- Department of Marine Sciences and Applied Biology, University of Alicante, Alicante, Spain
Artificial structures can be considered as high spatially structured habitats in the marine pelagic system, where patch connectivity would be strongly dependent on the exchange of larvae or dispersing individuals. Fish-farms located offshore may alter ecological connectivity, modifying trophic resources, and species dispersal among patches. High population densities of invertebrates can be found associated with fish-farm fouling communities, which can act as a seed source, contributing to the patterns of connectivity through individuals exchange between subpopulations or with sink populations. A field experiment was performed to analyse the role of fish-farms in the colonisation of new uninhabited habitats (floating experimental units) located at different positions relative to the fish farm and the main current, containing artificial habitats with and without feed pellets similar to those used in the fish farm. Amphipods were used as example of direct developing invertebrates for studying dispersing individuals from the fish farm to the new habitats. The richest and most abundant populations in this study were found close to and downstream of the fish farm, surpassing 1,000 amphipods at their maximum. Moreover, some floating habitats located more than 2 km from the fish farm were colonised in only 15 days. Thus, the role of fish farms has been shown to extend beyond a ‘stepping-stones’ effect in species dispersal, and have an additional effect on ecological connectivity by increasing population sizes and acting as population source. Our study aims to provide recommendations for coastal zone management in order to predict potential spread from fish farms to other platforms in the future and promote solutions related to interactions and consequences of connectivity within and between marine facilities.
Introduction
Understanding patterns of species dispersal is crucial to address questions of population persistence and community dynamics between groups of populations (Grantham et al., 2003). In spatially structured populations, or ‘metapopulations,’ source–sink or continent-islands dynamics can sustain local populations, such that populations with positive growth rate seed the populations with negative growth rate (Munguia et al., 2007). For fouling species, offshore facilities can be considered as high spatially structured habitats in the marine pelagic system, without obvious connectivity with other habitats, similar to the inhospitable terrestrial landscapes that surround and limit the dispersal of freshwater invertebrate populations (Bilton et al., 2001). So that, fouling communities on floating structures such as buoys, rafts or fish farms are isolated benthic habitats as they are surrounded by the sea and far from the seabed. Consequently, offshore facilities may lead to alteration of ecological connectivity, where patch connectivity would be strongly dependent on the exchange of larvae or dispersing individuals (Bishop et al., 2017).
Floating rafts and fish farms are expanding across the oceans since the practice of intensive marine aquaculture has steadily increased in many countries during the last five decades (FAO, 2014). As other artificial structures, they alter the local hydrodynamics and represent new inhabitable niches which may be used by some species apart from their natural substrates (Bishop et al., 2017). However, aquaculture facilities additionally provided an input of organic-enriched wastes that may also influence population sizes. Despite biogeographical variations, the most important sessile species found in shellfish and finfish farms are bivalves especially mussels, hydroids, algae, and tunicates (Cook et al., 2006; CRAB, 2007; Fitridge et al., 2012; Giangrande et al., 2020). Additionally, main free-living and epifaunal inhabitants are crustaceans, polychaetes, gastropods, and echinoderms (e.g., Hodson et al., 2000; Hincapié-Cárdenas, 2007; Guenther et al., 2010; Gonzalez-Silvera et al., 2015; Watts et al., 2015). Amphipods are however without doubt the most reported group of mobile fauna in aquaculture facilities, notably caprellids and tube-building amphipods (Hodson et al., 2000; Cook et al., 2006; Greene and Grizzle, 2007; Fernandez-Gonzalez and Sanchez-Jerez, 2017).
For most benthic invertebrate species, e.g., many groups of crustaceans, the larval phase is the dominant dispersal stage, usually undergone by pelagic larvae that can spend months in the plankton. This phase is one of the most sensitive within the life cycle of the animal (Thorson, 1950). This does not happen with other invertebrates such as peracarid crustaceans, which show a different and direct larval development, with a complete retention of the offspring, emerging fully-developed as juveniles from the ventral marsupium of the mother. This evolutionary adaptation influences the processes of population connectivity across fragmented habitats, which would depend on active migration of later stages such as juveniles or adults (Gutow and Franke, 2003). However, direct developing invertebrates has been overlooked on studies about population connectivity between offshore structures, which focus on marine invertebrates with planktonic larval development (e.g., Simons et al., 2016; Van der Molen et al., 2018; Coolen et al., 2020).
While the direct development of invertebrates limits dispersal, it may result to be essential in establishing and maintaining high-density populations in optimal habitats, due to local recruitment. In contrast to organisms such as decapods with higher fecundity and mortality in early life-stages, recruitment in peracarids does not depend on favourable oceanographic conditions (defined as a type III survivorship curve; Hedgecock, 1994). Brooding leads to a comparatively reduced fecundity (35.5 eggs per female for amphipods; Sainte-Marie, 1991), but local recruitment is ensured by a lower mortality rate in the early stages and a steady survival probability throughout their life history (Ito, 1980).
Amphipods are direct developing invertebrates that occur in high numbers in floating off-coast fish farms. Thus, local recruitment together with feed supply provided by aquaculture wastes could explain the high population densities amount thousands of amphipods per square meter associated with fish-farm fouling (Fernandez-Gonzalez, 2017). In such a situation, competition between juveniles and adults for both space and food may arise within the original habitat, so that when habitat patches are too crowded and competition for resources is hard, dispersal to new habitats can be spurred (Cadet et al., 2003).
In this sense, fish farms can act as a seed source and contribute to the patterns of connectivity through amphipod exchange between subpopulations or with sink populations. Thus, amphipod dispersal by drifting, rafting, dislodgement or migration may occur at different life-history stages such as juveniles or adults, leading to differences in initial population sizes, survivorship or population growth rates (Munguia et al., 2007; Munguia, 2015). Different drivers will determine the dispersal and successful colonisation of a new habitat, e.g., the biological condition of the source population, local currents and tides, distance from the source to the sink population or the existence of chemical cues that favour habitat selection by active swimming. Even as the numbers of immigrants may decrease with opposing currents or distance from their source, it is the conditions at the receiving population that will determine what level of subsidy is significant (Cowen and Sponaugle, 2009).
This study aims to understand the connectivity patterns for direct developing invertebrates in fragmented marine habitats, using amphipods as model organism. We analyse the potential role of fish-farm fouling as a source population of amphipods moving toward surrounding habitats located in the water column to determine its influence in the establishment and maintenance of local metapopulations. For this, the study was designed trying to avoid misinterpretations related to other sources of dispersal individuals or pathways such as hull fouling, floating objects or other offshore structures. Thus, we hypothesised that: (a) the main current and the intervening distance will affect the initial biofouling community and the dispersal of amphipods from a source population to surrounding habitats; (b) the suitability of the new floating habitats in terms of refuge and/or chemical stimuli from aquafeeds will elicit amphipod colonisation; and finally (c) initial population sizes in the new habitats will depend on the sex and life stage of the amphipods dispersing from the source population. A field experiment accompanied by zooplankton hauls was therefore performed in order to analyse differences in amphipod colonisation among new uninhabited habitats (floating experimental units) located at different positions relative to the fish farm and the main current, containing artificial habitats with and without feed pellets similar to those used in the fish farm. Additionally, the sex and life stage of both early colonists and migrants moving in the water column were noted so as to gain understanding about possible differences in initial population sizes attained in the new habitats.
Materials and Methods
Experimental Design
The field experiment was carried out at a fish farm off the coast of Guardamar del Segura (Alicante, Spain: 38° 5′45.88”N; 0°36′15.84”W) during July 2011. The fish farm consisted of 18 rings with a diameter of 19 m or 25 m and cage nets reaching depths from 12 to 15 m, set over a seabed at approximately 25 m depth. The cage structures were located 3 to 4 km off-coast and grow European sea bass Dicentrarchus labrax and gilthead sea bream Sparus aurata.
Fouling communities in this fish farm acting as source population of amphipods were characterised by a mean population density of 176,487 ± 38,893 ind.m–2, with a maximum of 1,008,727 ind.m–2 (Fernandez-Gonzalez and Sanchez-Jerez, 2017). Ten amphipod species were identified as part of the source population: Caprella dilatata, Caprella equilibra, Elasmopus rapax, Ericthonius punctatus, Jassa marmorata, Jassa slatteryii, Monocorophium insidiosum, Stenothoe georgiana, Stenothoe tergestina, and Stenothoe valida, being the gammarids J. slatteryi, E. rapax, E. punctatus and the caprellids C. dilatata, and C. equilibra the most abundant amphipod species. These species have been previously described as frequent and dominant in fish-farm samples from different points of the Mediterranean (Fernandez-Gonzalez and Sanchez-Jerez, 2017), confirming that this facility is representative of the Mediterranean aquaculture.
