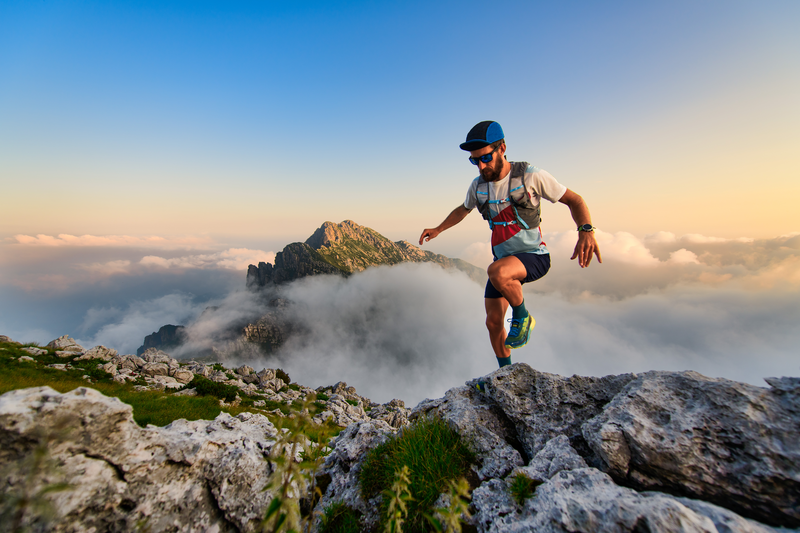
95% of researchers rate our articles as excellent or good
Learn more about the work of our research integrity team to safeguard the quality of each article we publish.
Find out more
ORIGINAL RESEARCH article
Front. Mar. Sci. , 06 December 2021
Sec. Marine Biology
Volume 8 - 2021 | https://doi.org/10.3389/fmars.2021.774274
This article is part of the Research Topic Biological Invasions in the Mediterranean Sea View all 13 articles
In this study, gamete release by Caulerpa cylindracea was investigated for the first time in the Tyrrhenian Sea (Sardinia, Western Mediterranean), focusing on the main environmental factors triggering gametogenesis and spawning of the species. For this purpose, a combination of field and laboratory experiments was conducted. First, a 2-year mensurative experiment was performed in the field during summer to individuate and quantify the occurrence of C. cylindracea gametogenesis and spawning events, linking them to environmental conditions. Then, based on the results of the field work, a laboratory experiment was performed to directly test, under controlled conditions, the effect on the above-mentioned processes of two abiotic factors that appeared to play a key role in species gamete release processes in the area: sea water temperature and water movement. During the 2 years of field research, several gametogenesis events were recorded in the study area and two spawning events also occurred. Significant differences were observed between the ratio of fertile and total thalli and between releasing thalli (RT) and fertile thalli (FT), among sites with different hydrodynamic conditions and sampling periods with different sea water temperatures. Overall, these results suggest that C. cylindracea sexual reproduction also occurs in the Tyrrhenian Sea, and that, therefore, new genotypes that favor the invasive profile of the species could be produced in the basin, where both its gametogenesis and spawning seem to be mainly regulated by water movement and sea water temperature.
Over the last decades, an increasing number of studies have considered the patterns of gametogenesis, sexual reproduction, and fertilization of seaweeds by means of an integrated biological and ecological approach (e.g., Brawley and Johnson, 1992; Santelices, 2002). Several experimental and field observations allowed to characterize gametogenesis, gamete release, and subsequent fertilization of broadcasting species as a tightly synchronized and environmentally controlled process (Santelices, 2002). Certainly, a remarkable seasonality in different phases of sexual reproduction (especially gametogenesis and spawning) results from a variety of environmental factors that satisfy physiological requirements for reproduction or from the use of environmental cues to coordinate reproduction (Lüning and Tom Dieck, 1989). However, detailed information in the literature is still scarce (Holmes and Brodie, 2004). Indeed, studies that simultaneously examine the effects of several environmental factors on sexual reproduction, in particular on gametogenesis and spawning, appear to be quite difficult. Nevertheless, they are necessary to delineate the conditions that must be simultaneously met for successful sexual reproduction, especially for alien species (Brawley and Johnson, 1992). Focusing on alien invasive species, knowledge of life cycle progression transcends pure academic interest (Liu et al., 2017), as establishing the occurrence of their sexual reproduction events has important environmental management implications, both for gametes and zygote dispersal and the potential to generate more invasive genotypes (Ellstrand and Schierenbeck, 2000, 2006).
The genus Caulerpa (Chlorophyta: Bryopsidales) is a group of marine green siphonous algae distributed in tropical and subtropical regions (Meinesz and Boudouresque, 1996; Ratana-Arporn and Chirapart, 2006), some of which have recently seriously invaded the Mediterranean Sea (Klein and Verlaque, 2008). The Caulerpa genus includes about 85 species (Guiry and Guiry, 2020), and it is considered one of the most widespread, conspicuous, and abundant groups of seaweeds worldwide (Silva, 2003). Species belonging to this genus are multinucleated (coenocyte) and colonial, and they have a modular organization. They are all characterized by the lack of cellulose in their cell walls (Silva, 1992), and they present great phenotypic plasticity and morphological variability that arise in several morphological forms and variants (Belleza and Liao, 2007). In the last few decades, the genus has attracted considerable research interest, because Caulerpa taxifolia (Vahl) C. Agardh and Caulerpa cylindracea Sonder have significantly expanded their range of distribution in the basin where they are currently considered as highly invasive (Verlaque et al., 2003; Piazzi et al., 2005; Klein and Verlaque, 2008). They represent an important threat to the diversity of benthic coastal ecosystems (Boudouresque and Verlaque, 2002), interfering with native species (Piazzi et al., 2001a; Piazzi and Cinelli, 2003) and modifying benthic assemblages (Argyrou et al., 1999).
Besides vegetative dispersion (Žuljević and Antolić, 2000) that represents the main dispersal mode for these species (Ceccherelli and Cinelli, 1999; Ceccherelli and Piazzi, 2001; Ceccherelli et al., 2002), Panayotidis and Žuljević (2001) suggested that such widespread and rapid spread is also probably supported by successful sexual reproduction. Indeed, sexual reproduction is common in the life cycle of the Caulerpa species, and it has been documented in populations from both tropical and temperate waters (Goldstein and Morrall, 1970; Enomoto and Ohba, 1987; Ohba et al., 1992; Clifton, 1997; Clifton and Clifton, 1999). While some authors claimed that the thallus found in nature has a haplodiplobiontic life cycle with heteromorphic alternation of generations (Goldstein and Morrall, 1970; Kapraun and Nguyen, 1994), other authors suggested that a diploid or haploid life cycle with only one generation is present (Ishihara et al., 1981; Ohba et al., 1992; Benzie et al., 2000). Anyway, several articles describe the thallus as monoecious, producing and releasing simultaneously both gametes and dying after gamete release (e.g., Žuljević and Antolić, 2000).
Several observations on sexual reproduction and gamete release by the Caulerpa species were reported for Caribbean reefs (e.g., Clifton, 1997; Clifton and Clifton, 1999), and indicated that sexual reproduction can also play an important role in the dynamics of these algae. Nevertheless, very scarce data on sexual reproduction and gamete release for the Caulerpa species in the Mediterranean Sea are currently present in the literature (Varela-Alvarez et al., 2012). In particular, with regard to two of the most abundant species in the basin, C. prolifera and C. taxifolia, only male or female gametes were observed (Meinesz, 1979; Žuljević and Antolić, 2000). Finally, considering C. cylindracea that is currently the most invasive Caulerpa species in the Mediterranean, even if there is a general agreement that C. cylindracea also reproduces sexually (e.g., Flagella et al., 2008; Klein and Verlaque, 2008; Katsanevakis et al., 2010), the entire lifecycle has not been described, and specific data for the Thyrrenian Sea are not present in the literature yet. Indeed, only two articles describing gametogenesis and spawning in the Mediterranean strain of this species are currently available in the literature. The first referred to the Adriatic Sea and the observation of only female gametes is reported (Žuljević et al., 2012). On the contrary, both male and female gametes were observed as well as planozygotes in the Aegean Sea, but only for a particular variant of the species [Caulerpa racemosa var. occidentalis (J. Agardh) Børgesen] (Panayotidis and Žuljević, 2001).
