These findings suggest that the maximum and not minimum water level affects population construction.
The trend of the C. aff. teleta population at each station had the following characteristics: at Stn A, the habitat was maintained by relying heavily on larval transfers from other stations; at Stn C, the habitat was susceptible to the lowering of the water level in the lagoon, and the more time the bottom sediment had to dry out, the more difficult it became for C. aff. teleta to inhabit the low water levels. However, despite a period of almost no habitat for approximately 11 months in the lagoon, high densities were again constructed at Stn C′ due to the transfer of planktonic larvae from other stations. Stn D showed the highest density between the three stations even after the summer of 2017, when the density was low throughout. By contrast, new recruitment also occurred due to transfers from other stations. Thus, each station showed different characteristics in the density and dynamics of C. aff. teleta in Gamo Lagoon.
New recruitments were seen in many months in all stations, and there were periods when new recruitments were seen despite the absence of females nearby, indicating that they were largely supported by the supply of planktonic larvae from other stations. Although the density varied between stations, at least one station had females during the period, suggesting that each station supplied planktonic larvae to the other and that they were interdependent and maintained as a single population throughout the Gamo Lagoon area. Therefore, it is important for populations to interact to maintain the C. aff. teleta population in Gamo Lagoon.
Due to the original topography of Gamo Lagoon, water tends to stagnate in the inner side of the lagoon and becomes a hypoxic and reducing environment under high water temperatures. In this study, we examined how C. aff. teleta, a capitellid polychaete that maintains its population in such an environment, was impacted during the 2 years when restoration work was underway. During the construction of a new levee, the balance of sedimentation dynamics was disrupted, the central part dried up, and water exchange with the riverside was lost, making the inner part isolated. This disrupted the supply of larvae over the lagoon, and the density decreased drastically and continued to be low. Later, an increase in the daily maximum water level initiated water to flow between the inner part and the riverside, and the density recovered. Thus, C. aff. teleta requires both environments—the inner part and the riverside of the lagoon—to maintain its population and that it is necessary to allow the water bodies to interact with each other.
The ecological adaptation of C. aff. teleta in Gamo Lagoon can be summarized as follows: C. aff. teleta prefers to live in the middle and innermost parts, namely, stations C and D, respectively, which are organic-rich environments. However, the innermost parts have a high risk of hypoxia and a low redox potential. When the population declines due to deterioration, it recovers by recruiting floating larvae from the riverside station A, which is not a favorable habitat; therefore, only a small number is maintained. In this lagoon, the presence of stations in different environments, both at the innermost part and the riverside, may be considered necessary to maintain the population in the lagoon as a whole, because both sides supply each other with planktonic larvae. Thus, water flow, which connects different environmental stations, is the most important factor for the maintenance of C. aff. teleta populations.
Many macrobenthos, including opportunistic polychaete species such as C. aff. teleta, have been affected by human activities that have altered their habitats, such as water flow and drought, but have continued to maintain their populations. It is important to understand the ecological characteristics of these animals, minimize the impact of habitat change, and maintain a sustainable marine ecosystem and biodiversity.
Data availability statement
The raw data supporting the conclusions of this article will be made available by the authors, if necessary.
Author contributions
WS-O conceived and designed the research. AH generated and analysed the data. WS-O wrote the first draft of the manuscript. All authors contributed to the article and approved the submitted version.
Funding
This study was supported by a grant from the Tohoku Ecosystem-Associated Marine Sciences (TEAMS) research program of the Ministry of Education, Culture, Sports, Science and Technology (MEXT). This study was partially supported by a research grant from JSPS KAKENHI Grant Number: JP15K07540, JP18K05777) to Waka Sato-Okoshi.
Acknowledgments
We are grateful to the members of the Laboratory of Biological Oceanography, Graduate School of Agricultural Science, Tohoku University, for their cooperation and assistance during field observations. Thanks especially to Dr. Tomohiko Kondoh for his continuous research advice and to Akane Nishioka for her cooperation in creating figures. We thank Miyagi Prefecture for their cooperation in providing us with an overview of the Gamo restoration works and photographs during the work period.
