- 1Department of Ecology and Marine Resources, Instituto de Investigaciones Marinas (IIM-CSIC), Vigo, Spain
- 2BioCost ResearchGroup, Facultad de Ciencias and CICA, Universidade da Coruña, A Coruña, Spain
Syngnathids are vulnerable fishes closely associated with seaweeds and seagrass, which provide shelter and food resources. Even though most syngnathids commonly feed on small crustaceans, the feeding regimes may differ depending on the species and prey availability. This is the first monitoring study to explain syngnathid abundances and dietary regimes within macroalgal beds in Cíes Archipelago (Atlantic Islands Marine National Park, North West Iberian Peninsula). We sampled the epifaunal assemblages in seaweed communities dominated by the canopy-forming macroalgae Gongolaria baccata and Codium spp. seasonally during 2 years. The epifaunal structure was mostly represented by harpacticoid copepods, amphipods (especially gammarids) and gastropods. Epifauna exhibited low plant-host specificity and a higher dominance of amphipods on the more structurally complex macroalgae G. baccata. The epifaunal assemblages and syngnathid specimens were assessed for trophic structure using stable isotopes (δ13C and δ15N). The three syngnathids (Hippocampus guttulatus, Syngnathus acus, and Entelurus aequoreus) inhabiting Cíes Archipelago were sympatric. They occupied highly similar trophic positions (TPs), but differed in niche size, in such a way that the snake pipefish E. aequoreus would likely feed on smaller prey. The assessment of the feeding regime in the dominant great pipefish S. acus revealed that amphipods mostly contributed to bulk diet, followed by isopods, carideans, and copepods, whereas mysidaceans were not highly consumed. Seasonal changes in both epifaunal structure and syngnathids abundance confirmed that syngnathids are seasonal residents in Cíes Archipelago, migrating to other areas in autumn when the seaweed cover is drastically reduced and the epifaunal structure modified. This study showed the importance of Gongolaria assemblages in Cíes Archipelago, providing rich dietary sources and potentially contributing to higher abundances and diversity of syngnathids. Ongoing cover reduction in Gongolaria assemblages in certain regions (e.g., Mediterranean) should be considered a potential ecological concern for syngnathids and accompanying fauna, requiring further investigations.
Introduction
Marine seaweeds and seagrasses are important benthic primary producers housing a great variety of small motile animals, with many different life forms, ecological characteristics and trophic patterns (Orth, 1973; Adams, 1976; Zimmerman et al., 1979; Orth et al., 1984; Edgar and Moore, 1986; Franco et al., 2006; Horinouchi et al., 2012). Vegetal communities are considered ecosystem engineers, providing habitat, refuge from predators, and a wide variety of food sources for a multitude of species (Howard and Koehn, 1985; Lawton and Jones, 1995; Vizzini and Mazzola, 2004; Espino et al., 2011; Ape et al., 2019). Marine macrophytes dominate sandy and rocky shores in temperate areas worldwide (Hemminga and Duarte, 2000; Mineur et al., 2015).
Macroalgae create spatial complex habitats that increase species richness and diversity (Schmidt and Scheibling, 2007; Bertelli and Unsworth, 2014). However, the abundance and distribution of epifauna differ among seaweeds, being strongly influenced by the architecture of the host macroalgae (Viejo, 1999; Villegas et al., 2008; Moore and Hovel, 2010; Png-González et al., 2014; Torres et al., 2015). Mobile epifauna is an essential source for secondary consumers and fishes associated with seagrass and seaweed assemblages (Adams, 1976; Jones, 1988; Brawley, 1992; Martin-Smith, 1993). The abundance of many epifaunal organisms in marine macrophytes may be positively related to the algal biomass (Chen et al., 2020), the root-rhizome mat, and/or the plant canopy (Orth et al., 1984), showing a preference for seaweeds with high morphological complexity (Gee and Warwick, 1994).
The Family Syngnathidae includes small and cryptic fishes (e.g., seahorses, pipefishes, and seadragons), generally associated with marine flora or other complex benthic habitats (Kuiter, 2009; Ahnesjö and Craig, 2011). In Europe, seahorses (Hippocampus) and pipefishes (e.g., Syngnathus, Nerophis, Entelurus) are members of the ichthyofauna inhabiting temperate sheltered and semi-sheltered coastal habitats dominated by seaweeds and seagrasses (Franzoi et al., 1993; Curtis and Vincent, 2005; Gristina et al., 2014; Correia et al., 2018; Scapin et al., 2018; Ape et al., 2019; Castro-Fernández et al., 2020; Planas et al., 2021a). Unlike many pipefishes, most seahorse species are characterized by limited home ranges and reduced mobility (Foster and Vincent, 2004) due to their morphology and body characteristics. There are special characteristics in syngnathids that make them vulnerable and threatened as a result of diverse anthropogenic impacts such as habitat loss, fishing pressure, or physical disturbances (i.e., noise) through boating and shipping (Foster and Vincent, 2004; Ripley and Foran, 2007; Vincent et al., 2011; IUCN, 2019).
Seahorses are relatively sedentary predators that follow a stationary ambush foraging strategy, slowly approaching prey (Howard and Koehn, 1985; Kendrick and Hyndes, 2005). Some syngnathids may feed on the adjacent water column, but epibenthic invertebrates largely contribute to the bulk diet in species that typically live on seaweeds and seagrasses (Manning et al., 2019, and references therein). Dietary and feeding studies in syngnathids pointed out different levels of specialization, a feature that very likely relies on differences in snout morphology, feeding behavior and kinematics of the mouth (Bergert and Wainwright, 1997; Kendrick and Hyndes, 2005; de Lussanet and Muller, 2007). Harpacticoid copepods and small-sized amphipods, as well as decapods, nematodes and polychaetes, have been considered among the principal targeted prey in the dietary regime of pipefishes and seahorses (Tipton and Bell, 1988; Flynn and Ritz, 1999; Kendrick and Hyndes, 2005; Kitsos et al., 2008; Gürkan et al., 2011; Yip et al., 2015; Valladares et al., 2017; Ape et al., 2019; Manning et al., 2019). Copepods are also an important dietary source in euryhaline pipefishes (e.g., S. abaster) inhabiting invaded freshwater habitats (Didenko et al., 2018). Species-specific studies of pipefish species (i.e., S. acus, S. typhle, S. abaster, N. ophidion, and N. maculatus) inhabiting diverse coastal areas in southern Europe revealed the importance of harpacticoid copepods, gammarids, carideans, isopods, and mysidaceans in their diet (Franzoi et al., 1993; Vizzini and Mazzola, 2004; Oliveira et al., 2007; Gürkan and Taskavak, 2019; Castro-Fernández et al., 2020; Planas et al., 2020a). However, habitat type and seasonal fluctuations in the composition and availability of food sources may govern variations in the dietary composition (Roelke and Sogard, 1993; Nenciu et al., 2018; Ape et al., 2019; Manning et al., 2019; Planas et al., 2021a). Other factors such as fish size, gender, reproductive status, or spatial changes may also drive prey preferences (Steffe et al., 1989; Woods, 2002; Curtis and Vincent, 2005; Oliveira et al., 2007; Valladares et al., 2017).
Habitat preferences, feeding behavior and prey availability in syngnathids have been widely studied in many regions worldwide (Kuiter, 2009; Ahnesjö and Craig, 2011; Scapin et al., 2018; Manning et al., 2019). However, studies on the Iberian Peninsula are scarce and mostly focused on specific topics for a few species, habitats and coastal areas (Curtis and Vincent, 2005, 2006; Correia et al., 2015, 2018; Valladares et al., 2017; Woodall et al., 2017; Planas et al., 2020a, 2021a, b). In this regard, marine protected areas (MPA) might play an important role in contributing to the knowledge and conservation of those flagship fishes in Iberian coastal areas. A recent study (Planas et al., 2021a) assessed the distribution and abundance of syngnathids (Hippocampus guttulatus and Syngnathus acus) in Cíes Archipelago (Atlantic Islands National Park, North West Spain) (Fernández et al., 2020). Most specimens inhabited vegetated rocky habitats and, less frequently, mixed sand and rock environments dominated by canopy-forming seaweeds such as Codium spp. and the fucacean Gongolaria baccata (Planas et al., 2021b). Although both seaweeds have comparable structural complexity, they differ in their morphology, with a more complex branched secondary structure in G. baccata. Consequently, higher species richness and diversity would be expected in the fucacean, especially in summer compared to autumn and spring (Viejo, 1999).
Some syngnathids are considered specialist predators, showing a great ability to efficiently exploit available resources among different habitats (Nenciu et al., 2018; Ape et al., 2019; Planas et al., 2021a). Some species may adapt to high abundances of certain species in local areas as shown by the high content of Balanus cypris in H. guttulatus at the Romania Black Sea coast (Nenciu et al., 2018). However, syngnathids show large unexplained dietary variation, likely as the result of large differences in prey availability. Sedentary species (e.g., many seahorse species) with shorter and broader snouts likely benefit from feeding on a wider range of prey in their complex habitats. Conversely, species with longer, narrower snouts (e.g., mobile pipefishes) would prefer smaller, faster prey in the water column (Manning et al., 2019). In sympatric species, inter-specific differences in habitat use may be consistent with differences in resources exploitation (Curtis and Vincent, 2005). Hence, species diversity and abundance in epifaunal assemblages may impose limitations to the establishment of certain syngnathid species. Consequently, assessing the trophic structure of epifauna and the dietary regimes in sympatric syngnathids will contribute to infer feeding behavior and potential inter-specific competition. For that, stable isotope analysis (SIA) is a useful and convenient tool in food-web studies (Peterson and Fry, 1987; Post, 2002; Caut et al., 2009; Wolf et al., 2009), especially in those involving vulnerable or threatened species where killing of individuals should be avoided (Sanderson et al., 2009; Valladares and Planas, 2012) in which the sacrifice of fishes for gut content analysis is not necessary. In wild syngnathids, dorsal fin-clipping is a common technique for easily obtaining tissue samples for further isotopic analysis and dietary regime estimation (Valladares et al., 2017; Planas et al., 2020a, 2021a).
The number of syngnathid species on the Iberian coast is limited (Dawson, 1986), and more than half are currently classified as Data Deficient (IUCN, 2019). Hence, further research is required to provide more knowledge on their biology and ecology in wild populations (IUCN, 2019). The main aims of our study were: (1) to qualitatively and quantitatively characterize epifaunal assemblages associated with algal communities in marine environments inhabited by syngnathid fishes on Cíes Archipelago (Atlantic Islands National Park, NW Spain); (2) to determine the host-plant specificity of the mobile epifauna in two main foundation seaweeds (G. baccata and Codium spp.); (3) to assess the structure and characteristics of the food web supporting syngnathid fishes, and (4) to evaluate trophic similarities across sympatric syngnathid species present in the National Park. Objectives 3 and 4 were addressed following an isotopic approach including δ13C and δ15N analyses. Eventually, the obtained information will increase the scarce knowledge on the trophic ecology in European syngnathids, confirming the importance of biogenic macroalgal biogenic habitats as preferred areas for syngnathids, and will provide supporting information for syngnathids conservation in Atlantic Islands National Park.
Materials and Methods
Bioethics
Samples were collected with the permission of the Regional Government (Xunta de Galicia, Spain) and Organismo Autónomo de Parques Nacionales Españoles (OAPN, Spain) in Atlantic Island National Park. Fish capture, handling and sampling procedures were approved by the Regional Government Xunta de Galicia (Reference REGA ES360570202001/16/FUN/BIOL.AN/MPO02) and conducted in compliance with all bioethics standards on animal experimentation of the Spanish Government (R.D. 1201/2005, 10th October 2005). All the procedures were approved by the Bioethics Committee of CSIC.
Study Sites
The study was conducted at three sites on the West coast of Cíes Archipelago, located on the outer area of the Ría de Vigo (NW Iberian Peninsula) (42°13′N, 8°54′W). Cíes Archipelago is composed of three islands and various islets (Figure 1), being part of the Atlantic Islands National Park (PNIA). PNIA was declared Spanish National Park in 2002, as well as Nature Reserve in 1980 and other levels of protection (Natural Park, Special Protection Area–SPA, Site of Community Importance–SCI, and OSPAR area). PNIA is located at the northern limit of the eastern boundary upwelling system off NW Africa and SW Europe (Nogueira et al., 1997; Álvarez et al., 2005). Seawater temperature is homogeneous in winter (13–16°C) and stratified in summer (12–18°C) due to the warming of upper layers. Surface water temperature typically ranges from 13.4 to 18.7°C in the southern coast and from 13.4 to 18.0°C in the northern coast (Puertos del Estado, 2017). The subtidal zones on the landward inner face are dominated by mixed substrates of rocky and sandy or rocky and gravel (Fernández et al., 2020). Rocky areas are sparsely covered by several seaweeds dominated by Gongolaria baccata, G. usneoides, Codium spp., and turf-forming algae (mostly Corallina officinalis and Falkenbergia rufolanosa) (Fernández et al., 2020; Piñeiro-Corbeira et al., 2020; Planas et al., 2021a, b). Cíes Archipelago hosts three species of syngnathids (Hippocampus guttulatus, Entelurus aequoreus, and Syngnathus acus) that inhabit semi-exposed and sheltered areas dominated by seaweed communities (Hernández-Urcera et al., 2021; Planas et al., 2021a).
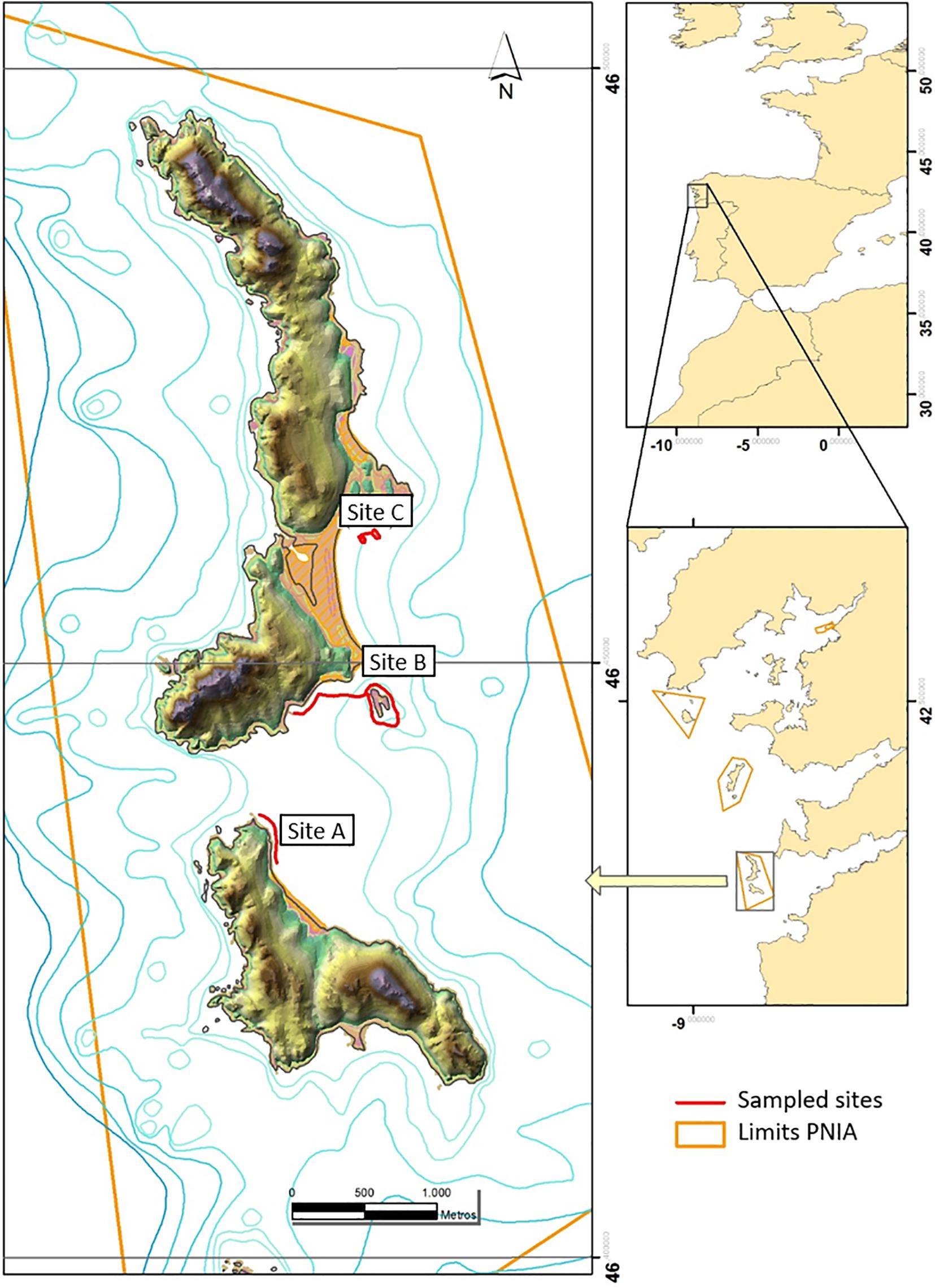
Figure 1. Sites (A–C) surveyed for epifauna assemblages associated with canopy-forming seaweeds in Cíes Archipelago (Galicia, NW Iberian Peninsula): samples collected in spring, summer, and autumn (2017–2018). Limits PNIA: Boundaries of Cíes Archipelago (Atlantic Islands National Park).
