- 1Department of Veterinary Medical Sciences, University of Bologna, Bologna, Italy
- 2Aquaculture Program, Institut de Recerca i Tecnologia Agroalimentàries, Centre de Sant Carles de la Ràpita, Sant Carles de la Ràpita, Spain
- 3Department of Cell Biology and Histology, University of Murcia, Murcia, Spain
To provide practical feeding management guidelines preceding a stressful episode during farming practices, European sea bass juveniles (initial weight: 72.3 g) were fed for 60-days different fish meal (FM) and fish oil (FO) dietary levels [high (30% FM, 15% FO, FM30/FO15), intermediate (20% FM, 7% FO, FM20/FO7), and low (10% FM, 3% FO, FM10/FO3)] in triplicate conditions. Fish were then fasted for 36 h and exposed to a 2-h acute crowding (80 kg m–3 biomass). Plasma biochemistry, skin mucus parameters and gene expression of stress and immune-related genes were performed before, at 2 and 24 h after crowding. At the end of the trial, the FM10/FO3 group showed lower final body weight, weight gain, and specific growth rate compared to the other treatments. Most of the plasma parameters were mainly affected by crowding condition rather than diet; however, after stress, lactate was higher in the FM30/FO15 group compared to the other treatments. Similarly, protease, antiprotease, peroxidase and lysozyme in skin mucus were mostly affected by crowding conditions, while fish fed FM10/FO3 displayed higher skin mucosal IgM and bactericidal activity against Vibrio anguillarum and V. harveyi. Most of the stress-related genes considered (hsp70 and gr-1 in the brain; hsp70, gr-1 and gr-2 in the head kidney), showed an overall expression pattern that increased over time after stress, in addition, hsp70 in the head kidney was also up-regulated in fish fed FM30/FO15 after stress. Higher plasmatic lactate together with the up-regulation of some stress-related transcripts suggest a higher reactivity to acute crowding of the stress-response mechanism in fish fed high FM and FO dietary levels. Otherwise, the higher skin mucosal IgM and bactericidal activity observed in fish fed FM10/FO3 dietary levels seems to indicate that acute crowding was able to activate a higher pro-inflammatory response in this treatment. Overall, the results of the present study seem to indicate that 10% FM and 3% FO dietary levels might affect stress and immune responses.
Introduction
Nowadays, fish farmers are urged to apply feeding management practices that implement feed formulations which address both optimal growth and fish welfare issues, especially for coping with exposures to various types of stress (Boyd et al., 2020; Reverter et al., 2021). Certainly, under current aquaculture intensive rearing conditions, fish are continuously exposed to many stressors both biotic and abiotic; among them, husbandry-related stressors such as handling, crowding, transportation, or confinement are the most important (Tort and Teles, 2012). When experienced, these stressors are well-known to cause a wide range of physiological alterations, thus, worsening overall fish performance (Barton et al., 2005; Bagni et al., 2007; Castro et al., 2018; Balasch and Tort, 2019). In recent years, for several fish species including Mediterranean carnivorous ones, significant amounts of dietary fishmeal (FM) and fish oil (FO) have been successfully replaced with alternative ingredients mainly derived from commodity agricultural crops (Kaushik et al., 2004; Sitjà-Bobadilla et al., 2005; Médale and Kaushik, 2009; Hardy, 2010; Sales and Glencross, 2011; Nasopoulou and Zabetakis, 2012; Bonvini et al., 2018c; Sutili et al., 2018; Parma et al., 2019; Kwasek et al., 2020). Among Mediterranean fish species, European sea bass (Dicentrarchus labrax) feed formulation has been evolving constantly, successfully reaching very low contents of FM and FO while providing for optimal rearing conditions; thus, demonstrating their potential for commercial use (Torrecillas et al., 2017a; Bonvini et al., 2018a; Parma et al., 2019). However, at the commercial farm scale this species is highly susceptible to stressful rearing-related problems such as netting, handling, sorting, transportation, and crowding giving rise to a wide range of negative physiological responses affecting their rearing performance and welfare (Gornati et al., 2004; Poltronieri et al., 2007; Fanouraki et al., 2011; Maricchiolo et al., 2011; Ellis et al., 2012; Tsalafouta et al., 2015; Samaras et al., 2017; Serradell et al., 2020). To counteract the negative impact of these stressors in fish, several feeding strategies have been developed to boost animal welfare, most of them including the utilization of functional feed additives (Volpatti et al., 2013; Gisbert et al., 2018; Serradell et al., 2020). However, only limited studies have investigated the interaction between very low dietary levels of FM and FO and animal welfare under suboptimal rearing conditions (Gisbert et al., 2018; Machado et al., 2019; Serradell et al., 2020). The effects of chronic stress on the immune system of fish are known to be clearly immunosuppressive; however, acute stress-induced immunomodulation is still poorly understood (Guo and Dixon, 2021). For instance, a recent study in European sea bass juveniles exposed to acute stress (netting and 1 min air exposure) and fed high levels of vegetable oils (VO) and carbohydrates resulted in depression of the immune response in both undisturbed and stressed fish (Machado et al., 2019). In order to find practical feeding management guidelines for fish farmers, the aim of this study was to evaluate the effect of different FM and FO dietary levels on growth performance, feed efficiency and, stress and immune-related biomarkers in European sea bass exposed to acute crowding. Fish responses to acute confinement were evaluated by analysis of different humoral immune biomarkers from skin mucus, plasma biochemistry, and gene expression of stress and immune-related genes in order to provide insight into the overall effects of experimental diets on fish welfare.
Materials and Methods
Experimental Diets
Three isonitrogenous (46% protein) and isolipidic (18% lipid) extruded diets (pellet size = 4.0 mm) were formulated to contain high, medium and low dietary levels of FM and FO (30% FM, 15% FO, FM30/FO15; 20% FM and 7% FO, FM20/FO7 and 10% FM and 3% FO, FM10/FO3, respectively). Diets were formulated with both, FM and a mixture of vegetable ingredients currently used for European sea bass aquafeeds (Parma et al., 2019), and were produced by SPAROS Lda (Portugal). Ingredients, proximate composition and fatty acid profile of the experimental diets are presented in Tables 1, 2.
Fish and Rearing Conditions
The experiment was carried out at the Laboratory of Aquaculture, Department of Veterinary Medical Sciences of the University of Bologna (Cesenatico, Italy). European sea bass (Dicentrarchus labrax) specimens were obtained from an Italian fish farm (Orbetello, GR) and adapted to the laboratory facilities for 1 week before the beginning of the trial. Afterward, 50 fish tank–1 (initial weight: 72.2 ± 1.3 g, mean ± standard deviation, SD) were randomly distributed in nine 800 L volume tanks. Each diet was randomly assigned and administered to triplicate tanks over 60 days. Tanks were provided with natural seawater and connected to a closed recirculation system (overall water volume: 13 m3). The rearing system consisted of a mechanical sand filter (PTK 1200, Astralpool, Barcelona, Spain), ultraviolet lights (SH-88, BLUGEO S.r.l., Parma, Italy) and a biofilter (PTK 1200, Astralpool, Barcelona, Spain). The water exchange rate within each tank was 100% every hour, while the overall water renewal amount in the system was 5% daily. During the trial, the temperature was kept at 23.0 ± 0.5°C and an artificial photoperiod of 12 h light and 12 h dark. The oxygen level was kept constant (8.0 ± 1.0 mg L–1) through a liquid oxygen system regulated by a software program (B&G Sinergia snc, Chioggia, Italy). Ammonia (total ammonia nitrogen ≤ 0.1 mg L–1) and nitrite (≤0.2 mg L–1) were daily monitored spectrophotometrically (Spectroquant Nova 60, Merck, Lab business, Darmstadt, Germany), while salinity (30 g L–1) was measured by a salt refractometer (106 ATC, Giorgio Bormac S.r.l., Carpi, Italy). Sodium bicarbonate was added on a daily basis to keep pH at 7.8–8.2. Fish were fed twice a day (8:30 and 16:30 h) for 6 days a week by automatic feeders to provide 10% overfeeding as reported by Bonvini et al. (2018a). Each meal lasted 1 h, after which the uneaten pellets of each tank were collected, dried overnight at 105°C, and weighed for further calculations of feed intake.
Sampling
At the beginning and at the end of the experiment, all the fish in each tank were anesthetized by MS222 at 100 mg L–1 and individually weighed (BW, g). Proximate composition of carcasses was determined at the beginning of the trial on a pooled sample of 10 fish and on a pooled sample of five fish per tank at the end of the trial. Furthermore, wet weight of body, viscera, liver, perivisceral fat and total length were individually recorded for five fish per tank to determine viscerosomatic (VSI), hepatosomatic (HSI) and mesenteric fat (MFI) indices, and condition factor (CF).
At the end of the 60-day period of feeding, animals were fasted for 36 h. Subsequently, a crowding stress challenge (80 kg m–3 biomass) was conducted by lowering the water level in tanks for 2 h. At the end of this time frame, the water level was brought back to the initial level, allowing the animals to recover from the stressful event for 24 h. Before (time 0, T0), at the end of the 2-h exposure (time 1, T1) and 24 h after confinement at high density (time 2, T2), eight fish per tank were euthanized with an overdose of MS222 (300 mg L–1) and sampled at each considered time point (T0, T1, and T2) in order to explore the capacity of the different diets to provide any advantage or disadvantage in front of the challenge test.
Skin mucus samples were collected from eight fish per tank according to Parma et al. (2020). Briefly, skin mucus was collected by gently scraping the dorsolateral surface of specimens using a cell scraper, taking care to avoid contamination with urinogenital and intestinal excretions. Collected mucus samples (3 mL each) were then stored at −80°C until analysis.
For the assessment of plasma biochemistry, blood from five fish per tank (n = 15 fish per diet treatment, same fish used for skin mucus samples) was collected from the caudal vein and placed in 4 mL heparin tubes (Vacutest Kima, Arzergrande, Italy). Samples were then centrifuged (3,000 × g, 10 min, 4°C) and plasma aliquots were stored at −80°C until analysis (Bonvini et al., 2018b).
Similarly, two fish per tank (n = 6 fish per diet treatment at T0, T1, and T2) were randomly sampled, euthanized with an overdose anesthetic as previously described, then head kidney (both sides) and brain (whole) were dissected to gain 100 mg of tissue in order to evaluate the impact of dietary treatments on genetic markers of stress-response in fish that had been submitted to crowding stress. The brain and head kidney samples were kept at 4°C overnight into RNA Later (Sigma-Aldrich), and then stored at −80°C until further analysis.
All experimental procedures were evaluated and approved by the Ethical-Scientific Committee for Animal Experimentation of the University of Bologna (protocol ID 942/2019) in accordance with European directive 2010/63/UE on the protection of animals used for scientific purposes.
Growth Performance, Feed Efficiency, and Body Condition Indices
In order to evaluate the effect of experimental diets, the following formulae were employed to calculate growth performance, somatic and nutritional index values:
Weight gain (WG, g) = BWf- BWi (where BWf and BWi represent the final and the initial body weight).
Specific growth rate (SGR,% day–1) = 100 * (ln BWf- ln BWi)/days
Feed intake (FI, g kg ΔBW–1 day–1) = ((1000*total feed ingestion)/(ΔBW))/days.
Feed conversion ratio (FCR) = FI/WG.
Condition factor (CF) = 100 * (BWf/total body length3).
Viscerosomatic index (VSI,%) = 100 * (viscera weight/BWf).
Hepatosomatic index (HSI,%) = 100 * (liver weight/BWf).
Mesenteric fat index (MFI,%) = 100 * (mesenteric fat weight/BWf).
Protein efficiency rate (PER) = (BWf – BWi)/total protein intake.
Gross protein efficiency (GPE,%) = 100 * [(% final body protein content * BWf) – (% initial body protein * BWi)]/total protein intake fish.
Lipid efficiency rate (LER) = (BWf – BWi)/total lipid intake.
Gross lipid efficiency (GLE,%) = 100 * [(% final body lipid content * BWi) – (% initial body lipid * BWi)]/total lipid intake fish.
Proximate Composition Analyses
Diets and whole bodies of fish were analyzed for proximate composition. Moisture content was obtained by weight loss after drying samples in a stove at 105°C overnight. Crude protein was determined as total nitrogen (N*6.25) using the Kjeldahl’s method according to the AOAC International (AOAC, 2010). Total lipids were determined according to Bligh and Dyer’s (1959) extraction method. Ash content was estimated by incineration in a muffle oven at 450°C overnight. Gross energy was determined by a calorimetric bomb (Adiabatic Calorimetric Bomb Parr 1261; PARR Instrument, IL, United States). Fatty acid analyses composition of diets was performed according to ISO16958:2015.
Stress and Metabolic Biomarkers in Plasma
Plasma samples (500 μL) were analyzed by automated analyzer (AU 480; 220 Olympus/Beckman Coulter, Brea, CA, United States) using specific methods (Olympus system 221 reagent, OSR) detecting the levels of glucose (GLU), triglycerides (TRIG), lactate, total protein, aspartate aminotransferase (AST), alanine transaminase (ALT), alkaline phosphatase (ALP), creatine kinase (CK), lactate dehydrogenase (LDH), calcium (Ca+2), inorganic phosphorus (P), potassium (K+) sodium (Na+), magnesium (Mg) according to Pelusio et al. (2021). In addition, plasma cortisol (CORT) concentration was determined using a chemiluminescence immunoassay (Immulite cortisol, Diagnostic Product Corporation, Los Angeles, CA, United States) using an automated analyzer (Immulite XP2000, Siemens) (Pelusio et al., 2021).
