- 1CPC Inc., Galveston, TX, United States
- 2NOAA Office of National Marine Sanctuaries, Flower Garden Banks National Marine Sanctuary, Galveston, TX, United States
- 3Louisiana Universities Marine Consortium (LUMCON), Chauvin, LA, United States
The continental shelf of the northwestern Gulf of Mexico harbors extensive reefs and banks that support diverse coral reefs and mesophotic communities. Mesophotic communities range in depth from 40 to 200 m and, in this region, foster some of the densest coral forests [aggregations of mesophotic octocoral, antipatharian, and branching stony coral communities] reported in published literature (10.23 ± 9.31 col/m2). The geologic features underlying the exposed substrates that harbor mesophotic communities are targeted for extensive hydrocarbon exploration and extraction, as they often contain oil and/or natural gas. The Bureau of Ocean Energy Management regulates offshore energy development in the United States and is tasked with protecting sensitive biological communities from impacts related to oil and gas activities. This study analyzed alpha and beta diversity of mesophotic coral forests on fourteen topographic banks in the northwestern Gulf of Mexico. The objective of the study was to examine differences in structure and community in relation to lease stipulations established by the Bureau of Ocean and Energy Management. It was determined that dense and diverse mesophotic coral forests and carbonate producers exist in present regulatory zones that prohibit oil and gas activities; however, the coral communities exist in higher densities, diversity, and richness in low relief substrates outside of these regulatory zones. Our findings suggest low relief hard substrates serve as important habitat for mesophotic coral forests; thus, we suggest the expansion of current stipulations should be considered to provide better protection to vulnerable coral communities on low relief features. Furthermore, additional studies to refine the relationship between low relief structures and biodiversity are needed to develop more meaningful habitat definitions to support resource management and improve resource protection in the future.
Introduction
In the Gulf of Mexico (GOM), mesophotic communities occur on many hard substrates between 40 and 200 m depth (Semmler et al., 2016). In these assemblages, corals and sponges contribute three-dimensional structural complexity and promote ecosystem development (Roberts et al., 2009; Hogg et al., 2010; Hourigan et al., 2017; Rossi et al., 2017). Similar to structurally complex terrestrial ecosystems, these mesophotic coral forests provide refuge, resources and substrate for a multitude of other organisms (Rossi et al., 2017), and create hotspots of biological diversity in deep water environments (Hourigan et al., 2017; Chimienti et al., 2019, 2020).
The continental shelf of the northwestern GOM contains scattered reef and bank features that encompass a wide variety of habitats from 15 to 200 m depth, including coral reefs and mesophotic communities. Above 50 m, light dependent scleractinian corals dominate the cnidarian community, including several threatened species of Orbicella. Below 70 m is predominantly composed of antipatharians [protected against international trade by the Convention on International Trade in Endangered Species (CITES)], octocorals, and few branching corals (Hickerson and Schmahl, 2005; Schmahl et al., 2008). These communities are considered vulnerable due to their susceptibility to bottom disturbing activities, including fishing, dredging, and hydrocarbon exploration, production, and decommissioning (Gass and Roberts, 2006; Lumsden et al., 2007; Reed et al., 2007; Heifetz et al., 2009; Yoklavich et al., 2018; Chimienti et al., 2019). Their life history traits, including slow growth rates, late maturity, low recruitment, and long life spans (Fossa et al., 2002; Hall-Spencer et al., 2002; Mortensen and Buhl-Mortensen, 2004; Bo, 2008; Roark et al., 2009; Clark et al., 2016), make them unlikely to recover quickly from detrimental impacts (Roark et al., 2009; Sherwood and Edinger, 2009; Clark et al., 2010; Prouty et al., 2011). Activities that cause physical damage, including bottom fishing (with trawling regarded as the most damaging), anchoring, and underwater construction, can reduce the three-dimensional structure created by the corals into rubble fields (Hall-Spencer et al., 2002; Clark and Koslow, 2007; Clark et al., 2010; Cordes et al., 2016), further impacting other communities, such as fish, that utilize the habitat created by the coral forest. Sedimentation from dredging, construction, and drilling can cause smothering or burial of coral polyps and shade light dependent corals, and depending on the duration of the event and the sensitivity of the corals impacted, can result in tissue necrosis and promote disease and infection (Brooke et al., 2009; Trannum et al., 2011; Erftemeijer et al., 2012; Cordes et al., 2016; Jones et al., 2019). Accidental discharges, spills, and dredging can introduce contaminants and pollutants into the water column that, depending on the specific chemicals and duration of the event, can cause patchy tissue death and non-acute general declines in coral condition (Esslemont et al., 2004; Erftemeijer et al., 2012; White et al., 2012; Hsing et al., 2013; Girard and Fisher, 2018).
The shallow hermatypic coral reefs and coral communities of the Flower Garden and Stetson Banks have been extensively studied (Nuttall et al., 2020; Johnston et al., 2021), were recognized as features of national significance, and were protected by the National Oceanic and Atmospheric Administration (NOAA) as Flower Garden Banks National Marine Sanctuary (FGBNMS) in 1992 and 1996, respectively. Exploration and characterization of the deeper communities (>40 m) in this region has increased over the past two decades, with modeling studies suggesting that the northern GOM has 20 times more potential habitat from 40 to 150 m than the United States Caribbean and Hawaii combined. This represents the most extensive potential habitat in this depth range in United States waters (Locker and Hine, 2020). In 2021, FGBNMS expanded, adding an additional 14 primarily mesophotic reefs and banks (15 CFR Part 922 – Subpart L, 2021), selecting boundaries that aligned with areas historically identified by regulators charged with protection of sensitive assemblages from activities on oil and gas leases (NTL No. 2009-G39, 2009).
Approximately 17% of total United States crude oil and 5% of domestic gas are produced offshore in the GOM (EIA, 2021). The surface expressions of underlying salt domes targeted for hydrocarbon extraction serve as the primary substratum for mesophotic coral communities in the northwestern GOM (Sammarco et al., 2016a). This geologic tie to vulnerable organisms raises the concern for their preservation. The protection of sensitive biological communities within the United States Exclusive Economic Zone (EEZ), where these features occur, are under the jurisdiction of the Department of the Interior, Bureau of Ocean Energy Management (BOEM). Since 1973, BOEM has taken a precautionary approach in the northwestern GOM to protect biological communities in water depths less than 300 m through stipulations that require either avoidance or other restrictions on allowable activities. The most recent stipulations are detailed in a Notice to Lessees (NTL No. 2009-G39, 2009) and are based on biological characterizations from the 1980s (Bright and Rezak, 1978; Rezak and Bright, 1981; Rezak et al., 1985). The crest of topographic features that provide hard-bottom habitats and support high biomass and diversity of animal and plant communities are protected through No Activity Zones (NAZs) designated using depth contours (either at the 55 or 85 m isobath). The NAZ prohibits bottom-disturbing activities and has associated buffer zones that restrict the release of drilling wastes. The NTL classifies medium to high relief features (≥2.44 m) outside of NAZs, which provide habitat for the growth of benthic invertebrates and attract large numbers of fish, as Potentially Sensitive Biological Features (PSBF). Bottom-disturbing activities are also prohibited in these areas. However, these features are not delimited and instead are identified on a case by case basis through environmental assessment. The remaining habitat that falls outside of the NAZ and PSBF definitions in the NTL are reviewed by BOEM during environmental assessments but are not subject to the protective stipulations. Studies indicate that the remaining and undiscovered hydrocarbon reserves, of which 80 – 85% reside below 120 m, will continue producing for many decades (Kaiser and Narra, 2019; Locker and Hine, 2020) and, therefore, evaluation and updates of these mitigation strategies are needed to ensure best management practices into the future.
No Activity Zones (NAZs) and PSBFs were developed based on the best available information in the 1980’s and have successfully provided significant protection to sensitive communities from oil and gas activities. However, since their designation, extensive mapping, exploration, and characterization has occurred along the continental shelf in the northwestern GOM. In recognition of this, BOEM initiated collaboration with NOAA to determine whether current NAZs and PSBFs are sufficient to protect the full range of sensitive biological communities or if updated classifications are needed to achieve management goals. This study combines previously examined BOEM datasets (Sammarco et al., 2016a,b,c) with additional NOAA data to investigate the biodiversity of vulnerable mesophotic corals in the northwestern GOM. These robust datasets were used to compare coral biodiversity and community structure in current NAZs and PSBFs with lower relief areas, examine how location and multibeam derived variables effect coral biodiversity, and provide recommendations on ways to improve resource protection and management.
Materials and Methods
Study Location
This study focused on the northwestern GOM, generally recognized as the area from the Texas-Mexico border to the state line between Louisiana and Mississippi, in the United States (Figure 1A). The analysis targeted 14 banks subject to the existing “Topographic Features” lease stipulations, including: Sonnier, 29 Fathom, Alderdice, Bouma, Rezak, McGrail, Parker, Sidner, Rankin, 28 Fathom, Bright, Horseshoe, Elvers, and Geyer Banks (Figure 1B). With the exception of 29 Fathom Bank, these areas are also of particular interest given their recent inclusion into FGBNMS (15 CFR Part 922 – Subpart L, 2021).
