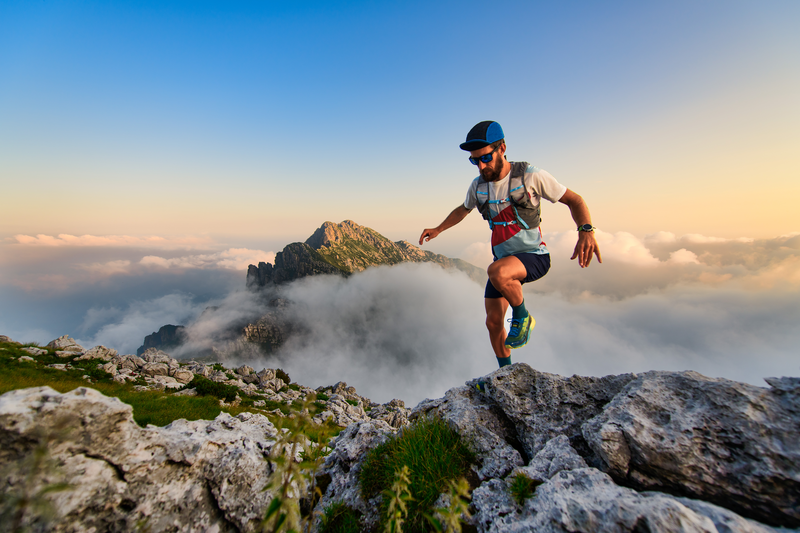
94% of researchers rate our articles as excellent or good
Learn more about the work of our research integrity team to safeguard the quality of each article we publish.
Find out more
OPINION article
Front. Mar. Sci. , 20 December 2021
Sec. Marine Fisheries, Aquaculture and Living Resources
Volume 8 - 2021 | https://doi.org/10.3389/fmars.2021.813091
Shrimp farming is growing worldwide, with a key driving factor being its high value in global seafood markets. In 2018, the first-sale value of farmed crustaceans was 69,300 million USD, in which Penaeus (Litopenaeus) vannamei was the most cultivated species with an annual growth rate of 8.78% (FAO, 2020). As a result, the importance of farmed shrimp as a source of food, employment, and economic development is indisputable. The increasing demand and shortage of resources, such as land and water, have pushed the industry toward intensifying shrimp farming (Xu et al., 2021). However, conventional intensive farming practices with high water exchange rates can lead to disease outbreaks and crop losses (Thitamadee et al., 2016). In this sense, the current trend suggests an increased demand for more “controlled intensive systems” with increased efficiency and biosecurity.
In large production areas (e.g., Asia and some Latin American countries), Biofloc Technology (BFT) became an alternative to overcome these issues, providing increased predictability and consistency (El-Sayed, 2021). The BFT contributes to nutrient optimization as studies reported that bioflocs could contribute to ~20% of the protein required by shrimp (Avnimelech, 2012). Burford et al. (2004) suggested that up to 29% of daily nitrogen retention of the shrimp was sourced by the natural biota (bioflocs). These microbial aggregates also provide vitamins, bioactive compounds, and beneficial bacteria (probiotics) (Yu et al., 2021). Under suitable conditions, BFT improves feed efficiency, stimulates growth, enhances the immune system (Hostins et al., 2019), and promotes a better physiological status compared to shrimp farmed in traditional systems (Cardona et al., 2016).
Nowadays, after ~20 years of evolution, BFT has been adapted and adjusted to different regions, locations, salinities, applied to one single-phase (nursery) or multiple phases, outdoor and indoor conditions, and according to different farm infrastructure, operational and resources limitations. The accumulated BFT knowledge has provided a baseline for the development of other related microbial-based (e.g., Aquamimicry, synbiotics, and semi-biofloc; Zeng et al., 2020; El-Sayed, 2021; Hussain et al., 2021), hybrid (e.g., BioRAS; Xu et al., 2020), and integrated (e.g., shrimp + fish) systems (Wright, 2015), with at least nine different variations. However, regardless of the system chosen and adopted, it is crucial to consider the local specificities (e.g., technological, social, financial, and environmental), tailoring day-to-day management practices and ongoing production strategies accordingly. In addition, considering the higher production costs compared to traditional low-input systems, the commercialization and post-harvest aspects are crucial to guarantee the resilience and competitiveness of BFT-based shrimp farms. In this opinion manuscript, we describe some key BFT characteristics regarding its past and present, while highlighting future challenges and opportunities aiming to contribute to the sustainability of intensive shrimp farming.
