- 1Laboratory of Marine Zoology, Graduate School of Fisheries and Environmental Sciences, Nagasaki University, Nagasaki, Japan
- 2Center for Bioinformatics & Computational Biology, University of Delaware, Newark, DE, United States
Batoids comprise five of the seven most threatened families of sharks and rays. The East Asian endemic Naru eagle ray Aetobatus narutobiei is a large bodied ray whose estuarine habitat overlaps with an economically valuable bivalve fishery. In response to decreased bivalve yields, the government initiated a predator control program and as a result, Naru eagle rays have faced intense and targeted fishing pressure during the last two decades. The long-term impacts of the predator control program on the population of rays and bivalves and their balance in the ecosystem are unknown because the life history of the Naru eagle ray has not been characterized. To begin to fill these critical knowledge gaps, the reproductive life history of the Naru eagle was described. Females mature at a larger size than males and require nearly twice as many years to reach maturity (DW50, 952.0 mm vs. 764.2 mm; Age50, 6.0 years vs. 3.5 years). Both males and females reproduce annually and their reproductive cycles are synchronized and seasonal. Females have a single ovary and paired uteri, are viviparous, and reproduce via matrotrophic histotrophy. Mating occurs in August and September and gestation lasts approximately 12 months including a 9.5-month diapause that begins soon after mating and ends in June of the following year, leaving 2.5 months for embryos to complete development. Fecundity ranged from 1 to 7 embryos per brood (n = 158, mean ± SD = 3.36 ± 1.26) and was positively correlated with female disc width (linear regression; F = 105.73, d.f. = 151, P < 0.05). Naru eagle rays are vulnerable to overfishing because of their low fecundity, long reproductive cycle and long time to reach sexual maturity. Obligate embryonic diapause during overwintering and seasonal migrations is a survival strategy that benefits the adults and neonates. This research is a valuable resource to help guide science-based management, conservation and protection of the endemic Asian A. narutobiei and its nursery areas.
Introduction
Climate change and anthropogenic disturbances can have significant and deleterious effects on coastal ecosystems and regional biodiversity. Elasmobranchs are especially susceptible to environmental change (Crear et al., 2020; Nowicki et al., 2021) and increased pressure from fisheries (Camhi et al., 1998; Musick, 1999) due to their generally slow maturity, low fecundity and low population growth rates (Hoenig and Gruber, 1990; Camhi et al., 1998). According to the International Union for Conservation of Nature (IUCN) Red List of Threatened Species, 33.7% (387 of 1174) of sharks and rays are threatened with extinction (Dulvy et al., 2021). Additionally, the global abundance of oceanic sharks and rays has declined by 71% owing to increase fishing pressure in the past 50 years (Pacoureau et al., 2021). Among elasmobranchs, large-bodied and shallow-water species are at the greatest risk of extinction, and five out of the seven most threatened families are batoids (i.e., rays, skates, guitarfish, and sawfish) (Dulvy et al., 2014). Batoids are more threatened than sharks and chimeras (Dulvy et al., 2021) and they play an important role as mesopredators and energetic links in ecosystems, however, they have received comparatively little attention from researchers and policy makers compared to their more charismatic shark counterparts. As a result, establishing science-based management of batoids is stymied by the lack of comprehensive data and information on their reproductive biology, early life history and nursery area requirements (Martins et al., 2018). For endemic elasmobranchs with a narrow range, identification and preservation of critical habitat is an urgent conservation need.
The East Asian Naru eagle ray Aetobatus narutobiei is one of five species of pelagic eagle rays and are endemic to East Asia. Aetobatus narutobiei is very similar in appearance to the long-headed eagle ray A. flagellum, a tropical species distributed throughout the Indo-West Pacific, with which it was formerly considered conspecific (Yamada and Miya, 1989; McEachran and Seret, 1990; Yamaguchi et al., 2005; White et al., 2013; White and Moore, 2013). The Naru eagle ray range is narrow, and their main habitat is Ariake Bay where large aggregations of rays are observed from spring to autumn. The rays are not targeted commercially for consumption. Ariake Bay, located in the southwestern of Japan, is a unique ecosystem with a muddy substrate encompassing the largest estuary in Japan. Its estuarine and tidal flats serve as conduits for nutrients to pass into productive coastal waters. Ariake Bay also provides essential spawning and nursery grounds for many species of bivalves, teleost fishes, and elasmobranchs such as the red stingray Hemitrygon akajei (Furumitsu et al., 2019) and scalloped hammerhead shark Sphyrna lewini (Yamaguchi and Kume, 2011). Historically, the bay is referred to as “the sea of treasures,” with an extensive estuary, nicknamed the “uterus.” Ariake Bay is renowned for its highly productive bivalve fisheries, especially pen-shells Atrina spp. and short neck clams Ruditapes philippinarum.
By 1984, the bivalve fishery was experiencing significantly reduced yields compared to the previous decade (Kyushu Regional Agricultural Administration Office, 1964–2013) and by the 1990s, when global warming impacts first were observed in western coastal areas of Japan (Ogino et al., 2019), an increase in the population size of Naru eagle rays in Ariake Bay was reported (Kawahara et al., 2004; Kimura et al., 2018). Warmer waters may have extended the time Naru eagle rays stay within Ariake bay and/or increased overwintering survivorship. Despite the timeline, with bivalve yields declining before Naru eagle ray populations increased, because the Naru eagle ray diet is dominated by mollusks (Yamaguchi et al., 2005), rays were assumed to be competing with the bivalve fishery causing significant reductions in yield. The national and local government responded to the decline in bivalve fishery with a government-supported and sanctioned predator control program with the goal of reducing Naru eagle ray populations as a mechanism to restore bivalve yields. As a result, the rays in Ariake Bay have been under intense fishing pressure for the last two decades. Since 2001, systematic surveys of rays culled as part of the predator control program in Japan were performed to describe the age, growth, feeding habits, and seasonal migration patterns of the Naru eagle ray (Yamaguchi et al., 2005). The largest catches of Naru eagle rays from the predator control program, including pregnant females and neonates, were from Ariake Bay, suggesting this area also might serve as a nursery ground for the species (Yamaguchi et al., 2005). Periodic assessment of bivalve and ray populations in Ariake Bay historically have not been completed and accordingly, the efficacy of the predator control program as a mechanism to recover bivalve yield is unknown. Despite the lack of data and understanding of ray predator–prey interactions that might affect bivalve population dynamics and ecosystem functions (Flowers et al., 2021), the government’s predator control program remains broadly adopted as an effective control measure.
Myliobatiformes is the largest order of rays with 218 species (Last et al., 2016). All Myliobatiformes rays are viviparous and reproduce via lipid histotrophy. Despite this commonality, myliobatid reproductive features vary widely, and comprehensive studies of their reproductive biology and embryonic development, especially for large pelagic species from the family Aetobatidae and Mobulidae are incomplete for all species. Basic anatomical information including the number of functional ovaries and uteri is lacking for many species even though it is essential to determine fecundity, a critical parameter for population management. Myliobatiform rays are a diverse group in terms of their morphology, habitat, and ecology; therefore, understanding their reproductive biology and embryonic development is of interest evolutionarily as well as from a biodiversity standpoint.
Select elasmobranchs include embryonic diapause, a temporary slowing or suspension of embryonic development that extends the time required to complete a reproductive cycle, as a life history trait. Diapause lasting from 4 to 10 months has been reported previously for 16 elasmobranch species (2 sharks and 14 rays), including A. narutobiei as summarized by Wyffels (2009) and Waltrick et al. (2012). In addition, an abbreviated diapause, less than 2 months in duration, is suspected for the red stingray (Furumitsu et al., 2019). Embryonic diapause in elasmobranchs has independently evolved among species with a range of reproductive strategies, suggesting that it may be regulated via several different mechanisms (Waltrick et al., 2012). Diapause is assumed to benefit neonates thereby increasing reproductive fitness of adults (Simpfendorfer, 1992; White et al., 2002), but this hypothesis has not been rigorously tested. It is essential to consider the reproductive biology and life-history parameters of a species holistically in order to understand the potential survival strategies and advantages afforded by embryonic diapause.
Recently, the Ministry of the Environment, Japan, categorized the Naru eagle ray as “Near Threatened (NT)” (Ministry of the Environment, 2017; Kimura et al., 2018). A subsequent assessment of Naru eagle ray by the IUCN Red List of Threatened Species listed Naru eagle rays as “Vulnerable,” a higher risk of extinction than what previously was assessed by the Ministry of the Environment, Japan (Rigby et al., 2021). To reduce the extinction risk of the Naru eagle ray and establish a science-based management policy for population conservation, it is essential and timely to fill biological and ecological knowledge gaps for this species. Understanding the eagle ray life history will guide future research and management measures that enable measures that reduce conflicts with bivalve fishery and guarantee the conservation of this threatened species.
The study aims to characterize the reproductive biology, including embryonic diapause and development of the Naru eagle ray in a great estuarine ecosystem and provide comment on the urgent need for conservation. Knowledge of the reproductive life history and strategies for survival for this pelagic ray will further our understanding of the reproductive systems of elasmobranchs in general, and the evolution of viviparous rays worldwide.
Materials and Methods
Study Location and Survey Techniques
Specimens examined (n = 1189) were collected by commercial vessels as part of the predator control program using gill nets (20 cm mesh size, 400 m length) at depths of 8–20 m in the northern part of Ariake Bay between August 2001 and November 2019 (Figure 1). Despite continuing efforts by fishers, few Naru eagle ray were caught during December and none were caught from January to March in the first few years of the predator control program (Yamaguchi et al., 2005). As a result, the predator control program operates annually from May to November.
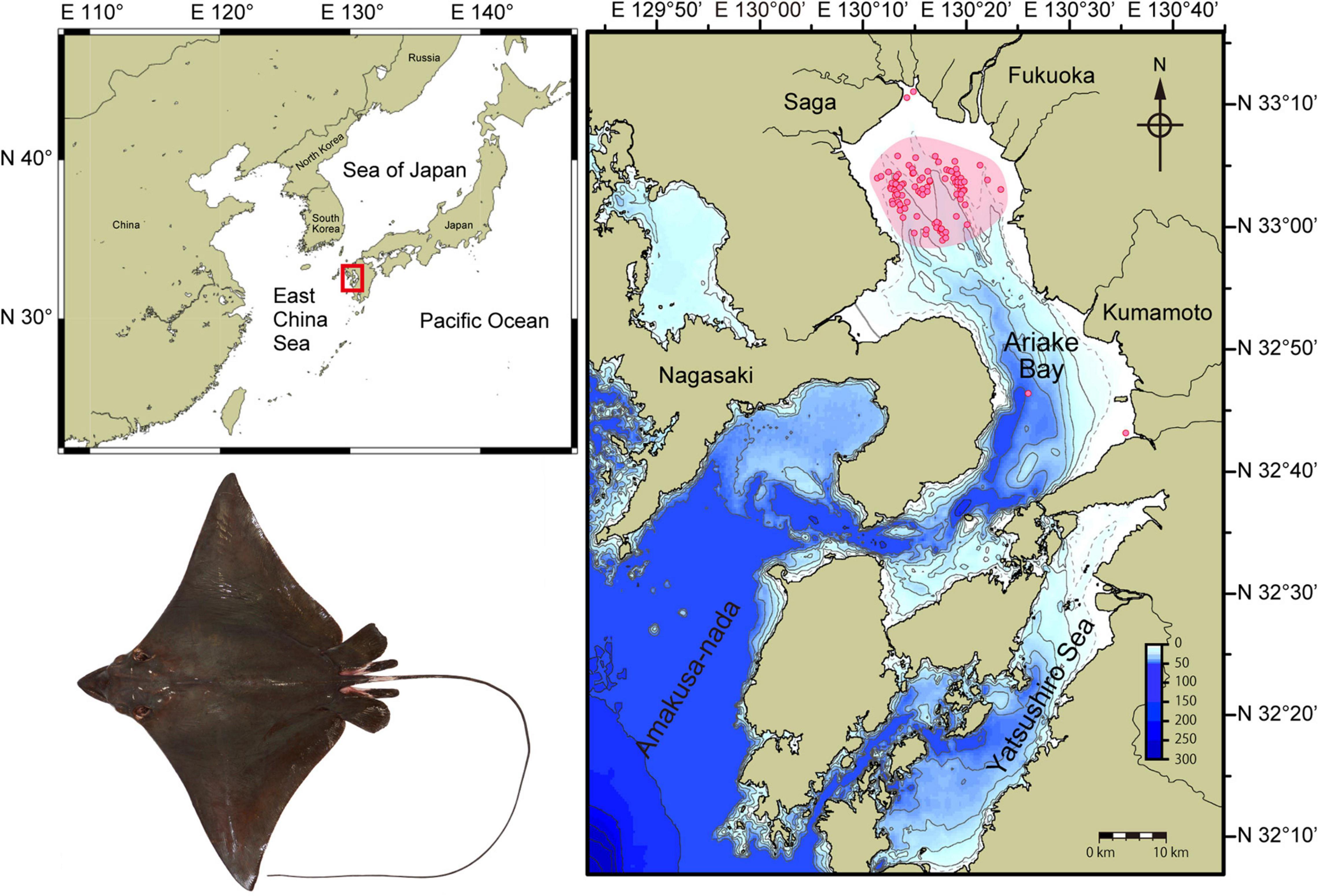
Figure 1. Ariake Bay (red rectangle) is on the western coastline of Japan and open to the East China Sea. Aetobatus narutobiei sampling areas within Ariake Bay are shown in detail (inset) with pink shading representing regions used by commercial vessels or as part of the predator control program and predominantly using gill nets. Red circles indicate specific sampling sites of vessels that contributed specimens for the study. Few rays were sampled from the river delta regions and rays were not sampled from deeper regions of the bay except during the migration seasons.
Morphometrics and Reproductive Data
Immediately after collection, disc width (DW, nearest 0.5 mm), body mass (BM, nearest 0.001 g for embryos or 1 g for field specimens), and sex were recorded for each Naru eagle ray. For females, uterus mass (UM, nearest 0.1 g) was measured. For males, inner clasper length (CL, nearest 0.01 mm), distance from the anteriormost limit of the cloaca to the tip of the clasper, was measured and the presence or absence of semen in the seminal vesicles was noted. Male maturity stages were determined based on the degree of development of the testes and claspers as described previously (Furumitsu et al., 2019): (1) immature—testes and claspers undeveloped; (2) premature or sub-adult—testes becoming lobular, vas deferens beginning to thicken and coil, clasper elongated and flexible, not calcified; and (3) mature—testes and seminal vesicles fully developed, vas deferens fully developed and coiled, claspers calcified. Female maturity stages were determined by the degree of development of the ovaries and uterus: (1) immature—ovary comprising white, undifferentiated tissues, uterus thin; (2) premature or sub-adult—ovary containing numerous immature follicles pale yellow or whitish in color and translucent, uterus thickening but without contents; and (3) mature—ovary containing opaque yellow vitellogenic follicles, uterus thick and may contain eggs or embryos (Furumitsu et al., 2019). Clasper length and UM relative to disc width were used as indicators of maturity (Yamaguchi and Kume, 2009). Analysis of covariance (ANCOVA) was used to test for differences between sexes in the log-transformed linear DW–BM relationship (KyPlot 6.0, Kyenslab, Tokyo, Japan) with a critical probability threshold of 0.05 for statistical significance.
Size and Age at Maturity and Natural Mortality Rate
The percentage of mature individuals in relation to immature and premature individuals for each 10 mm DW size group was calculated and fitted to the logistic model, Y = [1 + e(aX + b)]–1, where Y is the proportion of individuals mature at size X, and a and b are empirical parameters derived using the statistical software KyPlot 5.0 (KyensLab Inc.) (White and Dharmadi, 2007; Kume et al., 2009). Age at sexual maturity was calculated from established length-at-age relationships for the Naru eagle ray (Yamaguchi et al., 2005). Size (L50) and age (A50) at 50% maturity were calculated using the equation L50 or A50 = −ba–1 (Kume et al., 2009). Using previously reported age composition data for this species (Yamaguchi et al., 2005) in combination with the age at maturity determined in this study, a natural mortality rates of adult rays was calculated by a catch-curve method (Ricker, 1975).
Male and Female Reproductive Status
Masses (nearest 0.1 g) of the liver and gonads (with associated epigonal organ) were collected for mature rays to assess reproductive status. The gonadosomatic index (IG) was calculated using the formula: IG = [gonad mass/(body mass − gonad mass)] × 100. The monthly IG for mature males was used to approximate the mating season. Livers were removed and weighed to the nearest 0.1 g and the hepatosomatic index (IH) was calculated using the formula: IH = [liver mass/(body mass − liver mass)] × 100.
Testes removed from mature males were fixed in Bouin’s solution for 48 h and transferred to 70% ethanol. They were subsequently dehydrated and embedded in paraffin wax, sectioned to a thickness of 4–5 μm, and stained with hematoxylin and eosin (HE). For the purpose of defining the developmental stages of spermatogenesis, differentiating sperm cells were classified as spermatogonia (SG), primary spermatocytes (SC1), secondary spermatocytes (SC2), spermatids (ST), immature spermatozoa (IS), and mature spermatozoa (MS), according to Conrath and Musick (2002) and Poulakis and Grier (2014). A representative cross-section was selected from the middle of the right or left lobe and the testis were classified into one of seven stages of spermatogenesis (I–VII) based on the most developed stage of the sperm observed (Maruska et al., 1996; Furumitsu et al., 2019). Monthly proportions of the testis stages were calculated with and without the degenerate zone (VII), to characterize the proportion of newly formed spermatocytes in testis that are predominately degenerating.
For each mature female, the diameter of the largest ovarian follicles was measured (nearest 0.01 mm) with an electronic caliper and averaged. Because the Naru eagle ray was susceptible to capture-related uterine discharge, specimens that were observed to abort one or more embryos were removed from fecundity and uterine egg and embryo analyses. For mature females, 20 trophonemata were randomly selected and their length measured (nearest 0.01 mm) with an electronic caliper and averaged. The relationship between trophonemata length and stage of embryo and trophonemata length and female DW were tested using linear regression. Uterine egg and embryo mass (nearest 0.001 g), embryo DW (nearest 0.01 mm), and embryo sex (when possible) were recorded. The number of male and female embryos for all gravid rays was subjected to an χ2 test to determine if the sex ratio differed from parity. The relationship between the female DW and average uterine egg mass and female DW and fecundity were tested using linear regression (KyPlot 6.0, Kyenslab, Tokyo, Japan) with a critical probability threshold of 0.05 for statistical significance. Size at birth was estimated from the largest embryos and the smallest free-swimming neonates. Embryos were classified into 11 developmental cohorts based on easily recognized morphological characteristics (Table 1).
