- 1School of Civil Engineering and Architecture, Zhejiang University of Science and Technology, Hangzhou, China
- 2School of Civil Engineering, Architecture and Environment, Hubei University of Technology, Wuhan, China
- 3College of Marine Sciences, South China Agricultural University, Guangzhou, China
- 4Guangxi Key Laboratory of Marine Natural Products and Combinatorial Biosynthesis Chemistry, Biophysical and Environmental Science Research Center, Institute of Eco-Environmental Research, Guangxi Academy of Sciences, Nanning, China
- 5Southern Marine Science and Engineering Guangdong Laboratory, Zhuhai, China
The existence of microplastics (MPs) poses a potential threat to the entire ecosystem and has gained wide public attention. As an essential source of aquatic products, aquaculture industries are inevitably subjected to the pollution of MPs, particularly when the plastic products are widely used in aquaculture. Even so, the identification of MPs in aquaculture is rarely reported. Hence, high-efficient analytical methods for accurate detection of MPs in the aquaculture environment are of utmost significance. This review comprehensively summarizes the analytical methods for MPs in aquaculture, including sampling, extraction, and qualitative and quantitative analyses of MPs. MPs are identified and quantified mainly by visual inspection, spectroscopy, or thermal analysis. In addition, this review also points out the limitations of these methods and the accuracy of quality control. Finally, the need for establishing standard methods is emphasized, and suggestions for future research are also proposed.
Introduction
The ever-increasing human population has generated a remarkable amount of plastic waste. According to the statistical analysis, the global production of plastics has reached 370 million tons by 2019 (PlasticsEurope, 2020). Plastics are widely utilized in all aspects of our daily life due to their low cost, durability, good ductility, and lightweight, resulting in the accumulation of plastic wastes in the environment, which are inevitably introduced into the ocean through various pathways. Therefore, the ocean may become an immense reservoir for plastic wastes. The natural decomposition of plastics is extremely slow. The plastic waste will be broken into small plastic pieces after physical, chemical, and/or biological action (Wright et al., 2013). Among these, plastics with particle size <5 mm are defined as “microplastics” (MPs) (Erni-Cassola et al., 2017), which was first proposed by Thompson et al. (2004).
In general, MPs are classified into primary MPs and secondary MPs (Figure 1). The primary MPs are derived from microbeads in cosmetics, cleaning products, and air-blasting media, which can directly enter the environment (Du and Wang, 2021). The secondary MPs are derived from the decomposition of larger plastic pieces. Pollution of MPs has gained significantly more research attention in recent years due to its persistence in the natural environment and potential adverse impacts on organisms. MPs can migrate in various environmental compartments, such as air (Dris et al., 2016), soil (Blaesing and Amelung, 2018), oceans (Andrady, 2011), and freshwater (Dris et al., 2015; Figure 2). They are easily ingested by organisms and translocated to higher trophic levels through food web. The exposure of MPs to humans aroused severe health concerns, encouraging to explore the removal techniques of MPs. At present, we have been plagued by the pollution of MPs. Therefore, techniques with high sensitivity and selectivity should be developed for the detection, identification, and localization of MPs. MPs can be detected either directly in environmental samples (e.g., aerosols, sediments, soils, and water) or within organisms originated from various habitats. So far, research on the detection of MPs in the aquatic environment has been conducted in some typical farms (Supplementary Table 1). These results exhibited that MPs have become a potential source of pollutants in the aquatic environment. Aquatic products are a significant source of human food. In recent years, world aquaculture production of cultured aquatic animals has grown steadily. To achieve the goal of sustainable aquaculture, some countries intend to produce more aquatic animals from aquaculture. Thus, aquaculture environments, such as ponds, lakes, rivers, and oceans, were inevitably contaminated by a plethora of pollutants including MPs that can be detrimental to the growth and development of aquatic organisms and, finally, end up in the human body through food chain, posing a serious threat to human health (Figure 3). In contrast, the review regarding the pollution of MPs in aquaculture systems is limited.
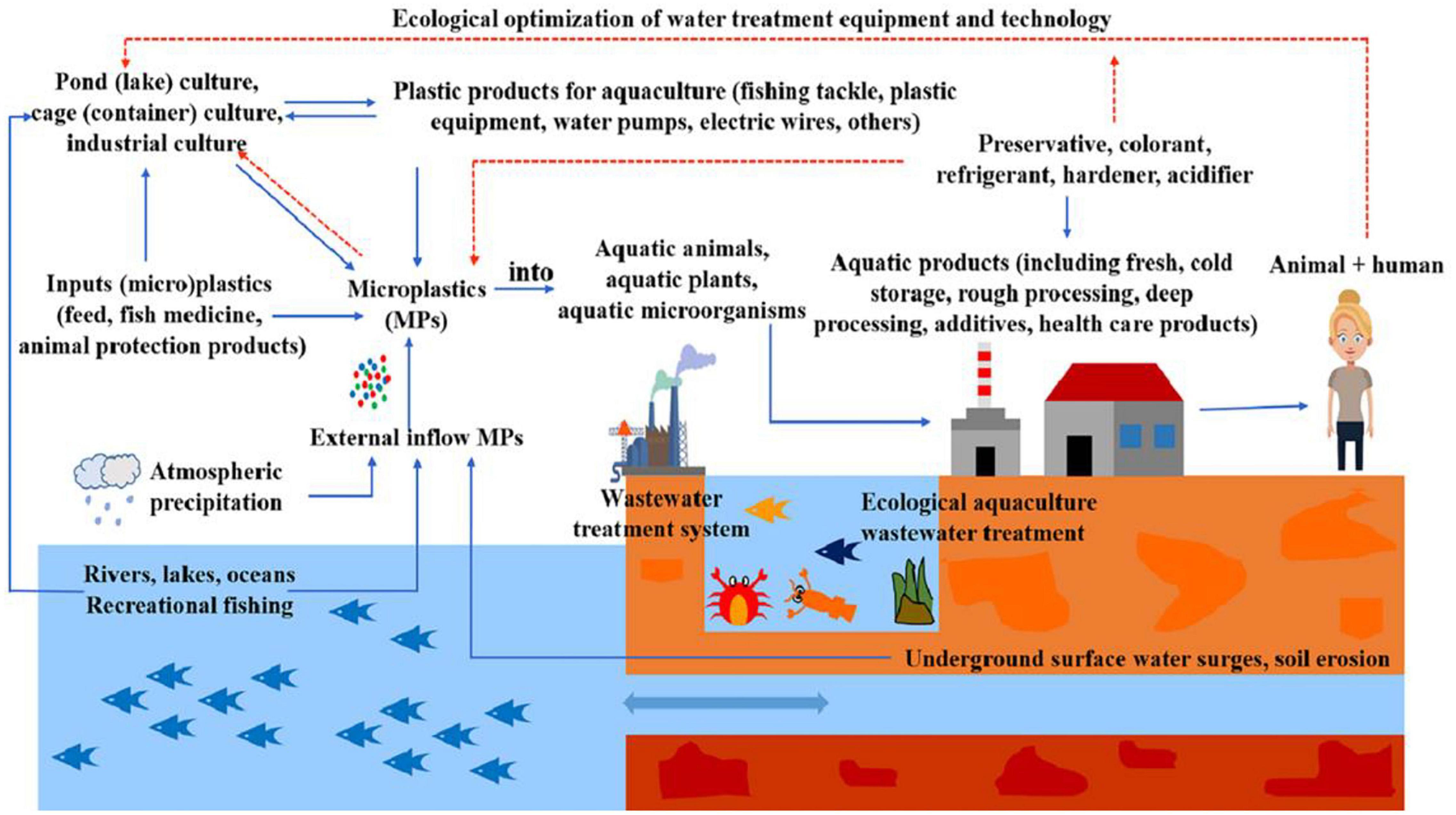
Figure 3. The migration of microplastics (MPs) in aquaculture system (reproduced with permission from Zhou et al., 2021).
