- 1Biodiversity Research Center, Academia Sinica, Taipei, Taiwan
- 2Division of Biological Science, Faculty of Science, Prince of Songkla University, Songkhla, Thailand
- 3Department of Biology, Faculty of Science, Burapha University, Chonburi, Thailand
The present study investigated the phylogeography of the intertidal barnacle Tetraclita in the Gulf of Thailand ecoregion (Sunda Shelf Province in the Pacific) and the Andaman Sea Coral Coast ecoregion (Andaman Province in the Indian Ocean) in Thailand’s waters. Tetraclita species were identified by a combined morphological and molecular approach using mitochondrial gene fragments (CO1 and 12S rRNA). Tetraclita singaporensis is a major occupiers on the Andaman coast but is sparse in the western Gulf of Thailand. Tetraclita squamosa inhabits almost all of our collection sites in the Gulf of Thailand but has a very low abundance in the Andaman Sea. Tetraclita kuroshioensis has two genetically distinct populations, one in the Andaman Sea and the other in the West Pacific region. S-DIVA analysis showed that the most recent common ancestor (MRCA) of T. kuroshioensis was distributed in both the Andaman Sea and West Pacific region, with a relative probability of 63%; the analysis further identified two molecular subclades, one on each side of the Sunda Shelf by vicariance about 0.53 million years ago, far before the Last Glacial Maximum (LGM). The MRCA of T. squamosa was based in the West Pacific region (relative probability: 90%), and dispersed into the Andaman Sea after the LGM. The MRCA of T. singaporensis was in the Andaman Sea (relative probability: 89.5%) and dispersed into the Gulf of Thailand via monsoonal currents through the Malacca Strait after the LGM. Presently, T. singaporensis is absent from the West Pacific region, further supporting its origin in the Andaman Sea. The distribution of intertidal barnacles in the Sunda Shelf and Andaman provinces is a result of the interplay between geological events and present day oceanographic currents.
Introduction
The Indo-Pacific region contains the world’s highest marine biodiversity (Roberts et al., 2002), a result of interactions among ocean topography, complicated oceanographic current patterns, and geological events such as sea level fluctuations (Ni et al., 2014). Intertidal species often have planktonic larval stages and a benthic adult stage. Intertidal species are mainly dispersed by the planktonic phases which can be affected by the length of the planktonic larval development period, present day oceanographic current patterns, and geological historical events, such as Pleistocene glaciations and sea level fluctuations (Tsang et al., 2012).
Intertidal barnacles of the genus Tetraclita are common in the mid to low shores of the rocky intertidal zone in the Indo-Pacific region (Newman and Ross, 1976). Before the use of DNA barcodes in species diversity studies, Tetraclita squamosa was believed to be distributed throughout the Indo-Pacific waters (Pilsbry, 1916; Newman and Ross, 1976). However, recent population genetic studies revealed that T. squamosa is composed of several distinct Evolutionary Significant Units (ESU), some of which were identified as cryptic species, including Tetraclita kuroshioensis and Tetraclita singaporensis (Chan et al., 2007a,b). In the West Pacific region, T. squamosa is present on the continental coastlines of southern China in the South China Sea region. T. kuroshioensis is common in the Pacific waters, including Taiwan and Japan, but absent from the continental coasts of China. T. singaporensis is mainly distributed in the Malay Peninsula. According to previous phylogenetic analyses (Chan et al., 2007b; Tsang et al., 2015), these three Tetraclita species are closely related to the family Tetraclitidae. Among them, T. singaporensis is the most recently derived species. Moreover, Tetraclita serrata, distributed in the West Indian Ocean, is sister to T. kuroshioensis (Tsang et al., 2012, 2015).
The coastlines of Thailand cover two marine ecosystems and two marine provinces, and supports a high diversity of Tetraclita species (Figures 1A,B; Pochai et al., 2017). The Gulf of Thailand ecoregion is located in Sunda Shelf Province (Eastern coastline of Thailand) and the Andaman Coral Coast ecoregion in Andaman Province (Western coastline of Thailand) (Spalding et al., 2007). The Gulf of Thailand receives a strong southwest flow of currents from the South China Sea along the gulf entrance. Within the gulf, there are counterclockwise eddies during the southwest monsoon season and a clockwise circulation during the northeast monsoon (Sojisuporn et al., 2010; Figures 1C,D). It appears that the planktonic larvae in the Gulf of Thailand can be retained from these cyclonic eddies. The Andaman Coral Coast ecoregion is located in the Andaman Sea facing the Indian Ocean. The Andaman Sea experiences current circulation generated from equatorial forces mediated by local winds (Figures 1C,D). During the Pleistocene glaciation periods, sea level decreased 120 m, totally exposing the Gulf of Thailand and partially isolating the Andaman Sea (Hall, 1998; Voris, 2000). The species living in the Gulf of Thailand colonized this region after the sea level rises and thus are relatively younger in geological age than the Andaman Sea. As a result, a phylogeographic break formed between the Gulf of Thailand and Andaman Sea for some species, including sea urchins (Coppard et al., 2021) and the barnacles Chthamalus malayensis (Tsang et al., 2012) and Octomeris brunnea (Chan et al., 2020). The currents and environmental differences between the two sides of the Malay Peninsula resulted in variation in the diversity and morphology of seaweeds in the Gulf of Thailand and Andaman Sea (Pongparadon et al., 2015, 2017). However, there are many marine species that have genetically homogeneous population across these two ecoregions, probably due to high dispersal abilities following the glaciations (e.g., the trumpetfish Aulostomus chinensis, Bowen et al., 2001; sea urchin Tripneustes, Lessios et al., 2003, gastropod Echinolittorina reticulata, Reid et al., 2006, seaweed Sargassum, Chan et al., 2013, 2014).
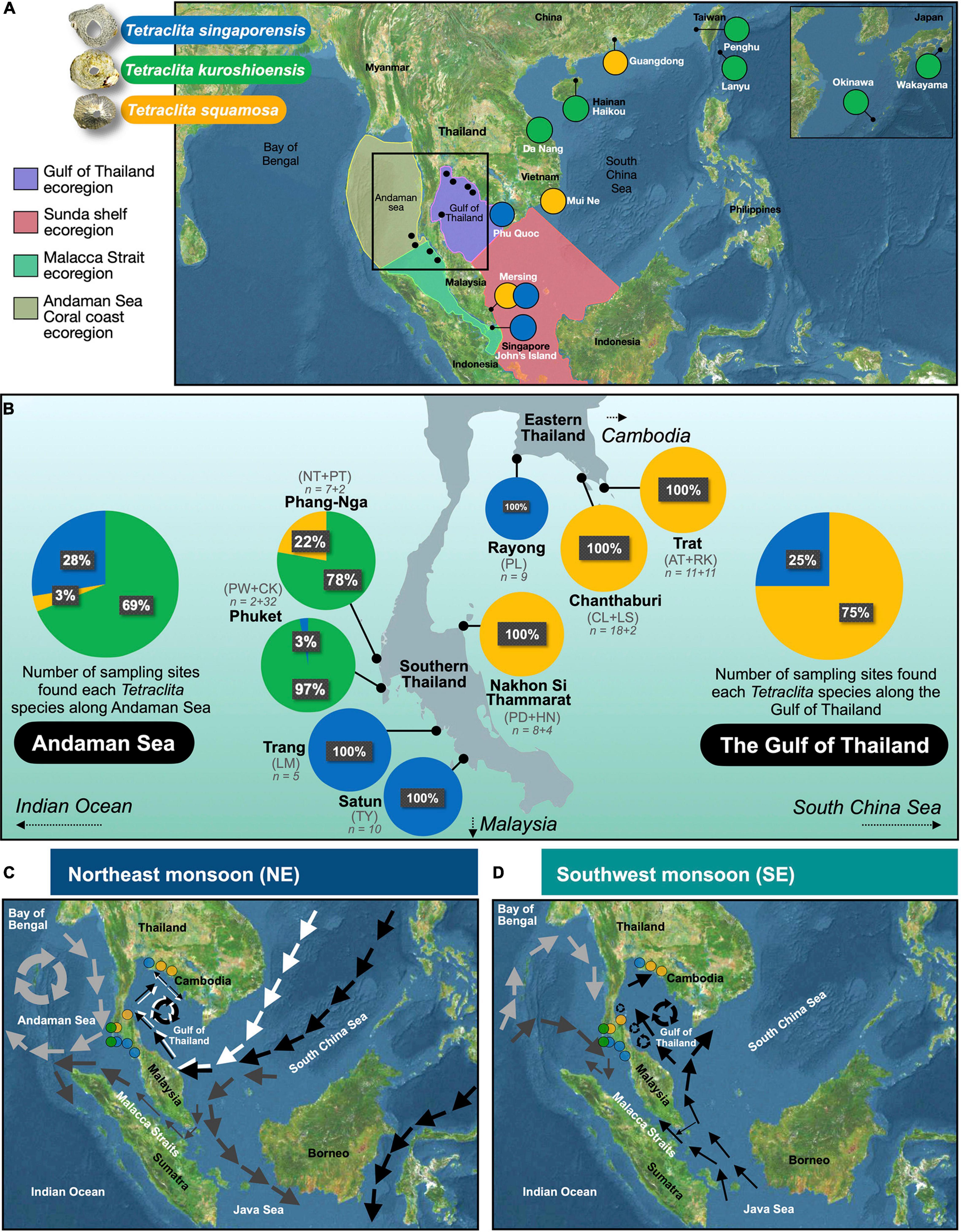
Figure 1. (A) Sampling sites in the Gulf of Thailand and Andaman Sea of Thailand waters (represented by black dots in the rectangle, which are magnified in B). The dots outside the rectangle represents locations of Tetraclita sequences obtained from Genbank and used in the present study. (B) Sampling site of Tetraclita spp. in the present study and the relative percentage of Tetraclita spp. in each site. (C) Oceanographic currents in the South China Sea and Andaman Sea in the NE monsoon. (D) Oceanographic currents in the South China Sea and Andaman Sea in the SW monsoon. Current patterns following the illustration in Pongparadon et al. (2015). During the northeast monsoon, there are circular clockwise currents around the Nicobar Islands that pass through the upper Andaman Sea. Dark gray arrows represent the currents from the South China Sea passing through the Singapore Straits and entering Malacca Strait. White and black arrows represent currents from the west Pacific and Philippines region that enter the Gulf of Thailand. During the SW monsoon, light gray arrows represent currents from the Indian Ocean passing through the Andaman Islands and flowing around the upper Andaman Sea. Dark gray arrows indicate currents from the Indian Ocean passing through the southern waters of Nicobar Islands and entering the lower region of the Andaman Sea. Black arrows indicate currents from the Java Sea flowing northwards to Singapore, then the eastern coast of the Malay Peninsula, and entering the Gulf of Thailand. Coordinates of study sites are in Table 1. Tetraclita singaporensis (blue dot), Tetraclita kuroshioensis (green dot), and Tetraclita squamosa (yellow dot). Ecoregions stated in Spalding et al. (2007) are highlighted in (A). Panel (A,C,D) is based on map generated by Ahoy Map Maker.
