- 1Programa de Pós-Graduação em Dinâmica dos Oceanos e da Terra, Universidade Federal Fluminense, Niterói, Brazil
- 2Departamento de Biologia Marinha, Universidade Federal Fluminense, Niterói, Brazil
- 3Departamento de Ciências Humanas e Tecnologias, Universidade do Estado da Bahia, Salvador, Brazil
- 4Departamento de Biologia Marinha, Instituto de Biologia, Universidade Federal do Rio de Janeiro, Rio de Janeiro, Brazil
- 5Programa de Pós Graduação em Genética, Instituto de Biologia, Universidade Federal do Rio de Janeiro, Rio de Janeiro, Brazil
- 6Programa de Pós-Graduação em Ecologia, Universidade Federal Universidade Federal do Rio de Janeiro, Rio de Janeiro, Brazil
- 7Departamento de Ciências do Mar, Universidade Federal de São Paulo, São Paulo, Brazil
- 8Laboratório de Avaliação e Monitoramento da Biodiversidade, Núcleo Rogério Valle de Produção Sustentável (SAGE), Instituto Alberto Luiz Coimbra de Pós-Graduação e Pesquisa de Engenharia, Universidade Federal do Rio de Janeiro, Rio de Janeiro, Brazil
Benthic cyanobacterial mats (BCMs) are conspicuous components of coral reef communities, where they play key ecological roles as primary producers among others. BCMs often bloom and might outcompete neighboring benthic organisms, including reef-building corals. We investigated the cyanobacterial species composition of three BCMs morphotypes from the marginal reef complex of Abrolhos Bank (Southeastern Brazil). Also, we assessed their allelopathic effects on coral zooxanthellae, their susceptibility to herbivory by fish, and their toxicity to brine shrimp nauplii. Morphology and 16S rDNA sequencing unveiled the cyanobacteria Moorena bouillonii, Okeania erythroflocculosa, Adonisia turfae, Leptolyngbya sp., and Halomicronema sp. as components of BCMs from Abrolhos. BCMs cell-free filtrates and extracts exerted an allelopathic effect by reducing the growth of the ex hospite Symbiodinium sp. in culture. BCMs-only treatments remained untouched in field susceptibility assays in contrast to macroalgae only and mixed BCMs-macroalgae treatments that had the macroalgae fully removed by reef fish. Crude aqueous extracts from BCMs were toxic to brine shrimps in acute assays. Besides unveiling the diversity of BCMs consortia in Abrolhos, our results cast some light on their allelopathy, antiherbivory, and toxicity properties. These antagonistic interactions might promote adverse cascading effects during benthic cyanobacteria blooms and in gradual shifts to BCMs-dominated states.
Introduction
Benthic cyanobacterial mats (BCMs) are ubiquitous in coral reef ecosystems, where they contribute to critical functions such as nitrogen fixation, sulfate reduction, and primary production (Charpy et al., 2007). The term BCMs is used to define a range of cyanobacteria-rich mats that might display diverse morphologies, from periphytic biofilms and horizontally-spreading mats over sediment and rubble, to tufts and fronds of various heights, up to tens of centimeters (Graham and Wilcox, 2000; Charpy et al., 2007; Cissell et al., 2019; Cissell and McCoy, 2021). Filamentous cyanobacteria can also be found entangled in other structurally more complex benthic assemblages, namely turf (Connell et al., 2014). These mats can be formed by a single genus (Paul et al., 2005) or by a consortium of multiple taxa (Echenique-Subiabre et al., 2015), including protists and non-cyanobacterial, heterotrophic prokaryotes, and viruses (Cissell and McCoy, 2021). Despite its relevance, the identity of BCMs components is often overlooked by morphology-based taxonomy (Engene et al., 2013a). The globally distributed genus Lyngbya C. Agardh ex Gomont is a foremost example of the cryptic diversity within benthic marine cyanobacteria. The recent combination of molecular, chemical, and morphological analyses revealed three additional genera [Moorena Engene and Tronholm, Okeania N. Engene et al., and Dapis Engene et al. (2018)] and several new species within the Lyngbya complex (Engene et al., 2012, 2013b,2018; Tronholm and Engene, 2019).
Increasing seawater warming and nutrient uploads stimulate BCMs growth which might lead to blooms in coastal areas (Sneed et al., 2017) and might be associated with the coral cover decline (Ford et al., 2018). Reef degradation generates newly available substrates easily occupied by fast-growing BCMs (e.g., Albert et al., 2005). BCMs can accentuate their presence even under limited space by growing directly over living organisms, such as scleractinian corals, fleshy algae, and seagrass (Yamamuro, 1999; Ritson-Williams et al., 2005; Puyana and Prato, 2013).
Cyanobacteria produce various cyclic and linear peptides, alkaloids, macro lactones, and heterocyclic compounds (Carroll et al., 2021). These chemicals can act as grazing deterrents against fishes (e.g., Thacker et al., 1997; Capper et al., 2016), sea urchins, mollusks, and crustaceans (see Leão et al., 2012), although some specialist sea hare (Capper and Paul, 2008; Ribeiro et al., 2017) and reef fish (Cissell et al., 2019) can actively graze on BCMs. Although cyanobacteria are an important source of nitrogen for herbivorous fish in marine (Yamamuro, 1999; Nicholson and Clements, 2020), and freshwater systems (Yang and Dudgeon, 2010), their toxicity and nutritional value is varied among taxa and within subpopulations (Nagarkar et al., 2004; Groendahl and Fink, 2017). Such variability also constrains the feeding behavior of consumers to meet their nutritional requirements (Mendes et al., 2018).
Cyanobacterial compounds also have allelopathic properties against competitors and may significantly impact the physiology of reef organisms (Thacker et al., 1998; Chadwick and Morrow, 2011), through microbiome disruption (Morrow et al., 2011). Such antagonistic interactions can generate associated cascading effects since competition strongly determines the structure and composition of coral reef communities (Birkeland, 1997). Benthic cyanobacterial mat allelopathic effects in coral reef environments are still poorly investigated (Brumley et al., 2018). Few studies have demonstrated that BCMs and the allelochemicals they produce can damage the coral’s tissue and microbiome (e.g., Titlyanov and Titlyanova, 2009; Morrow et al., 2011). In the nutrified and turbid reef systems of Abrolhos (Leão et al., 2016), BCMs have been associated with long-term impacts on coral growth (Ribeiro et al., 2018) and altered states of benthic dynamics (Teixeira et al., 2021).
Blooms of BCMs covering several benthic organisms have been observed in Abrolhos reefs during the summer (Ribeiro et al., 2017). Whereas the concentration of allelopathic chemicals depends on the BCMs assemblage structure (Brumley et al., 2018), the diffused compounds in benthic boundary layers could harm corals and alter trophic interactions (Brocke et al., 2015). Considering the coral tissue suppression by cyanobacteria previously reported (Ribeiro et al., 2018), we aimed to investigate the interaction between cyanobacteria and coral photosymbionts. Although symbiotic dinoflagellates are embedded in the gastrodermis, the constant mass transfer through boundary layers and tissues could expose them to toxic metabolites from the environment (Patterson, 1992). This pathway would be enhanced in the current global warming scenario (Teixeira et al., 2019) since tissue thickness is reduced in thermally stressed corals (Ainsworth et al., 2008), which increases mass transfer rates (Patterson, 1992).
We hypothesized that potentially toxic cyanobacteria within BCMs undermine coral physiology through the impairment of endosymbionts and disturb consumer–prey interactions. Here, we characterize the BCMs taxonomic composition of Abrolhos and evaluate BCM’s allelopathy, susceptibility to herbivory, and toxicity.
Materials and Methods
Sampling and Establishment of Cyanobacterial Cultures
Benthic cyanobacterial mats of three distinct morphotypes were manually collected by SCUBA divers between 3 and 8 m depth in the Abrolhos Bank (16°40′, 19°40′S—39°10′, 37°20′W) (Figure 1). Sampling was performed at two different sites on pinnacle reef tops: the nearshore Pedra de Leste (PLES) of Parcel das Paredes reef and the offshore site of Parcel dos Abrolhos reef (PAB). Throughout the text samples, PLES and PAB will be referred to as PLESBCM and PABBCM, respectively. The BCMs cover and long-term survey data are described in Teixeira et al. (2021). An aliquot of each sample was rinsed with filtered (GF/F) seawater and snap-frozen or fixed in 1% glutaraldehyde. Additional aliquots were transferred to vials containing f/2 medium (Guillard, 1975), prepared with 0.22 μm filtered Abrolhos seawater or filtered seawater, and transported to the laboratory. Single cyanobacterial filaments were isolated in a flow cytometer (MoFlo, Beckman Coulter, United States) (Fistarol et al., 2018) or by manual micromanipulation under an inverted microscope in case of wider filaments. Collected filaments were chosen to encompass distinct diameter diversities within conspicuous BCMs morphotypes in the Abrolhos Bank. Isolated filaments were transferred to f/2 medium (Guillard, 1975), prepared with 0.22 μm filtered Abrolhos seawater, and kept at 26°C with a 14:10-h light/photoperiod and ca. 20 μmol photons m–2 s–1. Established cultures were deposited in the Culture Collection of Microorganisms at UFRJ (CCMR).
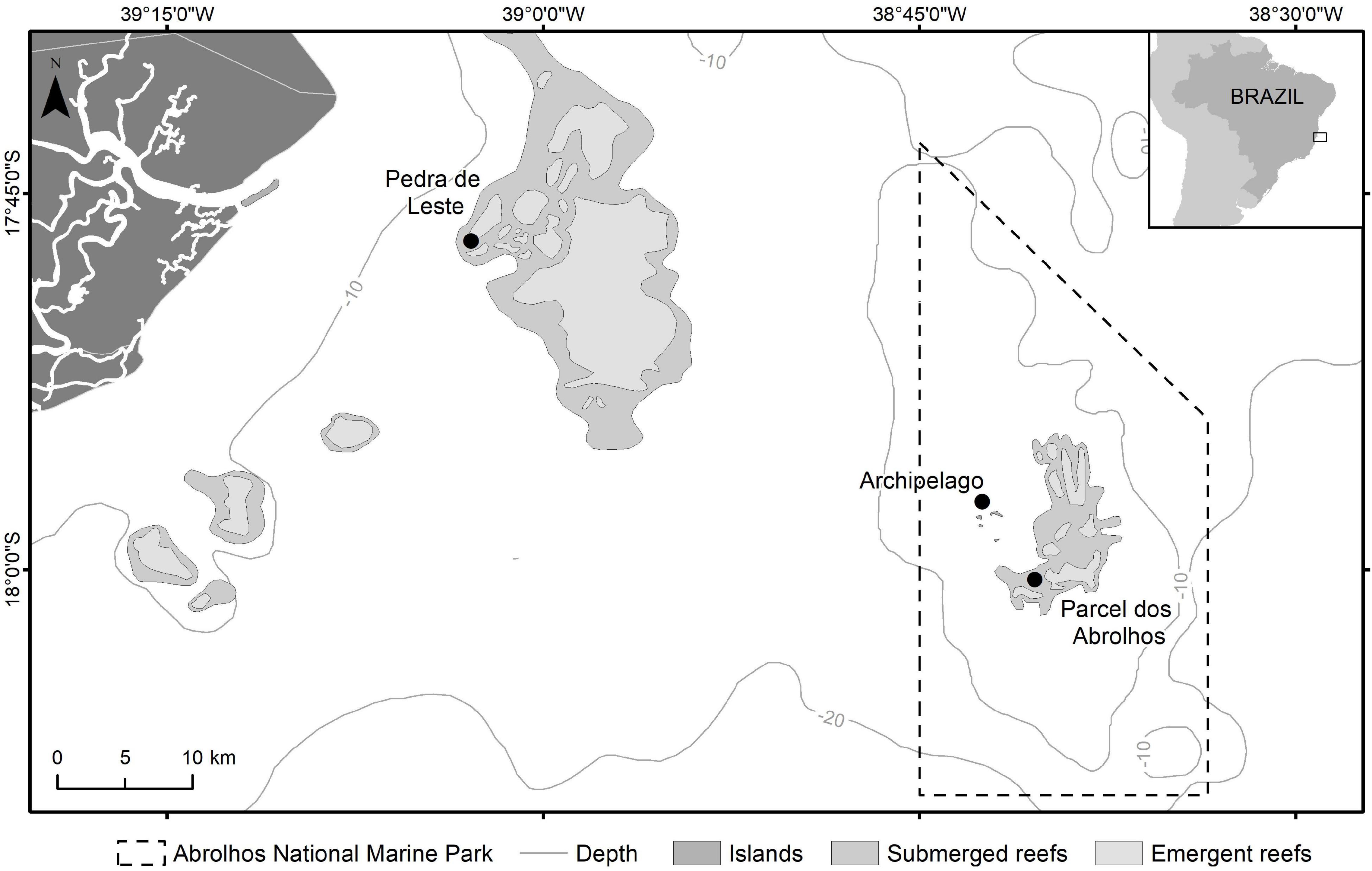
Figure 1. Study area. Symbols refer to Pedra de Leste Reef (PLES), Parcel dos Abrolhos Reef (PAB), and Abrolhos Archipelago. Dashed line = Abrolhos National Marine Park.
