- Estonian Marine Institute, University of Tartu, Tallinn, Estonia
Coastal regions are biologically active areas with significant ecological and socioeconomic values. These regions are increasingly being affected by marine litter. The impact of macro-sized marine litter on biomass and net primary production of hard and soft bottom communities was investigated by using a manipulative field experiment. Plastic bags were used to mimic the disturbance caused by litter to benthic vegetation and fauna. The experiment was carried out on a soft substrate community dominated by sago pondweed Stuckenia pectinata (L.) Boerner and bladder wrack Fucus vesiculosus L. as a foundation species of a hard substrate. A rapid negative impact of the plastic bag cover on vegetation biomass of soft-bottom community was detected, while the impact on the biomass of hard bottom vegetation was non-significant. Plastic bag cover substantially reduced the net production rates of hard substrate species Fucus and the biomass of associated zoobenthos. The difference in net production rates of Stuckenia with and without plastic cover was negligible.
Introduction
Pollution of the oceans in the world by anthropogenic litter has become a serious global environmental concern. Macroplastic enters the marine environment through both marine (e.g., fishing, shipping, aquaculture, and tourism) and land (e.g., industry, sewage, tourism, and coastal littering) sources (Napper and Thompson, 2020). Plastic constitutes the major component of litter found in the marine environment with most of that comprised of single-use plastic such as bags and bottles (Castro-Jiménez et al., 2019; Rothäusler et al., 2019). These plastic materials are known to decompose slowly or not at all (Barboza et al., 2019; Napper and Thompson, 2019).
Typically, plastic waste initially floats on the sea surface as it consists of light polymeric material. Only a minority of plastic that floats along shorelines will eventually wash ashore. In contrast, the majority of plastic objects float until they either become too heavy from biota growing on their surface or because they become waterlogged resulting in sinking (Hammer et al., 2012). Thus, up to 70% of all floating plastic objects are believed to eventually settle onto the seafloor (Green et al., 2015; Weber et al., 2018).
Due to their wide distribution and long-term persistence within the environment, macroplastic affects biota in several ways. The most common threats come in the form of ingestion, entanglement, the formation of artificial substrates, the release of toxic substances, and the promoted transmission of alien species (de Carvalho-Souza et al., 2018; Angiolillo and Fortibuoni, 2020). Awareness of the problem is greatest in regard to the suffering of seabirds, fish, turtles, and marine mammals by entanglement or ingestion of litter. However, we currently have limited knowledge on the implications of marine litter for the much less “charismatic” species and groups, e.g., invertebrates, that typically escape the public perception but by no means play a less important role in marine ecosystem function (Bergmann et al., 2015; Buhl-Mortensen and Buhl-Mortensen, 2018).
The majority of studies with the effect of marine litter have focused on coral reef habitats (de Carvalho-Souza et al., 2018; Angiolillo and Fortibuoni, 2020) as well as deep-sea areas (Consoli et al., 2018, 2020; Canals et al., 2021). Marine litter can cause direct damage to the fragile branches of many corals (Mulochau et al., 2020), as well as increase the risk of disease (Lamb et al., 2018). Thereby, such factors can lead to a reduction in ecologically important coral cover (Edward et al., 2020). Furthermore, the accumulation of plastic litter and fishnets is reported for many deep areas (Fabri et al., 2014). Discarded fishing gear can cause the entanglement of numerous benthic organisms (e.g., fishes, sea urchins, crabs, and turtles) (van den Beld et al., 2017; Beneli et al., 2020; Stelfox et al., 2020), and ingestion of macrolitter by demersal fishes is reported (Valente et al., 2020). Such factors often lead to the death of an organism (Hammer et al., 2012).
To date, information about the effect of macrolitter that has on shallow coastal region benthic communities and habitats remains poorly understood and probably underestimated (Green et al., 2015; Angiolillo and Fortibuoni, 2020). The few mesocosm experiments that have utilized seagrasses have shown the increase of the intraspecific and interspecific competition (Balestri et al., 2017), reduction of the cover of vegetation, as well as the vulnerability of seagrasses to sedimentation where plastic bags are buried in the sediment (Menicagli et al., 2021). Furthermore, in situ experiments have only demonstrated the effect of macrolitter on benthic fauna of soft-bottom habitats. As such, increased cover by plastic litter has been shown to change the structure of benthic communities (Katsanevakis et al., 2007; Green et al., 2015) and prolong food searching time for gastropods (Aloy et al., 2011). However, we could not find any literature references of assessments of the impact of macrolitter on species or habitats of coastal rocky shores (Barboza et al., 2019).
The aim of this study was (1) to evaluate experimentally the response of benthic communities to the disturbance caused by plastic bag cover in shallow coastal areas and (2) to compare the resilience of hard and soft bottom communities. We predicted that the disturbance would have a noticeable effect on the production and biomass of benthic vegetation.
Materials and Methods
Study Area
The field experiment was conducted in shallow, tideless, semi-enclosed Kõiguste Bay, northern Gulf of Riga, NE Baltic Sea (58.3713°N, 22.9820°E). Kõiguste Bay is characterized by a wide coastal zone with both a diverse bottom topography and composition of macrophyte communities. Most of the bay is shallower than 3 m with a maximum depth of 7 m. Within the bay, a soft sediment bottom prevails with the presence of boulders and stones increasing toward the open-sea area. Depending on the water exchange between the Gulf of Riga and the open Baltic Sea, salinity in Kõiguste Bay ranges from 5 to 7 PSU. During the experiment, the observed salinity of seawater ranged from 5.5 to 5.8 PSU. The experimental area was located in a sheltered part of Kõiguste Bay. The presence of marine macrolitter originating from outside the experiment was not observed in the area while conducting the study.
Experimental Setup
The manipulative experiment was carried out from June to October 2018 in Kõiguste Bay (58.3711°N, 22.9817°E). To evaluate the response of benthic communities to the disturbance caused by marine litter, the experimental setup comprised of areas covered with plastic bags, which was contrasted with undisturbed natural areas as controls. Light blue McLean waste bags, which were made of HDPE material, thickness of 16 microns, with dimensions of 56 × 60 cm, and capacity of 35 L, were selected. Reinforcement carcass (mesh size 15 × 15 cm) was used to keep the waste bags on the bottom (Figure 1). The setup simulated real-world conditions were limited water exchange and reduced light conditions under plastic bags.
