- 1Institute of Zoology, Zoological Society of London, London, United Kingdom
- 2Department of Genetics, Evolution and Environment, University College London, London, United Kingdom
- 3Key Laboratory of Aquatic Biodiversity and Conservation, Institute of Hydrobiology, Chinese Academy of Sciences, Wuhan, China
- 4Biosciences, College of Life and Environmental Sciences, University of Exeter, Exeter, United Kingdom
Conservation management requires evidence, but robust data on key parameters such as threats are often unavailable. Conservation-relevant insights might be available within datasets collected for other reasons, making it important to determine the information content of available data for threatened species and identify remaining data-gaps before investing time and resources in novel data collection. The Yangtze finless porpoise (Neophocaena asiaeorientalis asiaeorientalis) has declined severely across the middle-lower Yangtze, but multiple threats exist in this system and the relative impact of different anthropogenic activities is unclear, preventing identification of appropriate mitigation strategies. Several datasets containing information on porpoises or potential threats are available from past boat-based and fishing community surveys, which might provide novel insights into causes of porpoise mortality and decline. We employed multiple analytical approaches to investigate spatial relationships between live and dead porpoises and different threats, reproductive trends over time, and sustainable offtake levels, to assess whether evidence-based conservation is feasible under current data availability. Our combined analyses provide new evidence that mortality is spatially associated with increased cargo traffic; observed mortality levels (probably a substantial underestimate of true levels) are unsustainable; and population recruitment is decreasing, although multiple factors could be responsible (pollutants, declining fish stocks, anthropogenic noise, reduced genetic diversity). Available data show little correlation between patterns of mortality and fishing activity even when analyzed across multiple spatial scales; however, interview data can be affected by multiple biases that potentially complicate attempts to reconstruct levels of bycatch, and new data are required to understand dynamics and sustainability of porpoise-fisheries interactions. This critical assessment of existing data thus suggests that in situ porpoise conservation management must target multiple co-occurring threats. Even limited available datasets can provide new insights for understanding declines, and we demonstrate the importance of an integrative approach for investigating complex conservation problems and maximizing evidence in conservation planning for poorly known taxa.
Introduction
Conservation practitioners recognize the need for evidence-based conservation, where robust data are used to understand the dynamics of decline and guide best-practice management (Sutherland et al., 2004; Bower et al., 2018). However, data for many threatened species are limited in quantity and/or quality, hindering informed decision-making (Catullo et al., 2008; McDonald-Madden et al., 2008; Lindenmayer et al., 2013). In such cases, precautionary conservation is often applied (Carr and Raimondi, 1999; Pan and Huntington, 2015; Sampaio et al., 2015). This approach deals with uncertainty using defensive intervention, and typically advocates action even in data-poor contexts (Cooney, 2004). However, precautionary conservation can increase the risk of suboptimal outcomes and inefficient use of time and resources, as interventions are more poorly informed and might not target key problems effectively (VanderWerf et al., 2006). Further research can overcome data limitation, but requires investment in resources that could be allocated to more practical activities, can yield diminishing investment returns or risk replicating collection of existing data (Grantham et al., 2008), and can generate delays that reduce the guarantee of improved conservation prospects (Jaramillo-Legorreta et al., 2007). Alternatively, conservation-relevant insights might be provided through analysis of existing datasets originally collected for other reasons (McDonald-Madden et al., 2008; Zhang and Vincent, 2017). It is thus important to determine the information content of available data on threatened species before investing in novel data collection. This may involve multiple analyses of limited data to extract maximally useful conservation baselines and identify remaining data-gaps (MacMillan and Marshall, 2006; Thieme et al., 2007; Rodrigues, 2011).
The Yangtze finless porpoise (Neophocaena asiaeorientalis asiaeorientalis) is a freshwater cetacean endemic to the middle-lower Yangtze drainage in eastern China, occurring in the 1,700 km river mainstem between Yichang-Shanghai and in the appended Dongting and Poyang lakes. It is usually considered a freshwater subspecies of narrow-ridged finless porpoise, but has recently been proposed as a recently diverged distinct species on the basis of its genetic adaptation to freshwater conditions and complete genetic isolation from marine finless porpoise populations (Zhou et al., 2018). The Yangtze River experienced the extinction of the Yangtze River dolphin or baiji (Lipotes vexillifer) in the early 2000s (Turvey et al., 2007), and the porpoise population has also declined severely, falling in the mainstem from ∼2,700 in the 1990s (Zhang et al., 1993) to ∼500 in 2012 (Mei et al., 2014). It is now one of the few cetaceans listed as Critically Endangered by IUCN (2021). The middle-lower Yangtze drainage is heavily industrialized, supports extremely large human populations, and is impacted by numerous anthropogenic pressures including intensive legal and illegal fishing activities (free-floating nets, hook-based gears such as rolling-hook long-lines, fixed nets, and electrofishing), high vessel traffic, pollution, resource depletion, and habitat loss/degradation. These activities are all proposed as potential drivers of porpoise decline through elevated direct mortality, reduced survivorship, or reduced carrying capacity (Zhao et al., 2008; Wang, 2009; Mei et al., 2014; Huang et al., 2017).
The relative impact of different potential porpoise threats has been the subject of limited research. Previous studies consist largely of investigating local-scale porpoise distributions in relation to available resources (Kimura et al., 2012; Wang et al., 2014), and opportunistically-observed porpoise deaths associated with specific threats including fisheries bycatch, propeller collisions, and pollutants (Zhou and Wang, 1994; Wang et al., 2000, 2015; Dong et al., 2006; Yang et al., 2008; Turvey et al., 2013; Xiong et al., 2019). Two population viability analyses have been conducted to model future decline (Zhang and Wang, 1999; Huang et al., 2017); however, neither attempted to quantify sustainable levels of offtake (the number of individuals removed from the environment through hunting, harvesting, or other killing by humans; cf. Ingram et al., 2015), or incorporated mortality data associated with different threats. The only population-level study into drivers of decline used a mortality dataset derived from interviews with artisanal fishers (Turvey et al., 2013). This study suggested that propeller-related mortality has increased over time and might be driving decline, whereas bycatch mortality has decreased over time and might therefore merely be tracking decline. However, the relative significance of only these two threats could be assessed, and most deaths could not be attributed to known causes.
Rapid, effective action is needed to prevent Yangtze finless porpoise extinction. However, mitigation of different threats requires different approaches, but in the absence of robust data on primary driver(s) of decline, it is difficult to identify priority management targets. Indeed, in ecosystems where cetaceans face multiple threats, each threat might have reduced individual risk, resulting in reduced power to identify drivers of decline (Thompson et al., 2000). A precautionary approach has been generally recommended for cetacean conservation, especially for threatened species lacking robust data on causes of decline (Thompson et al., 2000), and precautionary measures have been initiated for Yangtze finless porpoise conservation. Porpoises are awarded national-level protection under China’s 1989 Wildlife Protection Act, several in situ and ex situ reserves have been established (with regulation of boat traffic within some reserves), and seasonal fishing bans have been implemented across the middle-lower Yangtze since 2003 to mitigate against bycatch and fish stock depletion (Wang, 2009), based on the assumption that fisheries impacts are an important driver of decline (Zhou and Wang, 1994; Wang et al., 1998; Zhao et al., 2008). However, existing in situ management potentially risks further declines if other factors are primarily responsible for regulating porpoise population dynamics (cf. Rojas-Bracho et al., 2019).
Several datasets containing information on porpoises and/or potential threats are available for the Yangtze system, including from boat-based surveys in 2006 and 2012 that followed identical survey protocols across the same area and same time of year (November–December) (Turvey et al., 2007; Zhao et al., 2008; Mei et al., 2014), and interview surveys in artisanal fishing communities in 2008 and 2011–2012 (Turvey et al., 2013, 2015a). These datasets date from a period when the porpoise population was experiencing a rapid decline (Mei et al., 2014), and so might be able to provide important insights into the drivers of this decline. We investigated the information content of this existing evidence-base using multiple analytical approaches, to determine whether available datasets can strengthen our baseline for understanding primary causes of porpoise mortality, investigate relative impacts of different possible threats, identify remaining data-gaps, and assess whether evidence-based porpoise conservation is feasible under current data availability or whether a more precautionary approach is justified. Our study reveals novel insights into the dynamics and probable drivers of porpoise population depletion, and illustrates an approach to maximize evidence in conservation planning and prioritization for highly threatened taxa.
Materials and Methods
Spatial Mortality and Threat Patterns
100 km-Section Analysis
Spatial data on counts of live porpoise sightings, cargo vessels and fishing vessels were collected along the Yangtze mainstem (Yichang-Shanghai) during the 2006 boat-based survey (Turvey et al., 2007; Zhao et al., 2008; Figure 1). Spatial data on reported porpoise deaths and locally used fishing gear types were collected across the same area during the 2008 interview survey of 599 respondents in 27 fishing communities (see Turvey et al., 2013 for survey methods and questionnaire design). Associated information reported by respondents on dates and locations of specific dead porpoise observations indicates that these all represented independent mortality events, rather than multiple reports of the same animals (Turvey et al., 2013). To investigate relationships between reported mortality levels and spatial distributions of different threats on a Yangtze-wide scale, data from both surveys were grouped into 17,100 km river sections, to control for spatially uneven distribution of fishing communities and match study design in Turvey et al. (2013) (Supplementary Table 1). The furthest downstream section was excluded from analysis because respondents here fished partly at sea, so reports likely also refer to marine narrow-ridged finless porpoises (Neophocaena asiaeorientalis sunameri) and marine-type gears.