Sampling
To examine the availability and abundance of potential prey for syngnathids in Cíes Archipelago, samples of whole canopy-forming seaweeds Codium spp. (including C. tomentosum and C. vermilara), Gongolaria baccata (S.G. Gmelin) Molinari and Guiry 2020 (formerly Cystoseira baccata and Treptacantha baccata), and Asparagopsis armata Harvey 1855 were collected on three areas preferred by syngnathid fishes (Planas et al., 2021a; Hernández-Urcera et al., 2021). Diurnal underwater visual censuses (UVC) were carried out on sites located on the western coast of Cíes Archipelago (Figure 1): A–Pau de Bandeira, B–Illote de Viños/Carracido, and C–Borrón. Each site was visited seasonally (spring, summer and autumn) in 2017 and 2018 to obtain an overview of habitat characteristics, seaweed dominance and epifaunal assemblages. Further information is provided by Planas et al. (2021a, 2021b). Adverse winter conditions prevented sampling during that season.
At each sampling site, two patches (10 × 10 m) on rocky substrates were selected per survey to minimize the effect of spatial heterogeneity in epifaunal assemblages. Up to five canopy-forming individuals (including canopy and holdfast included) of the most abundant seaweeds were randomly collected at each patch (10 algal individuals per site and season). Each individual was gently removed from the substratum with a scraper, collected in separate cloth bags, and transferred into plastic buckets filled with 70% ethanol. Additionally, samples of mysidaceans (Siriella armata) were also collected in the water column (mesh size: 125 μm). The samples were transported to the laboratory on the same day of sampling, and the non-sessile epifauna was washed off each alga through a nylon mesh (mesh size: 125 μm), and transferred to separate plastic boats and preserved in 95% ethanol until being identified and quantified to the lowest feasible taxonomic level. Flora and faunal nomenclature followed the codes of Guiry and Guiry (2020) and WoRMS Editorial Board (2020). Abundances were expressed relative to the total fauna present in the sample. As the large number of samples did not allow for a rapid identification and separation of the fauna, the resulting faunal groups were maintained separately at 4°C in polypropylene tubes filled with 95% ethanol for further SIA (see below for further details on stable isotope procedures).
Sighted syngnathids were collected by hand, introduced in numbered plastic bags and transferred to a support boat. The fishes were morphologically identified, anaesthetized with Ethyl 3-aminobenzoate methane sulfonate (MS-222; 0.1 g L-1; Sigma-Aldrich Co., United States) and dorsal fin-clipped (Valladares and Planas, 2012). Fin samples were transferred to screw-capped tubes containing 95% ethanol and conserved at 4°C for further genetic identification (see Planas et al., 2021a) and SIA. All fishes were released at the capture site within 2–3 h after sampling. Further details on UVC and syngnathid sampling are provided by Planas et al. (2020a, 2021a).
Stable Isotope Analysis
Stable isotopes (δ13C and δ15N) were analyzed in faunal groups with >2% relative abundance. The samples were rinsed with distilled water, frozen at −20°C, freeze-dried and manually homogenized with a mortar and pestle. Analyses were run on sub-samples of 0.2–0.5 mg dry weight biomass. Before SIA, external shells were manually removed in mollusks, whereas calcium carbonate was removed from ophiuroids by adding 10% HCl drop-by-drop, until no more release of CO2 was observed (Jaschinski et al., 2008; Vafeiadou et al., 2013). C/N values indicated that lipid content was higher than 5% for some epifaunal samples (Planas et al., 2021a). Since high lipid content might cause significant alterations in δ13C and, to a lesser extent, in δ15N values (Post et al., 2007; Logan et al., 2008), specific conversion factors constructed for lipid normalization and acidification on faunal groups were applied when necessary (Supplementary Table 1). Normalization was not necessary for fin samples (Valladares and Planas, 2012; Planas et al., 2020b).
δ13C, δ15N, and elemental composition analyses (total C and N percentage) were carried out at Servizos de Apoio á Investigación (SAI) of the University of A Coruña (Spain). Samples were measured by continuous-flow isotope ratio mass spectrometry using a FlashEA1112 elemental analyzer (Thermo Finnigan, Italy) coupled to a Delta Plus mass spectrometer (FinniganMat, Bremen, Germany) through a Conflo II interface. Carbon and nitrogen stable isotope abundance was expressed as permil (‰) relative to VPDB (Vienna Pee Dee Belemnite) and Atmospheric Air, according to the following equation:
where X is 13C or 15N and R is the corresponding 13C/12C or 15N/14N ratio. International reference materials for δ15N (IAEA-N-1, IAEA-N-2, IAEA-NO-3) and δ13C (NBS 22, IAEA-CH-6, USGS24) estimates were run with each batch of samples. The precision (standard deviation) for the analysis of δ13C and δ15N in the laboratory standard (acetanilide) was ± 0.15‰ (1-sigma, n = 10). Standards were run every 10 biological samples.
Data Analysis
Epifaunal assemblages were compared with analysis of similarities (ANOSIM). PERMANOVA was not applied due to dispersion heterogeneity in some datasets. ANOSIM is a non-parametric test widely used in ecology (Clarke, 1993) that uses a ranked dissimilarity matrix to test the null hypothesis that the similarities within groups are smaller than or equal to between-group similarities. ANOSIM’s R statistic ranges from 0 to 1, with values close to 1 indicating that all within-group similarities are less than any between-group similarity, and values close to 0 suggesting that groups are indistinguishable.
The faunal similarity between grouped samples was visualized by non-metric Multidimensional Scaling (NMDS; vegan v.2.5-7 package in R) (Oksanen et al., 2020) based on a Bray-Curtis (BC) dissimilarity matrix (Clarke, 1993).
A comparative analysis of epifauna on canopy-forming seaweeds was performed specifically for Codium spp. and G. baccata, which were regularly sampled from summer-2017 until autumn-2018. Spring-2017 was excluded from the analyses, as G. baccata was not sampled due to its extreme scarcity. Asparagopsis armata was not included in the analyses as it was only collected in spring-2017 when it was extremely abundant. Thereafter, the abundance of A. armata decreased sharply and the species was no longer sampled. The fucacean G. baccata is a Cystoseira-like species that forms structurally complex habitats on the lower shore and the sublittoral zone attached to rocks or in intertidal pools. The species measures up to 1 m in length or more, with a simple or branched axis, and distichous lateral branch systems, radially symmetrical in a repeatedly pinnate fashion. It is attached by a thick, conical attaching disc to the substrate (Roberts, 1967). In the Mediterranean, it is considered of special concern within the European Water Framework Directive (WFD, 2000/60/EC) (Orfanidis et al., 2001; Ballesteros et al., 2007; Orfanidis, 2007) since many assemblages are regressing or disappearing due to habitat degradation. Besides, this brown macroalgal is used as a water quality indicator in coastal areas. Unlike the Mediterranean, Gongolaria and other fucaceans are under continuous expansion in NW Iberia (Piñeiro-Corbeira et al., 2016; Barrientos et al., 2020). Codium species are green seaweeds including one to several erect fronds (up to 1 m in length), dichotomous branches, and a bushy thallus. Codium is attached by a small discoid holdfast to the substrate (Silva, 1955). Gongolaria and Codium are markedly different in morphology, surface texture and shape of leaves.
Epifaunal abundances in canopy-forming seaweeds were used to estimate univariate statistics for each algal species (Codium spp. vs. G. baccata) across sampled periods (from summer-2017 to autumn-2018): species richness (S), Shannon diversity (H′), Simpson dominance (D′) and Pielou’s evenness (J′). Biodiversity estimates were compared with a Kruskal-Wallis non-parametric test with the help of BiodiversityR 2.12-3 package in R (Kindt and Coe, 2005).
The association between epifauna or functional groups and combination of seaweed types or sampling periods (depending on the type of analysis) was assessed with the multipatt (Multi-level pattern) function in the indicspecies R package (version 1.7.9) (De Cáceres and Legendre, 2009).
For the assessment of the trophic structure in faunal assemblages, the assignment of OTUs to functional groups was performed considering the clusters provided by the Similarity Profile Analysis (SIMPROF) using the clustsig package in R v.1.1. (Clarke et al., 2008). The fauna was assigned to the following functional groups (FG) SF–suspension feeders, G–grazers and herbivores, D–deposit feeders, O–omnivores, C–primary carnivores (syngnathids not included), and C_Syng–syngnathids). The trophic assessment was conducted with isotopic data (δ13C and δ15N) from 32 OTUs (28 epifauna, 1 mysidacean, and 3 syngnathids) (Table 3).
The trophic position (TP) of faunal groups (syngnathids and selected taxa or operational taxonomic units–OTUs) is commonly estimated as follows (Post, 2002):
where λ is the TP of the organism used to estimate δ15Nbaseline (e.g., 1 for primary producers), δ15Nsecondary consumer (δ15Nsc, or any higher consumer) was measured directly, and TDF (trophic discrimination factor) is the enrichment in δ15N per trophic level.
Faunal TP was estimated using the tRophicPosition package v. 0.7.7 (Quezada-Romegialli et al., 2018), an R package incorporating a Bayesian model for the calculation of consumer TP at the population level using δ15N values, with the bivalve Musculus costulatus as the baseline. The model combines Markov Chain Monte Carlo simulations through JAGS and statistical and graphical analyses. Consumer and baseline observations were treated as random variables and modeled using relevant statistical distributions. The calculation of TP for one baseline follows standard equations linking 15N enrichment per trophic level and the TP of the baseline. It is generally assumed that TDF values for 15N range between 3 and 4‰ (Peterson and Fry, 1987). In epifauna, we applied a generalist value of 3.4‰, which is frequently used when TDF for a given species or faunal group is unknown (Minagawa and Wada, 1984; Cabana and Rasmussen, 1996; Post, 2002). In syngnathids, we applied the TDF value (TDF = 4.1) experimentally derived for these fishes and used it to estimate prey preferences in the studied area (Planas et al., 2020a).
Niche regions and pairwise niche overlap in faunal groups (functional groups and syngnathid fishes) were assessed using δ13C and δ15N bi-plot as multidimensional niche indicator data. The niche region was defined as the joint probability density function of the multidimensional niche indicators at a probability alpha of 95%. Uncertainty was accounted for in a Bayesian framework. The analysis provides directional estimates of niche overlap, accounts for species-specific distributions in multivariate niche space, and produces unique and consistent bivariate projections of the multivariate niche region (Swanson et al., 2015). The packages SIBER v.2.1.4 (Jackson et al., 2011) and NicheRover v.1.1.0 (Lysy et al., 2014) were used to assess differences in the trophic niche features between faunal functional groups. The total convex hull areas (TA) and core trophic niche breadths were estimated for faunal groups using SIBER (Stable Isotope Bayesian Ellipses) while correcting for variable sample sizes (SEAc). Total trophic overlap values for 95% TA were estimated using nicheROVER, a method that is insensitive to sample size and incorporates a statistical uncertainty using Bayesian methods (Swanson et al., 2015).
From the isotopic profiles in potential prey and consumers (Syngnathids), we estimated the dietary regime in S. acus, the most representative syngnathid in Cíes Archipelago. The whole procedure was fully described in Planas et al. (2020a). Briefly, a SIMM polygon was constructed to determine the proportion of consumers included inside the mixing polygon bound by all potential sources (with isotopic profiles adjusted for TDFs) (Phillips and Gregg, 2003; Jackson et al., 2011; Smith et al., 2013). Bayesian Stable Isotope Mixing Models (SIMM) (Stock and Semmens, 2016; Stock et al., 2018) were applied using amphipods, harpacticoid copepods, carideans, isopods and mysidaceans as potential prey sources previously selected through hierarchical clustering analysis (Planas et al., 2020a).
A P-value threshold of 0.05 was considered significant in all statistical analyses.
Results
A total of 82 Operating Taxonomic Units (OTUs) or multispecies groupings were identified in epifauna samples seasonally collected in 2017–2018 in Cíes Archipelago (Supplementary Table 2). Regarding main faunal groups, harpacticoid copepods were highly dominant (61.5% relative abundance), followed by gastropods (20.9%), amphipods (10.9%), bivalves (5.2%), and other minor epifauna groups (<0.35%) (Figure 2). The most representative taxa among amphipods were gammaridae (74%), followed by calliopiidae (10.6%), amphilochidae (6.4%), corophiidae (4.5%), caprellidae (4.4%), and phliantidae (0.2%) (Supplementary Table 2 and Figure 2). The most dominant OTUs were Musculus costulatus (3.8%), Elysia spp. (2.9%), Tricolia pullus (1.8%), Peringia ulvae (0.9%), and Apherusa spp. (0.64%) (Supplementary Table 2). The majority of bivalves found in seaweed assemblages were juveniles (∼1–2 mm in length). Among amphipods, the most important taxa were gammaridae (74%) (Supplementary Table 2 and Figure 2). Other much less dominant groups were calliopiidae (10.6%), amphilochidae (6.4%), corophiidae (4.5%), caprellidae (4.4%), and phliantidae (0.2%).
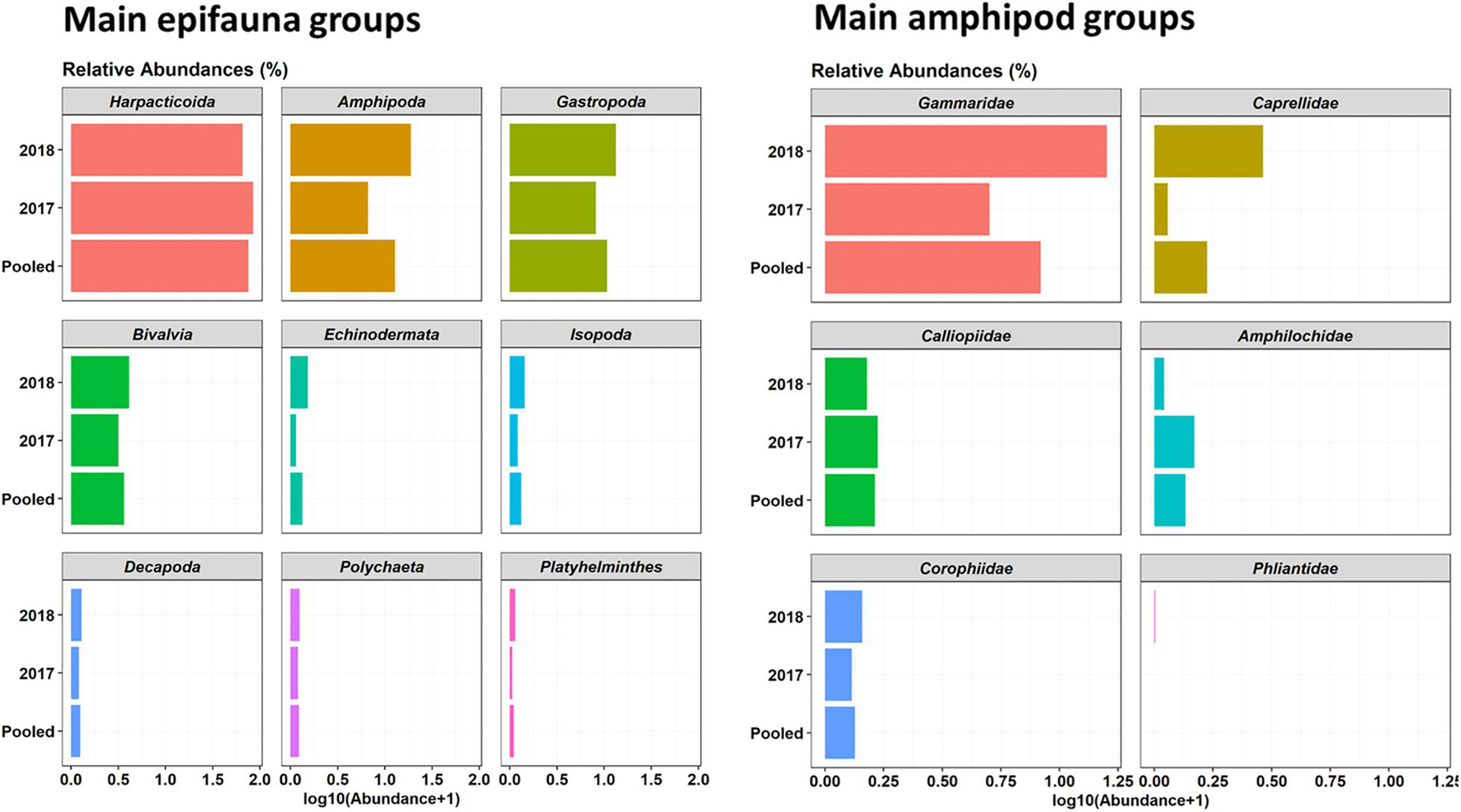
Figure 2. Relative abundances (%) of main epifauna taxa (left; only taxa with > 1% are provided) and Amphipods (right) associated with canopy-forming seaweeds in 2017–2018 samples collected in Cíes Archipelago.