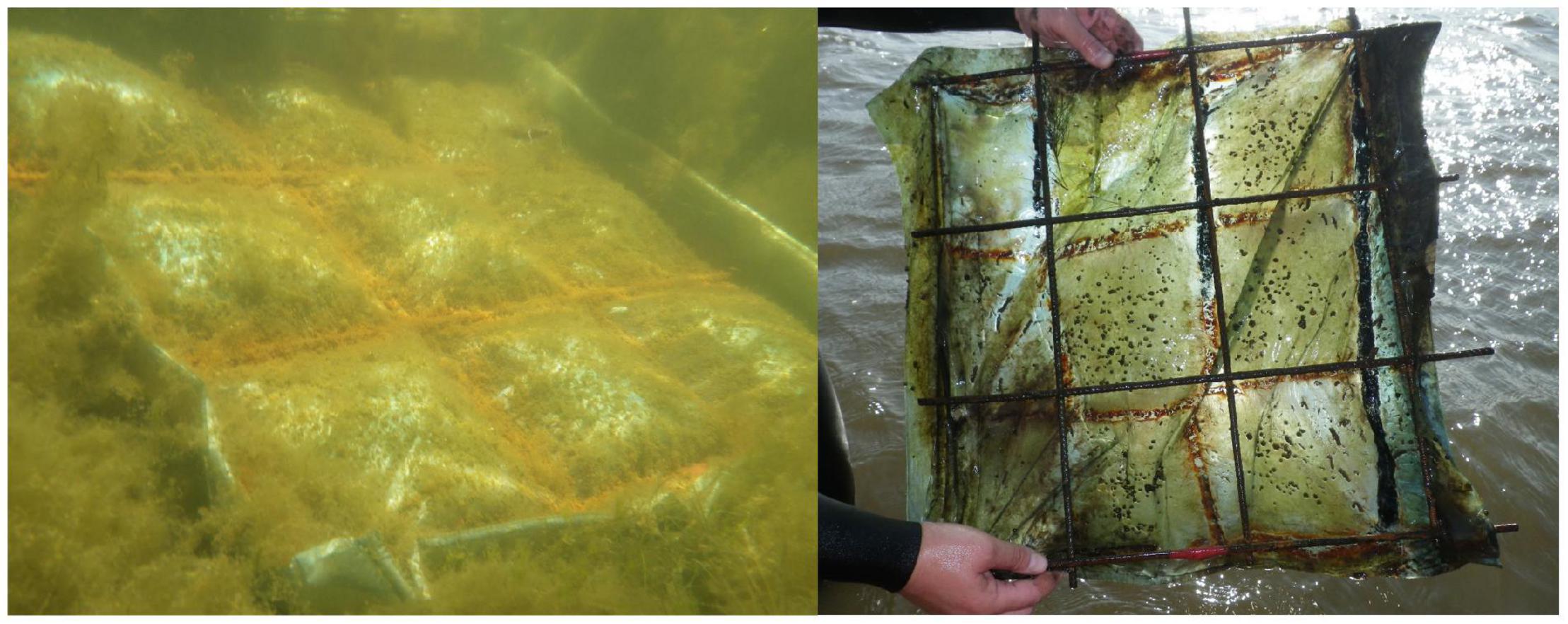
Figure 1. Soft substrate community covered by plastic bag after 1 month of exposure (left) and biofilm on plastic after 2 months of exposure (right). The amount of loose sediment covering the bag varied daily dependent on current hydrodynamic conditions.
The experiment was carried out on a soft substrate community dominated by the angiosperm Stuckenia pectinata (L.) Boerner and perennial brown macroalga Fucus vesiculosus L. as a characteristic species of a hard substrate. Both species are common and widespread throughout Baltic Sea coastal regions. Sago pondweed, S. pectinata, is the most frequent soft substrate species in Estonian coastal waters (Kotta et al., 2014) and plays an important role in structuring invertebrate community patterns (Maarse et al., 2021). Bladderwrack, F. vesiculosus, is a major community structuring species localized on the hard substrate in shallow sheltered or moderately exposed areas (Wikström and Kautsky, 2007).
The experiments were conducted on sheltered, smooth seafloor that comprised of the soft substrate (sand with mud), at a depth of 0.7 m. Specimens of Fucus were collected from the close vicinity of the experimental site, in the outer area of Kõiguste Bay (58.3611°N, 22.9946°E) where hard substrate prevails at depths of 1–1.5 m. Attached Fucus individuals of a similar thallus size were collected with the attached stone and associated epifauna, packed underwater, and transported to the experimental site.
Experimental plots were arranged as four transects (12 plots in each). The distance between each transect line was kept to 3 m as a minimum. Along with two of the transect lines, experimental plots (size 60 × 60 cm) with plastic bag cover above the community (respectively, dominated by Stuckenia or Fucus) were installed. For both communities, respective transect lines without plastic cover served as controls. The distance between each of the individual plots along a transect was at least 0.5 m. For both communities, the sampling design included the following two factors: disturbance by plastic (plots covered with plastic film and control plots without cover) and time of sampling. Each plot was sampled only one time. In total, four sampling series were conducted with an interval between each series of 1 month. For each treatment, three sampled plots served as replicates.
At each plot, biomass and primary production were measured. Biomass samples of the soft substrate community were collected within a 20 × 20 cm area. Fucus thalli were detached from stones in the laboratory. Samples were stored in deep frozen (−18°C) until analysis. In the laboratory, all species were identified to the species level. The dry weight of the species was obtained after drying the individuals at 60°C for 2 weeks.
The temperature was measured continuously using a General Oceanics thermologger set to measure at 4-h intervals and positioned above the seabed within the natural community and below the plastic cover. The rest of the environmental variables were measured once per month. Light reduction by the plastic cover was recorded using an Odyssey photosynthetically active radiation (PAR) logger. PAR was logged every minute for 2-3 h in three replicates with both uncovered loggers (controls) and loggers covered with plastic removed from the experimental plots prior to sampling. Oxygen concentration on the sea bottom was measured with an OptiOx optical dissolved oxygen sensor connected to a SevenGo DO meter (Mettler Toledo, Columbus, OH, United States), and pH values were measured with a Mettler Toledo InLab Expert Pro-ISM electrode.
Photosynthetic Production Measurements
The net photosynthetic rate of Fucus and Stuckenia was measured in triplicate using 600 ml glass bottles filled with seawater from the study site. Bottles individually containing ∼0.5 g dry weight of disturbed and undisturbed plant material were incubated in situ at a depth of 0.7 m. Bottles without plant material served as controls. The hourly net production rates (mg O2 g dry weight–1 h–1) were calculated from the differences in oxygen concentrations, measured over the incubation period (2–3 h). The dry weight of specimens was determined after drying at 60°C for 48 h. Throughout the experiment, the PAR (μmol m–2 s–1) at the incubation depth was measured using an Odyssey PAR logger.
Statistical Analyses
The results of the field experiment were statistically analyzed separately for hard and soft substrates using the factorial ANOVA. Prior to the analyses, the normality and homoscedasticity of the data were checked using Shapiro-Wilk and Levene’s tests accordingly. The effect of factors, i.e., (a) disturbance with two levels: under plastic and control and (b) sampling time with four levels: July, August, September, and October (except for PAR: five levels, i.e., including additional data on measurements in June), on the dependent variables (i.e., vegetation biomass, zoobenthos biomass, zoobenthos abundance, zoobenthos species richness, net primary production of Fucus and Stuckenia specimens, PAR, temperature, and oxygen concentration), was tested. Zoobenthos abundance was defined as the number of specimens and the species richness as the number of taxa. Effects were considered to be statistically significant at p < 0.05. When significant differences among main factors or their interactions were found, subsequent multiple comparisons of the means were performed using Tukey’s HSD post hoc test. Statistical analysis was carried out using STATISTICA (TIBCO Software Inc., RRID:SCR_014213) version 10.
Results
During the course of the experiment, the surface of the submerged plastic bags formed a biofilm reducing light penetration. At the commencement of the experiment, the plastic covering reduced PAR by 12%, after a one month period PAR decreased 55%, after 2 months 75%, after 3 months 79%, and finally by 95% compared with the simultaneous PAR measurements without cover (Figure 2). Temperature, oxygen concentrations, and pH levels were not significantly different beneath the plastic compared with the control. However, a temperature increase of 0.5–2°C was recorded for the plots covered by plastic during the summer months for more than 40% of the measurements taken.
