- 1Fisheries College, Jimei University, Xiamen, China
- 2Ornamental Aquarium Engineering Research Centre in Universities of Fujian Province, Xiamen, China
- 3Centre for Sustainable Tropical Fisheries and Aquaculture, College of Science and Engineering, James Cook University, Townsville, QLD, Australia
The paracalanid copepod, Bestiolina amoyensis, is a widely distributed species occurring in subtropical inshore waters across the Pacific Ocean. Its small size, herbivorous feeding habit, and high adaptability make the species one of the most promising candidates as potential live feed for hatchery larval rearing. This study investigated effects of different feeding density of microalgae Isochrysis spp. (1 × 105, 2 × 105, 3 × 105, 4 × 105, and 5 × 105 cells ml–1) and photoperiod (8L:16D, 12L:12D, and 16L:8D) on productivity-related parameters, including egg production, female life expectancy and population dynamics of B. amoyensis. Results showed that total egg output over female lifespan, final population size and intrinsic rate of population of 12L:12D photoperiod treatments were always the highest among three photoperiod conditions, especially at the food concentration of 4 × 105 cells ml–1, indicating B. amoyensis had high reproductive performance and the population was in a more stable status. The number of nauplii from 4 × 105 cells ml–1 algal concentration treatment accounted for 75% of the population, and the ratio of females to males approaching 1:1 when photoperiod was 12L:12D; female life expectancy was 10.5 ± 0.6 days. In conclusion, our results showed that Isochrysis spp. is a suitable feed for B. amoyensis with an optimal concentration at 4 × 105 cells mL–1; the optimal photoperiod for B. amoyensis rearing is 12L:12D. The relatively long reproductive lifespan and high intrinsic population increase rate make B. amoyensis a good candidate to develop culture techniques for hatchery larval rearing.
Introduction
Providing palatable nutritional food of appropriate size to marine larvae at different development stages is one of the most challenging tasks in marine hatcheries. For some larvae with small mouth gapes, the traditional live prey of rotifers (mean length 135 μm of SS-type rotifer Brachionus rotundiformis) and Artemia nauplii (typically between 400–500 μm) could be too large to be ingested by them (Fielder et al., 2000; Assavaaree et al., 2003; Gopakumar and Santhosi, 2009; Wullur et al., 2009; Conceição et al., 2010; Lindley et al., 2011; Hagiwara et al., 2014), and lacking of appropriate live prey to feed such larvae has been a crucial technical bottleneck. Copepods are the natural food for most marine larvae and nauplii of many copepods are less than 100 μm in size, which can meet the first feeding requirement of even small mouth- gaped fish larvae (McKinnon et al., 2003; Camus and Zeng, 2010; Bradley et al., 2013; Hill et al., 2020). Moreover, copepods typically develop through six naupliar and six copepodite stages with each stage of different size, making them suitable prey for fish larvae with different sizes of mouth gapes, as well as at different developmental stages (Schipp et al., 1999).
Several paracalanid species, including Parvocalanus crassirostris (Alajmi and Zeng, 2015) and Bestiolina similis (McKinnon et al., 2003; Camus et al., 2009; VanderLugt et al., 2009; Camus and Zeng, 2010), have been recommended as good candidates as larvae prey for fish larvae with small mouth gape. B. amoyensis, a species belonging to the same genus of B. similis, has been reported to co-exists with B. similis in the tropical coastal and estuary waters (McKinnon et al., 2003). The species distributes widely in Pacific Ocean, especially in western Pacific Ocean, and it is a dominant species during autumn (August – November) in subtropical Xiamen Harbor and Dongshan Bay of China (Yang, 2007; Zheng, 2009). However, no attempts have been made to culture the species as the live prey for feeding fish larvae previously.
Comparing to the traditional live prey, the main problem of copepods as live feed for larval culture is their difficulty for intensive culture and/or low culture productivity. Therefore, optimizing the culture conditions has been the focus of previous research. Many factors could affect copepod culture reproductivity and past research has been focused on temperature, salinity, and both quality and quantity of their food (e.g., Holste and Peck, 2006; Camus et al., 2009; Camus and Zeng, 2010; Nogueira et al., 2017; Nguyen et al., 2020; Choi et al., 2021; Dayras et al., 2021), while the effects of photoperiod is less studied despite photoperiod has been shown to significantly affect culture productivity of two Acartia species, i.e., Acartia tonsa (Peck and Holste, 2006) and Acartia sinjiensis (Camus and Zeng, 2008). Photoperiod is well known as one of the most important environmental factors regulating reproductive activity of aquatic animals, including copepods, and it has been reported to influence number of eggs produced by females, as well as the type (i.e., subitaneous vs. diapause eggs) of eggs produced (Marcus, 1982; Peck and Holste, 2006; Camus, 2012; Fereidouni et al., 2015). Furthermore, the physiological “clock” of aquatic animals allows them to response to changes in light condition, which could affect their feeding and reproductive activity (Miliou, 1992). For example, marine zooplankton, especially copepods, are known to undertake diurnal vertical migration (Haney, 1988) with a typical feeding pattern showing higher ingestion rate during night (Fuller, 1937; Petipa, 1958; Dagg and Grill, 1980; Stearns, 1986; Saito and Taguchi, 1996; Calbet et al., 1999), and some calaniod copepods, such as P. crassirostris (Sun, 2008) and Calanus (Harding et al., 1951; Zhang, 2003), spawn occurs more frequently at night. Hence, the goal of the current study was to evaluate the effects of photoperiod and microalgae feeding concentration and their interaction effects on culture productivity of B. amoyensis, seeking to answer the questions such as is there an optimum photoperiod regime for the cultivation of B. amoyensis? Whether there exist interactions between algae feeding concentration and photoperiod? For instance, whether longer darkness hours leading to enhanced egg production may require more abundant food supply to sustain higher productivity?
Materials and Methods
Microalgal Culture
The microalgae Isochrysis spp. was cultivated in a temperature-controlled room. Several 70 L cylindrical acrylic cylinders filled with 0.01 μm filtered, UV irradiated, and Chlorine treated seawater (salinity 28 psu) were used for the algae culture. All cultures were maintained at 25 ± 1°C with vigorous aeration and f/2 medium was used for the cultures (Guillard and Ryther, 1962). The photoperiod was set as constant light (24 h light) with a light intensity of approximately 4000–5000 Lux. The algal cultures in its exponential growth phase were used for feeding copepods.
Sampling and Isolation of Copepods
Bestiolina amoyensis were initially obtained from a plankton tow performed in coastal water of Jimei District, Xiamen City, Fujian Province, China, on May 20th, 2019. The plankton sample was immediately transported back to a laboratory at Jimei University for isolation of B. amoyensis.
To isolate B. amoyensis, healthy copepods were firstly attracted to a light source, they were then transferred by a pipette to several petri dishes, where they were sorted according to their morphological characteristics and movement mode. The B. amoyensis identified (Li and Huang, 1984; Lian et al., 2018) were gently pipetted individually to wells of a 12-well plate filled with autoclaved seawater, wells were then carefully checked to make sure there was no contamination of other animals. Female B. amoyensis, identified by their swollen genital segments, were further sorted and individually cultured in 12-well cell culture plates. The culture volume of each well was 5 ml with Isochrysis spp. added at 1 × 105 cells ml–1. Plates were incubated in a unit with light illustrated from down under. The light intensity was 500–800 Lux and photoperiod was set as 12 h L:12 h D.
Bestiolina amoyensis Stock Culture
Following separation, B. amoyensis cultures were gradually scaled up and eventually kept in several 300 L tanks filled with 0.01 μm filtered seawater and with gentle aeration. The salinity was 28 ± 1 psu and temperature was maintained at 26 ± 1°C. Light intensity was ∼ 500 Lux with a light: dark cycle of 12 h:12 h. Approximately 30% of the culture water was exchanged daily by gentle siphoning with a 5 cm diameter hollow cylindrical isolation device fitted to the front of the siphon tube, the bottom of which was covered with 48 μm mesh to prevent removal of copepods and reduce damage. B. amoyensis was fed daily with microalgae Isochrysis spp. at a concentration of 1 × 105 cells ml–1. Culture vessels were totally drained every 15 days for cleaning when adult copepods were caught with 500 μm special nets and juveniles with 75 μm nets, and the juveniles were transferred to culture carboys cleaned and sterilized with chlorine.
Experimental Design and Setup
A series of experiments were carried out to assess the influence of food concentration and photoperiod on the following parameters related to B. amoyensis culture productivity: (1) egg production, (2) adult female life expectancy, and (3) population growth over a 12 days culture period.
