- 1Centro de Física Aplicada y Tecnología Avanzada, Universidad Nacional Autónoma de México, Santiago de Querétaro, Mexico
- 2División de Ciencias Ambientales, Instituto Potosino de Investigación Científica y Tecnológica, San Luis Potosí, Mexico
- 3Instituto de Ingeniería, Universidad Nacional Autónoma de México, Mexico City, Mexico
- 4Unidad Académica de Sistemas Arrecifales–Puerto Morelos, Instituto de Ciencias del Mar y Limnología, Universidad Nacional Autónoma de México, Puerto Morelos, Mexico
- 5Facultad de Ciencias Naturales, Universidad Autónoma del Carmen, Ciudad del Carmen, Mexico
- 6Consejo Nacional de Ciencia y Tecnología, Universidad Autónoma del Carmen, Ciudad del Carmen, Mexico
- 7Facultad de Derecho, Universidad Autónoma de Querétaro, Santiago de Querétaro, Mexico
- 8Creamos Más S.A. de C.V., Guadalajara, Mexico
The recent periodic influx of massive quantities of pelagic Sargassum spp. (sargasso) into the Caribbean has posed ecological, social, and economic challenges to the region. Sustainable use of the biomass is crucial to mitigate negative impacts of beached algae. The current uses of sargasso in Mexico are reviewed, and a biorefinery approach is proposed to optimize its commercialization. The commercialization potential of sargasso in the Mexican Caribbean is analyzed using the strengths, weaknesses, opportunities, and threats (SWOT) analysis. The unpredictability of the influx to the Mexican shores is considerable, both in time and space, also, some areas receive consistently more sargasso than others. The lack of available technology, local infrastructure and regulations concerning sargasso are relevant, as is the urgent need to establish proper regulatory measures. In the context of the Mexican legal system, the category “special waste” can best be applied to sargasso that is collected from the beach or nearshore waters for the purpose of regulation. Lessons learnt from in Mexico may be applied elsewhere, with adaptations for each specific location. More importantly, mutual understanding of the constraints and possibilities of sargasso management in other countries, or territories, may facilitate the coordinated management of this transboundary macroalgae bloom.
Introduction
Mexico has an Exclusive Marine Economic Zone of 3.15 million km2 (Silva et al., 2014). Bioprospecting, i.e., the systematic search for natural components or organisms for the development of products, with applications in the pharmaceutical, biotechnological, agronomical, cosmetic, or bioremediation industries (Bhatia and Chugh, 2015), is amongst the economic activities in this zone. Such bioprospecting has been carried out on sixteen seaweed species found along the Mexican Atlantic coast (Ortega et al., 2001); however, pelagic Sargassum spp. (sargasso from now on) were not amongst them; as until 2014, its landings were sporadic, and of low to moderate biomass (Godínez-Ortega et al., 2021). The origin of the sargasso landings used to be the Sargasso Sea [see Figure 1, modified from Putman and He (2013)], but from 2011 the Great Atlantic Sargassum Belt began to form. Although the total estimated biomass in this new area of sargasso concentration was small at first (less than three million wet tons in total), it has increased over the years, reaching a maximum of 20 million wet tons of seaweed in June 2018 (Wang et al., 2019; see Figure 1).
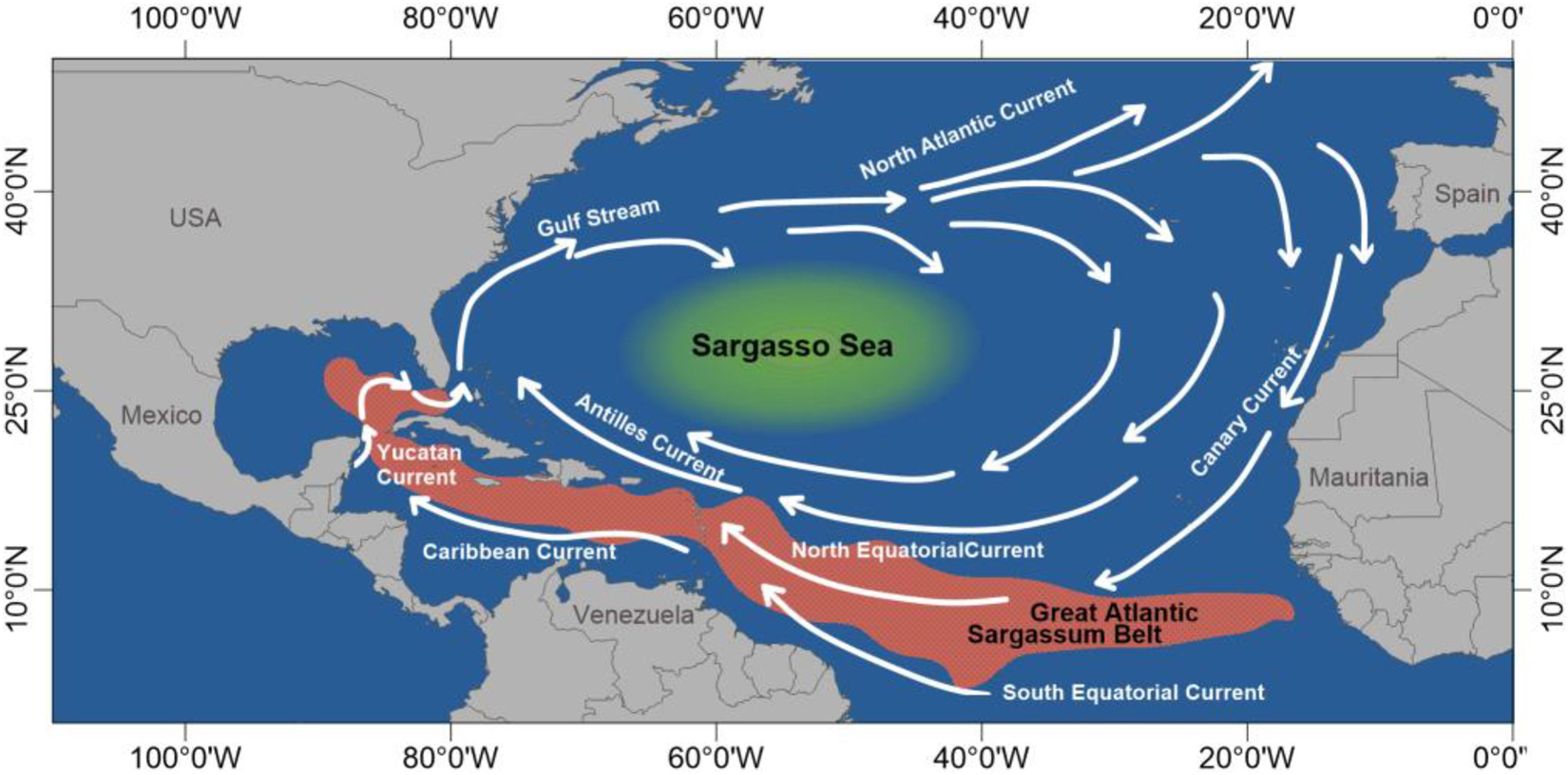
Figure 1. Major currents in the North Atlantic, the Sargasso Sea, and the Great Atlantic Sargassum Belt.
The periodic arrival of millions of tons of sargasso masses on the shores of the Caribbean has caused alterations in the environment (Smetacek and Zingone, 2013; van Tussenbroek et al., 2017; Chávez et al., 2020), with social and economic consequences that have affected the tourist industry in particular (Chávez et al., 2020). The increasingly massive influxes arriving on the Mexican coast could be seen as an opportunity to develop products with added-value. Initially, there was uncertainty as to whether the influxes of sargasso would recur; at present it is generally accepted that periodic massive sargasso inundations on the shores is the new “normal,” and that adaptation and mitigation strategies must be developed (e.g., Oxenford et al., 2021), including the commercialization of sargasso. The hypothetical commercial value of sargasso is becoming increasingly recognized, and many local communities throughout the Caribbean are becoming aware of its remarkable potential (Desrochers et al., 2020). Bioprospecting and subsequent commercialization of sargasso could potentially cover the costs of removing it near to the shore or on beaches, thereby mitigating their impacts on the environment (Silva et al., 2016; Chávez et al., 2020; Robledo et al., 2021; Thompson et al., 2021).