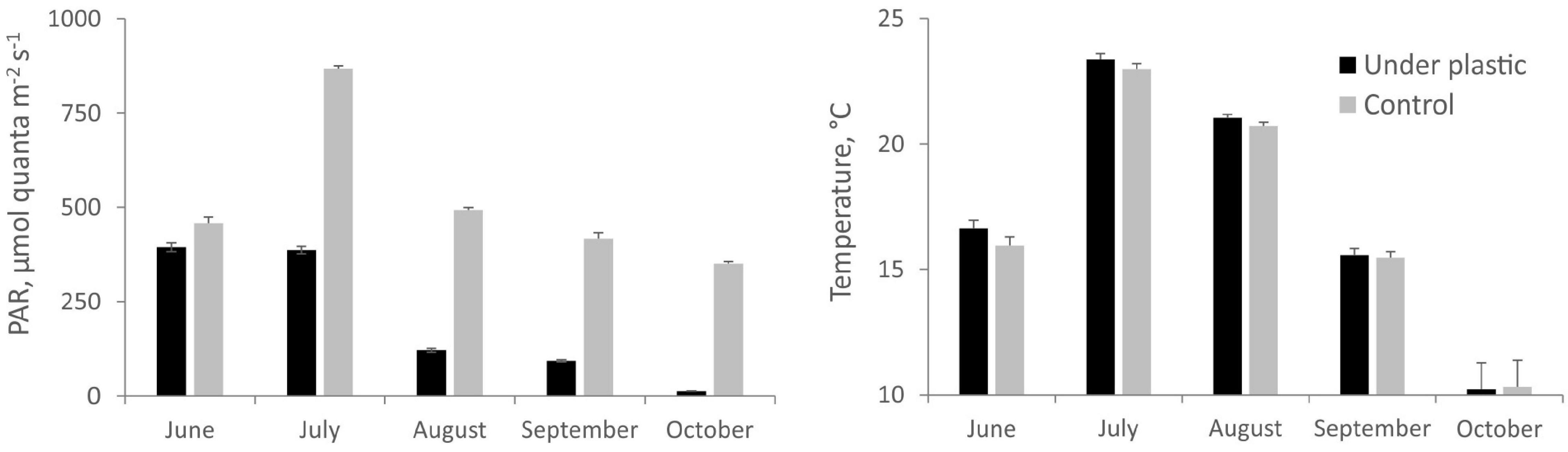
Figure 2. Temporal variation of average light availability [photosynthetically active radiation (PAR)] and temperature under the plastic cover and without cover (control). Values are means ± SE.
On the soft bottom, the total biomass of vegetation was remarkably lower beneath the plastic bag (Table 1 and Figure 3). In July, August, and September, statistically significant differences between the vegetation biomasses under the plastic covers and control series were found (Tukey’s HSD, p < 0.05). Although the biomass of hard bottom vegetation appeared to be lower beneath the plastic cover after a 3-month period (Figure 3), the observed differences between covered and not covered vegetation were statistically not significant. The biomass of zoobenthos was affected by the plastic cover only on hard substrate (Table 1 and Figure 3). On the soft bottom, the abundance of macrozoobenthos was significantly lower beneath the plastic cover compared with uncovered samples throughout the experiment (Figure 3). Gammarid amphipods and larval stages of the Chironomidae were the most abundant taxa for both soft and hard bottom communities. Species richness of macrozoobenthos in disturbed communities did not show a distinct difference when compared with that of undisturbed communities (ANOVA, p > 0.05).
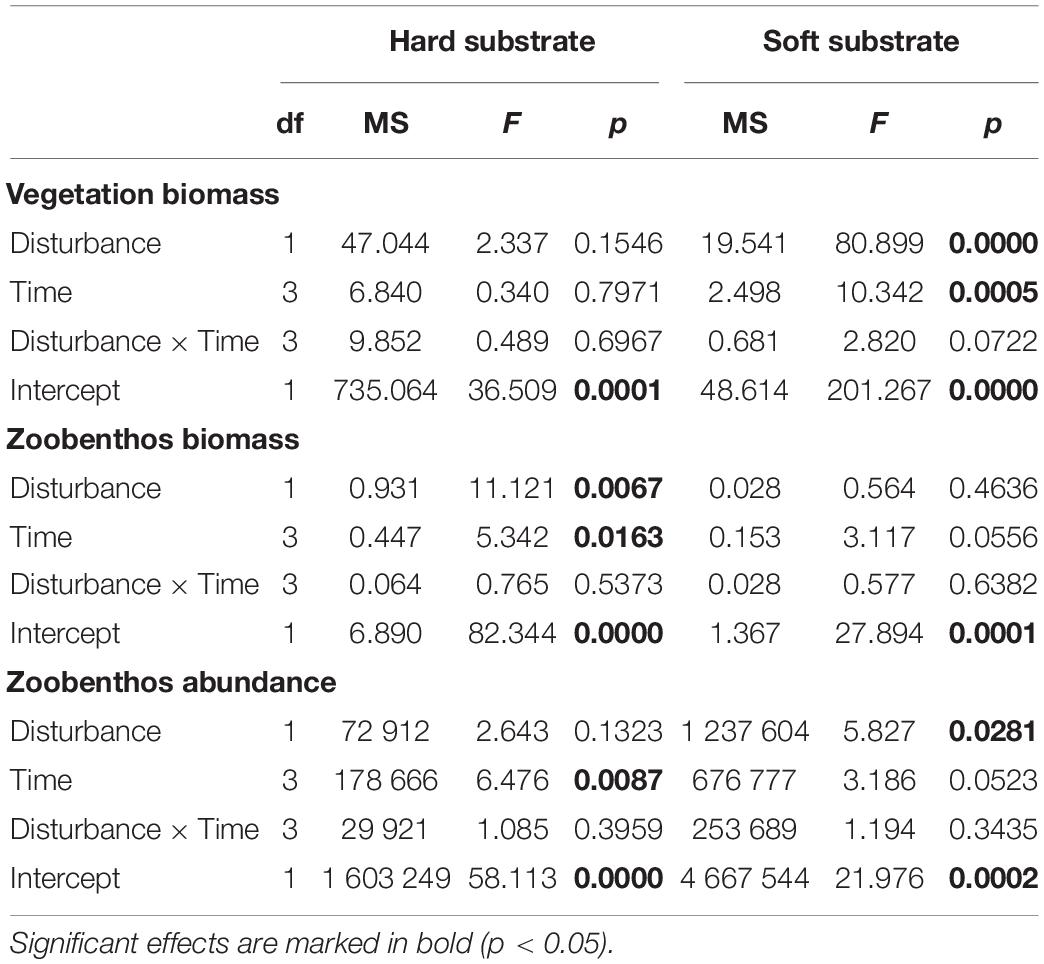
Table 1. Results of factorial ANOVA on the individual and combined effects of disturbance by plastic cover (presence vs. absence) and time (July, August, September, and October) on the biomass and abundance of benthic communities.