Taxonomic Characterization of the Benthic Cyanobacterial Mats
For each BCMs sample, single wide and narrow filaments were selected from the tufts under an inverted microscope (Leica DMi8®) and thoroughly rinsed with a sterile f/2 medium. The selected filaments were photographed with a digital camera for further morphometric analysis using Leica Application Suite X 3.4.1.17822 (Leica®, Mannheim, Germany) software. We measured a minimum of 20 filaments of each morphotype from the same sample, whenever possible, using the AxioVs40 v4.8.2.0 imaging system (Carl Zeiss, Jena, Germany). Single filaments were transferred to ca. 1 μL of medium to 0.5 mL in PCR tubes and digested with 0.5 μL of proteinase K (23 mg ml–1) at 37°C for 1 h, followed by 80°C for 5 min to denature the enzyme. These digested mixtures were further used directly in PCR to amplify the 16S rDNA gene. For cyanobacteria maintained in cultures, DNA was extracted from a cell pellet with 2% CTAB buffer (hexadecyl-trimethyl-ammonium bromide, pH 8.0), followed by chloroform/isoamyl alcohol (24:1, v/v) to take off organic compounds. DNA was precipitated with 95% ethanol and 0.3 M NaOAc, rinsed two times with cold 70% ethanol, and resuspended in 25 μL of TE buffer (10 mM Tris–HCl, 1 mM EDTA, pH 8.0). After DNA extraction, the integrity of the genetic material was checked on a 1% agarose gel. DNA quantification was done in a Nanodrop 1000 spectrophotometer (Thermo Scientific, United States).
Amplification of the 16S rDNA was performed according to Lane (1991) by nested PCR, using external universal 16S rDNA primers (forward 27F 5′-AGAGTTTGATCMTGGCTCAG-3′; reverse 1492R 5′-GGTTACCTTGTTACGACTT-3′) and subsequently internal cyanobacteria-specific primers (forward CYA359F 5′-GGGGAATYTTCCGCAATGGG-3′ and CYA106F 5′-CGGACGGGTGAGTAACGCGTGA-3′; reverse CYA781R 5′-GACTACTGGGGTATCTAATCCCWTT-3′), following previously described procedures (Nübel et al., 1997). Fragments using CYA106F were chosen to be amplified and confirmed by electrophoresis in agarose gel. The PCR products were purified with ExoSAP-IT (Thermo Scientific, United States). Sample sequencing was obtained by the SANGER method (Applied Biosystem 3500). Contigs were assembled with SeqMan Pro v.11.1, and electropherograms were visually checked for peak quality or reading errors and compared among them after alignments, and also to published sequences using the BLASTn tool in GenBank. In this study, 15 cyanobacterial sequences from Abrolhos were produced. A set of sequences from cultures with high similarity to these, sequences from type specimens, and sequences from closely related groups were retrieved to be used in phylogenetic reconstruction (49 sequences in total). Also, an external sequence from E. coli (NC000913) was chosen to root the topology. Sequences were aligned in ClustalW implemented in MEGA X (Kumar et al., 2018) with further manual refinement. Bayesian information criterion (BIC) determined the evolutionary substitution model with the model test, also implemented in MEGA X. Phylogenetic reconstructions were done by Bayesian and maximum likelihood (ML) methods. Mrbayes 3.2 (Ronquist et al., 2012) was used with 1 M of MCMC searching, discarding 10% as burn-in, and web-IQTREE (Trifinopoulos et al., 2016) with the UFBoot, ultrafast bootstrap approximation tool (Hoang et al., 2018), and the SH-aLRT test (Guindon et al., 2010). The Bayesian topology was edited in FigTree (Rambaut, 2018), and ML branch supports were added to the final figure.
Effect of Cell-Free Filtrates on Coral Zooxanthellae
To investigate the allelopathic effects of cyanobacterial chemicals on Symbiodinium sp. CCMR0100 (ITS2 type A4) (viz. Silva-Lima et al., 2015) isolated from the coral Mussismilia braziliensis (Verrill, 1868) in the same reef system, we exposed the photosymbionts to cell-free filtrates of cultured Moorena bouillonii (L. Hoffmann and Demoulin) Engene Tronholm (N = 4) and Adonisia turfae Walter et al. (N = 5), isolated from PABBCM. Strains were grown in an f/2 medium at 26°C, ca. 20 μE m–2s–1, and a 14:10-h light/dark photoperiod. Cyanobacterial cultures were grown for ca. 1 month, depending on the strain, until enough biomass was accumulated. The cells were then removed by filtration through glass microfiber filters (GF/F, 25 mm). Aliquots of 9 ml of filtrate from each cyanobacterial culture were distributed into quadruplicate 20 ml glass vials containing 9 ml of Symbiodinium sp. culture (harvested in the late exponential phase) in an f/2 medium. Controls were made by adding f/2 medium to the symbiont cultures instead of cyanobacteria culture filtrate. Two milliliters of fresh medium were added to each treatment and control vial to avoid nutrient limitation. Each experimental unit had an initial dinoflagellate concentration of ca. 6 × 104 cells ml–1. The experiment was run for 72 h at temperature and irradiance as described above. Cell counts were performed daily either by flow cytometry (BD Accuri C6) or by microscopy using Palmer–Maloney counting chambers. The allelopathic effect was assessed daily by measuring the difference in symbiont cell number between each treatment and its respective control. Negative effects resulted in significantly fewer cells in the treatment exposed to the filtrate than in the control. Positive effects resulted in more cells in the treatment flasks (i.e., greater cell number than the control).
Effect of Benthic Cyanobacterial Mat Extracts on Coral Zooxanthellae
To evaluate the allelopathic properties of secondary metabolites stored intracellularly in comparison with tests using naturally exuded allelochemicals (see previous section), we extracted a pooled biomass of PABBCM using organic solvents. Approximately 300 g (wet weight) of BCMs fronds was frozen in the field, transported to the laboratory, and freeze-dried. At that site, the BCMs is predominantly composed of M. bouillonii, A. turfae, Halomicronema sp., and Leptolyngbya sp. (Table 1). Exhaustive and successive organic extractions were done using ethyl acetate/methanol (1:1), yielding a homogeneous extract. Aliquots of this extract were solubilized in DMSO (dimethyl sulfoxide) and added to vials containing Symbiodinium sp. CCMR0100 to a final concentration proportional to the cyanobacteria biovolume used to prepare the extract, plus three dilutions by a factor of 10, that is, 10, 100, and 1,000 times of initial concentration. Symbiodinium cells were exposed to these extracts following the same experimental design as explained above for the cell-free filtrates. Cells were counted using an automated imaging system (FlowCam, Fluid Imaging Technologies, Inc.) before the experiment and after 48 h of incubation with the extracts to characterize the allelopathic effect of the cyanobacterial chemicals.
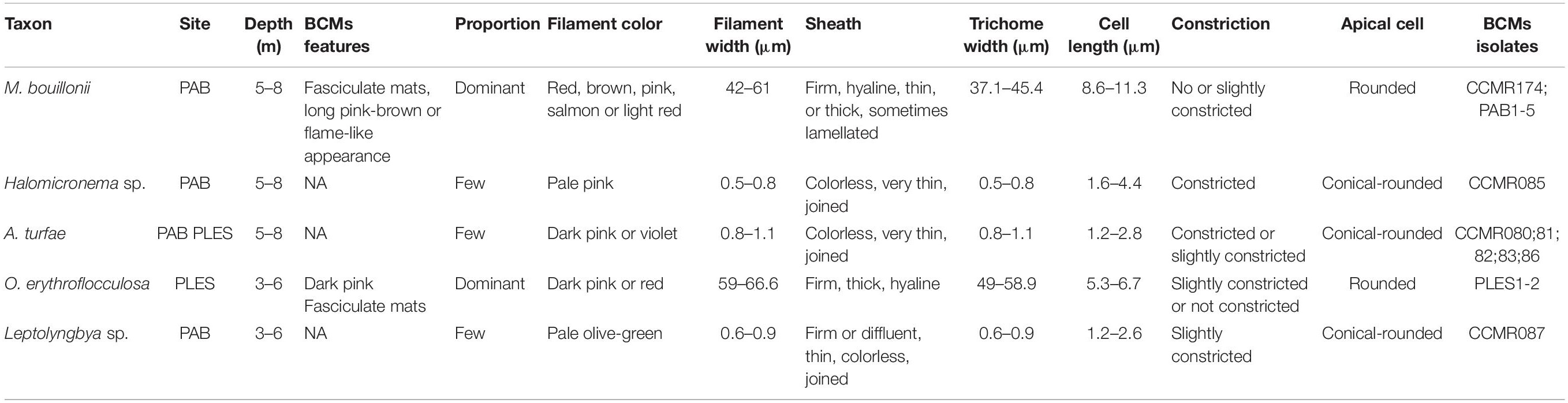
Table 1. Morphological and ecological features of the filaments found in the BCMs of Abrolhos Bank, Brazil.
