- 1Jiangsu Key Laboratory of Marine Bioresources and Environment, Jiangsu Ocean University, Lianyungang, China
- 2Co-Innovation Center of Jiangsu Marine Bio-Industry Technology, Jiangsu Ocean University, Lianyungang, China
- 3Jiangsu Key Laboratory of Marine Biotechnology, Jiangsu Ocean University, Lianyungang, China
- 4Jiangsu Institute of Marine Resources Development, Jiangsu Ocean University, Lianyungang, China
- 5Key Laboratory of Marine Ecosystem and Biogeochemistry, Second Institute of Oceanography, Ministry of Natural Resources, Hangzhou, China
Barnacles and bivalves are two well-known sessile invertebrates that play important roles in marine ecosystems. Microplastic (MP) pollution has attracted widespread attention. Barnacles and wild bivalves are smaller than farmed individuals; thus, they may be more sensitive to MPs. However, less is known about the abundance and spatial distribution of MPs in wild bivalves along with the coastal areas of China. This study evaluates MP pollution in the most abundant bivalves and barnacles (Crassostrea gigas, Ostrea cucullata, Mytilus edulis, and Balanus albicostatus) at five stations in the intertidal zone of the Yellow Sea. B. albicostatus was divided into ecotype A and ecotype B. The abundance of MPs in barnacles, wild bivalves barnacles, and wild bivalves varied from 0 to 2.25 items/individual and 0 to 118.21 items/g. O. cucullata and B. albicostatus (ecotype A) had the highest abundance of MPs, with average abundances of 0.56 ± 0.36 items/individual and 21.59 ± 27.26 items/g, respectively. The types of MPs found in bivalves and barnacles include fibers, fragments, films, and microbeads. The most abundant size was less than 1,000 μm, which accounted for 53% of the total MPs. Cellophane (CP), polypropylene (PP), polyethylene (PE), and polyethylene terephthalate (PET) were the main polymer types in bivalves and barnacles. This study suggests that the abundance of MPs in wild bivalves is close to that of farmed bivalves with commercial specifications, despite their smaller size. The MP abundance of barnacles in the Yellow Sea is higher than that in other areas in terms of items per gram. In addition, the ecological type may affect the ability of barnacles to accumulate MPs. Ingestion of MPs by barnacles and wild bivalves should be of concern because they may enter the human body through the food web and may pose a potential threat to human health.
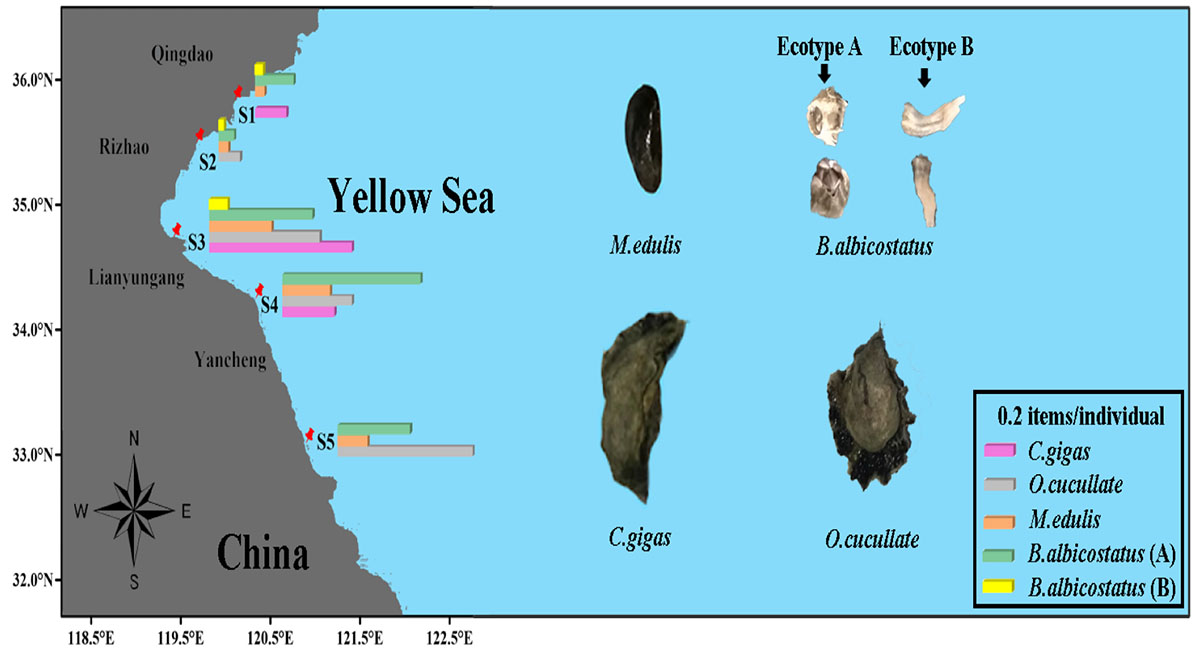
GRAPHICAL ABSTRACT. Microplastics in wild bivalves and wild bivalves on the coast of the Yellow Sea.
Introduction
Since plastic was invented in the middle of the last century, it has changed our life due to its multiple advantages, resulting in the massive replacement of traditional materials with plastic (Thompson et al., 2004; Barnes et al., 2009). Actually, the annual production of plastic in the world exceeded an estimate of 335 million tons in 2016 (Plastics Europe, 2018). A considerable amount of plastic waste is discharged into the ocean due to ineffective disposal by humans. As a result, plastic materials account for 60–80% of all marine debris in the ocean (Gregory and Ryan, 1997). It has become a potentially important menace to the marine environment on this planet (Zalasiewicz et al., 2016).
Microplastics (MPs) are defined as any plastic particles smaller than 5 mm in diameter (Thompson et al., 2004), including primary MPs and secondary MPs. Primary MPs are usually derived from industrial production (Costa et al., 2010), while secondary MPs are produced from mesoplastics and macroplastics by physical damage, photodegradation, oxidation, and biodegradation (Thompson et al., 2004; Auta et al., 2017; Isobe et al., 2019). Due to the small size of MPs, they are more easily dispersed in the ocean than large plastics. Consequently, MPs are ubiquitous in ocean environmental media (Rachel, 2018; Zhao et al., 2018; Zhu et al., 2018; Deanna and Mona, 2019). This leads to the ingestion of MPs by many marine organisms, including plankton (Lima et al., 2014), marine worms (Besseling et al., 2013), sea cucumber (Mohsen et al., 2019), bivalves (Von Moos et al., 2012; Li et al., 2018; Qu et al., 2018; Teng et al., 2019), crab (Zhang et al., 2021), fish (Foekema et al., 2013; Feng et al., 2019; Su et al., 2019), and even sea birds (Provencher et al., 2018). Moreover, MPs have the ability to carry out more toxic matters due to their large specific surface area and are more difficult to remove (Zhu et al., 2019). Therefore, MPs not only pose a threat to wildlife but are also potentially harmful to the global marine environment (Lebreton et al., 2017; Haward, 2018).
Seafood provides approximately 20% of the animal protein intake of nearly 3 billion people worldwide, and the annual consumption of mollusks per person in China is 9.98 kg (FAO, 2017). During bivalve and barnacle feeding, a large amount of seawater is filtered, consequently accumulating MPs from the seawater. Moreover, previous studies have shown that MPs are present in cultured bivalves around the world (Lisbeth and Colin, 2014; Dannielle, 2016; Li et al., 2018; Qu et al., 2018Teng et al., 2019; Feng et al., 2020c). However, there are few studies on MP pollution in wild bivalves, especially those attached to rocks in the intertidal zone as easily accessible seafood. In addition, bivalves and barnacles play a vital role in coastal ecosystems as an important part of the food chain. Some barnacles and wild bivalves are even thought to be ideal biological indicators in monitoring MP pollution (Li et al., 2018; Qu et al., 2018; Xu et al., 2020). Therefore, it is necessary to conduct more investigations on MP pollution in bivalves and barnacles. Moreover, wild individuals are often small, while cultured individuals are large, which may affect their MP levels. Breeding individuals often use breeding appliances, such as net cages, which are considered to be a source of marine MPs (Feng et al., 2020b). In addition, the difference in density per unit area of wild and cultured individuals is also an important factor affecting the abundance of biological MPs (Sfriso et al., 2020).
This study aims to increase our knowledge about the distribution of MPs in different barnacles and wild bivalves and to quantify MP variability between four species widely distributed in the Yellow Sea, China. The abundance and characteristics of MPs ingested by barnacles and wild bivalves collected from intertidal reefs along the Yellow Sea were investigated. In addition, two different ecotypes of barnacles were of particular concern. This study provides evidence for future evaluation of the selection of indicator organisms and the ecological and health risks caused by MP pollution of wild seafood in the marine environment.