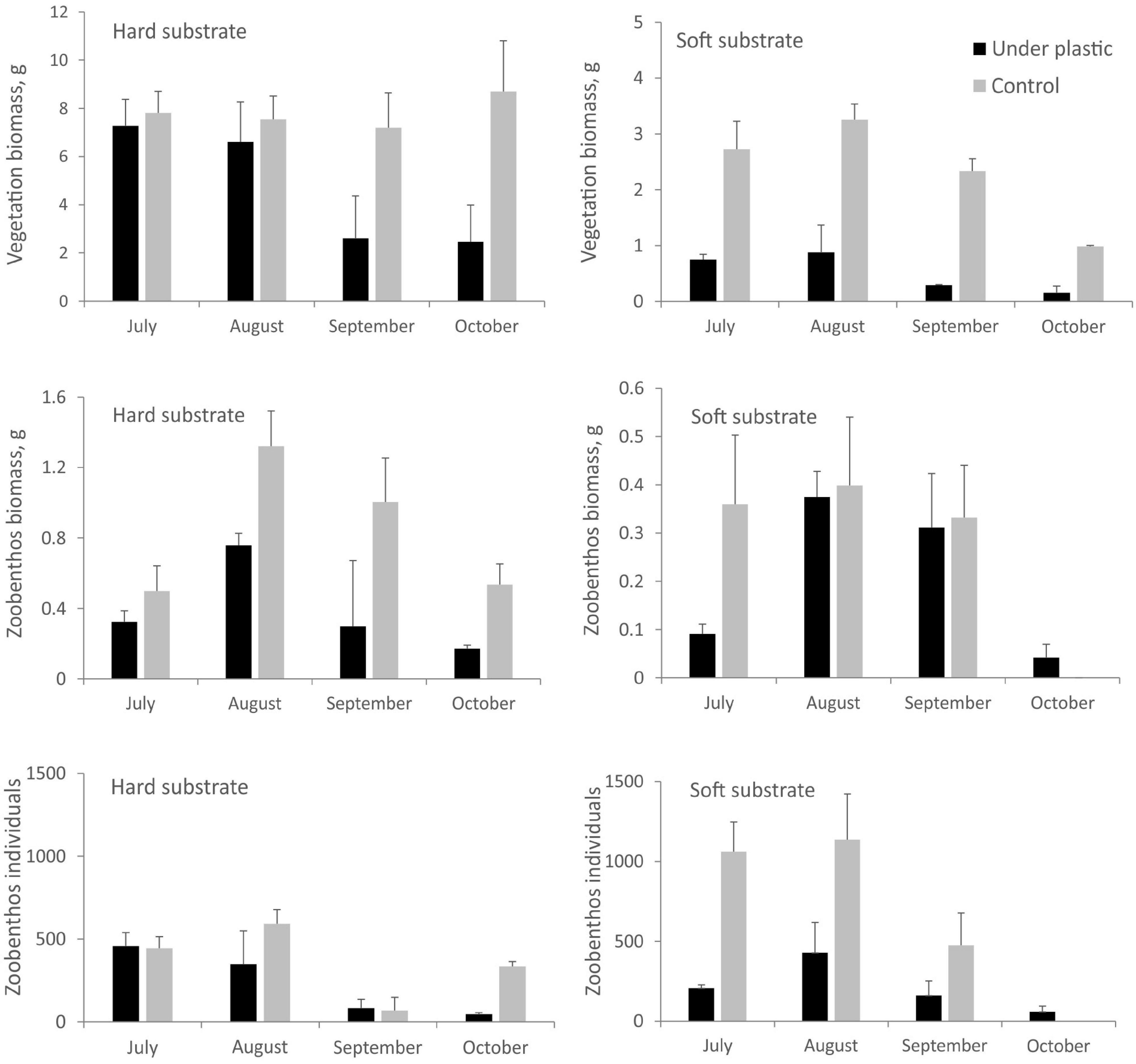
Figure 3. Total biomass of vegetation and zoobenthos and the number of zoobenthos individuals under the plastic cover and without cover (control) for both hard and soft substrates. Values are means ± SE.
Across the entirety of the experiment, the net production rates of Fucus were remarkably lower under the plastic cover (Table 2 and Figure 4). The most significant differences in the net production rates measured beneath the plastic cover in relation to the control were detected in October (Tukey’s post hoc test, p < 0.05) (Figure 4). At the same time, based on visual estimations, the covered specimens were in poor condition, when contrasted with the undisturbed specimens which had newly formed fronds. The difference in net production rates between disturbed and control specimens of Stuckenia was negligible.
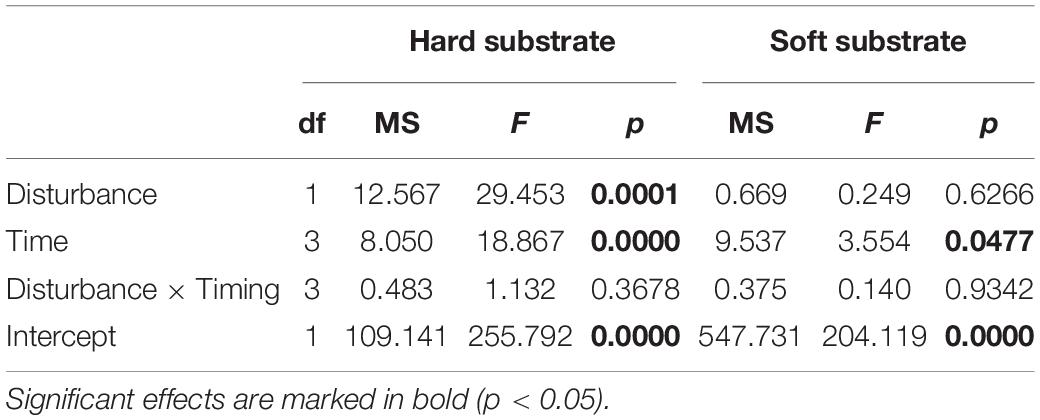
Table 2. Results of factorial ANOVA on the individual and combined effects of disturbance by plastic cover (presence vs. absence) and time (July, August, September, and October) on the net primary production.
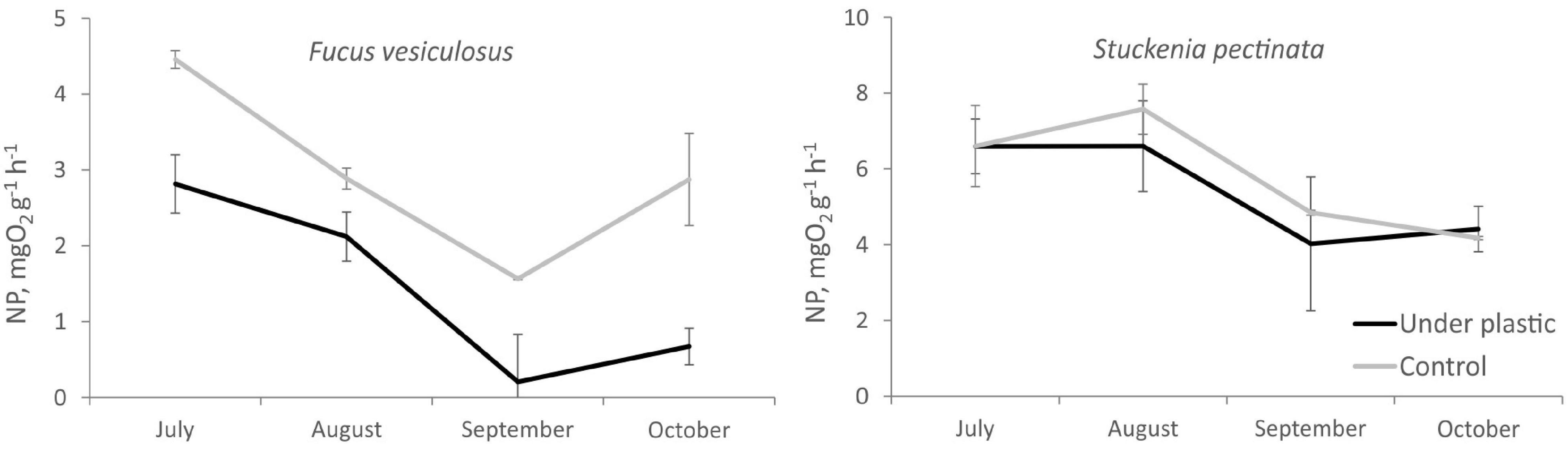
Figure 4. Net primary production rates of Fucus vesiculosus and Stuckenia pectinata. Values are means ± SE.