Benthic Cyanobacterial Mat Susceptibility to Herbivory
To evaluate the susceptibility of PABBCM to consumption and the potential influence of BCMs presence on consumer–prey interactions, we performed susceptibility assays of macroalgae and cyanobacteria in the field. We hypothesized that reef fish would refrain from consuming PABBCM filaments due to their high toxicity and/or low nutritional value. A small piece (5–10 cm) of fresh macroalgae and cyanobacteria was fixed to a 20-cm length 3-strand polypropylene rope with both ends fastened to a fishing weight, according to conventional methods (Hay et al., 1988), with modifications. Halymenia sp. was selected as a palatable macroalgae reference to evaluate BCMs consumption since it was readily consumed in previous tests (the authors, unpublished). Assays recorded grazing in three different treatments: a mix of macroalgae and BCMs, BCMs only, and macroalgae. A GoPRO camera was fastened to the bottom, and all treatments were placed within the camera’s field of view. The experimental design was conceived after running pilot studies in which we repeatedly observed no consumption of stranded BCMs, and full consumption of Halymenia when offered separately to consumers. Due to such strong contrast, we added the mixed treatment to evaluate the grazing behavior toward Halymenia baits in face of unpalatable BCMs of similar color covering the thalli. We performed three sequential trials, using a single replicate for each treatment in every trial (n = 3) and recorded each one for 3 h, independent of the total consumption of any given treatment. The consumer species, number of bites, and time before consumption of the total biomass offered in a single bait were identified and recorded in each video.
Benthic Cyanobacterial Mat Toxicity to Artemia salina
Samples of PABBCM (i.e., Moorena-dominated tufts, see Table 1) were snap-frozen and taken to the laboratory. The toxicity was assessed by exposing Artemia salina nauplii within 24 h after eclosion to crude aqueous extracts of BCMs biomass. The BCMs biomass was harvested on 25-mm fiberglass filters (GF/C), moistened with seawater, and preweighted. Filters containing the biomass were immediately weighted to calculate the wet weight of the cyanobacteria samples. Filters were then transferred to 15-ml polystyrene vials filled with 8 ml of filtered seawater. Cyanobacteria cells were disrupted by three cycles of sonication (1 min each with pulses of 1 s, full power, in an ice bath) interspersed with freeze–thaw at −18°C. Then, the homogenates were filtered on 25-mm fiberglass filters (GF/C) to remove cell debris, 9 ml of AMF was added to 3 ml of the cell-free filtrates, and 6 ml of AMF was added into six 1:1 dilutions of the homogenate. Dilution series were prepared to achieve six concentrations from a max mean (n = 11) concentration of 26, 29 mg f.w. eq. ml–1. Aliquots of 2 ml from each filtrate dilution were added to a 5-mL well plate in triplicates. Each well contained 2 ml of filtered seawater and 20 A. salina nauplii, which were observed after 48 h and scored as alive (motile) or dead (non-motile).
The specific growth rates of Symbiodinium cultures in incubations were calculated based on the cell concentrations, according to Equation 1:
Where N1 and N0 are the cell concentrations at t1 and t0, and t is the time, in days, between sampling occasions.
Statistical Analyses
Allelopathy data were analyzed with ANOVA with growth rate as response variable and treatment as fixed factor, followed by Tukey’s post hoc test. Validity of equal variance, normality, and independence assumptions on the error terms was confirmed using the Shapiro–Wilk test and visual inspection of the plotted residuals. Susceptibility data were analyzed with the Kruskal–Wallis non-parametric test to check for differences between treatments with Halymenia sp. Toxicity was measured as LC50-48 h (median lethal concentration with 95% confidence intervals after 48 h of exposure) calculated by the Trimmed Spearman–Karber method (Hamilton et al., 1977). All statistical analyses were performed in the R environment (R Core Team, 2016).
Results
Taxonomic Characterization of the Benthic Cyanobacterial Mats
The BCMs were predominantly composed of M. bouillonii or O. erythroflocculosa, non-ramified cyanobacteria species enclosed in exopolysaccharide sheaths and exhibit discoid cells arranged in wide trichomes, corresponding to the Oscillatoriales order (Komárek and Anagnostidis, 2005). In addition, Adonisia turfae Walter et al., Leptolyngbya sp., and Halomicronema sp., were also found in the BCMs. However, they belong to the Synechococcales order, which has thin, non-ramified filaments, with cells longer than wide.
The BCMs from PABBCM presented different macroscopic morphologies and cyanobacteria taxonomic compositions than those collected from PLESBCM. In PAB, the BCMs form fasciculate tufts of flame-like appearance, dark-red contours, and a pale inner region (Figure 2A), or as long, pink-brown fasciculate tufts (Figure 2C). These BCMs were predominantly composed of M. bouillonii (Figures 2D,E) and a few entangled filaments of A. turfae (Figure 2H) and Halomicronema sp. (Figure 2I). In BCMs from PLES, O. erythroflocculosa was the predominant taxon (Figure 2F) with Leptolyngbya sp. and A. turfae (Figures 2G,H), as relatively less abundant taxa, forming dark pink fasciculate, horizontally-spreading mats (Figure 2B and Table 1).
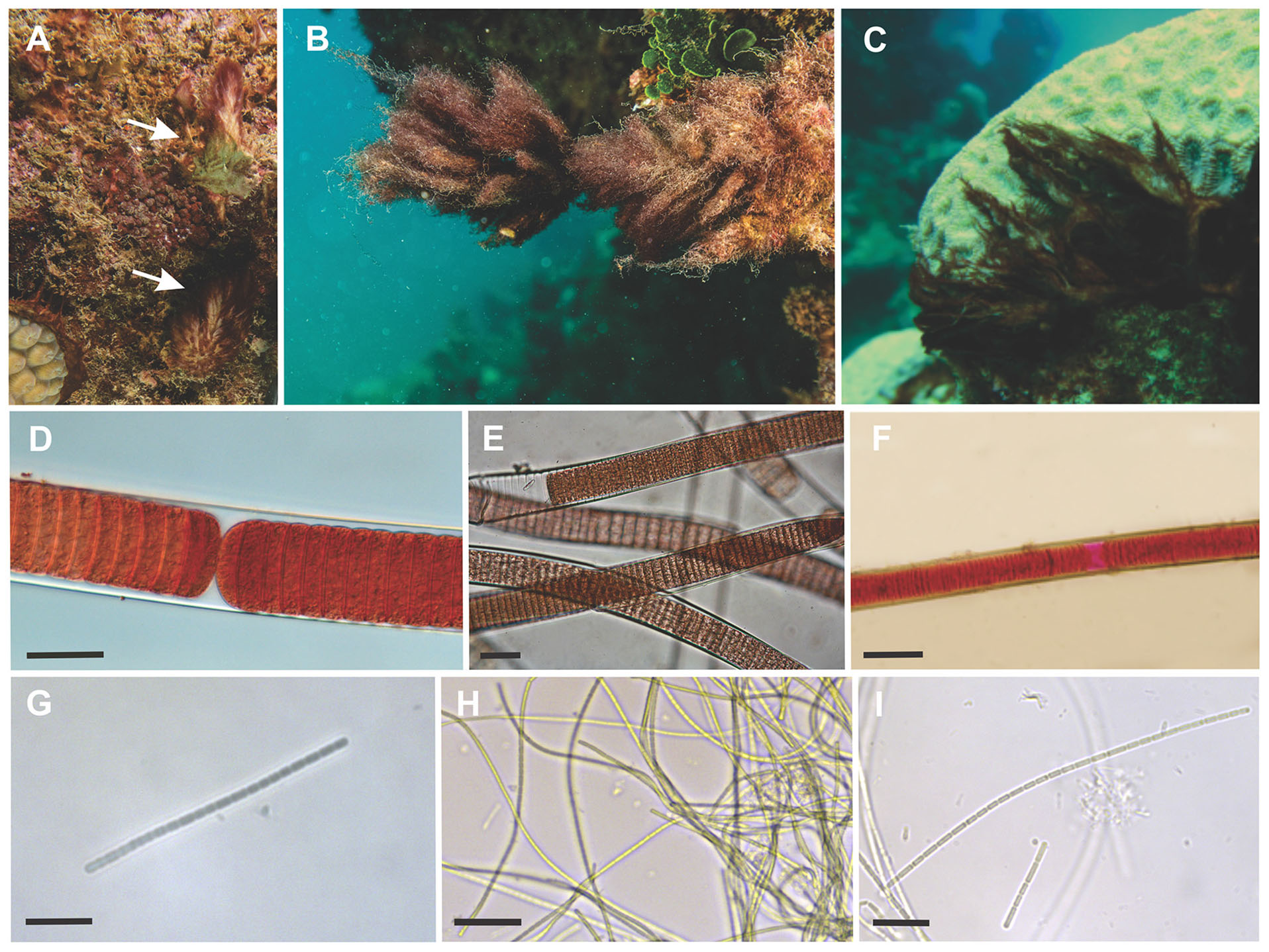
Figure 2. (A–C) Macroscopic aspect of BCMs observed in Abrolhos: (A,C) BCMs from PAB composed of M. bouillonii (predominant), A. Turfae, and Halomicronema sp.; (B,F) BCMs from PLES composed of O. erythroflocculosa (predominant), A. Turfae, and Leptolyngbya sp.; (D–I) General aspect of cyanobacterial filaments associated with BCMs; (D,E) M. bouillonii; (F) O. erythroflocculosa; (G) Leptolyngbya sp.; (H) A. turfae; (I) Halomicronema sp. Scale bars: panel (H) = 25 μm; panel (D) = 30 μm; panel (E) = 50 μm; panel (F) = 100 μm; panels (G,I) = 10 μm.
Phylogenetic inference (Figure 3) grouped the 16S rDNA sequences from broad filaments into two well-supported clades (maximum likelihood, UF-bootstrap percentage/Bayesian analyses, posterior probability), Moorena (97/0.99) and Okeania (99/1.00). The Moorena clade included the isolates CCMR0174, PAB1, 2, 3, 4, and 5, identified as M. bouillonii. The sequences of PLES1 and PLES2, identified as O. erythroflocculosa, clustered together in the Okeania clade (98/0.96). The seven sequences from thin filament isolates were distributed within two clades, one represented by Halomicronema sp. (92/0.95), including the strain CCMR0085, and the other (−/0.81) composed of the remaining six isolates, identified as A. turfae (CCMR0080, CCMR0081, CCMR0082, CCMR0083, and CCMR0086) and Leptolyngbya sp. (CCMR0087).