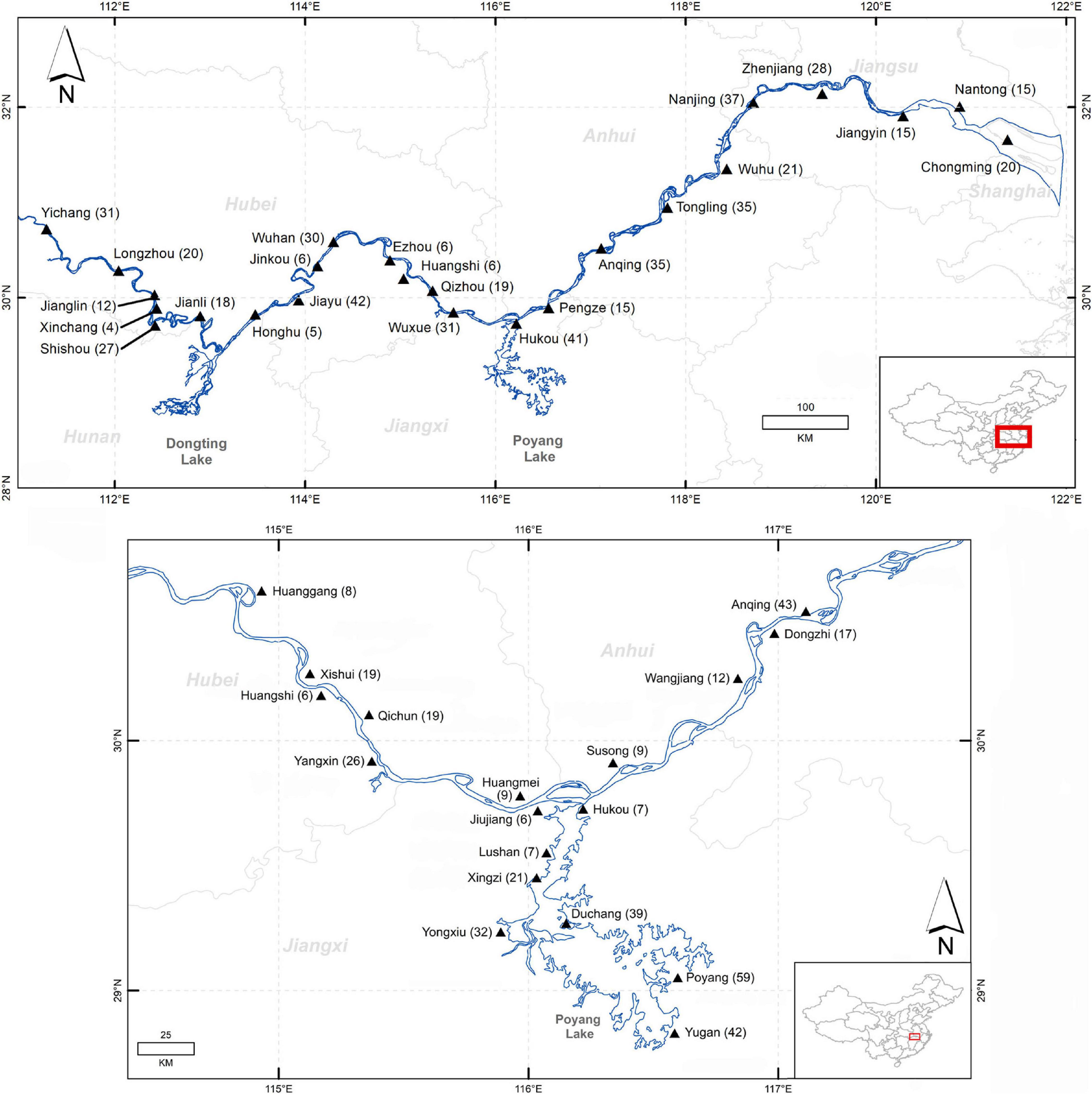
Figure 1. (Top) Middle-lower Yangtze drainage (Yichang-Shanghai), showing 2008 fisher survey localities and number of interviews. (Bottom) 2011–2012 fisher survey localities and number of interviews.
The relationship between mortality levels and threats in the remaining 16,100 km sections was investigated using a binomial generalized linear model (GLM) framework in R v3.4.3 (R Development Core Team, 2017). Proportion of respondents who had seen a dead porpoise during the previous 12 months was used as the response variable; data on numbers of dead porpoise sightings per respondent were too imprecise to analyze, as most responses were qualitative (e.g., “a few”) rather than quantitative. Predictor variables included counts of cargo vessels, fishing vessels and live porpoises, and proportions of respondents who reported using different functional fishing gear types (free-floating nets, fixed nets, hook-type gears), and who thought electrofishing was locally a problem. Vessel counts had much larger values and ranges than other variables, so were individually rescaled to a mean of 0 and standard deviation of 1. An indirect electrofishing metric was necessary because this activity is illegal and heavily penalized, so direct reporting data are prone to bias by omission.
Data were over-dispersed and so were fitted in a beta-binomial framework, which takes sample size information into account rather than simply using raw proportion data (Harrison, 2015). Models were ranked by Akaike Information Criterion corrected for small sample size (AICc). We considered all models within Δ6 units of the top model as competitive, but also applied the nesting rule (Richards, 2008) to remove models that were more complex versions of those with better AICc support and so likely to contain uninformative parameters. We applied model averaging to this best-model set. As this dataset is relatively small (n = 16 replicates), data were also fitted into a logit Gaussian framework to check robustness and directionality of significant predictors from the beta-binomial model. Influence of spatial autocorrelation on final models was discounted (Supplementary Material).
County-Level Analysis
Additional data on reported porpoise deaths and fishing activities were collected from all mainstem riverside counties between Huangshi-Dongzhi (∼270 km, 13 counties, 205 respondents) and all Poyang Lake counties within the distribution of the lake’s porpoise population (6 counties, 201 respondents) during the 2011–2012 interview survey (Figure 1; see Turvey et al., 2015a for survey methods and questionnaire design), allowing investigation into predictors of mortality at finer-scale resolution (Supplementary Table 2).
County-level mortality and fishing activity patterns were again investigated within a binomial GLM framework with models ranked by AICc, using proportion of respondents who had seen a dead porpoise during the previous 12 months as the response variable, and the same four functional gear categories analyzed above as predictors. The indirect electrofishing metric used here was mean percentage/county based on the question “What percentage of this village practices electrofishing?” with random values calculated from reported ranges (e.g., “10–30%”), and excluding vague responses. County-level count data of cargo and fishing vessels and live porpoises were unavailable for Poyang Lake, so could not be included as predictors. The model was over-dispersed within a binomial framework and was not successfully corrected with a quasibinomial model, so a logit Gaussian model that successfully fitted the data was also used, with logit transformation of response variable. Spatial autocorrelation was not detected in any model parameters (Supplementary Material).
Across-River Overlap of Porpoises and Fishing Gear
Along-river analyses were complemented by investigation of spatial correlation of porpoises and threats over an across-river transect. Porpoise sightings from both boat-based surveys between Ezhou-Zhenjiang, the ∼650 km mainstem section containing ∼80% of all sightings (Zhao et al., 2008; Mei et al., 2014; Figure 1), have been categorized by distance from bank into four distance bins (0–100, 101–300, 301–500 m, >500 m) (unpublished data). Fishers interviewed in 2011–2012 between Huangshi-Dongzhi (all located between Ezhou-Zhenjiang) were asked the distance from bank they used different gears; these data were categorized into the same distance bins used for porpoise data. Individual gear-use responses were assigned to all bins within a reported range (e.g., “100–150 m” was assigned to 0–100 and 101–300 m bins). Only free-floating nets and hook-type gears were analyzed, as reported use of other gears was very low. Chi-squared goodness-of-fit tests and Spearman rank correlation were used to investigate whether porpoise observations and gears showed similar distributions.
Reproductive Patterns Over Time
Boat-based surveys recorded whether observations were adults or calves (2006, 348 adults, 90 calves; 2012, 318 adults, 23 calves; unpublished data). To investigate whether reproduction has been uniform over time, we used a binary GLM to quantify differences in the proportion of calves in each survey year, where individuals were coded as 1 if calf and 0 if adult. We fitted year as the sole predictor in a GLM with a binomial error structure, and assessed significance by likelihood ratio test against a model containing only the intercept.
Estimating Sustainable Offtake
Levels of offtake that will maintain or achieve a sustainable Yangtze finless porpoise population were estimated using a Potential Biological Removal (PBR) model and a logistic population growth model, two population models commonly used to predict cetacean population change (Wade, 1998; Monnahan et al., 2015). Theoretical removal limits were then compared with numbers of dead porpoises reported during the 2008 interview survey, to assess whether direct mortality and known causes of mortality could have driven population decline.
Potential Biological Removal Model
PBR values were calculated using the formula in Wade (1998):
where Nmin = 20th percentile of estimated population size, Rmax = maximum annual population growth rate, and Fr = recovery factor.
Nmin is calculated by:
where Oabs = survey estimate of absolute abundance, Z = standard normal deviate, and CVabs = population estimate’s coefficient of variation.