Conflict of interest
The authors declare that the research was conducted in the absence of any commercial or financial relationships that could be construed as a potential conflict of interest.
Publisher’s note
All claims expressed in this article are solely those of the authors and do not necessarily represent those of their affiliated organizations, or those of the publisher, the editors and the reviewers. Any product that may be evaluated in this article, or claim that may be made by its manufacturer, is not guaranteed or endorsed by the publisher.
References
Blake J. A. (2009). Redescription of Capitella capitata (Fabricius) from West Greenland and designation of a neotype (Polychaeta, capitellidae). Zoosymposia 2, 55–80. doi: 10.11646/zoosymposia.2.1.7
Blake J. A., Grassle J. P., Eckelbarge K. J. (2009). Capitella teleta, a new species designation for the opportunistic and experimental Capitella sp. I, with a review of the literature for confirmed records. Zoosymposia 2, 25–53. doi: 10.11646/zoosymposia.2.1.6
Chareonpanich C., Montani S., Tsutsumi H., Matsuoka S. (1993). Modification of chemical characteristics of organically enriched sediment by Capitella sp. I. Mar. Poll. Bull. 26, 375–379. doi: 10.1016/0025-326X(93)90184-L
Diaz R. J., Rosenberg R. (1995). Marine benthic hypoxia: A review of its ecological effects and the behavioural responses of benthic macrofauna. oceanogr. Mar. Biol. 3, 245–303.
Eckelbarger K. J., Grassle J. P. (1983). Ultrastructral differences in the eggs and ovarian follicle cells of Capitella (Polychaeta) sibling species. Biol. Bull. 165, 379–393. doi: 10.2307/1541203
Fabricius O. (1780). Fauna Groenlandica, systematice sistens animalia groenlandiae occidentalis hactenus indagata, quoad nomen specificium, triviale, vernaculumque, synonyma auctorum plurimum, descriptionem, locum, victum, generationem, mores, usum capturamque singuli, pro ut detegendi occasio fuit, maximaque parte secundum proprias observationes.
Gamenick I., Jahn A., Vopel K., Giere O. (1996). Hypoxia and sulphide as structuring factors in a macrozoobenthic community on the Baltic Sea shore: Colonization studies and tolerance experiments. Mar. Poll. Bull. 114, 73–85.
Gamenick I., Vismann B., Grieshaber M. K., Giere O. (1998). Ecophysiological differentiation of Capitella capitata (Polychaeta). Sibling species from different sulfidic habitats. Mar. Ecol. Prog. Ser. 175, 155–166. doi: 10.3354/meps175155
Grassle J. P., Grassle J. F. (1976). Sibling species in the marine pollution indicator Capitella (Polychaeta). Science 192, 567–569. doi: 10.1126/science.1257794
Gray J. S., Wu R. S., Or Y. Y. (2002). Effects of hypoxia and organic enrichment on the coastal marine environment. Mar. Poll. Bull. 238, 249–279. doi: 10.3354/meps238249
Hargrave B. T. (2008). Towards a classification of organic enrichment in marine sediments based on biogeochemical indicators. Mar. Poll. Bull. 5, 810–824. doi: 10.1016/j.marpolbul.2008.02.006
Hartman O. (1959). Capitellidae and Nereidae (marine annelids) from the Gulf side of Florida with a review of freshwater Nereidae. Bull. Mar Sci. Gulf Caribbean. 9, 153–168.
Heijs S. K., Jonhers H. M., Gemerden H. V., Schaub B. E. M. (1999). The buffering capacity towards free sulphide in sediments of a coastal lagoon (Bassin d' arcachon, France) -the relative importance of chemical and biological processes. Estuar. Coast. Shelf. Sci. 49, 21–35. doi: 10.1006/ecss.1999.0482
Honma Y., Kitami T., Ito S. (1974). Fauna of lake kamo-ko, sado island in the Japan Sea (a preliminary report). Proc. Jap. Soc Syst. Zool. 10, 63–73.
