- Flanders Marine Institute (VLIZ), Ostend, Belgium
Climate driven changes and anthropogenic pressures on the marine environment have been shown to favor the increase in certain potentially harmful species. Among them, Noctiluca scintillans, a common dinoflagellate, often blooms during warm summers and is known to affect plankton communities. In this study, we assessed the dynamics in abundance and cell size of N. scintillans as well as the relationship between N. scintillans and small soft-bodied zooplankton in the Belgian part of the North Sea (BPNS), since negative correlations between these plankton groups have been previously reported for nearby regions. This study is the first to present consistently counted N. scintillans cell numbers and measured cell lengths, through the analysis of ZooScan images from samples taken monthly at stations throughout the coastal zone of the BPNS. The results show that N. scintillans demonstrated clear seasonal dynamics with both high densities and large cell sizes in spring/summer (May-July). The occurrence of N. scintillans in the analyzed plankton samples and the abundance of N. scintillans at the observed peak intensities nearly tripled over a period of 5 years. A zero-inflated model showed a correlation of N. scintillans abundance with temperature as well as with phosphate concentrations, suggesting that anthropogenic influences such as climate change and riverine nutrient inputs could affect the temporal dynamics of the species. The results, on the other hand, did not show any negative impact of N. scintillans on the soft-bodied plankton community.
Introduction
Over the last decades, climate driven changes and anthropogenic pressures have increasingly influenced the marine environment. Nutrient inputs of anthropogenic origin have notably led to the eutrophication of marine systems, particularly in coastal areas. In the southern North Sea (Lancelot et al., 1998; Daro et al., 2006), major western European rivers like the Rhine, Meuse and Scheldt (Van Bennekom and Wetsteijn, 1990; Lacroix et al., 2004) have a dominant influence on the water nutrient balance and thus can greatly influence the spring algal bloom dynamics. Notable shifts from diatom to flagellate dominance such as flagellate dominance of Noctiluca scintillans, have been observed over the last decades in this zone (Vasas et al., 2007).
The blooms of certain flagellates and phytoplankton species can be associated with negative effects on the marine environment, human health and the economy (Granéli and Turner, 2006) and are hence referred to as “harmful algal blooms” (Glibert et al., 2005). Noctiluca scintillans is one of the most important and abundant red tide organisms and its blooms have been linked to increased fish and marine invertebrate mortality (Huang and Qi, 1997; Thangaraja et al., 2007), affecting yields in fisheries and aquaculture. The high accumulations of toxic ammonia during blooms, which assist in the buoyancy of the cells (Fonda Umani et al., 2004), possibly act as the killing agent for other organisms (Okaichi and Nishio, 1976; Faust and Gulledge, 2002). In addition, the large size (0.2–2 mm) and voracious feeding behavior of N. scintillans enables it to feed on a broad spectrum of organisms including fish eggs, phytoplankton, zooplankton, detritus and bacteria (Schaumann et al., 1988; Kirchner et al., 1996; Quevedo et al., 1999). Other species feeding on the same food sources as N. scintillans can be affected due to food competition (Enomoto, 1956; Quevedo et al., 1999) which might explain the negative correlations observed between various soft-bodied zooplankton species and N. scintillans (Heyen et al., 1998; Kovalev and Piontkovski, 1998; Fock and Greve, 2002). At present, no consensus has yet been found on the exact drivers of the dynamics or bloom formation of N. scintillans. However, many studies found a variety of factors that correlated with N. scintillans densities, including eutrophication (Polishchuk and Ghilarov, 1981; Boni, 1983; Porumb, 1992; Bologa et al., 1995), specific nutrients [more particularly phosphate (Degobbis et al., 1995)], chlorophyll a (Isinibilir et al., 2008), other plankton species presence [diatom spring blooms (Weston et al., 2008), zooplankton biomass (Cataletto et al., 1995; Fonda Umani et al., 2004; Yılmaz et al., 2005)], physical characteristics of the water column [winter sea surface temperature (Heyen et al., 1998), tides (Holligan, 1979), stratification (Boni, 1983)], and weather conditions [rainfall (Miyaguchi et al., 2006), wind direction (Yamamoto et al., 1997; Nakamura and Hirata, 2006)]. This shows that the dynamics of N. scintillans is complex and depends on many factors, which can differ among locations.
During bloom formation, N. scintillans can reach high densities and can often constitute a significant part of the plankton community. Because manually counting N. scintillans cells is too time consuming, the species is often excluded from microscopy counts (Nohe et al., 2020). Hence, little is known about its dynamics in the Belgian part of the North Sea (BPNS). However, novel imaging techniques such as the ZooScan allow for an accurate assessment of the densities and associated size measurements of such taxa. Due to the potential adverse effects of N. scintillans on the marine environment as well as its potential to increase in abundance as a result of climate change and ocean acidification (Moore et al., 2008), substantial research is needed to gain knowledge on the blooms, drivers and effects of N. scintillans in the BPNS. The ZooScan data series used in this research included data on soft-bodied species, for which time series are very scarce (Aubert et al., 2018). Because of the availability of this unique data series and also due to the significant negative correlations found between N. scintillans and small soft-bodied zooplankton in neighboring areas (Heyen et al., 1998; Fock and Greve, 2002), it was decided to focus on the potential impact of N. scintillans on small soft-bodied plankton in the BPNS. Based on the ZooScan data, this study explores the potential of ZooScan imaging for cell density and size estimates of species of interest such as N. scintillans and aims to unravel the population dynamics of N. scintillans in the BPNS, to determine the drivers of its dynamics, as well as to investigate the impact of the species on small soft-bodied zooplankton taxa.
Materials and Methods
Study Area
The Belgian part of the North Sea (BPNS, ca. 3,600 km2) is located in the Southern Bight of the North Sea. It is a relatively shallow area with maximum depths of 40 meters. The strong tidal currents result in a well-mixed water column with very weak salinity and temperature stratification (Fettweis and Nechad, 2011). The BPNS is known as an eutrophicated ecosystem, due to anthropogenically induced nutrient inputs through the discharge of major West-European rivers such as the Ijzer, Scheldt, and Maas (Nihoul and Hecq, 1984; Lancelot et al., 1998; Daro et al., 2006; Goffin et al., 2015).
Data Acquisition
Open-access time series data (2014–2018) from Flanders Marine Institute (VLIZ) (2019a,b) were used for this analysis. Zooplankton samples and associated water quality parameters were collected on a monthly basis since 2014 at nine stations in the BPNS (Figure 1). Zooplankton was sampled with a 200 μm WP2 net which was deployed vertically and equipped with a flowmeter. Zooplankton collected in the cod-end was then sedated by sodawater and fixated in 4% formalin. In the lab, the fixative was changed to 70% ethanol. All 404 samples were digitized by the ZooScan plankton imaging device and processed by ZooProcess and Plankton Identifier (PkID) in order to detect and classify the digitized objects (Grosjean et al., 2004; Gorsky et al., 2010). The associated water quality parameters (nutrients, pigments and suspended particulate matter) are based on water samples collected with Niskin bottles at 3 m depth. For pigments, seawater was filtered through Whatman GF/F glass fiber filters (47 mm). The filter was subsequently folded, dried and stored frozen. For nutrients, around 200 mL of water was filtered through a 47 mm, 0.2 μm cellulose-acetate filter for residual water. When the filter ran dry, 150mL of the filtered water was poured into a recipient and stored at −24°C. After filtration and processing on board, all samples were sent to specialized companies for further analysis. High Pressure Liquid Chromatography (HPLC) was used for the determination of pigments. Nutrient samples were analyzed by means of a SEAL QuAAtro analysis system or by means of discrete analysis system and spectrophotometric detection with a Skalar AutoAnalyser system. Information on the conductivity, temperature and depth of the water column was obtained by means of a CTD profile. The methodology of these two dataseries is fully described in Mortelmans et al. (2019a,b).
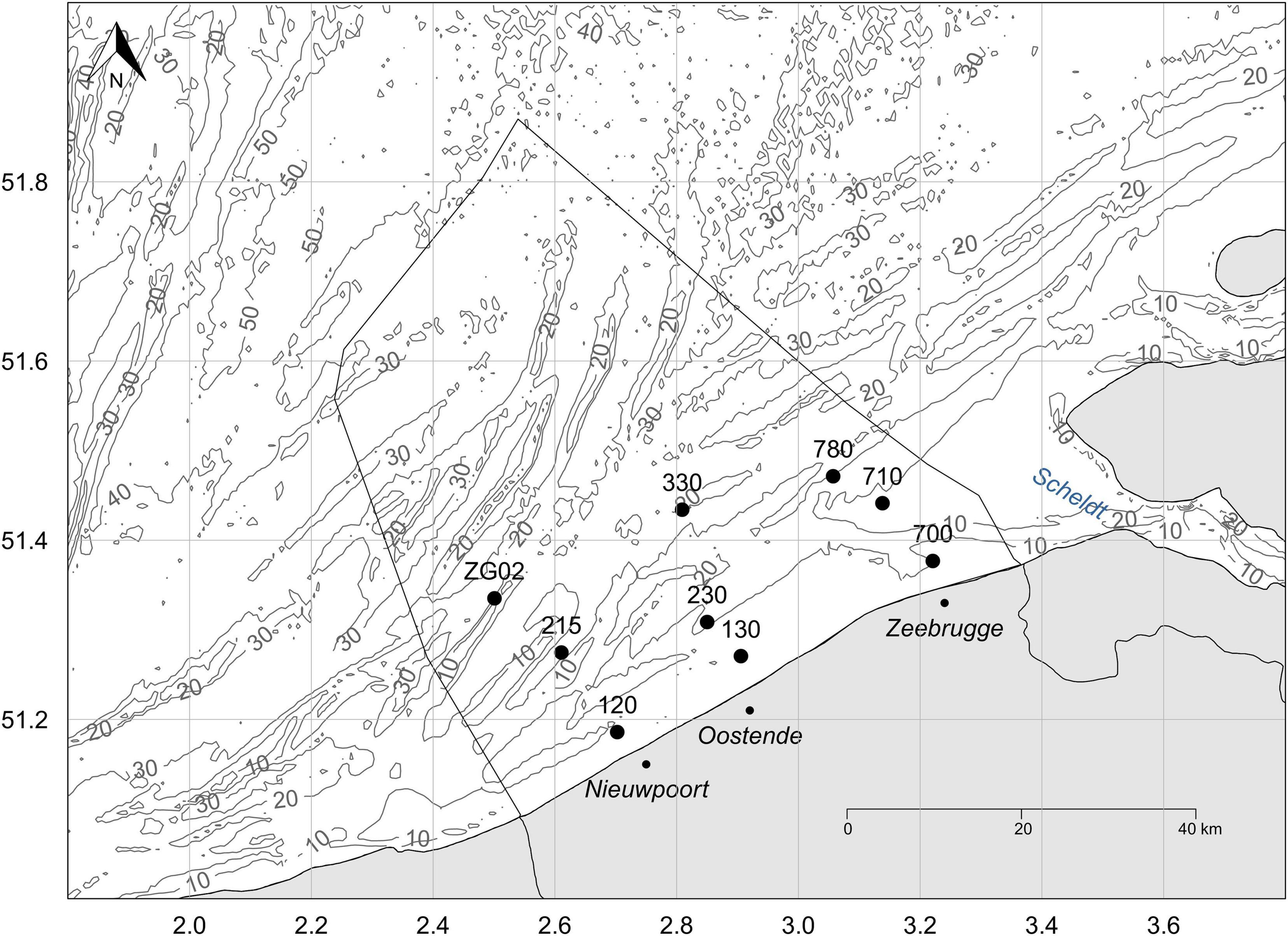
Figure 1. Map of the Belgian part of the North Sea, outlined by the black line in the sea, with indication of the nine stations included in the analysis. The gray lines and numbers indicate the depth [m] of the water column.
