- Leibniz Institute for Baltic Sea Research Warnemünde, Rostock, Germany
Dissolved and particulate organic carbon (DOC, POC) and nitrogen (DON, PON) constitute essential nutrient and energy sources to heterotrophic microbes in aquatic systems. Especially in the shallow coastal ocean, the concentrations are highly variable on short timescales, and cycling is heavily affected by different sources and environmental drivers. We analyzed surface water organic carbon and nitrogen concentrations determined weekly from 2010 to 2020 in the nearshore southwestern Baltic Sea (Heiligendamm, Germany) in relation to physical, chemical and biological parameters available since 1988. Mixing of low-DOC North Sea water with high-DOC Baltic Sea water, as well as in situ primary production, were confirmed as the main drivers of organic carbon and nitrogen concentrations. Tight coupling between POC, PON, chlorophyll a and phytoplankton carbon with DON seasonal dynamics corroborated the close relationship between phytoplankton production and degradation of organic nutrients with preferential remineralization of nitrogen. Significant changes in air and water temperature, salinity, and inorganic nutrients over time indicated effects of climate change and improved water quality management in the eutrophic Baltic Sea. Bulk organic nutrient concentrations did not change over time, while the salinity-corrected fraction of the DOC increased by about 0.6 μmol L–1yr–1. Concurrently, chlorophyll a and Bacillariophyceae and Cryptophyceae carbon increased, denoting a potential link to primary productivity. The high variability of the shallow system exacerbates the detection of trends, but our results emphasize the value of these extended samplings to understand coupled biogeochemical cycling of organic matter fractions and to detect trends in these important carbon reservoirs.
Introduction
Coastal oceans, although only occupying 7% of the surface area or 0.5% of the water volume of the global oceans, are responsible for about one-fifth of oceanic organic matter production (Gattuso et al., 1998; Wollast, 1998; Borges, 2005). Nearshore systems are highly dynamic environments: due to the high light and nutrient availability, they are hotspots of dissolved and particulate organic carbon (DOC, POC) and nitrogen (DON, PON) cycling. The organic compounds in the seawater do not only act as nutrient sources but also impact chemical and physical properties such as light availability, alkalinity, or trace element solubility and contaminant transport (Krop et al., 2001; Sandberg et al., 2004; Kerr et al., 2008; Aksnes et al., 2009; Hammer et al., 2017; McGovern et al., 2019). The organic matter can be sourced autochthonously, and in addition, the input from rivers, groundwater discharge, or release from sediments can be high (Cauwet, 2002; Burnett et al., 2003; Szymczycha et al., 2014; Donis et al., 2017). From the coastal waters, where organic nutrients are usually present in excess, they can be exported to the open ocean and support heterotrophic activity in the more nutrient-depleted waters (Duarte and Cebrián, 1996; del Giorgio and Duarte, 2002; Barrón and Duarte, 2015).
Particulate and dissolved phases present in a continuum of sizes are operationally separated by either passing through or being retained on a filter of a certain pore size [usually 0.1–0.8 μm, (Benner and Amon, 2015)]. The particulate organic matter (POM) pool is mainly comprised of biomass from phytoplankton or large bacteria, detritus including dead cells and fecal pellets, or other aggregated materials (Graff et al., 2015; Kharbush et al., 2020). POM usually experiences a high seasonality and peaks during spring or summer phytoplankton blooms (Lutz et al., 2007; Winogradow et al., 2019). Most of the POM is degraded in the water column or sinks to the sediments where it is buried and preserved. About half of the primary production is channeled into the dissolved pool via direct release, sloppy feeding, or after the death of the phytoplanktonic organisms (Duarte and Cebrián, 1996; Carlson et al., 2002). In the open ocean, only around 1% of the carbon fixed in the surface reaches the sediment. In the highly productive, shallow coastal systems this percentage can be much higher, but preservation differs widely according to POM origin, redox conditions, sediment morphology, and prevailing currents (Hedges and Keil, 1995; McKee et al., 2004; Leipe et al., 2011; Herndl and Reinthaler, 2013). Resuspension, especially in shallow systems, can resupply the partially degraded organic matter to the water column (Hopkinson, 1985; Wainright, 1987). In the global surface ocean, the DOC inventory exceeds the POC pool by an order of magnitude (Middelburg, 2019). Surface DOC concentrations in the Atlantic, Pacific and Indian Oceans lie at 70–80 μmol L–1 while values in the coastal ocean average around 290 μmol L–1 DOC (Hansell et al., 2009; Barrón and Duarte, 2015). Seasonal increases in surface DOC are usually delayed by a few days to weeks and pronounced during bloom demise (Nausch et al., 2008; Szymczycha et al., 2017). The dissolved organic matter (DOM) fixed during the productive season is then taken up and used as molecular building blocks or respired to CO2 throughout the winter by heterotrophic organisms. DOM comprises thousands to millions of different molecular structures that are more or less available for biotic or abiotic degradation with lifetimes ranging from minutes to millennia (Hansell, 2013; Dittmar, 2014; Zark et al., 2017). Freshly produced DOM, including carbohydrates, proteins or amino acids, is easily taken up, while a refractory carbon pool comprising more than 96% of all organically bound carbon in the oceans is stable over thousands of years (Hansell, 2013).
Particulate carbon and nitrogen concentrations increased in the upper 100 m of the northwestern Sargasso Sea near Bermuda from 1971 to 1998, with a larger relative increase in particulate nitrogen, which was attributed to enhanced dinitrogen fixation as a result of changing climate conditions (Karl, 1999; Hebel and Karl, 2001); DON and DOC concentrations were shown to increase in the euphotic zone in the 1990s in the same area (Church et al., 2002). In subarctic to temperate climate, the total organic nitrogen and carbon in Finnish boreal rivers (Lepistö et al., 2021) and DON export from Norwegian rivers to the Skagerrak coastline rose as a result of climate change and anthropogenic pressures (Deininger et al., 2020). Worrall et al. (2004) describe increasing DOC over time since the 1960s in British rivers and lakes, usually with small slopes. Possible reasons in this temperate environment are complex changes in discharge during the drier summers and wetter winters, eutrophication from nutrients, temperature increase, and changes in land management. Räike et al. (2012), however, do not observe a significant increase in DOC export from the Finnish Baltic Sea catchment between 1975 and 2010. The future impact of climate change on ocean biogeochemistry is associated with high uncertainties (Burd et al., 2016) and the effects of climate change on organic carbon concentrations and cycling are likely different on stratified and seasonally stratified open ocean systems and the coastal ocean (Lønborg et al., 2020). Long-term monitoring is important to provide a basis for robust predictions, especially in highly dynamic systems such as the Baltic Sea, where anthropogenic and natural factors entail environmental changes on different timescales (Lennartz et al., 2014).
The Baltic Sea is a unique brackish-marine inland sea ecosystem where terrestrial inputs are a significant source of DOM and POM (Bianchi et al., 1997; Osburn and Stedmon, 2011; Deutsch et al., 2012; Seidel et al., 2017). The enclosed basin experiences high freshwater input, especially in the North, and limited exchange with North Sea water through the Skagerrak and Kattegat leading to an estuarine-like circulation (Meier et al., 2006b). A stable salinity gradient forms from freshwater values in the Northeastern Bothnian Bay to ranging between 8 and >20 in the Arkona Basin surface and bottom waters in the southwest (Lass and Mohrholz, 2003). The water column is stratified throughout most of the year, supported by the absence of a tidal cycle, Major Baltic Inflow (MBI) events carrying oxygen-rich, saline waters to the deeps in the Baltic Proper happen only irregularly (Mohrholz, 2018).
DOC concentrations in the Baltic Sea are highly influenced by mixing of high DOC freshwater, sometimes carrying more than 1,000 μmol L–1 DOC, with lower DOC marine waters from the North Sea, which supply 100–200 μmol C L–1 (Kuliński and Pempkowiak, 2008; Kulinski and Pempkowiak, 2012; Maciejewska and Pempkowiak, 2014; Osterholz et al., 2016b; Seidel et al., 2017). In the southwestern Baltic Sea, the DOC-to-POC ratio lies around 45, with DOC concentrations that average on the same level as global lake concentrations at 320 μmol C L–1 (Nausch et al., 2008; Toming et al., 2020). Compared to the high background concentrations, the seasonal variability of DOC is small (Nausch et al., 2008). The rivers entering the Baltic Sea from densely populated catchments carry substantial nutrient loads from anthropogenic activities, including agricultural land use, industrial and household wastewater, marine traffic, and maritime activities (Nausch et al., 2008; Gustafsson et al., 2012). The nutrients are available to phytoplankton production favoring large bloom formation. Since 1960, the frequency and intensity of cyanobacterial blooms have increased (Terttu et al., 2001). The neighboring states make efforts to reduce the eutrophication of the system (HELCOM, 2018), and nutrients inputs have been significantly reduced since the 1990s (Kuss et al., 2020). However, climate change impacts become visible in the Baltic Sea: the air and water temperatures increase and the phytoplankton growing season extends, while water transparency decreases (Fleming-Lehtinen and Laamanen, 2012; Wasmund et al., 2019; Meier et al., 2021). Higher amounts of POC supplied to the sediments would support increasing anoxic conditions, during which organic carbon mineralization is slowed down (Carstensen et al., 2014; Asmala et al., 2018) and buried phosphorus is released from the sediment to the water column (Emil et al., 2007; Koch et al., 2018).
The Leibniz Institute for Baltic Sea Research Warnemünde, Rostock, Germany, conducts weekly nearshore sampling for inorganic nutrients, chlorophyll a, salinity, and temperature in the Bay of Mecklenburg in the southwestern Baltic Sea water since 1988, first at Warnemünde. After the demolition of the pier, the sampling was moved a few kilometers west to the Heiligendamm Pier in 1998. POC, PON and DOC, DON concentrations are available since 2010. The high-resolution sampling intervals over 11 years allow us to (I) elucidate the main drivers of organic nutrient concentrations, (II) explore the relationship of particulate and dissolved carbon and nitrogen fractions, and (III) observe potential changes in concentration and quality over time in an extremely variable nearshore, brackish-marine system.
Materials and Methods
Most samples were taken weekly at the same time of the day (10:30 am) from Heiligendamm Pier located at 54°8′46.68″N, 11°49′59.88″E, reaching about 170 m from the shoreline at around 5–6 m water depth (Figure 1). Additionally, water samples from Warnemünde Pier (54°10′59.88″N, 12°3′29.88″E, ∼100 m from the shoreline, 4–5 m water depth) from 1988 to end of November 1997, and from Kühlungsborn Pier (54°9′22.93″N, 11°45′46.65″E, ∼200 m from the shoreline, ∼5 m water depth) during March, April and October of 2001, and February to end of May 2007, are included in the time series. Only very few samplings were missed overall, e.g., in December 1997, January 1998, November and December of 2006, and during spring/summer 2020 some weeks were not sampled due to SARS-CoV-2 restrictions. The German coast of the southwestern Baltic Sea is characterized by flat topography, urbanized cities, and agricultural land use (von Ahn et al., 2021). Several small drainage channels enter the Baltic Sea around Heiligendamm. The largest of them, the 7 km long Mühlenfließ, receives the municipal waste water treatment plant output of the city of Bad Doberan with 60,000 inhabitants, carrying high ammonium and phosphate loads at times, and entering the Baltic Sea about 2 km east of the Heiligendamm Pier. The lowland Warnow River enters the Warnow Estuary about 15 km inland at a flow rate of 16.5 m3 s–1. The estuary discharges into the Baltic Sea in Warnemünde around 20 km east of Heiligendamm and the plume is deflected eastward (Jurasinski et al., 2018). The coast in the area is dominated by sandy barriers and sandy dunes with flat topology (Labuz, 2015), and the sediments in the Wismar Bay west of Heiligendamm consist of quaternary glaciofluvial sands with intercalated tills (Schafmeister and Darsow, 2011; von Ahn et al., 2021). During the weekly sampling, water temperature and salinity were determined in situ with a WTW Cond 1970i conductivity meter (xylem, United States). The surface water samples obtained by bucket were transported to the laboratory within 30 min and processed immediately. For POC and PON quantification, 200–500 mL of water were filtered through 0.7 μm precombusted glass fiber filters (GFF, 450°C, 6 h). The filters were stored frozen until analysis using a vario MICRO cube (Elementar, Germany). Calibration was done in the range of 0.01–4 mg acetanilide. Reproducibility based on the measurements of 20 filters from the same Baltic Sea water sample was better than 7.3 and 12.0% for POC and PON, respectively. Due to the lack of a POM reference sample, Marine Sediments MS2 and MS3 obtained from Quasimeme (Netherlands) were used to assess method- and laboratory bias. Precision was better than 8 and 12%, accuracy was better than 5 and 8% for POC and PON, respectively. Samples for DOC and total dissolved nitrogen (TDN) analysis were obtained from the filtrate and stored frozen until analysis via high temperature catalytic combustion using a Shimadzu TOC analyzer with TN module. Calibration was done in the range of 41–416 μmol C L–1 with acetanilide. Reproducibility was assessed from repeated measurements of a Baltic Sea water sample for DOC and TDN. Precision was calculated from repeated measurements of Quasimeme Low Nutrient Seawater from the Eastern Atlantic Ocean for DOC and from deep Florida Strait reference material (DSR), provided by D. A. Hansell (University of Miami, FL, United States), for TDN. Precision was better than 2 and 4%, accuracy was better than 4 and 3% for DOC and TDN, respectively. Nitrate, nitrite, phosphate (dissolved inorganic phosphorus, DIP), and silicate were analyzed from GFF-filtered (precombusted, 450°C, 6 h) water samples using manual or air-segmented continuous-flow spectrophotometry, ammonium was determined manually from unfiltered water as indophenol blue by photometry (Hansen and Koroleff, 1999). Nutrient analyses were performed in the long tradition of strict quality assurance (Grasshoff, 1976), and stability of the analytical methods was assured through regular intercalibration. Measurement uncertainties were 4.5, 5.0, 3.4, 3.8, and 9.2% for nitrate + nitrite, nitrite, phosphate, silicate, and ammonium, respectively. Dissolved inorganic nitrogen (DIN) was calculated from nitrate and nitrite, but not ammonium. Ammonium concentrations were only measured regularly between 1988 and 1997 and then from April to September until 2000, and therefore not taken into account for a consistent DIN term. Ammonium concentrations were 1.3 ± 1.6 μmol L–1 (April to September) and 3.4 ± 2.1 μmol L–1 (October to March). Dissolved organic nitrogen (DON) was determined by subtracting DIN from TDN. For chlorophyll a (Chl a) analysis, 0.5 L of water were passed through a GFF filter. The filters were then extracted with 97% ethanol for 3–5 h, and chlorophyll a was quantified with a TD10 AU-005-CE fluorometer (Turner Designs, United States). The phytoplankton samples (200 mL) were fixed with Acid Lugol’s solution (1 mL). Phytoplankton counts were performed according to HELCOM guidelines using an inverted light microscope following the Utermöhl method (Utermöhl, 1958) and evaluated on class taxonomic level. During counting, individuals are not only classified according to taxa, but also size classes (Olenina et al., 2006). At least 50 individuals of the most abundant species, and generally at least 500 individuals were counted per sample. Conversion to carbon was done according to Menden-Deuer and Lessard (2000). Counting, calculation, and data output were facilitated by the software “OrgaCount,” provided by AquaEcology (Oldenburg, Germany).