Production of Adult Copepods for the Experiment
For all experiments, mature B. amoyensis were retrieved with a special 500 μm sieve from stock culture and immediately transferred to a 2.5 L container with the bottom covered by a 75 μm mesh, the container was suspended in a 5 L transparent vessel containing 4 L of 0.01 μm filtered seawater. This design facilitated the separation of adult copepods and eggs produced by the females. After leaving the copepods to spawn for 6 h, each 2.5 L container containing the adult copepods was transferred to a new 5 L container, but the eggs produced by them were kept in the original 5 L container for incubation until hatching. The newly hatched nauplii were cultured for 3 days in the 5 L container before being transferred to an 18 L container for further cultivation until reaching the copepodite V stage (CV). The culture conditions and feeding were initially the same as those described for stock culture (i. e. 26 ± 1°C; 28 ± 1 psu) and the concentration of feeding algae was 1 × 105 cells mL–1. The last stage copepodites were then transferred to 30 ml Petri dishes and were monitored for molting daily with a microscope until they all developed to adults and ready for the following experiments. Adult B. amoyensis were transferred to a 1 L container containing filtered seawater and acclimated for 24 h to eliminate potential residual effects under the previous culture condition. The mixed population of both males and females during acclimation ensured that copepods used for the experiment were fertilized before being introduced into the Petri dishes for experiment.
Egg Production and Adult Female Life Expectancy Experiment
Following the acclimation, pairs of adult B. amoyensis that were actively swimming and with intact appendages were selected from the 1L beaker and carefully transferred to each of a 30 mL Petri dishes containing 20 mL seawater to monitor daily egg production. In the case that a male died during the experiment, a new male was introduced (Hall and Burns, 2001). If a female had not produced any eggs by the 3rd days of the experiment, a new female was introduced. Cultures were maintained in a constant temperature incubator and light intensity was set as ∼ 500 Lux.
This experiment used 5 × 3 factorial design with five food concentrations (1 × 105, 2 × 105, 3 × 105, 4 × 105 and 5 × 105 cells ml–1) and 3 photoperiod conditions (8 h:16 h, 12 h:12 h, and 16 h:8 h), hence a total of 15 treatments. With 12 replicate pairs of adult copepods per treatment, a total of 180 Petri dishes were set up. The copepods were starved for 24 h prior to the formal commencement of the trial. Microalgae in exponential growth phase were use in present experiment and five samples were measured daily using an automated cell counter (Count-star IA1000, Shanghai Ruiyu Biotech Co., Ltd.) to maintain the designated concentrations of the experiment. Constant temperature (26°C) incubators were used to set up three photoperiod conditions and the light intensity was ∼ 500 Lux. During the experiment, each pair of copepods were removed from the Petri dish and transferred to new one with fresh seawater and microalgae supplied at the designated concentration every 24 h. The egg production was monitored and recorded using a Leica DMi8 microscope. Any deaths over the 24 h period was also recorded. Experiment was continued until all copepods in a treatment had died, and the mean life expectancy of adult B. amoyensis in the treatment was obtained by averaging individual lifespans all 12 replicates of the treatment. The daily egg production and the total egg production over female lifespan for each treatment was calculated by averaging the data obtained from 12 replicates (Camus and Zeng, 2010).
Population Growth Experiment
The same 5 × 3 factorial design of 5 food concentrations and 3 photoperiod conditions was used for the population growth experiment, which lasted for 12 days. There were three replicates per treatment. Adult copepods were produced as described in the section “Production of Adult Copepods for the Experiment.” After acclimation, 12 B. amoyensis adults (4 males and 8 females) were introduced into each of a series of 45 × 1 L beakers (each 1 L beaker as a replicate) to start the experiment. Cultures were maintained in an incubator with constant temperature (∼ 26°C), salinity 28 psu, and a light intensity of ∼ 500 Lux over 12 days. Approximately 30% of the culture water was exchanged daily by gently siphoning using the cylindrical isolation device described above.
After 12 days of culture, the contents of each beaker were emptied onto a 48 μm sieve, all eggs, nauplii, copepodites and adults retained on the sieve were subsequently rinsed into Petri dishes, and 4–5% formalin was added to fix them for later counting. Eggs, nauplii, copepodites and adults of B. amoyensis were counted in each replicate and the final population size averaged from three replicates. Intrinsic rate (r) of population growth was then calculated for each treatment using the following formulation:
where N0 = population number at the beginning of the experiment, N1 = population number at the end of the experiment while t (days) is the duration of the experiment (Fenchel, 1974). Meanwhile, adults were sexed to yield the sex ratio of the population.
Data Analysis
Data are presented as mean ± standard error (SE). A statistical probability of p < 0.05 was accepted as significant in all tests. The effects of food concentration and photoperiod and their interaction on egg production, female life expectancy and population dynamics were analyzed using two-way ANOVA. All data were tested for normality (Shapiro-Wilk test) and homogeneity of variance (Levene’s test) prior to analysis of ANOVA. When significant interaction between the two factors of food concentration and photoperiod was found, the Tukey’s multiple comparisons test was performed to determine specific significant differences among treatments. If the assumption of normality was not met, the correlation between two variables was tested by the nonparametric Scheirer-Ray-Hare test (daily egg production data).
Results
Daily Egg Production
Figure 1 shows average daily egg production of B. amoyensis when cultured under various combinations of microalgae concentrations and photoperiods. Statistical analysis showed that there was an interaction effect between food concentration and photoperiod on daily egg production (p < 0.001). However, there was no significant effects among different photoperiods at all 5 diet concentrations (p > 0.05, Figure 1). On the other hand, the average daily egg production of the diet concentration treatment of 1 × 105 and 2 × 105 cells ml–1 were significantly lower than that of 3 × 105, 4 × 105, and 5 × 105 cells ml–1 treatment (p < 0.01) under all 3 photoperiod regimes (Figure 1).
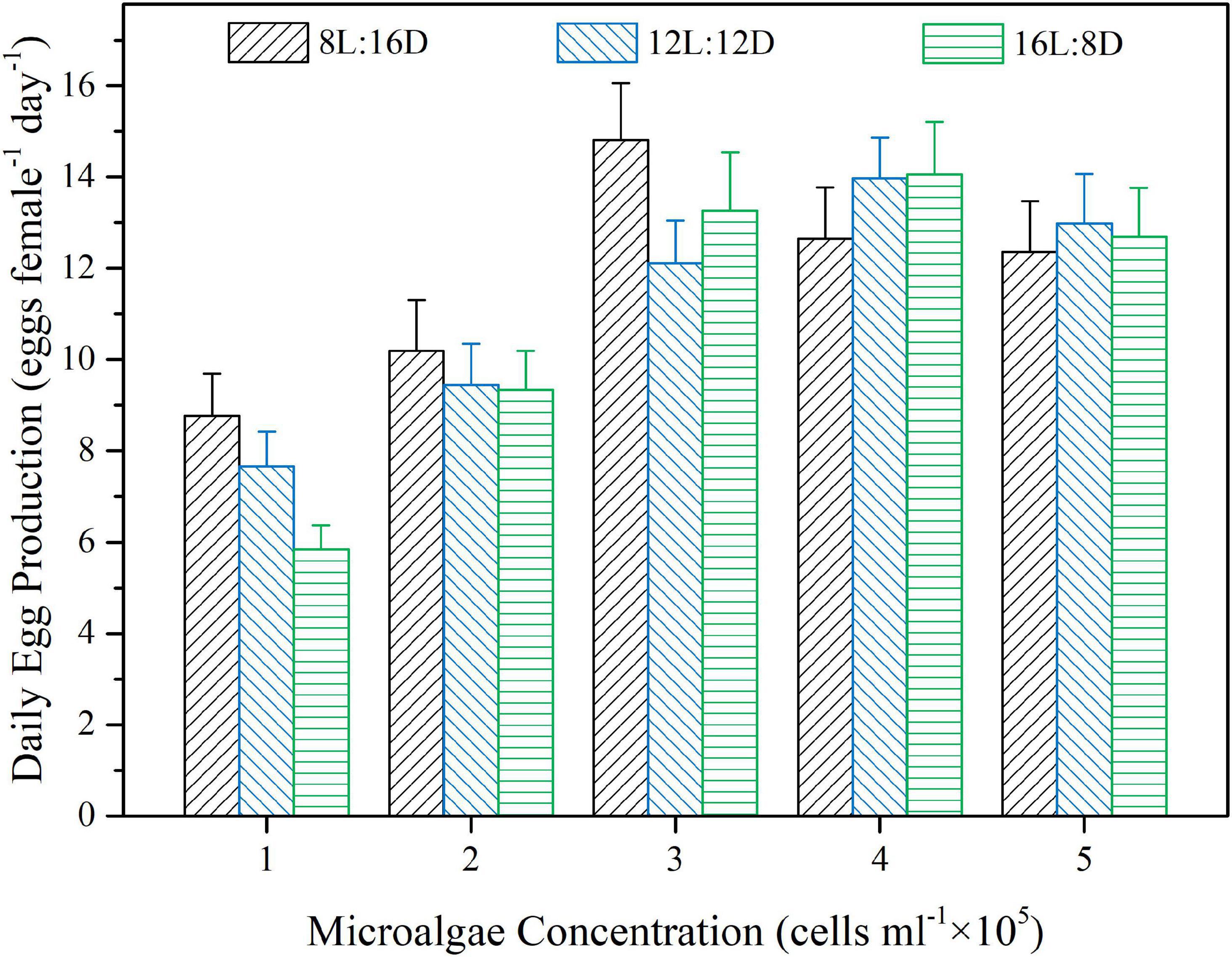
Figure 1. Effects of different concentrations of the microalgal diet and photoperiods on average daily egg production of Bestiolina amoyensis (N = 12). Data is presented as mean ± standard error (SE).