Size estimations of N. scintillans were based on the dataset “Flanders Marine Institute (VLIZ) (2019a),” according to the calculations given in Gorsky et al. (2010). These size estimations were performed on regions of interest (ROI’s), a portion of an image presenting a certain particle or organism of interest. Because some ROI’s had overlap between the actual organism of interest and other particles on the scanning bed, size estimations were overestimated in these particular ROI’s. In PkID, a new category called “Noctiluca_unsuitable” was created to store ROI’s containing overlapping particles, ROI’s where the N. scintillans cells are not fully in view and ROI’s of damaged or reproducing N. scintillans cells. After revalidation of the entire dataset, the original category, “Noctiluca,” only held the ROI’s with one N. scintillans cell. About half of the images were transferred to the “Noctiluca_unsuitable” folder, which came down to 33,624 of the 66,142 images. The size estimations were only based on the “Noctiluca” category in the further analysis, whereas density measurements were based on all ROI’s, including the images with overlapping particles. The diameter of a N. scintillans cell was calculated as the mean of the major and minor axis of the ROI [mm]. It should be noted that fixation in formaldehyde affects the N. scintillans cells, as they shrink and can get damaged (Yang et al., 2016). Living N. scintillans cells are thus larger than the size measurements used in this study, and real size can be recalculated as: volume of live cell = volume of intact fixed cell/0.61 (Yang et al., 2016). However, it was decided to work with the length measurements as measured by the ZooScan and not with the recalculated cell measurements, as a matter of comparability with most other studies which used length measurements of formaldehyde fixed cells (e.g., Dela-Cruz et al., 2003; Miyaguchi et al., 2006).
Data Analysis
Before analysis, samples from stations where N. scintillans was not encountered were added to the dataset. Environmental variables provided through the dataset “Flanders Marine Institute (VLIZ) (2019b)” were selected based on literature (Harrison et al., 2011) and availability in the dataset: conductivity, salinity, temperature, ammonia (NH4), phosphate (PO4), and chlorophyll a were taken into the analysis. Conductivity and salinity were collinear, and as salinity was available for fewer samples compared to conductivity, it was removed from further analysis. Samples missing one of the selected environmental variables were not included in the analysis, leading to a total of 311 samples considered over a period of 5 years in the present analysis. The data was subsequently explored (Zuur et al., 2010) and graphically represented per year, month or station.
All analyses were performed with RStudio (version 1.4.1106; RStudio Team, 2020). Kruskal-Wallis test was performed to analyze the spatio-temporal dynamics of N. scintillans abundance and a Wilcoxon test was executed to see which stations, months or years differed significantly from each other. To investigate which environmental variables influenced the dynamics of N. scintillans, generalized linear models (GLMs) were first tested but they did not predict the absence of N. scintillans well. Zero-inflated (ZI) models (Zuur and Ieno, 2016) were subsequently applied as the data contained a significant amount of zero values (e.g., 58% of the N. scintillans abundance data). Akaike information criterion (AIC) scores of different models showed that a ZI model with negative binomial distribution (ZINB) revealed to be the best suited for the overdispersed dataset. The Vuong test supported this and showed that a ZINB model was a significant improvement over a GLM with negative binomial distribution. In our dataset, the densities of the organisms were essentially count data converted to a fixed volume. As ZI models (“pscl” package) can only deal with the dependent variable being a natural number, the densities were rounded. Upwards rounding was, however, chosen to not change the low abundance values (low presence) to zero (absence). Based on AIC scores it was determined which variables were included in the count (abundance) and binary (presence/absence) part of the ZINB. The model with the lowest AIC score of which all included variables were significant, was selected as the final model. The same approach was used to define the models for the soft-bodied taxa: Appendicularia, Chaetognatha, Cnidaria, and Ctenophora. Autocorrelation patterns were analyzed with the autocorrelation function (ACF) in RStudio. A Redundancy Data Analysis (RDA) was performed to complement the modeling approach by investigating and visualizing the relationship between plankton taxa and environmental predictors. For this, plankton data was log(x+1) transformed to stabilize variance and to reduce the influence of dominant variables on the arrangement.
Results
Seasonal and Inter-Annual Variations of Noctiluca scintillans Abundances
Abundances of N. scintillans displayed a clear seasonal pattern (Figure 2) in the BPNS over the 5-year period. Densities started to increase in spring up to a peak in late spring/early summer (May to July). The peak was subsequently followed by a density decrease starting in August. The distribution of the species (Figure 3) showed that it was the most widespread during the end of spring/summer peak (May to July) with high densities at all stations. From August to March, some stations did not observe the species. The lowest observation frequency corresponded to the month January for which N. scintillans was found at only one station.
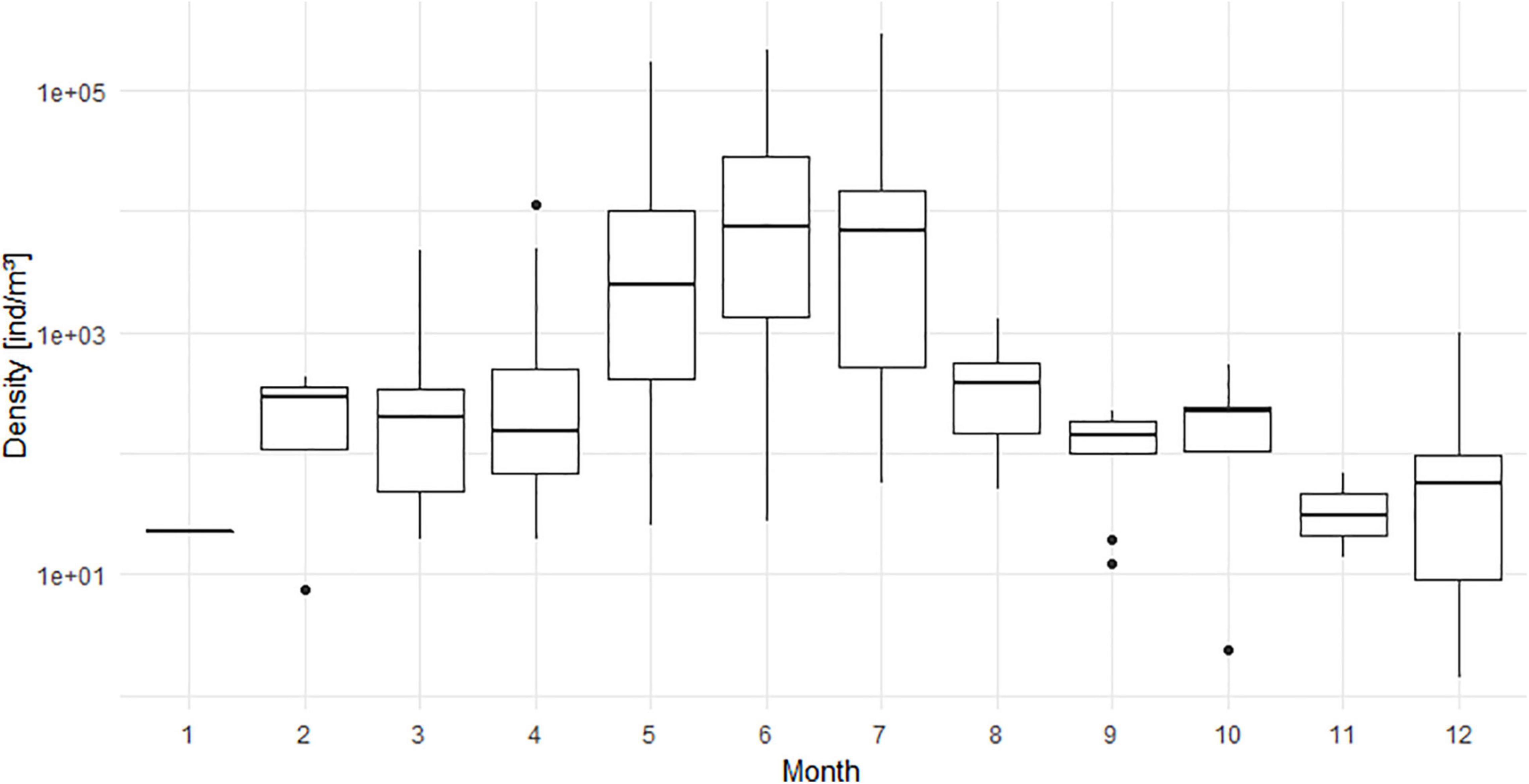
Figure 2. Monthly variations shown as boxplots of abundances of N. scintillans [ind/m3] averaged for the period from 2014 to 2018 for all stations and including the sampled stations where N. scintillans was not encountered. Y-axis has a log10 scale.