In this article, gamete release by C. cylindracea in the Thyrrenian Sea (Sardinia, Western Mediterranean) was investigated for the first time, focusing on the main environmental factors triggering the gametogenesis and spawning of the species. To this purpose, a combination of field and laboratory experiments was conducted in 2018–2019. First, a 2-year mensurative experiment was performed in the field during summer to individuate for the first time and, eventually, quantify the occurrence of C. cylindracea gametogenesis and spawning events, linking them to environmental conditions. Furthermore, based on the results of the field work, a complementary laboratory experiment was performed to directly test, under controlled conditions, the effect of the abiotic factors that appeared to play a key role in gamete release processes of the species in the area.
The field mensurative experiment was conducted in Tavolara Punta Coda Cavallo Marine Protected Area, along the North-Eastern Sardinian coast, in the summer period of 2018 and 2019. Field observations were carried out by snorkeling in Cala Bua bay (CB: 40°52.586′ N; 9°38.173′ E) and Porto Taverna bay (PT: 40°51′55.2″N, 9°39′47.6″E) at a depth of about 2 m (Figure 1). Both the study sites are characterized by big patches of C. cylindracea, overgrowing autochthonous macroalgae, especially on sandy bottoms, and they have a similar substratum type (rocks, cobbles, and sand were present) and assemblage [composed mainly by Acetabularia acetabulum (L.) Silva, dark filamentous algae (DFA), Laurencia spp., Liagora spp., and Padina pavonica (L.) Thivy]. In each site, two different study areas of about 20 m2 were selected where C. cylindracea was present (mean substratum percent cover: CB: 21.34%; PT: 19.23%); in particular, a sheltered area (SA) and an exposed area (EA) were considered in each site (Figure 2), considering the main winds blowing in the area in the summer months.
Figure 1. Map of Tavolara Punta Coda Cavallo MPA with the detailed position of the two considered study sites.
Figure 2. Schematic representation of the sampling design for the two performed experiments. Sites: CB: Cala Bua; PT: Porto Taverna. Water motion: NH: no water motion; LH: low water motion; HH: high water motion. Temperatures: LT: lower temperature (18°C); ET: equal temperature (24°C); HG: higher temperature (30°C).
During 15 randomly selected days from the beginning of July until the end of September, when the maximum development of C. cylindracea occurs (Klein and Verlaque, 2009), its populations were sampled in the study sites. A first survey was conducted in the afternoon to detect the presence of eventual fertile thalli (FT), and to estimate their abundance (refer to Section “Laboratory Experiment: Gametogenesis and Spawning Under Controlled Conditions” for sampling methods). FT (Figure 3) were detected observing fronds in detail, in order to individuate the changes in thallus appearance that usually accompany gamete formation, according to Panayotidis and Žuljević (2001) and Žuljević et al. (2012). If present, FT, easily detected because of depigmentation of blades, were marked (refer to Figure 3C) with a large (to not damage them) rubber band, in order to check them in case of possible gamete release the following morning, basing on the protocol described by Žuljević and Antolić (2000) for C. taxifolia. Considering that gamete release by the Caulerpa species usually occurs 15 min before sunrise (Clifton and Clifton, 1999; Žuljević et al., 2012), morning observations started about 30 min before the sunrise. As the morphological changes interesting the thallus and the branchlets during sexual reproduction can be visible at most 2 days prior of gamete release, if no release was observed the first morning, a new survey was repeated the following day. During each morning survey, releasing thalli (RT), detectable because of the presence of a green cloud around the plant, were visually counted, and the main environmental parameters were also measured according to the methods described below that were the same used for the laboratory experiment, in order to obtain uniform data (refer to section “Laboratory Experiment: Gametogenesis and Spawning Under Controlled Conditions” for the sampling methods). According to Agrawal (2012) and Liu et al. (2017), the main environmental factors triggering gametogenesis and spawning in algae were considered: water motion, seawater temperature, light availability (in terms of irradiance), salinity, and nutrients (N and P concentration).
Figure 3. (A–C) Fertile depigmented thalli with papillae in aquaria (A,B) and directly in the field (C). (C,D) A particular colored branchlet is presented in (A), while several discharge tubes are visible in (B,D), the observed gametes with and without flagella.
All data regarding the environmental factors were tri-replicated.
Data were collected using a multiparametric probe (Hanna Instruments HI991300).
Irradiance was estimated in terms of photosynthetically active radiation (PAR) by means of an underwater PAR meter (Apogee MQ-210).
Water samples (125 ml) in each site were randomly taken at approximately 50 cm from C. cylindracea patches. After collection, the water samples were shaken, filtered (0.45-mm mesh size filter), and frozen, as suggested by Balata et al. (2010). Then, in the laboratory, the concentrations of total inorganic N and P were estimated by oxidation with potassium peroxodisulphate according to the most recent method described by Cozzi et al. (2010). The same method was used also to estimate the concentration of N and P in the aquaria.
Water movement was estimated by measuring the rate of dissolution of initially dried (60°C, 24 h) and weighed gypsum balls (diameter 3 cm) prepared following Gambi et al. (1989) and mounted on stainless steel sticks. The balls were exposed to dissolution for 24 h. After recovery, the gypsum balls were rinsed lightly with fresh water to remove salts, dried at 60°C for 24 h, and weighed. The dissolution (weight loss) of each ball was converted to an estimate of flux (cms–1) according to the following function obtained by Bailey-Brock (1979):
where Me is the weight loss of each gypsum ball during field exposure, and Mc is the weight loss of the calibration balls (left in still seawater).
Water motion estimation in the aquaria was conducted before inserting algae, using the same method.
Within each study area, 10 quadrats of 15 cm × 15 cm were placed in random positions on the substratum, and thallus abundance was considered in each. To obtain comparable data, quadrats with less than five thalli were excluded before sampling, and thalli with a length < of 5 cm and/or with less than five branches were not considered. In each quadrat, the total number of thalli (TT) and the number of eventual FT and RT were counted (Engelen et al., 2005). For each sampling day, data obtained from the 10 quadrats of each area were pooled together, and the mean value was used; in this way, five replicates for each of the three sampling months were, on the whole, considered for each area.
Caulerpa cylindracea sterile thalli were collected along the coasts of Tavolara Punta Coda Cavallo Marine Protected Area, in Porto Taverna bay (40°51′55.2″N, 9°39′47.6″E) (Figure 1) in the late summer of 2019 (approximately 15–20 days before spawning events were observed in 2018). During collection, the main environmental parameters (seawater temperature, salinity, hydrodynamic stress, light availability, and N and P concentrations) were measured according to the same sampling methods used in the field in order to obtain homogeneous data (refer to Section “Field Experiment: Gametogenesis and Spawning in Tavolara Punta Coda Cavallo MPA” for the sampling methods). They all resulted in accordance with those described in the literature for the summer period in NW Mediterranean (Reñé et al., 2011; Caronni et al., 2014, 2016).
About 230 thalli (around 1 mm in diameter and 7 cm in length with at least five blades) were collected from widely separated areas at about 12 m depth. A mid-collection depth was chosen, because in the mid-depth range (5−15 m) C. cylindracea usually finds particularly favorable growth conditions (De Biasi et al., 1999). Moreover, such a collection depth avoided thallus exposition to significant fluctuations of the main environmental parameters characterizing shallow waters (Sarà et al., 1999) that could influence their survival and acclimation to laboratory conditions. After collection, the thalli were maintained in seawater and immediately transported to the laboratory. Once in the laboratory, the thalli were kept in small 20 L aerated aquaria with subsand filters. Artificial seawater was used, and a thin layer (1 cm) of fine sand (1 mm) was prepared as the substratum for algae settlement. All the aquaria were initially maintained in a growth chamber for 3 weeks, simulating the environmental conditions observed in the field. Water temperature was set at 24°C, and salinity and N and P concentrations were adjusted to 33 psu and 0.7 and 0.9 μM, respectively. The sea water was enriched with Provasoli’s solution (Provasoli et al., 1968), as suggested by Sudatti et al. (2011). The thalli were exposed to cool-white fluorescent light with an irradiance of 100 μmol photons m–2s–1 and a 15-h:9-h light-dark cycle for an acclimation period of 3 weeks (Phillips, 2009). During this period, the C. cylindracea thalli remained healthy in the aquaria and did not show relevant acclimation problems (the health status of the algae was evaluated considering both the color intensity of the blades and the presence of injuries and damaged portions, according to Bennett et al., 2015).