In term of total egg production over female lifespan, both food concentration (p < 0.001) and photoperiod (p < 0.001) had a main effect, and there was also a significant interaction effect between food concentration and photoperiod on the total egg production (p < 0.05, two-way ANOVA). Under all 3 photoperiods tested, the total egg production over female lifespan increased continuously with increasing food concentration, and reached a peak at 4 × 105 cells ml–1 (p < 0.05), however, the trend reversed as microalgae concentration further increased to 5 × 105 cells ml–1 (p < 0.05, Figure 2). Indeed, copepod from the 4 × 105 cells ml–1 treatment produced the highest number of eggs under all 3 photoperiod conditions, which was 2.3 times higher than the lowest value of the 1 × 105 cells ml–1 treatment (Figure 2). Similarly, photoperiod also had a significant effect on the total egg production of female at various food concentrations (p < 0.001): At microalgae concentration of 3 × 105, 4 × 105, and 5 × 105 cells ml–1, the highest egg production was all found under the photoperiod of 12 h L:12hD, followed by the photoperiod of 8 h L: 16 h D, while the 16 h L:8 h D photoperiod always had the lowest egg production (Figure 2). Overall, the highest total egg production over female lifespan was found under the combination of 4 × 105 cells ml–1 microalgae and 12 h L:12 h D photoperiod, which reached 139.7 ± 4.4 eggs female–1 (Figure 2).
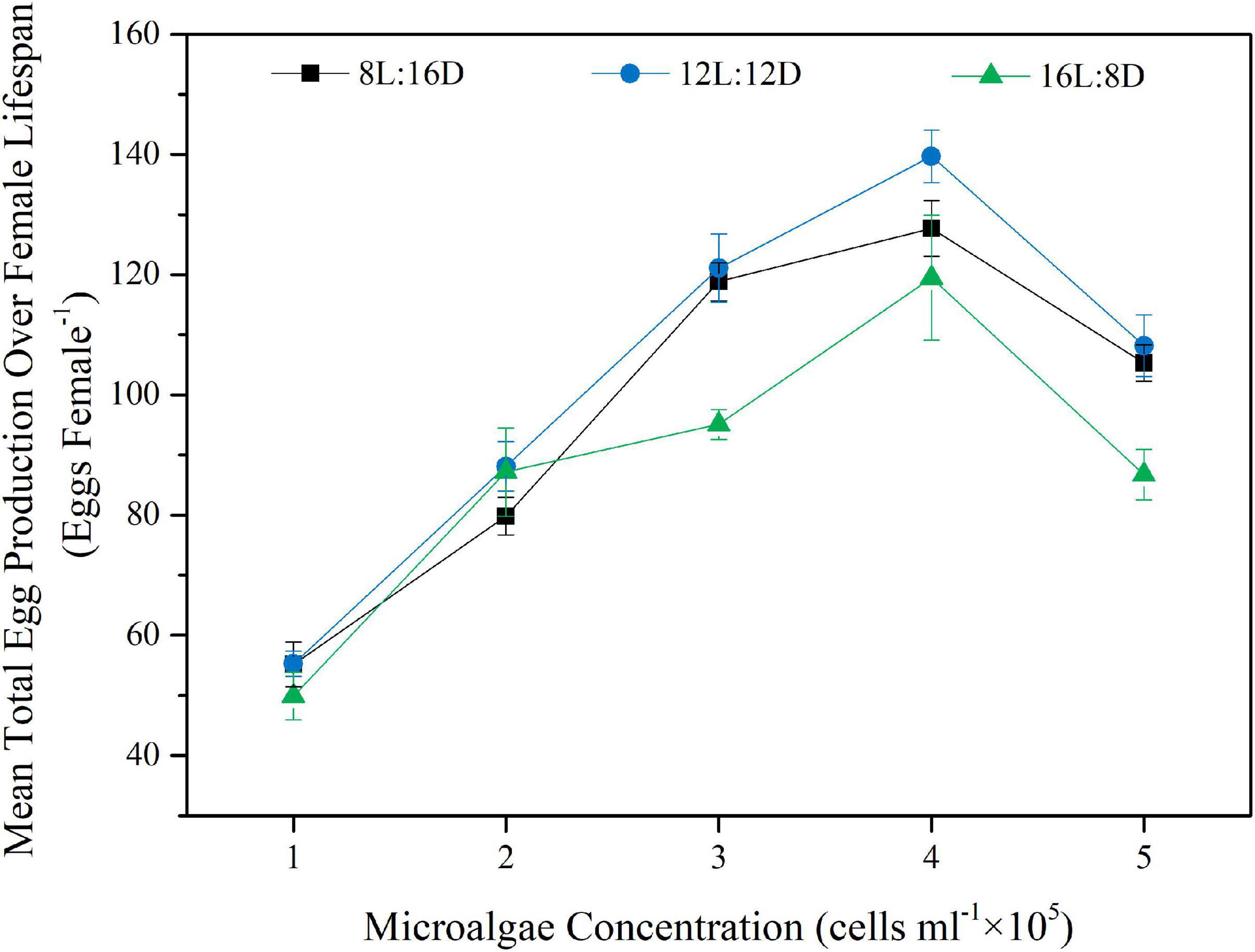
Figure 2. Effects of different concentrations of the microalgal diet and photoperiods on total egg production over female lifespan of B. amoyensis (N = 12). Data is presented as mean ± SE.
Adult Female Life Expectancy
Both food concentration and photoperiod significantly impacted female lifespan of B. amoyensis, and there was a significant interaction between the two factors (p < 0.01, Figure 3). The female life expectancy of 12L:12D photoperiod was not significantly different from that of other 2 photoperiod groups (p > 0.05) in 1 × 105 cells mL–1 group, while that of 16L:8D photoperiod group (11 ± 0.8 d) was significantly higher than that of the 8L:16D photoperiod group (8 ± 1.4 d) (p < 0.01). In 3 × 105cells mL–1 group, the female life expectancy of the 12L:12D photoperiod group (10 ± 2.1 d) was significantly higher than that of the other 2 photoperiod groups (p < 0.05), and the difference between 8L:16D photoperiod and 16L:8D photoperiod groups was not significant (p > 0.05). When food concentrations were 2 × 105cells mL–1, 4 × 105cells mL–1 and 5 × 105cells mL–1, the female life expectancy from 3 photoperiod groups did not differ significantly (p > 0.05, Figure 3).