Discussion
For the first time, the effect of plastic bag disturbance on both soft and hard bottom vegetation and their associated fauna was evaluated under the same environmental conditions. Based on our results, both hard and soft substrate communities are sensitive to littering with the level of effect depending on the structure of the underlying community. In shallow areas, macroplastic tends to accumulate in vegetated habitats rather than in unvegetated ones (Cozzolino et al., 2020). Therefore, in our case, the impact on the whole community including that of macrovegetation was studied. To simulate real-world conditions (plastic bags entangled between rocks or partially buried in sediment), the bags were secured on top of developed communities with mature vegetation present. This allowed for partial water exchange and the movement of benthic invertebrates.
Our study demonstrates that plastic litter can have a substantial impact on benthic community structure and function within the marine environment. A rapid negative effect of the plastic bag cover on the biomass of soft-bottom vegetation was detected. In contrast, no statistically significant impact on the biomass of the hard bottom community from plastic coverings was observed. The biomass of zoobenthos was affected by the plastic cover only on the hard substrate. Plastic bag cover did not affect the species richness of macrozoobenthos, and although, it did decrease the species abundance found in soft substrate communities. A covering of plastic bags was observed to significantly reduce the net production rate of the hard substrate species F. vesiculosus, while the difference in net production rates between specimens of S. pectinata with and without plastic cover was negligible.
Floating plastic bags eventually settle to the seafloor, and thereafter, they are trapped by rocks or buried partially or completely in sediment. Settled marine litter has an impact on the community below it through both shading, which affects light conditions, and smothering effects. The biomass of the annual species Stuckenia with its tender thalli decreased significantly after 1 month of plastic cover, while the effect of plastic cover on primary production was negligible. However, the effect on the perennial Fucus with its sturdy thalli was exactly the opposite. Contradictory effects of the disturbance on biomass can be explained by the growth strategies of the species. The growth rate of the perennial species is low, while annual species must grow fast as a consequence of the short vegetative season in high latitudes. Decreased light conditions and the prevention of growth toward the water surface due to the physical plastic barrier inhibited the growth and biomass gain of the Stuckenia community beneath the cover. In addition, a similar effect caused by light reduction on the growth of new shoots has been recorded for eelgrass Zostera marina L. (Eriander, 2017). Although the negative effect on Fucus biomass was statistically non-significant, due to the limited number of replicates, a difference (healthy vs. partly decayed thalli) could be detected visually following 3 months of smothering.
In plots exposed to covering by plastic, the net primary production values for Fucus decreased more than two times compared with that of undisturbed conditions. In general, limited light availability causes plants to reduce their growth and ultimately disappear from the area. However, it has been previously reported that soft-bottom angiosperms and charophytes are able to adapt to reduced lighting conditions with no significant decline in their primary production rates (Marquardt and Schubert, 2009; Ochieng et al., 2010; Kovtun-Kante et al., 2014). Such findings are supported by the results presented in this study.
It is known that different coastal environments have different plastic trapping efficiencies (Harris et al., 2021). As a result, the impact of littering is considered to be greater on the hard substrate whereby rocky bottoms retain more litter than soft bottoms (Melli et al., 2017).
An analog manipulative experiment was carried out in a coastal area to investigate the effect of plastic bag coverage on infauna and microphytobenthos. For this, plastic was anchored firmly to a pure mud-flat sediment seafloor (Green et al., 2015). Plastic bag cover reduced the number of individuals and had no effect on the species richness of the benthic fauna on a shore near Dublin, Ireland (Green et al., 2015). Such a finding is similar to the one found in this study further validating the results. The decrease in observed zoobenthos abundance was most probably caused by the major change in the structure of the plant community as the film cover flattened it down. The effect of biomass loss and limited water movement cannot be fully excluded. In contrast, an increase in the number of species in littered soft bottom areas was demonstrated by Katsanevakis et al. (2007) and Song et al. (2021). The increase of species richness was found to be caused by the litter, which provided refuge for mobile species and substrate for sessile species (Katsanevakis et al., 2007; Clemente et al., 2018; Santín et al., 2020). However, a shift in species composition cannot be considered as a positive effect of marine litter on biota, as it affects the structure and functioning of the whole community (Kiessling et al., 2015).
In the soft substrate, plastic bags may be completely buried in the sediment. Studies utilizing mesocosm experiments have demonstrated that plastic bags buried in marine sediment can affect the growth of marine plants. For example, when plastic bags were buried in sediment, Mediterranean seagrass growth was observed to decline, leading to a reduction in competitiveness with invasive algae, increasing the vulnerability of seagrass to exclusion (Menicagli et al., 2021). Additionally, the changes in the geochemical parameters (reduced porewater oxygen concentrations and pH levels) of sediment were described by Balestri et al. (2017). In our case, the limited water exchange was possible below the plastic cover, thus, nullifying said effect. We measured water temperature, oxygen concentrations, and seawater pH, observing only minor increases in temperature below plastic cover while other parameters remained identical when compared with untreated locations.
The awareness of the potential threat posed by marine litter to the Baltic Sea is currently growing. However, the abundance of marine litter is lower compared with the North or Mediterranean Seas (Kammann et al., 2018; Rothäusler et al., 2019). Marine litter, in combination with other anthropogenic stressors (e.g., eutrophication and climate change), represents a substantial additional challenge to marine biodiversity (Werner et al., 2016). In the Baltic Sea, several species living near the limit of their salinity tolerance are at especially high risk of decline or extinction under additional cumulative pressures (Torn et al., 2020). Previously, a decrease in abundance and distribution of the perennial Fucus and an increase of filamentous algae coverage was observed in the Baltic Sea (Torn et al., 2006; Maarse et al., 2020). The replacement of slow-growing species with opportunistic ones has also been observed under the influence of litter (Akoumianaki et al., 2008; Angiolillo and Fortibuoni, 2020). Marine litter also offers opportunities for the dispersal of invasive species by providing artificial substrate for colonization and transport, and thereby, invaders may outcompete native species (Mantelatto et al., 2020; Teng et al., 2021; Wilson et al., 2021). Fucus is the major habitat-forming species on the hard substrate in the Baltic Sea (Wikström and Kautsky, 2007). Therefore, any additional pressure on Fucus or other foundation species can cause drastic changes throughout the entire coastal ecosystem.