Materials and Methods
Sampling Collection
Wild bivalve and barnacle samples were collected at five locations (S1–S5) in the intertidal zone of the Yellow Sea in November 2018 using new stainless steel tools and knives (Figure 1). The collected barnacles and wild bivalves were held in aluminum foil bags. After the collection was completed, they were temporarily stored in a cooler (−5°C) and then stored in a refrigerator at −20°C in the laboratory for further analysis. Prior to dissection and digestion, all wild bivalve and barnacle samples were kept for not more than 3 weeks. Balanus albicostatus specimens were divided into two ecotypes based on whether there was a tube at their base (ecotype A without tube and ecotype B with tube).
Quality Assurance and Control
New tools made of stainless steel were used to collect wild bivalve and barnacle samples. When sampling, researchers in this study used aluminum foil bags and quickly packed the collected stemless invertebrates separately to reduce atmospheric pollution. Laboratory processing was performed in a laminar flow cabinet (SW-CJ-2F, SUJING, China). Operators in the laboratory were kept to a minimum, and outdoor air circulation was minimized. All liquids were filtered with glass microfibers (Φ = 8 μm, d = 47 mm) before use. Clothing and instrument cleaning procedures of the operator were consistent with those in our previous studies (Feng et al., 2020b). The MP pollution status in the laboratory passed the program blank (without wild bivalve and barnacle samples) detection, and five program blanks were set for each batch of sample processing.
Sampling Dissection and Digestion
Samples were selected to remove damaged individuals before digestion. The shells of stemless invertebrates were thoroughly rinsed with deionized water after defrosting. Due to their small size and large numbers, the digestion of barnacles and wild bivalves was not suitable for single sample digestion from the perspective of economy and operability. Therefore, this study uses 30–50 sessile organisms to be digested and processed in groups. The wet weight of soft tissues and total individuals was determined by a precision electronic balance (BS124S, Sartorius Electronic Balance, Beijing). The digestion process was based on previous studies (Foekema et al., 2013; Karami et al., 2017; Feng et al., 2020a). After complete digestion, the clear yellow light digestion solution was transferred and filtered through 8-μm pore size glass microfiber filter membranes (Haining Jinzheng, China) using a filtration unit with one Büchner funnel (AP-01P, Autoscience, Tianjin). The filter membranes were placed in clean Petri dishes with lids and dried at room temperature.
Microplastic Identification and Data Analysis
The observation and identification of MPs were consistent with previous studies (Zhao et al., 2016; Cai et al., 2019; Feng et al., 2019; Zhang et al., 2021). The data were analyzed using SPSS version 23 (IBM, New York, NY, United States) and visualized using Origin 2021 (Originlab, Northampton, Massachusetts, United States). The MP size data conformed to a normal distribution (Shapiro–Wilk, P > 0.05), and the variances could be considered equal (Levene’s test, P > 0.05). One-way ANOVA was conducted to assess differences in MP size among different stations. The least significant difference was determined by ANOVA post hoc investigation. Kruskal–Wallis ANOVA was used to determine whether there was a significant difference in MP abundance for different species and various sampling stations. A CI of 95% was set for all tests. A linear regression analysis was applied to determine the significant correlation between MP abundance and wild bivalve and barnacle soft tissue weight.
Results
Characteristics of Barnacles and Wild Bivalves
A total of 3,067 barnacles and wild bivalves were collected from five sites along the Yellow Sea (Table 1). These barnacles and wild bivalves were identified as four species, namely, Crassostrea gigas (C. gigas), Ostrea cucullata (O. cucullata), Mytilus edulis (M. edulis), and B. albicostatus. B. albicostatus was found in two different ecotypes, one of which had bases without tubes (ecotype A), while the other had bases with tubes (ecotype B), as shown in the Graphical Abstract. The numbers of C. gigas, O. cucullata, M. edulis, B. albicostatus (ecotype A), and B. albicostatus (ecotype B) were 200, 233, 940, 660, and 1,034, respectively.
Microplastic Abundance in Barnacles and Wild Bivalves in Different Areas
Microplastics were detected visually in more than 91% of the groups, with a total number of 600 items among all barnacles and wild bivalves. MPs were found in all barnacles and wild bivalves at sites S3 and S4. The lowest detection rate was from S2, which also reached 77.78% (Figure 2).
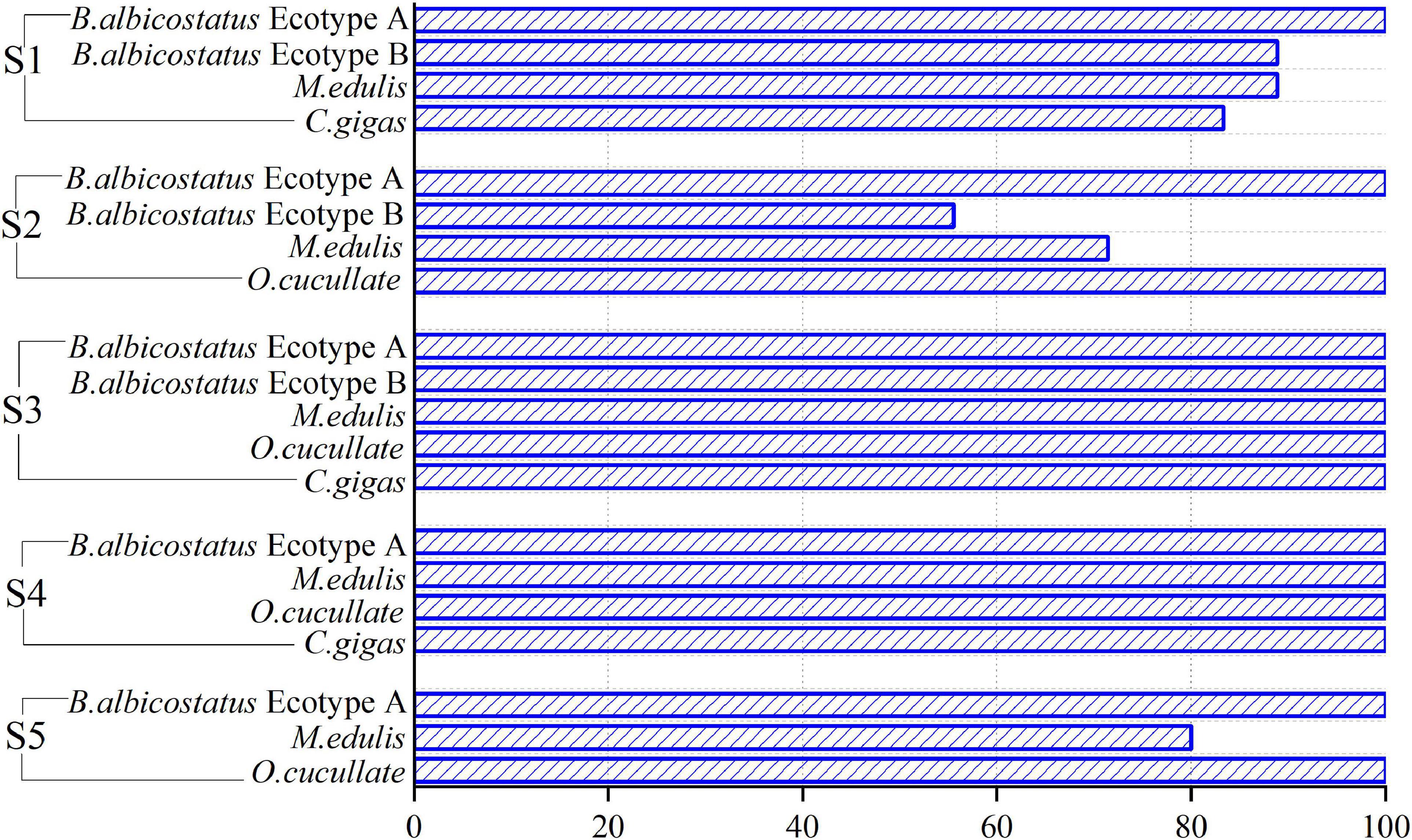
Figure 2. Microplastic (MP) detection rates at five sampling sites; 100% of the sessile organism groups from S3 to S4 were detected.
The MP abundance in barnacles and wild bivalves from five areas was revealed (Figure 3). MP abundance in barnacles and wild bivalves from different areas was significantly different for items per individual (Kruskal–Wallis, P = 0.000) and items per gram (Kruskal–Wallis, P = 0.000). When both normalized to individual or mass, barnacles and wild bivalves from S3 had the highest MP abundance (0.52 ± 0.45 items/individual and 16.85 ± 23.42 items/g), while in terms of items per individual, MP abundance in S2 samples had the lowest level, at 0.08 ± 0.08 items/individual and 3.13 ± 3.50 items/g.
