- 1Laboratorio de Ecología Molecular, Facultad de Ciencias, Instituto de Ecología y Biodiversidad (IEB), Universidad de Chile, Santiago, Chile
- 2Facultad de Ciencias, Escuela de Medicina Veterinaria, Universidad Mayor, Santiago, Chile
- 3Centro de Investigación Eutropia, Santiago, Chile
- 4Millennium Institute Biodiversity of Antarctic and Subantarctic Ecosystems (BASE), Santiago, Chile
- 5Centro de Estudios Avanzados en Zonas Áridas (CEAZA), La Serena, Chile
- 6Marine Mammal Institute, Oregon State University, Newport, OR, United States
- 7MARBEC, Université de Montpellier-CNRS-IFREMER-IRD, Séte, France
- 8Centre d’Etudes Biologiques de Chizé (CEBC) CNRS-ULR, UMR 7273, Villiers-en-Bois, France
- 9Littoral Environnement et Sociétés (LIENSs), UMR 7266, CNRS-Université de la Rochelle, La Rochelle, France
- 10South Atlantic Environmental Research Institute (SAERI), Stanley Cottage, Stanley, Falkland Islands
- 11Laboratorio de Mamíferos Marinos, Centro para el Estudio de Sistemas Marinos (CESIMAR-CONICET), Puerto Madryn, Argentina
Commerson’s dolphins (Cephalorhynchus commersonii) are separated into the subspecies C. c. commersonii, found along southern South America (SA) and the Falkland Islands/Islas Malvinas (FI/IM), and C. c. kerguelenensis, restricted to the subantarctic Kerguelen Islands (KI). Following the dispersal model proposed for the genus, the latter is thought to have originated from SA after a long-distance dispersal event. To evaluate this biogeographic scenario, a distribution-wide, balanced sampling of mitochondrial DNA (mtDNA) control region sequences was designed. New tissue samples from southern Chile, Argentina, FI/IM, and KI were added to published sequences from SA and KI, for a total of 256 samples. Genetic diversity indices, genetic and phylogeographic structure, and migration rates were calculated. One haplotype was shared between subspecies, with which all haplotypes of C. c. kerguelenensis formed a distinct group in the haplotype network. A new haplotype for C. c. kerguelenensis is reported. Differentiation in haplotype frequencies was found among localities within the distribution of C. c. commersonii, yet the phylogeographic signal was only statistically significant between subspecies. Coalescent-based historical gene flow estimations indicated migration between the northern and southern portions of the species’ range in SA as well as between SA and the FI/IM, but not between these and the KI. The net nucleotide divergence between dolphins from SA and the FI/IM was lower than the recommended threshold value suggested for delimiting subspecies, unlike that found between C. c. commersonii and C. c. kerguelenensis. The results are consistent with the model of post-glacial colonization of KI by South American C. commersonii, followed by an ongoing divergence process and subspecies status. Thus, C. c. kerguelenensis may represent the most recent diversification step of Cephalorhynchus, where isolation from their source population is driving a process of incipient speciation.
Introduction
Past climate fluctuations are known to have influenced the distribution of many species (Hewitt, 2000), as well as their population genetic structure (Collin and de Maintenon, 2002; Taylor and Hellberg, 2006). The late Pleistocene presented alternating glacial and interglacial periods which had an impact on a global scale (Mackensen, 2004) and shaped the geographic distribution and diversification of several marine organisms, especially within coastal and benthic habitats (Bennett, 1990; Thatje et al., 2005). Glaciations during the late Pleistocene induced changes in the distribution of pinnipeds, such as the South American fur seal (Arctocephalus australis), and the South American sea lion (Otaria byronia) (Túnez et al., 2013, 2010; Oliveira et al., 2017). Changes in the geographic range of southern elephant seals (Mirounga leonina) during the Holocene are thought to have been driven by similar processes (de Bruyn et al., 2009). Such climatic and oceanographic variations have also influenced the current distribution patterns of cetacean species. For example, demographic inference based on mitochondrial DNA (mtDNA) suggests that after glacial events during the Pleistocene white-beaked dolphins (Lagenorhynchus albirostris) expanded into the North Atlantic (Banguera-Hinestroza et al., 2010). In a similar manner, it has been shown that the population expansion of beluga whales (Delphinapterus leucas) into the western Nearctic and their dispersal routes from glacial refuges relates to the retreat of the Pleistocene ice cover (O’Corry-Crowe et al., 1997).
Climatic variations have also shaped the distribution and genetic population patterns of dolphins in the genus Cephalorhynchus. This genus comprises four small-sized species which live in coastal, temperate to subantarctic waters around the Southern Hemisphere (Dawson, 2018). According to the radiation model proposed for this genus, speciation was promoted by periods of changing climate, and followed the direction of the West Wind Drift, ultimately giving rise to the current discontinuous distribution of the four recognized species (Pichler et al., 2001). This process is thought to have started in southern Africa, where presently Heaviside’s dolphins (C. heavisidii) occur off the west coast of South Africa and Namibia. Subsequently, Cephalorhynchus arrived at the inshore waters of New Zealand, giving rise to Hector’s dolphins (C. hectori) and later diverging into two subspecies, C. hectori hectori in the South Island and C. hectori maui in the North Island (Baker et al., 2002). The radiation process of the genus continued eastward across the South Pacific, arriving at southern South America (SA) where there are now two recognized species, the Chilean dolphin (C. eutropia) and the Commerson’s dolphin (C. commersonii). Chilean dolphins inhabit the coastal waters of central and southern Chile, from Valparaíso to Isla Navarino (Goodall, 1994) and a few have also been documented on the Atlantic coast at Puerto Deseado, Argentina (Morgenthaler et al., 2014). Their current genetic structure along the Chilean coastline is consistent with predictions from the Expansion-Contraction biogeographic model with a poleward post-glacial shift (Pérez-Alvarez et al., 2016).
Commerson’s dolphins are mainly found along the coast of Argentina south to the central and eastern Strait of Magellan, and the Falkland Islands/Islas Malvinas (FI/IM) (Brownell and Praderi, 1985; Dawson, 2018). It has been suggested that Commerson’s dolphins in the latter region might have diverged significantly from their conspecifics off SA, even corresponding to a separate subspecies (Cipriano et al., 2011). Commerson’s dolphins are also found 8,500 km away from SA, in the coastal waters of the subantarctic Kerguelen Islands (KI) in the southern Indian Ocean (Dawson, 2018). This wide gap in distribution is in marked contrast with the distribution of the other Cephalorhynchus species and makes the geographic range of C. commersonii the broadest in the genus (Goodall et al., 1988). In addition to the conspicuous geographical distance between SA and KI, morphological and coloration differences (Robineau, 1984) as well as acoustic differences (Dziedzic and De Buffrenil, 1989) have been noted between individuals of both areas. Some of these lines of evidence led to a subspecies proposal (Robineau, 1986) and a subsequent formal description as C. c. kerguelenensis, based on morphological, skeletal and genetic characters (Robineau et al., 2007). Following the diversification model proposed for the genus, C. c. kerguelenensis is thought to have originated around 10,000 years ago through the establishment of a few migrants from SA (Pichler et al., 2001), akin to a long-distance dispersal and an establishment model (as defined by Crisp et al., 2011). This model represents an allopatric speciation process, originating with an exceptional dispersal event, from which a new population then establishes across a barrier at a sufficient distance to limit gene flow between the two populations (Crisp et al., 2011). Since the subspecies description, additional studies of the genetic diversity of C. commersonii have been conducted along the southern Argentine coast (Pimper et al., 2010; Cipriano et al., 2011). However, no further investigation has been conducted on the population genetic structure over the complete distribution of the two subspecies.
To explore the establishment of C. commersonii in the KI and to test whether genetic data support the proposed long-distance dispersal and establishment model, we designed a geographically extensive, balanced sampling scheme throughout the global distribution of Commerson’s dolphins using new and published mtDNA control region sequences. We included samples from the previously unsurveyed areas of FI/IM, southern Chile (Fitzroy), Argentina (Bahía Camarones, Punta Quilla, and Monte León), new samples from Puerto Deseado (Argentina), and additional samples from the KI. To complete a general phylogeographic overview of the species, we combined this new information with published sequences from the Strait of Magellan, Tierra del Fuego (Argentina), and the KI.
Materials and Methods
Origin of Samples
Skin samples were collected with a biopsy dart from Commerson’s dolphins in Fitzroy (Chile, 52°43.28′ S, 71°23.32′ W), the FI/MI (52°8.85′ S, 60°1.77′ W), and the KI (49°25.82′ S, 70°10.75′ W) and stored in 90% ethanol. Samples from the Argentine localities of Bahía Camarones (45°2.61′ S, 65°33.68′ W), Puerto Deseado (47°45.97′ S, −65°49.83′ W), Punta Quilla (50°30.84′ S, 68°58.39′ W), and Monte León (50°8.38′ S, 68°18.13′ W) were collected by skin “swabbing” from dolphins (Harlin et al., 1999). In order to obtain a balanced, distribution-wide sampling of sequences, we also included previously published sequences from missing or under-sampled areas in the distribution of this species, particularly 49 from SA off the Strait of Magellan, Chile, Tierra del Fuego (site Area A3), and Argentina (Pimper et al., 2010; Cipriano et al., 2011) and 11 from the KI (Robineau et al., 2007). Haplotype frequencies were retrieved from their respective publications. We decided against including additional published sequences because their geographic proximity to some of our sampling sites could potentially imbalance our analyses.