Values were calculated across several populations and parameters: (1) using 2006 and 2012 population estimates, with a coefficient of variation (CVabs) of 0.133 for 2006 and 0.159 for 2012 (Zhao et al., 2008; Mei et al., 2014); (2) using maximum annual population growth rates (Rmax) of 0.04 for Indo-Pacific finless porpoise (Neophocaena phocaenoides; Taylor et al., 2007), and 0.035 for a semi-wild translocated Yangtze finless porpoise population (Huang et al., 2017); and (3) using mainstem-only and mainstem+lakes population estimates. A default recovery factor (Fr) value of 0.1 was used (Wade and Angliss, 1997), and the standard normal deviate Z was fixed at -0.842 (Wade, 1998). Nmin (20th percentile of estimated population size) was calculated specifically for each estimate.
Logistic Growth Model
Logistic population growth for 2006–2012 was modeled in an Ordinary Differential Equation framework in R v3.4.3 with the DeSolve package (Soetaert et al., 2010), using:
where N = population estimate in any given year, t = year, K = population size at carrying capacity, and Ct = removal rate in year t, and with annual additions (A) to the population determined by:
The values of state, r, K, and Rmax were modified for each specific model. Identical Rmax values were used as for PBR analysis. In the absence of known carrying capacity values, highest recorded population estimates were used as proxies for population size at carrying capacity (K): mainstem = 2,546, Dongting Lake = 50, Poyang Lake = 450, total = 3,146 (Zhang et al., 1993; Zhao et al., 2008; Mei et al., 2014). Range-wide population estimates were 1,800 individuals in 2006 (Zhao et al., 2008) and 1,040 individuals in 2012 (Mei et al., 2014). Models were re-run using multiple values for starting population size and K: (1) range-wide model with N(2006) = 1,800 and K = 3,146; (2) mainstem-only with N(2006) = 1,225 and K = 2,546; (3–4) models 1 and 2 re-run to estimate maximum sustainable removal rates to maintain 2006 and 2012 range-wide and mainstem-only population estimates; (5–6) models 1 and 2 re-run to estimate actual removal rates required for observed range-wide and mainstem-only declines.
Results
For 100 km-section mainstem analysis, beta-binomial best-fit models and logit Gaussian model-averaged estimates both indicate that cargo vessel and live porpoise counts are significant positive predictors of reported mortality (Tables 1, 2). Fishing vessel density is also included in the final beta-binomial and logit Gaussian model sets as a negative predictor, but 95% confidence intervals include 0 in logit Gaussian model-averaged estimates, providing weaker support for a possible relationship. Best-fit models do not include other fishing activity indices. The null (intercept-only) model is included in both beta-binomial best-fit models and logit Gaussian model-averaged estimates.
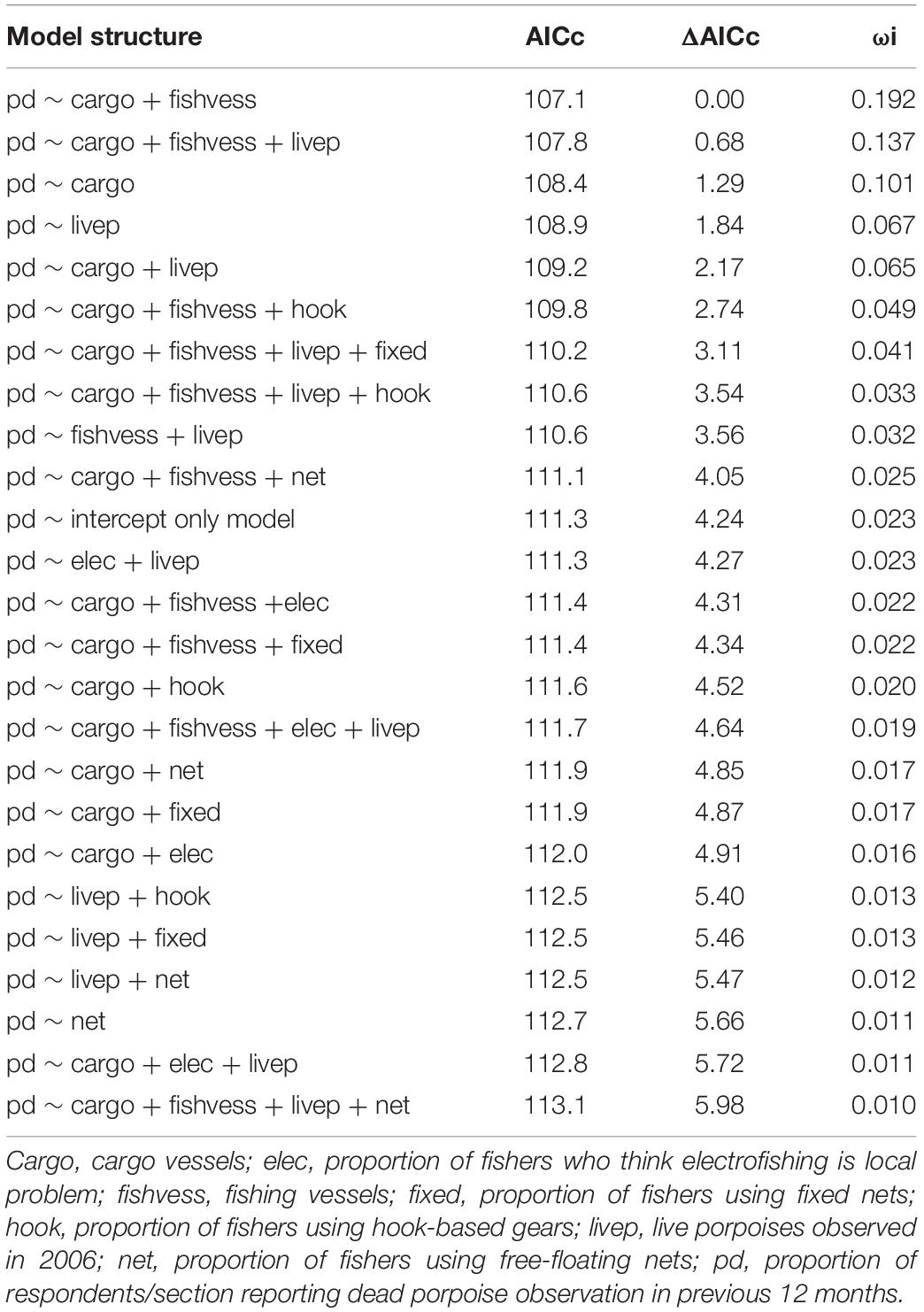
Table 1. Beta-binomial model selection showing Δ6 set for Yangtze-wide analysis, reporting Akaike Information Criterion corrected for small sample sizes (AICc), AICc scores (ΔAICc) and Akaike’s weight (ωi).
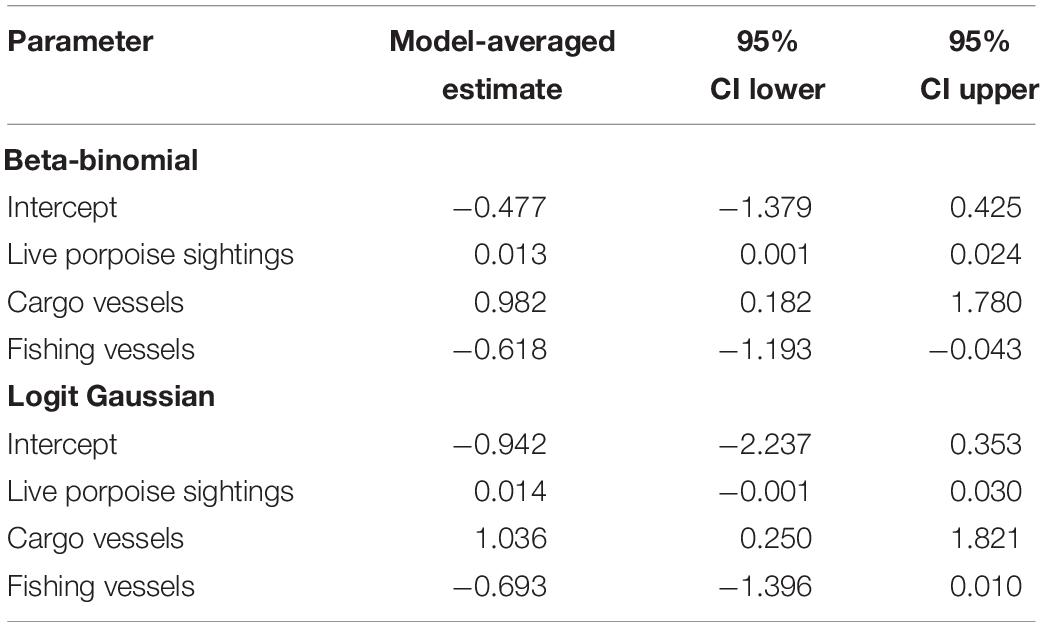
Table 2. Beta-binomial and logit Gaussian model-averaged parameter estimates for Yangtze-wide analysis.
For county-level analysis, model outputs indicate that mortality is not predicted by any fishing-based predictors. Final model selection includes only the null model, with all other model structures nested and discounted (Supplementary Table 3).