Humoral Immunity Markers in Skin Mucus
The peroxidase activity was measured by oxidation of 3,3′,5,5′-Tetramethylbenzidine (TMB) according to Quade and Roth (1997). Aliquots of 10 μL of mucus solution were diluted with Hanks’ buffer (HBSS) to a final volume of 50 μL in a flat-bottomed 96-well plate. As substrate, H2O2 was added, and the color change reaction was halted by adding 50 μL 2 M H2SO4. The optical density (OD) was read at λ = 450 nm in a plate reader (FLUOstar Omega, BMG Labtech, Germany). Samples without mucus solution were used as blanks. One unit was defined as the amount producing an absorbance change of 1, and the activity was expressed as U mg protein–1.
Protease activity was quantified using the azocasein hydrolysis assay according to the method described by Ross et al. (2000). Briefly, 100 μL of skin mucus were incubated with 100 μL of ammonium bicarbonate buffer and 125 μL of 0.7% azocasein (Sigma, United States) in sterile Eppendorf vials. The reaction was then halted by adding 250 μL of 4.6% trichloroacetic acid (TCA, Sigma, United States) and the OD was read at λ = 450 nm using a plate reader. Skin mucus was replaced by trypsin (5 mg mL–1, Sigma) for the positive controls or by ammonium bicarbonate buffer for the negative one. The activity for each sample was expressed as % protease activity in relation to the controls.
Antiprotease activity was determined by the capacity of skin mucus to inhibit trypsin activity (Hanif et al., 2004). Briefly, 100 μL of skin mucus were incubated (10 min, RT) with 10 μL of trypsin solution (5 mg mL–1, Sigma, United States). Subsequently, 100 μL of ammonium bicarbonate buffer and 125 μL of 0.7% azocasein (Sigma, United States) were added and mixtures incubated for 2 h at RT. Afterward, 250 μL of 4.6% trichloroacetic acid (TCA; Sigma, United States) were added and samples incubated for an additional 30 min. Later 100 μL of 0.5 N NaOH were added and the OD was read at λ = 450 nm using a plate reader. Buffer without sample or trypsin was used as positive controls and a combination of buffer and trypsin solution was used for the negative controls. The activity for each sample was expressed as% trypsin inhibition in relation to the controls.
Lysozyme activity was measured according to the turbidimetric method described by Parry et al. (1965). Volumes of 10 μL of skin mucus diluted 1:2 with 10 mM PBS, pH 6.2, were placed in a flat-bottomed 96-well plate. To each well, 200 μL of freeze-dried Micrococcus lysodeikticus in the above buffer (0.3 mg mL–1, Sigma) were added as lysozyme substrate. The reduction in absorbance at λ = 450 nm was measured. One unit of lysozyme activity was defined as a reduction in absorbance of 0.001 units min–1. The units of lysozyme present in skin mucus were obtained from a standard curve made with hen’s egg-white lysozyme (HEWL, Sigma), and the results were expressed as U mg protein–1.
Alkaline phosphatase activity was measured by incubating 100 μL of skin mucus with an equal volume of 4 mM p-nitrophenyl liquid phosphate (Sigma, United Kingdom) in 100 mM ammonium bicarbonate buffer containing 1 mM MgCl2 (pH 7.8, 30°C) (Ross et al., 2000). The OD was continuously measured at 1 min intervals over 3 h at 405 nm in a plate reader and one unit of activity was defined as the amount of enzyme required to release 1 mmol of p-nitrophenol product in 1 min. The activity was expressed as U mg protein–1.
Esterase activity was measured by incubating aliquots of 100 μL of skin mucus with an equal volume of 0.4 mM p-nitrophenylmyristate substrate (Sigma, United Kingdom) in 100 mM ammonium bicarbonate buffer containing 0.5% Triton X-100 (Sigma, United Kingdom) (pH 7.8, 30°C) in a flat-bottomed 96-well plate, as described by Ross et al. (2000). The OD and activity were determined and expressed as previously described for the alkaline phosphatase.
Total skin mucus immunoglobulin M (IgM) levels were analyzed by ELISA method as described by Hanif et al. (2004). Samples of 100 μL per well of skin mucus diluted 1:5 with 50 mM carbonate-bicarbonate buffer (35 mM NaHCO3 and 15 mM Na2CO3, pH 9.6), the proteins were adsorbed during an overnight incubation at 4°C and blocked for 2 h at room temperature with 200 μL blocking buffer containing 3% BSA in PBS-T, followed by three new rinses with PBS-T. Plates were then incubated (1 h with 100 μL per well) with mouse anti-European sea bass IgM monoclonal antibody (Aquatic Diagnostics Ltd., 1/100 in blocking buffer) and with 100 μL per well of secondary antibody anti-mouse IgG-HRP (1/1000 in blocking buffer, Sigma-Aldrich). After exhaustive rinsing with PBS-T the plates were developed using 100 μL of a 0.42 mM solution of 3,3,5,5-tetramethyl benzidine hydrochloride (TMB, Sigma-Aldrich). The reaction was allowed to proceed for 10 min and halted by the addition of 50 μL 2 M H2SO4 and the plates were then read at λ = 450 nm. Negative controls consisted of samples without skin mucus or without primary antibody, whose OD values were subtracted from each sample value.
Two bacteria, pathogenic for marine fish species (Vibrio harveyi and Vibrio anguillarum), were used in the bactericidal assays. Bacteria were cultured for 48 h at 25°C in Tryptic Soy Agar (TSA, Difco Laboratories), and then single colonies were inoculated in Tryptic Soy Broth (TSB, Difco Laboratories), both supplemented with NaCl to a final concentration of 1% (w/v), and grown with continuous shaking (100 rpm) for 24 h. Bactericidal activity was determined according to the method of Stevens et al. (1991). For this, aliquots of 100 μL of bacteria were added to wells of a flat-bottomed 96-well plate and incubated with the same volume of skin mucus. The absorbance of the dissolved formazan was measured at λ = 570 nm. Bactericidal activity was expressed as a percentage of non-viable bacteria (the difference between the absorbance of surviving bacteria in test samples compared with the absorbance of bacteria from positive controls).
Soluble total protein concentration in mucus samples was determined by the Bradford dye binding method, using bovine serum albumin (BSA, Sigma-Aldrich) as a standard (Bradford, 1976).
Gene Expression in Brain and Head-Kidney
RNA from tissues (50 mg per sample) was extracted using the RNeasy Mini Kit (Qiagen, Spain) following the instructions of the manufacturer. RNA concentration and quality were determined by spectrophotometry (λ = 260/280) (Nanodrop-2000® spectrophotometer; Thermo Scientific, United States) and denaturing electrophoresis in TAE agarose gel (1.5%) (Masek et al., 2005). For each tissue sample, ca. l μg of RNA was reverse transcribed into cDNA using Super-Script™ First-Strand Synthesis System for RT-PCR (Invitrogen™, Life Technologies, Warrington, United Kingdom) primed with oligo (dT)12–18 and random hexamers. Primers for quantitative PCR analyses were obtained for most genes by searching the literature for previously reported gene expression studies in this species (Table 3). When genes of interest were not previously published, an alignment of marine teleost homologs for the gene of interest obtained from GenBank, was made using BioEdit Sequence Alignment Editor ver 7.0.5.2 (Hall, 1999). Consensus primers designed from conserved regions identified in these alignments were used for D. labrax-specific qPCR assays (Table 2). Quantitative real-time polymerase chain reaction (qPCR) was performed using a C1000 Touch Thermal Cycler (BioRad, Spain). Beta actin (β-actin) was used as the reference gene. All cDNA samples were diluted 1:20 in molecular biology grade water. An aliquot of 5 μL from each diluted sample was pooled to provide a “calibrator sample” that was included as a calibrator reaction (β-actin) sample on each plate to correct for variation among experimental plates. A standard curve was prepared by amplification of a dilution series of this pooled cDNA for calculation of the efficiency (%E) for each assay to ensure that efficiency was close to 100%.
Quantitative PCR analyses for each gene were performed in triplicate in a total volume of 20 μL containing 2 μL cDNA, 20 μmol of each primer, 10 μL SYBR™ Green (Life Technologies, Warrington, United Kingdom) and 7 μL of sterile water. A negative control was included (NTC = no template control) for each set of reactions on each 96-well plate. All reactions were performed using the following conditions: 1 cycle at 95°C for 10 min; 40 cycles at 95°C for 15 s, Ta°C (primer-specific annealing temperature) for 30 s. A final melt curve stage completed the program, enabling confirmation of the amplification of a single product in each reaction. The final relative mRNA levels were then calculated following Pflaff’s method (Pfaffl, 2004).
Statistical Analysis
All data are presented as mean ± SD. A tank was used as the experimental unit for analyzing growth performance, whereas a pool of five sampled fish was considered the experimental unit for analyzing carcass composition and somatic indices. Individual fish were used for analyzing plasma biochemistry, skin mucus parameters and relative expression of stress and immune-related transcripts. At the end of the feeding trial with diets differing in the FM and FO levels (60 days), data of growth performance, nutritional indices and somatic indices were analyzed by a one-way analysis of variance (ANOVA) and in case of significance (P ≤ 0.05) Tukey’s post hoc test was performed. In order to provide the effect of diets on the basal status of skin mucus parameters, plasma and gene expression before the stress episode; results were analyzed by a two-tier statistical approach analyzing first the data at T0 by one way ANOVA and followed by a two-way ANOVA considering sampling points and diets as factors. When statistically significant differences were found among groups (P ≤ 0.05), Tukey’s post hoc test was performed to determine differences among experimental groups. Prior to ANOVA analyses, all data were checked for normality and homogeneity of variance by means of the Shapiro Wilk and Levene tests, respectively. All the statistical analyses were conducted using GraphPad Prism and Statistical Package for Social Science (SPSS) for Windows version 20.0.
Results
Key Performance Indicators and Body Proximate Composition
Results on growth performance and feeding efficiency parameters are summarized in Table 4. Significant differences were found in terms of BWf, WG, and SGR, while the lowest values were found in animals fed the FM10/FO7 diet. In particular, European sea bass fed FM30/FO15 and FM20/FO7 diets were 11.2 and 9.2% heavier than those from the FM10/FO3 dietary treatment.However, FCR, FI, and survival values displayed no significant differences among experimental groups.
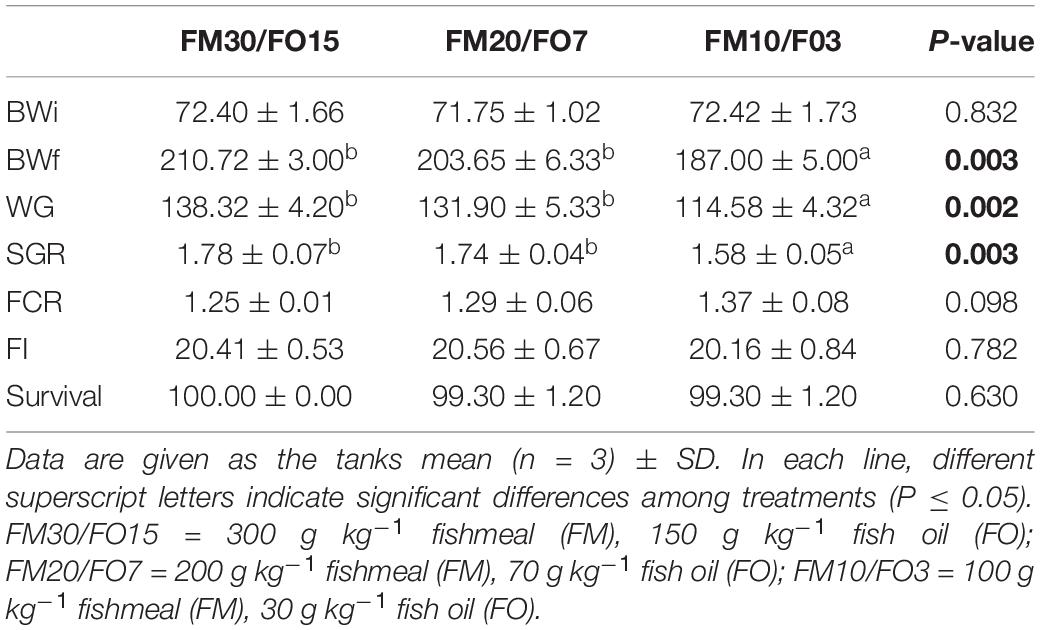
Table 4. Growth performance of European seabass fed experimental diets over 60 days before crowding stress exposure.
Results on proximate body composition and, nutritional and somatic indices are reported in Table 5. No significant differences were found on any of the analyzed parameters.