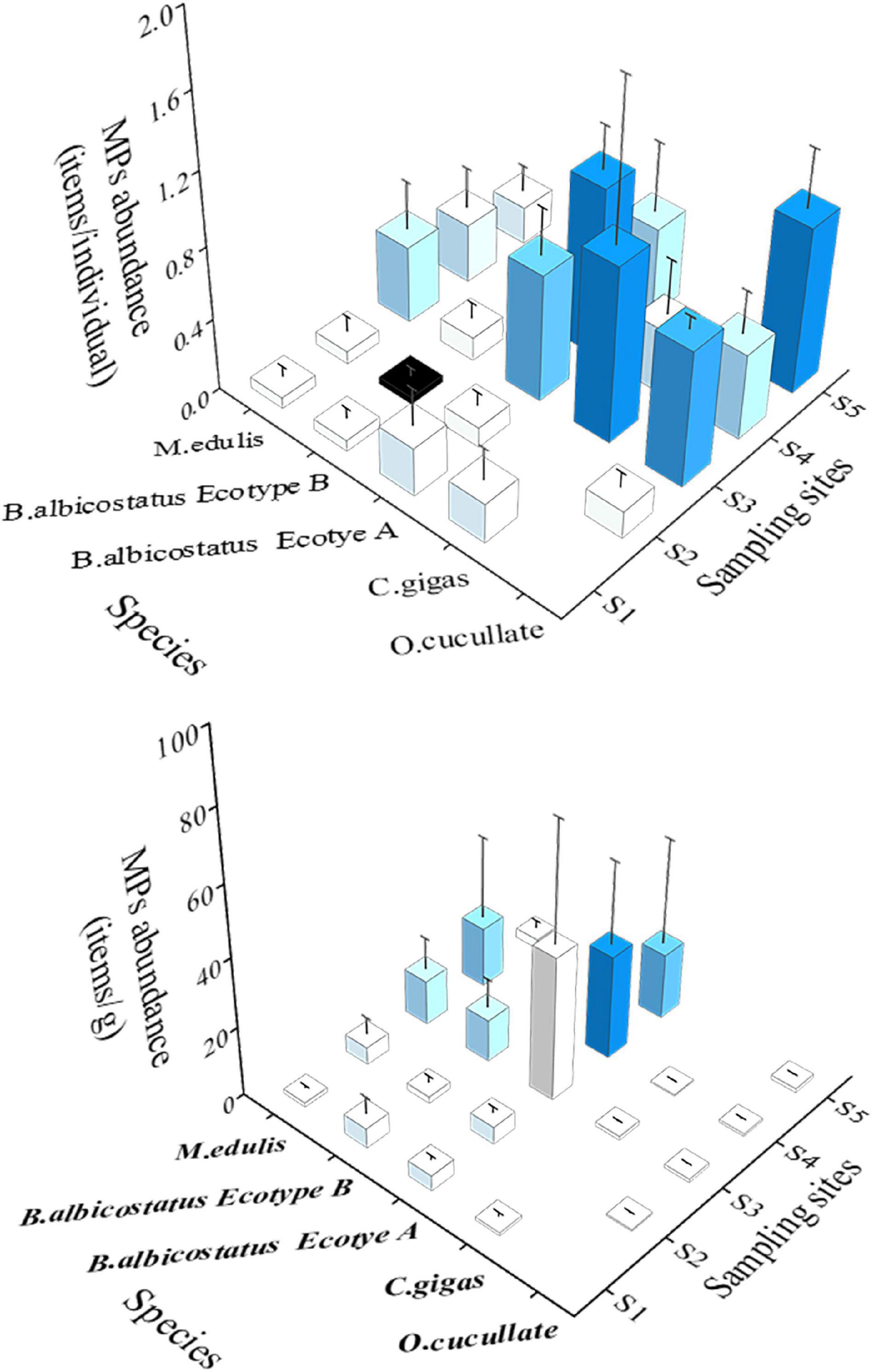
Figure 3. Abundance of MPs (mean ± SD) in four species of barnacles and wild bivalves from different sites in the Yellow Sea.
The abundance of MPs of barnacles and wild bivalves for different species was further studied (Figure 3). Kruskal–Wallis ANOVA showed that the MP abundance of various barnacles and wild bivalves was significantly different for both items per individual (P = 0.002) and items per gram (P = 0.000). The highest MP abundance in items per individual was O. cucullata (0.56 ± 0.36), while M. edulis was the lowest (0.21 ± 0.21). B. albicostatus had the highest value (14.09 ± 21.31), and C. gigas was 0.77 ± 0.81 when it was normalized to items per gram.
Characteristics of Microplastics in Barnacles and Wild Bivalves
Multiple types of MPs, including fibers, fragments, films, and microbeads, occurred in the tissues of the barnacles and wild bivalves (Figure 4). The most diverse types were observed as fibers, followed by fragments. The most popular colors were black, blue, green, and red, which were over 90% (Figure 5). The size of MPs varied from 135.12 to 2,917.69 μm, 86.55 to 4,867.23 μm, 137.85 to 3,331.06 μm, 69.83 to 3,743.20 μm, and 194.66 to 2,885.65 μm in C. gigas, O. cucullata, M. edulis, and B. albicostatus ecotype A and ecotype B, respectively. MPs less than 1 mm in size were the most common. As the size of MPs increased, the abundance trend of MPs decreased exponentially (R2 = 0.713, P < 0.01) (Figure 6). The materials of suspected MPs in the barnacles and wild bivalves were also confirmed (Figure 7). Most of them were cellophane (CP, 28.17%), followed by polypropylene (PP, 25.35%) and polyethylene (PE, 23.94%).
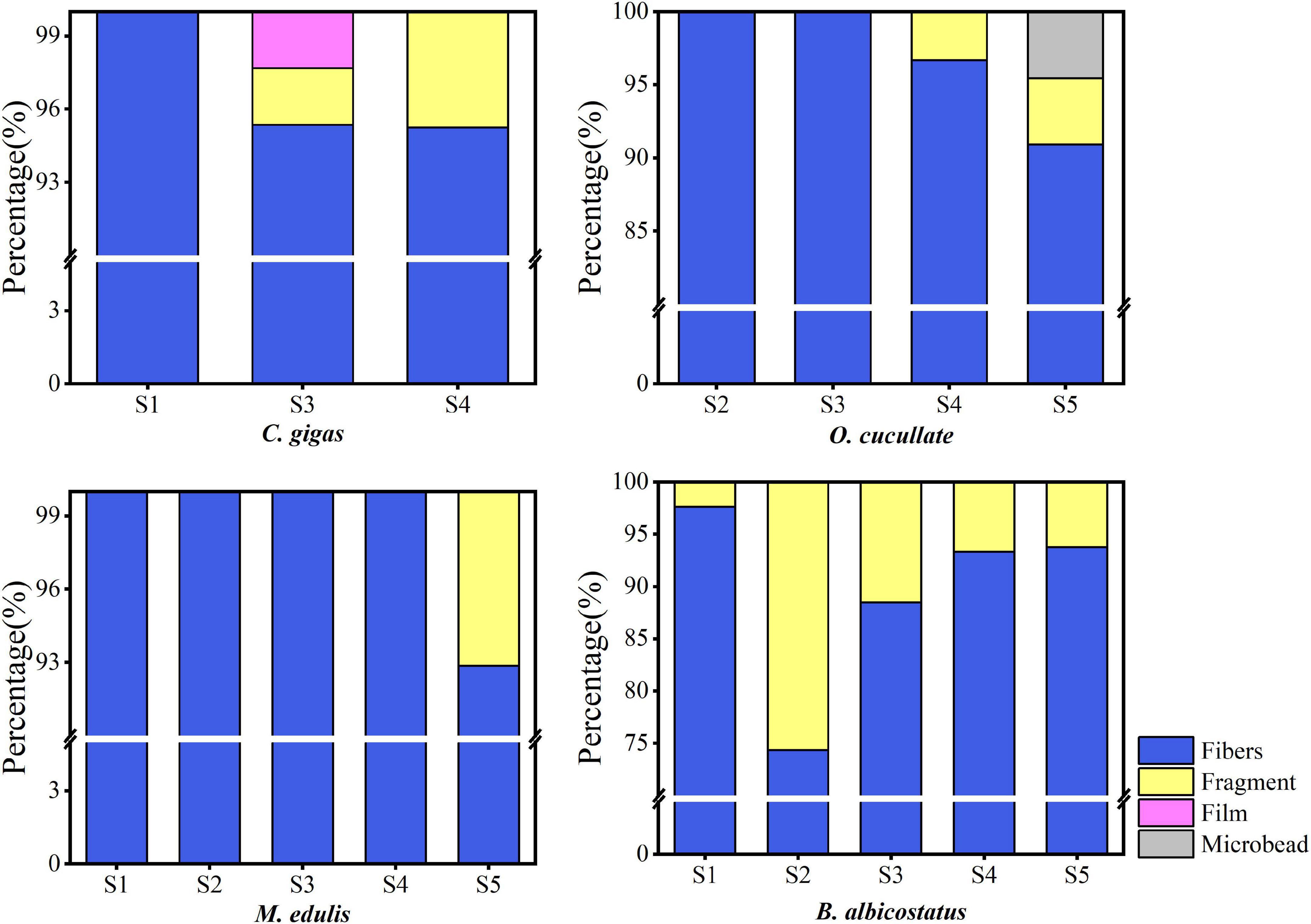
Figure 4. Shape distribution of MPs in four species of barnacles and wild bivalves from different sites in the Yellow Sea.