The systematic search for economically valuable products is based on the scientific study of biological samples. This “added-value approach” goes alongside developments in laws on intellectual property rights, environmental protection (e.g., to avoid overexploitation), new bio-rational approaches for specimen collection, as well as product development (Scott, 2001). The ocean is currently being “re-discovered” for bioprospecting, attracting stake holders who were previously oblivious to the sea. Although the ocean has been extensively used for a range of purposes throughout human history, it is still often perceived as “no man’s land,” or “Mare Nullius,” with resources “for free” (Bennett et al., 2021). Marine, or blue, biotechnology is becoming a blue bioeconomy, which includes prospecting for macro-algae, such as sargasso (Golberg et al., 2020).
The sargasso bloom that created the Great Atlantic Sargassum Belt affects many countries across and territories the wider Caribbean, equatorial Brazil, and part of the west coast of Africa. However, the severity and nature of the environmental, and socio-economic impacts differ according to the geographic region (UNEP-CEP, 2021). The Caribbean has numerous political entities, varying from small island states to larger territories with considerable stretches of coastline; these territories also have different levels of development as well as different levels of dependence on resources from the sea, such as livelihood/sustenance, fishing industries, or tourism. The UNEP-CEP (2021) identified that the socio-economic impacts of massive sargasso beaching depend on:
(1) The quantities arriving, according to geomorphology and exposure;
(2) Assets or resources present in the impacted areas, such as tourist infrastructure or natural resources, such as reefs;
(3) The capacity to mobilize resources to mitigate the massive beaching events.
The Mexican Caribbean has a coastline of >800 km, which has received sargasso yearly average volumes of 3.2 × 103 and 1.7 × 103 m3/km/month in the peak years, 2018 and 2019, respectively (Chávez et al., 2020). In this manuscript, we describe the massive beaching, harvesting, and commercialization efforts in Mexico, together with thoughts on the legal constraints and possibilities for the regulation of these efforts. It is hoped that the experiences in this part of Mexico may be adapted and applied elsewhere. Together with an improved understanding of the constraints and possibilities of sargasso management in other countries would facilitate coordinated management of this transboundary bloom.
Distribution of Sargasso in Waters Along the Mexican Caribbean Coast
Cost-effective management of sargasso demands spatially explicit information on where this alga mostly accumulates for efficient collecting, and for optimizing storage and primary processing, which ideally takes place near areas with regular mass landings, to reduce transport costs. We used remote sensing techniques to obtain the spatial distribution of frequent sargasso landings on a regional scale.
Satellite images were used to observe sargasso accumulations along the Mexican Caribbean coastline, from Cancun to the Sian Ka’an Natural Reserve. The sargasso cover was estimated in hexagonal lattices of 2 km diameter, using Landsat 8 OLI imagery from 2016 to 2019. The presence of sargasso was detected following Cuevas et al. (2018), with a temporal resolution of 16 days between images. The prevalence (number of days that sargasso accumulations were detected in a hexagon as a percentage of the total number of observed days in that hexagon), cumulative sum (the sum of hectares covered by satellite where sargasso was detected in a hexagon, considering all the processed images from 2016 to 2019), and variability of sargasso (standard deviation of the satellite detected sargasso cover in a hexagon over the study time) were calculated for each of the hexagons. Jenks-natural breaks method was used to classify the hexagons in terms of sargasso presence (Table 1).

Table 1. Statistics for multivariate classification based on the prevalence, variability, and total area covered by sargasso from 2016 to 2019, along the northern coast of the Mexican Caribbean.
The central part of the coastline studied, between Tulum and Puerto Morelos including Cozumel Island, almost always had large aggregations of sargasso during the periods of influx [usually from March until October (García-Sánchez et al., 2020)], although the variability in the amounts was high (class 4, red areas in Figure 2B). This part of the coastline which receiving most sargasso represents ≈20% of the coastline, with a length of ≈90 km. The remaining ≈80% of the coastline studied received smaller volumes of sargasso, but with a high prevalence and low variability (class 2, orange areas in Figure 2B), distributed in a relatively narrow strip (30 km width). Further offshore, medium-size patches with low prevalence (class 3, purple areas in Figure 2B) were detected, with a certain concentration in the sea to the west and southwest of Cozumel Island. Finally, class 1 (light yellow areas in Figure 2B) relates mainly to oceanic waters (but also includes a small part of the coast, south of Tulum) where large accumulations of sargasso do not occur. Most sargasso further offshore is probably transitory and does not reach the Mexican shore. Thus, open ocean harvesting of sargasso in the northern part of the Mexican Caribbean may be undesirable; the sargasso does not form large concentrations in fixed areas, and they are likely to stay in the ocean to be carried away, north, by the Yucatan Current.
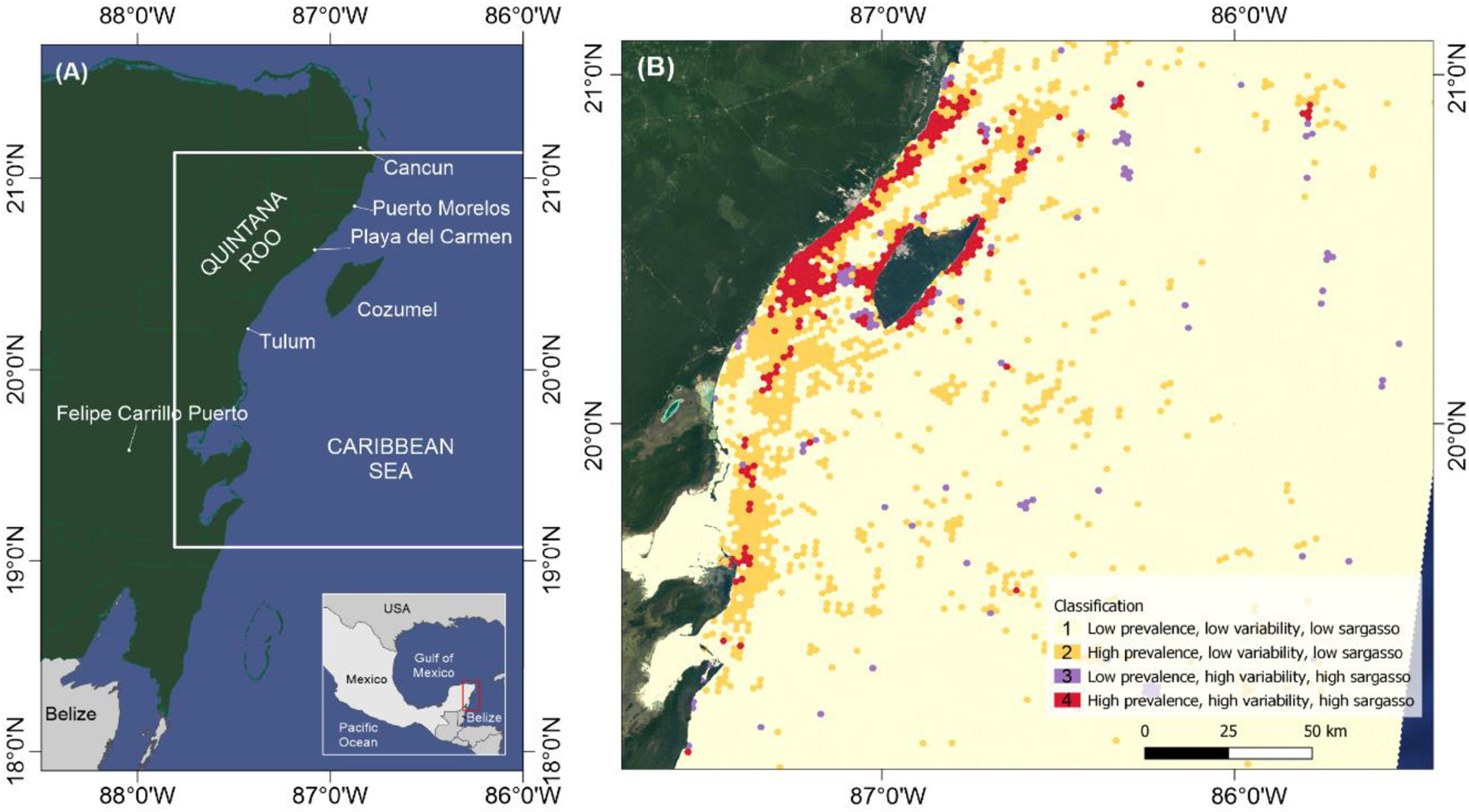
Figure 2. (A) The Mexican Caribbean and (B) map showing the classification of sargasso, based on LandSat satellite data from 2016 to 2019.