Mitochondrial Control Region Amplification
Laboratory analyses were conducted at the Universidad de Chile, for samples collected in SA and the KI; at the Molecular Core Facility at the Université de La Rochelle for samples collected at the KI; and at and at Oregon State University for samples collected in the FI/IM. DNA extractions were performed on the tissue samples following either a modified salt-extraction protocol (Aljanabi and Martinez, 1997), with an additional proteinase K digestion, or a standard phenol/chloroform extraction (e.g., Pichler et al., 2001). Amplification of the mtDNA control region was done with the primers M13 Dlp1.5 5′-TGTAAAACGACAGCCAGTTCACCCAAAGCTGRARTTCTA-3′ (forward) and 8G 5′-GGAGTACTATGTCCTGTAACCA-3′ (reverse) (Dalebout et al., 2005). We performed the amplifications in a total reaction volume of 25.6 μL, consisting of 12.7 μL water, 5 μL 10X Buffer (Invitrogen), 2 μL 50 mM MgCl2 (Invitrogen), 2 μL 10 pM dNTPs (Invitrogen), 1 μL 10 pM of each primer (2 μL total), 0.5 μL Taq Polymerase (recombinant) and Platinum (Invitrogen), and 70–150 ng of DNA. Amplification was conducted with the following profile: preliminary denaturation of 2 min at 94°C; followed by 30 cycles of, denaturation for 30 s at 94°C, annealing for 40 s at 56°C, polymerase extension for 40 s at 72°C; and a final polymerase extension for 10 min at 72°C and an infinite hold temperature of 4°C. Each PCR amplification included positive and negative controls.
PCR products were run on a 1% agarose gel, stained with either EtBr or 0.3% GelRed and bands were visualized under UV light. PCR products were purified and sequenced in both directions either at Oregon State University or Macrogen Inc., Seoul, South Korea, on a 3730XL DNA Analyzer (Applied Biosystems).
Genetic Diversity and Genetic Structure
We manually aligned all sequences and visually checked polymorphic sites either in Sequencher 4.7 (GeneCodes) or in Proseq 3.5 (Filatov, 2009). A Median-Joining haplotype network was constructed in Network 10.2.0.01 (Bandelt et al., 1999) and the final figure was prepared in Popart (Leigh and Bryant, 2015). We estimated the following genetic diversity indices in Dnasp 5.10.01 (Librado and Rozas, 2009) for each sampling locality: number of haplotypes (h), number of polymorphic sites (S), haplotype diversity (Hd), nucleotide diversity (π), and pairwise differences between sequences (Π). We evaluated pairwise population genetic differentiation between sampling localities in Arlequin 3.5.2 (Excoffier and Lischer, 2010) by calculating both genetic structure (FST) and phylogeographic structure (ΦST) indices. Statistical significance of both indices was evaluated with 1,000 permutations and a significance level of 0.05, and we generated False Discovery Rates adjusted p-values to correct for multiple comparisons in each analysis (Pike, 2011). To explore genetic and phylogeographic structure at a larger scale, we organized the sampling localities in two additional ways: the first followed a geographic criterion and considered three groups (SA, FI/IM, and KI) and the second grouping criterion followed the distribution of each subspecies and considered two groups, C. c. commersonii (SA and FI/IM) and C. c. kerguelenensis (KI). With these two grouping schemes, an Analysis of Molecular Variance (AMOVA) (Excoffier et al., 1992) was performed to assess the portion of genetic variance among-groups, among-populations within groups and within-population (Excoffier et al., 1992) and to evaluate if and how the distribution of variance changes if FI/IM samples are treated a separate group from SA samples. Similarly, we used Permut 2.0 (Pons and Petit, 1996) to test for the existence of phylogeographic structure between geographic areas (SA, FI/IM, and KI) and between subspecies. This was achieved by permuting the calculated FST and ΦST values 10,000 times. Since FST is a genetic differentiation index between populations based on haplotype frequencies, and ΦST considers both haplotype frequencies and genetic distance between haplotypes, a higher value of ΦST compared to FST would indicate the existence of significant phylogeographic structure. The percentage of permutations equal or higher than the observed ΦST value determines the p-value. Finally, with the aim of evaluating the delimitation of cetacean subspecies using genetic data (Taylor et al., 2017) we calculated net nucleotide divergence (dA) (Nei, 1987) in DnaSP. All new samples were checked for duplicates by either identifying individual markings in the field and/or by genotyping microsatellites (Fitzroy, KI, and FI/IM).
Historical Biogeography and Migration Rates
For the estimation of effective population size (Ne) and migration rates with the software Lamarc 2.1.10 (Kuhner, 2006; Kuhner and Smith, 2007), we grouped sampling locations in continental SA following a biogeographical criterion (Balech and Ehrlich, 2008) rather than genetic differentiation indices. Low sample sizes and matrilineal effects may generate genetic structures at small geographic scales that do not necessarily reflect a migration process. The samples from central Argentina Bahía Camarones, Puerto Deseado, and Monte León/Punta Quilla were included into a group named “northern SA” (NSA), while samples from the Strait of Magellan, Fitzroy, and Tierra del Fuego were pooled into “southern SA” group (SSA). FI/IM and KI were each kept as a separate group. Lamarc estimates these and other population parameters using coalescence theory, sampling genealogies from a tree space using a Metropolis Monte Carlo sampling technique. Likelihood searches used a MCMC approach with 12 runs of 20 short chains of 1,000 steps and three long chains of 20,000 steps, with a burn-in of 10,000 genealogies. We present the mean and standard deviation of the estimated Ne and Nm values obtained from 12 runs. Ne was calculated as twice the estimated female effective population size (Nef) obtained through Lamarc’s estimation of θ = 2Nef, and using a substitution rate of 5.8% for the mtDNA control region (Alter and Palumbi, 2009).
Results
DNA Sequences
Mitochondrial DNA control region haplotypes were successfully amplified and sequenced from skin samples collected in Fitzroy, Chile (n = 7); Bahía Camarones (n = 37), Puerto Deseado (n = 41), Punta Quilla (n = 25), and Monte León (n = 11) along the Argentine coastline; FI/IM (n = 52) and KI (n = 24). Additionally, we retrieved sequences from previously published work, particularly from three areas in the Strait of Magellan (n = 28), Tierra del Fuego (particularly 21 samples from sampling site called “area A3′’ in the study) (Pimper et al., 2010; Cipriano et al., 2011) and the KI (n = 11) (Robineau et al., 2007). The final dataset comprised a total of 256 control region sequences (Table 1) from sampling points throughout the distribution of the species (Figure 1). We edited the sequences retrieved from Robineau et al. (2007) in order to correct for sequencing errors as described by Pimper et al. (2010). Before calculating diversity indices and conducting analyses, we grouped the samples from Punta Quilla, and Monte León together (n = 36) as well as Fitzroy and the Strait of Magellan (n = 35), in both cases due to their geographical proximity and small sample size (Table 1).