The variability in the structure of epifaunal assemblages across Years and Seasons is graphically provided by two-dimensional NMDS plots (Figure 3), whereas the information on the taxa significantly associated with Years and Seasons are indicated in Supplementary Table 3.
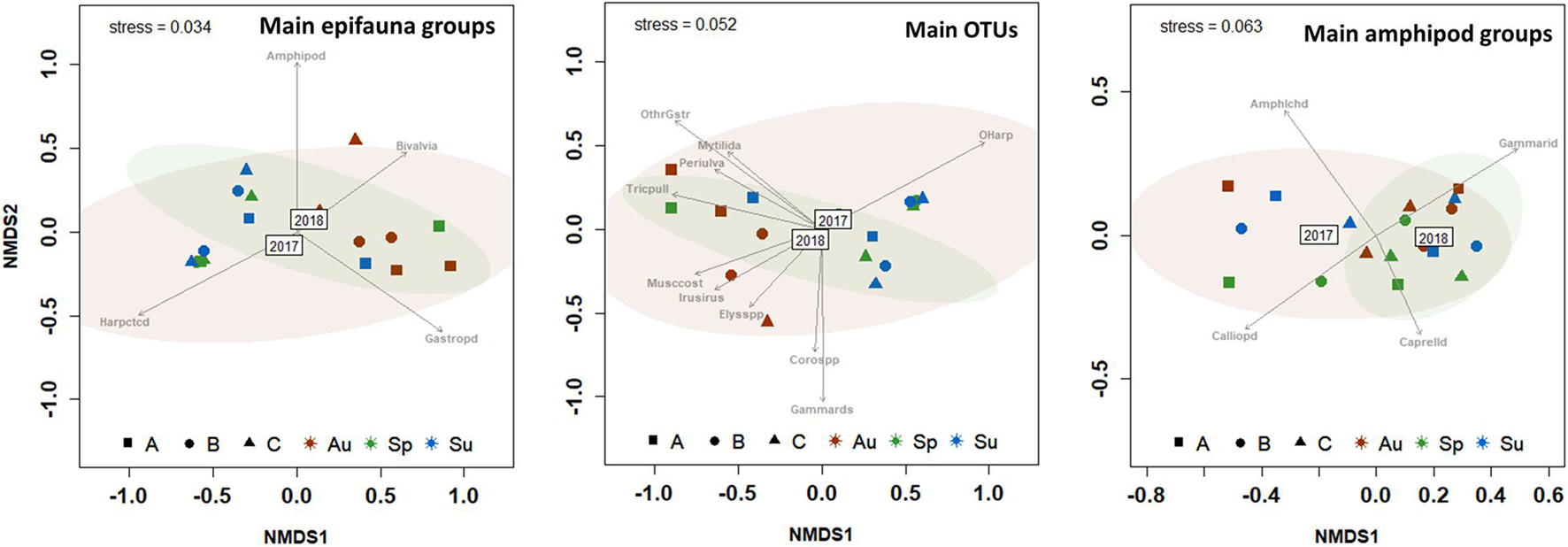
Figure 3. Two-dimensional non-metric multidimensional scaling (NMDS; Bray–Curtis similarities) plot of the variation in epifauna community composition based on the proportion of main faunal groups associated with canopy-forming seaweeds in Cíes Archipelago (2017–2018 samples). The confidence limits for ellipses (95% confidence) are shown. The most influencing groups (P < 0.05) are indicated by arrows. Eighteen marks are presented in the NMDS plot, corresponding to the eighteen samples.
Epifauna on Canopy-Forming Seaweeds
Gongolaria baccata was present on all three surveyed sites, whereas Codium spp. preferentially inhabited site A. The analysis of epifaunal biodiversity parsed into main foundation canopy-forming seaweeds (Figure 4 and Supplementary Table 4) revealed that richness (as number of OTUs per seaweed sampled) was, on average, consistently higher in G. baccata (20.7 ± 6.4; n = 45) than in Codium spp. (16.9 ± 5.4; n = 90) (Kruskal-Wallis test; p < 0.0001), and differed across Sampled periods (p < 0.0001). Shannon diversity (H′) was similar in both seaweeds (p = 0.119) but differed significantly across Sampled periods (p < 0.0001), with a significant increase in autumn-2017. Simpson dominance (D′) and Pielou’s evenness (J′) were slightly higher in Codium spp. than in G. baccata assemblages (p = 0.070 and 0.009, respectively). Dominance and evenness varied with Sampling periods (p < 0.0001).
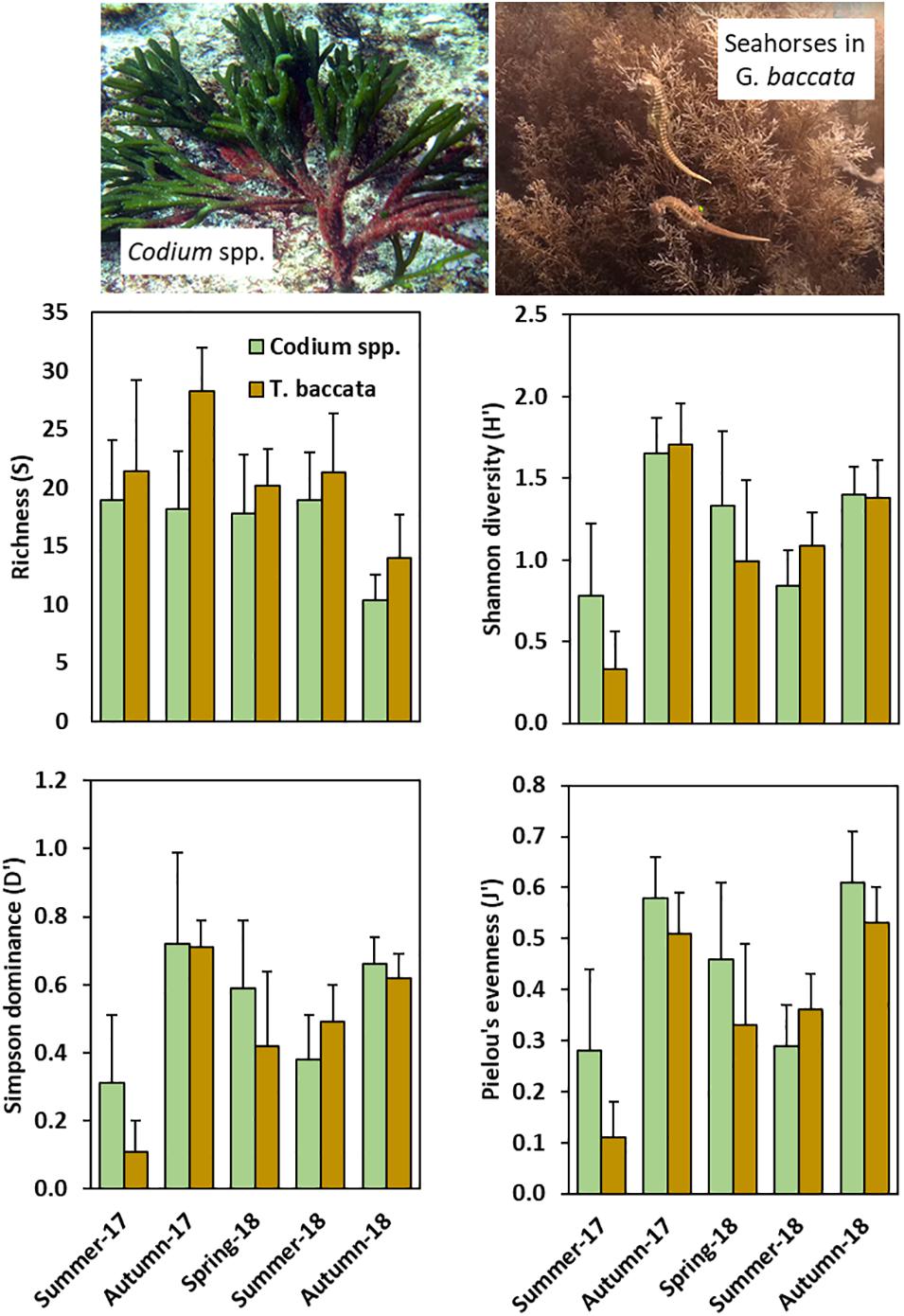
Figure 4. Species richness (S; mean ± standard deviation), Shannon diversity (H′; Mean ± SD), Simpson dominance (D′; mean ± SD), and Pielou’s evenness (J′; mean ± SD) per season (all sites pooled) in epifaunal assemblages associated to Codium spp. and Gongolaria baccata.
Analysis of similarities results on main OTUs revealed faunal dissimilarities for Seaweeds (ANOSIM statistic R = 0.160; p < 0.0001), and Sampling periods (R = 0.472; p < 0.0001). Gastropods (including Elysia sp., Tricolia pullus, Peringia ulvae, and others) were significantly associated with Codium assemblages (p < 0.0001), whereas Gammaridae, Amphipholis squamata, Corophium spp., and Dynamene bidentata (P = 0.021) were mostly related with G. baccata (Supplementary Table 5). Regarding the amphipods, the most significant difference between seaweeds was the strong association of gammaridae and corophidae with G. baccata (Supplementary Table 5). Those taxa together with amphilochidae significantly drove the differences across seasons, especially in autumn and summer. Globally, autumn was the period that contributed most to seasonal differences among samples.
The above results are graphically summarized on the two-dimensional nMDS plots, and the most influential (p < 0.05) groups of epifauna are provided (Figure 5). In general, spring samples denoted a transition of the community from autumn to summer. The autumn-spring-summer transition was less evident in amphipod groups, which showed higher inter-group similitudes and lower sample dispersion.
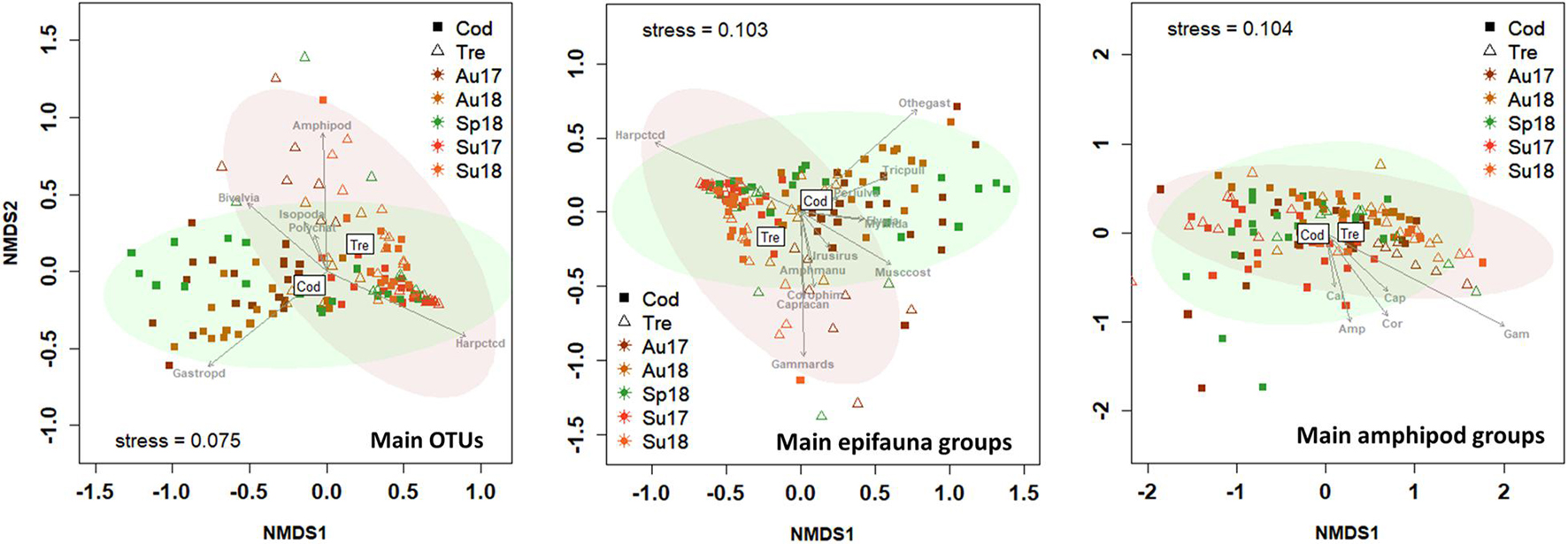
Figure 5. Two-dimensional non-metric multidimensional scaling (NMDS; Bray–Curtis similarities) plot of the seasonal (Sp, Spring; Su, Summer; Au, Autumn) variation in epifauna community composition associated to seaweeds Codium spp. (Cod) and Gongolaria baccata (Gon) in 2017 and 2018. The confidence limits for ellipses (95% confidence) are shown. The most influencing epifaunal groups (P < 0.05) are indicated by arrows. Main epifauna groups only include OTUs with >0.2% relative abundances. Amphipods: Amp, Amphilochidae; Cal, Calliopiidae; Cap, Caprellidae; Cor, Corophiidae; Gam, Gammaridae; Phl, Phliantidae.
Syngnathid Fishes
A total of 166 syngnathids were collected, including the long-snouted seahorse Hippocampus guttulatus Cuvier, 1829 (n = 6), and the pipefishes Syngnathus acus Linnaeus, 1758 (n = 151) and Entelurus aequoreus (Linnaeus, 1758) (n = 9) (Supplementary Table 6). Syngnathids were mainly collected in spring (48.8%) and summer (39.2%). S. acus was present on all three sampled sites (34, 48, and 69% in sites A, B, and C, respectively), whereas H. guttulatus and E. aequoreus were only present in site C.
Syngnathid fishes provided the highest δ15N values (11.24 ± 0.07‰), with small inter-specific differences (range = 0.31‰). Average δ13C values were rather stable (Max-Min = 0.24‰; mean = −15.45 ± 0.14‰) but ANOSIM results revealed significant isotopic differences across Years, and especially across Sampled seasons (see also NMDS plots in Supplementary Figure 1). The specimens collected in 2017 were characterized by more negative δ13C values (Multipatt, stat = 0.199; p < 0.0123). Spring was characterized by higher δ15N levels (Multipatt, stat = 0.421; p < 0.0001), whereas δ13C levels were lower in spring and summer samples (stat = 0.381; p < 0.0001). The three syngnathid species displayed similar isotopic profiles.
Trophic Niche Structure and Trophic Positions
Isotopic differences on the whole trophic cascade accounted for 2.6‰ in δ13C and 5.5‰ in δ15N. Musculus costulatus (i.e., isotopic baseline) occupied the lowest position, with isotopic values of −17.71‰ ± 0.07 for δ13C and 5.32 ± 0.26‰ for δ15N. This bivalve exhibited a rather stable isotopic profiles across seasons thorough the whole study, with values ranging from −18.99 to −17.58‰ for δ13C, and from 5.01 to 6.09‰ for δ15N (Table 1).
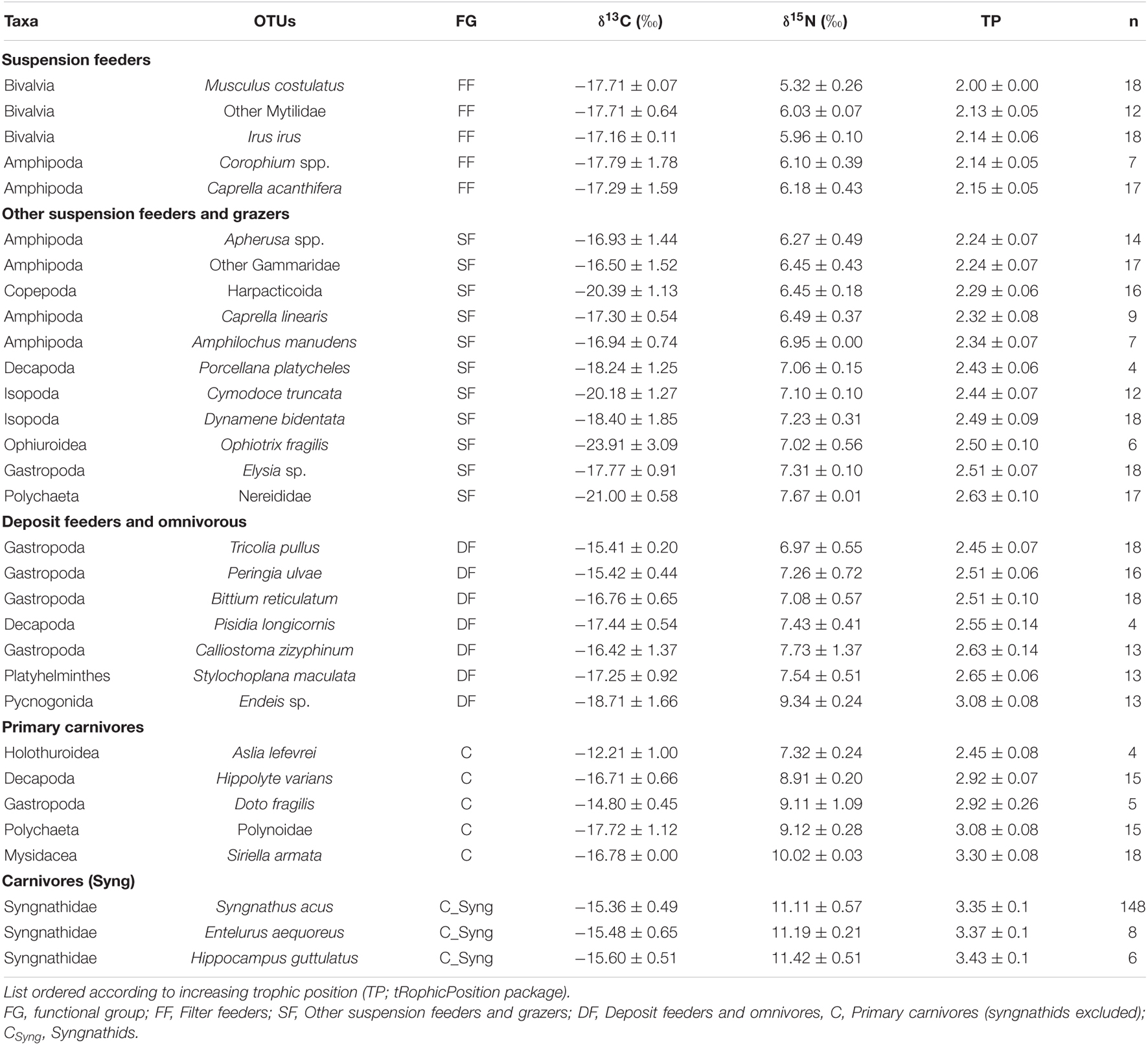
Table 1. Isotopic values (mean ± sd) in main epifauna (mysidacea also included) sampled in spring, summer, and autumn (2017–2018) on Cíes Archipelago.