The BFT system originated in the 1970s at the French Research Institute for Exploitation of the Sea (IFREMER) located in Tahiti, French Polynesia. It was later expanded to commercial shrimp farms in partnership with private sector companies from the U.S. In the 1990s and early-2000s, R&D efforts and commercial adoption began in the U.S. and Central America enabling further development of commercial large-scale shrimp operations, including Asia and Latin America (Burford et al., 2004; Ulloa-Walker et al., 2020).
However, by that time, the lack of understanding of the different components (e.g., engineering, production management, and aquatic microbiology) led to poor system control. As a result, imbalance of the microbial population and toxic N-compounds issues were commonly observed, as well as the excessive concentration of suspended solids (Ray et al., 2010), poor alkalinity (Furtado et al., 2015), and C:N ratio management (Panigrahi et al., 2019). During the evolution process, a wide variety of designs, conditions, strategies, and equipment have been evaluated, including aeration systems (Lara et al., 2017); clarification methods (Ray et al., 2010); water depths (Krummenauer et al., 2016), indoor and outdoor conditions (Xu et al., 2021), and stocking densities (Krummenauer et al., 2011; Da Silveira et al., 2020). Several training courses were carried out, and the trained human resources from the 1990s and more recently from the 2000s, now spread globally, supported the BFT expansion and the implementation of various commercial operations worldwide (Ulloa-Walker et al., 2020).
In the past, BFT was mostly restricted to universities and research centres. Nowadays, it has become consolidated as a production system in many countries. Locations once considered unsuitable for shrimp farming are now starting to produce in indoor closed systems (e.g., Germany and Canada), mainly due to the advances in technology over previous years in genetics, nutrition, engineering, and management in particular (El-Sayed, 2021). Currently, BFT has been applied in the nursery (especially in the Americas and, more recently, Asia) and grow-out phases. Stocking densities impact the shrimp harvest size (Krummenauer et al., 2011) and may influence the operational risks (e.g., disease outbreaks) and production costs. Large commercial operations normally adopt conservative stocking densities, whereas small indoor “boutique farms” tend to stock with higher densities aiming to optimize resources/infrastructure (Figure 1). In terms of shrimp performance, water quality parameters in optimal levels, proper feed management, and desirable microbial profile of the water are key points in BFT. Moreover, the use of high-quality diets associated with automatic feeders (promoting better spatial and temporal feed distribution) has improved growth and uniformity. Similarly, water probiotics (Hostins et al., 2019), and feed additives such as organic acids (Da Silva et al., 2016), probiotics (Kesselring et al., 2019), prebiotics, and immunostimulants (Zhou et al., 2020) have been contributing to the improvement of shrimp health and diseases resistance.
Figure 1. Different scales currently utilized in commercial biofloc-based shrimp farming: large-scale farm in Vietnam, Asia (left); and small-scale “boutique close to market” farm in Brazil, Latin America (right).
Regarding water quality, new aeration systems are emerging (Susanti et al., 2021) but still need validation at a larger scale. Although vastly improved, issues with N-compounds and management of suspended solids persist in some farms leading to poor growth, low DO levels, pathogenic vibrio outbreaks, and eventually mortalities. In terms of solids, some strategies have been adopted in small operations, e.g., clarifiers (Ray et al., 2010), RAS filtration systems and skimmers (Fleckenstein et al., 2020); and in large-scale farms, the solids are concentrated and removed through “shrimp toilets” by limited water exchanges (Afroz and Alam, 2013). Regardless of the strategy, proper waste management is a current challenge. Additionally, other production strategies have been implemented and led to an increase in actual production rates; for example, the maintenance of alkalinity within optimal levels (Furtado et al., 2015), adoption of partial harvests (Da Silveira et al., 2022), artificial substrates (Legarda et al., 2018) and “bacterial-rich” inoculums from a mature tank or pond (Ferreira et al., 2020). These processes can optimize the use of resources, speed up and maintain the nitrification process and improve the water quality parameters. Unfortunately, not all these practices have been fully adopted at a commercial level, mostly due to the lack of knowledge or logistics issues scaling them up into large operations.