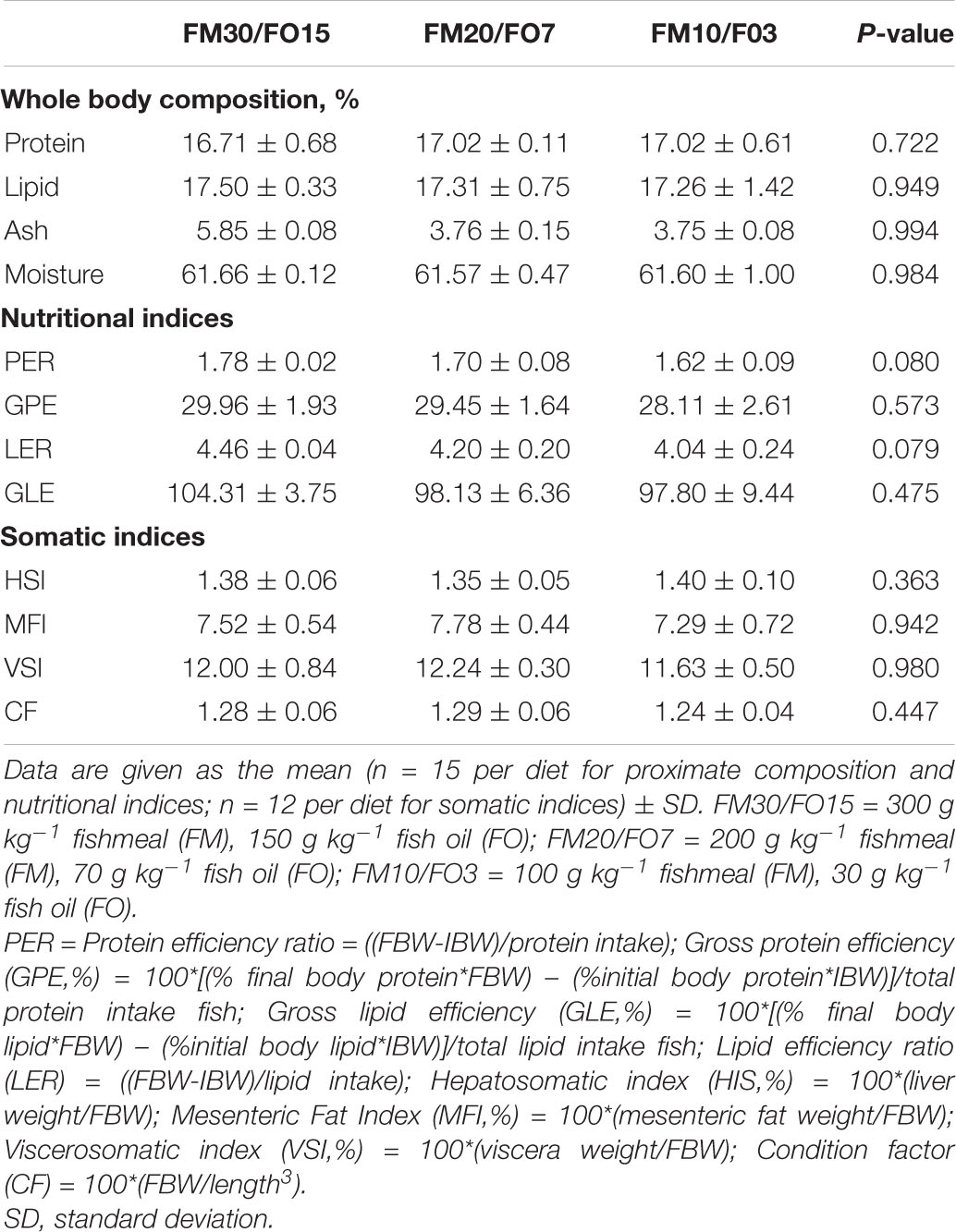
Table 5. Body composition and, nutritional and somatic indices of European seabass fed experimental diets over 60 days before crowding stress exposure.
Plasma Biochemistry
Plasma biochemistry results are summarized in Supplementary Table 1 and Table 6. At the end of the growth trial (T0) dietary treatments did not affect all the parameters analyzed except cortisol which was higher in FM30/FO15 than the other treatments (One-way ANOVA – Supplementary Table 1). Table 6 reports plasma results in relation to dietary treatments and time after stress (Two-way ANOVA). Glucose was affected by diet and time after stress where FM30/FO15 showed higher values than FM20/FO7 and values were higher at T1 compared to T0 and T2. Triglyceride were affected by time where T0 groups displayed higher values than those of T1 and T2. Similarly, plasma protein was affected by time with higher values found at T1 and T2 compared to T0. Concerning AST and ALP, no significant diet- nor time-dependant effects were found among experimental groups while a significant time effect was found in ALT, with higher values found in T0 than T2 groups. CK and LDH were affected by time with lower values found in T2 compared to T0. Ca2+ was affected by time where T1 and T2 showed higher values than T0. Both significant diet and time effects were found in P where FM30/FO15 and T0 displayed higher values than the other diets and time groups, respectively. K+ was affected by time with higher values at T0 compared to the other sampling times. Concerning Na+, significant diet, time and interaction effects were found and the combined effect of diet and time resulted in an increasing pattern from T0 to T1 only in FM10/F03 diets. In addition, at T1, FM10/FO3 displayed higher values than the other dietary treatments. Plasma Mg2+ levels were influenced by time; specifically, values were lower at T2 in comparison to T0 and T1. Cortisol level was affected by time; specifically, values were higher at T1 in comparison to T0 and T2. A significant diet and interaction effect were observed in lactate, in particular the lactate levels recorded at T1 were higher in the FM30/FO15 group in comparison to those observed in FM20/FO7 and FM10/FO3 at the same time point. In addition, at T0 FM30/FO15 displayed higher lactate values at T1 than the other time points, while in FM20/FO7 values were higher at T0 than T1. In FM10/FO3 no significant difference within time points were observed.
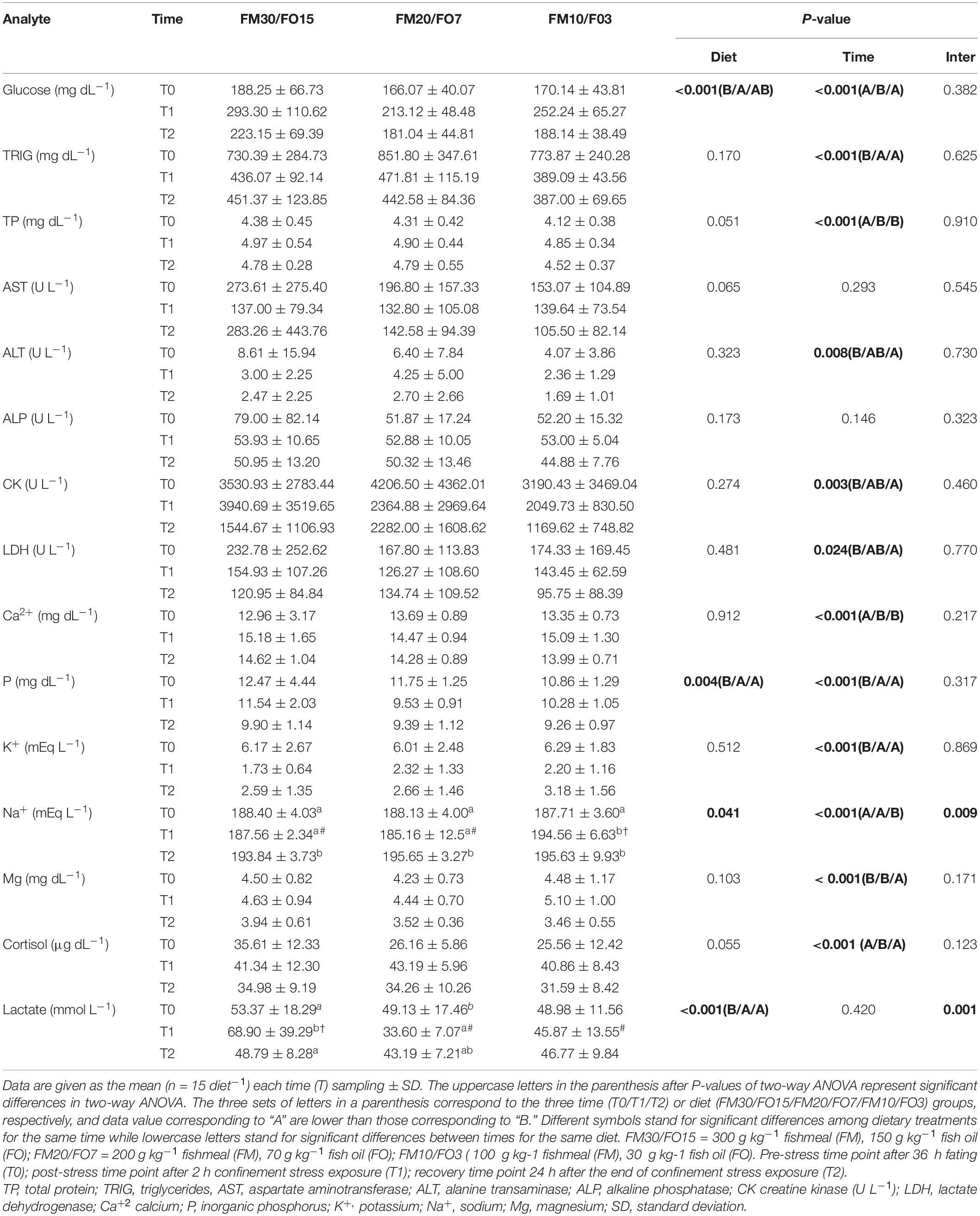
Table 6. Plasma biochemistry values for European seabass fed the experimental diets and exposed to crowding stress and recovery after small fasting period.
Humoral Immune Biomarkers in Skin Mucus
Results concerning humoral immune biomarkers in skin mucus are presented in Supplementary Figures 1A–I and in Figures 1A–I. At the end of the growth trial (T0) dietary treatments did not affect all the parameters analyzed except esterase which was lower in FM20/FO7 compared to the other treatments (One-way ANOVA – Supplementary Figure 1). In addition, bactericidal activities against V. anguillarum and V. harveyi were higher in FM10/FO3. Figure 1 reports humoral immune biomarkers results in relation to dietary treatments and time after stress (Two-way ANOVA). Protease activity was affected by time after stress displaying higher values in T0 than T1 and T2 (Figure 1A). Antiprotease activity was affected by time with significant diet × time effect which resulted to increasing values at T1 and T2 in FM10/FO3. In addition, at T0, FM20/FO7 was higher than FM10/FO3 (Figure 1B). Peroxidase activity was significantly affected by time and diet, showing higher values at T2 compared to T0 and T1 (Figure 1C). In addition, peroxidase activity was also lower in fish fed FM10/FO3 than FM30/FO15. Esterase activity was affected by time with higher values at T2 than T1 and T0 (Figure 1D). Alkaline phosphatase activity showed a diet, time and interaction effect with higher values at T2 in the FM30/FO15 and FM10/FO3 compared to the same dietary groups recorded at T0 and T1. In addition at T2, FM20/FO7 was lower than the other diets while FM10/FO3 was lower than FM30/FO15 (Figure 1E). Lysozyme was affected by time with general increasing values at T1 even if not specific differences were detected in the post hoc test (Figure 1F). IgM levels showed a diet, time and interaction effect; specifically, values increased in animals fed the FM10/FO3 diet and sampled at T1 and T2 compared to those sampled at T0 within the same treatment. In contrast, fish from the FM20/FO7 group were characterized by lower IgM values at T2 compared to T0, while those from the FM30/FO15 group showed lower values at T1 compared to T0 and T2 sampling points (Figure 1G). In addition, at T1 FM30/FO15 displayed lower values than FM20/FO7 and FM10/FO3, while at T2, FM10/FO3 showed higher values than the other diets and FM30/FO15 was higher than FM20/FO7.
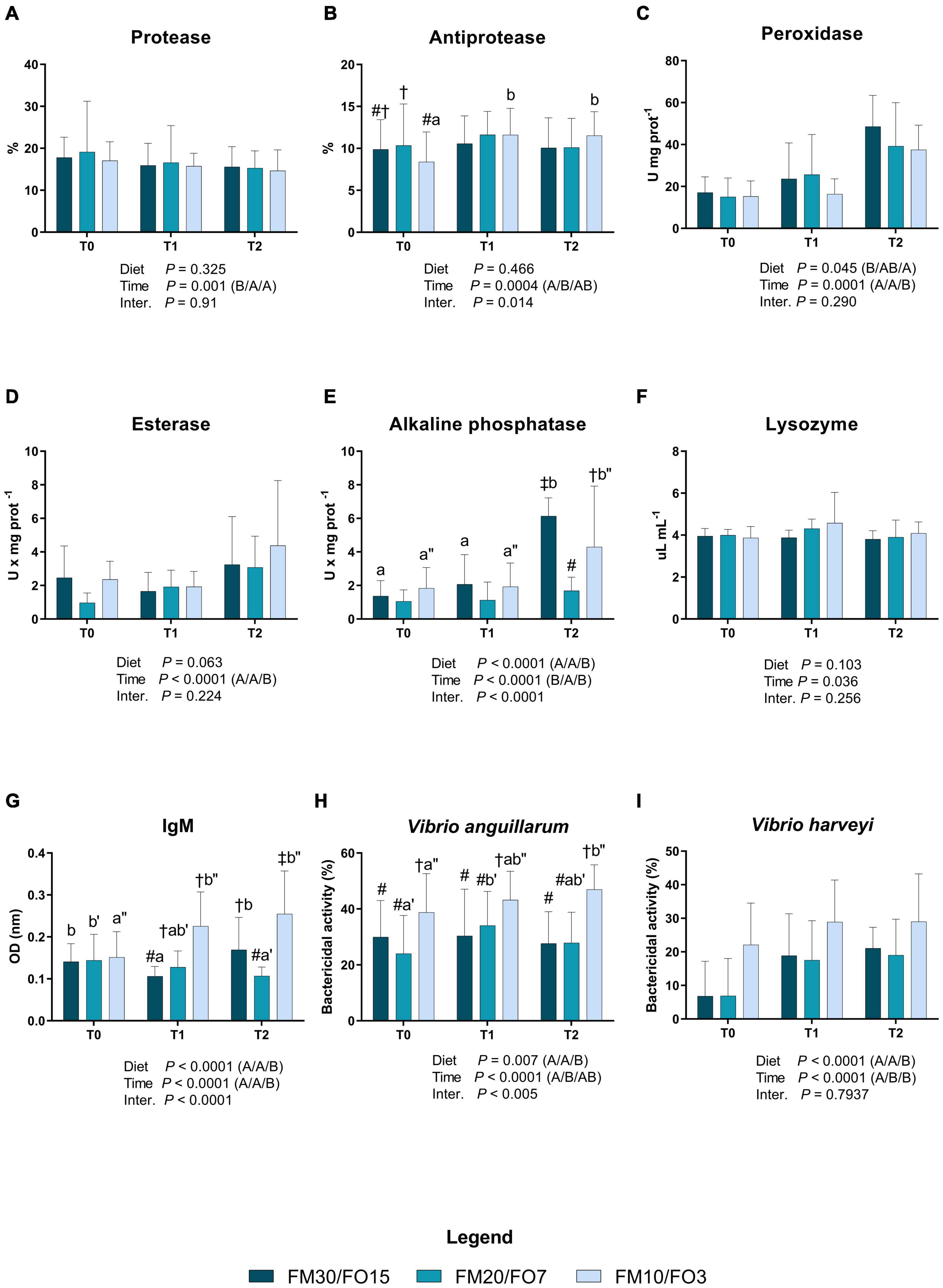
Figure 1. Humoral immune biomarkers in skin mucus of European sea bass fed the experimental diets and exposed to crowding stress (2 h T1) and recovery (24 h T2) after fasting period (36 h, T0). FM30/FO15 = 300 g kg– 1 fishmeal (FM), 150 g kg– 1 fish oil; (FO); FM20/FO7 = 200 g kg– 1 FM; 70 g kg– 1 FO; FM10/FO3 = 100 g kg– 1 FM; 30 g kg– 1 FO. Data represent the mean ± S.D. (N = 24). The uppercase letters in the parenthesis after P-values of two-way ANOVA represent significant differences in two-way ANOVA (P < 0.05). The three sets of letters in a parenthesis correspond to the three times (T0/T1/T2) or diets (FM30/FO15/FM20/FO7/FM10/FO3) groups, respectively, and data value corresponding to “A” are lower than those corresponding to “B.” Different symbols stand for significant differences among dietary treatments for the same time while lowercase letters (a b c, FM30/FO15; a’b’c’ FM20/FO7; a”b”c” FM10/FO3) stand for significant differences between times for the same diet. (A) Protease activity (%); (B) antiprotease activity (%); (C) peroxidase activity (U* mg prot– 1); (D) esterase activity (U* mg prot– 1); (E) alkaline phosphatase activity (U* mg prot– 1); (F) lysozyme activity (μL* mg prot– 1); (G) IgM (OD nm); (H) bactericidal activity (%) against Vibrio anguillarum; (I) bactericidal activity (%) against Vibrio harveyi.