Pochai et al. (2017) and Sukparangsi et al. (2019) surveyed the diversity of barnacles in Thailand and revealed that the species composition of barnacles is different between the Gulf of Thailand and Andaman Coral Sea ecoregions. This suggest that species diversity in these two ecoregions can be affected by present-day oceanographic currents or geological events. To further understand these two effects, it is essential to conduct fine-scale spatial samplings and study how barnacles differ genetically among multiple sites within each ecoregion. In the present study, we conducted samplings of Tetraclita species across their intertidal range from multiple sites in the Gulf of Thailand and Andaman Coral Coast ecoregions, including the specimens from other sampling sites in the West Pacific region. We test the hypothesis that there are genetic differentiations in Tetraclita, including T. singaporensis and T. kuroshioensis, within and between the Gulf of Thailand and Andaman Coral Coast ecoregions.
Materials and Methods
Study Sites
We collected Tetraclita spp. from 13 sampling stations distributed in the Gulf of Thailand and Andaman coast of Thailand (Table 1 and Figure 1B). We also included specimens from nine sampling stations distributed in Japan, Taiwan, Vietnam, Malaysia, and Singapore (Supplementary Table 1).
Sampling Collection
Specimens were collected during the period of spring low tides with the lowest tides of the year at all sampling sites. As a result, all Tetraclita spp. were collected from their entire vertical distribution ranges. Specimens were immediately stored in 95% Ethanol before morphological and DNA analyses. The collection of barnacles followed the ethical principles and guidelines for the use of animals in Thailand (Protocol number: IACUC 001/2564 for project: Barnacle Database in Eastern Thailand).
Morphological Analysis
Soft body tissues and opercular plates were removed from shell plates. Photographs of shell plates and opercular plates were taken using a stereomicroscope equipped with a digital camera. Cirri and mouth parts were dissected and examined under compound light microscope with a digital camera. Species identifications and diagnoses followed Chan (2001) and Chan et al. (2007a,b).
DNA Extraction, Amplification, and Sequencing
Total genomic DNA was extracted from the muscle tissue using Qiagen DNeasy Blood & Tissue Kits (Qiagen, CA, United States) according to the manufacturer’s instructions. Partial sequences of mitochondrial DNA markers, CO1, and 12S rRNA were amplified following the protocol from previous studies (Folmer et al., 1994; Mokady et al., 1994; Schubart and Huber, 2006; Tsang et al., 2009; Chen et al., 2012; Supplementary Table 2). PCR reactions were conducted in a DNA Engine Thermal Cycler (Bio-Rad, Richmond, CA, United States), and the products were checked by electrophoresis on 1.5% agarose gel in 1 × TAE buffer. DNA sequencing was performed by Genomics BioSci & Tech Ltd. (New Taipei City, Taiwan). The sequences were assembled and edited in Geneious Prime 2020.1.11.
DNA Barcoding for Species Identification
The following sequences were downloaded from GenBank and used for reference: 29 of Tetraclita spp., three of Tetraclitella divisa, three of Tesseropora rosea, one of Yamaguchiella coerulescens, and one of Balanus (Chan et al., 2007a,b; Tsang et al., 2007, 2012, 2015; Zardus et al., 2013; Shen et al., 2015; Song et al., 2017; Feng et al., 2020; Ji et al., 2021; Supplementary Table 1). All the sequences were aligned with MAFFT in Geneious Prime 2020.1.1 (see Text Footnote 1). Automatic Barcode Gap Discovery (ABGD) was used for species delimitation with the default option (Puillandre et al., 2012). K2P genetic distances were also calculated in MEGA X (Kumar et al., 2018).
Genetic Diversity and Demographic Analysis
The CO1 and 12S rRNA sequences of Tetraclita spp. from Thailand were aligned with MAFFT and trimmed to the same length in Geneious Prime 2020.1.1 (see Text Footnote 1). Nucleotide diversity (π) and haplotype diversity (h) were calculated using DnsSP version 6.11.01 (Rozas et al., 2017).
The datasets of CO1 and 12S rRNA were concatenated in Geneious Prime 2020.1.1 (see Text Footnote 1) before further analysis. Haplotypes of concatenated sequences were identified by DnaSP version 6.11.01 (Rozas et al., 2017). Population demography was estimated by mismatch distribution under the sudden expansion model using Arlequin version 3.5.2.2 (Excoffier and Lischer, 2010). The time since population expansion (t) was further estimated with the equation t = τ/2μ, where τ was calculated by Arlequin version 3.5.2.2 with the CO1 dataset, and μ is evolutionary rate × sequence length × generation time (Rogers and Harpending, 1992; Excoffier and Lischer, 2010). Two published CO1 evolutionary rates for the intertidal barnacles were used (1.55 × 10–8 and 2.76 × 10–8 substitutions per site per generation; Wares, 2001; Wares and Cunningham, 2001), and the generation time was assumed to be 2 years (Dawson et al., 2010). The relationships among haplotypes within different Tetraclita spp. were inferred by the TCS network using PopART (Leigh and Bryant, 2015).
Phylogenetic Inference and Divergence Time Estimation
The phylogenetic trees were inferred from CO1 and 12S rRNA concatenated sequences using Bayesian inferences (BI) and maximum likelihood (ML) in MrBayes 3.2.6 and W-IQ-TREE, respectively (Ronquist and Huelsenbeck, 2003; Nguyen et al., 2015; Trifinopoulos et al., 2016). Selected sequences downloaded from GenBank—including Tetraclita japonica (AB126701), T. kuroshioensis (MW298526), T. rufotincta (KY865100), T. serrata (KJ434948), T. squamosa (MT232759), T. rosea (KY865099), and T. divisa (KJ754822)—were included in the analysis (Shen et al., 2015; Song et al., 2017; Tsang et al., 2017; Cai et al., 2018; Feng et al., 2020; Ji et al., 2021). The sequences of Balanus balanus (KM660676), Chthamalus antennatus (KP294312), and Pollicipes polymerus (AY456188) were also used as outgroups (Lavrov et al., 2004; Shen et al., 2016).
Bayesian inferences were conducted with 5 × 106 generations with six MCMC chains. GTR + I + G was selected for both markers according to the results by jModeltest2 (Guindon and Gascuel, 2003; Darriba et al., 2012). The analyses were performed using three independent runs. Trees were saved every 1,000 generations, and the first 10% of trees were discarded as burn-in. The results of the three independent runs were combined using Tracer v1.7 and the effective sample size (ESS) of all parameters was >200 (Rambaut et al., 2018).
Maximum likelihood was conducted with 1,000 bootstrap replicates for a Shimodaira–Hasegawa approximate likelihood ratio test (SH-aLRT) and ultrafast bootstrap approximation (UFB) (Guindon et al., 2010; Hoang et al., 2018). GTR + F + I + G4 and TPM3u + F + G4 were selected as the best-fit model under the Bayesian information criterion for CO1 and 12S rRNA, respectively (Kalyaanamoorthy et al., 2017).
RealTime-ML analysis was conducted with the GTR + I + G model to estimate divergence time using MEGA X (Kumar et al., 2018). Two fossil records from the Upper Cretaceous and Lower Miocene were used as calibration points and distributed as exponential priors (Buckeridge, 1983, 2008; Pérez-Losada et al., 2008; Table 2).
Biogeographic Histories Inference
To infer the biogeographic histories for the Tetraclita spp. in Thailand, a statistical dispersal-vicariance analysis (S-DIVA) was conducted by RASP version 4.2 (Yu et al., 2010, 2015). The post-burnin trees and condensed tree from BI results were used as the input data. The distributions of haplotypes were categorized into two regions: the Indian Ocean region—including the Andaman Sea and the Malacca Strait ecoregions—and the West Pacific region—including the Gulf of Thailand ecoregion, Sunda shelf ecoregion, South China Sea, and sampling stations in Japan and Taiwan. We also designated the T. serrata specimen from the West Indian Ocean into the Indian Ocean region.
Results
Molecular Identification and Morphological Description of Tetraclita
According to the results of the ABGD analysis (Supplementary Table 1), all the specimens collected from Thailand were designated into three species, T. kuroshioensis, T. singaporensis, and T. squamosa. The K2P genetic distances are shown in Supplementary Table 3. For CO1 sequences, the intraspecific K2P distances were 0.006 for T. singaporensis, 0.011 for T. kuroshioensis, and 0.002 for T. squamosa. The interspecific K2P distances between these three species and other Tetraclitids (Tetraclitella and Tesseropora) ranged from 0.125 to 0.250. For 12S rRNA sequences, the intraspecific K2P distances were 0.008 for T. singaporensis, 0.011 for T. kuroshioensis, and 0.001 for T. squamosa. The interspecific K2P distances between these three species and other Tetraclitadae (Tetraclitella and Tesseropora) ranged from 0.073 to 0.227. The diagnostic morphological descriptions are summarized below.
Tetraclita singaporensis (Chan et al., 2007b)
Materials examined (based on CO1 and 12S rRNA): Total 25 specimens including Rayong, 9 specimens (T1–T2, T4–T10); Phuket, 1 specimen (T75); Trang, 5 specimens (T78–T82); Satun, 10 specimens (T83–T92), see detailed voucher information in Supplementary Table 1.
GenBank accession number: CO1: OK667807–OK667815, OK667877–OK667891; 12S rRNA: OK667951–OK667959, OK668025–OK668039.
Diagnosis: Shell dark green or greenish-gray with multi-tubiferous basis; Shell operculum small, Tergum not beaked, narrow and vertically elongated (Figure 2 and Supplementary Figure 1).