From an educational perspective, the farm technicians are usually receptive to adopting new practices, but ongoing training and incorporation of specialized personnel are often required. An example of a lack of training was the “brown water” nursery system in Ecuador. The “brown water” refers to BFT heterotrophic-based (mostly using molasses and commercial probiotics to control ammonia) operated in small indoor lined concrete tanks. Stoichiometric calculations were poorly applied in some cases, leading to N-compounds issues, and therefore high water exchange rates were frequently observed. As a result, several nursery facilities were abandoned, unsatisfactory growth performance was observed, and most farmers switched to “green water” earthen pond-based nurseries (Ching et al., 2020). On the other hand, higher rates of BFT adoption (or similar microbial-based approaches) have been observed in Brazil, Peru, Guatemala, and Mexico, particularly during the nursery phase. In Asia, Vietnamese intensive farms seem to be very adaptable to new microbial-based protocols applied to nursery and grow-out phases (Arnold et al., 2020; Boaventura et al., 2020).
Past and present are supporting the BFT future development. Although such technique was conceived to close the gap toward sustainable aquatic production, constraints such as high energy costs and proper management of the residual solids remain unsolved (Ray et al., 2010). For that, alternative processes and energy sources (Badiola et al., 2018), Integrated Multi-trophic Aquaculture (IMTA) (Poli et al., 2019; Holanda et al., 2020; Legarda et al., 2021), and new recycling options for the wastes generated (solid and liquid fractions) could enhance the circularity of BFT (Bauer et al., 2012), ultimately generating “green-labelable” products and promoting the creation of startups focused on sustainable solutions (Subasinghe et al., 2009). Likewise, new sensor technologies and data management & cloud systems coupled with machine learning, smart feeding technologies (e.g., hydroacoustic), and management decision support tools are examples of precision farming solutions that could contribute to a more functional BFT system (Rahman et al., 2021; Reis et al., 2022). Nevertheless, adoption at scale and economics analysis will likely determine the feasibility of such technologies.
Efficient microbial management is mandatory in BFT. In this sense, biotechnology tools and particularly the “omics” sciences will be required to identify and understand the microbial properties forming bioflocs, including the microbiota, mycobiota, and phytobiota. The biofouling process, the interaction between the microbes, the quorum sensing, and other communications processes are practical examples that must be better understood (Dobretsov et al., 2009). Indeed, the screening of microbial profiles could be connected with management decision support tools and apps, and help to control better and manipulate these communities to achieve improved biofloc nutritional quality, pathogen control, and production outcomes. In addition, information about the effect of bioflocs on shrimp quality is still scarce. Initial studies documented that biofloc consumption did not impact the post-harvest sensorial attributes (Martinez-Porchas et al., 2020). Moreover, tailored feeds, broader access to postlarvae from BFT-tailored breeding programs and BFT applied to other penaeid species are also expected. An extensive review done by Ulloa-Walker et al. (2020) described and highlighted promising results with non-L. vannamei species in nursery, grow-out, and broodstock conditions. However, few initiatives have been reported at larger scales, and future adoptions are foreseen, especially in the nursery phase.
Biofloc technology (hybrid and other microbial-based systems) can widely contribute to the sustainable intensification of shrimp production. The technique evolved from an experimental system to become a large-scale production system applied in many countries, particularly in the nursery phase. During this journey, a better understanding of the system, including engineering, roles of microbial communities, water quality, and feed management, has allowed for a more comprehensive and predictable environment (Table 1). In the future, scalability and incorporating circular economy concepts might help overcome some existing issues, reducing the carbon footprint and environmental impacts. In addition, more examples of industry-academia collaborations are needed and expected.