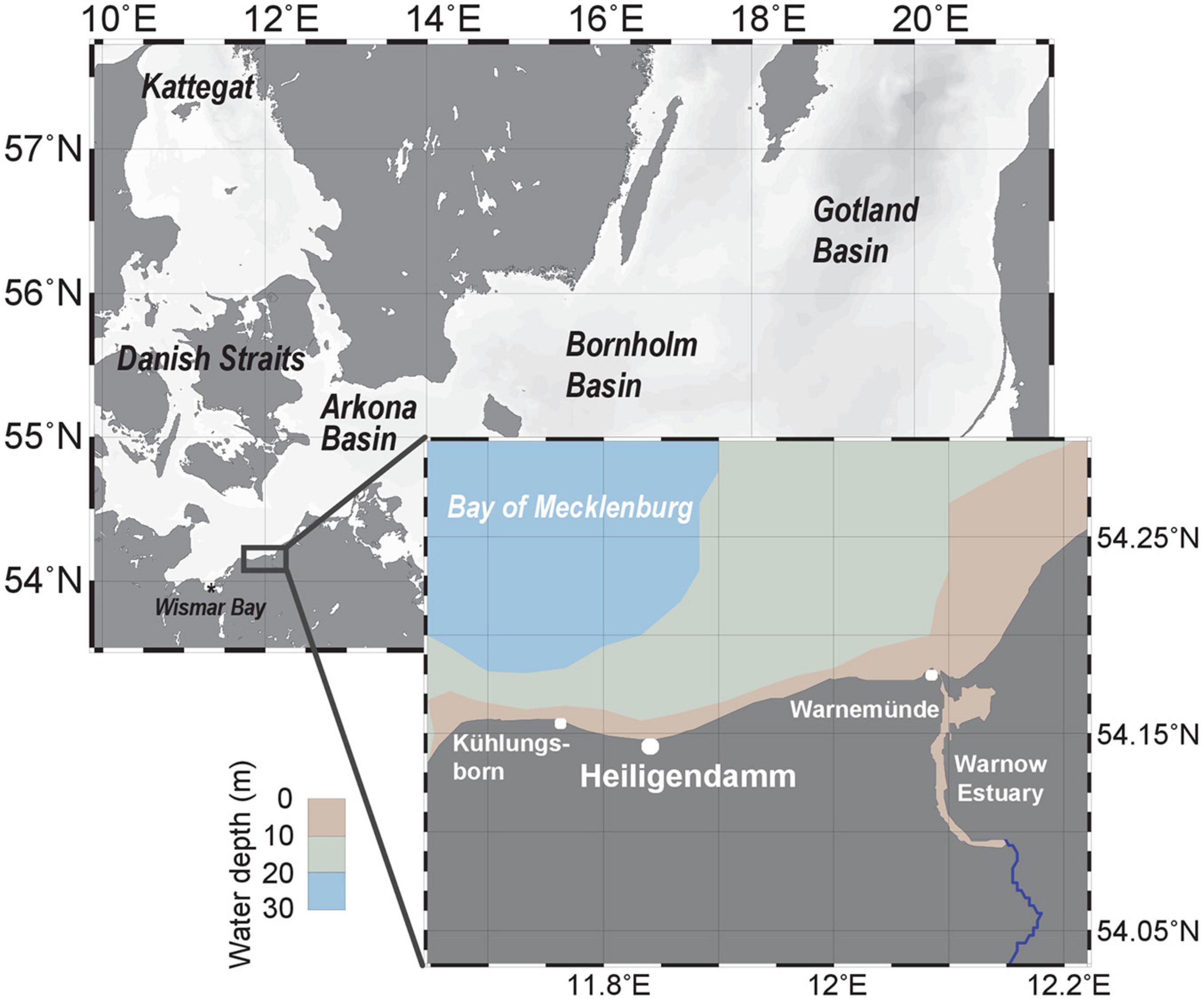
Figure 1. Southwestern Baltic Sea with insert showing sampling points between 1988 and 2020. Of 1,626 observations, most were made at Heiligendamm (n = 1116, 69%), followed by Warnemünde (n = 478, 29%), and Kühlungsborn (n = 32, 2%). Map produced with Ocean Data View (Schlitzer, 2020).
For this study, we used all data from Warnemünde, Kühlungsborn, and Heiligendamm Piers available at the ODIN II database of the Leibniz Institute for Baltic Sea Research Warnemünde1 between June 1988 and December 2020. For an overview of parameters and time frames, see Supplementary Table 1. Outliers in temperature, salinity, inorganic nutrients, Chl a, and organic carbon and nitrogen concentrations were identified and removed for statistical analysis applying Rosner’s test for multiple outliers (Rosner, 1983). Data were log-transformed before the outlier test if distributions were skewed, i.e., log-transformations were applied for POC, PON, nitrate, nitrite, Chl a and phytoplankton carbon. For time series analyses, missing data were replaced by means from two preceding and two successive values. As DOC was highly correlated to salinity and thus influenced by mixing (see section “Results”), we also calculated the salinity-corrected concentration DOCSC. For this, we first fitted a linear model for DOC ∼ S and then subtracted the obtained values from the environmental concentrations, i.e., DOCSC = DOCmeas − (−7.30 × S + 380.03), where S is salinity, and DOCmeas is the measured DOC concentration. Daily mean air temperature, wind speed, and wind direction (since 1947) were obtained from the Climate Data Center (CDC) of the German National Meteorological Service (Deutscher Wetterdienst, DWD) for the station Warnemünde (Station ID 4271, downloaded January 19, 2021). Daily means of net heat flux were calculated as the sum of solar radiation, sensible heat, latent heat, and net longwave radiation using NCEP/NCAR Reanalysis 1 data at 56°11′21.48″N, 11°15′0″E from 1948 to 2020 (Kalnay et al., 1996). In our study, negative net heat flux or its components indicate heat loss of the water column, whereas positive values indicate heat gain by the ocean. Most statistical analyses were performed using R (Version 4.0.5) with the “vegan” package (Oksanen et al., 2020). Multiple linear regressions were performed including all available data and separately for cold (∼November to March) and warm (∼May to October) season by splitting the dataset at the median of the bimodally distributed water temperature of 12.1°C. For time series analyses based on monthly mean data, we used the openair (Carslaw and Ropkins, 2012), trend (Pohlert, 2020), and TTR (Ulrich, 2020) packages. The non-parametric Mann–Kendall test (Hirsch et al., 1982) with bootstrap permutations was applied to test for monotonic upward or downward trends across deseasonalized monthly mean data. The slope was represented as Theil Sen’s slope (Sen, 1968; Theil, 1992). Here, the slopes between all pairs of data points are calculated and the Theil Sen’s slope estimator represents the median of all slopes, rendering it robust against non-normal distribution of data, heteroscedasticity, and outliers.
Results
Seasonality of Hydrography, Inorganic Nutrients, and Organic Carbon
The salinity at Heiligendamm Pier varied seasonally with increased salinity in winter due to exchange with the saline North Sea, averaging 12.2 ± 2.6. Salinities between 7.5 to 21.1 cover much of the salinity range of the Baltic Sea, but extreme values were detected only infrequently (Figures 2, 3). Coldest water temperatures below 5°C mostly occurred from December to March, while from June to September, temperatures often exceeded 15°C in the shallow water. Net heat flux with a strong seasonal signal varied from −325 W m–2 heat loss in winter to 312 W m–2 heat gain in summer. Total biota carbon from phytoplankton cell counts showed the spring bloom in March extending to April and a broad summer to fall bloom from July, declining only in November. Based on carbon content overall timepoints, classes Bacillariophyceae (Figure 3G, 39%) and Dinophyceae (26%) dominated the phytoplankton community. Ciliates (Mesodinium rubrum, 9%), Dictyochophyceae (8%), Cryptophyceae (6%), Cyanophyceae (5%), Euglenophyceae (3%), and Prymnesiophyceae (2%) contributed minor amounts of carbon. All inorganic nutrients, including silicate, phosphate, nitrate, and nitrite, declined in March with the onset of the spring bloom. Silicate and phosphate concentrations were rarely depleted and increased again from April. Nitrate and nitrite were mostly depleted from May through October.
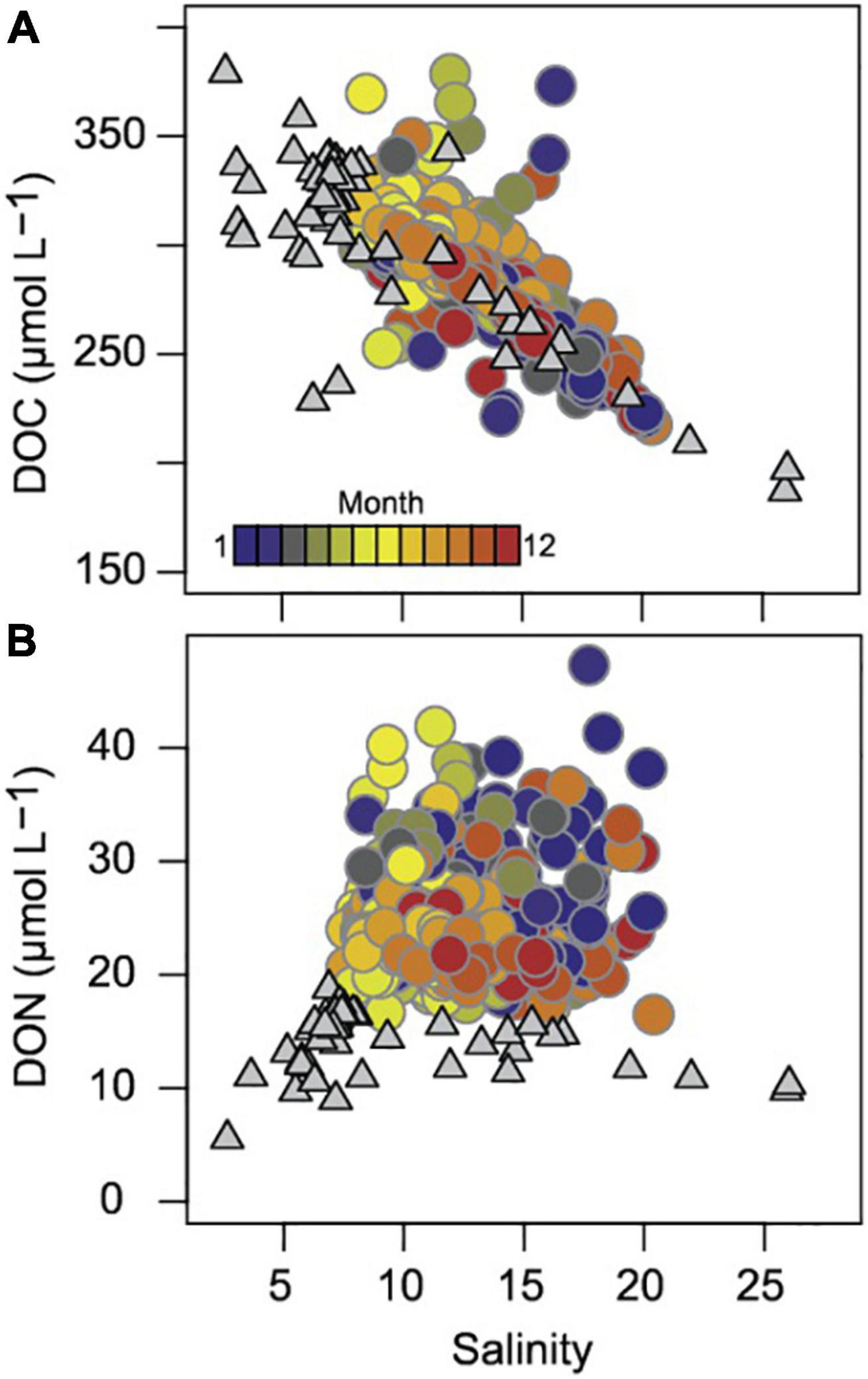
Figure 2. DOC (A) and DON (B) vs. salinity over the Baltic Sea surface water range (gray triangles) and at Heiligendamm (circles, colored by month). The Baltic Sea data are from R/V Meteor expeditions M86 and M87 (November 2011, June 2012) surface water (<70 m), including all stations except M87’s North Sea station S1 (Seidel et al., 2017).
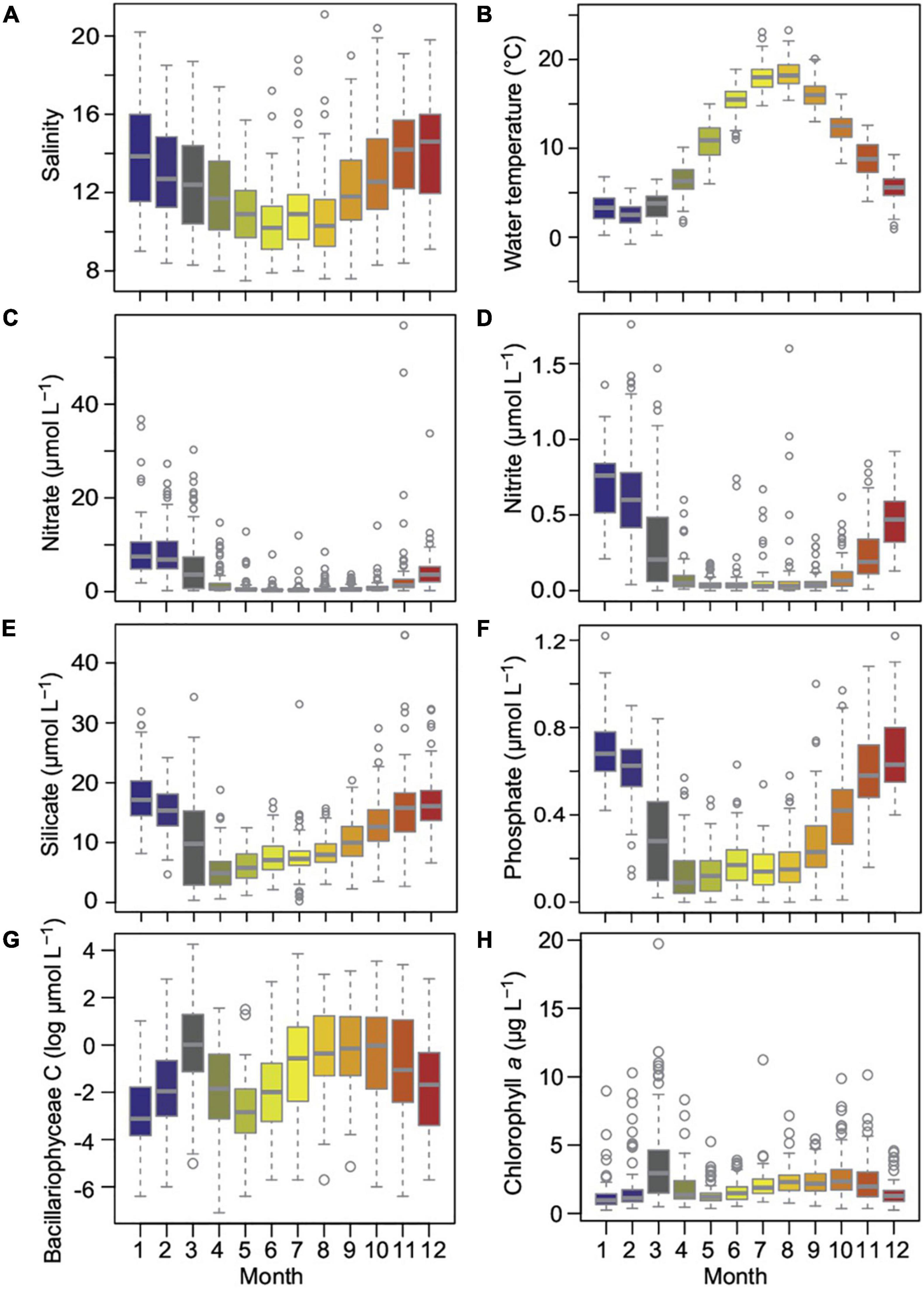
Figure 3. Annual cycles of salinity (A) and water temperature (B), inorganic nutrients (C–F), Bacillariophyceae carbon (G) exemplary as most abundant phytoplankton class, and chlorophyll a (H). Boxplots display minimum, first quartile, median, third quartile, and maximum, circles denote values outside the interquartile range. Colors are by month as in Figure 2.