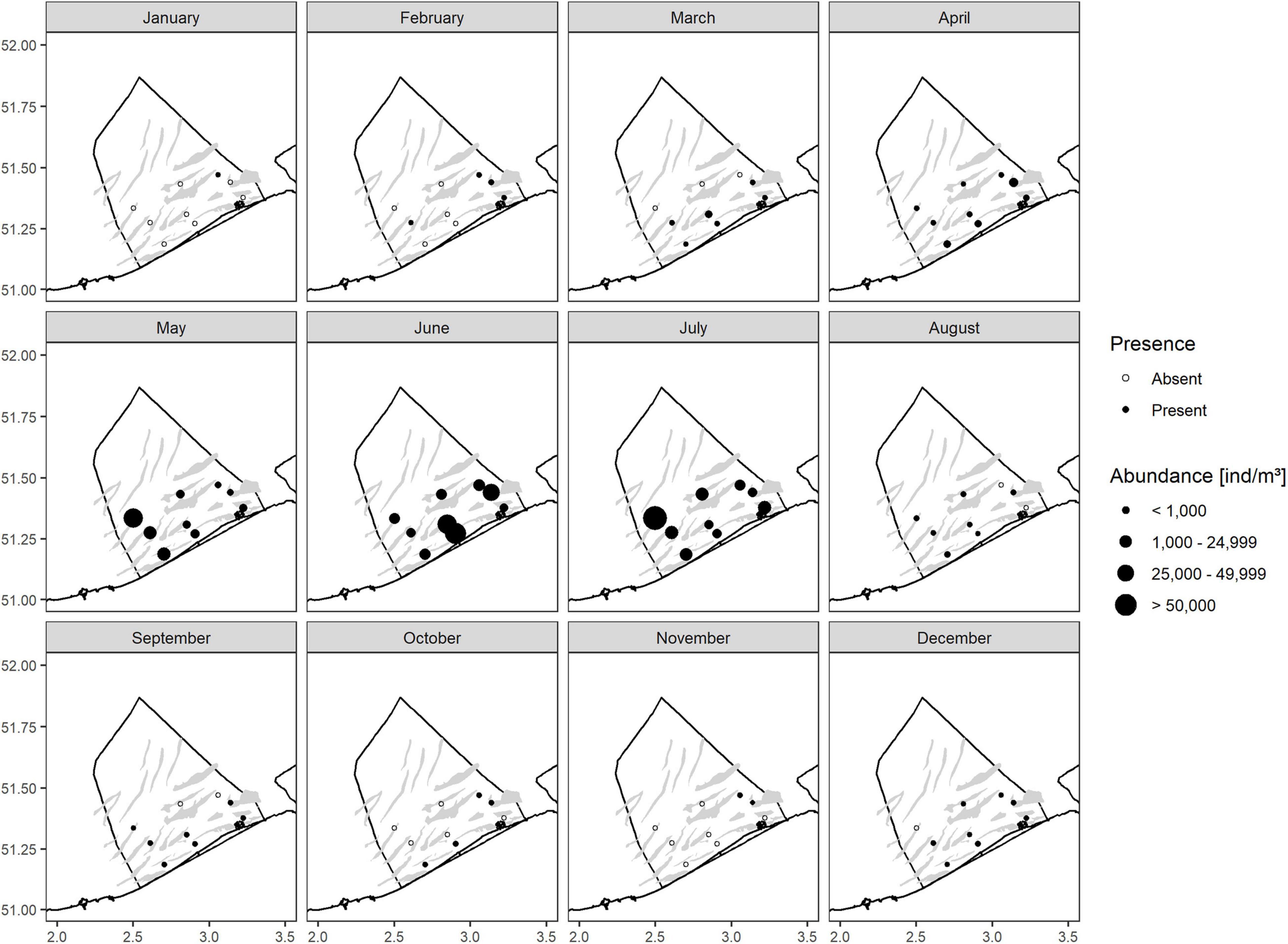
Figure 3. Monthly abundances [ind/m3] of N. scintillans per station for the period from 2014 to 2018 for each sampled station in the BPNS.
What strikes for the period from 2014 to 2018, is the strong increase in prevalence (Table 1) and abundance (Figure 4A) of N. scintillans. In a period of 5 years, we observed that the species was encountered approximately three times more often in the samples, and that peaks during late spring/summer became more numerous per year within the season and became more important in terms of density. The highest density was observed in July 2018 at station ZG02 with a peak density of 290,181 ind/m3. The Kruskal-Wallis test (Supplementary Material 1) showed that the abundance of N. scintillans significantly differed among years (p < 0.001) and months (p < 0.001), but not among stations. A Wilcoxon test demonstrated that 2014 significantly differed from the years 2016 up to 2018 included, and that 2015 differed from 2017 and 2018. December was the month that showed significant differences with all the other months at the exception of March, October and September. Furthermore, January, February and March each significantly differed from the months of April to July. April additionally also differed significantly from June, July, September, October and November. The months May, June and July differed as well from all the months comprised between August and November.
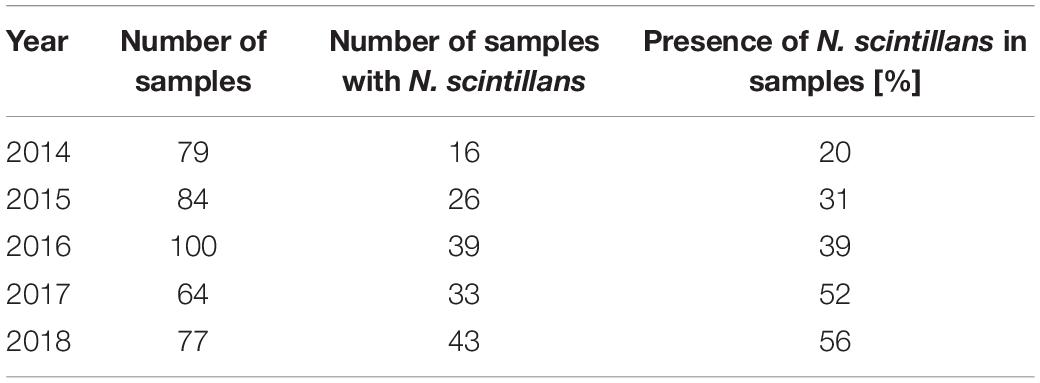
Table 1. Summary of the sampling effort in terms of number of samples per year and indication of the number and percentage of samples containing N. scintillans.
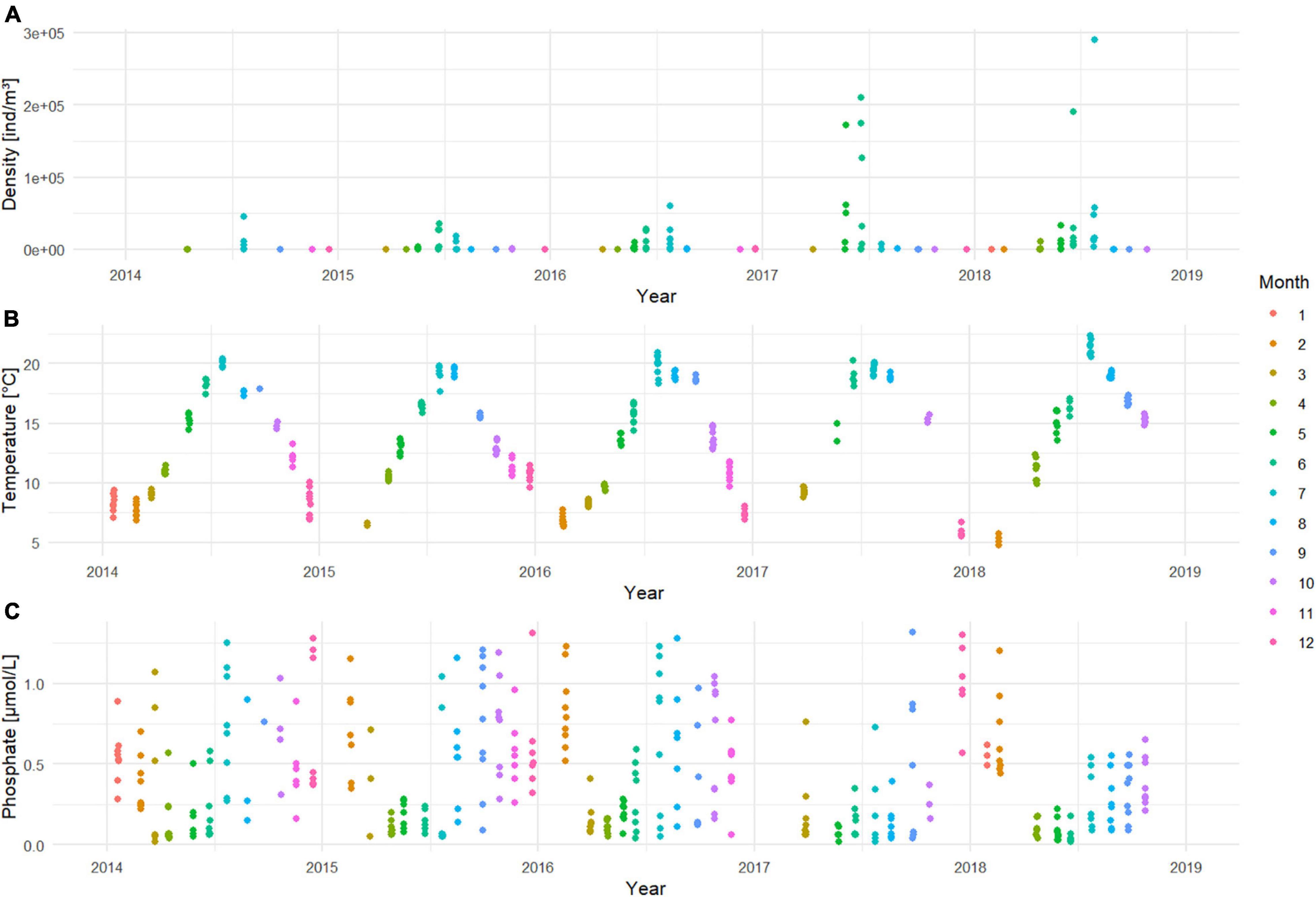
Figure 4. Time series of (A) N. scintillans densities [ind/m3] on a log10 scale; (B) temperature [°C] and (C) phosphate concentrations [μmol/L] for the period 2014–2018.
After evaluating models with the five selected variables (conductivity, temperature, ammonia, phosphate and chlorophyll a), a ZINB model (Supplementary Material 2) with the following variables best explained the dynamics of N. scintillans:
N. scintillans ∼ Temperature | Temperature + PO4 (M1)
The first part of this model proposed temperature (p < 0.001) as the main variable driving the abundance of N. scintillans. With higher temperatures, higher N. scintillans abundances will be observed. The second part of the model proposed that the presence or absence of N. scintillans was determined by both temperature (p < 0.01) and phosphate (p < 0.05). The model predicts that the chance for N. scintillans to be present is significantly larger in high temperatures and in low phosphate concentrations compared to low temperatures and high phosphate concentrations. This matches with the in situ seasonal patterns of temperature and phosphate concentrations (Figures 4B,C). The dispersion statistic of the model was 1.21, indicating some overdispersion. This means that significant results may not always appear significant in the model and that other variables can also potentially influence the seasonality of N. scintillans. A clear autocorrelation trend could be discerned for temperature, and correlations for small lags for N. scintillans abundance and PO4 were detected by the ACF, possible indicating time correlation. However, these were not taken into account in M1. The RDA ordination matched with the correlations found in M2 and indicate that N. scintillans was negatively correlated with phosphate concentrations and strongly positively correlated with temperature, which was visually represented in the RDA analysis bi-plot (Figure 5). It also showed a seasonal effect with a separation of e.g., the winter and summer season. The percentage of explained variance and the correlation coefficients with environmental factors are given in Supplementary Material 3.