To better map the abundance of MPs in the aquaculture environment and potential damage to organisms, future research should focus on the identification and quantification of MPs across the world. The analysis of MPs, including the sampling, preparation, and identification, has been reported (Figure 4). Nonetheless, to date, the standardized protocols for sample collection and subsequent analysis have not been proposed. At present, the approaches utilized to detect MPs in the aquaculture environment are rarely reported.
In summary, the goal of this review is to (1) present the most common method for the identification and quantification of MPs in the aquaculture system, (2) discuss the limitations, quality control, and quality assurance of these methodologies, and (3) propose the existing knowledge gaps and recommendations for future direction on the detection of MPs in the aquaculture environment.
Literature Search Strategy
A systematic literature review was performed to retrieve literature regarding the detection of MPs in aquaculture environment resulting from Google Scholar, Science Direct, Web of Science, PubMed, and other commonly used databases using the combination of keywords, including MPs, detection, identification, quantification, and aquaculture system. The obtained items were further refined to peer-reviewed research articles. The special keywords “microplastic and aquaculture” were applied as the key research criteria. We also examined and retrieved the reference list of each retrieved paper when it is necessary. Overall, research articles about the detection of MPs in the aquaculture environment were involved in this review.
Sampling Collection
Collection of samples is the first step of sampling methods of MPs. The most common sampling methods of MPs include selective sampling, volume-reduced sampling, and bulk sampling (Hidalgo-Ruz et al., 2012). MPs are directly extracted from samples by visual identification, which is defined as selective sampling. Volume-reduced sampling indicates that samples are filtered and sieved, and thus the target components can be used for additional analysis at the sampling location. Bulk sampling does not separate components on-site and keeps all samples. For large water body areas, static sampling is carried out at each sampling point, and filtering sample collection is generally selected. For example, Wang et al. (2017) used a precleaned 12 V DC Teflon pump to collect 20 L of surface water sample (0–20 cm in depth) and then the samples obtained were passed through a 50-μm stainless steel sieve.
Microplastics in aquaculture environment can be collected from the water surface or the water column at certain depths (Wang and Wang, 2018). For surface water sampling, neuston nets, and manta trawls are the most commonly utilized tools, but for water column sampling, near-bottom trawls, multiple opening–closing nets, continuous plankton recorders, bongo nets, and plankton nets are the main tools. Some alternative equipment are occasionally applied in surface water or water column sampling for MPs, such as water intake pumps, water collection bottles, or plankton traps. The smaller the size of the mesh, the smaller the particle size of the collected MPs (Uddin et al., 2020). The mesh can be blocked by algae or organisms if the aperture size becomes small. However, when the aperture size was increased, the toxic and small plastic particles may not be collected. In the aquatic environment, the depth of the water should be considered for sampling, and it is generally acknowledged that the abundance of MP surface is higher than that from water depth of 1–2 m. The used trawl nets varied between sampling depths. Samples from surface water used trawl-type sampling devices such as Manta trawl (Ta and Babel, 2020a,b). Bongo nets for mid-water samples and benthic trawls for deeper water from the bottom layer were applied. Typically, the mesh apertures most commonly used for the net are 333–335 μm (Du et al., 2021; Tirkey and Upadhyay, 2021).
Separation and Purification
Density Separation
Microplastics tend to float on the water surface due to their lower density than water. The target component and impurities can be separated by density flotation according to their density differences. To be specific, for density separation, the flotation solution was added to the sample, and then MPs were collected through a series of processes such as stirring, mixing, standing, and settling, and finally, the supernatant was separated. The density of most MPs is in the range of 0.80–1.40 g/cm3 (Ivleva et al., 2017). Generally speaking, MPs with a density of 1.40 g/cm3 can be obtained using a flotation solution.
The solution of NaCl is used extensively for the separation of MPs because it is cheap, readily available, green, and non-toxic (Eerkes-Medrano et al., 2015; Ma et al., 2020). Other flotation solutions are more efficient but limited to their expensive (SPT, NaI, etc.) or may pose a threat to the environment (ZnCl2, etc.) (Ivleva et al., 2017). In recent years, researchers have developed several MP separators based on density separation to improve their recovery rate by optimizing flotation patterns (Imhof et al., 2012; Nuelle et al., 2014; Karlsson et al., 2017). Moreover, Imhof et al. (2012) successfully developed a new device that can separate MPs <20 μm. Studies on the salt solutions for polymer separation are summarized in Supplementary Table 2.
Filtration and Sieving
Filtering or sieving is the most commonly used approach for separating the supernatant containing MPs from sediment samples and MPs from density separation of water samples. However, there exist some differences. For example, for filtration, the MPs onto filter membrane are obtained using a vacuum pump (Su et al., 2016), and sieving is performed directly onto screens with different pore sizes through gravity (Baldwin et al., 2016). The particle size of MPs collected depends on the size of the sieve and filter apertures. Generally speaking, the pore size (0.45–2 μm) of the filter membrane is smaller than that of the screen (Desforges et al., 2015; Fok and Cheung, 2015; Kim et al., 2015).