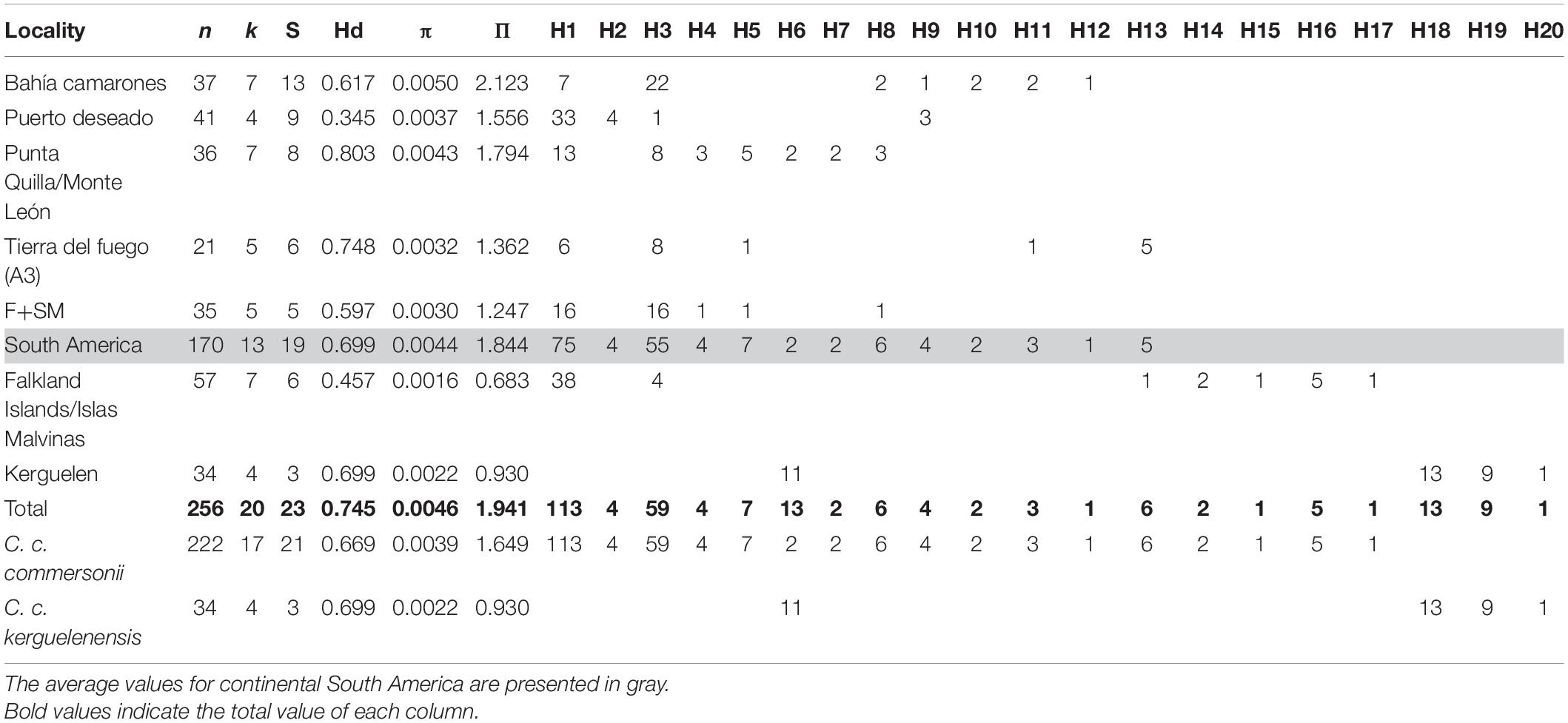
Table 1. Genetic diversity indices per locality and Cephalorhynchus commersonii subspecies: number of sequences (n), number of haplotypes (k), number of polymorphic sites (S), haplotype diversity (Hd), nucleotide diversity (π), pairwise differences between sequences (Π), and frequency of each found haplotype (H1-20) per locality.
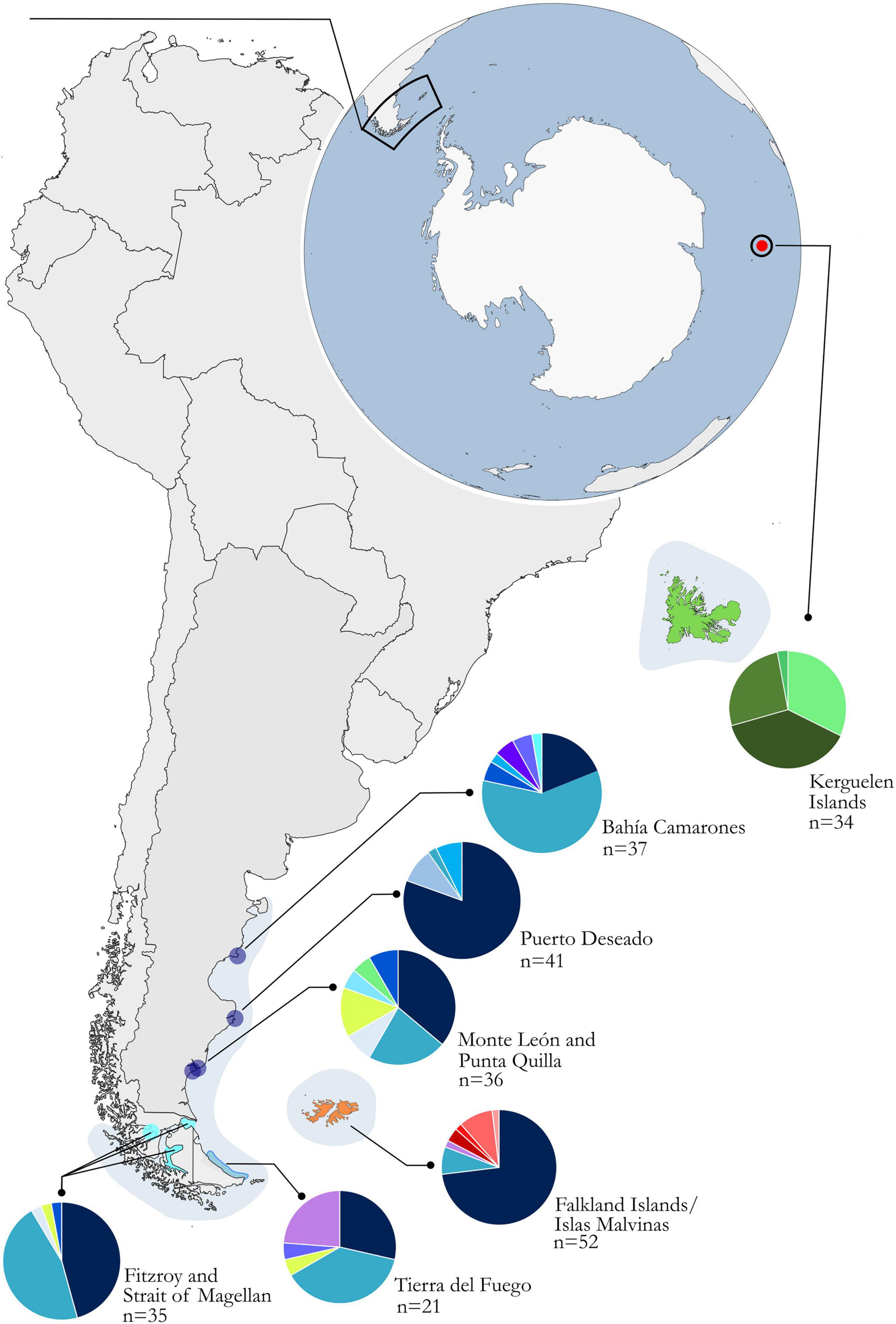
Figure 1. Geographic origin of samples within the distribution of Cephalorhynchus commersonii. Pie charts of each locality detail the respective haplotype frequencies. Haplotype frequencies are available in Table 1. The localities of Bahía Camarones, Puerto Deseado, and Punta Quilla with Monte León are included in the greater group of Northern South America (dark blue); Tierra del Fuego (blue), and Fitzroy and Strait of Magellan (light blue) in the group Southern South America; Falkland Islands/Islas Malvinas (orange) and Kerguelen Islands (green). Map adapted from FreeVectorMaps.com (https://freevectormaps.com).
The initial exploration of the haplotype network revealed two hypervariable sites, located at the base pairs 325 and 420. These sites were excluded as they produced several non-informative loops in the haplotype network and five median vectors (nodes which represent hypothesized ancestral haplotypes), affecting its interpretation. Nevertheless, the network including both hypervariable sites is shown in the Supplementary Material (Supplementary Figure 1). The final consensus fragment presented a length of 422 base pairs.
Genetic Diversity
From the 256 sequences, we identified 20 haplotypes defined by 23 variable sites (Figure 2 and Table 1). The combined South American dataset (n = 170) contained 13 haplotypes, while the FI/IM (n = 52) contained seven haplotypes and the KI (n = 35) contained four haplotypes. Two haplotypes, H1 (n = 113) and H3 (n = 59), were found in most individuals sequenced (n = 172 of 256) and were shared by all localities excluding KI (Figure 2 and Table 1).