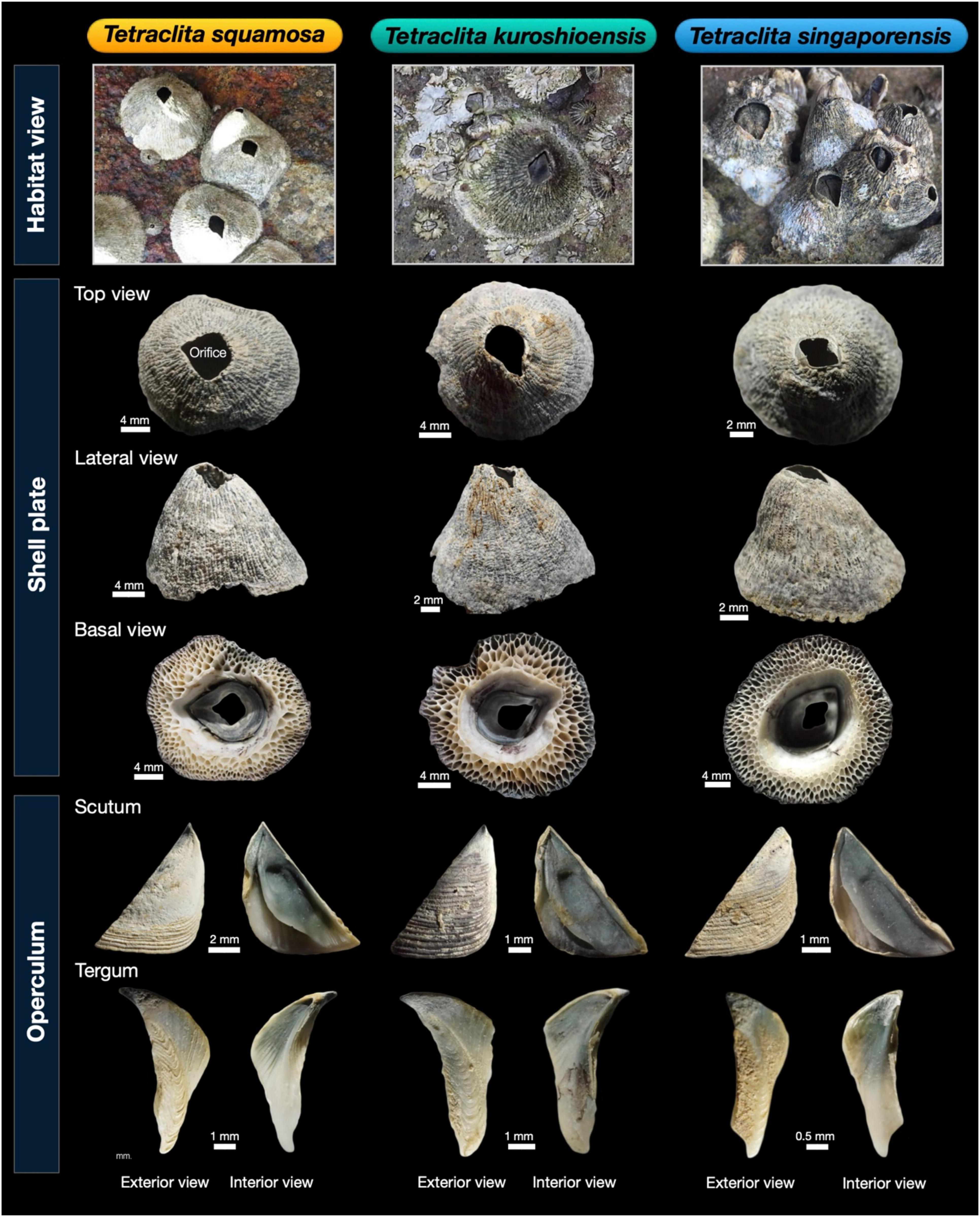
Figure 2. Morphology of shell and opercular plates (tergum and scutum) of Tetraclita spp. specimens from Thailand.
Remarks: T. singaporensis was identified in Singapore by Chan et al. (2007b). Subsequently, Tsang et al. (2011) revealed this species to be present along the coastline of the Malay peninsula, including the Andaman Sea and Gulf of Thailand. Detailed taxonomic identification refers to Chan et al. (2007b) and Supplementary Information.
Tetraclita kuroshioensis (Chan et al., 2007a,b)
Materials examined (based on CO1 and 12S rRNA): Total 40 specimens including Phang-Nga, 7 specimens (T66–T72); Phuket, 33 specimens (T76, T93–T124), see detailed information and voucher specimen number in Supplementary Table 1.
GenBank accession number: CO1: OK667869–OK667874, OK667892–OK667923; 12S rRNA: OK668014–OK668020, OK668024, OK668040–OK668071.
Diagnosis: Shell dark green or greenish-gray with multitubiferous basis; Tergum not beaked, width of the basal portion of tergum is twice as T. singaporensis (Figure 2 and Supplementary Figure 1).
Remarks: Detailed morphological description in Chan et al. (2007a).
Tetraclita squamosa (Bruguière, 1789)
Materials examined (based on CO1 and 12S rRNA): Total 56 specimens including Chanthaburi, 20 specimens (T11–T30); Trat, 22 specimens (T31–T53); Nakhon Si Thammarat, 12 specimens (T54–T65); Phang-Nga, two specimens (T73–T74), see detailed voucher information in Supplementary Table 1.
GenBank accession number: CO1: OK667816–OK667868, OK667875–OK667876; 12S rRNA: OK667960–OK668013, OK668021–OK668022.
Diagnosis: Shell dark green or greenish-gray with multitubiferous basis; Tergum beaked, carinal margin strongly curved, spur sharp when compared to the other two T. singaporensis and T. kuroshioensis (Figure 2 and Supplementary Figure 1).
Remarks: T. squamosa was reported to be the widest distributed species from Pilsbry (1916). From molecular DNA barcode analysis, T. squamosa composed of several cryptic species including T. kuroshioensis and T. singaporensis, which has different geographical ranges. Morphological description of T. squamosa can referred to Chan et al. (2007a).
Biogeography of Tetraclita in the Gulf of Thailand and Andaman Sea
There is diagnostic variation in the distribution of Tetraclita spp. between the Andaman Sea Coral Coast ecoregion and the Gulf of Thailand ecoregion. T. singaporensis was found mostly in the Andaman Sea Coral Coast ecoregion, including Phuket (3% relative abundance among T. kuroshioensis, T. squamosa, and T. singaporensis), Trang (100%), and Satun (100%). Trang and Satun are located closer to Malaysia in the Strait of Malacca side and colonized exclusively with T. singaporensis. In addition to T. singaporensis, T. kuroshioensis was found in parts of the Andaman Sea, including Phang-nga (78%) and Phuket (97%) (Figure 1B). In the Gulf of Thailand ecoregion, T. squamosa occupied almost all sampling sites, including Chanthaburi (100%), Trat (100%), and Nakorn Si Thammarat (100%). However, T. singaporensis was found in the Gulf of Thailand, in Rayong (100%), which is the closest sampling site to the upper region of the Gulf of Thailand and Chao Phraya estuary. In the Andaman Coral Coast ecoregion, T. squamosa was only found in Phang-nga (22%).
Genetic Diversity and Population Demography
The concatenated sequences (CO1 + 12S) of Tetraclita spp. were 1,077 bp, including 578 bp of CO1 and 500 bp of 12S rRNA sequences. Genetic variations were shown in Table 3. Among these three species, the nucleotide and haplotype diversities in T. kuroshioensis and T. singaporensis were much higher than in T. squamosa. For T. kuroshioensis and T. singaporensis, the nucleotide and haplotype diversities in the West Pacific region were higher than in the Indian Ocean region, except for the nucleotide diversity of CO1 in T. singaporensis. We only found two specimens of T. squamosa in the Indian Ocean region, and these specimens had identical 12S rRNA sequences and only differed by 1 bp in their CO1 sequences. In the West Pacific region, we collected many more specimens of T. squamosa than the other two species; however, only six haplotypes in CO1 and seven in 12S rRNA were found.
The mismatch distribution in T. singaporensis (Figure 3A) was multimodal, T. squamosa was unimodal (Figure 3B) and T. kuroshioensis was multimodal (Figure 3C). The sum of squared deviation (SSD) and Harpending’s raggedness index (R) of all these three Tetraclita species were not significantly different (Table 4). It was suggested that the sudden expansion model cannot be rejected for all three species. Tajama’ D and Fu’s Fs values were all significantly negative (Table 4). The estimated time since the population expansions were about 0.18–0.10 million years ago (mya) for T. singaporensis, 0.08–0.05 mya for T. squamosa, and 0.04–0.03 mya for T. kuroshioensis, based on τ values of 6.416, 3.000, and 1.658, respectively.