Seventy-two artificial collectors (ACs) were deployed in the dispersal and colonisation experiment, with an estimated volume of 250 ml. Three kinds of ACs were used: ‘Habitat’ collectors (H), based on those used by Vázquez-Luis et al. (2012), consisting of a mesh bag with the artificial habitat (plastic raffia), ‘Habitat and Pellets’ collectors (HP), identical to the previous but with feed pellets inside them as used at the fish farm and ‘Control’ collectors (C), with only the external mesh bag used in making the AC. Each AC represented a kind of possible receiving population in such a way that ‘H’ represented habitats offering refuge, ‘HP’ simulated those conditions found in fish farm fouling with available refuge and food while ‘C’ was a control for the treatment, to check the effect of the external mesh bag. ACs were suspended from a submerged buoy anchored by a rope at approximately 15 m from the sea bottom and between 5 and 8 m below the surface (Figure 1). The whole structure was totally underwater to avoid misinterpretations related to dispersal via hull fouling or other floating objects. Three replicates of the same kind of AC were attached on each rope with a minimal distance of 1m between them to ensure independence among replicates. Different ropes were used for each kind of collector in order to avoid interferences, particularly those related to the chemical cues from fish feed, and separated by several metres. In the field, the effects of distance and current on the dispersal and colonisation processes were tested by placing ACs near (50 m) and far (more than 2 km) from the fish farm and up and down the mainstream, at two different sites. Sites were randomly selected and separated by tens of metres. The main current was obtained from the historical data from the two previous summer periods recorded by the national government in the region1.
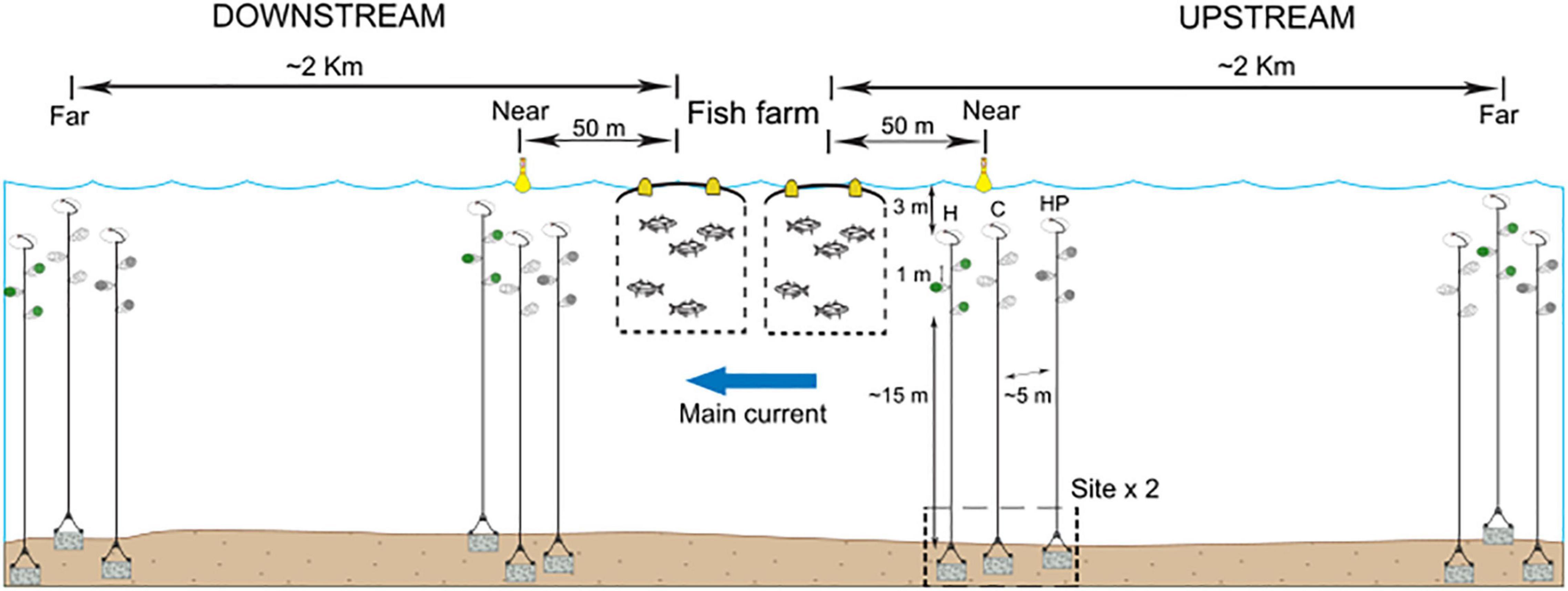
Figure 1. Experimental design and scheme of the deployment of the three types of ACs containing habitat and feed pellets (HP), only habitat (H) or control (C).
After 15 days, ACs were collected, covering them with independent plastic bags, and retrieved by divers. In the laboratory, the collected samples were preserved in 4% formalin seawater solution and later sieved through a 250 μm mesh. Artificial habitat and the biofilm that developed on the AC surface were checked and all amphipods were sorted. Biofilm and the initial fouling community on each AC were quantified by filtering with pre-weighed GFC Whatman filters and drying at 80°C.
The field experiment was combined with plankton hauls, in order to detect amphipods drifting in the water column. Vertical plankton hauls were performed using a conical 250 μm-mesh plankton net 0.6 m in diameter from the maximum depth to the surface. Samples were collected taking into account the same zones where ACs were located, i.e., near and far from the fish farm and up and down the mainstream. Plankton hauls were repeated 2 days during the course of the experiment, considering day and night-time to cover the possible nocturnal migrations of amphipods in these areas. Three replicates were taken in each case, resulting in a total of 48 plankton hauls. At the end of each trawl, the net was washed down with seawater and the retained material was also preserved in 4% formalin seawater solution.
All amphipods from AC and plankton hauls were sorted, identified to species level whenever possible, classified according to sex and life-history stage as males, females, brooding females or juveniles and finally counted. Sexual dimorphism was used to distinguish males and females adults by the size and shape of the gnathopods and the presence of oostegites or penial papillae. Females with eggs or juveniles in the brood pouch were termed brooding females. Small amphipods that could not be clearly identified as adult males or females were considered juveniles.
Data Analysis
To evaluate the influence of floating aquaculture facilities as source populations on surrounding floating habitats, data from AC were analysed taking into account a 4-factor mixed design: ‘Habitat suitability’ (orthogonal and fixed; three levels: habitat ‘H,’ habitat and feed pellets ‘HP’ and control treatment ‘C’); ‘Distance’ (orthogonal and fixed; two levels: ‘Near’ and ‘Far’), ‘Current’ (orthogonal and fixed; two levels: ‘up’ and ‘downstream’) and ‘Site’ (nested in the triple interaction and random, two levels), with three replicates for each treatment.
Biomass of the sessile colonising community, amphipod total abundance, species richness and abundance of the most important amphipod species were analysed using Generalised linear mixed models (GLMs) to univariate responses. Mixed-effects GLMs were fitted by means of the ‘lme4’ (Bates et al., 2007) and ‘lmerTest’ packages (Kuznetsova et al., 2017) to test for differences between main factors. Models were fitted using a Gaussian, or a negative binomial, family distribution of residuals, with a square-root link function. Moreover, GLMs were also fitted to the multivariate abundance data matrix, by means of the ‘mvabund’ package (Wang et al., 2012), using a negative binomial family error distribution due to over dispersion of abundance data, and P-values were calculated using 999 resampling iterations via a PIT-trap resampling procedure. The relative contribution of each of the three main fixed factors to explain the overall multivariate variance was estimated via the ‘best.r.sq’ function.
For all fitted GLMs, diagnosis plots of residuals and Q-Q plots were visually inspected to check the appropriateness of fitted models (Harrison et al., 2018). To select models with the largest parsimony, the AIC (Akaike Information Criterion) was obtained for the null model and models containing only the response variable and the entire set of predictors (full model including the four factors). The ‘Site’ factor did not help to explain any degree of variability with respect to the null model and the AIC was larger when this factor was included, hence finally not considered ‘Site’ in none of the univariate or multivariate models.
All modelling and testing were here implemented in the R3.6.1 statistical environment (R Core Team, 2019)2.
Results
Development of the Biofilm and Biofouling Community on Artificial Collectors
After 15 days of the experiment, ACs showed a colonising community formed of the initial biofilm community and sessile hydroids belonging to Campanulariidae family (mainly Obelia sp.). Higher biomass was found on ACs near the fish farm and downstream along the main current. Moreover, ACs containing fish pellets generated a significantly higher quantity of colonising community than the other ACs (P < 3.85e-6, Figure 2 and Table 1). Thus, the three main factors tested in this study: ‘Distance’ from fish farm, orientation to main ‘Current’ and ‘Habitat suitability’ showed independently significant differences regarding the biomass of colonising community generated (Figure 2 and Table 1).