Bactericidal activity against V. anguillarum was affected by time, diet and interaction; specifically, in fish fed the FM10/FO3 values were higher at T2 than T0 within the same dietary group, while in fish fed FM20/FO7 values were higher at T1 compared to T0. At each considered time point, FM10/FO3 showed higher values than the other dietary treatments.
Similarly, bactericidal activity against V. harveyi was also affected by time and diet with higher values at T1 and T2 than T0 and higher values reported in FM10/FO3 compared to the other treatments.
Gene Expression of Stress and Immune-Related Genes in Brain and Head-Kidney
Supplementary Figure 2 and Figure 2 show the relative expression values of the four target genes involved in the stress response measured in the brain and the head kidney.
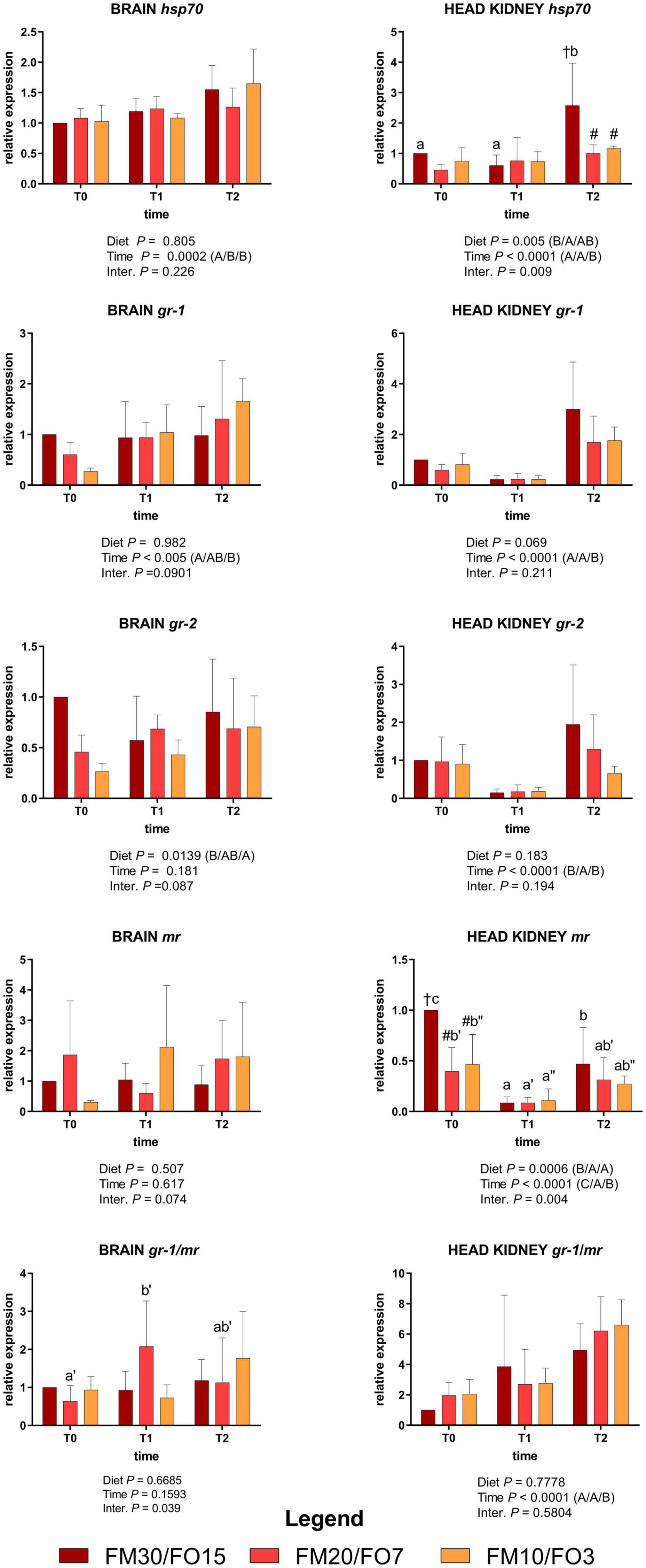
Figure 2. Relative expression of stress-related genes in brain and head kidney of European sea bass fed the experimental diets and exposed to crowding stress (2 h T1) and recovery (24 h T2) after fasting period (36 h, T0). FM30/FO15 = 300 g kg– 1 fishmeal (FM), 150 g kg– 1 fish oil; (FO); FM20/FO7 = 200 g kg– 1 FM; 70 g kg– 1 FO; FM10/FO3 = 100 g kg– 1 FM; 30 g kg– 1 FO. Data represent the mean ± S.D. (N = 6). The uppercase letters in the parenthesis after P-values of two-way ANOVA represent significant differences in two-way ANOVA (P < 0.05). The three sets of letters in a parenthesis correspond to the three times (T0/T1/T2) or diets (FM30/FO15/FM20/FO7/FM10/FO3) groups, respectively, and data value corresponding to “A” are lower than those corresponding to “B.” Different symbols stand for significant differences among dietary treatments for the same time while lowercase letters (a b c, FM30/FO15; a’b’c’ FM20/FO7; a”b”c” FM10/FO3) stand for significant differences between times for the same diet. Genes are reported as follows: gr-1, glucocorticoid receptor-1; gr-2 glucocorticoid receptor-2; mr, melanocortin receptor; hsp70, heat shock protein 70.
At the end of the growth trial (T0) brain gr-1 and gr-2 relative expression decreased at decreasing FMFO level, while in the head kidney hsp70 was lower in FM20/FO7 than FM30/FO15 and mr was lower in FM20/FO7 and FM10/FO3 compared to FM30/FO15 (One-way ANOVA – Supplementary Figure 2).
Figure 2 reports the same stress target genes in relation to dietary treatments and time after stress (Two-way ANOVA).
In the brain, hsp70 were affected by time displaying lower values at T0 than T1 and T2 while brain gr-1 was lower in T0 than T2. Brain gr-2 was affected by diet supported mainly by the higher values observed in FM30/FO15 than FM10/FO3. No significant time nor diet effects were reported for the relative expression of mr in the brain.
In the head kidney, hsp70 was up-regulated at T2 in fish fed the FM30/FO15 diet with higher values compared to the other time points within dietary treatment and in comparison to the other diets at T2. In addition, head kidney gr-1 and gr-2 were affected by time; specifically, gr-1 showed higher values at T2 than T1 and T0 while gr-2 showed higher values at T0 and T2 compared to T1. In the same tissue, mr was affected by time, diet and interaction; specifically, in FM30/FO15 transcripts decreased from T0 to T1, increasing again at T2 while in FM20/FO7 and FM10/FO3 values were higher at T0 than T1. At T0, FM30/FO15 displayed higher values than the other diets.
Concerning gr-1/mr a significant interaction effect was observed for brain where FM20/FO7 displayed higher values at T1 than T0. The gr-1/mr in the head kidney showed a time effect with values higher at T2 compared to the other time points.
The expression of the selected genes involved in the immune response for the brain and head kidney are depicted in Supplementary Figure 3 and Figure 3. At the end of the growth trial (T0) brain tgf was lower in FM20/FO7 compared to FM10/FO3 while brain tnf-α was lower in FM10/FO3 than FM30/FO15 and FM20/FO7. At the same time brain cox2 was lower in FM10/FO3 compared to FM30/FO15, while brain csp3 was lower in FM10/FO3 than FM20/FO7 (One-way ANOVA – Supplementary Figure 3). Concerning head kidney, at the end of the growth trial (T0) FM20/FO7 and FM10/FO3 showed tnf-α, cox2 and csp3 values lower than FM30/FO15, while lys and il10 values of FM20/FO7 were lower than FM30FO15 (One-way ANOVA – Supplementary Figure 3).
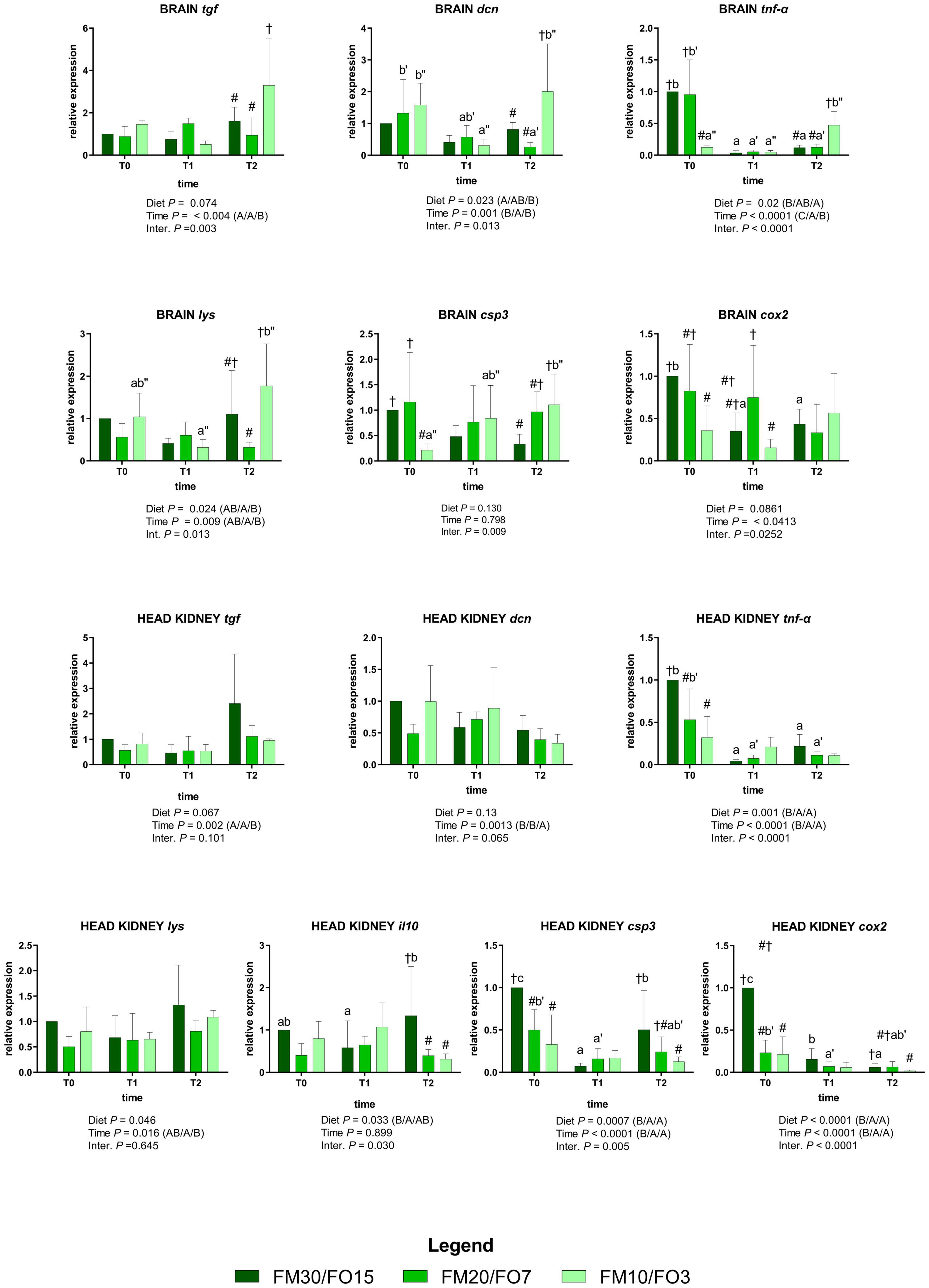
Figure 3. Relative expression of immune-related genes in brain and head kidney of European sea bass fed the experimental diets and exposed to crowding stress (2 h T1) and recovery (24 h T2) after fasting period (36 h, T0). FM30/FO15 = 300 g kg– 1 fishmeal (FM), 150 g kg– 1 fish oil; (FO); FM20/FO7 = 200 g kg– 1 FM; 70 g kg– 1 FO; FM10/FO3 = 100 g kg– 1 FM; 30 g kg– 1 FO. Data represent the mean ± S.D. (N = 6). The uppercase letters in the parenthesis after P-values of two-way ANOVA represent significant differences in two-way ANOVA (P < 0.05). The three sets of letters in a parenthesis correspond to the three times (T0/T1/T2) or diets (FM30/FO15/FM20/FO7/FM10/FO3) groups, respectively, and data value corresponding to “A” are lower than those corresponding to “B.” Different symbols stand for significant differences among dietary treatments for the same time while lowercase letters (a b c, FM30/FO15; a’b’c’ FM20/FO7; a”b”c” FM10/FO3) stand for significant differences between times for the same diet. Genes are reported as follows: csp3, caspase 3; hsp70, heat shock protein 70; tgf, transforming growth factor; tnf-α, tumor necrosis factor α; il10, interleukin 10; dcn, dicentrarcin, cox2, cyclooxygenase 2, lys, lysozyme.