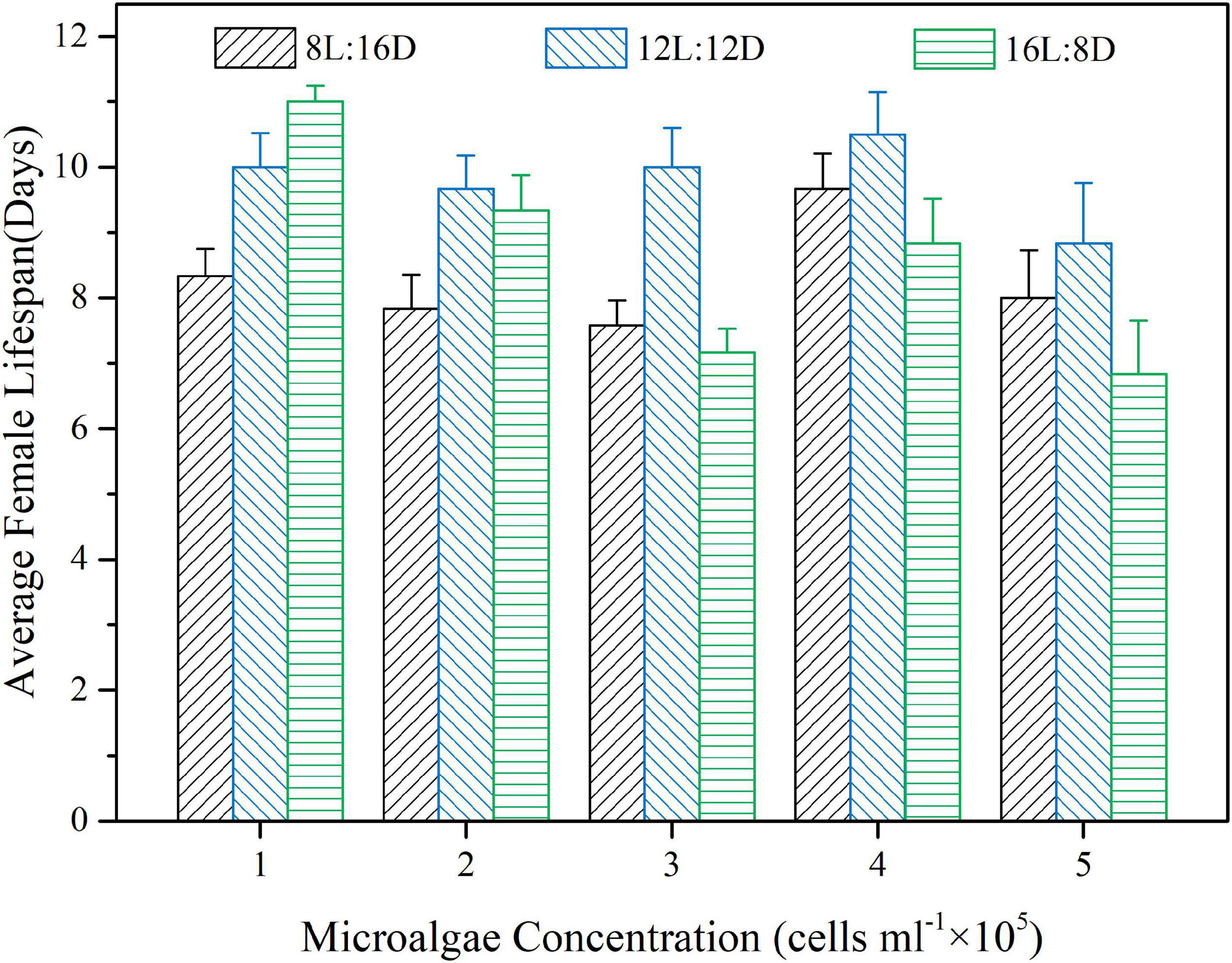
Figure 3. Effects of different concentrations of the microalgal diet and photoperiods on female lifespan of B. amoyensis (N = 12). Data is presented as mean ± SE.
There was no statistical difference among different food concentrations under 8L: 16D and 12L: 12D photoperiods for female life expectancy (p > 0.05, Figure 3). Under the photoperiod regime of 16L:8D, the female lifespan of the 1 × 105 cells mL–1 diet treatment was not significantly different from that of the 2 × 105 cells mL–1, and 4 × 105 cells mL–1 diet treatments (p > 0.05), but significantly higher than those of the 3 × 105 cells mL–1, and 5 × 105 cells mL–1 food concentrations (p < 0.01, Figure 3). At the same time, the female life expectancy of the 2 × 105 cells mL–1 treatment was significantly higher than that of the 5 × 105 cells mL–1 treatment (p < 0.05) although both of them were not significantly different from that of the 3 × 105 cells mL–1, and 4 × 105 cells mL–1 treatments (p > 0.05, Figure 3).
Population Growth and Composition
The average final population sizes of B. amoyensis after 12 days of culture under different combinations of food concentrations and photoperiods are presented in two categories: “All Stages Included”, which included eggs, and “All Post-Egg-Stages”, which excluded eggs (Table 1). For “All Stages Included” final population, statistical analysis showed that the final population sizes were significantly affected by both food concentration (p < 0.01) and photoperiod (p < 0.01), and there was an interaction effect between food concentration and photoperiod (p < 0.001).
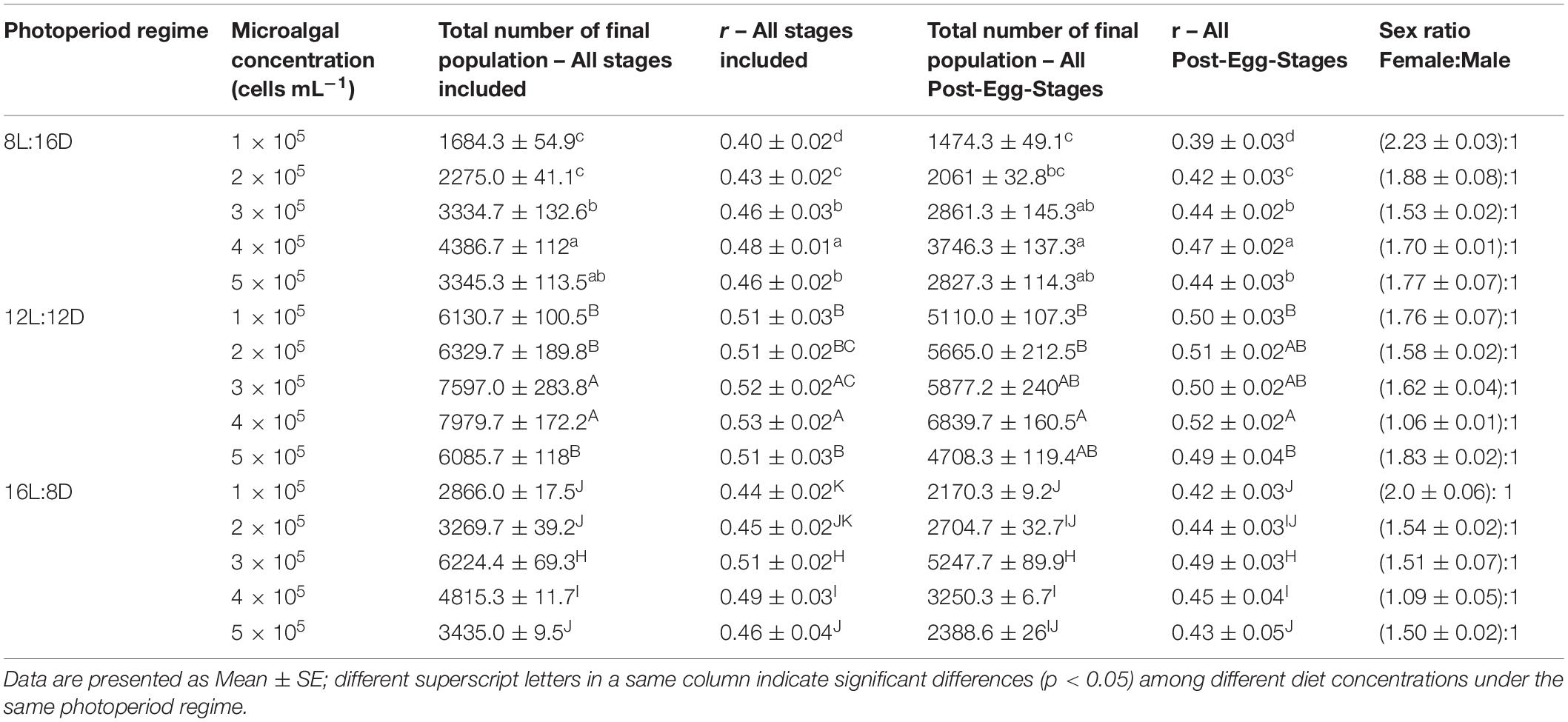
Table 1. Final population size and intrinsic rate (r) of B. amoyensis cultured over a 12 days period fed on different microalgal diets under three photoperiod regimes.
Of the 3 photoperiods tested, at a same algal concentration, the 12 h L:12 h D photoperiod always produced the largest and significantly bigger final population than those of the other two photoperiods (p < 0.01, Figure 4). Meanwhile, under both 8 h L:16 h D and 12 h L:12 h D photoperiods, population size increased with increasing food concentration, and reached a peak at 4 × 105 cells ml–1 (4386.7 ± 112 and 7979.7 ± 172.2 for photoperiod 8 h L:16 h D and 12 h L:12 h D, respectively) before dropped when food concentration further increased to 5 × 105 cells ml–1 (Figure 4). At photoperiod regime of 16 h L:8 h D, population size similarly increased significantly with increasing algal concentration but the maximum population size was observed at microalgae concentration of 3 × 105 cells ml–1 as population decreased significantly as algal concentration further increased to 4 × 105 and 5 × 105 cells ml–1, respectively (Figure 4). It is worth noting that levels when compared to the other two photoperiods, at all food concentrations the final population of the photoperiod 12 h L:12 h D remained at relatively high levels (Figure 4).