The COVID 19 pandemic forced shrimp farmers globally to be much more efficient in managing production costs. Biofloc technology still has a high production cost when compared to traditional (earthen pond) systems. Especially in medium-large operations, the adoption of economic modellings and sensitivity analysis, precision farming technologies, holistic health monitoring, and improved biosecurity protocols will help identify opportunities to reduce costs and production-associated risks, improving the system predictability, competitiveness, and resilience.
ME: article conceptualization, writing, and review. AM-B, MM-P, MP, and FV: writing and review. All authors contributed to the article and approved the submitted version.
The authors declare that the research was conducted in the absence of any commercial or financial relationships that could be construed as a potential conflict of interest.
All claims expressed in this article are solely those of the authors and do not necessarily represent those of their affiliated organizations, or those of the publisher, the editors and the reviewers. Any product that may be evaluated in this article, or claim that may be made by its manufacturer, is not guaranteed or endorsed by the publisher.
We gratefully acknowledge the work of our numerous students, technical staff, industry collaborators, and colleagues who have contributed to the body of knowledge related to the biofloc technology in shrimp farming.
Afroz, T., and Alam, S. (2013). Sustainable shrimp farming in Bangladesh: a quest for an Integrated Coastal Zone Management. Ocean Coastal Manage. 71, 275–283. doi: 10.1016/j.ocecoaman.2012.10.006
Arnold, S., Emerenciano, M. G. C., Cowley, J. A., Little, B., Rahman, A., and Perrin, T. (2020). Collaboration drives innovations in super-intensive indoor shrimp farming. Glob. Seafood Alliance.
Avnimelech, Y. (2012). Biofloc Technology: A Practical Guide Book. Baton Rouge, Louisiana: The World Aquaculture Society.
Badiola, M., Basurko, O., Piedrahita, R., Hundley, P., and Mendiola, D. (2018). Energy use in recirculating aquaculture systems (RAS): a review. Aquac. Eng. 81, 57–70. doi: 10.1016/j.aquaeng.2018.03.003
Bauer, W., Prentice-Hernandez, C., Tesser, M. B., Wasielesky, W. Jr, and Poersch, L. H. (2012). Substitution of fishmeal with microbial floc meal and soy protein concentrate in diets for the pacific white shrimp Litopenaeus vannamei. Aquaculture 342, 112–116. doi: 10.1016/j.aquaculture.2012.02.023
Boaventura, M., Hetzel, G., Huynh Tran, C., Bakker, S., and Marc, C. (2020). A practical experience at a shrimp nursery system in Vietnam. Aquac. Asia Pac.
Burford, M. A., Thompson, P. J., Mcintosh, R. P., Bauman, R. H., and Pearson, D. C. (2004). The contribution of flocculated material to shrimp (Litopenaeus vannamei) nutrition in a high-intensity, zero-exchange system. Aquaculture 232, 525–537. doi: 10.1016/S0044-8486(03)00541-6
Cardona, E., Lorgeoux, B., Chim, L., Goguenheim, J., Le Delliou, H., and Cahu, C. (2016). Biofloc contribution to antioxidant defence status, lipid nutrition and reproductive performance of broodstock of the shrimp Litopenaeus stylirostris: consequences for the quality of eggs and larvae. Aquaculture 452, 252–262. doi: 10.1016/j.aquaculture.2015.08.003
Ching, C., Crespo, C., Pazmiño, A., and Ron, E. (2020). Sistemas Multifasicos: Una oportunidad para crecer. Boletin Nicovita.