Coastal regions are biologically highly active areas with significant ecological and socioeconomic values. Shallow coastal areas and beaches are becoming increasingly impacted by marine litter (Scotti et al., 2021; Zalewska et al., 2021). In addition to its impact on biota, marine litter affects the human quality of life, particularly in coastal areas (Hardesty et al., 2015). Plastic constitutes the largest proportion of marine litter overall (Pham et al., 2014). As single-use plastic bags are one of the most consumed items globally, appropriate measures must be taken to reduce their release into the environment (UNEP (United Nations Environment Programme), 2020). In general, the options to reduce the impact of single-use plastic bags on the environment are to ban their use, adopt plastic bag taxes, and increase public awareness surrounding the issue. An efficient waste management and recycling system must be in place to prevent plastic bags from ending up in the sea (UNEP (United Nations Environment Programme), 2020). To date, single-use plastic bags bans and/or plastic bag taxes are in use as a regulatory measure in many countries worldwide (Xanthos and Walker, 2017; Knoblauch et al., 2018).
Member States of the European Union are required to ensure environmentally sound waste management in order to prevent and reduce marine litter from all sources. Therefore, in 2021, single-use plastic and oxo-degradable plastics will be banned in the European Union. In Europe, the objective of the European Strategy for Plastics is to ensure that all plastic packages in the Union market are reusable or easily recyclable (EU, 2019). Another powerful legislative instrument within Europe is the Marine Strategy Framework Directive (EC, 2008). This directive designates marine litter as one descriptor of the Good Environmental Status (GES). As such, the abundance of litter and the impact it has on biota needs to be assessed by the Member States of the European Union in order to describe human impacts on the marine environment. The implementation of the Directive has promoted a significant increase in the amount of monitoring as well as the number of studies dedicated to marine litter. This has made it possible to take appropriate measures in less littered areas, such as the Baltic Sea, before the accumulation of the problem.
Conclusion
Based on our results, the primary production rate of hard substrate communities in shallow coastal systems is sensitive to littering by plastic, while in soft-bottom communities, the impact is expressed as the reduction in vegetation biomass. This study also provides evidence that habitat forming perennial species, due to particular threat from marine litter, can lead to the changes in the functioning of coastal shallow-water ecosystems. Thus, in temperate shallow coastal areas, the nature and scale of the impact of plastic litter on marine biota is dependent on the habitat and given coastal morphology of an area. As such, these factors should be taken into consideration when planning future assessments of the harmful effects of marine litter. In the context of the Baltic Sea, the species living near their salinity tolerance limit are at especially high risk of decline or extinction under additional cumulative pressures. Therefore, any additional pressure, e.g., anthropogenic litter on habitat-forming species has potentially a high impact on the coastal ecosystem.
It is shown that when plastic litter ends up in the sea, it decomposes slowly, affecting the biota and benthic habitats. Thus, there is an urgent need for political decisions to promote environmentally friendly production, reuse and recycling of plastic, as well as raise awareness around its use to consumers in order to slow down this process. At present, the abundance of macrolitter in the Baltic Sea is lower than in other European seas. However, we can be sure that if the situation escalates, habitat-forming species will be the most vulnerable as they already have to survive the elevated pressure caused by natural conditions, man-made stressors, and climate change.
Data Availability Statement
The raw data supporting the conclusions of this article will be made available by the authors, without undue reservation.
Author Contributions
KT, GM, and GR performed the experiments. KT, TP, and GR analyzed the data. KT and TP drafted the manuscript. All authors designed the study, discussed the results, edited the manuscript, and approved the submitted version.
Funding
This study was supported by the European Regional Development Fund via the Mobilitas Pluss (MOBERA12) of the Estonian Research Council.
Conflict of Interest
The authors declare that the research was conducted in the absence of any commercial or financial relationships that could be construed as a potential conflict of interest.
Publisher’s Note
All claims expressed in this article are solely those of the authors and do not necessarily represent those of their affiliated organizations, or those of the publisher, the editors and the reviewers. Any product that may be evaluated in this article, or claim that may be made by its manufacturer, is not guaranteed or endorsed by the publisher.
Acknowledgments
We would like to thank Imbi Püss, Tiia Möller, and Remo Torn for their help in fieldwork. We also thank Jack R. Hall for his help in editing the manuscript.
References
Akoumianaki, I., Kontolefas, P., Katsanevakis, S., Nicolaidou, A., and Verriopoulos, G. (2008). Subtidal littering: indirect effects on soft substratum macrofauna? Medit. Mar. Sci. 9, 35–52. doi: 10.12681/mms.131
Aloy, A. B., Vallejo, B. M. Jr., and Juinio-Meñez, M. A. (2011). Increased plastic litter cover affects the foraging activity of the sandy intertidal gastropod Nassarius pullus. Mar. Pollut. Bull. 62, 1772–1779. doi: 10.1016/j.marpolbul.2011.05.021
Angiolillo, M., and Fortibuoni, T. (2020). Impacts of marine litter on Mediterranean reef systems: from shallow to deep waters. Front. Mar. Sci. 7:826. doi: 10.3389/fmars.2020.581966
Balestri, E., Menicagli, V., Vallerini, F., and Lardicci, C. (2017). Biodegradable plastic bags on the seafloor: a future threat for seagrass meadows? Sci. Total Environ. 605, 755–763. doi: 10.1016/j.scitotenv.2017.06.249
Barboza, L. G. A., Cózar, A., Gimenez, B. C. G., Barros, T. L., Kershaw, P. J., and Guilhermino, L. (2019). “Macroplastics pollution in the marine environment,” in World Seas: An Environmental Evaluation, ed. C. Sheppard (Cambridge, MA: Academic Press), 305–328. doi: 10.1016/B978-0-12-805052-1.00019-X
Beneli, T. M., Pereira, P. H. C., Nunes, J. A. C. C., and Barros, F. (2020). Ghost fishing impacts on hydrocorals and associated reef fish assemblages. Mar. Environ. Res. 161:105129. doi: 10.1016/j.marenvres.2020.105129
Bergmann, M., Gutow, L., and Klages, M. (2015). Marine Anthropogenic Litter. Berlin: Springer International Publishing. doi: 10.1007/978-3-319-16510-3
Buhl-Mortensen, P., and Buhl-Mortensen, L. (2018). Impacts of bottom trawling and litter on the seabed in Norwegian waters. Front. Mar. Sci. 5:42. doi: 10.3389/fmars.2018.00042
Canals, M., Pham, C. K., Bergmann, M., Gutow, L., Hanke, G., Van Sebille, E., et al. (2021). The quest for seafloor macrolitter: a critical review of background knowledge, current methods and future prospects. Environ. Res. Lett. 16:023001.