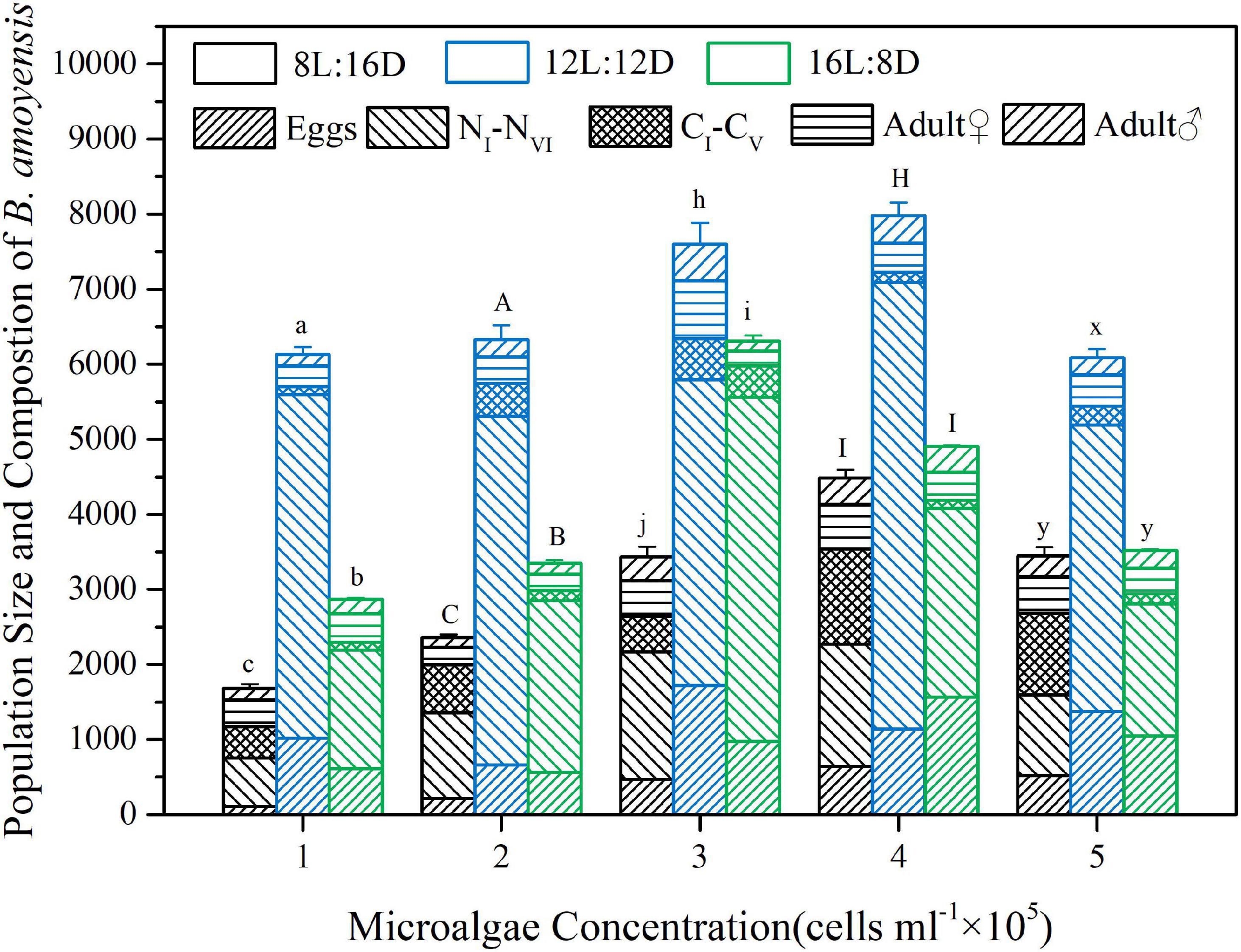
Figure 4. Effects of different concentrations of the microalgal diet and photoperiod regimes on population size and population structure of B. amoyensis over 12 days of cultivation (N = 3). Data is presented as mean ± SE. Different letters on the top of bars indicate significant differences of different photoperiod treatments at the same microalgae feeding concentration (p < 0.05).
Figure 4 shows final population composition of B. amoyensis (eggs, nauplii, copepodites, female and male adults) at the end of the experiment. The maximum amount of nauplii and copepodite was reached under photoperiod of 8L:16D in all diet treatments (Figure 4, Table 1). Under photoperiod 12 h L:12 h D and 16 h L:8 h D, nauplii stage accounted for more than 50% of the population size. In particular, under the culture condition of photoperiod 12 h L:12 h D and 4 × 105 cells ml–1 algal feeding concentration, nauplii accounted for 75% of the total population (Figure 4).
For the female to male sex ratio, under photoperiod 8 h L:16 h D, it ranged from 1.5:1 of 3 × 105 cells ml–1 food concentration to 2.2:1 of 1 × 105 cells ml–1 food concentration (Table 1). Under both photoperiods 12 h L:12 h D and 16 h L:8 h D, the lowest female to male sex ratio was both 1.1:1 and from the 4 × 105 cells ml–1 food concentration treatment. For other food concentration treatments, the sex ratio ranged from 1.6:1 to 1.8 and 1.5:1 to 2.0:1 under photoperiod 12 h L:12 h D and 16 h L:8 h D, respectively (Table 1).
It is worth noting that both food concentration and photoperiod had a significantly effect on the intrinsic population increase rates(r) of B. amoyensis (p < 0.001). The intrinsic rates under photoperiod 12 h L:12 h D was significantly higher than that of other 2 photoperiods (p < 0.01) at all 5 food concentrations. The intrinsic rates of 8L:16D photoperiod treatment was significantly lower than that of other 2 photoperiods in 1 × 105 cells ml–1 to 3 × 105 cells ml–1 food concentrations (p < 0.001), while at 4 × 105 cells ml–1 and 5 × 105 cells ml–1 food concentrations, the intrinsic population increase rates(r) of 8L:16D photoperiod group were not significantly different from that of 16L:8D photoperiod (Table 1). When all stages were included, the intrinsic rates of 12 h L:12 h D photoperiod ranged from 0.51 to 0.53, the highest r value was reached at food concentration of 4 × 105 cells ml–1, which was significantly higher than other food concentration treatments except the 3 × 105 cells ml–1 treatment. On the other hand, the intrinsic rates of under photoperiods 8 h L:16 h D and 16 h L:8 h D were ≤0.48 (4 × 105 cells ml–1) and 0.51 (3 × 105 cells ml–1), respectively (Table 1). Similar trend was shown when eggs were excluded with the intrinsic rates ranged from 0.39 to 0.47, 0.49 to 0.52, and 0.42 to 0.49 for photoperiod of 8 h L:16 h D, 12 h L:12 h D, and 16 h L:8 h D, respectively (Table 1).
Discussion
Reproductive ability of copepods is affected by the nutritional condition of mature females (Castro-Longoria, 2003), and food quantity is an important factor that can significantly affect female condition. For example, the egg production has been reported to typically increase with the food concentration, reach a maximum level at a certain concentration (Runge, 1984; Hirche et al., 1997; Camus, 2012), but tend to decrease when the food concentration exceeds a threshold level (Zhao et al., 2014). Therefore, determining the optimal microalgae feeding quantity is crucial for optimizing the culture protocol of B. amoyensis.
Most of paracalanid copepods are herbivorous (Paffenhöfer, 1984) although a few of them have been reported as could be carnivorous (Suzuki et al., 1999). Similar to its congener B. similis (Vineetha et al., 2018; Siddique et al., 2021), we found B. amoyensis as herbivorous, feeding mainly on phytoplankton. In the present study, the microalgae Isochrysis spp., one of the most commonly used marine unicellular algae for feeding calanoid copepods (Payne and Rippingale, 2000; Vengadeshperumal et al., 2010; Santhanam et al., 2013; El-Tohamy et al., 2021), was confirmed could fulfill the growth and reproduction needs of B. amoyensis. Isochrysis spp. is known for their high nutritional value, such as rich in polyunsaturated fatty acids (PUFAs), e.g., DHA and EPA (Sukenik and Wahnon, 1991; Gouveia et al., 2008) and grows well in mass cultures, either indoors or outdoors (Kaplan et al., 1986; Fidalgo et al., 1998). When at exponential phase of growth, the flagellated microalgae are not easily accumulated at the bottom like some other microalgae species, such a feature benefited the present experiments: the concentrations of Isochrysis spp. largely remained at designated levels during the 24 h period between daily water changes as confirmed by intermittent sampling of culture waters from replicates to estimate the algal concentrations from different treatments (see Supplementary Table 1).