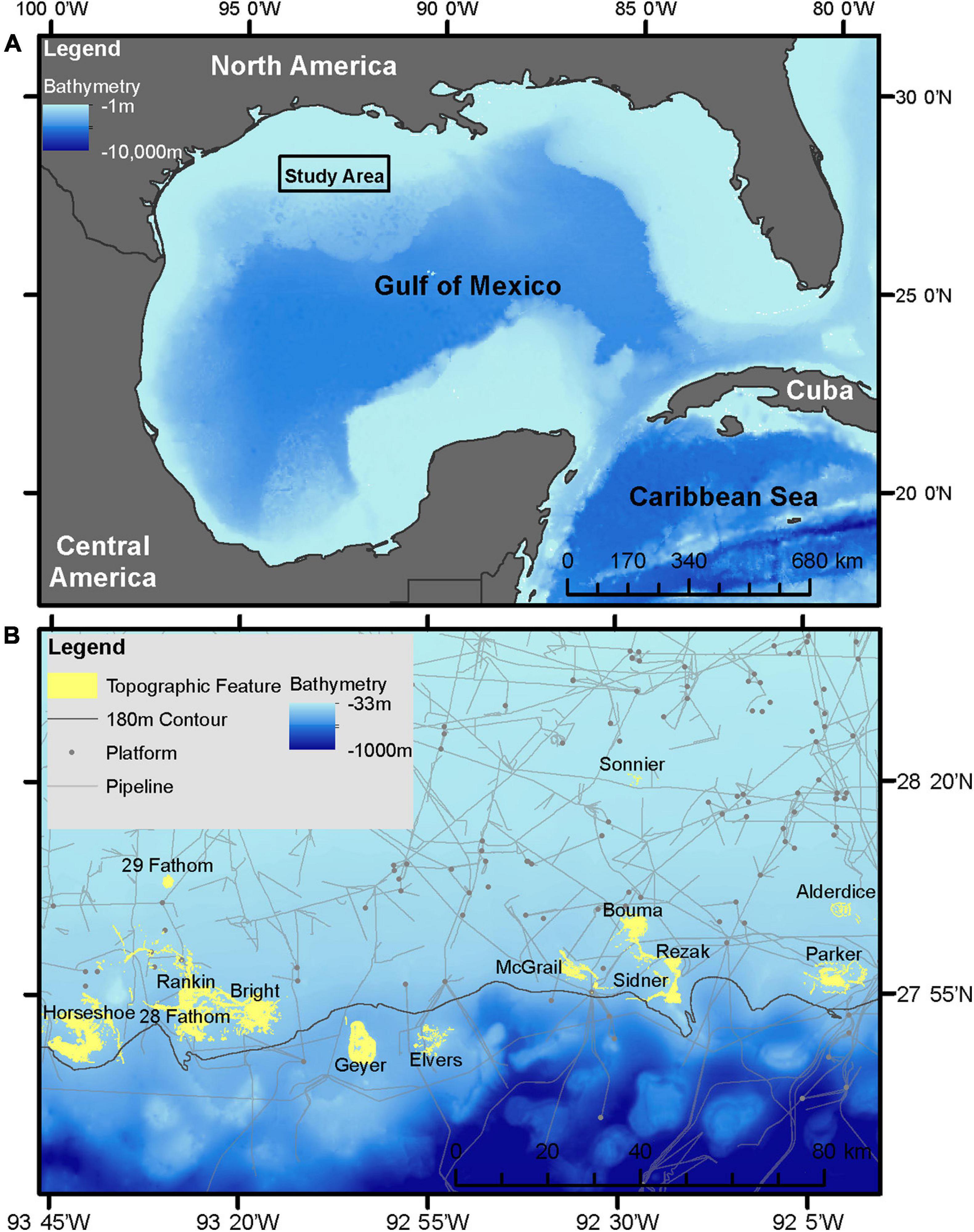
Figure 1. (A) Study area in the northwestern GOM. (B) Inset of study area with study site locations, 180 m isobath, and current oil and gas pipeline and platform infrastructure.
Remotely Operated Vehicle Surveys
From 2011 to 2013, remotely operated vehicle (ROV) surveys were conducted on selected hard substrates outside of BOEM designated NAZs associated with the 14 targeted banks. Habitats examined included PSBFs and other low relief hard substrates. The Deep Ocean Engineering Phantom S-2 ROV, owned and operated by University of North Carolina at Wilmington Undersea Vehicle Program (UNCW-UVP), was equipped with a downward facing Insite-Tritech Scorpio Plus digital still camera with strobes, dual scale lasers set at 10 cm, an ORE Offshore 4410C Trackpoint II underwater acoustic tracking system with an ORE Offshore 4377A transponder with depth telemetry and altimeter, and a Northstar 951XD differential GPS.
From 2016 to 2018, additional ROV surveys were conducted on sites of interest to FGBNMS for expansion. This included habitats within NAZs, PSBFs, other low relief hard substrates, and soft substrates. The SubAtlantic MOHAWK 18 ROV (owned by National Marine Sanctuary Foundation, and operated by UNCW-UVP) was equipped with a forward facing Kongsberg Maritime OE14-408 10 mp digital still camera, Insite Pacific Mini Zeus II HD, OE11-442 strobe, two Sidus SS501 50 mW green spot lasers set at 10 cm in the still camera frame for scale, and an ORE transponder with ORE TrackPoint II.
Transect Delineation/Determination
All transects were constrained within the “core biological zone” (CBZ) of each feature, developed by the Office of National Marine Sanctuaries in 2007 (ONMS, 2016; Figure 1). This polygon represents an aggregation of important biological and geological features associated with each bank, identified through visual interpretation of seafloor topography and previous scuba and submersible investigations demonstrating the presence of high-diversity coral reefs, coralline algal reefs and deep coral. Within the CBZ, habitats were further characterized into four groups; NAZ, PSBF, low relief rock substrate (LRRS), and flat substrate (FS), based on 4 m2 resolution multibeam bathymetric data. NAZs were identified for each bank by drawing a polygon along the isobath designated in NTL No. 2009-G39 (either 55 or 85 m). The remaining habitats were identified based on local relief. Local relief was calculated from bathymetric data using a focal window to calculate the depth range within a 2 × 2 grid cell window using ESRIs ArcGIS. The resulting grid was converted to polygons where PSBFs were >2.44 m relief, LRRSs were between 0.33 and 2.44 m relief, and FSs were <0.33 m.
The objective of the 2011 to 2013 transects was to characterize biological communities associated with PSBFs inside the CBZ and outside NAZs (Sammarco, 2016). Ten dive sites were randomly generated at each bank within PSBFs and LRRSs, proportionally distributed by area in each habitat and with a minimum distance of 100 m between sites. Due to the objectives of the study, NAZs and soft substrates were excluded from site selections. Dive sites represented the geographic point at which the ROV made contact with the bottom to conduct transects (Figure 2). The ROV maintained a distance of 1 – 1.5 m above the bottom and traveled at 0.5 knots while completing five, non-overlapping, 10-min transects at each dive site. Transects were conducted along hard substrate and extensive soft substrate areas were avoided to align with the study objectives. On each transect, downward facing digital still images were taken every 30 s.
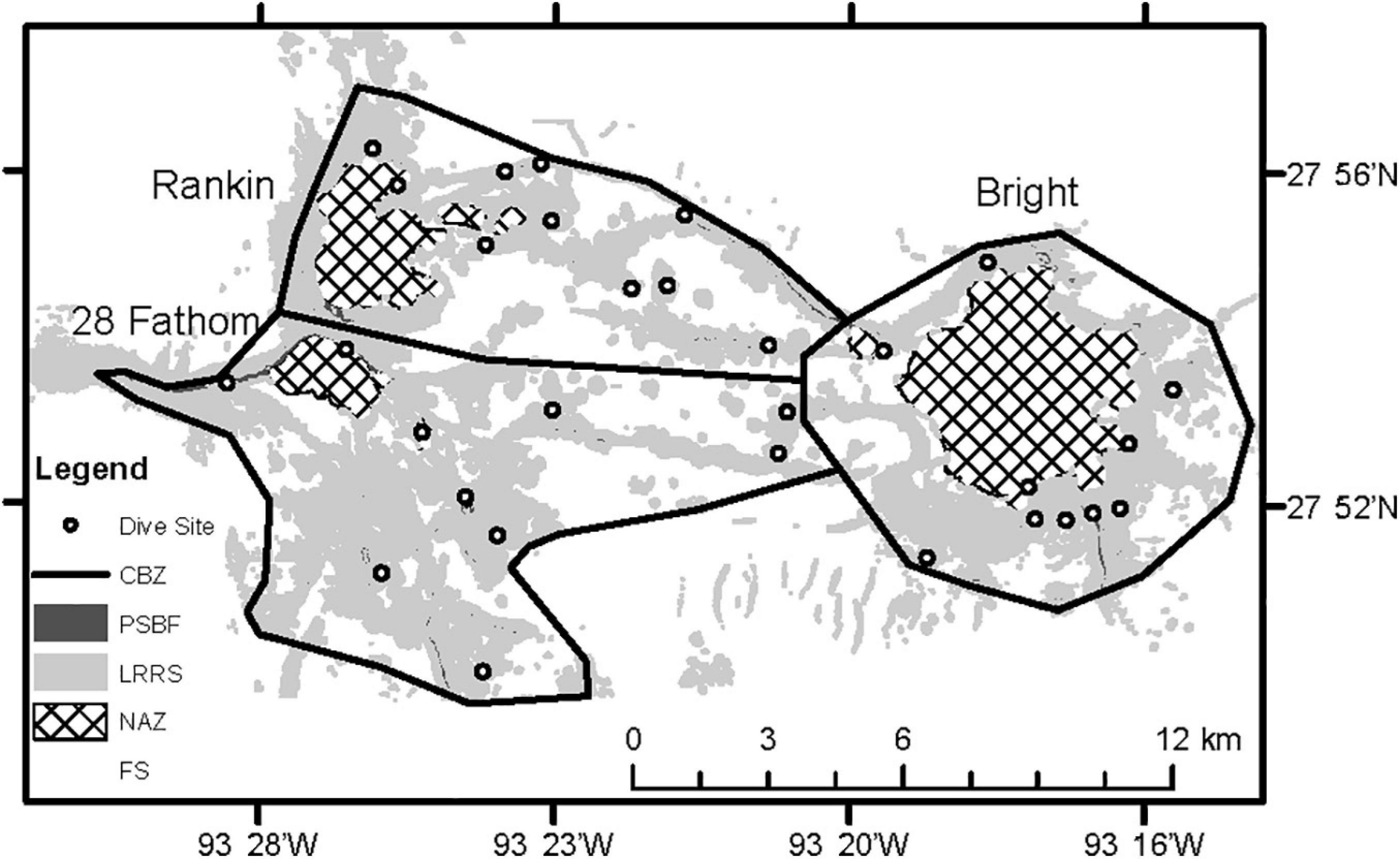
Figure 2. Inset of three topographic features showing habitats identified by multibeam bathymetry: No Activity Zone (NAZ), Potentially Sensitive Biological Features (PSBF), Low Relief Rock Substrate (LRRS), Flat Substrate (FS), and Core Biological Zone (CBZ). Each circle denotes a dive site.