At Heiligendamm, DOC contributed 290 ± 25 μmol L–1 (n = 535), while POC comprised 33 ± 19 μmol L–1 (n = 538). The major nitrogen fraction was DON with 17.2 ± 1.6 μmol L–1 (n = 502), followed by PON 4.8 ± 2.5 μmol L–1 (n = 538) and DIN 3.1 ± 5.5 μmol L–1 (n = 1575). DON concentrations at Heiligendamm were higher than in the Baltic Sea surface waters and not strongly correlated with salinity (Figure 2). DON concentrations, however, were likely overestimated, especially during winter months as ammonium could not be included in the calculation (see section “Materials and Methods”). POC and PON concentrations were overall more variable due to their high seasonal production and low background (Coefficient of Variation, CV: 58 and 51%) than DOC and DON concentrations (CV: 9% both). POC concentrations fluctuated most in March during the spring bloom. After a slight decrease during April and May, the summer and fall blooms persisted until September or October, after which POC decreased. DOC and DON were moderately positively related (Pearson’s R = 0.49, p < 0.001), while POC and PON were strongly positively correlated (Pearson’s R = 0.90, p < 0.001, Supplementary Figure 1). POC and DOC, as well as PON and DON, were weakly positively related with R < 0.15 (Pearson Product Moment correlation, p < 0.05). Over the annual cycle, the carbon-to-nitrogen ratio in the particulate phase (POC/PON) declined during the warm months, with the lowest values of 6.6 ± 1.3 from June to September (Figure 4). DOC concentrations were most variable during the winter months from October to February, and an increase in concentration exceeding the overall average of around 290 μmol DOC L–1 occurred between April and September. The carbon-to-nitrogen ratio in the dissolved phase (DOC/DON) was highest from May to August at 17.6 ± 1.0.
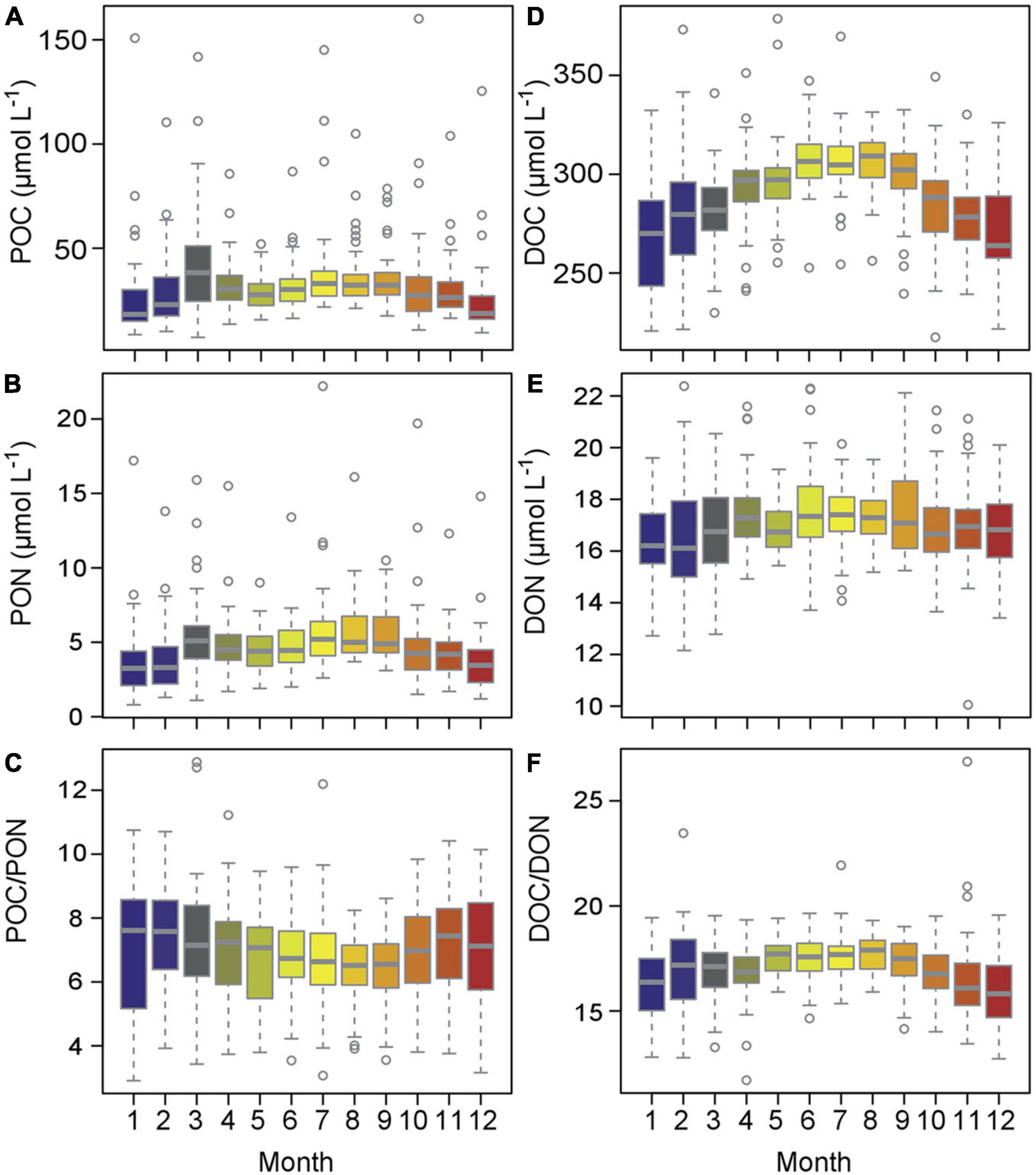
Figure 4. Annual cycles of POC, PON, POC/PON (A–C) and DOC, DON, DOC/DON (D–F). Boxplots display minimum, first quartile, median, third quartile and maximum, circles denote values outside the interquartile range. Colors are by month as in Figure 2.
Environmental Drivers of Organic Carbon and Nitrogen Fractions
Organic carbon and nitrogen fractions showed an overall consistent seasonal pattern but also high interannual variability that became evident when examining the yearly successions (Figure 3 and Supplementary Figure 2). For example, a distinct POC increase attributable to a spring bloom occurred in spring 2015 (days 40–120), but almost no increase during this time of the year was captured in 2018. DOC concentrations sharply declined at the onset of winter (2014, 2015, 2016) or showed almost no decrease after day 300 (2010, 2012, 2017, 2020).
We assessed the environmental drivers of organic carbon and nitrogen fractions via multiple linear regression (MLR) analysis including water temperature, salinity, precipitation, Chl a, biota carbon, inorganic nutrients nitrate, nitrate, phosphate, silicate, and additionally PON for DON and DOCSC. We tested the correlation of 1-day, 2-day, and 3-day wind speed means to POC and DOC as longer periods with elevated wind speed may be needed for significant wave generation and thus sediment resuspension. DOC was not related to any wind speed parameters, but we found the highest correlation to POC for 1-day means (Pearson’s R = 0.34), while correlations with 2-day and 3-day means were weaker (Pearson’s R = 0.22 and 0.20, respectively) and therefore used 1-day mean wind speed in the regression analyses. Including precipitation did not improve any regression, and also nitrite and nitrate were not included in any model as other parameters improved the models to a stronger degree, but cross-correlations should be considered here (Supplementary Figure 1). Repeated multiple linear regression analyses separately for warm and cold seasons did not considerably improve the models, and few significant model terms changed. DOC highly inversely correlated to salinity, and multiple linear regression analysis showed the best fit when including salinity, water temperature, Chl a and phosphate (adj. R2 = 0.69, model regression R = 0.83, degrees of freedom DF = 517). Only in the cold season were precipitation and silicate important to describe DOC concentrations, while wind speed weakly contributed during warm months and phosphate was excluded. The correlation of DON with salinity was weak (Pearson’s R = 0.33, p < 0.001), although presumably underestimated as ammonium was not included in the calculation of DON, but weakly positively correlated to salinity (Pearson’s R = 0.38, p < 0.001). No satisfactory fit was found for DON when excluding DOC as a potential parameter. The best fit was obtained when including salinity, PON, and Chl a (adj. R2 = 0.15, regression R = 0.39, DF = 493), Chl a and PON, however, did not provide explanatory power to cold season DON. Similarly, no good fit was found for DOCSC, with the best model including water temperature and phosphate (adj. R2 = 0.14, regression R = 0.48, DF = 519). POC was best modeled with biota carbon, Chl a, water temperature, phosphate, and 1-day mean wind speed (adj. R2 = 0.56, regression R = 0.75, DF = 419). When POC concentrations are low during the cold season, only Chl a and biota carbon contributed significantly to the MLR (adj. R2 = 0.50, Pearson’s R = 0.71, DF = 115). For the years 2010 and 2011, phaeopigment concentrations were available along with POC. This data showed that POC was best approximated including Chl a and phaeopigment concentrations (adj. R2 = 0.78, regression R = 0.89, DF = 45). PON strongly correlated to POC (Pearson’s R = 0.90, p < 0.001) and was best approximated including Chl a, biota carbon, temperature, and wind speed, (adj. R2 = 0.42, regression R = 0.65, DF = 425). Wind speed was not a significant term in warm season MLR, but salinity contributed significantly (adj. R2 = 0.38, regression R = 0.63, DF = 293).
Long Term Trends
Time series analyses revealed that air temperature increased since the start of the measurements in 1947 in Warnemünde at 0.027°C yr–1 (Table 1). The air temperature increase, calculated including only the daily means of each sampling day, was only slightly higher at 0.029°C yr–1. Water temperature, monitored since 1988 through the weekly campaign, increased by 0.037°C yr–1 (Table 1 and Figure 5). Precipitation did not change over time. Inorganic nutrients nitrate, nitrite, and phosphate significantly decreased since 1989. Especially for phosphorus, the concentrations decreased strongly until the late 90s, after which the pattern flattened. Silicate concentrations increased by 0.046 μmol L–1yr–1 over all data, but not significantly between 2010 and 2020. Chl a significantly increased, and the increase accelerated to 0.086 μg L–1 yr–1 in the 2010 to 2020 period. Of the phytoplankton carbon contributions, Bacillariophyceae and Cryptophyceae carbon increased, while that of the photosynthetic ciliate Mesodinium rubrum decreased. Salinity increased at the nearshore station since 1988. No significant change was found for DOC, POC, DON, and PON monthly mean concentrations. The contribution of DON to total dissolved nitrogen did not significantly change over time even when inorganic nitrogen species concentrations declined. However, DOCSC increased by about 0.6 μmol L–1yr–1 since the start of the observation in 2010. The cross-correlation of POC and DOC observations revealed significant peaks of DOC at lags of 0–1 month after POC peaked. The decomposition of the time series into seasonal, trend, and random fractions provided better insight, showing in the seasonal decomposition that the POC peaked first, followed by a broad DOC peak that overlapped with a second peak in POC after April (Figure 6). The DON seasonal decomposition was more similar to the POC pattern with two distinct peaks for spring and summer/fall blooms than to the DOC pattern.
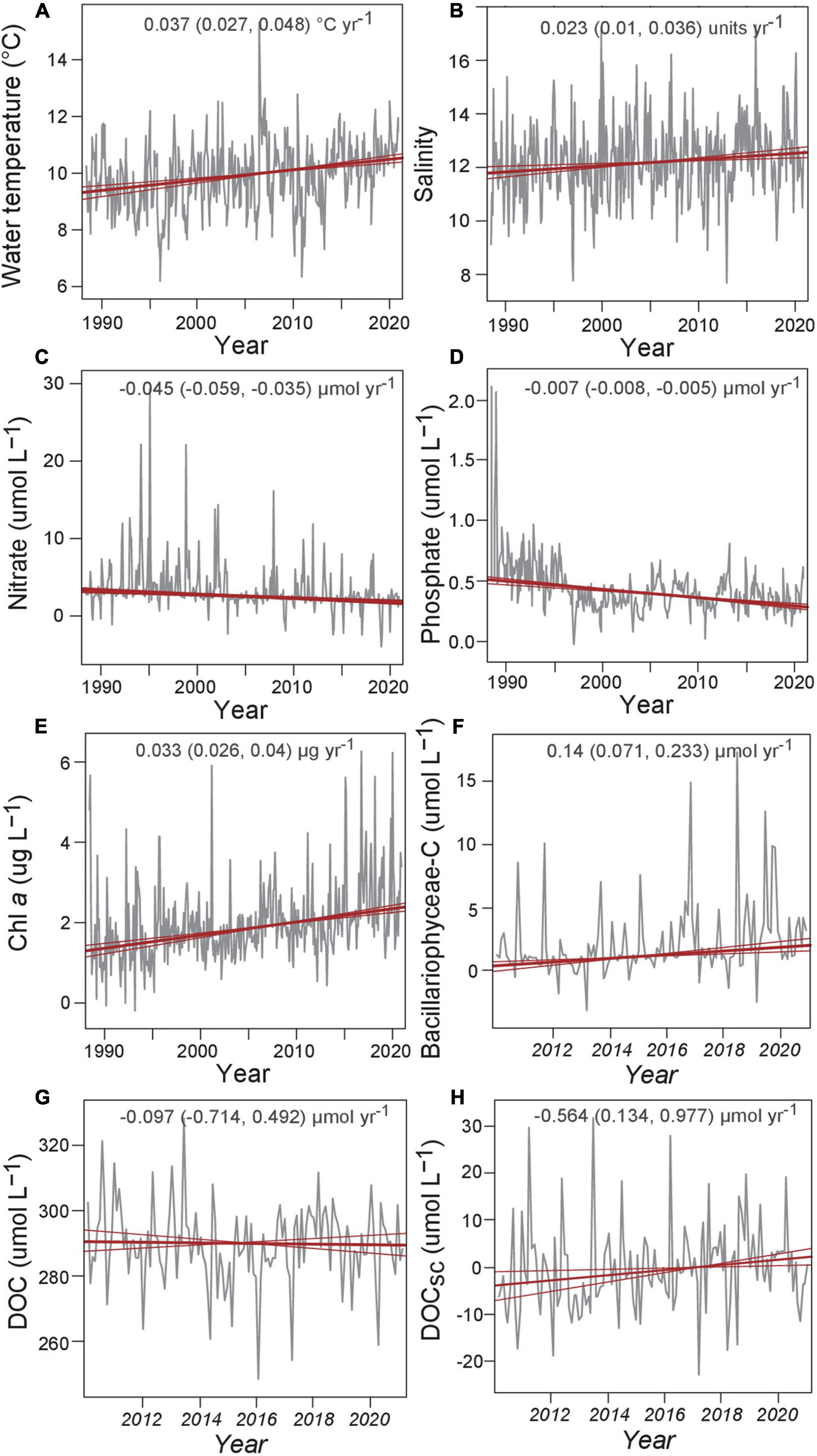
Figure 5. Deseasonalized monthly means of selected water parameters at Heiligendamm Pier. Thick red lines show the Theil-Sen trend estimate and the thin red lines show the 95% confidence intervals for the trend based on resampling methods. The overall trend is given at the top, as well as the 95% confidence intervals in the slope. Note different time spans in panels (A–E) and (F–H).