Da Silva, B. C. E., Vieira, F. D. N., Mouriño, J. L. P., Bolivar, N., and Seiffert, W. Q. (2016). Butyrate and propionate improve the growth performance of Litopenaeus vannamei. Aquac. Res. 47, 612–623. doi: 10.1111/are.12520
Da Silveira, L. G. P., Krummenauer, D., Poersch, L. H., Fóes, G. K., Rosas, V. T., and Wasielesky, W. Jr. (2022). The effect of partial harvest on production and growth performance of Litopenaeus vannamei reared in biofloc technologic system. Aquaculture 546:737408. doi: 10.1016/j.aquaculture.2021.737408
Da Silveira, L. G. P., Krummenauer, D., Poersch, L. H., Rosas, V. T., and Wasielesky, W. Jr. (2020). Hyperintensive stocking densities for Litopenaeus vannamei grow-out in biofloc technology culture system. J. World Aquac. Soc. 51, 1290–1300. doi: 10.1111/jwas.12718
Dobretsov, S., Teplitski, M., and Paul, V. (2009). Mini-review: quorum sensing in the marine environment and its relationship to biofouling. Biofouling 25, 413–427. doi: 10.1080/08927010902853516
El-Sayed, A. F. M. (2021). Use of biofloc technology in shrimp aquaculture: a comprehensive review, with emphasis on the last decade. Rev. Aquacult. 13, 676–705. doi: 10.1111/raq.12494
FAO (2020). The State of World Fisheries and Aquaculture 2020: Sustainability in Action. Food and Agriculture Organization of the United Nations.
Ferreira, G. S., Silva, V. F., Martins, M. A., Da Silva, A. C. C. P., Machado, C., Seiffert, W. Q., et al. (2020). Strategies for ammonium and nitrite control in Litopenaeus vannamei nursery systems with bioflocs. Aquac. Eng. 88:102040. doi: 10.1016/j.aquaeng.2019.102040
Fleckenstein, L. J., Tierney, T. W., Fisk, J. C., and Ray, A. J. (2020). The effects of different solids and biological filters in intensive pacific white shrimp (Litopenaeus vannamei) production systems. Aquac. Eng. 91:102120. doi: 10.1016/j.aquaeng.2020.102120
Furtado, P. S., Poersch, L. H., and Wasielesky, W. (2015). The effect of different alkalinity levels on Litopenaeus vannamei reared with biofloc technology (BFT). Aquac. Int. 23, 345–358. doi: 10.1007/s10499-014-9819-x
Holanda, M., Santana, G., Furtado, P., Rodrigues, R. V., Cerqueira, V. R., Sampaio, L. A., et al. (2020). Evidence of total suspended solids control by Mugil liza reared in an integrated system with pacific white shrimp Litopenaeus vannamei using biofloc technology. Aquac. Rep. 18:100479. doi: 10.1016/j.aqrep.2020.100479
Hostins, B., Wasielesky, W., Decamp, O., Bossier, P., and De Schryver, P. (2019). Managing input C/N ratio to reduce the risk of acute Hepatopancreatic Necrosis Disease (AHPND) outbreaks in biofloc systems–A laboratory study. Aquaculture 508, 60–65. doi: 10.1016/j.aquaculture.2019.04.055
Hussain, A. S., Mohammad, D. A., Sallam, W. S., Shoukry, N. M., and Davis, D. A. (2021). Effects of culturing the Pacific white shrimp Penaeus vannamei in “biofloc” vs “synbiotic” systems on the growth and immune system. Aquaculture 542:736905. doi: 10.1016/j.aquaculture.2021.736905
Kesselring, J. C., Gruber, C., Standen, B., and Wein, S. (2019). Continuous and pulse-feeding application of multispecies probiotic bacteria in whiteleg shrimp, Litopenaeus vannamei. J. World Aquac. Soc. 50, 1123–1132. doi: 10.1111/jwas.12640
Krummenauer, D., Peixoto, S., Cavalli, R. O., Poersch, L. H., and Wasielesky, W. Jr. (2011). Superintensive culture of white shrimp, Litopenaeus vannamei, in a biofloc technology system in southern Brazil at different stocking densities. J. World Aquac. Soc. 42, 726–733. doi: 10.1111/j.1749-7345.2011.00507.x