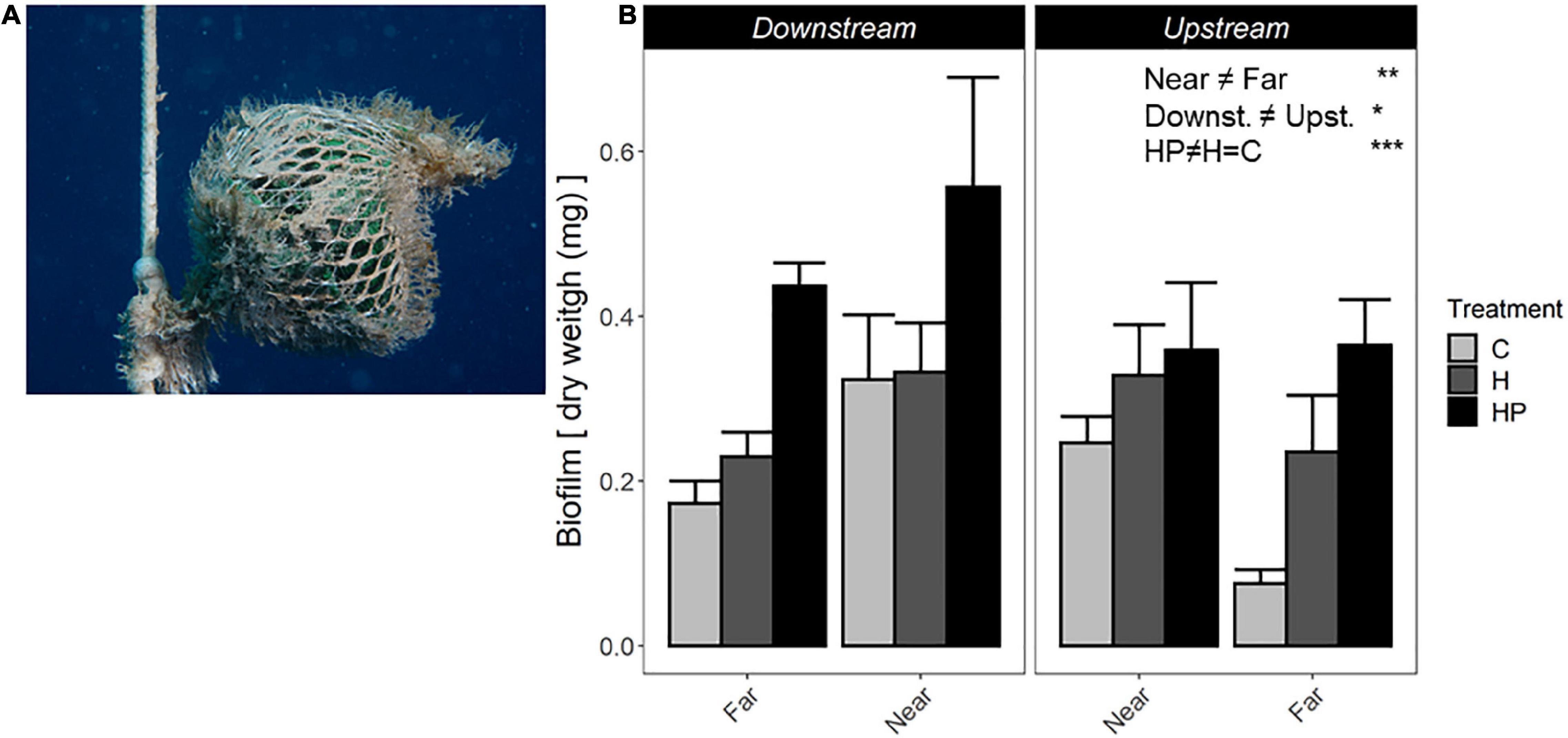
Figure 2. (A) Fouling community on an AC after 15 experimental days. (B) Biomass of sessile colonising community, including biofilm and small hydroids, expressed as dry weight (mg). ACs are represented as ACs containing habitat and feed pellets (HP), only habitat (H) or control (C). Significant GLM results are expressed as *p < 0.05; **p < 0.01; and ***p < 0.001.
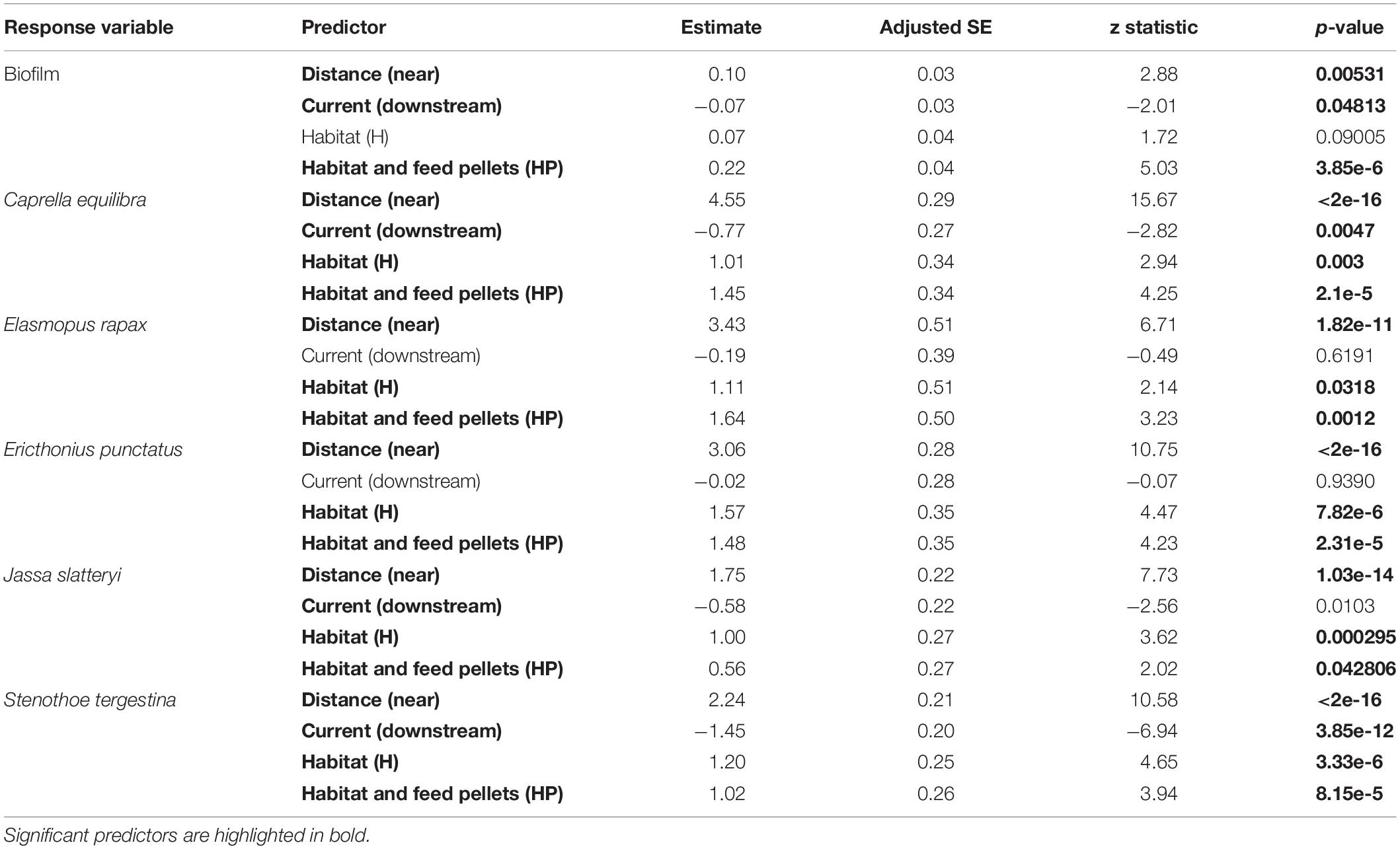
Table 1. Results of the GLM testing for the effects of “Distance,” “Current,” and “Habitat suitability” for the biofilm and the most abundant amphipods of the assemblage, including associated P-values.
Effects of Main Current and Distance in Amphipod Dispersal and the Importance of Habitat Suitability in the Colonisation of the New Habitat
A total of 16,211 individuals belonging to ten amphipod species were identified. Total abundance and number of species were higher on ACs located near the fish farm and downstream (453.9 ± 99.2 ind.; 7.56 ± 0.24 species) than upstream (366.2 ± 106.7 ind.; 6.28 ± 0.25 species) and far from it, both downstream (49.9 ± 11.1 ind.; 5.28 ± 0.29 species) and upstream (21.6 ± 7.7 ind., 2.17 ± 0.27 species). Thus, the interaction between the factors ‘Distance’ and ‘Current’ was significantly different for both variables (P < 0.036 for the abundance; P < 0.006 for the richness; Figures 3, 4).