However, for the screen, some disadvantages, including severe blockage, its inapplicability to a wide range of sizes, and time-consuming (Mai et al., 2018), still exist. The separation efficiency of MPs can be improved by screens with various aperture sizes. However, the filtration may face the disadvantage that MPs may adhere tightly to the filter membrane, and it is difficult to remove the MPs. To address this issue, Hoffman and Turner (2015) found that C3H8O (50%, V/V) was a suitable detergent for the removal of components onto the filter membrane.
Digestion
Environmental samples contain biological materials that are often confused with MPs, resulting in the overestimation of environmental concentrations and increasing the number of MPs subjected to further analysis. The objective of digestion is to remove organic impurities that interfere with the identification of MPs. It is widely used in the preparation of biological, sewage, and sludge samples. Three common methods are used for the sample pretreatment, such as enzymatic digestion, alkaline digestion, and acid digestion. In particular, for enzymatic digestion, which is a time-consuming process, each enzyme works under its optimal temperature and pH condition, which must be monitored and retained through the experiment (Tirkey and Upadhyay, 2021). Wang et al. (2019) first extracted the MPs, then treated the dried sample with 30% H2O2 to digest the organic matter, and finally suspended and filtered the MPs by adding a saturated NaCl solution. Anderson et al. (2017) found that the solution containing H2O2 and Fe2+ was more beneficial to the oxidation of organic compounds. Researchers applied different kinds of digestion solutions including 100 g/L KOH, 10 mol/L NaOH, 30% H2O2, 69% HNO3, HNO3:HCl (1:1, V/V), and HNO3:HClO4 (4:1, V/V) to digest MPs, and they concluded that KOH (100 g/L, 60°C) posed severe damage to MPs (Zou et al., 2019).
Microplastic Identification
Visual Observation
Visual observation is suitable for plastic particles with large size (>1 mm) (Song et al., 2015). MPs are manually identified and sorted before being counted according to their physical characteristics (e.g., color, shape, and hardness). With the help of optical microscopes, electron microscopes, and scanning probe microscopes, it is possible to obtain more information on MPs. For example, scanning electron microscopy (SEM) can provide extremely high magnification and clear images with a resolution of up to 0.1 μm, which is capable of distinguishing MPs from organic particles. Ribeiro-Claro et al. (2017) believed that particles of MPs with various shapes (e.g., irregular polyhedral, hexagons, spheres, and fibers) and sizes can be accurately determined by SEM. Patterson et al. (2019) initially identified MPs from the Tuticorin coast, Gulf of Mannar, Southeastern India, by using stereomicroscope and then verified the composition of the polymers by Fourier transform infrared (FTIR)-attenuated total reflection (ATR). These results showed that polyethylene (PE) fiber (0.25–0.5 mm) is most common in oysters and seawater. Zhu et al. (2019) characterized the morphology of MPs with the assist of a stereomicroscope and then identified some plastics-like using μ-FTIR. They found that MPs were abundant in the Maowei Sea, a typical mariculture bay.
This method has the advantages of simple operation, low cost, and non-toxic. However, there are many substances similar to MPs in the environment, which readily cause misjudgment (Eriksen et al., 2013). Nile red (NR) can enhance the recognition efficiency. For example, Shim et al. (2016) demonstrated that MPs can be readily identified using an NR solution (5 mg/L) in n-hexane that can effectively dye plastics.
Fourier Transform Infrared
Three modes including ATR (Klein et al., 2015; Mani et al., 2015; Imhof et al., 2017), reflection (Harrison et al., 2012; Vianello et al., 2013; Ter Halle et al., 2017), and transmission (Frias et al., 2014; Löder et al., 2015) modes are applied for FTIR. FTIR mainly provides chemical bond information of compounds. The generation of peak types and specific spectrum rely on the bond structure. In comparison with the standard library, MPs can be distinguished from other organic and inorganic substances. The composition of MPs can be identified if the matching degree of MPs detected by infrared spectroscopy reaches more than 70% with the standard library.
The FTIR method was also widely used for the characterization of MPs because of simple operation and accurate identification. However, this method is time-consuming (Käppler et al., 2018), and it is easily affected by plastic inhomogeneity and material aging (Zhou et al., 2015). In addition, some plastic particles with size <20 μm cannot be detected. Micro-FTIR not only improved spatial resolution but also enabled the detection of smaller plastic particles. Garcia et al. (2020) used micro-FTIR to identify MPs isolated from fish tissues and found that the main polymers are PET, PES, and PE. Käppler et al. (2016) identified MPs in the environment using Raman spectroscopy and FTIR and compared and analyzed their advantages and disadvantages. They found that the samples were capable of being detected in different spectral ranges for synthetic polymers, as shown in Figure 5.
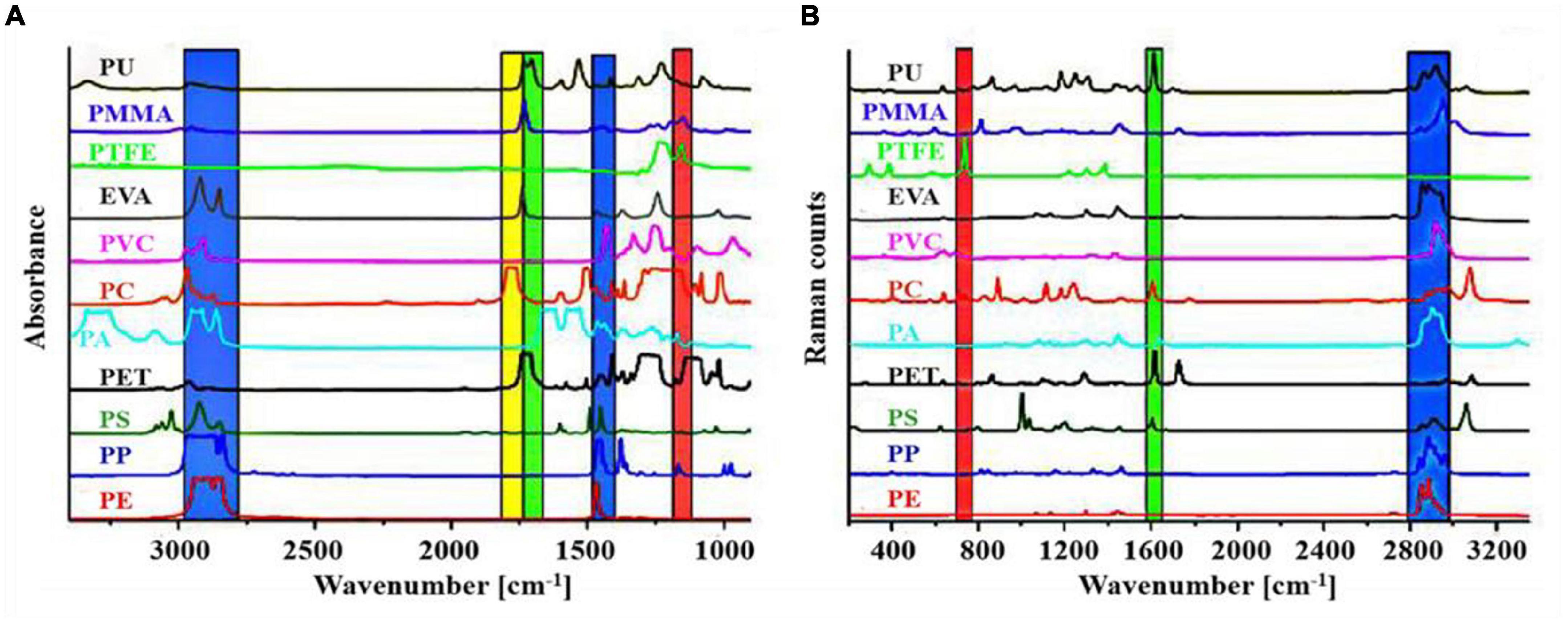
Figure 5. (A) Fourier transform infrared (FTIR) (transmission mode) and (B) Raman spectra of various kinds of microplastics (MPs) (reproduced with permission from Käppler et al., 2016).