The thalli were then exposed to different temperature and hydrodynamic conditions (the two parameters that show differences among sites during the field mensurative experiment) in an environmental test chamber for 45 days. According to Phillips (2009), indeed, this period of time is sufficient to observe, if environmental conditions are favorable, a significant number of reproductive episodes for Caulerpa species. In particular, six different combinations of treatments (including one simulating the values recorded on the occasion of the spawning events observed in natural conditions) were tested according to a fully orthogonal experimental design. Three replicates for each combination of treatments were considered (three different aquaria for each), and 12 different thalli with at least five branches were transferred in each of the experimental aquaria. In particular, three different temperatures [LT: lower than the mean summer Sardinian one (18°C) vs. ET: equal to the mean summer Sardinian one (24°C) vs. HT: higher than the mean summer Sardinian one (30°C)], and three different hydrodynamic conditions [NH: no water motion (0 cm s–1) vs. LH: low water motion (∼5 cm s–1) vs. HH: high water motion (∼13 cm s–1)] (Figure 2). Chamber temperature was set in order to obtain desired seawater temperatures in the aquaria, avoiding overheating. The different hydrodynamic conditions were, instead, obtained directly in the aquaria by means of adjustable aquarium stirring pumps. The other parameters (irradiance, nutrient concentration, salinity, and photoperiod) were maintained stable (refer to Section “Laboratory Experiment: Gametogenesis and Spawning Under Controlled Conditions” for the sampling methods).
At the beginning of the experiment, according to Phillips (2009), the thalli were examined daily to immediately detect fertile ones. When they showed signs of gamete formation processes (considering the morphological changes described for the species and, in particular, depigmentation and papillae development (Panayotidis and Žuljević, 2001; Žuljević et al., 2012), they were counted and separated into 1-L beakers with the aim of obtaining gamete samples for microscopic observation. For practical reasons, in the afternoon before gamete release, according to Phillips (2009), the FT were placed in total darkness to postpone gamete release for about 2–3 h. The number of RT was then determined.
During release under controlled conditions, 20 separate suspensions of gametes were collected with a micropipette (50 μl each) near each release cloud and pipetted on a microscope graduated slide for microscope observation (×40 and ×100 magnification was used). In this way, estimation of the abundance and size of gametes produced during each event was possible (the abundances obtained in the 20 suspensions were pooled together to obtain a reliable value for each event). In particular, the length and width of at least 30 gametes obtained from each separate suspension event were measured. Data regarding the abundance and the size of gametes relative to all the events that occurred in each aquarium were pooled together, and the means were considered, in order to obtain three replicate size and abundance values for each combination of treatments.
The ratio between the number of FT and the total TT was considered as the main response variable to evaluate gametogenesis during both experiments. Moreover, for the second experiment, the ratio between the number of RT and that of fertile ones (FT) was also considered as the response variable. Finally, three additional response variables were also taken into account for each treatment in the second experiment: the total number of spawning events, the abundance of released gametes, and their size (length and width).
The data were analyzed by means of univariate statistical analyses using the software GMAV5 (Underwood et al., 2002).
A four-way ANOVA was performed with the data collected during the first experiment. The considered factors were: year (two random levels: 2018 vs. 2019), site (two fix levels: CB vs. PT), zone (two fix levels: LH vs. HH), and month (three fix levels: July vs. August vs. September). Moreover, further, six four-way ANOVAs were performed to analyze data regarding each of the considered environmental parameters.
With regard to the second experiment, instead, five two-way ANOVAs (considered variables: FT/TT and RT/FT ratios, gamete abundance, length, and width) were performed considering as fixed factors water motion (three levels: NH vs. LH vs. HH) and temperature (three levels: LT vs. ET vs. HT).
Shapiro–Wilk and Cochran’s tests were performed prior to each ANOVA to test for normality and homogeneity of variances. The Student–Newman–Keuls (SNK) test was performed for a posteriori comparison of the means in case of significant ANOVA results (Underwood, 1997).
Finally, Pearson correlation was used to test the relationship between gametogenesis events and environmental conditions for the first experiment (only temperature and water motion).
Some significant differences were found among the six environmental parameters (Figure 4 and Supplementary Materials (Tables 1A,B). Figure 4 shows the mean values recorded for each environmental variable in each area of the two sites. While there were no differences among sites, years, areas, and months for salinity, irradiance, and total N and P concentrations, significant differences were observed among areas and months for both sea water temperature and water motion. With regard to seawater temperature, higher values were recorded in the sheltered areas when compared with the exposed ones (SA: 25.3°C and EA: 24.1°C). However, the most relevant temperature differences were recorded among the sampling months. Indeed, mean values appeared to be significantly higher in July and in the first part of August (25.1°C), while they were lower in the second part of August and in September (24.3°C) (Figure 4 and Supplementary Tables 1A,B). On the contrary, with regard to water motion, the main differences were observed between the two areas of each site, as in the sheltered areas the mean values appeared to be significantly lower than in the exposed ones (SA: 5.58 cm s–1 vs. EA: 10.69 cm s–1).
Figure 4. Mean (±ES) value for each of the considered environmental factors (seawater temperature, salinity, water motion, light availability, and N and P concentrations) for each of the two areas in the two study sites, during each of the three periods considered for each study year.
Table 1. (A) Results of the ANOVA on data regarding the fertile thalli (FT)/total number of thalli (TT) ratio in relation to the considered factors: Site (Si): CB vs PT; Year (Ye): 2018 vs. 2019; Area (Ar): sheltered vs. exposed; Period (Pe): July vs. August vs. September.
During the 15 sampling days of each of the 2 years of field research, about 600 gametogenesis events were, on the whole, recorded in the two study sites (at least one fertile thallus was observed during 82 of the 120 observations performed during the study) and during about 35% of the observations, half of the thalli present in the considered quadrats appeared to be fertile. Nevertheless, only two spawning events were observed throughout the whole study period (one event for each year) in Cala Bua (CB).
The FT appeared as depigmented protoplasmic masses, easily distinguishable from colored, not fertile ones; papillae and discharge tubes were well developed on the frond axes and the top of the stolon, and the upper part of the branchlets was orange-brown (Figures 3A–C).
Significant differences in their ratio (FT/TT) were observed between the exposed and sheltered areas and among the sampling months, while no differences were recorded among the sites and years (Figure 4, Table 1, and Supplementary Table 1). In Figure 5, the mean FT/TT ratio is presented for each study area and site in each period of the 2 years. In particular, in both sites, the FT/TT ratio was significantly higher in the sheltered areas (21%) than in the exposed ones (7.98 %). Moreover, for both years, such a ratio assumed a significantly higher value in the second part of August and in September (21%) (Figure 5 and Tables 1A,B).
Figure 5. Mean (±ES) ratio between the number of fertile thalli and the total number of thalli (FT/TT %) in the two areas of the two study sites during each of the three periods considered for each study year. Spawning events are indicated with *.
Also, the two spawning events that were detected thanks to the occurrence of the typical cloud, occurred in Cala Bua in September; each lasted about 9−10 min, starting 14 and 13 min before sunrise– respectively. Despite, both the spawning events were observed in quadrats in which some other FT were also present (FT/TT ratio: 55.12 and 23.16%, respectively, in the quadrat containing the first and second releasing thallus), the releasing thallus was distinctly identified, as the gamete cloud were localized only around the interested branchlets.