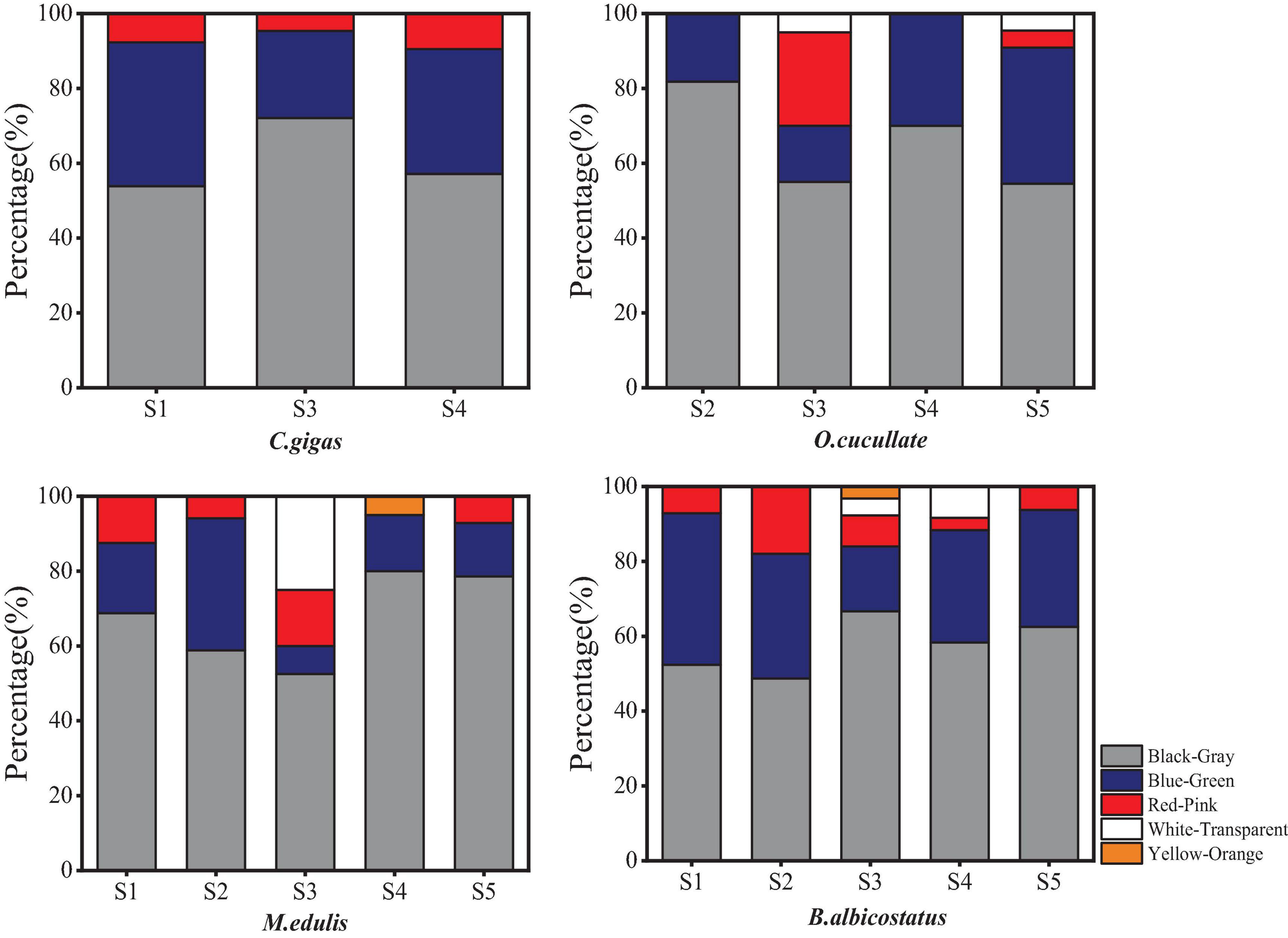
Figure 5. Color distribution of MPs in four species of barnacles and wild bivalves from different sites in the Yellow Sea.
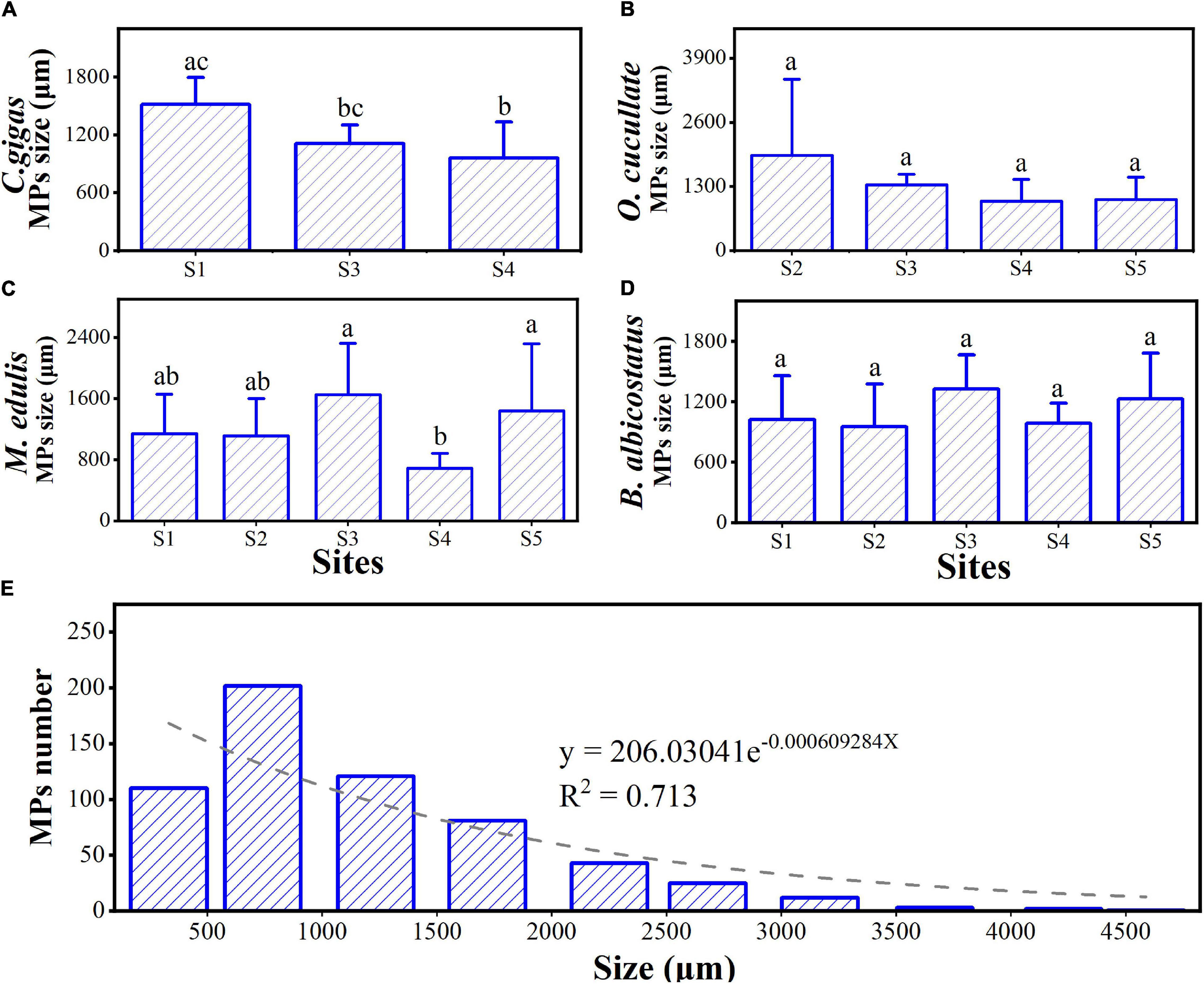
Figure 6. Mean MP size (± SD) in four species of barnacles and wild bivalves from different sites (A–D) in the Yellow Sea and the size distribution of the MPs in all wild bivalves and barnacle samples (E). Different letters above the bars indicate significant differences among species (one-way ANOVA, P < 0.05). The gray dashed represents the fit between the number and the particle size of MPs.