Raman Spectroscopy
The vibrational spectroscopy technique based on the inelastic scattering of light is defined as Raman spectroscopy. Based on the scattering spectra of different frequencies of the incident light, it is possible to obtain the molecular structure of substances (Chen et al., 2020). Characteristic spectral fingerprints can be achieved by detecting the molecular vibration of a sample through Raman spectroscopy, and the composition of the sample is identified by comparing it with a known reference spectrum.
Not only Raman spectroscopy can obtain information on the functional groups on the surface of MPs, but it also allows the observation of local microscopic features (Collard et al., 2015). Raman spectroscopy with a high spatial resolution (<l μm) (Oßmann et al., 2018) is not sensitive to interference signals from water and atmospheric carbon dioxide. Moreover, the fluorescence of the sample influences the Raman spectroscopy signal. Zhao et al. (2015) identified the MPs (polypropylene and PE) in the three estuaries of China using Raman spectroscopy. Collard et al. (2015) proposed a new extraction method based on hypochlorite digestion and ultrasonic treatment for the separation of MPs from membranes. This method is appropriate for the subsequent analysis of Raman spectra. It can avoid fluorescence and allows better identification of artificial particles in fish stomachs.
Raman spectroscopy has the advantages of higher spatial resolution and no interference from water, and some information can be obtained using Raman spectroscopy rather than infrared spectroscopy. However, the fluorescence effect from the pigment, additive, or contaminant in the environmental sample affects the measurements of sample with Raman spectroscopy, and the detection time of Raman imaging is remarkably higher than FTIR imaging.
Pyrolysis Gas Chromatography/Mass Spectrometry
Currently, pyrolysis gas chromatography/mass spectrometry (Pyr-GC/MS) is the most commonly applied method for the characterization of polymers. In the Pyr-GC/MS technique, the polymer was pyrolyzed under an inert atmosphere, and then the pyrolyzed products were fed to GC-MS, where GC separates them and pyrogram is formed. The pyrogram of the unknown samples is compared with developed or available reference pyrogram to know the composition of the polymer mass under investigation (Käppler et al., 2016). This technique has the advantages of small sample amount, qualitative and quantitative analyses, no additional reagents, and so on, but it requires stringent experimental conditions (Dekiff et al., 2014). Fischer and Scholz-Böttcher (2017) used the Pyr-GC-MS method to simultaneously identify and quantify many typical MPs including PE, PP, PS, PVC, PA6, PMMA, PET, and PC. Hermabessiere et al. (2018) characterized MPs with Pyr-GC/MS by optimizing the experimental conditions, and thus the detection signal was enhanced and detection time was shortened greatly.
TDS (thermodesorption)-GC/MS first heats the sample up to 800°C under inert conditions such as N2 and then detects the sample composition by GC-MS (Li et al., 2017). It analyzes sample volumes of up to 100 mg, but it is limited to qualitative analysis. Dümichen et al. (2015) identified and quantified the characteristic decomposition products of spiked PE in complex environmental samples by combining thermogravimetric analysis coupled to solid-phase extraction (TGA-SPE) and TDS-GC/MS. This method with fast sample cleanup does not require any visual classification. However, it is only suitable for the samples that can be easily cleaned and for known polymers. In summary, the identification and quantification of MPs in the aquaculture environment by these analytical techniques are exhibited in Supplementary Table 3.
Quality Assurance and Quantity Control
During the entire sampling process, quality assurance and quantity control are vital for data accuracy. Researchers should wear 100% cotton clothes and latex gloves. The collected samples are sealed in polythene bags and aluminum foil to avoid the interference of atmospheric MPs (Noik and Tuah, 2015). Since MPs are ubiquitous in the air, a series of blank tests must be conducted to minimize the impact of environmental pollution. The MPs collected on-site should be compared with those in the standards library (Ng and Obbard, 2006).
During the on-site sampling process, a procedure blank and standard addition blank should be prepared (Hanke et al., 2013). Non-plastic materials such as glass bottles should be used during the detection process. The glass bottle should be prewashed in a nitric acid bath and then rinsed with ultrapure water three times.
Conclusion and Future Recommendations
This review briefly summarized the sampling, separation, and purification of MPs in the aquaculture environment (Figure 6). In addition, we also compare the superiority and limitations of various characterization techniques. Nonetheless, some knowledge gaps still exist. Future recommendations for the detection of MPs are as follows.
a) During the sampling process, for surface water in lakes or oceans, manta trawls or nets are suitable. For the separation of MPs, density separation is recommended. For the purification of MPs, the Fenton reaction that can effectively oxidize the organic compounds was recommended.
b) It is very difficult to quantify and identify MPs with a single method. The combination of various techniques is recommended for the identification of MPs.
c) MPs can be detected using electrochemical method because surface of MPs with the similar properties to colloid is easy to be charged in the aqueous environment.
d) Establishing standards for quantitative and qualitative analyses of MPs is extremely essential.