Castro-Jiménez, J., González-Fernández, D., Fornier, M., Schmidt, N., and Sempéré, R. (2019). Macro-litter in surface waters from the Rhone River: plastic pollution and loading to the NW Mediterranean Sea. Mar. Pollut. Bull. 146, 60–66. doi: 10.1016/j.marpolbul.2019.05.067
Clemente, C. C., Paresque, K., and Santos, P. J. (2018). The effects of plastic bags presence on a macrobenthic community in a polluted estuary. Mar. Pollut. Bull. 135, 630–635. doi: 10.1016/j.marpolbul.2018.07.070
Consoli, P., Andaloro, F., Altobelli, C., Battaglia, P., Campagnuolo, S., Canese, S., et al. (2018). Marine litter in an EBSA (ecologically or biologically significant area) of the central Mediterranean Sea: abundance, composition, impact on benthic species and basis for monitoring entanglement. Environ. Pollut. 236, 405–415. doi: 10.1016/j.envpol.2018.01.097
Consoli, P., Sinopoli, M., Deidun, A., Canese, S., Berti, C., Andaloro, F., et al. (2020). The impact of marine litter from fish aggregation devices on vulnerable marine benthic habitats of the central Mediterranean Sea. Mar. Pollut. Bull. 152:110928. doi: 10.1016/j.marpolbul.2020.110928
Cozzolino, L., Nicastro, K. R., Zardi, G. I., and Carmen, B. (2020). Species-specific plastic accumulation in the sediment and canopy of coastal vegetated habitats. Sci. Total Environ. 723:138018. doi: 10.1016/j.scitotenv.2020.138018
de Carvalho-Souza, G. F., Llope, M., Tinôco, M. S., Medeiros, D. V., Maia-Nogueira, R., and Sampaio, C. L. (2018). Marine litter disrupts ecological processes in reef systems. Mar. Pollut. Bull. 133, 464–471. doi: 10.1016/j.marpolbul.2018.05.049
EC (2008). Directive 2008/56/EC of the European Parliament and of the Council of 17 June 2008 Establishing A Framework for Community Action in the Field of Marine Environmental Policy (Marine Strategy Framework Directive). Available online at: http://data.europa.eu/eli/dir/2008/56/oj (accessed August 23, 2021)
Edward, J. P., Mathews, G., Raj, K. D., Laju, R. L., Bharath, M. S., Kumar, P. D., et al. (2020). Marine derbis — an emerging threat to the reef areas of Gulf of Mannar, India. Mar. Pollut. Bull. 151:110793. doi: 10.1016/j.marpolbul.2019.110793
Eriander, L. (2017). Light requirements for successful restoration of eelgrass (Zostera marina L.) in a high latitude environment – acclimatization, growth and carbohydrate storage. J. Exp. Mar. Biol. Ecol. 496, 37–48. doi: 10.1016/j.jembe.2017.07.010
EU (2019). Directive 2019/904 of the European Parliament and of the Counscil of 5 June 2019 on the Reduction of the Impact of Certain Plastic Products on the Environment. Available online at: http://data.europa.eu/eli/dir/2019/904/oj (accessed August 23, 2021)
Fabri, M. C., Pedel, L., Beuck, L., Galgani, F., Hebbeln, D., and Freiwald, A. (2014). Megafauna of vulnerable marine ecosystems in French Mediterranean submarine canyons: spatial distribution and anthropogenic impacts. Deep Sea Res. II 104, 184–207. doi: 10.1016/j.dsr2.2013.06.016
Green, D. S., Boots, B., Blockley, D. J., Rocha, C., and Thompson, R. (2015). Impacts of discarded plastic bags on marine assemblages and ecosystem functioning. Environ. Sci. Technol. 49, 5380–5389. doi: 10.1021/acs.est.5b00277
Hammer, J., Kraak, M. H., and Parsons, J. R. (2012). “Plastics in the marine environment: the dark side of a modern gift,” in Reviews of Environmental Contamination and Toxicology, Vol. 220, ed. D. Whitacre (New York, NY: Springer), 1–44. doi: 10.1007/978-1-4614-3414-6_1
Hardesty, B. D., Good, T. P., and Wilcox, C. (2015). Novel methods, new results and science-based solutions to tackle marine debris impacts on wildlife. Ocean Coast. Manage. 115, 4–9. doi: 10.1016/j.ocecoaman.2015.04.004
Harris, P. T., Westerveld, L., Nyberg, B., Maes, T., Macmillan-Lawler, M., and Appelquist, L. R. (2021). Exposure of coastal environments to river-sourced plastic pollution. Sci. Total Environ. 769:145222. doi: 10.1016/j.scitotenv.2021.145222
Kammann, U., Aust, M. O., Bahl, H., and Lang, T. (2018). Marine litter at the seafloor – abundance and composition in the North Sea and the Baltic Sea. Mar. Pollut. Bull. 127, 774–780. doi: 10.1016/j.marpolbul.2017.09.051
Katsanevakis, S., Verriopoulos, G., Nicolaidou, A., and Thessalou-Legaki, M. (2007). Effect of marine litter on the benthic megafauna of coastal soft bottoms: a manipulative field experiment. Mar. Pollut. Bull. 54, 771–778. doi: 10.1016/j.marpolbul.2006.12.016
Kiessling, T., Gutow, L., and Thiel, M. (2015). “Marine litter as habitat and dispersal vector,” in Marine Anthropogenic Litter, eds M. Bergmann, L. Gutow, and M. Klages (Cham: Springer Open), 141–181. doi: 10.1007/978-3-319-16510-3_6
Knoblauch, D., Mederake, L., and Stein, U. (2018). Developing countries in the lead — what drives the diffusion of plastic bag policies? Sustainability 10:1994. doi: 10.3390/su10061994
Kotta, J., Möller, T., Orav-Kotta, H., and Pärnoja, M. (2014). Realized niche width of a Brackish water submerged aquatic vegetation under current environmental conditions and projected influences of climate change. Mar. Environ. Res. 102, 88–101. doi: 10.1016/j.marenvres.2014.05.002
Kovtun-Kante, A., Torn, K., and Kotta, J. (2014). In situ production of charophyte communities under reduced light conditions in a brackish-water ecosystem. Estonian J. Ecol. 63, 28–38. doi: 10.3176/eco.2014.1.03
Lamb, J. B., Willis, B. L., Fiorenza, E. A., Couch, C. S., Howard, R., Rader, D. N., et al. (2018). Plastic waste associated with disease on coral reefs. Science 359, 460–462. doi: 10.1126/science.aar3320
Maarse, F. E., Salovius-Laurén, S., and Snickars, M. (2020). Long-term changes in the phytobenthos of the southern Åland Islands, northern Baltic Sea. Nord. J. Bot. 38:e02751. doi: 10.1111/njb.02751
Maarse, F. E., Salovius-Laurén, S., and Snickars, M. (2021). Physical drivers of epi- and infauna communities related to dominating macrophytes in shallow bays in the Northern Baltic Sea. Estuar. Coast. 262:107600. doi: 10.1016/j.ecss.2021.107600
Mantelatto, M. C., Póvoa, A. A., Skinner, L. F., de Araujo, F. V., and Creed, J. C. (2020). Marine litter and wood debris as habitat and vector for the range expansion of invasive corals (Tubastraea spp.). Mar. Pollut. Bull. 160:111659. doi: 10.1016/j.marpolbul.2020.111659
Marquardt, R., and Schubert, H. (2009). Photosynthetic characterisation of Chara vulgaris in bioremediation ponds. Charophytes 2, 1–8.