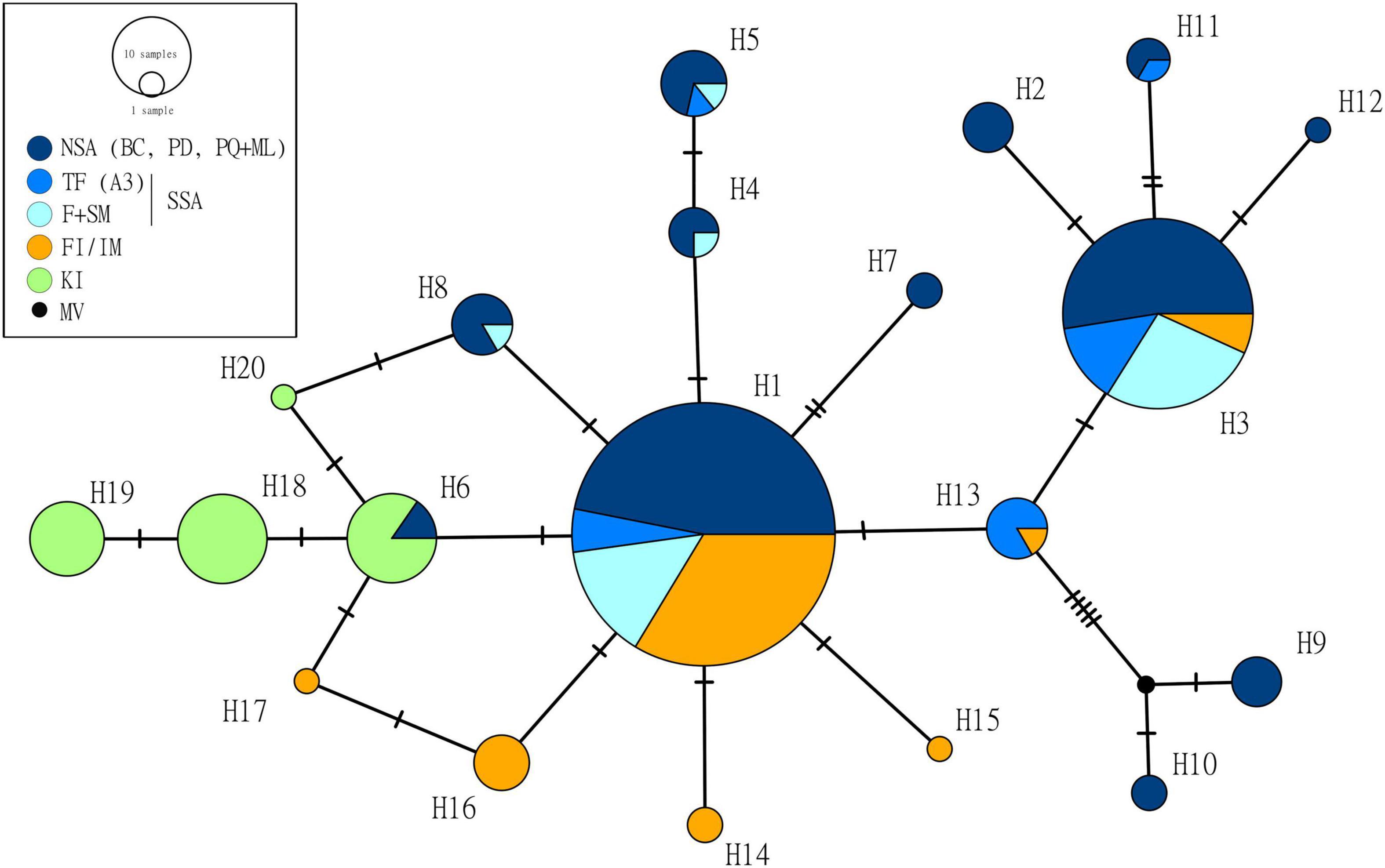
Figure 2. Haplotype network of the 256 sequences. To focus on the greater distribution-wide patterns, localities were color-coded to broadly show their origin within the distribution of Commerson’s dolphins, as in the map: NSA (dark blue): Bahía Camarones (BC), Puerto Deseado (PD), and Punta Quilla and Monte León (PQ + ML); SSA ARG: Tierra del Fuego (A3, blue), and Fitzroy and Strait of Magellan (F + SM, light blue), Falkland Islands/Islas Malvinas (FI/IM, orange), and Kerguelen Islands (KI, green). MV, median vector. Transversal dashes indicate one mutational step. Haplotype frequencies are available in Table 1.
Three haplotypes (H1, H3, and H13) were shared between SA and the FI/IM and one (H6) between SA (Punta Quilla) and the KI (Figure 2). This haplotype was reported previously by Pimper et al. (2010) (haplotype Ccom-M) in a sample from a locality in Tierra del Fuego (TDF-A2 in that study). However, this locality (and hence the haplotype) was not included because of its too large sample size (n = 92), which would have unbalanced our sampling, and its proximity to an area which was already included (TDF-A3). No haplotypes were shared between the FI/IM and the KI.
South America contained the highest number of private haplotypes (n = 9), while the FI/IM and the KI had four and three, respectively (Table 1). Populations with the lowest and highest genetic diversities were both found in SA, Puerto Deseado (Hd = 0.345), and Punta Quilla/Monte León (Hd = 0.803). The genetic diversity of FI/IM was lower than KI (Hd = 0.457 vs. 0.699) despite having almost twice as many haplotypes (k = 7 vs. 4) (Table 1). Unlike the other locations, the KI was not comprised of one common haplotype but rather three haplotypes of relatively equal frequencies (n = 11, 13, and 9) and one low-frequency haplotype. Overall, haplotypes from SA and the FI/IM appear to be homogeneously distributed in the haplotype network. In contrast, samples from KI appear to cluster together, forming a distinct haplogroup (Figure 2). Overall, the two subspecies had similar genetic diversity (C. c. commersonii Hd = 0.669 vs. C. c. kerguelenensis Hd = 0.699) (Table 1).
Genetic Structure and Genetic Differentiation
Among the 21 possible genetic structure comparisons (FST), we found only six to be non-significant. Five of these occurred between South American sampling sites, while one was found between the FI/IM and Puerto Deseado, Argentina (Table 2). Similarly, the only two statistically non-significant phylogeographic structure comparisons occurred within SA (Table 2). All FST and ΦST comparisons involving the FI/IM and the KI were statistically significant, apart from FI/IM and Puerto Deseado (FST). The KI showed most of the highest structure values, which was especially evident in the phylogeographic structure analysis (Table 2). Statistical significance did not change after the FDR correction for multiple comparisons. The AMOVA analysis showed that a higher percentage of the among groups variance was explained by grouping samples according to subspecies, rather than following the geographic grouping (i.e., SA, FI/IM, and KI). Respective percentages of variation were 47.83% for the subspecies grouping, and 31.18% for the geographic grouping criterion (Table 3). The test for phylogeographic signal performed in PERMUT between SA and the FI/IM was not statistically significant (p = 0.107). Conversely, this test was significant when comparing subspecies (SA and FI/IM against KI), as no simulated phylogeographic structure value was equal or larger than the observed value. Finally, for the calculations of net nucleotide divergence between areas, for SA and FI/IM we obtained dA = 0.051%; between SA and KI dA = 0.424%; while between FI/IM and KI we obtained a value of dA = 0.346%. The global net nucleotide divergence value we obtained between the two subspecies, i.e., SA and the FI/IM against KI, was dA = 0.396%.
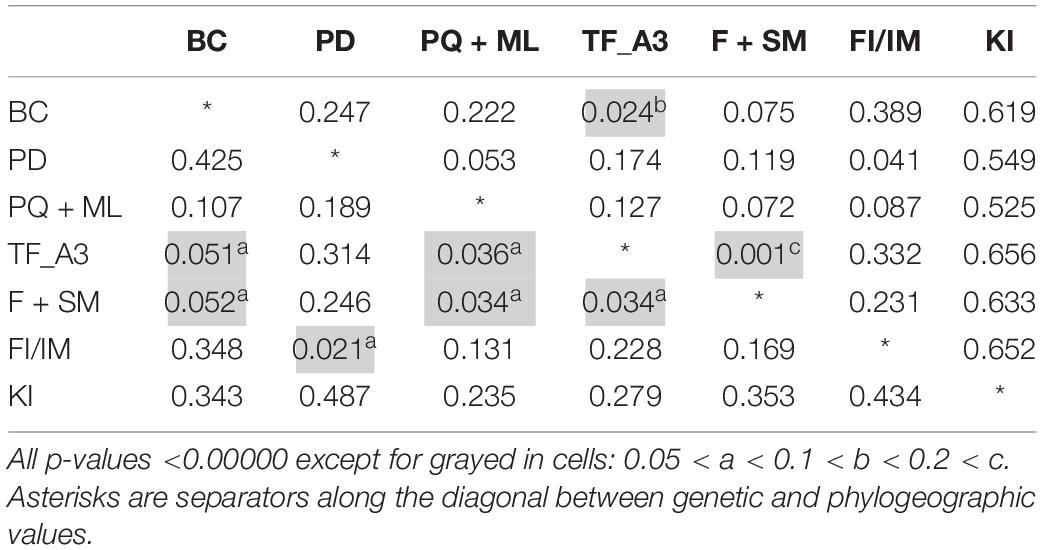
Table 2. Genetic (FST, below diagonal) and phylogeographic (ΦST, above diagonal) structure values between the South American localities of Bahía Camarones (BC), Puerto Deseado (PD), Punta Quilla and Monte León (PQ/ML), Tierra del Fuego (TF_A3), Fitzroy and the Strait of Magellan (F + SM); the Falkland Islands/Islas Malvinas (FI/IM); and Kerguelen Islands (KI).