Neither porpoises nor gear categories are distributed evenly across nearshore-offshore bins (porpoises, 2006: X2 = 62.19, p < 0.0001; porpoises, 2012: X2 = 40.46, p < 0.0001; free-floating nets, X2 = 106.95, p < 0.0001; hook-type gears, X2 = 40.48, p < 0.0001; df = 3 for all analyses; Figure 2). Spearman rank tests show no correlation in porpoise or gear relative abundance across bins [porpoises vs. free-floating nets (2006), rs = 0.80, p = 0.333; porpoises vs. free-floating nets (2012), rs = −0.20, p = 0.917; porpoises vs. hook-type gears (2006), rs = 0.74, p = 0.262; porpoises vs. hook-type gears (2012), rs = −0.32, p = 0.684]. Gear use is biased toward near-bank habitats, being predominantly used ≤ 100 m from shore (hook-type gears, 53.9%; free-floating nets, 50.2%), whereas porpoises were observed most frequently 101–300 m from shore (2006, 36.4%; 2012, 43.6%). Across-river porpoise distribution also differs significantly between survey years (X2 = 24.48, p < 0.0001), with 32.1% observed ≤ 100 m from shore in 2006 but only 13.3% in 2012.
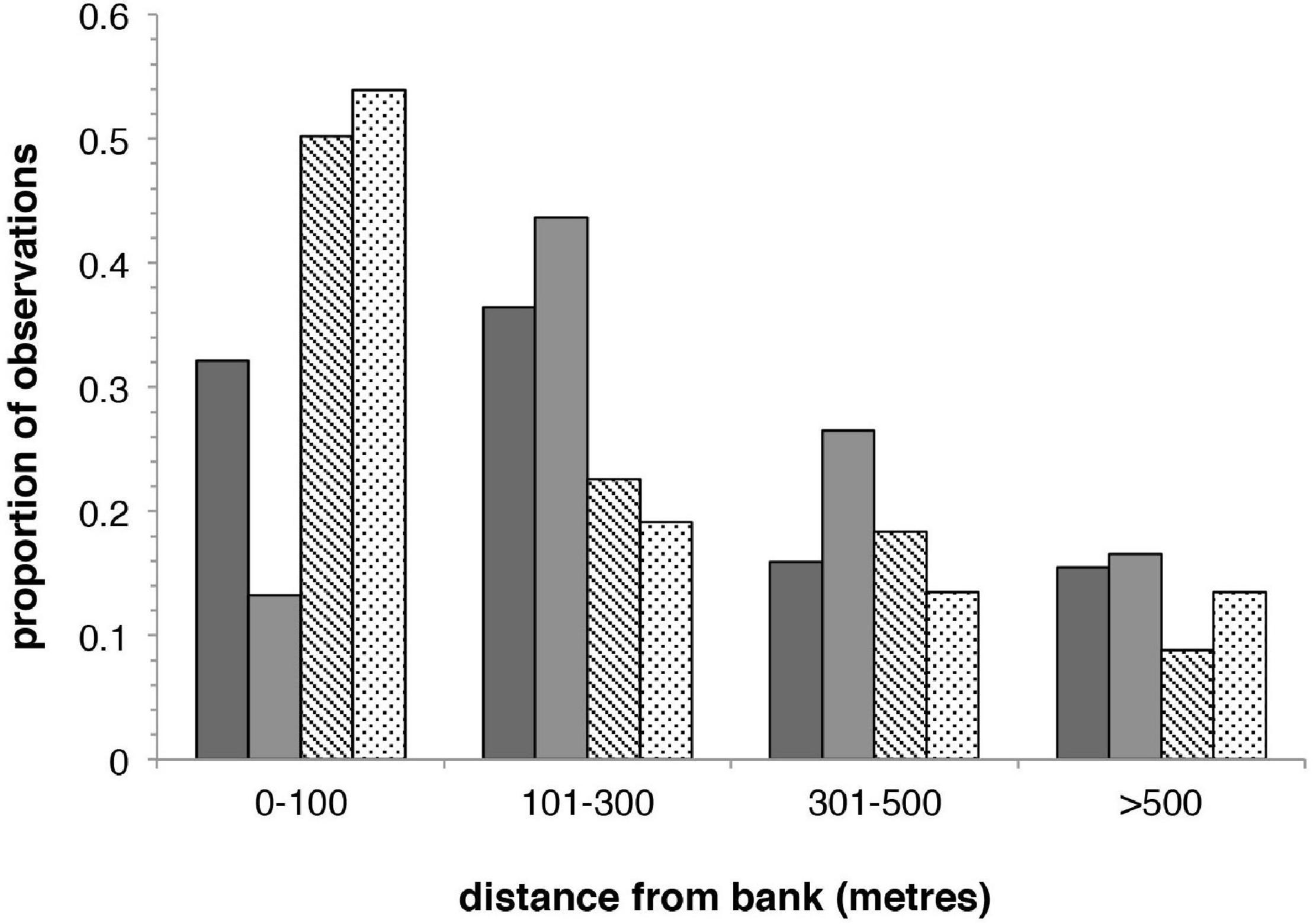
Figure 2. Proportions of porpoise observations and reported use of fishing gears in four distance-from-bank bins across Yangtze mainstem. Dark gray, 2006 porpoise data (n = 439); mid-gray, 2012 porpoise data (n = 181); hashed bar, free-floating nets (n = 283); dotted bar, hook-type gears (n = 89).
There was a significant decrease between survey years in the proportion of calves: in 2006, mean proportion of calves was 0.20 (95% confidence intervals, 0.17–0.24), whereas in 2012, mean proportion was 0.067 (0.045–0.10) (X2 = 31.7, df = 1, p < 0.001).
Using the PBR model, maximum annual removal from the total population to allow an optimum sustainable population is always ≤ 3.35 individuals (Table 3). The logistic model estimates removal values that are nearly ten times higher, and predicts a maximum annual removal of 30 individuals using 2006 population size to allow an optimum sustainable population (Table 4). To generate the observed 2006–2012 decline, the logistic model always requires annual removal of ≥ 140 individuals. Thirty mainstem deaths were reported by respondents as having been observed during the 12 months before the 2008 interview survey (fishery-based trauma, n = 6; vessel collisions, n = 7; unknown cause, n = 17; Turvey et al., 2013). Using this baseline minimum removal rate, the 2006 logistic model predicts a decline, but not severe enough to produce the observed 2012 mainstem-only estimate of 505 individuals; observed deaths account for 21.0–21.5% of total predicted annual mortality (Supplementary Table 4).
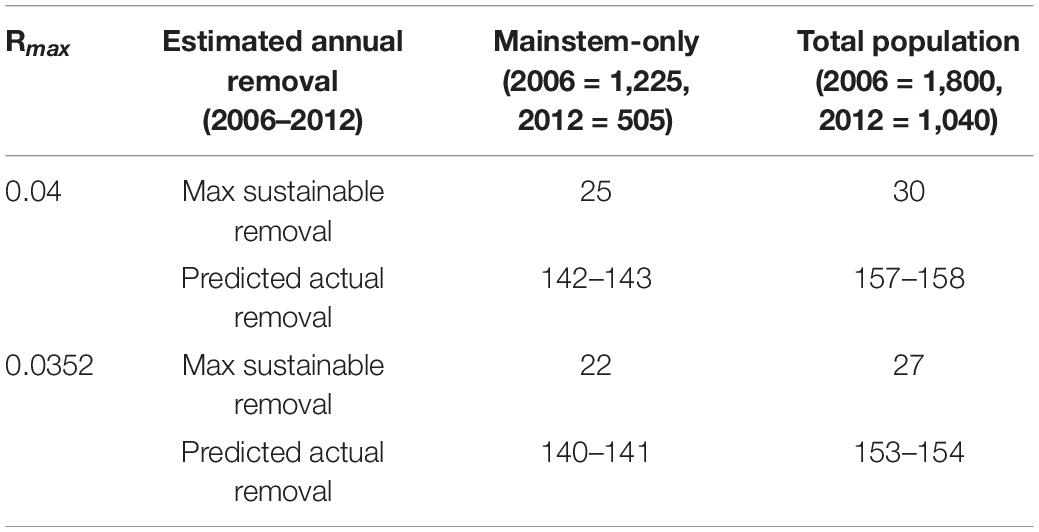
Table 4. Estimated annual removals in logistic model from mainstem-only and total porpoise populations required to maintain stable 2006 population size (maximum sustainable removal) or result in observed 2012 population size (predicted actual removal).
Discussion
Our combined analyses of different potential threats to the Critically Endangered Yangtze finless porpoise demonstrate the importance of an integrative approach for investigating complex conservation problems, rather than only considering single anthropogenic threats and their impacts within multi-threat landscapes. By assessing the information content of existing conservation data, we demonstrate that critical consideration of even limited available datasets can provide important new insights to help understand population decline and guide future work. These findings support the possibility of evidence-based conservation management even for data-poor species.
Although our along-river analyses were conducted at a relatively coarse spatial scale, this spatial framework has been sufficient to provide useful conservation-relevant insights in previous studies (Turvey et al., 2010, 2013), and best-model sets contained better models than the null model. This approach identified a correlation between high cargo vessel densities and increased dead porpoise observations, supporting the suggestion that direct and/or indirect impacts of ship traffic (e.g., collisions, disturbance, anthropogenic noise) might be responsible for reducing porpoise viability and causing mortality (Li et al., 2008; Wang, 2009; Turvey et al., 2013). These findings highlight the urgent need for improved monitoring of vessel traffic and its environmental impact in the Yangtze, and further research into the dynamics and sustainability of interactions between porpoises and cargo vessels, which remain difficult to detect or infer in the absence of specific targeted studies.