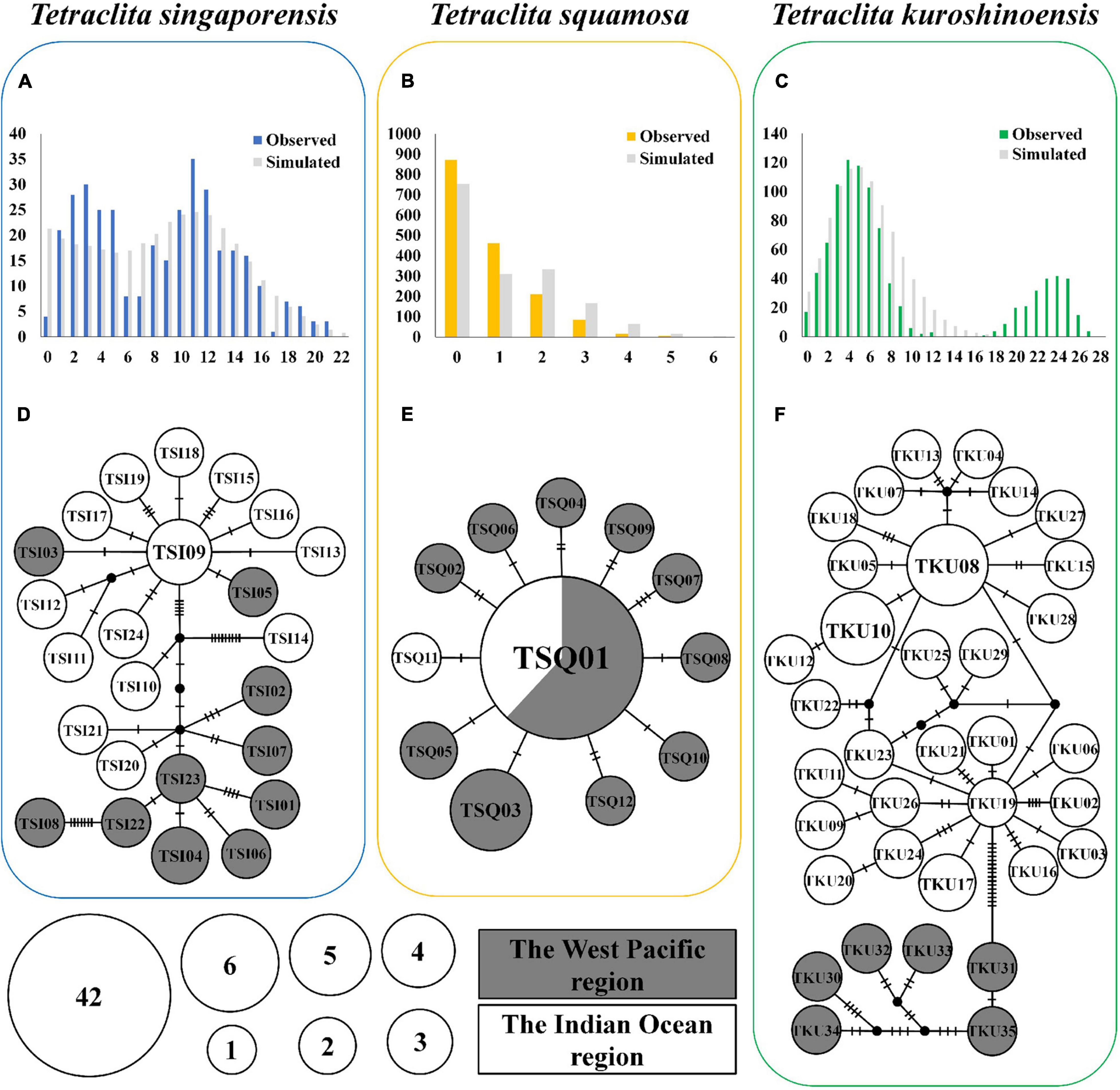
Figure 3. Mismatch distribution (A–C) and haplotype network (D–F) from CO1 and 12S rRNA concatenated sequences of Tetraclita spp. The results of mismatch distribution are shown in the histograms. The abscissa indicates the number of pairwise difference between specimens, and the ordinate indicates the frequency of each value. The colored and gray bars represent the frequency distribution of the observed and expected pairwise difference, respectively, under the sudden expansion model. For the haplotype network, the size of each circle represents the number of specimens. The haplotype numbers are shown in each circle, and details on each haplotype can be found in Supplementary Table 1.
A total of 71 haplotypes were observed from CO1 and 12S rRNA concatenated sequences: 24 of T. singaporensis, 12 of T. squamosa, and 35 of T. kuroshioensis (Figures 3D–F and Supplementary Table 1). Shared haplotypes from different localities were observed in T. singaporensis and T. squamosa (TSI09, TSQ01, and TSQ03) (Supplementary Table 1). Among these haplotypes, TSQ01 had the widest distribution—not only the Gulf of Thailand and Andaman Sea, but also Mui Ne, Vietnam (TKMNV01) and Mersing, Malaysia (TKMSM08) (Figure 3E and Supplementary Table 1). For the TCS network, haplotypes from the same region were not always connected to each other in T. singaporensis (Figure 3D). For T. squamosa, the network structure was star-shaped, with 11 haplotypes connected to the central haplotype TSQ01 (Figure 3E). For T. kuroshioensis, haplotypes can be separated into two groups: those from the Indian Ocean region (TKU01–29) and those from the Western Pacific region (TKU30–35). Among these haplotypes, TKU08 and TKU19 distributed in Phuket (CK) were connected to more than six haplotypes from Phuket (CK) and Phang-nga (NT) (Figure 3F).
Phylogeny and Divergence Times
Phylogenetic trees inferred by BI and ML were both consistent with the previous studies (Chan et al., 2007b; Tsang et al., 2012, 2015; Figure 4). The three Tetraclita spp. formed a monophyletic clade with T. serrata. Among them, T. singaporensis was derived the earliest. T. kuroshioensis was sister to T. serrata with high posterior probabilities and bootstrap support and was further divided into two subclades, one consisting of the specimens from Phang-Nga and Phuket, Thailand (Andaman Sea) and the other consisting of the specimens from Vietnam, Taiwan, and Japan (Western Pacific region); this is consistent with the results of the haplotype network (Figures 3F, 4). However, the posterior probability and bootstrap support were not high for subclade 2 (<85).
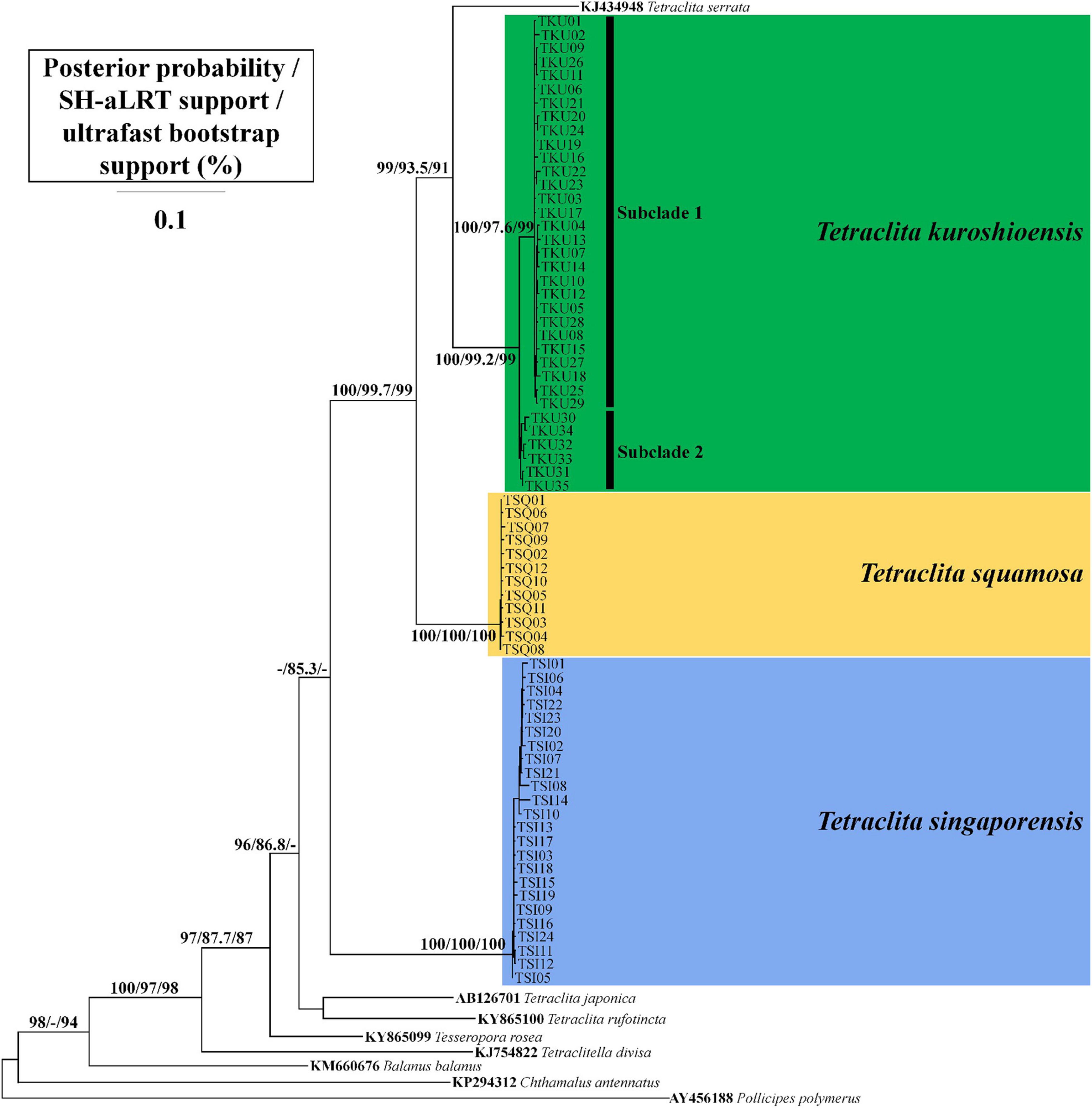
Figure 4. Phylogenetic tree of Tetraclita spp. in Thailand based on the CO1 and 12S rRNA concatenated sequences by maximum likelihood (ML). Posterior probability for Bayesian inference (BI), SH-aLRT, and ultrafast bootstrap support for ML are presented at the main nodes when >85.
Tetraclita singaporensis derived from the other three species (including T. serrata) was inferred at 12.65 mya during the Middle-Late Miocene (Figure 5A). T. squamosa was then derived from T. kuroshioensis and T. serrata at 6.70 mya during the Late Miocene (Figure 5A). The divergence time between T. kuroshioensis and T. serrata were inferred at 4.66 mya during the Pliocene (Figure 5A).