Results
Size Distributions, Disc Width, Age at Sexual Maturity, and Natural Mortality Rate
Males averaged 686.6 ± 174.5 mm DW (range 298–1038 mm) and 5881.5 ± 3797.2 g (range 409.3–17350 g). Females averaged 834.2 ± 290.7 mm DW (range 297–1536 mm) and 13186.4 ± 11495.1 g (range 411.6–60000 g). The largest female was approximately 1.5 times the disc width and 3.5 times the mass of the largest male (Figure 2A). The relationship between DW and BM was significantly different (F = 25.983, d.f. = 1, P < 0.001) between males BM = (4 × 10–9) DW3.2112 (n = 540, r2 = 0.986) and females BM = (4 × 10–9) DW3.1936 (n = 636, r2 = 0.992). The overall sex ratio (n = 1189, F:M = 1:0.86) was significantly different from 1 (χ2 = 6.66, d.f. = 1, P < 0.05).
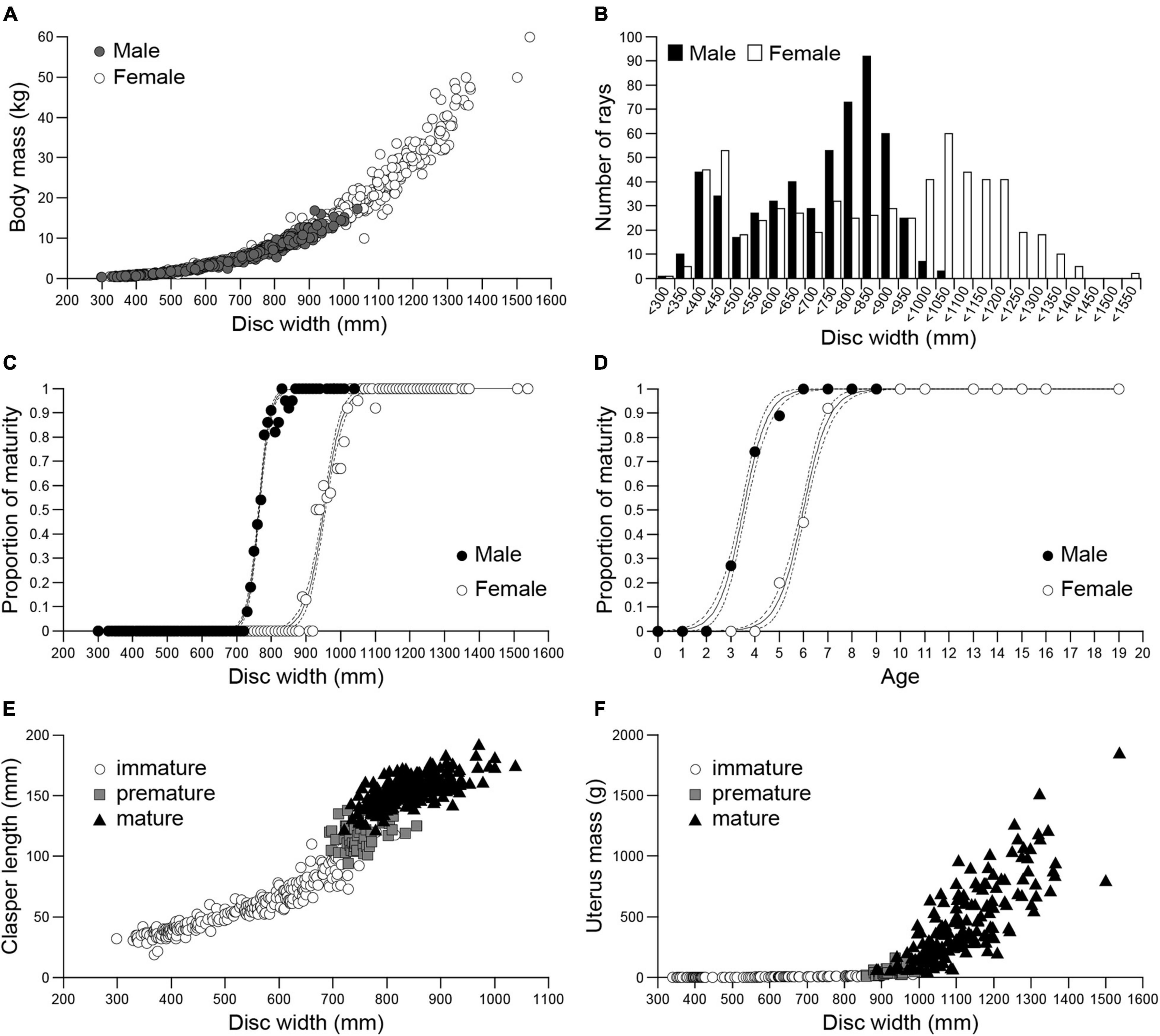
Figure 2. Size, sex, and maturity of Naru eagle rays Aetobatus narutobiei from Ariake Bay. (A) The relationship between the disc width (DW) and body mass (BM) for males (closed circle) and females (open circle). (B) Size class frequencies for males (black bar) and females (white bar). Size at maturity (C) and age at sexual maturity (D), for males (black circle) and females (white circle). Dashed lines represent 95% confidence intervals; The relationship between disc width and (E) clasper length for Aetobatus narutobiei males and (F) uterus mass for females.
The distributions of the DW frequencies for both sexes were bimodal (Figure 2B). The first mode represents immature specimens, 350–400 mm DW for males and 400–450 mm DW for females, and the second mode represents mature specimens, 800–850 mm DW for males and 1000–1050 mm DW for females (Figure 2B).
At sexual maturity female DW was greater than males (Figure 2C). All specimens with DWs larger than 855 mm for males and 1096 mm for females were sexually mature. The smallest sexually mature male was 721 mm DW and smallest sexually mature female was 885 mm DW. The size at 50% sexual maturity, or DW50 (±95% CI) was 764.2 (764.2–764.2) mm for males and 952.0 (951.9–952.0) mm for females (Figure 2C). Male specimens began maturing at age 2 and females began maturing at age 4 with first attained maturity at age 3 and 5, respectively. All specimens over age 6 for males and age 8 for females were mature. The age at 50% sexual maturity (±95% CI) was 3.5 (3.5–3.5) years for males and 6.0 (6.0–6.0) for females (Figure 2D). Clasper length increased abruptly when DW was 700 mm (premature males, n = 52), and clasper growth slowed when DW was ≥800 mm, concomitant with the onset of sexual maturity (Figure 2E). Uterus mass began to increase when DW was 850 mm, concomitant with the observations of premature females (n = 48), and continued to increase thereafter (Figure 2F). Natural mortality rate for mature females (more than 6 years old) is 0.23 and that for mature males (more than 4 years old) is 0.75.
Temporal Periodicity of IG and IH
Monthly IG for males (Figure 3A) showed clear seasonal variations. Male IG increased from May to June, reached its maximum in July, and decreased precipitously from August to September. In contrast, there were no seasonal trends in IG for females during the same timeframe (Figure 3B). Monthly IH showed a similar trend between the sexes, although there were different seasonal patterns for IH vs. IG for both sexes. The IH was lowest from April to July for males and April to August for females, and for both sexes increased thereafter until November (Figures 3C,D). In November, just before the overwintering period, male and female IH was approximately two-fold higher than the lowest values measured in early spring.
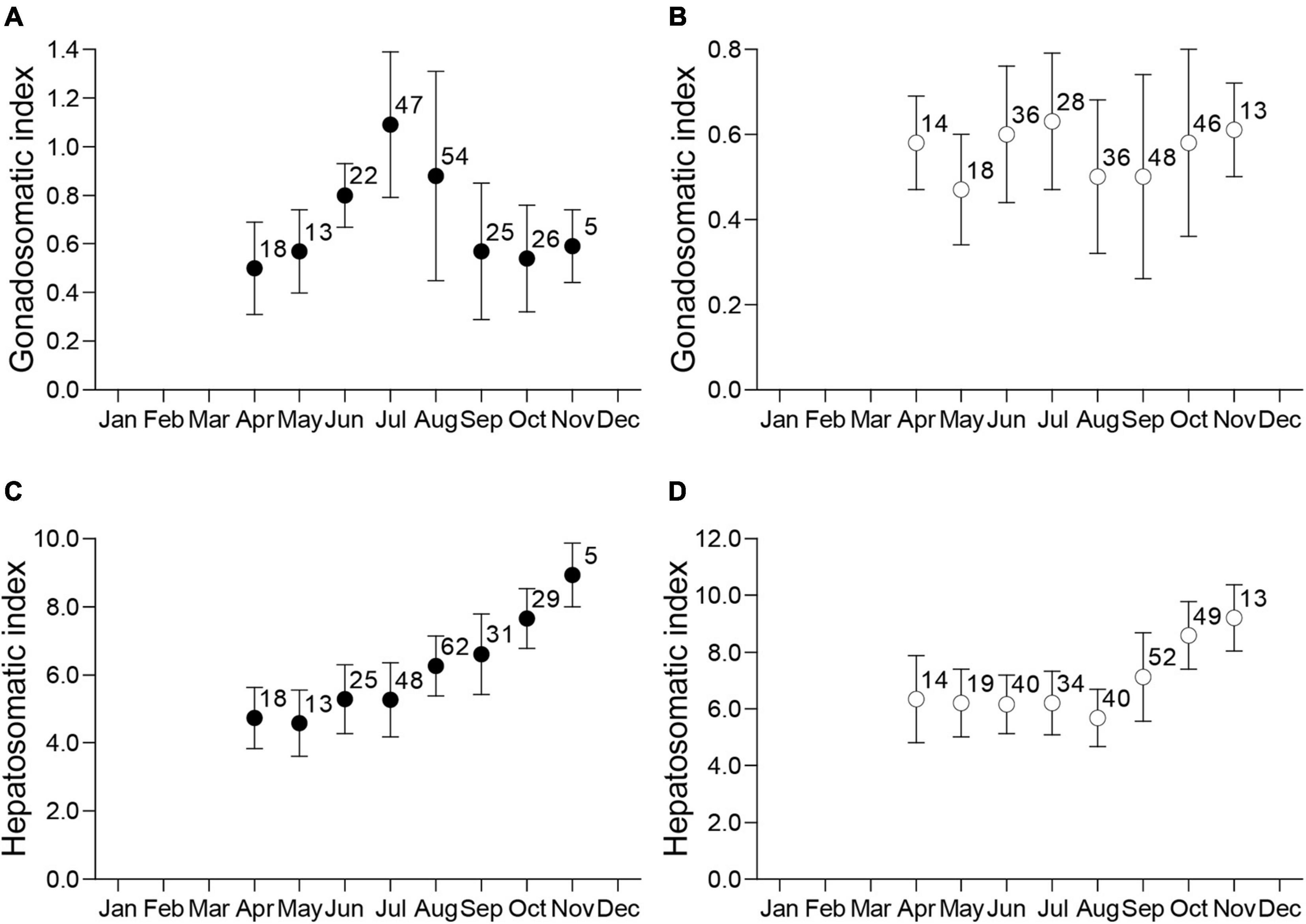
Figure 3. Monthly distributions of the mean ± standard deviation and sample size for the gonadosomatic index (IG) for mature males (A) and females (B), and hepatosomatic index (IH) for mature males (C) and females (D).
Testis Morphology, Spermatogenesis, and Temporal Periodicity
The testes of Naru eagle rays are paired, dorsoventrally flattened organs on the dorsal surface of the epigonal organ located in the anterior peritoneal cavity and suspended from the dorsal body wall by mesorchia (Figure 4A). Both testes are well developed and functional. The germinal zone of each testis lies in the central (middle) portion of each lobe, not visible externally (Figures 4B,C). Spermatogenesis proceeds radially from the central germinal zone, toward the outer margin of the lobe where spermatocysts connect to efferent ductules. Spermatocysts in the final stages of spermatogenesis are visible around the circumferential perimeter of each lobe (Figures 4B,C).
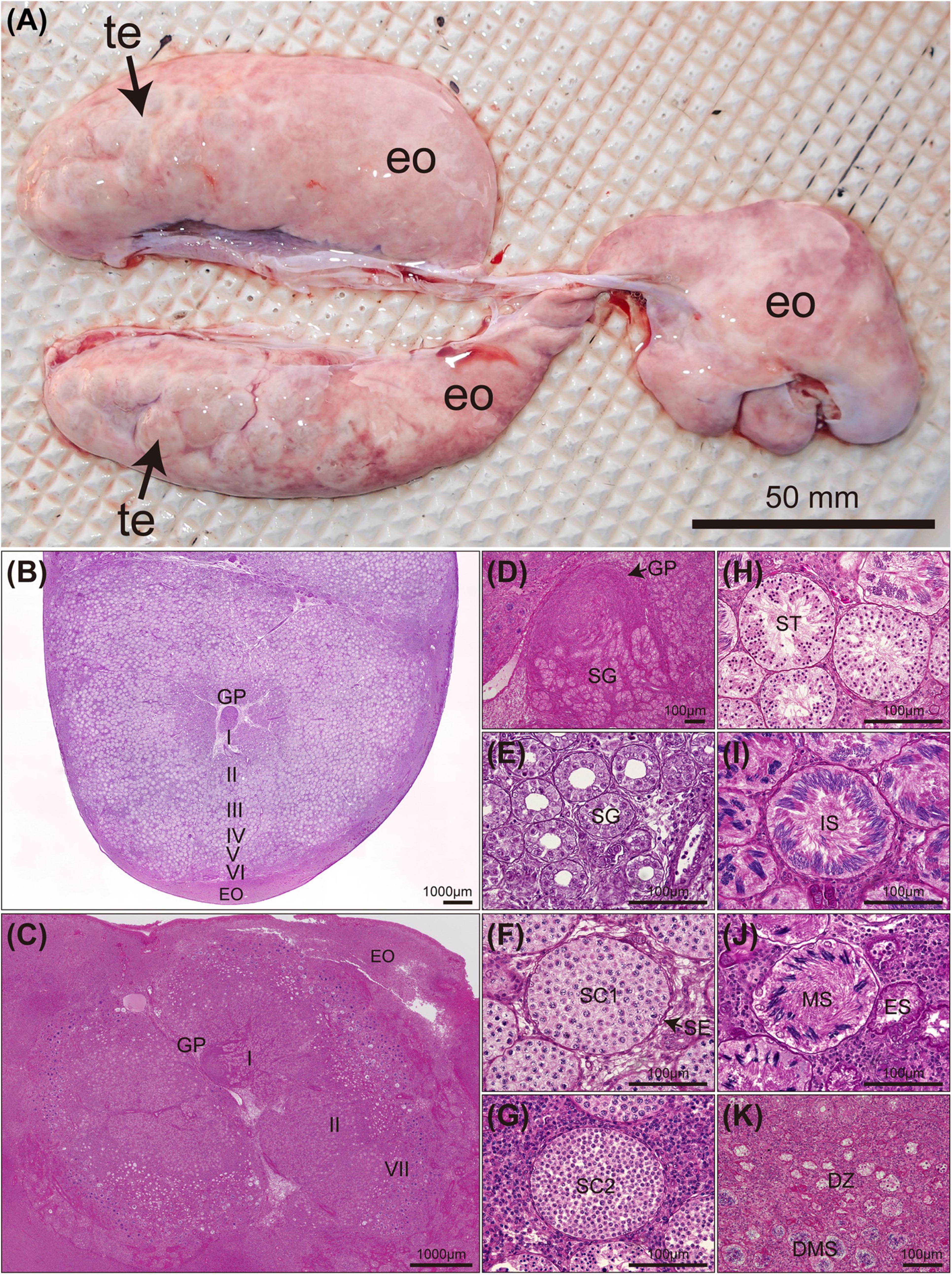
Figure 4. Mature Aetobatus narutobiei testes anatomy and stages of spermatogenesis (A) (te) testis lobe, (eo) epigonal organ and histology (B–K). (B) Cross section through a single testicular lobe with germinal papilla (GP) and epigonal organ (EO) (August). (C) Cross section through a single testicular lobe (October). (D) Stage I primary spermatogonia (SG). (E) Stage II early spermatocysts with spermatogonial cells (SG). (F) Stage III primary spermatocytes (SC1) with sertoli cells (SE). (G) Stage III secondary spermatocytes (SC2). (H) Stage IV spermatids (ST). (I) Stage V immature sperm (IS). (J) Stage VI mature sperm (MS) and an empty spermatocyst (ES). (K) Stage VII degenerate zone (DZ) and degenerate mature sperm (DMS).
Stage I (Figure 4D) was observed in all months. Stage II spermatocysts (Figure 4E) occurred in April–June, October, and November, peaking in April and May (100%), and reached stage III (Figures 4F,G) in June, July, and September to November (Figure 5B). Stage IV spermatocysts (Figure 4H) were observed in July and September, and stage V (Figure 4I) in July and August (Figure 5B). Stage VI spermatocysts (Figure 4J) were observed from August until October (Aug 96.6%, Sep 86.7%, Oct 8.3%). Stage VII spermatocysts (Figures 4J,K) were observed in April and September–November (Figure 5A). In October, approximately 67% of males had degenerate zones in their testes. Sperm production was confirmed by direct observation of semen in the seminal vesicles of rays from August through October (Aug 91.7%, Sep 100%, Oct 75.0%).