Author Contributions
SX and YX: conceptualization. XS: resources. SX and HD: data curation. SX and XS: writing—original draft preparation. JW and SX: writing—review and editing. JW: visualization, supervision, project administration, and funding acquisition. All authors have read and agreed to the published version of this manuscript.
Funding
This study was funded by the National Key Research and Development Program of China (2018YFD0900604), the National Natural Science Foundation of China (42077364), the Guangdong Province Universities and Colleges Pearl River Scholar Funded Scheme (2018), the Innovation Group Project of Southern Marine Science and Engineering Guangdong Laboratory (Zhuhai) (311021006), and the Key Research Projects of Universities in Guangdong Province (2019KZDXM003 and 2020KZDZX1040).
Conflict of Interest
The authors declare that the research was conducted in the absence of any commercial or financial relationships that could be construed as a potential conflict of interest.
Publisher’s Note
All claims expressed in this article are solely those of the authors and do not necessarily represent those of their affiliated organizations, or those of the publisher, the editors and the reviewers. Any product that may be evaluated in this article, or claim that may be made by its manufacturer, is not guaranteed or endorsed by the publisher.
Acknowledgments
We appreciate the provision of SCAU Wushan Campus Teaching and Research Base.
Supplementary Material
The Supplementary Material for this article can be found online at: https://www.frontiersin.org/articles/10.3389/fmars.2021.804208/full#supplementary-material
References
Anderson, P. J., Warrack, S., Langen, V., Challis, J. K., Hanson, M. L., and Rennie, M. D. (2017). Microplastic contamination in Lake Winnipeg, Canada. Environ. Pollut.225, 223–231. doi: 10.1016/j.envpol.2017.02.072
Andrady, A. L. (2011). Microplastics in the marine environment. Mar. Pollut. Bull. 62, 1596–1605. doi: 10.1016/j.marpolbul.2011.05.030
Baldwin, A. K., Corsi, S. R., and Mason, S. A. (2016). Plastic debris in 29 great lakes tributaries: relations to watershed attributes and hydrology. Environ. Sci. Technol. 50, 10377–10385. doi: 10.1021/acs.est.6b02917
Blaesing, M., and Amelung, W. (2018). Plastics in soil: analytical methods and possible sources. Sci. Total Environ. 612, 422–435. doi: 10.1016/j.scitotenv.2017.08.086
Browne, M. A., Dissanayake, A., Galloway, T. S., Lowe, D. M., and Thompson, R. C. (2008). Ingested microscopic plastic translocates to the circulatory system of the mussel, Mytilus edulis (L). Environ. Sci. Technol. 42, 5026–5031. doi: 10.1021/es800249a
Chen, G., Fu, Z., Yang, H., and Wang, J. (2020). An overview of analytical methods for detecting microplastics in the atmosphere. TrAC Trends Anal. Chem. 130:115981. doi: 10.1016/j.trac.2020.115981
Claessens, M., De Meester, S., Van Landuyt, L., De Clerck, K., and Janssen, C. R. (2011). Occurrence and distribution of microplastics in marine sediments along the Belgian coast. Mar. Pollut. Bull. 62, 2199–2204. doi: 10.1016/j.marpolbul.2011.06.030
Collard, F., Gilbert, B., Eppe, G., Parmentier, E., and Das, K. (2015). Detection of anthropogenic particles in fish stomachs: an isolation method adapted to identification by Raman spectroscopy. Arch. Environ. Contam. Toxicol. 69, 331–339. doi: 10.1007/s00244-015-0221-0
Dekiff, J. H., Remy, D., Klasmeier, J., and Fries, E. (2014). Occurrence and spatial distribution of microplastics in sediments from Norderney. Environ. Pollut. 186, 248–256. doi: 10.1016/j.envpol.2013.11.019
Desforges, J. P., Galbraith, M., and Ross, P. S. (2015). Ingestion of microplastics by zooplankton in the northeast Pacific ocean. Arch. Environ. Contam. Toxicol. 69, 320–330. doi: 10.1007/s00244-015-0172-5
Ding, J., Jiang, F., Li, J., Wang, Z., Sun, C., Wang, Z., et al. (2019). Microplastics in the coral reef systems from Xisha Islands of South China Sea. Environ. Sci. Technol. 53, 8036–8046. doi: 10.1021/acs.est.9b01452
Dris, R., Gasperi, J., Saad, M., Mirande, C., and Tassin, B. (2016). Synthetic fibers in atmospheric fallout: a source of microplastics in the environment? Mar. Pollut. Bull. 104, 290–293. doi: 10.1016/j.marpolbul.2016.01.006
Dris, R., Imhof, H., Sanchez, W., Gasperi, J., Galgani, F., Tassin, B., et al. (2015). Beyond the ocean: contamination of freshwater ecosystems with (micro-)plastic particles. Environ. Chem. 12, 539–550. doi: 10.1071/en14172
Du, H., and Wang, J. (2021). Characterization and environmental impacts of microplastics. Gondwana Res. 98, 63–75. doi: 10.1016/j.gr.2021.05.023
Du, H., Xie, Y., and Wang, J. (2021). Environmental impacts of microplastics on fishery products: an overview. Gondwana Res. (in press). doi: 10.1016/j.gr.2021.08.013
Dümichen, E., Barthel, A. K., Braun, U., Bannick, C. G., Brand, K., Jekel, M., et al. (2015). Analysis of polyethylene microplastics in environmental samples, using a thermal decomposition method. Water Res. 85, 451–457. doi: 10.1016/j.watres.2015.09.002
Eerkes-Medrano, D., Thompson, R. C., and Aldridge, D. C. (2015). Microplastics in freshwater systems: a review of the emerging threats, identification of knowledge gaps and prioritisation of research needs. Water Res. 75, 63–82. doi: 10.1016/j.watres.2015.02.012
Eriksen, M., Mason, S., Wilson, S., Box, C., and Amato, S. (2013). Microplastic pollution in the surface waters of the Laurentian Great Lakes. Mar. Pollut. Bull. 77, 177–182. doi: 10.1016/j.marpolbul.2013.10.007
