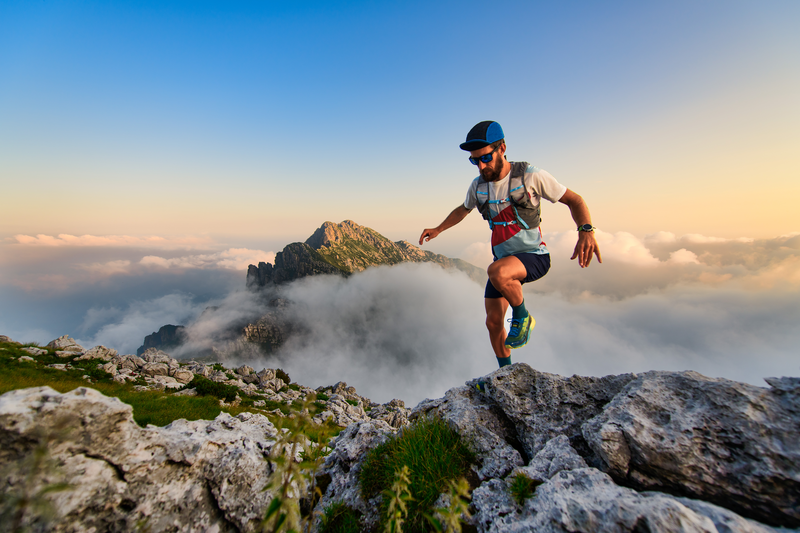
95% of researchers rate our articles as excellent or good
Learn more about the work of our research integrity team to safeguard the quality of each article we publish.
Find out more
ORIGINAL RESEARCH article
Front. Mar. Sci. , 10 December 2021
Sec. Global Change and the Future Ocean
Volume 8 - 2021 | https://doi.org/10.3389/fmars.2021.784586
Gracilariopsis lemaneiformis (G. lemaneiformis) is an important marine red macroalgae with high economic and ecological value all over the world. To date, global warming is a key issue that has a great impact on all living organisms, such as macroalgae. L-arginine (Arg) is a precursor of nitric oxide (NO) and polyamines (PAs), which can induce stress defense responses in land plants. However, its role in inducing algae resistance at high temperature (HT) is unclear. In this study, G. lemaneiformis thalli were treated with different concentrations of Arg to investigate its effect and the mechanism on the tolerance of G. lemaneiformis against HT stress. It turned out that exogenous Arg significantly alleviated the HT-induced oxidative damage as indicated by a markedly decrease in malondialdehyde (MDA) content. Notably, Arg remarkably improved the relative growth rate (RGR) and phycobiliprotein (PBP) contents of G. lemaneiformis at HT. Moreover, Arg significantly elevated the activities of antioxidant enzymes, such as superoxide dismutase (SOD), peroxidase (POD), and catalase (CAT), to efficiently scavenge reactive oxygen species (ROS). In addition, it also promoted the accumulation of free amino acids (AAs) as compared to those in the control treatment (CK) group under HT conditions. To investigate the mechanism of G. lemaneiformis to Arg, a transcriptome analysis was performed and revealed 1,414 and 3,825 differentially expressed genes (DEGs) in Arg-treated groups as compared to CK groups at 24 and 48 h of HT stress, respectively. Results showed that Arg significantly upregulated the expression of genes encoding antioxidant enzymes, heat shock proteins, and triggered transcription factors (TFs) signaling during HT stress. Moreover, Arg enhanced the DEGs involved in arginine and proline (Pro) metabolism, AAs biosynthesis, glycolysis, tricarboxylic acid (TCA) cycle, and oxidative phosphorylation. These results may help in understanding the role of Arg in G. lemaneiformis resistance to HT and provide a practical viewpoint for obtaining heat-tolerant G. lemaneiformis to further promote the development of the cultivated seaweed industry in the future.
Gracilariopsis lemaneiformis (G. lemaneiformis; Rhodophyta) is one of the most cultivated seaweeds around the world, especially in China and Indonesia (Kim et al., 2017), ranking second in Chinese cultivated macroalgae production with an annual yield exceeding 300,000 tons based on data in 2019 (China Fishery Statistics Yearbook, 2020). Gracilariopsis lemaneiformis is a valuable resource with high protein and low lipid and rich in polysaccharides, dietary fiber, minerals, essential amino acids (AAs), and trace elements (Han et al., 2012). It is not only widely used in the agar industry, but also be applied to mitigate red tide and eutrophication for marine ecological restoration (Zhou et al., 2006; Sun et al., 2018).
Gracilariopsis lemaneiformis 981, the thermo-tolerant cultivar, is still unable to survive in summer in the South China Sea area. When it is exposed to a temperature higher than 26°C, its normal metabolism will be disrupted and lead to algae decay and disease (Fu et al., 2014; Lv et al., 2019). Especially with the rising global and local temperatures, the G. lemaneiformis cultivation in the southern coast of China has been seriously affected.
High temperature (HT) exerts an adverse influence on the membrane stability of algae, ultimately reducing the algal growth rate and yield (Wang et al., 2017). The most adverse effect of HT stress is the overaccumulation of reactive oxygen species (ROS), which resulted in nucleic acids, protein, and membrane lipid peroxidation (Xu et al., 2006). However, plants can protect themselves from oxidative damage via regulating antioxidant enzymes, such as superoxide dismutase (SOD), catalase (CAT), and peroxidase (POD) (Anderson, 2002). Additionally, the enzymes, such as ascorbate peroxidase (APX), monodehydroascorbate reductase (MDAR), thioredoxin (TRX), and peroxiredoxins (PRXR), are also important in H2O2 scavenging (Pérez-Pérez et al., 2009; Smirnoff, 2010). Previous studies have demonstrated that exogenous salicylic acid and methyl jasmonate can improve heat tolerance by enhancing those genes encoding antioxidant enzymes in marine algae (Wang et al., 2017). Besides the phytohormones, AAs have also various prominent functions in plant stress response. Arginine, as one of the most functional diverse AAs in living cells, has been reported to modify plant stress tolerance through the manipulation of antioxidant mechanisms (Karpets et al., 2018). However, the roles of arginine in alleviating HT stress-induced ROS damage in G. lemaneiformis are not yet known.
Arginine is a precursor for the biosynthesis of nitric oxide (NO), polyamines (PAs), which are related to stress resistance (Liu et al., 2006). Exogenous or pre-treated with arginine has been reported its positive effect via modification of those arginine-derived stress-related substances under abiotic stress, such as heat, salinity, water deficit, and heavy metal, respectively, in rice, wheat, sugarcane, and Hyoscyamus niger (Kakkar et al., 2000; Nasibi et al., 2013; Karpets et al., 2018; Silveira et al., 2021). The application of arginine can also play a vital role in enhancing the defense resistance system against diseases in tomato fruits via its effects on NO biosynthesis and defensive enzyme activity (Zheng et al., 2011). In terms of temperature stress, exogenously applied arginine could alleviate the adverse effects of fluctuating temperature stress on maize growth and development (Matysiak et al., 2020) and enhance the seedling resistance to the damaging heating in collaboration with the NO-signaling system (Karpets et al., 2018). However, it remains unclear whether the unique roles of arginine in G. lemaneiformis are attributable to stress-related substances under HT conditions.
The present study investigated the possible involvement of exogenous arginine in G. lemaneiformis resistance to HT stress by combining physiological assays with transcriptome analysis. The aim of our study was to investigate the effect of arginine on algae growth, antioxidant enzymes, and AAs changes in G. lemaneiformis under HT stress. Further RNA-seq indicated the underlying role of arginine in regulating various processes, such as antioxidant defense, AAs biosynthesis, and energy metabolism, which allowed the algae to adapt to alleviating HT stress in G. lemaneiformis.
Gracilariopsis lemaneiformis 981 was originally collected in March 2020 with great growth, more lateral whiskers, purple-red color, no disease, and less miscellaneous algae from aquaculture base of Xiapu (26°65′N, 119°66′E), Fujian Province, China. Then algae were sampled into a bubble chamber containing ice mass and delivered to the laboratory quickly. The tender thalli (approximately 8 cm) with good growth status were washed repeatedly in filtered seawater to remove sediment and epiphytes. Materials were cultivated in an illumination incubator (GXZ-280B, Dongnan Instrument Factory, Ningbo, China) at 23°C with a photoperiod of 12 h light and 12 h darkness, and 40 μmol photons m–2 s–1 light intensity as pre-treatment, sterile seawater with a salinity of 25 was conducted as the medium and was renewed every 3 days during this period. After pretreatment for 2 weeks, the thalli were transferred into sterile seawater enriched with Provasoli medium (Provasoli, 1968).