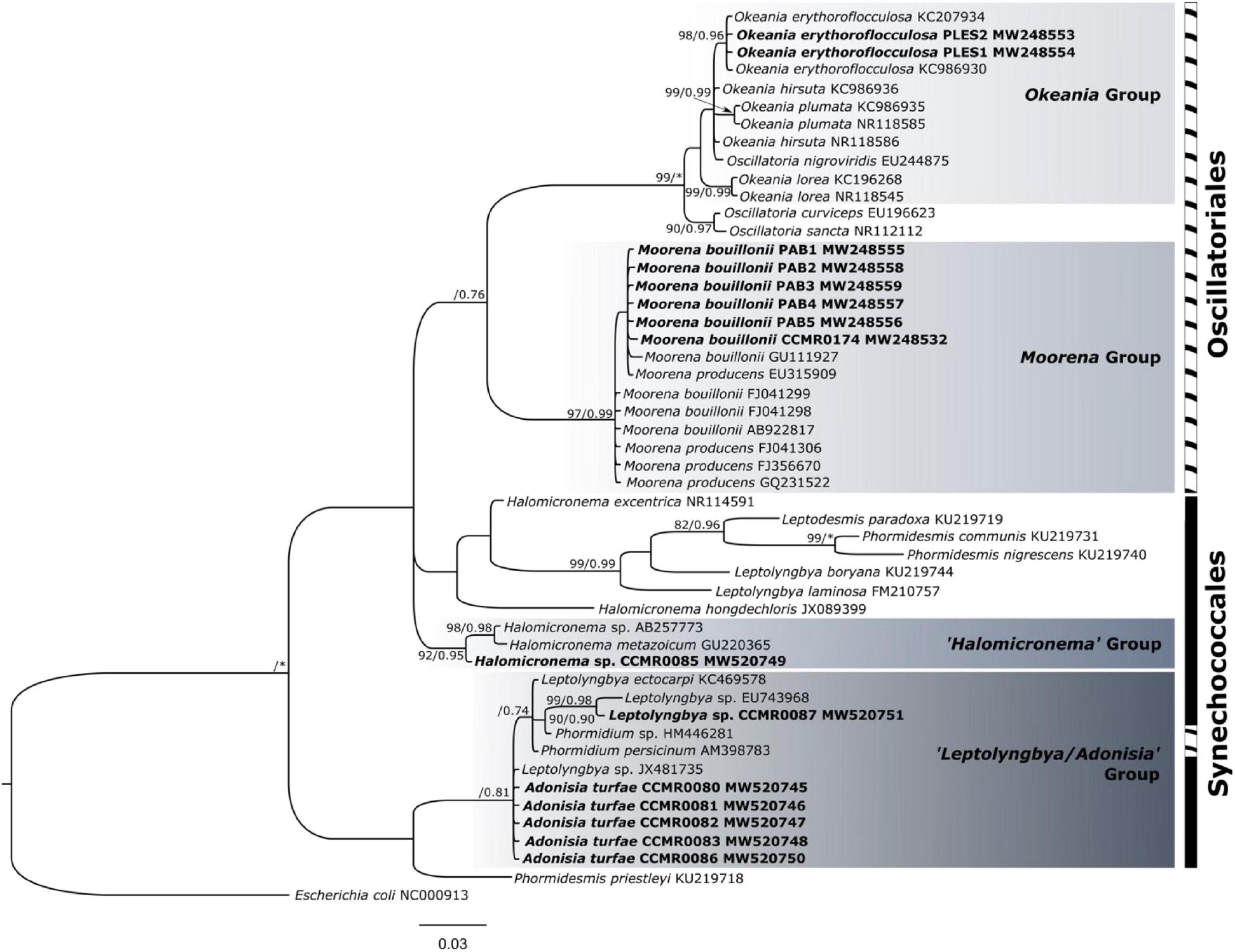
Figure 3. Bayesian tree of the 16s rDNA genomic region of cyanobacteria from Abrolhos Bank (ntax = 49). The inference was constructed with K2P + G as a substitution rate model. Only support values > 70% are indicated. Maximum likelihood bootstrap values are on the left, and Bayesian posterior probabilities are on the right. Asterisks indicate 100% support. Sequences obtained in this study are indicated in bold. Scale bar = 0.03 substitutions per nucleotide position.
The 16S rDNA sequences presented a considerable similarity to others already published, such as PLES1 and PLES2, whose sequences showed 100% similarity with O. erythroflocculosa KC986930 (Engene et al., 2013b). The sequences of PAB1, 2, 3, 4, 5, and CCMR0174 were 99–100% similar to M. bouillonii GU111927 (Engene et al., 2012). Regarding the thin filaments, sequences of strains CCMR0080, CCMR0081, CCMR0082, CCMR0083, and CCMR0086 previously identified as A. turfae had 100% similarity to Leptolyngbya sp. JX481735 (Konstantinou et al., 2019). The sequence of the strain CCMR0085 presented 99% similarity to Halomicronema sp. AB257773 (Yamazaki et al., 2008), which was found in the skeleton of the reef-building coral Goniastrea aspera Verrill, 1866. The sequence of the strain CCMR0087 was 99% similar to Leptolyngbya sp. EU743968, collected on the coral Orbicella annularis Ellis and Solander, 1786, which is one of the species responsible for causing Black band disease (BBD) (Gantar et al., 2009).
Allelopathic Effect of Cyanobacterial Exudates and Benthic Cyanobacterial Mat Extracts
Growth rates of the ex hospite Symbiodinium sp. exposed to cell-free filtrates (i.e., exudates) of the cultured M. bouillonii and A. turfae were significantly lower than those of corresponding controls (Supplementary Table 1 and Figure 4). Cell-free filtrates from cultures of the remaining taxa did not cause significant effects on Symbiodinium. Organic extracts of PABBCM significantly reduced the growth rates of Symbiodinium sp. at natural concentrations after a 48-h incubation (Supplementary Table 1 and Figure 5).
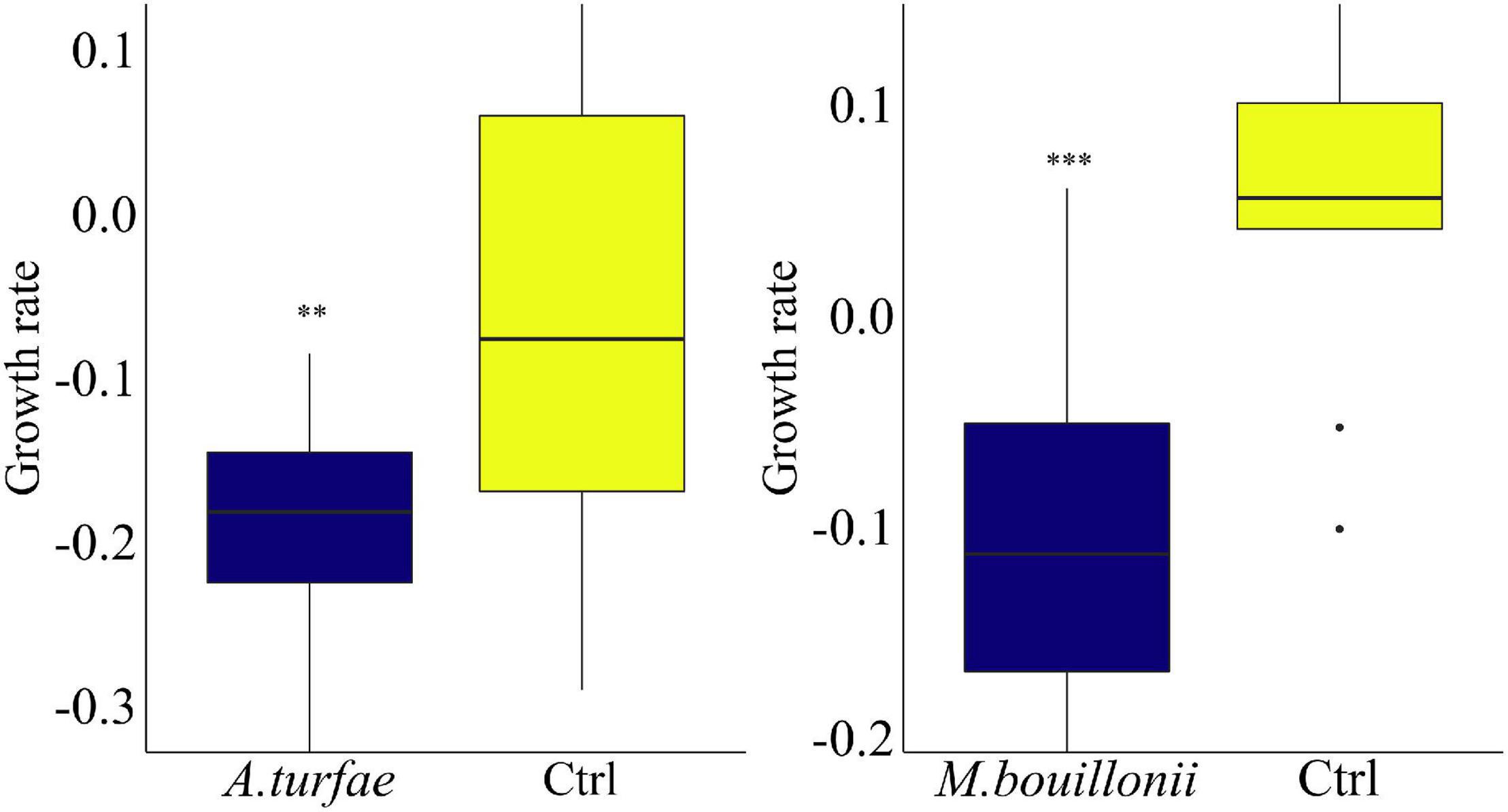
Figure 4. Growth rates of Symbiodinium sp. incubated with cell-free filtrates with M. bouillonii (left) and A. turfae (right). Whiskers: standard error. *Statistical significance compared to controls, p-value < 0.05. Asterisks represent significant p-values (alpha = 5%): p < 0.05*; p < 0.01**; p < 0.001***.
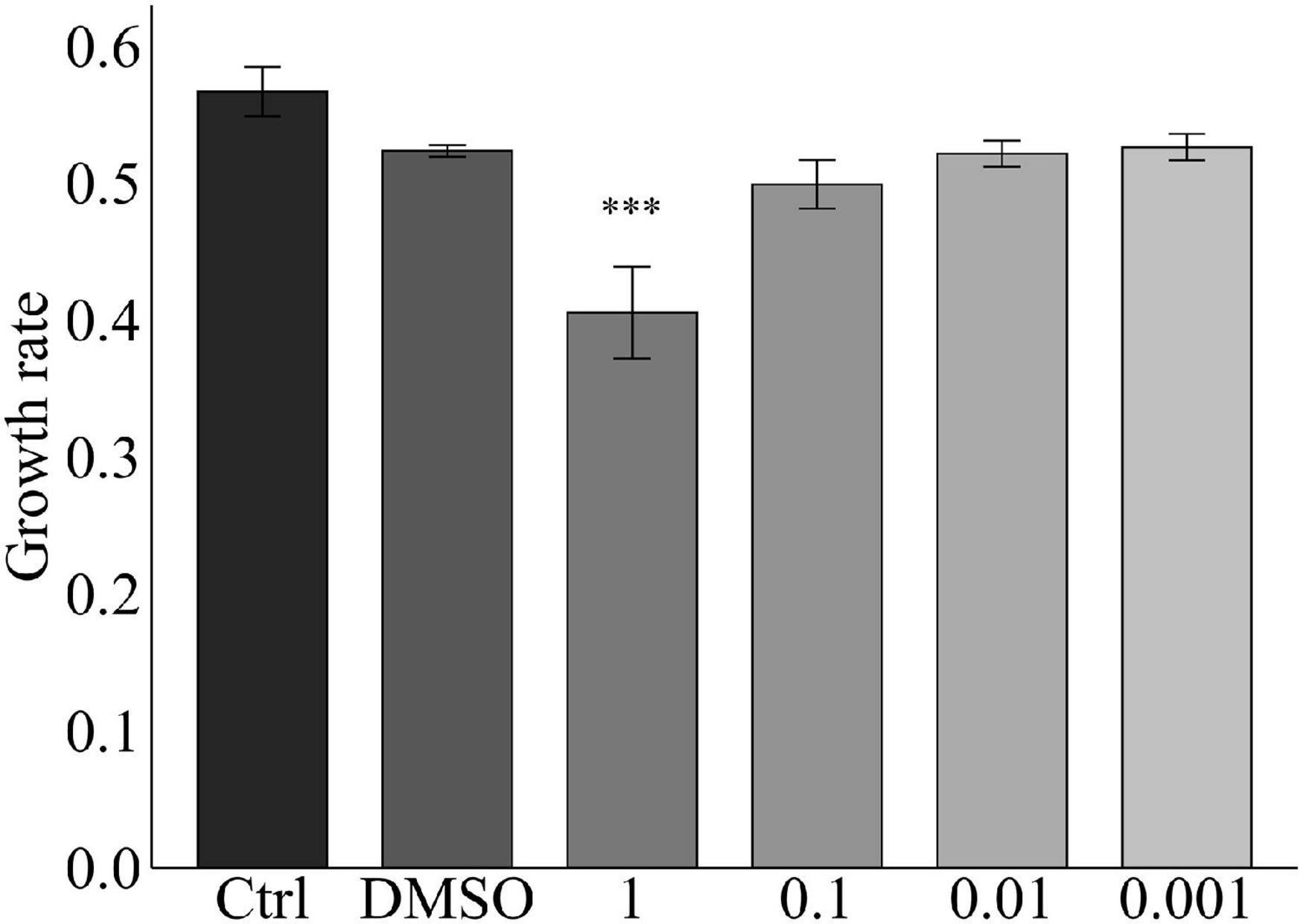
Figure 5. Growth rates, m (d−1), for the incubation with crude extract in the natural concentration (1), diluted 10x (0.1), diluted 100x (0.01) diluted 1000x (0.001). Error bars are standard errors. *Statistical significance compared to controls, p < 0.05. Asterisks represent significant p-values (alpha = 5%): p < 0.001***.