The objective of the transects conducted in 2016 – 2018 was to characterize previously unexplored areas, or interesting features, within the CBZ. At each dive site, one transect was conducted for each habitat encountered, based on the six habitats [coral reefs, coral communities, algal nodules (rhodolith substrate), coralline algae reefs, deep coral reefs, and soft bottom] described by Schmahl et al. (2008) (Table 1). Transects were 5 min in length, corresponding to a survey distance of approximately 100 m. The ROV traveled at 0.5 knots at an altitude of approximately 1 m off the bottom. Video and still cameras, maintaining a wide and fixed frame, were angled 45° toward the substrate, and high definition (HD) video was collected for the duration of the transect along with photographs every 30 s.
Image Analysis
From 2011 to 2013, images that consisted of >50% soft bottom or shadow, or were out of focus or silted, were removed from analysis. Nine to 11 images were randomly selected from each transect for analysis, where counts of octocoral, antipatharian, stony, and soft coral species were recorded to family level. The area of each image was calculated using scale lasers and summed over the transect to obtain transect area.
In 2016, HD video was analyzed, where the entire 5-min survey was processed for colony counts. Corals were identified to family level and counted. Mean area was estimated by reviewing still frames and calculating mean field of view using scale lasers from all transects (0.86 m). This mean field of view was then multiplied over the approximately 100 m transect, resulting in a transect area of 86.2 m2 for each transect in 2016.
In 2017 and 2018, still images were analyzed. Images that consisted of >50% water column (i.e., off bottom images), or were out of focus or silted, were removed from the analysis. Fifteen to 20 images were randomly selected for colony count analysis. Corals were identified to the family level and counted. Area measurements were estimated each year by averaging the field of view of two randomly selected images from each transect, resulting in a transect area of 2.6 m2 and 6.5 m2 in 2017 and 2018, respectively.
For all datasets, colony counts were summed across the transect and converted to density (col/m2) by dividing the transect colony count by the transect area. The predominant habitat for each survey was identified using the local scheme described by Schmahl et al. (2008) (Table 1), which included coral community (CC), deep reef (DR), coralline algae reef (CAR), rhodolith substrate (RS), and soft substrate (SS). Site summaries are presented in Table 2.
Location and Multibeam Derived Variables
These variables were obtained for the start location of transects in all datasets using the ROV’s onboard tracking system. Location was recorded as latitude and longitude in decimal degrees (DD). Due to the east-west orientation of the coastline in this region, latitude is closely related to distance from shore (Figure 1A). Depth was extracted from 4 m2 resolution multibeam bathymetry and depth derived habitat complexity variables (slope and local relief) were calculated using spatial analyst and zonal statistics in ESRI’s ArcGIS. Transects were classified into four a priori categories based on location (designated NAZ) or local relief (PSBF, LRRS, or FS). Coherent curves were used to examine covariance between latitude, longitude, depth, slope, and local relief using a Type III similarity profile permutation test on Pearson correlations of normalized environmental variables to define clusters of coherent variables which were statistically indistinguishable (Somerfield and Clarke, 2013). Variables found to have a significant effect on coral biodiversity were presented as bivariate scatter plots against α-diversity statistics, with a locally weighted scatterplot smoothing line (LOWESS). Coherent curves were generated in PRIMER version 7 (Clarke and Gorley, 2015) and scatterplots and smoothed lines were generated using the “PerformanceAnalytics” library (Peterson et al., 2020) in the R statistical package (R Core Team, 2018).
Coral Diversity and Community Structure Measures
Both alpha (α) and beta (β) diversity measures were calculated to assess multidimensional patterns in biodiversity. Alpha-diversity quantifies diversity at each sample site while β-diversity describes the variation in community structure. For this study, α-diversity was quantified by four indices: family richness, Shannon-Wiener diversity (H’), Pielou evenness (J’), and total density. Beta-diversity was calculated following Anderson et al. (2006), where β-diversity represented the variation in community composition in multivariate space. This was calculated using distance-to-centroid as defined by the Jaccard index similarity on presence/absence transformed family density data.
Statistical Analysis
Independently, α-diversity measures provide limited information about a community, but when combined in multivariate space, can provide a more powerful understanding of community diversity. Therefore, permutational multivariate analysis of variance (PERMANOVA; Anderson, 2017) using a multivariate similarity matrix was used to examine α-diversity. Rank similarity in α-diversity was determined using a Bray-Curtis distance matrix and examined for significant differences between categories (NAZ, PSBF, LRRS, FS) and effects of habitat complexity measures (depth, slope, and local relief) and location (latitude and longitude) using PERMANOVA. To reduce the influence of numerically dominant families and improve normal distribution, density was square root transformed and local relief was fourth root transformed to improve the normal distribution of the data. Between-group dissimilarities were calculated with similarity percentages (SIMPER; Clarke, 1993; Clarke et al., 2014), using Bray-Curtis similarity index and transformations, to examine how α-diversity measures contributed to significant differences. Significant differences in β-diversity between categories were tested with permutational analysis of multivariate dispersions (PERMDISP; Anderson et al., 2008). Statistical significance was determined by a threshold of p < 0.05. PERMANOVA, SIMPER, and PERMDISP were performed in PRIMER version 7 (Clarke and Gorley, 2015).
Multivariate regression trees (MRTs) are statistical descriptions of relationships between multispecies data and environmental characteristics. MRTs split multivariate data into hierarchical clusters based on explanatory variables, where each step minimizes dissimilarity within the cluster (De’ath, 2002). Each cluster represents a biodiversity assemblage where environmental values define the association (De’ath, 2002). This sequential testing process enables tree-based models to handle variable collinearity well. Overall fit is measured by relative error. MRTs were used to examine the relationship between α-diversity and habitat complexity variables (latitude, depth, and local relief) with a significant effect on coral biodiversity. Trees were developed with 100 iterations and the best tree, within one standard error (SE), was selected. MRT analysis was performed in R using the “mvpart” library (Therneau et al., 2014) in the R statistical package (R Core Team, 2018).
Due to the different study objectives (one excluding soft substrates and the other not), survey methods, area calculations, and image processing techniques, each dataset was analyzed independently in all calculations and multivariate analyses. The exclusion of soft substrates in 2011 – 2013 transects means these habitats are underrepresented in this dataset, potentially resulting in an inflated coral density in FS as all habitats were hard substrate. The survey methods and area calculations in 2011 – 2013 transects were more exact than 2016 – 2018 transects, where depth of field assumptions were made and mean area calculated from either a subset of assumed representative images or an assumed transect length of 100 m, increasing the uncertainty of density calculations in these transects.
Results
Coral Diversity and Community Structure
Within the four categories (NAZ, PSBF, LRRS, FS) surveyed, five major habitats, as defined by Schmahl et al. (2008), were identified: CC, DR, CAR, RS, and SS. CC, DR, and CAR represent sedimentary rock substrates, RS is a biogenic substrate, and SS is unconsolidated substrate. Within NAZs, RS and CAR were the predominant habitats, while outside NAZs, CAR and DR dominated (Figure 3A). FS was primarily composed of DR and CAR rock pavements or rubble.
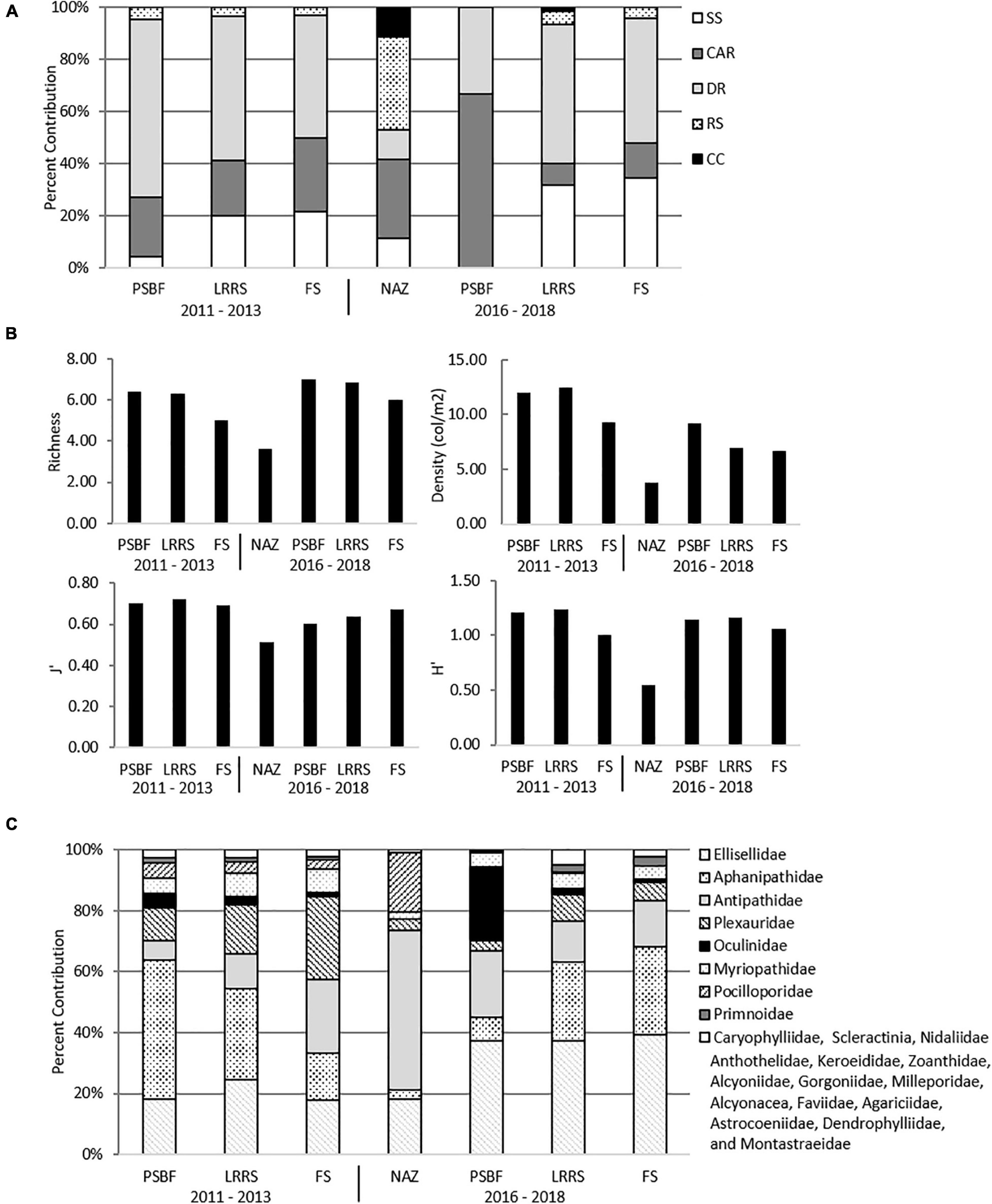
Figure 3. Characteristics of No Activity Zone (NAZ), Potentially Sensitive Biological Features (PSBF), Low Relief Rock Substrate (LRRS), and Flat Substrate (FS) (A) Proportion of habitats across categories. (B) Mean values for richness, density, evenness (J’), and diversity (H’) across categories. (C) Proportion of family density across categories.