The concentration of L-arginine (Arg; 0.5 mM) was chosen based on a preliminary test with a range of concentrations (0, 0.1, 0.5, and 1 mM) for the most effective and economic concentration on phenotypic changes. Then algae thalli were subjected to 30°C conditions (day/night = 12:12) with or without 0.5 mM Arg treatment for variable time periods. Each concentration was performed in biological triplicate. After treatment, G. lemaneiformis samples were frozen with liquid nitrogen and stored at − 80°C for subsequent experiments.
The effects of Arg on the growth of G. lemaneiformis at 30°C were based on the change of fresh weight (FW) after 7 days. The calculation formula for Relative Growth Rate (RGR) was conducted as follows:
RGR (% d–1) = ln (Wt/W0)/t × 100, where Wt represents the final weight after t days, W0 is the weight at the initial, and t refers to the treatment time (d).
For the determination of Malondialdehyde (MDA), tissues (0.1 g) were homogenized with 1 ml 10% tricarboxylic acid (TCA) solution for each sample, the supernatant was measured using the MDA test kit (Jianchengbio, Nanjing, China) after centrifuged at 8,000 g for 10 min at 4°C.
Measurement of H2O2 content and SOD, POD, and CAT enzyme activity determination can be referred to the method described by Sun et al. (2016) and Zhang et al. (2019). For the antioxidant enzyme activity, briefly, approximately 0.1 g fresh seaweeds were powdered with liquid nitrogen, then extracted separately with 1 ml extracting solution from the detection kit. After centrifugation at 8,000 g for 10 min, the absorbance at 560, 470, and 240 nm was recorded and used to calculate, respectively, according to the instructions of the manufacturer (Cominbio, Suzhou, China).
Phycoerythrin (PE) and phycocyanin (PC) were measured according to the method reported with slight modification (Wang et al., 2015). Approximately 0.1 g powder was weighed and extracted with 10 ml phosphate buffer (10 mM, pH 5.5) at 4°C. After centrifugation at 15,000 g for 30 min, the supernatant was used and determined by UV-Vis spectrophotometer (UV-6100, METASH, Shanghai, China).
The contents of PE and PC were calculated using the following formulas:
where V represents the volume of extract solution (ml), and g refers to the weight of algae powder (g).
Proline (Pro) content was determined with the reagents in assay kits (Cominbio, Suzhou, China). Approximate 0.1 g fresh seaweeds were powdered in liquid nitrogen and extracted with 1 ml extracting solution from the detection kit. After centrifugation at 8,000 g for 10 min, the absorbance of the supernatant was measured at 520 nm and used to calculate Pro content according to the instructions of the manufacturer.
An autoanalyzer based on the ninhydrin method was used to quantify the 17 free AA contents according to the Chinese National Standard (GB 5009.124-2016).
Approximately 0.2 g of fresh G. lemaneiformis was weighed into the beaker with 25 ml 0.02 mol/L HCl, the solution was passed through the C18 column after ultrasonic extraction, then the mixture was filtrated through a 0.22 μm ultrafiltration membrane (Millipore, Temecula, CA, United States), and analyzed using AA automatic analyzer (LA8080, Hitachi, Japan). The content of free AA was calculated according to the following equations: X = A × D × V1/V2/10,000/Fw. In this study, X, A, D, V1, V2, and Fw refer to AA content (g/100 g), quality of AAs in the sample solution (ng), dilution ratio, the volume of extract solution (ml), sample liquid volume (μl), and FW of samples, respectively.
Total RNA was extracted from G. lemaneiformis thalli after 30°C treatment for 24 and 48 h using the RNeasy Plant Mini Kit (Qiagen, Shanghai, China) according to the instructions of the manufacturer. Briefly, the quality and quantification of RNA were separately determined by agarose gel electrophoresis and NanoDrop 2000 spectrophotometer (Thermo Fisher Scientific, Waltham, MA, United States). More accurate RNA quantification was performed by an Agilent 2100 Bioanalyzer (Agilent Technologies, Santa Clara, CA, United States) for 28S/18S and RNA integrity number (RIN).
A total of 12 samples were performed by mRNA library preparation kit (MGIEasy, MGI, Shenzhen, China) for the establishment of the cDNA library. The mRNAs were enriched with oligo magnetic adsorption, fragmented, then reversed into double-strand cDNAs, which were linked with sequencing adaptors after purification. The single-stranded circular DNA libraries were conducted by polymerase chain reaction (PCR) amplification and then sequenced according to 50 bp single-end sequencing method by using BGISEQ-500 platform performed by Wuhan Genomic Institution (BGI, Shenzhen, China).
RNA-seq, reads filtering, and unigene annotations were performed by using SOAPnuke software (BGI, Shenzhen, China). Adaptor sequences and reads with unknown bases > 10% and low-quality reads (reads with a mass value below 15 which accounted for more than 50% of the total base number) (Cock et al., 2010) were removed to obtain clean reads. Clean reads were then mapped to a mixed reference transcriptome assembled by using HISAT (Kim et al., 2015) and Bowtie2 (Langmead and Salzberg, 2012), respectively. The expression levels of the genes and transcripts were represented by fragments per kilobase per million fragments (FPKM) mapped values and were calculated by RSEM software (Li and Dewey, 2011). The DEGs were identified using the DESeq analysis (Wang et al., 2010). The criteria of adjusted p-value ≤ 0.001 and log2 (fold change) ≥ 1 were set as the threshold for DEGs. Q value was obtained from p-value and was adjusted using KOBAS 2.0 according to the Beyer-Hardwick (BH) method (Mao et al., 2005). The significance enrichment levels of gene oncology (GO) terms and Kyoto Encyclopedia of Genes and Genomes (KEGG) pathways were set at Q value < 0.05.
Ten genes were chosen randomly from the transcriptomic results for validation by qRT-PCR. The total RNA of each sample was prepared as described in Section “RNA Isolation and cDNA Library Construction.” First-strand cDNA was synthesized from 1 μg of total RNA by reverse transcription using the HiScript II Q RT SuperMix for qPCR (Vazyme, Nanjing, China) according to the protocol of the manufacturer. qPCR was performed on an ABI7500 qRT-PCR System (Applied Biosystems, Foster City, CA, United States) and each reaction contained 10 μl ChamQ Universal SYBR qPCR Master Mix (Vazyme, Nanjing, China), 0.1 μg cDNA, and 7.5 pmol of each gene-specific primer in a final volume of 20 μl. Three independent biological replicates were used for analyses and the relative expression level of genes was determined by the 2–ΔΔCT method using 18S rRNA as the respective reference gene. Primers for qPCR are listed in Supplementary Table 1.
The data are expressed as the mean and SD. Statistical difference of data was evaluated with one-way ANOVA and Duncan’s multiple range tests at the p < 0.05 level of significance using SPSS (version 19.0, SPSS Institute, Chicago, IL, United States).
To gain insights into the possible function of Arg on G. lemaneiformis under HT stress, we analyzed the effects of different Arg concentrations (0, 0.1, 0.5, and 1 mM) on morphological changes of algal thalli under HT stress. Under prolonged stress, it showed that the algae thalli in the control treatment (CK; 0 mM Arg) group exhibited an obvious accelerated yellowing phenotype as compared with those of 0.5 and 1 mM Arg treatment, however, no obvious morphological change in 0.1 mM Arg treatment as compared with the CK group (Supplementary Figure 1A) was observed. Then, we measured the content of PBP present in the red algae, such as PE and PC. Results showed that G. lemaneiformis with 0.5 and 1 mM Arg treatment contained higher content of PBP as compared with the CK group at 7 d under HT stress treatment, while 0.1 mM Arg treatment was comparable and remained unchanged to the CK group. Both 0.5 and 1 mM Arg treatments were efficient in alleviating HT stress-triggered decrease of algal PBP, but there was no significant difference between the two concentrations (Supplementary Figures 1B,C). Thus, 0.5 mM arginine was chosen in the following experiments for economic consideration.