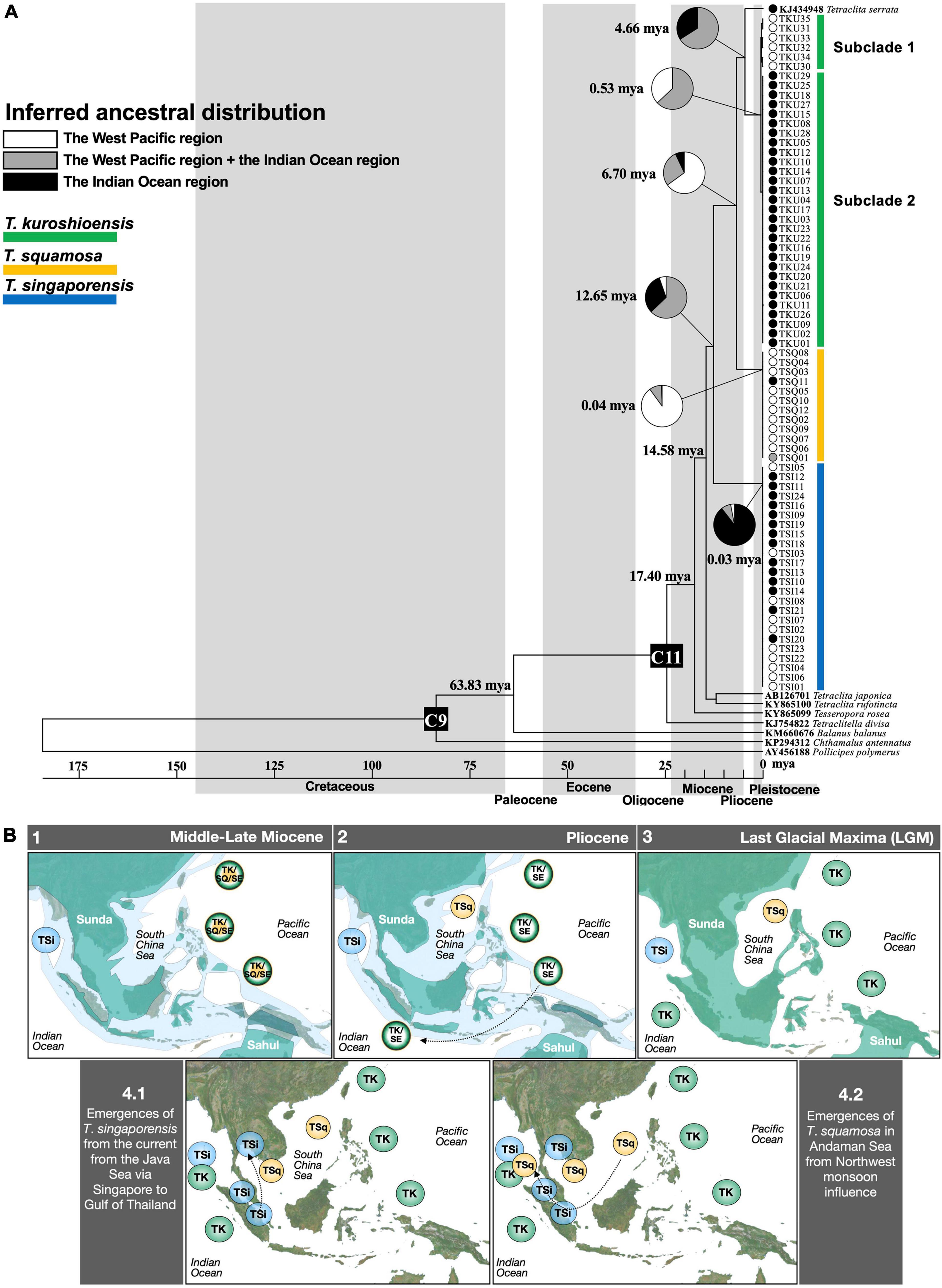
Figure 5. (A) Ancestral distributions inferred by statistical dispersal-vicariance analysis (S-DIVA) and divergence time. The probabilities of alternative ancestral ranges at each node are in different colors in the pie charts. The divergence times (million years ago) estimated by RealTime-ML analysis are also shown at the main nodes. C9 stands for the calibration point of the fossils of Pachydiadema (Catophragmus) cretacea. C11 stands for the calibration point of the fossils Tetraclitella judiciae. These two calibration points were used in Pérez-Losada et al. (2008). (B) Paleobiogeography hypothesis of Tetraclita distribution around the Sunda Shelf from the Middle-Late Miocene to present day. LGM and date of sea fluctuation are adapted from Crandall et al. (2012). See the Discussion section for detail. Green indicates the exposed land. Green in (3) indicates the region that was an exposed landmass during the Pleistocene glaciations. Light blue indicate shallow seas. Location of landmass in different geological time followed Hall (1998). TK, Tetraclita kuroshioensis; TSi, Tetraclita singaporensis; TSq, Tetraclita squamosa; TK/SQ/SE, common ancestor of T. kuroshioensis, T. squamosa and T. serrata; TK/SE, common ancestor of T. kuroshioensis and T. serrata.
Ancestral Distribution of Tetraclita spp.
The distribution of the most recent common ancestor (MRCA) of T. kuroshioensis, T. squamosa, T. singaporensis, and T. serrata was inferred in both the West Pacific and Indian Ocean regions with a relative probability of 62.8%, and T. singaporensis in the Indian Ocean region was further divided from the other three species in the West Pacific region by vicariance. The MRCA of T. squamosa was inferred in the West Pacific region (relative probability: 90%), and the MRCA of T. kuroshioensis was inferred in both the Indian Ocean and West Pacific region (relative probability: 63%) or the West Pacific region (relative probability: 37%).
Discussion
Tetraclita is an intertidal barnacle widely distributing in the Indo-West Pacific that comprises several morphologically similar cryptic species. It is difficult to analyze the biogeography and phylogeography of Tetraclita based only on morphological species identifications because they have large intra-specific morphological variations. Molecular data based on analysis of two mitochondrial DNA genes (CO1 and 12S rRNA) allowed us to precisely identify Tetraclita spp. in the Gulf of Thailand ecoregion and Andaman Coral Coast ecoregion. Previously, T. singaporensis, T. kuroshioensis, and T. squamosa in Thailand have been qualitatively reported by Pochai et al. (2017). Unlike the present study, Pochai et al. (2017) recorded T. singaporensis only in the Andaman Sea and T. squamosa only in the Gulf of Thailand; this is probably because Pochai et al. (2017) based their identifications solely on morphology and used fewer sampling sites. In the present study, we expanded the scale of biogeographical collection of Tetraclita spp. to cover both the Gulf of Thailand and Andaman Sea Coral Coast ecoregions. According to DNA analysis, T. singaporensis is present on both sides of the Malay Peninsula (Figure 1B) but absent from the West Pacific and South China Sea. T. squamosa’s major populations are in the South China Sea and Gulf of Thailand, with some scattered populations on the Andaman coasts. T. kuroshioensis is present in both the Andaman Sea and the West Pacific region (Tsang et al., 2011). There are two clades of T. kuroshioensis in the molecular phylogenetic tree: one in the Indian Ocean and the other in the Pacific Ocean.
The current distribution of the three Tetraclita species in the Indian and West Pacific Ocean may be a result of the interplay among geological events, the life history of species, and the present day oceanographic currents. Based on our population genetics analysis, T. kuroshioensis has a more persistent population than T. singaporensis and T. squamosa. The demographic expansion of T. kuroshioensis began 0.04–0.03 mya, prior to the Last Glacial Maxima (LGM). Prior to the LGM, T. kuroshioensis was present in both Indian and Pacific Oceans. The Sunda Shelf is an extension of the continental shelf of Southeast Asia and covers the Malay Peninsula. The Malay Peninsula connects the Andaman Sea with the Indian Ocean and the South China Sea via the Strait of Malacca. During the LGM and Pleistocene glaciations, sea levels were as much as 120 m lower than the present day (Chappell et al., 1996; Voris, 2000; Solihuddin, 2014), resulting in the Sunda Shelf and the Gulf of Thailand being exposed. Subsequently, the Indian and Pacific populations of T. kuroshioensis were separated and there was no gene flow. After the Pleistocene glaciations, the sea level rose and the connections between the Indian and Pacific Ocean opened again via the Strait of Malacca (Crandall et al., 2012).
Based on the present day distribution pattern, T. kuroshioensis is common and abundant in insular habitats in the Pacific Ocean, including Japan, Taiwan, and the Philippines (Chan et al., 2007b). However, T. kuroshioensis is absent from the Pacific side of the Malacca Peninsula and in the Malacca Strait that opens into the Indian Ocean. The absence of T. kuroshioensis on the East coast of the Malay Peninsula and in the Malacca Strait suggests that there may be no gene flow between the Pacific and Indian populations of T. kuroshioensis, thus resulting in distinct molecular differentiation between the Pacific and Indian Ocean populations. Oceanographic current patterns in the Andaman Sea suggest that planktonic larvae from the Indian Ocean are unlikely to have been transported to the Pacific via the Malacca Strait. Gyre or circular currents occurred around the Andaman Sea region in both seasons. The Malacca Strait experiences a continuous northwest-bound currents, in spite of the two monsoon seasons. As a result, water masses in the Andaman Sea cannot flow into the Malacca Strait and enter the South China Sea. This is also evidenced by a thermal boundary between the Andaman exit of the Malacca Strait and the Andaman Sea during the southwest monsoon season (Riza et al., 2010; Isa et al., 2020). This suggests that there is lack of larval dispersal and gene flow in the T. kuroshioensis populations in the Andaman Sea into the west Malacca Strait and subsequently into the South China Sea. A similar pattern was recorded for the Green Tiger Prawn on both sides of Malay Peninsula—there is a population genetic break between the Indian Ocean and South China Sea populations on the west coast of the Malay Peninsula in the Malacca Strait (Abdul Halim et al., 2021). The green algae Halimeda borneensis, Halimeda discoidea, and Halimeda opuntia were present in the Andaman Sea and northwest opening of the Malacca Strait but absent from the South China Sea (Pongparadon et al., 2015). Further examples of population genetic differentiation between Indian and Pacific populations due to a lack of gene flow after the LGM were observed in the intertidal barnacle O. brunnea (Chan et al., 2020), the Indo-Pacific tasselfish (Chenoweth and Hughes, 2003), vetigastropod and gastropod (Imron et al., 2007; Crandall et al., 2008a), and sea stars (Crandall et al., 2008b).
Tetraclita singaporensis is very common on the Andaman coastlines along the west Malacca Strait to the tip of the Malacca peninsula, including Singapore (Chan et al., 2007b). It has only been recorded in two sites on the west coast of the Gulf of Thailand and is absent from the rest of the West Pacific region (Tsang et al., 2011). S-DIVA results in the present study suggest that T. singaporensis’s most recent common ancestor was distributed in the Andaman Sea and the populations in the Gulf of Thailand expanded after the Pleistocene glaciations. Although the currents in the West Malacca Strait always flow westbound in spite of the two monsoons, there is a current from the Java Sea to Singapore and along eastern Malaysia to the Gulf of Thailand (Pongparadon et al., 2015). The relatively scattered populations in the Gulf of Thailand probably resulted from population expansions and larval dispersal from the tip of the Malacca Peninsula toward the Gulf of Thailand, after the sea levels rose following the Pleistocene.