Figure 3 reports the same immune response genes in relation to dietary treatments and time after stress (Two-way ANOVA).
In the brain, tgf was affected by time and interaction; specifically, values were up-regulated at T2 only in fish fed FM10/FO3. A similar trend occurred for dcn, where the relative expression in fish fed FM10/FO3 was higher than FM20/FO7 and FM30/FO15 at T2 and higher in comparison to the same dietary treatment at T1. In addition, values in FM20/FO7 were lower at T2 compared to the values from the same treatment recorded in T1. The relative expression of tnf-α was affected by time, diet and interaction; specifically, values decreased at T1 and T2 only in FM30/FO15 and FM20/FO7, while values increased at T2 compared to T1 in FM10/FO3. In addition, at T0 values were lower in FM10/FO3 than the other dietary treatments while the same diet displayed higher values at T2 than the other dietary treatments.
The expression of lys was affected by diet, time and interaction; specifically, values were higher in FM10/FO3 at T2 compared to fish fed the FM20/FO7 diet and compared to the same dietary treatment at T1.
The relative expression of csp3 displayed a significant diet x time interaction where fish from the FM10/FO3 group displayed an increasing trend from T0 to T2 sampling points. In addition, at T0 values were lower in FM10/FO3 than the other diets while at T2 the same diet displayed higher values than FM30/FO15. Brain cox2 reported significant time and interaction effects; specifically, values within FM30/FO15 were higher at T0 compared to T1 and T2. Data at T0 reported values in FM30/FO15 higher than FM10/FO3 while at T1 FM20/FO7 values were higher than FM10/FO3.
Regarding the assessment of gene expression patterns in the head kidney, tgf was affected by time with higher values at T2, than T1 and T0 while dcn displayed lower values in T2 than T0 and T1. The relative expression of tnf-α showed significant diet, time and interaction effect; specifically, values in FM30FO15 and FM20/FO7 were higher at T0 than T1 and T2, while at T0 FM30/FO15 values were higher the other diets.
Head kidney lys gene expression showed higher values at T2 than T1. The pro-inflammatory cytokine il10 displayed significant diet x time interaction effect with higher values in fish fed FM30/FO15 diet at T2 compared to the same diet at T1, while at T2 FM30/FO15 values were higher compared to the other dietary treatments. Caspase 3 displayed a significant diet, time and interaction effects. In particular FM30/FO15 displayed higher values at T0 compared to the same diet at T1 and T2, while the same diet was higher at T2 than T1. In addition, csp3 values at T0 were higher in FM30/FO15 than the other diets, while at T2 FM30/FO15 values were higher than FM10/FO3. The relative expression of cox2 was higher in FM30/FO15 group at T0 compared to the same treatment at T1 and T2, while FM20/FO7 at T0 was higher than T1 and T2. At T0 cox2 values were higher in FM30/FO15 than the other treatments, while at T2 FM30/FO15 values were higher than FM10/FO3.
Discussion
Key Performance Indicators and Body Proximate Composition
Recently, several studies have explored the feasibility of using very low FM and FO diets in European sea bass, as their effects on fish performance, gut morphology and microbiome, plasma biochemistry and filet quality have been described (Torrecillas et al., 2017b; Bonvini et al., 2018c; Parma et al., 2019; Henry et al., 2020). Although it has been shown that the FM and FO reduction may affect stress resistance, cellular immunity and oxidative status in different marine fish species (Sitjà-Bobadilla et al., 2005; Montero and Izquierdo, 2010; Geay et al., 2011; Gisbert et al., 2018), this topic still deserves further attention especially regarding this species, as it is highly susceptible to common stressors under intensive farming conditions.
In the present study, despite the absence of significant differences on feed intake and FCR values among diets, fish from the FM10/FO3 group showed a tendency for higher FCR; thus, leading to a negative outcome on BWf and SGR, values that were 11.3% lower than the FM30/FO15 group individuals. These findings are in contrast with those previously reported by Parma et al. (2019), where juveniles (22.8–68.2 g in BW) fed 10% FM as their sole animal protein source showed equal growth, feed and protein utilization in comparison to a control containing 20% FM. These differences could be related to the different feed formulation (i.e., lower FO inclusion) in the present trial. Similar results were reported also by Coutinho et al. (2017) where juveniles (7–28 g in BW) fed diets containing 15% FO and 8 and 2% (on dry weight basis) of FM and soluble fish protein concentrate, respectively. On the other hand, Bonvini et al. (2018a) reported a trend toward lower growth performance and protein efficiency at higher FM replacement levels in 70–200 g European sea bass when fed a diet containing 10% FM compared to their congeners fed diets containing 20 and 30% FM.
In FM10/FO3 the reduced level of marine ingredients with a consequence reduction of LC-PUFA could have affected growth performance as previously described in this species where the supplementation of n-3 and n-6 LC-PUFA in fish fed free FM and FO diet led to increased growth (Torrecillas et al., 2017a, 2018).
Concerning body composition and nutritional uptake, all treatments were found to be comparable under the present experimental conditions, results which agreed with those previously reported by Bonvini et al. (2018a) and Parma et al. (2019).
Plasma Biochemistry
Studying a farmed fish species blood biochemistry profile can be a valuable tool to assess nutritional and health status (Peres et al., 2014; Bonvini et al., 2015). Overall, the present results at T0 were within the range of normal values reported for this species under proper nutritional and non-stressing conditions (Bonvini et al., 2018b). Furthermore, different FM/FO dietary levels did not affect the basal status before stress even if cortisol level in FM30/FO15 was higher than the other treatments.
Data on plasma cortisol coupled with glucose levels is one of the first and main metabolites being released into the blood stream as a marker to acute stress response (Barton, 2002). European sea bass has been well characterized to have high basal and post-stress plasma cortisol concentrations (Fanouraki et al., 2011; Maricchiolo et al., 2011; Ellis et al., 2012) after even the minimum ordinary handling that occurs in fish farms (Tsalafouta et al., 2015; Samaras et al., 2017). In accordance with Di Marco et al. (2008), in our study the cortisol level peaked after stress exposure with a tendency to return to values similar to those reported in the pre-stress condition.
Plasma lactate values reflect metabolic and hormonal responses and can be considered an indicator of secondary stress responses (Saccol et al., 2018). The observed higher plasma lactate after stress exposure only in fish fed the FM30/FO15 diet may indicate a stronger response to crowding by increasing anaerobic muscle activity, as also reported in other fish species exposed to stressors such as chasing, netting, and air exposure (Ruane et al., 2002; Fanouraki et al., 2007).
Under current experimental conditions, confinement stress induced a significant increase of plasma glucose, which tended to return to initial basal values after a recovery time of 24 h. Notably, glucose was found to be slightly higher in groups fed FM30/FO15 at 2 h (T1) after confinement stress. Similar trends in plasmatic glucose levels and recovery time have also been reported in European sea bass exposed to 100 kg/m3 for 15 min (Di Marco et al., 2008). Basal TRIG levels before stress are within the range of values reported by Peres et al. (2014) on this species; however, they were lower than those reported by Bonvini et al. (2018a,b). The different basal levels among these studies might be attributed to different dietary formulations regarding lipid sources as well as to different post-prandial time at which analyses were conducted. In this work, TRIG levels decreased after 2 h post-stress exposure in all the treatments. A similar decrease was reported in European sea bass after 48–72 h post-stress challenge and in animals exposed to starvation (Pérez-Jiménez et al., 2007; Di Marco et al., 2008; Peres et al., 2014). The authors suggested that this decrease is probably due to its use as an energetic substrate and to the reduction in lipogenesis, which may have contributed to the maintenance of plasma glucose levels after an acute stress event (Pérez-Jiménez et al., 2007). Total protein level is a good potential indicator to predict the nutritional status of fish, since it is usually very stable in well-nourished animals. Under present experimental conditions, total protein increased after stress in all treatments. In contrast to our results, previous studies evidenced a plasma protein decline under fasting or stress conditions as a consequence of amino acid oxidation or peripheral proteolysis (Mommsen et al., 1999; Di Marco et al., 2008; Coeurdacier et al., 2011; Peres et al., 2014). However, Montero et al. (1999), described an increase of cortisol, glucose, and plasma protein in gilthead sea bream held at high stocking density as a strategy for increasing oxygen-carrying capacity of blood during periods of high energy demand. Such differences between studies may be related to the duration and intensity of the stressor, which would affect host metabolism and plasmatic protein levels.
Non-specific enzymes ALT, ALP, LDH, and CK are plasma indicators that may provide important information on fish health status, whose elevated levels in blood may indicate tissue damage in many organs including liver, muscle, spleen and kidney (Peres et al., 2013; Guardiola et al., 2018; Parma et al., 2020). Under current experimental conditions, ALT levels dropped after stress exposure and also continued to decrease after recovery time in all the treatments. An increasing level of plasma ALT may imply liver damage and less efficient absorption and transportation of lipids and carbohydrates from the intestine as described by other authors (Peres et al., 2014; Bonvini et al., 2018b), while ALT reduction may also be associated with changes in amino acid metabolism, since ALT degrades alanine for cellular energetic purposes.
The enzyme CK is particularly active in heart and skeletal muscle, while LDH converts lactate to pyruvate in cells; and their activity was described as being lowered by 30 and 50% respectively after 1 week fasting in European sea bass (Peres et al., 2014). Accordingly, CK and LDH concentration were found to be reduced at 24 h post-stress in a similar manner for all the treatments under current experimental conditions. Furthermore, plasma electrolytes are indicators of the secondary phase of stress response in fish, providing an indirect indication of altered plasma cortisol levels (Peres et al., 2014). Although fish may absorb some inorganic nutrients from the aquatic environment, when starvation or elevated stocking density occurs, a significant reduction in plasma phosphorus and calcium levels take place, confirming their importance as a general indicator of stress in fish (Lall, 2002; Hrubec and Smith, 2010; Guardiola et al., 2018). In this study, plasmatic calcium levels tended to increase after confinement stress and return to basal values at 24 h after stress in all dietary groups. Contrariwise, phosphorus concentration continued to decrease at T2, with generally lower values in animals fed FM20/FO7 and FM10/FO3 diets. This is consistent with a calcium and phosphorus drop and eventual resilience after starvation or stress described for this species (d’Orbcastel et al., 2010; Peres et al., 2014). Other plasma electrolytes such as potassium, sodium and magnesium alterations may be linked to acid-base balance and ions-transport disturbance (Roque et al., 2010; Bonvini et al., 2018b), which in the present study were found to tend to return to the initial values after the recovery period. However, at 2 h after stress only fish fed FM10/FO3 were characterized by an increase in the level of plasma sodium, finding which may be related to the reduced dietary LC-PUFA such as arachidonic acid which was able to attenuate the stressor-induced increase in plasma osmolality induced by acute crowding in gilthead sea bream (Van Anholt et al., 2004).
Humoral Immune Biomarkers in Skin Mucus
The study of the innate immune parameters of skin mucus (lysozyme, proteases, anti-proteases, peroxidase, esterase, alkaline phosphatase, and bactericidal activities) is of great importance in the evaluation of mucosal immunity of fish as this is the first line of the host’s defense. Their evaluation has been studied in relation to pathogens, environmental stress, feeding conditions, contaminants, crowding or handling in several fish species. However, data concerning the specific response of mucosal components to different dietary formulations and stressors such as crowding or handling in European sea bass are still scarce (Guardiola et al., 2014b,2016; Sanahuja et al., 2019).