Erni-Cassola, G., Gibson, M. I., Thompson, R. C., and Christie-Oleza, J. A. (2017). Lost, but found with Nile Red: a novel method for detecting and quantifying small microplastics (1 mm to 20 mu m) in environmental samples. Environ. Sci. Technol. 51, 13641–13648. doi: 10.1021/acs.est.7b04512
Fischer, M., and Scholz-Böttcher, B. M. (2017). Simultaneous trace identification and quantification of common types of microplastics in environmental samples by pyrolysis-gas chromatography-mass spectrometry. Environ. Sci. Technol. 51, 5052–5060. doi: 10.1021/acs.est.6b06362
Fok, L., and Cheung, P. K. (2015). Hong Kong at the Pearl River estuary: a hotspot of microplastic pollution. Mar. Pollut. Bull. 99, 112–118. doi: 10.1016/j.marpolbul.2015.07.050
Frias, J. P., Otero, V., and Sobral, P. (2014). Evidence of microplastics in samples of zooplankton from Portuguese coastal waters. Mar. Environ. Res. 95, 89–95. doi: 10.1016/j.marenvres.2014.01.001
Fries, E., Dekiff, J. H., Willmeyer, J., Nuelle, M. T., Ebert, M., and Remy, D. (2013). Identification of polymer types and additives in marine microplastic particles using pyrolysis-GC/MS and scanning electron microscopy. Environ. Sci. Proc. 15, 1949–1956. doi: 10.1039/c3em00214d
Garcia, A. G., Suárez, D. C., Li, J., and Rotchell, J. M. (2020). A comparison of microplastic contamination in freshwater fish from natural and farmed sources. Environ. Sci. Pollut. Res. 28, 14488–14497. doi: 10.1007/s11356-020-11605-2
Hanke, G., Galgani, F., Werner, S., Oosterbaan, L., Nilsson, P., Fleet, D., et al. (2013). Guidance on Monitoring of Marine Litter in European Seas. Luxembourg: Publications Office of the European Union, 117. doi: 10.2788/99475
Harrison, J. P., Ojeda, J. J., and Romero-González, M. E. (2012). The applicability of reflectance micro-Fourier-transform infrared spectroscopy for the detection of synthetic microplastics in marine sediments. Sci. Total Environ. 416, 455–463. doi: 10.1016/j.scitotenv.2011.11.078
Hermabessiere, L., Himber, C., Boricaud, B., Kazour, M., Amara, R., Cassone, A. L., et al. (2018). Optimization, performance, and application of a pyrolysis-GC/MS method for the identification of microplastics. Anal. Bioanal. Chem. 410, 6663–6676. doi: 10.1007/s00216-018-1279-0
Hidalgo-Ruz, V., Gutow, L., Thompson, R. C., and Thiel, M. (2012). Microplastics in the marine environment: a review of the methods used for identification and quantification. Environ. Sci. Technol. 46, 3060–3075. doi: 10.1021/es2031505
Hoffman, A., and Turner, K. (2015). Microbeads and engineering design in chemistry: no small educational investigation. J. Chem. Educ. 92, 742–746. doi: 10.1021/ed500623k
Imhof, H. K., Schmid, J., Niessner, R., Ivleva, N. P., and Laforsch, C. (2012). A novel, highly efficient method for the separation and quantification of plastic particles in sediments of aquatic environments. Limnol. Oceanogr. Methods 10, 524–537. doi: 10.4319/lom.2012.10.524
Imhof, H. K., Sigl, R., Brauer, E., Feyl, S., Giesemann, P., Klink, S., et al. (2017). Spatial and temporal variation of macro-, meso- and microplastic abundance on a remote coral island of the Maldives, Indian Ocean. Mar. Pollut. Bull. 116, 340–347. doi: 10.1016/j.marpolbul.2017.01.010
Ivleva, N. P., Wiesheu, A. C., and Niessner, R. (2017). Microplastic in aquatic ecosystems. Angew. Chem. Int. Ed. 56, 1720–1739. doi: 10.1002/anie.201606957
Käppler, A., Fischer, D., Oberbeckmann, S., Schernewski, G., Labrenz, M., Eichhorn, K.-J., et al. (2016). Analysis of environmental microplastics by vibrational microspectroscopy: FTIR, Raman or both? Anal. Bioanal. Chem. 408, 8377–8391. doi: 10.1007/s00216-016-9956-3
Käppler, A., Fischer, M., Scholz-Böttcher, B. M., Oberbeckmann, S., Labrenz, M., Fischer, D., et al. (2018). Comparison of μ-ATR-FTIR spectroscopy and py-GCMS as identification tools for microplastic particles and fibers isolated from river sediments. Anal. Bioanal. Chem. 410, 5313–5327. doi: 10.1007/s00216-018-1185-5
Karlsson, T. M., Vethaak, A. D., Almroth, B. C., Ariese, F., van Velzen, M., Hassellöv, M., et al. (2017). Screening for microplastics in sediment, water, marine invertebrates and fish: method development and microplastic accumulation. Mar. Pollut. Bull. 122, 403–408. doi: 10.1016/j.marpolbul.2017.06.081
Kim, I. S., Chae, D. H., Kim, S. K., Choi, S., and Woo, S. B. (2015). Factors influencing the spatial variation of microplastics on high-tidal coastal beaches in Korea. Arch. Environ. Contam. Toxicol. 69, 299–309. doi: 10.1007/s00244-015-0155-6
Klein, S., Worch, E., and Knepper, T. P. (2015). Occurrence and spatial distribution of microplastics in river shore sediments of the Rhine-Main area in Germany. Environ. Sci. Technol. 49, 6070–6076. doi: 10.1021/acs.est.5b00492
Krüger, L., Casado-Coy, N., Valle, C., Ramos, M., Sánchez-Jerez, P., Gago, J., et al. (2020). Plastic debris accumulation in the seabed derived from coastal fish farming. Environ. Pollut. 257:113336. doi: 10.1016/j.envpol.2019.113336
Lee, K. W., Shim, W. J., Kwon, O. Y., and Kang, J. H. (2013). Size-dependent effects of micro polystyrene particles in the marine copepod Tigriopus japonicus. Environ. Sci. Technol. 47, 11278–11283. doi: 10.1021/es401932b
Li, J., Liu, H., and Chen, J. P. (2017). Microplastics in freshwater systems: a review on occurrence, environmental effects, and methods for microplastics detection. Water Res. 137, 362–374. doi: 10.1016/j.watres.2017.12.056
Löder, M. G. J., Kuczera, M., Mintenig, S., Lorenz, C., and Gerdts, G. (2015). Focal plane array detector-based micro-Fourier-transform infrared imaging for the analysis of microplastics in environmental samples. Environ. Chem. 12, 563–581. doi: 10.1071/EN14205