Copepods productivity has commonly been indicated by daily egg production of females (Klepper et al., 1998). However, Camus and Zeng (2010) have adopted total egg production over female lifespan to estimate female productivity, and noted that this method is more accurate since it takes female life expectancy and reproductivity changes during lifespan into account. In this study, we have measured both daily egg production and egg production over female lifespan to provide a comprehensive information on egg production of B. amoyensis under different culture conditions. Our results showed that under all 3 photoperiods used, average daily egg production of B. amoyensis was significantly higher when they were fed Isochrysis spp. at a concentration ≥3 × 105 cells ml–1 when compared to that at ≤2 × 105 cells ml–1. In the case of total egg production over female lifespan, under all photoperiods used, the lowest total egg production was found in the lowest algal concentration of 1 × 105 cells mL–1, and total egg production increased by the algal concentration, reaching a maximum level at 4 × 105 cells mL–1 before decreased with further increase of food concentration. Similar results were also reported for other copepod species, such as Calanus pacificus (Runge, 1984), Calanus finmarchicus (Hirche et al., 1997), and Tisbe furcate (Zhao et al., 2014). Such a result likely can be explained by that when food concentration is low, copepods need to move actively to catch more algae and with increase movement frequency of feeding appendage to enhance feeding (Paffenhöfer and Lewis, 1990), which increases energy expenditure. As the result, less energy can be channeled for egg production. Indeed, it has been observed that in some calanoids, clearance rate, i.e., the water volume sweeps clear by a copepod per unit time, increased with decreasing food concentration (Corner et al., 1972). Clearly, clearance rate increase is associated with higher energy expenditure. With higher food concentrations, copepods could obtain enough food with a lower clearance rate, which reduces energy consumption while still meeting the energy demands for both maintenance and reproduction (Zhao et al., 2014). However, when the food concentration is too high, it was found that microalgae could adhere to the feeding appendages and body surface of copepods, which could impair their feeding and motility, leading to lower food ingestion, and subsequent lower egg output and shorter female life expectancy. While Garrido et al. (2013) found that ingestion rate of calanoid copepod Centropages chierchiae increased linearly with the range of food concentrations they used (6.4–393.8 μC L–1), but out results suggest that the ingestion rate of B. amoyensis does not increase infinitely with food concentration and a threshold exists, beyond which the clearance rate would not further increase, or even decrease.
Female life expectancy is one of the factors that could affect how much a female contributes to the total pool of eggs produced in the culture, and hence culture population growth. When food concentrations were ≥3 × 105 cells mL–1, adult females cultured under 16 h L:8 h D photoperiod had a shorter life expectancy than other two photoperiods. The prolonged light phase likely kept B. amoyensis active for longer, and the higher metabolic rate may result in shorter female lifespan. Similarly, A. sinjiensis cultured under constant light had a relative shorter life expectancy than other photoperiods (Camus and Zeng, 2008).
The results of population growth experiment showed that feeding on Isochrysis spp. alone, B. amoyensis could significantly expanded its population at all concentrations tested, ranging from 1 × 105 to 5 × 105 cells ml–1 and under different photoperiods. Interestingly, at all 5 algal feeding concentrations, B. amoyensis cultured under 12 h L:12 h D photoperiod consistently had the maximum and significantly bigger population sizes at the end of the experiment than other two photoperiods, indicating the important of photoperiod in regulating population growth. A possible explanation of such a result is that 12 h L:12 h D mimics typical photoperiod of Xiamen region during autumn when B. amoyensis reportedly is a dominant copepod species in Xiamen Harbor of China (Yang, 2007) where B. amoyensis used in this study were collected from. Indeed, the photoperiod of Xiamen region in spring and autumn ranges from 12 h L:12 h D, hence B. amoyensis may have adapted to such a photoperiod condition for maximum production. Indeed, under 12 h L:12 h D photoperiod, female B. amoyensis typically had the longest life expectancy among 3 photoperiods except at 1 × 105 cells ml–1 algal concentration, which was also not significant different from the longest life expectancy under 16 h L:8 h D. This should also lead to higher overall egg production while suggesting that 12 h L:12 h D is in fact optimal photoperiod for B. amoyensis.
The relative numbers of nauplii and copepodites in a culture population typically indicate the status of the culture (VanderLugt et al., 2009). VanderLugt and Lenz (2009) pointed out that populations dominated by nauplii typically indicate high reproductive rates of females. The maximum numbers and proportion of nauplii in the final populations of the population growth experiment were also found from the 12 h L:12 h D photoperiod, and at all 5 algae concentrations exceeding 50% of the total population. In particular, the number of nauplii of the 4 × 105 cells mL–1 treatment achieved 75% (Figure 3), indicating that B. amoyensis had a high reproductive rate under 12 h L:12 h D photoperiod and when fed Isochrysis spp. at 4 × 105 cells mL–1.
Sex-determining in copepods are related to the population size and sex ratio (Mauchline, 1998; Voordouw and Anholt, 2002). In planktonic calanoid copepods, sex may also be controlled by environmental factors (Mauchline, 1998). The female-biased adult sex ratios had been reported in many species, including Acrocalanus sp., Parvocalanus sp., Paracalanus indicus, B. similis (McKinnon and Duggan, 2001; McKinnon et al., 2005; Duggan et al., 2008; Gusmão and McKinnon, 2009), Paracalanus nanus (McKinnon and Duggan, 2001; Gusmão and McKinnon, 2009), and Paracalanus aculeatus (McKinnon and Duggan, 2001; McKinnon et al., 2005; Gusmão and McKinnon, 2009). The sex ratios of Calanus spp. vary seasonally, and the food quality and quantity could also significantly affect sex ratio. For example, more males could be observed during the phytoplankton boom season comparing to the pre-phytoplankton boom season (Irigoien et al., 2000). Similarly, there were more male B. amoyensis found in the populations of 4 × 105 cells mL–1 treatments under 12 h L:12 h D photoperiod with the sex ratio approaching 1:1, indicating the culture population was at a stable status (Fisher, 1930) under the culture condition.
Conclusion
The results of this study showed that Isochrysis spp. is a suitable diet for B. amoyensis culture as the population expanded significantly when it is fed to the copepod at concentrations ranging from 1 × 105 to 5 × 105 cells ml–1, and under photoperiods of 8 h L:16 h D, 12 h L:12 h D and 16 h L:8 h D. However, summarizing the outcomes of a range productivity related parameters measured in B. amoyensis, including egg production, female life expectancy, and population growth and composition, the optimum Isochrysis spp. feeding concentration and photoperiod for the culture productivity of the species was determined to be 4 × 105 cells mL–1 and 12 h L:12 h D, respectively.
Data Availability Statement
The original contributions presented in the study are included in the article/Supplementary Material, further inquiries can be directed to the corresponding author.
Author Contributions
SW and LW conceived the original idea of this study and designed the experiments. LW conducted the experiments and collected the data. LW, SW, and CsZ performed the data analyses. LW wrote the draft of the article and revised it with SW and CsZ. YW and CxZ attended the field works and performed part of data collection. SW supervised the research. All authors contributed to the article and approved the submitted version.
Funding
This work was contribution to the Regional Demonstration Project of the 13th Five-Year Plan of Marine Economy Innovation & Development in Xiamen (funded by the State Oceanic Administration, People’s Republic of China, Grant No. 16PZY002SF18).
Conflict of Interest
The authors declare that the research was conducted in the absence of any commercial or financial relationships that could be construed as a potential conflict of interest.
Publisher’s Note
All claims expressed in this article are solely those of the authors and do not necessarily represent those of their affiliated organizations, or those of the publisher, the editors and the reviewers. Any product that may be evaluated in this article, or claim that may be made by its manufacturer, is not guaranteed or endorsed by the publisher.
Acknowledgments
We thank Qiaoqi Luo from Fujian Institute of Oceanography for kindly providing the original strain of Isochrysis spp.
Supplementary Material
The Supplementary Material for this article can be found online at: https://www.frontiersin.org/articles/10.3389/fmars.2021.788744/full#supplementary-material
Supplementary Table 1 | Concentrations of Isochrysis spp. from five different treatment groups after 24 h of incubation with the experimental Bestiolina amoyensis.
References
Alajmi, F., and Zeng, C. (2015). Evaluation of microalgal diets for the intensive cultivation of the tropical calanoid copepod, Parvocalanus crassirostris. Aquac. Res. 46, 1025–1038. doi: 10.1111/are.12254
Assavaaree, M., Hagiwara, A., Kogane, T., and Arimoto, M. (2003). Effect of temperature on resting egg formation of the tropical SStype rotifer Brachionus rotundiformis Tschugunoff. Fish. Sci. 69, 520–528. doi: 10.1046/j.1444-2906.2003.00652.x
Bradley, C. J., Strickler, J. R., Buskey, E. J., and Lenz, P. H. (2013). Swimming and escape behavior in two species of calanoid copepods from nauplius to adult. J. Plankton Res. 35, 49–65. doi: 10.1093/plankt/fbs088
Calbet, A., Saiz, E., Irigoien, X., Alcaraz, M., and Trepat, I. (1999). Short communication. Food availability and diel feeding rhythms in the marine copepods Acartia grani and Centropages typicus. J. Plankton Res. 21, 1009–1015. doi: 10.1093/plankt/21.5.1009
Camus, T. (2012). The Improvement of Copepods Intensive Culture Protocols as Live Feeds for Aquaculture Hatcheries. Ph.D. thesis. Townsville, QLD: James Cook University.