Exogenous application of 0.5 mM Arg was significantly decreased MDA content by 56.01% as compared with the CK group after 7 days of HT treatment (Figure 1A). Importantly, the RGR furtherly indicated that 0.5 mM Arg promoted the growth of G. lemaneiformis as compared with those without Arg treatment under HT stress (Figure 1B) since the RGR of G. lemaneiformis under HT stress (30°C) was significantly decreased by 20.45% compared with those cultivated under optimal temperature (23°C; Supplementary Figure 2). Collectively, these results indicated that the application of Arg could remarkably alleviate the membrane lipid peroxidation and the growth decline of G. lemaneiformis under HT stress.
Figure 1. Effect of L-arginine with 0.5 mM concentration on G. lemaneiformis under heat stress after 7 days. (A) Malonaldehyde (MDA) content and (B) relative growth rate (RGR). Data are means ± SD (n = 3), and different letters between different concentrations of arginine treatments indicate a significant difference (p < 0.05).
To explore the involvement of Arg in ROS scavenging against HT stress, we firstly measured the content of H2O2 in thalli under HT with or without Arg treatments after 1, 3, and 7 days of incubation. H2O2 content was continued to accumulate in algal thalli without Arg treatment, showing a significant increase at day 7, whereas the H2O2 content in the Arg group was declining in 7 days (Figure 2A).
Figure 2. Effect of L-arginine on ROS and antioxidant systems in G. lemaneiformis grown under high temperature with different treatments. (A) H2O2 content; (B) superoxide dismutase (SOD) activities; (C) catalase (CAT) activities; and (D) peroxidase (POD) activities. Data presented are the means ± SD from three independent experiments, and different letters above the columns indicate a significant difference (p < 0.05). ROS, reactive oxygen species.
We then measured the antioxidant enzyme (CAT, POD, and SOD) activities. CAT activity was remarkably activated in the Arg-treated group, reached a maximum at 3 days, with an obviously 59.11% increase in contrast to control (Figure 2C). Superoxide dismutase activity was slightly activated in the Arg-treated group as compared with the CK group at 3 days under HT stress (Figure 2B). But Arg treatment had no significant promotion in POD activity relative to CK under HT stress (Figure 2D). These results indicated that Arg could upregulate the activities of antioxidant enzymes, especially activities of CAT, thus effectively protecting the algal cells from heat damage.
To reveal the mechanism of the effect of Arg in enhancing G. lemaneiformis tolerance to HT stress, the algal thalli from both CK and Arg groups after HT treatment at 24 and 48 h were subjected to RNA-seq analysis. RNA samples were tested using the BGISEQ platform, generated 21.58 M clean reads average in each sample, and obtained a total of 23,479 unigenes, the detailed information of each sample was listed in Supplementary Table 2. The total mapping genome and gene ratio were average at 94.30 and 96.42%, respectively. Then all the unigenes were blasted against public databases (such as TFs, PRG, GO, KEGG, and NR) for identification of gene function, of which approximately 73.34% of unigenes annotated at least in one database except TFs and PRG (Supplementary Table 3).
Principal Component Analysis (PCA) was performed to describe transcriptome differences between Arg and CK groups under HT stress at 24 and 48 h. It suggested that Arg changed the transcriptome of G. lemaneiformis under HT stress, especially at 48 h (Figures 3A,B). The volcano plots of DEGs between Arg-treated and CK groups revealed a total of 1,414 unigenes (629 upregulated and 785 downregulated) at 24 h, and a total of 3,825 unigenes (2,219 upregulated and 1,606 downregulated) at 48 h (Supplementary Figure 3). To validate the reliability of the RNA-Seq results, 10 unigenes were randomly selected for RT-PCR analysis. The expression patterns of the 10 unigenes were generally in agreement with the RNA-Seq results (Supplementary Figure 4).
Figure 3. Overview of Arg-induced transcriptomic changes of G. lemaneiformis under HT stress. (A) Principal component analysis (PCA); (B) number of differentially expressed genes (DEGs) between different groups; (C) significantly enriched KEGG pathway at 24 h and (D) significantly enriched KEGG pathway at 48 h. KEGG, Kyoto Encyclopedia of Genes and Genomes.
To analyze the functions of the DEGs, GO and KEGG enrichment analyses were performed. GO analysis showed that DEGs at 24 h were enriched in membrane part, membrane, POD activity, oxidoreductase activity, an integral component of membrane, an intrinsic component of membrane, and antioxidant activity, etc. However, DEGs at 48 h were associated with the structural constituent of the cytoskeleton, generation of precursor metabolites and energy, thylakoid, and photosynthesis (Supplementary Figure 5). Kyoto Encyclopedia of Genes and Genomes enrichment analysis showed that there was only one remarkably enriched pathway at 24 h, the basal transcription factors (TFs; Figure 3C). While the top three significantly enriched KEGG pathways at 48 h were related to metabolisms and were mostly involved in AA metabolism, energy metabolism, global and overview maps, and carbohydrate metabolism (glycolysis, pyruvate metabolism, ascorbate, aldarate metabolism, and alactose metabolism; Figure 3D).
Transcriptome analysis revealed that the expression of unigenes encoding ROS scavenging enzymes was significantly changed by Arg treatment under heat stress. As shown in Figure 4, most genes had no significant difference at 24 h, but they were generally upregulated at 48 h. Genes encoding SOD (four up and one downregulated), APX (two up and one downregulated), MDAR (one upregulated), PRXR (four up and one downregulated), and TRX (seven up and one downregulated) were markedly upregulated at 48 h. However, genes (21 upregulated and 20 downregulated) encoding POD exhibited different expression patterns in G. lemaneiformis with Arg treatment under HT stress (Figure 4). These data indicated that most of the DEGs encoding ROS scavenging enzymes in G. lemaneiformis could be significantly upregulated by Arg under HT stress at 48 h treatment.
Figure 4. The clustering chart of Arg-induced DEGs related to antioxidant systems in G. lemaneiformis under high temperature. The same row represents the abundance change of one unigene at 24 h (left column) and 48 h (right column), the number in each square beside the enzyme code refers to the fold change of corresponding DEGs. Red and blue squares indicate upregulation and downregulation, separately, while white means are not expressed at this time point. DEGs, differentially expressed genes; Arg, L-Arginine.
In the present study, we found that genes involved in Heat Shock Proteins (HSPs) were markedly activated in G. lemaneiformis with Arg treatment under HT stress. Based on the results listed in Table 1, a total of four HSP families, containing 41 DEGs were identified. Out of these HSPs, the genes (24 genes, 14 upregulated) related to the HSP70 family were found to be the most abundant, followed by the HSP20 family (11 genes, 9 upregulated), HSP40 family (3 genes, 1 upregulated), and HSP90 family (3 genes, 2 upregulated) at 48 h. In summary, 26 up and 11 downregulated genes related to HSPs were dramatically activated in the Arg-treated groups, particularly at 48 h, while many of those genes were not significantly expressed at 24 h.
As shown in Table 2, many genes were annotated into TFs and sorted into 12 families, such as C2H2, TIG, HSF, and MYB. Of these genes, a total of 29 transcripts showed a significantly difference in transcript abundance compared with control, i.e., 13 and 21 upregulated genes in the Arg-treated groups at 24 and 48 h, respectively. Notably, the expression of DEGs involved in HSF was only upregulated at 24 h, and genes related to C2C2-GATA, Alfin-like, C3H, MADS, bZIP, and G2-like were significantly overexpressed at 48 h. Moreover, the five unigenes involved in TIG, FHA, and MYB were markedly upregulated at both 24 and 48 h.
The DEGs in arginine metabolism to Pro, PA, and γ-aminobutyric acid (GABA) synthesis and metabolism were significantly induced by Arg. For Pro synthesis, Pro can be converted by the glutamate metabolism via pyrroline-5-carboxylate (P5C) synthetase (P5CS, EC:2.7.2.11 1.2.1.41) and P5C reductase (P5CR, EC:1.5.1.2). We identified one DEG (Unigene4599_All) encoding P5CS and one DEG (Unigene3468_All) encoding P5CR, respectively. Arginine application did not change the expression level of P5CS, whereas upregulated the expression level of P5CR at 48 h, leading to a 1.74-fold change (Supplementary Table 4). In Pro metabolism, one unigene (Unigene12856_All) encoding 1-pyrroline-5-carboxylate dehydrogenase (P5CDH, EC:1.2.1.88) was upregulated in the Arg-treated groups at 48 h (Figure 5 and Supplementary Table 4).