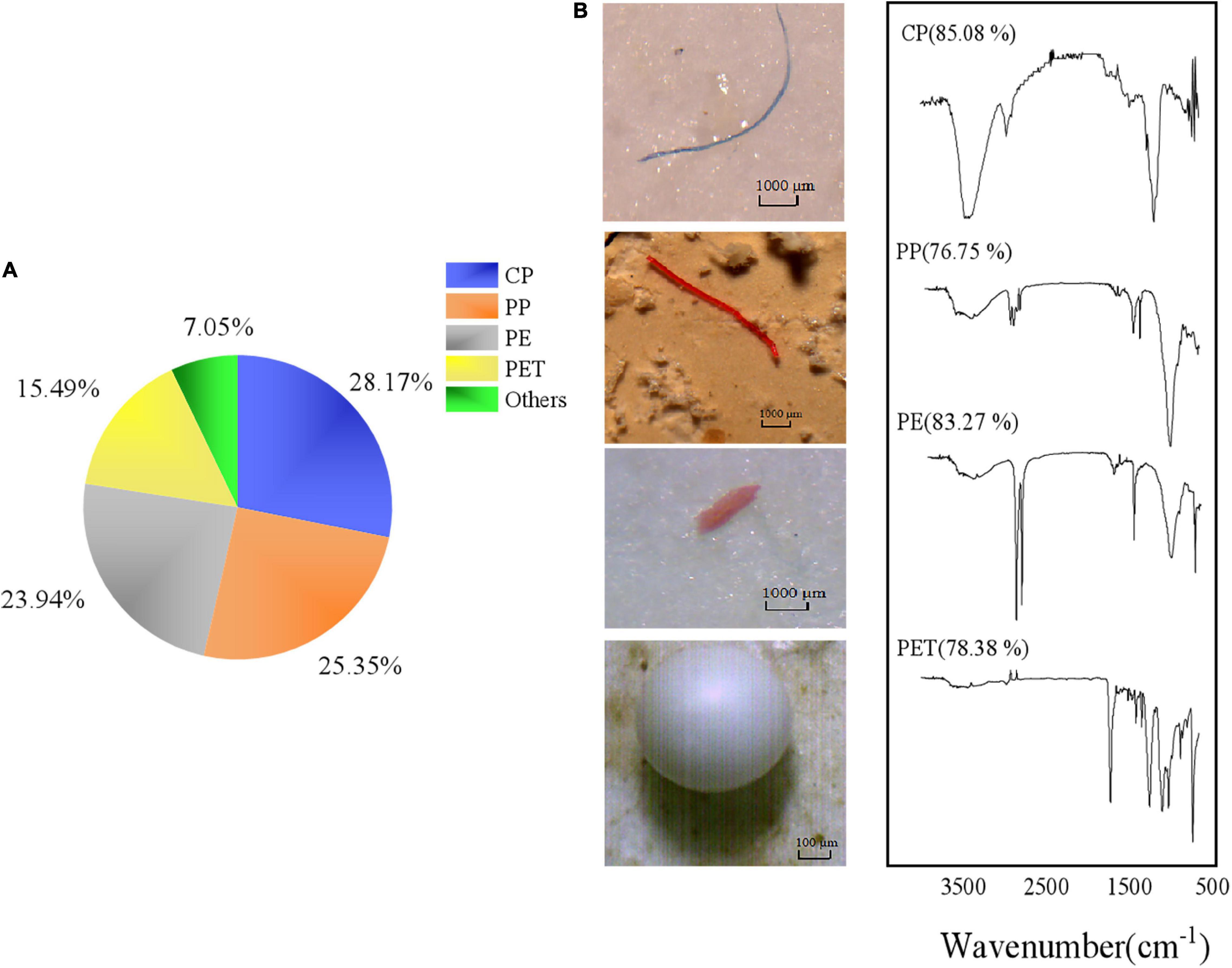
Figure 7. Type distribution (A) and material spectra (B) identified by μ-FT-IR microscopy of the MPs observed in barnacles and wild bivalves. Proportions less than 5% were classified as others, including polymethacrylates (PMA, 4.23%), polyacrylonitrile (PAN, 1.41%), and poly(urethane:ethylene terephthalate) (PU-PET, 1.41%).
Microplastic Pollution Features of Various Ecotypes
The abundance of MPs of different ecotypes and habitats of B. albicostatus was also analyzed (Figure 8). Ecotype A barnacles had a higher MP pollution level than ecotype B in terms of both items per individual and items per gram. An average of 0.52 ± 0.38 MPs was found in ecotype A, while 0.08 ± 0.08 MPs were found in ecotype B. In terms of items per gram, 21.59 ± 27.26 existed in ecotype A compared with 6.60 ± 7.25 in ecotype B. Both fibers and fragments were found in different B. albicostatus ecotypes, but slight differences in proportion existed (fibers occupied 98.14% in ecotype A and 94.29% in ecotype B). There was no significant difference in the particle size distribution of MPs below 3,000 μm. However, MPs with a particle size larger than 3,000 μm were only found in ecotype A barnacles.
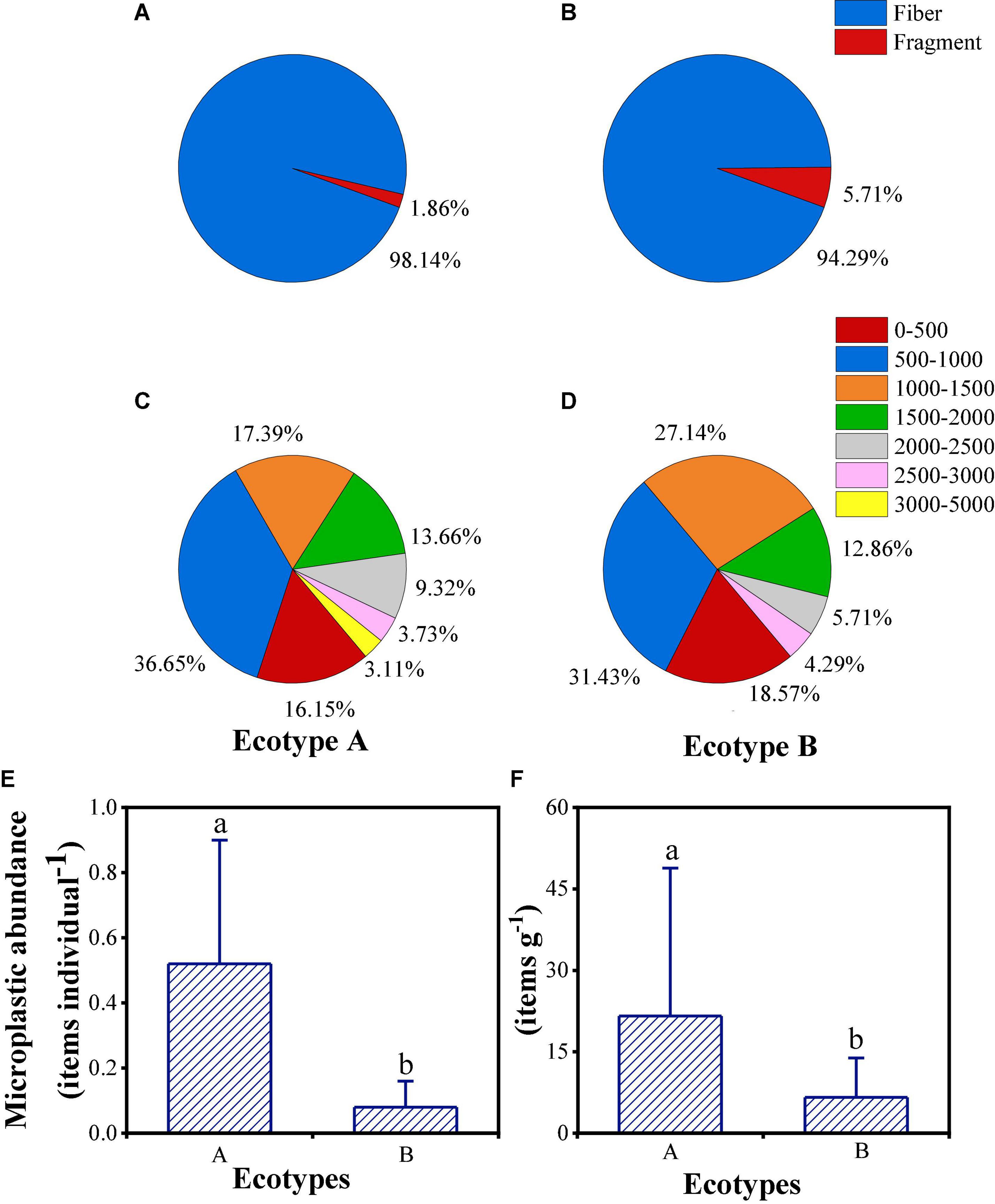
Figure 8. The shape, size and abundance of MPs in ecotypes A (A,C,E) and B (B,D,F) of barnacles. Different letters above the bars indicate significant differences among ecotypes (one-way ANOVA, P < 0.05). Different letters above the bars indicate significant differences among ecotypes (one-way ANOVA, P < 0.05).
Relationship Between Microplastic Abundance and Soft Tissue Weight of Barnacles and Wild Bivalves
To further explore the relationship between the biological characteristics of sessile organisms and MP pollution levels, the relationship between MP abundance and soft tissue weight was analyzed (Figure 9). The results suggested that the MP value was highly positively related to the tissue weight of O. cucullata (r = 0.680, P < 0.01) and B. albicostatus ecotype B (r = 0.684, P < 0.01) normalized to items per individual.