Among all datasets and categories, coral density ranged from 0 to 61.84 col/m2 on all transects, with PSBFs and LRRSs possessing the highest mean density in both 2011 – 2013 (12.03 ± 2.15 SE and 12.44 ± 0.64 col/m2, respectively) and 2016 – 2018 datasets (9.24 ± 5.83 and 6.99 ± 1.54 col/m2, respectively). Similarly, across both datasets PSBFs and LRRSs also had the highest richness and diversity measures. NAZs, only documented in 2016 – 2018 transects, possessed the lowest mean density (3.75 ± 0.52 col/m2) and had the lowest richness and diversity in that dataset (Figure 3B). Across all datasets, a total of 25 families were observed, and included scleractinian corals, antipatharians, octocorals, and soft corals (Supplementary Table 1). Different coral families were found between categories, where Aphanipathidae and Ellisellidae dominated in PSBF and LRRS, Antipathidae in NAZs, and Plexauridae and Ellisellidae in FS (Figure 3C).
In both 2011 – 2013 and 2016 – 2018 transects, slope and local relief covaried (correlation = 0.51, p = 0.130 and correlation = 0.59, p = 0.134, respectively) and depth and latitude covaried (correlation = 0.71, p = 0.138 and correlation = 0.62, p = 0.140, respectively).
Statistical analysis of 2011 – 2013 transects found significant differences in α diversity between categories and a significant effect of depth, local relief, and latitude (Table 3). While generally α-diversity measures increased with increasing square root local relief and decreased with increasing latitude (closer to shore), α-diversity measures were maximized at depths between 100 and 140 m (Figure 4A). Pairwise tests indicated α-diversity was significantly different between PSBF and FS (t = 2.604, p = 0.003), where greater family richness and density in PSBF contributed >80% of the dissimilarity (Table 3). Further, β-diversity indicated FS had significantly greater community variation as compared to PSBFs (t = 2.741, p = 0.027; mean distance from centroid = 37.77 and 31.57, respectively) and LRRSs (t = 4.478, p < 0.001; mean distance from centroid = 37.420 and 33.058, respectively).
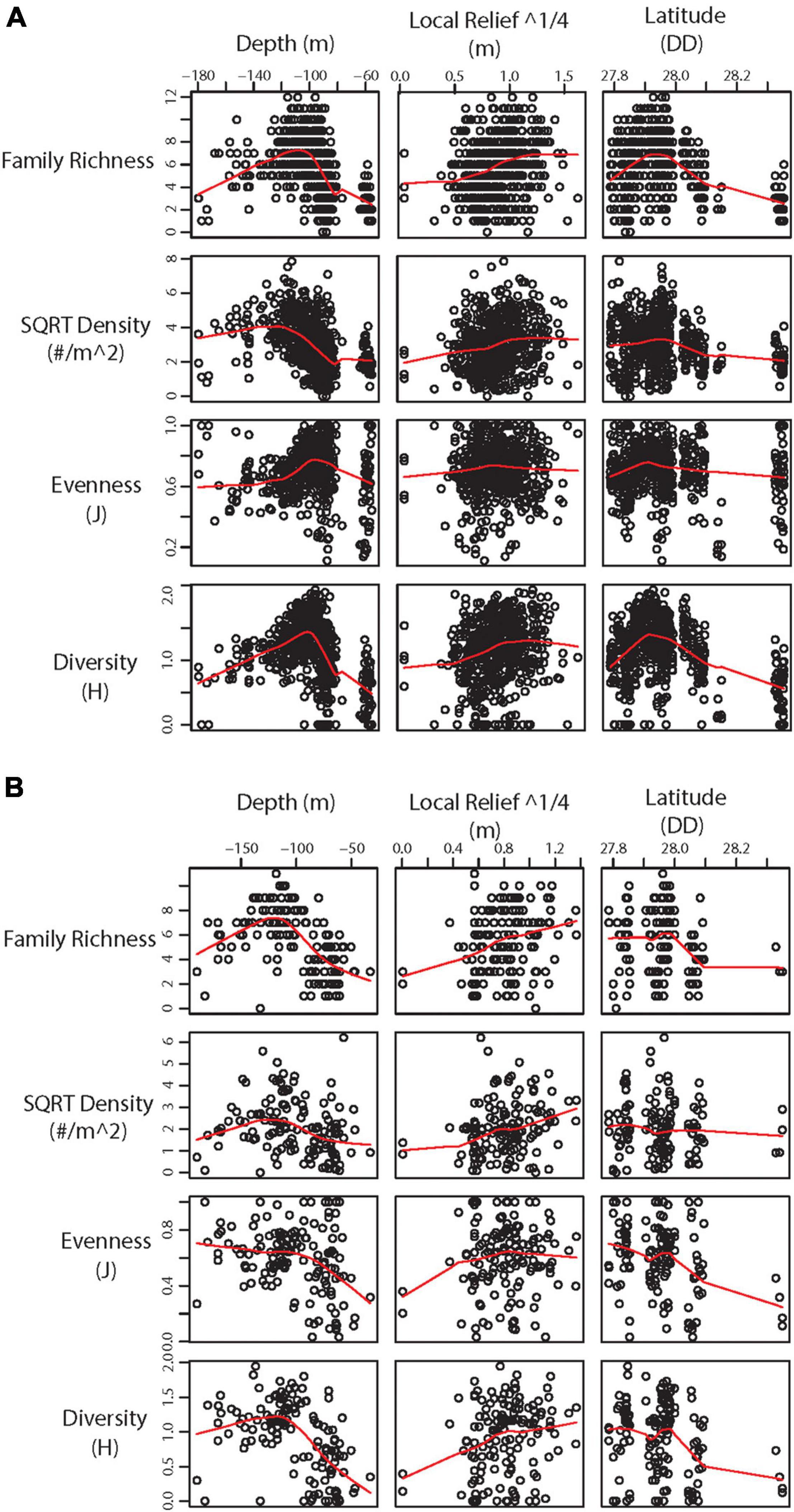
Figure 4. Scatter plot with LOWESS smoothed line (red) showing significant environmental variables against α-diversity statistics from (A) 2011 – 2013 transects and (B) 2016 – 2018 transects.
Similar to the 2011 – 2013 data, analysis of 2016 – 2018 transects found significant differences in α-diversity between categories and significant effect of depth, local relief, and latitude (Table 3). Again, α-diversity measures increased with increasing square root local relief and decreased with increasing latitude, while diversity measures were maximized at depths between 100 and 140 m (Figure 4B). Pairwise tests found significant differences between NAZ and LRRS (t = 3.508, p < 0.001), where greater family richness and density in LRRS contributed >80% of the dissimilarity (Table 3). Beta-diversity indicated NAZs had significantly greater community variation compared to LRRS (t = 3.443, p = 0.003; mean distance from centroid = 37.771 and 30.116, respectively).
In both datasets, NAZs and FSs had the greatest community variation but the lowest mean coral density and family richness, while PSBFs and LRRSs had the lowest community variation and the greatest mean coral density and family richness.
Coral Community Relationship to Location and Multibeam Derived Variables
In MRT analyses, depth was the most significant variable in both datasets. In 2011 – 2013 transects, surveys were split into shallow (<93.69 m) and deep (≥93.69 m), then further split by latitude. The shallower transects (<93.69 m) were split into mid shelf (≥28.08 DD), shelf edge (< 28.08 DD, ≥ 27.84 DD), and slope (< 27.87 DD) regions. Deeper transects (≥ 93.69 m) were separated into shelf edge (≥ 27.86 DD) and slope (< 27.86 DD) regions (Figures 5A, 6A). Deeper surveys had greater richness, density, and diversity, but lower evenness, compared to shallower surveys. In 2016 – 2018 transects, depth was the only split, where greater coral richness, density, and diversity, but lower evenness, were found in deeper (≥ 93.03 m) transects (Figures 5B, 6B).
