- 1Australian Institute of Marine Science, Townsville, QLD, Australia
- 2School of Science, Technology and Engineering, University of the Sunshine Coast, Maroochydore, QLD, Australia
- 3University of Rhode Island, Kingston, RI, United States
- 4MEGA Lab, University of Hawaii at Hilo, Hilo, HI, United States
- 5Scripps Institution of Oceanography, University of California, San Diego, San Diego, CA, United States
- 6Faculty of Science, School of Life and Environmental Sciences, The University of Sydney, Sydney, NSW, Australia
Editorial on the Research Topic
Advances in 3D Habitat Mapping of Marine Ecosystem Ecology and Conservation
Advances in 3D technology have enabled low-cost and accurate measurements of habitat structure and organism size in both terrestrial and marine environments. However, there is still a need for guidance on how to apply novel 3D technologies for marine ecology and conservation. Multiple teams from around the world are leading the application of 3D photogrammetry in marine ecosystems. The widespread adoption of 3D methodologies produces a growing need for agreed standards to assess the quality of 3D data (e.g., error metrics). Similarly, standardized techniques where possible, will ensure collaboration and compatibility of 3D data across space and time.
This Research Topic is a first step toward the standardization of methods and communication of the state of the field to the wider audience in marine science and conservation using or considering the use of 3D technologies. The Topic provides relevant information that: (1) defines standard methods for the application of 3D technologies to marine ecosystem ecology and conservation, (2) advances fundamental marine ecological and conservation knowledge relevant to the habitat structure of marine ecosystems; and (3) highlights knowledge gaps and directions to move toward a high-resolution 3D map of the world's oceans.
The Research Topic focuses on the use of high-resolution 3D reconstructions of underwater ecosystems. It includes 13 articles, of which six are original research articles, one is a perspective, and six are methodological advances. Most of the original research articles also included a methodological validation or comparison. The geographic scope of this Research Topic ranges from the Caribbean to Greenland to the Pacific Islands and Australasia. Nine articles were conducted in coral reefs, three in temperate rocky reefs, and one on a deep-sea cliff, which highlights the versatility of 3D technologies. The diverse group of articles explored the relationship between habitat features, benthic and fish abundance, health, diversity, the potential of reef scape genomics, and the effectiveness of marine protection. A range of equipment from action cameras and DSLRs, to machine vision cameras, underwater robots, and echo-sounders were used to generate 3D reconstructions, emphasizing how a broad range of 3D technologies can benefit a wide variety of end users and applications. Similarly, the breath of the research published in this Research Topic captured 3D reconstructions of sub-millimeter resolutions, as well as 3D maps across large spatial extents.
Key Findings in Original Research Articles
Advancing Fundamental Marine Ecological and Conservation Knowledge
Across large spatial extents, 3D technologies were used to characterize structural complexity and investigate its drivers and representation within marine parks. Asner et al. mapped reef rugosity (2 and 6 m resolution) to 22 m depth throughout the eight main Hawaiian Islands, and found rugosity was mainly driven by water depth and reef slope. Mayorga-Martínez et al. mapped slope, aspect, curvature, rugosity, and ruggedness (2.5 m resolution) for three submerged banks and two emerging reefs in the Southwestern Gulf of Mexico and found structural complexity increased with depth and was underrepresented within marine parks.
Three-dimensional technologies were used to investigate the relationship of surface rugosity and more traditional metrics of ecosystem health and state at the scale surveys are routinely conducted (hundreds of squared meters). Urbina-Berreto et al. explored the relationship between structural complexity and coral cover on reef slopes and lava-flows and found sites with the highest coral cover also had the highest structural complexity. Monfort et al. investigated the relationship between fish assemblages and surface rugosity in Mediterranean shallow rocky reefs and found total fish abundance and species richness increased with surface rugosity. Kanki et al. investigated the relationships between the prevalence of four benthic sessile organisms (ascidian, barnacle, polychaete, and articulated coralline algae) and terrain variables derived from 3D maps of the seafloor (cm resolution, 4 m2 extent). Filter-feeders were more abundant on vertical and/or high faces above the seafloor, likely due to higher current velocities. In contrast, algae occurred at various heights and on gentle slopes suitable for photosynthesis.