Harvesting
The large variability in the sargasso landings poses a challenge for near-shore harvesting or collection from the beaches. Planning for seasonal variability may be possible to a certain degree, although the enormous interannual variations mean that planning and logistics for the acquisition, temporal storage and maintenance of machinery, as well as the contracting of workers, is more difficult. For example, the interannual irregularity of the influx produces maintenance costs for equipment in non-sargasso years, which should be included in determining viable business scenarios. Oxenford et al. (2021) have already stated that the irregular supply of sargasso is a major challenge for the valorization of sargasso on an industrial scale. The overall amount of sargasso available as a raw material for industrial development cannot be guaranteed. To overcome the irregular supply and provide a supply of the raw material in years of low influx, harvested sargasso could be stored (at a cost) or sargasso could be cultivated (at a higher cost, probably).
Beach Collection
In Mexico, maintenance of the public beaches; including the collection of beached sargasso is the responsibility of the corresponding municipalities. Thus, the jurisdictional responsibility of the beached sargasso depends on the municipality and state government. Sargasso and its epibionts (Mendoza-Becerril et al., 2020), may contain heavy metals, such as arsenic (Rodríguez-Martínez et al., 2020) or other toxins [e.g., chlordecone, (Devault et al., 2021a)], but only if their concentrations are excessively high (which has yet to be established), can sargasso be considered hazardous, and as such become subject to federal regulations. Individual hotels are responsible for the maintenance of beach sections for which they have been granted concessions, which includes keeping it free from sargasso. The photographs in Figure 3 show sargasso collection along the 400 m long beach in front of a hotel in Puerto Morelos (20°49.7′N 86°53.6′W; see Figure 2A). Table 2 shows the sargasso collection costs for this hotel, through 2019 (Lucena, 2020), which give an idea of the temporal variability of the quantity of beached sargasso in the area and the costs involved. From January to July, the collection was possible without a tractor, given the small amount of beached sargasso.
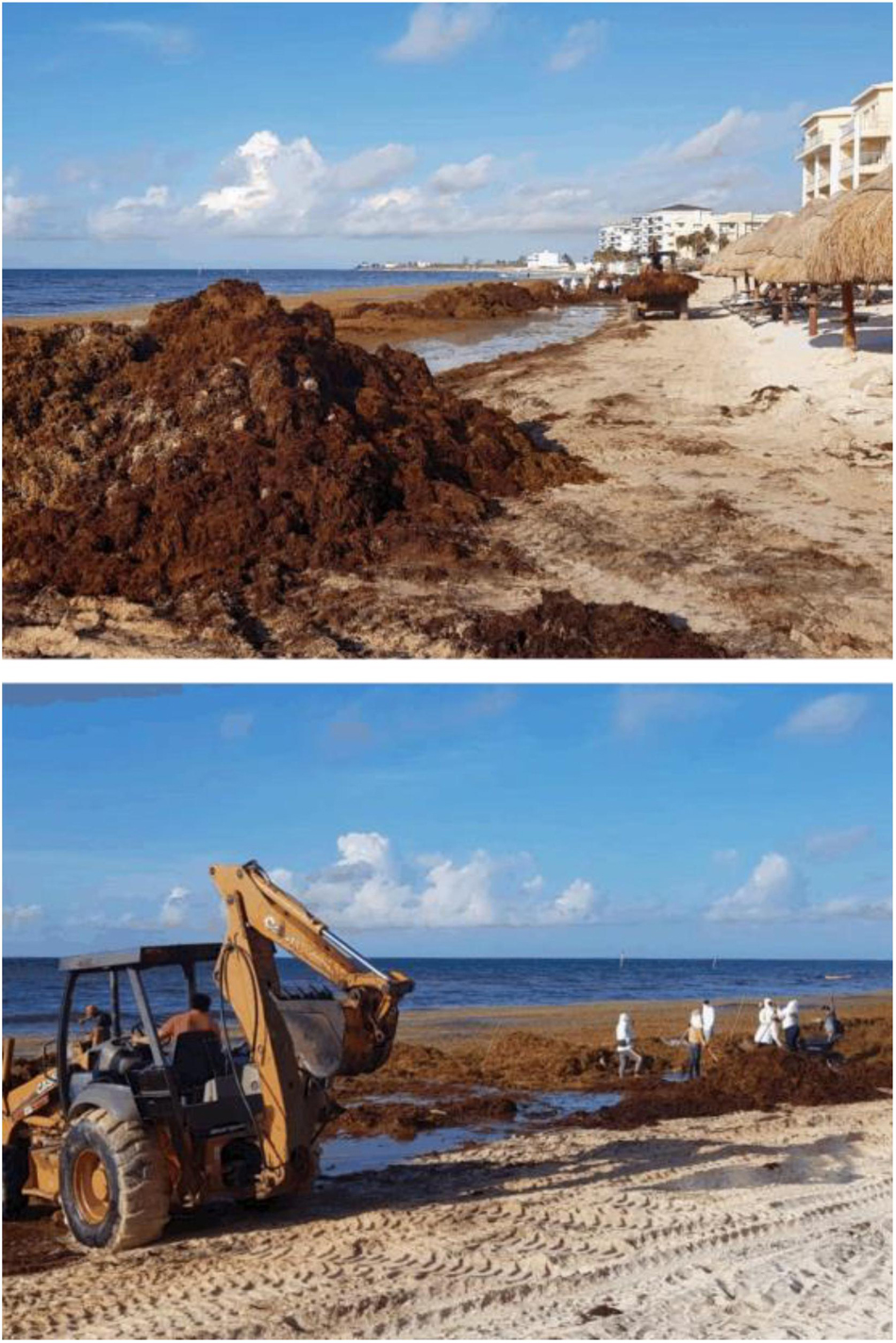
Figure 3. Sargasso collection at Puerto Morelos (20°49.7′N 86°53.6′W, see Figure 2A), June 2019.
Harvesting Sargasso in Nearshore Waters
Beached sargasso masses have a negative visual impact, cause damage to the nearshore ecosystems and beaches (van Tussenbroek et al., 2017), and if left to decompose, has an unpleasant smell, releasing corrosive gasses that may be a danger to public health (Chávez et al., 2020). The containment and collection of sargasso in nearshore and off-shore waters is a means of avoiding these undesirable effects. Companies such as Grupo Dakatso de México, S.A. de C.V. (Dakatso, 2020) offer these services in the Mexican Caribbean. The sargasso is monitored with satellite images and drones in a 2 km radius area, to keep that area free of beached sargasso (Dakatso, 2021a). Various types of barriers are used to contain or redirect the sargasso (Figure 4A), depending on local conditions. Small vessels with a sweeping system are employed to collect the sargasso detained by the barriers (Figure 4B), collecting up to 15 wet tons of sargasso from the barriers, or 7 wet tons of dispersed sargasso. The collected sargasso is then transferred to an authorized site for trituration (with permission is authorized by the Mexican Ministry of the Environment, when the site complies with the General Law for the Prevention and Comprehensive Management of Waste). The cost of renting barriers and maintenance, monitoring, collection, and trituration of the sargasso by Grupo Dakatso de México, S.A. de C.V is approximately $1,800,000.00 MXN (≈$87,500.00 USD) per km of protected coastline per month.
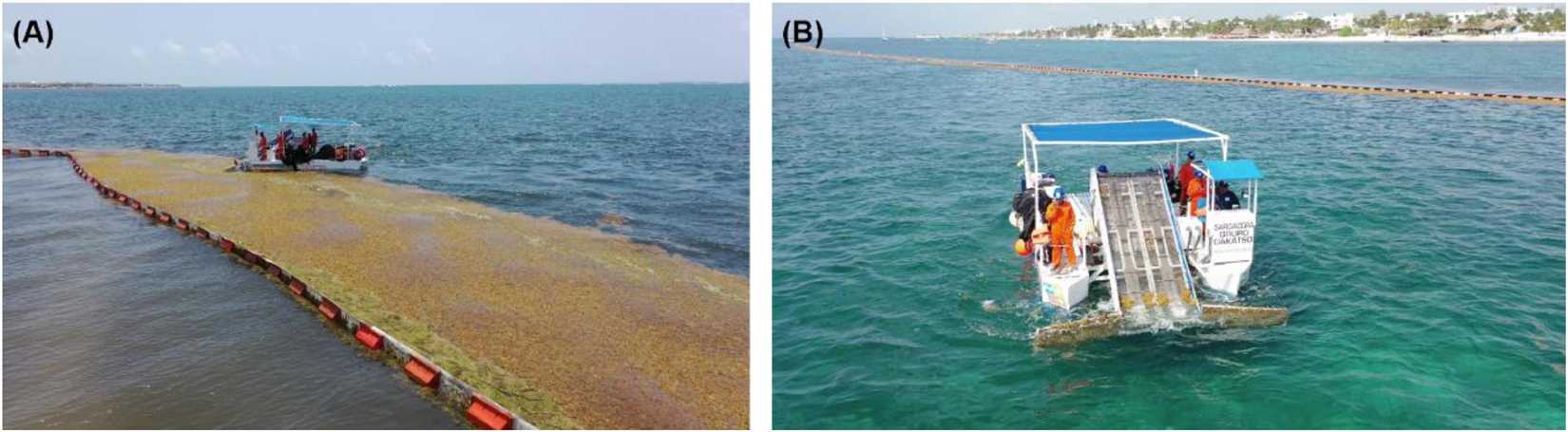
Figure 4. Barriers contain the sargasso (A) and it is collected at sea (B). Photos courtesy of Grupo Dakatso S.A. de C.V.