Figure 5. The construction of hypothetical arginine, proline, polyamines, and GABA metabolism pathway based on the KEGG database. The solid arrow indicates one-step reaction, the dotted arrow indicates poly-step reaction and omitted intermediate steps. The same row represents the abundance change of one unigene at 24 h (left column) and 48 h (right column), the number in each square beside the enzyme code refers to the fold change of corresponding DEGs. Red and blue squares indicate upregulation and downregulation, separately, while white means are not expressed at this time point. KEGG, Kyoto Encyclopedia of Genes and Genomes; GABA, γ-aminobutyric acid.
For PA synthesis, ornithine can be converted into polyamine putrescine (PUT), then be further processed to spermidine (SPD) and spermine (SPM). In this process, it involves ornithine aminotransferase (OAT, EC:2.6.1.13), ornithine decarboxylase (ODC, EC:4.1.1.17), SPD synthase (SPDS), and SPM synthase (SPMS) enzymes. In addition, SPM can be oxidated to generate SPD by spermine oxidase (SPMO, EC:1.5.3.16). In the present study, the genes encoding OAT (one upregulated gene) and SPDS (one upregulated) exhibited different expression patterns in Arg-treated G. lemaneiformis at 48 h, while most genes were downregulated at 24 h (Figure 5 and Supplementary Table 4). In addition, the genes encoding SPMO (three upregulated) were markedly activated both at 24 and 48 h. Moreover, PA can also be obtained via methionine (Met) metabolism involving S-adenosylmethionine synthetase (SAMS, EC:2.5.1.6) and S-adenosylmethionine decarboxylase (SAMDC, EC:4.1.1.50). Most genes encoding SAMS (three up and one downregulated) and SAMDC (one upregulated gene) were upregulated to generate S-Adenosyl-methioninamine (dcSAM) at 48 h, which further regulated the activity of SPDS and SPMS to produce PA (Figure 5 and Supplementary Table 4).
For GABA synthesis, PUT can be converted into 4-amino-butanal, then be processed into GABA. During this process, it involves polyamine oxidase (PAO, EC:1.5.3.-) and aldehyde dehydrogenase (NAD+, EC:1.2.1.3). The unigenes (CL2334.Contig1_All, CL2334.Contig4_All and Unigene2164_All) encoding PAO were all significantly upregulated in the Arg-treated groups both at 24 and 48 h. Besides, genes encoding NAD + were markedly upregulated in Arg-treated G. lemaneiformis after 48 h of HT (Figure 5 and Supplementary Table 4).
Besides the change of Arg metabolic process, biosynthesis of other AA pathways was also significantly activated induced by the application of exogenous Arg.
For aspartate (Asp) biosynthesis, oxaloacetate from the TCA cycle could be converted into Asp by Asp aminotransferase (GOT2, EC: 2.6.1.1), and the unigenes (four transcripts, four upregulated) encoding GOT2 were upregulated. Asp could be converted into Met, Thr, Lys, and histidine (His), in this process, it involves threonine synthase (THRS, EC:4.2.3.1), diaminopimelate decarboxylase (LYSA, EC:4.1.1.20), 5-methyltetrahydrofolate-homocysteine methyltransferase (METH, EC:2.1.1.13), cytosolic non-specific dipeptidase (CNDP2, EC:3.4.13.18), etc. Most genes encoding THRS, LYSA, and METH were significantly upregulated (Figure 6 and Supplementary Table 5).
Figure 6. Overview of amino acids biosynthesis and free amino acids content. The number and color in square represent the transcriptome information of DEGs at 24 h and 48 h, red and blue represent content increase and decrease, separately. The solid arrow indicates one-step reaction, the dotted arrow indicates poly-step reaction, and omitted intermediate steps. The same row represents the abundance change of one unigene at 24 h (left column) and 48 h (right column), the number in each square beside the enzyme code refers to the fold change of corresponding DEGs. Red, blue, and white squares indicate upregulation, downregulation, and not expressed, separately. DEGs, differentially expressed genes.
Moreover, some AAs [serine (Ser), glycine (Gly), Cys, and Ile] could be synthesized from glycerate-3P and D-erythrose 4-phosphate through glycolysis and pentose phosphate pathways. During this process, it involves phosphoserine phosphatase (PSPH, EC: 3.1.3.3), cysteine synthase (CYSK, EC:2.5.1.47), serine-glyoxylate transaminase (AGXT, EC: 2.6.1.45), cystathionine beta-synthase (CBS, EC: 4.2.1.22), etc. Many genes encoding PSPH, CYSK, and AGXT were all remarkably upregulated in G. lemaneiformis with Arg treatment at 48 h (Figure 6 and Supplementary Table 5).
Furthermore, we measured the content of 17 free AAs (14 detected and 3 undetected) at 72 h under HT condition by an AA automatic analyzer and found that 13 AA contents were increased (Table 3). Among those upregulated AAs, Asp was the most significantly increased AA, followed by Thr, Arg, Cys, His, Ser, and Gly, leading a 5. 27-, 4. 52-, 1, 75-, 1. 4-, 1.39-1, 28-, and 1.25-fold changes compared to that of the CK treatment under HT stress, respectively. However, the stress-related AA Pro was not detected, thus we measured it in the laboratory using a different method. It showed Arg treatment significantly promoted the accumulation of Pro at 3 d, leading 2.51-fold of increase as compared with the CK group (Supplementary Figure 6). In general, the content of AAs was consistent with the gene expression of their respective encoding AA synthase.
Arginine remarkably changed the energy metabolism pathway, i.e., glycolysis, TCA cycle, and oxidative phosphorylation. In the glycolysis pathway, glucose could be converted into pyruvate to generate energy with a series of reactions, this process involves enolase (ENO, EC: 4.2.1.11) and pyruvate kinase (PK, EC: 2.7.1.40). Most genes encoding ENO (nine upregulated) and PK (two upregulated) were markedly upregulated by Arg under HT stress (Figure 7 and Supplementary Table 6). In addition, pyruvate could be converted into acetyl-CoA enter the TCA cycle by pyruvate dehydrogenase complex (PDH). The genes (19 transcripts, 14 upregulated) encoding PDH were markedly upregulated at 48 h (Figure 7 and Supplementary Table 6). Citrate synthase (CSY, EC: 2.3.3.1), isocitrate dehydrogenase (IDH, EC: 1.1.1.42), and 2-oxoglutarate dehydrogenase complex (2-OGDH) are three key enzymes of the TCA cycle. Most genes encoding CSY (two upregulated), IDH (three upregulated), and 2-OGDH (seven upregulated) were markedly upregulated in Arg treatment under HT stress at 48 h (Figure 7 and Supplementary Table 6).
Figure 7. The transcriptomic changes in the glycolysis and TCA cycle. The enzyme codes and corresponding enzyme names were listed in Supplementary Table 6. The color of red and blue squares represent upregulation and downregulation, separately, while white square means that the unigenes were not expressed in this point. The solid and dotted arrows indicate one-step and poly-step reactions, respectively. The same row represents the abundance change of one unigene at 24 h (left column) and 48 h (right column), the number in each square beside the enzyme code refers to the fold change of corresponding DEGs. Red and blue squares indicate upregulation and downregulation, separately, while white means are not expressed at this time point. DEGs, differentially expressed genes.
As for oxidative phosphorylation, exogenous Arg significantly activated the most genes at 48 h, but the majority of these genes had no significant difference at 24 h (Figure 8 and Supplementary Table 7). The genes (63 transcripts, 40 up and 23 downregulated) related to oxidative phosphorylation, such as genes encoding NADH dehydrogenase (10 upregulated genes), cytochrome c reductase (6 upregulated), cytochrome c oxidase (7 upregulated), F-type ATPase (10 upregulated), and V-type ATPase (7 upregulated), which were remarkably upregulated in Arg-treated group at 48 h (Figure 8 and Supplementary Table 7). Generally, we speculate that exogenous Arg can increase the RGR of G. lemaneiformis via promoting glycolysis, TCA cycle, and oxidative phosphorylation which possibly offers more energy.