The assignment of OTUs to the clusters (functional groups) provided by SIMPROF analysis is provided in Figure 6. Average δ15N values for FF, SF, DF, and C were 5.9 ± 0.4, 7.0 ± 0.5, 7.4 ± 0.5, and 9.0 ± 0.9, respectively (Table 2). The TPs estimated by the Bayesian package tRophicPosition for those FG were 2.14, 2.40, 2.62, and 2.97, respectively (Table 2 and Figure 7). Syngnathids occupied the highest TP on the cascade (3.36 ± 0.05) (Table 2).
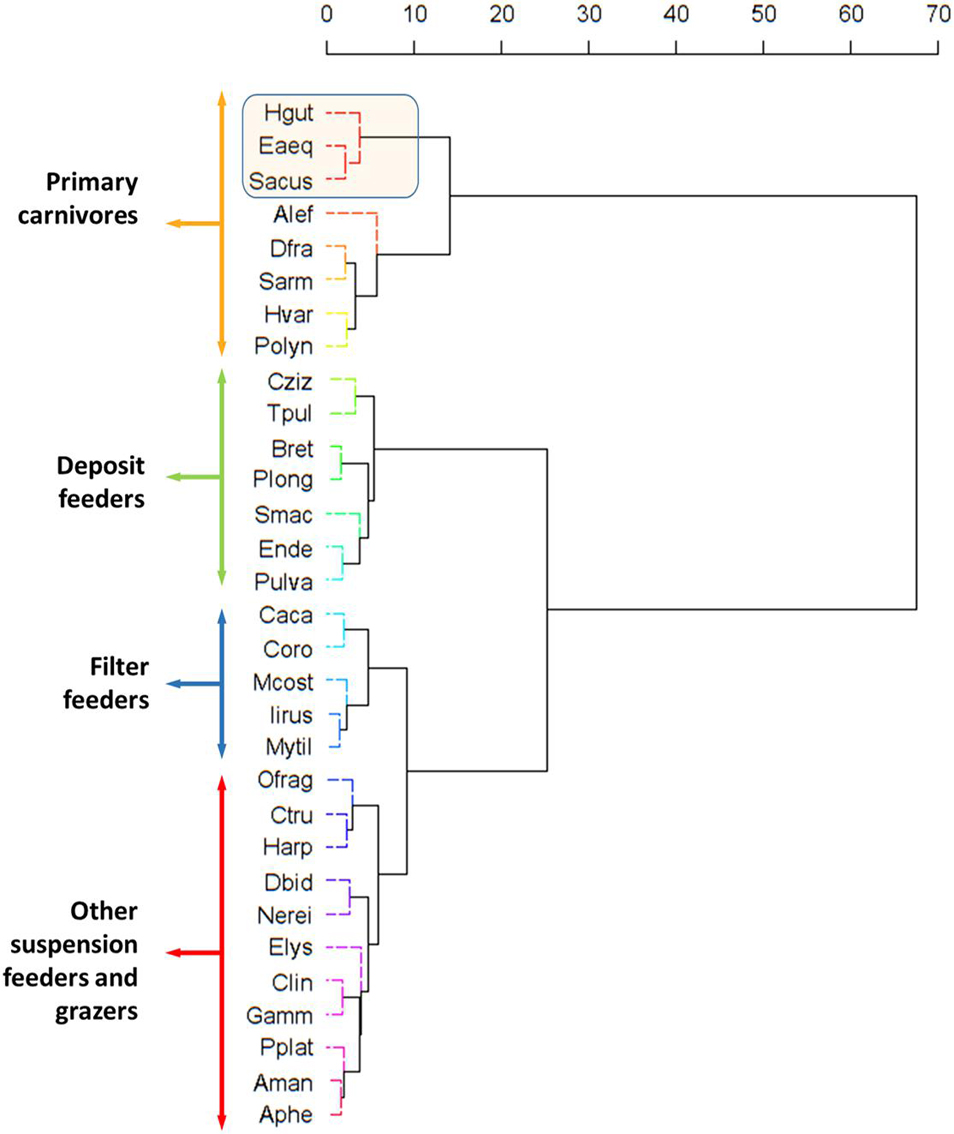
Figure 6. Similarity Profile Analysis (SIMPROF) dendrogram and associated epifauna based on the Bray-Curtis similarity matrix of isotopic data (δ13C and δ15N). Method Cluster: Ward.D; Method Distance: Bray-Curtis. Aman, Amphilochus manudens; Asqu, Amphipholis squamata; Aphe, Apherusa spp.; Alef, Aslia lefevrei; Bret, Bittium reticulatum; Cziz, Calliostoma zizyphinum; Caca, Caprella acanthifera; Clin, Caprella linearis; Coro, Corophium spp.; Ctru, Cymodoce truncata; Dfra, Doto fragilis; Dbid, Dynamene bidentata; Eaeq, Entelurus aequoreus; Elys, Elysia spp.; Ende, Endeis spp.; Gamm, Gammaridae; Hgut, Hippocampus guttulatus; Harp, Harpacticoida; Hvar, Hippolyte varians; Iirus, Irus irus; Mcost, Musculus costulatus; Mytil, Mytilidae; Nerei, Nereididae; Ofrag, Ophiotrix fragilis; Pulva, Peringia ulvae; Plong, Pisidia longicornis; Polyn, Polynoidae; Pplat, Porcellana platycheles; Sacus, Syngnathus acus; Sarm, Siriella armata; Smac, Stylochoplana maculata; Tpul, Tricolia pullus.
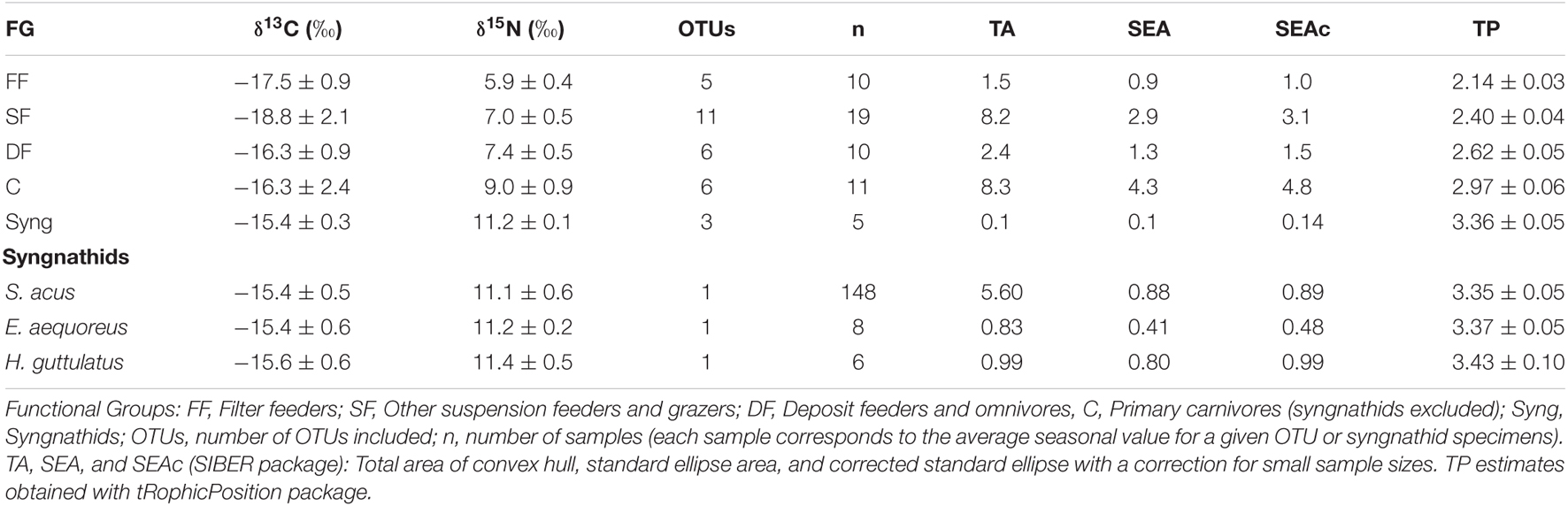
Table 2. Average (mean ± sd) isotopic values (δ13C and δ15N), estimated niche areas (TA, SEA, and SEAc), and trophic position (TP) in functional groups (FG) sampled in spring, summer, and autumn (2017–2018) on Cíes Archipelago.
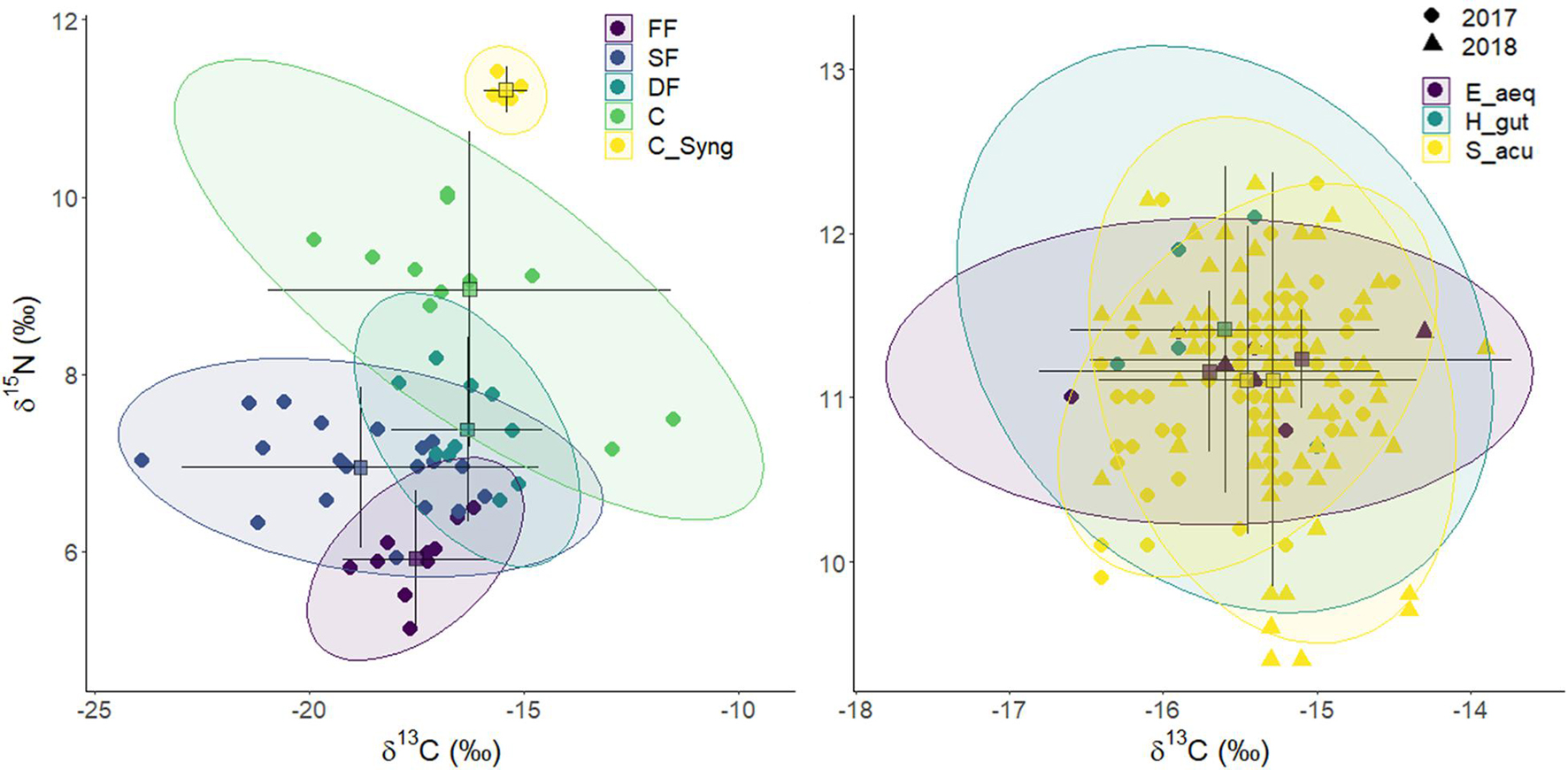
Figure 7. Stable isotope Bayesian ellipses showing trophic niche widths and overlaps between functional groups and syngnathids (E. aequoreus, H. guttulatus, and S. acus) (right) based on isotopic profiles (δ13C and δ15N). Ellipses with 95% credible intervals for the means are based on standard ellipses corrected for small sample sizes (SEAc; isotopic niche metrics; SIBER package). Samples were collected in spring, summer and autumn (2017–2018) on Cíes Archipelago. Each mark corresponds to mean annual isotopic values for each OTUs (left) or individuals (right). FF, Filter feeders; SF, Other suspension feeders and grazers; DF, Deposit feeders and omnivores, C, Primary carnivores (syngnathids excluded); CSyng, Syngnathids.
The two-dimensional NMDS plot (Supplementary Figure 2) of isotopic profiles (δ13C and δ15N) in functional groups revealed annual stability for the whole community (ANOSIM; R: 0.0168; P = 0.197). However, significant dissimilarities were encountered across FG (ANOSIM; R: 0.458; p < 0.0001), mainly due to higher δ15N values (Multipatt, stat = 0.846; p < 0.0001) and TPs (Multipatt, stat = 0.845, p < 0.0001) in carnivores and syngnathids. Mean TP in FF, SF, DF, and C were 2.14 ± 0.03, 2.40 ± 0.04, 2.62 ± 0.05, and 2.97 ± 0.06, respectively (Table 2). The stable isotope Bayesian ellipses showing trophic niche widths (Figure 7) displayed overlaps for all non-carnivorous guilts. Those FG showed large variation in δ13C values, especially in SF.
SIBER analyses revealed highly reduced trophic niche areas (SEAc) for FF (1.5) and syngnathids (0.1) compared to other FG (Table 2). The analysis applied to syngnathids indicated that the pipefish E. aequoreus occupied the smallest niche area (SEAc = 0.48), whereas the niches in S. acus and H. guttulatus were similar (0.89 and 0.99, respectively).
The trophic resemblance across taxa, functional groups and syngnathid species were assessed by the NicheROVER overlap analysis (Table 3). The results obtained confirmed a high degree of overlap between grazers, deposit feeders and omnivores, as well as between suspension feeders, grazers, and omnivores. Moreover, the probability that a syngnathid occupy the niche of lower trophic carnivores was relatively high (41.5%), but the opposite was extremely low (3.4%). Regarding syngnathid species, the trophic niche in E. aequoreus was largely overlapped by those of H. guttulatus (81.5%) and S. acus (90.9%), whereas the trophic niches of H. guttulatus and S. acus largely overlapped (89.2%).