Conversely, whereas fisheries bycatch is a key cause of mortality in small cetaceans (Reeves et al., 2013) and several Yangtze finless porpoise bycatch events are documented (Zhou and Wang, 1994; Wang et al., 2000, 2015; Turvey et al., 2013), available data show limited correlation between porpoise deaths and fishing activity even when analyzed across multiple spatial scales. Indeed, the only statistically significant correlation is an unexpected negative relationship between fishing vessel density and reported porpoise mortality in along-river analysis, although we note that this pattern is weak or questionable because 95% confidence intervals include 0 in some model-averaged estimates; any such relationship may potentially reflect a correlation with other landscape-level factors (e.g., greater localized amounts of fish resources for both subsistence fishers and porpoises in more anthropologically undisturbed river sections). If fisheries interactions are genuinely not involved in driving porpoise decline, our across-river analyses provide a potential reason why: porpoises and fishers tend not to use the same parts of the river, with porpoises observed most frequently 101–300 m from the bank, whereas free-floating nets and hook-type gears are used most frequently ≤ 100 m from the bank. Indeed, whereas hook-type gears account for 45.2% of observed porpoise bycatch events (Turvey et al., 2013), this gear category shows less overlap than free-floating nets with porpoise distribution.
However, we note that respondents reported similar levels of porpoises killed by fishery-based trauma and vessel collisions, suggesting that fisheries bycatch may also be an important driver of porpoise decline in this system. If this is indeed the case, various factors might explain the limited relationship we observed in our analyses between porpoise mortality and fishing activity. Although protocols have been developed to reduce reporting inaccuracy by respondents and detect ecological signals in interview datasets (e.g., Newing, 2011; Turvey et al., 2015b), these data can be affected by multiple biases (e.g., declining recall accuracy, misremembering) that potentially complicate our attempt to reconstruct porpoise mortality patterns. In particular, levels of bycatch reporting are often affected by respondent reticence in discussing sensitive or illegal behaviors, with fishers reluctant to report porpoise deaths (Lien et al., 1994; Slooten and Dawson, 2016). Furthermore, not all deaths will be observed, and carcasses might also drift downstream (Peltier et al., 2012; Moore et al., 2020); although we note that the coarse spatial scales used in along-river analyses should reduce mismatches between locations of porpoise deaths and subsequent carcass detections, and the fact that live porpoise counts are a significant positive predictor of dead porpoise counts in 100 km-section analysis suggests a local origin for observed dead individuals. However, our spatial data resolution may not detect finer-scale bycatch mortality patterns and correlates (especially for electrofishing, a potential threat for which we could only use indirect indices).
Porpoise distribution is influenced at local scales by hydrology, water quality, substrate and fish abundance (Wei et al., 2003; Zhang et al., 2015, 2018), so regional variation in habitat use by porpoises and fishers might be associated with higher overlap and bycatch risk. We therefore recommend additional field-based research into porpoise-fisheries interactions (including spatial mapping of areas of high fishing activity, and establishment of fisher observer programs) to identify possible bycatch-risk hotspots. Data on Yangtze finless porpoise prey species are still limited (Chen et al., 1980; Yang et al., 2021); future research into the identity and diversity of porpoise prey, and their local distribution, population trends and importance in regional fisheries, is also a priority. Furthermore, 2006 and 2012 survey data show marked differences in across-river porpoise distribution, with fewer porpoises observed near the bank in 2012. If this represents a genuine change in habitat use (versus undercounting of porpoises near the bank, and furthest from mid-channel survey vessels, in 2012), porpoise-gear overlap has decreased. Ecological reasons for this shift are unclear, but it could indicate increasing anthropogenic degradation of near-shore habitats, or elevated mortality from near-shore fishing.
Expected proportions of calves in a healthy Yangtze finless porpoise population are unknown; no pre-decline baseline exists, and data for other porpoise taxa vary between ∼2-27% of the population (Kasuya and Kureha, 1979; Siebert et al., 2006; Thomsen et al., 2007). Although our analysis of between-year reproductive variation is relatively simple, it indicates that significantly fewer calves were recorded in 2012 compared to 2006. We note that reproduction is an annually variable trait in many cetacean populations (e.g., Manlik et al., 2016), and several uncontrolled factors can influence cetacean count data (Dawson et al., 2008); however, both surveys were conducted using the same methods and across the same area (Zhao et al., 2008; Mei et al., 2014). We thus interpret these results as suggesting reproductive success has decreased between the two survey years. A comparable pattern was observed (although not tested statistically) during baiji decline, with proportion of observed immature individuals decreasing from 31 to 17% in 1985–1999 (Zhang et al., 2003). Multiple mechanisms could reduce porpoise reproductive success and/or survivorship, hindering identification of cause(s). High concentrations of pollutants that affect cetacean reproduction and calf survival (heavy metals, persistent organic pollutants) have been found in Yangtze finless porpoise carcasses (Dong et al., 2006; Yang et al., 2008; Xiong et al., 2019), Yangtze fish (Xian et al., 2008), and the Yangtze River (Müller et al., 2008), with highest mercury concentrations and polychlorinated biphenyl hazard quotients found in porpoise calves (Dong et al., 2006; Yang et al., 2008). Although few data exist on specific Yangtze fish decreases (including porpoise prey species), fish stocks have declined substantially through habitat loss/degradation and damming (Ye et al., 2013), and decreased prey availability is associated with reduced reproductive success in several cetaceans (Mann, 2000; Ford et al., 2009). Anthropogenic noise from vessels and industrial activities is a source of environmental stress for Yangtze cetaceans (Zhao et al., 2008; Wang, 2009), and is implicated in reduced porpoise reproductive success through physiological damage, hormonal stress, behavioral alteration, and ecological effects on prey species (Nabi et al., 2018). Reproductive fitness may also be affected by reduced genetic diversity seen in the surviving porpoise population (Chen et al., 2017).
Predicted sustainable offtake rates differ between our population models by an order of ∼10 due to differences in model structure and aims: whereas logistic modeling only considers demographic and ecological parameters (e.g., point estimates of N) and does not explicitly consider uncertainty, PBR uses Nmin and incorporates a precautionary recovery factor to identify whether sustainable removal thresholds have been exceeded in post-depletion populations (Robards et al., 2009). Conservation target-setting using the PBR approach can be affected by some sources of data uncertainty (e.g., bias in abundance estimates, catastrophic events, trends in natural mortality; Punt et al., 2020), but there is no evidence that these constitute significant concerns in this system. However, as PBR is a conservative management technique, higher removal rates might still be sustainable in the longer-term than predicted in our model. Furthermore, mainstem-only models might be invalidated if this section does not contain a closed population (Taylor, 1997), but instead experiences movement of individuals between the appended lakes, which is suggested although not demonstrated (Li et al., 2010; Huang et al., 2017). We have also assumed that modeled mortality levels represent human-caused deaths, because observed porpoise mortality has increased as the population declined over recent decades (Turvey et al., 2013); the number of deaths would instead have decreased with decreasing porpoise population size if these events were mostly due to natural causes, but we acknowledge that some observed deaths may be natural. Model outputs therefore represent guidelines only under certain population scenarios.
However, all models show that observed mortality levels are sufficient to drive decline, indicating that current direct mortality is likely to be unsustainable even with stable reproduction and carrying capacity. A precautionary approach suggests the lowest predicted sustainable removal threshold, the PBR estimates based upon 2012 porpoise population data, should constitute a management guideline (Milner-Gulland and Akçakaya, 2001). These predicted PBR sustainable removal thresholds are comparable to values estimated for other highly threatened cetaceans (e.g., Maui’s dolphin Cephalorhynchus hectori maui; Slooten and Dawson, 2008), and suggest that reported mortality from both fishing and vessel strikes exceed sustainable levels; even if either threat was mitigated effectively, direct mortality would remain too high to maintain a stable population. Using these PBR thresholds to guide management would make maximum allowable annual mortality less than one porpoise in the mainstem, and less than two porpoises for the entire Yangtze.
Conversely, reported deaths account for only ∼21% of predicted annual mortality required to drive observed mainstem decline in the logistic model. This might suggest that reduction in survivorship and/or carrying capacity have also been important in driving decline, as demonstrated in population modeling comparing relative effects of anthropogenic removal vs. reducing prey availability in other cetaceans (Williams et al., 2016). We note that available mortality records were derived from a small respondent sample; for example, only 27 fishers were interviewed between Huangshi-Dongzhi in 2008, whereas this section contained 1,982 licensed fishing families in 2013 (Turvey et al., 2015a). Additional interviews might therefore yield considerably more records. However, as discussed above, respondent data contain multiple sources of uncertainty, making it challenging to infer the level of underestimation in our data. Although actual mortality levels could be substantially greater than estimated, we caution against extrapolating mortality data from small respondent samples across much larger respondent populations.