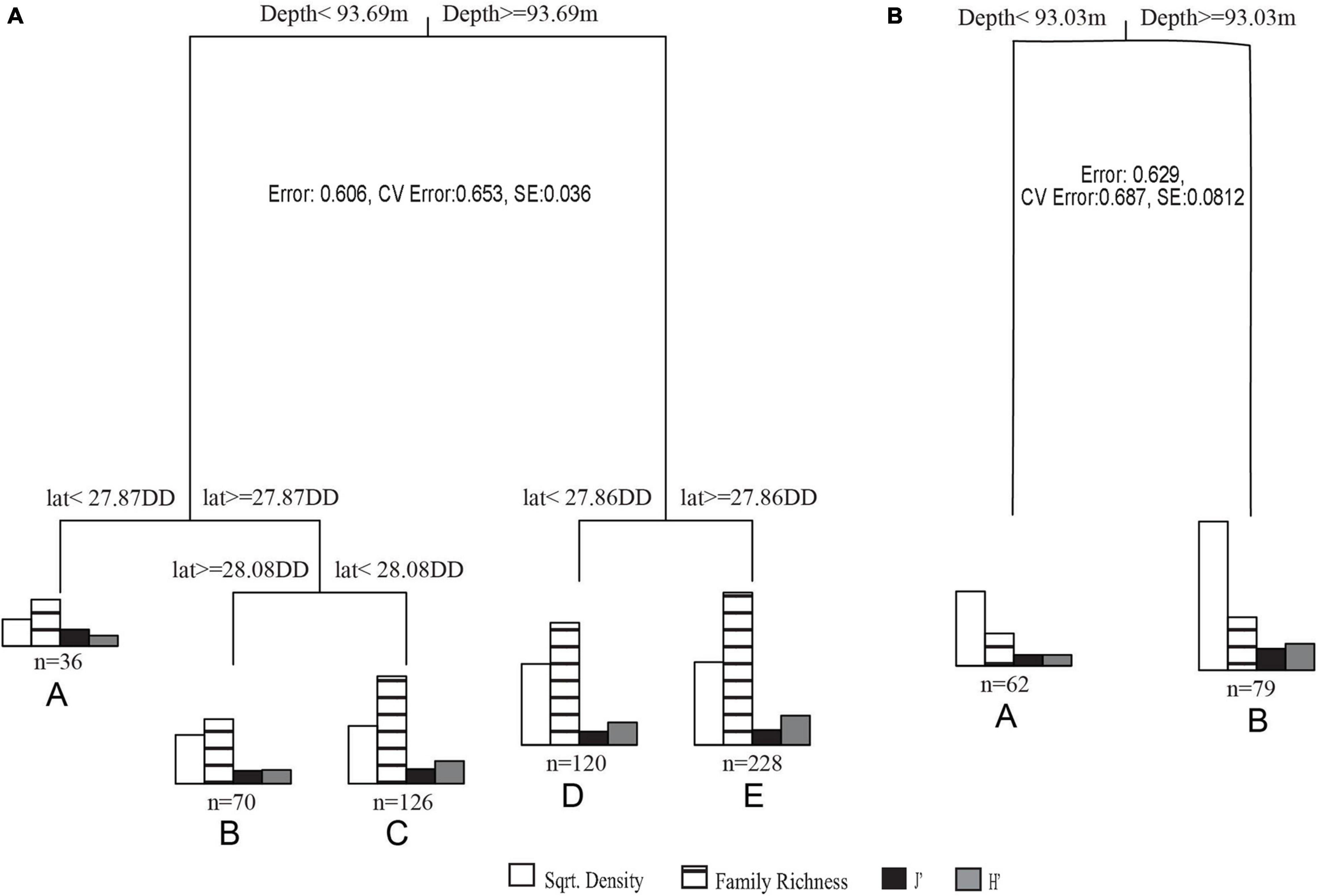
Figure 5. (A) 2011 – 2013 transects MRT where cluster A is <93.69 m on the slope, B is <93.69 m on the mid shelf, C is <93.69 m on the shelf edge, D is ≥93.69 m on the shelf edge, and E is ≥93.69 m on the slope. (B) 2016 – 2018 transects MRT where cluster A is <93.03 m and B is ≥93.03 m.
Discussion
The reefs and banks along the continental shelf of the northwestern GOM provide extensive habitats that support vulnerable mesophotic coral forests in densities greater than those documented to date in the Caribbean Sea (Slattery and Lesser, 2021), the North Atlantic Ocean (de Matos et al., 2014), and the Mediterranean Sea (Cau et al., 2015; Chimienti et al., 2020). However, most of the topographic features that support these communities represent surface expressions of underlying salt dome or diaper formations, whose geologic processes resulted in the formation of oil and natural gas deposits beneath the seafloor and, as a result, oil and gas exploration around these features has been extensive (Drew et al., 1982; Faucon, 2013). Routine activities associated with oil and gas exploration, production, and decommissioning can include, among others, anchoring, pile driving, platform placement and removal, explosive use, pipeline laying, and material disposal (Cordes et al., 2016). All of these can be detrimental to coral communities by causing sedimentation, contamination, and physical disturbance (Cordes et al., 2016). Therefore, BOEM has taken proactive steps to mitigate impacts through designated NAZ and PSBF (NTL No. 2009-G39, 2009) and accompanying lease stipulations. However, with the continued use and expansion of oil and gas infrastructure in the region, these mitigation strategies need periodic review to ensure management goals are being met.
We found that BOEM’s currently designated NAZs supported a distinct mesophotic coral forest of moderate density and variable community composition. Further, NAZs were found to be predominantly composed of rhodolith bed substrates. These are areas of active carbonate production and contributors to major ecosystem functions, including algal seed banks (Fredericq et al., 2019). They are also bioindicators for ocean acidification (Ragazzola et al., 2012) and they support a high biodiversity of reef fish (Moura et al., 2021), algae (Fredericq et al., 2019), and other invertebrates (Hinojosa-Arango and Riosmena-Rodríguez, 2004). Rhodolith beds are low relief, light dependent, communities and therefore contribute only a small proportion of the habitat within BOEM defined PSBFs, which were instead predominantly coralline algal reefs or deep reef substrates. PSBFs supported a greater density and diversity of mesophotic coral forests than NAZs, but were not found to support unique communities in terms of coral biodiversity and clustered with LRRSs. LRRSs, which are hard bottom features with less than 2.4 m of relief, are not currently identified as biologically sensitive underwater features in NTL No. 2009-G39. Both PSBFs and LRRSs supported the highest coral densities and diversities found in this study and community composition was the least variable. Despite these similarities, PSBFs and LRRSs supported different family assemblages, potentially due to the different proportion of habitat types encountered (Figure 3), where LRRSs had a greater proportion of interspersed soft substrate habitat than PSBFs. LRRSs were found to be similar in coral biodiversity and habitats as FS. However, due to the data collection methods, FS habitats were primarily represented by rock pavements or rubble features, while soft substrate habitats were underrepresented.
Remotely sensed datasets are commonly used as a cost effective and efficient surrogate for in situ measurements of biodiversity (McArthur et al., 2010). While BOEM primarily uses remotely sensed high resolution geophysical and seismic survey data to identify PSBFs, as they have a demonstrated ability to predict the presence of deep-water habitats (Roberts et al., 2000; Roberts et al., 2010), these datasets are proprietary and difficult to access in a raw format. However, other seafloor datasets, such as bathymetric maps, are readily available, and several bathymetry derived products, such as depth, slope, and local relief have been successfully used as indicators of biodiversity (Sammarco et al., 2016c; Silva and MacDonald, 2017; Hu et al., 2020). Together, seafloor observations, remotely sensed data products, and multivariate modeling can provide guidance for resource managers for planning exploration or extraction activities. Depth, latitude (as a proxy for distance from shore in this region), and local relief were found to have a significant effect on mesophotic coral abundance and diversity.
Depth was found to have the greatest explanatory power for changes in mesophotic coral biodiversity in this study. Richness, diversity, and density of mesophotic coral forests increased with depth, reaching a maximum around 100 m, and MRTs highlighted a significant hierarchical split at 93 m, where mesophotic coral forests occurring in shallower water had lower overall density and biodiversity. This depth split is similarly classified by BOEM designated NAZs for shelf edge banks (85 m isobath), delineating the cluster of coral biodiversity unique to NAZs. It is also within this depth range (80 – 130 m) that the cross-over occurs from sunlit nutrient poor waters to nutrient rich waters, where light penetration is insufficient to facilitate biological carbonate production (Cullen, 1982; Sigman and Hain, 2012) and a significant change in the coral community from light dependent to heterotrophic species is expected in this depth zone. A greater proportion of reef-building pocilloporid corals and biogenic RS was found in NAZs, further supporting that current NAZs are protecting an area of actively accreting reef-building communities responsible for creating biogenic rock and where the greatest carbonate production is occurring. Below the depth ranges encompassed by NAZs, heterotrophic coral families predominate. While these communities are not actively creating biogenic rock substrates, their living skeletons create three-dimensional coral forests, providing structure and habitat for a multitude of organisms. Active management and protection of these living communities is important to maintain their health and the health of the ecosystems they support.
Latitudinal location on the continental shelf was identified as a secondary contributor to changes in coral biodiversity. In this region, latitude directly corresponds with distance from shore (Figure 1A), where by as latitude decreases, distance from shore and depth increase and temperature and turbidity decrease. The changes in these physical parameters can drive changes in biodiversity (Rezak et al., 1985; Jordán-Garza et al., 2017) as tolerance varies amongst coral species and their degree of reliance on heterotrophy (Berkelmans and van Oppen, 2006; Grottoli et al., 2006; Roberts et al., 2009). While latitude and depth variables covaried, the MRT model for 2011 – 2013 identified significant latitudinal splits in the data, a relationship long documented in the northwestern GOM. Neumann (1958) originally classified these features into three distinct groups: near shore (within 17 miles of the shore), mid shelf (45–75 miles offshore), and shelf edge (75 miles to shelf edge). MRT results identified mid shelf features occurred at ≥ 28.08 DD, corresponding to approximately 110 miles from shore. For shelf edge features, the split occurred between 28.08 DD and 27.87 DD, corresponding to approximately 110 to 130 miles from shore. Both these splits occurred a greater distance from shore than the classification made by Neumann in 1958, indicating that the mid shelf bank classification has a broader geographic footprint than previously thought. In addition, it is becoming apparent that features occurring beyond the continental shelf break [180 m isobath (Gore, 1992)] possess unique biological communities (Sammarco et al., 2016a) and coral biodiversity (this study) and should be considered as a fourth geographic group: slope features. MRT results identified this split at <27.87 DD, corresponding to > 130 miles from shore. Mid shelf features supported the lowest coral diversity and density while shelf edge features supported the greatest.