Camus, T., and Zeng, C. (2008). Effects of photoperiod on egg production and hatching success, naupliar and copepodite development, adult sex ratio and life expectancy of the tropical calanoid copepod Acartia sinjiensis. Aquaculture 280, 220–226. doi: 10.1016/j.aquaculture.2008.05.008
Camus, T., and Zeng, C. (2010). Roles of microalgae on total egg production over female lifespan and egg incubation time, naupliar and copepodite survival, sex ratio and female life expectancy of the copepod Bestiolina similis. Aquac. Res. 41, 1717–1726. doi: 10.1111/j.1365-2109.2010.02565.x
Camus, T., Zeng, C., and McKinnon, A. D. (2009). Egg production, egg hatching success and population increase of the tropical paracalanid copepod, Bestiolina similis (Calanoida: Paracalanidae) fed different microalgal diets. Aquaculture 297, 169–175. doi: 10.1016/j.aquaculture.2009.09.018
Castro-Longoria, E. (2003). Egg production and hatching success of four Acartia species under different temperature and salinity regimes. J. Crust. Biol. 23, 289–299. doi: 10.1163/20021975-99990339
Choi, S. Y., Lee, E. H., Soh, H. Y., and Jang, M. C. (2021). Effects of temperature and salinity on egg production, hatching, and mortality rates in Acartia ohtsukai (Copepoda, Calanoida). Front. Mar. Sci. 8:704479. doi: 10.3389/fmars.2021.704479
Conceição, L. E., Yúfera, M., Makridis, P., Morais, S., and Dinis, M. T. (2010). Live feeds for early stages of fish rearing. Aquac. Res. 41, 613–640. doi: 10.1111/j.1365-2109.2009.02242.x
Corner, E. D. S., Head, R. N., and Kilvington, C. C. (1972). On the nutrition and metabolism of zooplankton. VIII. The grazing of Biddulphia cells by Calanus helgolandicus. J. Mar. Biol. Assoc. U. K. 52, 847–861. doi: 10.1017/S0025315400040595
Dagg, M. J., and Grill, D. W. (1980). Natural feeding rates of Centropages typicus females in the New York Bight. Limnol. Oceanogr. 25, 597–609. doi: 10.4319/lo.1980.25.4.0597
Dayras, P., Bialais, C., Sadovskaya, I., Lee, M. C., Lee, J. S., and Souissi, S. (2021). Microalgal diet influences the nutritive quality and reproductive investment of the cyclopoid copepod Paracyclopina nana. Front. Mar. Sci. 8:697561. doi: 10.3389/fmars.2021.697561
Duggan, S., McKinnon, A. D., and Carleton, J. H. (2008). Zooplankton in an Australian tropical estuary. Estuaries Coasts 31, 455–467. doi: 10.1007/s12237-007-9011-x
El-Tohamy, W., Qin, J., Abdel-Aziz, N., El-Ghobashy, A., and Dorgham, M. (2021). Suitable algal species and density for the culture of copepod Gladioferens imparipes as a potential live food for fish larvae. Aquac. Int. 29, 105–125. doi: 10.1007/s10499-020-00614-0
Fenchel, T. (1974). Intrinsic rate of natural increase: the relationship with body size. Oecologia 14, 317–326. doi: 10.1007/BF00384576
Fereidouni, A. E., Meskar, S., and Asil, S. M. (2015). Effects of photoperiod on offspring production, development and generation time, survival, adult sex ratio and total life span of freshwater cyclopoid copepod, Mesocyclops sp.: comments on individual variations. Aquac. Res. 46, 163–172. doi: 10.1111/are.12171
Fidalgo, J. P., Cid, A., Torres, E., Sukenik, A., and Herrero, C. (1998). Effects of nitrogen source and growth phase on proximate biochemical composition, lipid classes and fatty acid profile of the marine microalga Isochrysis galbana. Aquaculture 166, 105–116. doi: 10.1016/S0044-8486(98)00278-6
Fielder, D. S., Purser, G. J., and Battaglene, S. C. (2000). Effect of rapid changes in temperature and salinity on availability of the rotifers Brachionus rotundiformis and Brachionus plicatilis. Aquaculture 189, 85–99. doi: 10.1016/S0044-8486(00)00369-0
Fuller, J. L. (1937). The feeding rate of Calanus finmarchicus in relation to environmental conditions. Biol. Bull. 72, 233–246. doi: 10.2307/1537257
Garrido, S., Cruz, J., Santos, A. M. P., Ré, P., and Saiz, E. (2013). Effects of temperature, food type and food concentration on the grazing of the calanoid copepod Centropages chierchiae. J. Plankton Res. 35, 843–854. doi: 10.1093/plankt/fbt037
Gopakumar, G., and Santhosi, I. (2009). Use of copepods as live feed for larviculture of damselfishes. Asian Fish. Sci. 22, 1–6. doi: 10.33997/j.afs.2009.22.1.001
Gouveia, L., Coutinho, C., Mendonça, E., Batista, A. P., Sousa, I., Bandarra, N. M., et al. (2008). Functional biscuits with PUFA−ω3 from Isochrysis galbana. J. Sci. Food Agric. 88, 891–896. doi: 10.1002/jsfa.3166
Guillard, R. R., and Ryther, J. H. (1962). Studies of marine planktonic diatoms: I. Cyclotella nana Hustedt, and Detonula confervacea (Cleve) Gran. Can. J. Microbiol. 8, 229–239. doi: 10.1139/m62-029
Gusmão, L. F. M., and McKinnon, A. D. (2009). Sex ratios, intersexuality and sex change in copepods. J. Plankton Res. 31, 1101–1117. doi: 10.1093/plankt/fbp059
Hagiwara, A., Wullur, S., Marcial, H. S., Hirai, N., and Sakakura, Y. (2014). Euryhaline rotifer Proales similis as initial live food for rearing fish with small mouth. Aquaculture 432, 470–474. doi: 10.1016/j.aquaculture.2014.03.034
Hall, C. J., and Burns, C. W. (2001). Effects of salinity and temperature on survival and reproduction of Boeckella hamata (Copepoda: Calanoida) from a periodically brackish lake. J. Plankton Res. 23, 97–104. doi: 10.1093/plankt/23.1.97
Harding, J. P., Marshall, S. M., and Orr, A. P. (1951). Time of egg-laying in the planktonic copepod Calanus. Nature 167, 953–953. doi: 10.1038/167953a0
Hill, M., Pernetta, A., and Crooks, N. (2020). Size matters: a review of live feeds used in the culture of marine ornamental fish. Asian Fish. Sci. 33, 161–174. doi: 10.33997/j.afs.2020.33.2.007
Hirche, H. J., Meyer, U., and Niehoff, B. (1997). Egg production of Calanus finmarchicus: effect of temperature, food and season. Mar. Biol. 127, 609–620. doi: 10.1007/s002270050051
Holste, L., and Peck, M. A. (2006). The effects of temperature and salinity on egg production and hatching success of Baltic Acartia tonsa (Copepoda: Calanoida): a laboratory investigation. Mar. Biol. 148, 1061–1070. doi: 10.1007/s00227-005-0132-0
Irigoien, X., Obermüller, B., Head, R. N., Harris, R. P., Rey, C., Hansen, B. W., et al. (2000). The effect of food on the determination of sex ratio in Calanus spp.: evidence from experimental studies and field data. ICES J. Mar. Sci. 57, 1752–1763. doi: 10.1006/jmsc.2000.0960
Kaplan, D., Richmond, A. E., Dubinsky, Z., and Aaronson, S. (1986). “Algal nutrition,” in Handbook of Microalgal Mass Culture, ed. A. Richmond (New York, NY: CRC Press).
Klepper, G. S., Burkart, C. A., and Houchin, L. (1998). Nutrition and the regulation of egg production in the calanoid copepod Acartia tonsa. Limnol. Oceanogr. 43, 1000–1007. doi: 10.4319/lo.1998.43.5.1000
Li, S. J., and Huang, J. Q. (1984). On two new species of planktonic Copepoda from the estuary of Jiulong River, Fujian, China. J. Xiamen Univ. Nat. Sci. 3, 381–390.