Benthic Cyanobacterial Mat Susceptibility to Herbivory
There was no BCMs consumption by fish in susceptibility field assays performed in PAB (Table 2, Figure 6, and Supplementary Video). Treatments with only macroalgae were consumed first, before the Halymenia pieces in mixed treatments. All BCMs in the experiment remained untouched until the end of the trial. BCMs present in mixed treatments was avoided during delicate targeting of the macroalgae thalli. Halymenia sp. in macroalgae-only and mixed treatments were consumed in all trials compared with no-consumption of BCMs-only treatments (Table 2). There was no difference in the total number of bites between macroalgae treatments (chi-square = 0.2, df = 1, p > 0.05). The angelfish Pomacanthus paru and the surgeon fish Acanthurus bahianus were the primary consumers. They had distinct strategies to avoid BCMs while grazing the macroalgae in mixed treatments. Pomacanthus paru have a protruding feeding apparatus that allowed them to break the bait and selectively suck the pieces of interest floating in the water. The surgeon fishes have a smaller mouth and repeatedly bite the Halymenia pieces, taking out small fragments. Other eventual consumers were the juvenile of the parrotfish Sparisoma axillare, the yellowtail snapper Ocyurus chrysurus, and the damselfish Stegastes fuscus, which consumed Halymenia only in the mixed treatment.
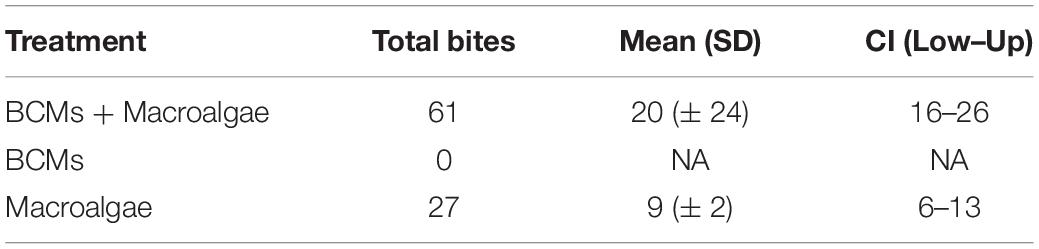
Table 2. Number of bites in the susceptibility to herbivory tests of BCMs and macroalgae in single and mixed treatments.
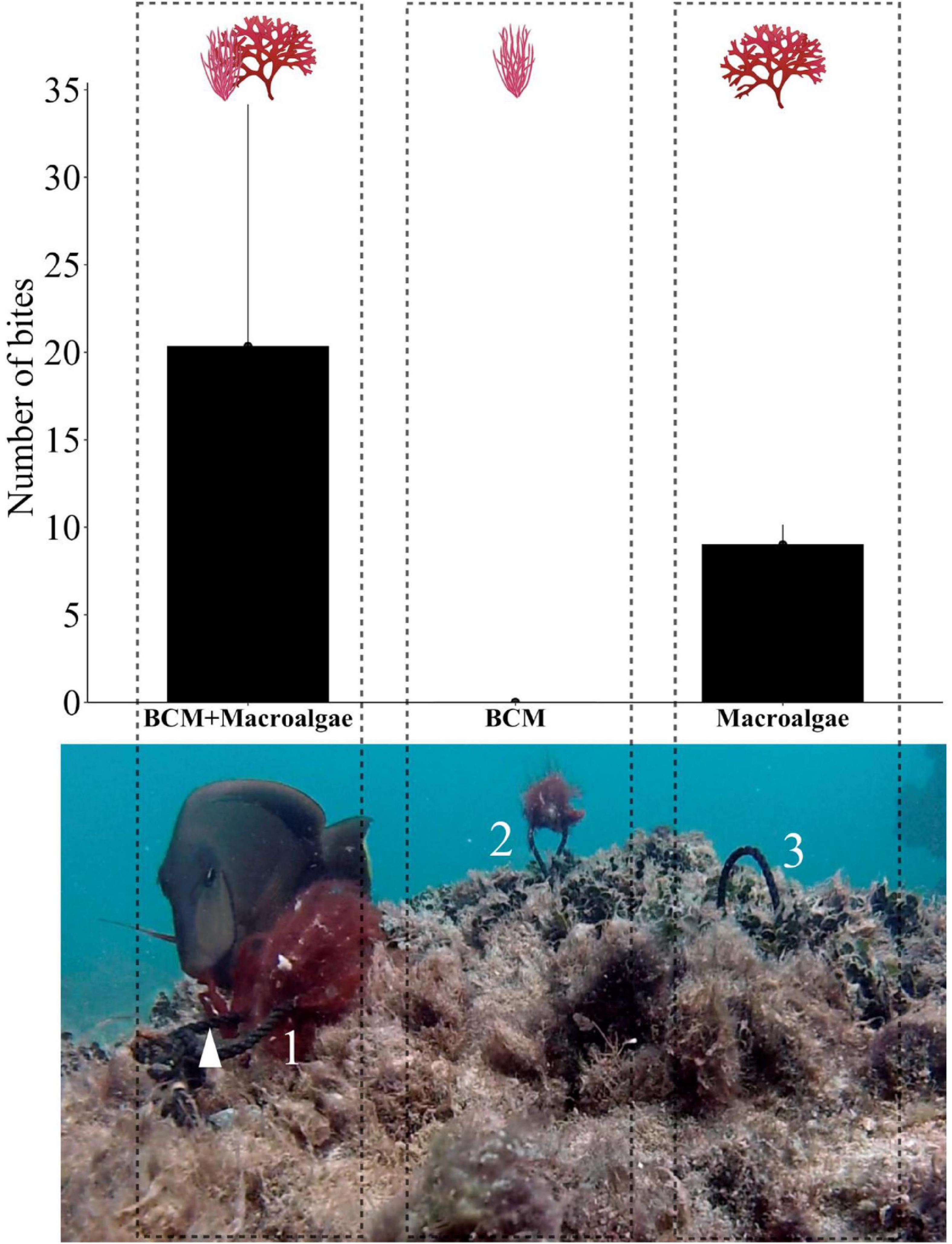
Figure 6. Susceptibility tests of BCMs and palatable macroalgae in single and mixed treatments. Bar plot of the mean number of bites in each treatment (N = 3), whiskers: standard error. Photo: Acanthurus bahianus biting macroalgae in the mixed treatment (BCMs + macroalgae) during the course of one of the trials when consumption had already taken place, hence the empty rope in the macroalgae-only treatment in 3. The arrow points to the macroalgae thalli. Numbers refer to treatments within each dashed line rectangle: 1 = mixed; 2 = BCMs; 3 = macroalgae. Snapshot from wide-angle footage. Size distortions causing close objects to appear larger than their actual size (please see Supplementary Video).
Benthic Cyanobacterial Mat Toxicity
Three out of 11 PABBCM samples caused more than 50% mortality to A. salina nauplii (Supplementary Figure 1). The LC50-48 h for these three samples was 9.8 mg w.w. eq. ml–1 (95% CI: 8.3–11.6 mg w.w. eq. ml–1), 9.3 mg w.w. eq. ml–1 (95% CI: 6.8–12.6 mg w.w. eq. ml–1), and 3.2 mg w.w. eq. ml–1 (95% CI: 2.5–4.1 mg w.w. eq. mL–1). The other eight samples had LC50-48 h values higher than the maximum concentration used in tests (i.e., > 13.7–32.6 mg w.w. eq. ml–1).
Discussion
Morphological and Molecular Characterization of the Cyanobacteria
Morphological and genetic analyses of cyanobacteria in the BCMs unveiled two species, M. bouillonii and O. erythroflocculosa, hitherto unknown to the Abrolhos reefs and the tropical Southwestern Atlantic (SWA). Species belonging to both genera have been reported in Florida, the Caribbean, and the Pacific regions (Engene et al., 2012). Okeania erythroflocculosa has been detected as an abundant component of the BCMs worldwide (Engene et al., 2012, 2013a). Despite the high gene abundance of Oscillatoriales cyanobacteria previously reported for Abrolhos cyanobacterial mats (Walter et al., 2016), the species have not been identified to date. The species-level identification of cyanobacteria with thin filaments requires diacritic features that are difficult to observe. Their genotypic diversity can be high within the different phenotypes, characterizing them as cryptotaxa (Engene et al., 2010; Caires et al., 2018). Here, the BCMs cryptotaxa were identified as A. turfae, previously reported (Walter et al., 2020), and two strains closely related to Leptolyngbya sp. and Halomicronema sp. The 16S rDNA sequences of the two latter cyanobacteria showed great similarities with the sequences found in the skeleton of scleractinian corals (Yamazaki et al., 2008; Gantar et al., 2009).
Allelopathic Effect of Cyanobacterial Exudates and Benthic Cyanobacterial Mat Extracts
Laboratory experiments evidenced allelopathic effects of chemical substances from cultured strains of cyanobacteria (M. bouillonii and A. turfae), expressed by inhibiting the growth of the ex-hospite Symbiodinium sp. isolated from M. braziliensis. This negative effect on Symbiodinium was also evidenced in incubations with organic extracts from BCMs collected in Abrolhos. Similar incubation bioassays reported a decrease in the number of Chlorella vulgaris cells after exposure to freshwater cyanobacteria exudates (Leão et al., 2009). Growth inhibition potential was also reported by the metabolites hapalindole from Fischerella sp. and calothrixin A from Calothrix sp., which affected growth and inhibited the genetic replication of prokaryotic and eukaryotic cells in bioassays (Doan et al., 2000). Also, exudates from the Baltic Sea cyanobacteria Nodularia spumigena and Anabaena sp. exhibited antagonistic effects on cryptophytes (Suikkanen et al., 2004). Cyanobacterin, a metabolite from Scytonema hofmanni, and fischerelin A, from Fischerella muscicula, were reported to affect the growth of photoautotrophs by inhibition of photosystem II (Gleason, 1990). Cyanobacteria extracts also exerted allelopathic effects in the field, manifested through a decreased pigment concentration of endosymbiotic dinoflagellates and the consequent bleaching of the Porites coral (Puyana et al., 2019).