Figure 8. The clustering chart of DEGs at oxidative phosphorylation on G. lemaneiformis. Color and number in each square indicate up- or downregulation and change level, respectively, white square means are not expressed at this time point. Note: the pattern diagram of oxidative phosphorylation is obtained from the KEGG website. DEGs, differentially expressed genes; KEGG, Kyoto Encyclopedia of Genes and Genomes.
High temperature negatively affects the morphology and physiology of algae and land plants. As for G. lemaneiformis, one of the world’s most cultivated seaweeds, an increase in temperature leads to large-scale production reduction. AAs are good candidates to be used as plant biostimulants, playing a beneficial role in growth and development under stress conditions (Haghighi et al., 2020). Especially for Arg, an AA with a high N/C ratio is involved in regulating many physiological processes under stress (Lv et al., 2020). Exogenous Arg could alleviate the adverse effects of temperature stress on maize height and the biomass of shoots and roots (Matysiak et al., 2020). However, no evidence has been found of the effects of Arg in the alleviation of HT stress in G. lemaneiformis. In the present study, the application of Arg significantly alleviated the HT stress-induced decrease in algae growth and the content of PBP, as algae not treated with Arg showed a much lower RGR and exhibited a more severe yellowing phenotype than Arg-treated algae (Figure 1B and Supplementary Figure 1). According to the results of RNA-seq analysis, Arg can activate a series of pathways genes in G. lemaneiformis, such as antioxidant system, HSPs, TFs, stress-related AAs, PAs, and energy metabolism, which may improve the heat tolerance of G. lemaneiformis, suggesting a potential role of Arg in increasing the yield of G. lemaneiformis in aquaculture as well.
During stress, ROS, such as superoxide radicals (O2–), singlet oxygen (1O2), hydrogen peroxide (H2O2), and hydroxyl radicals (OH∙), are produced, which exert a detrimental impact on plant cells and result in cellular damage and death (Thorpe et al., 2013). However, plants have evolved corresponding mechanisms to eliminate excessive ROS via ROS scavenging enzymes, such as SOD, CAT, POD, APX, MDAR, TRX, and PRXR (Anderson, 2002; Pérez-Pérez et al., 2009; Smirnoff, 2010; Das and Roychoudhury, 2014). In our study, DEG analysis showed that Arg treatment enhanced the transcript levels of most genes encoding those protective enzymes, such as SOD, APX, MDAR, PRXR, and TRX (Figure 4). Moreover, exogenous Arg reduced the content of H2O2 and elevated the activities of antioxidant enzymes, such as SOD and CAT in G. lemaneiformis under HT stress (Figure 2). However, it was an interesting phenomenon that the H2O2 content of the Arg-treated group was increased at 1 day. SPD could be catalyzed into 4-amino-butanal to produce H2O2 by PAO, and the expression of genes encoding PAO could be upregulated by Arg, which may be the reason for the H2O2 increase (Figure 5 and Supplementary Table 4). These results indicated the positive effects of Arg in regulating thermotolerance via upregulation of the antioxidant enzymes under HT stress.
Heat shock proteins, as vital cellular chaperones, play a pivotal role in conferring abiotic stress tolerance. Moreover, HSP can detoxify ROS by positively regulating the antioxidant enzymes system and improving the stability of cell membranes (ul Haq et al., 2019). At least six types of HSPs, i.e., HSP100, HSP90, HSP70, HSP60, HSP40, and small HSPs (sHSPs), have been identified in higher plants. HSP90 and HSP70 can interact with some co-chaperone proteins, such as HSP20 which regulates its activities and aid in the folding of specific substrate proteins to ameliorate heat damage and maintain cell homeostasis (Wang et al., 2004; Guo et al., 2020). HSP40 is an obligatory co-chaperone partner of HSP70 families, which provides HSP70s with the activities and functional specificity to ensure their chaperone function effectively (Craig et al., 2006). Many pieces of evidence have indicated the importance of HSPs and sHSPs in regulating high-temperature tolerance in algae, such as in red algae G. lemaneiformis, Cyanidioschyzon merolae, and green algae Chlamydomonas reinhardtii (Gu et al., 2012; Kobayashi et al., 2014). Our previous study showed that HSPs were involved in the regulation of phytohormone-mediated heat tolerance in response to heat stress (Wang et al., 2017). In the present study, most of the genes encoding HSP90, HSP70, HSP40, and sHSPs were induced by Arg application, particularly at 48 h, while many of those genes were not significantly expressed at 24 h. We speculate that these HSPs respond to the Arg-induced HT tolerance mainly at 48 h (Table 1), which is generally consistent with the expression pattern of other possibly HT-responsive DEGs analyzed in our study. Therefore, it indicated the crucial role of Arg in regulating thermotolerance via upregulation of HSPs when G. lemaneiformis responds to heat stress.
In the signaling network from the stress signals perception to the downstream expression of stress-responsive genes, TFs play an essential role (Hussain et al., 2011). TFs have also emerged as powerful tools for the manipulation of complex metabolic pathways (Hussain et al., 2011). Members of the HSF, MYB, ERF, bZIP, and WRKY TF families have already been implicated in the regulation of stress responses in algae (Strenkert et al., 2011; Ritter et al., 2014; Liang and Jiang, 2017; Ji et al., 2018). Notably, the MYB group of TFs renders tolerance to elevated temperatures, and this high tolerance is associated with enhanced AA metabolism (El-kereamy et al., 2012; Xing et al., 2021). In the present study, Arg remarkably enhanced several TF encoding genes, covering 12 families, such as HSF, C2H2, C2C2-GATA, bHLH, MADS, MYB, and bZIP (Table 2). Particularly, the transcript level of genes encoding MYB showed considerable enhancement both at 24 and 48 h in response to HT stress. It indicated that the upregulation of TFs may contribute to Arg-induced heat tolerance in G. lemaneiformis.
Polyamines, such ad PUT, SPM, and SPD, as second messengers, manifest a multifunction defensive role in conferring plant abiotic stresses tolerance (Liu et al., 2007; Hasanuzzaman et al., 2019). PA metabolism may lead to increased GABA accumulation, which also mediates plant responses to several environmental stresses (Al-Quraan, 2015). The synthesis of PA in plants requires Arg and Met metabolism (de Oliveira et al., 2018). The present study showed that Arg treatment enhanced the transcript levels of most PA synthesis-related genes (Figure 5 and Supplementary Table 4). This is similar to the results of several studies that the overexpression of PA biosynthetic genes provides increased stress tolerance in the plant (Liu et al., 2015). Moreover, exogenous application of Arg significantly upregulated the genes involved in the catabolism of PA to produce GABA (Figure 5). Then GABA from this step converts into succinate, which further enters the TCA cycle to generate more energy for stress acclimation. Collectively, these data indicated that exogenous Arg could promote PA and GABA syntheses that effectively improved the adaptive heat stress response in G. lemaneiformis.
Besides PA and GABA, Pro has also been proved that it can improve the resistance of plants under abiotic stress (Sairam et al., 2002; Haghighi et al., 2020; Wang X. et al., 2020). Algae and higher plants tend to accumulate Pro in response to salt and heavy metal stresses (Zhang et al., 2008; Cheng et al., 2014). In the present study, Arg treatments significantly increased Pro content, as compared to the non-treated group under high-temperature stress (Supplementary Figure 6) and upregulated the expression of the Pro synthesis genes (P5CR genes; Figure 5). Besides Pro, some other AAs, such as His, Ser, Met, Gly, alanine (Ala), Asp, and valine (Val), were also reported to play vital roles in the protection of plants against various stresses (Di Martino et al., 2003; Sharma and Dietz, 2006; Liu and Lin, 2020). In the present study, we measured the contents of 17 free AAs between the Arg and CK treatments under HT stress for 3 d. The levels of most AAs were increased, particularly, Asp, Thr, Arg, Ala, and His, which were markedly increased as compared to CK treatments under HT stress. This is similar to the transcriptome data that Arg upregulated the expression of AA synthesis genes encoding Gly, Cys, Ser, Pro, Met, His, Thr, Val, Asp, and Ala in the Arg-treated group, especially at 48 h (Figure 6 and Table 3). Collectively, these results indicated that Arg might promote algae HT tolerance by upregulating the synthesis of Pro and other stress-related AAs in G. lemaneiformis.