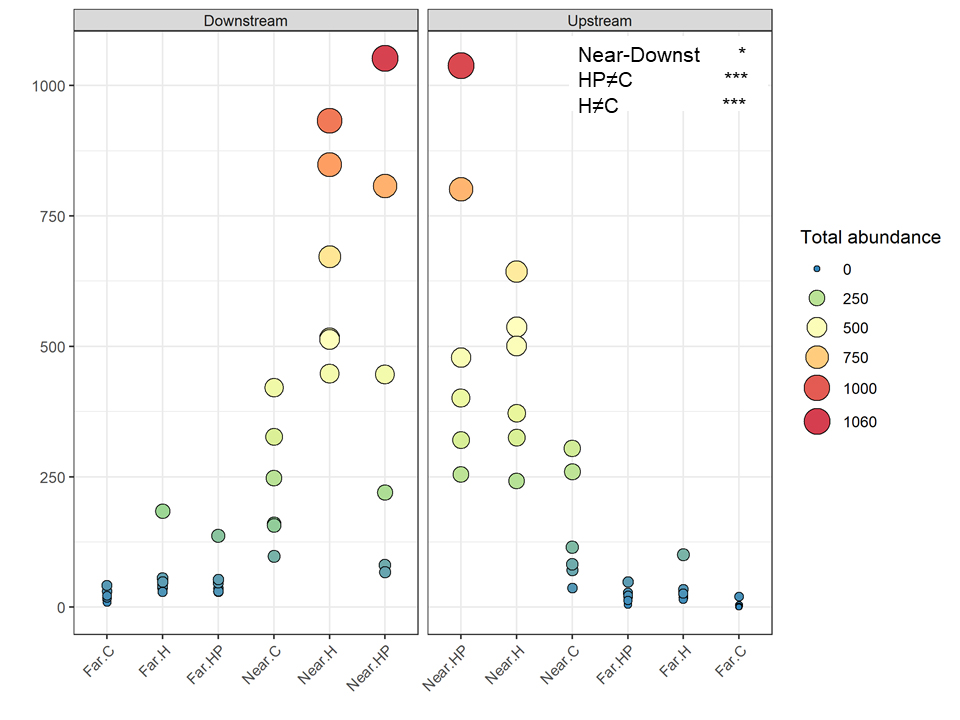
Figure 3. Mean total abundance of amphipods in the different ACs containing habitat and feed pellets (HP), only habitat (H) or control (C) located near and far downstream and near and far upstream. Significant GLM results are expressed as *p < 0.05 and ***p < 0.001.
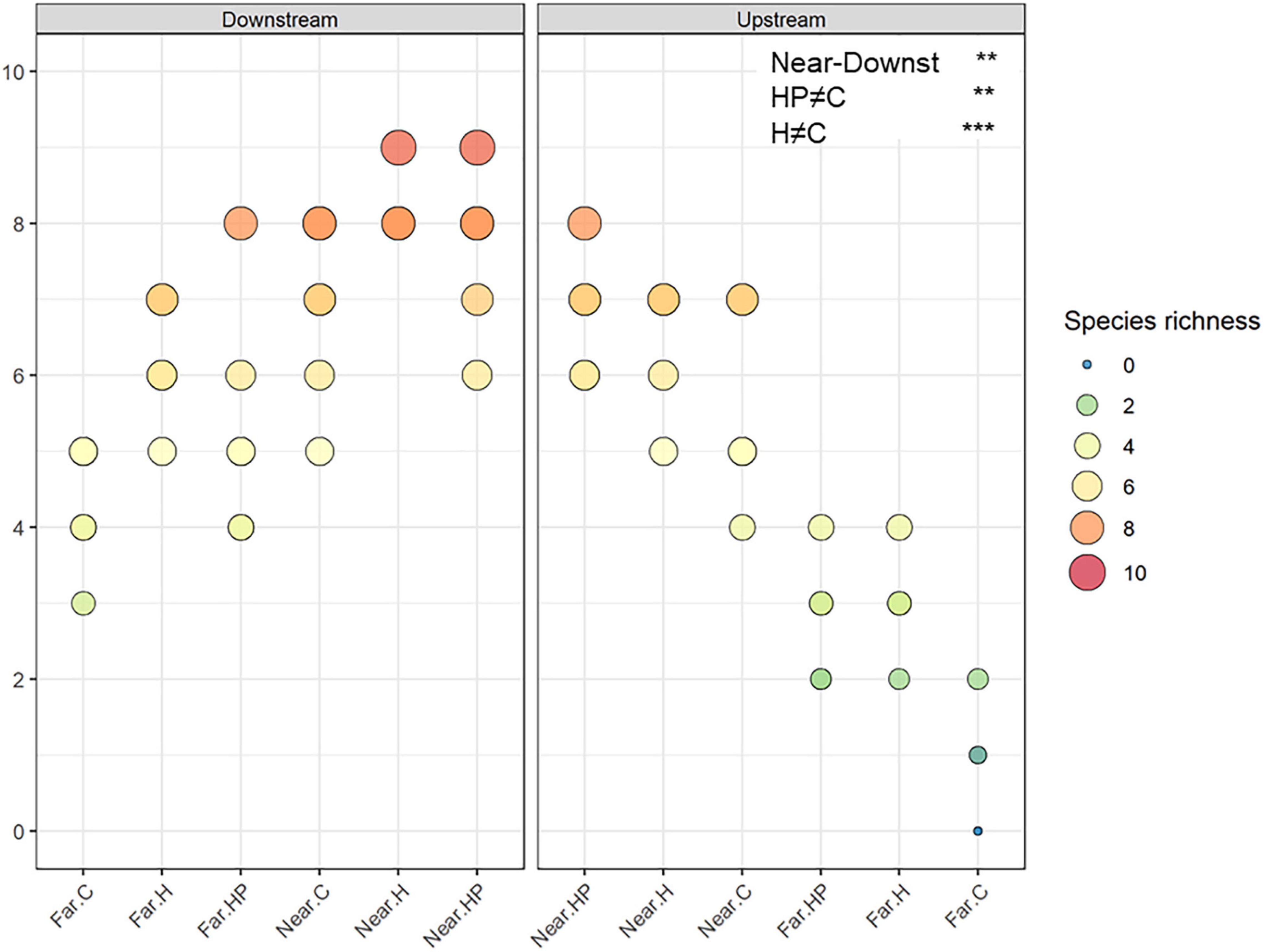
Figure 4. Amphipod species richness in the different ACs containing habitat and feed pellets (HP), only habitat (H) or control (C) located near and far downstream and near and far upstream. Significant GLM results are expressed as **p < 0.01 and ***p < 0.001.
The multivariate structure of amphipod assemblage composition showed independently significant differences for the three main factors tested in this study: ‘Distance,’ ‘Current,’ and ‘Habitat suitability’ (Table 2). The factors ‘Current’ and ‘Habitat suitability’ explained a larger amount of variation in the multivariate structure (30 and 27%, respectively) than did ‘Distance’ (21%). The most abundant amphipod species were Ericthonius punctatus, Caprella equilibra, Jassa slatteryi and Stenothoe tergestina, which were also responsible for differences between Near and Far treatments (Figure 5 and Table 1). To a large extent, all the amphipods tended to colonise ACs located downstream, and statistical differences were found for the factor ‘Current’ except for E. rapax and E. punctatus that also successfully colonised those nearer ACs located upstream (Figure 5).
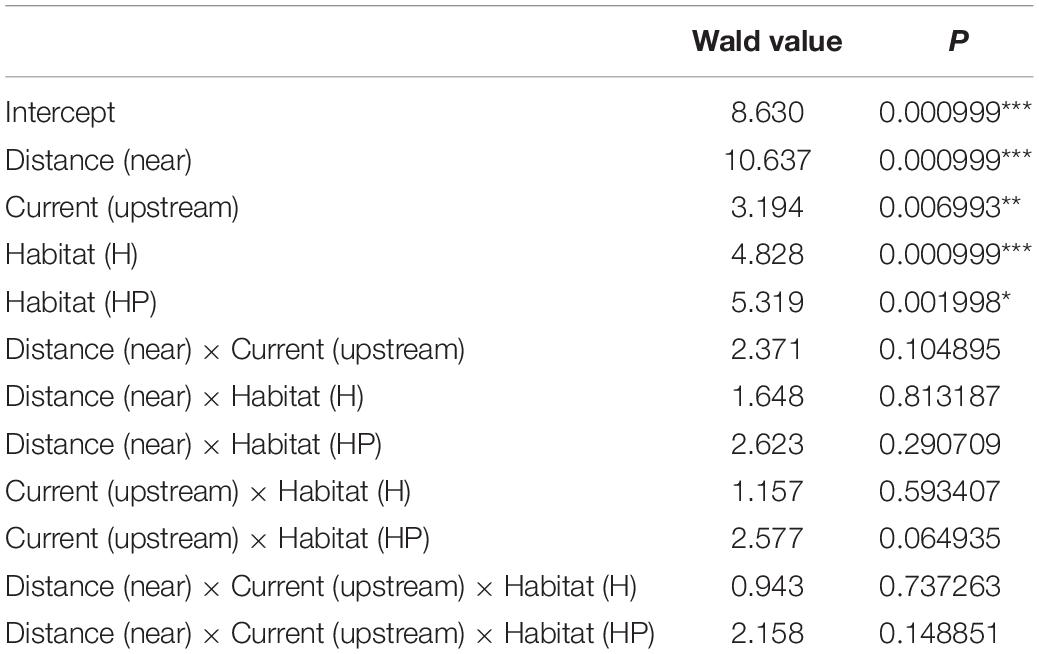
Table 2. Multivariate GLM results for the whole amphipod assemblage showing significant different between factor levels and associated P-values (*P < 0.05, **P < 0.01, and ***P < 0.0001).