The decrease in growth rate of Symbiodinium sp. cultures supports previous evidence of an antagonistic relationship between BCMs and reef builders in Abrolhos (Ribeiro et al., 2018). The effects shown here are likely involved in interactions at the BCMs-coral boundary, which accumulate and transfer metabolites (Wangpraseurt et al., 2012). The increase in cyanobacterial biomass observed during blooms could result in thicker diffusion boundary layers that enhance transfer mechanisms and have antagonistic effects on coral photosynthesis and respiration (Jorissen et al., 2016). Such enhanced mass transfer through boundary layers and tissues (Patterson, 1992) could expose Symbiodinium to combined stress due to molecules produced by heterotrophs under anoxic conditions within the BCMs, and exuded allelochemicals diffused during blooms (Brocke et al., 2015). An increase in BCMs cover would also be a threat to coral recruitment success (Ritson-Williams et al., 2020). Additionally, allelopathic effects during episodic cyanobacteria blooms can be further aggravated after acute disturbances such as heatwaves (Teixeira et al., 2019) when both the host and photosymbionts are physiologically impaired due to oxidative stress (Oakley and Davy, 2018).
Benthic Cyanobacterial Mat Toxicity and Susceptibility to Consumers
The mortality of brine shrimp after exposure to aqueous extracts of Abrolhos BCMs at natural cyanobacterial densities unveils the potential toxic properties of these benthic structures. Variable toxicity within the same BCMs that we observed (i.e., 3 out of 11 samples caused >50% mortality) should not imply a lack of chemical defense by cyanobacteria, as spatial and seasonal variability of chemical defenses against herbivores is expected due to metabolic responses to changes in the environment (Puglisi et al., 2019). The high spatial variability of Abrolhos reefs likely contributes to a context dependency of BCMs toxicity (Ribeiro et al., 2018; Teixeira et al., 2021). The large biomass of morbid sea slugs, Stilocheylus striatus, observed during cyanobacterial blooms in Abrolhos reefs (Ribeiro et al., 2017) is in line with the toxic potential of Abrolhos BCMs observed here. This opistobranch is a specialist consumer of BCMs and has been shown to sequester toxic compounds from the cyanobacteria, becoming unpalatable to predators (Pennings and Paul, 1993).
Our results indicate that O. erythroflocculosa, M. bouillonii, Leptolyngbya sp., and A. turfae are potential producers of toxic compounds within Abrolhos BCMs. Moorena, Okeania, and Lyngbya species have been reported to produce more than 40% of the cyanobacteria secondary metabolites described to date (Engene et al., 2011; Dittmann et al., 2015), many of which mediate interactions with grazers (Thacker et al., 1997) and competitors (Kuffner et al., 2006; Titlyanov and Titlyanova, 2009). The Leptolyngbya consortium produces cyanotoxins and is associated with BBD in corals (Gantar et al., 2009).
Field assays of susceptibility to herbivory showed that BCMs remained untouched by consumers, either in treatments with BCMs alone or mixed with Halymenia sp., a more palatable macroalgae. Even though the genus Halymenia produces ecologically relevant secondary metabolites (Malik et al., 2020), the presence of these types of chemicals did not seem to influence algal consumption by surgeon fish elsewhere (Wylie and Paul, 1988). Among consumers, both A. bahianus and S. axillare are detritivorous but consume macroalgae and have a scraper feeding mode. These species display high foraging plasticity and can explore different food items, including cyanobacteria, and also calcareous and sheet-like macroalgae (Ferreira and Gonçalves, 2006; Mendes et al., 2018). The angelfish P. paru has a varied, omnivorous diet, encompassing sessile invertebrates and several groups of macroalgae (Batista et al., 2012). These species readily consumed the macroalgae treatments in every trial, with just a few bites given their relatively large size and protruding feeding apparatus. Although reef fish may consume benthic cyanobacteria (Ferreira and Gonçalves, 2006; Cissell et al., 2019), our exploratory results suggest avoidance of BCMs by reef fishes in PAB. The limited sample size of our feeding trials requires caution in interpreting such results and making generalizations of this pattern to BCMs in general. Given that a significant discrepancy between feeding selectivity and nutrient content of cyanobacteria was reported for reef herbivores (Mendes et al., 2018), BCMs consumption and deterrence seem to be a trade-off between its composition, growth form, and chemistry (Cissell and McCoy, 2021; Ford et al., 2021). The bottom-up effects on reef herbivores remain elusive as contrasting evidence has recently emerged (Cissell et al., 2019; Ford et al., 2021). Consumers with different feeding strategies and nutritional demands may then target high nutritious cyanobacteria (Clements and Choat, 2018), and also associated non-cyanobacterial components (Cissell and McCoy, 2021), or avoid less nutritious (Nagarkar et al., 2004) and toxic cyanobacteria (Ford et al., 2021).
Phase shifts from coral dominated to opportunistic BCMs taxa have been reported worldwide (de Bakker et al., 2017; Ford et al., 2018), and such expansion disturbs reef trophic dynamics (Ford et al., 2021) by reducing energy flow and ultimately promotes a trophic simplification of the ecosystem (Ullah et al., 2018). This trophic disruption brings reef communities to alternative unstable states (Teixeira et al., 2021), because cyanobacteria are highly efficient in nutrient uptake and grow fast (den Haan et al., 2016; Ford et al., 2018), boosting their capacity to outcompete other benthic species and change community structure (e.g., de Bakker et al., 2017; Ribeiro et al., 2018; Teixeira et al., 2021). In the Abrolhos reef complex, BCMs can be structured by chemically defended species of cyanobacteria that display allelopathic, grazing deterrent, and toxic properties. These ecologically significant, albeit antagonistic, interactions with reef organisms could promote deleterious cascading effects on the ecosystem and contribute to the homogenization of reef communities. The disturbance of such trophic relations is probably amplified during episodic blooms of benthic cyanobacteria.
Data Availability Statement
The datasets presented in this study can be found in online repositories. The names of the repository/repositories and accession number(s) can be found below: https://www.ncbi.nlm.nih.gov/genbank/, mw248552 to 248559 and mw520745 to 520751.
Author Contributions
FR designed the study and experiments, performed fieldwork, analyzed data, wrote the manuscript, and revised the manuscript. TC performed taxonomical work, analyzed data, and wrote the manuscript. LV performed phylogenetic and molecular analyses and wrote the manuscript. MS and PH contributed in genetic sequencing and microscopy. GF and AC performed microalgae cultures and incubation experiments. GP-F analyzed macroalgae and cyanobacteria collection and revised the manuscript. RM contributed with study design and sampling, materials, resources and revised the manuscript. RP and PS designed the study and experiments and wrote the manuscript. All authors contributed to the article and approved the submitted version.
Funding
This work has been supported by the following Brazilian research agencies: CNPq (grants 30755/82019/3 and 430819/2018-8 to PS), and the Rio de Janeiro Research Foundation (FAPERJ) (grant E-26/202.911/2018). Partial funding was also provided by the Long-Term Ecological Research Program (PELD-Abrolhos/CNPq) and Fundação RENOVA/FEST.
Conflict of Interest
The authors declare that the research was conducted in the absence of any commercial or financial relationships that could be construed as a potential conflict of interest.
Publisher’s Note
All claims expressed in this article are solely those of the authors and do not necessarily represent those of their affiliated organizations, or those of the publisher, the editors and the reviewers. Any product that may be evaluated in this article, or claim that may be made by its manufacturer, is not guaranteed or endorsed by the publisher.
Acknowledgments
We thank the three reviewers for their insightful comments and suggestions that helped us to improve the manuscript. PS, RP, and RM would like to thank the Brazilian National Council for Scientific and Technological Development (CNPq) for Research Productivity fellowships. FR would like to thank “Coordenação de Aperfeiçoamento de Pessoal de Nível Superior” (CAPES) for DSc. fellowship. We would like to thank Prof. Inácio Domingos from UFRJ for microscopy support.
Supplementary Material
The Supplementary Material for this article can be found online at: https://www.frontiersin.org/articles/10.3389/fmars.2021.790277/full#supplementary-material
Supplementary Figure 1 | Concentration–response curves after 48 h of incubation of Artemia salina nauplii exposed to cell-free filtrates of PABBCM. Response expressed as average% mortality in each exposure concentration. LC50-48 h is shown for the three samples where this end point was calculable by the Trimmed Spearman–Karber method. The other samples have LC50-48 h higher than the highest exposure concentration. Whiskers: standard error.
Supplementary Table 1 | Summary of ANOVA type II results for comparisons between incubation treatments and Tukey’s HSD post hoc test to verify differences in Symbiodinium growth index μ (d-1), between treatments and respective controls (without exudates).
Supplementary Video 1 | Susceptibility of benthic cyanobacteria to herbivory in field experiments.
References
Ainsworth, T. D., Hoegh-Guldberg, O., Heron, S. F., Skirving, W. J., and Leggat, W. (2008). Early cellular changes are indicators of pre-bleaching thermal stress in the coral host. J. Exp. Mar. Biol. Ecol. 364, 63–71. doi: 10.1016/j.jembe.2008.06.032
Albert, S., O’Neil, J. M., Udy, J. W., Ahern, K. S., O’Sullivan, C. M., and Dennison, W. C. (2005). Blooms of the cyanobacterium Lyngbya majuscula in coastal Queensland, Australia: disparate sites, common factors. Mar. Poll. Bull. 51, 428–437. doi: 10.1016/j.marpolbul.2004.10.016
Batista, D., Muricy, G. R. D. S., Andréa, B. R., and Villaça, R. C. (2012). High intraspecific variation in the diet of the french angelfish Pomacanthus paru in the south-western Atlantic. Braz. J. Oceanogr. 60, 449–454.
Brocke, H. J., Polerecky, L., De Beer, D., Weber, M., Claudet, J., and Nugues, M. M. (2015). Organic matter degradation drives benthic cyanobacterial mat abundance on Caribbean coral reefs. PLoS One 10:e0125445. doi: 10.1371/journal.pone.0125445
Brumley, D., Spencer, K. A., Gunasekera, S. P., Sauvage, T., Biggs, J., Paul, V. J., et al. (2018). Isolation and characterization of anaephenes A-C, alkylphenols from a filamentous cyanobacterium (Hormoscilla sp., Oscillatoriales). J. Nat. Prod. 81, 2716–2721. doi: 10.1021/acs.jnatprod.8b00650
Caires, T. A., Lyra, G. M., Hentschke, G. S., Pedrini, A. G., Sant’Anna, C. L., and Nunes, J. M. C. (2018). Neolyngbya gen. nov. (Cyanobacteria): a new benthic marine taxon widely distributed on the Brazilian Coast. Mol. Phylogenet. Evol. 120, 196–211. doi: 10.1016/j.ympev.2017.12.009
Capper, A., and Paul, V. J. (2008). Grazer interactions with four species of Lyngbya in southeast Florida. Harmful Algae 7, 717–728. doi: 10.1016/j.hal.2008.02.004
Capper, A., Erickson, A. A., Ritson-Williams, R., Becerro, M. A., Arthur, K. A., and Paul, V. J. (2016). Palatability and chemical defences of benthic cyanobacteria to a suite of herbivores. J. Exp. Mar. Biol. Ecol. 474, 100–108.