Glycolysis, TCA cycle, and oxidative phosphorylation are cellular interconnected energy metabolic pathways, which generate carbon and nitrogen skeletons, the electron transfer chain (ETC), and provide ATP for plant and algae growth or defense (Fernie et al., 2004; Fan et al., 2018). Genes related to glycolysis and the TCA cycle, such as HK, PFK, GAPDH, PK, SDH, and MDH, were reported to be involved in the tolerance to temperature stress (Dong et al., 2020; Wang L. et al., 2020). As reported, HT stress downregulated energy metabolisms, such as glycolysis/gluconeogenesis, TCA cycle, oxidative phosphorylation, and starch and sucrose metabolism (Wang et al., 2018). In the present study, Arg remarkably enhanced the energy metabolism at 48 h by activating the expression of genes encoding numerous supplementary enzymes in carbon and nitrogen metabolism (e.g., pyruvate kinase, PDH, CSY, IDH, and 2-OGDH) and electron transport chain (e.g., NADH dehydrogenase, cytochrome c reductase, cytochrome c oxidase, and ATPase; Figures 7, 8), which were consistent with the enhanced RGR of G. lemaneiformis under HT stress (Figure 1B and Supplementary Figure 1).
Exogenous Arg can alleviate the growth decline of algae at HT. It upregulated the activities of enzymatic antioxidants and promoted the accumulation of free AAs. RNA-seq analysis further revealed that Arg upregulated the expression levels of genes encoding antioxidant enzymes (SOD, APX, MDAR, PRXR, and TRX), HSPs (HSP70s, HSP90s, and sHSPs), and triggered TF signaling during HT stress. Moreover, Arg enhanced the DEGs involved in arginine metabolism (Pro, PA, and GABA synthesis), AA biosynthesis, and energy metabolism (glycolysis, TCA cycle, and oxidative phosphorylation). Collectively, these results supported that exogenous Arg could increase the yield of G. lemaneiformis at HT and revealed the mechanism of Arg to G. lemaneiformis under HT stress, providing a potential gene pool for obtaining heat-tolerant G. lemaneiformis in the future (Figure 9).
Figure 9. A proposed model for Arg-induced HT tolerance in G. lemaneiformis associated with physiological and transcriptional changes of Gracilaria lemaneiformis under HT stress. The red font indicates upregulation, green font means downregulation. HT, high temperature; PE, phycoerythrin; PC, phycocyanin; MDA, malondialdehyde; SOD, superoxide dismutase; APX, ascorbate peroxidase; TRX, thioredoxin; MDAR, monodehydroascorbate reductase; PRXR, peroxiredoxins; OAT, ornithine–oxo-acid transaminase; P5CR, pyrroline-5-carboxylate reductase; SAMDC, S-adenosylmethionine decarboxylase; GABA, γ-aminobutyric acid; PAO, polyamine oxidases; NAD+, aldehyde dehydrogenase; Asp, aspartate; His, histidine; Gly, glycine; Cys, cystine; Ser, serine; Thr, threonine; Val, valine; Ala, Alanine; PK, pyruvate kinase; PDH, pyruvate dehydrogenase; ENO, enolase; TCA, tricarboxylic acid; IDH, isocitrate dehydrogenase; CSY, citrate synthase; αKGDH, 2-oxoglutarate dehydrogenase.
The datasets presented in this study can be found in online repositories (SRA data, PRJNA774936).
SL and NX designed the study. JZ performed the experiments and drafted the manuscript. SL, XC, and XS provided substantial contributions in this period. JZ, SL, and CH analyzed the data. SL, CH, and NX made important suggestions and provided manuscript revision. All the authors contributed to the article and approved the final manuscript.
This work was supported by the National Key R&D Program of China (2018YFD0901502), the National Natural Science Foundation of China (31672674 and 32001889), the Natural Science Foundation of Zhejiang Province (LY19C190003), and the Key Program of Science and Technology of the Ningbo Government (2019B10009).
The authors declare that the research was conducted in the absence of any commercial or financial relationships that could be construed as a potential conflict of interest.
All claims expressed in this article are solely those of the authors and do not necessarily represent those of their affiliated organizations, or those of the publisher, the editors and the reviewers. Any product that may be evaluated in this article, or claim that may be made by its manufacturer, is not guaranteed or endorsed by the publisher.
We would like to thank SL, XC, and XS for helpful comments and technical assistance in the experiment. We would also like to thank SL, CH, and NX for writing—review.
The Supplementary Material for this article can be found online at: https://www.frontiersin.org/articles/10.3389/fmars.2021.784586/full#supplementary-material
Al-Quraan, N. A. (2015). GABA shunt deficiencies and accumulation of reactive oxygen species under UV treatments: insight from Arabidopsis thaliana calmodulin mutants. Acta Physiol. Plant. 37, 1–11. doi: 10.1007/s11738-015-1836-5
Anderson, J. A. (2002). Catalase activity, hydrogen peroxide content and thermotolerance of pepper leaves. Sci. Hortic Amsterdam 95, 277–284. doi: 10.1016/S0304-4238(02)00076-6
Cheng, R. L., Feng, J., Zhang, B. X., Huang, Y., Cheng, J., and Zhang, C. X. (2014). Transcriptome and gene expression analysis of an oleaginous diatom under different salinity conditions. Bioenerg. Res. 7, 192–205. doi: 10.1007/s12155-013-9360-1
China Fishery Statistics Yearbook (2020). Beijing, China: Bureau of Fisheries, Ministry of Agriculture, 45.
Cock, P. J. A., Fields, C. J., Goto, N., Heuer, M. L., and Rice, P. M. (2010). The Sanger FASTQ file format for sequences with quality scores, and the Solexa/Illumina FASTQ variants. Nucleic Acids Res. 38, 1767–1771. doi: 10.1093/nar/gkp1137
Craig, E. A., Huang, P., Aron, R., and Andrew, A. (2006). The diverse roles of J-proteins, the obligate Hsp70 co-chaperone. Rev. Physiol. Bioch. P. 156, 1–21. doi: 10.1007/s10254-005-0001-0
Das, K., and Roychoudhury, A. (2014). Reactive oxygen species (ROS) and response of antioxidants as ROS-scavengers during environmental stress in plants. Front. Env. Sci Switz. 2:53. doi: 10.3389/fenvs.2014.00053
de Oliveira, L. F., Navarro, B. V., Cerruti, G. V., Elbl, P., Minocha, R., Minocha, S. C., et al. (2018). Polyamine- and amino acid-related metabolism: The roles of arginine and ornithine are associated with the embryogenic potential. Plant Cell Physiol. 59, 1084–1098. doi: 10.1093/pcp/pcy049
Di Martino, C., Delfine, S., Pizzuto, R., Loreto, F., and Fuggi, A. (2003). Free amino acids and glycine betaine in leaf osmoregulation of spinach responding to increasing salt stress. New Phytol. 158, 455–463. doi: 10.1046/j.1469-8137.2003.00770.x
Dong, W., Ma, X., Jiang, H., Zhao, C., and Ma, H. (2020). Physiological and transcriptome analysis of Poa pratensis var. anceps cv. Qinghai in response to cold stress. BMC Plant Biol. 20:362. doi: 10.21203/rs.3.rs-25290/v1
El-kereamy, A., Bi, Y. M., Ranathunge, K., Beatty, P. H., Good, A. G., and Rothstein, S. J. (2012). The rice R2R3-MYB transcription factor OsMYB55 is involved in the tolerance to high temperature and modulates amino acid metabolism. PLoS One. 7:e52030. doi: 10.1371/journal.pone.0052030