Tetraclita squamosa is mainly distributed in the South China Sea and their populations are believed to have expanded into the coastline of southern China following the Pleistocene glaciations (Chan et al., 2007a; Tsang et al., 2011). The species’ larval development period is about 14 days (Chan, 2003). The current in the Malacca Strait flows at 10–70 cm s–1 (Riza et al., 2010). Assuming they are transported passively by currents, larvae can travel as many as 846.7 km in 14 days, almost the length of the Malacca Strait. The presence of scattered populations along the Andaman coastline is believed to be the result of T. squamosa population expansion from the South China Sea into the Indian Ocean after the sea level rose during the Pleistocene period. During the northeast monsoon, oceanic interchange between the South China Sea (Pacific waters) and Andaman Sea (Indian Ocean) occurs through the Strait of Malacca and Singapore Strait (Figure 5B), thus bringing the larvae of T. squamosa into the Indian Ocean (Pongparadon et al., 2015).
Environmental conditions in different marine ecoregions appear to be related to the vertical and horizontal distribution of the Tetraclita species in the Gulf of Thailand, Malacca Strait, Sunda Shelf and Andaman Sea, and Coral Coast ecoregions. T. singaporensis appears to be a tropical species that inhabits the mid shores of the ecoregions with high water temperature and high chlorophyll a concentration, including the Malacca Strait and Gulf of Thailand ecoregions (Figure 6). T. squamosa appears to be less heat tolerant, and only present in the low shore region of the intertidal zone (Chan et al., 2008; Figure 6). T. kuroshioensis has the widest geographical range of the three species studied, which includes insular and continent habitats (Chan et al., 2007a), and is probably more tolerate to wide ranges of temperatures. T. kuroshioensis is also common in the mid shores of the intertidal zone in the West Pacific and Andaman Sea (Figure 6). Variations in environmental conditions among ecosystems in Southeast Asian waters have been shown to affect the distribution and morphology of the green algae Halimeda (Pongparadon et al., 2015).
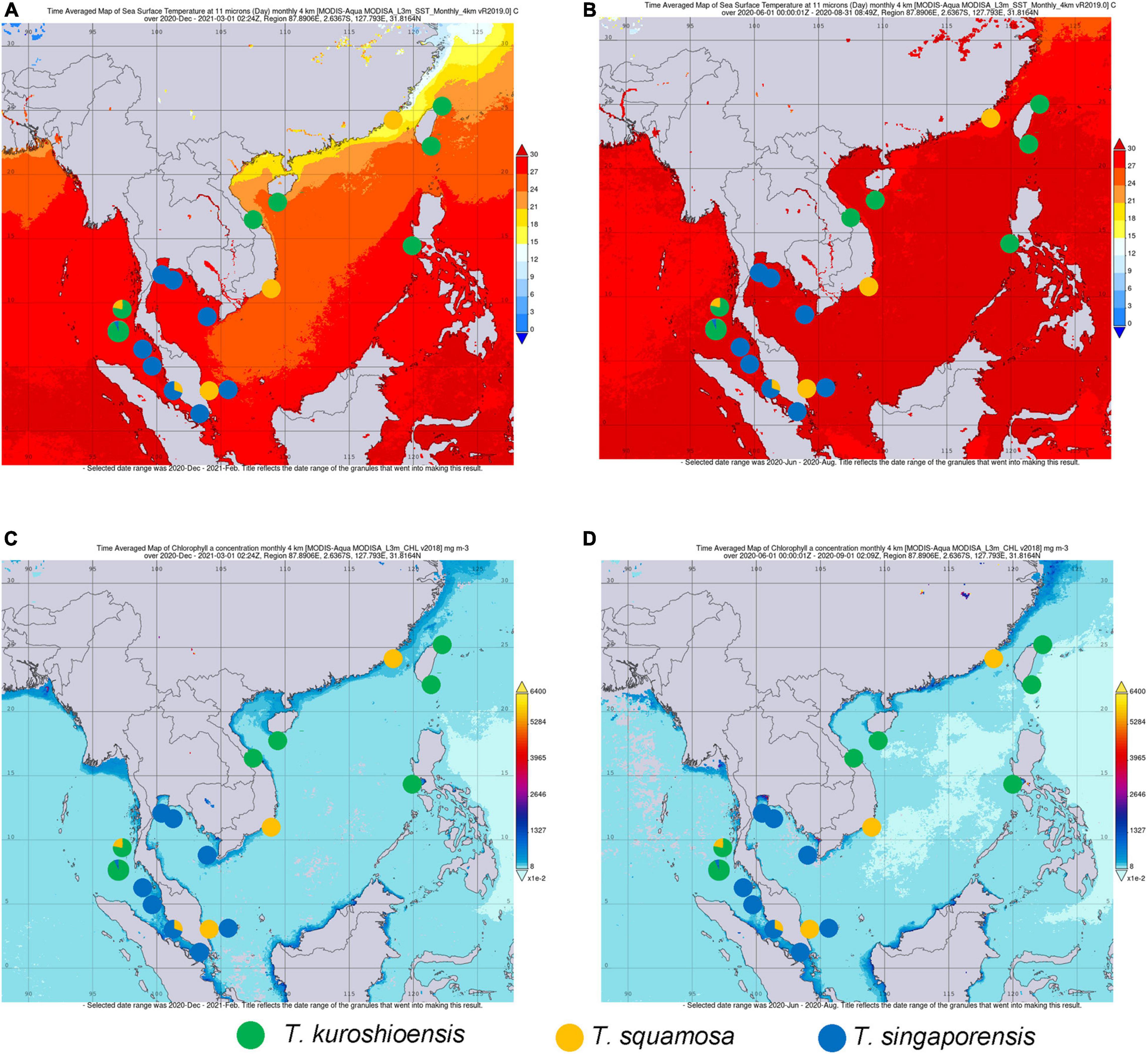
Figure 6. (A) Seawater temperature during the NE monsoon (December 2020–March 2021). (B) Seawater temperature during the SW monsoon (June 2020–August 2020). (C) Chlorophyll a concentration during the northeast monsoon (December 2020–March 2021). (D) Chlorophyll a concentration during the southwest monsoon in the Indo-Pacific waters. Seawater temperature and chlorophyll a concentration were determined from the NASA GIOVANNI database.
Climate change is occurring globally, and consequent changes in the geographical distribution of warm- and cold-water species have been well documented. In general, warm water species will expand their geographical ranges northward, and vice versa for cold water species. In the present study, T. squamosa lives in the low shores of the intertidal shores, below the zonation of T. kuroshioensis and T. singaporensis. This suggests that T. squamosa may have a lower thermal tolerance than T. kuroshioensis and T. singaporensis. It is possible that the distribution of these three Tetraclita species in the South China Sea and Indian Ocean will change under increasing water temperature trends. It is essential to survey the temporal variation in the geographical distribution of Tetraclita in Southeast Asia.
Data Availability Statement
The datasets presented in this study can be found in online repositories. The names of the repository/repositories and accession number(s) can be found below: NCBI (GenBank) OK667951–OK668081 and OK667807–OK667933.
Ethics Statement
The animal study was reviewed and approved by protocol number: IACUC 001/2564 for project: Barnacle Database in Eastern Thailand; Burapha University, Thailand.
Author Contributions
BKKC and WS designed the experiments and prepared the manuscript. KA and WS performed morphological analysis and tissue collection for DNA barcoding. BKKC and Y-FT performed DNA barcoding and phylogenetic analysis. WS contributed to securing the funding. All authors contributed to the sample collection.
Funding
This work was financially supported by the Thailand Science Research and Innovation (Grant No. 23.8/2564) for project: Barnacle Database in Eastern Thailand (RSPG-BUU Lifelong Learning Knowledge Center https://www.rspgburapha.com), Burapha University. BKKC is supported by the Senior Investigator Award, Academia Sinica.
Conflict of Interest
The authors declare that the research was conducted in the absence of any commercial or financial relationships that could be construed as a potential conflict of interest.
Publisher’s Note
All claims expressed in this article are solely those of the authors and do not necessarily represent those of their affiliated organizations, or those of the publisher, the editors and the reviewers. Any product that may be evaluated in this article, or claim that may be made by its manufacturer, is not guaranteed or endorsed by the publisher.
Acknowledgments
We thank Ashitapol Pochai and Salinee Khachonpisitsak for providing Tetraclita specimens from Natai, Phang-nga. We also thank the Department of Biology, Faculty of Science, Burapha University for providing laboratory equipment and the RSPG Burapha Team, particularly Pitak Sootanan, for managing the funding. Thanks to Noah Last of Third Draft Editing for his English language editing.
Supplementary Material
The Supplementary Material for this article can be found online at: https://www.frontiersin.org/articles/10.3389/fmars.2021.774041/full#supplementary-material
Footnotes
References
Abdul Halim, S. A. A., Othman, A. S., Akib, N. A. M., Jamaludin, N. A., Esa, Y., and Nor, S. A. M. (2021). Mitochondrial markers identify a genetic boundary of the Green Tiger Prawn (Penaeus semisulcatus) in the Indo-Pacific Ocean. Zool. Stud. 60:8. doi: 10.6620/ZS.2021.60-08
Bowen, W., Bass, A. L., Rocha, L. A., Grant, W. S., and Robertson, D. R. (2001). Phylogeography of the trumpetfishes (Aulostomus): ring species complex on a global scale. Evolution 55, 1029–1039.
Buckeridge, J. S. (1983). Fossil barnacles (Cirripedia: Thoracica) of New Zealand and Australia. N. Z. Geol. Surv. Paleont. Bull. 50:151.
Buckeridge, J. S. (2008). Two new species and a new subspecies of Tetraclitella (Cirripedia: Thoracica) from the Cainozoic of Australia and New Zealand and a consideration of the significance of tubiferous walls. Zootaxa 1897, 43–52.
Cai, Y. F., Shen, X., Zhou, L., Chu, K. H., and Chan, B. K. K. (2018). Mitochondrial genome of Tesseropora rosea: molecular evidence for non-monophyly of the genus Tetraclita. Mitochondrial DNA B Resour. 3, 92–94. doi: 10.1080/23802359.2017.1422412
Chan, B. K. K. (2001). Studies on Tetraclita squamosa and Tetraclita japonica (Cirripedia: Thoracica) I: adult morphology. J. Crust. Biol. 21, 616–630.
Chan, B. K. K. (2003). Studies on Tetraclita squamosa and Tetraclita japonica (Cirripedia: Thoracica) II: larval morphology and development. J. Crustac. Biol. 23, 522–547. doi: 10.1651/C-2350
Chan, B. K. K., Akihisa, M., and Lee, P. F. (2008). Latitudinal gradient in the distribution of the intertidal acorn barnacles of the Tetraclita species complex (Crustacea: Cirripedia) in NW Pacific and SE Asian waters. Mar. Ecol. Prog. Ser. 362, 201–210.