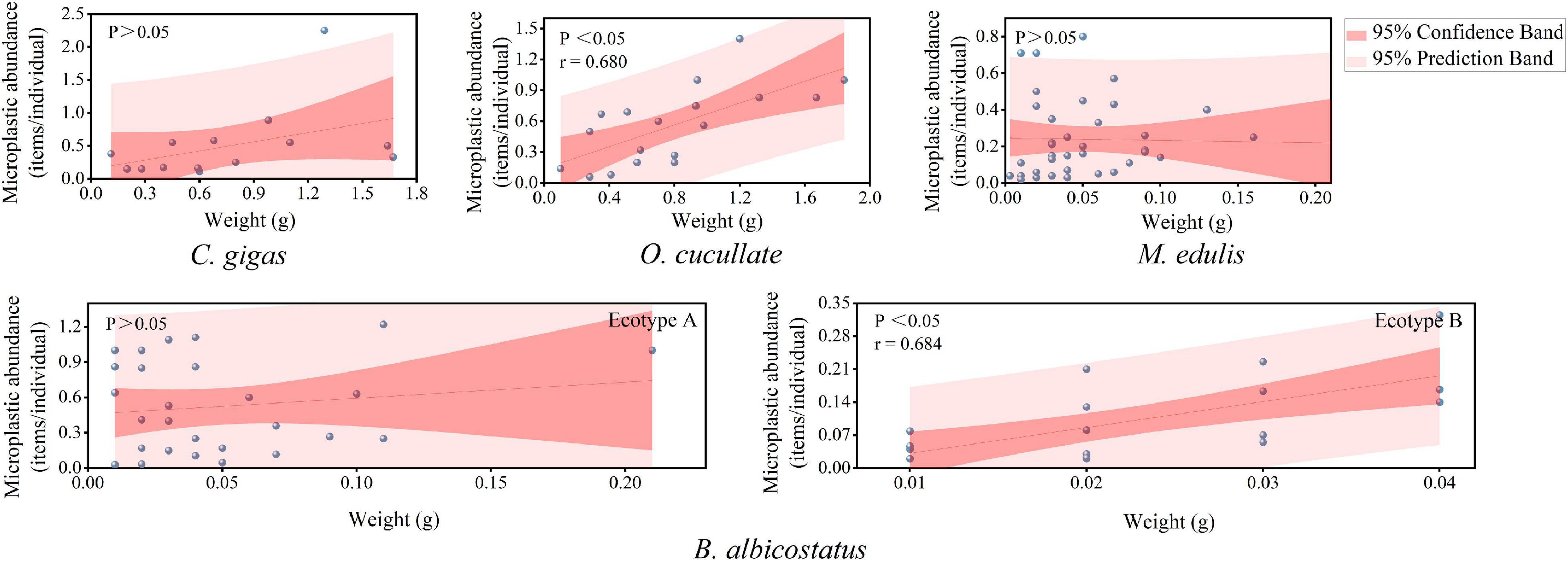
Figure 9. Correlation analysis between MP abundance and wet weight of the total tissues of barnacles and wild bivalves. The red line is the fitted trend line, gray ball represents the abundance value of MPs in different weights of organisms.
Discussion
Microplastic Pollution Level in Barnacles and Wild Bivalves of the Yellow Sea
In this study, MP abundance in four species of barnacles and wild bivalves in the Yellow Sea was investigated. The high MP detection rate (91.85%) indicates that MPs are widespread in barnacles and wild bivalves, suggesting that barnacles and wild bivalves can accumulate MPs. These results indicate that the intertidal zone of the Yellow Sea has been contaminated by MPs (Teng et al., 2019; Feng et al., 2020c). Therefore, barnacles and wild bivalves can be exposed to MPs in natural environments. However, the abundance of MPs in the barnacles and wild bivalves was significantly different, indicating significant spatial variability (Figure 8). The highest abundance of MPs and 100% MP detection rate were found in the barnacles and wild bivalves from the S3 region, whether calculated by an individual or by items per gram. The results were also consistent with previous studies on MPs in marine invertebrates (Carney and Eggert, 2019; Teng et al., 2019; Feng et al., 2020a,b), showing that the S3 area is more seriously affected by human activities, such as shipping and mariculture.
In contrast, the abundance of MPs showed great variation between the species of barnacles and wild bivalves, with range values of 0–2.25 items/individual and 0–118.21 items/g. The ability to ingest or eliminate MPs may lead to interspecific differences in MP abundance (Xu et al., 2020). Compared with previous studies (Table 2), the values of MP abundance in mussels showed a higher level by items per gram (Li et al., 2016; Qu et al., 2018), which was only lower than the results of Kolandhasamy et al. (2018). However, it was much lower when expressed by individuals (Li et al., 2016; Kolandhasamy et al., 2018; Qu et al., 2018). For the oysters in this study, both items per gram and items per individual showed intermediate levels of contamination compared with traditional studies (Gajahin et al., 2017; Li et al., 2018; Teng et al., 2019). Few studies have been conducted on MPs in barnacles, and our results show that the pollution level of barnacles in the Yellow Sea is much higher than that in the upper gulf in Thailand and the coast of Hong Kong in terms of items per gram (Gajahin et al., 2017; Xu et al., 2020). When calculated for individuals, the abundance of MPs in barnacles along the coast of Hong Kong was higher than that in this study (Xu et al., 2020). According to the current research results, the uptake of MPs by barnacles and wild bivalves is ubiquitous, and the degree of habitat pollution and the size of individuals are the main influencing factors. In particular, MPs are found in oysters and mussels, although they are not cultured on ropes or cages. In addition, it is worth noting that due to different methods, there may be differences in comparing the levels and abundance of MP pollution between different studies (Table 2).
Characteristics of Microplastic Pollution in Barnacles and Wild Bivalves
In this study, MPs with a size of <1,000 μm were the most abundant, which has also been found in many previous studies (Peng et al., 2017; Teng et al., 2019; Feng et al., 2020a). Browne et al. (2011) reported that MPs with small particle sizes may be more likely to be ingested and to accumulate in tissues, and MPs with small particle sizes may show extremely high toxic effects on copepod adults and offspring. In terms of the shape of particles in barnacles and wild bivalves, most MPs were fibrous, which was consistent with the results from seawater samples from the Yellow Sea (Sun et al., 2018). In the South Yellow Sea, the high-frequency use of plastic fishing tools (such as fishing gear, ropes, and fishing nets) has led to fibers becoming the most common shape in this area (Feng et al., 2019). In addition, fibers may also be the most abundant shape of MPs in the ocean (Frias et al., 2016; Andrés and Ruth, 2017). For materials, CP, PP, PE, and polyethylene terephthalate (PET) were the main polymer types in the barnacles and wild bivalves. These four material types are also the most abundant materials for MPs in coastal environments and organisms (Jabeen et al., 2017; Zhu et al., 2018; Feng et al., 2019; Mohsen et al., 2019; Teng et al., 2019; Zhang et al., 2019).
Microplastic Pollution in Different Ecotypes of Barnacles
In our study, the distribution of barnacles was found at Stations S1–S5. Among them, two types of barnacles with different ecotypes (ecotype A without tubes and ecotype B with tubes) were found at Stations S1–S3. The abundance of MPs in these two different ecotypes of barnacles was different, and the abundance of MPs in barnacles with tubes was significantly lower than that of tubeless barnacles. In addition, the characteristics of MPs of different ecotypes of barnacles are manifested only in the significant differences in the abundance of MPs, and there was no significant difference in type. These results may indicate that differences in ecotypes affect the accumulation of MPs in marine organisms (Figure 8). In this study, during the sampling process, we found that barnacles with ecotype B had a higher population density than those with ecotype A. We speculate that when the environment has a certain number of MPs, the larger the number of individuals is, the smaller the number of MPs that can be obtained. There were similar inferences in a study of MP pollution of the benthos (Sfriso et al., 2020). However, the mechanism of MP accumulation in individuals caused by different ecological types still needs further confirmation. In previous studies, the abundance of MPs in two different ecotypes of Ulva prolifera (drifting and attached) showed significant differences, which provides evidence supporting our conclusion (Feng et al., 2020b). We speculate that ecotypes may play an important role in the accumulation of MPs, not only in plants but also in animals. Thus, we suggested that the selection of MP indicator organisms should consider in-depth their ecological type (Figure 9).
Characteristics of Microplastic Pollution in Barnacles and Wild Bivalves
A large number of studies have confirmed that MPs can cause damage to marine invertebrates, including mechanical damage caused by abrasion or obstruction of the digestive tract, reduction in a filter-feeding rate and growth rate, energy consumption, and oxidative stress (Von et al., 2012; Rochman et al., 2013; Wright et al., 2013). In addition, the large specific surface area of MPs allows MPs to interact with pollutants in the environment. In particular, MPs have a strong adsorption capacity for persistent organic pollutants (POPs, Rochman et al., 2013; Wright et al., 2013). This adsorption behavior allows MPs to act as carriers for contaminants to enter the organism through the food chain (Derraik, 2002; Cole et al., 2011). In addition, barnacles and wild bivalves play an important ecological function in the intertidal zone. They are not only important feeders of phytoplankton and zooplankton but also serve as prey for various marine animals. This bioaccumulation effect of MPs may gradually have a negative impact on the diversity of coastal organisms and the functionality of ecosystems.