Finally, a negative correlation between the abundance of FT (in terms of FT/TT ratio) and water motion was found (R2 = –0.71, p < 0.05, n = 120) (Figure 6A). With regard to temperature, only a slightly negative correlation was, instead, observed (R2 = –0.59, p < 0.05, n = 120) (Figure 6B).
Figure 6. Relationship among FT/TT ratio, (A) water motion, and (B) sea water temperature (B) on the pooled data (n = 120). R values are provided in the text.
Several gametogenesis and spawning events were observed under controlled conditions with significant differences in relation to treatments. Figure 7 shows the mean FT/TT ratio for each water motion and temperature combination. In particular, higher ratios were observed at 24°C (67.59%) while lower values were recorded both at lower (18°C: 47.22%) and highest temperatures (30°C: 17.59%). Moreover, significantly higher FT/TT ratios were found in the aquaria with no water movement (63.89%), and they remarkably decreased in the aquaria with low (41.67%) and, in particular, high water motion (29.17%) (Figure 7A and Tables 2A,B).
Figure 7. Mean (±ES) ratio between the number of FT and the total number of thalli (FT/TT%) (A) and between the number of releasing thalli (RT) and that of fertile ones (RT/FT %) (B) in the aquaria with different temperature and water motion treatments.
Table 2. (A) Results of the ANOVAs on data regarding the FT/TT and the releasing thalli (RT)/FT ratios for each treatment: Temperature (T): 18 vs. 24 vs. 30°C and Water motion (W): no water motion (NH) vs. low water motion (LH) vs. high water motion (HH).
During the spawning events, several gametes were observed. The gametes were discharged within gametangia thanks to papillae in a stream of mucilaginous material spurting from different discharge tubes starting from the colored branchlets (Figures 3A–C). Gametangia are membrane-bound spherical structures that contain several gametes that, once released, form a green cloud that disperses within 10–20 min, depending on hydrodynamic conditions. In the aquaria with no water movement, the dispersion time was of about 15-17 min, while both with the low and high water motions it ranged between 10 and 12 min. After the end of the releasing event, the parental branchlets appeared white and suddenly died; the empty thalli usually disintegrated within about 1 day (24−36 h).
Despite the spawning events, overall, no zygotes were observed during the study.
All the gametes had a length that ranged from 5.5 to 6.1 μm, while their width ranged from 1.9 to 2.5 μm (Figure 3D). Moreover, no eyespot was observed in the analyzed gametes while some of them (about 15% of the analyzed ones) had two visible flagella (Figure 3D). No differences in the size of gametes were recorded in relation to treatments (Figures 8A,B). On the contrary, gametes were more abundant in the aquaria with low or null water motion and with a temperature of 24°C (NH: 104 cells ml–1; LH: 103 cells ml–1) (Figure 9 and Tables 3A,B).
Figure 8. Mean (±ES) length (A) and width (B) of the observed gametes in the aquaria with different temperature and water motion treatments.
Figure 9. Mean (±ES) abundance of the observed gametes in the aquaria with different temperature and water motion treatments.
Table 3. (A) Results of the ANOVA on data regarding the abundance, length, and width of gametes in relation to treatments: Temperature (T): 18 vs. 24 vs. 30°C; Water motion (W): no water motion (NH) vs. low water motion (LH) vs. high water motion (HH).
This study presents the first report of gametogenesis and spawning by C. cylindracea in the Thyrrenian Sea and provides useful information on the environmental factors triggering such processes. First of all, several gametogenesis events were observed in Tavolara Punta Coda Cavallo MPA during the period of the study, and two gamete release events also occurred, even if no zygotes were observed, as described in few previous studies on the species (Panayotidis and Žuljević, 2001; Žuljević et al., 2012).
Despite both the observed morphology of FT and the general pattern of gametogenesis of C. cylindracea appearing to be quite similar to that of other conspecific species (e.g., Miyake and Kunieda, 1937; Clifton, 1997; Phillips, 2009), some relevant differences also emerged.
With regard to timing, in Tavolara Punta Coda Cavallo MPA, the spawning events appeared to be shorter (10 min) than those described by Miyake and Kunieda (1937) (1 h), while their duration was quite similar to those reported by both Panayotidis and Žuljević (2001) and Žuljević et al. (2012) for other parts of the Mediterranean basin (5−10 min). These results suggest that the duration of spawning events can be quite variable, as already observed for other algae (Pearson and Serrao, 2006).
Moreover, according to Clifton (1997), generally, algae with external fertilization, like Bryopsidales, are characterized by a highly synchronized reproductive pattern, with tens to thousands of conspecific algal thalli (at least 3.5% of the population) becoming simultaneously fertile in the same small area overnight and several spawning events occurring together. Analyzing the data obtained during the field experiment, a different situation was instead recorded. The mean percentage of simultaneously observed FT in the 300 cm2 considered in each site exceed 50% (max. 63.64%) during only 6 of the 120 observations and, in most cases, only one or two FT were, on the whole, counted, with less than 1% of the population fertile. Moreover, only two single spawning events were overall observed. A quite different situation was, instead, observed under controlled conditions, where in some cases FT/TT ratio exceeded 90% and RT/FT ratio exceeded 100%. The lower rates of both FT and releasing events observed during the field study, in comparison to both the laboratory ones and those recorded by Clifton (1997) for reef environments, can be explained considering that the observations of Clifton (1997) referred to the tropical waters of which C. cylindracea is native and not to the Mediterranean, where the environmental conditions are certainly different and so, presumably, different cues act (tidal, photoperiod, lunar phases, etc.). According to Pearson et al. (1998), indeed, environmental cues should be taken into account among the most important triggers playing a key role in coordinating gametogenesis. Considering that the most energy-consuming phase of sexual reproduction is gametogenesis (Shinkawa et al., 2019), it is plausible to suppose that gamete production and release happen when the conditions are favorable in order not to waste energy.
According to Brawley and Johnson (1992), in particular, synchronicity can act by interfering with both gametogenesis and spawning in different ways, ensuring that mature gametes are available for synchronous release when favorable conditions occur (Pearson et al., 2004). On the contrary, Yund (2000) observed that, under non-ideal conditions, environmental cues usually do not act, with the consequence that no synchronous release will occur; in this case, only few FT and spawns are recorded. Therefore, despite the remarkable capability of C. cylindracea to adapt in response to the main ecological factors (Verlaque et al., 2000), it can be assumed that the quite low number of fertile and RT observed during the field study is due to the loss of cues regulating and coordinating sexual reproductive events.
The data from both the field and controlled condition experiments revealed remarkable differences in fertile and RT rates in relation to the environmental factors. These results suggest that also for C. cylindracea, gametogenesis and spawning are strictly related to environmental conditions, as already observed for several quite similar species (Clifton, 1997), and that successful reproduction usually depends on a delicate juxtaposition of different conditions (Santelices, 2002). Environmental factors directly operate to complete algal lifecycles (growth, reproduction, spore germination, and again growth) (Agrawal, 2012) and gametogenesis, and the other phases of sexual reproduction, which appeared to occur only when the main environmental factors (temperature, salinity, photoperiod, main nutrients, etc.) satisfy the physiological requirements for reproduction (Lüning and Tom Dieck, 1989).
Although during observations several environmental factors were considered as determinants for gamete production and release, no differences were observed for light and nutrient availability, as well as for salinity among the study areas and sampling periods. On the contrary, some interesting results were overall obtained considering seawater temperature and water motion. In particular, significant differences in FT/TT ratio were recorded among areas and periods with different hydrodynamics and seawater temperatures, respectively; furthermore, for the former, a clear negative correlation with the ratio of FT was highlighted from the analysis of data obtained under controlled conditions both for FT/TT and RT/FT. These results are in accordance with those of other studies that demonstrated the importance of water motion and temperature in triggering gametogenesis and spawning for other similar species (Giese and Kanatani, 1987; Clifton and Clifton, 1999), and they suggest that such factors must be considered when investigating C. cylindracea sexual reproduction.