Ide S., Baltay A., Beroza G. C. (2011). Shallow dynamic overshoot and energetic deep rupture in the 2011 mw 9.0 tohoku-oki earthquake. Science 332, 1426–1429. doi: 10.1126/science.1207020
Imajima M., Hartman O. (1964). “The polychaetous annelids of Japan,” in Allan Hancock Found publications occasional paper California, USA: University of Southern California, vol. 26, 1–452.
Kanaya G. (2014). Recolonization of macrozoobenthos on defaunated sediments in a hypertrophic brackish lagoon: Effects of sulfide removal and sediment grain size. Mar. Environ. Res. 95, 81–88. doi: 10.1016/j.marenvres.2013.12.014
Kanaya G., Kikuchi E. (2004). Relationships between sediment chemical buffering capacity and H2S accumulation: Comparative study in two temperate estuarine brackish lagoons. Hydrobiologia 528, 187–199. doi: 10.1007/s10750-004-2342-8
Kanaya G., Kikuchi E. (2011). Infuences of anthropogenic eutrophication on estuarine benthic ecosystems. Chikyu Kankyo 16, 33–44.
Kanaya G., Maki H., Suzuki T., Sato-Okoshi W., Kikuchi E. (2014). Tsunami-induced changes in a shallow brackish lagoon ecosystem (Gamo lagoon) in Sendai bay, Japan. Glob. Environ. Res. 18, 35–46.
Kanaya G., Nobata E., Toya T., Kikuchi E. (2005). Effects of different feeding habits of three bivalve species on sediment characteristics and benthic diatom abundance. Mar. Ecol. Prog. Ser. 229, 67–78. doi: 10.3354/meps299067
Kanaya G., Suzuki T., Kikuchi E. (2015). Impacts of the 2011 tsunami on sediment characteristics and macrozoobenthic assemblages in a shallow eutrophic lagoon, Sendai bay, Japan. PloS One 10 (8), e0135125. doi: 10.1371/journal.pone.0135125
Kanaya G., Suzuki T., Maki H., Nakamura Y., Miyajima Y., Kikuchi E. (2012). Effects of the 2011 tsunami on the topography, vegetation, and macrobenthic fauna in gamo lagoon, Japan. Jap. J. Benthol. 67, 20–32.
Kanaya G., Uehara T., Kikuchi E. (2016). Effects of sedimentary sulfide on community structure, population dynamics, and colonization depth of macrozoobenthos in organic-rich estuarine sediments. Mar. Poll. Bull. 109, 393–401. doi: 10.1016/j.marpolbul.2016.05.043
Kinoshita K., Tamaki S., Yoshioka M., Srithonguthai S., Kunihiro T., Hama D., et al. (2008). Bioremediation of organically enriched sediment deposited below fish farms with artificially masscultured colonies of deposit-feeding polychaete Capitella sp. I. Fish. Sci. 74, 77–87. doi: 10.1111/j.1444-2906.2007.01498.x
Kitamori R. (1960). The relation between the distribution of genus Capitella, polychaetous annelids, and pollution. Bull. Naikai. Reg. Fish. Res. Lab. 87, 1–10.
Kondoh T. (2017). Benthic community dynamics and life history strategies of dominant species in gamo lagoon (Miyagi prefecture, Japan) after the great East Japan earthquake and tsunami disturbance (Sendai: Tohoku University).
Kondoh T., Nakayama G., Sato-Okoshi W. (2020). “Preliminary report of impacts of the 2011 earthquake and tsunami and subsequent events on macrobenthic community in a shallow brackish lagoon in Sendai bay, Japan,” in Evolution of marine coastal ecosystems under the pressure of global changes, Ed. Ceccaldi H.-J., Henocque Y., Komatsu T., Prouzet P., Sautour B., Yoshida J., et al (Switzerland AG: Springer Nature), 163–172.