Chan, B. K. K., Tsang, L. M., and Chu, K. H. (2007a). Morphological and genetic differentiation of the acorn barnacle Tetraclita squamosa (Crustacea, Cirripedia) in East Asia and description of a new species of Tetraclita. Zool. Scr. 36, 79–91. doi: 10.1111/j.1463-6409.2007.00260.x
Chan, B. K. K., Tsang, L. M., and Chu, K. H. (2007b). Cryptic diversity of the Tetraclita squamosa complex (Crustacea: Cirripedia) in Asia: description of a new species from Singapore. Zool. Stud. 46, 46–56.
Chan, B. K. K., Tsao, Y. F., and Ganmanee, M. (2020). Morphological and molecular evidence support the intertidal barnacle Octomeris intermedia Nilsson-Cantell, 1921 (Thoracica, Chthamalidae) as a valid species in Indo-Pacific waters. Zookeys 914, 1–31. doi: 10.3897/zookeys.914.49328
Chan, S. W., Cheang, C. C., Gerung, G., Tharith, C., and Ang, P. (2013). Homogeneous population of the brown alga Sargassum polycystum in Southeast Asia: possible role of recent expansion and asexual propagation. PLoS One 8:e77662. doi: 10.1371/journal.pone.0077662
Chan, S. W., Cheang, C. C., Yeung, C. W., Chirapart, A., Gerung, G., and Anh, P. (2014). Recent expansion led to the lack of genetic structure of Sargassum aquifolium populations in Southeast Asia. Mar. Biol. 161, 785–795.
Chappell, J., Omura, A., Esat, T., McCulloch, M., Pandolfi, J., Ota, Y., et al. (1996). Reconciliation of late quaternary sea levels derived from coral terraces at Huon Peninsula with deep sea oxygen isotope records. Earth Planet. Sci. Lett. 141, 227–236. doi: 10.1016/0012-821X(96)00062-3
Chen, Y.-Y., Lin, H.-C., and Chan, B. K. K. (2012). Description of a new species of coral-inhabiting barnacle, Darwiniella angularis sp. n. (Cirripedia, Pyrgomatidae) from Taiwan. Zookeys 214, 43–74. doi: 10.3897/zookeys.214.3291
Chenoweth, S. F., and Hughes, J. M. (2003). Oceanic interchange and nonequilibrium population structure in the estuarine dependent Indo-Pacific tasselfish, Polynemus sheridani. Mol. Ecol. 12, 2387–2397. doi: 10.1046/j.1365-294x.2003.01921.x
Coppard, S. E., Jessop, H., and Lessios, H. A. (2021). Phylogeography, colouration, and cryptic speciation across the Indo-Pacific in the sea urchin genus Echinothrix. Sci. Rep. 11:16568. doi: 10.1038/s41598-021-95872-0
Crandall, E. D., Frey, M. A., Grosberg, R. K., and Barber, P. H. (2008a). Contrasting demographic history and phylogeographical patterns in two Indo-Pacific gastropods. Mol. Ecol. 17, 611–626. doi: 10.1111/j.1365-294X.2007.03600.x
Crandall, E. D., Jones, M. E., Muñoz, M. M., Akinronbi, B., Erdmann, M. V., and Barber, P. H. (2008b). Comparative phylogeography of two seastars and their ectosymbionts within the Coral Triangle. Mol. Ecol. 17, 5276–5290. doi: 10.1111/j.1365-294X.2008.03995.x
Crandall, E. D., Sbrocco, E. J., Deboer, T. S., Barber, P. H., and Carpenter, K. E. (2012). Expansion dating: calibrating molecular clocks in marine species from expansions onto the Sunda Shelf following the last glacial maximum. Mol. Biol. Evol. 29, 707–719. doi: 10.1093/molbev/msr227
Darriba, D., Taboada, G. L., Doallo, R., and Posada, D. (2012). jModelTest 2: more models, new heuristics and parallel computing. Nat. Methods 9:772. doi: 10.1038/nmeth.2109
Dawson, M. N., Grosberg, R. K., Stuart, Y. E., and Sanford, E. (2010). Population genetic analysis of a recent range expansion: mechanisms regulating the poleward range limit in the volcano barnacle Tetraclita rubescens. Mol. Ecol. 19, 1585–1605. doi: 10.1111/j.1365-294x.2010.04588.x
Excoffier, L., and Lischer, H. E. L. (2010). Arlequin suite ver 3.5: a new series of programs to perform population genetics analyses under Linux and Windows. Mol. Ecol. Resour. 10, 564–567. doi: 10.1111/j.1755-0998.2010.02847.x
Feng, M., Cao, W., Wang, C., Lin, S., Sun, D., and Zhou, Y. (2020). Complete mitochondrial genome of Tetraclita squamosa squamosa (Sessilia: Tetraclitidae) from China and phylogeny within Cirripedia. Mitochondrial DNA B: Resour. 5, 2121–2123. doi: 10.1080/23802359.2020.1765705
Folmer, O., Black, M., Hoeh, W., Lutz, R., and Vrijenhoek, R. (1994). DNA primers for amplification of mitochondrial cytochrome c oxidase subunit I from diverse metazoan invertebrates. Mol. Mar Biol. Biotechnol. 3, 294–299.
Guindon, S., Dufayard, J.-F., Lefort, V., Anisimova, M., Hordijk, W., and Gascuel, O. (2010). New algorithms and methods to estimate maximum-likelihood phylogenies: assessing the performance of PhyML3.0. Syst. Biol. 59, 307–321. doi: 10.1093/sysbio/syq010
Guindon, S., and Gascuel, O. (2003). A simple, fast, and accurate algorithm to estimate large phylogenies by maximum likelihood. Syst. Biol. 52, 696–704. doi: 10.1080/10635150390235520
Hall, R. (1998). “The plate tectonics of Cenozoic SE Asia and the distribution of land and sea,” in Biogeography and Geological Evolution of SE Asia, eds R. Hall and J. D. Holloway (Leiden: Backbury Publishers), 99–131.
Hoang, D. T., Chernomor, O., von Haeseler, A., Minh, B. Q., and Vinh, L. S. (2018). UFBoot2: improving the ultrafast bootstrap approximation. Mol. Biol. Evol. 35, 518–522. doi: 10.1093/molbev/msx281
Imron, Jeffrey, B., Hale, P., Degnan, B. M., and Degnan, S. M. (2007). Pleistocene isolation and recent gene flow in Haliotis asinina, an Indo-Pacific vetigastropod with limited dispersal capacity. Mol. Ecol. 16, 289–304. doi: 10.1111/j.1365-294X.2006.03141.x
Isa, N. S., Akhir, M. F., Kok, P. H., Daud, N. R., Khalil, I., and Roseli, N. H. (2020). Spatial and temporal variability of sea surface temperature during El-Niño Southern Oscillation and Indian Ocean dipole in the strait of Malacca and Andaman Sea. Reg. Stud. Mar. Sci. 39:101402. doi: 10.1016/j.rsma.2020.101402
Ji, N., Ge, T., Mao, S., Zhang, M., Mao, N., Cai, Y., et al. (2021). The first mitochondrial genome of Tetraclita kuroshioensis (Crustacea: Sessilia) from China: insight into the phylogeny within Cirripedia. Mitochondrial DNA B: Resour. 6, 988–989. doi: 10.1080/23802359.2021.1891984
Kalyaanamoorthy, S., Minh, B. Q., Wong, T. K. F., von Haeseler, A., and Jermiin, L. S. (2017). ModelFinder: fast model selection for accurate phylogenetic estimates. Nat. Methods 14, 587–589. doi: 10.1038/nmeth.4285
Kumar, S., Stecher, G., Li, M., Knyaz, C., and Tamura, K. (2018). MEGA X: molecular evolutionary genetics analysis across computing platforms. Mol. Biol. Evol. 35, 1547–1549. doi: 10.1093/molbev/msy096
Lavrov, D. V., Brown, W. M., and Boore, J. L. (2004). Phylogenetic position of the Pentastomida and (pan)crustacean relationships. Proc. Biol. Sci. 271, 537–544. doi: 10.1098/rspb.2003.2631
Leigh, J. W., and Bryant, D. (2015). POPART: full-feature software for haplotype network construction. Methods Ecol. Evol. 6, 1110–1116. doi: 10.1111/2041-210X.12410
Lessios, H. A., Kane, J., and Robertson, D. R. (2003). Phylogeography of the pantropical sea urchin Tripneustes: contrasting patterns of population structure between oceans. Evolution 57, 2026–2036. doi: 10.1111/j.0014-3820.2003.tb00382.x
Mokady, O., Rozenblatt, S., Graur, D., and Loya, Y. (1994). Coral-host specificity of Red Sea Lithophaga bivalves: interspecific and intraspecific variation in 12S mitochondrial ribosomal RNA. Mol. Mar. Biol. Biotechnol. 3, 158–164.
Newman, W. A., and Ross, A. (1976). Revision of the balanomorph barnacles; including a catalog of the species. Mem. San Diego Soc. Nat. Hist. 9, 1–108.
Nguyen, L.-T., Schmidt, H. A., von Haeseler, A., and Minh, B. Q. (2015). IQ-TREE: a fast and effective stochastic algorithm for estimating maximum-likelihood phylogenies. Mol. Biol. Evol. 32, 268–274. doi: 10.1093/molbev/msu300
Ni, G., Li, Q., Kong, L. F., and Yu, H. (2014). Comparative phylogeography in marginal seas of the northwestern Pacific. Mol. Ecol. 23, 534–548. doi: 10.1111/mec.12620
Pérez-Losada, M., Harp, M., Høeg, J. T., Achituv, Y., Jones, D., Watanabe, H., et al. (2008). The tempo and mode of barnacle evolution. Mol. Phylogenet. Evol. 46, 328–346. doi: 10.1016/j.ympev.2007.10.004
Pilsbry, H. A. (1916). The sessile barnacles (Cirripedia) contained in the collections of the U. S. National Museum; including a monograph of the American species. Bull. Am. Mus. Nat. Hist. 93, 1–366.