With regard to temperature, in the field, a significantly higher FT/TT ratio was observed in the second part of August and in September, when the mean seawater temperature was about 24°C, while in the first half of summer, when the temperature was higher, a lower number of FT were observed. Furthermore, the results of the laboratory experiment highlighted a similar trend, as the FT/TT ratio was significantly higher in the aquaria with a temperature of 24°C, while remarkably lower ratios were, instead, observed at both 18 and 30°C. These results are in accordance with those of other studies on macro and microalgae (e.g., Henry, 1988; Dieck, 1992; Kalita and Tytlianov, 2003; Agrawal, 2012), and they suggest that C. cylindracea must be included in the list of green algae characterized by a very narrow range of temperatures favorable for sexual reproduction. For most algae, indeed, the temperature range that allows for reproduction is considerably limited compared to that for vegetative growth, and they may reproduce asexually or sexually at different, also quite small, temperature ranges (League and Greulach, 1955).
Anyway, considering the few data available in the literature for the species, some differences can be noticed. A slightly higher gametogenesis temperature was, indeed, described both by Panayotidis and Žuljević (2001), with FT primarily observed at 26°C, and Žuljević et al. (2012) with a peak of FT recorded at 25°C, even if they asserted that on the whole C. racemosa in the Adriatic Sea becomes fertile when the seawater temperature is between 20 and 25°C.
Some quite interesting information can also be obtained by analyzing the correlation between FT/TT and RT/FT ratios and water motion, which resulted negative. Higher ratios, indeed, were observed in the aquaria where no water motion occurred. A similar pattern was also observed in the field, where the FT/TT ratio appeared to be related to a stimulating effect of calm condition for gametogenesis. These results suggest that a stimulatory effect of calm conditions seems to exist for gametogenesis and not only for gamete release, as already observed for other species of Bropsydales (Brawley et al., 1999; Speransky et al., 2000). According to Pearson et al. (1998), C. cylindracea seems to be among the algae that have the ability to perceive water motion via boundary layer bicarbonate levels and photosynthetic metabolism (Pearson and Brawley, 1996, 1998), and to consider it as a signal coordinating the different phases of its sexual reproduction.
Stressing the remarkable direct effects of wave forces affecting intertidal organisms, Denny (1995) asserted that they primarily included damage and, especially, displacement of unattached parts that usually occur for gametes floating in water more than adult individuals attached to the substratum. The hypothesis of gamete displacement and dilution is also confirmed by the significantly lower abundance of gametes in the water near the discharge tubes observed in aquaria with high water motion. Even if, in all the aquaria, gamete abundances appeared to be quite similar to those observed by Goldstein and Morrall (1970), for several species of the Caulerpa genus, significantly higher abundances were observed under no water motion conditions, especially when a temperature of 24°C were simulated.
From the obtained data, the effect of temperature is evident only in the optimal condition of water motion, thus allowing us to suppose that water motion is the main factor in C. cylindracea gametogenesis and spawning (as also confirmed by the differences in the Pearson correlation coefficient). Temperature, instead, seems to have, especially in some cases (e.g., 30°C when water motion is remarkable) a secondary additive effect. Actually, for some other seaweeds, gamete release is strictly restricted to calm intervals (Serrão et al., 1996), thereby preventing the gamete dilution predicted to occur by turbulent flow and water exchange (Pennington, 1985; Denny and Shibata, 1989; Levitan et al., 1992). Indeed, gamete production only under low water motion conditions represents an extremely valuable adaptation for an organism inhabiting the intertidal zone, as it allows to finalize gamete release, reducing dispersion. In this way, a large number of gametes appear to be available for gamete collision and fertilization, enhancing the probability of zygote formation.
These results also provide some interesting insights into the relationship between C. cylindracea diffusion and the climate changes that are interesting in the Mediterranean Sea (e.g., Adloff et al., 2015; Marbà et al., 2015). This basin is considered a miniature ocean highly affected by climate changes (Lejeusne et al., 2010). Furthermore, climatic models predict that this basin will be one of the regions that will experience the main effects of both the global warming trend and of an increase in extreme events significantly altering currents and water motion in it. Considering that the two main factors playing a key role in the gametogenesis and spawning of C. cylindracea appear to be precisely sea water temperature and motion, for which the species seems to have a narrow range of tolerance, it is plausible to hypothesize that ongoing climate changes can significantly reduce the chances of successful reproduction of the species in the basin. In case of not optimal environmental conditions, indeed, reproducing asexually could be more advantageous, as recently observed for other species (Endo et al., 2021). Therefore, a situation of increased water motion and temperature similar to one of those simulated during the laboratory experiment, especially in summer, when the vegetative growth of the species reaches its maximum (Piazzi et al., 2001b), will lead to a reduction in the number of FT and, consequently, in the probability of spawning events to occur.
A further observation that a favorable combination of several interacting factors must occur for FT production and gamete release (Serrao et al., 1999; Santelices, 2002) is represented by significantly lower FT/TT ratio that was observed during field observations when compared with both of those obtained under controlled conditions. Indeed, these data suggest that in the field, other factors presumably not acting in the aquaria played a role in such processes, as already observed for the Caulerpa genus during other studies (Phillips, 2009; Žuljević et al., 2012). Considering the other factors triggering gametogenesis and spawning for algae (e.g., extracellular organic substances, pollution, pH of the medium, osmotic stress, etc., according to Agrawal, 2012), an active role of pheromones and other inhibiting extracellular substances can be supposed for the above-mentioned processes. Pheromones are particularly important for the chemical communication between individuals preceding the sexual reproduction of algae (Maier, 1993). While such substances can be easily diluted in the water under natural conditions, at least when thallus abundance is not particularly high, their efficiency in aquaria is expected to be higher. Indeed, in the aquaria, gametogenesis and release can also be enhanced by the absence of extracellular organic substances produced by other algae that could have an inhibitory effect on the reproduction of the considered species, as observed by Wichard and Oertel (2010) and Lüning et al. (2000) for other seaweeds.
Finally, also considering the peculiar morphological features of the observed gametes, some interesting considerations can be made. All the gametes observed during the study had the same length (5.5 to 6.1 μm) and width (1. to 2.5 μm) and lacked the eyespot (Figure 3D). These results are in accordance with that of Goldstein and Morrall (1970) and to Žuljević et al. (2012) that observed that anisogamous gametes for the species with female and male differ for the size and the presence/absence of the eyespot. Therefore, all the observed gametes presumably were males while no female ones were observed, contrary to what was described by Žuljević et al. (2012) for the Adriatic Sea, who observed only larger female gametes with the eyespot. A quite similar result was instead obtained by Phillips (2009) for the congeneric species C. taxifolia, for which, even if a few female gametes were observed, a markedly male sex ratio was noticed. These results suggest that gender expression in Tavolara Punta Coda Cavallo MPA populations of C. cylindracea has deviated from the 1:1 male:female allocation, expected when sex is wholly genetically determined, as already observed for C. prolifera, for which either only male (Meinesz, 1979) or female gametes (Dostal, 1929) were described. Even if sex ratios in macroalgal species have been poorly studied (Holmes and Brodie, 2004), and the causal factors responsible for biased sex ratios are still almost unknown, these results can be explained by considering that Caulerpa populations can be composed of many male plants, few bisexual plants, and no female ones.
In conclusion, the obtained data suggest that C. cylindracea sexual reproduction occurs in the Thyrrenian Sea and that it should be taken into account when focusing on the invasion dynamics of the species. Moreover, as the gametogenesis and spawning of C. cylindracea are mainly regulated by water motion and temperature, such factors must be considered for the management of the species, considering that, as sexual reproduction occurs, new more invasive genotypes for the species could be produced in the Mediterranean, even if the ongoing climate changes must also be considered in the reproduction and diffusion dynamics of the species.
The raw data supporting the conclusions of this article will be made available by the authors, without undue reservation.