Explored Uses of Sargasso in Mexico
Alginates and Fucoidans
Polysaccharides in the cell walls of all brown algae, including Sargassum species (which include pelagic sargasso and benthic species) prevent the algae from drying out and also provide strength and flexibility (Puspita et al., 2020). The most abundant polysaccharide in sargasso is alginate (Liu et al., 2012), which has applications in the pharmaceutical and food industries, where they are used as thickeners and stabilizers; their viscous consistency is due to their water absorption capacity (Jesumani et al., 2019). Alginates extracted from other Sargassum spp. have shown anticancer and antiviral properties (de Sousa et al., 2007; Sinha et al., 2010), but regular intake of these alginates can bring health risks with alterations to the kidneys and liver. Studies on animals have demonstrated that the consumption of certain alginates causes hyperplasia in the spleen, glomerular and tubular hemorrhage in the kidney, and degeneration and hemorrhages in the liver (de Sousa et al., 2007).
The term alginate refers to alginic acid (sodium salts, calcium, and magnesium). Sodium salts have the highest solubility, and their extraction consists of transforming all types of alginate salts into sodium salt to facilitate their dilution in an aqueous solution (Fertah, 2017). For this, two conversions are employed: first, the alginate salts are converted to alginic acid by acid pre-treatment; and secondly, the alginic acid (in insoluble form) is converted into sodium alginate (soluble) by alkaline extraction. This allows the precipitation of the fibers and finally the solubilized sodium alginate is dried (Hernández-Carmona et al., 2012; Fertah, 2017). During these processes, parameters such as temperature, reaction time, and the percentage of aqueous ethanol must be controlled (Mazumder et al., 2016; Mohammed et al., 2020). Alkaline extraction at high temperatures (above 80°C) decreases the yield of sodium alginate due to the breaking of uric acid chains, resulting in lower viscosities (Mohammed et al., 2018). Longer reaction time improves the penetration of the liquid into the algal biomass and thereby the dilution and release of the alginate (Mazumder et al., 2016). Mazumder et al. (2016) report that the alginate yield increases with aqueous ethanol percentages of 70–100%. Alginate extraction is carried out in Mexico by Creamos Más S.A. de C.V. (Creamos_Más, 2020) using the procedure shown in Figure 5.
All Sargassum species also have a relatively high contents of fucoidans, also called fucans, which are polysaccharides containing fucose and sulfate (Sanjeewa et al., 2018), with potential antiviral, anticancer, antioxidant, anti-inflammatory, antimicrobial, and anticoagulant properties (Thinh et al., 2013; Sanjeewa et al., 2018). The bioactive properties of fucoidans are attributed to the content and position of sulfate and vary with the size and geometry of the molecule. According to Sinha et al. (2010), fucoidans from Sargassum tenerrimum can inhibit the binding and penetration of host cells by viruses, although the mechanism involved is unknown. Fucoidan has low toxicity and can therefore be used for the production of antiviral drugs. The anticancer activity of fucoidan has been determined in animal studies, which show that they can reduce the size (weight) of tumors. The anticoagulant properties of fucoidan depend on the degree of sulfating and may be higher than that of heparin, one of the most widely used anticoagulant drugs (Jesumani et al., 2019). Fucoidan is extracted from ground algae that are suspended in the buffer solution, followed by a precipitation process using ethanol (after the elimination of the alginates, using CaCl2) with optimal conditions of 60°C, and a pH of 4. Sinurat et al. (2016) filtered and centrifuged macerated dried Sargassum binderi with CaCl2 as a solvent, subsequently employing ethanol for the precipitation of fucoidan, yielding 4.02% and total sulfate content of 10.90%. Hifney et al. (2016) used a hot buffer process, obtaining fucoidan yields of 19.00% and sulfate content of 47.60%. It is important to note that specific compositions of fucoidan can be obtained depending on the conditions of the algae (e.g., wet or dry), the heterogeneity and structural complexity of the species, as well as the part of the plant, the season, and the extraction method used (Eluvakkal et al., 2010; Zayed and Ulber, 2019). Although fucoidan has promising applications in medicine, further studies into its effectiveness, potential cytotoxicity, and other secondary effects are needed. The extraction of fucoidan is carried out in Mexico by Creamos Más S.A. de C.V. (Creamos_Más, 2020) following the procedure depicted in Figure 6.
Complementary Animal Feed
Like all Sargassum species, sargasso is high in carbohydrates, essential amino acids, vitamins, minerals, and beta-carotene (Carrillo Domínguez et al., 2002; Casas-Valdez et al., 2006), which makes it suitable as (complementary) animal feed. Three studied benthic Sargassum species in Mexico have high percentage of carbohydrates (39%), but low crude protein (8%); in addition, they contain vitamin compounds, such as retinol and ascorbic acid with concentrations of 821.50 and 46.25 mg per 100 g of Sargassum spp., respectively (Casas-Valdez et al., 2006). The flour obtained from benthic Sargassum species has been fed to chickens, reducing the cholesterol in hens and increasing their egg quality (Meza, 1998). Casas-Valdez et al. (2006) evaluated Sargassum spp. as a complementary feed for goats in experiments with 25% of the flour from Sargassum spp. The results were similar when fed either on the usual feed, or the sargasso-based feed. These studies based on benthic Sargassum spp. have established that these algae provide large amounts of minerals that can be easily digested by animals (Gojon-Báez et al., 1998). However, it has yet to be established whether sargasso from the Mexican Caribbean has similar results; in addition, the high arsenic content found in Caribbean sargasso (Rodríguez-Martínez et al., 2020) must be addressed before implementing its use as an animal feed. The presence of epiphytic communities (Salter et al., 2020), mostly calcifying hydroids (Mendoza-Becerril et al., 2020), should also be considered before using sargasso as livestock feed, as they may alter its nutritional composition.
Complementary Fertilizer
Sargasso is rich in essential minerals, including potassium, but has low nitrogen and phosphorous contents and can thus be used as a complementary fertilizer. Sargasso can be applied directly, like manure, compost, or chemical extract. Table 3 shows the composition analysis of sargasso collected in February 2019. The samples are of Sargassum fluitans III, Sargassum natans I, and S. natans VIII, and the relative contribution of each species/morphological form is highly variable (García-Sánchez et al., 2020); therefore, the chemical composition of mixed algal masses may vary accordingly.