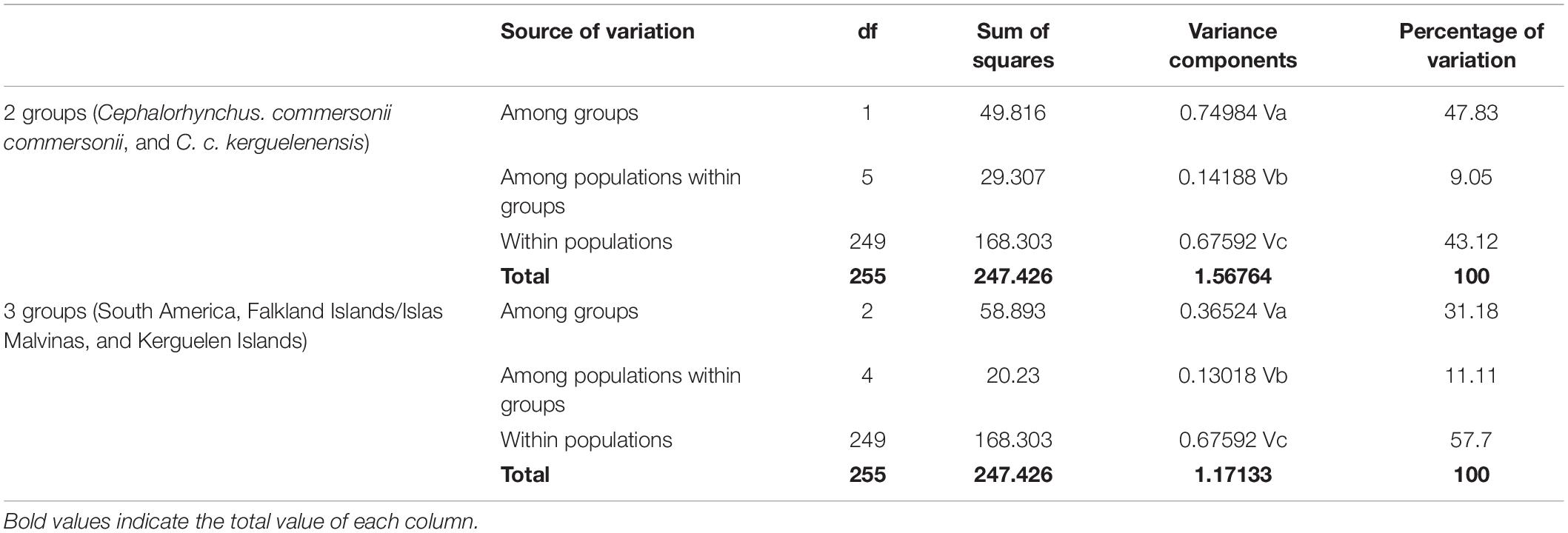
Table 3. Analysis of Molecular Variance performed with Cephalorhynchus commersonii samples grouped according to the subspecies status (two groups) and according to geographic distribution (three groups), after 1,000 permutations.
Historical Biogeography and Migration Rates
Coalescent-based estimations of historical gene flow (Figure 3) indicated that migrations have been occurring continuously between NSA and SSA (NmNSA→SSA = 4.45; NmSSA→NSA = 7.12). Lower levels of bidirectional migrations have been also connecting SSA and the FI/IM (NmSSA→FI/IM = 1.23; NmyFI/IM→SSA = 2.64) as well as the FI/IM and NSA (NmNSA→FI/IM = 0.75; NmFI/IM→NSA = 2.76). The KI are the most isolated population as the number of effective migrants sent or received per generation ranged from 0.56 to 0.04. The KI also showed the lowest value of theta (Θ = 0.001) and the lowest estimated effective population size (Ne = 1,716). Estimates of theta and effective population size for the remaining regions were, in ascending order: FI/IM (Θ = 0.0029, Ne = 4,941), SSA (Θ = 0.0034, Ne = 5,840) and NSA (Θ = 0.0067, Ne = 11,467).
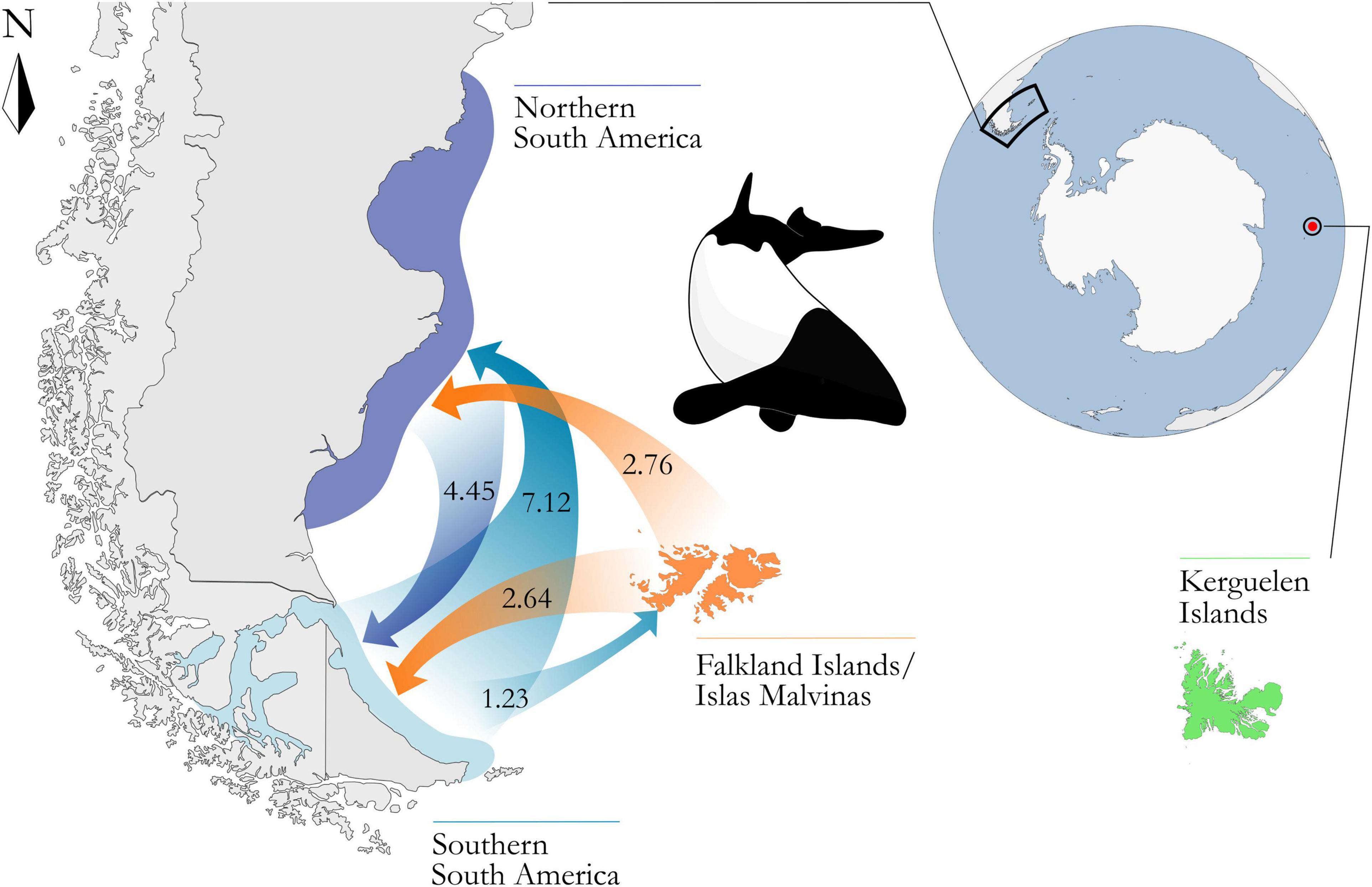
Figure 3. Schematic representation of the number of effective migrants estimated in Lamarc, between the four defined regions across the distribution of the Commerson’s dolphin: northern South America (Bahía Camarones, Puerto Deseado, and Punta Quilla and Monte León), southern South America [Strait of Magellan, Fitzroy and Tierra del Fuego (A3)], Falkland Islands/Islas Malvinas, and Kerguelen Islands. Only values greater than one effective migrant per generation are shown with arrows, representing continuous historical migration between these areas. Distance between South America and Kerguelen Islands is not to scale. Map adapted from FreeVectorMaps.com (https://freevectormaps.com).
Discussion
A geographically extensive sampling scheme, including both new and previously published sequences of the mtDNA control region, allowed us to estimate the genetic diversity and genetic structure across the entire range of the Commerson’s dolphin. From this, we evaluated the previously proposed establishment hypothesis of the KI population (Pichler et al., 2001) as a long-distance dispersal model (Crisp et al., 2011).
The genetic diversity indices we obtained for continental SA are similar to results from previous work conducted in this area. Along the southern Argentina coast, from Puerto Deseado to the Strait of Magellan (n = 57), Cipriano et al. (2011) found 11 haplotypes and a genetic diversity of Hd = 0.671. Further south, along the coast of Tierra del Fuego (n = 196), Pimper et al. (2010) found 20 haplotypes and Hd = 0.807, although many of the latter samples are also used in our study. Our genetic and phylogeographic structure results were also similar to those of Cipriano et al. (2011), showing genetic structure over similarly short distances (Table 2). In the absence of physical barriers along the Argentine coast, behavioral or environmental barriers have been suggested as drivers for this genetic structure (Pimper et al., 2010; Cipriano et al., 2011), as in studies on other species in the genus. In the sister species C. eutropia, strong mitochondrial and nuclear genetic differentiation between north and south populations has been described in the absence of physical barriers along the Chilean coast (Pérez-Alvarez et al., 2015) and coincides with the boundary of two recognized biogeographic provinces of the Southeastern Pacific (Camus, 2001; Escribano et al., 2003). The Chilean dolphin has also shown short movements along the shore (mean 23.1 km) and reduced home ranges (Heinrich, 2006). Similarly, along the South African coast, Heaviside’s dolphins also exhibit genetic population structure across short distances (Gopal et al., 2019). Such small scale genetic structure may be related to reduced home ranges as detected during tracking period of 54 days in C. heavisidii females (Elwen et al., 2006). Finally, Hector’s dolphins show genetic structure over distances as short as 100 km (Hamner et al., 2012), limited along-shore movements (average of 31 km) (Bräger et al., 2002) and display site fidelity and reduced home ranges (Bräger et al., 2002; Rayment et al., 2009).