Langerhans P. (1880). Die Wurmfauna von Madeira. III. Z. für wissenschaftliche Zoologie. 34, 87–143.
Linke-Gamenick I., Forbes V. E., Méndez N. (2000). Effect of chronic fluoranthene exposure on sibling species of Capitella with different development modes. Mar. Ecol. Prog. Ser. 203, 191–203. doi: 10.3354/meps203191
Magalhães F., Bailey-Brock J. H. (2012). Capitellidae grube 1862 (Annelida: Polychaeta) from the Hawaiian islands with description of two new species. Zootaxa 3581, 1–52. doi: 10.11646/zootaxa.3581.1.1
Marvin-DiPasquale M. C., Capone D. G. (1998). Benthic sulfate reduction along the Chesapeake bay central channel. i. spatial trends and controls. Mar. Ecol. Prog. Ser. 168, 213–228. doi: 10.3354/meps168213
Méndez N. (2002). Experimental evidence of polymorphysm of sexual development in Capitella sp. b (Polychaeta: Capitellidae) from Barcelona, Spain. Sci. Mar. 66, 103–110. doi: 10.3989/scimar.2002.66n2103
Méndez N. (2006). Life cycle of Capitella sp. y (Polychaeta: Capitellidae) from estero del yugo, mazatlán, Mexico. J. Mar. Biol. Ass. UK 86, 263–369. doi: 10.1017/S0025315406013117
Muto N., Kim K. S. (1986). Experimental studies of factors influencing the value of ORP―Water temperature, DO, H2S and pH―. Jap. J. Water pollut. Res. 9, 661–667. doi: 10.2965/jswe1978.9.661
Nielsen O. I., Kristensen E., Holmer M. (2003). Impact of Arenicola marina (Polychaeta) on sediment sulfur dynamics. Aquat. Microb. Ecol. 33, 95–105. doi: 10.3354/ame033095
Nishi E., Ueda L., Sakamoto A., Sugihara N., Shimosako K., Sanada S. (2010). Benthos fauna of artificially created tidal flats in Yokohama port, Tokyo bay. Nat. Hist. Rep. Kanagawa. 31, 29–33.
Ozawa S., Nishimura T., Suito H., Kobayashi T., Tobita M., Imakiire T. (2011). Coseismic and postseismic slip of the 2011 magnitude-9 tohoku-oki earthquake. Nature 475, 373–376. doi: 10.1038/nature10227
Public Works Department, Miyagi Prefecture (2021). Environmental consideration records of the reconstruction works on the rivers and coasts in Miyagi after the Great East Japan Earthquake/tsunami (Miyagi Prefecture: Public Works Department), 344.
Sakai S., Nakaya M., Sampei Y., Dettman D. L., Takayasu K. (2012). Hydrogen sulfide and organic carbon at the sediment-water interface in coastal brackish lake nakaumi, SW Japan. Environ. Earth Sci. 7, 1999–2006.
Silva C. F., Shimabukuro M., Alfaro-Lucas J. M., Fujiwara Y., Sumida P. Y. G., Amaral A. C. Z. (2016). A new Capitella polychaete worm (Annelida: Capitellidae) living inside whale bones in the abyssal south Atlantic. Deep-Sea. Res. I. 108, 23–31. doi: 10.1016/j.dsr.2015.12.004
Tanaka H., Mitobe Y., Hoang V. C. (2016). Estuarine morphological changes due to the 2015 kanto-tohoku heavy rainfall and their subsequent recovery. Tohoku. J. Natl. Disaster. Sci. 52, 67–72.