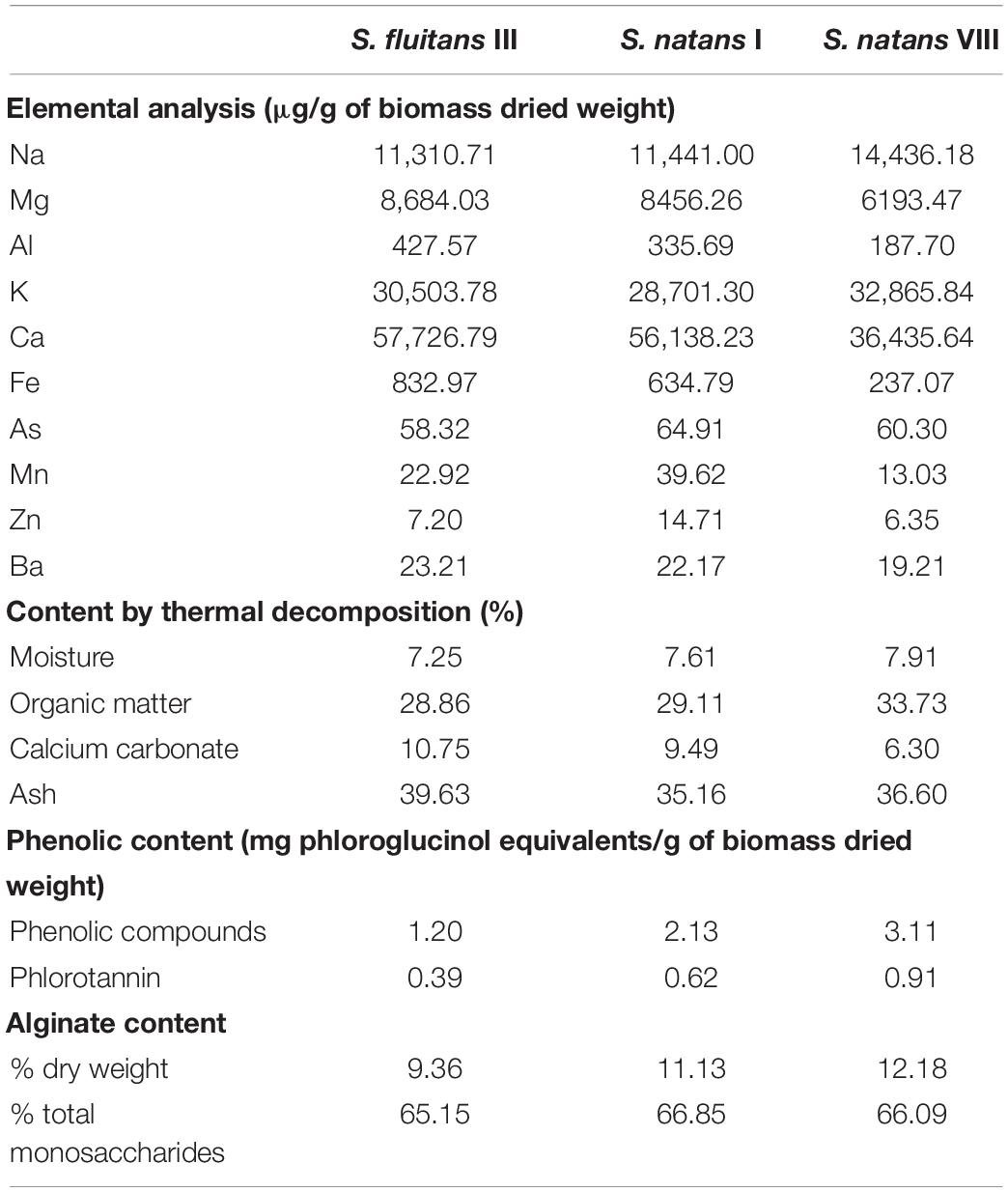
Table 3. Chemical composition of the different Sargassum species (Davis et al., 2021).
Extracts taken from Sargassum species have been used in crop production as foliar applications, soil drenches, or seed treatments (Kalaivanan and Venkatesalu, 2012; Mohanty et al., 2013). The concentration of sargasso can be controlled within the varying doses supplied (Mohanty et al., 2013). Sargasso extracts promote crop growth and yield (Anantharaj and Venkatesalu, 2002), increases the weight of the roots, the number of fruits, and the percentage of total soluble solids (Sutharsan et al., 2014). Liquid fertilizers also accelerate the growth of various parts of the plants, such as stems, leaves, flowers, and fruits (Kumar et al., 2012). Solid sargasso fertilizer includes the preparation of sargasso flour or the direct application of thalli to the soil (Kumar et al., 2012; Thompson et al., 2020b). In Mexico, the companies Salgax (2019), Dianco (2021), and C-Combinator (2021) have developed products based on algae, including sargasso. The high salt and arsenic contents of sargasso are issues which must be addressed when it is to be used as a complementary fertilizer.
Biofilters and Biosorbent Materials
The alginates in the cell walls of sargasso are porous and can absorb various polluting compounds (Saldarriaga-Hernandez et al., 2020); therefore, they can be used as a medium for the biosorption of metallic ions and organic dyes (Mahmood et al., 2017; Hannachi and Hafidh, 2020). Biosorption is a simple, safe, and inexpensive technique to remove toxic substances (Kanamarlapudi et al., 2018; Elgarahy et al., 2021). Certain metal ions can cause a range of problems in the environment, which in high concentrations can affect flora and fauna. As a biosorbent, sargasso can remove lead, mercury, zinc, and copper ions through physisorption, microprecipitation, ion exchange, and chemisorption (Robalds et al., 2016). In physisorption, cations are trapped on the biosorbent surface due to electrostatic and Van der Waals attractive forces. Microprecipitation is a crystallization process caused by the reaction between the metal ions and the polymers of the substrate. In ion exchange, the ions from sargasso are replaced by metal ions with similar charges. Finally, chemisorption is the result of the chemical chelation between the metal ions and the organic ligands of the substrate (Calderón et al., 2020; Singh et al., 2020). Besides metals, toxic, organic dyes, used in the textile and paper industry, can also be removed through biosorption (e.g., Hannachi and Hafidh, 2020; López-Miranda et al., 2020). If these substances are not removed and reach the environment via wastewater discharge, alterations may occur in ecosystems.
In Mexico, sargasso-based biofilters have recently been developed to remove contaminants in aqueous solutions (López-Miranda et al., 2020). Figure 7 shows the prototype used, details of the design, and construction of the filter. Figure 7A shows the tubular element of the filter housing. The water inlet and outlet are at the bottom and top of the tube, respectively. Figure 7B shows the cartridge inside the casing, which is made up of layers of approximately 7 g of dried processed sargasso. The processing consisted of placing the sargasso in a 10% hydrogen peroxide solution for 2 h, and then drying it in the shade in order to expose chemical bonds and increase the removal capacity. At the end of each of these parts is a nylon mesh preventing the sargasso from dragging. Removal efficiency is determined by the quality of the sargasso, the concentration of the contaminant, the inflow of the aqueous solution, the area of the biofilter, and the distribution of the sargasso within the filter.
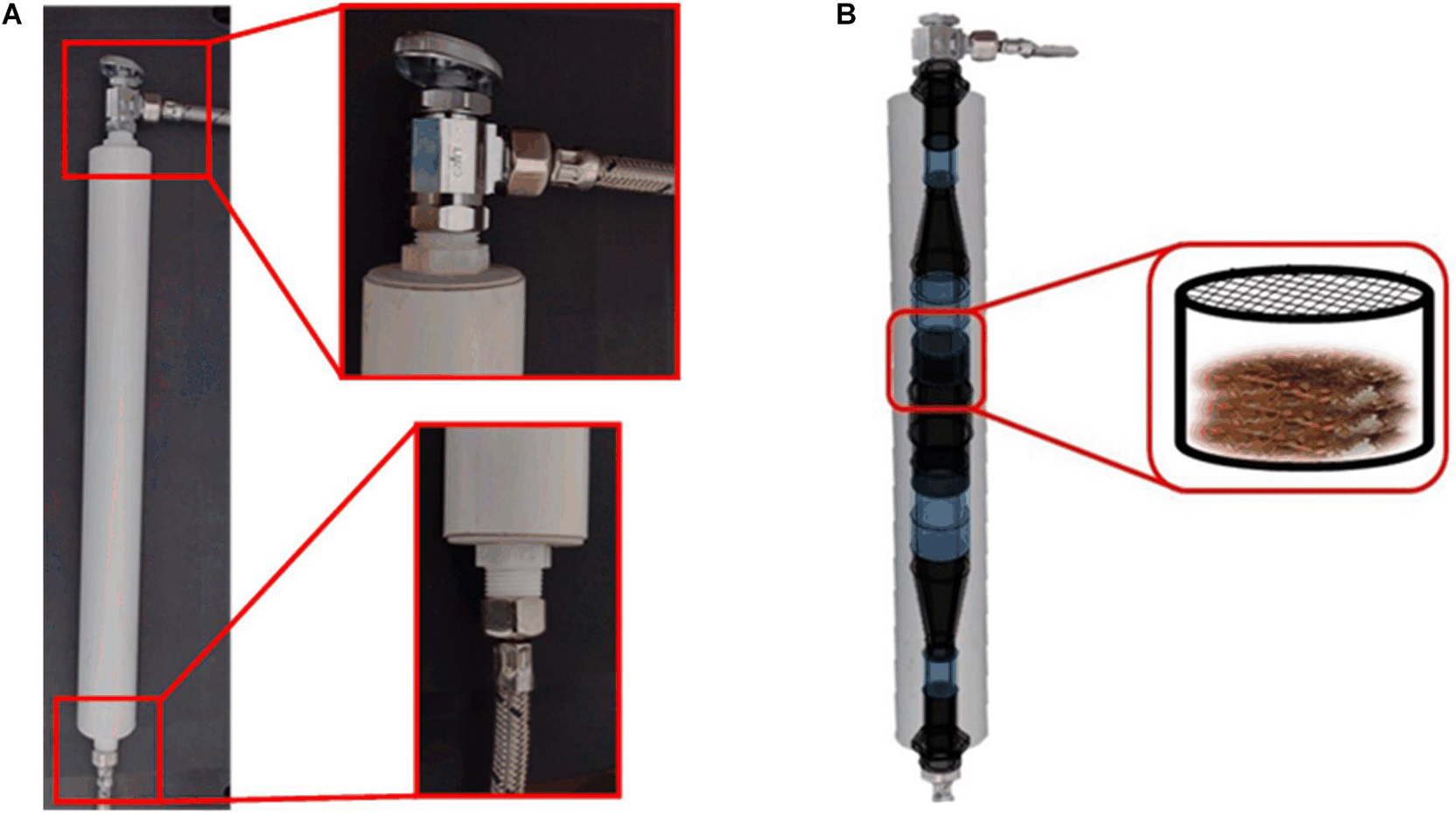
Figure 7. Filter prototype for testing the removal of contaminants: (A) design of filter housing and (B) filter cartridge containing sargasso.