Mussels, oysters, and some species of barnacles are important sources of seafood along the coast of China. Barnacles and wild bivalves distributed in the intertidal zone are also easy to obtain for consumption. Furthermore, they are consumed whole, without gut removal. As a result, the consumption of this contaminated seafood may increase the potential risk to human health (Hussain et al., 2001). However, detailed investigations should be conducted to understand the mutual transmission and accumulation of MPs through the food webs of coastal and marine ecosystems and the harmful effects for marine life in regard to MP ingestion.
Conclusion
Our study found that MP pollution in barnacles and wild bivalves is widespread in the intertidal zone of the Yellow Sea. Barnacles and wild bivalves can be used as ecological indicators of MPs. Different ecotypes may lead to differences in the accumulation of MPs in barnacles. However, the mechanism of MP accumulation in different ecotypes of barnacles remains to be studied. In addition, our study indicates that MPs are widespread in seafood, such as shellfish, representing a potential risk to human health.
Data Availability Statement
The original contributions presented in the study are included in the article/supplementary material, further inquiries can be directed to the corresponding author.
Author Contributions
TZ: conceptualization, methodology, writing original draft – reviewing and editing, supervision, and funding acquisition. KS: investigation, methodology, formal analysis, and visualization. LM: conceptualization, methodology, and writing, reviewing, and editing. RT and TS: investigation. WH: methodology. ZF: conceptualization, formal analysis, writing original draft, reviewing and editing, supervision, and funding acquisition. All authors contributed to the article and approved the submitted version.
Funding
This work was supported by Jiangsu Industry-University-Research Cooperation Project (BY2020428), Open-end Funds of Jiangsu Key Laboratory of Marine Bioresources and Environment (SH20201210), Provincial Policy Guidance Program (North Jiangsu Science and Technology Special Project) (SZ-LYG202131), College Student Innovation and Entrepreneurship Training Program of Jiangsu Province (202111641155Y), Universities Natural Science Research Project of Jiangsu Province (20KJB170029), Jiangsu Province Postdoctoral Research Foundation (2021K212B), and Postdoctoral Research Foundation of Lianyungang (LYG2021002).
Conflict of Interest
The authors declare that the research was conducted in the absence of any commercial or financial relationships that could be construed as a potential conflict of interest.
Publisher’s Note
All claims expressed in this article are solely those of the authors and do not necessarily represent those of their affiliated organizations, or those of the publisher, the editors and the reviewers. Any product that may be evaluated in this article, or claim that may be made by its manufacturer, is not guaranteed or endorsed by the publisher.
References
Andrés, R. S., and Ruth, P. (2017). Morphological and physical characterization of microplastics. Compr. Anal. Chem. 7, 49–66. doi: 10.1016/bs.coac.2016.10.007
Auta, H. S., Emenike, C. U., and Fauziah, S. H. (2017). Distribution and importance of microplastics in the marine environment: a review of the sources, fate, effects, and potential solutions. Environ. Int. 102, 165–176. doi: 10.1016/j.envint.2017.02.013
Barnes, D. K. A., Galgani, F., Thompson, R. C., and Barlaz, M. (2009). Accumulation and fragmentation of plastic debris in global environments. Philos. Trans. R. Soc. B Biol. Sci. 364, 1985–1998. doi: 10.1098/rstb.2008.0205
Besseling, E., Wegner, A., Foekema, E. M., Van Den Heuvel-Greve, M. J., and Koelmans, A. A. (2013). Effects of microplastic on fifitness and PCB bioaccumulation by the lugworm Arenicola marina (L.). Environ. Sci. Technol. 47, 593–600. doi: 10.1021/es302763x
Browne, M. A., Crump, P., Niven, S. J., Teuten, E., Tonkin, A., Galloway, T., et al. (2011). Accumulation of Microplastic on shorelines woldwide: sources and sinks. Environ. Sci. Technol. 45, 9175–9179. doi: 10.1021/es201811s
Cai, H. W., Du, F. N., Li, L. Y., Li, B. W., Li, J. N., and Shi, H. H. (2019). A practical approach based on FT-IR spectroscopy for identification of semi-synthetic and natural celluloses in microplastic investigation. Sci. Environ. 669, 692–701. doi: 10.1016/j.scitotenv.2019.03.124
Carney, A. B., and Eggert, H. (2019). Marine plastic pollution: sources, impacts, and policy tissues. Rev. Environ. Econ. Policy 13, 317–326. doi: 10.1093/reep/rez012
Cole, M., Lindeque, P., Halsband, C., and Galloway, T. S. (2011). Microplastics as contaminants in the marine environment: a review. Mar. Pollut. Bull. 62, 2588–2597. doi: 10.1016/j.marpolbul.2011.09.025
Costa, M. F., Ivar do, S. J. A., Silva-Cavalcanti, J. S., Araújo, M. C. B., Spengler, A., and Tourinho, P. S. (2010). On the importance of size of plastic fragments and pellets on the strandline: a snapshot of a Brazilian beach. Environ. Monit. Assess. 168, 299–304. doi: 10.1007/s10661-009-1113-4
Dannielle, S. G. (2016). Effects of microplastics on European flat oysters, Ostrea edulis and their associated benthic communities. Environ. Pollut. 216, 95–103. doi: 10.1016/j.envpol.2016.05.043
Deanna, R., and Mona, W. (2019). Characterization of microplastics in the surface waters of Kingston Harbour. Sci. Total Environ. 664, 753–760. doi: 10.1016/j.scitotenv.2019.01.319
Derraik, J. G. B. (2002). The pollution of the marine environment by plastic debris: a review. Mar. Pollut. Bull. 44, 842–852. doi: 10.1016/S0025-326X(02)00220-5
FAO (2017). Food and Agriculture Organization of the United Nations; The State of World Fisheries and Aquaculture. Rome: FAO, 230.
Feng, Z. H., Zhang, T., Li, Y., He, X. R., Wang, R., Xu, J. T., et al. (2019). The accumulation of microplastics in fish from an important fish farm and mariculture area, Haizhou Bay, China. Sci. Total Environ. 696:133948. doi: 10.1016/j.scitotenv.2019.133948
Feng, Z. H., Zhang, T., Wang, J. X., Huang, W., Wang, R., Xu, J. T., et al. (2020c). Spatio-temporal features of microplastics pollution in macroalgae growing in an important mariculture area, China. Sci. Total Environ. 719:137490. doi: 10.1016/j.scitotenv.2020.137490
Feng, Z. H., Zhang, T., Shi, H. H., Gao, K. S., Huang, W., Xu, J. T., et al. (2020b). Microplastics in bloom-forming macroalgae: distribution, characteristics and impacts. J. Hazard. Mater. 397:122752. doi: 10.1016/j.jhazmat.122752
Feng, Z. H., Wang, R., Zhang, T., Wang, J. X., Huang, W., Li, J., et al. (2020a). Microplastics in specific tissues of wild sea urchins along the coastal areas of northern China. Sci. Total Environ. 728:138660. doi: 10.1016/j.scitotenv.2020.138660
Foekema, E. M., De Gruijter, C., Mergia, M. T., Van Franeker, J. A., Murk, A. T. J., and Koelmans, A. A. (2013). Plastic in North Sea fish. Environ. Sci. Technol. 47, 8818–8824. doi: 10.1021/es400931b
Frias, J. P. G. L., Gago, J., Otero, V., and Sobral, P. (2016). Microplastics in coastal sediments from Southern Portuguese shelf waters. Mar. Environ. Res. 114, 24–30. doi: 10.1016/j.marenvres.2015.12.006
Gajahin, G. N. T., Jayan, D. M. S., Amararatne, Y., and Suchana, C. (2017). Effects of Microplastics on barnacles and wild bivalves in the eastern coast of Thailand: an approach to coastal zone conservation. Mar. Pollut. Bull. 124, 349–355. doi: 10.1016/j.marpolbul.2017.06.010
Gregory, M. R., and Ryan, P. G. (1997). “Pelagic plastics and other seaborne persistent synthetic debris: a review of Southern Hemisphere perspectives,” in Marine Debris–Sources, Impacts and Solutions, eds J. M. Coe and D. B. Rogers (New York, NY: Springer), 49–66. doi: 10.1007/978-1-4613-8486-1-6
Haward, M. (2018). Plastic pollution of the world’s seas and oceans as a contemporary challenge in ocean governance. Nat. Commun. 9:667. doi: 10.1038/s41467-018-03104-3