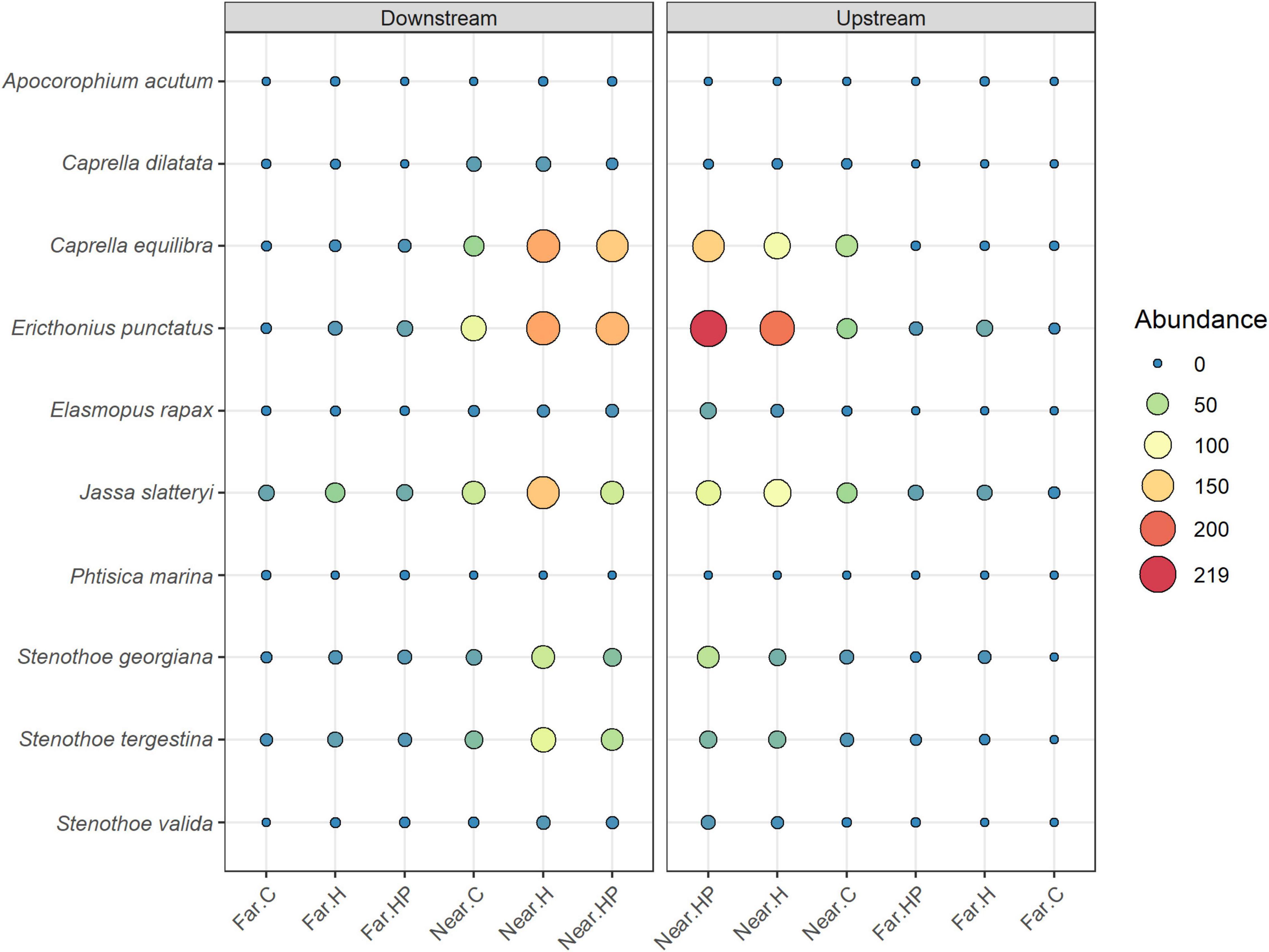
Figure 5. Mean abundances of the main amphipod species studies in the different ACs containing habitat and feed pellets (HP), only habitat (H) or control (C) located near and far downstream and near and far upstream.
Few differences were found regarding habitat suitability, except for the provision of refuge. Mean total abundance and species richness were significantly higher in those ACs containing artificial habitat, both HP (268.33 ± 67.16 ind., P < 7.44 e–5; 5.6 species, P < 0.001) and H (298.71 ± 58.04 ind., P < 2.67 e–7; 6.0 species, P < 0.00001), than control ones (101.83 ± 24.54 ind., 4.4 species; Figures 3, 4). Amphipod assemblage composition was also significantly different in control ACs (Table 2; H = HP≠C); this was mainly due to the significant lower abundances of E. punctatus, C. equilibra, J. slatteryi, Elasmopus rapax and S. tergestina found in the control ACs (Figure 5 and Table 1).
Sex and Life Stage of the Colonisers and Dispersing Amphipods
High amphipod abundances found in ACs after 2 weeks hindered the identification of sex and life stage of the colonisers, given that most of the species found in ACs near the fish farm showed a large proportion of juveniles (around 44.9%) and reproductive females, including brooding females and females with empty marsupium (16.2% approximately, Table 3). However, records of each individual being the only specimen of its species in an AC were identified and classified according to their sex and life stage, in order to consider them as first colonisers (assuming that some growth could have occurred at the new habitat). Data thus obtained reflected that most of the species tend to disperse as adults, but they are able to operate several dispersal modes as did C. dilatata which also arrived at ACs as juveniles (Table 3).
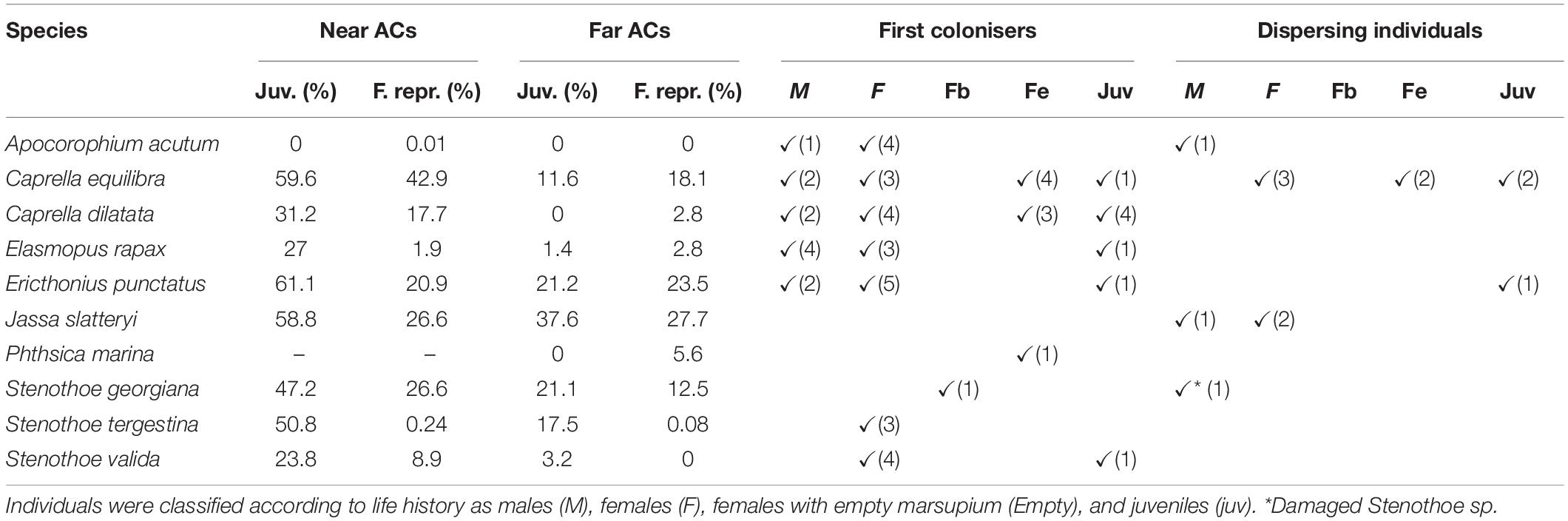
Table 3. Proportion of juveniles and reproductive females present in the artificial collectors (ACs) and sex and life-stage of the first colonisers in an AC and the dispersing habitat detected in this study.