Melli, V., Angiolillo, M., Ronchi, F., Canese, S., Giovanardi, O., Querin, S., et al. (2017). The first assessment of marine debris in a site of community importance in the north-western Adriatic Sea (Mediterranean Sea). Mar. Pollut. Bull. 114, 821–830. doi: 10.1016/j.marpolbul.2016.11.012
Menicagli, V., Balestri, E., Vallerini, F., De Battisti, D., and Lardicci, C. (2021). Plastics and sedimentation foster the spread of a non-native macroalga in seagrass meadows. Sci. Total Environ. 757:143812. doi: 10.1016/j.scitotenv.2020.143812
Mulochau, T., Lelabousse, C., and Séré, M. (2020). Estimations of densities of marine litter on the fringing reefs of Mayotte (France – South Western Indian Ocean) - impacts on coral communities. Mar. Pollut. Bull. 160:111643. doi: 10.1016/j.marpolbul.2020.111643
Napper, I. E., and Thompson, R. C. (2019). Environmental deterioration of biodegradable, oxo-biodegradable, compostable, and conventional plastic carrier bags in the sea, soil, and open-air over a 3-year period. Environ. Sci. Technol. 53, 4775–4783. doi: 10.1021/acs.est.8b06984
Napper, I. E., and Thompson, R. C. (2020). Plastic debris in the marine environment: history and future challenges. Glob. Chall. 4:1900081. doi: 10.1002/gch2.201900081
Ochieng, C. A., Short, F. T., and Walker, D. I. (2010). Photosynthetic and morphological responses of eelgrass (Zostera marina L.) to a gradient of light conditions. J. Exp. Mar. Biol. Ecol. 382, 117–124. doi: 10.1016/j.jembe.2009.11.007
Pham, C. K., Ramirez-Llodra, E., Alt, C. H., Amaro, T., Bergmann, M., Canals, M., et al. (2014). Marine litter distribution and density in European seas, from the shelves to deep basins. PLoS One 9:e95839. doi: 10.1371/journal.pone.0095839
Rothäusler, E., Jormalainen, V., Gutow, L., and Thiel, M. (2019). Low abundance of floating marine debris in the northern Baltic Sea. Mar. Pollut. Bull. 149:110522. doi: 10.1016/j.marpolbul.2019.110522
Santín, A., Grinyó, J., Bilan, M., Ambroso, S., and Puig, P. (2020). First report of the carnivorous sponge Lycopodina hypogea (Cladorhizidae) associated with marine debris, and its possible implications on deep-sea connectivity. Mar. Pollut. Bull. 159:111501. doi: 10.1016/j.marpolbul.2020.111501
Scotti, G., Esposito, V., D’Alessandro, M., Panti, C., Vivona, P., Consoli, P., et al. (2021). Seafloor litter along the Italian coastal zone: an integrated approach to identify sources of marine litter. Waste Manage. 124, 203–212. doi: 10.1016/j.wasman.2021.01.034
Song, X., Lyu, M., Zhang, X., Ruthensteiner, B., Ahn, I.-Y., Pastorino, G., et al. (2021). Last plastic debris dumps: new biodiversity hot spots emerging on the seep-sea floor. Environ. Sci. Technol. Lett. 8, 148–154. doi: 10.1021/acs.estlett.0c00967
Stelfox, M., Burian, A., Shanker, K., Rees, A. F., Jean, C., Willson, M. S., et al. (2020). Tracing the origin of olive ridley turtles entangled in ghost nets in the Maldives: a phylogeographic assessment of populations at risk. Biol. Conserv. 245:108499. doi: 10.1016/j.biocon.2020.108499
Teng, G., Jin, X., Fu, C., Guan, L., Jin, Y., Chen, Y., et al. (2021). Is seafloor litter contributing to sea anemone blooms? Sci. Total Environ. 759:143479. doi: 10.1016/j.scitotenv.2020.143479
Torn, K., Krause-Jensen, D., and Martin, G. (2006). Present and past depth distribution of bladderwrack (Fucus vesiculosus) in the Baltic Sea. Aquat. Bot. 84, 53–62. doi: 10.1016/j.aquabot.2005.07.011
Torn, K., Peterson, A., and Herkül, K. (2020). Predicting the impact of climate change on the distribution of the key habitat-forming species in the NE Baltic Sea. J. Coast. Res. 95, 177–181. doi: 10.2112/SI95-035.1
UNEP (United Nations Environment Programme) (2020). Single-Use Plastic Bags and Their Alternatives: Recommendations from Life Cycle Assessments. Available online at: https://www.lifecycleinitiative.org/single-use-plastic-bags-and-their-alternatives-recommendations-from-life-cycle-assessments/ (accessed August 23, 2021)
Valente, T., Scacco, U., and Matiddi, M. (2020). Macro-litter ingestion in deep-water habitats: is an underestimation occurring? Environ. Res. 186:109556. doi: 10.1016/j.envres.2020.109556
van den Beld, I. M., Guillaumont, B., Menot, L., Bayle, C., Arnaud-Haond, S., and Bourillet, J. F. (2017). Marine litter in submarine canyons of the Bay of Biscay. Deep Sea Res. II 145, 142–152. doi: 10.1016/j.dsr2.2016.04.013
Weber, M., Makarow, D., Unger, B., Mortier, N., De Wilde, B., van Eekert, M., et al. (2018). “Assessing marine biodegradability of plastic — towards an environmentally relevant international standard test scheme,” in Proceedings of the International Conference on Microplastic Pollution in the Mediterranean Sea, eds M. Cocca, E. Di Pace, M. E. Errico, G. Gentile, A. Montarsolo, and R. Mossotti (Cham: Springer International Publishing), 189–193. doi: 10.1007/978-3-319-71279-6_25
Werner, S., Budziak, A., van Franeker, J. A., Galgani, F., Hanke, G., Maes, T., et al. (2016). Harm Caused by Marine Litter. MSFD GES TG Marine Litter – Thematic Report. Luxembourg: European Union. doi: 10.2788/690366
Wikström, S. A., and Kautsky, L. (2007). Structure and diversity of invertebrate communities in the presence and absence of canopy-forming Fucus vesiculosus in the Baltic Sea. Estuar. Coast. Shelf Sci. 72, 168–176. doi: 10.1016/j.ecss.2006.10.009
Wilson, H. L., Johnson, M. F., Wood, P. J., Thorne, C. R., and Eichhorn, M. P. (2021). Anthropogenic litter is a novel habitat for aquatic macroinvertebrates in urban rivers. Freshw. Biol. 66, 524–534. doi: 10.1111/fwb.13657
Xanthos, D., and Walker, T. R. (2017). International policies to reduce plastic marine pollution from single-use plastics (plastic bags and microbeads): a review. Mar. Pollut. Bull. 118, 17–26. doi: 10.1016/j.marpolbul.2017.02.048
Keywords: marine litter, macroplastic, benthic ecology, photosynthetic production, coastal areas, disturbance, benthic vegetation, zoobenthos
Citation: Torn K, Martin G, Paalme T and Reisalu G (2022) A Manipulative Field Experiment to Evaluate the Impact of Discarded Plastic Bags on Shallow Vegetated Benthic Communities. Front. Mar. Sci. 8:774908. doi: 10.3389/fmars.2021.774908
Received: 13 September 2021; Accepted: 15 November 2021;
Published: 21 January 2022.
Edited by:
Stelios Katsanevakis, University of the Aegean, GreeceReviewed by:
Virginia Menicagli, University of Pisa, ItalyPierpaolo Consoli, University of Naples Federico II, Italy
Copyright © 2022 Torn, Martin, Paalme and Reisalu. This is an open-access article distributed under the terms of the Creative Commons Attribution License (CC BY). The use, distribution or reproduction in other forums is permitted, provided the original author(s) and the copyright owner(s) are credited and that the original publication in this journal is cited, in accordance with accepted academic practice. No use, distribution or reproduction is permitted which does not comply with these terms.
*Correspondence: Kaire Torn, a2FpcmUudG9ybkB1dC5lZQ==