Krummenauer, D., Poersch, L. H., Fóes, G., Lara, G., and Wasielesky, W. Jr. (2016). Survival and growth of Litopenaeus vannamei reared in BFT system under different water depths. Aquaculture 465, 94–99. doi: 10.1016/j.aquaculture.2016.09.002
Lara, G., Krummenauer, D., Abreu, P. C., Poersch, L. H., and Wasielesky, W. (2017). The use of different aerators on Litopenaeus vannamei biofloc culture system: effects on water quality, shrimp growth and biofloc composition. Aquac. Int. 25, 147–162. doi: 10.1007/s10499-016-0019-8
Legarda, E. C., Barcelos, S. S., Redig, J. C., Ramírez, N. C. B., Guimarães, A. M., Santo, C. M. D. E., et al. (2018). Effects of stocking density and artificial substrates on yield and water quality in a biofloc shrimp nursery culture. Rev. Bras. Zootecnia 47:e20170060. doi: 10.1590/rbz4720170060
Legarda, E. C., Da Silva, D., Miranda, C. S., Pereira, P. K. M., Martins, M. A., Machado, C., et al. (2021). Sea lettuce integrated with Pacific white shrimp and mullet cultivation in biofloc impact system performance and the sea lettuce nutritional composition. Aquaculture 534:736265. doi: 10.1016/j.aquaculture.2020.736265
Martinez-Porchas, M., Ezquerra-Brauer, M., Mendoza-Cano, F., Higuera, J. E. C., Vargas-Albores, F., and Martinez-Cordova, L. R. (2020). Effect of supplementing heterotrophic and photoautotrophic biofloc, on the production response, physiological condition and post-harvest quality of the whiteleg shrimp, Litopenaeus vannamei. Aquac. Rep. 16:100257. doi: 10.1016/j.aqrep.2019.100257
Panigrahi, A., Sundaram, M., Chakrapani, S., Rajasekar, S., Syama Dayal, J., and Chavali, G. (2019). Effect of carbon and nitrogen ratio (C:N) manipulation on the production performance and immunity of Pacific white shrimp Litopenaeus vannamei (Boone, 1931) in a biofloc-based rearing system. Aquaculture Res. 50, 29–41. doi: 10.1111/are.13857
Poli, M. A., Legarda, E. C., De Lorenzo, M. A., Martins, M. A., and Do Nascimento Vieira, F. (2019). Pacific white shrimp and Nile tilapia integrated in a biofloc system under different fish-stocking densities. Aquaculture 498, 83–89. doi: 10.1016/j.aquaculture.2018.08.045
Rahman, A., Xi, M., Dabrowski, J. J., Mcculloch, J., Arnold, S., Rana, M., et al. (2021). An integrated framework of sensing, machine learning, and augmented reality for aquaculture prawn farm management. Aquac. Eng. 95:102192. doi: 10.1016/j.aquaeng.2021.102192
Ray, A. J., Seaborn, G., Leffler, J. W., Wilde, S. B., Lawson, A., and Browdy, C. L. (2010). Characterization of microbial communities in minimal-exchange, intensive aquaculture systems and the effects of suspended solids management. Aquaculture 310, 130–138. doi: 10.1016/j.aquaculture.2010.10.019
Reis, J., Peixoto, S., Soares, R., Rhodes, M., Ching, C., and Davis, A. (2022). Passive acoustic monitoring as a tool to assess feed response and growth of shrimp in ponds and research systems. Aquaculture 546:737326. doi: 10.1016/j.aquaculture.2021.737326
Subasinghe, R., Soto, D., and Jia, J. (2009). Global aquaculture and its role in sustainable development. Rev. Aquac. 1, 2–9. doi: 10.1111/j.1753-5131.2008.01002.x
Susanti, L., Utomo, S. W., and Takarina, N. D. (2021). “Sustainability and feasibility assessments of nanobubble aeration technology in economic-socio environment of Penaeus vannamei shrimp farming,” in BIO Web of Conferences: EDP Sciences (Yogyakarta). doi: 10.1051/bioconf/20213305005
Thitamadee, S., Prachumwat, A., Srisala, J., Jaroenlak, P., Salachan, P. V., Sritunyalucksana, K., et al. (2016). Review of current disease threats for cultivated penaeid shrimp in Asia. Aquaculture 452, 69–87. doi: 10.1016/j.aquaculture.2015.10.028