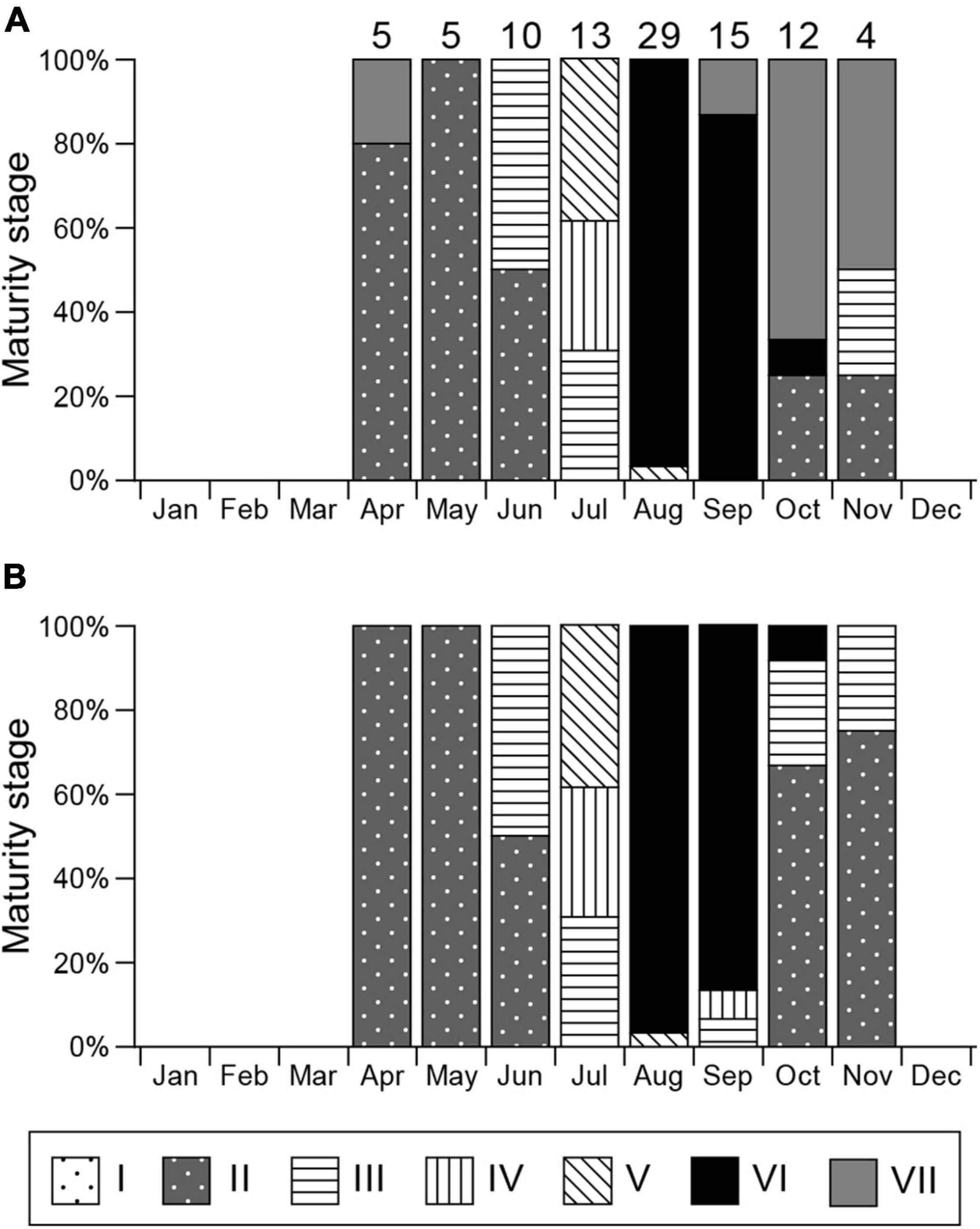
Figure 5. Monthly gonadal maturity stages for mature male Aetobatus narutobiei. (A) Considering the most mature stage and (B) most mature stage disregarding the degenerate zone. Sample size for each month is indicated above the bar.
Reproductive Cycle of Females
Similar to testes, the ovary of Naru eagle rays is observed on the dorsal surface of the epigonal organ in the anterior peritoneal cavity and is suspended from the dorsal body wall by mesorchia. Unlike the paired testes, only the left ovary was functional (Figures 6A,B). Maximum ovarian follicle diameter showed clear seasonal variations. Diameters were smallest during April and July, 10.11 ± 2.51 mm (range 5.40–18.50 mm), and abruptly increased in August, 25.20 ± 5.32 mm (range 13.50–32.00 mm) (Figure 6C). Pre-ovulatory ova were observed in females collected from August to September. The largest ovarian follicle diameter (32.20 mm) was observed in September. Mature females were post-ovulatory with encapsulated uterine eggs in early September with the exception of two females that cycled in August (7th and 30th). These observations indicate that ovulation and fertilization were synchronous and occurred in September.
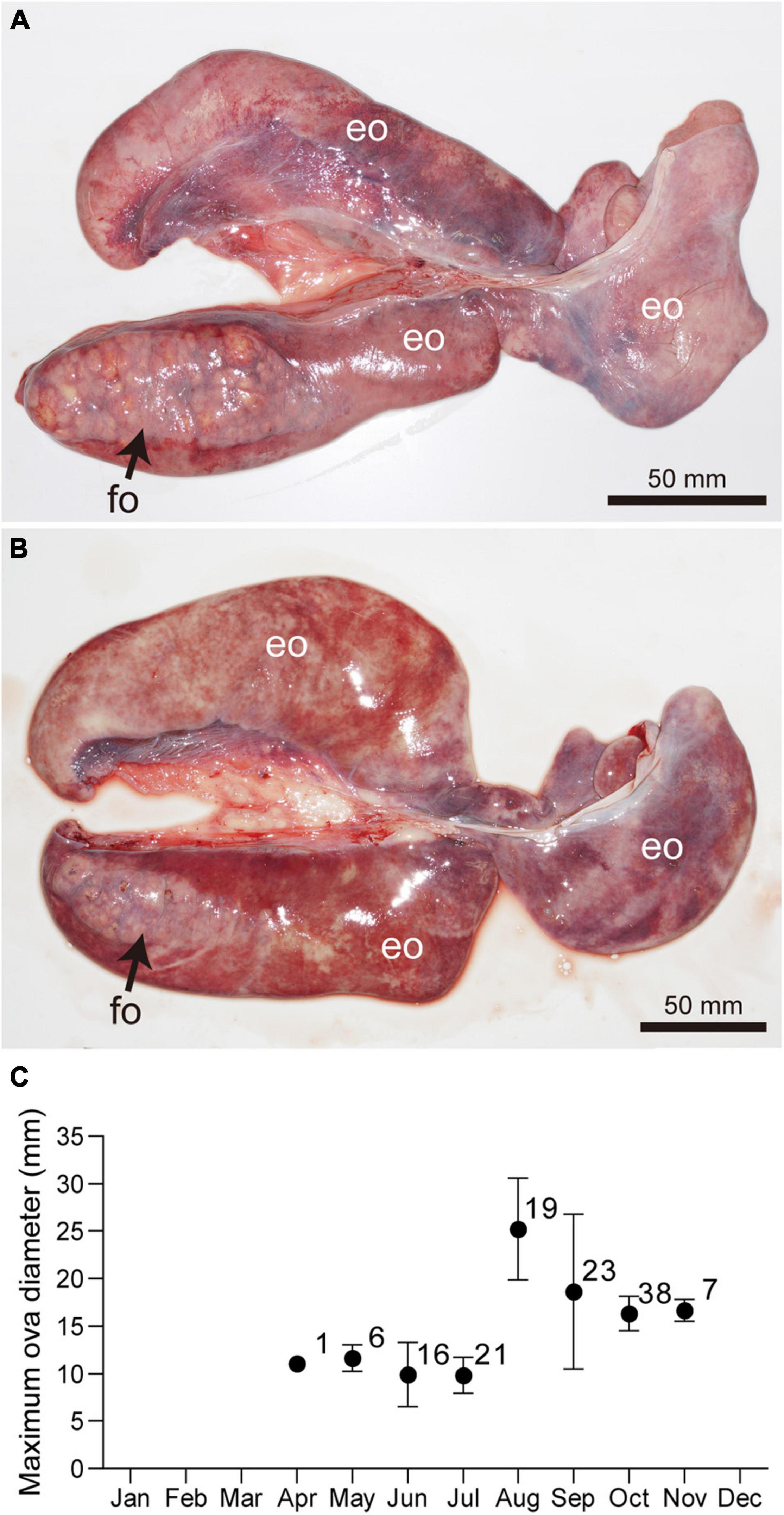
Figure 6. Ovaries of mature females Aetobatus narutobiei before (A) and after (B) ovulation. Only left ovary is functional. (C) Monthly ovarian follicle diameters (mean ± standard deviation and sample size) for mature female Aetobatus narutobiei. The peak of the maximum diameter of the ovarian follicles occurred in August, just prior to ovulation. (eo) epigonal organ, (fo) ovarian follicle.
Gestation and Embryonic Development
Aetobatus narutobiei had paired functional uteri and pregnant females had multiple eggs or embryos in both, apart from ten females that had a single egg or embryo in either the right or left uterus. The total number of eggs or embryos examined from the right (133 eggs and 73 embryos) and left (143 eggs and 61 embryos) uterus of pregnant females (n = 158) was not significantly different (right: left = 1.01:1, χ2 = 0.01, d.f. = 1, P > 0.05).
Uterine eggs were enclosed in a fragile, soft, smooth textured, light brown translucent tertiary egg envelope (Figure 7A). Mean uterine egg mass varied widely (3.145–8.019 g) among females with larger females producing eggs with greater mean mass (linear regression; F = 4.03, d.f. = 63, P < 0.05) (Figure 7B). There was no relationship between the number of uterine eggs per female and mean egg mass (linear regression; F = 1.07, d.f. = 63, P > 0.05) (Figure 7C).
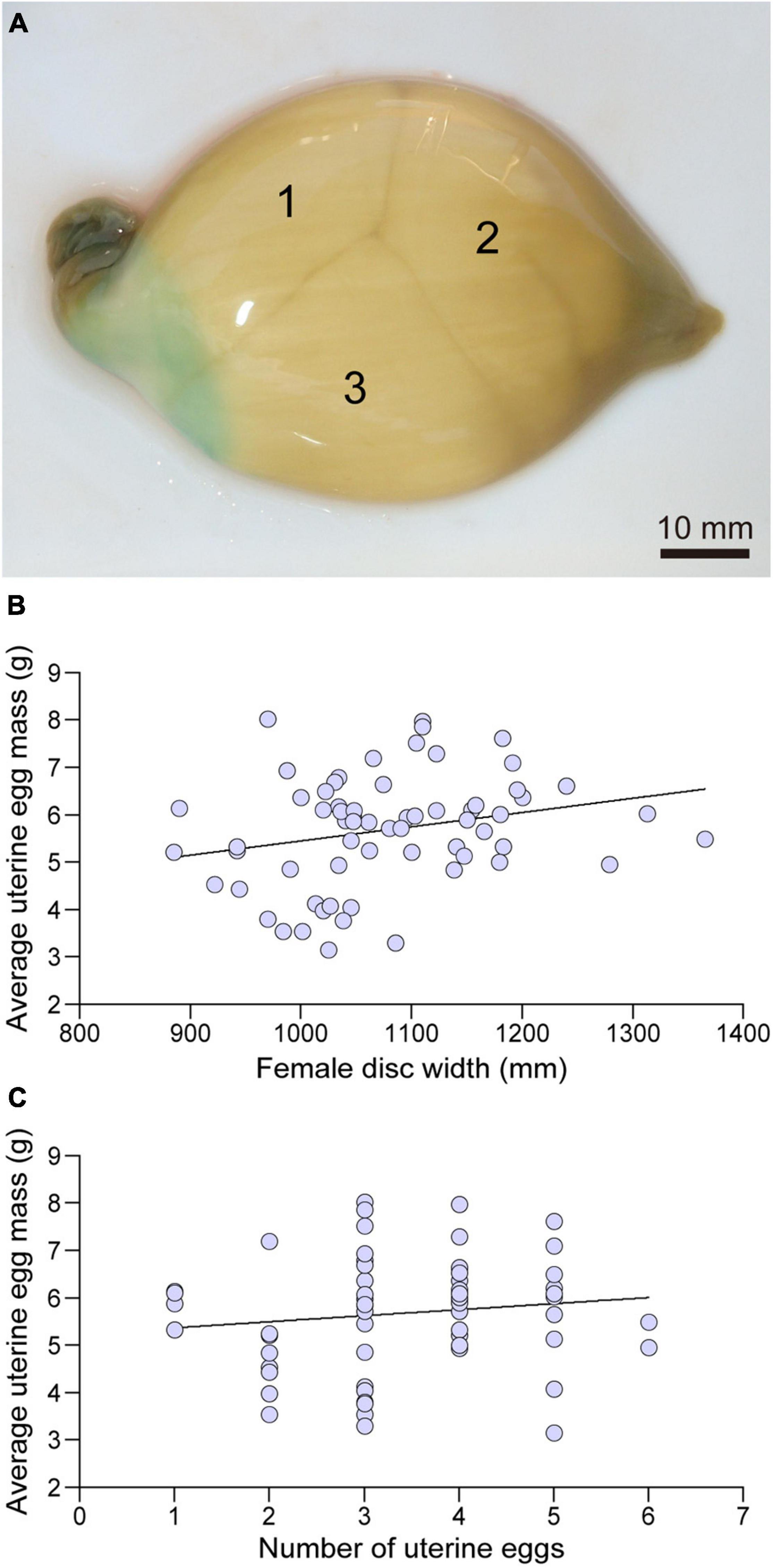
Figure 7. A uterine egg case collected in October containing three eggs (A), the relationship between the disc width (mm) and average uterine egg mass (g) (B), and the relationships between number of eggs in the egg case and average uterine egg mass (g) (C).
Uterine eggs without macroscopic embryos (stage 1, n = 86) were observed from April–June and September–November with the exception of two females with stage 1 embryos observed in August. No data is available from December to March because Naru eagle rays overwinter outside of the bay and specimen collection proved difficult (Figure 8). The ratio of pregnant females with stage 1 embryos was 100% in April and May, dropped to 18.2% in June and 0% in July before increasing to 8.7% in August, 80.6% in September and reaching 100% in October and November.
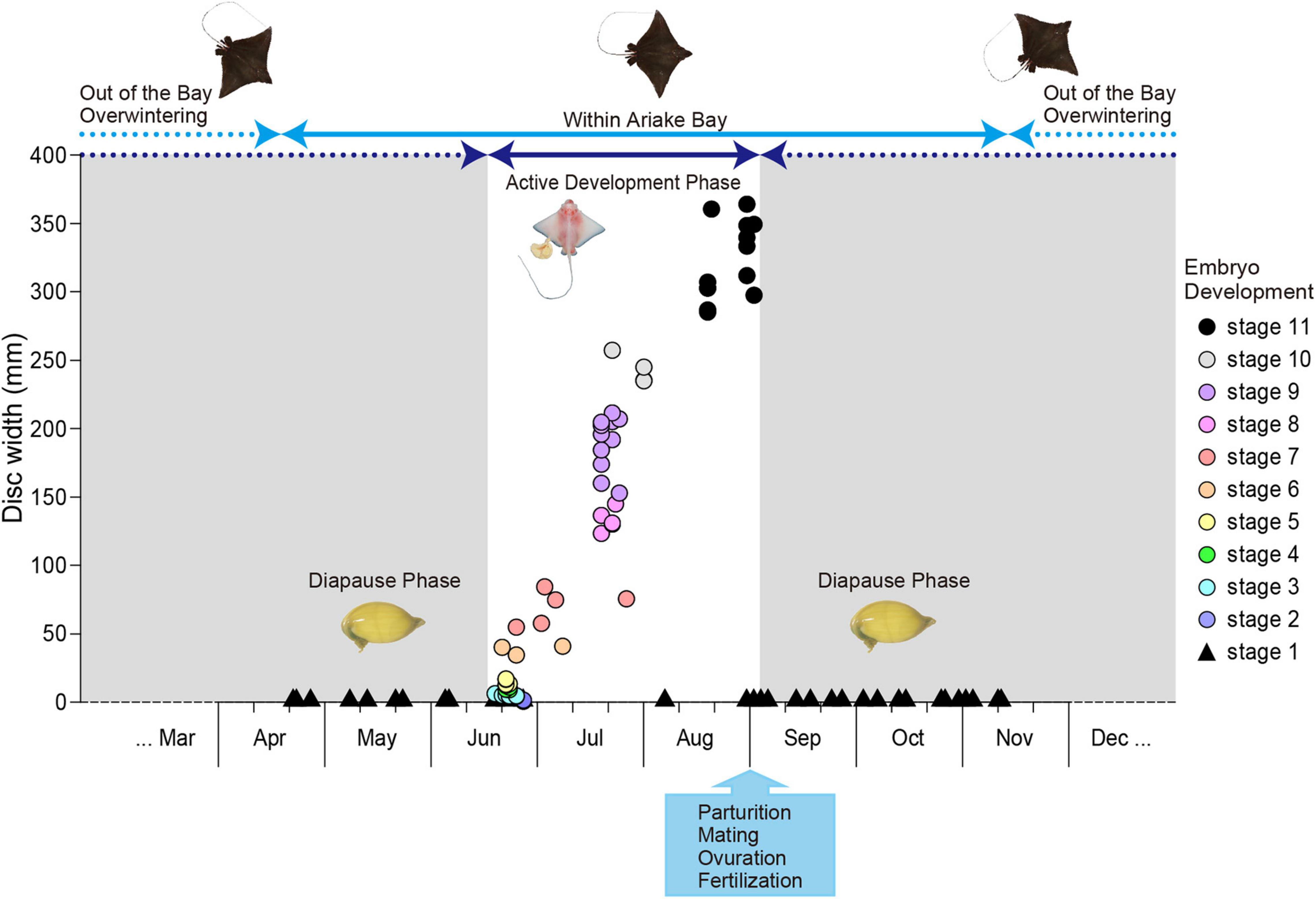
Figure 8. Aetobatus narutobiei embryonic diapause and growth. Embryonic disc width (circles) by month with circle color representing embryonic development stages 2–11 and triangles shown for uterine eggs without macroscopic embryos (stage 1).