In the present study, acute crowding slightly reduced protease activity in the skin mucus with a similar response for all the dietary treatments. In contrast, a different response of skin mucus protease activity to acute crowding was observed in gilthead sea bream, which was characterized by a decrease and increase after 2 and 24 h stress exposure, respectively. In addition, protease activity in gilthead sea bream was reduced when fish were fed low fish meal content (10% FM and 3% FO vs. 30 FM%, 15% FO), while chronic high density rearing conditions did not affect its activity (Parma et al., 2020). Antiprotease activity was significantly affected by crowding condition in fish fed the FM10/FO3 diet, which resulted in increasing values at 2 and 24 h after stress. In contrast, antiprotease activity was not affected by acute crowding in gilthead sea bream and, in agreement with our findings, antiprotease and protease were negatively correlated as a physiological response to compensate for the variations in protease activity (Guardiola et al., 2016). Peroxidase activity peaked at 24 h after stress for all the treatments. No data on skin mucus peroxidase are available for European sea bass exposed to acute crowding; however, in gilthead sea bream a similar response to crowding was observed with increasing values obtained at 48 h after stress. These differences between species could be attributed to species-specific factors, fish health status or exposure time to stress (Guardiola et al., 2014a; Sanahuja et al., 2019). In addition, in the present study a lower response of peroxidase activity was observed in fish fed the FM10/FO3 diet in comparison to those animals from the FM30/FO15 group, data that may indicate that these low FM/FO levels may reduce the specific action of this skin mucus component. Esterase activity in fish skin mucus has been previously studied mostly in terms of defensive activity against pathogens or in response to environmental chemical compounds, although their role is still not fully understood (Guardiola et al., 2014a; Sanahuja et al., 2019). No data are reported on esterase activity in fish skin mucus in response to acute crowding; however, our results indicated a positive response to stress of this mucus component in all dietary groups. Interestingly, in olive flounder (Paralichthys olivaceus) esterase activity of skin mucus was negatively correlated with protease activity. A similar trend occurred in the present trial, supporting the hypothesis that these enzymes may have a direct or indirect role in immune function either by acting alone or in association with other biologically active substances present in the skin mucus (Jung et al., 2012). Alkaline phosphatase is an antibacterial agent in mucus due to its hydrolytic activity, and increases in fish after physical or chemical stress, including skin regeneration (Sheikhzadeh et al., 2012a,b). In our study, ALP peaked at 24 h post-stress with a similar time-related effect as that observed for peroxidase and esterase, indicating possible synergistic effects of these three parameters in response to stress (Jung et al., 2012). Increased activity of ALP was also demonstrated in tiger barb (Puntius tetrazona) mucus during crowding stress as response to the increase in mucus secretion or improved mucosal immune responses (Hoseinifar et al., 2014; Roosta and Hoseinifar, 2016). Lysozyme activity was marginally affected by crowding conditions with an increasing level at 2 h post stress. Recent findings showed that acute crowding conditions in Atlantic salmon (Salmo salar) induced an increase in lysozyme activity of skin mucus only at 1 h post-stress, while it returned to basal values at 3 h after stress (Djordjevic et al., 2021). Conversely, high rearing density during 98 and 120 days in gilthead sea bream and turbot (Scophthalmus maximus), respectively, reduced skin mucus lysozyme activity as a possible immunodepressive effect due to chronic stress caused by high stocking density (Jia et al., 2016).
In addition, the bactericidal activity of IgM production in skin mucus reached a relevant post-stress peak mainly in fish fed the FM10/FO3 diet, which displayed higher values in comparison to other treatments. Our values were higher than those reported for skin mucus IgM in gilthead sea bream after crowding stress (transfer from 10 to 50 kg m–3 over 48 h) (Guardiola et al., 2016). The crowding stress employed in the present study seemed sufficient to activate a further excretion of IgM in fish fed the FM10/FO3 diet. Such a response may be interpreted as a mechanism to avoid possible invasion by pathogens when animals are highly challenged, subject to frequent surface abrasion, or vulnerable to infections. A further reinforcement for the idea of there being an enhanced humoral response in low FM/FO diet was the more elevated in vitro bactericidal activity against V. anguillarum and V. harveyi observed in fish fed the FM10/FO3 diet. These results may be the consequence of the sum of many intrinsic antimicrobial factors such as skin antimicrobial peptides and other innate immune components to fight pathogens. In particular, recent findings have shown the importance of LC-PUFA fractions in the composition of the skin mucus of gilthead seabream in relation to different FMFO dietary level, highlighting the needs for further studies to better understand the role of feed composition on skin mucus protective functions (Torrecillas et al., 2019).
Gene Expression of Stress and Immune-Related Genes in Brain and Head-Kidney
Glucocorticoid (gr-1, gr-2) and mineral corticoid receptors (mr) are receptors for cortisol found in many tissues including brain and immune cells, which are related to the regulatory role of cortisol in immune defense such as the secretion of pro- and anti-inflammatory cytokines (Reyes-López et al., 2018). In addition, hsp70 is responsible for repairing and preventing cellular damage from stress-induced protein denaturation and is considered an important indicator for stress in many species including European sea bass (Islam et al., 2021). In the present study, most of the stress-related genes considered, specifically hsp70 and gr-1, in the brain, and hsp70, gr-1, and gr-2 in head kidney, showed an overall time-dependent increasing pattern in expression after stress, findings which are in line with a positive response of the marker genes under consideration to crowding and handling stress in European sea bass and other fish species (Eissa and Wang, 2016).
Additionally, concerning gr-1 and gr-2, it was previously explained that different expression patterns among gr-1 and mr function as differential feed-forward and feed-back mechanisms, respectively, of cortisol on these receptor targets (Samaras et al., 2018). The gr1/mr ratio has been considered an appropriate indicator of stress load in fish species including European sea bass, as also reported in mammalian vertebrates (De Kloet et al., 2005, 2008; Samaras et al., 2018). In the present study, the gr1/mr ratio observed in the kidney increased after stress as result of the increasing, decreasing patterns of expression for gr-1 and mr, respectively. Interestingly, these genes were similarly expressed in the brain of European sea bass exposed to a combination of stresses including confinement, chasing and air exposure (Samaras et al., 2018).
Although not all the transcripts analyzed in the brain and head kidney showed a clear dietary effect, data reported for hsp70, gr1, gr2 in the head kidney seemed to indicate a more acute response to crowding stress for fish fed the FM30/FO15 diet compared to lower FM/FO levels. In general, these results are in agreement with previous findings in European sea bass fed high levels of VOs, since dietary lipids can alter the ability of fish to cope with different types of stressors (Montero et al., 2003; Machado et al., 2019). At the biochemical level, these data are consistent with the higher glucose and lactate plasma level found in FM30/FO15, while no evident relation with plasma cortisol level was found. The absence of a direct relation between cortisol plasma level and the gene expression of glucocorticoid receptors was also previously observed in this species (Pavlidis et al., 2011; Reyes-López et al., 2018). In addition, the effect of the diet on the expression of stress-related genes was less evident in the brain than in the head kidney, possibly indicating a difference in the degree to which there is involvement of the diet in different target tissues.
While fish fed the high FM/FO (FM30/FO15) diet showed a higher response to stress, a low FM diet (FM10/FO3) resulted in a higher up-regulation of several immune-related genes at 24 h post stress, including dcn, and lys in the brain. These data were in agreement with the higher level of IgM and bactericidal activity against V. anguillarum and V. harveyi observed in skin mucus from animals fed the FM10/FO3 diet at 24-h post-stress; collectively these data all indicate a stronger activation of the immune response in fish fed this diet. Several antimicrobial molecules including lysozyme and dicentracin are known to play a key role in the innate immune response as they possess powerful microbiocidal activity against a wide range of pathogens. In European sea bass particularly, it has been suggested that dicentracin is involved in the antimicrobial function against bacteria such as V. anguillarum and Photobacterium damselae and its expression was up-regulated in head kidney at 5h post-infection with nodavirus (Mosca et al., 2014; Meloni et al., 2015; Valero et al., 2015, 2020). The higher pro-inflammatory activity observed at mucosal immune effectors (IgM and bactericidal activity) and gene expression levels (lysozyme and dicentracin) in fish from the FM10/FO3 group could also be related to the lower stress response observed after crowding, as evidenced by the lower lactate, glucose and gene expression of stress-related genes (hsp70, gr-1, gr-2) obtained in fish from this treatment. It is well documented that stress and immune response are closely connected due to the existing crosstalk between these two systems, since a stressor induces alterations in immune response. For instance, in fish species the inhibition of the pro-inflammatory response related to high cortisol production is well known (Castillo et al., 2009; Reyes-López et al., 2018). However, in the head kidney a different pattern of expression of immune-related genes compared to the brain was observed: in particular tgf and il10 were up-regulated in fish fed the FM30/FO15 diet compared to the other treatments. Both of these cytokines have been shown to have significant autocrine activities for normal kidney function in mammals (Sinuani et al., 2013). Some types of nephropathy in mammals have been shown to benefit from increased expression of il10. Therefore, the up-regulation of anti-inflammatory cytokines such as il10 and tgf may support the beneficial dietary effect of high FM/FO levels enhancing anti-inflammatory response after exposure to stress (Busti et al., 2020).
Conclusion
In conclusion, 10% FM, 3% FO dietary inclusion levels showed a moderate reduction in growth performance, an effect that seemed to be related to the higher FCR reported in fish fed this diet. Although most of the plasma and skin mucus parameters analyzed were not affected by dietary treatments before stress, acute crowding induced different dietary-mediated responses. Specifically, a higher level of plasma lactate together with the up-regulation of some stress-related transcripts (hsp70, gr-2) suggests a higher reactivity to acute crowding of the stress-response mechanism, but a lower inflammatory response, as evidenced by higher expression of the anti-inflammatory tnf-α and il10 genes in fish fed high FM and FO dietary levels (FM30/FO15). Otherwise, the higher IgM levels and bactericidal activity observed in skin mucus of fish fed the FM10/FO3 diet seems to indicate that acute crowding was able to activate a higher pro-inflammatory response in this treatment. The results of the present study seem to indicate that the low FM/FO dietary level employed could affect stress and immune response. Repeated or chronic exposure to stressors would be needed in order to further evaluate the dietary effects on fish welfare.
Data Availability Statement
The original contributions presented in the study are included in the article/Supplementary Material, further inquiries can be directed to the corresponding author/s.
Ethics Statement
The animal study was reviewed and approved by Ethical-Scientific Committee for Animal Experimentation of the University of Bologna (protocol ID 942/2019) in accordance with European directive 2010/63/UE on the protection of animals used for scientific purposes.
Author Contributions
AB, NFP, LP, and PPG conceived and designed the experiment. NFP, AB, and LP wrote the first draft of the manuscript. NFP and LP carried out fish maintenance and sample collection. EG and KBA carried out the gene expression analysis and data processing. MAE carried out the skin mucus analysis and data processing. FD and MCS carried out plasma biochemistry analysis. All authors reviewed, improved the writing and approved the final manuscript.
Funding
This research was undertaken under the MedAID (Mediterranean Aquaculture Integrated Development) project, which has received funding from the European Union’s Horizon 2020 Research and Innovation Programme, Call H2020-SFS-23-2016, grant agreement no. 727315 (http://www.medaid-h2020.eu/).
Conflict of Interest
The authors declare that the research was conducted in the absence of any commercial or financial relationships that could be construed as a potential conflict of interest.
Publisher’s Note
All claims expressed in this article are solely those of the authors and do not necessarily represent those of their affiliated organizations, or those of the publisher, the editors and the reviewers. Any product that may be evaluated in this article, or claim that may be made by its manufacturer, is not guaranteed or endorsed by the publisher.
Acknowledgments
The authors would like to thank Gillian Forlivesi Heywood for English language editing and Antonina De Marco for assistance in fish maintenance during her master thesis degree accomplishment.
Supplementary Material
The Supplementary Material for this article can be found online at: https://www.frontiersin.org/articles/10.3389/fmars.2021.779053/full#supplementary-material
References
AOAC (2010). Officials Methods of Analysis, 17th Edn. Washington, DC: Association of Official Analytical Chemists.
Bagni, M., Civitareale, C., Priori, A., and Ballerini, A. (2007). Pre-slaughter crowding stress and killing procedures affecting quality and welfare in sea bass (Dicentrarchus labrax) and sea bream (Sparus aurata). Aquaculture 263, 52–60. doi: 10.1016/j.aquaculture.2006.07.049
Balasch, J. C., and Tort, L. (2019). Netting the stress responses in fish. Front. Endocrinol. 10:62. doi: 10.3389/fendo.2019.00062
Barton, B. A. (2002). Stress in fishes: a diversity of responses with particular reference to changes in circulating corticosteroids. Integr. Compar. Biol. 42, 517–525. doi: 10.1093/icb/42.3.517
Barton, B. A., Ribas, L., Acerete, L., and Tort, L. (2005). Effects of chronic confinement on physiological responses of juvenile gilthead sea bream, Sparus aurata L., to acute handling. Aquac. Res. 36, 172–179. doi: 10.1111/j.1365-2109.2004.01202.x
Bligh, E. G., and Dyer, W. J. (1959). A rapid method of total lipid extraction and purification. Can. J. Biochem. Physiol. 37, 911–917. doi: 10.1139/o59-099
Bonvini, E., Parma, L., Badiani, A., Fontanillas, R., Gatta, P. P., Sirri, F., et al. (2018c). Integrated study on production performance and quality traits of European sea bass (Dicentrarchus labrax) fed high plant protein diets. Aquaculture 484, 126–132. doi: 10.1016/J.aquaculture.2017.10.041
Bonvini, E., Bonaldo, A., Mandrioli, L., Sirri, R., Dondi, F., Bianco, C., et al. (2018a). Effects of feeding low fishmeal diets with increasing soybean meal levels on growth, gut histology and plasma biochemistry of sea bass. Animal 12, 923–930. doi: 10.1017/S1751731117002683
Bonvini, E., Bonaldo, A., Parma, L., Mandrioli, L., Sirri, R., Grandi, M., et al. (2018b). Feeding European sea bass with increasing dietary fibre levels: Impact on growth, blood biochemistry, gut histology, gut evacuation. Aquaculture 494, 1–9. doi: 10.1016/j.aquaculture.2018.05.017
Bonvini, E., Parma, L., Mandrioli, L., Sirri, R., Brachelente, C., Mongile, F., et al. (2015). Feeding common sole (Solea solea) juveniles with increasing dietary lipid levels affects growth, feed utilization and gut health. Aquaculture 449, 87–93. doi: 10.1016/j.aquaculture.2015.01.013
Boyd, C. E., D’Abramo, L. R., Glencross, B. D., Huyben, D. C., Juarez, L. M., Lockwood, G. S., et al. (2020). Achieving sustainable aquaculture: historical and current perspectives and future needs and challenges. J. World Aquac. Soc. 51, 578–633. doi: 10.1111/jwas.12714
Bradford, M. M. (1976). A rapid and sensitive method for the quantitation of microgram quantities of protein utilizing the principle of protein-dye binding. Anal. Biochem. 72, 248–254.