Lian, G. S., Wang, Y. G., Sun, R. X., and Hwang, J. S. (2018). Species Diversity of Marine Planktonic Copepods in China’s Seas. Beijing: China Ocean Paress. doi: 10.3724/SP.J.1003.2011.11130
Lindley, L. C., Phelps, R. P., Davis, D. A., and Cummins, K. A. (2011). Salinity acclimation and free amino acid enrichment of copepod nauplii for first-feeding of larval marine fish. Aquaculture 318, 402–406. doi: 10.1016/j.aquaculture.2011.05.050
Marcus, N. H. (1982). Photoperiodic and temperature regulation of diapause in Labidocera aestiva (Copepoda: Calanoida). Biol. Bull. 162, 45–52. doi: 10.2307/1540969
McKinnon, A. D., and Duggan, S. (2001). Summer egg production rates of paracalanid copepods in subtropical waters adjacent to Australia’s North West Cape. Hydrobiologia 453, 121–132. doi: 10.1023/A:1013115900841
McKinnon, A. D., Duggan, S., and De’ath, G. (2005). Mesozooplankton dynamics in nearshore waters of the Great Barrier Reef. Estuar. Coast. Shelf Sci. 63, 497–511. doi: 10.1016/j.ecss.2004.12.011
McKinnon, A. D., Duggan, S., Nichols, P. D., Rimmer, M. A., Semmens, G., and Robino, B. (2003). The potential of tropical paracalanid copepods as live feeds in aquaculture. Aquaculture 223, 89–106. doi: 10.1016/S0044-8486(03)00161-3
Miliou, H. (1992). Effects of light (photoperiod, spectral composition) on the population dynamics of Tisbe holothuriae Humes (Copepods, Harpacticoida). Hydrobiologia 232, 201–209. doi: 10.1007/BF00013705
Nguyen, T. T., Le, M. H., Doan, N. X., Nguyen, S. T., Truong, T. S., Vu, M. T., et al. (2020). Salinity and temperature effects on productivity of a tropical calanoid copepod Pseudodiaptomus incisus. Aquac. Res. 51, 3768–3779. doi: 10.1111/are.14727
Nogueira, N., Sumares, B., Andrade, C. A. P., and Afonso, A. (2017). The effects of temperature and photoperiod on egg hatching success, egg production and population growth of the calanoid copepod, Acartia grani (Calanoida: Acartiidae). Aquac. Res. 49, 93–103. doi: 10.1111/are.13437
Paffenhöfer, G. A. (1984). Food ingestion by the marine planktonic copepod Paracalanus in relation to abundance and size distribution of food. Mar. Biol. 80, 323–333. doi: 10.1007/BF00392828
Paffenhöfer, G. A., and Lewis, K. D. (1990). Perceptive performance and feeding behavior of calanoid copepods. J. Plankton Res. 12, 933–946. doi: 10.1093/plankt/12.5.933
Payne, M. F., and Rippingale, R. J. (2000). Evaluation of diets for culture of the calanoid copepod Gladioferens imparipes. Aquaculture 187, 85–96. doi: 10.1016/S0044-8486(99)00391-9
Peck, M. A., and Holste, L. (2006). Effects of salinity, photoperiod and adult stocking density on egg production and egg hatching success in Acartia tonsa (Calanoida: Copepoda): optimizing intensive cultures. Aquaculture 255, 341–350. doi: 10.1016/j.aquaculture.2005.11.055
Petipa, T. S. (1958). The diurnal feeding rhythm of the copepod crustacean Acartia clausi Giesbr. Dokl. Akad. Nauk SSSR 120, 435–437.
Runge, J. A. (1984). Egg production of the marine, planktonic copepod, Calanus pacificus Brodsky: laboratory observations. J. Exp. Mar. Biol. Ecol. 74, 53–66. doi: 10.1016/0022-0981(84)90037-6
Saito, H., and Taguchi, S. (1996). Diel feeding behavior of neritic copepods during spring and fall blooms in Akkeshi Bay, eastern coast of Hokkaido, Japan. Mar. Biol. 125, 97–107. doi: 10.1007/BF00350764
Santhanam, P., Jeyaraj, N., and Jothiraj, K. (2013). Effect of temperature and algal food on egg production and hatching of copepod, Paracalanus parvus. J. Environ. Biol. 34, 243–246.
Schipp, G. R., Bosmans, J. M., and Marshall, A. J. (1999). A method for hatchery culture of tropical calanoid copepods, Acartia spp. Aquaculture 174, 81–88. doi: 10.1016/S0044-8486(98)00508-0
Siddique, A., Bhowal, A., Purushothaman, J., Madhusoodhanan, R., Raghunathan, C., and Chandra, K. (2021). Seasonal oceanographic phenomenon promotes hitchhiking among the plankton in a coastal marine ecosystem: a tropical perspective. Ecol. Indic. 129:107914. doi: 10.1016/j.ecolind.2021.107914
Stearns, D. E. (1986). Copepod grazing behavior in simulated natural light and its relation to nocturnal feeding. Mar. Ecol. Prog. Ser. 30, 65–76. doi: 10.3354/MEPS030065
Sukenik, A., and Wahnon, R. (1991). Biochemical quality of marine unicellular algae with special emphasis on lipid composition. I. Isochrysis galbana. Aquaculture 97, 61–72. doi: 10.1016/0044-8486(91)90279-G
Sun, X. (2008). Population Dynamics of Small Copepod. Ph.D. thesis. Qingdao: Institute of oceanology, Chinese Academy of Sciences.
Suzuki, K., Nakamura, Y., and Hiromi, J. (1999). Feeding by the small calanoid copepod Paracalanus sp. on heterotrophic dinoflagellates and ciliates. Aquat. Microb. Ecol. 17, 99–103. doi: 10.3354/ame017099
VanderLugt, K., Cooney, M. J., Lechner, A., and Lenz, P. H. (2009). Cultivation of the paracalanid copepod, Bestiolina similis (Calanoida: Crustacea). J. World Aquac. Soc. 40, 616–628. doi: 10.1111/J.1749-7345.2009.00282.X
VanderLugt, K., and Lenz, P. H. (2009). Handbook for the Cultivation of Two Hawaiian Paracalanid Copepods. Honolulu, HI: University of Hawaii.
Vengadeshperumal, N., Damotharan, P., Rajkumar, M., Perumal, P., Vijayalakshmi, S., and Balasubramanian, T. (2010). Laboratory culture and biochemical characterization of the calanoid copepod, Acartia southwelli Sewell, 1914 and Acartia centrura Giesbrecht, 1889. Adv. Biol. Res. 4, 97–107.
Vineetha, G., Karati, K. K., Raveendran, T. V., Babu, K. I., Riyas, C., Muhsin, M. I., et al. (2018). Responses of the zooplankton community to peak and waning periods of El Niño 2015–2016 in Kavaratti reef ecosystem, northern Indian Ocean. Environ. Monit. Assess. 190:465. doi: 10.1007/s10661-018-6842-9
Voordouw, M. J., and Anholt, B. R. (2002). Environmental sex determination in a splash pool copepod. Biol. J. Linn. Soc. 76, 511–520. doi: 10.1046/j.1095-8312.2002.00087.x
Wullur, S., Sakakura, Y., and Hagiwara, A. (2009). The minute monogonont rotifer Proales similis de Beauchamp: culture and feeding to small mouth marine fish larvae. Aquaculture 293, 62–67. doi: 10.1016/j.aquaculture.2009.04.011
Yang, W. D. (2007). Studies of Zooplankton Grazing Impacts on Phytoplankton in Xiamen Harbor. Master’s thesis. Xiamen: Xiamen University.
Zhang, G. (2003). Reproductive Strategy of Calanus sinicus in the Southern Yellow Sea. Ph.D. thesis. Qingdao: Institute of Oceanology, Chinese Academy of Sciences.
Zhao, W., Liu, Q., and Zhang, P. (2014). Experimental Population Ecology and Technology of Culture and Utilization of Marine Copepods. Beijing: Science Press.
Keywords: copepod, sex ratio, productivity, live prey, algal feeding density
Citation: Wang L, Wang S, Zeng C, Wang Y and Zeng C (2021) Effects of Food Concentration and Photoperiod on Egg Production, Female Life Expectancy and Population Dynamics of the Paracalanid Copepod, Bestiolina amoyensis. Front. Mar. Sci. 8:788744. doi: 10.3389/fmars.2021.788744
Received: 03 October 2021; Accepted: 01 November 2021;
Published: 25 November 2021.
Edited by:
Sami Souissi, Lille University of Science and Technology, FranceReviewed by:
Mie Hylstofte Sichlau Winding, Greenland Climate Research Centre, GreenlandDonghui Guo, Xiamen University, China
Copyright © 2021 Wang, Wang, Zeng, Wang and Zeng. This is an open-access article distributed under the terms of the Creative Commons Attribution License (CC BY). The use, distribution or reproduction in other forums is permitted, provided the original author(s) and the copyright owner(s) are credited and that the original publication in this journal is cited, in accordance with accepted academic practice. No use, distribution or reproduction is permitted which does not comply with these terms.
*Correspondence: Shuhong Wang, shwang@jmu.edu.cn
†These authors have contributed equally to this work and share first authorship