Similar to previous findings examining in situ relief by Sammarco et al. (2016b), biodiversity increased with increasing local relief. However, a significant split in local relief was not identified as an explanatory variable for changes in mesophotic coral biodiversity in the model. This may be due to a survey design that favored hard substrates (2011 – 2013 project) and features of interest within the CBZ (2016 – 2019 project) where coral density would have been higher than in flat, soft, sediments away from hard substrate or outside of the CBZ. In this study, FS areas were predominantly composed of hardbottom pavements and rubble, not soft substrates, and the observed similarities in α-diversity of coral communities between FS and LRRS highlights that, while relief and biodiversity are positively correlated, flat hardbottom pavements and rubble fields provide potential habitat for mesophotic and deep-sea corals but require further examination. In order to draw conclusions about relief thresholds that characterize natural splits in mesophotic coral biodiversity, additional data needs to be collected to help define the relationship between flat habitats and coral biodiversity.
Extensive vulnerable mesophotic coral communities occur in association with each of the banks examined in this study and are the target of oil and gas exploration in the northwestern GOM due to their underlying salt diaper. As a precautionary management strategy, BOEM developed NAZ and PSBF designations to protect sensitive biological communities, but these designations have not been formally re-evaluated since the 1980s. This study presents an extensive new dataset and found that currently designated NAZs continue to represent a distinct biological coral community that is sufficiently captured within the isobath definition implemented by BOEM for shelf edge and slope features. NAZs support unique and moderately dense coral communities, and extensive rhodolith bed substrates that play a critical role in the ocean carbon cycle. The recently expanded FGBNMS boundaries closely resemble the NAZs and therefore provide additional protections to these habitats. However, areas with greater coral density and diversity exist outside, but in close proximity, to NAZs and are not included in the current definition of PSBFs due to their low relief.
The >2.44 m relief threshold used to define PSBFs by BOEM does not represent a low relief threshold for the development of the mesophotic coral communities and features with much lower relief support similar communities. Our findings suggest that low relief rock substrates serve as important habitat for abundant and diverse mesophotic coral forests that warrant consideration for protection from bottom disturbing activities. Further, we suggest an expansion of BOEMs lease stipulation should be considered in order to include biota on features of low relief (down to 0.33 m). An expansion of these lease stipulations will not only provide active protection for the vulnerable coral communities within the low relief substrates, but also support the preservation of broader ecosystems and ecosystem services (Moffitt et al., 2010; Edgar et al., 2014). Generally, the number of species protected increases with increasing size of the protected area, especially for species with smaller home ranges and where habitats are diverse (Moffitt et al., 2010). In dynamic open ocean environments like the study area, spatial prioritization to protect a wide representation of habitats is critical to support spatial connectivity at the population, genetic, and ecosystem level (Ban et al., 2014; Carr et al., 2017). Further, these protections should not be limited to a single sector (i.e., oil and gas) and instead need an integrated approach to better safeguard these vulnerable communities from other threats such as fishing and anchoring. Though not addressed here, further consideration should also be given to the delineating boundaries that can facilitate management and enforcement within these areas.
Conclusion
• The northwestern GOM harbors some of the densest mesophotic coral forests reported to date.
• Low relief rock substrates serve as important habitat for abundant and diverse mesophotic coral forests.
• BOEMs current NAZ and PSBF lease stipulations do not fully encompass vulnerable mesophotic communities and would need to be enlarged to include assemblages on features of lower relief (possibly those >0.33 m).
• Mesophotic coral forest diversity changes with latitude, which in this region correlates with distance from shore. Banks classified as “mid-shelf” based on their biotic
composition were found to occur at a greater distance from shore than previous research identified and a fourth geographic group, slope features, may be warranted.
Data Availability Statement
The raw data supporting the conclusions of this article will be made available by the authors, without undue reservation.
Author Contributions
MN contributed to data processing, led the analysis, and writing of the manuscript. EH and PS were a principal investigator on data collection cruises, assisted with the development of, and provided edits for this manuscript. RB contributed to data processing, assisted with the development of, and provided edits for this manuscript. GS was the superintendent of FGBNMS, guided the development of the studies presented, assisted with the development of, and provided edits for this manuscript. All authors contributed to the development of the ideas and results presented in this manuscript.
Funding
The funding for this project was provided, in part, by the U.S. Department of the Interior/BOEM/Environmental Studies Program, through Interagency Agreement M11AC00005 with the NOAA’s National Ocean Service/Office of National Marine Sanctuaries/Flower Garden Banks National Marine Sanctuary and by NOAA’s Deep-Sea Coral Research and Technology Program.
Conflict of Interest
MN and RB were employed by company CPC Inc.
The remaining authors declare that the research was conducted in the absence of any commercial or financial relationships that could be construed as a potential conflict of interest.
Publisher’s Note
All claims expressed in this article are solely those of the authors and do not necessarily represent those of their affiliated organizations, or those of the publisher, the editors and the reviewers. Any product that may be evaluated in this article, or claim that may be made by its manufacturer, is not guaranteed or endorsed by the publisher.
Acknowledgments
We would like to recognize the extensive contribution of the people who collected and processed the datasets used in this manuscript including the crew of the R/V Manta, University of North Carolina at Wilmington Undersea Vehicle Program ROV pilots (Eric Glidden, Lance Horn, Glen Taylor, and Jason White), Flower Garden Banks National Marine Sanctuary contractors (Kaitlin Buhler, Ryan Eckert, John Embesi, Allison Kuba, Kelly O’Connell, Travis Sterne, and Jimmy MacMillan), Louisiana Universities Marine Consortium staff (Daniel Beltz), BOEM staff (Mark Belter, Alicia Caporaso, and Michelle Nannen) and BSEE staff (James Sinclair). The scientific results and conclusions, as well as any views or opinions expressed herein, are those of the author(s) and do not necessarily reflect the views of NOAA or the Department of Commerce.
Supplementary Material
The Supplementary Material for this article can be found online at: https://www.frontiersin.org/articles/10.3389/fmars.2021.780248/full#supplementary-material
References
15 CFR Part 922 – Subpart L (2021). National marine sanctuary regulations, Flower Garden Banks National Marine Sanctuary. Fed. Regist. 15, 120–123.
Anderson, M., Gorley, R., and Clarke, K. (2008). PERMANOVA+ for PRIMER. Guide to Software and Statistical Methods. Plymouth: PRIMER-E.
Anderson, M. J. (2017). “Permutational multivariate analysis of variance (PERMANOVA),” in Wiley StatsRef: Statistics Reference Online, eds N. Balakrishnan, T. Colton, B. Everitt, W. Piegorsch, and F. Ruggeri and J. L. Teugels (Chichester: John Wiley & Sons, Ltd.), 1–15. doi: 10.1002/9781118445112.stat07841
Anderson, M. J., Ellingsen, K. E., and McArdle, B. H. (2006). Multivariate dispersion as a measure of beta diversity. Ecol. Lett. 9, 683–693. doi: 10.1111/j.1461-0248.2006.00926.x
Ban, N. C., Maxwell, S. M., Dunn, D. C., Hobday, A. J., Bax, N. J., Ardron, J., et al. (2014). Better integration of sectoral planning and management approaches for the interlinked ecology of the open oceans. Mar. Policy 49, 127–136. doi: 10.1016/j.marpol.2013.11.024
Berkelmans, R., and van Oppen, M. J. H. (2006). The role of zooxanthellae in the thermal tolerance of corals: a ‘nugget of hope’ for coral reefs in an era of climate change. Proc. R. Soc. Biol. Sci. U.S.A. 273, 2305–2312. doi: 10.1098/rspb.2006.3567
Bo, A. (2008). Taxonomy and Ecology of Antipatharians. Ph.D. dissertation in Marine Biology and Ecology. Ancona: Universita Politecnica Delle Marche, 212.
Bright, T., and Rezak, R. (1978). Northwestern Gulf of Mexico Topographic Features Study. Final Report to the BLM. Contract no AA550-CT7-15. College Station, TX: Texas A&M Research Foundation and Texas A&M Dept of Oceanography.
Brooke, S., Homes, M., and Young, C. M. (2009). Sediment tolerance of two different morphotypes of the deep-sea coral Lophelia pertusa from the Gulf of Mexico. Mar. Ecol. Prog. Ser. 390, 137–144. doi: 10.3354/meps08191
Carr, M. H., Robinson, S. P., Wahle, C., Davis, G., Kroll, S., Murray, S., et al. (2017). The central importance of ecological spatial connectivity to effect coastal marine protected areas and to meeting the challenges of climate change in the marine environment. Aquat. Conserv. Mar. Freshw. Ecosyst. 27, 6–29. doi: 10.1002/aqc.2800
Cau, A., Follesa, M. C., Moccia, D., Alvito, A., Bo, M., Angiolillo, M., et al. (2015). Deepwater corals biodiversity along roche du large ecosystems with different habitat complexity along the south Sardinia continental margin (CW Mediterranean Sea). Mar. Biol. 162, 1865–1878. doi: 10.1007/s00227-015-2718-5
Chimienti, G., Bo, M., Taviani, M., and Mastrototaro, F. (2019). “19 Occurrence and biogeography of mediterranean cold-water corals,” in Mediterranean Cold-Water Corals: Past, Present and Future: Understanding the Deep-Sea Realms of Coral, eds C. Orejas and C. Jiménez (Cham: Springer International Publishing), 213–243. doi: 10.1007/978-3-319-91608-8_19
Chimienti, G., De Padova, D., Mossa, M., and Mastrototaro, F. (2020). A mesophotic black coral forest in the Adriatic sea. Sci. Rep. 10:8504. doi: 10.1038/s41598-020-65266-9
Clark, M. R., Althaus, F., Schlacher, T. A., Williams, A., Bowden, D. A., and Rowden, A. A. (2016). The impacts of deep-sea fisheries on benthic communities: a review. ICES J. Mar. Sci. 73, i51–i69.