In the other hand, only 25 amphipods were captured using vertical plankton hauls. According to these results for dispersing amphipods, C. equilibra were detected dispersing in the water column in different stages as non-brooding females, females with empty marsupium and juveniles, while dispersing A. acutum and J. slatteryi individuals were adults and those of E. punctatus were juveniles.
Discussion
Artificial structures near and offshore may lead to alteration of ecological connectivity of some species (Bishop et al., 2017). Offshore facilities provide habitat in the pelagic system in such a way that can be considered as subpopulations with patch connectivity strongly dependent on the exchange of individuals. Fish farms as highly productive habitats may maintain increasing biomass of inhabiting organisms to the limit of their carrying capacity and have a strong influence promoting and maintaining surrounding sink populations. Thus, according with this study, the role of fish farms extends beyond that of a subpopulation by acting as a source population. Population connectivity have been demonstrated for amphipods which lack of planktonic larvae and depend on active migration of dispersal individuals. As expected, distance between the source and receiving habitats and their position down the main current are determining factors in the successful colonisation of the new habitats. In fact, the richest and most abundant populations in this study were found close to and downstream of the fish farm, surpassing 1000 amphipods at their maximum. Moreover, high connectivity between floating habitats seems to exist when even those located more than 2 km from the fish farm were colonised in only 15 days.
After dispersal through the water column, planktonic larvae or dispersing individuals must locate a suitable substrate on which to settle, which may depend on the presence and strength of cues to which the larvae may respond (Kingsford et al., 2002). The surface film of microorganisms has long been recognised as a prerequisite for the settlement of many fouling species (Pawlik, 1992). By the end of the field experiment, the clean surfaces provided by the new habitats had been covered by an initial biofouling layer consisting of a biofilm and some small hydroids. Fish farming activity can enhance biofilm biomass near the facility (Sanz-Lázaro et al., 2011) and in fact, the presence of feed pellets in experimental units led to a greater quantity of this initial biofouling, as did proximity and the location downstream from the fish farm.
In our study, higher abundances were detected downstream but amphipods were able to colonise all the habitats close to the fish farm, even those located upstream. This can be explained by both physical and biological processes. The structural framework of fish farms locally affects the oceanographic conditions, deviating currents and reducing their speed (Klebert et al., 2013). Therefore, the altered pattern of the main current, together with other secondary currents and eddies may well promote passive dispersal to upstream locations. Furthermore, active swimming and nocturnal migrations related to the lunar cycle may also be responsible for the arrival in new habitats of dispersing invertebrates against the main current (Fernandez-Gonzalez et al., 2014, 2016). This is more conspicuous when the species show strong swimming capabilities, such as the gammarids E. rapax or E. punctatus in the case of this study, which is probably why these species reached higher abundances in upstream locations. The effect of the main current on amphipod dispersal was more patent with distance, where ‘far’ habitats located downstream of the fish farm were colonised with higher abundances than those upstream.
Dispersal is often density-dependent in such a way that populations with a positive growth rate can promote emigration of individuals to other local populations (Pulliam, 1988; Amarasekare, 2004). Thus, density-dependent dispersal has important consequences on source-sink dynamics. Density-dependent changes seem to drive the flow of individuals from the fish farm to new habitats, since three of the most abundant species of direct developing invertebrates in the fish-farm fouling (E. punctatus, C. equilibra, and J. slatteryi) were also the most abundant in new ‘near’ habitats.
Conditions in the newly available habitats may not be appropriate to allow a species to complete its life history, in such a way that even when a species is able to disperse and arrive at a new site, it will not occur if its niche or preferred habitat are absent (Pulliam, 1988). The physical structure of the substrates and habitat complexity play an important role in the abundance of associated invertebrates (Hacker and Steneck, 1990; Aikins and Kikuchi, 2001; Hauser et al., 2006) as well as the existence of trophic resources may produce chemical cues that increase the colonisation rate. Refuge or greater surface available seem to be the most important issues in amphipod colonisation in this study, since higher densities and species richness were reached in the experimental units that offered an additional artificial habitat. Thus, tube-building amphipods such as E. punctatus and J. slatteryi colonise all new habitats including control ACs, regardless of their distance from the source, by attaching their tubes to any kind of hard substrate. They can even occupy clean surfaces or those in an initial stage of colonisation that fulfil their ecological requirements (Beermann, 2014).
Food stimuli seem to be unrelated to the selection of a new site to settle, at least regarding chemical cues from feed pellets. When living in fish-farm fouling, amphipods are able to assimilate the organic matter derived from food pellets and other farming activity as a trophic resource (Gonzalez-Silvera et al., 2015) but in this study, no differences were detected in the number or abundance of amphipod species in those ACs containing fish pellets, even though the biofouling community on them was also higher. Some issues could explain the lack of response to the availability of food. For example, the enriched environment near the fish farm could hinder finding differences between experimental units with and without fish pellets, due to both the strong presence of the same chemical cues or other food available in the water column around the farm. Other scenarios include the possible inability of the amphipod to detect chemical cues from feed pellets, especially when they are juveniles, or the interferences with other wild fauna attracted to ACs with pellets such as fish. Further studies about the potential influence of chemical cues from aquafeeds on amphipods should be performed in the laboratory in order to resolve these issues, avoiding possible interferences in this field experiment.
Differences in life-history stages influence the dispersal potential of each species as well as the processes of population connectivity (Levin, 2006). Indeed, dispersal as juveniles or adults can lead to different initial population sizes, survivorship or population growth rates (e.g., Highsmith, 1982; Munguia et al., 2007; Munguia, 2015). According to the results of this study, most of the species tended to disperse as adults, except for some dispersing juveniles of C. equilibra, E. punctatus and C. dilatata, whose presence in the plankton, together with juveniles of E. rapax, was also confirmed through more selective methods like light traps (Fernandez-Gonzalez et al., 2014). Species that disperse mainly as juveniles usually colonise more slowly and disperse more evenly than species dispersing as adults, sometimes dominating them in terms of numerical abundance (Munguia et al., 2007), which seems to be in accordance with the abundances attained by these species in the new habitats. Nevertheless, caprellid females with empty marsupium were also detected as dispersing individuals and first colonisers, suggesting that dispersal may occur at different stages. Dispersal just before or during the reproductive period, as ovigerous females, facilitates the colonisation process and may explain population persistence even at very low densities (Gilpin, 2012). Such breeding dispersal could be assumed for E. punctatus, since around 20% of swimmers belonging to this species captured with light traps were ovigerous females (Fernandez-Gonzalez et al., 2014) or for J. slatteryi, given the initial population sizes and the high proportion of reproductive females in the new habitats. Indeed, dispersal as adults promotes higher initial population sizes when a new habitat is colonised, especially when an ovigerous female reaches and releases her brood into these new habitats (Mills, 1967; Munguia et al., 2007). This is even more so when one single brood of J. slatteryi consists of up to 40 embryos (Jeong et al., 2007) or 10 embryos for E. punctatus (Rumbold et al., 2016).
Once the species arrived in the new habitats, especially the closer ones, all of them (except A. acutum) showed all developmental stages (adult males, adult females, including brooding females and juveniles). This indicates they can all undergo their complete life cycle in the new habitats and maintain local populations, not only receiving individuals from the fish farm but also through self-recruitment. Nevertheless, as the respective proportions of each stage varied among the samples, the dynamics of the local populations had still not become stabilised (Gutow and Franke, 2003).
Complex mechanisms explain the connectivity between highly productive habitats and newly available habitats in the water column. The case of fish farms in off-coast areas is very special because is an optimal habitat, in the case of amphipods with population densities above 170,000 individuals per m2 (Fernandez-Gonzalez, 2017). The near-saturated populations found in these habitats promote the dispersal of individuals ready to start breeding immediately (presaturation dispersal), and even less fit individuals such as juveniles (saturation dispersal; Hansson, 1991). This experiment confirms that connectivity in highly spatially structured populations such as suspended underwater habitats is feasible at a scale of hundreds of metres, where individuals move across a distressing environment like the water column with the risk of predation or failing in an unsuitable habitat. This successful colonisation happens in only 15 days even when species with limited dispersal such as direct developing invertebrates have been considered. Thus, higher connectivity can be expected for species with pelagic dispersal phase, which could potentially travel up to 10 km (Simons et al., 2016). Moreover, the fact that the new habitats colonised also include those without obvious trophic resources suggests that different kinds of floating habitat beyond the aquaculture facilities may be colonised.