Uterine eggs with macroscopic embryos were observed first in mid to late June and embryonic growth was rapid (Figure 8). Parturition occurred in late August with the exception of four pregnant females examined in the first 3 days of September, approximately 2.5 months after the smallest macroscopic embryos first were observed. All females examined after September 3 had encapsulated uterine eggs in stage 1 of development. Embryos (n = 224) were sexed when possible (n = 132). Embryos were assigned to one of 11 developmental cohorts (Table 1): Stage 1 (n = 86); Stage 2 (n = 2); Stage 3 (n = 7); Stage 4 (n = 4); Stage 5 (n = 5); Stage 6 (n = 3); Stage 7 (n = 4); Stage 8 (n = 6); Stage 9 (n = 11); Stage 10 (n = 4); and Stage 11 (n = 13), based on easily recognized external morphological characteristics (Figure 9). Embryo sex ratio (F:M 1:1.03) was not different from 1:1 (χ2 = 0.03, d.f. = 1, P > 0.05). At the onset of stage 2, embryonic development was rapid and embryos reached stage 10 after only 4–5 weeks (Figure 8). Easily observed morphological changes in body form were complete by stage 10 with large increases in embryo size during stages 10 and 11, the months preceding parturition (Figure 8). Monthly embryo DW (average ± SEM) increased from 14.25 ± 2.98 mm in June (n = 23) to 328.23 ± 24.22 mm in September (n = 4).
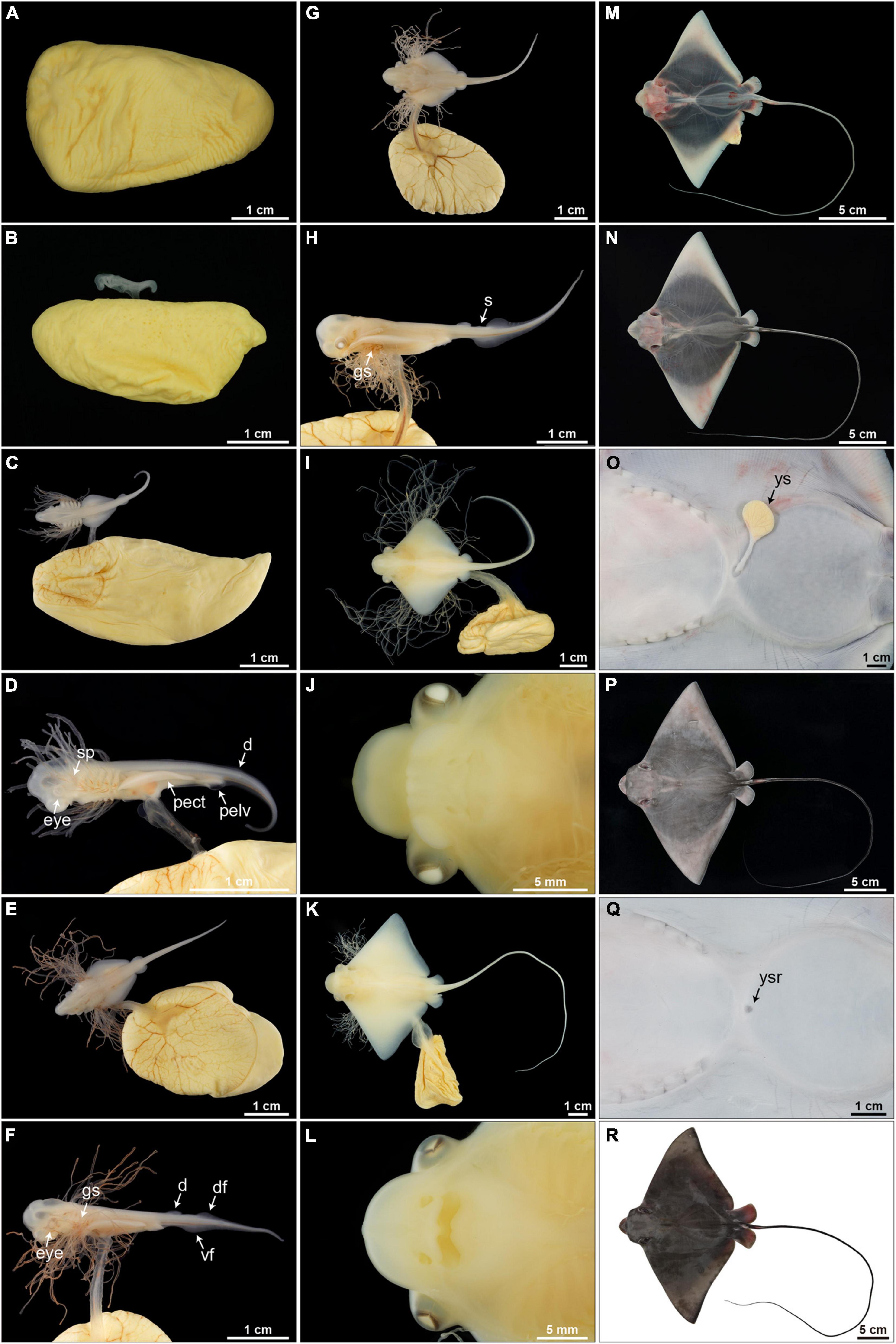
Figure 9. Embryonic development (stages 1–11) of Aetobatus narutobiei. (A) Stage 1: The egg is within an egg case and without a macroscopic embryo. (B) Stage 2: Macroscopic embryos on uterine eggs within an egg case (11.72 mm TL). (C,D) Stage 3: The pectoral fins within the posterior margins of the gills (6.56 mm DW). (E,F) Stage 4: The pectoral fins beyond the posterior margin of the gills but within the anterior margins of the gills (10.79 mm DW). (G,H) Stage 5: The pectoral fins beyond the gills but within the posterior margin of the eyes (16.10 mm DW). (I,J) Stage 6: The pectoral fins are fused forming the disc, the cephalic lobes are developing (36.09 mm DW). (K,L) Stage 7: The cephalic lobes are formed completely, the yolk sac and gill filaments persist (75.04 mm DW). (M) Stage 8: Gill filaments are resorbed but the yolk sac remains, muscle tissue in the center of the disc appears black but the disc margins remain translucent (132.80 mm DW). (N,O) Stage 9: The yolk sac is diminished and the body is free of pigmentation (116.35 mm DW). (P,Q) Stage 10: The yolk sac is resorbed and the dorsal surface is begins to turn gray–black (241.00 mm DW). (R) Stage 11: Body pigmentation is present (312.00 mm DW). d, dorsal fin; df, dorsal fold; gs, gill slit; pect, pectoral fin; pelv, pelvic fin; s, spine; vf, ventral fold; ys, external yolk sac; ysr, yolk sac remnant.
As embryo DW increased, mass of the external yolk sac decreased (Figure 10). External yolk sac mass ranged 2.47–5.73 g during embryonic stages 2–6, and was mostly absorbed (<0.015 g) by stage 10. Embryo body mass increased exponentially once embryos reached stage 5 and by stage 11 embryo mass was (average ± SEM) 624.4 ± 53.6 g. The heaviest embryo was 1089 g, representing an increase in wet weight of 12,874–34,626% compared to the lightest (3.145 g) and heaviest (8.459 g) egg mass measured from stage 1 eggs or 19,179% compared to the average egg mass (5.678 g) observed for females of similar DW.
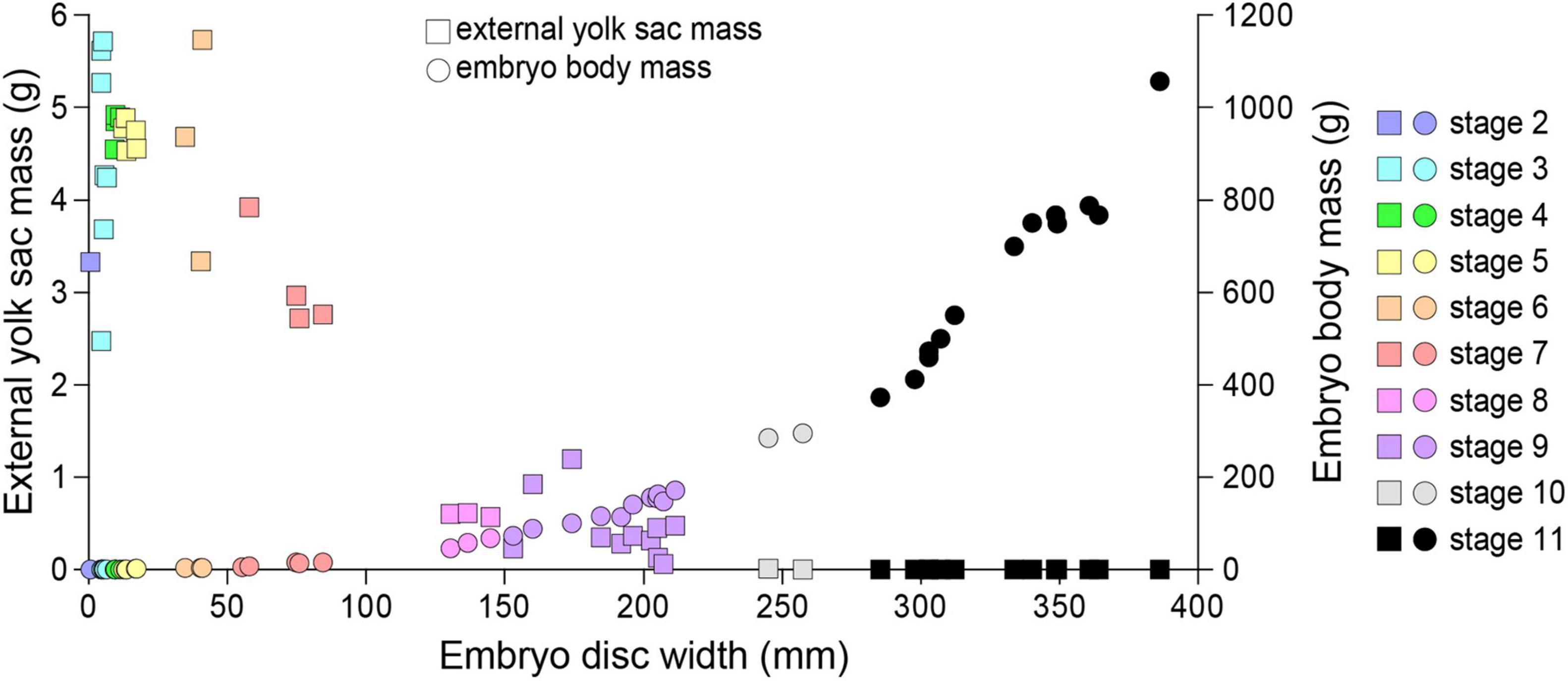
Figure 10. The relationship between the embryo disc width, embryo body mass and the external yolk sac mass for Aetobatus narutobiei.
Parturition occurred during late August through to early-September, and the DW at birth ranged from 300 to 400 mm. The earliest free-swimming specimen (329 mm DW and 540 g) was observed on August 22nd and the smallest free-swimming specimen (297 mm DW and 420 g) was observed on August 30th. Both the largest and the latest embryos in stage 11 (390 mm DW and 1089 g) were observed on September 2nd. Gestation was almost 1 year including a 9.5 months embryonic diapause phase and 2.5 months embryonic growth phase.
Trophonemata and Embryonic Growth
Embryo body mass increased rapidly after external yolk was consumed (Figure 10). In conjunction with yolk depletion, embryonic nutrition transitioned to histotroph. Trophonemata were conspicuous as villous and highly vascular projections of the uterine mucosa (Figure 11A). There was no relationship between the length of the trophonemata and the yolk mass or embryo DW during the embryonic growth phase (Figure 11B). However, there was an increasing trend for trophonemata length from stages 1 to 4 and a decreasing trend from stages 10–11 (Figure 11C). Trophonemata length increased with disc width of pregnant females (linear regression; F = 56.37, d.f. = 50, P < 0.05) (Figure 11D). Histotroph was not observed for females with stage 9 embryos (Figure 11E) but it was present in uteri of females with stage 11 embryos (Figure 11F). When or at what embryonic stage (10 or 11) histotroph is available to embryos was not determined.
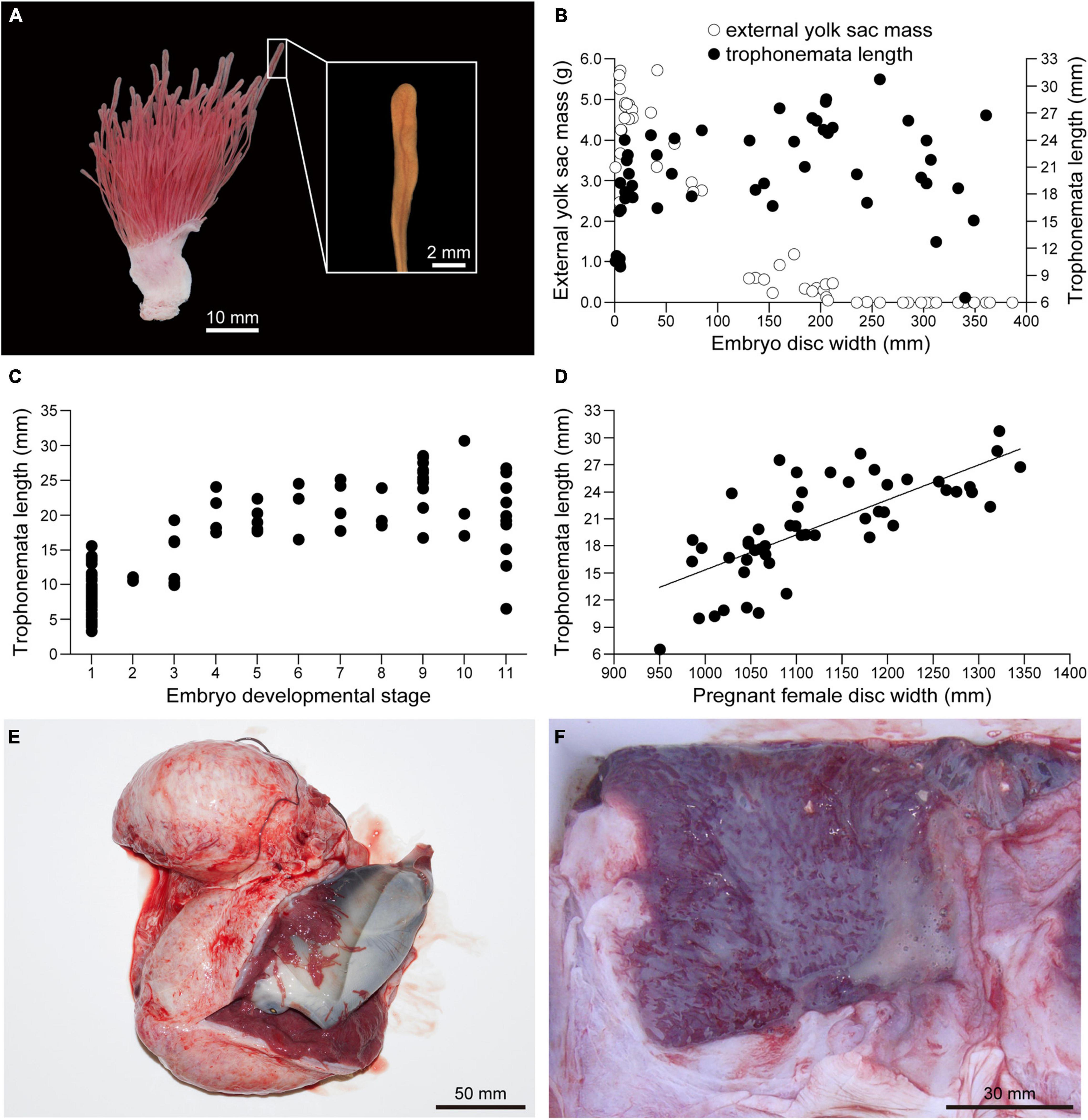
Figure 11. Embryonic yolk consumption and uterine trophonemata of Aetobatus narutobiei. (A) The uterine mucosa with trophonemata Gross appearance of the uterine wall and mucosa with trophonemata representative of a female with stage 11 embryos. (B) The relationship between embryo disc width, trophonemata length and the external yolk mass and (C) embryo development stage versus trophonemata length. (D) The relationship between the pregnant female disc width and trophonemata length. (E) Stage 9 embryo within the uterus. (F) Histotroph (pale yellow material) in the uterus of a female with late term, stage 11 embryos.
Fecundity
Fecundity for 158 pregnant females with uterine eggs (n = 86) and embryos (n = 72) ranged from 1 to 7 embryos per brood (n = 158, mean ± SD = 3.36 ± 1.26) and was positively correlated with female disc width (linear regression; F = 105.73, d.f. = 151, P < 0.05) (Figure 12).
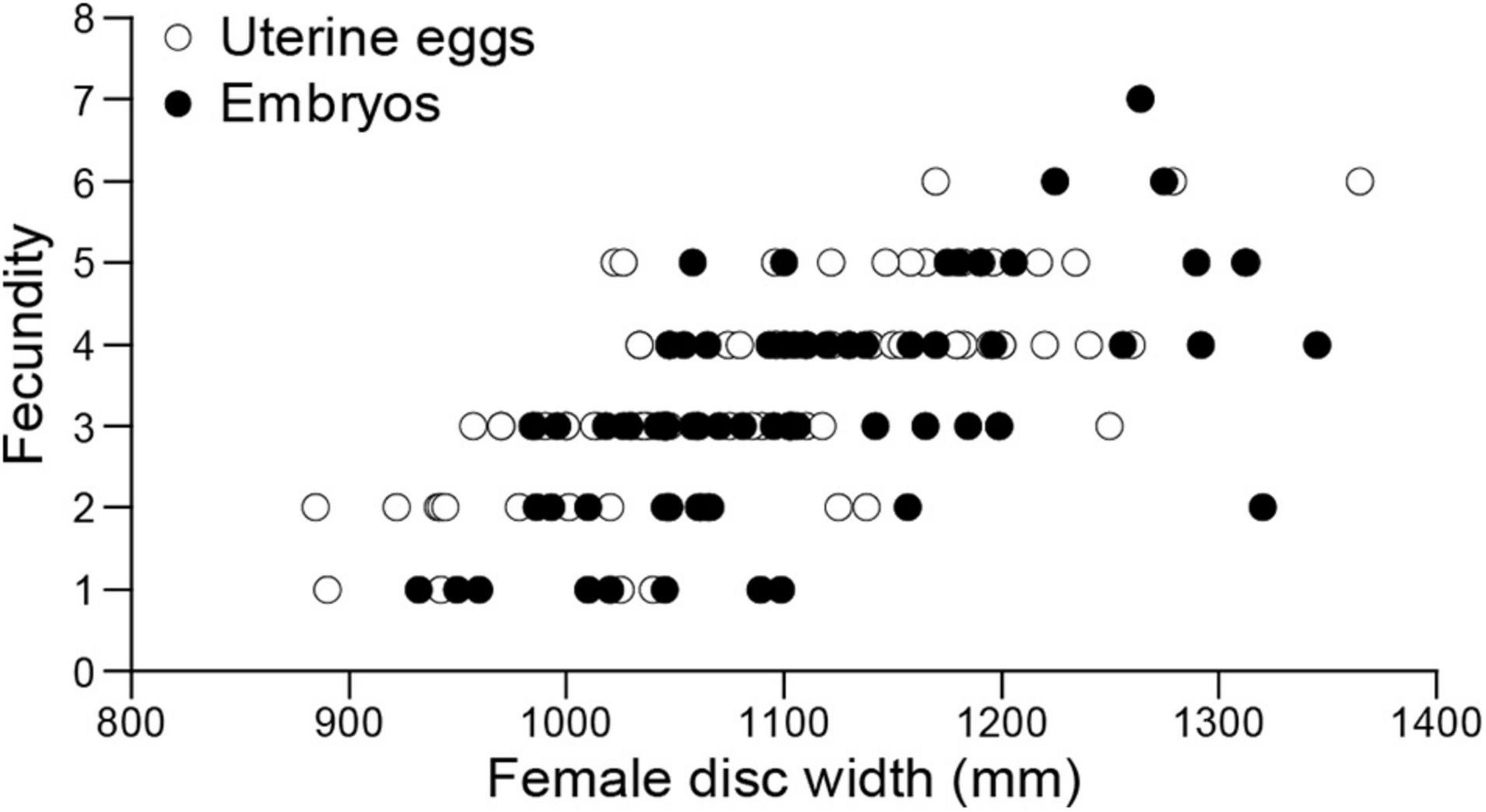
Figure 12. Aetobatus narutobiei disc width and fecundity for females (n = 158) from Ariake Bay. Fecundity ranged from 1 to 7 embryos per litter (n = 158, mean ± SD = 3.36 ± 1.26) and was positively correlated with female disc width (linear regression; F = 105.73, d.f. = 151, P < 0.05).