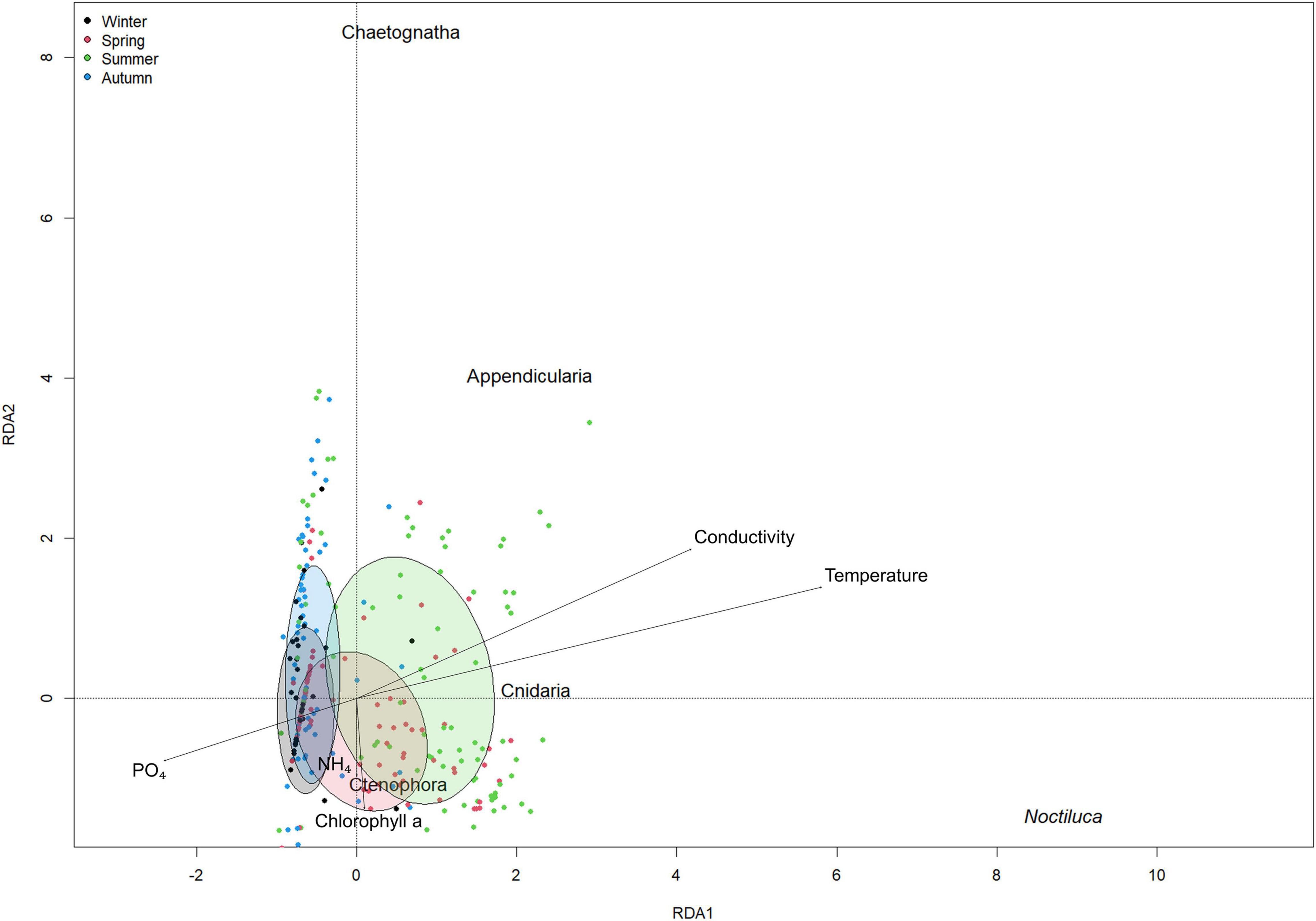
Figure 5. Redundancy analysis plot for environmental variable vectors and for N. scintillans, Appendicualira, Cnidaria, Ctenophora, and Chaetognatha. Dots and ellipses in black: winter months (December, January, February), red: spring months (March, April, May), green: summer months (June, July, August) and blue: autumn months (September, October, November).
Seasonal and Inter-Annual Variations of Noctiluca scintillans Cell Size
The cell size of N. scintillans ranged between 261 and 1,121 μm over the study period from 2014 to 2018, with smaller mean cell sizes in December and larger ones in May and June (Figure 6). The averaged monthly cell size variation for the 5-year period depicted an increase in mean cell size during the spring season, from 443 μm in January to 475 μm in April, which was subsequently followed by a peak during late spring/early summer (571 and 555 μm in May and June, respectively). At the end of summer and autumn (July–November) the mean cell size ranged between 419 and 480 μm. In December, a decrease of the mean cell size could be observed with a mean cell size of 379 μm. These monthly differences in cell size can also be clearly observed from the ZooScan images of N. scintillans in Figures 7A,B.
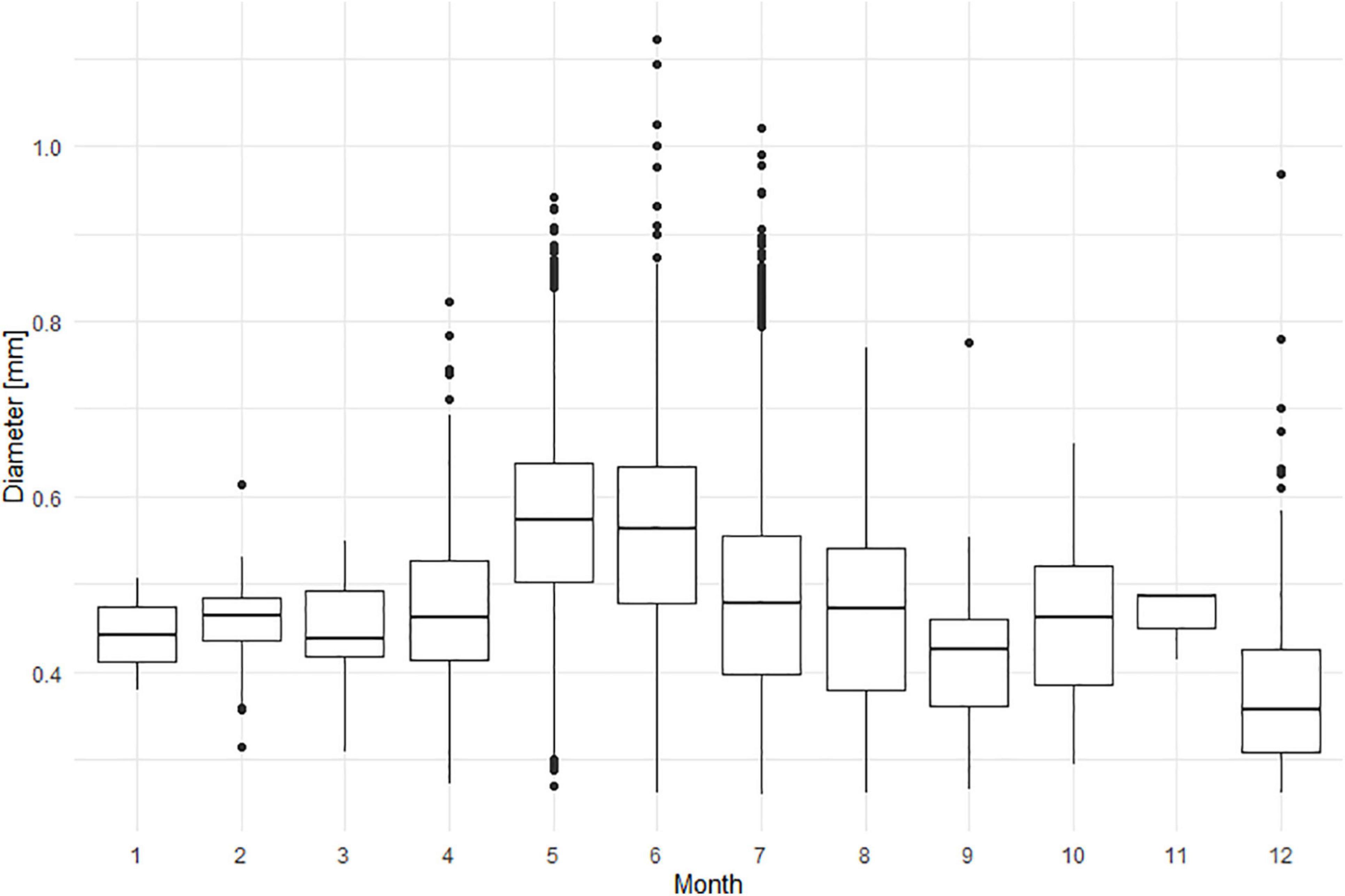
Figure 6. Diameter [mm] of individual N. scintillans cells per month, in the studied period from 2014 to 2018.
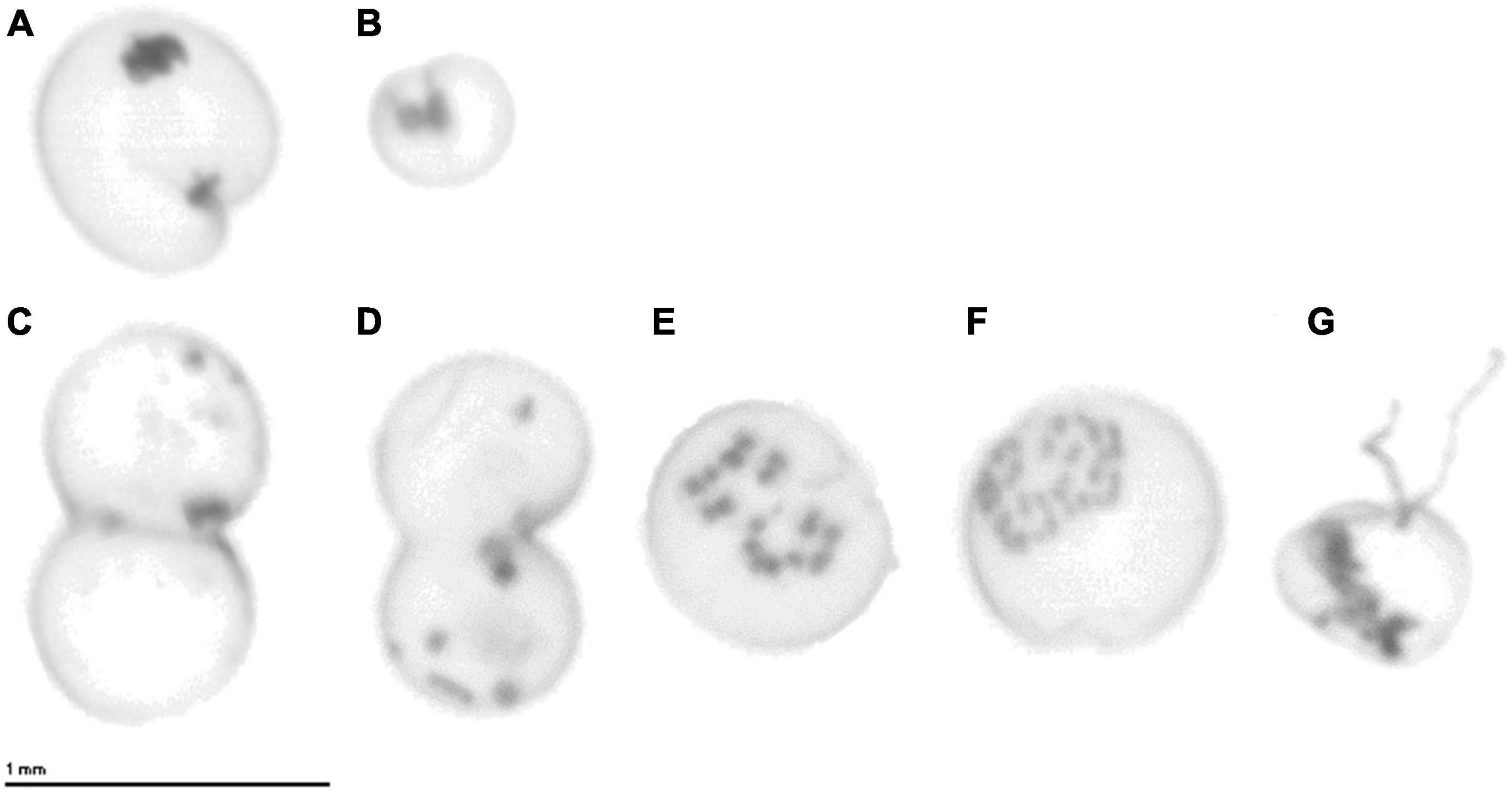
Figure 7. ZooScan images of individual N. scintillans cells from (A) May 2016 and (B) November 2016. ZooScan images of N. scintillans cells performing (C,D) binary fission and (E–G) sexual reproduction. (E,F) Progamete stages and (G) isogamete stage with two flagella.