Busti, S., Bonaldo, A., Dondi, F., Cavallini, D., Yúfera, M., Gilannejad, N., et al. (2020). Effects of different feeding frequencies on growth, feed utilisation, digestive enzyme activities and plasma biochemistry of gilthead sea bream (Sparus aurata). Aquaculture 529, 735616. doi: 10.1016/j.aquaculture.2020.735616
Castillo, J., Teles, M., Mackenzie, S., and Tort, L. (2009). Stress-related hormones modulate cytokine expression in the head kidney of gilthead seabream (Sparus aurata). Fish Shellf. Immunol. 27, 493–499. doi: 10.1016/j.fsi.2009.06.021
Castro, C., Peréz-jiménez, A., Coutinho, F., and Corraze, G. (2018). Nutritional history does not modulate hepatic oxidative status of European sea bass (Dicentrarchus labrax) submitted to handling stress. Fish Physiol. Biochem. 44, 911–918. doi: 10.1007/s10695-018-0480-6
Coeurdacier, J. L., Dutto, G., Gasset, E., and Blancheton, J. P. (2011). Is total serum protein a good indicator for welfare in reared sea bass (Dicentrarchus labrax)? Aquat. Liv. Resourc. 24, 121–127. doi: 10.1051/alr/2011130
Cordero, H., Morcillo, P., Cuesta, A., Brinchmann, M. F., and Esteban, M. A. (2016). Differential proteome profile of skin mucus of gilthead seabream (Sparus aurata) after probiotic intake and/or overcrowding stress. J. Proteomics 132, 41–50. doi: 10.1016/j.jprot.2015.11.017
Coutinho, F., Simões, R., Oliva-Teles, A., Oliva-Teles, A., Peres, H., Monge-Ortiz, R., et al. (2017). Effects of dietary methionine and taurine supplementation to low-fish meal diets on growth performance and oxidative status of European sea bass (Dicentrarchus labrax) juveniles. Aquaculture 479, 447–454. doi: 10.1016/J.aquaculture.2017.06.017
De Kloet, E. R., Joëls, M., and Holsboer, F. (2005). Stress and the brain: from adaptation to disease. Nat. Rev. Neurosci. 6, 463–475. doi: 10.1038/nrn1683
De Kloet, E. R., Karst, H., and Joëls, M. (2008). Corticosteroid hormones in the central stress response: quick-and-slow. Front. Neuroendocrinol. 29:268–272. doi: 10.1016/j.yfrne.2007.10.002
Di Marco, P., Priori, A., Finoia, M. G., Massari, A., Mandich, A., and Marino, G. (2008). Physiological responses of European sea bass Dicentrarchus labrax to different stocking densities and acute stress challenge. Aquaculture 275, 319–328. doi: 10.1016/j.aquaculture.2007.12.012
Djordjevic, B., Morales-Lange, B., Press, C. M., Olson, J., Lagos, L., Mercado, L., et al. (2021). Comparison of circulating markers and mucosal immune parameters from skin and distal intestine of Atlantic salmon in two models of acute stress. Int. J. Mol. Sci. 22, 1–13. doi: 10.3390/ijms22031028
d’Orbcastel, E. R., Lemarié, G., Breuil, G., Petochi, T., Marino, G., Triplet, G., et al. (2010). Effects of rearing density on sea bass (Dicentrarchus labrax) biological performance, blood parameters and disease resistance in a flow through system. Aquat. Liv. Resourc. 23, 109–117. doi: 10.1051/alr/2009056
Eissa, N., and Wang, H.-P. (2016). Transcriptional stress responses to environmental and husbandry stressors in aquaculture species. Rev. Aquac. 8, 61–88. doi: 10.1111/raq.12081
El Aamri, F., Real, F., Acosta, F., Bravo, J., Román, L., Déniz, S., et al. (2015). Differential innate immune response of European seabass (Dicentrarchus labrax) against Streptococcus iniae. Fish Shellf. Immunol. 46, 436–441. doi: 10.1016/j.fsi.2015.05.054
Ellis, T., Yildiz, H. Y., López-Olmeda, J., Spedicato, M. T., Tort, L., and Øverli, Ø, et al. (2012). Cortisol and finfish welfare. Fish Physiol. Biochem. 38, 163–188. doi: 10.1007/s10695-011-9568-y
Fanouraki, E., Divanach, P., and Pavlidis, M. (2007). Baseline values for acute and chronic stress indicators in sexually immature red porgy (Pagrus pagrus). Aquaculture 265, 294–304. doi: 10.1016/j.aquaculture.2007.01.006
Fanouraki, E., Mylonas, C. C., Papandroulakis, N., and Pavlidis, M. (2011). Species specificity in the magnitude and duration of the acute stress response in Mediterranean marine fish in culture. Gen. Compar. Endocrinol. 173, 313–322. doi: 10.1016/j.ygcen.2011.06.004
Geay, F., Ferraresso, S., Zambonino-Infante, J. L., Bargelloni, L., Quentel, C., Vandeputte, M., et al. (2011). Effects of the total replacement of fish-based diet with plant-based diet on the hepatic transcriptome of two European sea bass (Dicentrarchus labrax) half-sibfamilies showing different growth rates with the plant-based diet. BMC Genomics 12:522. doi: 10.1186/1471-2164-12-522
Gisbert, E., Fournier, V., Solovyev, M., Skalli, A., and Andree, K. B. (2018). Diets containing shrimp protein hydrolysates provided protection to European sea bass (Dicentrarchus labrax) affected by a Vibrio pelagius natural infection outbreak. Aquaculture 495, 136–143. doi: 10.1016/j.aquaculture.2018.04.051
Gornati, R., Papis, E., Rimoldi, S., Terova, G., Saroglia, M., and Bernardini, G. (2004). Rearing density influences the expression of stress-related genes in sea bass (Dicentrarchus labrax, L.). Gene 341, 111–118. doi: 10.1016/j.gene.2004.06.020
Guardiola, F. A., Cuesta, A., Abellán, E., Meseguer, J., and Esteban, M. A. (2014a). Comparative analysis of the humoral immunity of skin mucus from several marine teleost fish. Fish Shellf. Immunol. 40, 24–31. doi: 10.1016/j.fsi.2014.06.018
Guardiola, F. A., Cuesta, A., and Esteban, M. A. (2016). Using skin mucus to evaluate stress in gilthead seabream (Sparus aurata L.). Fish Shellf. Immunol. 59, 323–330. doi: 10.1016/j.fsi.2016.11.005
Guardiola, F. A., Cuesta, A., Arizcun, M., Meseguer, J., and Esteban, M. A. (2014b). Comparative skin mucus and serum humoral defence mechanisms in the teleost gilthead seabream (Sparus aurata). Fish Shellf. Immunol. 36, 545–551. doi: 10.1016/j.fsi.2014.01.001
Guardiola, F. A., Saraiva-Fraga, M., Cuesta, A., and Esteban, M. A. (2018). Changes in natural haemolytic complement activity induced by stress in gilthead seabream (Sparus aurata L.). Fish Shellf. Immunol. 78, 317–321. doi: 10.1016/j.fsi.2018.04.056
Guo, H., and Dixon, B. (2021). Understanding acute stress-mediated immunity in teleost fish. Fish Shellf. Immunol. Rep. 2:100010. doi: 10.1016/j.fsirep.2021.100010
Hall, T. (1999). BioEdit: a user-friendly biological sequence alignment editor and analysis program for Windows 95/98/NT. Nucleic Acids Symp. Ser. 41, 95–98.
Hanif, A., Bakopoulos, V., and Dimitriadis, G. J. (2004). Maternal transfer of humoral specific and non-specific immune parameters to sea bream (Sparus aurata) larvae. Fish Shellf. Immunol. 17, 411–435. doi: 10.1016/j.fsi.2004.04.013
Hardy, R. W. (2010). Utilization of plant proteins in fish diets: effects of global demand and supplies of fishmeal. Aquac. Res. 41, 770–776. doi: 10.1111/j.1365-2109.2009.02349.x
Henry, M. A., Fountoulaki, E., Vasilaki, A., Rigos, G., Kokou, F., and Karalazos, V. (2020). Dietary micronutrient supplementation in low fishmeal based diets for optimum growth and immune status of European sea bass (Dicentrarchus labrax) juveniles. Aquaculture 528:735479. doi: 10.1016/j.aquaculture.2020.735479
Hoseinifar, S. H., Sharifian, M., Vesaghi, M. J., Khalili, M., and Esteban, M. A. (2014). The effects of dietary xylooligosaccharide on mucosal parameters, intestinal microbiota and morphology and growth performance of Caspian white fish (Rutilus frisii kutum) fry. Fish Shellf. Immunol. 39, 231–236. doi: 10.1016/j.fsi.2014.05.009
Hrubec, T. C., and Smith, S. S. (2010). “Hematology of fishes,” in Schalm’s Veterinary Hematology, 6th Edn, eds D. Weiss, K. Wardrop, and O. Schalm (Hoboken, NJ: Wiley-Blackwell), 994–1003.
Islam, M. J., Kunzmann, A., and Slater, M. J. (2021). Extreme winter cold-induced osmoregulatory, metabolic, and physiological responses in European seabass (Dicentrarchus labrax) acclimatized at different salinities. Sci. Total Environ. 771:145202. doi: 10.1016/j.scitotenv.2021.145202
Jia, R., Liu, B.-L., Han, C., Huang, B., and Lei, J.-L. (2016). Influence of stocking density on growth performance, antioxidant status, and physiological response of juvenile turbot, Scophthalmus maximus, reared in land-based recirculating aquaculture system. J. World Aquac. Soc. 47, 587–599. doi: 10.1111/jwas.12295
Jung, T. S., Del Castillo, C. S., Javaregowda, P. K., Dalvi, R. S., Nho, S. W., Park, S. B., et al. (2012). Seasonal variation and comparative analysis of non-specific humoral immune substances in the skin mucus of olive flounder (Paralichthys olivaceus). Dev. Compar. Immunol. 38, 295–301. doi: 10.1016/j.dci.2012.06.005
Kaushik, S. J., Covès, D., Dutto, G., and Blanc, D. (2004). Almost total replacement of fish meal by plant protein sources in the diet of a marine teleost, the European seabass, Dicentrarchus labrax. Aquaculture 230, 391–404. doi: 10.1016/S0044-8486(03)00422-8
Kwasek, K., Thorne-Lyman, A. L., and Phillips, M. (2020). Can human nutrition be improved through better fish feeding practices? A review paper. Crit. Rev. Food Sci. Nutr. 60, 3822–3835. doi: 10.1080/10408398.2019.1708698
Lall, S. P. (2002). “The minerals,” in Fish Nutrition, 3rd Edn, eds J. E. Halver and R. W. Hardy (Cambridge, MA: Academic Press), 824.
Machado, M., Castro, C., Oliva-Teles, A., and Costas, B. (2019). Interactive effects of dietary vegetable oil and carbohydrate incorporation on the innate immune response of European seabass (Dicentrarchus labrax) juveniles subjected to acute stress. Aquaculture 498, 171–180. doi: 10.1016/j.aquaculture.2018.08.050
Maricchiolo, G., Mirto, S., Caruso, G., Caruso, T., Bonaventura, R., Celi, M., et al. (2011). Welfare status of cage farmed European sea bass (Dicentrarchus labrax): a comparison between submerged and surface cages. Aquaculture 314, 173–181. doi: 10.1016/j.aquaculture.2011.02.001
Masek, T., Vopalensky, V., Suchomelova, P., and Pospisek, M. (2005). Denaturing RNA electrophoresis in TAE agarose gels. Anal. Biochem. 336, 46–50. doi: 10.1016/j.ab.2004.09.010
Médale, F., and Kaushik, S. (2009). Les sources protéiques dans les aliments pour les poissons d’élevage. Cah. Agric. 18, 103–111. doi: 10.1684/agr.2009.0279
Meloni, M., Candusso, S., Galeotti, M., and Volpatti, D. (2015). Preliminary study on expression of antimicrobial peptides in European sea bass (Dicentrarchus labrax) following in vivo infection with Vibrio anguillarum. A time course experiment. Fish Shellf. Immunol. 43, 82–90. doi: 10.1016/j.fsi.2014.12.016
Mommsen, T. P., Vijayan, M. M., and Moon, T. W. (1999). Cortisol in teleosts: dynamics, mechanisms of action, and metabolic regulation. Rev. Fish Biol. Fish. 9, 211–268. doi: 10.1023/a:1008924418720
Montero, D., and Izquierdo, M. (2010). “Welfare and health of fish fed vegetable oils as alternative lipid sources to fish oil,” in Fish Oil Replacement and Alternative Lipid Sources in Aquaculture Feeds, eds G. M. Turchini, W.-K. Ng, and D. R. Tocher (Boca Raton, FL: CRC Press), 439–485.