Fan, M., Sun, X., Liao, Z., Wang, J., Li, Y., and Xu, N. (2018). Comparative proteomic analysis of Ulva prolifera response to high temperature stress. Proteome Sci. 16:17. doi: 10.1186/s12953-018-0145-5
Fernie, A. R., Carrari, F., and Sweetlove, L. J. (2004). Respiratory metabolism: glycolysis, the TCA cycle and mitochondrial electron transport. Curr. Opin. Plant Biol. 7, 254–261. doi: 10.1016/j.pbi.2004.03.007
Fu, F., Sui, Z. H., Zhou, W., Wang, J. G., Chang, L. P., and Ci, S. F. (2014). UV-irradiation mutation of tetraspores of Gracilariopsis lemaneiformis and screening of thermotolerant strains. J. Appl. Phycol. 26, 647–656. doi: 10.1007/s10811-013-0087-3
Gu, Y. H., Zhang, X., Lu, N., Zang, X. N., Zhang, X. C., and Li, G. Q. (2012). Cloning and transcription analysis of hsp70-1 and hsp70-2 of Gracilaria lemaneiformis under heat shock. Aquaculture 358, 284–291. doi: 10.1016/j.aquaculture.2012.05.040
Guo, L. M., Li, J., He, J., Liu, H., and Zhang, H. M. (2020). A class I cytosolic HSP20 of rice enhances heat and salt tolerance in different organisms. Sci Rep. UK 10:1383. doi: 10.1038/s41598-020-58395-8
Haghighi, M., Saadat, S., and Abbey, L. (2020). Effect of exogenous amino acids application on growth and nutritional value of cabbage under drought stress. Sci. Hortic Amsterdam 272:109561. doi: 10.1016/j.scienta.2020.109561
Han, T., Jiang, Z., Fang, J., Zhang, J., Mao, Y., Zou, J., et al. (2012). Carbon dioxide fixation by the seaweed Gracilaria lemaneiformis in integrated multi-trophic aquaculture with the scallop Chlamys farreri in Sanggou Bay. China. Aquacult. Int. 21, 1035–1043. doi: 10.1007/s10499-012-9610-9
Hasanuzzaman, M., Alhaithloul, H. A. S., Parvin, K., Bhuyan, M., Tanveer, M., Mohsin, S. M., et al. (2019). Polyamine action under metal/metalloid stress: Regulation of biosynthesis, metabolism, and molecular interactions. Int. J. Mol. Sci. 20:3215. doi: 10.3390/ijms20133215
Hussain, S. S., Kayani, M. A., and Amjad, M. (2011). Transcription factors as tools to engineer enhanced drought stress tolerance in plants. Biotechnol. Prog. 27, 297–306. doi: 10.1002/btpr.514
Ji, C. L., Mao, X., Hao, J. Y., Wang, X. D., Xue, J. N., Cui, H. L., et al. (2018). Analysis of bZIP transcription factor family and their expressions under salt stress in Chlamydomonas reinhardtii. Int. J. Mol. Sci. 19:2800. doi: 10.3390/ijms19092800
Kakkar, R. K., Bhaduri, S., Rai, V. K., and Kumar, S. (2000). Amelioration of NaCl stress by arginine in rice seedlings: changes in endogenous polyamines. Biol. Plantarum. 43, 419–422. doi: 10.1023/A:1026715032115
Karpets, Y. V., Kolupaev, Y. E., Lugovaya, A. A., Shvidenko, N. V., and Yastreb, T. O. (2018). Effects of nitrate and L-arginine on content of nitric oxide and activities of antioxidant enzymes in roots of wheat seedlings and their heat resistance. Russ. J. Plant Physl. 65, 908–915. doi: 10.1134/S1021443718050096
Kim, D., Landmead, B., and Salzberg, S. L. (2015). HISAT: a fast spliced aligner with low memory requirements. Nat. Methods 12, 357–U121. doi: 10.1038/nmeth.3317
Kim, J. K., Yarish, C., Hwang, E. K., Park, M., and Kim, Y. (2017). Seaweed aquaculture: cultivation technologies, challenges and its ecosystem services. Algae Seoul 32, 1–13. doi: 10.4490/algae.2017.32.3.3
Kobayashi, Y., Harada, N., Nishimura, Y., Saito, T., Nakamura, M., Fujiwara, T., et al. (2014). Algae sense exact temperatures: small heat shock proteins are expressed at the survival threshold temperature in Cyanidioschyzon merolae and Chlamydomonas reinhardtii. Genome Biol. Evol. 6, 2731–2740. doi: 10.1093/gbe/evu216
Langmead, B., and Salzberg, S. L. (2012). Fast gapped-read alignment with Bowtie 2. Nat. Methods 9, 357–U354. doi: 10.1038/nmeth.1923
Li, B., and Dewey, C. N. (2011). RSEM: accurate transcript quantification from RNA-Seq data with or without a reference genome. BMC Bioinform. 12:323. doi: 10.1186/1471-2105-12-323
Liang, M. H., and Jiang, J. G. (2017). Analysis of carotenogenic genes promoters and WRKY transcription factors in response to salt stress in Dunaliella bardawil. Sci. Rep UK. 7:37025. doi: 10.1038/srep37025
Liu, J. H., Kitashiba, H., Wang, J., Ban, Y., and Moriguchi, T. (2007). Polyamines and their ability to provide environmental stress tolerance to plants. Plant Biotechnol. Nar. 24, 117–126. doi: 10.5511/plantbiotechnology.24.117
Liu, J. H., Nada, K., Honda, C., Kitashiba, H., Wen, X. P., Pang, X. M., et al. (2006). Polyamine biosynthesis of apple callus under salt stress: importance of the arginine decarboxylase pathway in stress response. J. Exp. Bot. 57, 2589–2599. doi: 10.1093/jxb/erl018
Liu, J. H., Wang, W., Wu, H., Gong, X., and Moriguchi, T. (2015). Polyamines function in stress tolerance: from synthesis to regulation. Front. Plant Sci. 6:827. doi: 10.3389/fpls.2015.00827
Liu, L., and Lin, L. (2020). Effect of heat stress on Sargassum fusiforme leaf metabolome. J. Plant Biol. 63, 229–241. doi: 10.1007/s12374-020-09247-5
Lv, J. H., Pang, Q. Q., Chen, X. Q., Li, T., Fang, J. G., Lin, S. Y., et al. (2020). Transcriptome analysis of strawberry fruit in response to exogenous arginine. Planta 82:252. doi: 10.1007/s00425-020-03489-w
Lv, Y., Sun, P., Zhang, Y. Y., Xuan, W. Y., Xu, N. J., and Sun, X. (2019). Response of trehalose, its degrading enzyme, sucrose, and floridoside/isofloridoside under abiotic stresses in Gracilariopsis lemaneiformis (Rhodophyta). J. Appl. Phycol. 31, 3861–3869. doi: 10.1007/s10811-019-01869-8
Mao, X. Z., Cai, T., Olyarchuk, J. G., and Wei, L. P. (2005). Automated genome annotation and pathway identification using the KEGG Orthology (KO) as a controlled vocabulary. Bioinformatics 21, 3787–3793. doi: 10.2307/1592215
Matysiak, K., Kierzek, R., Siatkowski, I., Kowalska, J., Krawczyk, R., and Miziniak, W. (2020). Effect of exogenous application of amino acids L-arginine and glycine on maize under temperature stress. Agronomy Basel. 10:769. doi: 10.3390/agronomy10060769
Nasibi, F., Heidari, T., Asrar, Z., and Mansoori, H. (2013). Effect of arginine pre-treatment on nickel accumulation and alleviation of the oxidative stress in Hyoscyamus niger. J. Soil Sci. Plant. Nut. 13, 680–689. doi: 10.4067/S0718-95162013005000015
Pérez-Pérez, M. A. E., Mata-Cabana, A., Saìnchez-Riego, A. M. A., Lindahl, M., and Florencio, F. J. (2009). A comprehensive analysis of the peroxiredoxin reduction system in the Cyanobacterium Synechocystis sp. strain PCC 6803 reveals that all five peroxiredoxins are thioredoxin dependent. J. Bacteriol. 191, 7477–7489. doi: 10.1128/JB.00831-09
Provasoli, L. (1968). “Media and prospects for the cultivation of marine algae,” in Cultures and Collections of Algae. Proceedings of the US-Japan Conference, eds A. Watanabe and A. Hattori (Hakone: Japanese Society of Plant Physiologists), 63–75.