A Kruskal-Wallis test (Supplementary Material 4) showed that the cell diameter was highly variable between years (p < 0.05) and months (p < 0.001), but also between stations (p < 0.05). Year 2014 significantly differed from 2017 and 2018; and 2016 differed from 2015 and 2017. May and June significantly differed from February, March, July, August, September and December; and the month December differed from April. Station 330 significantly differed from station 130, 230, and 700.
Seasonal Variations of Noctiluca scintillans and Soft-Bodied Plankton
A majority of the soft-bodied taxa considered in this study exhibited a distinguishable seasonal trend (Figure 8) with low abundances in winter, which increased at the end of spring/early summer, although subtle differences were observable among taxa. Ctenophora tended to peak before N. scintillans, whereas Chaetognatha experienced a later peak. For Cnidaria it was unclear when its peak occurred. The seasonal trend of Appendicularia appeared as the most similar to the one of N. scintillans with Appendicularia peaking at the end of spring and beginning of summer, just before the N. scintillans peaks. A ZINB model was drawn up for each taxon, and only Appendicularia covaried significantly with N. scintillans abundances (Supplementary Material 5). The best-fitted model found for Appendicularia was the following:
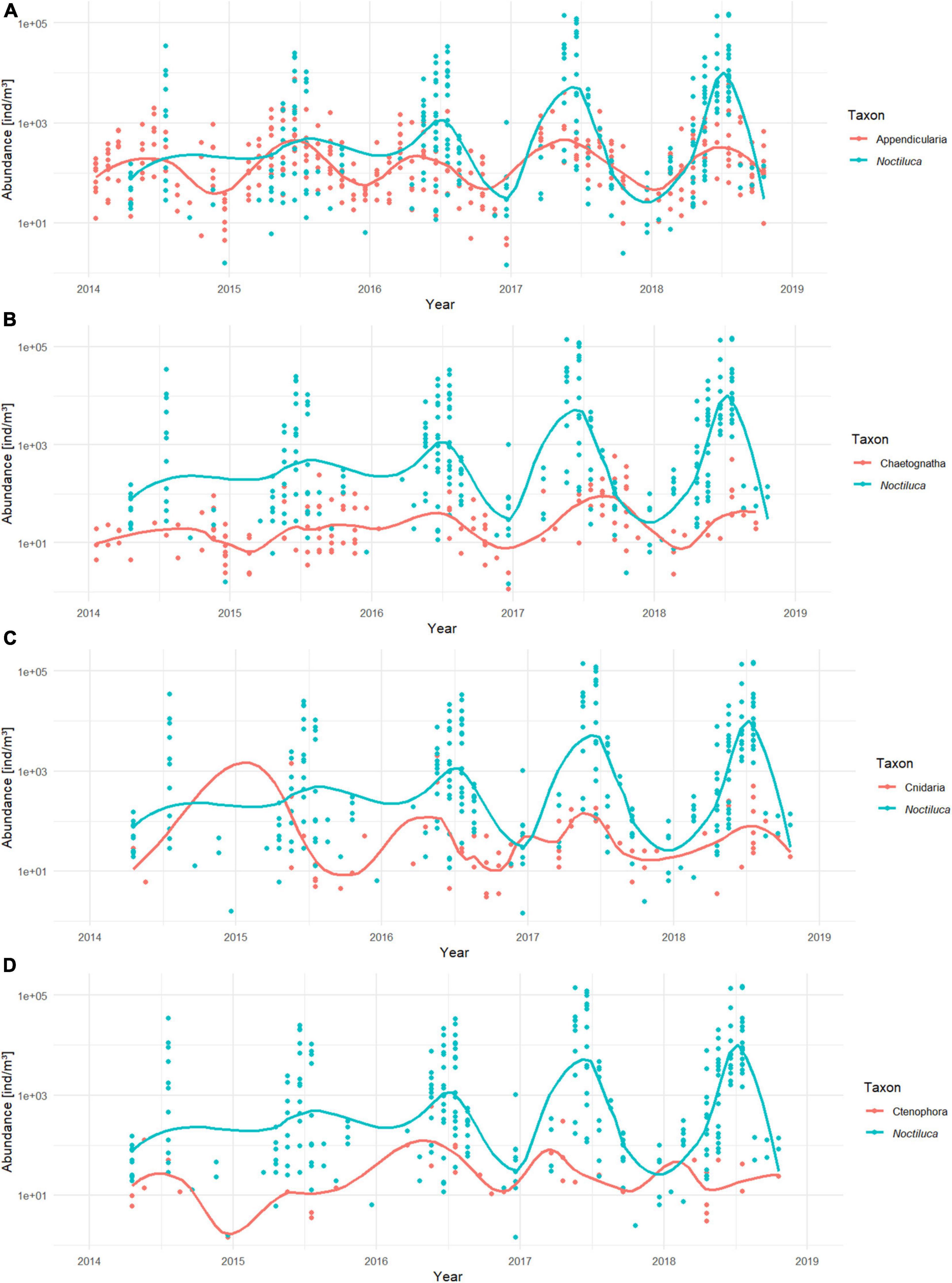
Figure 8. Time series of sampled abundances [ind/m3] of N. scintillans and (A) Appendicularia, (B) Chaetognatha, (C) Cnidaria, and (D) Ctenophora from 2014 to 2018. Noctiluca scintillans is represented in blue whereas the soft-bodied plankton taxa are orange. The solid lines are the smoothed conditional means.
Appendicularia ∼ N. scintillans + PO4 | 1 (M2)
The model reports that the abundances of Appendicularia positively covariated with N. scintillans abundances (p < 0.001) and negatively with phosphate concentrations (p < 0.001) and that both covariations were highly significant. The dispersion statistic for the model was 1.24, indicating overdispersion. Similarly to M1, correlations for small lags for N. scintillans abundance, Appendicualria abundance and PO4 were detected by the ACF. The RDA ordination shows the negative correlation of Appendicularia with phosphate concentrations and positive correlation with N. scintillans abundances (Figure 5 and Supplementary Material 2). The other soft-bodies species are represented as well on the RDA analysis bi-plot, which showed that NH4 and chlorophyll a concentrations were positively related to Ctenophora and negatively to Chaetognatha. Cnidaria showed a positive correlation with temperature and conductivity, and a negative one with phosphate concentrations.
Discussion
Seasonal and Interannual Dynamics of Noctiluca scintillans
N. scintillans has a worldwide distribution with, in general, a density peak in spring or summer and in some cases an additional lower peak in another season (Harrison et al., 2011). In the German Bight in the North Sea, N. scintillans was reported to usually peak in spring (March to late June), after which its abundances decrease significantly in August. In winter, a small population remains (Uhlig and Sahling, 1990). Similar seasonal patterns have been observed in the Dutch North Sea (Kat, 1979; Zevenboom et al., 1991) and in areas near Helgoland in the North Sea (Uhlig and Sahling, 1985). The seasonal dynamics of N. scintillans in the BPNS was consistent with the seasonal dynamics found in other parts of the North Sea and other parts of the world: a large peak at the end of spring/early summer (June-July) and a small winter population in December. Additionally, the important abundance decrease in August after the peak was concurrent with the findings of Uhlig and Sahling (1990) and Miyaguchi et al. (2006).
In the BPNS, N. scintillans cell size demonstrated a seasonal pattern characterized by larger sizes in May-June (555–571 μm) and smaller ones in December (379 μm). The concurrence of the largest cell sizes and abundance peak in spring/summer contradicts the findings of Miyaguchi et al. (2006) who noted an increase in N. scintillans abundance concurrent with a decrease in cell volume during the spring period. Miyaguchi et al. (2006) hypothesized that the small volume and large abundances of N. scintillans might be an indication that cells actively conduct binary fission during bloom formation. The ZooScan recorded images of N. scintillans performing both binary fission and sexual reproduction (Figure 7) in May, June, and July of the covered sampling period, despite the relative large size of the cells during these months. This confirms that cells do indeed conduct binary fission during abundance maxima, but more importantly, our results highlight that this does not exclusively occur when cell size is small or decreasing. In general, cell size has been considered as a valuable indicator of the condition of N. scintillans population. When small, the cell is considered in a good nutritional status and capable of population growth, while a large cell has been considered as in a poor condition (Murray and Suthers, 1999; Dela-Cruz et al., 2003). The size of what is defined “small” or “large” ranges between 340 and 525 μm and 400 and 1,200 μm, respectively, depending on the study. Dela-Cruz et al. (2003) designated the cutoff boundary at 525 μm, based on the modal cell-size class determined by the total number of cells measured in their study. Although cell size was larger in spring/summer in our study, a considerable part of the N. scintillans cells in the BPNS were still smaller than this cutoff value. Therefore, a large part of the N. scintillans population were most likely healthy and capable of population growth, allowing for a high spring/summer bloom as it was observed.
In a timespan of only 5 years, the occurrence of N. scintillans in the samples nearly tripled, which could not be attributed to the sampling effort (Table 1). High abundance peaks also increased in cell concentration values over the covered period (Figure 4A). This increasing trend differs from the 3-year interval oscillations observed over 20 years in the German Bight (Uhlig and Sahling, 1990), whereby a year with a relatively high abundance was followed by 2 years of relatively low abundances. In the BPNS, both 2017 and 2018 were characterized by high N. scintillans abundances. The highest abundance peak was observed in July 2018 with densities rising up to 290,181 ind/m3. The peak densities of N. scintillans found in spring/summer in the present dataset were higher than previous peak densities reported in the BPNS: Van Ginderdeuren et al. (2014) observed a maximum of 39,800 ind/m3 in July–August between 2009 and 2010, and Daro et al. (2006) reported peak maxima of 16,000 ind/m3 in the period from 1988 to 2004. It should be noted, however, that these authors used a different methodology, which hampers direct comparisons of peak densities with our measurements. Annual maximum densities can differ strongly among methodologies, but also among years as shown by the high inter-annual variability in N. scintillans reported by Uhlig and Sahling (1990). Thus, while our observations suggest an increasing trend in N. scintillans over the years, further observations to evaluate and confirm this trend are necessary. The present peak density values in the end of spring/summer were 30–300 times lower than densities reported in the German Bight from 1968 to 1988 (∼107–108 ind/m3; Uhlig and Sahling, 1990). Such a difference could partly be attributed to the fact that N. scintillans in the German Bight was sampled with bottle samplers near the water surface (0.5 and 5 m depth), where it has been shown to accumulate due to its positive buoyancy (Omori and Hamner, 1982; Uhlig and Sahling, 1985). Noctiluca scintillans cells normally are positively buoyant due to their large cell vacuoles filled with ammonium ions (Elbrachter and Qi, 1998). They only tend to sink to the bottom if they are well fed, where they remain until digestion and defecation have taken place (Omori and Hamner, 1982). Observations made during VLIZ sampling campaigns support the accumulation in the surface layer, where N. scintillans aggregated in the top layer of water samples. Additionally, data collected in May 2020 with a Video Plankton Recorder in the BPNS showed that while N. scintillans was present in the whole water column, its densities were 4.5 times higher in the upper layers (personal observations). The magnitude of the difference is still too large to be attributed to sampling depth alone, and it is likely that regional and temporal factors also contributed to this difference (Uhlig and Sahling, 1990).