Conclusion
Our combined analyses of available datasets highlight what we can currently infer about threats associated with Yangtze finless porpoise decline, and also highlight what we still do not know. We provide evidence of a spatial association between mortality and cargo traffic; observed mortality levels (probably underestimates of true levels) are unsustainable; and recruitment is decreasing, although multiple factors could be driving this trend. Conversely, although we demonstrate that even a small annual mortality rate from bycatch is enough to drive porpoise decline, we cannot clarify a relationship between porpoise mortality and fishing activities using existing data, and we emphasize the urgent need for further applied research into the significance and spatial dynamics of this potential threat. These findings also suggest that existing precautionary management efforts that focus upon mitigating potential fisheries impacts within the Yangtze system may be insufficient to prevent further porpoise declines, and highlight the importance of adopting an evidence-based approach to investigate the information content of available datasets.
Uncertainty persists over impacts of different anthropogenic activities on porpoises across the Yangtze, and we recognize that porpoise decline might represent a cumulative effect of multiple stressors, a widely recognized problem in many aquatic systems facing complex threats; we encourage further research to investigate combined effects and potential interactions (additive, synergistic or antagonistic) between stressors in the Yangtze system (Crain et al., 2008; Côté et al., 2016). In particular, we recognize that the inevitable biases inherent to interview datasets are likely to represent a key limitation to our current understanding of patterns, levels and drivers of porpoise mortality in the Yangtze, especially with regard to fishers reporting sensitive information to outsiders on porpoise-fisheries interactions. To reduce such data limitations, it is crucial to conduct more systematic investigation of porpoise mortality to obtain direct data on deaths caused by different anthropogenic factors, ideally through a strandings investigation program that includes post-mortem examinations of all dead individuals using internationally established protocols (Wang et al., 2015; IJsseldijk et al., 2019).
However, our new baseline can help guide future research priorities, highlighting the need for targeted monitoring, research and modeling of potential threats across priority habitats. The current precautionary approach to reducing fishing pressure may provide beneficial impacts on wider biodiversity, but in situ management should also mitigate additional threats. More widely, critical assessment of available data for other threatened cetaceans can hopefully be used to understand sustainability or otherwise of different human activities in other poorly understood freshwater and marine systems, as a tool for reducing bycatch associated with commercial fishing operations (e.g., to meet the newly established Import Provisions of the United States Marine Mammal Protection Act; NOAA Fisheries, 2021). We encourage greater collaboration and sharing of existing datasets for threatened species, to maximize understanding of conservation-relevant data content and prevent time and resources from being wasted in biodiversity conservation (Mace et al., 2000; Haddaway, 2015).
Data Availability Statement
The original contributions presented in the study are included in the article/Supplementary Material, further inquiries can be directed to the corresponding author/s.
Ethics Statement
The studies involving human participants were reviewed and approved by the Zoological Society of London Ethics Committee. Written informed consent for participation was not required for this study in accordance with national legislation and institutional requirements.
Author Contributions
LM and ST conceived ideas, designed methodology, and led writing of manuscript. ST, ZM, YH, and DW collected the data. LM, XH, and ST analyzed the data. All authors contributed critically to drafts and gave final approval for publication.
Funding
Funding was provided by a Ph.D. studentship from the Natural Environment Research Council doctoral training partnership.
Conflict of Interest
The authors declare that the research was conducted in the absence of any commercial or financial relationships that could be construed as a potential conflict of interest.
Publisher’s Note
All claims expressed in this article are solely those of the authors and do not necessarily represent those of their affiliated organizations, or those of the publisher, the editors and the reviewers. Any product that may be evaluated in this article, or claim that may be made by its manufacturer, is not guaranteed or endorsed by the publisher.
Acknowledgments
We thank Helen Chatterjee for logistical support.
Supplementary Material
The Supplementary Material for this article can be found online at: https://www.frontiersin.org/articles/10.3389/fmars.2021.791484/full#supplementary-material
References
Bower, S. D., Brownscombe, J. W., Birnie-Gauvin, K., Ford, M. I., Moraga, A. D., Pusiak, R. J. P., et al. (2018). Making tough choices: picking the appropriate conservation decision-making tool. Conserv. Lett. 11:e12418. doi: 10.1111/conl.12418
Carr, M. H., and Raimondi, P. T. (1999). Marine protected areas as a precautionary approach to management. CalCOFI Rep. 40, 71–76.
Catullo, G., Masi, M., Falcucci, A., Maiorano, L., Rondinini, C., and Boitani, L. (2008). A gap analysis of southeast Asian mammals based on habitat suitability models. Biol. Conserv. 141, 2730–2744. doi: 10.1016/j.biocon.2008.08.019
Chen, M., Fontaine, M. C., Chehida, Y. B., Zheng, J., Labbé, F., Mei, Z., et al. (2017). Genetic footprint of population fragmentation and contemporary collapse in a freshwater cetacean. Sci. Rep. 7:14449. doi: 10.1038/s41598-017-14812-z
Chen, P., Liu, P., Liu, R., Lin, K., and Pilleri, G. (1980). Distribution, ecology, behaviour and conservation of the dolphins of the middle reaches of Changjiang (Yangtze) River (Wuhan-Yueyang). Investig. Cetacea 10, 87–103.
Cooney, R. (2004). The Precautionary Principle in Biodiversity Conservation and Natural Resource Management: An Issues Paper for Policy-Makers, Researchers and Practitioners. Gland: IUCN.
Côté, I. M., Darling, E. S., and Brown, C. J. (2016). Interactions among ecosystem stressors and their importance in conservation. Proc. R. Soc. B 283:20152592. doi: 10.1098/rspb.2015.2592
Crain, C. M., Kroeker, K., and Halpern, B. S. (2008). Interactive and cumulative effects of multiple human stressors in marine systems. Ecol. Lett. 11, 1304–1315.
Dawson, S., Wade, P., Slooten, E., and Barlow, J. (2008). Design and field methods for sighting surveys of cetaceans in coastal and riverine habitats. Mamm. Rev. 38, 19–49. doi: 10.1111/j.1365-2907.2008.00119.x
Dong, W., Xu, Y., Wang, D., and Hao, Y. (2006). Mercury concentrations in Yangtze finless porpoises (Neophocaena phocaenoides asiaeorientalis) from eastern Dongting Lake, China. Fresenius Environ. Bull. 15, 441–447.
Ford, J. K. B., Ellis, G. M., Olesiuk, P. F., and Balcomb, K. C. (2009). Linking killer whale survival and prey abundance: food limitation in the oceans’ apex predator? Biol. Lett. 6, 139–142. doi: 10.1098/rsbl.2009.0468
Grantham, H. S., Moilanen, A., Wilson, K. A., Pressey, R. L., Rebelo, T. G., and Possingham, H. P. (2008). Diminishing return on investment for biodiversity data in conservation planning. Conserv. Lett. 1, 190–198. doi: 10.1038/s41598-019-52241-2
Haddaway, N. R. (2015). A call for better reporting of conservation research data for use in meta-analyses. Conserv. Biol. 29, 1242–1245. doi: 10.1111/cobi.12449
Harrison, X. A. (2015). A comparison of observation-level random effect and beta-binomial models for modelling overdispersion in binomial data in ecology & evolution. PeerJ 3:e1114. doi: 10.7717/peerj.1114
Huang, S. L., Mei, Z., Hao, Y., Zheng, J., Wang, K., and Wang, D. (2017). Saving the Yangtze finless porpoise: time is rapidly running out. Biol. Conserv. 210, 40–46. doi: 10.1016/j.biocon.2016.05.021
IJsseldijk, L. L., Brownlow, A. C., and Mazzariol, S. (2019). Best Practice on Cetacean Post Mortem Investigation and Tissue Sampling. ACCOBAMS-MOP7/2019/Doc 33. Rotterdam: ACCOBAMS/ASCOBANS.
Ingram, D. J., Coad, L., Collen, B., Kümpel, N. F., Breuer, T., Fa, J. E., et al. (2015). Indicators for wild animal offtake: methods and case study for African mammals and birds. Ecol. Soc. 20:40.
IUCN (2021). The IUCN Red List of Threatened Species. Version 2021-1. Available online at: http://www.iucnredlist.org (accessed August 9, 2021).
Jaramillo-Legorreta, A., Rojas-Bracho, L., Brownell, R. L. Jr., Read, A. J., Reeves, R. R., Ralls, K., et al. (2007). Saving the vaquita: immediate action, not more data. Conserv. Biol. 21, 1653–1655. doi: 10.1111/j.1523-1739.2007.00825.x
Kasuya, T., and Kureha, K. (1979). The population of finless porpoise in the Inland Sea of Japan. Sci. Rep. Whales Res. Inst. 31, 1–44. doi: 10.5181/cetology.0.23_1
Kimura, S., Akamatsu, T., Li, S., Dong, L., Wang, K., Wang, D., et al. (2012). Seasonal changes in the local distribution of Yangtze finless porpoises related to fish presence. Mar. Mamm. Sci. 28, 308–324.
Li, S., Akamatsu, T., Wang, D., Wang, K., Dong, S., Zhao, X., et al. (2008). Indirect evidence of boat avoidance behavior of Yangtze finless porpoises. Bioacoustics 17, 174–176. doi: 10.1080/09524622.2008.9753806
Li, S., Dong, S., Kimura, S., Akamatsu, T., Wang, K., and Wang, D. (2010). “Detection of Yangtze finless porpoises in the Poyang Lake mouth area via passive acoustic data-loggers,” in Biology, Evolution and Conservation of River Dolphins Within South America and Asia, eds J. M. Shostell and M. Ruiz-Garcia (New York, NY: Nova Science Publishers), 341–356.