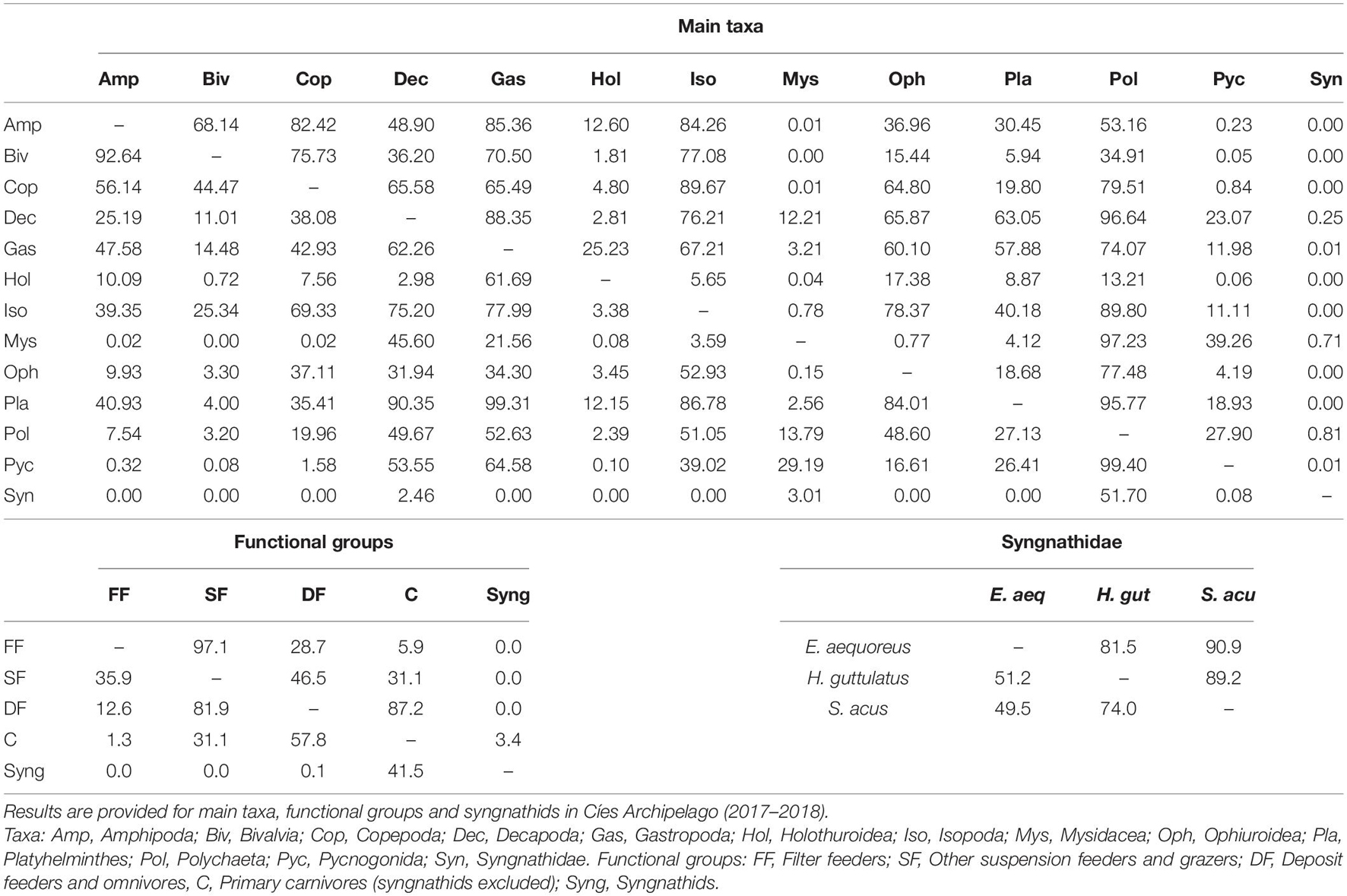
Table 3. Niche overlap estimates (NicheROVER package) showing posterior probabilities (alpha = 0.95) that individuals indicated by the rows will be found within the niches indicated by the column header.
Discussion
The epifauna associated with main canopy-forming macroalgae in Cíes Archipelago was highly dominated by harpacticoid copepods, followed by gastropods, amphipods and bivalves (mainly juveniles), in decreasing order. Globally, epifaunal assemblages showed high annual stability and a marked seasonality effect was noticed, especially from summer to autumn. Seasonal changes in epifauna may be driven by changes in macroalgal assemblages. The rocky bottom biomass in Cíes Archipelago is largely dominated by seasonal and pseudoperennials seaweeds that reach high cover in spring and summer but mostly disappear in autumn and winter (Fernández et al., 2020; Piñeiro-Corbeira et al., 2020; Planas et al., 2021a). This pattern also applies to Cystoseira sensu lato and Codium spp., two sets of locally abundant pseudoperennial seaweeds that shed their phylloids and branches in fall-winter while retaining only the perennial axes until the following spring (Ballesteros, 1990; García-Fernández and Bárbara, 2016). As a result, the biomass and the three-dimensional structure of these seaweeds vary considerably throughout the year (Tuya et al., 2006), possibly explaining at least part of the seasonal variability of the epifaunal assemblage observed during this study.
Changes in epiphytic algal growth (Johnson and Scheibling, 1987; Lutz et al., 2019; Cacabelos et al., 2010) may also affect epifaunal composition as shown in herbivorous gammarids on the North coast of Spain, which augmented abundances in summer in response to the increase of epiphytic algae (Viejo, 1999). Epifaunal abundances are likely governed by epiphytic algal structure rather than macrophyte structure (Martin-Smith, 1993). Accordingly, greater epiphyte load may increase habitat complexity, increasing the space available for epifaunal colonization. However, other studies failed in establishing a relationship between habitat complexity and epifaunal abundance and diversity (Kelaher, 2003). Interestingly, epiphyte load and epifaunal structure may also vary spatially and quantitatively between native and non-indigenous species (Bellan-Santini et al., 1996; Wikström and Kautsky, 2004; Buschbaum et al., 2006; Gestoso et al., 2010) or subspecies, as observed in C. fragile subspecies on rocky intertidal shores in Australia (Lutz et al., 2010).
Epifauna on Canopy-Forming Seaweeds
The morphological characteristics of the seaweeds seemingly explain much of the variation in epifaunal assemblages detected among the seaweed investigated in the Cíes Archipelago. The complex algal morphology-habitat heterogeneity is one of the drivers of host development on seaweed structures, impacting their availability to superior trophic consumers. Some studies also reported differences in the composition of epifauna assemblages depending on the type of seaweed (Bates, 2009; Liuzzi and Gappa, 2011; Png-González et al., 2014) and even across congeneric taxa (Chiarore et al., 2019), but a lack of specificity has been reported in others (Duffy and Hay, 1991; Taylor and Cole, 1994; Russo, 1997; Viejo, 1999). Hence, the evidence and the mechanisms underlying plant-host specificity are conflicting (Wikström and Kautsky, 2004). Anyway, it seems that the physical structure and morphology of seaweeds might strongly determine the biodiversity and abundance of associated faunal assemblages (Orth et al., 1984; Kelaher, 2002, 2003), with coarsely structured algal species supporting lower epifaunal densities than those finely structured (Taylor and Cole, 1994).
Gongolaria baccata supported systematically greater seasonal species richness, but diversity was generally higher in Codium assemblages. Similarities in the composition of their epifauna between both macroalgae became more apparent in spring-summer, when these two pseudoperennial seaweeds have recovered their biomass for the autumn-winter low. Harpacticoids were the most representative epifauna on both macroalgae, but their relative abundances dropped in autumn, accompanying the epiphytic biomass decline (Johnson and Scheibling, 1987). García-Fernández and Bárbara (2016) observed epiphytic seasonality, suggesting the absence of host-specific epiphytes in Cystoseira assemblages in northern Atlantic Iberia. Similarly, Otero-Schmitt and Pérez-Cirera (1996) reported strong seasonal variations for the growth of many filamentous seaweeds, mostly ephemerophyceae, in Cystoseira tamariscifolia (currently Ericaria senegalensis). Epifaunal assemblages are frequently seaweed-dependent, varying among seasons, which in turn also govern algal cover and diversity, and the structure and abundance of epifaunal organisms (Seed et al., 1981; Hicks, 1985; Viejo, 1999; Kumagai and Aoki, 2003; Winkler et al., 2017). Cíes Archipelago is positioned on the mouth of Ría de Vigo, the southern coastal embayment in the NW Iberian Peninsula. Hydrodynamically, the Ría is governed by the patterns of circulation in estuarine systems, and by the coastal upwelling (Prego and Fraga, 1992; Álvarez-Salgado et al., 1993; Nogueira et al., 1997). The high dominance of copepods in spring-early autumn was in agreement with peaks in chlorophyll a in May and September as a result of spring and early autumn blooms (Bode et al., 2020).
Most epifaunal organisms in Cíes Archipelago showed a weak relationship with their host plant and only a few taxa evidenced plant-host specificity. G. baccata seemed to provide less-suitable substratum or less-preferred food sources for gastropods (e.g., P. ulvae, Elysia sp., T. pulus). Fucaceans are finely structured seaweeds that support diversified faunal assemblages, provide habitat, a better refuge for predators, as well as shelter and food resources for a wide variety of organisms (see Chiarore et al., 2019, and references therein). This type of seaweed would provide a greater surface area for periphyton, a suitable surface for grasping, and attachment points to resist water movement in many species such as amphipods (Taylor and Cole, 1994), which contribute significantly to the dietary regime to many species, syngnathid fishes included (Manning et al., 2019). Some amphipods have shown a strong host specificity (Duffy and Hay, 1991; Poore and Steinberg, 1999; Schmidt and Scheibling, 2007; Gestoso et al., 2014). In Cíes, the relative abundance of amphipods was notably higher in G. baccata (22.79%) than in Codium (9.38%). Amphipods would benefit from more attachment sites, preferring more structurally complex macroalgae (G. baccata) over simpler seaweeds (Codium spp.). However, intrinsic host-plant qualities (i.e., presence of nonpolar secondary metabolites or heavy metals) must not be discarded as a driver of amphipod-host preferences, as shown in the herbivorous amphipod Peramphithoe parmerong (Poore and Steinberg, 1999; Roberts et al., 2006).
Trophic Structure
The filter feeder Musculus costulatus occupied the lowest position on the trophic cascade and was selected as the isotopic baseline organism in our study. The species also showed the lowest (and rather stable) δ15N values and high relative abundances. This bivalve is common in many canopy-forming algae on rocky substrates, including Sargassaceae such as Gongolaria, Ericaria, or Cystoseira (Fernández, 2015; Chiarore et al., 2019).
Inter-functional group differences in average δ15N values were 1.1 (FF-SF), 0.4 (SF-DF), 1.6 (DF-C), and 2.2 (C-Syngnathids). Considering that FF and C (syngnathids excluded) occupied the lower and top positions, respectively, in our epifaunal structure cascad, the resulting trophic discrimination factor (TDF) for 15N was 3.1‰, a value within the range of the generalist values (2–5‰) commonly applied in trophic studies (DeNiro and Epstein, 1981; Minagawa and Wada, 1984; Post, 2002; Caut et al., 2009). The TDF estimated between FF and syngnathids (5.3‰) was slightly higher than those experimentally derived for adult H. guttulatus fed on mysidaceans or a mixture of mysidaceans and Artemia (3.9–4.2‰) (Planas et al., 2020a). Dissimilarities would rely on the fact that TDF values are diet-dependent (Caut et al., 2008, 2009). The values for δ13C did not follow any pattern on the trophic structure, reflecting the effect of different organic matter sources and their seasonal fluctuations on the diet of each organism. This feature was already reported by Vizzini and Mazzola (2004) in a pipefish community from a western Mediterranean seagrass meadow. Nevertheless, the estimated TDF for δ13C was 2.1‰, a slightly lower value than the experimentally derived TDF (2.5–3.2‰) (Planas et al., 2020a).
Syngnathids
From the point of view of potential habitats for syngnathids, the macroalgal communities that characterize sites A and B were dominated by Codium spp. (Planas et al., 2021b), whereas site C was dominated by Gongolaria spp. Aside from differences in epifaunal composition and habitat complexity, the macroalgal community on site C promoted a higher diversity and abundance of syngnathids by providing better camouflage and shelter. One of the main concerns regarding seaweed conservation in Cíes Archipelago is a progressive increase the abundance of less optimal turf species (Halopteris scoparia, Chondria coerulescens, or Corallina spp.), and non-native (Codium fragile, Asparagopsis armata) (Planas et al., 2021a). Even though the abundance of some syngnathids (e.g., E. aequoreus) can be promoted by the new habitats provided by some introduced seaweeds (Polte and Buschbaum, 2008), the changes observed in recent years in Cíes Archipelago would likely induce a decrease in the structural heterogeneity possibly representing a major threat to syngnathid populations. Thus, a decreased habitat complexity may negatively impact (i.e., being more easily preyed upon) species with fragmented distribution and a high spatial demographic heterogeneity such as H. guttulatus (Gristina et al., 2014; Lazic et al., 2018).
Lower epifaunal richness and complexity was recorded in autumn due to the drastic decrease of seaweed cover. This finding is common in faunal communities of southern Europe when seaweeds disappear due to storms and strong currents (López de la Rosa et al., 2002). From the perspective of wave exposure, sites A and C were the most and less exposed, respectively (Hernández-Urcera et al., 2021). Castejón-Silvo et al. (2021) recently demonstrated that the declines in biogenic habitat structure (e.g., kelp or seagrass beds) increase water flow velocity and wave exposure, impacting associated pipefishes through increased energy expenditure. Hence, increasing structural complexity in less exposed seaweed assemblages (e.g., site C) would alleviate adverse environmental conditions caused by currents, especially in species (e.g., S. acus and E. aequoreus) lacking a prehensile tail to hold on to vegetation, and may partly explain the higher occurrence and diversity of syngnathids in site C.
Predator-prey interactions are influenced by vegetational densities, leave shape, shoots density, and the foraging strategy of the predator (Orth et al., 1984). Syngnathids are ambush predators that capture the prey by rapid suction (de Lussanet and Muller, 2007; Roos et al., 2009). Their diets are strongly related to head characteristics (i.e., head shape, relative snout lengths, and gape sizes) (Manning et al., 2019). The feeding apparatus of syngnathids is highly specialized for a fast and powerful pivot feeding (Leysen et al., 2011) with its tiny and elongate tubular snout but toothless jaws. Seahorses are tail-attached predators with enlarged strike distances compared to pipefishes (Van Wassenbergh et al., 2011). A comparative study on several syngnathids concluded that the more specialized a species (longer snout), the more morphologically constrained it is (less variation) (Manning et al., 2019). The study was based on the assumption that long-snouted syngnathids are more specialized (Franzoi et al., 1993; Kendrick and Hyndes, 2005). Syngnathids inhabiting Cíes Archipelago conformed to the elongate snout form. Data on head and gape biometrics are not available, though direct observations showed that mouth gape size was smaller in the snake pipefish. This feature agrees with our results of TP and niche overlap estimates in Cíes, which indicate that the three syngnathids species have similar but not identical feeding regimes. Feeding sources were likely narrower in the snake pipefish E. aequoreus, a pelagic fish in the temperate northeastern Atlantic that can be regularly found offshore throughout its range at low densities (Harris et al., 2007), and is rarely present in coastal ecosystems in our region.
Vertical migrations may have a great influence on the configuration of epifaunal assemblages associated with vegetal communities (Sánchez-Jerez et al., 1999). Since our surveys were systematically carried out in the morning, the effect of daily vertical migrations in the structure of the epifauna was not assessed. Like most syngnathids, the species identified in Cíes Archipelago are considered diurnal feeders (James and Heck, 1994; Kendrick and Hyndes, 2005; Felicio et al., 2006). However, their feeding behavior and swimming capabilities differ considerably. In contrast to the active swimming of both pipefishes, the seahorse H. guttulatus tends to be a “sit-and-wait” predator that occasionally pursues prey. Nevertheless, this distinguishing feature does not seem to significantly affect its TP.
Syngnathids in Cíes Archipelago were mostly large adults that most likely stay there temporarily (spring–early autumn) for reproduction, migrating to more sheltered sites before the seaweed assemblages vanish in autumn and winter due to storms and strong sea currents (Lazzari and Able, 1990; Vincent et al., 1995; Monteiro et al., 2001; Masonjones et al., 2010; Planas et al., 2021a). Their presence in Cíes Archipelago largely coincides with their breeding season (March-April until September-October). The release of newborn batches by males was synchronized with the period of maximal relevance of phytal harpacticoid copepods (Supplementary Figure 3), one of the main food sources for early developing specimens (Tipton and Bell, 1988; Vizzini and Mazzola, 2004), suggesting that free-living juveniles possibly benefit from a higher food supply during the breeding season (Polte and Buschbaum, 2008).
Reconstruction of Syngnathids Diet
The SIMM polygon is provided in Figure 8 and the models run without informative priors revealed that S. acus mostly fed on amphipods (42 ± 7%), followed by isopods, carideans and copepods, whereas mysidaceans did not seem to contribute largely to the diet (Table 4). The estimates provided by SIMMs run with informative priors displayed similar results for amphipods (41 ± 8%), with lower contributions of copepods and mysidaceans and higher percentages for carideans and isopods. The large standard deviations of the means would be the result of inter-individual differences in the feeding regime.