In addition to exhibiting genetic structure within areas with no obvious barriers, Hector’s dolphins also present marked genetic and phylogeographic structure over areas with potential dispersal barriers, such as the Cook Strait, which separates New Zealand’s North and South Islands (Baker et al., 2002; Hamner et al., 2012). In this latter case, combined genetic and morphologic evidence led to the description of two different subspecies, C. h. hectori, also referred to as the South Island Hector’s dolphin; and the critically endangered C. h. maui exclusively found off the West Coast of the North Island and known as the Mâui dolphin (Baker et al., 2002). SA and the FI/IM are separated by 400–550 km of open ocean at their closest point, much more than the 20 km that separate New Zealand’s North and South Islands. In contrast to C. h. hectori and C. h. maui, Commerson’s dolphins from populations along the SA coast and the FI/IM share several haplotypes (Table 1) and do not show high levels of genetic differentiation (Table 2). The net nucleotide divergence between FI/IM and SA of dA = 0.051% is an order of magnitude below the lower subspecies threshold suggested for cetaceans (dA = 0.4%) (Taylor et al., 2017). Furthermore, the migration analysis suggested moderate migration rates between SA and the FI/IM. The estimated number of effective migrants fits within the range of one to four, which may indicate a migration-drift equilibrium between these two regions. Alternatively, some degree of isolation may be occurring between the FI/IM and SA populations. On one hand, the low diversity in FI/IM may suggest a past founder effect after a possible migration from SA. On the other hand, the low but significant phylogeographic structure may also suggest an incipient divergence between them. In this context, the several haplotypes shared between these regions would be explained by slow linage sorting related to their population sizes. However, based on the recognized, currently accepted, consensus criteria, Commerson’s dolphins of SA and the FI/IM lack enough genetic distinctiveness to be considered different evolutionary units. These results contribute genetic data on a cetacean species to the body of information which supports grouping the FI/IM into the same biogeographic province as SSA (>40°S), the Magellanic biogeographic province (Cousseau et al., 2019). Even if these results are based on a single mitochondrial marker, they suggest that female-mediated gene flow between the two areas did occur at least until recently, which is also supported by the presence of several shared haplotypes. Commerson’s dolphins are capable of occasionally traveling long-distances, which makes continued movements between SA and FI/IM possible. They have been recorded over 180 km out at sea in waters over 1,000 m in depth (Pedraza, 2008), as far out as the Drake Passage (Aguayo, 1975) and off the coast of South Africa (de Bruyn et al., 2006). In this genus, such ventures are not exclusive to Commerson’s dolphins; long-distance movements exceeding 400 km over deep waters have also been described for Hector’s dolphins (Hamner et al., 2014). Nevertheless, these long-range movements are considered unconventional for Commerson’s dolphins, as most records lack proper evidence and are speculative (Brownell and Praderi, 1985; van Waerebeek et al., 2010).
The 8,500 km gap in distribution range of C. commersonii, between SSA, the FI/IM, and the KI (Dawson, 2018), is the widest gap for this genus and presents a potentially significant barrier to dispersal. Previously described osteological, morphological, acoustic and genetic differences between dolphins from these two distant regions (Robineau, 1986; Dziedzic and De Buffrenil, 1989; Robineau et al., 2007) lead to them being considered two subspecies, C. c. commersonii and C. c. kerguelenensis. In relation to previous studies (Robineau et al., 2007; Pimper et al., 2010; Cipriano et al., 2011), here we present a geographically broader sampling scheme, including new areas like Fitzroy, Chile, and the FI/IM, while increasing sampling size in the KI. This allowed us to contribute a fuller picture of the global phylogeography of the species. The phylogeographic structure values between the two subspecies (ΦST = 0.524–0.655) are well above the next highest value (ΦST < 0.39), found between the northernmost locality of Bahía Camarones and FI/IM, i.e., between C. c. commersonii populations (Table 2). The detection of a phylogeographic signal between the samples corresponding to each subspecies by PERMUT further supports this, as well as the results obtained with Lamarc. All migration estimates involving the KI (i.e., samples from the range of C. c. kerguelenensis) were generally much lower than one effective migrant per generation, far below the minimum number to avoid divergence between population. The presence of a single haplotype (H6) shared at very low frequency by KI and SA, as well as the other private haplotypes closely related to H6, may reflect a founder effect followed by the isolation of the colonizers, establishing a newly isolated population. The small population size of the KI population may also have accelerated the lineage sorting process, as observed between Hector’s and Maui’s dolphins in New Zealand (Baker et al., 2002; Hamner et al., 2012). Finally, the net nucleotide divergence of dA = 0.396% is within rounding error of the recommended interval for the sub-species classification of dA = 0.4–2.0% (Taylor et al., 2017) and is thus consistent with the current subspecies ranking. The obtained dA complements the previously described morphological differences between Commerson’s dolphins from SA and the KI (Robineau et al., 2007). Future work could explore the second criterion of diagnosticability (Taylor et al., 2017) using genetic data, such as nuclear markers. In this regard, the KI subspecies may be considered as an incipient, divergent clade of C. commersonii. Pichler et al. (2001) proposed a model of evolution for this genus in which a long-distance dispersal event from South Africa would have permitted the establishment of a new population in New Zealand, which further evolved to C. hectori. In a same manner, a small group of C. hectori would have reached SA, giving rise to both C. eutropia on the Pacific side and C. commersonii on the Atlantic. In this context, C. c. kerguelenensis may represent the most recent and ongoing step in the diversification of Cephalorhynchus, where isolation from their source population is driving an incipient process of future speciation.
Data Availability Statement
All haplotypes obtained from new sequences were deposited in GenBank under the accession numbers OL452036-OL452049 and OL624846-OL624872.
Ethics Statement
The animal study was reviewed and approved by the Undersecretariat for Fisheries and Aquaculture permits RES 665/2009, RES 67/2010, and RES 334/2012, approved by the Bioethical Committee at the Universidad de Chile. Administración de Parques Nacionales (APN) de Argentina. Oregon State University permit IACUP 4671 licensed by the Government of the Falkland Islands/Islas Malvinas. Permit order n°2013-109, issued by le préfet, administrateur supérieur des Terres australes et antarctiques françaises.
Author Contributions
MP-A, RM, CB, PT, CG, AV, PB, MC, EC, CO, and RL: sample collection. MP-A, EP, and SK: contributed reagents, materials, and analysis tools, conceived and designed the analysis, analyzed the data, and wrote the manuscript. SK, MP-A, AV, and DS: laboratory work. MP-A, SK, AV, DS, CD, and RL: acquisition of data. SK: elaboration of figures and tables. All authors read, edited, commented, and approved the final manuscript.
Funding
Financial support for this project was provided by the CONICYT Postdoctoral FONDECYT Program 3140513, CONICYT Program FONDECYT Iniciación 11170182. Instituto de Ecología y Biodiversidad (IEB, Proyecto CONICYT PIA APOYO CCTE AFB170008), Proyecto PIA CONICYT ACT172065, Réserve Naturelle Nationale des Terres Australes Françaises.
Conflict of Interest
The authors declare that the research was conducted in the absence of any commercial or financial relationships that could be construed as a potential conflict of interest.
Publisher’s Note
All claims expressed in this article are solely those of the authors and do not necessarily represent those of their affiliated organizations, or those of the publisher, the editors and the reviewers. Any product that may be evaluated in this article, or claim that may be made by its manufacturer, is not guaranteed or endorsed by the publisher.
Acknowledgments
Samples from Chile were collected under a permit from the Chilean Undersecretariat for Fisheries and Aquaculture RES 665/2009, RES 67/2010, and RES 334/2012 and approved by the Bioethical Committee at the Universidad de Chile. Samples from Argentina were collected by members of Marine Mammal Lab (CESIMAR-CONICET) under permits from Administración de Parques Nacionales, available Technical Report “Crespo et al. (2005) – Estudio de línea de base y plan de monitoreo de mamíferos marinos del futuro Parque Nacional Marino Monte León.” Samples from the Kerguelen Islands were collected under permit order n°2013-109, issued by le préfet, administrateur supérieur des Terres australes et antarctiques française. The collection and analysis of genetic samples from the Falkland Islands/Islas Malvinas was conducted as part of the research program, “Dolphins of the kelp,” funded by a grant from the National Geographic Society/Waitt Foundation Program and a Darwin Plus award (DPLUS0042) from the United Kingdom, Department for Environment, Food and Rural Affairs. For assistance with sampling in the Falkland Islands/Islas Malvinas we thank Steve Cartwright and Maria Isabel Garcia Rojas. Institutional approval for the collection of biopsy samples was granted by the Oregon State University IACUP 4671 and licensed by the Government of the Falkland Islands/Islas Malvinas.