Fetal Mortality and Abnormal Embryo
Two dead embryos and four undeveloped uterine eggs were observed among 224 embryos from six of 72 females examined from late June to early September (Figures 13A–C). Assuming the undeveloped uterine eggs were fertilized, the fetal mortality rate for Naru eagle ray, in Ariake Bay was 2.68%. Females with fetal mortality (n = 6) had a combination of live embryos with one or more dead embryos or undeveloped eggs. Fetal mortality rates were 50% (n = 2), 33.3% (n = 3) and 16.7% (n = 1) by brood. The first female (1010 mm DW) with fetal mortality was collected August 2003. She had one embryo and one unfertilized or undeveloped egg, i.e., 50.0% fetal mortality. The remaining five females (with DWs of 1142 mm, September 2003; 1275 mm, June 2010; 1047 and 1081 mm, July 2018; 1026 mm, July 2019) had undeveloped eggs, or dead embryos in their uteri, resulting in individual fetal mortality rates of 33.3%, 16.7%, 50.0%, 33.3%, and 33.3%, by brood, respectively.
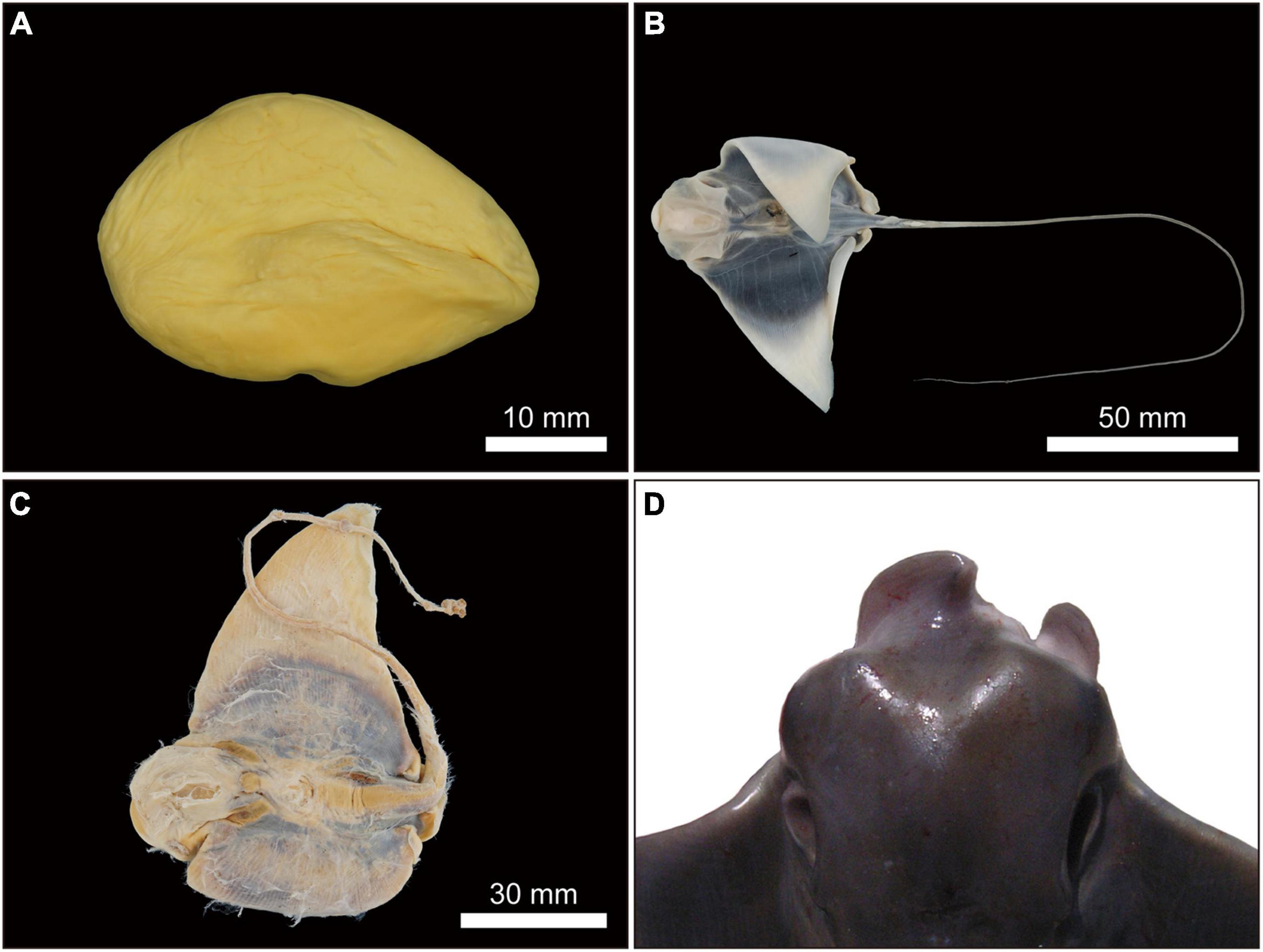
Figure 13. Undeveloped or unfertilized uterine eggs or dead embryos and abnormal morphological embryos of Aetobatus narutobiei. (A) Egg without embryonic development from a female collected in June 2010; (B,C) dead embryos from females collected in July 2019 (B) and September 2003 (C) and (D) an abnormal embryo in September 2009.
One morphologically abnormal but live embryo, alongside two healthy embryos was observed in a single gravid female (DW of 1058 mm) collected on 2 September 2009. All other females (n = 65) presented normal embryos. The abnormal embryo was at embryonic stage 11 and its deformities were associated with fusion of the cranial margin of the pectoral fins or disc (Figure 13D). The percentage of morphologically abnormal Naru eagle ray embryos observed in Ariake Bay was 0.45% (n = 1).
Discussion
This research represents the first comprehensive reproductive life history study for an Aetobatidae ray and provides data fundamental for the development of future science-based management strategies of A. narutobiei. Naru eagle rays are considered specialists that feed mainly on bivalves (Yamaguchi et al., 2005) whose foraging activities are assumed to negatively impact populations of commercial bivalves, for both fisheries and aquaculture. Consequently, developing programs to reduce their population rather than protecting the rays as an important component of the ecosystems has been the focus of previous research (Fukuda and Zenitani, 2010; Yokoyama et al., 2014). This research identifies Ariake Bay as the site of mating, gestation and parturition and a nursery ground for Naru eagle rays (Heupel et al., 2007). Therefore, coastal areas such as Ariake Bay that are important bivalve fishery grounds also serve as critical habitat for all life stages of Naru eagle rays.
Naru eagle rays reproduce synchronously and annually. They are especially vulnerable to overfishing because of their low fecundity and long reproductive cycle. Their reproductive strategy includes rapid embryonic development, parturition, and mating in Ariake Bay during late summer, and a long period of embryonic diapause in relation to seasonal migrations outside of Ariake Bay.
Reproduction and Size at Sexual Maturity
Size and age at 50% sexual maturity for Naru eagle ray females was 20% and 42% greater than that of males, respectively. In the family Aetobatidae, size and age at sexual maturity were known previously for only male spotted eagle ray A. narinari (ocellated eagle ray A. ocellatus at present) from Australia (DW at 50% sexual maturity of 130.6 cm) (Schluessel et al., 2010). Size and age disparity between sexes has been reported for other stingray species such as the longtail butterfly ray Gymnura poecilura (Menon et al., 2020), whiptail stingray Dasyatis chrysonota (Ebert and Cowley, 2009), and bat ray Myliobatis californica (Martin and Cailliet, 1988b) might be typical of Myliobatiforms and related to their reproductive mode (Martin and Cailliet, 1988b; Capapé, 1993; Furumitsu et al., 2010, 2019). For viviparous species larger females are advantageous, as the increased internal space for developing embryos enables larger young and/or higher fecundity at parturition (Furumitsu et al., 2019). However, the long time needed for females to reach maturity makes A. narutobiei especially vulnerable to anthropic pressure, particularly targeted fishing efforts that remove gestating females such as the Ariake Bay predator control program.
The bimodal size distribution for male and female A. narutobiei in Ariake Bay was similar to the size distributions of red stingrays in Ariake Bay (Furumitsu et al., 2019) and Atlantic stingray Hypanus sabinus from Florida (Snelson et al., 1988). This bimodal pattern may be the result of differing habitat preferences for smaller (the immature or premature stage) and larger size classes. Naru eagle rays were usually sampled from size-segregated schools of immature or premature rays (including both sexes), and mature (males and females, separately) life stages. The observed bimodal distribution in sizes in Ariake Bay also may be related to fishing pressure with larger rays and bigger aggregations of eagle rays targeted by fisher, presumably to maximize profit from the predator control program.
Reproductive Seasonality
In general, sampling Naru eagle rays proved to be extremely challenging because of their large size and mass. Moreover, the testis and ovaries were particularly susceptible to post-mortem deterioration. Pregnant females were prone to spontaneous abortion when caught and brought onto the vessel as has been reported for many elasmobranchs, especially rays (Adams et al., 2018). This may be responsible for the lack of reproductive life history information for Naru eagle rays, especially fecundity and gestation time, despite its importance for conservation and management.
In common with many elasmobranch species that reproduce seasonally, the IG for males decreased in advance of the mating season (Yamaguchi et al., 1997, 2000; Kyne and Bennett, 2002). Similarly, A. narutobiei IG peaked 1 month prior to the observation of semen in seminal vesicles and mating activity. The decrease in IG represents seasonal testicular atrophy, where active spermatogenesis has ceased and sperm are stored in the seminal vesicles. The presence of mature sperm in the testes and seminal vesicles coincided with parturition and observation of pre-ovulatory ova for females.
Naru eagle rays undertake annual and seasonal migrations between the inner and outer bay areas (Yamaguchi et al., 2005). Male and female body condition deteriorated during winter and early spring while outside Ariake Bay. Seasonal patterns for IG were different between males and females and may be a consequence of the energetic demands of reproduction. However, monthly variation in IH was not different between males and females and may be attributed to common seasonal migration and feeding habits. In April, when rays return to Ariake Bay, male and female IH was at its nadir. Before leaving the bay in November, IH had doubled. Peak IH corresponded with completion of spermatogenesis for males and the end of gestation and parturition for females. Hepatic energy stored from feeding activity in Ariake Bay during late summer and fall is used as an energy source during the overwintering period and for spring and early summer reproductive activity.
Male Reproductive Anatomy
Shark and ray testes are classified into three major organizational types: radial, diametric, and compound (Pratt, 1988). However, the testis organization type for some batoids does not conform to one of these types and has been described as lobate with radial or compound organization (Pratt, 1988). This research is, to the best of our knowledge, the first to describe testis structure and spermatogenesis for rays in the Aetobatidae family. Aetobatus narutobiei testes had internal lobes composed of spermatocysts that developed radially from a central germinal zone. Lobate testes with radial development were observed also for a Potamotrygon ray (Pratt, 1988). Other rays where testis organization has been described [Atlantic stingray (Maruska et al., 1996), red stingray (Furumitsu et al., 2019), bat ray (Pratt, 1988), smooth butterfly ray Gymnura micrura (Pratt, 1988), cownose ray Rhinoptera bonasus (Poulakis, 2013) and Fluvitrygon signifer (Chatchavalvanich et al., 2005)] have lobate testes with compound development of spermatocysts across each lobe. Future studies on the testicular organization for other batoids may reveal additional diversity in reproductive anatomy as well as provide insight into the implications of these alternative organizational patterns on reproduction.
Female Reproductive Anatomy and Fecundity
Elasmobranchs utilize a plethora of reproductive modes and their reproductive anatomy and physiology is equally diverse (Wourms, 1977; Hamlett and Koob, 1999; Lutton et al., 2005). All myliobatid rays are viviparous and histotrophic, with female reproductive anatomy, species specific with respect to the symmetry and functionality of ovaries and uteri. This is important because the number of functional ovaries and uteri may affect fecundity and ultimately fitness for viviparous species. Previously, little was known about fecundity for family Aetobatidae. Fecundity for spotted eagle ray based on 60 pregnancies for rays held at six aquaria worldwide (Underwater World Singapore, SeaWorld Antonio, Discovery Cove, Georgia Aquarium, Burger’s Zoo, Disney’s The Seas, Okinawa Expo Aquarium and Ripley’s Aquarium of the Smokies) ranged from 1 to 4 young with a mean of 1.8 ± 0.6 young per litter, and neonate DW 44.7 ± 5.1cm at birth (Swider et al., 2017). The left ovary and uterus were functional and the right ovary and uterus vestigial based on examination of a single post-partum spotted eagle ray (Swider et al., 2017). However, at least one of the included institutions (Okinawa Expo Aquarium) houses ocellated eagle ray that were previously identified as a spotted eagle rays. Uchida et al. (1990) reported fecundity was 1 or 2 with DW at birth 50–59 cm for the ocellated eagle rays. In situ spotted eagle rays sampled from Venezuela have one functional uterus and their fecundity ranges from 1 to 5 (mean 3.09) (Tagliafico et al., 2012). Both ovaries and uteri are functional for Naru eagle rays and their fecundity (max 7) is the highest of all Aetobatids. Although fecundity of in situ spotted eagle rays from Venezuela is similar to in situ Naru eagle rays (this study), the maximum DW (2260 mm) of the largest Venezuelan female was almost 1.5 times that of the largest female Naru eagle ray from Ariake Bay (Tagliafico et al., 2012). This suggests that the reproductive life history strategy of Naru eagle rays maximizes the number of young over their size at birth. This contrasts with the majority of myliobatids, whose reproductive strategy includes producing a small number of large neonates. Fecundity of 23 eagle ray species ranges from 1 to 12 with 14 species less than 2 (Figure 14A), putting the relatively high fecundity of Naru eagle rays into phylogenetic perspective. From an anatomical perspective, fecundity for Myliobatidae and Aetobatidae species with paired uteri was higher compared with Rinopteridae and Mobulidae species with one functional uterus and usually, only one offspring (Bigelow and Schroeder, 1953; Notarbartolo-di-Sciara and Serena, 1988; White et al., 2006; Mendonça et al., 2012). The Naru eagle ray is the only Aetobatid species with paired uteri and females had higher fecundity and smaller neonates at birth than similarly sized congener females (Figure 14B). Overall, embryo size at birth is smaller among myliobatids with two uteri, regardless of female size. However, even though Naru eagle ray fecundity is high among myliobatid species, it is lower than that reported for 21 dasyatid species with only a single uterus (Furumitsu et al., 2019), and therefore the call for conservation of Naru eagle rays remains an urgent necessity. Functionally there was no relationship between maximum female size and fecundity among eagle ray species (Figure 14A), but larger species generally bore larger young, similar to dasyatid species (Figure 14B).