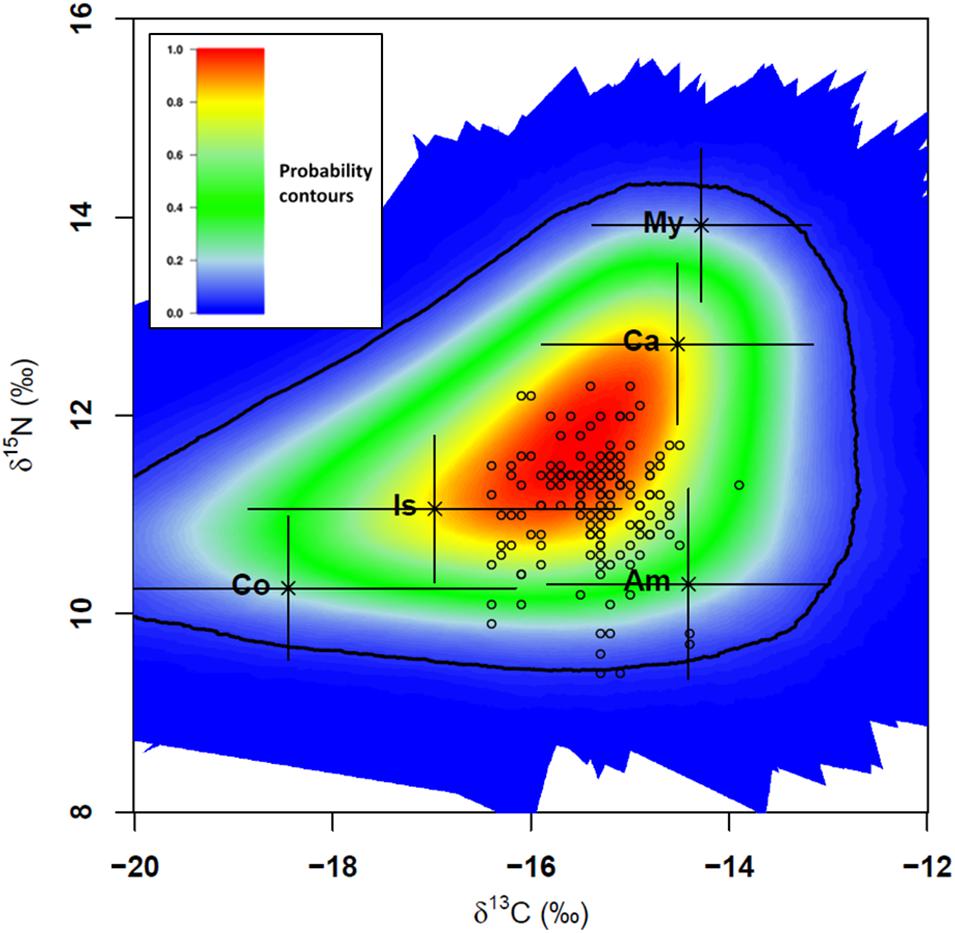
Figure 8. Stable Isotope Mixing Models (SIMM) polygon for Syngnathus acus (n = 148; 2017 and 2018 surveys) relative to average δ13C and δ15N signatures of five potential prey sources (mean ± SD; black crosses) adjusted for trophic discrimination factor (TDF) values and consumers (open circles). TDF values: 2.5 for 13C and 3.9 for 15N (Planas et al., 2020a). The colored region represents the 95% confidence interval. Probability contours are at the 5% level. Two consumers with a low probability (<5%) were not included in the subsequent mixing model (Smith et al., 2013). Sources: Am, Amphipoda; Co, Copepoda; Ca, Caridea; Is, Isopoda; My, Mysidacea.
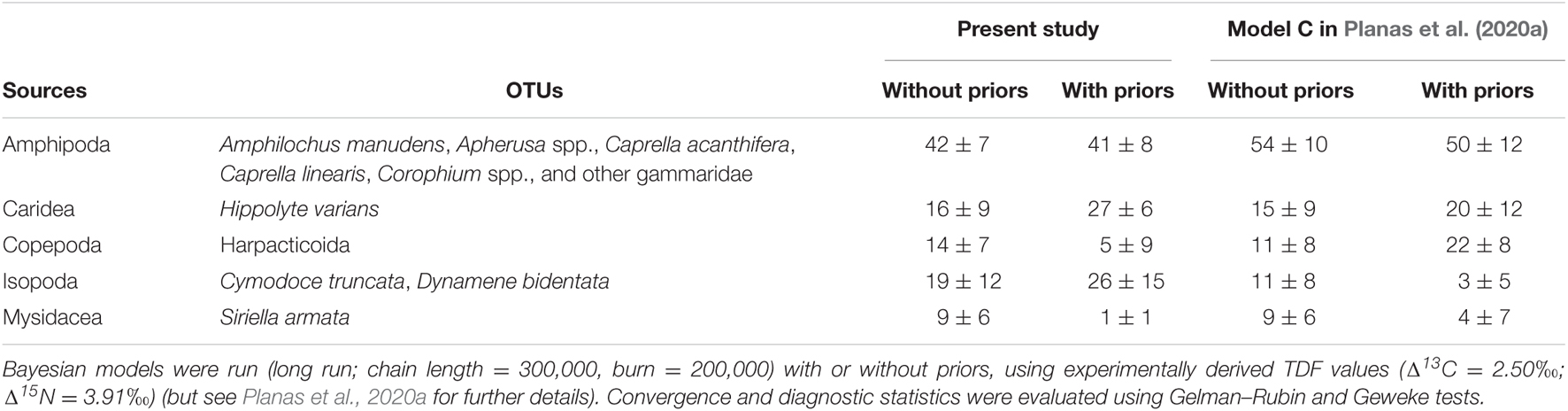
Table 4. Percent diet (mean ± sd) composition for prey sources as estimated by Bayesian Stable Isotope Mixing Model (SIMM) (MixSIAR package in R v. 3.1.12; Stock et al., 2018) based on dorsal fin tissue of the pipefish Syngnathus acus (n = 146) sampled on Cíes Archipelago in 2017 and 2018.
Our results strongly agree with a preliminary survey carried out in spring and summer 2016 (Planas et al., 2020a). In that study, S. acus was considered a specialist predator foraging preferentially on amphipods near the bottom. Patches of mysidaceans in Cíes Archipelago are commonly found above the seabed, being less accessible to syngnathids than other epiphytic prey. A recent study using DNA metabarcoding did not reveal the presence of mysidaceans in H. guttulatus feces from Taranto Mar Piccolo (SE Italy) (Lazic et al., 2021). It is feasible that mysidaceans may be part of the bulk diet in S. acus when other preferred prey are less available, as reported in other syngnathids (e.g., S. taenionotus) (Franzoi et al., 1993). The same hypothesis could be also applied to Hippolyte varians, a caridean with low average abundances (0.19%) but remarkably represented in the bulk diet of S. acus (>16%). Syngnathids staying in Cíes in autumn, when food supply and diversity decreased, would benefit from the maximal values reached by H. varians in that season, especially in Codium spp. (0.45–0.55%).
The three syngnathid species identified in our study occurred sympatrically in site C, and they probably compete for similar, but not identical, food resources. Dietary regimes for H. guttulatus and E. aequoreus were not estimated due to scarce availability. However, their limited abundances should not entail restrictions in prey availability. Furthermore, the spatial segregation observed in some pipefishes (Malavasi et al., 2007) was not investigated in detail in Cíes Archipelago. However, direct observations showed that seahorses were generally attached to canopies above the substrate whereas the pipefishes were located on micro-habitats near the substrate. As indicated above, it is unlikely that the diet in the seahorse species differed substantially from that in S. acus (Valladares et al., 2017), but the dietary regime in E. aequoreus remains uncertain and needs further research. The TP of this pipefish did not differ significantly from that of the other two species. However, its small gape size and narrower trophic niche would promote the feeding on smaller and less mobile prey (e.g., copepods) (Kendrick and Hyndes, 2005; Polte and Buschbaum, 2008), foraging on a micro-habitat as pointed out in other sympatric pipefishes (Franzoi et al., 1993).
Conclusion
The differences in epiphytic communities in Cíes Archipelago could be partly explained by the structural complexity of canopy-forming macroalgae and seasonality. Assemblages of the fucacean Gongolaria baccata showed greater seasonal richness in epifaunal species compared to Codium spp., but the diversity was similar for both seaweed assemblages. Only a few epifaunal groups revealed a plant-host specificity, with amphipods being among the most representative epifauna on G. baccata. The three syngnathid species identified in our study preferred sheltered sites dominated by the more structurally complex macroalgae G. baccata, but the dominant snake pipefish Syngnathus acus appeared to be more generalist considering global abundances and seasonal fluctuations. Regarding dietary regimes, all species occupied the same TP; however, the range of prey sources in the pipefish Entelurus aequoreus was likely narrower than in S. acus and the seahorse Hippocampus guttulatus. The study revealed the significance of site C (Borrón–Rodas Beach) as a marine reserve for syngnathids on the National Park and confirmed amphipods as the main contributor to the dietary regime. However, trophic plasticity underlying spatial and habitat effects would be expected and a spatio-temporal analysis is currently ongoing. The results achieved also suggest the importance of Gongolaria-like species for European syngnathids and other fauna on the Atlantic coasts and the need for their conservation.
Data Availability Statement
The original contributions presented in the study are included in the article/Supplementary Material, further inquiries can be directed to the corresponding author.
Ethics Statement
The animal study was reviewed and approved by the Regional Government Xunta de Galicia: Reference REGA ES360570202001/16/FUN/BIOL.AN/MPO02.
Author Contributions
MP: funding acquisition, project administration, conceptualization, methodology, stable isotopes, data curation, formal analysis, visualization, writing–original draft preparation, and writing–review and editing. CP-C: field sampling, conceptualization, methodology, identification and quantification of epifauna, data curation, and assistance in manuscript writing. LI, RN, SC, AJ, and MR: identification and quantification of epifauna, stable isotope analyses, and data curation. RB: data curation and assistance in manuscript writing. All authors revised and approved the manuscript.
Funding
This study was financially supported by the Spanish Government with project Hippoparques (Ref. 1541S/2015, Organismo Autónomo de Parques Nacionales de España, Ministerio para la Transición Ecológica y Reto Demográfico, MITECO, Spain).
Conflict of Interest
The authors declare that the research was conducted in the absence of any commercial or financial relationships that could be construed as a potential conflict of interest.
Publisher’s Note
All claims expressed in this article are solely those of the authors and do not necessarily represent those of their affiliated organizations, or those of the publisher, the editors and the reviewers. Any product that may be evaluated in this article, or claim that may be made by its manufacturer, is not guaranteed or endorsed by the publisher.
Acknowledgments
We acknowledge A. Chamorro, M. E. Garci, I. Bárbara, V. Peña, P. Díaz-Tapia, R. Chamorro, J. Hernández-Urcera, I. Ferreiro, D. Costas, J. Cremades, K. Nedelec, the staff and keepers of PNIA and PNAC, and the Regional Government Xunta de Galicia for their support in the project. We also acknowledge support of the publication fee by the CSIC Open Access Publication Support Initiative through its Unit of Information Resources for Research (URICI).
Supplementary Material
The Supplementary Material for this article can be found online at: https://www.frontiersin.org/articles/10.3389/fmars.2021.773367/full#supplementary-material
References
Adams, S. A. (1976). The ecology of eelgrass, Zostera marina (L.), fish communities. I. Structural analysis. J. Exp. Mar. Biol. Ecol. 22, 269–291. doi: 10.1016/0022-0981(76)90007-1
Ahnesjö, I., and Craig, J. F. (2011). The biology of Syngnathidae: pipefishes, seadragons and seahorses. J. Fish Biol. 78, 1597–1602. doi: 10.1111/j.1095-8649.2011.03008.x
Álvarez, I., de Castro, M., Gómez-Gesteira, M. G., and Prego, R. (2005). Inter- and intra-annual analysis of the salinity and temperature evolution in the Galician Rías Baixas-ocean boundary (northwest Spain). J. Geophys. Res. 110:C04008. doi: 10.1029/2004JC002504
Álvarez-Salgado, X. A., Rosón, G., Pérez, F. F., and Pazos, Y. (1993). Hydrographic variability of the Rías Baixas (NW Spain) during the upwelling season. J. Geophys. Res. 98, 14447–14455. doi: 10.1029/93JC00458
Ape, F., Corriero, G., Mirto, S., Pierri, C., Lazic, T., and Gristina, M. (2019). Trophic flexibility and prey selection of the wild long-snouted seahorse Hippocampus guttulatus Cuvier, 1829 in three coastal habitats. Estuar. Coast. Shelf Sci. 224, 1–10. doi: 10.1016/j.ecss.2019.04.034
Ballesteros, E. (1990). Structure and dynamics of the Cystoseira caespitosa Sauvageau (Fucales, Phaeophyceae) community in the North-Western Mediterranean. Sci. Mar. 54, 155–168.
Ballesteros, E., Torras, X., Pinedo, S., Garcia, M., Mangialajo, L., and de Torres, M. (2007). A new methodology based on littoral community cartography dominated by macroalgae for the implementation of the European Water Framework Directive Mar. Pollut. Bull. 55, 172–180. doi: 10.1016/j.marpolbul.2006.08.038
Barrientos, S., Barreiro, R., Cremades, J., and Piñeiro-Corbeira, C. (2020). Setting the basis for a long-term monitoring network of intertidal seaweed assemblages in northwest Spain. Mar. Environ. Res. 160:105039. doi: 10.1016/j.marenvres.2020.105039
Bates, C. R. (2009). Host taxonomic relatedness and functional-group affiliation as predictors of seaweed–invertebrate epifaunal associations. Mar. Ecol. Prog. Ser. 387, 125–136. doi: 10.3354/MEPS08089
Bellan-Santini, D., Arnaud, P. M., Bellan, G., and Verlaque, M. (1996). The influence of the introduced tropical alga Caulerpa taxifolia, on the biodiversity of the Mediterranean marine biota. J. Mar. Biol. Assoc. U. K. 76, 235–237. doi: 10.1017/S0025315400029180
Bergert, B. A., and Wainwright, P. C. (1997). Morphology and kinematics of prey capture in the syngnathid fishes Hippocampus erectus and Syngnathus floridae. Mar. Biol. 127, 563–570.
Bertelli, C. M., and Unsworth, R. K. (2014). Protecting the hand that feeds us: seagrass (Zostera marina) serves as commercial juvenile fish habitat. Mar. Pollut. Bull. 83, 425–429.
Bode, A., Álvarez, M., García, L. M., Louro, M. A., Nieto-Cid, M., Ruíz-Villarreal, M., et al. (2020). Climate and local hydrography underlie recent regime shifts in plankton communities off Galicia (NW Spain). Oceans 1, 181–197. doi: 10.3390/oceans1040014
Brawley, S. (1992). “Mesoherbivores,” in Plant–Animal Interactions in the Marine Benthos, eds D. M. John, S. J. Hawkins, and J. H. Price (Oxford: Clarendon Press), 235–263.
Buschbaum, C., Chapman, A. S., and Saier, B. (2006). How an introduced seaweed can affect epibiota diversity in different coastal systems. Mar. Biol. 148, 743–754. doi: 10.1007/s00227-005-0128-9
Cabana, G., and Rasmussen, J. B. (1996). Comparison of aquatic food chains using nitrogen isotopes. Proc. Natl. Acad. Sci. U.S.A. 93, 10844–10847. doi: 10.1073/pnas.93.20.10844
Cacabelos, E., Olabarria, C., Incera, M., and Troncoso, J. S. (2010). Effects of habitat structure and tidal height on epifaunal assemblages associated with macroalgae. Estuar. Coast. Shelf Sci. 89, 43–52. doi: 10.1016/j.ecss.2010.05.012
Castejón-Silvo, I., Terrados, J., Nguyen, T., Jutfelt, F., and Infantes, E. (2021). Increased energy expenditure is an indirect effect of habitat structural complexity loss. Funct. Ecol. 2021, 1–13. doi: 10.1111/1365-2435.13876
Castro-Fernández, J., Castejón-Silvo, I., Arechavala-López, P., Terrados, J., and Morales-Nin, B. (2020). Feeding ecology of pipefish species inhabiting Mediterranean seagrasses. Mediterr. Mar. Sci. 21, 705–718. doi: 10.12681/mms.22455
Caut, S., Angulo, E., and Courchamp, F. (2008). Caution on isotopic model use for analyses of consumer diet. Can. J. Zool. 86, 438–445. doi: 10.1139/Z08-012
Caut, S., Angulo, E., and Courchamp, F. (2009). Variation in discrimination factors (Δ15N and Δ13C): the effect of diet isotopic values and applications for diet reconstruction. J. Appl. Ecol. 46, 443–453. doi: 10.1111/j.1365-2664.2009.01620.x
Chiarore, A., Bertocci, I., Fioretti, S., Meccariell, A., Saccone, G., Crocett, F., et al. (2019). Syntopic Cystoseira taxa support different molluscan assemblages in the Gulf of Naples (southern Tyrrhenian Sea). Mar. Freshw. Res. 70, 1561–1575. doi: 10.1071/MF18455
Chen, Y. Y., Cooper, P., and Fulton, C. J. (2020). Sargassum epifaunal communities vary with canopy size, predator biomass and seascape setting within a fringing coral reef ecosystem. Mar. Ecol. Prog. Ser. 640, 17–30. doi: 10.3354/meps13282
Clarke, K. R. (1993). Non-parametric multivariate analyses of changes in community structure. Austral Ecol. 18, 117–143. doi: 10.1111/j.1442-9993.1993.tb00438.x
Clarke, K. R., Somerfield, P. J., and Gorley, R. N. (2008). Testing of null hypotheses in exploratory community analyses: similarity profiles and biota-environment linkage. J. Exp. Mar. Biol. Ecol. 366, 56–69. doi: 10.1016/j.jembe.2008.07.009
Correia, M., Caldwell, I., Koldewey, H., Andrade, J. P., and Palma, J. (2015). Seahorse (Hippocampinae) population fluctuations in the Ria Formosa Lagoon, south Portugal. J. Fish Biol. 87, 679–690. doi: 10.1111/jfb.12748
Correia, M., Koldewey, H. J., Andrade, J. P., Esteves, E., and Palma, J. (2018). Identifying key environmental variables of two seahorse species (Hippocampus guttulatus and Hippocampus hippocampus) in the Ria Formosa lagoon, South Portugal. Environ. Biol. Fishes 101, 1357–1367. doi: 10.1007/s10641-018-0782-7
Curtis, J. M. R., and Vincent, A. C. J. (2005). Distribution of sympatric seahorse species along a gradient of habitat complexity in a seagrass-dominated community. Mar. Ecol. Prog. Ser. 291, 81–91. doi: 10.3354/meps291081
Curtis, J. M. R., and Vincent, A. C. J. (2006). Life history of an unusual marine fish: survival, growth and movement patterns of Hippocampus guttulatus (Cuvier 1829). J. Fish Biol. 68, 707–733. doi: 10.1111/j.1095-8649.2006.00952.x
Dawson, C. E. (1986). “Syngnathidae,” in Fishes of the North-Eastern Atlantic and the Mediterranean, eds P. J. P. Whitehead, M. L. Bauchot, J. C. Hereau, J. Nielsen, and E. Tortonese (Paris: UNESCO), 628–639.