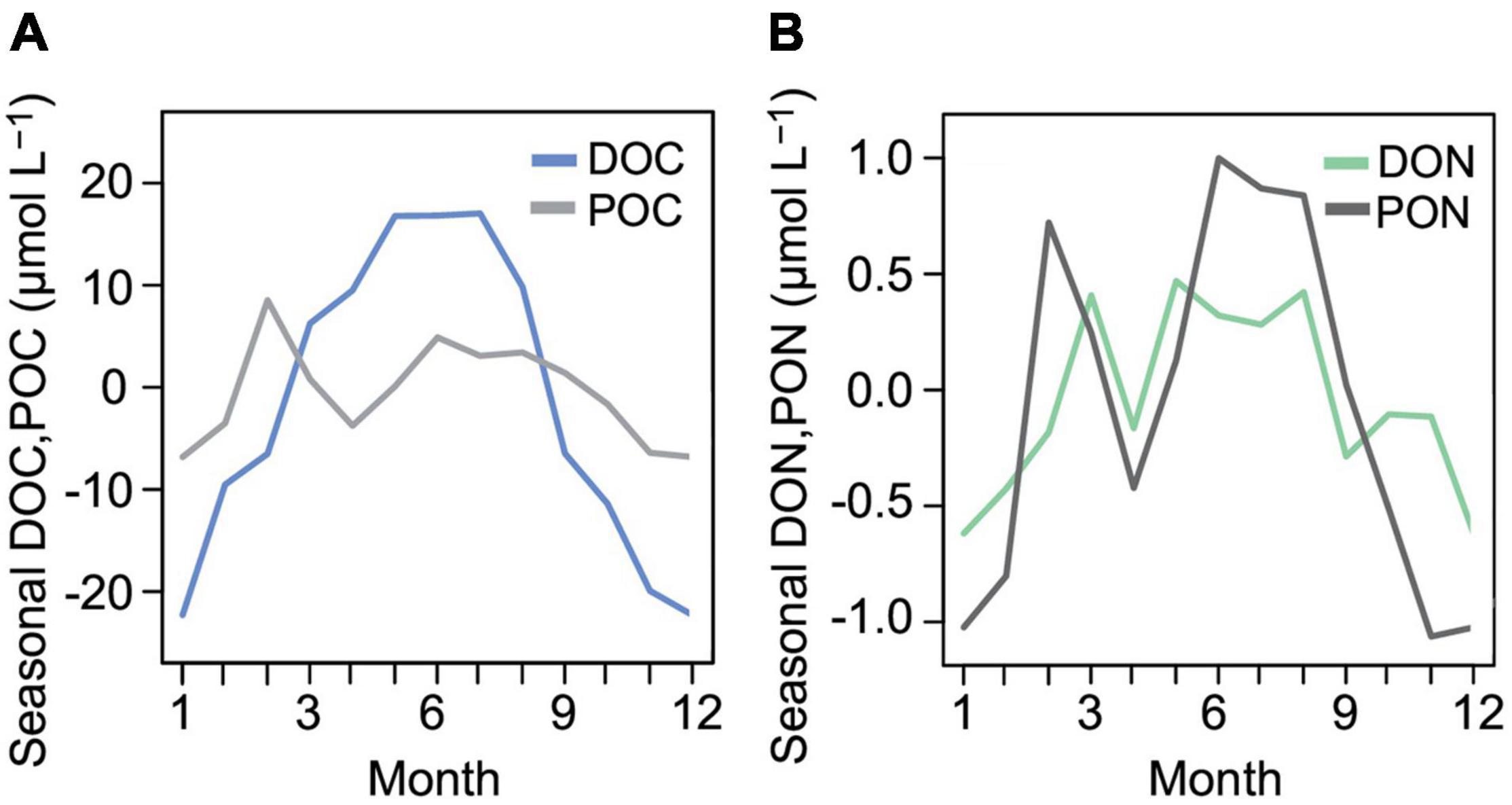
Figure 6. Seasonal cycle of DOC and POC (A) and DON and PON (B) from time series decomposition including 11 years of weekly measurements.
Discussion
Concentrations and Drivers of Particulate and Dissolved Organic Matter Fractions
Mixing of saline North Sea water with Baltic Sea water of lower salinity impacted by riverine input, especially to the Bothnian Bay and the Gulf of Finland, groundwater influx, primary productivity in the warm period, particle sinking and sediment resuspension, microbial degradation, photodegradation, and flocculation have previously been described to impact organic carbon and nitrogen concentrations in the water column of the Baltic Sea (Gustafsson et al., 2000; Kuliński and Pempkowiak, 2008; Nausch et al., 2008; Hordoir and Meier, 2010; Kulinski and Pempkowiak, 2012; Szymczycha et al., 2014; Hoikkala et al., 2015; Kuliński et al., 2016; Niemistö and Lund-Hansen, 2019; Piontek et al., 2019; Voss et al., 2020). Particulate and dissolved organic carbon and nitrogen seasonal progressions were highly variable between the years, as also observed in other marine settings, e.g., the Fram Strait (Engel et al., 2019), but a clear pattern emerged from the long term data series. Out of the numerous potential drivers of organic carbon and nitrogen in particulate and dissolved form, we confirmed water mixing and primary production as the most important drivers to the nearshore setting sampled weekly at Heiligendamm Pier.
POC peaked during phytoplankton bloom phases and was moderately well explained by biota carbon, Chl a, and wind speed. Results from 1 year (phaeopigment data only available for 2010) showed that including phaeopigment analysis may improve POC prediction to a level of confidence similar to DOC. PON dynamics were tightly coupled to POC and best approximated using similar parameters, prominently including Chl a and biota carbon. The proportion of Chl a to POC is variable across ecosystems (Graff et al., 2015) and also in the Northern Baltic Sea (Andersson and Rudehäll, 1993) according to predominant species and the state of the bloom, which explains why additional primary production indicators improved POC prediction. POC and PON concentrations were within the range of those observed in the surface waters of the Gdansk Deep and the Arkona and Bornholm Basins, but higher than values observed at deeper stations in the Baltic Proper (Nausch et al., 2008; Szymczycha et al., 2017; Winogradow et al., 2019), where the terrigenous impact is less strong and vertical particle flux exports POM from the upper water column; additionally, resuspension events are much less frequent and influence bottom waters only (Christiansen et al., 2002). POC concentrations were in the upper range of those observed over an annual cycle in the central North Sea, PON concentrations were slightly increased. This results in POC/PON ratios at Heiligendamm below those in the North Sea, where high fall and winter values > 10 were attributed to resuspension from bottom sediments at water depth mostly exceeding 30 m, which likely contain more degraded material than the shallow sediments at Heiligendamm (Suratman et al., 2009). DOC comprised the largest fraction of the organic carbon pool at Heiligendamm Pier, contributing 90 ± 5% overall, and was most strongly driven by water mixing. In the brackish setting of Heiligendamm, located in Mecklenburg Bay, strong salinity variations between 7.5 and 21 occurred. Water is continuously exported through the Danish Straits, and favorable wind conditions, especially during the winter months, lead to an influx of North Sea water, carrying less DOC at concentrations typically below 120 μmol L–1 (Döös et al., 2004; Osterholz et al., 2016b). A seasonal DOC signal was detected on the high background concentration, though small: warm season (April to October) DOC averaged around 301 μmol L–1 and only dropped to around 281 μmol L–1 during cold months (November to March), resulting in less than 10% change for all within-year differences, which lies within the ranges previously reported for the Baltic Proper (Hoikkala et al., 2012; Maciejewska and Pempkowiak, 2014). Considering that the main drivers of DOC concentrations work in the same direction from warm to the cold season, this constitutes a small change: low-DOC North Sea water influx decreases DOC concentrations in the southern Baltic Sea in winter through dilution, remineralization slows down at low temperature but still occurs, and primary production that would lead to increases in DOC is almost absent. Seasonal DOC accumulation is generally thought to be stronger in oligotrophic to mesotrophic environments such as the Sargasso Sea or the Western Mediterranean Sea (Copin-Montégut and Avril, 1993; Carlson et al., 1994), and DOC easily doubles in the coastal Dutch North Sea over the annual cycle (Suratman et al., 2009; Sintes et al., 2010). However, even in the eutrophic Black Sea, the seasonal DOC accumulation resulted in an increase from 210 to 280 μmol C L–1 in summer. The high terrigenous background DOC, large scale mixing through the basin, and rapid cycling of available organics are likely responsible for the small DOC variability observed at Heiligendamm. DON concentrations at Heiligendamm were high and varied little over an annual cycle. DON concentration across the Baltic Sea salinity transect reveals a source of DON in the mid-salinity range and high DON concentrations at the Heiligendamm Pier compared to the open water stations (Seidel et al., 2017; Figure 2). DON export to the Baltic Sea has been shown to increase with agricultural land use and is dominant in the southern Baltic Sea catchments (Seitzinger et al., 2002; Thomas et al., 2003; Stedmon et al., 2006; Voss et al., 2020). DON release from autochthonous sources such as the abundant cyanobacteria (Voss et al., 2005) was also hypothesized as a potential DON source to the Baltic Sea water column by Seidel et al. (2017).
An additional parameter that potentially contributes to the observed organic nutrient pattern is, amongst others, benthic flux, e.g., diffusive or through submarine groundwater discharge (SGD). SGD along the Polish and German Baltic Sea coastline is estimated to be responsible for a DOC flux of 11 ± 1kT to the coastal waters, representing around 10% of the carbon load discharged with river runoff (Szymczycha et al., 2014). In the Hel Bight, groundwater introduces phosphate and silicate to the Baltic Sea, promoting increased seep-site specific net community production but lower microbial respiration due to the input of recalcitrant DOC (Donis et al., 2017). von Ahn et al. (2021) describe groundwater from different sources in shallow sediments of the Wismar Bay close to Heiligendamm. The 224Ra activities especially in the confined, southeastern Wismar Bay were much higher than those detected at sampling stations along the coastline, including Heiligendamm, where water exchange with the open Baltic Sea is much stronger. Parameters suitable for SGD detection, such as radon and radium, or water isotopic compositions, were not determined in our study. Using e.g. silicate, phosphate or DOC to trace SGD to the coastal waters is a challenge in the examined system. Szymczycha et al. (2014) show variation in SGD flow, highly correlated to precipitation, which can entail runoff from land and increased river/drainage channel discharge, also introducing high nutrient loads. We examined nutrient-salinity-temperature diagrams but found no pattern hinting at specific sources in the mixed surface water sample.
Not all possible drivers of DOM and POM concentrations could be assessed with the available parameter set. Severe weather conditions could, due to strong wind and rain, lead to short-term, local increases in particulate or dissolved carbon and nitrogen concentrations from terrigenous inputs or resuspension. Naturally, the processes discussed here best apply to the mid-salinity region of the Baltic Sea. Across the salinity gradient of the Baltic Sea, the contributions of the processes can vary, e.g., as described for Indian estuaries along a latitudinal gradient, where relative contributions of terrestrial and phytoplankton particulate carbon and nitrogen as well as agricultural impact vary from North to South. Nevertheless, the coastal type around Heiligendamm Pier is representative of much of the coasts found along the ∼8,000 km of Baltic Sea shoreline (Labuz, 2015), and we capture main processes affecting estuarine DOM and POM described previously (Hoikkala et al., 2012; Maciejewska and Pempkowiak, 2014; Osterholz et al., 2016a).
Impact of Severe Weather Events and Major Baltic Inflows
At the shallow sampling station, sediment resuspension, increased terrigenous organic matter supply from the land through drainage channels or small rivers potentially impact inorganic and organic nutrient concentrations during periods of severe winds and precipitation on short timescales. Wind speed was overall weakly correlated to POC. The fact that 1-day means of wind speed more strongly correlated to POC than the respective 2-day and 3-day means indicated an immediate effect of short-term disturbances in the shallow system. Besides the weak correlation of POC and wind speed, no further correlations were detected between weather parameters (speed, direction, precipitation) and organic matter concentrations or inorganic nutrients that deviated from patterns observed in conjunction with salinity.
In addition to overall correlations, we examined some single events in detail. In 2011, unusually high rainfall occurred in July and August (Mehl et al., 2014), especially in the coastal region west of Heiligendamm. This was not mirrored in DOM and POM concentrations. A storm surge on January 4–5 2017 did not lead to notable increases in particulate or dissolved carbon and nitrogen concentrations. A storm event on January 4–5, 2019, broke the dyke of the coastal peat bog Hütelmoor east of Heiligendamm. During this time, a distinct peak in POC occurred, but no increase in DOC was visible. These few events showed that the weather events can impact organic matter supply in the shallow waters, but no consistent observations could be made using the weekly sampling data, indicating that a weekly sampling is too scarce to detect such momentary events in a shallow coastal environment.
The particulate and dissolved organic matter time series covered the period of a Major Baltic Inflow (MBI) event that took place between December 2014 and November 2015. MBIs transport saline, oxygenated North Sea water to the deep basins of the Baltic Sea and are characterized by the co-occurrence of oxygen with salinity ≥ 17.2 for the Bornholm Basin (Gräwe et al., 2015; Mohrholz et al., 2015; Mohrholz, 2018). However, the MBIs are only observed along the deep channels through which the saline waters propagate toward the Baltic Proper, not in the shallow, nearshore waters. Accordingly, no salinity extremes or impacts on nutrients were observed at Heiligendamm during winter 2014/2015 MBI.
Relationships Between Organic Carbon and Nitrogen Fractions
Succession and proportions of the major elements in DOM and POM, carbon and nitrogen, are indicators of organic matter source, cycling and quality. Within the microbial loop, photosynthetic microbes fix CO2 and contribute to the POM pool. Some DOM is released by living cells, but most of it is released upon cell death and lysis to the microbial community (Azam et al., 1983), leading to a peak in DOC coinciding or shortly after the peak in phytoplankton biomass or POC. Huang et al. (2018), however, showed that the correlation between POC and DOC is strong for global, combined data of lakes, oceans and coastal waters but weak for individual water types. Accordingly, the correlation of POC and DOC was not strong at the Heiligendamm station. Time series cross-correlation based on monthly mean data showed a significant lag of DOC zero to 1 month after POC; thus the monthly interval is too short to examine this relationship in detail. Time series decomposition revealed different modes for POC and DOC: POC peaked twice, reflecting a short but intense spring bloom followed by a longer summer/fall bloom peak, while DOC peaked unimodal with a maximum in July. However, the spring bloom-induced rise in POC started only very shortly before the DOC peak. These patterns were not evident from the concentrations over single years (Supplementary Figure 2); only through time series decomposition a seasonal signal emerged. The detrended data revealed that the seasonality in DON, as well as the salinity-corrected DOCSC, were more similar to the seasonality of POC and PON than to DOC, indicative of a POM source. The POC/PON ratio determined at Heiligendamm (6.9 ± 1.6) was on average only marginally above the C/N ratio of planktonic organisms of 6.6 according to Redfield (1934), denoting a predominantly phytoplankton origin. POC/PON ratios in the open ocean increase with depth due to the preferential remineralization of PON during sinking (Schneider et al., 2003), imprinted here in the elevated POC/PON ratios toward winter as remineralization progresses.