SCa, SCi, and AN conceived and designed the experiments. SCa, MD, and PP performed the study. SCa, RG, and CM analyzed the collected data. SCa and SCi wrote the manuscript. All authors contributed to the article and approved the submitted version.
The authors declare that the research was conducted in the absence of any commercial or financial relationships that could be construed as a potential conflict of interest.
All claims expressed in this article are solely those of the authors and do not necessarily represent those of their affiliated organizations, or those of the publisher, the editors and the reviewers. Any product that may be evaluated in this article, or claim that may be made by its manufacturer, is not guaranteed or endorsed by the publisher.
We would like to thank Sebastiano Caronni Orsenigo and Giulia Piazza for their precious help during the field and laboratory studies, respectively.
The Supplementary Material for this article can be found online at: https://www.frontiersin.org/articles/10.3389/fmars.2021.774274/full#supplementary-material
Adloff, F., Somot, S., Sevault, F., Jordà, G., Aznar, R., Déqué, M., et al. (2015). Mediterranean Sea response to climate change in an ensemble of twenty first century scenarios. Clim. Dyn. 45, 2775–2802. doi: 10.1007/s00382-015-2507-3
Agrawal, S. C. (2012). Factors controlling induction of reproduction in algae review: the text. Folia Microbiol. 57, 387–407. doi: 10.1007/s12223-012-0147-0
Argyrou, M., Demetropoulos, A., and Hadjichristophorou, M. (1999). Expansion of the macroalga Caulerparacemosa and changes in soft bottom macrofaunal assemblages in Moni Bay, Cyprus. Oceanol. Acta 22, 517–528. doi: 10.1016/S0399-1784(00)87684-7
Bailey-Brock, J. H. (1979). Sediment trapping by chaetopterid polychaetes on a Hawaiian fringing reef. J. Mar. Res. 37, 643–656.
Balata, D., Piazzi, L., Nesti, U., Bulleri, F., and Bertocci, I. (2010). Effects of enhanced loads of nutrients on epiphytes on leaves and rhizomes of Posidoniaoceanica. J. Sea Res. 63, 173–179. doi: 10.1016/j.seares.2009.12.001
Belleza, D. F. C., and Liao, L. M. (2007). Taxonomic inventory of the marine green algal genus Caulerpa (Chlorophyta, Bryopsidales) at the University of San Carlos (Cebu) herbarium. Philipp. Sci. 44, 71–104. doi: 10.3860/psci.v44i0.380
Bennett, S., Wernberg, T., De Bettignies, T., Kendrick, G. A., Anderson, R. J., Bolton, J. J., et al. (2015). Canopy interactions and physical stress gradients in subtidal communities. Ecol. Lett. 18, 677–686. doi: 10.1111/ele.12446
Benzie, J. A., Ballment, E., Chisholm, J. R., and Jaubert, J. M. (2000). Genetic variation in the green alga Caulerpataxifolia. Aquat. Bot. 66, 131–139. doi: 10.1016/S0304-3770(99)00068-6
Boudouresque, C. F., and Verlaque, M. (2002). Biological pollution in the Mediterranean Sea: invasive versus introduced macrophytes. Mar. Pollut. Bull. 44, 32–38. doi: 10.1016/s0025-326x(01)00150-3
Brawley, S. H., and Johnson, L. E. (1992). Gametogenesis, gametes and zygotes: an ecological perspective on sexual reproduction in the algae. Br. Phycol. J. 27, 233–252. doi: 10.1080/00071619200650241
Brawley, S. H., Johnson, L. E., Pearson, G. A., Speransky, V., Li, R., and Serrao, E. (1999). Gamete release at low tide in fucoid algae: maladaptive or advantageous? Am. Zool. 39, 218–229. doi: 10.1093/icb/39.2.218
Caronni, S., Calabretti, C., Ceccherelli, G., Delaria, M. A., Grechi, M., Macri, G., et al. (2016). Gli effetti della mucillagine della microalga invasiva Chrysophaeum taylorii Lewis & Bryan (Pelagophyceae) sulla resilienza delle macroalghe al disturbo meccanico. Biol. Mar. Mediterr. 23, 66–69. doi: 10.1007/s00227-014-2461-3
Caronni, S., Delaria, M. A., Navone, A., Panzalis, P., Sechi, N., and Ceccherelli, G. (2014). Relevant scales of variability of the benthic allochthonous microalga Chrysophaeum taylorii. Mar. Biol. 161, 1787–1798. doi: 10.3354/meps182299
Ceccherelli, G., and Cinelli, F. (1999). The role of vegetative fragmentation in dispersal of the invasive alga Caulerpataxifolia in the Mediterranean. Mar. Ecol. Prog. Ser. 182, 299–303. doi: 10.1515/BOT.2001.027
Ceccherelli, G., and Piazzi, L. (2001). Dispersal of Caulerparacemosa fragments in the Mediterranean: lack of detachment time effect on establishment. Bot. Mar. 44, 209–213. doi: 10.1016/S0022-0981(02)00336-2
Ceccherelli, G., PiazzI, L., and Balata, D. (2002). Spread of introduced Caulerpa species in macroalgal habitats. J. Exp. Mar. Biol. Ecol. 280, 1–11. doi: 10.1126/science.275.5303.1116
Clifton, K. E. (1997). Mass spawning by green algae on coral reefs. Science 275, 1116–1118. doi: 10.1046/j.1529-8817.1999.3510024.x
Clifton, K. E., and Clifton, L. M. (1999). The phenology of sexual reproduction by green algae (Bryopsidales) on Caribbean coral reefs. J. Phycol. 35, 24–34.
Cozzi, S., Margiotta, F., and Saggiomo, V. (2010). “Metodi automatici di analisi dei nutrienti,” in Metodologie di Studio del Plancton Marino, eds G. Socal, I. Buttino, M. Cabrini, O. Mangoni, A. Penna, and C. Totti (Roma: ISPRA, Manuali e Linee Guida), 87–100.
De Biasi, A. M., Gai, F., and Vannucci, A. (1999). Biologia delle Secche della Meloria: considerazioni sull’ecologia di Caulerpa racemosa (Frosskål). J. Agardh. Biol. Mar. Mediterr. 6, 376–379.
Denny, M. W., and Shibata, M. F. (1989). Consequences of surf-zone turbulence for settlement and external fertilization. Am. Nat. 134, 859–889.
Denny, T. P. (1995). Involvement of bacterial polysaccharides in plant pathogenesis. Annu. Rev. Phytopathol. 33, 173–197. doi: 10.1146/annurev.py.33.090195.001133
Dieck, T. I. (1992). North Pacific and North Atlantic digitate Laminaria species (Phaeophyta): hybridization experiments and temperature responses. Phycologia 31, 147–163. doi: 10.2216/i0031-8884-31-2-147.1
Dostal, R. (1929). Untersuchungen über Protoplasmamobilisation beiCaulerpa prolifera. JbWiss Bot. 71, 596–667.
Ellstrand, N. C., and Schierenbeck, K. A. (2000). Hybridization as a stimulus for the evolution of invasiveness in plants? Proc. Natl. Acad. Sci. U.S.A. 97, 7043–7050. doi: 10.1073/pnas.97.13.7043
Ellstrand, N. C., and Schierenbeck, K. A. (2006). Hybridization as a stimulus for the evolution of invasiveness in plants? Euphytica 148, 35–46. doi: 10.1007/s10681-006-5939-3
Endo, H., Mizuta, H., and Uji, T. (2021). α-aminoisobutyric acid mimics the effect of 1-aminocyclopropane-1-carboxylic acid to promote sexual reproduction in the marine red alga Pyropiayezoensis (Rhodophyta). J. Appl. Phycol. 33, 1081–1087. doi: 10.1007/s10811-020-02326-7
Engelen, A. H., Berg, P. A., Olsen, J. L., Stam, W. T., and Breeman, A. M. (2005). Effects of wave exposure and depth on biomass, density and fertility of the fucoid seaweed Sargassumpolyceratium (Phaeophyta, Sargassaceae). J. Phycol. 40, 149–158. doi: 10.1080/09670260500109210
Enomoto, S., and Ohba, H. (1987). Culture studies on Caulerpa (Caulerpales, Chlorophyceae). Reproduction and development of C. racemosa var. laetevirens. Jpn. J. Phycol. 35, 167–177.