Tomioka S., Kondoh T., Sato-Okoshi W., Ito K., Kajihara H. (2016). Cosmopolitan or cryptic species? A case study of Capitella teleta (Annelida: Capitellidae). Zool. Sci. 33, 545–555. doi: 10.2108/zs160059
Tsutsumi H. (1987). Population dynamics of Capitella capitata (Polychaeta; capitellidae) in an organically polluted cove. Mar. Ecol. Prog. Ser. 36, 139–149. doi: 10.3354/meps036139
Tsutsumi H. (1990). Population persistence of Capitella sp. (Polychaeta; capitellidae) on a mud flat subject to environmental disturbance by organic enrichment. Mar. Ecol. Prog. Ser. 63, 147–156. doi: 10.3354/meps063147
Tsutsumi H. (2005). Production of planktonic and non-planktonic larvae in a single brood of Capitella sp. I and its implications for population persistence in disturbed environment due to organic enrichment of the sediments. Benthos. Res. 60, 17–24. doi: 10.5179/benthos1996.60.1_17
Tsutsumi H., Kikuchi T. (1984). Study of the life history of Capitella capitata (Polychaeta: Capitellidae) in amakusa, south Japan including a comparison with other geographical regions. Mar. Biol. 80, 315–321. doi: 10.1007/BF00392827
Tsutsumi H., Kikuchi T., Tanaka M., Higashi T., Imasaka K., Miyazaki M. (1991). Benthic fauna succession in a cove organically polluted by fish farming. Mar. Pollut. Bull. 23, 233–238.
Tsutsumi H., Kinoshita K., Srithongouthai S., Sato A., Nagata S., Inoue A., et al. (2005). Treatment of the organically enriched sediment below the fish farm with the biological activities of artificially mass-cultured colonies of a small deposit feeding polychaete, Capitella sp. I. Benthos. Res. 60, 25–38. doi: 10.5179/benthos1996.60.1_25
Tsutsumi H., Montani S. (1993). Utilization of biological activities of capitellid polychaete for treatment of “hedoro” (organically enriched sediment) deposited on the marine bottom below fish net pen culture. Nippon. Suisan. Gakkaishi. 59, 1343–1347. doi: 10.2331/suisan.59.1343
Ueno S., Yamamoto G. (1982). Physiological tolerance in the polychaetous annelids Capitella capitata and Paraprionospio pinnata. Benthos Res. 23, 60–68.
Volkenborn N. (2007). Effects of bioturbation and bio irrigation by lugworms (Arenicola marina) on physical and chemical sediment properties and implications for intertidal habitat succession. Estuar. Coast. Shelf. Sci. 74, 331–343. doi: 10.1016/j.ecss.2007.05.001
Warren L. M. (1991). Problems in capitellid taxonomy. the genera Capitella, Capitomastus, and Capitellides (Polychaeta). Ophelia Suppl 5, 272–282.
Keywords: Capitella aff. teleta, population dynamics, lagoon, water level, planktonic larvae, restoration work
Citation: Hashimoto A and Sato-Okoshi W (2022) Population dynamics of Capitella aff. teleta (Polychaeta, Capitellidae) in Gamo Lagoon, northeastern Japan, during a series of restoration works following the 2011 Great East Japan Earthquake and tsunami. Front. Mar. Sci. 9:1001925. doi: 10.3389/fmars.2022.1001925
Received: 24 July 2022; Accepted: 27 September 2022;
Published: 31 October 2022.
Edited by:
Victoria Louise Georgia Todd, Ocean Science Consulting Ltd., United KingdomReviewed by:
Jongseong Ryu, Anyang University, South KoreaAlvar Carranza, Universidad de la República, Uruguay
Copyright © 2022 Hashimoto and Sato-Okoshi. This is an open-access article distributed under the terms of the Creative Commons Attribution License (CC BY). The use, distribution or reproduction in other forums is permitted, provided the original author(s) and the copyright owner(s) are credited and that the original publication in this journal is cited, in accordance with accepted academic practice. No use, distribution or reproduction is permitted which does not comply with these terms.
*Correspondence: Waka Sato-Okoshi, waka.sato-okoshi.d3@tohoku.ac.jp