De Cáceres, M., and Legendre, P. (2009). Associations between species and groups of sites: indices and statistical inference. Ecology 90, 3566–3574. doi: 10.1890/08-1823.1
de Lussanet, M. H. C., and Muller, M. (2007). The smaller your mouth, the longer your snout: predicting the snout length of Syngnathus acus, Centriscus scutatus and other pipette feeders. J. R. Soc. Interface 4, 561–573. doi: 10.1098/rsif.2006.0201
DeNiro, M. J., and Epstein, S. (1981). Influence of diet on the distribution of nitrogen isotopes in animals. Geochim. Cosmochim. Act. 45, 341–351. doi: 10.1016/0016-7037(81)90244-1
Didenko, A., Kruzhylina, S., and Gurbyk, A. (2018). Feeding patterns of the black-striped pipefish Syngnathus abaster in an invaded freshwater habitat. Environ. Biol. Fishes 101, 917–931. doi: 10.1007/s10641-018-0747-x
Duffy, J. E., and Hay, M. E. (1991). Food and shelter as determinant of food choice by an herbivorous marine amphipod. Ecology 72, 1286–1298. doi: 10.2307/1941102
Edgar, G. J., and Moore, P. G. (1986). “Macro-algae as habitats for motile macrofauna,” in Monografias Biológicas. Simposio Internacional. Usos Funciones Ecológicas de las Algas Marinas Bentónicas, ed. B. Santelices (New York, NY: Springer), 255–277.
Espino, F., Tuya, F., Brito, A., and Haroun, R. (2011). Ichthyofauna associated with Cymodocea nodosa meadows in the Canarian Archipelago (central eastern Atlantic): community structure and nursery role. Cienc. Mar. 37, 157–174. doi: 10.7773/cm.v37i2.1720
Felicio, A. K. C., Rosa, I. L., Souto, A., and Freitas, R. H. A. (2006). Feeding behavior of the longsnout seahorse Hippocampus reidi Ginsburg, 1933. J. Ethol. 24, 219–225. doi: 10.1007/s10164-005-0189-8
Fernández, D. (2015). La reserva Marina de Interés Pesquero Os Miñarzos: Línea Base de las Comunidades Biológicas y Análisis del Efecto Reserva Temprano. Doctoral Thesis. A Coruña: University of A Coruña, 315.
Fernández, E., Barañano, C., Alejo, I., Barreiro, R., Bellas, J., Besada, V., et al. (2020). Islas Cíes: Un Ecosistema en la Frontera. Vigo: Concello de Vigo.
Flynn, A. J., and Ritz, D. A. (1999). Effect of habitat complexity and predatory style on the capture success of fish feeding on aggregated prey. J. Exp. Mar. Biol. Ecol. 79, 487–494. doi: 10.1017/S0025315498000617
Foster, S. J., and Vincent, A. C. J. (2004). Life history and ecology of seahorses: implications for conservation and management. J. Fish Biol. 65, 1–61. doi: 10.1111/j.0022-1112.2004.00429.x
Franco, A., Franzoi, P., Malavasi, S., Riccato, F., and Torricelli, P. (2006). “Fish assemblages in different shallow water habitats of the Venice Lagoon,” in Marine Biodiversity. Developments in Hydrobiology, Vol. 183, eds K. Martens, H. Queiroga, M. R. Cunha, A. Cunha, M. H. Moreira, V. Quintino, et al. (Dordrecht: Springer). doi: 10.1007/1-4020-4697-9_13
Franzoi, P., Maccagnani, R., Rossi, R., and Ceccherelli, V. U. (1993). Life cycles and feeding habits of Syngnathus taenionotus and S. abaster (Pisces, Syngnathidae) in a Brackish Bay of the Po River Delta (Adriatic Sea). Mar. Ecol. Prog. Ser. 97, 71–81. doi: 10.3354/meps097071
García-Fernández, A., and Bárbara, I. (2016). Studies of Cystoseira assemblages in Northern Atlantic Iberia. An. Jard. Bot. Madr. 73:e035. doi: 10.3989/ajbm.2403
Gee, M. J., and Warwick, R. M. (1994). Body-size distribution in a marine metazoan community and the fractal dimensions of macroalgae. J. Exp. Mar. Biol. Ecol. 178, 247–259. doi: 10.1016/0022-0981(94)90039-6
Gestoso, I., Olabarria, C., and Troncoso, J. S. (2010). Variability of epifaunal assemblages associated with native and invasive macroalgae. Mar. Freshw. Res. 61, 724–731. doi: 10.1071/MF0925
Gestoso, I., Olabarria, C., and Troncoso, J. S. (2014). Selection of habitat by a marine amphipod. Mar. Ecol. 35, 103–110. doi: 10.1111/maec.12068
Gristina, M., Cardone, F., Carlucci, R., Castellano, L., Passarelli, S., and Corriero, G. (2014). Abundance, distribution and habitat preference of Hippocampus guttulatus and Hippocampus hippocampus in a semi-enclosed central Mediterranean marine area. Mar. Ecol. 36, 57–66. doi: 10.1111/maec.12116
Guiry, M. D., and Guiry, G. M. (2020). AlgaeBase. World-wide Electronic Publication, National University of Ireland. 2020. Available online at: https://www.algaebase.org (accessed February 26 2020)
Gürkan, Ş, Taşkavak, E., Sever, T. M., and Akalın, S. (2011). Gut contents of two European seahorses Hippocampus hippocampus and Hippocampus guttulatus in the Aegean Sea, Coasts of Turkey. Pak. J. Zool. 43, 1197–1201.
Gürkan, S., and Taskavak, E. (2019). The relationships between gut length and prey preference of three pipefish (Syngnathus acus, Syngnathus typhle, Nerophis ophidion Linnaeus, 1758) species distributed in Aegean Sea, Turkey. Iran. J. Fish. Sci. 18, 1093–1100. doi: 10.22092/ijfs.2019.118277
Harris, M. P., Beare, D., Toresen, R., Nottestad, L., Kloppmann, M., Dorner, H., et al. (2007). A major increase in snake pipefish (Entelurus aequoreus) in northern European seas since 2003: potential implications for seabird breeding success. Mar. Biol. 151, 973–983. doi: 10.1007/s00227-006-0534-7
Hernández-Urcera, J., Murillo, F. J., Regueira, M., Cabanella-Reboredo, M., and Planas, M. (2021). Preferential habitats prediction in syngnathids using species distribution models. Mar. Environ. Res. 172, 105488. doi: 10.1016/j.marenvres.2021.105488
Hicks, G. R. F. (1985). “Meiofauna associated with rocky shore algae,” in The Ecology of Rocky Coasts, eds P. G. Moore and R. Seed (London: Hodder and Stoughton), 36–56.
Horinouchi, M., Tongnunui, P., Furumitsu, K., Nakamura, Y., Kanou, K., Yamaguchi, A., et al. (2012). Food habits of small fishes in seagrass habitats in Trang, southern Thailand. Fish. Sci. 78, 577–587. doi: 10.1007/s12562-012-0485-5
Howard, R., and Koehn, J. (1985). Population dynamics and feeding ecology of pipefish (Syngnathidae) associated with eelgrass beds of Western Port, Victoria. Mar. Freshw. Res. 36, 361–370. doi: 10.1071/MF9850361
IUCN (2019). The IUCN Red List of Threatened Species. Version 2019-1. 2019. Available online at: http://www.iucnredlist.org. (accessed October 16, 2019)
Jackson, A. L., Inger, R., Parnell, A. C., and Bearhop, S. (2011). Comparing isotopic niche widths among and within communities: SIBER–Stable isotope Bayesian ellipses in R. J. Anim. Ecol. 80, 595–602. doi: 10.1111/j.1365-2656.2011.01806.x
James, P. L., and Heck, K. L. Jr. (1994). The effects of habitat complexity and light intensity on ambush predation within a simulated seagrass habitat. J. Exp. Mar. Biol. Ecol. 176, 187–200. doi: 10.1016/0022-0981(94)90184-8
Jaschinski, S., Hansen, T., and Sommer, U. (2008). Effects of acidification in multiple stable isotope analyses. Limnol. Oceanogr. Methods 6, 12–15. doi: 10.4319/lom.2008.6.12
Johnson, S. C., and Scheibling, R. E. (1987). Structure and dynamics of epifaunal assemblages on intertidal macroalgae Ascophyllum nodosum and Fucus vesiculosus in Nova Scotia, Canada. Mar. Ecol. Prog. Ser. 37, 209–227. doi: 10.3354/meps037209
Jones, G. P. (1988). Ecology of rocky reef fish of noith-eastern New Zealand: a review. N. Z. J. Mar. Freshw. Res. 22, 445–462. doi: 10.1080/00288330.1988.9516315
Kelaher, B. P. (2002). Influence of physical characteristics of coralline turf on associated macrofaunal assemblages. Mar. Ecol. Prog. Ser. 232, 141–148. doi: 10.3354/MEPS232141
Kelaher, B. P. (2003). Changes in habitat complexity negatively affect diverse gastropod assemblages in coralline algal turf. Oecologia 135, 431–441. doi: 10.1007/s00442-003-1196-5
Kendrick, A. J., and Hyndes, G. A. (2005). Variations in the dietary compositions of morphologically diverse syngnathid fishes. Environ. Biol. Fishes 72, 415–427. doi: 10.1007/s10641-004-2597-y
Kindt, R., and Coe, R. (2005). Tree Diversity Analysis. A Manual and Software for Common Statistical Methods for Ecological and Biodiversity Studies. Nairobi: World Agroforestry Centre (ICRAF).
Kitsos, M. S., Tzomos, T., Anagnostopoulou, L., and Koukouras, A. (2008). Diet composition of the seahorses, Hippocampus guttulatus Cuvier, 1829 and Hippocampus hippocampus (L., 1758) (teleostei, syngnathidae) in the Aegean Sea. J. Fish Biol. 72, 1259–1267. doi: 10.1111/j.1095-8649.2007.01789.x
Kumagai, N., and Aoki, M. (2003). Seasonal changes in the epifaunal community on the shallow-water gorgonian Melithaea flabellifera. J. Mar. Biol. Assoc. U. K. 83, 1221–1222. doi: 10.1017/S002531540300852X
Lawton, J. H., and Jones, C. G. (1995). “Linking species and ecosystems: organisms as ecosystem engineers,” in Linking Species and Ecosystems, eds C. G. Jones and J. H. Lawton (Boston, MA: Springer). doi: 10.1007/978-1-4615-1773-3_14
Lazic, T., Pierri, C., Gristina, M., Carlucci, R., Cardone, F., Colangelo, P., et al. (2018). Distribution and habitat preferences of Hippocampus species along the Apulian coast. Aquat. Conserv. 28, 1317–1328. doi: 10.1002/aqc.2949
Lazic, T., Pierri, C., Corriero, G., Balech, B., Cardone, F., Deflorio, M., et al. (2021). Evaluating the efficiency of DNA metabarcoding to analyze the diet of Hippocampus guttulatus (Teleostea: Syngnathidae). Life 11:998. doi: 10.3390/life11100998
Lazzari, K., and Able, K. W. (1990). Northern pipefish, Syngnathus fuscus, occurrences over the Mid-Atlantic Bight continental shelf: evidence of seasonal migration. Environ. Biol. Fishes 27, 177–185. doi: 10.1007/BF00001671
Leysen, H., Roos, G., and Adriaens, D. (2011). Morphological variation in head shape of pipefishes and seahorses in relation to snout length and developmental growth. J. Morphol. 272, 1259–1270. doi: 10.1002/jmor.10982
Liuzzi, M. G., and Gappa, J. L. (2011). Algae as hosts for epifaunal bryozoans: role of functional groups and taxonomic relatedness. J. Sea Res. 65, 28–32. doi: 10.1016/j.seares.2010.06.007
López de la Rosa, I., Raso, J., and Rodríguez, A. (2002). Evolution of a decapod community (Crustacea) of shallow soft bottoms with seaweeds from southern Europe. J. Mar. Biol. Assoc. U. K. 82, 85–95. doi: 10.1017/S0025315402005209
Logan, J. M., Jardine, T. D., Miller, T. J., Bunn, S. E., Cunjak, R. A., and Lutcavage, M. E. (2008). Lipid corrections in carbon and nitrogen stable isotope analyses: comparison of chemical extraction and modelling methods. J. Anim. Ecol. 77, 838–846. doi: 10.1111/j.1365-2656.2008.01394.x
Lutz, M. L., Davis, A. R., and Minchinton, T. E. (2010). Non-indigenous macroalga hosts different epiphytic assemblages to conspecific natives in Southeast Australia. Mar. Biol. 157, 1095–1103. doi: 10.1007/s00227-010-1391-y
Lutz, M. L., Minchinton, T. E., and Davis, A. R. (2019). Differences in architecture between native and non-indigenous macroalgae influence associations with epifauna. J. Exp. Mar. Biol. Ecol. 514–515, 76–86. doi: 10.1016/j.jembe.2019.03.006
Lysy, M., Stasko, A. D., and Swanson, H. K. (2014). nicheROVER: (Niche) (R)egion and Niche(Over)lap Metrics for Multidimensional Ecological Niches. R package Version 1.1.0. Available online at: https://CRAN.R-project.org/package=nicheROVER (accessed November 10, 2020).
Malavasi, S., Franco, A., Riccato, F., Valerio, C., Torricelli, P., and Franzoi, P. (2007). Habitat selection and spatial segregation in three pipefish species. Estuar. Coast. Shelf Sci. 5, 143–150. doi: 10.1016/j.ecss.2007.02.022
Manning, C. G., Foster, S. J., and Vincent, A. C. J. (2019). A review of the diets and feeding behaviours of a family of biologically diverse marine fishes (Family Syngnathidae). Rev. Fish Biol. Fish 29, 197–221. doi: 10.1007/s11160-019-09549-z
Martin-Smith, K. M. (1993). Abundance of mobile epifauna: the role of habitat complexity and predation by fishes. J. Exp. Mar. Biol. Ecol. 174, 243–260. doi: 10.1016/0022-0981(93)90020-O
Masonjones, H. D., Rose, E., McRae, L. B., and Dizson, D. L. (2010). An examination of the population dynamics of syngnathid fishes within Tampa Bay, Florida, USA. Curr. Zool. 56, 118–133. doi: 10.1093/CZOOLO/56.1.118
Minagawa, M., and Wada, E. (1984). Stepwise enrichment of 15N along food chains: further evidence and the relation between δ15N and animal age. Geochim. Cosmochim. Acta 48, 1135–1140. doi: 10.1016/0016-7037(84)90204-7
Mineur, F., Arenas, F., Assis, J., Davies, A. J., Engelen, A. H., Fernandes, F., et al. (2015). European seaweeds under pressure: consequences for communities and ecosystem functioning. J. Sea Res. 98, 91–108. doi: 10.1016/j.seares.2014.11.004
Monteiro, N. M., Almada, V. C., Santos, A. M., and Vieira, M. N. (2001). The breeding ecology of the pipefish Nerophis lumbriciformis and its relation to latitude and water temperature. J. Mar. Biol. Assoc. U. K. 81, 1031–1033. doi: 10.1017/S002531540100501X
Moore, E. C., and Hovel, K. A. (2010). Relative influence of habitat complexity and proximity to patch edges on seagrass epifaunal communities. Oikos 119, 1299–1311. doi: 10.1111/j.1600-0706.2009.17909.x
Nenciu, M. I., Harcota, G. E., Totoiu, A., Bisinicu, E., Filimon, A., and Nita, V. N. (2018). Prey preference of the long-snouted seahorse (Hippocampus guttulatus Cuvier, 1829) at the Romanian Black Sea coast. Sci. Pap. Ser. D Anim. Sci. LXI, 348–355.