The role of anthropogenic structures providing habitat that could facilitate species range expansions and the introduction of non-native species have been widely suggested in published literature (e.g., Boos et al., 2011; Mineur et al., 2012; Fernandez-Gonzalez and Sanchez-Jerez, 2013; Adams et al., 2014). However, the presence of hard substrata in offshore areas is extremely limited in comparison to the surfaces available in shallow coastal waters (e.g., natural rocky habitats, breakwaters and jetties; Bulleri and Chapman, 2010). Few studies have previously explored potential connectivity among existing offshore structures or their possible role in the dispersal of marine species. Here, the role of fish farms has been shown to extend beyond a ‘stepping-stones’ effect, and have an additional effect on ecological connectivity by increasing population sizes and acting a population source. Dispersal of direct developing species may particularly benefit from these structures which, however, has been rarely investigated in ecological connectivity studies.
Models or approaches to assess connectivity are needed and should consider these factors in order to predict potential spread to other artificial structures in the future with the aim to favour or reduce interactions within and between marine facilities (Van der Molen et al., 2018). Considering distance and position regarding the main current from fish farms to different marine facilities is particularly important in order to reduce the risk of spreading in case of non-indigenous species. It should be considered that the successful colonisation of a new habitat depends on the presence or not of a resident population and local factors such as competition or predation.
In the opposite side, artificial structures can reduce connectivity by acting as physical barriers to the movement of organisms within and among habitats (Bishop et al., 2017). In the light of the results, coupling drop-ropes with collectors to the existing floating cages could act as physical barrier and achieve potential accessory cultures as part of more environment-friendly models such as integrated multi-trophic aquaculture (IMTA) systems (Fernandez-Gonzalez et al., 2018). Further studies are necessary to assess this and other possible consequences of the connectivity between floating artificial habitats in off-coast areas.
Data Availability Statement
The raw data supporting the conclusions of this article will be made available by the authors, without undue reservation.
Author Contributions
VF-G and PS-J conceived the idea, designed methodology, and collected the samples. VF-G and SN-M analysed samples at the laboratory and performed statistical analysis. VF-G led the writing of the manuscript. SN-M and PS-J contributed critically to the drafts and gave final approval for publication.
Conflict of Interest
The authors declare that the research was conducted in the absence of any commercial or financial relationships that could be construed as a potential conflict of interest.
Publisher’s Note
All claims expressed in this article are solely those of the authors and do not necessarily represent those of their affiliated organizations, or those of the publisher, the editors and the reviewers. Any product that may be evaluated in this article, or claim that may be made by its manufacturer, is not guaranteed or endorsed by the publisher.
Acknowledgments
We would like to thank staff of the fish farms that gave us access and help for the study. We are grateful to colleagues of the Marine Biology Laboratory at the University of Alicante and Fernando Tuya (University of Las Palmas de Gran Canaria, Spain) for their cooperation along the course of this work. We would also like to thank Guido Jones for carried out English proofreading.
Footnotes
References
Adams, T. P., Miller, R. G., Aleynik, D., and Burrows, M. T. (2014). Offshore marine renewable energy devices as stepping stones across biogeographical boundaries. J. Appl. Ecol. 51, 330–338. doi: 10.1111/1365-2664.12207
Aikins, S., and Kikuchi, E. (2001). Studies on habitat selection by amphipods using artificial substrates within an estuarine environment. Hydrobiologia 457, 77–86.
Amarasekare, P. (2004). The role of density-dependent dispersal in source–sink dynamics. J. Theor. Biol. 226, 159–168. doi: 10.1016/j.jtbi.2003.08.007
Bates, D., Sarkar, D., Bates, M. D., and Matrix, L. (2007). The lme4 Package. R Package Version, Vol. 2, 74.
Beermann, J. (2014). Spatial and seasonal population dynamics of sympatric Jassa species (Crustacea. Amphipoda). J. Exp. Mar. Biol. Ecol. 459, 8–16. doi: 10.1016/j.jembe.2014.05.008
Bilton, D. T., Freeland, J. R., and Okamura, B. (2001). Dispersal in freshwater invertebrates. Annu. Rev. Ecol. Syst. 32, 159–181.
Bishop, M. J., Mayer-Pinto, M., Airoldi, L., Firth, L. B., Morris, R. L., Loke, L. H., et al. (2017). Effects of ocean sprawl on ecological connectivity: impacts and solutions. J. Exp. Mar. Biol. Ecol. 492, 7–30. doi: 10.1016/j.jembe.2017.01.021
Boos, K., Ashton, G. V., and Cook, E. J. (2011). “The Japanese skeleton shrimp Caprella mutica (Crustacea, Amphipoda): a global invader of coastal waters,” in In the Wrong Place - Alien Marine Crustaceans: Distribution, Biology and Impacts, eds B. S. Galil, P. F. Clark, and J. T. Carlton (Dordrecht: Springer), 129–156.
Bulleri, F., and Chapman, M. G. (2010). The introduction of coastal infrastructure as a driver of change in marine environments. J. Appl. Ecol. 47, 26–35. doi: 10.1111/j.1365-2664.2009.01751.x
Cadet, C., Ferrière, R., Metz, J. A., and Van Baalen, M. (2003). The evolution of dispersal under demographic stochasticity. Am. Nat. 162, 427–441. doi: 10.1086/378213
Cook, E. J., Black, K. D., Sayer, M. D. J., Cromey, C. J., Angel, D. L., Spanier, E., et al. (2006). The influence of caged mariculture on the early development of sublittoral fouling communities: a pan-European study. ICES J. Mar. Sci. 63, 637–649. doi: 10.1016/j.icesjms.2005.12.007
Coolen, J. W., Boon, A. R., Crooijmans, R., Van Pelt, H., Kleissen, F., Gerla, D., et al. (2020). Marine stepping-stones: connectivity of Mytilus edulis populations between offshore energy installations. Mol. Ecol. 29, 686–703. doi: 10.1111/mec.15364
Cowen, R. K., and Sponaugle, S. (2009). Larval dispersal and marine population connectivity. Annu. Rev. Mar. Sci. 1, 443–466. doi: 10.1146/annurev.marine.010908.163757
CRAB (2007). European Best Practice in Aquaculture Biofouling. Collective Research on Aquaculture Biofouling. Available online at: https://www.yumpu.com/user/crabproject.com
FAO (2014). The State of World Fisheries and Aquaculture 2014. Rome: Food and Agricultural Organization of the United Nations.
Fernandez-Gonzalez, V. (2017). Fouling Amphipods on Marine Aquaculture Facilities: Ecological Interactions and Potential Applications. Ph.D. Dissertation. Alicante: University of Alicante.
Fernandez-Gonzalez, V., Fernandez-Jover, D., Toledo-Guedes, K., Valero-Rodriguez, J. M., and Sanchez-Jerez, P. (2014). Nocturnal planktonic assemblages of amphipods vary due to the presence of coastal aquaculture cages. Mar. Environ. Res. 101, 22–28. doi: 10.1016/j.marenvres.2014.08.001
Fernandez-Gonzalez, V., Martinez-Garcia, E., and Sanchez-Jerez, P. (2016). Role of fish farm fouling in recolonisation of nearby soft-bottom habitats affected by coastal aquaculture. J. Exp. Mar. Biol. Ecol. 474, 210–215.
Fernandez-Gonzalez, V., and Sanchez-Jerez, P. (2013). First occurrence of Caprella scaura Templeton, 1836 (Crustacea: Amphipoda) on off-coast fish farm cages in the Mediterranean sea. Helgol. Mar. Res. 68, 187–191. doi: 10.1007/s10152-013-0375-y
Fernandez-Gonzalez, V., and Sanchez-Jerez, P. (2017). Fouling assemblages associated with off-coast aquaculture facilities: an overall assessment of the Mediterranean Sea. Med. Mar. Sci. 18, 87–96.
Fernandez-Gonzalez, V., Toledo-Guedes, K., Valero-Rodriguez, J. M., Agraso, M. D. M., and Sanchez-Jerez, P. (2018). Harvesting amphipods applying the integrated multitrophic aquaculture (IMTA) concept in offshore areas. Aquaculture 489, 62–69. doi: 10.1016/j.aquaculture.2018.02.008
Fitridge, I., Dempster, T., Guenther, J., and de Nys, R. (2012). The impact and control of biofouling in marine aquaculture: a review. Biofouling 28, 649–669. doi: 10.1080/08927014.2012.700478
Giangrande, A., Lezzi, M., Del Pasqua, M., Pierri, C., Longo, C., and Gravina, M. F. (2020). Two cases study of fouling colonization patterns in the Mediterranean Sea in the perspective of integrated aquaculture systems. Aquac. Repo. 18:100455. doi: 10.1016/j.aqrep.2020.100455
Gilpin, M. (2012). Metapopulation Dynamics: Empirical and Theoretical Investigations. London: Academic Press.
Gonzalez-Silvera, D., Izquierdo-Gomez, D., Fernandez-Gonzalez, V., Martínez-López, F. J., López-Jiménez, J. A., and Sanchez-Jerez, P. (2015). Mediterranean fouling communities assimilate the organic matter derived from coastal fish farms as a new trophic resource. Mar. Pollut. Bull. 91, 45–53. doi: 10.1016/j.marpolbul.2014.12.029
Grantham, B. A., Eckert, G. L., and Shanks, A. L. (2003). Dispersal potential of marine invertebrates in diverse habitats. Ecol. Appl. 13, S108–S116.