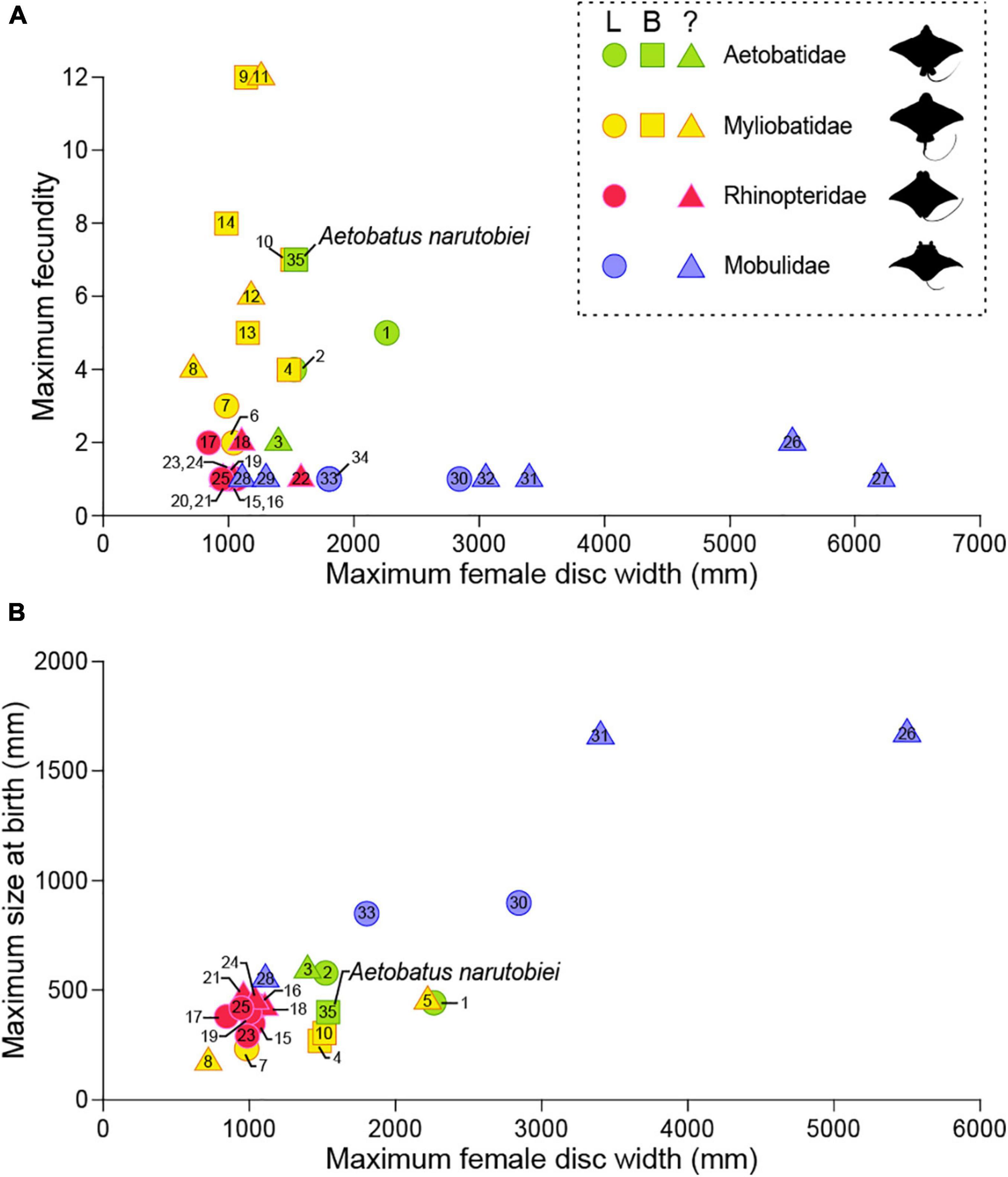
Figure 14. Variability in the number of functional uteri compared to (A) fecundity and (B) maximum size at birth for subfamily Myliobatinae. Rays with two functional uteri are represented by squares, circles are shown for rays that have a single (left) functional uterus, and triangles are shown for species that have not been described. Aetobatus narinari: 1 (Tagliafico et al., 2012) and 2 (Swider et al., 2017); Aetobatus ocellatus: 3 (Uchida et al., 1990); Aetomylaeus bovinus: 4 (Seck et al., 2002) and 5 (Dulčić et al., 2008); Aetomylaeus maculatus: 6 (Devadoss, 1982); Aetomylaeus nichofii: 7 (Devadoss, 1998) and 8 (Last and Stevens, 2009); Myliobatis aquila: 9 (Capapé et al., 2007); Myliobatis californicus: 10 (Martin and Cailliet, 1988a) and 11 (Villavicencio Garayzar, 1996); Myliobatis freminvillei: 12 (Tagliafico et al., 2016); Myliobatis goodei: 13 (Araújo et al., 2016); Myliobatis ridens: 14 (Araújo et al., 2016); Rhinoptera bonasus: 15 (Neer and Thompson, 2005), 16 (Pérez-Jiménez, 2011), 17 (Poulakis, 2013), 18 (Fisher, 2010) and 19 (Smith and Merriner, 1986); Rhinoptera brasiliensis: 20 (Domingues et al., 2009); Rhinoptera javanica: 21 (Uchida et al., 1990) and 22 (James, 1962, 1971); Rhinoptera marginata: 23 (Tıraşın and Başusta, 2018); Rhinoptera steindachneri: 24 (Bizzarro et al., 2007) and 25 (Burgos-Vázquez et al., 2019); Mobula alfredi: 26 (Marshall and Bennett, 2010); Mobula birostris: 27 (Cabanillas-Torpoco et al., 2019); Mobula hypostoma: 28 (Bigelow and Schroeder, 1953); Mobula kuhlii cf. eregoodootenkee: 29 (Broadhurst et al., 2018); Mobula mobular: 30 (White et al., 2006) and 31 (Notarbartolo-di-Sciara and Serena, 1988); Mobula tarapacana: 32 (Notarbartolo-di-Sciara, 1988); Mobula thurstoni: 33 (Notarbartolo-di-Sciara, 1988) and 34 (Mendonça et al., 2012); Aetobatus narutobiei: 35 this study.
Regardless of reproductive mode, reproductive output of viviparous species is confined by internal space. If the total area available to developing embryos is similar for females with a single uterus versus two uteri, then overall reproductive output might be similar from a total mass standpoint but not necessarily from a reproductive output standpoint. Females maximize the number of young or the size of young as part of their reproductive strategy. The potential maximum size of young at birth for viviparous species is ultimately bound by the size of the uterus. Aetobatus is an important species to study in order to improve our understanding of the trade-offs between number of functional uteri and the size and number of young produced during each reproductive cycle and their influence on other life history characteristics. For example, manta and devil rays have a single functional uterus and produce a small number of very large young. They have acquired larger bodies and are among the largest of all batoids with expansive oceanic and pelagic ranges.
Functional Morphology of the Uterus
Elasmobranch reproduction includes lecitotrophic and matrotrophic reproductive modes (Wourms, 1977; Hamlett et al., 2005). Histotrophy is a type of matrotrophy where embryos are provided supplemental nutrition as histotroph or uterine milk that contains variable proportions of lipids, carbohydrates and proteins. All stingrays are histotrophic and the uterine mucosa is specialized with villous projections termed trophonemata (Hamlett et al., 1985, 2005; Compagno, 1990; Hamlett and Hysell, 1998). Trophonemata are involved in one or more critical physiological processes during gestation including gas exchange, nutrient provision, and osmoregulation (Babel, 1967; Hamlett et al., 1985, 1996; Smith and Merriner, 1986; Johnson and Snelson, 1996; Hamlett and Hysell, 1998).
Aetobatus narutobiei trophonemata length was proportional to female size but not embryo size. This suggests that trophonemata elongation and histotroph (uterine milk) secretion are not directly related and that gas exchange and osmoregulation may be more important. A similar finding was observed for Myliobatis goodei where the prominent vascularity and the thin epithelium of trophonemata aids gaseous exchanges to supply the oxygen demands of the embryos (Colonello et al., 2013). Previous studies on the uterus of the cownose ray (Hamlett et al., 1985), southern stingray Hypanus americanus (Hamlett et al., 1996), and manta ray Mobula alfredi (Tomita et al., 2012) suggest that oxygen may be supplied to the uterine fluid from the uterine mucosa by diffusion. Colonello et al. (2013) proposed that the source of the histotroph for M. goodei was the basal crypts observed between the trophonemata, especially later in gestation, when embryonic nutritional requirements are increased. The function(s) of Naru eagle ray uterine trophonemata may include one or more critical aspects of embryonic respiration and nutrition but further study is needed for confirmation.
The energy derived from consumption of yolk and histotroph (uterine milk) resulted in a 34,626% increase in embryo mass at birth compared to the mass of the egg yolk. Increases in embryo mass of more than 3,000% during gestation are common for histotrophic rays, such as Bathytoshia centroura (approximately 3,000%) (Capapé, 1993), Aetomylaeus bovina (3,120%) (Seck et al., 2002), Pteroplatytrygon violacea (1,680%) (Babel, 1967), butterfly ray (3,564%) (Yokota et al., 2012), red stingray (2,080–15,643%) (Furumitsu et al., 2019) and yellow stingray Urobatis jamaicensis (4,600%) (Fahy et al., 2007). For Naru eagle rays, the percent increase in embryo mass is 2–10 times higher in comparison. Aetobatus narutobiei embryos showed the greatest degree of matrotrophy or growth efficiency of any ray; however, based on female size we suspect Mobulidae might have an even higher degree of matrotrophy. Future work will focus on characterizing histotroph and uterine mucosa dynamics to identify the source of histotroph and its nutritional content for Naru eagle rays. This information could help to elucidate mechanisms for the extreme degree of matrotrophy observed for Naru eagle rays and the reproductive strategy of the largest eagle and manta rays.
Embryonic Diapause for Overwintering Survival
Aetobatus narutobiei gestation was nearly a year, but embryos were in diapause for 79.2% of the duration. Embryonic diapause, a temporary arrest of development, is a reproductive strategy that extends the time required to complete a reproductive cycle. For Naru eagle rays diapause was obligate, occurring each year and observed for every pregnant female. In elasmobranchs, diapause is recognized among species with seasonal and synchronous reproductive cycles, when females possess uterine eggs without macroscopic signs of embryonic development during sequential months (Wyffels, 2009). This is the first observation of embryonic diapause for any species of the family Aetobatidae, though diapause has been observed for nearly 20 elasmobranch species (Waltrick et al., 2012). Embryonic diapause is typically thought to enable young to be born when environmental conditions such as prey abundance, predator presence, and water temperature are optimal for their growth and survival (Simpfendorfer, 1992; Marshall et al., 2007). Diapause may also be due to the energetic demands of reproduction for adults and the seasonal availability of mates. It is likely that the drivers and advantage(s) of diapause are species and habitat specific, requiring a thorough understanding of the complete life history of each species under investigation (Waltrick et al., 2012).
Considering the advantages of diapause from the perspective of Naru eagle ray neonates, prey abundance, water temperature, and predator presence are all factors potentially influencing gestation time. At the end of summer when A. narutobiei give birth, bivalves are abundant in Ariake Bay’s estuary. Adult hammerhead sharks Sphyrna spp. and white cheek sharks Carcharhinus tjutjot are the most likely predators of Naru eagle rays. These species also use Ariake Bay as mating and nursery grounds, but migrate into the bay in May and depart in July after breeding. Only neonate sharks remain in the bay, thereby minimizing the risk of predation on neonate Naru eagle rays. Summer water temperature in Ariake Bay when Naru eagle rays give birth is high, but with the onset of the autumn season water temperature declines. The water temperature of the estuary and shallow areas of Ariake Bay is below 10°C during the winter period, which can threaten survival of Naru eagle rays. Therefore, when water temperature reaches 18–19°C in late October and November, Naru eagle rays gradually move from the shallow regions to the deeper, southern habitat within Ariake Bay, before migrating to the open sea to overwinter. Our observations include a 200 km migration from the innermost area of Ariake Bay to Amakusa-nada in the East China Sea over the period of a month (Yamaguchi and Furumitsu, unpublished data). The timing of parturition for Naru eagle rays occurs when water temperatures are highest, predator presence lowest, and is optimized for young to maximize food consumption and energy stores in the 2–3 months period prior to their winter migration.
The active embryonic development phase occurs quickly, from June to August, after a protracted diapause phase where embryonic development is arrested at a very early stage. The timing of parturition gives young an optimized environment for survival, but the drivers of diapause also should be considered from the perspective of the adult Naru eagle rays. Diapause may allow females to recover body condition depleted from the demands of reproduction and migration (Waltrick et al., 2012). During summer, A. narutobiei females produce ova for the next reproductive cycle at the same time as secreting histotroph for actively developing embryos. The energy demands from reproduction were reflected in female body condition that did not improve until September, after parturition and ovulation. Male and female body condition was at its maximum, nearly twice as high as in April, by the end of autumn just before migration out of Ariake Bay. Females were pregnant prior to migration and overwintering. During overwintering the embryos are arrested and as a result, the energetic demands of pregnancy may be minimal and relatively constant. The rays remained in deeper waters in the open sea where the water temperature is stable during the winter, and likely use stored energy from feeding in Ariake Bay as sustenance in the absence of prey. As the water temperature in Ariake Bay increased in the spring, A. narutobiei migrated from the depths of the open sea to the shallow estuaries of Ariake Bay where bivalves were prevalent from April to June allowing them to recover depleted body condition before meeting the energetic demands of histotroph production and concurrent folliculogenesis. In autumn, the size and the stock of their main food, bloody clam (ark shell) Scapharca kagoshimensis, increased after the end of the fishing season (Masaki and Onohara, 2003) providing energy for their winter migration.
There are few potential predators for the adult Naru eagle rays in Ariake Bay during late summer and autumn. Their migration to the open sea to avoid low water temperatures in Ariake Bay is expected to be extremely dangerous with regard to large predator encounters, long-distance movement, and acquisition of food. Males are smaller than females (Max: 1000 mm DW vs. 1500 mm DW) and their lifespans (9 years vs. 19 years) were less than half that of the females (Yamaguchi et al., 2005). The natural mortality rate of males is 3.2 times higher than for females. The smaller size of males may have left them more susceptible to predation and thus, particularly higher mortality rates for the males compared to females during migration and overwintering can be expected. Thus, mating prior to exposure to risks associated with overwintering is advantageous for adult males.
Neonates are born when food is abundant, water temperature is highest, and predator presence is lowest. Adult females take advantage of diapause to allow them to recover body condition lost during overwintering before the active phase of embryonic development begins and they need to simultaneously produce histotroph and undergo folliculogenesis, both energy intensive physiological processes. Parturition is timed to allow females several months to improve body condition before winter migration. Similar to females, adult males have several months after returning to Ariake Bay after overwintering to recover body condition before the mating season and have several months after mating to gain condition before migration. Therefore, embryonic diapause benefits both A. narutobiei adults and neonates.
The reproductive life history of up to nine batoid species in Ariake Bay may include embryonic diapause (Furumitsu, 2009; Kume et al., 2009; Yamaguchi and Kume, 2009; Furumitsu et al., 2019; Yamaguchi, unpublished data). Embryonic development periods are 2.5–3 months for most species; however, the timing of parturition is different for each species, from July to September (e. g., Hemitrygon laevigata in July; H. akajei, Rhinobatis hynnicephalus, and Platyrhina tangi in July and August; and Myliobatis tobijei and A. narutobiei in August and September). We hypothesize another strategy where species utilize the Ariake Bay estuaries in turn by controlling the length of the diapause phase of gestation based on environmental conditions (water temperature, predator presence, competition, and food supply). Ariake Bay is remarkable with a high concentration of species that have adopted diapause as a reproductive life history strategy. Further research including the identification of endogenous and exogenous factors that initiate diapause and resumption of embryonic development are needed.
Fetal Mortality and Morphologically Abnormal Embryos
The fetal mortality rate for wild-caught viviparous rays including observations of dead embryos and unfertilized or undeveloped uterine eggs has been reported previously (1.25%) for only one species, red stingray (Furumitsu et al., 2019). In comparison, the fetal mortality rate for A. narutobiei was 2.68%, or twice as high. For both Ariake Bay species, the observation of mummified embryos was rare compared to undeveloped or unfertilized eggs. No estimates of fetal mortality rate are available for viviparous sharks, however, similar observations of mummified embryos and unfertilized eggs have been reported (Clark and von Schmidt, 1965; Randall, 1977; Rosa-Molinar et al., 1983; Sandoval-Castillo and Villavicencio-Garayzar, 2008; Baremore and Hale, 2012). Unfertilized eggs waste both the energy required for ova formation during folliculogenesis and uterine space during gestation. In the absence of a fitness advantage, females would be expected to minimize unfertilized eggs in a reproductive cycle. Multiple mating is one strategy females may use to ensure fertilization of all ova and unfertilized uterine eggs could be attributed to reduced mating or insufficient sperm. Of the two Ariake Bay species, only A. narutobiei is a target of the predator control program. The higher rate of fetal mortality for A. narutobiei, particularly unfertilized eggs, may be a result of lower numbers of male available for mating due to the predator control program and natural mortality. This would be especially important if A. narutobiei include multiple paternity as part of their reproductive life history (Portnoy et al., 2007).
The frequency of morphologically abnormal embryonic specimens for A. narutobiei in Ariake Bay was relatively low (0.45%), and similar to the red stingray (0.72%) from Ariake Bay (Furumitsu et al., 2019). All abnormalities observed for A. narutobiei in Ariake Bay were associated with fusion of the pectoral fins. This physical deformity is common and has been reported among embryos of other batoids (Honma and Sugihara, 1971; Lamilla et al., 1995; Yamaguchi, 2004; Escobar-Sánchez et al., 2009; Blanco-Parra and Niño-Torres, 2011; Ramírez-Amaro et al., 2013). Most reports of morphological abnormalities describe embryos close to their birth size, an indication that the deformity does not affect ray survival in utero. Indeed, adult specimens with abnormal morphologies are observed rarely in batoid species, recently summarized by Furumitsu et al. (2019). The rate of developmental abnormalities described for Naru eagle rays and red stingrays are similar and may represent the natural incidence of birth defects for rays in a healthy ecosystem. As anthropogenic influences and climate change increasingly affect critical habitats like Ariake Bay, monitoring the rate of mummified embryos, unfertilized and undeveloped eggs and developmental abnormalities might be a useful to ensure the incidence does not increase over time.
Predator Control Programs and Science-Based Ecosystem Management
A government sponsored predator control program was implemented to increase productivity of the bivalve fishery by reducing predation on bivalves from Naru eagle rays based on the marine trophic cascade hypothesis (Myers et al., 2007). According to the hypothesis, a decline in large shark species and subsequent increase in mesopredator rays led to a decline in bivalve yield in the Chesapeake Bay. This trophic cascade imbalance was used to justify the development of targeted cownose ray fisheries in the northwest Atlantic (Myers et al., 2007). Subsequent analyses using expanded regional data and temporal analyses for shark, ray and bivalve abundance found little support for the shark-mediated trophic cascade or vilification of cownose rays in the fishery collapse (Grubbs et al., 2016). Similarly, for Naru eagle rays and bivalves in Ariake Bay, bivalve catches were in decline already since the mid-1980s (Yamaguchi et al., 2005). Therefore, increased eagle ray populations observed in the late-1990s do not overlap with long-term declines of bivalve catches. Therefore, it is essential to test the hypothesized trophic cascade using predator–prey linkages in this estuarine ecosystem and the impacts of long-term fishing pressures on the Naru eagle ray.