Montero, D., Izquierdo, M. S., Tort, L., Robaina, L., and Vergara, J. M. (1999). High stocking density produces crowding stress altering some physiological and biochemical parameters in gilthead seabream, Sparus aurata, juveniles. Fish Physiol. Biochem. 20, 53–60. doi: 10.1023/a:1007719928905
Montero, D., Kalinowski, T., Obach, A., Robaina, L., Tort, L., Caballero, M. J., et al. (2003). Vegetable lipid sources for gilthead seabream (Sparus aurata): effects on fish health. Aquaculture 225, 353–370. doi: 10.1016/S0044-8486(03)00301-6
Mosca, F., Ciulli, S., Volpatti, D., Romano, N., Volpe, E., Bulfon, C., et al. (2014). Defensive response of European sea bass (Dicentrarchus labrax) against Listonella anguillarum or Photobacterium damselae subsp. piscicida experimental infection. Vet. Immunol. Immunopathol. 162, 83–95. doi: 10.1016/j.vetimm.2014.10.002
Nasopoulou, C., and Zabetakis, I. (2012). Benefits of fish oil replacement by plant originated oils in compounded fish feeds. A review. LWT 47, 217–224. doi: 10.1016/j.lwt.2012.01.018
Parma, L., Pelusio, N. F., Gisbert, E., Esteban, M. A., D’Amico, F., Soverini, M., et al. (2020). Effects of rearing density on growth, digestive conditions, welfare indicators and gut bacterial community of gilthead sea bream (Sparus aurata, L. 1758) fed different fishmeal and fish oil dietary levels. Aquaculture 518:734854. doi: 10.1016/j.aquaculture.2019.734854
Parma, L., Yúfera, M., Navarro-Guillén, C., Moyano, F. J., Soverini, M., D’Amico, F., et al. (2019). Effects of calcium carbonate inclusion in low fishmeal diets on growth, gastrointestinal pH, digestive enzyme activity and gut bacterial community of European sea bass (Dicentrarchus labrax L.) juveniles. Aquaculture 510, 283–292. doi: 10.1016/j.aquaculture.2019.05.064
Parry, R. M., Chandan, R. C., and Shahani, K. M. (1965). A Rapid and sensitive assay of muramidase. Exp. Biol. Med. 119, 384–386. doi: 10.3181/00379727-119-30188
Pavlidis, M., Karantzali, E., Fanouraki, E., Barsakis, C., Kollias, S., and Papandroulakis, N. (2011). Onset of the primary stress in European sea bass Dicentrarhus labrax, as indicated by whole body cortisol in relation to glucocorticoid receptor during early development. Aquaculture 315, 125–130. doi: 10.1016/j.aquaculture.2010.09.013
Pelusio, N. F., Scicchitano, D., Parma, L., Dondi, F., Brini, E., D’Amico, F., et al. (2021). Interaction between dietary lipid level and seasonal temperature changes in gilthead sea bream Sparus aurata: effects on growth, fat deposition, plasma biochemistry, digestive enzyme activity, and gut bacterial community. Front. Mar. Sci. 8:664701. doi: 10.3389/fmars.2021.664701
Peres, H., Santos, S., and Oliva-Teles, A. (2013). Selected plasma biochemistry parameters in gilthead seabream (Sparus aurata) juveniles. J. Appl. Ichthyol. 29, 630–636. doi: 10.1111/j.1439-0426.2012.02049.x
Peres, H., Santos, S., and Oliva-Teles, A. (2014). Blood chemistry profile as indicator of nutritional status in European seabass (Dicentrarchus labrax). Fish Physiol. Biochem. 40, 1339–1347. doi: 10.1007/s10695-014-9928-5
Pérez-Jiménez, A., Guedes, M. J., Morales, A. E., and Oliva-Teles, A. (2007). Metabolic responses to short starvation and refeeding in Dicentrarchus labrax. Effect of dietary composition. Aquaculture 265, 325–335. doi: 10.1016/j.aquaculture.2007.01.021
Pfaffl, M. W. (2004). “Quantification strategies in real-time PCR,” in AZ of Quantitative PCR, ed. S. A. Bustin (La Jolla, CA: International University Line), 89–113.
Poltronieri, C., Maccatrozzo, L., Simontacchi, C., Bertotto, D., Funkenstein, B., Patruno, M., et al. (2007). Quantitative RT-PCR analysis and immunohistochemical localization of HSP70 in sea bass Dicentrarchus labrax exposed to transport stress. Eur. J. Histochem. 51, 125–136. doi: 10.4081/1134
Quade, M. J., and Roth, J. A. (1997). A rapid, direct assay to measure degranulation of bovine neutrophil primary granules. Vet. Immunol. Immunopathol. 58, 239–248. doi: 10.1016/s0165-2427(97)00048-2
Reverter, M., Tapissier-Bontemps, N., Sarter, S., Sasal, P., and Caruso, D. (2021). Moving towards more sustainable aquaculture practices: a meta-analysis on the potential of plant-enriched diets to improve fish growth, immunity and disease resistance. Rev. Aquac.e 13, 537–555. doi: 10.1111/raq.12485
Reyes-López, F. E., Aerts, J., Vallejos-Vidal, E., Ampe, B., Dierckens, K., Tort, L., et al. (2018). Modulation of innate immune-related genes and glucocorticoid synthesis in gnotobiotic full-sibling European sea bass (Dicentrarchus labrax) larvae challenged with Vibrio anguillarum. Front. Immunol. 9:914. doi: 10.3389/fimmu.2018.00914
Roosta, Z., and Hoseinifar, S. H. (2016). The effects of crowding stress on some epidermal mucus immune parameters, growth performance and survival rate of tiger barb (Pentius tetrazona). Aquac. Res. 47, 1682–1686. doi: 10.1111/are.12616
Roque, A., Yildiz, H. Y., Carazo, I., and Duncan, N. (2010). Physiological stress responses of sea bass (Dicentrarchus labrax) to hydrogen peroxide (H2O2) exposure. Aquaculture 304, 104–107. doi: 10.1016/j.aquaculture.2010.03.024
Ross, N. W., Firth, K. J., Wang, A., Burka, J. F., and Johnson, S. C. (2000). Changes in hydrolytic enzyme activities of naïve Atlantic salmon Salmo salar skin mucus due to infection with the salmon louse Lepeophtheirus salmonis and cortisol implantation. Dis. Aquat. Org. 41, 43–51. doi: 10.3354/dao041043
Ruane, N. M., Huisman, E. A., and Komen, J. (2002). The influence of feeding history on the acute stress response of common carp (Cyprinus carpio). Aquaculture 210, 245–257. doi: 10.1016/S0044-8486(01)00819-5
Saccol, E. M. H., Parrado-Sanabria, Y. A., Gagliardi, L., Jerez-Cepa, I., Mourão, R. H. V., Heinzmann, B. M., et al. (2018). Myrcia sylvatica essential oil in the diet of gilthead sea bream (Sparus aurata L.) attenuates the stress response induced by high stocking density. Aquac. Nutr. 24, 1381–1392. doi: 10.1111/anu.12675
Sales, J., and Glencross, B. (2011). A meta-analysis of the effects of dietary marine oil replacement with vegetable oils on growth, feed conversion and muscle fatty acid composition of fish species. Aquac. Nutr. 17, e271–e287. doi: 10.1111/j.1365-2095.2010.00761.x
Samaras, A., Pavlidis, M., Lika, K., Theodoridi, A., and Papandroulakis, N. (2017). Scale matters: performance of European sea bass, Dicentrarchus labrax, L. (1758), reared in cages of different volumes. Aquac. Res. 48, 990–1005. doi: 10.1111/are.12942
Samaras, A., Santo, C. E., Papandroulakis, N., Mitrizakis, N., Pavlidis, M., Höglund, E., et al. (2018). Allostatic load and stress physiology in European seabass (Dicentrarchus labrax L.) and gilthead seabream (Sparus aurata L.). Front. Endocrinol. 9:451. doi: 10.3389/fendo.2018.00451
Sanahuja, I., Fernández-Alacid, L., Ordóñez-Grande, B., Sánchez-Nuño, S., Ramos, A., Araujo, R. M., et al. (2019). Comparison of several non-specific skin mucus immune defences in three piscine species of aquaculture interest. Fish Shellf. Immunol. 89, 428–436. doi: 10.1016/j.fsi.2019.04.008
Serradell, A., Torrecillas, S., Makol, A., Valdenegro, V., Fernández-Montero, A., Acosta, F., et al. (2020). Prebiotics and phytogenics functional additives in low fish meal and fish oil based diets for European sea bass (Dicentrarchus labrax): effects on stress and immune responses. Fish Shellf. Immunol. 100, 219–229. doi: 10.1016/j.fsi.2020.03.016
Sheikhzadeh, N., Heidarieh, M., Karimi Pashaki, A., Nofouzi, K., Ahrab Farshbafi, M., and Akbari, M. (2012a). Hilyses®, fermented Saccharomyces cerevisiae, enhances the growth performance and skin non-specific immune parameters in rainbow trout (Oncorhynchus mykiss). Fish Shellf. Immunol. 32, 1083–1087. doi: 10.1016/j.fsi.2012.03.003
Sheikhzadeh, N., Karimi Pashaki, A., Nofouzi, K., Heidarieh, M., and Tayefi-Nasrabadi, H. (2012b). Effects of dietary Ergosan on cutaneous mucosal immune response in rainbow trout (Oncorhynchus mykiss). Fish Shellf. Immunol. 32, 407–410. doi: 10.1016/j.fsi.2011.11.028
Sinuani, I., Beberashvili, I., Averbukh, Z., and Sandbank, J. (2013). Role of IL-10 in the progression of kidney disease. World J. Transplant. 3, 91–98. doi: 10.5500/wjt.v3.i4.91
Sitjà-Bobadilla, A., Peña-Llopis, S., Gómez-Requeni, P., Médale, F., Kaushik, S., and Pérez-Sánchez, J. (2005). Effect of fish meal replacement by plant protein sources on non-specific defence mechanisms and oxidative stress in gilthead sea bream (Sparus aurata). Aquaculture 249, 387–400. doi: 10.1016/j.aquaculture.2005.03.031
Stevens, M. G., Kehrli, M. E., and Canning, P. C. (1991). A colorimetric assay for quantitating bovine neutrophil bactericidal activity. Vet. Immunol. Immunopathol. 28, 45–56. doi: 10.1016/0165-2427(91)90042-b
Sutili, F. J., Gatlin, D. M., Heinzmann, B. M., and Baldisserotto, B. (2018). Plant essential oils as fish diet additives: benefits on fish health and stability in feed. Rev. Aquac. 10, 716–726. doi: 10.1111/raq.12197
Torrecillas, S., Betancor, M. B., Caballero, M. J., Rivero, F., Robaina, L., Izquierdo, M., et al. (2018). Supplementation of arachidonic acid rich oil in European sea bass juveniles (Dicentrarchus labrax) diets: effects on growth performance, tissue fatty acid profile and lipid metabolism. Fish Physiol. Biochem. 44, 283–300. doi: 10.1007/s10695-017-0433-5
Torrecillas, S., Mompel, D., Caballero, M. J., Montero, D., Merrifield, D., Rodiles, A., et al. (2017a). Effect of fishmeal and fish oil replacement by vegetable meals and oils on gut health of European sea bass (Dicentrarchus labrax). Aquaculture 468, 386–398. doi: 10.1016/j.aquaculture.2016.11.005
Torrecillas, S., Montero, D., Domínguez, D., Robaina, L., and Izquierdo, M. (2019). Skin mucus fatty acid composition of gilthead sea bream (Sparus aurata): a descriptive study in fish fed low and high fish meal diets. Fishes 4:15. doi: 10.3390/fishes4010015
Torrecillas, S., Robaina, L., Caballero, M. J., Montero, D., Calandra, G., Mompel, D., et al. (2017b). Combined replacement of fishmeal and fish oil in European sea bass (Dicentrarchus labrax): production performance, tissue composition and liver morphology. Aquaculture 474, 101–112. doi: 10.1016/j.aquaculture.2017.03.031
Tort, L., and Teles, M. (2012). Genomic responses to stress challenges in fish. Funct. Genom. Aquac. 7, 147–168. doi: 10.1002/9781118350041.ch7
Tsalafouta, A., Papandroulakis, N., and Pavlidis, M. (2015). Early life stress and effects at subsequent stages of development in European sea bass (D. labrax). Aquaculture 436, 27–33. doi: 10.1016/j.aquaculture.2014.10.042
Valero, Y., Arizcun, M., Cortés, J., Ramírez-Cepeda, F., Guzmán, F., Mercado, L., et al. (2020). NK-lysin, dicentracin and hepcidin antimicrobial peptides in European sea bass. Ontogenetic development and modulation in juveniles by nodavirus. Dev. Compar. Immunol. 103:103516. doi: 10.1016/j.dci.2019.103516
Valero, Y., García-Alcázar, A., Esteban, M. A., Cuesta, A., and Chaves-Pozo, E. (2015). Antimicrobial response is increased in the testis of European sea bass, but not in gilthead seabream, upon nodavirus infection. Fish Shellf. Immunol. 44, 203–213. doi: 10.1016/j.fsi.2015.02.015
Van Anholt, R. D., Spanings, F. A. T., Koven, W. M., Nixon, O., and Wendelaar Bonga, S. E. (2004). Arachidonic acid reduces the stress response of gilthead seabream Sparus aurata L. J. Exp. Biol. 207, 3419–3430. doi: 10.1242/jeb.01166
Keywords: aquaculture, feeding strategy, dietary fishmeal and fishoil, crowding stress, growth, skin mucus, plasma biochemistry, gene expression
Citation: Pelusio NF, Bonaldo A, Gisbert E, Andree KB, Esteban MA, Dondi F, Sabetti MC, Gatta PP and Parma L (2022) Different Fish Meal and Fish Oil Dietary Levels in European Sea Bass: Welfare Implications After Acute Confinement Stress. Front. Mar. Sci. 8:779053. doi: 10.3389/fmars.2021.779053
Received: 17 September 2021; Accepted: 13 December 2021;
Published: 04 January 2022.
Edited by:
Aires Oliva-Teles, University of Porto, PortugalReviewed by:
Manuel Gesto, Technical University of Denmark, DenmarkPatricia Diaz-Rosales, Centro de Investigación en Sanidad Animal (CISA), Instituto Nacional de Investigaciones Agrarias-Consejo Superior de Investigaciones Científicas (INIA-CSIC), Spain
Copyright © 2022 Pelusio, Bonaldo, Gisbert, Andree, Esteban, Dondi, Sabetti, Gatta and Parma. This is an open-access article distributed under the terms of the Creative Commons Attribution License (CC BY). The use, distribution or reproduction in other forums is permitted, provided the original author(s) and the copyright owner(s) are credited and that the original publication in this journal is cited, in accordance with accepted academic practice. No use, distribution or reproduction is permitted which does not comply with these terms.
*Correspondence: Luca Parma, luca.parma@unibo.it