Biotic and Abiotic Factors Driving the Dynamics of Noctiluca scintillans
Temperature and phosphate concentrations are potential factors shaping the dynamics of N. scintillans, as suggested by the present results. Temperature is known to play an important role in the reproduction and lifecycle of many plankton species (Richardson, 2008) and the presence of N. scintillans has been correlated to temperature in previous studies (Tada et al., 2004; Jang et al., 2010). Noctiluca scintillans has been reported to occur over large temperature ranges from temperatures below 0–30°C. The maximum growth rate measured was found at 23–24°C in laboratory settings (Uhlig and Sahling, 1985; Lee and Hirayama, 1992). Higher temperatures, above 25°C (Nakamura, 1998; Liu and Wong, 2006), 28°C (Jang et al., 2010), and 30°C (Qi et al., 2004), were shown to decrease the growth rate of the species and were referred to as the cause of the decline or disappearance of the species. In the BPNS, water temperatures above 25°C have been rarely observed and therefore, a decline of N. scintillans abundance due to temperature increase is unlikely to occur. The maximum observed temperature in the dataset was 22.32°C in July 2018. It actually shows that summer temperatures in the BPNS are in line with the optimal growth conditions for N. scintillans populations.
In the BPNS, high nutrient concentrations are generally observed in winter, while in spring (April–May), phosphate concentrations are low (Van der Zee et al., 2007). Our results for the period 2014–2018 match the typical seasonal dynamics in phosphate concentrations. The ZINB model highlighted that phosphate negatively correlated with N. scintillans abundance, which is concurrent with the findings of Mohamed and Mesaad (2007) in the Red Sea. Noctiluca scintillans abundance was potentially indirectly linked to phosphate concentrations through food availability. Usually, low phosphate concentrations in spring are an indication of phytoplankton uptake as the bloom develops which, in turn, forms an abundant food source for N. scintillans (Sahayak et al., 2005). This is in accordance with other parts of the world where N. scintillans has been shown to often bloom after eutrophication-induced phytoplankton blooms (Painting et al., 1993; Hayward et al., 1995; Mohamed and Mesaad, 2007).
When framing our observations in a broader context of general dinoflagellate dynamics over the past decades in the North Sea, similarities can be seen regarding trends and drivers. Nohe et al. (2020) stated that in the BPNS the total abundance and biovolume of dinoflagellates significantly increased from 1970s to the 2000s and that dinoflagellates increased year-round, although more specifically in summer. Climate warming was identified as one of the possible drivers for the overall increase of dinoflagellates in summer in the 2000s, as dinoflagellates are generally associated with warmer conditions (Baretta-Bekker et al., 2009). The capacity of many dinoflagellates for mixotrophic growth, and more specifically their ability to access alternative phosphorous sources, may have also contributed to their dominance during summer when nutrient levels were at their lowest (Burson et al., 2016). Our results, despite covering a shorter time series of 5 years, are thus in line with the trends and potential drivers proposed by Nohe et al. (2020). Although N. scintillans in the BPNS is not considered mixotrophic, it predates on a wide spectrum of particles and organisms, from several μm to 600–800 μm (Nikishina et al., 2011), and is therefore not dependent on one food source (Schaumann et al., 1988; Kirchner et al., 1996; Quevedo et al., 1999). Diatoms, Phaeocystis aggregates, copepod eggs and molts, fecal pellets and various protists such as dinoflagellates, ciliates and suctorians were reported in the food vacuoles of N. scintillans in the BPNS (Daro et al., 2006). The positive trend in abundance and peak intensity of N. scintillans over the studied period is most likely the result of a combination of factors: the ability of the species to predate on a wide food range leading to a competitive food advantage over many other zooplankton species, its optimum growth rate concordant with warmer temperatures in the BPNS in summer, as well as its ability to multiply quickly rendering it highly competitive and most likely explain its positive trend in abundance and peak intensity over the studied period.
Noctiluca scintillans in Relation to Soft-Bodied Plankton
The dynamics of N. scintillans has previously been correlated to the dynamics of other zooplankton species. Several studies observed inverse relationships with copepod abundance and the biomass of the overall zooplankton community, most likely due to the predation of N. scintillans on zooplankton itself and on their eggs as well as due to the competition for similar food sources (Fonda Umani et al., 2004; Yılmaz et al., 2005; Isinibilir et al., 2008). Negative interactions were also suggested between N. scintillans and small soft-bodied plankton species, such as Pleurobrachia pileus (Ctenophora), Pleurobrachia bachei (Ctenophora), chaetognaths (Chaetognatha), Rathkea octopunctata (Cnidaria) and Lizzia blondina (Cnidaria) (Heyen et al., 1998; Kovalev and Piontkovski, 1998; Fock and Greve, 2002). In the Black Sea, significant negative inter-annual correlations between N. scintillans and Pleurobrachia bachei were found (Kovalev and Piontkovski, 1998), whereas Fock and Greve (2002) found a strong indication that interactions occurred between N. scintillans, Pleurobrachia pileus, chaetognaths, and hydromedusae. Heyen et al. (1998) observed that a late timing or high abundances of P. pileus led to late timing and low abundances of N. scintillans. They concluded that the timing of N. scintillans not only depends on sea surface temperature, but also on the timing and abundance of P. pileus. Such a conclusion could not be supported by our results, as N. scintillans did not correlate with Ctenophora, nor with Chaetognatha or Cnidaria. In case the plankton groups used in this study contained plankton species with very different dynamics, then it might be possible that species-specific relationships of soft-bodied species with N. scintillans were not detected due to the inability of the ZooScan to identify organisms to species level. On the other hand, the ZINB model indicated that appendicularians were significantly positively correlated with N. scintillans, contrary to what was expected. It is most likely that the dynamics of Appendicularia is governed by similar factors as N. scintillans dynamics, rather than N. scintillans having a direct positive effect on the species, especially considering that N. scintillans peaks later than Appendicularia. Studies found that certain phytoplankton blooms (of e.g., Coccolithophores or Phaeocystis) had a strong positive effect on the nutrition of both N. scintillans and Appendicularia (Lancelot et al., 2002; Amelina et al., 2017). Beside the raptorial feeding habit, N. scintillans is also capable of producing mucoid strands that bind suspended particles, small diatoms and other algae in a non-selective manner (Kiϕrboe and Titelman, 1998). Appendicularia on the other hand feed within a mucous house that processes large volumes of water and concentrates small particles inside the house prior to feeding (Deibel, 1986). These two taxa are both considered fine particle feeders (Amelina et al., 2017) and their similar trends in dynamics might be linked to their ability for non-selective food uptake.
Conclusion
This study is the first one to provide consistent counts of N. scintillans cell concentrations as well as measured cell lengths for the region of the BPNS, which demonstrates that imaging methods such as the ZooScan are a powerful tool to monitor and study the N. scintillans population. Although N. scintillans occurred more frequently and in higher abundances in the most recent years of our time series, no negative impact of this species was observed on the small soft-bodied plankton community. The results of this study show that temperature and phosphate concentrations are potential drivers of the dynamics of N. scintillans in the BPNS, factors which can be influenced by anthropogenic pressures. The presence and density of N. scintillans are likely to increase in the future, notably due to global warming with warmer water temperatures (IPCC, 2021) forming the ideal growth condition for the species. The potential of N. scintillans to negatively influence other plankton in addition to its increasing prominent role in the plankton community, underlines the importance of further studying its role and impact on marine food webs. While there is still a large number of knowledge gaps related to the poorly studied gelatinous organisms (Aubert et al., 2018), especially when it comes to their inter-relationships, the new era of advanced optical techniques can bring more insight. Imaging techniques such as the Video Plankton Recorder (Davis et al., 2004) or the Continuous Particle Imaging and Classification System (Gallager, 2016) enable to register and quantify the gelatinous plankton community more effectively than techniques based on net sampling, as fragile gelatinous species can be observed in situ without being damaged (Remsen et al., 2004). The use of in situ optical methods should be thus encouraged for poorly studied gelatinous species as it could bring a major contribution to future research into the dynamics, distribution and impact of species such as N. scintillans.
Data Availability Statement
Publicly available datasets were analyzed in this study. The datasets used in this study are: Flanders Marine Institute (VLIZ), Belgium (2019a): LifeWatch observatory data: zooplankton observations in the Belgian part of the North Sea (https://doi.org/10.14284/329) and Flanders Marine Institute (VLIZ), Belgium (2019b): LifeWatch observatory data: nutrient, pigment, suspended matter and secchi measurements in the Belgian part of the North Sea (https://doi.org/10.14284/328).
Author Contributions
AO: processing of the data, statistical analyses, and writing. JM: processing of the data, project supervision, and reviewing. AA: reviewing. KD: project supervision. MV: project supervision and reviewing. All authors contributed to the article and approved the submitted version.
Funding
This work was primarily financed by the Flanders Marine Institute (VLIZ). It makes use of data and infrastructure provided by the VLIZ and funded by the Research Foundation—Flanders (FWO) as part of the Belgian contribution to the LifeWatch European Research Infrastructure (I000819N-LIFEWATCH and I002021N-LIFEWATCH).
Conflict of Interest
The authors declare that the research was conducted in the absence of any commercial or financial relationships that could be construed as a potential conflict of interest.
Publisher’s Note
All claims expressed in this article are solely those of the authors and do not necessarily represent those of their affiliated organizations, or those of the publisher, the editors and the reviewers. Any product that may be evaluated in this article, or claim that may be made by its manufacturer, is not guaranteed or endorsed by the publisher.
Acknowledgments
We wish to thank the crew of the RV Simon Stevin, as well as the many students and interns who have been processing the zooplankton samples with the ZooScan over the years.
Supplementary Material
The Supplementary Material for this article can be found online at: https://www.frontiersin.org/articles/10.3389/fmars.2021.777999/full#supplementary-material
References
Amelina, A. B., Sergeeva, V. M., Arashkevich, E. G., Drits, A. V., Louppova, N. E., and Solovyev, K. A. (2017). Feeding of the dominant herbivorous plankton species in the black sea and their role in coccolithophorid consumption. Oceanology 57, 806–816. doi: 10.1134/s000143701706011x
Aubert, A., Antajan, E., Lynam, C., Pitois, S., Pliru, A., Vaz, S., et al. (2018). No more reason for ignoring gelatinous zooplankton in ecosystem assessment and marine management: concrete cost-effective methodology during routine fishery trawl surveys. Mar. Policy 89, 100–108. doi: 10.1016/j.marpol.2017.12.010
Baretta-Bekker, J. G., Baretta, J. W., Latuhihin, M. J., Desmit, X., and Prins, T. C. (2009). Description of the long-term (1991–2005) temporal and spatial distribution of phytoplankton carbon biomass in the Dutch North Sea. J. Sea Res. 61, 50–59. doi: 10.1016/j.seares.2008.10.007
Bologa, A. S., Bodeanu, N., Petran, A., Tiganus, V., and Zaitsev, Y. P. (1995). Major modifications of the Black Sea benthic and planktonic biota in the last three decades. Bull. Inst. Oceanogr. 1, 85–110.