DON concentrations at Heiligendamm Pier (17 ± 1.6 μmol L–1) and much of the Baltic Sea are higher than values determined for the coastal and open North Sea with 11 and 5 μmol L–1 (Van Engeland et al., 2010; Hoikkala et al., 2015). Preferential uptake of organic nitrogen over carbon is observed in nutrient-limited systems, and as inorganic nitrogen species are depleted early in the season, the DON pool presents a potential nutrient source also in the Baltic Sea (Veuger et al., 2004; Bronk et al., 2007). However, due to the high standing stocks and partial decoupling from DOC dynamics, this seems to be valid only for a part of the DON pool here. Knudsen-Leerbeck et al. (2017) found a similar proportion of DON to the total nitrogen pool in the Great Belt and Roskilde Fjord (∼70%) as was present at Heiligendamm, and could attribute 17 ± 5.5% of the DON as bioavailable. Accordingly, small, rapidly cycled molecules such as free amino acids, urea or amines make up only a small portion of the DON pool, while non-hydrolyzable amide-N compounds are major constituents of the deep ocean high-molecular weight DON pool that resists further biodegradation on long timescales exceeding hundreds or even thousands of years (McCarthy et al., 1997; Aluwihare et al., 2005). This fraction of complex biomolecules is likely to also accumulate in the land-locked Baltic Sea.
Besides a refractory DON pool, the Baltic Sea also holds a large amount of persistent DOC. The background levels in the Baltic Sea are 5–10 times higher than organic matter production through photosynthetic processes (Nausch et al., 2008). The narrow entrance to the North Sea and the succession of sills and basins in the Baltic Sea allow little exchange with the North Sea (Schneider and Müller, 2018), so that the DOC in the Baltic Sea carries a strong terrigenous signal. The concentration of suspended lignin phenols, for example, decreased from north to south along the salinity gradient of the Baltic Sea (Bianchi et al., 1997; Hernes and Benner, 2003), and terrestrial solid-phase extractable carbon decreased from ∼100% near the Kalix River outflow to the Bothnian Bay to 53% in the North Sea water inflow in the deeper Kattegat water (Seidel et al., 2017). Terrigenous DOM is usually depleted in nitrogen (Benner et al., 2005; Sipler and Bronk, 2015), and even though DON concentrations are high, this holds true for the Baltic Sea DOM. DOC/DON values are around 12–14 in the North Sea and other surface oceans, and increase to 15–34 in deep waters that hold primarily refractory DOC (Hopkinson and Vallino, 2005; Van Engeland et al., 2010; Hansell, 2013). The unfavorable C/N ratio in both terrigenous and deep ocean DOM, derived from preferential degradation of nitrogen in the deep ocean, and intrinsic to terrestrial humic acids, which are less aliphatic than marine humic acids (Stuermer et al., 1978), render them less attractive to microbial degradation and contribute to DOM accumulation in the Baltic Sea. Nevertheless, stable carbon isotope ratios of high-molecular weight (HMW) DOM revealed non-conservative behavior and removal of terrestrial HMW DOM in the Baltic Sea (Alling et al., 2008; Deutsch et al., 2012). Inferring terrigenous contribution from C/N ratios of DOM alone is difficult in the southern Baltic Sea, where riverine freshwater inputs from the north have already been mixed during circulation through the Baltic Proper, and the few available DOC/DON ratios vary widely (Voss et al., 2020).
Long Term Trends
The inflow of DOC into the land-locked sea is roughly balanced with the outflow of DOC through the Danish Straits, but this balance will remain only as long as the biological turnover and/or the hydrodynamic situation in the Baltic Sea do not experience disturbances (Nausch et al., 2008). The long term development of the organic nutrients POC, PON, DOC and DON, which comprise an important, actively processed fraction of the global carbon cycle, is directly related to primary productivity but is much less researched in the Baltic Sea region.
We showed that inorganic N and P concentrations, as important nutrient supply to primary producers, significantly decreased at the Heiligendamm time series station. This development was previously described by Kuss et al. (2020) for the Western Baltic Sea and at the Boknis Eck time series station at the entrance of the Eckernförde Bay, regularly sampled since 1986 (Lennartz et al., 2014), and attributed to decreased inputs through freshwater endmembers where improved water management strategies imposed by HELCOM and EU reduced anthropogenic pressure on the ecosystem (Reusch et al., 2018). Similarly, the total Baltic Sea nitrogen load decreased by 31 kiloton yr–1 in recent years, with regional differences according to the main source, i.e., coastal areas are dominated by riverine inputs that peaked in the 1980s to early 1990s, or atmospheric deposition and N2 fixation more strongly impacting the Baltic Proper (Voss et al., 2005; Lønborg and Markager, 2021). Corresponding decline in river nutrient loads was shown for many areas in northern Europe since the 1980s (De Jonge, 1997; de Vries et al., 1998; Stålnacke et al., 2004; Soetaert et al., 2006). However, ecosystem responses are not straightforward in the sense that reduced nutrient inputs lead to a reduction in primary production and alleviate eutrophication, as manifested in the increase of Chl a concentration at the Heiligendamm time series station. The effects of decreased nutrient influx are counteracted by internal nutrient feedback as well as changes in water clarity, light climate, solar radiation, and temperature with complex implications such as species shifts and prolonged growing periods (Emil et al., 2007; McQuatters-Gollop et al., 2007; Dupont and Aksnes, 2013; Wasmund et al., 2019).
Higher temperature can induce species shifts and promote heterotrophic metabolism and thus affect organic matter recycling more strongly than the autotrophic process of carbon fixation (O’Connor et al., 2009). Air temperature at the closest weather station (since 1947) reflected globally increasing air temperature at a rate of 0.029°C yr–1 at the southern Baltic Sea coast, similar to the trend reported by Deininger et al. (2020) for all of Norway. The change point detected in the air temperature time series in 1988 was close to the global change point in 1987 attributed to rapid climate change and natural forcing (Reid et al., 2016). Warming propagated into the shallow water column at Heiligendamm Pier, where water temperature increased by 0.027°C yr–1. This value was the same as the water temperature increase of 0.02°C yr–1 reported for surface water at Boknis Eck (Lennartz et al., 2014) and 0.03°C yr–1 reported for the North Sea/Baltic Sea region by Mackenzie and Schiedek (2007). Here, no significant change point was detected. Low- and mid-latitude coastal waters were shown to actually experience cooling after 1998, termed the hiatus period (Liao et al., 2015), but the signal was mixed in the Baltic Sea region, and water temperature change only including the time after 1998 at Heiligendamm remained positive at 0.040°C yr–1 and thus even accelerated. Our findings contrast the results by Omstedt and Nohr (2004), who found no increase in Baltic Sea annual mean temperature and no trend in Baltic Sea net heat flux during their study period from 1970 to 2002, suggesting that the very nearshore waters respond differently to global warming.
In the Baltic Sea, especially in the southwest, salinity depends strongly on wind flow and river runoff (Meier and Kauker, 2003; Omstedt and Nohr, 2004), although direct influence of the largest river in the area, the Warnow River discharging into the Baltic Sea in Warnemünde ∼ 20 km east of Heiligendamm, is not observed as the plume is usually transported eastward with the main Baltic Sea circulation (Jurasinski et al., 2018). At Heiligendamm, despite the high fluctuation, a seasonal signal with increased salinity in winter from North Sea water inflow emerged, and salinity significantly increased over time. The saline waters carry less DOC, which could lead to a decrease in bulk DOC concentration in the southwestern basins and the Baltic Proper, but there are more consequences to this pattern: higher saltwater input in the south and higher freshwater input in the north amplify the north-south salinity gradient of the Baltic Sea with complex ramifications for species distributions and element cycling, extending to organic carbon fractions.
At Heiligendamm, the bulk DOC, DON, POC, and PON concentrations did not change significantly within the examined time frame from 2010 to 2020, though several recent studies show increased carbon and nitrogen concentrations in rivers that are exported to the coastal ocean in different northern European regions (Worrall et al., 2004; Deininger et al., 2020; Lepistö et al., 2021). Unlike Deininger et al. (2020), who found DON to the Skagerrak from Norwegian rivers to increase from 1990 to 2017, we detected no change in DON concentration. This may be due to the fact that our time series for DON starting in 2010 was much shorter than their dataset covering 27 years. Also, it did not cover the period of the most pronounced decrease in nitrate and nitrite in the late nineties. On the other hand, Van Engeland et al. (2010) covered a crucial time frame in N-reduction in their analysis of DON in the North Sea from 1995 to 2005, but were not able to detect significant trends. Consequently, the length of the observed dataset may not be sufficient, but also intense water mixing and organic matter (OM) cycling in the coastal and open ocean settings may blur trends in DON. Further, at our study site, there was no consistent change in bulk DOC over time, but the salinity-corrected fraction of DOCSC increased by 0.56 μmol L–1yr–1. Elevated bulk DOC concentrations were found in water bodies throughout Great Britain (Worrall et al., 2004). Elevated TOC concentrations were also shown for forested Finish catchments (Lepistö et al., 2021), but export from Finnish rivers into the Baltic Sea did not significantly increase in a 36-year period from 1975 (Räike et al., 2012). High uncertainties exist regarding future discharge to the Baltic Sea (Meier et al., 2006a), and the effect may be stronger in in the northern Baltic Sea where riverine input from forested catchments is high compared to Heiligendamm. DOC can be actively removed through microbial metabolism or can be lost through flocculation, aggregation and precipitation (Eisma, 1986; Hullar et al., 1996) in the river estuaries before reaching the Baltic Sea where it is mixed into a large water body, dampening the effects of increased concentrations in inland waters. The DOCSC increase was paralleled by an increase in Chl a since 2010 at Heiligendamm station. For other parts of the Baltic Sea, long-term trends in primary productivity through Chl a are mixed: prolongation of the growing period is visible through time shifts in the appearance of certain diatom species and less in augmented Chl a (Kahru et al., 2016; Wasmund et al., 2019). The occurrence of the widespread cyanobacteria blooms oscillates between years, regulated in part by the amount of available phosphate, water temperature and weather conditions, but overall driving factors are less well studied (Kahru et al., 2018). In a recent study, Beltran-Perez and Waniek (2021) defined the favorable conditions defining the phenology of the cyanobacteria for the Gotland Basin using water and air parameters. They argue that the type of heat exchange between water surface and overlying atmosphere plays an underestimated role in bloom onset and demise. Though cyanobacteria are not the main contributors to phytoplankton C at Heiligendamm, observed changes in water temperature likely impact phytoplankton community composition.
Primary production and Chl a were elevated in the northern Baltic Proper, the Gulf of Finland, and the Gulf of Gdansk, while the Baltic Proper experienced a decline in line with the decreased eutrophication of the Baltic Sea (Fleming et al., 2008; HELCOM, 2018; Zdun et al., 2021). At Heiligendamm, as the DOC/DON ratio, total DON and DOC, POC and PON, and %DON of TDN did not reveal significant changes over time so that the composition and fate of the DOCSC are unclear. As a potential source, we found the Bacillariophyceae carbon to increase over time at Heiligendamm, though at a rate 3.6 times lower than the DOCSC. Cryptophyceae carbon increased as well, but together with Bacillariophyceae still does not add up to the observed increase in DOC. Ciliate carbon was dominated Mesodinium rubrum. The phototroph ciliate has been shown to be abundant in the Arkona Basin in summer (Setälä and Kivi, 2003), and is important in element cycling in upwelling and coastal areas, excreting 1.8–12.5% of total fixed carbon but also actively taking up amino acids and DOC (Smith and Barber, 2008). Due to these roles, its decreasing abundance is difficult to interpret. In the northern parts of the Baltic Sea, especially in the Gulf of Finland, an ongoing shift from diatom dominance toward dinoflagellates has been observed (Spilling et al., 2018). However, Wasmund et al. (2011) showed increasing numbers of Bacillariophyceae in fall in the southwestern Baltic Sea since 2000. Nutrient availability, temperature, and other environmental factors impact phytoplankton C/N ratios. Diatoms exhibit very variable C/N ratios (C/N 5–9), and Dinophyceae C/N ratios are usually high [C/N 7–9, Garcia et al. (2018)]. Increased Bacillariophyceae have, however, not yet impacted the C/N ratios determined at the time series station. Further, dead diatoms sink out of the euphotic zone more quickly than dinoflagellates, which either form inert spores or lyse during sinking (Spilling et al., 2018), thereby affecting the water column nutrient supply in the opposite direction to the increase in DOC observed at Heiligendamm. Cryptophyceae carbon quality has not been assessed, but it is known that different phytoplankton species release varying DOM. For example, cyanobacteria-OM is less easily degraded by microorganisms but still more easily degraded than humic OM (Landa et al., 2014; Liu et al., 2020; Patriarca et al., 2020). The direct connections between changes in phytoplankton abundance and community composition to DOM and POM at Heiligendamm remain to be established, but the composition of the additional DOM is of direct consequence for its fate, either stimulating bacterial turnover or accumulating in the land-locked Baltic Sea Basin. In the long run, this unbalances the stable organic carbon concentrations in the system maintained out through the water exchange with the North Sea. Overall, the equilibrium between POC and DOC production impacts the food web, the interactions of phytoplankton and heterotrophs, and ultimately the carbon sequestration by the biological pump (Karl et al., 1998).
Conclusion
The time series station at Heiligendamm provides valuable insights into nearshore nutrient cycling in the Baltic Sea and reveals that seasonal cycles can be detected even in a highly dynamic ecosystem. Particulate organic carbon and nitrogen concentrations in the nearshore Baltic Sea closely follow phytoplankton patterns, indicating biotic sources. Dissolved organic carbon and nitrogen seasonal annual cycles are less pronounced due to the high standing stocks and presumed rapid cycling of the available fractions. Significant changes in temperature and Chl a over time, however, were not (yet) matched by changes in bulk organic carbon and nitrogen fractions. A weak increase in the salinity-corrected DOC fraction DOCSC was detected, warranting further observation as impacts of climate change on biochemical cycles are expected. The setting in the southwestern Baltic Sea is representative of many estuarine, brackish coastal systems worldwide under high anthropogenic pressure. Not all observations can be explained with the currently measured parameters, and water and carbon isotopic analyses are currently implemented; autonomous sensor systems to assess shorter-term variability (<1 week) are considered. The role of organic matter quantity and quality in transport and fate of pollutants needs to receive more attention in the anthropogenically influenced Baltic Sea with high maritime, shipping, and agricultural impacts in the future. All water column data are available to the scientific community upon registration through the oceanographic database of the Leibniz Institute for Baltic Sea Research Warnemünde, ODIN 2 (see text footnote 1).