Supplementary Material
The Supplementary Material for this article can be found online at: https://www.frontiersin.org/articles/10.3389/fmars.2021.782512/full#supplementary-material
Abbreviations
SA, South America; FI/IM, Falkland Islands/Islas Malvinas; KI, Kerguelen Islands; NSA, northern South America; SSA, southern South America.
Footnotes
References
Aguayo, A. (1975). Progress report on small cetacean research in chile. J. Fish. Res. Bd. Can. 32, 1123–1143. doi: 10.1139/f75-134
Aljanabi, S. M., and Martinez, I. (1997). Universal and rapid salt-extraction of high quality genomic DNA for PCR-based techniques. Nucleic Acids Res. 25, 4692–4693. doi: 10.1093/nar/25.22.4692
Alter, S. E., and Palumbi, S. R. (2009). Comparing evolutionary patterns and variability in the mitochondrial control region and cytochrome b in three species of baleen whales. J. Mol. Evol. 68, 97–111. doi: 10.1007/s00239-008-9193-2
Baker, A. N., Smith, A. N. H., and Pichler, F. B. (2002). Geographical variation in Hector’s dolphin: recognition of new subspecies of Cephalorhynchus hectori. J. R. Soc. N. Z. 32, 713–727. doi: 10.1080/03014223.2002.9517717
Balech, E., and Ehrlich, M. D. (2008). Esquema biogeográfico del mar argentino. Rev. Investig. Desarro. Pesquero 19, 45–75.
Bandelt, H. J., Forster, P., and Röhl, A. (1999). Median-joining networks for inferring intraspecific phylogenies. Mol. Biol. Evol. 16, 37–48. doi: 10.1093/oxfordjournals.molbev.a026036
Banguera-Hinestroza, E., Bjørge, A., Reid, R. J., Jepson, P., and Hoelzel, A. R. (2010). The influence of glacial epochs and habitat dependence on the diversity and phylogeography of a coastal dolphin species: Lagenorhynchus albirostris. Conserv Genet. 11, 1823–1836. doi: 10.1007/s10592-010-0075-y
Bennett, K. D. (1990). Milankovitch cycles and their effects on species in ecological and evolutionary time. Paleobiology 16, 11–21. doi: 10.1017/S0094837300009684
Bräger, S., Dawson, S. M., Slooten, E., Smith, S., Stone, G. S., and Yoshinaga, A. (2002). Site fidelity and along-shore range in Hector’s dolphin, an endangered marine dolphin from New Zealand. Biol. Conserv. 108, 281–287. doi: 10.1016/S0006-3207(02)00124-6
Brownell, R. L., and Praderi, R. (1985). Distribution of commerson’s Cephalorhynchus commersonii and the rediscovery of the type of Lagenorhynchus floweri. Sci. Rep. Whales Res. Inst. 36, 153–164.
Camus, P. A. (2001). Biogeografía marina de chile continental. Rev. Chil. Hist. Nat. 74, 587–617. doi: 10.4067/S0716-078X2001000300008
Cipriano, F., Hevia, M., and Iñíguez, M. (2011). Genetic divergence over small geographic scales and conservation implications for commerson’s dolphins (Cephalorhynchus commersonii) in southern Argentina. Mar. Mamm. Sci. 27, 701–718. doi: 10.1111/j.1748-7692.2010.00434.x
Collin, R., and de Maintenon, M. (2002). Integrative approaches to biogeography: patterns and processes on land and in the sea. Integr. Comp. Biol. 42, 911–912. doi: 10.1093/icb/42.5.911
Cousseau, M. B., Pequeño, G., Mabragaña, E., Lucifora, L. O., Martínez, P., and Giussi, A. (2019). The magellanic province and its fish fauna (South America): several provinces or one? J. Biogeogr. 47, 220–234. doi: 10.1111/jbi.13735
Crisp, M. D., Trewick, S. A., and Cook, L. G. (2011). Hypothesis testing in biogeography. Trends Ecol. Evol. 26, 66–72. doi: 10.1016/j.tree.2010.11.005
Dalebout, M. L., Robertson, K. M., Frantzis, A., Engelhaupt, D., Mignucci-Giannoni, A. A., Rosario-Delestre, R. J., et al. (2005). Worldwide structure of mtDNA diversity among Cuvier’s beaked whales (Ziphius cavirostris): implications for threatened populations. Mol. Ecol. 14, 3353–3371. doi: 10.1111/j.1365-294X.2005.02676.x
Dawson, S. M. (2018). “Cephalorhynchus dolphins,” in Encyclopedia of Marine Mammals, eds B. G. Würsig, J. G. M. Thewissen, and K. M. Kovacs (Cambridge, MA: Academic Press), 166–172. doi: 10.1016/b978-0-12-804327-1.00086-8
de Bruyn, M., Hall, B. L., Chauke, L. F., Baroni, C., Koch, P. L., and Hoelzel, A. R. (2009). Rapid response of a marine mammal species to holocene climate and habitat change. PLoS Genet 5:e1000554. doi: 10.1371/journal.pgen.1000554
de Bruyn, P. J. N., Hofmeyr, G. J. G., and de Villiers, M. S. (2006). First record of a vagrant commerson’s dolphin, Cephalorhynchus commersonii, at the southern African continental shelf. Afr. Zool. 41, 131–133. doi: 10.3377/1562-7020(2006)41[131:froavc]2.0.co;2
Dziedzic, A., and De Buffrenil, V. (1989). Acoustic signals of the commerson’s dolphin, Cephalorhynchus commersonii, in the Kerguelen Islands. J. Mammal. 70, 449–452. doi: 10.2307/1381541
Elwen, S., Meÿer, M. A., Best, P. B., Kotze, P. G. H., Thornton, M., and Swanson, S. (2006). Range andmovements of female Heaviside’s dolphins (Cephalorhynchus heavisidii), as determined by satellite-linked telemetry. J. Mammal. 87, 866–877. doi: 10.1644/05-MAMM-A-307R2.1
Escribano, R., Fernández, M., and Aranís, A. (2003). Physical-chemical processes and patterns of diversity of the chilean eastern boundary pelagic and benthic marine ecosystems: an overview. Gayana 67, 190–205. doi: 10.4067/S0717-65382003000200008
Excoffier, L., and Lischer, H. E. L. (2010). Arlequin suite ver 3.5: a new series of programs to perform population genetics analyses under Linux and Windows. Mol. Ecol. Resour. 10, 564–567. doi: 10.1111/j.1755-0998.2010.02847.x
Excoffier, L., Smouse, P. E., and Quattro, J. M. (1992). Analysis of molecular variance inferred from metric distances among DNA haplotypes: application to human mitochondrial DNA restriction data. Genetics 131, 479–491. doi: 10.1093/genetics/131.2.479
Filatov, D. A. (2009). Processing and population genetic analysis of multigenic datasets with ProSeq3 software. Bioinformatics 25, 3189–3190. doi: 10.1093/bioinformatics/btp572
Goodall, R. N. P. (1994). “Chilean dolphin Cephalorhynchus eutropia (Gray 1846),” in Handbook of Marine Mammals, eds S. H. Ridgway and R. Harrison (Cambridge, MA: Academic Press), 269–287.
Goodall, R. N. P., Galeazzi, A. R., Leatherwood, S., Miller, K. W., Cameron, I. S., Kastelein, R., et al. (1988). “Studies of commerson’s dolphins, Cephalorhynchus commersonii, off Tierra del Fuego, 1976-1984, with a review of information on the species in the South Atlantic,” in Biology of the genus Cephalorhynchus, eds R. L. Brownell Jr. and G. P. Donovan (Cambridge, UK: International Whaling Commission), 3–70.
Gopal, K., Karczmarski, L., and Tolley, K. A. (2019). Patterns of geographic variation between mitochondrial and nuclear markers in Heaviside’s (Benguela) dolphins (Cephalorhynchus heavisidii). Integr. Zool. 14, 506–526. doi: 10.1111/1749-4877.12380
Hamner, R. M., Constantine, R., Oremus, M., Stanley, M., Brown, P., and Scott Baker, C. (2014). Long-range movement by Hector’s dolphins provides potential genetic enhancement for critically endangered Maui’s dolphin. Mar. Mamm. Sci. 30, 139–153. doi: 10.1111/mms.12026
Hamner, R. M., Pichler, F. B., Heimeier, D., Constantine, R., and Baker, C. S. (2012). Genetic differentiation and limited gene flow among fragmented populations of New Zealand endemic Hector’s and Maui’s dolphins. Conserv. Genet. 13, 987–1002. doi: 10.1007/s10592-012-0347-9
Harlin, A. D. Würsig, B., Baker, C. S., and Markowitz, T. M. (1999). Skin swabbing for genetic analysis: application to dusky dolphins (Lagenorhynchus obscurus). Mar. Mamm. Sci. 15, 409–425. doi: 10.1111/j.1748-7692.1999.tb00810.x
Heinrich, S. (2006). Ecology of Chilean Dolphins and Peale’s Dolphins at Isla Chiloe, Southern Chile. Ph.D. Thesis. St Andrews: University of St Andrews.