Clark, M. R., and Koslow, J. A. (2007). “Impacts of fisheries on seamounts in seamounts: ecology, fisheries, and conservation,” in Blackwell Fisheries and Aquatic Resources Series 12, eds T. J. Pitcher, T. Morato, P. J. B. Hart, M. R. Clark, N. Haggan, and R. S. Santos (Oxford: Blackwell Publishing), 413–441. doi: 10.1002/9780470691953.ch19
Clark, M. R., Rowden, A. A., Schlacher, T., Williams, A., Consalvey, M., Stocks, K. I., et al. (2010). The ecology of seamounts: structure, function and human impacts. Annu. Rev. Mar. Sci. 2, 253–278. doi: 10.1146/annurev-marine-120308-081109
Clarke, K. (1993). Non−parametric multivariate analyses of changes in community structure. Aust. J. Ecol. 18, 117–143. doi: 10.1111/j.1442-9993.1993.tb00438.x
Clarke, K. R., Gorley, R., Somerfield, P. J., and Warwick, R. (2014). Change in Marine Communities: An Approach to Statistical Analysis and Interpretation. Plymouth: PRIMER-E.
Clarke, K. R., and Gorley, R. N. (2015). PRIMER v7: User Manual/Tutorial. Plymouth: Primer-E Limited, 296.
Cordes, E. E., Jones, D. O. B., Schlacher, T. A., Amon, D. J., Bernardino, A. F., Brooke, S., et al. (2016). Environmental impacts of the deep-water oil and gas industry: a review to guide management strategies. Front. Environ. Sci. 4:58. doi: 10.3389/fenvs.2016.00058
Cullen, J. J. (1982). The deep chlorophyll maximum: comparing vertical profiles of chlorophyll a. Can. J. Fish. Aquat. Sci. 39, 791–803. doi: 10.1139/f82-108
de Matos, V., Gomes-Pereira, J. N., Tempera, F., Ribeiro, P. A., Braga-Henriques, A., and Porteiro, F. (2014). First record of Antipathella subpinnata (Anthozoa, Antipatharia) in the Azores (NE Atlantic), with description of the first monotypic garden for this species. Deep Sea Res. II Top. Stud. Oceanogr. 99, 113–121. doi: 10.1016/j.dsr2.2013.07.003
De’ath, G. (2002). Multivariate regression trees: a new technique for modeling species-environment relationships. Ecology 83, 1105–1117.
Drew, L. J., Schuenemeyer, J. H., and Bawiec, W. J. (1982). Estimation of the Future Rates of Oil and Gas Discoveries in the Western Gulf of Mexico. Geological Survey. Newark, DE: Delaware University.
Edgar, G. J., Stuart-Smith, R. D., Willis, T. J., Kininmonth, S., Baker, S. C., Banks, S., et al. (2014). Global conservation outcomes depend on marine protected areas with five key elements. Nature 506, 216–220. doi: 10.1038/nature13022
EIA (2021). Gulf of Mexico Fact Sheet. Energy Information Administration. Available online at: https://www.eia.gov/special/gulf_of_mexico/ (accessed June 4, 2021).
Erftemeijer, P. L. A., Riegl, B., Hoeksema, B. W., and Todde, P. A. (2012). Environmental impacts of dredging and other sediment disturbances on corals: a review. Mar. Pollut. Bull. 64, 1737–1765. doi: 10.1016/j.marpolbul.2012.05.008
Esslemont, G., Russell, R. A., and Maher, W. A. (2004). Coral record of harbor dredging: Townsville, Australia. J. Mar. Syst. 52, 51–64.
Faucon, B. (2013). Oil Companies Go Deep. The Wall Street Journal. Available online at: https://www.wsj.com/articles/SB10001424052702303442004579123560225082786 (accessed June 1, 2021).
Fossa, J. H., Mortensen, P. B., and Furevik, D. M. (2002). The deep-water coral Lophelia pertusa in Norwegian waters: distribution and fishery impacts. Hydrobiologia 471, 1–12.
Fredericq, S., Krayesky-Self, S., Sauvage, T., Richards, J., Kittle, R., Arakaki, N., et al. (2019). The critical importance of rhodoliths in the life cycle completion of both macro- and microalgae, and as holobionts for the establishment and maintenance of marine biodiversity. Front. Mar. Sci. 5:502. doi: 10.3389/fmars.2018.00502
Gass, S. E., and Roberts, J. M. (2006). The occurrence of the cold-water coral Lophelia pertusa (Scleractinia) on oil and gas platforms in the North Sea: colony growth, recruitment and environmental controls on distribution. Mar. Pollut. Bull. 52, 549–559. doi: 10.1016/j.marpolbul.2005.10.002
Girard, F., and Fisher, C. R. (2018). Long-term impact of the Deepwater Horizon oil spill on deep-sea corals detected after seven years of monitoring. Biol. Conserv. 225, 117–127. doi: 10.1016/j.biocon.2018.06.028
Grottoli, A., Rodrigues, L., and Palardy, J. E. (2006). Heterotrophic plasticity and resilience in bleached corals. Nature 440, 1186–1189. doi: 10.1038/nature04565
Hall-Spencer, J., Allain, V., and Fossa, J. H. (2002). Trawling damage to Northeast Atlantic ancient coral reefs. Proc. R. Soc. Lond. B Biol. 269, 507–511. doi: 10.1098/rspb.2001.1910
Heifetz, J., Stone, R. P., and Shotwell, S. K. (2009). Damage and disturbance to coral and sponge habitat of the Aleutian archipelago. Mar. Ecol. Prog. Ser. 397, 295–303. doi: 10.3354/meps08304
Hickerson, E. L., and Schmahl, G. P. (2005). “The state of coral reef ecosystems of the Flower Garden Banks, Stetson Bank, and other banks in the northwestern Gulf of Mexico,” in The State of Coral reef Ecosystems of the United States and Pacific Freely Associated States, ed. J. E. Waddell (Silver Spring, MD: NOAA Technical Memorandum NOS NCCOS 11), 189–217.
Hinojosa-Arango, G., and Riosmena-Rodríguez, R. (2004). Influence of rhodolith-forming species and growth-form on associated fauna of rhodolith beds in the central-west Gulf of California, México. Mar. Ecol. 25, 109–127. doi: 10.1111/j.1439-0485.2004.00019.x
Hogg, M., Tendal, O., Conway, K., Pomponi, S., Gutt, J., Krautter, M., et al. (2010). Deep-Sea Sponge Grounds: Reservoirs of Biodiversity WCMC Biodiversity Series. Cambridge: UNEP-WCMC, 86.
Hourigan, T. F., Etnoyer, P. J., and Cairns, S. D. (2017). The State of Deep-Sea Coral and Sponge Ecosystems of the United States. NOAA Technical Memorandum NMFS−OHC−4. Silver Spring, MD: NOAA Technical Memo, 467.
Hsing, P. Y., Fu, B., Larcom, E. A., Berlet, S. P., Shank, T. M., Govindarajan, A. F., et al. (2013). Evidence of lasting impact of the Deepwater Horizon oil spill on a deep Gulf of Mexico coral community. Elem. Sci. Anthropocene 1:000012. doi: 10.12952/journal.elementa.000012
Hu, Z., Hu, J., Hu, H., and Zhou, Y. (2020). Predictive habitat suitability modeling of deep-sea framework-forming scleractinian corals in the Gulf of Mexico. Sci. Total Environ. 742:140562. doi: 10.1016/j.scitotenv.2020.140562
Johnston, M. A., O’Connell, K., Blakeway, R. D., MacMillan, J., Nuttall, M. F., Hu, X., et al. (2021). Long-Term Monitoring at East and West Flower Garden Banks: 2019 Annual Report National Marine Sanctuaries Conservation Series ONMS-21-02. Galveston, TX: U.S. Department of Commerce, National Oceanic and Atmospheric Administration, Flower Garden Banks National Marine Sanctuary, 88.
Jones, R., Fisher, R., and Bessell-Browne, P. (2019). Sediment deposition and coral smothering. PLoS One 14:e0216248. doi: 10.1371/journal.pone.0216248
Jordán-Garza, A. G., González-Gándara, C., Salas-Pérez, J. J., and Morales-Barragan, A. M. (2017). Coral assemblages are structured along a turbidity gradient on the Southwestern Gulf of Mexico, Veracruz. Cont. Shelf Res. 138, 32–40. doi: 10.1016/j.csr.2017.03.002
Kaiser, M. J., and Narra, S. (2019). A retrospective of oil and gas field development in the U.S. outer continental shelf Gulf of Mexico, 1947–2017. Nat. Resour. Res. 28, 685–715. doi: 10.1007/s11053-018-9414-3
Locker, S. D., and Hine, A. C. (2020). “An overview of the geologic origins of hydrocarbons and production trends in the Gulf of Mexico,” in Scenarios and Responses to Future Deep Oil Spills, eds S. Murawski, C. Ainsworth, S. Gilbert, D. Hollander, C. B. Paris, M. Schlüter, et al. (Cham: Springer), 60–74. doi: 10.1007/978-3-030-12963-7_4
Lumsden, S. E., Hourigan, T. F., Bruckner, A. W., and Dorr, G. (eds) (2007). The State of Deep Coral Ecosystems of the United States. NOAA Technical Memorandum CRCP-3. Silver Spring MD: NOAA Technical Memo, 365.
McArthur, M., Brooke, B., Przeslawaki, R., Ryan, D., Lucieer, V., Nicol, S., et al. (2010). A Review of Surrogates for Marine Benthic Biodiversity. Geoscience Australia, Record 2009/42. Canberra, ACT: Geoscience Australia, 61.
Moffitt, E. A., White, J. W., and Botsford, L. W. (2010). The utility and limitations of size and spacing guidelines for designing marine protected area (MPA) networks. Biol. Conserv. 144, 306–318. doi: 10.1016/j.biocon.2010.09.008
Mortensen, P. B., and Buhl-Mortensen, L. (2004). Distribution of deep-water gorgonian corals in relation to benthic habitat features in the Northeast Channel (Atlantic Canada). Mar. Biol. 144, 1223–1238. doi: 10.1007/s00227-003-1280-8
Moura, R. L., Abieri, M. L., Castro, G. M., Carlos-Júnior, L. A., Chiroque-Solano, P. M., Fernandes, N. C., et al. (2021). Tropical rhodolith beds are a major and belittled reef fish habitat. Sci. Rep. 11:794. doi: 10.1038/s41598-020-80574-w
Neumann, A. C. (1958). The Configuration and Sediments of Stetson Bank, Northwestern Gulf of Mexico. Master’s thesis. College Station, TX: Texas A&M University.