Flagella, M. M., Lorenti, M., and Buia, M. C. (2008). Temperature response in a shallow-water Mediterranean population of Caulerparacemosa var. cylindracea (Caulerpales, Chlorophyta), and a possible strategy of season anticipation. Bot. Mar. 51, 278–284. doi: 10.1515/BOT.2008.037
Gambi, M. C., Buia, M. C., Casola, E., and Scardi, M. (1989). “Estimates of water movement in Posidoniaoceanica beds: a first approach,” in International Workshop on Posidonia Beds, ed. C. F. Boudouresque (Paris: GIS Posidonie), 101–112.
Giese, A. C., and Kanatani, H. (1987). “Maturation and spawning,” in Reproduction of Marine Invertebrates, eds A. C. Giese, J. S. Pearse, and V. B. Pearse (Palo Alto, CA: Blackwell Scientific Press), 251–329.
Goldstein, M., and Morrall, S. (1970). Gametogenesis and fertilization in Caulerpa. N. Y. Acad. Sci. Ann. 175, 660–672. doi: 10.1111/j.1749-6632.1970.tb00304.x
Guiry, M. D., and Guiry, G. M. (2020). AlgaeBase. World-Wide Electronic Publication. Design Studio. Available online at: https://www.algaebase.org (accessed July 25, 2020)
Henry, E. C. (1988). Regulation of reproduction in brown algae by light and temperature. Bot. Mar. 31, 353–358. doi: 10.1515/botm.1988.31.4.353
Holmes, M. J., and Brodie, J. (2004). Morphology, seasonal phenology and observations on some aspects of the life history in culture of Porphyradioica (Bangiales, Rhodophyta) from Devon, UK. Phycologia 43, 176–188. doi: 10.2216/i0031-8884-43-2-176.1
Ishihara, K., Shimizu, K., and Yamada, Y. (1981). Pore water pressures measured in sand deposits during an earthquake. Soils Found 21, 85–100. doi: 10.3208/sandf1972.21.4_85
Kalita, T. L., and Tytlianov, E. A. (2003). Effect of temperature and illumination on growth and reproduction of the green alga Ulva fenestrata. Russ. J. Mar. Biol. 29, 316–322. doi: 10.1023/A:1026361627982
Kapraun, D. F., and Nguyen, M. N. (1994). Karyology, nuclear DNA quantification and nucleus-cytoplasmic domain variations in some multinucleate green algae (Siphonocladales, Chlorophyta). Phycologia 33, 42–52. doi: 10.2216/i0031-8884-33-1-42.1
Katsanevakis, S., Issaris, Y., Poursanidis, D., and Thessalou-Legaki, M. (2010). Vulnerability of marine habitats to the invasive green alga Caulerparacemosa var. cylindracea within a marine protected area. Mar. Environ. Res. 70, 210–218. doi: 10.1016/j.marenvres.2010.05.003
Klein, J. C., and Verlaque, M. (2009). Macroalgal assemblages of disturbed coastal detritic bottoms subject to invasive species. Estuar. Coast. Shelf Sci. 82, 461–468. doi: 10.1016/j.ecss.2009.02.003
Klein, J., and Verlaque, M. (2008). The Caulerparacemosa invasion: a critical review. Mar. Pollut. Bull. 56, 205–225.
League, E. A., and Greulach, V. A. (1955). Effects of daylength and temperature on the reproduction of Vaucheriasessilis. Bot. Gaz. 117, 45–51. doi: 10.1086/335889
Lejeusne, C., Chevaldonné, P., Pergent-Martini, C., Boudouresque, C. F., and Pérez, T. (2010). Climate change effects on a miniature ocean: the highly diverse, highly impacted Mediterranean Sea. Trends Ecol. Evol. 25, 250–260. doi: 10.1016/j.tree.2009.10.009
Levitan, D. R., Sewell, M. A., and Chia, F. S. (1992). How distribution and abundance influence fertilization success in the sea urchin Strongylocentotusfranciscanus. Ecology 73, 248–254. doi: 10.2307/1938736
Liu, X., Bogaert, K., Engelen, A. H., Leliaert, F., Roleda, M. Y., and De Clerck, O. (2017). Seaweed reproductive biology: environmental and genetic controls. Bot. Mar. 60, 89–108. doi: 10.1515/bot-2016-0091
Lüning, K., and Tom Dieck, I. (1989). Environmental triggers in algal seasonality. Bot. Mar. 32, 389–397.
Lüning, K., Wagner, A., and Buchholz, C. (2000). Evidence for inhibitors of sporangium formation in Laminariadigitata (Phaeophyceae) during the season of rapid growth. J. Phycol. 36, 1129–1134.
Maier, I. (1993). Gamete orientation and induction of gametogenesis by pheromones in algae and plants. Plant Cell Environ. 16, 891–907. doi: 10.1111/j.1365-3040.1993.tb00513.x
Marbà, N., Jordà, G., Agusti, S., Girard, C., and Duarte, C. M. (2015). Footprints of climate change on Mediterranean Sea biota. Front. Mar. Sci. 2:56. doi: 10.3389/fmars.2015.00056
Meinesz, A. (1979). Contribution to the study of Caulerpaprolifera (Forsskål) lamouroux (Chlorophyceae, Caulerpales). III. Standing crop and primary productivity at a station off the French continental coast of the Mediterranean Sea. Bot. Mar. 22, 123–128. doi: 10.1515/botm.1979.22.2.123
Meinesz, A., and Boudouresque, C. F. (1996). Sur l’origine de CaulerpataxifoliaenMéditerranée. Sciences 319, 603–614.
Miyake, K., and Kunieda, H. (1937). On the sexual reproduction of Caulerpa (preliminary note). Cytologia 8, 205–207. doi: 10.1508/cytologia.8.205
Ohba, H., Nashima, H., and Enomoto, S. (1992). Culture studies on Caulerpa (Caulerpales, Chlorophyceae) III. Reproduction, development and morphological variation of laboratory-cultured C. racemosa var. peltata. Bot. Mag. 105:589. doi: 10.1007/BF02489433
Panayotidis, P., and Žuljević, A. (2001). Sexual reproduction of the invasive green alga Caulerparacemosa var. occidentalis in the Mediterranean Sea. Oceanolacta 24, 199–203. doi: 10.1016/S0399-1784(01)01142-2
Pearson, G. A., and Brawley, S. H. (1996). Reproductive ecology, of Fucusdistichus (Phaeophyceae): an intertidal alga with successful external fertilization. Mar. Ecol. Prog. Ser. 143, 211–223. doi: 10.3354/meps143211
Pearson, G. A., and Brawley, S. H. (1998). A model for signal transduction during gamete release in the fucoid alga Pelvetiacompressa. Plant Physiol. 118, 305–313. doi: 10.1104/pp.118.1.305
Pearson, G. A., and Serrao, E. A. (2006). Revisiting synchronous gamete release by fucoid algae in the intertidal zone: fertilization success and beyond? Integr. Comp. Biol. 46, 587–597. doi: 10.1093/icb/icl030
Pearson, G. A., Serrão, E. A., and Brawley, S. H. (1998). Control of gamete release in fucoid algae: sensing hydrodynamic conditions via carbon acquisition. Ecology 79, 1725–1739.