Boni, L. (1983). Red tides of the coast of Emilia Romagna (north-western Adriatic sea) from 1975 to 1982. Inf. Bot. Ital. 15, 18–24.
Burson, A., Stomp, M., Akil, L., Brussaard, C. P. D., and Huisman, J. (2016). Unbalanced reduction of nutrient loads has created an offshore gradient from phosphorus to nitrogen limitation in the North Sea. Limnol. Oceanogr. 61, 869–888. doi: 10.1002/lno.10257
Cataletto, B., Feoli, E., Fonda Umani, S., and Cheng-Yong, S. (1995). Eleven years of time-series analysis on the net-zooplankton community in the Gulf of Trieste. ICES J. Mar. Sci. 52, 669–678. doi: 10.1016/1054-3139(95)80080-8
Daro, M. H., Breton, E., Antajan, E., Gasparini, S., and Rousseau, V. (2006). “Do Phaeocystis colony blooms affect zooplankton in the Belgian coastal zone?” in Current Status of Eutrophication in the Belgian Coastal Zone, eds V. Rousseau et al. (Oostende: Flanders Marine Institute (VLIZ)) 61–72. doi: 10.1016/0077-7579(86)90061-x
Davis, C. S., Hu, Q., Gallager, S. M., Tang, X., and Ashjian, C. J. (2004). Real-time observation of taxa-specific plankton distributions: an optical sampling method. Mar. Ecol. Prog. Ser. 284, 77–96.
Degobbis, D., Fonda-Umani, S., Franco, P., Malej, A., Precali, R., and Smodlaka, N. (1995). Changes in the northern Adriatic ecosystem and the hypertrophic appearance of gelatinous aggregates. Sci. Total Environ. 165, 43–58. doi: 10.1016/0048-9697(95)04542-9
Deibel, D. (1986). Feeding mechanism and house of the appendicularian Oikopleura vanhoeffeni. Mar. Biol. 93, 429–436. doi: 10.1007/bf00401110
Dela-Cruz, J., Middleton, J. H., and Suthers, I. M. (2003). Population growth and transport of the red tide dinoflagellate, Noctiluca scintillans, in the coastal waters off Sydney Australia, using cell diameter as a tracer. Limnol. Oceanogr. 48, 656–674. doi: 10.4319/lo.2003.48.2.0656
Elbrachter, M., and Qi, Y. Z. (1998). “Aspects of Noctiluca (Dinophyceae) population dynamics,” in Physiological Ecology of Harmful Algal Blooms. NATO ASI Series, eds D. M. Anderson, A. D. Cembella, and G. M. Hallegraeff (Berlin: Springer-Verlag), 315–335.
Enomoto, Y. (1956). On the Occurrence and the Food of Noctiluca Scintillans (Macartney) in the Waters Adjacent to the West Coast of Kyushu, with Special Reference to the Possibility of the Damage Caused to the Fish Eggs by That Plankton. Nippon Suisan Gakkaishi 22, 82–88. doi: 10.2331/suisan.22.82
Faust, M. A., and Gulledge, R. A. (2002). Identifying Harmful Marine Dinoflagellates. Washington, DC: Smithsonian Institution.
Fettweis, M. P., and Nechad, B. (2011). Evaluation of in situ and remote sensing sampling methods for SPM concentrations, Belgian continental shelf (southern North Sea). Ocean Dyn. 61, 157–171. doi: 10.1007/s10236-010-0310-6
Flanders Marine Institute (VLIZ) (2019a). LifeWatch observatory data: zooplankton observations in the Belgian Part of the North Sea. Geosci. Data J. 6, 76–84. doi: 10.3897/BDJ.8.e57236
Flanders Marine Institute (VLIZ) (2019b). LifeWatch Observatory Data: Nutrient, Pigment, Suspended Matter and Secchi Measurements in the Belgian Part of the North Sea. (Oostende: Flanders Marine Institute (VLIZ)).
Fock, H. O., and Greve, W. (2002). Analysis and interpretation of recurrent spatio-temporal patterns in zooplankton dynamics: a case study on N. scintillans in the German Bight. Mar. Biol. 140, 59–73. doi: 10.1007/s002270100685
Fonda Umani, S., Beran, A., Parlato, S., Virgilio, D., Zollet, T., De Olazabal, A., et al. (2004). N. scintillans Macartney in the Northern Adriatic Sea: long-term dynamics, relationships with temperature and eutrophication, and role in the food web. J. Plankton Res. 26, 545–561. doi: 10.1093/plankt/fbh045
Gallager, S. M. (2016). The Continuous Plankton Imaging and Classification Sensor (CPICS): a sensor for quantifying Mesoplankton Biodiversity and Community Structure. Am. Geophys.Union 2016, 52A–57A.
Glibert, P., Anderson, D., Gentien, P., Granéli, E., and Sellner, K. (2005). The Global, Complex Phenomena of Harmful Algal Blooms. Oceanography 18, 136–147. doi: 10.5670/oceanog.2005.49
Goffin, A., Van den Bergh, E., Meire, P., Mostaert, F., Lescrauwaet, A. K., and Pirlet, H. (2015). “Scheldt Estuary,” in Compendium for Coast and Sea 2015: An Integrated Knowledge Document about the Socio-Economic, Environmental and Institutional Aspects of the Coast and Sea in Flanders and Belgium, eds H. Pirlet, et al. (Oostende: Flanders Marine Institute (VLIZ)), 233–242.
Gorsky, G., Ohman, M. D., Picheral, M., Gasparini, S., Stemmann, L., Romagnan, J. B., et al. (2010). Digital zooplankton image analysis using the ZooScan integrated system. J. Plankton Res. 32, 285–303.
Granéli, E., and Turner, J. T. (2006). “An Introduction to Harmful Algae,” in Ecology of Harmful Algae. Ecological Studies (Analysis and Synthesis), Vol. 189, eds E. Granéli and J. T. Turner (Berlin: Springer), 3–7.
Grosjean, P., Picheral, M., Warembourg, C., and Gorsky, G. (2004). Enumeration, measurement, and identification of net zooplankton samples using the ZOOSCAN digital imaging system. ICES J. Mar. Sci. 61, 518–525. doi: 10.1016/j.icesjms.2004.03.012
Harrison, P. J., Furuya, K., Glibert, P. M., Xu, J., Liu, H. B., Yin, K., et al. (2011). Geographical distribution of red and green Noctiluca scintillans. Chin. J. Oceanol. Limnol. 29, 807–831. doi: 10.1007/s00343-011-0510-z
Hayward, T. L., Cayan, D. R., Franks, P. J. S., Lynn, R. J., Mantyla, A. W., McGowan, J. A., et al. (1995). The State of the California Current in 1994–1995, a Period of Transition. CalCOFI Rep., 36, 19–39.
Heyen, H., Fock, H., and Greve, W. (1998). Detecting relationships between the interannual variability in ecological time series and climate using a multivariate statistical approach – a case study on Helgoland Roads zooplankton. Clim. Res. 10, 179–191. doi: 10.3354/cr010179
Holligan, P. M. (1979). “Dinoflagellate blooms associated with tidal fronts around the British Isles,” in Toxic Dinoflagellate Blooms in North Holland, eds D. L. Taylor and H. H. Seliger (New York, NY: Elsevier), 249–256.
Huang, C., and Qi, Y. (1997). The abundance cycle and influence factors on red tide phenomena of Noctiluca scintillans (Dinophyceae) in Dapeng Bay, the South China Sea. J. Plankton Res. 19, 303–318. doi: 10.1093/plankt/19.3.303
IPCC (2021). “Summary for Policymakers,” in Climate Change 2021: The Physical Science Basis. Contribution of Working Group I to the Sixth Assessment Report of the Intergovernmental Panel on Climate Change, eds V. Masson-Delmotte, P. Zhai, A. Pirani, S. L. Connors, C. Péan, S. Berger, et al. (Cambridge: Cambridge University Press).
Isinibilir, M., Kideys, A. E., Tarkan, A. N., and Yilmaz, I. N. (2008). Annual cycle of zooplankton abundance and species composition in Izmit Bay (the northeastern Marmara Sea). Estuar. Coast. Shelf Sci. 78, 739–747. doi: 10.1016/j.ecss.2008.02.013
Jang, M.-C., Shin, K.-S., Jang, P.-G., and Lee, W.-J. (2010). Relationship between Environmental Factors and Short-term Variations of Mesozooplankton During Summer in Jangmok Bay, South Coast of Korea. Ocean Polar Res 32, 41–52. doi: 10.4217/opr.2010.32.1.041
Kat, M. (1979). Toxic Dinoflagellate Blooms. Developments in Marine Biology. (New York, NY: Elsevier), 215–220.
Kirchner, M., Sahling, G., Uhlig, G., Gunkel, W., and Klings, K. W. (1996). Does the red tide-forming dinoflagellate Noctiluca scintillans feed on bacteria? Sarsia 81, 45–55.
Kiϕrboe, T., and Titelman, J. (1998). Feeding, prey selection and prey encounter mechanisms in the heterotrophic dinoflagellate Noctiluca scintillans. J. Plankton Res 20, 1615–1636. doi: 10.1093/plankt/20.8.1615
Kovalev, A. V., and Piontkovski, S. A. (1998). Interannual changes in the biomass of the Black Sea gelatinous zooplankton. J. Plankton Res. 20, 1377–1385. doi: 10.1093/plankt/20.7.1377
Lacroix, G., Ruddick, K., Ozer, J., and Lancelot, C. (2004). Modelling the impact of the Scheldt and Rhine/Meuse plumes on the salinity distribution in Belgian waters (southern North Sea). J. Sea Res. 52, 149–163. doi: 10.1016/j.seares.2004.01.003
Lancelot, C., Keller, M., Rousseau, V., Smith, W. O. Jr., and Mathot, S. (1998). “Autecology of the marine Haptophyte Phaeocystis sp,” in Physiological Ecology of Harmful Algal Blooms. NATO ASI Series G: Ecological sciences, Vol. 41, eds D. M. Anderson, et al. (Brussels: NATO), 209–224.
Lancelot, C., Rousseau, V., Becquevort, S., Parent, J. Y., Breton, E., Déliat, C., et al. (2002). “Study and modeling of eutrophication-related changes in coastal planktonic food–webs: a contribution of the AMORE (Advanced Modeling and Research on Eutrophication) consortium,” in Sustainable Management of the North Sea: Presentation of Research Results, 21-22/01/2002.
Lee, J. K., and Hirayama, K. (1992). Effects of salinity, food level and temperature on the population growth on Noctiluca scintillans (Macarthy). Bull. Fac. Fish. Nagasaki Univ. 71, 163–168.