Greene, J. K., and Grizzle, R. E. (2007). Successional development of fouling communities on open ocean aquaculture fish cages in the western Gulf of Maine, USA. Aquaculture 262, 289–301. doi: 10.1016/j.aquaculture.2006.11.003
Guenther, J., Misimi, E., and Sunde, L. M. (2010). The development of biofouling, particularly the hydroid Ectopleura larynx, on commercial salmon cage nets in Mid-Norway. Aquaculture 300, 120–127.
Gutow, L., and Franke, H. D. (2003). Metapopulation structure of the marine isopod Idotea metallica, a species associated with drifting habitat patches. Helgol. Mar. Res. 56, 259–264. doi: 10.1007/s10152-002-0126-y
Hacker, S. D., and Steneck, R. S. (1990). Habitat architecture and the abundance and body-size-dependent habitat selection of a phytal amphipod. Ecology 71, 2269–2285. doi: 10.2307/1938638
Hansson, L. (1991). Dispersal and connectivity in metapopulations. Biol. J. Linn. Soc. 42, 89–103. doi: 10.1016/b978-0-12-284120-0.50009-7
Harrison, X. A., Donaldson, L., Correa-Cano, M. E., Evans, J., Fisher, D. N., Goodwin, C. E. D., et al. (2018). A brief introduction to mixed effects modelling and multi-model inference in ecology. PeerJ 6:e4794. doi: 10.7717/PEERJ.4794
Hauser, A., Attrill, M. J., and Cotton, P. A. (2006). Effects of habitat complexity on the diversity and abundance of macrofauna colonising artificial kelp holdfasts. Mar. Ecol. Prog. Ser. 325, 93–100. doi: 10.3354/meps325093
Hedgecock, D. (1994). “Does variance in reproductive success limit effective population sizes of marine organisms,” in Genetics and Evolution of Aquatic Organisms, ed. A. Beaumont (London: Chapman & Hall), 122–134.
Highsmith, R. C. (1982). Reproduction by fragmentation in corals. Mar. Ecol. Prog. Ser. 7, 207–226. doi: 10.3354/meps007207
Hincapié-Cárdenas, C. (2007). Macrobiofouling on Open-Ocean Submerged Aquaculture Cages in Puerto Rico. Ph.D. thesis. Mayaguez: University of Puerto Rico.
Hodson, S. L., Burke, C. M., and Bissett, A. P. (2000). Biofouling of fish-cage netting: the efficacy of a silicone coating and the effect of netting colour. Aquaculture 184, 277–290.
Ito, Y. (1980). “Chapter 2 Strategies of survival,” in Comparative Ecology, 2 Edn, ed. Cambridge University Press (Cambridge: University of Cambridge), 47–94.
Jeong, S. J., Yu, O. H., and Suh, H. L. (2007). Life history and reproduction of Jassa slatteryi (Amphipoda, Ischyroceridae) on a seagrass bed (Zostera marina L.) in southern Korea. J. Crust. Biol. 27, 65–70. doi: 10.1651/s-2739.1
Kingsford, M. J., Leis, J. M., Shanks, A., Lindeman, K. C., Morgan, S. G., and Pineda, J. (2002). Sensory environments, larval abilities and local self-recruitment. Bull. Mar. Sci. 70, 309–340.
Klebert, P., Lader, P., Gansel, L., and Oppedal, F. (2013). Hydrodynamic interactions on net panel and aquaculture fish cages: a review. Ocean. Eng. 58, 260–274. doi: 10.1016/j.oceaneng.2012.11.006
Kuznetsova, A., Brockhoff, P. B., and Christensen, R. H. B. (2017). lmerTest package: tests in linear mixed effects models. J. Stat. Softw. 82, 1–26. doi: 10.18637/JSS.V082.I13
Levin, L. A. (2006). Recent progress in understanding larval dispersal: new directions and digressions. Integr. Comp. Biol. 46, 282–297. doi: 10.1093/icb/icj024
Mills, E. L. (1967). The biology of an ampeliscid amphipod crustacean sibling species pair. J. Fish Board. Can. 24, 305–355. doi: 10.1139/f67-030
Mineur, F., Cook, E. J., Minchin, D., Bohn, K., Macleod, A., and Maggs, C. A. (2012). Changing coasts : marine aliens and artificial structures. Oceanogr. Mar. Biol. 50, 189–234.
Munguia, P. (2015). Role of sources and temporal sinks in a marine amphipod. Biol. Lett. 11:20140864. doi: 10.1098/rsbl.2014.0864
Munguia, P., Mackie, C., and Levitan, D. R. (2007). The influence of stage-dependent dispersal on the population dynamics of three amphipod species. Oecologia 153, 533–541. doi: 10.1007/s00442-007-0762-7
Pawlik, J. R. (1992). Chemical ecology of the settlement of benthic marine invertebrates. Oceanogr. Mar. Biol. Annu. Rev. 30, 273–335.
Pulliam, H. R. (1988). Sources, sinks, and population regulation. Am. Nat. 132, 652–661. doi: 10.1086/284880
R Core Team (2019). R: A Language and Environment for Statistical Computing. Vienna: R Foundation for Statistical Computing.
Rumbold, C. E., Barlett, T. R., Gavio, M. A., and Obenat, S. M. (2016). Population dynamics of two invasive amphipods in the Southwestern Atlantic: Monocorophium acherusicum and Ericthonius punctatus (Crustacea). Mar. Biol. Res. 12, 268–277. doi: 10.1080/17451000.2016.1142091
Sainte-Marie, B. (1991). A review of the reproductive bionomics of aquatic gammaridean amphipods: variation of life history traits with latitude, depth, salinity and superfamily. Hydrobiologia 223, 189–227. doi: 10.1007/bf00047641
Sanz-Lázaro, C., Navarrete-Mier, F., and Marín, A. (2011). Biofilm responses to marine fish farm wastes. Environ. Pollut. 159, 825–832. doi: 10.1016/j.envpol.2010.10.035
Simons, R. D., Page, H. M., Zaleski, S., Miller, R., Dugan, J. E., Schroeder, D. M., et al. (2016). The effects of anthropogenic structures on habitat connectivity and the potential spread of non-native invertebrate species in the offshore environment. PLoS One 11:e0152261. doi: 10.1371/journal.pone.0152261
Thorson, G. (1950). Reproductive and larval ecology of marine bottom invertebrates. Biol. Rev. 25, 1–45. doi: 10.1111/j.1469-185x.1950.tb00585.x
Van der Molen, J., García-García, L. M., Whomersley, P., Callaway, A., Posen, P. E., and Hyder, K. (2018). Connectivity of larval stages of sedentary marine communities between hard substrates and offshore structures in the North Sea. Sci. Rep. 8:14772. doi: 10.1038/s41598-018-32912-2
Vázquez-Luis, M., Borg, J. A., Sanchez-Jerez, P., and Bayle-Sempere, J. T. (2012). Habitat colonisation by amphipods: comparison between native and alien algae. J. Exp. Mar. Biol. Ecol. 432, 162–170. doi: 10.1016/j.jembe.2012.07.016
Wang, Y., Neuman, U., Wright, S., and Warton, D. I. (2012). mvabund: an R package for model-based analysis of multivariate abundance data. Methods Ecol. Evol. 3, 471–474. doi: 10.1111/J.2041-210X.2012.00190.X
Keywords: marine metapopulations, amphipods, artificial habitats, colonisation, aquaculture
Citation: Fernandez-Gonzalez V, Navarro-Mayoral S and Sanchez-Jerez P (2021) Connectivity Patterns for Direct Developing Invertebrates in Fragmented Marine Habitats: Fish Farms Fouling as Source Population in the Establishment and Maintenance of Local Metapopulations. Front. Mar. Sci. 8:785260. doi: 10.3389/fmars.2021.785260
Received: 28 September 2021; Accepted: 29 October 2021;
Published: 23 November 2021.
Edited by:
Stefano Aliani, National Research Council, Consiglio Nazionale delle Ricerche (CNR), ItalyReviewed by:
Stefano Masier, Centre d’Ecologie Fonctionnelle et Evolutive, FranceAdriana Giangrande, University of Salento, Italy
Copyright © 2021 Fernandez-Gonzalez, Navarro-Mayoral and Sanchez-Jerez. This is an open-access article distributed under the terms of the Creative Commons Attribution License (CC BY). The use, distribution or reproduction in other forums is permitted, provided the original author(s) and the copyright owner(s) are credited and that the original publication in this journal is cited, in accordance with accepted academic practice. No use, distribution or reproduction is permitted which does not comply with these terms.
*Correspondence: Victoria Fernandez-Gonzalez, victoria.fernandez@ua.es