Ma, J., Niu, X., Zhang, D., Lu, L., Ye, X., Deng, W., et al. (2020). High levels of microplastic pollution in aquaculture water of fish ponds in the Pearl River estuary of Guangzhou, China. Sci. Total Environ. 744:140679. doi: 10.1016/j.scitotenv.2020.140679
Mai, L., Bao, L. J., Shi, L., Wong, C. S., and Zeng, E. Y. (2018). A review of methods for measuring microplastics in aquatic environments. Environ. Sci. Pollut. Res. 25, 11319–11332. doi: 10.1007/s11356-018-1692-0
Mani, T., Hauk, A., Walter, U., and Burkhardt-Holm, P. (2015). Microplastics profile along the Rhine River. Sci. Rep. 5:17988. doi: 10.1038/srep17988
Mohsen, M., Wang, Q., Zhang, L., Sun, L., Lin, C., and Yang, H. (2019). Microplastic ingestion by the farmed sea cucumber Apostichopus japonicus in China. Environ. Pollut. 245, 1071–1078. doi: 10.1016/j.envpol.2018.11.083
Ng, K. L., and Obbard, J. P. (2006). Prevalence of microplastics in Singapore’s coastal marine environment. Mar. Pollut. Bull. 52, 761–767. doi: 10.1016/j.marpolbul.2005.11.017
Noik, V. J., and Tuah, P. M. (2015). A first survey on the abundance of plastics fragments and particles on two sandy beaches in Kuching, Sarawak, Malaysia. Mater. Sci. Eng. 78:012035. doi: 10.1088/1757-899X/78/1/012035
Nuelle, M. T., Dekiff, J. H., Remy, D., and Fries, E. (2014). A new analytical approach for monitoring microplastics in marine sediments. Environ. Pollut. 184, 161–169. doi: 10.1016/j.envpol.2013.07.027
Oßmann, B. E., Sarau, G., Holtmannspötter, H., Pischetsrieder, M., Christiansen, S. H., and Dicke, W. (2018). Small-sized microplastics and pigmented particles in bottled mineral water. Water Res. 141, 307–316. doi: 10.1016/j.watres.2018.05.027
Park, T. J., Lee, S. H., Lee, M. S., Lee, J. K., Lee, S. H., and Zoh, K. D. (2020). Occurrence of microplastics in the Han River and riverine fish in South Korea. Sci. Total Environ. 708:134535. doi: 10.1016/j.scitotenv.2019.134535
Patterson, J., Jeyasanta, K. I., Sathish, N., Booth, A. M., and Edward, J. (2019). Profiling microplastics in the Indian edible oyster, Magallana bilineata collected from the Tuticorin coast, Gulf of Mannar, Southeastern India. Sci. Total Environ. 691, 727–735. doi: 10.1016/j.scitotenv.2019.07.063
PlasticsEurope (2020). An Analysis of European Plastics Production, Demand and Waste Data: Plastics – The Facts. Available online at: https://www.plasticseurope.org/en/resources/market-data/ (accessed 17 December 17, 2020).
Primpke, S., Fischer, M., Lorenz, C., Gerdts, G., and Scholz-Böttcher, B. M. (2020). Comparison of pyrolysis gas chromatography/mass spectrometry and hyperspectral FTIR imaging spectroscopy for the analysis of microplastics. Anal. Bioanal. Chem. 412, 8283–8298. doi: 10.1007/s00216-020-02979-w
Quinn, B., Murphy, F., and Ewins, C. (2017). Validation of density separation for the rapid recovery of microplastics from sediment. Anal. Methods 9, 1491–1498. doi: 10.1039/c6ay02542k
Ribeiro-Claro, P., and Nolasco, M. M.Araújo, C. (2017). “Chapter 5-Characterization of microplastics by Raman spectroscopy”, in Comprehensive Analytical Chemistry, Vol. 75, eds T. A. P. Rocha-Santos and Duarte, A. C. (Amsterdam: Elsevier), 119–151. doi: 10.1016/bs.coac.2016.10.001
Rodrigues, M. O., Gonçalves, A. M. N., Gonçalves, F. J. M., and Abrantes, N. (2020). Improving cost-efficiency for MPs density separation by zinc chloride reuse. MethodsX 7:100785. doi: 10.1016/j.mex.2020.100785
Shim, W. J., Song, Y. K., Hong, S. H., and Jang, M. (2016). Identification and quantification of microplastics using Nile Red staining. Mar. Pollut. Bull. 113, 469–476. doi: 10.1016/j.marpolbul.2016.10.049
Song, Y. K., Hong, S. H., Jang, M., Han, G. M., Rani, M., Lee, J., et al. (2015). A comparison of microscopic and spectroscopic identification methods for analysis of microplastics in environmental samples. Mar. Pollut. Bull. 93, 202–209. doi: 10.1016/j.marpolbul.2015.01.015
Su, L., Xue, Y., Li, L., Yang, D., Kolandhasamy, P., Li, D., et al. (2016). Microplastics in Taihu Lake, China. Environ. Pollut. 216, 711–719. doi: 10.1016/j.envpol.2016.06.036
Ta, A. T., and Babel, S. (2020a). Microplastics pollution with heavy metals in the aquaculture zone of the Chao Phraya River estuary, Thailand. Mar. Pollut. Bull. 161:111747. doi: 10.1016/j.marpolbul.2020.111747
Ta, A. T., and Babel, S. (2020b). Microplastic contamination on the lower Chao Phraya: abundance, characteristic and interaction with heavy metals. Chemosphere 257:127234. doi: 10.1016/j.chemosphere.2020.127234
Ter Halle, A., Jeanneau, L., Martignac, M., Jardé, E., Pedrono, B., Brach, L., et al. (2017). Nanoplastic in the North Atlantic subtropical gyre. Environ. Sci. Technol. 51, 13689–13697. doi: 10.1021/acs.est.7b03667
Thompson, R. C., Olsen, Y., Mitchell, R. P., Davis, A., Rowland, S. J., John, A. W., et al. (2004). Lost at sea: where is all the plastic? Science 304:838. doi: 10.1126/science.1094559
Tirkey, A., and Upadhyay, L. S. B. (2021). Microplastics: an overview on separation, identification and characterization of microplastics. Mar. Pollut. Bull. 170:112604. doi: 10.1016/j.marpolbul.2021.112604
Uddin, S., Fowler, S. W., Saeed, T., Naji, A., and Al-Jandal, N. (2020). Standardized protocols for microplastics determinations in environmental samples from the Gulf and marginal seas. Mar. Pollut. Bull. 158:111374. doi: 10.1016/j.marpolbul.2020.111374
Van Cauwenberghe, L., Vanreusel, A., Mees, J., and Janssen, C. R. (2013). Microplastic pollution in deep-sea sediments. Environ. Pollut. 182, 495–499. doi: 10.1016/j.envpol.2013.08.013