López-Miranda et al. (2020) showed that these biofilters remove methylene blue (100%), methyl red (36%), and methyl orange (65%), when applied in 4–6 ppm concentrations. The removal capacity of lead in solution is also high (96%) and is constant for up to 5 L of contaminated water; thus, the volume of water that can be treated in this way is >700 L per kg of dried sargasso. This type of biofilter can be used in both domestic and industrial applications.
Construction Materials
In Mexico, the Blue & Green company (Blue-Green, 2018) produced the first bricks made of sargasso, called a “sargablock.” The first house using sargablock has already been built, made of 40% dry sargasso. It is estimated that a 40 m2 construction requires 2,150 blocks, and 20 wet tons of sargasso. Studies carried out by the Secretariat of Ecology and Environment of Quintana Roo state, Mexico, found that the resistance of the bricks is 75–120 kgf/cm2, while its durability can be up to 120 years, regardless of the region or climate type where they are used (Desrochers et al., 2020). Currently there is no other sustainable material as resistant, or with such a long useful life. Sargabricks are therefore seen as a viable and safe material for the creation of ecofriendly buildings.
Another application for the construction industry is “sargacreto,” produced by Grupo Dakatso S.A. de C.V., Mexico (Dakatso, 2021b); sargasso mixed with concrete, to give it a more attractive esthetic appearance. The sargasso is dehydrated then mixed with a 60% concrete concentration. It can be used to build sidewalks, blocks, joists, and vaults. As yet there are no detailed studies on the properties and characteristics of the blocks made out of sargacreto.
For both sargablocks and sargacreto, further studies are required to test the environment around such constructions for Sick Building Syndrome, indoor air quality, acoustics, and radiation (Joshi, 2008).
Other Products
A Mexican-based company, Renovare (2021) designed eco-friendly shoes. For each sole 100 g of dried, crushed sargasso is used, while the upper part of the shoe is made from recycled plastic bottles. Sargasso is also locally used by craftspeople who mix it with resin to make plant pots, vases and ornaments.
Paper can be made from the cellulose in sargasso, thus reducing the demand for trees in paper production. According to a patent (Nicolucci and Monegato, 1996), paper made from algae is manufactured through an 8 stage process (Figure 8). In Mexico today, the Salgax (2019) and Sarganico (2021) companies make various paper and cardboard products from sargasso.
A Proposal for a Sargasso Biorefinery
A biorefinery is a facility for processing biomass in a sustainable way to obtain a spectrum of high added-value products and bioenergy (Balina et al., 2017). The products with the highest added-value are obtained first, and the solid residues generated at the end of the process are used for the production of bioenergy, such as biogas and biochar. The aim of this cascade approach is that waste from the biorefinery is minimal (Rajak et al., 2020).
Brown seaweeds, including sargasso, are generally rich in polysaccharides (30–50% dry weight), and contain practically no lignin (Yanagisawa et al., 2013), in contrast to lignocellulosic biomass that comes from plants (Zoghlami and Paës, 2019), making extracting the polysaccharides easier. This feature, along with the faster growth rates of seaweeds compared to plants, has positioned macroalgae as an appropriate feedstock for biorefineries. The trend is to develop new and simple bioprocesses that allow a combination of high added-value products to be recovered, not just one product. For example, a fucoidan, with health benefits and nutritional benefits, could be placed in the market as one product (Lange et al., 2020). To achieve this, new biotechnologies specifically designed for macroalgae must be developed.
In a sargasso-based biorefinery at least two conversion processes could operate, depending on the end products it seeks to deliver: chemical conversion to extract alginates and fucoidans, and biological conversion to produce bioenergy. Operational units such as grinding, heating, decanting, solvent extraction, and centrifuging would be indispensable for extracting the value-added products in the first step, and the final solid residue could be used for biogas production (Flórez-Fernández et al., 2021). Another option could be to extract the sugars through saccharification and use them for other purposes (Davis et al., 2020), such as ethanol production; in that case, a fermentation step is required, and the operational units necessary for recovering ethanol. In this option, an anaerobic digester would also be necessary to convert the final residues into biogas (Figure 9). Although the total carbohydrate content of sargasso could be around 11% (Desrochers et al., 2020), the composition of the polysaccharides may differ between morphotypes of S. natans and S. fluitans (Davis et al., 2020). Saccharification, using the enzymes Cellic CTec2 (developed for lignocellulosic biomass) and a generic amyloglucosidase, releases galactose, glucose, and mannose from sargasso (Davis et al., 2020), which are key intermediaries for producing biofuels. Various companies or consortia in Mexico are exploring biorefinery of sargasso, such as Creamos Más S.A. de C.V. (Creamos_Más, 2020) and Metco (2021). Currently, in Mexico, there are no reports of integrated biorefineries based on sargasso that produce and commercialize high-added value products, biofuels, and other side products such as animal feed, plant stimulants, and soil improvement products.
Products of High Added-Value
Alginates (9–40% dry weight) and fucoidans (5–20%) can be used in the pharmaceutical, cosmetic and food industries for their properties as emulsifiers, stabilizers, and in the case of fucoidans as skin protectants (Priyan Shanura Fernando et al., 2019), and are thus high added-value products. The antioxidant properties of sargasso make it an attractive material for developing cosmetic products (Sanjeewa et al., 2018). The various pigments it contains can also protect the skin against UV rays (Kim et al., 2013). In addition, it has a high vitamin content, making it interesting as a raw material for skincare products (Jesumani et al., 2019). The polysaccharides in sargasso also inhibit collagenase and elastase activity in the skin; proteins are responsible for the deterioration of the skin, making it attractive in the manufacture of anti-aging products (Jesumani et al., 2019).
Phenolic compounds, mainly phlorotannins, are bioactive components of brown seaweeds with antioxidant, bactericidal, and anticancer properties (Li et al., 2011). At present, in Mexico, phenolic compounds are not extracted from sargasso for commercialization, but this may be possible in a biorefinery. Phenolic compounds have been reported in various species of Sargassum (Puspita et al., 2020), whereas Davis et al. (2020) determined the content of phenolic compounds in sargasso (S. natans and S. fluitans), using the methodology of Folin-Ciocalteu. They found that S. natans contained up to 3.10 mg/g of dry biomass and S. fluitans 1.20 mg/g of dry biomass. However, variations in the phenolic content are probably great, depending on the trajectory of the seaweed, as well as the collection, transport, and storage conditions. The enzymatic extracts (Viscozyme) of Sargassum muticum contained 6% of phenolic compounds (dry weight) and showed free radical scavenging activity vs. 2,2-diphenyl-1-picrylhydrazyl (DPPH) and ferric reducing antioxidant power; the extract did not show antibacterial activity but avoided the formation of biofilms of Pseudomonas aeruginosa and Escherichia coli (Puspita et al., 2017). The anticancer activity of phlorotannins from brown seaweeds has not reached clinical trials yet; nonetheless, several works highlight the anticancer role of phlorotannins in vitro and in vivo (Erpel et al., 2020). Currently, there are no reports showing the antioxidant, bactericidal, or anticancer properties of sargasso extracts, but bioprospecting for components with such properties may be promising, in a biorefinery approach.