Estuaries serve as nursery grounds for many coastal fishes (Takita and Yamaguchi, 2009). This study identified the Ariake Bay estuary as critical habitat for reproduction and as a nursery for A. narutobiei. Previous studies have identified coastal areas as nurseries for elasmobranchs (Simpfendorfer and Milward, 1993; Yokota and Lessa, 2006; Rangel et al., 2018) but there are few estuarine habitats that function as nurseries for elasmobranchs (Heupel and Simpfendorfer, 2011; Francis, 2013). In Ariake Bay, many elasmobranchs, including scalloped hammerhead sharks and white cheek sharks, use the estuary for parturition and breeding during early summer and then depart the bay. Their neonates remain in the bay until autumn and are frequently caught by commercial fisheries using set nets and gill nets. Stingrays such as the red stingray also use the estuary as a nursery (Furumitsu et al., 2019). Thus, abundant neonates for several elasmobranch species during summer and autumn are dependent on the estuarine areas of Ariake Bay. The use of the estuarine areas may be a successful ecological strategy to improve survival rates, because of reduced predation (Heupel and Simpfendorfer, 2011).
Naru eagle rays have specific environmental requirements for food, reproduction, and overwintering, and Ariake Bay, an expansive estuary ecosystem, is extremely important and one of the only habitats known to support their requirements for life. The rays leave the bay in winter, when water temperatures are coldest, and are dependent on the estuary to replenish depleted energy stores from overwintering and migration and to complete gestation, parturition and mating in rapid succession during the short summer season. The same estuary also serves as a nursery area. Adults leverage diapause to minimize the energetic demands of reproduction during migration and overwintering outside of the estuary and diapause benefits neonates by delaying parturition until summer, the optimum season for their survival. The combination of long lifespan, slow maturity, low fecundity, and long gestation along with increased and targeted fishing pressure during periods when rays are aggregated for reproduction has resulted in rapid population decline. These same life-history traits will prevent a rapid recovery. Science-based management is essential to ensure survival of this threatened and endemic ray. This study details consequences of targeted removal of an apex/meso predator from a complex ecosystem and serves as a model to assess the effects of natural and anthropogenic marine environmental change.
Data Availability Statement
Datasets collected and analyzed as part of this research are available from the corresponding author upon reasonable request.
Ethics Statement
Ethical review and approval was not required for this animal study in accordance with institutional requirements. Animal samples used in this study were collected from operators involved in a predator control program authorized by various national and prefectural governments.
Author Contributions
AY conceived the study and obtained funding. AY and KF completed field surveys and JW joined when in country. KF curated and analyzed the data. AY, KF, and JW interpreted the data and wrote and reviewed the manuscript. All authors approved the final manuscript.
Funding
This study was supported in part by the Japan Society for the Promotion of Science KAKENHI Grant Nos. 19H02977, 23380112, 20580205, and 26660161.
Conflict of Interest
The authors declare that the research was conducted in the absence of any commercial or financial relationships that could be construed as a potential conflict of interest.
Publisher’s Note
All claims expressed in this article are solely those of the authors and do not necessarily represent those of their affiliated organizations, or those of the publisher, the editors and the reviewers. Any product that may be evaluated in this article, or claim that may be made by its manufacturer, is not guaranteed or endorsed by the publisher.
Acknowledgments
We are indebted to the fishers: Y. Kobayashi, T. Shimoda, and other members of the Ooura Fishermen’s Cooperative; Saga and S. Yoshida of the Shimabara Fishermen’s Cooperative, Nagasaki; T. Jinkawa of the Ashikari Fishermen’s Cooperative, Saga; and Y. Yoshida of the Higashiyoka-cho Fisherman’s Cooperative, Saga, who supported our efforts to examine their eagle ray catch for more than a decade. The samples used in this study were caught as part of Harmful Organism Control programs of the Fisheries Agency of Agriculture, Forestry and Fisheries or prefectural offices. We thank G. Kume (Kagoshima University, Kagoshima), T. Ito, Y. Oka, K. Hara, M. Watanabe, Y. Ogino and many students from the Yamaguchi Laboratory (Nagasaki University, Nagasaki) for assistance with examination of specimens and field work. We received generous support from K. Aoyagi and T. Yuasa (National Federation of Fisheries Co-operative Associations) during the course of this study. We thank the reviewers for questions and suggestions that improved the clarity and impact of the research detailed in this manuscript.
References
Adams, K. R., Fetterplace, L. C., Davis, A. R., Taylor, M. D., and Knott, N. A. (2018). Sharks, rays and abortion: The prevalence of capture-induced parturition in elasmobranchs. Biol. Conserv. 217, 11–27. doi: 10.1016/j.biocon.2017.10.010
Araújo, P. R. V., Oddone, M. C., and Velasco, G. (2016). Reproductive biology of the stingrays, Myliobatis goodei and Myliobatis ridens (Chondrichthyes: Myliobatidae), in southern Brazil. J. Fish Biol. 89, 1043–1067. doi: 10.1111/jfb.13015
Babel, J. S. (1967). Reproduction, Life History, and Ecology of the Round Stingray, Urolophus halleri Cooper. California: University of Southern California.
Baremore, I. E., and Hale, L. F. (2012). Reproduction of the sandbar shark in the western North Atlantic Ocean and Gulf of Mexico. Mar. Coast. Fish.: Dyn. Manag. Ecosyst. Sci. 4, 560–572. doi: 10.1080/19425120.2012.700904
Bigelow, H. B., and Schroeder, W. C. (1953). Fishes of the Western North Atlantic, Part 2. Sawfishes, Guitarfishes, Skates and Rays; Chimaeroids. Sears Foundation for Marine Research. New Haven: Yale University.
Bizzarro, J. J., Smith, W. D., Márquez-Farías, J. F., and Hueter, R. E. (2007). Artisanal fisheries and reproductive biology of the golden cownose ray, Rhinoptera steindachneri Evermann and Jenkins, 1891, in the northern Mexican Pacific. Fish. Res. 84, 137–146. doi: 10.1016/j.fishres.2006.10.016
Blanco-Parra, M. P., and Niño-Torres, C. A. (2011). Morphological abnormality in a diamond stingray, Dasyatis dipterura (Jordan & Gilbert, 1880) (Chondrichthyes: Dasyatidae), from the Gulf of California, Mexico. Cah. Biol. Mar. 52, 357–360.
Broadhurst, M. K., Laglbauer, B. J. L., Burgess, K. B., and Coleman, M. A. (2018). Reproductive biology and range extension for Mobula kuhlii cf. eregoodootenkee. Endanger. Spec. Res. 35, 71–80. doi: 10.3354/esr00876
Burgos-Vázquez, M. I., Chávez-García, V. E., Cruz-Escalona, V. H., Navia, A. F., and Mejía-Falla, P. A. (2019). Reproductive strategy of the Pacific cownose ray Rhinoptera steindachneri in the southern Gulf of California. Mar. Freshw. Res. 70, 93–106. doi: 10.1071/MF18096
Cabanillas-Torpoco, M., Forsberg, K., Siccha-Ramirez, R., Cisneros, P., Luque, C., Purizaca, W., et al. (2019). First description of a giant manta ray fetus Mobula birostris (Walbaum 1792) from Tumbes, Peru (Southeast Pacific). Zootaxa 4603, 397–400. doi: 10.11646/zootaxa.4603.2.12
Camhi, M., Fowler, S., Musick, J., Bräutigam, A., and Fordham, S. (1998). Sharks and Their Relatives: Ecology and Conservation. Cambridge: IUCN Publications Services Unit.
Capapé, C. (1993). New data on the reproductive biology of the thorny stingray, Dasyatis centroura (Pisces: Dasyatidae) from off the Tunisian coasts. Environ. Biol. Fish. 38, 73–80. doi: 10.1007/978-94-017-3450-9_7
Capapé, C., Guélorget, O., Vergne, Y., and Quignard, J. P. (2007). Reproductive biology of the common eagle ray Myliobatis aquila (Chondrichthyes: Myliobatidae) from the coast of Languedoc (southern France, northern Mediterranean). Vie Milieu 57, 125–130.
Chatchavalvanich, K., Thongpan, A., and Nakai, M. (2005). Structure of the testis and genital duct of freshwater stingray, Himantura signifer (Elasmobranchii: Myliobatiformes: Dasyatidae). Ichthyol. Res. 52, 123–131. doi: 10.1007/s10228-004-0262-2
Clark, E., and von Schmidt, K. (1965). Sharks of the central Gulf Coast of Florida. Bull. Mar. Sci. 15, 13–83.
Colonello, J. H., Christiansen, H. E., Cousseau, M. B., and Macchi, G. J. (2013). Uterine dynamics of the southern eagle ray Myliobatis goodei (Chondrichthyes: Myliobatidae) from the southwest Atlantic Ocean. Ital. J. Zool. 80, 187–194. doi: 10.1080/11250003.2012.742146
Compagno, L. J. V. (1990). Alternative life-history styles of cartilaginous fishes in time and space. Environ. Biol. Fish. 28, 33–75. doi: 10.1007/BF00751027
Conrath, C. L., and Musick, J. A. (2002). Reproductive biology of the smooth dogfish, Mustelus canis, in the northwest Atlantic Ocean. Environ. Biol. Fish. 64, 367–377. doi: 10.1023/A:1016117415855
Crear, D. P., Latour, R. J., Friedrichs, M. A. M., St-Laurent, P., and Weng, K. C. (2020). Sensitivity of a shark nursery habitat to a changing climate. Mar. Ecol. Prog. Ser. 652, 123–136. doi: 10.3354/meps13483
Devadoss, P. (1982). On the embryonic stage of the mottled ray, Aetomylus maculatvs (Gray), with a note On its breeding season. Indian J. Fish. 29, 253–255.
Devadoss, P. (1998). Observations on the breeding and development in some batoid fishes. Indian J. Fish. 45, 271–283.
Domingues, R. R., Gonzalez, M. M. B., and Amorim, A. F. (2009). First reported occurrence of pregnant and neonato, Rhinoptera brasiliensis (Chondrichthyes, Rhinopteridae) caught off Guaruja city, São Paulo state, Brazil. J. Aquat. Sci. 4, 605–608.
Dulčić, J., Lipej, L., Orlando Bonaca, M., Jenko, R., Grbec, B., Guélorget, O., et al. (2008). The bull ray, Pteromylaeus bovinus (Myliobatidae), in the northern Adriatic Sea. Cybium 32, 119–123.
Dulvy, N. K., Fowler, S. L., Musick, J. A., Cavanagh, R. D., Kyne, P. M., Harrison, L. R., et al. (2014). Extinction risk and conservation of the world’s sharks and rays. elife 3:e00590. doi: 10.7554/eLife.00590
Dulvy, N. K., Pacoureau, N., Rigby, C. L., Pollom, R. A., Jabado, R. W., Ebert, D. A., et al. (2021). Overfishing drives over one-third of all sharks and rays toward a global extinction crisis. Curr. Biol. 31, 4773–4787. doi: 10.1016/j.cub.2021.08.062
Ebert, D. A., and Cowley, P. D. (2009). Reproduction and embryonic development of the blue stingray, Dasyatis chrysonota, in southern African waters. J. Mar. Biol. Assoc. U. K. 89, 809–815. doi: 10.1017/S0025315408002907
Escobar-Sánchez, O., Galván-Magaña, F., Downton-Hoffmann, C. A., Carrera-Fernández, M., and Alatorre-Ramírez, V. G. (2009). First record of a morphological abnormality in the longtail stingray Dasyatis longa (Myliobatiformes: Dasyatidae) in the Gulf of California, Mexico. Mar. Biodiv. Rec. 2, 1–3. doi: 10.1017/S1755267208000304
Fahy, D. P., Spieler, R. E., and Hamlett, W. C. (2007). Preliminary observations on the reproductive cycle and uterine fecundity of the yellow stingray, Urobatis jamaicensis (Elasmobranchii: Myliobatiformes: Urolophidae) in southeast Florida, U.S.A. Raffles Bull. Zool. Supp. 14, 131–139.
Fisher, R. A. (2010). Life History, Trophic Ecology, & Prey Handling by Cownose Ray, Rhinoptera bonasus, From Chesapeake Bay. Virginia: Virginia Institute of Marine Science.
Flowers, K. I., Heithaus, M. R., and Papastamatiou, Y. P. (2021). Buried in the sand: Uncovering the ecological roles and importance of rays. Fish Fish. 22, 105–127. doi: 10.1111/faf.12508
Francis, M. P. (2013). Temporal and spatial patterns of habitat use by juveniles of a small coastal shark (Mustelus lenticulatus) in an estuarine nursery. PLoS One 8:e57021. doi: 10.1371/journal.pone.0057021
Fukuda, Y., and Zenitani, H. (2010). Estimation of abundance and catchability by DeLury’s method of longheaded eagle ray Aetobatus flagellum (Bloch and Schneider) in Nakatsu of Suo-Nada, Seto Island Sea, Japan. Nippon Suisan Gakk. 76, 10–19. doi: 10.2331/suisan.76.10
Furumitsu, K. (2009). Studies on taxonomy and life history of genus Dasyatis from eastern Asia. [Nagasaki, Japan]: Nagasaki University. PhD Thesis.
Furumitsu, K., Wyffels, J. T., and Yamaguchi, A. (2019). Reproduction and embryonic development of the red stingray Hemitrygon akajei from Ariake Bay, Japan. Ichthyol. Res. 66, 419–436. doi: 10.1007/s10228-019-00687-9
Furumitsu, K., Zhang, J., and Yamaguchi, A. (2010). Redescription of a poorly known stingray, Dasyatis laevigata (Chondrichthyes: Dasyatidae), with notes on some biological aspects in Ariake Sea, Japan. Species Divers. 15, 139–154. doi: 10.12782/specdiv.15.139
Grubbs, R. D., Carlson, J. K., Romine, J. G., Curtis, T. H., McElroy, W. D., McCandless, C. T., et al. (2016). Critical assessment and ramifications of a purported marine trophic cascade. Sci. Rep. 6:20970. doi: 10.1038/srep20970
Hamlett, W. C., and Hysell, M. K. (1998). Uterine specializations in elasmobranchs. J. Exp. Zool. 282, 438–459. doi: 10.1002/(SICI)1097-010X(199811/12)282
Hamlett, W. C., and Koob, T. J. (1999). “Female reproductive system,” in Sharks, Skates and Rays the Biology of Elasmobranch Fishes, ed. W. C. Hamlett (Baltimore, MD: The Johns Hopkins University Press), 398–443.
Hamlett, W. C., Kormanik, G., Storrie, M., Stevens, B., and Walker, T. I. (2005). “Chondrichthyan parity, lecithotrophy and matrotrophy,” in Reproductive Biology and Phylogeny of Chondrichthyes: Sharks, Batoids and Chimaeras, ed. W. C. Hamlett (Enfield, NH: Science Publishers), 395–434.
Hamlett, W. C., Musick, J. A., Eulitt, A. M., Jarrell, R. L., and Kelly, M. A. (1996). Ultrastructure of uterine trophonemata, accommodation for uterolactation, and gas exchange in the southern stingray, Dasyatis americana. Can. J. Zool. 74, 1417–1430. doi: 10.1139/z96-157
Hamlett, W. C., Wourms, J. P., and Smith, J. W. (1985). Stingray placental analogues: structure of trophonemata in Rhinoptera bonasus. J. Submicrosc. Cytol. 17, 541–550.
Heupel, M. R., Carlson, J. K., and Simpfendorfer, C. A. (2007). Shark nursery areas: concepts, definition, characterization and assumptions. Mar. Ecol. Prog. Ser. 337, 287–297. doi: 10.3354/meps337287
Heupel, M. R., and Simpfendorfer, C. A. (2011). Estuarine nursery areas provide a low-mortality environment for young bull sharks Carcharhinus leucas. Mar. Ecol. Prog. Ser. 433, 237–244. doi: 10.3354/meps09191
Hoenig, J. M., and Gruber, S. H. (1990). “Life-history patterns in the elasmobranchs: implications for fisheries management,” in Elasmobranchs as Living Resources: Advances in the Biology, Ecology, Systematics, and the Status of the Fisheries NOAA Technical Report NMFS 90, eds J. H. L. Pratt, S. H. Gruber, and T. Taniuchi (Seattle, WA: U.S. Department of Commerce,), 1–16.
Honma, Y., and Sugihara, C. (1971). A stingray, Dasyatis akajei, with aberrant pectoral fins from the Japan Sea. JPN. J. Ichthyol. 18, 187–189. doi: 10.11369/jji1950.18.187
James, P. S. B. R. (1962). Observations on shoals of the Javanese cownose ray Rhinoptera javanica Müller & Henle from the Gulf of Mannar, with additional notes on the species. J. Mar. Biol. Assoc. India 4, 217–223.
James, P. S. B. R. (1971). Further observations on shoals of the Javanese cownose ray Rhinoptera javanica Müller & Henle from the Gulf of Mannar with a note on the teeth structure in the species. J. Mar. Biol. Assoc. India 12, 151–157.
Johnson, M. R., and Snelson, J. (1996). Reproductive life history of the Atlantic stingray, Dasyatis sabina (Pisces, Dasyatidae), in the freshwater St. Johns River, Florida. Bull. Mar. Sci. 59, 74–88.
Kawahara, I., Ito, S., and Yamaguchi, A. (2004). Influence of the Bullnose Ray, Aetobatus flagellum, on the Pen-Shell, Atrina pectinata, in Ariake Sound. Bull. Saga Prefect. Ariake Fish. Res. Dev. Cent. 22, 29–33.
Kimura, S., Senou, H., Yamaguchi, A., Suzuki, T., and Shigeta, T. (2018). Outline and issues of the Red List of threatened marine fishes in Japan. JPN. J. Ichthyol. 65, 97–116.
Kume, G., Furumitsu, K., Tanaka, S., and Yamaguchi, A. (2009). Reproductive biology of the guitarfish Rhinobatos hynnicephalus (Batoidea: Rhinobatidae) in Ariake Bay. Japan Environ. Biol. Fish. 85, 289–298. doi: 10.1007/s10641-009-9487-2
Kyne, P. M., and Bennett, M. B. (2002). Reproductive biology of the eastern shovelnose ray, Aptychotrema rostrata (Shaw & Nodder, 1794), from Moreton Bay, Queensland, Australia. Mar. Freshw. Res. 53, 583–589. doi: 10.1071/MF01063
Kyushu Regional Agricultural Administration Office (1964–2013). Annual Report of Statistics on Agriculture, Forestry and Fisheries. Japan: Ministry of Agriculture Forestry and Fisheries of Japan.
Lamilla, J. G., Pequeño, G. R., and Kong, I. U. (1995). Dasyatis brevis (Garman, 1880) segunda especie de Dasyatidae registrada para Chile (Chondrichthyes, Myliobatiformes). Estud. Oceanol. 14, 23–27.