Data Availability Statement
The data presented in this study can be found in online repositories. The parameter identifiers are given in Supplementary Table 1, the database can be accessed through odin2.iowarnemuende.de.
Author Contributions
CB, SB, MD, JJ, LK, and BS performed weekly sampling and analyzed the samples. HO analyzed the data, with inputs from HF, AK, JJW, and DES-B. HO wrote the manuscript. All authors critically reviewed the final version, agreed to be listed, and approved the submitted version.
Conflict of Interest
The authors declare that the research was conducted in the absence of any commercial or financial relationships that could be construed as a potential conflict of interest.
Publisher’s Note
All claims expressed in this article are solely those of the authors and do not necessarily represent those of their affiliated organizations, or those of the publisher, the editors and the reviewers. Any product that may be evaluated in this article, or claim that may be made by its manufacturer, is not guaranteed or endorsed by the publisher.
Acknowledgments
The authors are indebted to everyone involved in the time-consuming sampling and analyses over the years. In particular, this work would not have been possible without the contributions of former IOW colleagues Klaus Nagel, Monika Nausch, Günther Nausch, and Norbert Wasmund. The costs for personnel, infrastructure and analyses of the collected samples were entirely covered by the Leibniz Institute for Baltic Sea Research.
Supplementary Material
The Supplementary Material for this article can be found online at: https://www.frontiersin.org/articles/10.3389/fmars.2021.795028/full#supplementary-material
Footnotes
References
Aksnes, D., Dupont, N., Staby, A., Fiksen, Ø., Kaartvedt, S., and Aure, J. (2009). Coastal water darkening and implications for mesopelagic regime shifts in Norwegian fjords. Mar. Ecol. Prog. Ser. 387, 39–49. doi: 10.3354/meps08120
Alling, V., Humborg, C., Mörth, C.-M., Rahm, L., and Pollehne, F. (2008). Tracing terrestrial organic matter by δ34S and δ13C signatures in a subarctic estuary. Limnol. Oceanogr. 53, 2594–2602. doi: 10.4319/lo.2008.53.6.2594
Aluwihare, L. I., Repeta, D. J., Pantoja, S., and Johnson, C. G. (2005). Two chemically distinct pools of organic nitrogen accumulate in the ocean. Science 308, 1007–1010. doi: 10.1126/science.1108925
Andersson, A., and Rudehäll, Å. (1993). Proportion of plankton biomass in particulate organic carbon in the northern Baltic Sea. Mar. Ecol. Prog. Ser. 95, 133–139. doi: 10.3354/meps095133
Asmala, E., Haraguchi, L., Markager, S., Massicotte, P., Riemann, B., Staehr, P. A., et al. (2018). Eutrophication leads to accumulation of recalcitrant autochthonous organic matter in coastal environment. Glob. Biogeochem. Cycles 32, 1673–1687. doi: 10.1029/2017GB005848
Azam, F., Fenchel, T., Field, J. G., Gray, J. S., Meyerreil, L. A., and Thingstad, F. (1983). The ecological role of water-column microbes in the sea. Mar. Ecol. Prog. Ser. 10, 257–263. doi: 10.3354/meps010257
Barrón, C., and Duarte, C. M. (2015). Dissolved organic carbon pools and export from the coastal ocean. Glob. Biogeochem. Cycles 29, 1725–1738. doi: 10.1002/2014GB005056
Beltran-Perez, O. D., and Waniek, J. J. (2021). Environmental window of cyanobacteria bloom occurrence. J. Mar. Syst. 224:103618. doi: 10.1016/j.jmarsys.2021.103618
Benner, R., and Amon, R. M. W. (2015). The Size-reactivity continuum of major bioelements in the ocean. Annu. Rev. Mar. Sci. 7, 185–205. doi: 10.1146/annurev-marine-010213-135126
Benner, R., Louchouarn, P., and Amon, R. M. W. (2005). Terrigenous dissolved organic matter in the Arctic Ocean and its transport to surface and deep waters of the North Atlantic. Glob. Biogeochem. Cycles 19:GB2025. doi: 10.1029/2004GB002398
Bianchi, T. S., Rolff, C., and Lambert, C. D. (1997). Sources and composition of particulate organic carbon in the Baltic Sea: the use of plant pigments and lignin-phenols as biomarkers. Mar. Ecol. Prog. Ser. 156, 25–31. doi: 10.3354/meps156025
Borges, A. V. (2005). Do we have enough pieces of the jigsaw to integrate CO2 fluxes in the coastal ocean? Estuaries 28, 3–27. doi: 10.1007/BF02732750
Bronk, D. A., See, J. H., Bradley, P., and Killberg, L. (2007). DON as a source of bioavailable nitrogen for phytoplankton. Biogeosciences 4, 283–296. doi: 10.5194/bg-4-283-2007
Burd, A. B., Frey, S., Cabre, A., Ito, T., Levine, N. M., Lønborg, C., et al. (2016). Terrestrial and marine perspectives on modeling organic matter degradation pathways. Glob. Change Biol. 22, 121–136. doi: 10.1111/gcb.12987
Burnett, W. C., Bokuniewicz, H., Huettel, M., Moore, W. S., and Taniguchi, M. (2003). Groundwater and pore water inputs to the coastal zone. Biogeochemistry 66, 3–33. doi: 10.1023/B:BIOG.0000006066.21240.53
Carlson, C. A., Ducklow, H. W., and Michaels, A. F. (1994). Annual flux of dissolved organic carbon from the euphotic zone in the northwestern Sargasso Sea. Nature 371, 405–408. doi: 10.1038/371405a0
Carlson, C. A., Giovannoni, S. J., Hansell, D. A., Goldberg, S. J., Parsons, R., Otero, M. P., et al. (2002). Effect of nutrient amendments on bacterioplankton production, community structure, and DOC utilization in the northwestern Sargasso Sea. Aquat. Microb. Ecol. 30, 19–36. doi: 10.3354/ame030019
Carslaw, D., and Ropkins, K. (2012). openair - An R package for air quality data analysis. Environ. Model. Softw. 27, 52–61. doi: 10.1016/j.envsoft.2011.09.008
Carstensen, J., Andersen, J. H., Gustafsson, B. G., and Conley, D. J. (2014). Deoxygenation of the Baltic Sea during the last century. Proc. Natl. Acad. Sci. U.S.A. 111, 5628–5633. doi: 10.1073/pnas.1323156111
Cauwet, G. (2002). “DOM in the coastal zone,” in Biogeochemistry of Marine Dissolved Organic Matter, eds D. A. Hansell and C. A. Carlson (Boston, MA: Academic Press), 579–609. doi: 10.1016/B978-012323841-2/50014-2
Christiansen, C., Edelvang, K., Emeis, K., Graf, G., Jähmlich, S., Kozuch, J., et al. (2002). Material transport from the nearshore to the basinal environment in the southern Baltic Sea: I. Processes and mass estimates. J. Mar. Syst. 35, 133–150. doi: 10.1016/S0924-7963(02)00126-4
Church, M. J., Ducklow, H. W., and Karl, D. M. (2002). Multiyear increases in dissolved organic matter inventories at Station ALOHA in the North Pacific Subtropical Gyre. Limnol. Oceanogr. 47, 1–10. doi: 10.4319/lo.2002.47.1.0001
Copin-Montégut, G., and Avril, B. (1993). Vertical distribution and temporal variation of dissolved organic carbon in the North-Western Mediterranean Sea. Deep Sea Res. I Oceanogr. Res. Pap. 40, 1963–1972. doi: 10.1016/0967-0637(93)90041-Z
De Jonge, V. N. (1997). High remaining productivity in the Dutch western Wadden Sea despite decreasing nutrient inputs from riverine sources. Mar. Pollut. Bull. 34, 427–436. doi: 10.1016/S0025-326X(96)00143-9
de Vries, I., Duin, R. N. M., Peeters, J. C. H., Los, F. J., Bokhorst, M., and Laane, R. W. P. M. (1998). Patterns and trends in nutrients and phytoplankton in Dutch coastal waters: comparison of time-series analysis, ecological model simulation, and mesocosm experiments. ICES J. Mar. Sci. 55, 620–634. doi: 10.1006/jmsc.1998.0399
Deininger, A., Kaste, Ø., Frigstad, H., and Austnes, K. (2020). Organic nitrogen steadily increasing in Norwegian rivers draining to the Skagerrak coast. Sci. Rep. 10:18451. doi: 10.1038/s41598-020-75532-5
del Giorgio, P. A., and Duarte, C. M. (2002). Respiration in the open ocean. Nature 420, 379–384. doi: 10.1038/nature01165
Deutsch, B., Alling, V., Humborg, C., Korth, F., and Mörth, C. M. (2012). Tracing inputs of terrestrial high molecular weight dissolved organic matter within the Baltic Sea ecosystem. Biogeosciences 9, 4465–4475. doi: 10.5194/bg-9-4465-2012
Dittmar, T. (2014). “Reasons behind long-term stability of dissolved organic matter,” in The Biogeochemistry of Marine Dissolved Organic Matter, 2nd Edn, eds D. A. Hansell and C. A. Carlson (Cambridge, MA: Academic Press). doi: 10.1016/B978-0-12-405940-5.00007-8
Döös, K., Meier, H. E. M., and Döscher, R. (2004). The baltic haline conveyor belt or the overturning circulation and mixing in the Baltic. Ambio 33, 261–266. doi: 10.1579/0044-7447-33.4.261
Donis, D., Janssen, F., Liu, B., Wenzhöfer, F., Dellwig, O., Escher, P., et al. (2017). Biogeochemical impact of submarine ground water discharge on coastal surface sands of the southern Baltic Sea. Estuar. Coast. Shelf Sci. 189, 131–142. doi: 10.1016/j.ecss.2017.03.003
Duarte, C. M., and Cebrián, J. (1996). The fate of marine autotrophic production. Limnol. Oceanogr. 41, 1758–1766. doi: 10.4319/lo.1996.41.8.1758
Dupont, N., and Aksnes, D. L. (2013). Centennial changes in water clarity of the Baltic Sea and the North Sea. Estuar. Coast. Shelf Sci. 131, 282–289. doi: 10.1016/j.ecss.2013.08.010
Eisma, D. (1986). Flocculation and de-flocculation of suspended matter in estuaries. Neth. J. Sea Res. 20, 183–199. doi: 10.1016/0077-7579(86)90041-4
Emil, V., Conley, D. J., Gustafsson, B. G., Kuosa, H., Pitkänen, H., Oleg, P. S., et al. (2007). Internal ecosystem feedbacks enhance nitrogen-fixing cyanobacteria blooms and complicate management in the Baltic Sea. Ambio 36, 186–194. doi: 10.1579/0044-7447(2007)36[186:IEFENC]2.0.CO;2
Engel, A., Bracher, A., Dinter, T., Endres, S., Grosse, J., Metfies, K., et al. (2019). Inter-Annual variability of organic carbon concentration in the eastern fram strait during summer (2009–2017). Front. Mar. Sci. 6:187. doi: 10.3389/fmars.2019.00187
Fleming, V., Laamanen, M., Kuosa, H., Haahti, H., and Olsonen, R. (2008). Long-term Development of Inorganic Nutrients and Chlorophyll α in the Open Northern Baltic Sea. Ambio 37, 86–92. doi: 10.1579/0044-7447(2008)37[86:LDOINA]2.0.CO;2
Fleming-Lehtinen, V., and Laamanen, M. (2012). Long-term changes in Secchi depth and the role of phytoplankton in explaining light attenuation in the Baltic Sea. Estuar. Coast. Shelf Sci. 10, 1–10. doi: 10.1016/j.ecss.2012.02.015
Garcia, N. S., Sexton, J., Riggins, T., Brown, J., Lomas, M. W., and Martiny, A. C. (2018). High variability in cellular stoichiometry of carbon, nitrogen, and phosphorus within classes of marine eukaryotic phytoplankton under sufficient nutrient conditions. Front. Microbiol. 9:543. doi: 10.3389/fmicb.2018.00543
Gattuso, J.-P., Frankignoulle, M., and Wollast, R. (1998). Carbon and carbonate metabolism in coastal aquatic ecosystems. Annu. Rev. Ecol. Syst. 29, 405–434. doi: 10.1146/annurev.ecolsys.29.1.405
Graff, J. R., Westberry, T. K., Milligan, A. J., Brown, M. B., Dall’olmo, G., Dongen-Vogels, V. V., et al. (2015). Analytical phytoplankton carbon measurements spanning diverse ecosystems. Deep Sea Res. I Oceanogr. Res. Pap. 102, 16–25. doi: 10.1016/j.dsr.2015.04.006
Gräwe, U., Naumann, M., Mohrholz, V., and Burchard, H. (2015). Anatomizing one of the largest saltwater inflows into the Baltic Sea in December 2014. J. Geophys. Res. Oceans 120, 7676–7697. doi: 10.1002/2015JC011269
Gustafsson, B. G., Schenk, F., Blenckner, T., Eilola, K., Meier, H. E. M., Müller-Karulis, B., et al. (2012). Reconstructing the Development of Baltic Sea Eutrophication 1850–2006. Ambio 41, 534–548. doi: 10.1007/s13280-012-0318-x
Gustafsson, Ö., Widerlund, A., Andersson, P. S., Ingri, J., Roos, P., and Ledin, A. (2000). Colloid dynamics and transport of major elements through a boreal river — brackish bay mixing zone. Mar. Chem. 71, 1–21. doi: 10.1016/S0304-4203(00)00035-9
Hammer, K., Schneider, B., Kuliński, K., and Schulz-Bull, D. E. (2017). Acid-base properties of Baltic Sea dissolved organic matter. J. Mar. Syst. 173, 114–121. doi: 10.1016/j.jmarsys.2017.04.007
Hansell, D. A. (2013). Recalcitrant dissolved organic carbon fractions. Annu. Rev. Mar. Sci. 5, 421–445. doi: 10.1146/annurev-marine-120710-100757
Hansell, D. A., Carlson, C. A., Repeta, D. J., and Schlitzer, R. (2009). Dissolved organic matter in the ocean - a controversy stimulates new insights. Oceanography 22, 202–211. doi: 10.5670/oceanog.2009.109
Hansen, H. P., and Koroleff, F. (1999). “Determination of nutrients,” in Methods of Seawater Analysis, eds K. Grasshoff, K. Kremling, and M. Ehrhardt (Hoboken, NJ: Wiley), 159–228. doi: 10.1002/9783527613984.ch10
Hebel, D. V., and Karl, D. M. (2001). Seasonal, interannual and decadal variations in particulate matter concentrations and composition in the subtropical North Pacific Ocean. Deep Sea Res. II Top. Stud. Oceanogr. 48, 1669–1695. doi: 10.1016/S0967-0645(00)00155-7
Hedges, J. I., and Keil, R. G. (1995). Sedimentary organic matter preservation: an assessment and speculative synthesis. Mar. Chem. 49, 81–115. doi: 10.1016/0304-4203(95)00008-F
HELCOM (2018). “State of the Baltic Sea – Second HELCOM holistic assessment 2011-2016,” in Proceedings of the Baltic Sea Environment 2018, Helsinki.