Ulloa-Walker, D. A., Morales-Suazo, M. C., and Emerenciano, M. G. C. (2020). Biofloc technology: principles focused on potential species and the case study of Chilean river shrimp Cryphiops caementarius. Rev. Aquac. 12, 1759–1782. doi: 10.1111/raq.12408
Xu, W., Xu, Y., Su, H., Hu, X., Xu, Y., Li, Z., et al. (2021). Production performance, inorganic nitrogen control and bacterial community characteristics in a controlled biofloc-based system for indoor and outdoor super-intensive culture of Litopenaeus vannamei. Aquaculture 531:735749. doi: 10.1016/j.aquaculture.2020.735749
Xu, W., Xu, Y., Su, H., Hu, X., Yang, K., Wen, G., et al. (2020). Characteristics of ammonia removal and nitrifying microbial communities in a hybrid biofloc-RAS for intensive Litopenaeus vannamei culture: a pilot-scale study. Water 12:3000. doi: 10.3390/w12113000
Yu, Z., Dai, Z. Y., Li, L., Qin, G. X., and Wu, L. F. (2021). Dietary supplementation with biofloc promotes growth, improves immune and antioxidant status, and upregulates NF-κB/Nrf2 signalling molecules and stress resistance in Rhynchocypris lagowskii Dybowski. Aquac. Nutr. 27, 225–239. doi: 10.1111/anu.13180
Zeng, S., Khoruamkid, S., Kongpakdee, W., Wei, D., Yu, L., Wang, H., et al. (2020). Dissimilarity of microbial diversity of pond water, shrimp intestine and sediment in Aquamimicry system. AMB Express 10, 1–11. doi: 10.1186/s13568-020-01119-y
Zhou, L., Li, H., Qin, J. G., Wang, X., Chen, L., Xu, C., et al. (2020). Dietary prebiotic inulin benefits on growth performance, antioxidant capacity, immune response and intestinal microbiota in Pacific white shrimp (Litopenaeus vannamei) at low salinity. Aquaculture 518:734847. doi: 10.1016/j.aquaculture.2019.734847
Keywords: Litopenaeus vannamei, water quality, production costs, hybrid system, circular economy, microbial approaches, intensification, sustainability
Citation: Emerenciano MGC, Miranda-Baeza A, Martínez-Porchas M, Poli MA and Vieira FdN (2021) Biofloc Technology (BFT) in Shrimp Farming: Past and Present Shaping the Future. Front. Mar. Sci. 8:813091. doi: 10.3389/fmars.2021.813091
Received: 11 November 2021; Accepted: 23 November 2021;
Published: 20 December 2021.
Edited by:
Hüseyin Sevgili, Isparta University of Applied Sciences, TurkeyReviewed by:
Jia-Song Zhang, Chinese Academy of Fishery Sciences (CAFS), ChinaCopyright © 2021 Emerenciano, Miranda-Baeza, Martínez-Porchas, Poli and Vieira. This is an open-access article distributed under the terms of the Creative Commons Attribution License (CC BY). The use, distribution or reproduction in other forums is permitted, provided the original author(s) and the copyright owner(s) are credited and that the original publication in this journal is cited, in accordance with accepted academic practice. No use, distribution or reproduction is permitted which does not comply with these terms.
*Correspondence: Maurício G. C. Emerenciano, bWF1cmljaW8uZW1lcmVuY2lhbm9AY3Npcm8uYXU=
Disclaimer: All claims expressed in this article are solely those of the authors and do not necessarily represent those of their affiliated organizations, or those of the publisher, the editors and the reviewers. Any product that may be evaluated in this article or claim that may be made by its manufacturer is not guaranteed or endorsed by the publisher.
Research integrity at Frontiers
Learn more about the work of our research integrity team to safeguard the quality of each article we publish.