Ritter, A., Dittami, S. M., Goulitquer, S., Correa, J. A., Boyen, C., Potin, P., et al. (2014). Transcriptomic and metabolomic analysis of copper stress acclimation in Ectocarpus siliculosus highlights signaling and tolerance mechanisms in brown algae. BMC Plant Biol. 14:116. doi: 10.1186/1471-2229-14-116
Sairam, R. K., Rao, K. V., and Srivastava, G. C. (2002). Differential response of wheat genotypes to long term salinity stress in relation to oxidative stress, antioxidant activity and osmolyte concentration. Plant Sci. 163, 1037–1046. doi: 10.1016/S0168-9452(02)00278-9
Sharma, S. S., and Dietz, K. J. (2006). The significance of amino acids and amino acid-derived molecules in plant responses and adaptation to heavy metal stress. J. Exp. Bot. 57, 711–726. doi: 10.1093/jxb/erj073
Silveira, N. M., Ribeiro, R. V., de Morais, S. F. N., de Souza, S. C. R., da Silva, S. F., Seabra, A. B., et al. (2021). Leaf arginine spraying improves leaf gas exchange under water deficit and root antioxidant responses during the recovery period. Plant Physiol. Bioch. 162, 315–326. doi: 10.1016/j.plaphy.2021.02.036
Smirnoff, N. (2010). The role of active oxygen in the response of plants to water deficit and desiccation. New Phytol. 125, 27–58. doi: 10.1111/j.1469-8137.1993.tb03863.x
Strenkert, D., Schmollinger, S., Sommer, F., Schulz-Raffelt, M., and Schroda, M. (2011). Transcription factor-dependent chromatin remodeling at heat shock and copper-responsive promoters in Chlamydomonas reinhardtii. Plant Cell. 23, 2285–2301. doi: 10.2307/41433466
Sun, X., Wu, J., Wang, G. C., Kang, Y. N., Ooi, H. S., Shen, T. T., et al. (2018). Genomic analyses of unique carbohydrate and phytohormone metabolism in the macroalga Gracilariopsis lemaneiformis (Rhodophyta). BMC Plant Biol. 18:94. doi: 10.1186/s12870-018-1309-2
Sun, Y., Wang, H., Liu, S., and Peng, X. (2016). Exogenous application of hydrogen peroxide alleviates drought stress in cucumber seedlings. S. Afr. J. Bot. 106, 23–28. doi: 10.1016/j.sajb.2016.05.008
Thorpe, G. W., Reodica, M., Davies, M. J., Heeren, G., Jarolim, S., Pillay, B., et al. (2013). Superoxide radicals have a protective role during H2O2 stress. Mol. Biol. Cell. 24, 2876–2884. doi: 10.1091/mbc.E13-01-0052
ul Haq, S., Khan, A., Ali, M., Khattak, A. M., Gai, W. X., Zhang, H. X., et al. (2019). Heat shock proteins: Dynamic biomolecules to counter plant biotic and abiotic stresses. Int. J. Mol. Sci. 20:5321. doi: 10.3390/ijms20215321
Wang, F., Wang, C., Zou, T., Xu, N., and Sun, X. (2017). Comparative transcriptional profiling of Gracilariopsis lemaneiformis in response to salicylic acid- and methyl jasmonate-mediated heat resistance. PLoS One. 12:e0176531. doi: 10.1371/journal.pone.0176531
Wang, L., Ma, K. B., Lu, Z. G., Ren, S. X., Jiang, H. R., Cui, J. W., et al. (2020). Differential physiological, transcriptomic and metabolomic responses of Arabidopsis leaves under prolonged warming and heat shock. BMC Plant Biol. 20:86. doi: 10.1186/s12870-020-2292-y
Wang, L. K., Feng, Z. X., Wang, X., Wang, X. W., and Zhang, X. G. (2010). DEGseq: an R package for identifying differentially expressed genes from RNA-seq data. Bioinformatics 26, 136–138. doi: 10.1093/bioinformatics/btp612
Wang, W., Teng, F., Lin, Y., Ji, D., Xu, Y., Chen, C., et al. (2018). Transcriptomic study to understand thermal adaptation in a high temperature-tolerant strain of Pyropia haitanensis. PLoS One. 13:e0195842. doi: 10.1371/journal.pone.0195842
Wang, X., Xia, J. B., and Cao, X. B. (2020). Physiological and ecological characteristics of Periploca sepium Bunge under drought stress on shell sand in the Yellow River Delta of China. Sci. Rep UK 10:9567. doi: 10.1038/s41598-020-66717-z
Wang, Y., Feng, Y., Wang, H., Zhong, M., Chen, W., and Du, H. (2015). Physiological and proteomic analyses of two Gracilaria lemaneiformis strains in response to high-temperature stress. J. Appl. Phycol. 28, 1847–1858. doi: 10.13140/RG.2.2.11576.44800
Wang, W. X., Vinocur, B., Shoseyov, O., and Altman, A. (2004). Role of plant heat-shock proteins and molecular chaperones in the abiotic stress response. Trends Plant Sci. 9, 244–252. doi: 10.1016/j.tplants.2004.03.006
Xing, G. L., Li, J. Y., Li, W. L., Lam, S. M., Yuan, H. L., Shui, G. H., et al. (2021). AP2/ERF and R2R3-MYB family transcription factors: potential associations between temperature stress and lipid metabolism in Auxenochlorella protothecoides. Biotechnol. Biofuels 14:22. doi: 10.1186/s13068-021-01881-6
Xu, S., Li, J. L., Zhang, X. Q., Wei, H., and Cui, L. J. (2006). Effects of heat acclimation pretreatment on changes of membrane lipid peroxidation, antioxidant metabolites, and ultrastructure of chloroplasts in two cool-season turfgrass species under heat stress. Environ. Exp. Bot. 56, 274–285. doi: 10.1016/j.envexpbot.2005.03.002
Zhang, L. P., Mehta, S. K., Liu, Z. P., and Yang, Z. M. (2008). Copper-induced proline synthesis is associated with nitric oxide generation in Chlamydomonas reinhardtii. Plant Cell Physiol. 49, 411–419. doi: 10.1093/pcp/pcn017
Zhang, X., Hu, C., Sun, X., Zang, X., Zhang, X., Fang, T., et al. (2019). Comparative transcriptome analysis reveals chitooligosaccharides-induced stress tolerance of Gracilariopsis lemaneiformis under high temperature stress. Aquaculture 519:734876. doi: 10.1016/j.aquaculture.2019.734876
Zheng, Y., Sheng, J., Zhao, R., Zhang, J., Lv, S., Liu, L., et al. (2011). Preharvest L-arginine treatment induced postharvest disease resistance to Botrysis cinerea in tomato fruits. J. Agr. Food Chem. 59, 6543–6549. doi: 10.1021/jf2000053
Keywords: Gracilariopsis lemaneiformis, L-arginine, high-temperature stress, heat resistance, transcriptome
Citation: Zhang J, Liu S, Hu C, Chen X, Sun X and Xu N (2021) Physiological and Transcriptome Analysis of Exogenous L-Arginine in the Alleviation of High-Temperature Stress in Gracilariopsis lemaneiformis. Front. Mar. Sci. 8:784586. doi: 10.3389/fmars.2021.784586
Received: 28 September 2021; Accepted: 01 November 2021;
Published: 10 December 2021.
Edited by:
Peng Jin, University of Guangzhou, ChinaReviewed by:
Yong Zhang, Fujian Normal University, ChinaCopyright © 2021 Zhang, Liu, Hu, Chen, Sun and Xu. This is an open-access article distributed under the terms of the Creative Commons Attribution License (CC BY). The use, distribution or reproduction in other forums is permitted, provided the original author(s) and the copyright owner(s) are credited and that the original publication in this journal is cited, in accordance with accepted academic practice. No use, distribution or reproduction is permitted which does not comply with these terms.
*Correspondence: Shixia Liu, bGl1c2hpeGlhQG5idS5lZHUuY24=; Nianjun Xu, eHVuaWFuanVuQG5idS5lZHUuY24=
Disclaimer: All claims expressed in this article are solely those of the authors and do not necessarily represent those of their affiliated organizations, or those of the publisher, the editors and the reviewers. Any product that may be evaluated in this article or claim that may be made by its manufacturer is not guaranteed or endorsed by the publisher.
Research integrity at Frontiers
Learn more about the work of our research integrity team to safeguard the quality of each article we publish.