Herndl, G. J., and Reinthaler, T. (2013). Microbial control of the dark end of the biological pump. Nat. Geosci. 6, 718–724. doi: 10.1038/ngeo1921
Hernes, P. J., and Benner, R. (2003). Photochemical and microbial degradation of dissolved lignin phenols: Implications for the fate of terrigenous dissolved organic matter in marine environments. J. Geophys. Res. Oceans 108:3291. doi: 10.1029/2002JC001421
Hirsch, R. M., Slack, J. R., and Smith, R. A. (1982). Techniques of trend analysis for monthly water quality data. Water Resour. Res. 18, 107–121. doi: 10.1029/WR018i001p00107
Hoikkala, L., Kortelainen, P., Soinne, H., and Kuosa, H. (2015). Dissolved organic matter in the Baltic Sea. J. Mar. Syst. 142, 47–61. doi: 10.1016/j.jmarsys.2014.10.005
Hoikkala, L., Lahtinen, T., Perttilä, M., and Lignell, R. (2012). Seasonal dynamics of dissolved organic matter on a coastal salinity gradient in the northern Baltic Sea. Cont. Shelf Res. 45, 1–14. doi: 10.1016/j.csr.2012.04.008
Hopkinson, C. S. (1985). Shallow-water benthic and pelagic metabolism. Mar. Biol. 87, 19–32. doi: 10.1007/BF00397002
Hopkinson, C. S., and Vallino, J. J. (2005). Efficient export of carbon to the deep ocean through dissolved organic matter. Nature 433, 142–145. doi: 10.1038/nature03191
Hordoir, R., and Meier, H. E. M. (2010). Freshwater fluxes in the Baltic Sea: a model study. J. Geophys. Res. Oceans 115:C08028. doi: 10.1029/2009JC005604
Huang, C., Jiang, Q., Yao, L., Yang, H., Lin, C., Huang, T., et al. (2018). Variation pattern of particulate organic carbon and nitrogen in oceans and inland waters. Biogeosciences 15, 1827–1841. doi: 10.5194/bg-15-1827-2018
Hullar, M., Fry, B., Peterson, B. J., and Wright, R. T. (1996). Microbial utilization of estuarine dissolved organic carbon: a stable isotope tracer approach tested by mass balance. Appl. Environ. Microbiol. 62, 2489–2493. doi: 10.1128/aem.62.7.2489-2493.1996
Jurasinski, G., Janssen, M., Voss, M., Böttcher, M. E., Brede, M., Burchard, H., et al. (2018). Understanding the Coastal Ecocline: assessing sea–land interactions at non-tidal, low-lying coasts through interdisciplinary research. Front. Mar. Sci. 5:342. doi: 10.3389/fmars.2018.00342
Kahru, M., Elmgren, R., Di Lorenzo, E., and Savchuk, O. (2018). Unexplained interannual oscillations of cyanobacterial blooms in the Baltic Sea. Sci. Rep. 8:6365. doi: 10.1038/s41598-018-24829-7
Kahru, M., Elmgren, R., and Savchuk, O. P. (2016). Changing seasonality of the Baltic Sea. Biogeosciences 13, 1009–1018. doi: 10.5194/bg-13-1009-2016
Kalnay, E., Kanamitsu, M., Kistler, R., Collins, W., Deaven, D., Gandin, L., et al. (1996). The NCEP/NCAR 40-Year Reanalysis Project. Bull. Am. Meteorol. Soc. 77, 437–472. doi: 10.1175/1520-0477(1996)077<0437:TNYRP>2.0.CO;2
Karl, D. M. (1999). A sea of change: biogeochemical variability in the north pacific subtropical gyre. Ecosystems 2, 181–214. doi: 10.1007/s100219900068
Karl, D. M., Hebel, D. V., Björkman, K., and Letelier, R. M. (1998). The role of dissolved organic matter release in the productivity of the oligotrophic North Pacific Ocean. Limnol. Oceanogr. 43, 1270–1286. doi: 10.4319/lo.1998.43.6.1270
Kerr, S. C., Shafer, M. M., Overdier, J., and Armstrong, D. E. (2008). Hydrologic and biogeochemical controls on trace element export from Northern Wisconsin Wetlands. Biogeochemistry 89, 273–294. doi: 10.1007/s10533-008-9219-2
Kharbush, J. J., Close, H. G., Van Mooy, B. A. S., Arnosti, C., Smittenberg, R. H., Le Moigne, F. A. C., et al. (2020). Particulate organic carbon deconstructed: molecular and chemical composition of particulate organic carbon in the ocean. Front. Mar. Sci. 7:518. doi: 10.3389/fmars.2020.00518
Knudsen-Leerbeck, H., Mantikci, M., Bentzon-Tilia, M., Traving, S. J., Riemann, L., Hansen, J. L. S., et al. (2017). Seasonal dynamics and bioavailability of dissolved organic matter in two contrasting temperate estuaries. Biogeochemistry 134, 217–236. doi: 10.1007/s10533-017-0357-2
Koch, S., Kahle, P., and Lennartz, B. (2018). Spatio-temporal analysis of phosphorus concentrations in a North-Eastern German lowland watershed. J. Hydrol. Reg. Stud. 15, 203–216. doi: 10.1016/j.ejrh.2018.02.001
Krop, H. B., Van Noort, P. C. M., and Govers, H. A. J. (2001). “Determination and theoretical aspects of the equilibrium between dissolved organic matter and hydrophobic organic micropollutants in Water (Kdoc),” in Reviews of Environmental Contamination and Toxicology: Continuation of Residue Reviews, ed. G. W. Ware (New York, NY: Springer), 1–122. doi: 10.1007/978-1-4613-0107-3_1
Kuliński, K., Hammer, K., Schneider, B., and Schulz-Bull, D. (2016). Remineralization of terrestrial dissolved organic carbon in the Baltic Sea. Mar. Chem. 181, 10–17. doi: 10.1016/j.marchem.2016.03.002
Kuliński, K., and Pempkowiak, J. (2008). Dissolved organic carbon in the southern Baltic Sea: Quantification of factors affecting its distribution. Estuar. Coast. Shelf Sci. 78, 38–44. doi: 10.1016/j.ecss.2007.11.017
Kulinski, K., and Pempkowiak, J. (2012). Carbon Cycling in the Baltic Sea. Berlin : Springer-Verlag. doi: 10.1007/978-3-642-19388-0
Kuss, J., Nausch, G., Engelke, C., Weber, M. V., Lutterbeck, H., Naumann, M., et al. (2020). Changes of nutrient concentrations in the Western Baltic Sea in the transition between inner coastal waters and the central basins: time series from 1995 to 2016 With Source Analysis. Front. Earth Sci. 8:106. doi: 10.3389/feart.2020.00106
Labuz, T. (2015). “Environmental impacts—coastal erosion and coastline changes,” in Second Assessment of Climate Change for the Baltic Sea Basin, Regional Climate Studies, ed. The BACCII Author Team (Cham: Springer), 381–396. doi: 10.1007/978-3-319-16006-1_20
Landa, M., Cottrell, M. T., Kirchman, D. L., Kaiser, K., Medeiros, P. M., Tremblay, L., et al. (2014). Phylogenetic and structural response of heterotrophic bacteria to dissolved organic matter of different chemical composition in a continuous culture study. Environ. Microbiol. 16, 1668–1681. doi: 10.1111/1462-2920.12242
Lass, H. U., and Mohrholz, V. (2003). On dynamics and mixing of inflowing saltwater in the Arkona Sea. J. Geophys. Res. Oceans 108:3042. doi: 10.1029/2002JC001465
Leipe, T., Tauber, F., Vallius, H., Virtasalo, J., Uścinowicz, S., Kowalski, N., et al. (2011). Particulate organic carbon (POC) in surface sediments of the Baltic Sea. Geo Mar. Lett. 31, 175–188. doi: 10.1007/s00367-010-0223-x
Lennartz, S. T., Lehmann, A., Herrford, J., Malien, F., Hansen, H. P., Biester, H., et al. (2014). Long-term trends at the Boknis Eck time series station (Baltic Sea), 1957–2013: Does climate change counteract the decline in eutrophication? Biogeosciences 11, 6323–6339. doi: 10.5194/bg-11-6323-2014
Lepistö, A., Raike, A., Sallantaus, T., and Finer, L. (2021). Increases in organic carbon and nitrogen concentrations in boreal forested catchments - Changes driven by climate and deposition. Sci. Total Environ. 780:146627. doi: 10.1016/j.scitotenv.2021.146627
Liao, E., Lu, W., Yan, X.-H., Jiang, Y., and Kidwell, A. (2015). The coastal ocean response to the global warming acceleration and hiatus. Sci. Rep. 5:16630. doi: 10.1038/srep16630
Liu, S., Baetge, N., Comstock, J., Opalk, K., Parsons, R., Halewood, E., et al. (2020). Stable isotope probing identifies bacterioplankton lineages capable of utilizing dissolved organic matter across a range of bioavailability. Front. Microbiol. 11:580397. doi: 10.3389/fmicb.2020.580397
Lønborg, C., Carreira, C., Jickells, T., and Álvarez-Salgado, X. A. (2020). Impacts of global change on ocean dissolved organic carbon (DOC) cycling. Front. Mar. Sci. 7:466. doi: 10.3389/fmars.2020.00466
Lønborg, C., and Markager, S. (2021). Nitrogen in the Baltic Sea: long-term trends, a budget and decadal time lags in responses to declining inputs. Estuar. Coast. Shelf Sci. 261:107529. doi: 10.1016/j.ecss.2021.107529
Lutz, M. J., Caldeira, K., Dunbar, R. B., and Behrenfeld, M. J. (2007). Seasonal rhythms of net primary production and particulate organic carbon flux to depth describe the efficiency of biological pump in the global ocean. J. Geophys. Res. Oceans 112:C10011. doi: 10.1029/2006JC003706
Maciejewska, A., and Pempkowiak, J. (2014). DOC and POC in the water column of the southern Baltic. Part I. Evaluation of factors influencing sources, distribution and concentration dynamics of organic matter. Oceanologia 56, 523–548. doi: 10.5697/oc.56-3.523
Mackenzie, B. R., and Schiedek, D. (2007). Daily ocean monitoring since the 1860s shows record warming of northern European seas. Glob. Change Biol. 13, 1335–1347. doi: 10.1111/j.1365-2486.2007.01360.x
McCarthy, M., Pratum, T., Hedges, J., and Benner, R. (1997). Chemical composition of dissolved organic nitrogen in the ocean. Nature 390, 150–154. doi: 10.1038/36535
McGovern, M., Evenset, A., Borgå, K., De Wit, H. A., Braaten, H. F. V., Hessen, D. O., et al. (2019). Implications of Coastal Darkening for Contaminant Transport, Bioavailability, and Trophic Transfer in Northern Coastal Waters. Environ. Sci. Technol. 53, 7180–7182. doi: 10.1021/acs.est.9b03093
McKee, B. A., Aller, R. C., Allison, M. A., Bianchi, T. S., and Kineke, G. C. (2004). Transport and transformation of dissolved and particulate materials on continental margins influenced by major rivers: benthic boundary layer and seabed processes. Cont. Shelf Res. 24, 899–926. doi: 10.1016/j.csr.2004.02.009
McQuatters-Gollop, A., Raitsos, D. E., Edwards, M., Pradhan, Y., Mee, L., Lavender, S., et al. (2007). A lon‡ term chlorophyll dataset reveals regime shift in North Sea phytoplankton biomass unconnected to nutrient levels. Limnol. Oceanogr. 52, 635–648. doi: 10.4319/lo.2007.52.2.0635
Mehl, D., Miegel, K., and Schumann, A. (2014). Unusual rainfall events in Mecklenburg-Vorpommern during the summer of 2011 and their hydrological consequences - Part 2: Sydrological consequences. Hydrol. Wasserbewirtschaftung 58, 29–42.
Meier, H. E. M., and Kauker, F. (2003). Modeling decadal variability of the Baltic Sea: 2. Role of freshwater inflow and large-scale atmospheric circulation for salinity. J. Geophys. Res. Oceans 108:3368. doi: 10.1029/2003JC001799
Meier, H. E. M., Feistel, R., Jan, P., Arneborg, L., Burchard, H., Volker, F., et al. (2006b). Ventilation of the Baltic Sea deep water: a brief review of present knowledge from observations and models. Oceanologia 48, 133–164.
Meier, H. E. M., Kjellström, E., and Graham, L. P. (2006a). Estimating uncertainties of projected Baltic Sea salinity in the late 21st century. Geophys. Res. Lett. 33:L15705. doi: 10.1029/2006GL026488
Meier, H. E. M., Kniebusch, M., Dieterich, C., Gröger, M., Zorita, E., Elmgren, R., et al. (2021). Climate change in the baltic sea region: a summary. Earth Syst. Dyn. Discuss. 2021, 1–205. doi: 10.5194/esd-2021-67
Menden-Deuer, S., and Lessard, E. J. (2000). Carbon to volume relationships for dinoflagellates, diatoms, and other protist plankton. Limnol. Oceanogr. 45, 569–579. doi: 10.4319/lo.2000.45.3.0569
Middelburg, J. J. (2019). Marine Carbon Biogeochemistry - A Primer for Earth System Scientists. Cham: Springer. doi: 10.1007/978-3-030-10822-9
Mohrholz, V. (2018). Major Baltic Inflow Statistics – Revised. Front. Mar. Sci. 5:384. doi: 10.3389/fmars.2018.00384
Mohrholz, V., Naumann, M., Nausch, G., Krüger, S., and Gräwe, U. (2015). Fresh oxygen for the Baltic Sea – An exceptional saline inflow after a decade of stagnation. J. Mar. Syst. 53. 148, 152–166. doi: 10.1016/j.jmarsys.2015.03.005
Nausch, G., Nehring, D., and Nagel, K. (2008). “Nutrient concentrations, trends and their relation to eutrophication,” in State and Evolution of the Baltic Sea, 1952–2005, eds R. Feistel, G. Nausch, and N. Wasmund (Hoboken, NJ: John Wiley & Sons, Inc), 337–366. doi: 10.1002/9780470283134.ch12
Niemistö, J., and Lund-Hansen, L. C. (2019). Instantaneous Effects of Sediment Resuspension on Inorganic and Organic Benthic Nutrient Fluxes at a Shallow Water Coastal Site in the Gulf of Finland, Baltic Sea. Estuaries Coasts 42, 2054–2071. doi: 10.1007/s12237-019-00648-5
O’Connor, M. I., Piehler, M. F., Leech, D. M., Anton, A., and Bruno, J. F. (2009). Warming and resource availability shift food web structure and metabolism. PLoS Biol. 7:e1000178. doi: 10.1371/journal.pbio.1000178
Oksanen, J., Blanchet, F. G., Friendly, M., Kindt, R., Legendre, P., Mcglinn, D., et al. (2020). vegan Community Ecology Package Version 2.5-7. Available online at: https://rdrr.io/cran/vegan/ (accessed November, 2020).
Olenina, I., Hajdu, S., Edler, L., Andersson, A., Wasmund, N., Busch, S., et al. (2006). Biovolumes and size-classes of phytoplankton in the Baltic Sea. HELCOM Baltic Sea Environ. Proc. 106:144.