NTL No. 2009-G39 (2009). Biologically-Sensitive Underwater Features and Areas. Available online at: https://www.bsee.gov/sites/bsee.gov/files/notices-to-lessees-ntl/notices-to-lessees/09-g39.pdf (accessed November 11, 2021).
Nuttall, M. F., Somerfield, P. J., Sterne, T. K., MacMillian, J., Embesi, J. A., Hickerson, E. L., et al. (2020). Stetson Bank Long-Term Monitoring: 1993-2015. National Marine Sanctuaries Conservation Series ONMS-20-06. Galveston, TX: U.S. Department of Commerce, National Oceanic and Atmospheric Administration, Flower Garden Banks National Marine Sanctuary, 156.
ONMS (2016). Flower Garden Banks National Marine Sanctuary Expansion Draft Environmental Impact Statement. Silver Spring, MD: U.S. Department of Commerce, National Oceanic and Atmospheric Administration, Office of National Marine Sanctuaries, 153.
Peterson, B. G., Carl, P., Boudt, K., Bennett, R., Ulrich, J., Zivot, E., et al. (2020). Package ‘PerformanceAnalytics’. R Package Version 2.0.4. Available online at: https://cran.r-project.org/package=PerformanceAnalytics
Prouty, N. G., Roark, E. B., Buster, N. A., and Ross, S. W. (2011). Growth rate and age distribution of deep-sea black coral sin the Gulf of Mexico. Mar. Ecol. Prog. Ser. 432, 101–115. doi: 10.3354/mepg08953
R Core Team (2018). R: A Language and Environment for Statistical Computing. Vienna: R Foundation for Statistical Computing.
Ragazzola, F., Foster, L. C., Form, A., Anderson, P. S. L., Hansteen, T. H., and Fietzke, J. (2012). Ocean acidification weakens the structural integrity of coralline algae. Glob. Change Biol. 18, 2804–2812. doi: 10.1111/j.1365-2486.2012.02756.x
Reed, J. K., Koenig, C. C., and Shepard, A. N. (2007). Impacts of bottom trawling on a deep-water Oculina coral ecosystem off Florida. Bull. Mar. Sci. 81, 481–496.
Rezak, R., and Bright, T. (1981). Northern Gulf of Mexico Topographic Features Study. Final Report, Bureau of Land Management, Contract AA551-Ct8-35, Vol. 3. College Station, TX: Texas A&M Research Foundation and Texas A&M University, 99–127.
Rezak, R., Bright, T. J., and McGrail, D. W. (1985). Reefs and Banks of the Northwestern Gulf of Mexico: Their Geological, Biological, and Physical Dynamics. New York, NY: John Wiley and Sons, 259.
Roark, E. B., Guilderson, T. P., Dunbar, R. B., Fallon, S. J., and Mucciarone, D. A. (2009). Extreme longevity in proteinaceous deep-sea corals. Proc. Natl. Acad. Sci. U.S.A. 106, 5204–5208. doi: 10.1073/pnas.0810875106
Roberts, H. H., Coleman, J., Hunt, J. Jr., and Shedd, W. (2000). Surface amplitude mapping of 3D-seismic for improved interpretations of seafloor geology and biology from remotely sensed data. Gulf Coast Assoc. Geol. Soc. Trans. 50, 495–503.
Roberts, H. H., Feng, D., and Joye, S. B. (2010). Cold-seep carbonates of the middle and lower continental slope, northern Gulf of Mexico. Deep Sea Res. II Top. Stud. Oceanogr. 57, 2040–2054. doi: 10.1016/j.dsr2.2010.09.003
Roberts, J. M., Wheeler, A. J., Freiwald, A., and Cairns, S. D. (2009). Cold-Water Corals: The Biology and Geology of Deep-Sea Coral Habitats. Cambridge: Cambridge University Press, 1–334.
Rossi, S., Bramanti, L., Gori, A., and Orejas, C. (2017). “An overview of the animal forests of the world,” in Marine Animal Forests, eds S. Rossi, L. Bramanti, A. Gori, and C. Orejas (Cham: Springer), 1–26. doi: 10.1007/978-3-319-21012-4_1
Sammarco, P. W. (2016). Deepwater Reconnaissance of Potentially Sensitive Biological Features Surrounding Shelf-Edge Topographic Banks in the Northern Gulf of Mexico. OCS Study BOEM 2017-024. New Orleans, LA: US Dept. of the Interior, Bureau of Ocean Energy Management, Gulf of Mexico OCS Region, 1–109.
Sammarco, P. W., Nuttall, M. F., Beltz, D., Hickerson, E. H., and Schmahl, G. P. (2016a). Patterns of mesophotic benthic community structure on banks off vs inside the continental shelf edge, Gulf of Mexico. Gulf M. Sci. 33, 77–92.
Sammarco, P. W., Nuttall, M. F., Beltz, D., Horn, L., Taylor, G., Hickerson, E. L., et al. (2016b). The positive relationship between relief and species richness in mesophotic communities on offshore banks, including geographic patterns. Environ. Geosci. 23, 195–207. doi: 10.1306/eg.12071615020
Sammarco, P. W., Horn, L., Taylor, G., Beltz, D., Nuttall, M. F., Hickerson, E. L., et al. (2016c). A statistical approach to assessing relief on mesophotic banks: bank comparisons and geographic patterns. Environ. Geosci. 23, 95–122. doi: 10.1306/eg.01121615013
Schmahl, G. P., Hickerson, E. L., and Precht, W. F. (2008). “Biology and ecology of coral reefs and coral communities in the Flower Garden Banks Region, Northwestern Gulf of Mexico,” in Coral Reefs of the USA, eds B. M. Riegl and R. E. Dodge (Dordrecht: Springer), 221–261. doi: 10.1007/978-1-4020-6847-8_6
Semmler, R. F., Hoot, W. C., and Reaka, M. L. (2016). Are mesophotic coral ecosystems distinct communities and can they serve as refugia for shallow reefs? Coral Reefs 36, 433–444. doi: 10.1371/journal.pone.0188598
Sherwood, O. A., and Edinger, E. N. (2009). Ages and growth rates of some deep-sea gorgonian and antipatharian corals of Newfoundland and Labrador. Can. J. Fish. Aquat. Sci. 66, 142–152. doi: 10.1139/f08-195
Sigman, D. M., and Hain, M. P. (2012). The biological productivity of the ocean. Nat. Educ. Knowl. 3:21.
Silva, M., and MacDonald, I. R. (2017). Habitat suitability modeling for mesophotic coral in the northeastern Gulf of Mexico. Mar. Ecol. Prog. Ser. 583, 121–136. doi: 10.3354/meps12336
Slattery, M., and Lesser, M. P. (2021). Gorgonians are foundation species on sponge-dominated mesophotic coral reefs in the Caribbean. Front. Mar. Sci. 8:304. doi: 10.3389/fmars.2021.654268
Somerfield, P. J., and Clarke, K. R. (2013). Inverse analysis in non-parametric multivariate analyses: distinguishing groups of associated species which covary coherently across samples. J. Exp. Mar. Biol. Ecol. 449, 261–273. doi: 10.1016/j.jembe.2013.10.002
Therneau, T., Atkinson, B., Ripley, B., Oksanen, J., and De’Ath, G. (2014). mvpart: Multivariate Partitioning. R Package Version 1.6-2. Available online at: https://cran.r-project.org/package=mvpart
Trannum, H., Nilsson, H. C., Schaanning, M. T., and Norling, K. (2011). Biological and biogeochemical effects of organic matter and drilling discharges in two sediment communities. Mar. Ecol. Prog. Ser. 442, 23–36. doi: 10.3354/meps09340
White, H. K., Hsing, P. Y., Cho, W., Shank, T. M., Cordes, E. E., Quattrini, A. M., et al. (2012). Impact of the Deepwater Horizon oil spill on a deep-water coral community in the Gulf of Mexico. Proc. Natl. Acad. Sci. U.S.A. 109, 20303–20308. doi: 10.1073/pnas.1118029109
Yoklavich, M. M., Laidig, T. E., Graiff, K., Clarke, M. E., and Whitmire, C. E. (2018). Incidence of disturbance and damage to deep-sea corals and sponges in areas of high trawl bycatch near the California and Oregon border. Deep Sea Res. II Top. Stud. Oceanogr. 150, 156–163. doi: 10.1016/j.dsr2.2017.08.005
Keywords: mesophotic coral, potentially sensitive biological features, oil and gas, coral forest, no activity zone, Gulf of Mexico, lease stipulations, conservation policy
Citation: Nuttall MF, Hickerson EL, Blakeway RD, Schmahl GP and Sammarco PW (2022) Do Oil and Gas Lease Stipulations in the Northwestern Gulf of Mexico Need Expansion to Better Protect Vulnerable Coral Communities? How Low Relief Habitats Support High Coral Biodiversity. Front. Mar. Sci. 8:780248. doi: 10.3389/fmars.2021.780248
Received: 21 September 2021; Accepted: 06 December 2021;
Published: 12 January 2022.
Edited by:
William F. Precht, Dial Cordy and Associates, Inc., United StatesReviewed by:
Daniel Pech, El Colegio de la Frontera Sur, MexicoOrion Carlos Norzagaray, Autonomous University of Baja California, Mexico
Copyright © 2022 Nuttall, Hickerson, Blakeway, Schmahl and Sammarco. This is an open-access article distributed under the terms of the Creative Commons Attribution License (CC BY). The use, distribution or reproduction in other forums is permitted, provided the original author(s) and the copyright owner(s) are credited and that the original publication in this journal is cited, in accordance with accepted academic practice. No use, distribution or reproduction is permitted which does not comply with these terms.
*Correspondence: Marissa Faye Nuttall, TWFyaXNzYS5OdXR0YWxsQG5vYWEuZ292