Lien, J., Stenson, G. B., Carver, S., and Chardine, J. (1994). “How many did you catch? The effects of methodology on bycatch reports obtained from fishermen,” in Gillnets and Cetaceans, eds W. F. Perrin, G. P. Donovan, and J. Barlow (Cambridge: International Whaling Commission), 535–540.
Lindenmayer, D. B., Piggott, M. P., and Wintle, B. A. (2013). Counting the books while the library burns: why conservation monitoring programs need a plan for action. Front. Ecol. Environ. 11:549–555. doi: 10.1890/120220
Mace, G. M., Balmford, A., Boitani, L., Cowlishaw, G., Dobson, A. P., Faith, D. P., et al. (2000). It’s time to work together and stop duplicating conservation efforts. Nature 405:393. doi: 10.1038/35013247
MacMillan, D. C., and Marshall, K. (2006). The Delphi process—an expert-based approach to ecological modelling in data-poor environments. Anim. Conserv. 9, 11–19. doi: 10.1111/j.1469-1795.2005.00001.x
Manlik, O., McDonald, J. A., Mann, J., Raudino, H. C., Bejder, L., Krützen, M., et al. (2016). The relative importance of reproduction and survival for the conservation of two dolphin populations. Ecol. Evol. 6, 3496–3512. doi: 10.1002/ece3.2130
Mann, J. (2000). Female reproductive success in bottlenose dolphins (Tursiops sp.): life history, habitat, provisioning, and group-size effects. Behav. Ecol. 11, 210–219. doi: 10.1093/beheco/11.2.210
McDonald-Madden, E., Baxter, P. W. J., and Possingham, H. P. (2008). Making robust decisions for conservation with restricted money and knowledge. J. Appl. Ecol. 45, 1630–1638. doi: 10.1111/j.1365-2664.2008.01553.x
Mei, Z., Zhang, X., Huang, S.-L., Zhao, X., Hao, Y., Zhang, L., et al. (2014). The Yangtze finless porpoise: on an accelerating path to extinction? Biol. Conserv. 172, 117–123. doi: 10.1016/j.biocon.2014.02.033
Milner-Gulland, E. J., and Akçakaya, H. R. (2001). Sustainability indices for exploited populations. Trends Ecol. Evol. 16, 686–692. doi: 10.1016/s0169-5347(01)02278-9
Monnahan, C. C., Branch, T. A., and Punt, A. E. (2015). Do ship strikes threaten the recovery of endangered eastern North Pacific blue whales? Mar. Mamm. Sci. 31, 279–297. doi: 10.1111/mms.12157
Moore, M. J., Mitchell, G. H., Rowles, T. K., and Early, G. (2020). Dead cetacean? Beach, bloat, float, sink. Front. Mar. Sci. 7:333. doi: 10.3389/fmars.2020.00333
Müller, B., Berg, M., Yao, Z., Zhang, X., Wang, D., and Pfluger, A. (2008). How polluted is the Yangtze river? Water quality downstream from the Three Gorges Dam. Sci. Total Environ. 402, 232–247. doi: 10.1016/j.scitotenv.2008.04.049
Nabi, G., McLaughlin, R. W., Hao, Y., Wang, K., Zeng, X., Khan, S., et al. (2018). The possible effects of anthropogenic acoustic pollution on marine mammals’ reproduction: an emerging threat to animal extinction. Environ. Sci. Pollut. Res. 25, 19338–19345. doi: 10.1007/s11356-018-2208-7
Newing, H. (2011). Conducting Research in Conservation: A Social Science Perspective. Abingdon: Routledge. doi: 10.4324/9780203846452
NOAA Fisheries (2021). NOAA Fisheries Establishes International Marine Mammal Bycatch Criteria for U.S. Imports. Available online at: https://www.fisheries.noaa.gov/foreign/marine-mammal-protection/noaa-fisheries-establishes-international-marine-mammal-bycatch-criteria-us-imports (accessed December 1, 2021).
Pan, M., and Huntington, H. P. (2015). A precautionary approach to fisheries in the central Arctic ocean: policy, science, and China. Mar. Policy 63, 153–157. doi: 10.1016/j.marpol.2015.10.015
Peltier, H., Dabin, W., Daniel, P., Van Canneyt, O., Dorémus, G., Huon, M., et al. (2012). The significance of stranding data as indicators of cetacean populations at sea: modelling the drift of cetacean carcasses. Ecol. Indic. 18, 278–290. doi: 10.1371/journal.pone.0062180
Punt, A. E., Siple, M., Francis, T. B., Hammond, P. S., Heinemann, D., Long, K. J., et al. (2020). Robustness of potential biological removal to monitoring, environmental, and management uncertainties. ICES J. Mar. Sci. 77, 2491–2507. doi: 10.1093/icesjms/fsaa096
R Development Core Team (2017). R: A Language and Environment for Statistical Computing. Vienna: R Foundation for Statistical Computing.
Reeves, R. R., McClellan, K., and Werner, T. B. (2013). Marine mammal bycatch in gillnet and other entangling net fisheries, 1990 to 2011. Endanger. Species Res. 20, 71–97. doi: 10.3354/esr00481
Richards, S. A. (2008). Dealing with overdispersed count data in applied ecology. J. Appl. Ecol. 45, 218–227. doi: 10.1111/j.1365-2664.2007.01377.x
Robards, M. D., Burns, J. J., Meek, C. L., and Watson, A. (2009). Limitations of an optimum sustainable population or potential biological removal approach for conserving marine mammals: Pacific walrus case study. J. Environ. Manage. 91, 57–66. doi: 10.1016/j.jenvman.2009.08.016
Rodrigues, A. S. L. (2011). Improving coarse species distribution data for conservation planning in biodiversity-rich, data-poor, regions: no easy shortcuts. Anim. Conserv. 14, 108–110. doi: 10.1111/j.1469-1795.2011.00451.x
Rojas-Bracho, L., Brusca, R. C., Álvarez-Borrego, S., Brownell, R. L. Jr., Camacho-Ibar, V., Ceballos, G., et al. (2019). Unsubstantiated claims can lead to tragic conservation outcomes. Bioscience 69, 12–14. doi: 10.1093/biosci/biy138
Sampaio, F. D. F., Freire, C. A., Sampaio, T. V. M., Vitule, J. R. S., and Fávaro, L. F. (2015). The precautionary principle and its approach to risk analysis and quarantine related to the trade of marine ornamental fishes in Brazil. Mar. Policy 51, 163–168. doi: 10.1016/j.marpol.2014.08.003
Siebert, U., Gilles, A., Lucke, K., Ludwig, M., Benke, H., Kock, K. H., et al. (2006). A decade of harbour porpoise occurrence in German waters—analyses of aerial surveys, incidental sightings and strandings. J. Sea Res. 56, 65–80. doi: 10.1016/j.seares.2006.01.003
Slooten, E., and Dawson, S. M. (2008). Sustainable levels of human impact for Hector’s dolphin. Open Conserv. Biol. J. 2, 37–43. doi: 10.2174/1874839200802010037
Slooten, E., and Dawson, S. M. (2016). Hector’s and Maui Dolphin Bycatch 1985-2015. International Whaling Commission Report SC/66b/SM/15. Cambridge: International Whaling Commission.
Soetaert, K., Petzoldt, T., and Setzer, W. R. (2010). Solving differential equations in R: package deSolve. J. Stat. Softw. 33, 1–25. doi: 10.18637/jss.v033.i09
Sutherland, W. J., Pullin, A. S., Dolman, P. M., and Knight, T. M. (2004). The need for evidence based conservation. Trends Ecol. Evol. 19, 305–308. doi: 10.1016/j.tree.2004.03.018
Taylor, B. L. (1997). Defining ‘population’ to meet management objectives for marine mammals. Mol. Genet. Mar. Mamm. 3, 49–65. doi: 10.1111/gcb.14296
Taylor, B. L., Chivers, S. J., Larese, J., and Perrin, W. F. (2007). Generation Length and Percent Mature Estimates for IUCN Assessments of Cetaceans. National Marine Fisheries Administrative Report LJ-07-01. La Jolla, CA: Southwest Fisheries Science Center, 1–24.
Thieme, M., Lehner, B., Abell, R., Hamilton, S. K., Kellndorfer, J., Powell, G., et al. (2007). Freshwater conservation planning in data-poor areas: an example from a remote Amazonian basin (Madre de Dios River, Peru and Bolivia). Biol. Conserv. 135, 500–517.
Thompson, P. M., Wilson, B., Grellier, K., and Hammond, P. S. (2000). Combining power analysis and population viability analysis to compare traditional and precautionary approaches to conservation of coastal cetaceans. Conserv. Biol. 14, 1253–1263. doi: 10.1046/j.1523-1739.2000.00099-410.x
Thomsen, F., Laczny, M., and Piper, W. (2007). The harbour porpoise (Phocoena phocoena) in the central German Bight: phenology, abundance and distribution in 2002-2004. Helgol. Mar. Res. 61, 283–289. doi: 10.1007/s10152-007-0075-6
Turvey, S. T., Barrett, L. A., Hart, T., Collen, B., Hao, Y., Zhang, L., et al. (2010). Spatial and temporal extinction dynamics in a freshwater cetacean. Proc. R. Soc. B 277, 3139–3147. doi: 10.1098/rspb.2010.0584
Turvey, S. T., Hao, Y., and Wang, D. (2015a). Identifying Conservation Solutions for the Yangtze Finless Porpoise (Neophocaena asiaeorientalis asiaeorientalis) Through Community Research. International Whaling Commission. Available online at: http://www.iucn-csg.org/wp-content/uploads/2010/03/Turvey_IWC_final_report.pdf (accessed December 1, 2021).