Carroll, A. R., Copp, B. R., Davis, R. A., Keyzers, R. A., and Prinsep, M. R. (2021). Marine natural products. Nat. Prod. Rep. 38, 362–413. doi: 10.1039/D0NP00089B
Chadwick, N. E., and Morrow, K. M. (2011). “Competition among sessile organisms on coral reefs,” in Coral Reefs: An Ecosystem in Transition, eds Z. Dubinsky and N. Stambler (Heidelberg: Springer).
Charpy, L., Alliod, R., Rodier, M., and Golubic, S. (2007). Benthic nitrogen fixation in the SW New Caledonia lagoon. Aquat. Microb. Ecol. 47, 73–81. doi: 10.3354/ame047073
Cissell, E. C., and McCoy, S. J. (2021). Shotgun metagenomic sequencing reveals the full taxonomic, trophic, and functional diversity of a coral reef benthic cyanobacterial mat from Bonaire, Caribbean Netherlands. Sci. Total Environ. 755:142719. doi: 10.1016/j.scitotenv.2020.142719
Cissell, E. C., Manning, J. C., and McCoy, S. J. (2019). Consumption of benthic cyanobacterial mats on a Caribbean coral reef. Sci. Rep. 9:12693.
Clements, K. D., and Choat, J. H. (2018). “Nutritional ecology of parrotfishes (Scarinae, Labridae),” in The Biology and Ecology of Parrotfishes, eds A. S. Hoey and R. M. Bonaldo (Boca Raton, FL: CRC).
Connell, S. D., Foster, M. S., and Airoldi, L. (2014). What are algal turfs? Towards a better description of turfs. Mar. Ecol. Prog. Ser. 495, 299–307. doi: 10.3354/meps10513
de Bakker, D. M., Van Duyl, F. C., Bak, R. P., Nugues, M. M., Nieuwland, G., and Meesters, E. H. (2017). 40 Years of benthic community change on the Caribbean reefs of Curaçao and Bonaire: the rise of slimy cyanobacterial mats. Coral Reefs 36, 355–367. doi: 10.1007/s00338-016-1534-1539
den Haan, J., Huisman, J., Brocke, H. J., Goehlich, H., Latijnhouwers, K. R., Van Heeringen, S., et al. (2016). Nitrogen and phosphorus uptake rates of different species from a coral reef community after a nutrient pulse. Sci. Rep. 6: 28821.
Dittmann, E., Gugger, M., Sivonen, K., and Fewer, D. P. (2015). Natural product biosynthetic diversity and comparative genomics of the cyanobacteria. Trends Microbiol. 23, 642–652. doi: 10.1016/j.tim.2015.07.008
Doan, N. T., Rickards, R. W., Rothschild, J. M., and Smith, G. D. (2000). Allelopathic actions of the alkaloid 12-epi-hapalindole E isonitrile and calothrixin A from cyanobacteria of the genera Fischerella and Calothrix. J. Appl. Phycol. 12, 409–416. doi: 10.1023/A:1008170007044
Echenique-Subiabre, I., Villeneuve, A., Golubic, S., Turquet, J., Humbert, J.-F., and Gugger, M. (2015). Influence of local and global environmental parameters on the composition of cyanobacterial mats in a tropical lagoon. Microb. Ecol. 69, 234–244. doi: 10.1007/s00248-014-0496-490
Engene, N., Choi, H., Esquenazi, E., Rottacker, E. C., Ellisman, M. H., Dorrestein, P. C., et al. (2011). Underestimated biodiversity as a major explanation for the perceived rich secondary metabolite capacity of the cyanobacterial genus Lyngbya. Environ. Microbiol. 13, 1601–1610. doi: 10.1111/j.1462-2920.2011.02472.x
Engene, N., Coates, R. C., and Gerwick, W. H. (2010). 16S rRNA gene heterogeneity in the filamentous marine cyanobacterial genus Lyngbya. J. Phycol. 46, 591–601. doi: 10.1111/j.1529-8817.2010.00840.x
Engene, N., Gunasekera, S. P., Gerwick, W. H., and Paul, V. J. (2013a). Phylogenetic inferences reveal a large extent of novel biodiversity in chemically rich tropical marine cyanobacteria. Appl. Environ. Microb. 79, 1882–1888. doi: 10.1128/AEM.03793-3712
Engene, N., Paul, V. J., Byrum, T., Gerwick, W. H., Thor, A., and Ellisman, M. H. (2013b). Five chemically rich species of tropical marine cyanobacteria of the genus Okeania gen. nov. (Oscillatoriales, Cyanoprokaryota). J. Phycol. 49, 1095–1106. doi: 10.1111/jpy.12115
Engene, N., Rottacker, E. C., Kaštovskı, J., Byrum, T., Choi, H., Ellisman, M. H., et al. (2012). Moorea producens gen. nov., sp. nov. and Moorea bouillonii comb. nov., tropical marine cyanobacteria rich in bioactive secondary metabolites. Int. J. Syst. Evol. Microbiol. 62, 1171–1178. doi: 10.1099/ijs.0.033761-33760
Engene, N., Tronholm, A., and Paul, V. J. (2018). Uncovering cryptic diversity of Lyngbya: the new tropical marine cyanobacterial genus Dapis (Oscillatoriales). J. Phycol. 54, 435–446. doi: 10.1111/jpy.12752
Ferreira, C. E. L., and Gonçalves, J. E. A. (2006). Community structure and diet of roving herbivorous reef fishes in the Abrolhos Archipelago, south-western Atlantic. J. Fish Biol. 69, 1533–1551. doi: 10.1111/j.1095-8649.2006.01220.x
Fistarol, G. O., Hargreaves, P. I., Walter, J. M., Viana, T. V., Gomes, P. D. F., Lourenço, C. B., et al. (2018). Rapid isolation of culturable microalgae from a tropical shallow lake system. J. Appl. Phycol. 30, 1807–1819.
Ford, A. K., Bejarano, S., Nugues, M. M., Visser, P. M., Albert, S., and Ferse, S. C. A. (2018). Reefs under Siege - the rise, putative drivers, and consequences of benthic cyanobacterial mats. Front. Mar. Sci. 5:18. doi: 10.3389/fmars.2018.00018
Ford, A. K., Visser, P. M., van Herk, M. J., Jongepier, E., and Bonito, V. (2021). First insights into the impacts of benthic cyanobacterial mats on fish herbivory functions on a nearshore coral reef. Sci. Rep. 11:7147. doi: 10.1038/s41598-021-84016-z
Gantar, M., Sekar, R., and Richardson, L. L. (2009). Cyanotoxins from black band disease of corals and from other coral reef environments. Microb. Ecol. 58, 856–864. doi: 10.1007/s00248-009-9540-x
Gleason, F. K. (1990). The natural herbicide, cyanobacterin, specifically disrupts thylakoid membrane structure in Euglena gracilis strain. FEMS Microbiol. Lett. 68, 77–81.
Groendahl, S., and Fink, P. (2017). High dietary quality of non-toxic cyanobacteria for a benthic grazer and its implications for the control of cyanobacterial biofilms. BMC Ecol. 17:20. doi: 10.1186/s12898-017-0130-3
Guillard, R. R. L. (1975). “Culture of phytoplankton for feeding marine invertebrates,” in Culture of Marine Invertebrate Animals, eds W. L. Smith and M. H. Chanley (New York, NY: Plenum Press), 26–60.
Guindon, S., Dufayard, J. F., Lefort, V., Anisimova, M., Hordijk, W., and Gascuel, O. (2010). New algorithms and methods to estimate maximum-likelihood phylogenies: assessing the performance of PhyML 3.0. Syst. Biol. 59, 307–332. doi: 10.1093/sysbio/syq010
Hamilton, M. A., Russo, R. C., and Thurston, R. V. (1977). Trimmed Spearman-Karber method for estimating median lethal concentrations in toxicity bioassays. Enviro. Sci. Tech. 11, 714–719.
Hay, M. E., Paul, V. J., Lewis, S. M., Gustafson, K., Tucker, J., and Trindell, R. N. (1988). Can tropical seaweeds reduce herbivory by growing at night? diel patterns of growth, nitrogen content, herbivory, and chemical versus morphological defenses. Oecologia 75, 233–245. doi: 10.1007/BF00378604
Hoang, D. T., Chernomor, O., Von Haeseler, A., Minh, B. Q., and Vinh, L. S. (2018). UFBoot2: improving the ultrafast bootstrap approximation. Mol. Biol. Evol. 35, 518–522. doi: 10.1093/molbev/msx281
Jorissen, H., Skinner, C., Osinga, R., Beer, D., and Nugues, M. M. (2016). Evidence for water-mediated mechanisms in coral - algal interactions. Proc. Biol. Sci. 283, 1–10. doi: 10.1098/rspb.2016.1137
Komárek, J., and Anagnostidis, K. (2005). “Cyanoprokaryota-2. Teil/2nd part: Oscillatoriales,” in Süsswasserflora von Mitteleuropa 19/778 2, eds B. Büdel, G. Gärtner, L. Krienitz, and M. Schagerl (Heidelberg: Elsevier/Spektrum).
Konstantinou, D., Voultsiadou, E., Panteris, E., Zervou, S.-K., Hiskia, A., and Gkelis, S. (2019). Leptothoe, a new genus of marine cyanobacteria (Synechococcales) and three new species associated with sponges from the Aegean Sea. J. Phycol. 55, 882–897. doi: 10.1111/jpy.12866
Kuffner, I. B., Walters, L. J., Becerro, M., Paul, V. J., Ritson-Williams, R., and Beach, K. (2006). Inhibition of coral recruitment by macroalgae and cyanobacteria. Mar. Ecol. Prog. Ser. 323, 107–117. doi: 10.3354/meps323107
Kumar, S., Stecher, G., Li, M., Knyaz, C., and Tamura, K. (2018). MEGA X: molecular evolutionary genetics analysis across computing platforms. Mol. Biol. Evol. 35, 1547–1549.
Lane, D. J. (1991). “16S/23S rRNA sequencing,” in Nucleic Acid Techniques in Bacterial Systematic, eds E. Stackebrandt and M. Goodfellow (New York, NY: John Wiley and Sons).
Leão, P. N., Engene, N., Antunes, A., Gerwick, W. H., and Vasconcelos, V. (2012). The chemical ecology of cyanobacteria. Nat. Prod. Rep. 29, 372–391. doi: 10.1093/molbev/msy096
Leão, P. N., Vasconcelos, M. T. S. D., and Vasconcelos, V. M. (2009). Allelopathic activity of cyanobacteria on green microalgae at low cell densities. Eur. J. Phycol. 44, 347–355. doi: 10.1080/09670260802652156
Leão, Z. M., Kikuchi, R. K. P., Ferreira, B. P., Neves, E. G., Sovierzoski, H. H., Oliveira, M. D., et al. (2016). Brazilian coral reefs in a period of global change: a synthesis. Braz. J. Oceanogr. 64, 97–116.