Pearson, G. A., Serrão, E. A., Dring, M., and Schmid, R. (2004). Blue-and green-light signals for gamete release in the brown alga, Silvetia compressa. Oecologia 138, 193–201. doi: 10.1007/s00442-003-1424-z
Pennington, J. T. (1985). The ecology of fertilization of echinoid eggs: the consequences of sperm dilution, adult aggregation, and synchronous spawning. Biol. Bull. 169, 417–430. doi: 10.2307/1541492
Phillips, J. A. (2009). Reproductive ecology of Caulerpataxifolia (Caulerpaceae, Bryopsidales) in subtropical eastern Australia. Eur. J. Phycol. 44, 81–88. doi: 10.1080/09670260802343640
Piazzi, L., and Cinelli, F. (2003). Evaluation of benthic macroalgal invasion in a harbour area of the western Mediterranean Sea. Eur. J. Phycol. 38, 223–231. doi: 10.1080/1364253031000136358
Piazzi, L., Balata, D., Ceccherelli, G., and Cinelli, F. (2001a). Comparative study of the growth of the two co-occurring introduced green algae Caulerpataxifolia and Caulerparacemosa along the Tuscan coast (Italy, western Mediterranean). Cryptogam. Algol. 22, 459–466. doi: 10.1016/S0181-1568(01)01059-5
Piazzi, L., Ceccherelli, G., and Cinelli, F. (2001b). Threat to macroalgal diversity: effects of the introduced green alga Caulerparacemosa in the Mediterranean. Mar. Ecol. Prog. Ser. 210, 149–159. doi: 10.3354/meps210149
Piazzi, L., Meinesz, A., Verlaque, M., Akcali, B., B, A., Argyrou, M., et al. (2005). Invasion of Caulerparacemosa var. cylindracea (Caulerpales, Chlorophyta) in the Mediterranean Sea: an assessment of the spread. Cryptogam. Algol. 26, 189–202.
Provasoli, L., Yamasu, T., and Manton, I. F. R. S. (1968). Experiments on the resynthesis of symbiosis in Convolutaroscoffensis with different flagellate cultures. J. Mar. Biol. Assoc. U. K. 48, 465–478. doi: 10.1017/S0025315400034603
Ratana-Arporn, P., and Chirapart, A. (2006). Nutritional evaluation of tropical green seaweeds Caulerpalentillifera and Ulva reticulata. Agric. Nat. Resour. 40, 75–83.
Reñé, A., Satta, C. T., Garcés, E., Massana, R., Zapata, M., Anglès, S., et al. (2011). Gymnodiniumlitoralis sp. nov. (Dinophyceae), a newly identified bloom-forming dinoflagellate from the NW Mediterranean Sea. Harmful Algae 12, 11–25. doi: 10.1016/j.hal.2011.08.008
Santelices, B. (2002). Recent advances in fertilization ecology of macroalgae. J. Phycol. 38, 4–10. doi: 10.1046/j.1529-8817.2002.00193.x
Sarà, G., Leonardi, M., and Mazzola, A. (1999). Spatial and temporal changes of suspended matter in relation to wind and vegetation cover in a Mediterranean shallow coastal environment. Chem. Ecol. 16, 151–173. doi: 10.1080/02757549908037644
Serrao, E. A., Brawley, S. H., Hedman, J., Kautsky, L., and Samuelsson, G. (1999). Reproductive success of Fucusvesiculosus (Phaeophyceae) in the Baltic Sea. J. Phycol. 35, 254–269.
Serrão, E. A., Kautsky, L., and Brawley, S. H. (1996). Distributional success of the marine seaweed Fucusvesiculosus L. in the brackish Baltic Sea correlates with osmotic capabilities of Baltic gametes. Oecologia 107, 1–12. doi: 10.1007/BF00582229
Shinkawa, H., Kajikawa, M., and Nomura, Y. (2019). Algal protein kinase, triacylglycerol accumulation regulator 1, modulates cell viability and gametogenesis in carbon/nitrogen-imbalanced conditions. Plant Cell Physiol. 60, 916–930. doi: 10.1093/pcp/pcz010
Silva, P. (1992). Geographic patterns of diversity in benthic marine algae. Pacific Sci. 46, 429–437.
Speransky, S. R., Brawley, S. H., and Halteman, W. A. (2000). Gamete release is increased by calm conditions in the coenocytic green alga Bryopsis (Chlorophyta). J. Phycol. 36, 730–739. doi: 10.1046/j.1529-8817.2000.99199.x
Sudatti, D. B., Fujii, M. T., Rodrigues, S. V., Turra, A., and Pereira, R. C. (2011). Effects of abiotic factors on growth and chemical defenses in cultivated clones of Laurenciadendroidea J. Agardh (Ceramiales, Rhodophyta). Mar. Biol. 158, 1439–1446. doi: 10.1007/s00227-011-1660-4
Underwood, A. J. (1997). Experiments in Ecology: Their Logical Design and Interpretation Using Analysis of Variance. Cambridge: Cambridge University Press.
Underwood, A. J., Chapman, M. G., and Richards, S. A. (2002). GMAV-5 for Windows. An Analysis of Variance Programme. Camperdown, NSW: University of Sydney.
Varela-Alvarez, E., Garreta, A. G., Lluch, J. R., Salvador Soler, N., Serrao, E. A., and Siguán, M. A. (2012). Mediterranean species of Caulerpa are polyploid with smaller genomes in the invasive ones. PLoS One 7:e47728. doi: 10.1371/journal.pone.0047728
Verlaque, M., Boudouresque, C. F., Meinesz, A., and Gravez, V. (2000). The Caulerparacemosacomplex (Caulerpales, Ulvophyceae) in the Mediterranean sea. Bot. Mar. 43, 49–68. doi: 10.1515/BOT.2000.005
Verlaque, M., Durand, C., Huisman, J. M., Boudouresque, C. F., and Le Parco, Y. (2003). On the identity and origin of the Mediterranean invasive Caulerparacemosa (Caulerpales, Chlorophyta). Eur. J. Phycol. 38, 325–339. doi: 10.1080/09670260310001612592
Wichard, T., and Oertel, W. (2010). Gametogenesis and gamete release of Ulva mutabilis and Ulva lactuca (Chlorophyta): regulatory effects and chemical characterization of the “swarming inhibitor”1. J. Phycol. 46, 248–259.
Yund, P. (2000). How severe is sperm limitation in natural populations of marine free-spawners? Trends Ecol. Evol. 15, 10–13. doi: 10.1016/s0169-5347(99)01744-9
Žuljević, A., and Antolić, B. (2000). Synchronous release of male gametes of Caulerpataxifolia (Caulerpales, Chlorophyta) in the Mediterranean Sea. Phycologia 39, 157–159. doi: 10.2216/i0031-8884-39-2-157.1
Keywords: Tyrrhenian Sea, Caulerpa cylindracea, gametogenesis, spawning, environmental factors, temperature
Citation: Caronni S, Delaria MA, Gentili R, Montagnani C, Navone A, Panzalis P and Citterio S (2021) First Report of Gametogenesis and Spawning for the Invasive Alga Caulerpa cylindracea in the Tyrrhenian Sea: The Key Role of Water Motion and Temperature. Front. Mar. Sci. 8:774274. doi: 10.3389/fmars.2021.774274
Received: 11 September 2021; Accepted: 28 October 2021;
Published: 06 December 2021.
Edited by:
Periklis Kleitou, Marine and Environmental Research Lab (MER), CyprusReviewed by:
Ana Alexandre, University of Algarve, PortugalCopyright © 2021 Caronni, Delaria, Gentili, Montagnani, Navone, Panzalis and Citterio. This is an open-access article distributed under the terms of the Creative Commons Attribution License (CC BY). The use, distribution or reproduction in other forums is permitted, provided the original author(s) and the copyright owner(s) are credited and that the original publication in this journal is cited, in accordance with accepted academic practice. No use, distribution or reproduction is permitted which does not comply with these terms.
*Correspondence: Sarah Caronni, YXJhaC5jYXJvbm5pQHVuaW1pYi5pdA==
†These authors have contributed equally to this work
Disclaimer: All claims expressed in this article are solely those of the authors and do not necessarily represent those of their affiliated organizations, or those of the publisher, the editors and the reviewers. Any product that may be evaluated in this article or claim that may be made by its manufacturer is not guaranteed or endorsed by the publisher.
Research integrity at Frontiers
Learn more about the work of our research integrity team to safeguard the quality of each article we publish.