Turvey, S. T., Trung, C. T., Quyet, V. D., Nhu, H. V., Thoai, D. V., Tuan, V. C. A., et al. (2015b). Interview-based sighting histories can inform regional conservation prioritization for highly threatened cryptic species. J. Appl. Ecol. 52, 422–433. doi: 10.1111/1365-2664.12382
Turvey, S. T., Pitman, R. L., Taylor, B. L., Barlow, J., Akamatsu, T., Barrett, L. A., et al. (2007). First human-caused extinction of a cetacean species? Biol. Lett. 3, 537–540. doi: 10.1098/rsbl.2007.0292
Turvey, S. T., Risley, C. L., Moore, J. E., Barrett, L. A., Hao, Y., Zhao, X., et al. (2013). Can local ecological knowledge be used to assess status and extinction drivers in a threatened freshwater cetacean? Biol. Conserv. 157, 352–360. doi: 10.1016/j.biocon.2012.07.016
VanderWerf, E. A., Groombridge, J. J., Fretz, J. S., and Swinnerton, K. J. (2006). Decision analysis to guide recovery of the po’ouli, a critically endangered Hawaiian honeycreeper. Biol. Conserv. 129, 383–392. doi: 10.1016/j.biocon.2005.11.005
Wade, P. R. (1998). Calculating limits to the allowable human-caused mortality of cetaceans and pinnipeds. Mar. Mamm. Sci. 14, 1–37. doi: 10.1111/j.1748-7692.1998.tb00688.x
Wade, P. R., and Angliss, R. (1997). Guidelines for Assessing Marine Mammal Stocks: Report of the GAMMS Workshop. NOAA Technical Memorandum NMFS-OPR-12. Seattle, WA: U.S. Department of Commerce, 1–93.
Wang, D. (2009). Population status, threats and conservation of the Yangtze finless porpoise. Bull. Chin. Acad. Sci. 27, 3473–3484.
Wang, D., Liu, R., Zhang, X., Yang, J., Wei, Z., Zhao, Q., et al. (2000). “Status and conservation of the Yangtze finless porpoise,” in Biology and Conservation of Freshwater Cetaceans in Asia, eds R. R. Reeves, B. D. Smith, and T. Kasuya (Gland: IUCN), 81–85.
Wang, D., Zhang, X., and Liu, R. (1998). “Conservation status and its future of baiji and Yangtze finless porpoise in China,” in Ecology and Environmental Protection of Large Irrigation Projects in Yangtze River in 21st Century, eds Z. Hua, B. Fu, and Y. Yang (Beijing: Environmental Science Press), 218–226. doi: 10.1065/espr2006.10.350
Wang, Y., Li, W., and van Waerebeek, K. (2015). Strandings, bycatches and injuries of aquatic mammals in China, 2000-2006, as reviewed from official documents: a compelling argument for a nationwide strandings programme. Mar. Policy 51, 242–250.
Wang, Z., Akamatsu, T., Wang, K., and Wang, D. (2014). The diel rhythms of biosonar behavior in the Yangtze finless porpoise (Neophocaena asiaeorientalis asiaeorientalis) in the port of the Yangtze River: the correlation between prey availability and boat traffic. PLoS One 9:e97907. doi: 10.1371/journal.pone.0097907
Wei, Z., Wang, D., Zhang, X., Zhao, Q., Kuang, X., Wang, X., et al. (2003). Habitat use and preliminary evaluation of the habitat status of the Yangtze finless porpoise (Neophocaena phocaenoides asiaeorientalis) in the Balijiang section of the Yangtze River, China. Acta Zool. Sin. 49, 163–170.
Williams, R., Thomas, L., Ashe, E., Clark, C. W., and Hammond, P. S. (2016). Gauging allowable harm limits to cumulative, sub-lethal effects of human activities on wildlife: a case-study approach using two whale populations. Mar. Policy 70, 58–64. doi: 10.1016/j.marpol.2016.04.023
Xian, Q., Ramu, K., Isobe, T., Sudaryanto, A., Liu, X., Gao, Z., et al. (2008). Levels and body distribution of polybrominated diphenyl ethers (PBDEs) and hexabromocyclododecanes (HBCDs) in freshwater fishes from the Yangtze River, China. Chemosphere 71, 268–276. doi: 10.1016/j.chemosphere.2007.09.032
Xiong, X., Qian, Z., Mei, Z., Wu, J., Hao, Y., Wang, K., et al. (2019). Trace elements accumulation in the Yangtze finless porpoise (Neophocaena asiaeorientalis asiaeorientalis)—a threat to the endangered freshwater cetacean. Sci. Total Environ. 686, 797–804. doi: 10.1016/j.scitotenv.2019.06.031
Yang, F., Zhang, Q., Xu, Y., Jiang, G., Wang, Y., and Wang, D. (2008). Preliminary hazard assessment of polychlorinated biphenyls, polybrominated diphenyl ethers, and polychlorinated dibenzo-p-dioxins and dibenzofurans to Yangtze finless porpoise in Dongting Lake, China. Environ. Toxicol. Chem. 27, 991–996. doi: 10.1897/07-381.1
Yang, J., Wang, K., Mei, Z., Xu, J., Zheng, J., Wan, X., et al. (2021). Temporal variation in the diet of Yangtze finless porpoise calls for conservation of semi-migratory fish. Freshw. Biol. 66, 992–1001. doi: 10.1111/fwb.13692
Ye, S., Li, Z., Zhang, T., Liu, J., and Xie, S. (2013). Assessing fish distribution and threats to fish biodiversity in the Yangtze River Basin, China. Ichthyol. Res. 61, 183–188. doi: 10.3897/BDJ.9.e76669
Zhang, X., Liu, R., Zhao, Q., Zhang, G., Wei, Z., Wang, X., et al. (1993). The population of finless porpoise in the middle and lower reaches of Yangtze River. Acta Theriol. Sin. 13, 260–270.
Zhang, X., and Vincent, A. C. J. (2017). Integrating multiple datasets with species distribution models to inform conservation of the poorly-recorded Chinese seahorses. Biol. Conserv. 211, 161–171. doi: 10.1016/j.biocon.2017.05.020
Zhang, X., Wang, D., Liu, R., Wei, Z., Hua, Y., Wang, Y., et al. (2003). The Yangtze River dolphin or baiji (Lipotes vexillifer): population status and conservation issues in the Yangtze River, China. Aquat. Conserv. 13, 51–64. doi: 10.1002/aqc.547
Zhang, X., Wang, H., Wan, A., Chen, M., Wang, K., and Yu, D. (2018). Effects of macrozoobenthos and zooplankton on the occurrence of Yangtze finless porpoise via the bottom-up force at a confluence of rivers. Nat. Environ. Pollut. Technol. 17, 175–182.
Zhang, X., and Wang, K. (1999). Population viability analysis for the Yangtze finless porpoise. Acta Ecol. Sin. 19, 529–533. doi: 10.1007/s11626-011-9448-x
Zhang, X., Yu, D., Wang, H., Wan, A., Chen, M., Tao, F., et al. (2015). Effects of fish community on occurrences of Yangtze finless porpoise in confluence of the Yangtze and Wanhe rivers. Environ. Sci. Pollut. Res. Int. 22, 9524–9533. doi: 10.1007/s11356-015-4102-x
Zhao, X., Barlow, J., Taylor, B. L., Pitman, R. L., Wang, K., Wei, Z., et al. (2008). Abundance and conservation status of the Yangtze finless porpoise in the Yangtze River, China. Biol. Conserv. 141, 3006–3018. doi: 10.1065/espr2006.10.350
Zhou, K., and Wang, X. (1994). Brief review of passive fishing gear and incidental catches of small cetaceans in Chinese waters. Rep. Int. Whal. Comm. Spec. Issue 15, 347–354.
Keywords: cetacean, conservation effectiveness, evidence-based conservation, interview survey, precautionary principle, sustainable offtake, uncertainty
Citation: Mogensen LMW, Mei Z, Hao Y, Harrison XA, Wang D and Turvey ST (2022) Precautionary Principle or Evidence-Based Conservation? Assessing the Information Content of Threat Data for the Yangtze Finless Porpoise. Front. Mar. Sci. 8:791484. doi: 10.3389/fmars.2021.791484
Received: 08 October 2021; Accepted: 08 December 2021;
Published: 04 January 2022.
Edited by:
Jeff Moore, Southwest Fisheries Science Center (NOAA), United StatesReviewed by:
Xuming Zhou, Institute of Zoology, Chinese Academy of Sciences (CAS), ChinaTom Jefferson, Clymene Enterprises, United States
Copyright © 2022 Mogensen, Mei, Hao, Harrison, Wang and Turvey. This is an open-access article distributed under the terms of the Creative Commons Attribution License (CC BY). The use, distribution or reproduction in other forums is permitted, provided the original author(s) and the copyright owner(s) are credited and that the original publication in this journal is cited, in accordance with accepted academic practice. No use, distribution or reproduction is permitted which does not comply with these terms.
*Correspondence: Samuel T. Turvey, c2FtdWVsLnR1cnZleUBpb3ouYWMudWs=