Malik, S. A. A., Bedoux, G., Robledo, D., García-Maldonado, J. Q., Frelle-Pelegrín, Y., and Bourgougon, N. (2020). Chemical defense against microfouling by allelopathic active metabolites of Halymenia foresii (Rhodophyta). J. Appl. Phycol. 32, 2673–2687. doi: 10.1007/s10811-020-02094-2094
Mendes, T. C., Ferreira, C. E. L., and Clements, K. D. (2018). Discordance between diet analysis and dietary macronutrient content in four nominally herbivorous fishes from the Southwestern Atlantic. Mar. Biol. 165:180. doi: 10.1007/s00227-018-3438-3434
Morrow, K. M., Paul, V. J., Liles, M. R., and Chadwick, N. E. (2011). Allelochemicals produced by Caribbean macroalgae and cyanobacteria have species-specific effects on reef coral microorganisms. Coral Reefs 30, 309–320. doi: 10.1007/s00338-011-0747-741
Nagarkar, S., Williams, G. A., Subramanian, G., and Saha, S. K. (2004). “Cyanobacteria-dominated biofilms: a high quality food resource for intertidal grazers,” in Proceedings of the Asian Pacific Phycology in the 21st Century: Prospects and Challenges (Dordrecht: Springer).
Nicholson, G. M., and Clements, K. D. (2020). Resolving resource partitioning in parrotfishes (Scarini) using microhistology of feeding substrata. Coral Reefs 39, 1313–1327.
Nübel, U., Garcia-Pichel, F., and Muyzer, G. (1997). PCR primers to amplify 16S rRNA genes from cyanobacteria. Appl. Environ. Microbiol. 63, 3327–3332.
Oakley, C. A., and Davy, S. K. (2018). “Cell biology of coral bleaching,” in Coral Bleaching. Ecological Studies (Analysis and Synthesis), eds M. Van Oppen and J. Lough (Cham: Springer).
Patterson, M. R. (1992). A mass transfer explanation of metabolic scaling relations in some aquatic invertebrates and algae. Science 255, 1421–1423. doi: 10.1126/science.255.5050.1421
Paul, V. J., Thacker, R. W., Banks, K., and Golubic, S. (2005). Benthic cyanobacterial bloom impacts the reefs of South Florida (Broward County. USA). Coral Reefs 24, 693–697. doi: 10.1007/s00338-005-0061-x
Pennings, S. C., and Paul, V. J. (1993). Sequestration of dietary secondary metabolites by three species of sea hares: location, specificity and dynamics. Mar. Biol. 117, 535–546. doi: 10.1007/BF00349763
Puglisi, M. P., Sneed, J. M., Ritson-Williams, R., and Young, R. (2019). Marine chemical ecology in benthic environments. Nat. Prod. Rep. 36, 410–429. doi: 10.1039/C8NP00061A
Puyana, M., and Prato, J. (2013). Overgrowth of reef organisms by benthic cyanobacteria in the Colombian Caribbean. Mutis 3, 58–60. doi: 10.21789/22561498.885
Puyana, M., Prato, J. A., Nieto, C. F., Ramos, F. A., Castellanos, L., Pinzón, P., et al. (2019). Experimental approaches for the evaluation of allelopathic interactions between hermatypic corals and marine benthic cyanobacteria in the Colombian Caribbean. Acta Biol. Colomb. 24, 243–254. doi: 10.15446/abc.v24n2.72706
Rambaut, A. (2018). FigTree, Version 1.4. 4. Program Distributed by the Author. Institute of Evolutionary Biology: University of Edinburgh.
R Core Team (2016). R: a Language and Environment for Statistical Computing. Vienna: R Foundation for Statistical Computing.
Ribeiro, F. V., Padula, V., Moura, R. L., Moraes, F. C., Salomon, P. S., Gibran, F. Z., et al. (2017). Massive opisthobranch aggregation in the largest coralline reefs in the South Atlantic Ocean: are mesoherbivores underestimated top-down players? Bull. Mar. Sci. 93, 915–916. doi: 10.5343/bms.2016.110
Ribeiro, F. V., Sá, J. A., Fistarol, G. O., Salomon, P. S., Pereira, R. C., Souza, M. L. A. M., et al. (2018). Long-term effects of competition and environmental drivers on the growth of the endangered coral Mussismilia braziliensis (Verril, 1867). PeerJ 6:e5419. doi: 10.7717/peerj.5419
Ritson-Williams, R., Arnold, S. N., and Paul, V. J. (2020). The impact of macroalgae and cyanobacteria on larval survival and settlement of the scleractinian corals Acropora palmata, A. cervicornis and Pseudodiploria strigosa. Mar. Biol. 167:31.
Ritson-Williams, R., Paul, V. J., and Bonito, V. (2005). Marine benthic cyanobacteria overgrow coral reef organisms. Coral Reefs 24:629. doi: 10.1007/s00338-005-0059-54
Ronquist, F., Teslenko, M., Van Der Mark, P., Ayres, D. L., Darling, A., Höhna, S., et al. (2012). MrBayes 3.2: efficient Bayesian phylogenetic inference and model choice across a large model space. Syst. Biol. 61, 539–542. doi: 10.1093/sysbio/sys029
Silva-Lima, A. W., Walter, J. M., Garcia, G. D., Ramires, N., Ank, G., Meirelles, P. M., et al. (2015). Multiple Symbiodinium strains are hosted by the Brazilian endemic corals Mussismilia spp. Microb. Ecol. 70, 301–310. doi: 10.1007/s00248-015-0573-z
Sneed, J. M., Meickle, T., Engene, N., Reed, S., Gunasekera, S., and Paul, V. J. (2017). Bloom dynamics and chemical defenses of benthic cyanobacteria in the Indian River Lagoon. Florida. Harmful Algae 69, 75–82. doi: 10.1016/j.hal.2017.10.002
Suikkanen, S., Fistarol, G. O., and Granéli, E. (2004). Allelopathic effects of the Baltic cyanobacteria Nodularia spumdigena, Aphanizomenon flosaquae and Anabaena lemmermannii on algal monocultures. J. Exp. Mar. Biol. Ecol. 308, 85–101. doi: 10.1016/j.jembe.2004.02.012
Teixeira, C. D., Chiroque-Solano, P. M., Ribeiro, F. V., Carlos-Júnior, L. A., Neves, L. M., Salomon, P. S., et al. (2021). Decadal (2006-2018) dynamics of Southwestern Atlantic’s largest turbid zone reefs. PLoS One 16:e0247111. doi: 10.1371/journal.pone.0247111
Teixeira, C. D., Leitão, R. L., Ribeiro, F. V., Moraes, F. C., Neves, L. M., Bastos, A. C., et al. (2019). Sustained mass coral bleaching (2016-2017) in Brazilian turbid-zone reefs: taxonomic, cross-shelf and habitat-related trends. Coral Reefs 38, 801–813. doi: 10.1007/s00338-019-01789-1786
Thacker, R. W., Becerro, M. A., Lumbang, W. A., and Paul, V. J. (1998). Allelopathic interactions between sponges on a tropical reef. Ecology 79, 1740–1750. doi: 10.2307/176792
Thacker, R. W., Nagle, D. G., and Paul, V. J. (1997). Effects of repeated exposures to marine cyanobacterial secondary metabolites on feeding by juvenile rabbitfish and parrotfish. Mar. Ecol. Prog. Ser. 147, 21–29.
Titlyanov, E. A., and Titlyanova, T. V. (2009). The dynamics of the restoration of mechanical damage to colonies of the scleractinian coral Porites lutea under conditions of competition with algal settlers for substratum. Russ. J. Mar. Biol. 35, 230–235. doi: 10.1134/S1063074009030067
Trifinopoulos, J., Nguyen, L. T., von Haeseler, A., and Minh, B. Q. (2016). W-IQ-TREE: a fast online phylogenetic tool for maximum likelihood analysis. Nucleic Acids Res. 44, W232–W235. doi: 10.1093/nar/gkw256
Tronholm, A., and Engene, N. (2019). Moorena gen. nov., a valid name for “Moorea Engene & al.” nom. inval. (Oscillatoriaceae. Cyanobacteria). Not. Algar. 122, 1–2.
Ullah, H., Nagelkerken, I., Goldenberg, S. U., and Fordham, D. A. (2018). Climate change could drive marine food web collapse through altered trophic flows and cyanobacterial proliferation. PLoS Biol. 16:e2003446. doi: 10.1371/journalpbio.2003446
Walter, J. M., Coutinho, F. H., Leomil, L., Hargreaves, P. I., Campeão, M. E., Vieira, V. V., et al. (2020). Ecogenomics of the marine benthic filamentous cyanobacterium Adonisia. Microb. Ecol. 80, 249–265. doi: 10.1007/s00248-019-01480-x
Walter, J. M., Tschoeke, D. A., Meirelles, P. M., de Oliveira, L., Leomil, L., Tenório, M., et al. (2016). Taxonomic and functional metagenomic signature of turfs in the Abrolhos reef system (Brazil). PLoS One 11:e0161168. doi: 10.1371/journal.pone.0161168
Wangpraseurt, D., Weber, M., Røy, H., Polerecky, L., de Beer, D., Suharsono, et al. (2012). In situ oxygen dynamics in coral-algal interactions. PLoS One 7:e31192. doi: 10.1371/journal.pone.0031192
Wylie, C. R., and Paul, V. J. (1988). Feeding preferences of the surgeonfish Zebrasoma flavescens in relation to chemical defenses of tropical algae. Mar. Ecol. Prog. Ser. 45, 23–32. doi: 10.3354/meps045023
Yamamuro, M. (1999). Importance of epiphytic cyanobacteria as food sources for heterotrophs in a tropical seagrass bed. Coral Reefs 18, 263–271.
Yamazaki, S. S., Nakamura, T., Yuen, Y. S., and Yamasaki, H. (2008). “Reef-building coral Goniastrea aspera harbor a novel filamentous cyanobacterium in their skeleton,” in Proceedings of the 11th International Coral Reef Symposium (Ft. Lauderdale, FL).
Keywords: BCMs, Oscillatoriales, Synechococcales, zooxanthellae, interspecific interactions, Southwestern Atlantic, turbid reefs, chemical mediation
Citation: Ribeiro FV, Caires TA, Simões MAA, Hargreaves PI, Villela LB, Fistarol GO, Cazelgrandi Junior AB, Pereira-Filho GH, Moura RL, Pereira RC and Salomon PS (2022) Benthic Cyanobacterial Diversity and Antagonistic Interactions in Abrolhos Bank: Allelopathy, Susceptibility to Herbivory, and Toxicity. Front. Mar. Sci. 8:790277. doi: 10.3389/fmars.2021.790277
Received: 06 October 2021; Accepted: 23 November 2021;
Published: 06 January 2022.
Edited by:
Mirko Mutalipassi, Anton Dohrn Zoological Station, ItalyReviewed by:
Ethan C. Cissell, Florida State University, United StatesOlivier P. Thomas, National University of Ireland Galway, Ireland
Lucia Barra, Zoological Station Anton Dohrn, Italy
Copyright © 2022 Ribeiro, Caires, Simões, Hargreaves, Villela, Fistarol, Cazelgrandi Junior, Pereira-Filho, Moura, Pereira and Salomon. This is an open-access article distributed under the terms of the Creative Commons Attribution License (CC BY). The use, distribution or reproduction in other forums is permitted, provided the original author(s) and the copyright owner(s) are credited and that the original publication in this journal is cited, in accordance with accepted academic practice. No use, distribution or reproduction is permitted which does not comply with these terms.
*Correspondence: Paulo Sergio Salomon, cHNzYWxvbW9uQGdtYWlsLmNvbQ==
†Present address: Renato Crespo Pereira, Instituto de Pesquisas Jardim Botânico do Rio de Janeiro, Rio de Janeiro, Brazil