At colony scales, Richaume et al. investigated the effect of longevity of no-take zones on populations of red coral (Corallium rubrum) in France, by using photogrammetry to measure morphometrics. They found after 5-years under protection red coral colonies were taller and had more branches inside no-take zones, the difference persisted after 40 years. Million et al. explored relationships between linear extension and growth metrics in the staghorn coral (Acropora cervicornis) using photogrammetry. They found growth in higher order metrics was not a linear function of growth in branch length and concluded the use of early growth to predict future performance of corals is limited.
Methodological Enhancements Over Traditional Metrics
Several studies independently compared traditional methods with photogrammetry, unanimously concluding that metrics derived using 3D technologies are comparable to traditional metrics. Specifically, several studies agreed that photogrammetric methods can provide data with higher precision, lower error, extraction of additional and useful metrics, and improved representativeness. Limitations of 3D technologies included its effectiveness for species-level taxonomic identification, a requirement for initial investment and a steep learning curve. However, photogrammetric methods were also regarded as more efficient once established.
Million et al. developed a photogrammetry processing pipeline for fragments of staghorn coral. 3D models were built with Agisoft Metashape, which was automated to run on a high-performance computing system to serially process models. They showed photogrammetry is an information rich method for quantifying colony-level morphometrics and is compatible with field measurements. Couch et al. compared data from in-water surveys to photogrammetry-derived metrics for assessing coral demography, bleaching, and diversity. They concluded most metrics did not vary significantly between methods regardless of the habitat type or depth, and that photogrammetry offers a unique opportunity to quantify and mitigate inter-observer error rigorously. Urbina-Berreto et al. compared field and digital estimates of coral cover using the Line Intercept Transect (LIT) method and found in situ estimates were higher than digitized estimates. They also performed surface analyses on the orthomosaics to quantify coral cover and found these yielded the most precise estimates. They concluded surface analysis was the most efficient method and outperformed other methods in terms of data outputs and representativeness of the ecosystem. Conley and Hollander found no significant difference in surface area measurements derived using traditional methods (wax dipping, geometrical calculation, and buoyant weight) and photogrammetry. They used this to characterize the relationship between buoyant weight and surface area over time for the coral species Stylophora pistillata.
Several studies published in this Research Topic presented 3D approaches to derive novel metrics, maximize limited bottom-time, and explore difficult-to-access environments. Kanki et al. proposed a new photogrammetric method to 3D map the seafloor (cm resolution, 4 m2 extent) and quantify terrain variables and the prevalence of sessile organisms. Macedo Cruz de Oliveira et al. compared methods for classification of marine organisms from photogrammetric reconstructions of cold-water coral habitats, and found 3D support vector machine outperformed other methods, but each method explored has advantages for specific applications. Van Audenhaege et al. demonstrated a novel approach for mapping and classifying vertical habitats (e.g., cliffs, drop-offs) that are difficult to access and challenging to study. Hatcher et al. developed and tested a new mapping platform (SQUID-5), capable of 2.5D mapping complex coral reef habitats (between 3 and 9 m of depth) and is capable of measuring changes in the morphology and location of seafloor features over time (~3 cm resolution) without pre-existing ground controls. Finally, Bongaerts et al. discussed the potential of “reef-scape genomics” that uses recent advances in underwater 3D technology to enable spatially explicit molecular ecology studies on coral reefs.
Challenges as We Move Toward a 3D Map of the World's Oceans
Future applications of 3D mapping, based on photogrammetry or other technologies, should meet standard requirements that allow comparisons across environments, sensors, and processing approaches and ensure a robust assessment of the product's quality and uncertainties. Following the recommendations by James et al., we suggest that: (1) the selection of sensors and methods is clearly described and justified as fit for purpose, (2) error reporting includes both the performance of the model fitting and, importantly, the quality of the 3D product is assessed using independent measurements, and (3) uncertainties (e.g., in bias or precision) are explicitly acknowledged and managed, especially when performing change detection analyses.