Hewitt, G. (2000). The genetic legacy of the quaternary ice ages. Nature 405, 907–913. doi: 10.1038/35016000
Kuhner, M. K. (2006). LAMARC 2.0: maximum likelihood and Bayesian estimation of population parameters. Bioinformatics 22, 768–770. doi: 10.1093/bioinformatics/btk051
Kuhner, M. K., and Smith, L. P. (2007). Comparing likelihood and bayesian coalescent estimation of population parameters. Genetics 175, 155–165. doi: 10.1534/genetics.106.056457
Leigh, J. W., and Bryant, D. (2015). popart: full-feature software for haplotype network construction. Methods Ecol. Evol. 6, 1110–1116. doi: 10.1111/2041-210X.12410
Librado, P., and Rozas, J. (2009). DnaSP v5: a software for comprehensive analysis of DNA polymorphism data. Bioinformatics 25, 1451–1452. doi: 10.1093/bioinformatics/btp187
Mackensen, A. (2004). Changing Southern Ocean palaeocirculation and effects on global climate. Antartic Sci. 16, 369–386. doi: 10.1017/S0954102004002202
Morgenthaler, A., Fernández, J., Moraga, R., and Olavarría, C. (2014). Chilean dolphins on the Argentine Atlantic coast. Mar. Mam. Sci. 30, 782–787. doi: 10.1111/mms.12052
O’Corry-Crowe, G. M., Suydam, R. S., Rosenberg, A., Frost, K. J., and Dizon, A. E. (1997). Phylogeography, population structure and dispersal patterns of the beluga whale Delphinapterus leucas in the western Nearctic revealed by mitochondrial DNA. Mol. Ecol. 6, 955–970. doi: 10.1046/j.1365-294X.1997.00267.x
Oliveira, L. R., Gehara, M. C. M., Fraga, L. D., Lopes, F., Túnez, J. I., Cassini, M. H., et al. (2017). Ancient female philopatry, asymmetric male gene flow, and synchronous population expansion support the influence of climatic oscillations on the evolution of South American sea lion (Otaria flavescens). PLoS One 12:e0179442. doi: 10.1371/journal.pone.0179442
Pedraza, S. N. (2008). Ecología de la Tonina Overa (Cephalorhynchus commersonii) en el Litoral Patagónico. Ph.D. Thesis. Buenos Aires: Universidad de Buenos Aires.
Pérez-Alvarez, M. J., Olavarría, C., Moraga, R., Baker, C. S., Hamner, R. M., and Poulin, E. (2016). Historical dimensions of population structure in a continuously distributed marine species: the case of the endemic Chilean dolphin. Sci. Rep. 6:35507. doi: 10.1038/srep35507
Pérez-Alvarez, M. J., Olavarría, C., Moraga, R., Baker, C. S., Hamner, R. M., and Poulin, E. (2015). Microsatellite markers reveal strong genetic structure in the endemic chilean dolphin. PLoS One 10:e0123956. doi: 10.1371/journal.pone.0123956
Pichler, F. B., Robineau, D., Goodall, R. N. P., Meyer, M. A., Olivarria, C., and Baker, C. S. (2001). Origin and radiation of Southern Hemisphere coastal dolphins (genus Cephalorhynchus). Mol. Ecol. 10, 2215–2223. doi: 10.1046/j.0962-1083.2001.01360.x
Pike, N. (2011). Using false discovery rates for multiple comparisons in ecology and evolution: false discovery rates for multiple comparisons. Methods Ecol. Evol. 2, 278–282. doi: 10.1111/j.2041-210X.2010.00061.x
Pimper, L. E., Baker, C. S., Goodall, R. N. P., Olavarría, C., and Remis, M. I. (2010). Mitochondrial DNA variation and population structure of commerson’s dolphins (Cephalorhynchus commersonii) in their southernmost distribution. Conserv. Genet. 11, 2157–2168. doi: 10.1007/s10592-010-0102-z
Pons, O., and Petit, R. J. (1996). Measuring and testing genetic differentiation with ordered versus unordered alleles. Genetics 144, 1237–1245. doi: 10.1093/genetics/144.3.1237
Rayment, W., Dawson, S., Slooten, E., Bräger, S., Fresne, S. D., and Webster, T. (2009). Kernel density estimates of alongshore home range of Hector’s dolphins at Banks Peninsula, New Zealand. Mar. Mamm. Sci. 25, 537–556. doi: 10.1111/j.1748-7692.2008.00271.x
Robineau, D. (1984). Morphologie externe et pigmentation du dauphin de commerson, Cephalorhynchus commersonii (Lacépède, 1804), en particulier celui des îles Kerguelen. Can. J. Zool. 62, 2465–2475. doi: 10.1139/z84-363
Robineau, D. (1986). Valeur adaptative des caractères morphologiques distinctifs (taille et pigmentation) d’une population isolée d’un dauphin subantarctique, Cephalorhynchus commersonii (Lacépède, 1804). Mammalia 50, 172–180. doi: 10.1515/mamm.1986.50.3.357
Robineau, D., Goodall, R. N. P., Pichler, F., and Baker, C. S. (2007). Description of a new subspecies of commerson’s dolphin, Cephalorhynchus commersonii (Lacépède, 1804), inhabiting the coastal waters of the Kerguelen Islands. Mammalia 71, 172–180. doi: 10.1515/MAMM.2007.034
Taylor, B. L., Archer, F. I., Martien, K. K., Rosel, P. E., Hancock-Hanser, B. L., Lang, A. R., et al. (2017). Guidelines and quantitative standards to improve consistency in cetacean subspecies and species delimitation relying on molecular genetic data. Mar. Mam. Sci. 33, 132–155. doi: 10.1111/mms.12411
Taylor, M. S., and Hellberg, M. E. (2006). Comparative phylogeography in a genus of coral reef fishes: biogeographic and genetic concordance in the Caribbean. Mol. Ecol. 15, 695–707. doi: 10.1111/j.1365-294X.2006.02820.x
Thatje, S., Hillenbrand, C.-D., and Larter, R. (2005). On the origin of Antarctic marine benthic community structure. Trends Ecol. Evol. 20, 534–540. doi: 10.1016/j.tree.2005.07.010
Túnez, J. I., Cappozzo, H. L., Nardelli, M., and Cassini, M. H. (2010). Population genetic structure and historical population dynamics of the South American sea lion, Otaria flavescens, in north-central Patagonia. Genetica 138, 831–841. doi: 10.1007/s10709-010-9466-8
Túnez, J., Cappozzo, H., Pavés, H., Albareda, D., and Cassini, M. (2013). The role of pleistocene glaciations in shaping the genetic structure of South American fur seals (Arctocephalus australis). N. Z. J. Mar. Freshw. Res. 47, 139–152. doi: 10.1080/00288330.2012.753463
Keywords: Cephalorhynchus commersonii, colonization, divergence, last glacial maximum, mitochondrial DNA, phylogeography
Citation: Kraft S, Pérez-Alvarez M, Olavarría C, Moraga R, Baker CS, Steel D, Tixier P, Guinet C, Viricel A, Brickle P, Costa M, Crespo E, Durante C, Loizaga R and Poulin E (2021) From Settlers to Subspecies: Genetic Differentiation in Commerson’s Dolphins Between South America and the Kerguelen Islands. Front. Mar. Sci. 8:782512. doi: 10.3389/fmars.2021.782512
Received: 24 September 2021; Accepted: 15 November 2021;
Published: 13 December 2021.
Edited by:
Marc Girondot, Université Paris-Sud, FranceReviewed by:
Aimee R. Lang, Southwest Fisheries Science Center (NOAA), United StatesRobin Cristofari, University of Turku, Finland
Copyright © 2021 Kraft, Pérez-Alvarez, Olavarría, Moraga, Baker, Steel, Tixier, Guinet, Viricel, Brickle, Costa, Crespo, Durante, Loizaga and Poulin. This is an open-access article distributed under the terms of the Creative Commons Attribution License (CC BY). The use, distribution or reproduction in other forums is permitted, provided the original author(s) and the copyright owner(s) are credited and that the original publication in this journal is cited, in accordance with accepted academic practice. No use, distribution or reproduction is permitted which does not comply with these terms.
*Correspondence: MJosé Pérez-Alvarez, bWFyaWEucGVyZXpAdW1heW9yLmNs
†Present address: Sebastián Kraft, Centre of Marine Sciences (CCMAR), University of the Algarve, Campus de Gambelas, Faro, Portugal