Liu, X.-J., and Wong, C. K. (2006). Seasonal and spatial dynamics of Noctiluca scintillans in a semi-enclosed bay in the northeastern part of Hong Kong. Bot. Mar. 49, 145–150. doi: 10.1515/bot.2006.019
Miyaguchi, H., Fujiki, T., Kikuchi, T., Kuwahara, V. S., and Toda, T. (2006). Relationship between the bloom of Noctiluca scintillans and environmental factors in the coastal waters of Sagami Bay, Japan. J. Plankton Res. 28, 313–324. doi: 10.1093/plankt/fbi127
Mohamed, Z. A., and Mesaad, I. (2007). First report on Noctiluca scintillans blooms in the Red Sea off the coasts of Saudi Arabia: consequences of eutrophication. Oceanologia 49, 337–351.
Moore, S. K., Trainer, V. L., Mantua, N. J., Parker, M. S., Laws, E. A., Backer, L. C., et al. (2008). Impacts of climate variability and future climate change on harmful algal blooms and human health. Environ. Health 7(Suppl. 2):S4. doi: 10.1186/1476-069x-7-s2-s4
Mortelmans, J., Deneudt, K., Cattrijsse, A., Beauchard, O., Daveloose, I., Vyverman, W., et al. (2019a). Nutrient, pigment, suspended matter and turbidity measurements in the Belgian part of the North Sea. Sci. Data 6:22. doi: 10.1038/s41597-019-0032-7
Mortelmans, J., Goossens, J., Amadei Martínez, L., Deneudt, K., Cattrijsse, A., and Hernandez, F. (2019b). LifeWatch observatory data: zooplankton observations in the Belgian part of the North Sea. Geosci. Data J. 6, 76–84. doi: 10.1002/gdj3.68
Murray, S., and Suthers, I. M. (1999). Population ecology of Noctiluca scintillans Macartney, a red-tide-forming dinoflagellate. Mar. Freshw. Res. 50, 243–252. doi: 10.1071/mf98052
Nakamura, Y. (1998). Biomass, feeding and production of Noctiluca scintillans in the Seto Inland Sea, Japan. J. Plankton Res. 20, 2213–2222. doi: 10.1093/plankt/20.11.2213
Nakamura, Y., and Hirata, A. (2006). Plankton community structure and trophic interactions in a shallow and eutrophic estuarine system, Ariake Sound, Japan. Aquat. Microb. Ecol. 44, 45–57. doi: 10.3354/ame044045
Nihoul, J. C. J., and Hecq, J. H. (1984). Influence of the residual circulation on the physico-chemical characteristics of water masses and the dynamics of ecosystems in the Belgian coastal zone. Cont. Shelf Res. 3, 167–174. doi: 10.1016/0278-4343(84)90005-0
Nikishina, A. B., Drits, A. V., Vasilyeva, Y. V., Timonin, A. G., Solovyev, K. A., Ratkova, T. N., et al. (2011). Role of the Noctiluca scintillans population in the trophic dynamics of the Black Sea plankton over the spring period. Oceanology 51, 1029–1039. doi: 10.1134/s0001437011060129
Nohe, A., Goffin, A., Tyberghein, L., Lagring, R., De Cauwer, K., Vyverman, W., et al. (2020). Marked changes in diatom and dinoflagellate biomass, composition and seasonality in the Belgian Part of the North Sea between the 1970s and 2000s. Sci. Total Environ. 716:136316. doi: 10.1016/j.scitotenv.2019.136316
Okaichi, T., and Nishio, S. (1976). Identification of ammonia as the toxic principle of red tide of Noctiluca miliaris. Bull. Plankton Soc. Jpn. 23, 75–80.
Omori, M., and Hamner, W. M. (1982). Patchy distribution of zooplankton: Behavior, population assessment and sampling problems. Mar. Biol. 72, 193–200. doi: 10.1007/bf00396920
Painting, S., Lucas, M., Peterson, W., Brown, P., Hutchings, L. Mitchell-lnnes, B., et al. (1993). Dynamics of bacterioplankton, phytoplankton and mesozooplankton communities during the development of an upwelling plume in the southern Benguela. Marine Ecol. Prog. Ser. 100, 35–53. doi: 10.3354/meps100035
Polishchuk, L. V., and Ghilarov, A. M. (1981). Comparison of two approaches used to calculate zooplankton mortality. Limnol. Oceanogr. 26, 1162–1168. doi: 10.4319/lo.1981.26.6.1162
Porumb, F. (1992). “On the development of Noctiluca scintillans under eutrophication of Romanian Black Sea waters,” in Marine Coastal Eutrophication, eds R. A. Vollenweider, R. Marchetti, and R. Viviani (Amsterdam: Elsevier), 907–920. doi: 10.1016/b978-0-444-89990-3.50078-x
Qi, Y., Chen, J., Wang, Z., Xu, N., Wang, Y., Shen, P., et al. (2004). Some observations on harmful algal bloom (HAB) events along the coast of Guangdong, southern China in 1998. Hydrobiologia 512, 209–214. doi: 10.1023/b:hydr.0000020329.06666.8c
Quevedo, M., Gonzalez-Quiros, R., and Anadon, R. (1999). Evidence of heavy predation by Noctiluca scintillans on Acartia clausi (Copepoda) eggs off the central Cantabrian coast (NW Spain). Oceanol. Acta 22, 127–131. doi: 10.1016/s0399-1784(99)80039-5
Remsen, A., Hopkins, T. L., and Samson, S. (2004). What you see is not what you catch: a comparison of concurrently collected net, Optical Plankton Counter, and Shadowed Image Particle Profiling Evaluation Recorder data from the northeast Gulf of Mexico. Deep Sea Res. I Oceanogr. Res. Pap. 51, 129–151. doi: 10.1016/j.dsr.2003.09.008
Richardson, A. J. (2008). In hot water: zooplankton and climate change. ICES J. Mar. Sci. 65, 279–295. doi: 10.1093/icesjms/fsn028
Sahayak, S., Jyothibabu, R., Jayalakshmi, K. J., Habeebrehman, H., Sabu, P., Prabhakaran, M. P., et al. (2005). Red tide of Noctiluca miliaris off south of Thiruvananthapuram subsequent to the ‘stench event’at the southern Kerala coast. Curr. Sci. 89, 1472–1473.
Schaumann, K., Gerdes, D., and Hesse, K. J. (1988). Hydrographic and biological characteristics of a N. scintillans red tide in the German Bight, 1984. Meeresforschung 32, 77–91.
Tada, K., Pithakpol, S., and Montani, S. (2004). Seasonal variation in the abundance of Noctiluca scintillans in the Seto Inland Sea, Japan. Plankton Biol. Ecol. 51, 7–14.
Thangaraja, M., Al-Aisry, A., and Al-Kharusi, L. (2007). Harmful algal blooms and their impacts in the middle and outer ROPME sea area. Int. J. Oceans Oceanogr. 2, 85–98.
Uhlig, G., and Sahling, G. (1985). Blooming and red tide phenomenon in Noctiluca scintillans. Bull. Mar. Sci. 37, 779–780.
Uhlig, G., and Sahling, G. (1990). Long-term studies on Noctiluca scintillans in the German Bight population dynamics and red tide phenomena 1968–1988. Neth. J. Sea Res. 25, 101–112. doi: 10.1016/0077-7579(90)90012-6
Van Bennekom, A. J., and Wetsteijn, F. J. (1990). The winter distribution of nutrients in the Southern Bight of the North Sea (1961–1978) and in the estuaries of the scheldt and the rhine/meuse. Neth. J. Sea Res. 25, 75–87. doi: 10.1016/0077-7579(90)90010-e
Van der Zee, C., Roevros, N., and Chou, L. (2007). Phosphorus speciation, transformation and retention in the Scheldt estuary (Belgium/The Netherlands) from the freshwater tidal limits to the North Sea. Mar. Chem. 106, 76–91. doi: 10.1016/j.marchem.2007.01.003
Van Ginderdeuren, K., Van Hoey, G., Vincx, M., and Hostens, K. (2014). The mesozooplankton community of the Belgian shelf (North Sea). J. Sea Res. 85, 48–58. doi: 10.1016/j.seares.2013.10.003
Vasas, V., Lancelot, C., Rousseau, V., and Jordán, F. (2007). Eutrophication and overfishing in temperate nearshore pelagic food webs: a network perspective. Mar. Ecol. Prog. Ser. 336, 1–14. doi: 10.3354/meps336001
Weston, K., Greenwood, N., Fernand, L., Pearce, D. J., and Sivyer, D. B. (2008). Environmental controls on phytoplankton community composition in the Thames plume, UK. J. Sea Res. 60, 246–254. doi: 10.1016/j.seares.2008.09.003
Yamamoto, T., Okai, M., Takeshita, K., and Hashimoto, T. (1997). Characteristics of meteorological conditions in the years of intensive red tide occurrence in Mikawa Bay, Japan. Bull. Jpn. Soc. Fish. Oceanogr. 61, 114–122.
Yang, Y., Sun, X., and Zhao, Y. (2016). Effects of Lugol’s iodine solution and formalin on cell volume of three bloom-forming dinoflagellates. Chin. J. Oceanol. Limnol. 35, 858–866. doi: 10.1007/s00343-017-5378-0
Yılmaz, İN., Okus, E., and Yüksek, A. (2005). Evidences for influence of a heterotrophic dinoflagellate (Noctiluca scintillans) on zooplankton community structure in a highly stratified basin. Estuar. Coast. Shelf Sci 64, 475–485. doi: 10.1016/j.ecss.2005.03.011
Zevenboom, W., Rademaker, M., and Colijn, F. (1991). Exceptional Algal Blooms in Dutch North Sea Waters. Water Sci. Technol. 24, 251–260. doi: 10.2166/wst.1991.0298
Zuur, A. F., and Ieno, E. N. (2016). Beginner’s Guide to Zero-Inflated Models with R. Newburgh: Highland Statistics Limited.
Keywords: Noctiluca, ZooScan, gelatinous zooplankton, time-series, Belgian coast, plankton blooms
Citation: Ollevier A, Mortelmans J, Aubert A, Deneudt K and Vandegehuchte MB (2021) Noctiluca scintillans: Dynamics, Size Measurements and Relationships With Small Soft-Bodied Plankton in the Belgian Part of the North Sea. Front. Mar. Sci. 8:777999. doi: 10.3389/fmars.2021.777999
Received: 16 September 2021; Accepted: 23 November 2021;
Published: 10 December 2021.
Edited by:
Helga Do Rosario Gomes, Lamont Doherty Earth Observatory (LDEO), United StatesReviewed by:
Akash R. Sastri, Institute of Ocean Sciences, CanadaSusumu Ohtsuka, Hiroshima University, Japan
Copyright © 2021 Ollevier, Mortelmans, Aubert, Deneudt and Vandegehuchte. This is an open-access article distributed under the terms of the Creative Commons Attribution License (CC BY). The use, distribution or reproduction in other forums is permitted, provided the original author(s) and the copyright owner(s) are credited and that the original publication in this journal is cited, in accordance with accepted academic practice. No use, distribution or reproduction is permitted which does not comply with these terms.
*Correspondence: Anouk Ollevier, anouk.ollevier@vliz.be