Despite the important contribution of this Research Topic toward a future 3D map of the world's oceans, several important questions remain unanswered. Especially in regard to good practices when scaling across datasets of different spatial resolutions (e.g., grain size) and extents (e.g., window size), defining the key parameters to compare datasets from different sources (e.g., echosounder vs. cameras), and the potential and limitations of machine learning for automatic segmentation and classification of 3D data (Figure 1).
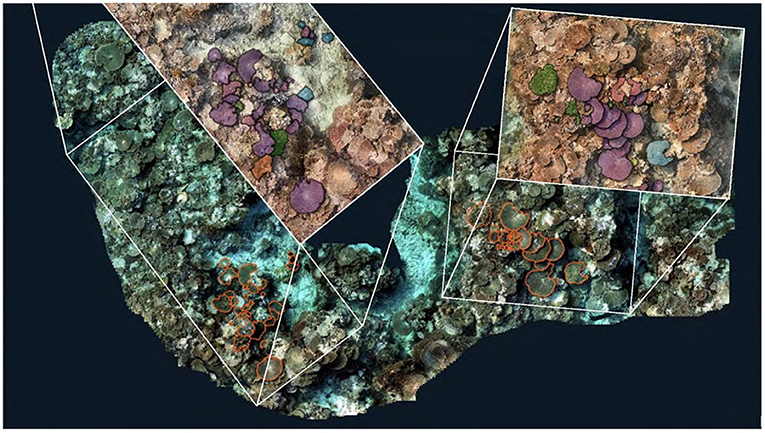
Figure 1. Example of two datasets collected with different platforms over the same area of a coral reef. The larger dataset was collected using action cameras and has a ground sampling distance of approximately 1 cm, the smaller excerpts were collected with a digital single-lens reflex camera and has a ground sampling distance of approximately 1 mm.
Author Contributions
RF conceived the Research Topic, invited guest editors, read all articles, summarized, analyzed the research findings across the Research Topic, and wrote this editorial. All other authors reviewed manuscript and contributed equally to editing and reviewing several articles submitted for publication in Research Topic.
Conflict of Interest
The authors declare that the research was conducted in the absence of any commercial or financial relationships that could be construed as a potential conflict of interest.
Publisher's Note
All claims expressed in this article are solely those of the authors and do not necessarily represent those of their affiliated organizations, or those of the publisher, the editors and the reviewers. Any product that may be evaluated in this article, or claim that may be made by its manufacturer, is not guaranteed or endorsed by the publisher.
Acknowledgments
We appreciate the help of Tiny Remmers Barry to source the panels for the figure used in this editorial and to the authors of all articles published in this Research Topic. RF and MG-R were funded through the Reef Restoration and Adaptation Program and other internal projects within the Australian Institute of Marine Science. AD was supported by the USDA National Institute of Food and Agriculture, Hatch Formula project accession no. 1017848.
Keywords: 3D structural complexity, photogrammetry, rugosity, standardization, error reporting, cross-platform, marine ecology, structure-from-motion
Citation: Ferrari R, Leon JX, Davies AJ, Burns JHR, Sandin SA, Figueira WF and Gonzalez-Rivero M (2022) Editorial: Advances in 3D Habitat Mapping of Marine Ecosystem Ecology and Conservation. Front. Mar. Sci. 8:827430. doi: 10.3389/fmars.2021.827430
Received: 02 December 2021; Accepted: 21 December 2021;
Published: 21 January 2022.
Edited by:
Wei-Bo Chen, National Science and Technology Center for Disaster Reduction (NCDR), TaiwanReviewed by:
Lida Teneva, Independent Researcher, Sacramento, United StatesCopyright © 2022 Ferrari, Leon, Davies, Burns, Sandin, Figueira and Gonzalez-Rivero. This is an open-access article distributed under the terms of the Creative Commons Attribution License (CC BY). The use, distribution or reproduction in other forums is permitted, provided the original author(s) and the copyright owner(s) are credited and that the original publication in this journal is cited, in accordance with accepted academic practice. No use, distribution or reproduction is permitted which does not comply with these terms.
*Correspondence: Renata Ferrari, R.FerrariLegorreta@aims.gov.au