Vermeiren, P., Muñoz, C., and Ikejima, K. (2020). Microplastic identification and quantification from organic rich sediments: a validated laboratory protocol. Environ. Pollut. 262:114298. doi: 10.1016/j.envpol.2020.114298
Vianello, A., Boldrin, A., Guerriero, P., Moschino, V., Rella, R., Sturaro, A., et al. (2013). Microplastic particles in sediments of Lagoon of Venice, Italy: first observations on occurrence, spatial patterns and identification. Estuar. Coast. Shelf Sci. 130, 54–61. doi: 10.1016/j.ecss.2013.03.022
Wang, F., Wang, B., Duan, L., Zhang, Y., and Yu, G. (2020). Occurrence and distribution of microplastics in domestic, industrial, agricultural and aquacultural wastewater sources: a case study in Changzhou, China. Water Res. 182:115956. doi: 10.1016/j.watres.2020.115956
Wang, J., Lu, L., Wang, M., Jiang, T., Liu, X., and Ru, S. (2019). Typhoons increase the abundance of microplastics in the marine environment and cultured organisms: a case study in Sanggou Bay, China. Sci. Total Environ. 667, 1–8. doi: 10.1016/j.scitotenv.2019.02.367
Wang, Q., Zhu, X., Hou, C., Wu, Y., Teng, J., Zhang, C., et al. (2021). Microplastic uptake in commercial fishes from the Bohai Sea, China. Chemosphere 263:127962. doi: 10.1016/j.chemosphere.2020.127962
Wang, W., Ndungu, A. W., Li, Z., and Wang, J. (2017). Microplastics pollution in inland freshwaters of China: a case study in urban surface waters of Wuhan, China. Sci. Total Environ. 575, 1369–1374. doi: 10.1016/j.scitotenv.2016.09.213
Wang, W., and Wang, J. (2018). Investigation of microplastics in aquatic environments: an overview of the methods used, from field sampling to laboratory analysis. TrAC Trends Anal. Chem. 108, 195–202. doi: 10.1016/j.trac.2018.08.026
Wright, S. L., Thompson, R. C., and Galloway, T. S. (2013). The physical impacts of microplastics on marine organisms: a review. Environ. Pollut. 178, 483–492. doi: 10.1016/j.envpol.2013.02.031
Wu, F., Wang, Y., Leung, J., Huang, W., Zeng, J., Tang, Y., et al. (2019). Accumulation of microplastics in typical commercial aquatic species: a case study at a productive aquaculture site in China. Sci. Total Environ. 708:135432. doi: 10.1016/j.scitotenv.2019.135432
Wu, M., Yang, C., Du, C., and Liu, H. (2020). Microplastics in waters and soils: occurrence, analytical methods and ecotoxicological effects. Ecotoxicol. Environ. Saf. 202:110910. doi: 10.1016/j.ecoenv.2020.110910
Yuan, W., Liu, X., Wang, W., Di, M., and Wang, J. (2019). Microplastic abundance, distribution and composition in water, sediments, and wild fish from Poyang Lake, China. Ecotoxicol. Environ. Saf. 170, 180–187. doi: 10.1016/j.ecoenv.2018.11.126
Zhang, D., Cui, Y., Zhou, H., Jin, C., Yu, X., Xu, Y., et al. (2019). Microplastic pollution in water, sediment, and fish from artificial reefs around the Ma’an Archipelago, Shengsi, China. Sci. Total Environ. 703:134768. doi: 10.1016/j.scitotenv.2019.134768
Zhang, D., Fraser, M. A., Huang, W., Ge, C., Wang, Y., Zhang, C., et al. (2020). Microplastic pollution in water, sediment, and specific tissues of crayfish (Procambarus clarkii) within two different breeding modes in Jianli, Hubei province, China. Environ. Pollut. 272:115939. doi: 10.1016/j.envpol.2020.115939
Zhao, S., Zhu, L., and Li, D. (2015). Microplastic in three urban estuaries, China. Environ. Pollut. 206, 597–604. doi: 10.1016/j.envpol.2015.08.027
Zheng, Y., Li, J., Cao, W., Liu, X., Jiang, F., Ding, J., et al. (2019). Distribution characteristics of microplastics in the seawater and sediment: a case study in Jiaozhou Bay, China. Sci. Total Environ. 674, 27–35. doi: 10.1016/j.scitotenv.2019.04.008
Zhou, A., Zhang, Y., Xie, S., Chen, Y., Li, X., Wang, J., et al. (2021). Microplastics and their potential effects on the aquaculture systems: a critical review. Rev. Aquacult. 13, 719–733. doi: 10.1111/raq.12496
Zhou, Q., Zhang, H. B., Yuan, L. I., and Luo, Y. M. (2015). Progress on microplastics pollution and its ecological effects in the coastal environment. Chin. Sci. Bull. 60:3210. doi: 10.1360/N972015-00714
Zhu, J., Zhang, Q., Li, Y., Tan, S., Kang, Z., Yu, X., et al. (2019). Microplastic pollution in the Maowei Sea, a typical mariculture bay of China. Sci. Total Environ. 658, 62–68. doi: 10.1016/j.scitotenv.2018.12.192
Keywords: microplastics, analytical method, sampling, identification, aquaculture environment
Citation: Xiang S, Xie Y, Sun X, Du H and Wang J (2022) Identification and Quantification of Microplastics in Aquaculture Environment. Front. Mar. Sci. 8:804208. doi: 10.3389/fmars.2021.804208
Received: 29 October 2021; Accepted: 15 November 2021;
Published: 06 January 2022.
Edited by:
Li Zhang, South China Sea Institute of Oceanology, Chinese Academy of Sciences (CAS), ChinaReviewed by:
Ke Pan, Shenzhen University, ChinaHailong Zhou, Hainan University, China
Jiye Hu, University of Science and Technology Beijing, China
Xiangrong Xu, South China Sea Institute of Oceanology, Chinese Academy of Sciences (CAS), China
Copyright © 2022 Xiang, Xie, Sun, Du and Wang. This is an open-access article distributed under the terms of the Creative Commons Attribution License (CC BY). The use, distribution or reproduction in other forums is permitted, provided the original author(s) and the copyright owner(s) are credited and that the original publication in this journal is cited, in accordance with accepted academic practice. No use, distribution or reproduction is permitted which does not comply with these terms.
*Correspondence: Xuemei Sun, sunxuemei@hbut.edu.cn; Hao Du, duhao2020@scau.edu.cn; Jun Wang, wangjun2016@scau.edu.cn