Omstedt, A., and Nohr, C. (2004). Calculating the water and heat balances of the Baltic Sea using ocean modelling and available meteorological, hydrological and ocean data. Tellus A 56, 400–414. doi: 10.3402/tellusa.v56i4.14428
Osburn, C. L., and Stedmon, C. A. (2011). Linking the chemical and optical properties of dissolved organic matter in the Baltic–North Sea transition zone to differentiate three allochthonous inputs. Mar. Chem. 126, 281–294. doi: 10.1016/j.marchem.2011.06.007
Osterholz, H., Singer, G., Wemheuer, B., Daniel, R., Simon, M., Niggemann, J., et al. (2016b). Deciphering associations between dissolved organic molecules and bacterial communities in a pelagic marine system. ISME J. 10, 1717–1730. doi: 10.1038/ismej.2015.231
Osterholz, H., Kirchman, D. L., Niggemann, J., and Dittmar, T. (2016a). Environmental drivers of dissolved organic matter molecular composition in the delaware estuary. Front. Earth Sci. 4:95. doi: 10.3389/feart.2016.00095
Patriarca, C., Sedano-Núñez, V. T., Garcia, S. L., Bergquist, J., Bertilsson, S., Sjöberg, P. J. R., et al. (2020). Character and environmental lability of cyanobacteria-derived dissolved organic matter. Limnol. Oceanogr. 66, 496–509. doi: 10.1002/lno.11619
Piontek, J., Endres, S., Le Moigne, F. A. C., Schartau, M., and Engel, A. (2019). Relevance of nutrient-limited phytoplankton production and its bacterial remineralization for carbon and oxygen fluxes in the Baltic Sea. Front. Mar. Sci. 6:581. doi: 10.3389/fmars.2019.00581
Pohlert, T. (2020). trend: Non-Parametric Trend Tests and Change-Point Detection. R package version 1.1.4. Available online at: https://cran.r-project.org/web/packages/trend/trend.pdf (accessed July 4, 2021).
Räike, A., Kortelainen, P., Mattsson, T., and Thomas, D. N. (2012). 36year trends in dissolved organic carbon export from Finnish rivers to the Baltic Sea. Sci. Total Environ. 435-436, 188–201. doi: 10.1016/j.scitotenv.2012.06.111
Redfield, A. C. (1934). “On the proportions of organic derivations in sea water and their relation to the composition of plankton,” in James Johnstone Memorial Volume, ed. R. J. Daniel (Liverpool: Liverpool University Press), 177–192. doi: 10.1111/gcb.13106
Reid, P. C., Hari, R. E., Beaugrand, G., Livingstone, D. M., Marty, C., Straile, D., et al. (2016). Global impacts of the 1980s regime shift. Glob. Change Biol. 22, 682–703. doi: 10.1111/gcb.13106
Reusch, T. B. H., Dierking, J., Andersson, H. C., Bonsdorff, E., Carstensen, J., Casini, M., et al. (2018). The Baltic Sea as a time machine for the future coastal ocean. Sci. Adv. 4:eaar8195. doi: 10.1126/sciadv.aar8195
Rosner, B. (1983). Percentage points for a generalized ESD many-outlier procedure. Technometrics 25, 165–172. doi: 10.1080/00401706.1983.10487848
Sandberg, J., Andersson, A., Johansson, S., and Wikner, J. (2004). Pelagic food web structure and carbon budget in the northern Baltic Sea: potential importance of terrigenous carbon. Mar. Ecol. Prog. Ser. 268, 13–29. doi: 10.3354/meps268013
Schafmeister, M.-T., and Darsow, A. (2011). “Potential change in groundwater discharge as response to varying climatic conditions – an experimental model study at catchment scale,” in The Baltic Sea Basin, eds J. Harff, S. Björck, and P. Hoth (Berlin: Springer), 391–404. doi: 10.1007/978-3-642-17220-5
Schlitzer, R. (2020). Ocean Data View. Available online at: https://odv.awi.de (accessed November 19, 2021).
Schneider, B., and Müller, J. D. (2018). “The main hydrographic characteristics of the Baltic Sea,” in Biogeochemical Transformations in the Baltic Sea: Observations through Carbon Dioxide Glasses, eds B. Schneider and J. D. Müller (Cham: Springer), 35–41. doi: 10.1007/978-3-319-61699-5
Schneider, B., Schlitzer, R., Fischer, G., and Nöthig, E.-M. (2003). Depth-dependent elemental compositions of particulate organic matter (POM) in the ocean. Glob. Biogeochem. Cycles 17:1032. doi: 10.1029/2002GB001871
Seidel, M., Manecki, M., Herlemann, D. P. R., Deutsch, B., Schulz-Bull, D., Jürgens, K., et al. (2017). Composition and transformation of dissolved organic matter in the Baltic Sea. Front. Earth Sci. 5:31. doi: 10.3389/feart.2017.00031
Seitzinger, S. P., Sanders, R. W., and Styles, R. (2002). Bioavailability of DON from natural and anthropogenic sources to estuarine plankton. Limnol. Oceanogr. 47, 353–366. doi: 10.4319/lo.2002.47.2.0353
Sen, P. K. (1968). Estimates of the regression coefficient based on Kendall’s Tau. J. Am. Stat. Assoc. 63, 1379–1389. doi: 10.1080/01621459.1968.10480934
Setälä, O., and Kivi, K. (2003). Planktonic ciliates in the Baltic Sea in summer: distribution, species association and estimated grazing impact. Aquat. Microb. Ecol. 32, 287–297. doi: 10.3354/ame032287
Sintes, E., Stoderegger, K., Parada, V., and Herndl, G. J. (2010). Seasonal dynamics of dissolved organic matter and microbial activity in the coastal North Sea. Aquat. Microb. Ecol. 60, 85–95. doi: 10.3354/ame01404
Sipler, R. E., and Bronk, D. A. (2015). “Chapter 4 - Dynamics of Dissolved Organic Nitrogen,” in Biogeochemistry of Marine Dissolved Organic Matter, 2nd Edn, eds D. A. Hansell and C. A. Carlson (Boston, MA: Academic Press), 127–232. doi: 10.1016/B978-0-12-405940-5.00004-2
Smith, W., and Barber, R. (2008). A carbon budget for the autrophic ciliate Mesodinium rubrum. J. Phycol. 15, 27–33. doi: 10.1111/j.1529-8817.1979.tb02957.x
Soetaert, K., Middelburg, J. J., Heip, C., Meire, P., Van Damme, S., and Maris, T. (2006). Long-term change in dissolved inorganic nutrients in the heterotrophic Scheldt estuary (Belgium, The Netherlands). Limnol. Oceanogr. 51, 409–423. doi: 10.4319/lo.2006.51.1_part_2.0409
Spilling, K., Olli, K., Lehtoranta, J., Kremp, A., Tedesco, L., Tamelander, T., et al. (2018). Shifting Diatom—Dinoflagellate dominance during spring bloom in the baltic sea and its potential effects on biogeochemical cycling. Front. Mar. Sci. 5:327. doi: 10.3389/fmars.2018.00327
Stålnacke, P., Vandsemb, S. M., Vassiljev, A., Grimvall, A., and Jolankai, G. (2004). Changes in nutrient levels in some Eastern European rivers in response to large-scale changes in agriculture. Water Sci. Technol. 49, 29–36.
Stedmon, C. A., Markager, S., Søndergaard, M., Vang, T., Laubel, A., Borch, N. H., et al. (2006). Dissolved organic matter (DOM) export to a temperate estuary: seasonal variations and implications of land use. Estuaries Coasts 29, 388–400. doi: 10.1007/BF02784988
Stuermer, D. H., Peters, K. E., and Kaplan, I. R. (1978). Source indicators of humic substances and proto-kerogen. Stable isotope ratios, elemental compositions and electron spin resonance spectra. Geochim. Cosmochim. Acta 42, 989–997. doi: 10.1016/0016-7037(78)90288-0
Suratman, S., Weston, K., Jickells, T., and Fernand, L. (2009). Spatial and seasonal changes of dissolved and particulate organic C in the North Sea. Hydrobiologia 628, 13–25. doi: 10.1007/s10750-009-9730-z
Szymczycha, B., Maciejewska, A., Winogradow, A., and Pempkowiak, J. (2014). Could submarine groundwater discharge be a significant carbon source to the southern Baltic Sea? Oceanologia 56, 327–347. doi: 10.5697/oc.56-2.327
Szymczycha, B., Winogradow, A., Kuliński, K., Koziorowska, K., and Pempkowiak, J. (2017). Diurnal and seasonal DOC and POC variability in the land-locked sea. Oceanologia 59, 379–388. doi: 10.1016/j.oceano.2017.03.008
Terttu, F., Kaisa, K., Riitta, O., and Kerstin, W. (2001). The History of Cyanobacterial Blooms in the Baltic Sea. Ambio 30, 172–178. doi: 10.1579/0044-7447-30.4.172
Theil, H. (1992). “A rank-invariant method of linear and polynomial regression analysis,” in Henri Theil’s Contributions to Economics and Econometrics: Econometric Theory and Methodology, eds B. Raj and J. Koerts (Dordrecht: Springer), 345–381. doi: 10.1007/978-94-011-2546-8
Thomas, H., Pempkowiak, J., Wulff, F., and Nagel, K. (2003). Autotrophy, nitrogen accumulation and nitrogen limitation in the Baltic Sea: a paradox or a buffer for eutrophication? Geophys. Res. Lett. 30:2130. doi: 10.1029/2003GL017937
Toming, K., Kotta, J., Uuemaa, E., Sobek, S., Kutser, T., and Tranvik, L. (2020). Predicting lake dissolved organic carbon at a global scale. Sci. Rep. 10:8471. doi: 10.1038/s41598-020-65010-3
Ulrich, J. (2020). TTR: Technical Trading Rules. R Package Version 0.24.2. Available online at: https://cran.r-project.org/web/packages/TTR/TTR.pdf (accessed on July 4, 2021).
Utermöhl, H. (1958). Zur Vervollkommnung der quantitativen Phytoplankton-methodik. Mittellungen Int. Vererin. Limnol. 9, 1–38. doi: 10.1080/05384680.1958.11904091
Van Engeland, T., Soetaert, K., Knuijt, A., Laane, R. W. P. M., and Middelburg, J. J. (2010). Dissolved organic nitrogen dynamics in the North Sea: a time series analysis (1995–2005). Estuar. Coast. Shelf Sci. 89, 31–42. doi: 10.1016/j.ecss.2010.05.009
Veuger, B., Middelburg, J. J., Boschker, H. T. S., Nieuwenhuize, J., Van Rijswijk, P., Rochelle-Newall, E. J., et al. (2004). Microbial uptake of dissolved organic and inorganic nitrogen in Randers Fjord. Estuar. Coast. Shelf Sci. 61, 507–515. doi: 10.1016/j.ecss.2004.06.014
von Ahn, C. M. E., Scholten, J. C., Malik, C., Feldens, P., Liu, B., Dellwig, O., et al. (2021). A Multi-Tracer Study of Fresh Water Sources for a Temperate Urbanized Coastal Bay (Southern Baltic Sea). Front. Environ. Sci. 9:642346. doi: 10.3389/fenvs.2021.642346
Voss, M., Asmala, E., Bartl, I., Carstensen, J., Conley, D. J., Dippner, J. W., et al. (2020). Origin and fate of dissolved organic matter in four shallow Baltic Sea estuaries. Biogeochemistry 154, 385–403. doi: 10.1007/s10533-020-00703-5
Voss, M., Emeis, K. C., Hille, S., Neumann, T., and Dippner, J. W. (2005). Nitrogen cycle of the Baltic Sea from an isotopic perspective. Glob. Biogeochem. Cycles 19:GB3001. doi: 10.1029/2004GB002338
Wainright, S. C. (1987). Stimulation of heterotrophic microplankton production by resuspended marine sediments. Science 238, 1710–1712. doi: 10.1126/science.238.4834.1710
Wasmund, N., Nausch, G., Gerth, M., Busch, S., Burmeister, C., Hansen, R., et al. (2019). Extension of the growing season of phytoplankton in the western Baltic Sea in response to climate change. Mar. Ecol. Prog. Ser. 622, 1–16. doi: 10.3354/meps12994
Wasmund, N., Tuimala, J., Suikkanen, S., Vandepitte, L., and Kraberg, A. (2011). Long-term trends in phytoplankton composition in the western and central Baltic Sea. J. Mar. Syst. 87, 145–159. doi: 10.1016/j.jmarsys.2011.03.010
Winogradow, A., Mackiewicz, A., and Pempkowiak, J. (2019). Seasonal changes in particulate organic matter (POM) concentrations and properties measured from deep areas of the Baltic Sea. Oceanologia 61, 505–521. doi: 10.1016/j.oceano.2019.05.004
Wollast, R. (1998). “Evaluation andcomparison of the global carbon cycle in the coastal zone and in the open ocean,” in The Global Coastal Ocean – Processes and Methods, eds K. H. Brink and A. R. Robinson (New York, NY: John Wiley & Sons), 213–252. doi: 10.1016/j.jmarsys.2011.03.010
Worrall, F., Harriman, R., Evans, C. D., Watts, C. D., Adamson, J., Neal, C., et al. (2004). Trends in Dissolved Organic Carbon in UK Rivers and Lakes. Biogeochemistry 70, 369–402. doi: 10.1007/s10533-004-8131-7
Zark, M., Christoffers, J., and Dittmar, T. (2017). Molecular properties of deep-sea dissolved organic matter are predictable by the central limit theorem: evidence from tandem FT-ICR-MS. Mar. Chem. 191, 9–15. doi: 10.1016/j.marchem.2017.02.005
Keywords: DOC, DON, POC, PON, biogeochemical cycling, coastal ocean, inorganic nutrients, Baltic Sea
Citation: Osterholz H, Burmeister C, Busch S, Dierken M, Frazão HC, Hansen R, Jeschek J, Kremp A, Kreuzer L, Sadkowiak B, Waniek JJ and Schulz-Bull DE (2021) Nearshore Dissolved and Particulate Organic Matter Dynamics in the Southwestern Baltic Sea: Environmental Drivers and Time Series Analysis (2010–2020). Front. Mar. Sci. 8:795028. doi: 10.3389/fmars.2021.795028
Received: 14 October 2021; Accepted: 25 November 2021;
Published: 24 December 2021.
Edited by:
Christian Lønborg, Aarhus University, DenmarkReviewed by:
Xiaolin Li, Xiamen University, ChinaLiyang Yang, Fuzhou University, China
Beata Szymczycha, Institute of Oceanology, Polish Academy of Sciences (PAN), Poland
Ding He, Hong Kong University of Science and Technology, Hong Kong SAR, China
Jibiao Zhang, Guangdong Ocean University, China
Copyright © 2021 Osterholz, Burmeister, Busch, Dierken, Frazão, Hansen, Jeschek, Kremp, Kreuzer, Sadkowiak, Waniek and Schulz-Bull. This is an open-access article distributed under the terms of the Creative Commons Attribution License (CC BY). The use, distribution or reproduction in other forums is permitted, provided the original author(s) and the copyright owner(s) are credited and that the original publication in this journal is cited, in accordance with accepted academic practice. No use, distribution or reproduction is permitted which does not comply with these terms.
*Correspondence: Helena Osterholz, aGVsZW5hLm9zdGVyaG9sekBpby13YXJuZW11ZW5kZS5kZQ==