Last, P. R., and Stevens, J. D. (2009). “Family Myliobatidae,” in Sharks and Rays of Australia, Second Edition, eds P. R. Last and J. D. Stevens (Australia: CSIRO Publishing), 465–472.
Last, P. R., White, W. T., de Carvalho, M. R., Séret, B., Stehmann, M. F. W., and Naylor, G. J. P. (2016). Rays of the World. Australia: CSIRO publishing.
Lutton, B. V., St George, J., Murrin, C. R., Fileti, L. A., Callard, I. P., and Hamlett, W. C. (2005). “The elasmobranch ovary,” in Reproductive Biology and Phylogeny of Chondrichthyes: Sharks, Batoids and Chimaeras, Vol. 3, ed. W. C. Hamlett (Enfield, NH: Science Publishers), 237–281.
Marshall, A. D., and Bennett, M. B. (2010). Reproductive ecology of the reef manta ray Manta alfredi in southern Mozambique. J. Fish Biol. 77, 169–190. doi: 10.1111/j.1095-8649.2010.02669.x
Marshall, L. J., White, W. T., and Potter, I. C. (2007). Reproductive biology and diet of the southern fiddler ray, Trygonorrhina fasciata (Batoidea: Rhinobatidae), an important trawl bycatch species. Mar. Freshw. Res. 58, 104–115. doi: 10.1071/MF05165
Martin, L. K., and Cailliet, G. M. (1988a). Aspects of the reproduction of the bat ray, Myliobatis californica, in central California. Copeia 1988, 754–762. doi: 10.2307/1445398
Martin, L. K., and Cailliet, G. M. (1988b). Age and growth determination of the bat ray, Myliobatis californica Gill, in central California. Copeia 1988, 762–773. doi: 10.2307/1445399
Martins, A. P. B., Heupel, M. R., Chin, A., and Simpfendorfer, C. A. (2018). Batoid nurseries: definition, use and importance. Mar. Ecol. Prog. Ser. 595, 253–267. doi: 10.3354/meps12545
Maruska, K. P., Cowie, E. G., and Tricas, T. C. (1996). Periodic gonadal activity and protracted mating in elasmobranch fishes. J. Exp. Zool. 276, 219–232. doi: 10.1002/(SICI)1097-010X(19961015)276:3<219::AID-JEZ6>3.0.CO;2-Q
Masaki, K., and Onohara, T. (2003). Condition of Fisheries and Distribution of Ark Shell, Scapharca sativa in the Saga Portion of the Ariake Sound. Bull. Saga Prefect. Ariake Fish. Res. Dev. Cent. 21, 29–36.
McEachran, J. D., and Seret, B. (1990). “Myliobatididae,” in Check-List of the Fishes of the Eastern Tropical Atlantic (CLOFETA), eds J. C. Quéro, J. C. Hureau, C. Karrer, A. Post, and L. Saldanha (Paris: UNESCO), 67–70.
Mendonça, S. A., Macena, B. C. L., Creio, E., Viana, D. L., Viana, D. F., and Hazin, F. H. V. (2012). Record of a pregnant Mobula thurstoni and occurrence of Manta birostris (Myliobatiformes: Mobulidae) in the vicinity of Saint Peter and Saint Paul Archipelago (Equatorial Atlantic). J. Aqua. Sci. 7, 21–26.
Menon, M., Maheswarudu, G., Ramulu, K. S., and Kizhakudan, S. J. (2020). Reproductive biology and diet of the longtail butterfly ray Gymnura poecilura (Shaw, 1804) along western Bay of Bengal. J. Mar. Biol. Assoc. U.K. 100, 461–470. doi: 10.1017/S0025315420000259
Ministry of the Environment (2017). The Japanese Red Lists on marine fishes. Available online at: http://www.env.go.jp/press/files/jp/106403.pdf [accessed on May 17, 2021]
Musick, J. A. (1999). “Ecology and conservation of long-lived marine animals,” in Life in the Slow Lane: Ecology and Conservation of Long-Lived Marine Animals American Fsheries Society Symposium 23, ed. J. A. Musick (Bethesda, MD: American Fisheries Society), 1–10.
Myers, R. A., Baum, J. K., Shepherd, T. D., Powers, S. P., and Peterson, C. H. (2007). Cascading effects of the loss of apex predatory sharks from a coastal ocean. Science 315, 1846–1850. doi: 10.1126/science.1138657
Neer, J. A., and Thompson, B. A. (2005). Life history of the cownose ray, Rhinoptera bonasus, in the northern Gulf of Mexico, with comments on geographic variability in life history traits. Environ. Biol. Fish. 73, 321–331. doi: 10.1007/s10641-005-2136-5
Notarbartolo-di-Sciara, G. (1988). Natural history of the rays of the genus Mobula in the Gulf of California. Fish. Bull. 86, 45–66.
Notarbartolo-di-Sciara, G., and Serena, F. (1988). Term embryo of Mobula mobular (Bonnaterre, 1788) from the northern Tyrrhenian Sea. Atti Soc. Ital. Sci. Nat. Mus. Civ. Stor. Nat. Milano 129, 396–400.
Nowicki, R. J., Thomson, J. A., Fourqurean, J. W., Wirsing, A. J., and Heithaus, M. R. (2021). Loss of predation risk from apex predators can exacerbate marine tropicalization caused by extreme climatic events. J. Anim. Ecol. 90, 2041–2052. doi: 10.1111/1365-2656.13424
Ogino, Y., Furumitsu, K., Kiriyama, T., and Yamaguchi, A. (2019). Using optimised otolith sectioning to determine the age, growth and age at sexual maturity of the herbivorous fish Kyphosus bigibbus: with a comparison to using scales. Mar. Freshw. Res. 71, 855–867. doi: 10.1071/MF19231
Pacoureau, N., Rigby, C. L., Kyne, P. M., Sherley, R. B., Winker, H., Carlson, J. K., et al. (2021). Half a century of global decline in oceanic sharks and rays. Nature 589, 567–571. doi: 10.1038/s41586-020-03173-9
Pérez-Jiménez, J. C. (2011). Biología reproductiva de la raya Rhinoptera bonasus (Elasmobranchii) en el sureste del Golfo de México. Hidrobiológica 21, 159–167.
Portnoy, D. S., Piercy, A. N., Musick, J. A., Burgess, G. H., and Graves, J. E. (2007). Genetic polyandry and sexual conflict in the sandbar shark, Carcharhinus plumbeus, in the western North Atlantic and Gulf of Mexico. Mol. Ecol. 16, 187–197. doi: 10.1111/j.1365-294X.2006.03138.x
Poulakis, G. R. (2013). Reproductive biology of the cownose ray in the Charlotte Harbor estuarine system, Florida. Mar. Coast. Fish. 5, 159–173. doi: 10.1080/19425120.2013.795509
Poulakis, G. R., and Grier, H. J. (2014). Ontogenetic testicular development and spermatogenesis in rays: the cownose ray, Rhinoptera bonasus, as a model. Environ. Biol. Fish. 97, 1013–1029. doi: 10.1007/s10641-014-0267-2
Pratt, J. H. L. (1988). Elasmobranch gonad structure: a description and survey. Copeia 1988, 719–729. doi: 10.2307/1445394
Ramírez-Amaro, S. R., González-Barba, G., Galván-Magaña, F., and Cartamil, D. (2013). First record of abnormal cephalic horns in the California bat ray Myliobatis californica. Mar. Biodivers. Rec. 6, 1–4. doi: 10.1017/S1755267213000146
Randall, J. E. (1977). Contribution to the biology of the whitetip reef shark (Triaenodon obesus). Pac. Sci. 31, 143–164.
Rangel, B. S., Rodrigues, A., and Moreira, R. G. (2018). Use of a nursery area by cownose rays (Rhinopteridae) in southeastern Brazil. Neotrop. Ichthyol. 16:e170089. doi: 10.1590/1982-0224-20170089
Ricker, W. E. (1975). Computation and interpretation of biological statistics of fish populations. Bull. Fish. Res. Board Canada 191, 1–382.
Rigby, C. L., Derrick, D., Dyldin, Y. V., Herman, K., Ishihara, H., Jeong, C.-H., et al. (2021). Aetobatus narutobiei. The IUCN Red List of Threatened Species 2021. Available online at: https://www.iucnredlist.org/species/104021947/104021988 [accessed on April 22, 2021]
Rosa-Molinar, E., Williams, C. S., and Collard, S. (1983). Fetal mummification in the sandbar shark, Carcharhinus plumbeus (Nardo, 1827). J. Wildl. Dis. 19, 156–158. doi: 10.7589/0090-3558-19.2.156
Sandoval-Castillo, J., and Villavicencio-Garayzar, C. (2008). Fetal mummification in silky shark (Carcharhinus falciformis) from the Gulf of California, Mexico. Braz. Arch. Biol. Technol. 51, 551–554. doi: 10.1590/S1516-89132008000300015
Schluessel, V., Bennett, M. B., and Collin, S. P. (2010). Diet and reproduction in the white-spotted eagle ray Aetobatus narinari from Queensland, Australia and the Penghu Islands, Taiwan. Mar. Freshw. Res. 61, 1278–1289. doi: 10.1071/MF09261
Seck, A. A., Diatta, Y., Gueye-Ndiaye, A., and Capapé, C. (2002). Observations on the reproductive biology of the Bull Ray, Pteromylaeus bovinus (E. Geoffroy Saint-Hilaire, 1817)(Chondrichthyes: Myliobatidae) from the coast of Senegal (eastern tropical Atlantic). Acta Adriat. 43, 87–96.
Simpfendorfer, C. A. (1992). Reproductive strategy of the Australian sharpnose shark, Rhizoprionodon taylori (Elasmobranchii: Carcharhinidae), from Cleveland Bay, northern Queensland. Mar. Freshw. Res. 43, 67–75. doi: 10.1071/MF9920067
Simpfendorfer, C. A., and Milward, N. E. (1993). Utilisation of a tropical bay as a nursery area by sharks of the families Carcharhinidae and Sphyrnidae. Environ. Biol. Fish. 37, 337–345. doi: 10.1007/BF00005200
Smith, J. W., and Merriner, J. V. (1986). Observations on the reproductive biology of the cownose ray, Rhinoptera bonasus, in Chesapeake Bay. Fish. Bull. 84, 871–877.
Snelson, F. F. J., Williams-Hooper, S. E., and Schmid, T. H. (1988). Reproduction and ecology of the Atlantic stingray, Dasyatis sabina, in Florida coastal lagoons. Copeia 1988, 729–739. doi: 10.2307/1445395
Swider, D. A., Corwin, A. L., Kamerman, T. Y., Zimmerman, S. L., Violetta, G. C., Davis, J., et al. (2017). “Reproduction of spotted eagle rays, Aetobatus narinari, in aquaria,” in The Elasmobranch Husbandry Manual II: Recent Advances in the Care of Sharks, Rays and their Relatives, eds M. Smith, D. Warmolts, D. Thoney, R. Hueter, M. Murray, and J. Ezcurra (Columbus, OH: Ohio Biological Survey, Inc), 433–442.
Tagliafico, A., Ehemann, N., Rangel, M. S., and Rago, N. (2016). Exploitation and reproduction of the bullnose ray (Myliobatis freminvillei) caught in an artisanal fishery in La Pared, Margarita Island, Venezuela. Fish. Bull. 114, 114–152. doi: 10.7755/FB.114.2.2
Tagliafico, A., Rago, N., Rangel, S., and Mendoza, J. (2012). Exploitation and reproduction of the spotted eagle ray (Aetobatus narinari) in the Los Frailes Archipelago, Venezuela. Fish. Bull. 110, 307–316.
Takita, T., and Yamaguchi, A. (2009). Fishes in estuarine and tidal flat ecosystems. Status of endangered fishes in Ariake Sound. Japan: Tokai University Press.
Tıraşın, E. M., and Başusta, N. (2018). Near-term embryos and gravid females of Lusitanian cownose ray (Rhinoptera marginata) in Mersin Bay, eastern Mediterranean Sea. Mar. Freshw. Res. 69, 1365–1371. doi: 10.1071/MF17356
Tomita, T., Toda, M., Ueda, K., Uchida, S., and Nakaya, K. (2012). Live-bearing manta ray: how the embryo acquires oxygen without placenta and umbilical cord. Biol. Lett. 8, 721–724. doi: 10.1098/rsbl.2012.0288
Uchida, S., Toda, M., and Kamei, Y. (1990). “Reproduction of elasmobranchs in captivity,” in Elasmobranchs as living resources: advances in the biology, ecology, systematics, and the status of the fisheries NOAA Technical Report NMFS 90, eds J. H. L. Pratt, S. H. Gruber, and T. Taniuchi (Seattle, WA: U.S. Department of Commerce), 211–237.
Villavicencio Garayzar, C. J. (1996). Tallas, proporción de sexos y reproducción de Myliobatis califomica y M. longirostris (Pisces: Myliobatidae) en Baja California Sur, México. Rev. Biol. Trop. 43, 291–295.
Waltrick, D., Awruch, C., and Simpfendorfer, C. (2012). Embryonic diapause in the elasmobranchs. Rev. Fish Biol. Fish. 22, 849–859. doi: 10.1007/s11160-012-9267-5
White, W. T., and Dharmadi. (2007). Species and size compositions and reproductive biology of rays (Chondrichthyes, Batoidea) caught in target and non-target fisheries in eastern Indonesia. J. Fish Biol. 70, 1809–1837. doi: 10.1111/j.1095-8649.2007.01458.x
White, W. T., Furumitsu, K., and Yamaguchi, A. (2013). A new species of eagle ray Aetobatus narutobiei from the Northwest Pacific: an example of the critical role taxonomy plays in fisheries and ecological sciences. PLoS One 8:e83785. doi: 10.1371/journal.pone.0083785
White, W. T., Giles, J., and Dharmadi, and Potter, I. C. (2006). Data on the bycatch fishery and reproductive biology of mobulid rays (Myliobatiformes) in Indonesia. Fish. Res. 82, 65–73. doi: 10.1016/j.fishres.2006.08.008
White, W. T., Hall, N. G., and Potter, I. C. (2002). Reproductive biology and growth during pre- and postnatal life of Trygonoptera personata and T. mucosa (Batoidea: Urolophidae). Mar. Biol. 140, 699–712. doi: 10.1007/s00227-001-0756-7
White, W. T., and Moore, A. B. M. (2013). Redescription of Aetobatus flagellum (Bloch & Schneider, 1801), an endangered eagle ray (Myliobatoidea: Myliobatidae) from the Indo-West Pacific. Zootaxa 3752, 199–213. doi: 10.11646/zootaxa.3752.1.12
Wourms, J. P. (1977). Reproduction and development in chondrichthyan fishes. Am. Zool. 17, 379–410. doi: 10.1093/icb/17.2.379
Wyffels, J. T. (2009). “Embryonic development of chondrichthyan fishes—a review,” in Development of Non-Teleost Fishes, eds Y. W. Kunz, C. A. Luer, and B. G. Kapoor (Enfield, NH: Science Publishers), 1–103.
Yamaguchi, A. (2004). Abnormal specimens of Dasyatis akajei from Japan Sea, Myliobatis tobijei and Aetobatus fragellum from Ariake Sound. Rep. Japan. Soc. Elasmobranch Stud. 40, 44–45.
Yamaguchi, A., Kawahara, I., and Ito, S. (2005). Occurrence, growth and food of longheaded eagle ray, Aetobatus flagellum, in Ariake Sound, Kyushu, Japan. Environ. Biol. Fish. 74, 229–238. doi: 10.1007/s10641-005-0217-0
Yamaguchi, A., and Kume, G. (2009). Reproductive biology of the fanray, Platyrhina sinensis (Batoidea: Platyrhinidae) in Ariake Bay, Japan. Ichthyol. Res. 56, 133–139. doi: 10.1007/s10228-008-0078-6
Yamaguchi, A., and Kume, G. (2011). Treasurable shallow water of Ariake Sea as the cradle for fishes. Sci. J. Kagaku 81, 446–449.
Yamaguchi, A., Taniuchi, T., and Shimizu, M. (1997). Reproductive biology of the starspotted dogfish Mustelus manazo from Tokyo Bay, Japan. Fish. Sci. 63, 918–922. doi: 10.2331/fishsci.63.918
Yamaguchi, A., Taniuchi, T., and Shimizu, M. (2000). Geographic variations in reproductive parameters of the starspotted dogfish, Mustelus manazo, from five localities in Japan and in Taiwan. Environ. Biol. Fish. 57, 221–233. doi: 10.1023/A:1007558324902
Yokota, L., Goitein, R., Gianeti, M. D., and Lessa, R. T. P. (2012). Reproductive biology of the smooth butterfly ray Gymnura micrura. J. Fish Biol. 81, 1315–1326. doi: 10.1111/j.1095-8649.2012.03413.x
Yokota, L., and Lessa, R. P. (2006). A nursery area for sharks and rays in Northeastern Brazil. Environ. Biol. Fish. 75, 349–360. doi: 10.1007/s10641-006-0038-9
Keywords: elasmobranch, Myliobatidae, viviparous, trophonemata, fecundity, nursery area, uterus, estuary ecosystem
Citation: Yamaguchi A, Furumitsu K and Wyffels J (2021) Reproductive Biology and Embryonic Diapause as a Survival Strategy for the East Asian Endemic Eagle Ray Aetobatus narutobiei. Front. Mar. Sci. 8:768701. doi: 10.3389/fmars.2021.768701
Received: 01 September 2021; Accepted: 15 November 2021;
Published: 21 December 2021.
Edited by:
David M. P. Jacoby, Lancaster University, United KingdomReviewed by:
Michelle S. Passerotti, National Marine Fisheries Service (NOAA), NEFSC Narragansett Laboratory, United StatesNatascha Wosnick, Federal University of Paraná, Brazil
Copyright © 2021 Yamaguchi, Furumitsu and Wyffels. This is an open-access article distributed under the terms of the Creative Commons Attribution License (CC BY). The use, distribution or reproduction in other forums is permitted, provided the original author(s) and the copyright owner(s) are credited and that the original publication in this journal is cited, in accordance with accepted academic practice. No use, distribution or reproduction is permitted which does not comply with these terms.
*Correspondence: Atsuko Yamaguchi, eS1hdHN1a29AbmFnYXNha2ktdS5hYy5qcA==