Hussain, N., Jaitley, V., and Florence, A. T. (2001). Recent advances in the understanding of uptake of microparticulates across the gastrointestinal lymphatics. Adv. Drug Deliv. Rev. 50, 107–142. doi: 10.1016/S0169-409X(01)00152-1
Isobe, A., Iwasaki, S., Uchida, K., and Tokai, T. (2019). Abundance of non-conservative microplastics in the upper ocean from 1957 to 2066. Nat. Commun. 10:417. doi: 10.1038/s41467-019-08316-9
Jabeen, K., Su, L., Li, J., Yang, D., Tong, C., Mu, J., et al. (2017). Microplastics and mesoplastics in fish from coastal and fresh waters of China. Environ. Pollut. 221, 141–149. doi: 10.1016/j.envpol.2016.11.055
Karami, A., Golieskardi, A., Choo, C. K., Romanoc, N., Yu, B. H., and Salamatinia, B. (2017). A high-performance protocol for extraction of microplastics in fish. Sci. Total Environ. 578, 485–494. doi: 10.1016/j.scitotenv.2016.10.213
Kolandhasamy, P., Su, L., Li, J., Qu, X., Jabeen, K., and Shi, H. H. (2018). Adherence of microplastics to soft tissue of mussels: a novel way to uptake microplastics beyond ingestion. Sci. Total Environ. 610–611, 635–640. doi: 10.1016/j.scitotenv.2017.08.053
Lebreton, L. C. M., Joost, V. D. Z., Damsteeg, J. W., Slat, B., Andrady, A., and Reisser, J. (2017). River plastic emissions to the world’s oceans. Nat. Commun. 8:15611. doi: 10.1038/ncomms15611
Li, H. X., Ma, L. S., Lin, L., Ni, Z. X., Xu, X. R., Shi, H. H., et al. (2018). Microplastics in oysters Saccostrea Cucullata, along the Pearl River Estuary, China. Environ. Pollut. 236, 619–625. doi: 10.1016/j.envpol.2018.01.083
Li, J. N., Qu, X. Y., Su, L., Zhang, W. W., Yang, D. Q., Kolandhasamy, P., et al. (2016). Microplastics in mussels along the coastal waters of China. Environ. Pollut. 214, 177–184. doi: 10.1016/j.envpol.2016.04.012
Lima, A. R. A., Costa, M. F., and Barletta, M. (2014). Distribution patterns of microplastics within the plankton of a tropical estuary. Environ. Res. 132, 146–155. doi: 10.1016/j.envres.2014.03.031
Lisbeth, V. C., and Colin, R. J. (2014). Microplastics in bivalves cultured for human consumption. Environ. Pollut. 193, 65–70. doi: 10.1016/j.envpol.2014.06.010
Mohsen, M., Wang, Q., Zhang, L. B., Sun, L. N., Lin, C. G., and Yang, H. S. (2019). Microplastic ingestion by the farmed sea cucumber Apostichopus japonicus in China. Environ. Pollut. 245, 1071–1078. doi: 10.1016/j.envpol.2018.11.083
Peng, G. Y., Zhu, B. S., Yang, D. Q., Su, L., Shi, H. H., and Li, D. D. (2017). Microplastics in sediments of the Changjiang Estuary, China. Environ. Pollut. 225, 283–290. doi: 10.1016/j.envpol.2016.12.064
Plastics Europe (2018). Plastics–The Facts 2017-An Analysis of European Plastics Production, Demand and Waste Data. Frankfurt: Plastics Europe.
Provencher, J. F., Vermaire, J. C., Avery-Gomm, S., Braune, B. M., and Mallory, M. L. (2018). Garbage in guano? Microplastic debris found in faecal precursors of seabirds known to ingest plastics. Sci. Total Environ. 644, 1477–1484. doi: 10.1016/j.scitotenv.2018.07.101
Qu, X. Y., Su, L., Li, H. X., Liang, M. Z., and Shi, H. H. (2018). Assessing the relationship between the abundance and properties of microplastics in water and in mussels. Sci. Total Environ. 621, 679–686. doi: 10.1016/j.scitotenv.2017.11.284
Rachel, W. O. (2018). Microplastics in Polar Regions: the role of long range transport. Curr. Opin. Environ. Sci. Health 1, 24–29. doi: 10.1016/j.coesh.2017.10.004
Rochman, C. M., Hoh, E., Kurobe, T., and The, S. J. (2013). Ingested plastic transfers hazardous chemicals to fish and induces hepatic stress. Sci. Rep. 3:3263. doi: 10.1038/srep03263
Sfriso, A. A., Tomio, Y., Rosso, B., Gambaro, A., Sfriso, A., Corami, F., et al. (2020). Microplastic accumulation in benthic invertebrates in Terra Nova Bay (Ross Sea, Antarctica). Environ. Int. 137:105587. doi: 10.1016/j.envint.2020.105587
Su, L., Deng, H., Li, B., Chen, Q., Pettigrove, V., Wu, C., et al. (2019). The occurrence of microplastic in specific organs in commercially caught fishes from coast and estuary area of East China. J. Hazard. Mater. 365, 716–724. doi: 10.1016/j.jhazmat.2018.11.024
Sun, X. X., Liang, J. H., Zhu, M. L., Zhao, Y. F., and Zhang, B. (2018). Microplastics in seawater and zooplankton from the Yellow Sea. Environ. Pollut. 242, 585–595. doi: 10.1016/j.envpol.2018.07.014
Teng, J., Wang, Q., Ran, W., Wu, D., Liu, Y. F., Sun, S., et al. (2019). Microplastic in cultured oysters from different coastal areas of China. Sci. Total Environ. 653, 1282–1292. doi: 10.1016/j.scitotenv.2018.11.057
Thompson, R., Olsen, Y., Mitchell, R., Davis, A., Rowland, S. J., John, A. W. G., et al. (2004). Lost at sea: where is all the plastic? Science 304:838. doi: 10.1126/science.1094559
Von Moos, N., Burkhardt-Holm, P., and Kohler, A. (2012). Uptake and effects of microplastics on cells and tissue of the blue mussel Mytilus edulis L. after an experimental exposure. Environ. Sci. Technol. 46, 11327–11335. doi: 10.1021/es302332w
Wright, S. L., Thompson, R. C., and Galloway, T. S. (2013). The physical impacts of microplastics on marine organisms: a review. Environ. Pollut. 178, 483–492. doi: 10.1016/j.envpol.2013.02.031
Xu, X. Y., Wong, C. Y., Tam, N. F. Y., Liu, H. M., and Cheung, S. G. (2020). Barnacles as potential bioindicator of microplastic pollution in Hong Kong. Mar. Pollut. Bull. 154:111081. doi: 10.1016/j.marpolbul.2020.111081
Zalasiewicz, J., Waters, C. N., Sul, J. I. D., Corcoran, P. L., and Yonan, Y. (2016). The geological cycle of plastics and their use as a stratigraphic indicator of the Anthropocene. Anthropocene 13, 4–17. doi: 10.1016/j.ancene.2016.01.002
Zhang, F., Wang, X. H., Xu, J. Y., Zhu, L. X., Peng, G. Y., Xu, P., et al. (2019). Food-web transfer of microplastics between wild caught fish and crustaceans in East China Sea. Mar. Pollut. Bull. 146, 173–182.
Zhang, T., Sun, Y. X., Song, K. X., Du, W. G., Huang, W., Gu, Z. Q., et al. (2021). Microplastics in different tissues of wild crabs at three important fishing grounds in China. Chemosphere 271:129479. doi: 10.1016/j.chemosphere.2020.129479
Zhao, J. M., Ran, W., Teng, J., Liu, Y. L., Liu, H., Yin, X. N., et al. (2018). Microplastic pollution in sediments from the Bohai Sea and the Yellow Sea, China. Sci. Total Environ. 64, 637–645. doi: 10.1016/j.scitotenv.2018.05.346
Zhao, S. Y., Danley, M., Ward, J. E., Li, D., and Mincer, T. J. (2016). An approach for extraction, characterization and quantitation of microplastic in natural marine snow using Raman microscopy. Anal. Methods 9, 1470–1478. doi: 10.1039/C6AY02302A
Zhu, L., Bai, H., Chen, B., Sun, X., Qu, K., and Xia, B. (2018). Microplastic pollution in North Yellow Sea, China: observations on occurrence, distribution and identification. Sci. Total Environ. 636, 20–29.
Keywords: microplastic, native oysters, mussels and barnacles, pollution, ecosystem
Citation: Zhang T, Song K, Meng L, Tang R, Song T, Huang W and Feng Z (2022) Distribution and Characteristics of Microplastics in Barnacles and Wild Bivalves on the Coast of the Yellow Sea, China. Front. Mar. Sci. 8:789615. doi: 10.3389/fmars.2021.789615
Received: 05 October 2021; Accepted: 09 December 2021;
Published: 13 January 2022.
Edited by:
Xiaoshan Zhu, Tsinghua University, ChinaCopyright © 2022 Zhang, Song, Meng, Tang, Song, Huang and Feng. This is an open-access article distributed under the terms of the Creative Commons Attribution License (CC BY). The use, distribution or reproduction in other forums is permitted, provided the original author(s) and the copyright owner(s) are credited and that the original publication in this journal is cited, in accordance with accepted academic practice. No use, distribution or reproduction is permitted which does not comply with these terms.
*Correspondence: Zhihua Feng, fengzhihua@jou.edu.cn
†These authors have contributed equally to this work and share first authorship