Bioenergy
Biogas
Biogas is produced from the anaerobic digestion of organic biomass; typically, 50–70% of the gas produced is methane, and 30–50% is CO2 (Angelidaki et al., 2019). The biomethane potential of brown seaweeds is between 200 and 380 L CH4/kg volatile solids (VS), which is generally lower (<50%) than the theoretical potential and higher than lignocellulosic biomass (100–260 L CH4/kg VS), for example. This low yield is attributed to the complexity of the polysaccharides, including the degree of polymerization (alginates) and the sulfur content (fucoidans), as well as the concentration of polyphenols and salinity (Thompson et al., 2019). It is also important to consider that the biogas produced must be upgraded to increase the biomethane content. In addition to CO2 and CH4, raw biogas may contain low concentrations of hydrogen sulfide, water vapor, and NH3, among other gases. These compounds, along with CO2, reduce the calorific value of the biogas and need to be removed before it can be used as fuel (biomethane content >95%). Several physicochemical methods can be used to remove CO2, including water- and organic-scrubbing, absorption, adsorption, and membrane separation (Angelidaki et al., 2019).
Information on biogas production from solid sargasso residues, following the extraction of high added-value compounds, is limited. At laboratory scale, the biomethane potential from the anaerobic digestion of the solid residue biomass (0.15 L CH4/kg total solids or TS) and fresh biomass of Sargassum mucticum (0.18 L CH4/kg TS) were very similar (Flórez-Fernández et al., 2021). Both biomasses were subjected to washing, grinding, freezing, and thawing; the solid residue was obtained after autohydrolysis at 150–170°C, to extract alginates, fucoidans, and phenolic compounds. Surprisingly, the biomethane potential was slightly higher after the organic compounds were extracted, but anaerobic digestion of alginates has resulted in low biomethane production yields (Milledge et al., 2019). Overall, the work of Flórez-Fernández et al. (2021) on S. muticum supports the biorefinery approach for obtaining a combination of high added-value products and bioenergy from sargasso, rather than using it only to produce biogas (a single product approach). Other authors have also stated that the only way that biofuel production from macroalgae would be economically attractive is to also produce marketable, high added-value products (Song et al., 2015; IEA-Bioenergy, 2017; Kumar et al., 2018; Rajak et al., 2020).
Even without a biorefinery, or if the added-value products are not economically feasible due to seasonality, the sargasso biomass can still be used to produce biogas. The main advantage of sargasso as feedstock for anaerobic digestion is its low or zero lignin content (Konda et al., 2015). However, pre-treatments are necessary to break the cell wall structure and release the organic matter. The most promising pre-treatment for biogas production from sargasso is hydrothermal pre-treatment, where the biomass is heated with hot water or steam at 100–180°C. Using hydrothermal pre-treatment (140°C, 30 min) with a severity factor of 2.65, the biomethane potential reached 116 L CH4/kg VS in contrast to 41 L CH4/kg VS for non-pretreated pelagic S. fluitans (Thompson et al., 2020a). The severity factor relates to the extent of a reaction based on the temperature and time; this factor predicts deconstruction and polymer yield of complex biomass during saccharification (de Farias Silva and Bertucco, 2018). Another pre-treatment applied to sargasso in Mexico to improve the biomethane potential is using the fungus Trametes hirsuta and its enzymatic extract (Tapia-Tussell et al., 2018). The biological pre-treatment with the fungus yielded a biomethane potential of 104 L CH4/kg VS, which is 21% higher than the sargasso exposed only to the fungus enzymatic extract and 28% higher than the non-pretreated sargasso.
Bioethanol
The main challenge in transforming sargasso into bioethanol is converting the variety of carbohydrates into fermentable sugars that not only include glucose, but also other hexoses (galactose, mannose) and pentoses (xylose) (Devault et al., 2021b). Pre-treatment to deconstruct the biomass, while assuring the release of polysaccharides, is needed. This can be achieved by hydrothermal processes, steam explosion, wet oxidation, and simply milling (Harun et al., 2013). The next step is to convert the polysaccharides into monosaccharides, which can be achieved by thermochemical processes (dilute acid or alkali) and using mild temperatures (50–100°C), avoiding the formation of inhibitory compounds, such as furfurals. An alternative is enzymatic saccharification; however, the efficiency is suboptimal due to their low specificity as some enzymes are designed for lignocellulosic materials. Enzymes obtained from marine sources, such as alginate lyases and laminarases, can be used for saccharification, but have low efficiencies (Torres et al., 2019). After obtaining the monosaccharides, fermentation can be carried out using Saccharomyces cerevisiae, Pichia stipitis, or Pachysolen tannophilus.
For the brown alga Laminaria digitata, hydrolyzed with acid and enzymes after fermentation with S. cerevisiae the bioethanol yield reached 94% (Kostas et al., 2017), whereas Sargassum species had a yield of 65–85% after pre-treating with dilute acid (Borines et al., 2013). As far as we know, no fermentation processes using sargasso for bioethanol production are currently applied in Mexico. The challenge will be to achieve a greater degree of hydrolysis conversion from polysaccharides to monosaccharides, and to find, or even design through metabolic engineering, more flexible yeasts that use glucose and the other sugars found in the hydrolyzates.
Legal Frame Work for Valorization of Sargasso
In the evolving regulations concerning natural resources and waste in Mexico, three legal perspectives are possible concerning the valorization of sargasso: (1) as a natural resource (focusing on the ecosystem services it provides); (2) as a raw material (focusing on its extraction and transformation); (3) as waste (unclaimed beached sargasso, i.e., once beached, or destined to arrive on a beach).
When sargasso is considered a natural resource, found in the sea, it should be regulated by constitutional provisions [e.g., (DOF, 2021a, b, c, d), see Supplementary Material 1], under the federal jurisdiction of the Secretaría de Marina (the Mexican Navy). When it is considered a raw material, the Comisión Nacional de Pesca (the Ministry of Fisheries) should be the federal body with the responsibility of overseeing its exploitation.
Before sargasso can be employed as a raw material for products, it needs to be stored temporally. When it is not to be used for any purpose, it must be disposed of properly. The state government has the legal responsibility for the temporal storage or disposal of sargasso, unless it is considered as a hazardous waste. Sargasso contains heavy metals [e.g., arsenic (Rodríguez-Martínez et al., 2020)] and other toxins [e.g., chlordecone, (Devault et al., 2021a)]; if these levels are excessively high, sargasso may be considered hazardous waste and becomes subject to federal regulations. The federation would also be responsible for the (decaying) beached sargasso, which at present is cleaned by municipalities and concessionaries of the beach sections (see above). However, this potential toxicity should be further studied; although the concentrations of toxic elements may be too high for certain uses, they may be below the levels of hazardous waste.
To regulate beached sargasso as a potential resource (when collected from the beach or nearshore waters with barriers), it is best categorized as a “waste requiring special treatment” from a legal perspective, even though it may appear contradictory to consider a potential resource as a waste. The main problem in viewing beached sargasso as waste, is that the regulations concerning its management stipulate that the generator is the owner of the waste, and since no specific individual/legal entity is identified as owning or responsible for the generation of sargasso, it must be seen as “orphan waste.” As such, the Mexican federation is responsible for the regulation of this waste in all its facets: from the management of its contamination effects and potential damage to human and ecosystem health to establishment of comprehensive, circular management processes, including valorization.
Therefore, a National Strategy for the Use of Sargasso must be developed, to handle the processing cycle of sargasso from a regenerative approach, using biopolitical indicators founded on scientific evidence, rather than relying on purely administrative decisions (Pantoja, 2020, 2021). Examples of such biopolitical indicators are the climatological models and indicators of environmental vulnerability (e.g., to droughts and floods), implemented to assign microcredits from green funds to compensate for climate change, and the program for the temporary regularization of migrants from countries affected by natural disasters in the United States (Bolaños Guerra, 2018).
Challenges for the Sustainable Use of Sargasso
Recent reports (Oxenford et al., 2021; Robledo et al., 2021) have already highlighted the general challenges in the valorization, and industrialization of sargasso in the Caribbean. A strengths, weaknesses, opportunities, and threats (SWOT) analysis helps to identify the strengths, weaknesses, opportunities and threats related to the commercialization of sargasso in Mexico (Figure 10).