Nogueira, E., Pérez, F. F., and Ríos, A. F. (1997). Seasonal patterns and long-term trends in an estuarine upwelling ecosystem (Ría de Vigo, NW Spain). Estuar. Coast. Shelf Sci. 44, 285–300. doi: 10.1006/ecss.1996.0119
Oksanen, J., Guillaume Blanchet, F., Friendly, M., Kindt, R., Legendre, P., McGlinn, D., et al. (2020). vegan: Community Ecology Package. R package version 2.5-7. Available online at: https://CRAN.R-project.org/package=vegan (accessed March 8, 2021).
Oliveira, F., Erzini, K., and Gonçalves, J. M. S. (2007). Feeding habits of the deep-snouted pipefish Syngnathus typhle in a temperate coastal lagoon. Estuar. Coast. Shelf Sci. 72, 337–347. doi: 10.1016/j.ecss.2006.11.003
Orfanidis, S. (2007). Comments on the development of new macroalgal indices to assess water quality within the Mediterranean Sea. Mar. Pollut. Bull. 54, 626–627. doi: 10.1016/j.marpolbul.2007.01.004
Orfanidis, S., Panayotidis, P., and Stamatis, N. (2001). Ecological evaluation of transitional and coastal waters: a marine benthic macrophytes-based model. Mediterr. Mar. Sci. 2, 45–65. doi: 10.12681/mms.266
Orth, R. J., Heck, K. L., and van Montfrans, J. (1984). Faunal communities in seagrass beds: a review of the influence of plant structure and prey characteristics on predator-prey relationships. Estuaries 7, 339–350. doi: 10.2307/1351618
Otero-Schmitt, J., and Pérez-Cirera, J. L. (1996). Epiphytism on Cystoseira (Fucales, Phaeophyta) from the Atlantic Coast of Northwest Spain. Botanica Marina 39, 445–465. doi: 10.1515/botm.1996.39.1-6.445
Peterson, B. J., and Fry, B. (1987). Stable isotopes in ecosystem studies. Annu. Rev. Ecol. Evol. Syst. 18, 293–320. doi: 10.1146/annurev.es.18.110187.001453
Phillips, D. L., and Gregg, J. W. (2003). Source partitioning using stable isotopes: coping with too many sources. Oecologia 136, 261–269. doi: 10.1007/s00442-003-1218-3
Piñeiro-Corbeira, C., Barreiro, R., and Cremades, J. (2016). Decadal changes in the distribution of common intertidal seaweeds in Galicia (NW Iberia). Mar. Environ. Res. 113, 106–115. doi: 10.1016/j.marenvres.2015.11.012
Piñeiro-Corbeira, C., Barreiro, R., Olmedo, M., and De la Cruz-Modino, R. (2020). Recreational snorkeling activities to enhance seascape enjoyment and environmental education in the Islas Atlánticas de Galicia National Park (Spain). J. Environ. Manage. 272, 111065. doi: 10.1016/j.jenvman.2020.111065
Planas, M., Chamorro, A., Paltrinieri, A., Campos, S., Nedelec, K., and Hernández-Urcera, J. (2020a). Effect of diet on breeders and inheritance in Syngnathids: application of isotopic experimentally derived data to field studies. Mar. Ecol. Prog. Ser. 650, 107–123. doi: 10.3354/meps13315
Planas, M., Paltrinieri, A., Dias-Carneiro, M. D., and Hernández-Urcera, J. (2020b). Effects of tissue preservation on carbon and nitrogen stable isotope signatures in Syngnathid fishes and prey. Animals 2020, 2301. doi: 10.3390/ani10122301
Planas, M., Piñeiro-Corbeira, C., Bouza, C., Castejón-Silvo, I., Vera, M., Regueira, M., et al. (2021a). A multidisciplinary approach to identify priority areas for the monitoring of a vulnerable family of fishes in Spanish Marine National Parks. BMC Ecol. Evol. 21:4. doi: 10.1186/s12862-020-01743-z
Planas, M., Piñeiro-Corbeira, C., Bouza, C., et al. (2021b). “Signátidos de los parques nacionales de las islas atlánticas y del archipiélago de cabrera,” in Proyectos de Investigación en Parques Nacionales: 2015-2018, ed. J. Amengual (Madrid: Organismo Autónomo de Parques Nacionales, Ministerio de Agricultura, Alimentación y Medio Ambiente).
Png-González, L., Vázquez-Luis, M., and Tuya, F. (2014). Comparison of epifaunal assemblages between Cymodocea nodosa and Caulerpa prolifera meadows in Gran Canaria (eastern Atlantic). J. Mar. Biol. Assoc. U. K. 94, 241–253. doi: 10.1017/S0025315413001513
Polte, P., and Buschbaum, C. (2008). Native pipefish Entelurus aequoreus are promoted by the introduced seaweed Sargassum muticum in the northern Wadden Sea, North Sea. Aquat. Biol. 3, 11–18. doi: 10.3354/AB00071
Poore, A. G. B., and Steinberg, P. D. (1999). Preference-performance relationships and effects of host plant choice in an herbivorous marine amphipod. Ecol. Monogr. 69, 443–464.
Post, D. M. (2002). Using stable isotopes to estimate trophic position: models, methods, and assumptions. Ecology 83, 703–718.
Post, D. M., Layman, C. A., Arrington, D. A., Takimoto, G., Quatrocchi, J., and Montaña, C. G. (2007). Getting to the fat of the matter: models, methods and assumptions for dealing with lipids in stable isotope analyses. Oecologia 152, 179–189. doi: 10.1007/s00442-006-0630-x
Prego, R., and Fraga, F. (1992). A simple model to calculate the residual flows in a Spanish Ria. Hydrographic consequences in the Ría of Vigo. Estuar. Coast. Shelf Sci. 34, 603–615. doi: 10.1016/S0272-7714(05)80065-4
Puertos del Estado (2017). Available online at: http://www.puertos.es/es-es/oceanografia/Paginas/portus.aspx (accessed December 30, 2019)
Quezada-Romegialli, C., Jackson, A. L., Hayden, B., Kahilainen, K. K., Lopes, C., and Harrod, C. (2018). tRophicPosition, an R package for the Bayesian estimation of trophic position from consumer stable isotope ratios. Methods Ecol. Evol. 9:1592–1599. doi: 10.1111/2041-210X.13009
Ripley, J. L., and Foran, C. M. (2007). Influence of estuarine hypoxia on feeding and sound production by two sympatric pipefish species (Syngnathidae). Mar. Environ. Res. 63, 350–367. doi: 10.1016/j.marenvres.2006.10.003
Roberts, M. (1967). Studies on marine algae of the British Isles. 4. Cystoseira baccata (Gmelin) silva. Br. Phycol. Bull. 3, 367–378. doi: 10.1080/00071616700650251
Roberts, D. A., Poore, A. G. B., and Johnston, E. L. (2006). Ecological consequences of copper contamination in macroalgae: effects on epifauna and associated herbivores. Environ. Toxicol. Chem. 25, 2470–2479. doi: 10.1897/05-661R.1
Roelke, D. L., and Sogard, S. M. (1993). Gender-based differences in habitat selection and activity level in the northern pipefish (Syngnathus fuscus). Copeia 1993, 528–532. doi: 10.2307/1447155
Roos, G., Van Wassenbergh, S., Herrel, A., and Aerts, P. (2009). Kinematics of suction feeding in the seahorse Hippocampus reidi. J. Exp. Biol. 212, 3490–3498. doi: 10.1242/jeb.033050
Russo, A. R. (1997). Epifauna living on sublittoral seaweeds around Cyprus. Hydrobiologia 344, 169–179. doi: 10.1023/A:1002970714963
Sánchez-Jerez, P., Barberá-Cebrián, C., and Ramos-Esplá, A. (1999). Daily vertical migrations in the epifauna associated with Posidonia oceanica meadows. J. Mar. Biol. Assoc. U. K. 79, 971–977. doi: 10.1017/S0025315499001204
Sanderson, B. L., Tran, D. D., Coe, H. J., Pelekis, V., Steel, E. A., and Reichert, W. L. (2009). Nonlethal sampling of fish caudal fins yields valuable stable isotope data for threatened and endangered fishes. Trans. Am. Fish. Soc. 138, 1166–1177. doi: 10.1577/T08-086.1
Scapin, L., Cavraro, F., Malavasi, S., Riccato, F., Zucchetta, M., and Franzoi, P. (2018). Linking pipefishes and seahorses to seagrass meadows in the Venice lagoon: Implications for conservation. Aquat. Conserv. Mar. Freshw. Ecosyst. 28, 282–295. doi: 10.1002/aqc.2860
Schmidt, A. L., and Scheibling, R. E. (2007). Effects of native and invasive macroalgal canopies on composition and abundance of mobile benthic macrofauna and turf-forming algae. J. Exp. Mar. Biol. Ecol. 341, 110–130. doi: 10.1016/j.jembe.2006.10.003
Seed, R., Elliott, M. N., Boaden, P. J. S., and O’Connor, R. J. (1981). The composition and seasonal changes amongst the epifaunal associted with Fucus serratus L. in Strangford Lough, Northern Ireland. Cah. Biol. Mar. 22, 243–266.
Silva, P. C. (1955). The dichotomous species of Codium in Britain. J. Mar. Biol. Assoc. U. K. 34, 565–577. doi: 10.1017/S0025315400008821
Smith, J. A., Mazumder, D., Suthers, I. M., and Taylor, M. D. (2013). To fit or not to fit: evaluating stable isotope mixing models using simulated mixing polygons. Methods Ecol. Evol. 4, 612–618. doi: 10.1111/2041-210X.12048
Steffe, A. S., Westoby, M., and Bell, J. D. (1989). Habitat selection and diet in two species of pipefish from seagrass: sex differences. Mar. Ecol. Prog. Ser. 55, 23–30. doi: 10.3354/meps055023
Stock, B., and Semmens, B. (2016). MixSIAR GUI User Manual. 2016; Version 3. Available online at: https://github.com/brianstock/MixSIAR (accessed April 5, 2017). doi: 10.5281/zenodo.1209993
Stock, B. C., Jackson, A. L., Ward, E. J., Parnell, A. C., Phillips, D. L., and Semmens, B. X. (2018). Analyzing mixing systems using a new generation of Bayesian tracer mixing models. PeerJ 6:e5096. doi: 10.7717/peerj.5096
Swanson, H. K., Lysy, M., Power, M., Stasko, A. D., Johnson, J. D., and Reist, J. D. (2015). A new probabilistic method for quantifying n-dimensional ecological niches and niche overlap. Ecology 96, 318–324. doi: 10.1890/14-0235.1
Taylor, R. B., and Cole, R. G. (1994). Mobile epifauna on subtidal brown seaweeds in northeastern New Zealand. Mar. Ecol. Prog. Ser. 115, 271–282. doi: 10.3354/MEPS115271
Tipton, K., and Bell, S. S. (1988). Foraging patterns of two syngnathid fishes: importance of harpacticoid copepods. Mar. Ecol. Prog. Ser. 47, 31–43. doi: 10.3354/meps047031
Torres, A. C., Veiga, P., Rubal, M., and Sousa-Pinto, I. (2015). The role of annual macroalgal morphology in driving its epifaunal assemblages. J. Exp. Mar. Biol. Ecol. 464, 96–106. doi: 10.1016/j.jembe.2014.12.016
Tuya, F., Martín, J. A., and Luque, A. (2006). Seasonal cycle of a Cymodocea nodosa seagrass meadow and of the associated ichthyofauna at Playa Dorada (Lanzarote, Canary Islands, eastern Atlantic). Cienc. Mar. 32, 695–704. doi: 10.7773/cm.v32i4.1158
Vafeiadou, A. M., Adão, H., De Troch, M., and Moens, T. (2013). Sample acidification effects on carbon and nitrogen stable isotope ratios of macrofauna from a Zostera noltii bed. Mar. Freshw. Res. 64, 741–745. doi: 10.1071/mf12169
Valladares, S., and Planas, M. (2012). Non-lethal dorsal fin sampling for stable isotope analysis in seahorses. Aquat. Ecol. 46, 363–370. doi: 10.1007/s10452-012-9407-y
Valladares, S., Soto, D. X., and Planas, M. (2017). Dietary composition of endangered seahorses determined by stable isotope analysis. Mar. Freshw. Res. 68, 831–839. doi: 10.1071/MF16013
Van Wassenbergh, S., Roos, G., and Ferry, L. (2011). An adaptive explanation for the horse-like shape of seahorses. Nat. Commun. 2, 164. doi: 10.1038/ncomms1168
Viejo, R. M. (1999). Mobile epifauna inhabiting the invasive Sargassum muticum and two local seaweeds in northern Spain. Aquat. Bot. 64, 131–149. doi: 10.1016/S0304-3770(99)00011-X
Villegas, M. J., Laudien, J., Sielfeld, W., and Arntz, W. E. (2008). Macrocystis integrifolia and Lessonia trabeculata (Laminariales; Phaeophyceae) kelp habitat structures and associated macrobenthic community off northern Chile. Helgol. Mar. Res. 62, 33–43. doi: 10.1007/s10152-007-0096-1
Vincent, A. C. J., Berglund, A., and Ahnesjö, I. (1995). Reproductive ecology of five pipefish species in one eelgrass meadow. Environ. Biol. Fishes 44, 347–361. doi: 10.1007/BF00008250
Vincent, A. C. J., Foster, S. J., and Koldewey, H. J. (2011). Conservation and management of seahorses and other Syngnathidae. J. Fish Biol. 78, 1681–1724. doi: 10.1111/j.1095-8649.2011.03003.x
Vizzini, S., and Mazzola, A. (2004). The trophic structure of the pipefish community (Pisces: Syngnathidae) from a western Mediterranean seagrass meadow based on stable isotope analysis. Estuaries 27, 325–333. doi: 10.1007/BF02803388
Wikström, S. A., and Kautsky, L. (2004). Invasion of a habitat-forming seaweed: Effects on associated biota. Biol. Invasions 6, 141–150. doi: 10.1023/B:BINV.0000022132.00398.14
Wolf, N., Carleton, S. A., and Martínez del Rio, C. (2009). Ten years of experimental animal isotopic ecology. Funct. Ecol. 23, 17–26. doi: 10.1111/J.1365-2435.2009.01529.X
Winkler, N. S., Pérez-Matus, A., Villena, A. A., and Thiel, M. (2017). Seasonal variation in epifaunal communities associated with giant kelp (Macrocystis pyrifera) at an upwelling-dominated site. Austral Ecol. 42, 132–144. doi: 10.1111/aec.12407
Woodall, L. C., Otero-Ferrer, F., Correia, M., Curtis, J. M. R., Garrick-Maidment, N., Shaw, P. W., et al. (2017). A synthesis of European seahorse taxonomy, population structure, and habitat use as a basis for assessment, monitoring and conservation. Mar. Biol. 165:19. doi: 10.1007/s00227-017-3274-y
Woods, C. M. C. (2002). Natural diet of the seahorse Hippocampus abdominalis. N. Z. J. Mar. Freshw. Res. 36, 655–660. doi: 10.1080/00288330.2002.9517121
WoRMS Editorial Board (2020). World Register of Marine Species. Available online at: http://www.marinespecies.org. (accessed January 11, 2020).
Yip, M. Y., Lim, A. C. O., Chong, V. C., Lawson, J. M., and Foster, S. J. (2015). Food and feeding habits of the seahorses Hippocampus spinosissimus and Hippocampus trimaculatus (Malaysia). J. Mar. Biol. Assoc. U. K. 95, 1033–1040. doi: 10.1017/S0025315414001660
Keywords: epifauna, seaweed assemblages, trophic web, stable isotopes, syngnathids, national park, feeding regime
Citation: Piñeiro-Corbeira C, Iglesias L, Nogueira R, Campos S, Jiménez A, Regueira M, Barreiro R and Planas M (2021) Structure and Trophic Niches in Mobile Epifauna Assemblages Associated With Seaweeds and Habitats of Syngnathid Fishes in Cíes Archipelago (Atlantic Islands Marine National Park, North West Iberia). Front. Mar. Sci. 8:773367. doi: 10.3389/fmars.2021.773367
Received: 09 September 2021; Accepted: 06 October 2021;
Published: 11 November 2021.
Edited by:
Bernardo Antonio Perez Da Gama, Fluminense Federal University, BrazilReviewed by:
Jan Vanaverbeke, Royal Belgian Institute of Natural Sciences, BelgiumMaria Eggertsen, Swedish University of Agricultural Sciences, Sweden
Copyright © 2021 Piñeiro-Corbeira, Iglesias, Nogueira, Campos, Jiménez, Regueira, Barreiro and Planas. This is an open-access article distributed under the terms of the Creative Commons Attribution License (CC BY). The use, distribution or reproduction in other forums is permitted, provided the original author(s) and the copyright owner(s) are credited and that the original publication in this journal is cited, in accordance with accepted academic practice. No use, distribution or reproduction is permitted which does not comply with these terms.
*Correspondence: Miquel Planas, bXBsYW5hc0BpaW0uY3NpYy5lcw==