Pochai, A., Kingtong, S., Sukparangsi, W., and Khachonpisitasak, S. (2017). The diversity of acorn barnacles (Cirripedia, Balanomorpha) across Thailand’s coasts: the Andaman Sea and Gulf of Thailand. Zoosyst. Evol. 93, 13–34. doi: 10.3897/zse.93.10769
Pongparadon, S., Zuccarello, G. C., Phang, S. M., Kawai, H., Hanyuda, T., and Prathep, A. (2015). Diversity of Halimeda (Chlorophyta) from the Thai–Malay Peninsula. Phycologia 54, 349–366.
Pongparadon, S., Zuccarello, G. C., and Prathep, A. (2017). High morpho-anatomical variability in Halimeda macroloba (Bryopsidales, Chlorophyta) in Thai waters. Phycol. Res. 65, 136–145.
Puillandre, N., Lambert, A., Brouilleft, S., and Achaz, G. (2012). ABGD, automatic barcode gap discovery for primary species delimitation. Mol. Ecol. 21, 1864–1877. doi: 10.1111/j.1365-294X.2011.05239.x
Rambaut, A., Drummond, A. J., Xie, D., Baele, G., and Suchard, M. A. (2018). Posterior summarization in Bayesian phylogenetics using Tracer 1.7. Syst. Biol. 67, 901–904. doi: 10.1093/sysbio/syy032
Reid, D. G., Lal, K., Mackenzie-Dodds, J., Kaligis, F., Littlewood, D. T. J., and Williams, S. T. (2006). Comparative phylogeography and species boundaries in Echinolittorina snails in the central Indo-West Pacific. J. Biogeogr. 33, 990–1006. doi: 10.1111/j.1365-2699.2006.01469.x
Riza, S., Setiawan, I., Iskandar, T., Ilhamsyah, Y., Wahid, M. A., and Musman, M. (2010). Currents simulation in the Malacca straits by using three-dimensional numerical model. Sains Malay. 39, 519–524.
Roberts, C. M., McClean, C. J., Veron, J. E. N., Hawkins, J. P., Allen, G. R., McAllister, D. E., et al. (2002). Marine biodiversity hotspots and conservation priorities for tropical reefs. Science 295, 1280–1284. doi: 10.1126/science.1067728
Rogers, A. R., and Harpending, H. (1992). Population growth makes waves in the distribution of pairwise genetic differences. Mol. Biol. Evol. 9, 552–569. doi: 10.1093/oxfordjournals.molbev.a040727
Ronquist, F., and Huelsenbeck, J. P. (2003). MrBayes 3: Bayesian phylogenetic inference under mixed models. Bioinformatics 19, 1572–1574. doi: 10.1093/bioinformatics/btg180
Rozas, J., Ferrer-Meta, A., Sánchez-DelBarrio, J. C., Guirao-Rico, S., Librado, P., Ramos-Onsins, S. E., et al. (2017). DnsSP 6: DNA sequence polymorphism analysis of large data sets. Mol. Biol. Evol. 1, 3299–3302. doi: 10.1093/molbev/msx248
Schubart, C. D., and Huber, M. G. J. (2006). Genetic comparisons of German population of the stone crayfish: Austropotamobius torrentium (Crustacea: Astacidae). Bull. Fr. Pêche Piscic. 380-381, 1019–1028. doi: 10.1051/kmae:2006008
Shen, X., Tsang, L. M., Chu, K. H., Achituv, Y., and Chan, B. K. K. (2015). Mitochondrial genome of the intertidal acorn barnacle Tetraclita serrata Darwin, 1854 (Crustacea: Sessilia): gene order comparison and phylogenetic consideration within Sessilia. Mar. Genomics 22, 63–69. doi: 10.1016/j.margen.2015.04.004
Shen, X., Tsoi, K.-H., and Cheang, C.-C. (2016). The model barnacle Balanus Linnaeus, 1758 (Crustacea: Maxillopoda: Sessilia) mitochondrial genome and gene rearrangements within the family Balanidae. Mitochondrial DNA A DNA Mapp. Seq. Anal. 27, 2112–2114. doi: 10.3109/19401736.2014.982581
Sojisuporn, P., Morimoto, A., and Yanagi, T. (2010). Seasonal variation of sea surface current in the Gulf of Thailand. Coast. Mar. Sci. 34, 91–102.
Solihuddin, T. (2014). A drowning Sunda Shelf model during last glacial maximum (LGM) and Holocene: a review. Indones. J. Geosci. 1, 99–107. doi: 10.17014/ijog.v1i2.182
Song, J., Shen, X., Chu, K. H., and Chan, B. K. K. (2017). Mitochondrial genome of the acorn barnacle Tetraclita rufotincta Pilsbry, 1916: highly conserved gene order in Tetraclitidae. Mitochondrial DNA B Resour. 2, 936–937. doi: 10.1080/23802359.2017.1413305
Spalding, M. D., Fox, H. E., Allen, G. R., Davidson, N., Ferdaña, Z. A., Finlayson, M., et al. (2007). Marine ecoregions of the world: a bioregionalization of coastal and shelf areas. Bioscience 57, 573–583.
Sukparangsi, W., Pochai, A., Wongkunanusorn, C., and Khachonpisitsak, S. (2019). Discovery of Neonrosella vitiata (Darwin) and Newmanella spinosus Chan & Cheang (Balanomorpha, Tetraclitidae) from the Andaman Sea, eastern Indian Ocean. Zookeys 833, 1–20. doi: 10.3897/zookeys.833.30689
Trifinopoulos, J., Nguyen, L.-T., von Haeseler, A., and Minh, B. Q. (2016). W-IQ-TREE: a fast online phylogenetic tool for maximum likelihood analysis. Nucleic Acids Res. 44, W232–W235. doi: 10.1093/nar/gkw256
Tsang, L. M., Achituv, Y., Chu, K. H., and Chan, B. K. K. (2012). Zoogeography of intertidal communities in the West Indian Ocean as determined by ocean circulation systems: patterns from the Tetraclita barnacles. PLoS One 7:e45120. doi: 10.1371/journal.pone.0045120
Tsang, L. M., Chan, B. K. K., Ma, K. Y., Hsu, C.-H., and Chu, K. H. (2007). Lack of mtDNA and morphological differentiation between two acorn barnacles Tetraclita japonica and T. formosana differing in parietes colours and geographical distribution. Mar. Biol. 151, 147–155. doi: 10.1007/s00227-006-0460-8
Tsang, L. M., Chan, B. K. K., Shih, F.-L., Chu, K. H., and Chen, C. A. (2009). Host-associated speciation in the coral barnacle Wanella milleporae (Cirripedia: Pyrgomatidae) inhabiting the Millepora coral. Mol. Ecol. 18, 1463–1475. doi: 10.1111/j.1365-294X.2009.04090.x
Tsang, L. M., Chu, K. H., Achituv, Y., and Chan, B. K. K. (2015). Molecular phylogeny of the acorn barnacle family Tetraclitidae (Cirripedia: Balanomorpha: Tetraclitoidea): validity of shell morphology and arthropodal characteristics in the systematics of Tetraclitid barnacles. Mol. Phylogenet. Evol. 82 (Pt A), 324–329. doi: 10.1016/j.ympev.2014.09.015
Tsang, L. M., Shen, X., Cheang, C. C., Chu, K. H., and Chan, B. K. K. (2017). Gene rearrangement and sequence analysis of mitogenomes suggest polyphyly of Archaeobalanid and Balanid barnacles (Cirripedia: Balanomorpha). Zool. Scr. 46, 729–739. doi: 10.1111/zsc.12246
Tsang, L. M., Wu, T. H., Ng, W. C., Williams, G. A., Chan, B. K. K., and Chu, K. H. (2011). “Comparative phylogeography of Indo-West Pacific intertidal barnacles,” in Phylogeography and Population Genetics in Crustacea, eds C. Held, S. Koenemann, and C. D. Schubart (Milton Park: Taylor and Francis Group publisher).
Voris, H. K. (2000). Maps of Pleistocene sea levels in Southeast Asia: shorelines, river systems and time durations. J. Biogeogr. 27, 1153–1167.
Wares, J. P. (2001). Patterns of speciation inferred from Mitochondrial DNA in North American Chthamalus (Cirripedia: Balanomorpha: Chthamaloidea). Mol. Phylogenet. Evol. 18, 104–116. doi: 10.1006/mpev.2000.0857
Wares, J. P., and Cunningham, C. W. (2001). Phylogeography and historical ecology of the north Atlantic intertidal. Evolution 55, 2455–2469. doi: 10.1111/j.0014-3820.2001.tb00760.x
Yu, Y., Harris, A. J., Blair, C., and He, X. (2015). RASP (Reconstruct Ancestral State in Phylogenies): a tool for historical biogeography. Mol. Phylogenet. Evol. 87, 46–49. doi: 10.1016/j.ympev.2015.03.008
Yu, Y., Harris, A. J., and He, X. (2010). S-DIVA (Statistical Dispersal-Vicariance Analysis): a tool for inferring biogeographic histories. Mol. Phylogenet. Evol. 56, 848–850. doi: 10.1016/j.ympev.2010.04.011
Keywords: acorn barnacle, Cirripedia, Tetraclitidae, distribution, molecular phylogeny
Citation: Chan BKK, Tsao Y-F, Wangkulangkul K, Amjud K and Sukparangsi W (2022) Biogeography and Biodiversity of the Intertidal Barnacle Tetraclita Species in the Gulf of Thailand and Andaman Sea – Influences of Oceanographic Currents and Pleistocene Glaciations. Front. Mar. Sci. 8:774041. doi: 10.3389/fmars.2021.774041
Received: 10 September 2021; Accepted: 29 December 2021;
Published: 26 January 2022.
Edited by:
Anna Rita Rossi, Sapienza University of Rome, ItalyReviewed by:
Zi-Min Hu, Yantai University, ChinaDongsheng Zhang, Second Institute of Oceanography, Ministry of Natural Resources, China
Copyright © 2022 Chan, Tsao, Wangkulangkul, Amjud and Sukparangsi. This is an open-access article distributed under the terms of the Creative Commons Attribution License (CC BY). The use, distribution or reproduction in other forums is permitted, provided the original author(s) and the copyright owner(s) are credited and that the original publication in this journal is cited, in accordance with accepted academic practice. No use, distribution or reproduction is permitted which does not comply with these terms.
*Correspondence: Woranop Sukparangsi, d29yYW5vcEBnby5idXUuYWMudGg=