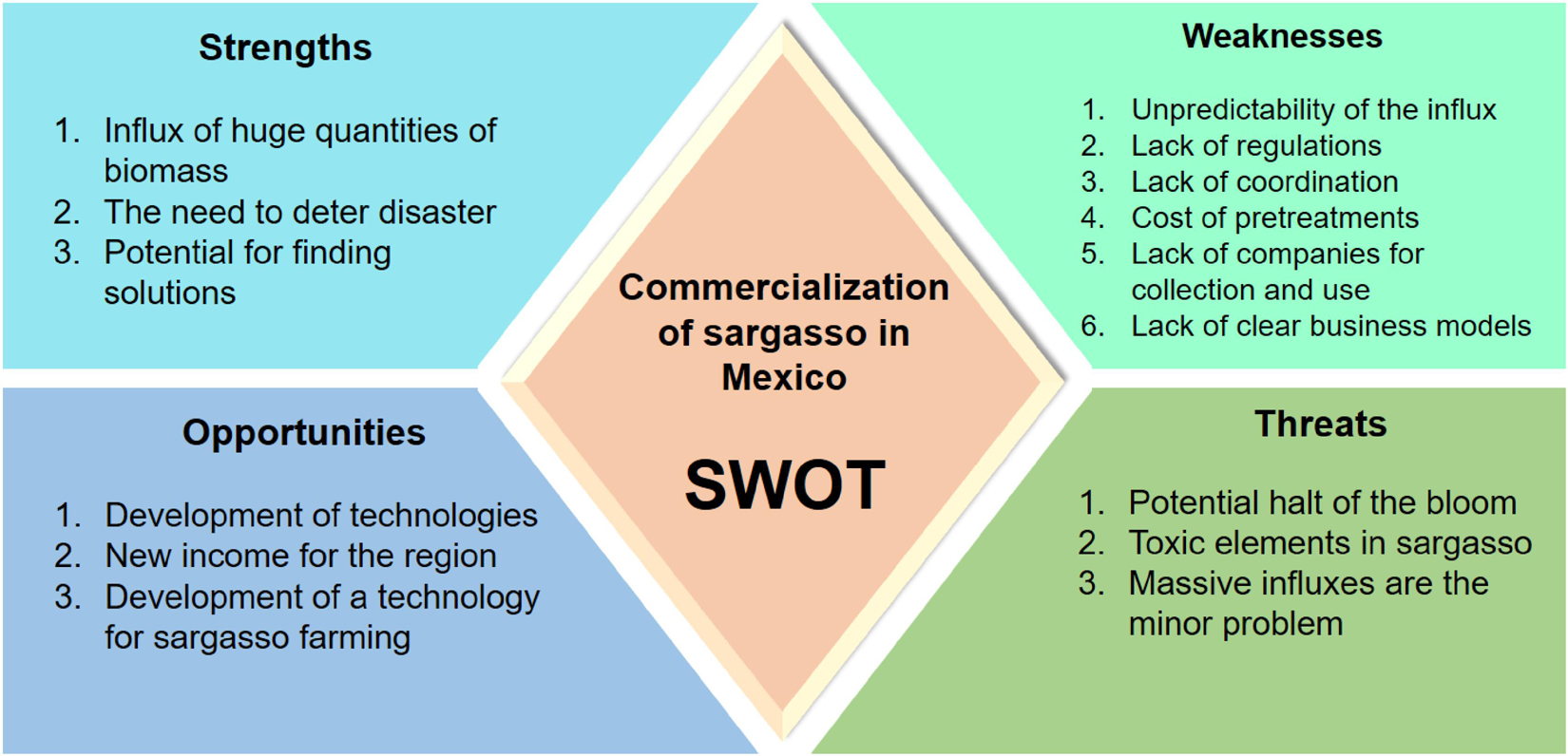
Figure 10. Analysis of strengths, weaknesses, opportunities, and threats (SWOT) related to the commercialization of sargasso in Mexico.
The strengths are: (1) the long coastline receiving enormous quantities of sargasso, which allows for valorization/commercialization on an industrial scale; (2) the need deter ecological, economic and social disaster through harvesting/collecting and disposing of sargasso in any possible way; (3) the potential of finding solutions through the ongoing development of sargasso-based products by the academic sector and entrepreneurs.
Weaknesses include: (1) the unpredictability of how much sargasso can be expected to arrive each year; (2) the lack of norms and regulations for the harvest, transport, and exploitation of sargasso. Such regulations are also essential to guarantee legal certainty for investors; (3) the lack of a comprehensive approach to the valorization of sargasso. At present there is no, or insufficient, coordination among academic institutions, industries and the (different levels of) government to promote and normalize the use of sargasso; (4) the costs of pre-treatments to enhance the properties of sargasso; (5) the lack of companies that collect and process sargasso in the Mexican Caribbean; (6) the lack of clear business models that assess whether valorization is economically viable (with, or without, the gains obtained in protecting the environment, economy and society through the collection of sargasso).
Opportunities in the commercialization of sargasso include: (1) the development of technologies and patents; creation of new “know-how” that can be passed on to other nations which face similar influxes; (2) the establishment of an alternative income for the region, as at present the economy of the Mexican Caribbean is almost entirely dependent on the conjuncture-sensitive tourism industry; and (3) the development of a technology for sargasso farming.
Threats include: (1) the uncertainty regarding the continuance of the Great Atlantic Sargasso Belt, as macro algal blooms often have at “boom-bust” cycle (Tyler et al., 2001; Human et al., 2015); however, such cycles are usually on an estuary scale, involving local nutrient recycling, whereas sargasso is drifting in the open ocean. The current consensus is that it is likely to continue, and that adaptation to periodic massive influxes is required; (2) sargasso may be unsuitable for certain uses; when it contains arsenic and/or chlordecone it should not be used in any process in the food chain (Devault et al., 2021a); (3) the factors that are thought to have caused the sargasso bloom (human-induced climate change, corresponding changes in oceanic currents, upwellings and region-wide eutrophication) may cause other environmental hazards that cannot be solved.
Toward a Circular Economy
The circular economy is defined as the economic space where the value of products, materials, and resources is maintained in the economy for as long as possible, minimizing waste (Carus and Dammer, 2018). Reusing, recycling, remanufacturing, and optimizing the durability of products is indispensable in the circular economy framework, to ensure that the loop is closed (Sverko Grdic et al., 2020). A biorefinery aims to use the renewable carbon of biomass and minimize waste and emissions (Cherubini, 2010), coming closer to a circular bioeconomy framework (Ubando et al., 2020). In addition, sargasso fixes the (excessive) carbon in the atmosphere and takes up the (excessive) nutrients in the ocean, converting them into biomass. The natural environment will improve, if this biomass is adequately retrieved and processed, avoiding the return of carbon to the atmosphere, or nutrients to the ocean. This could be achieved with a circular, cascading approach, with zero waste, as pursued in biorefineries (Torres et al., 2019), with the valorization of bio-products. Sustainability would be assessed through life cycle, and techno-economic and social-economic analyses (Ubando et al., 2020). Currently, there are no biorefineries based on the pelagic sargasso arriving in the Caribbean, but we can learn from other experiences and adopt a biorefinery approach to process sargasso in multiple products minimizing or eliminating waste.
This economic model implies systemic transformations that call for theoretical, empirical, and political reflection on the use of assets and on human rights guarantees. Renewable resources are managed in flows, and finite resources by inventory, assuming their finite nature (Pantoja, 2020). The main drivers of the circular economy are increasing price volatility, restrictions on the supply of primary resources, environmental policies and a new consumer culture. The efficiency of a circular model can be measured by the distance between the useful life of a product and its reintegration into the production process: the shorter the distance, the higher the yield, as the product is inserted in more, and varied, life cycles (EMF, 2021).
The antithesis of the circular economy is the linear economy, “the economy of consumption,” of extract, use and throw away (EMF, 2021). The linear economy ignores the finite nature of many materials, concentrating on their recovery and valorization by analyzing the life cycles of materials and resources from a regenerative perspective. If we ignore the scarcity element of resources as a fundamental element of economic science (Balmaceda, 2017), we omit activities such as conservation, natural heritage, energy efficiency, the reduction of greenhouse gases, the recovery, valorization, and recycling of its waste. In this way we have created models of excessive resource exploitation and a disproportionate generation of waste, thus destroying the capacity of the environment to regenerate or adapt to such changes (Pantoja, 2021).
The circular economy model will allow the Mexican Federal State, and particularly the state of Quintana Roo, to face the arrival of massive quantities of sargasso on the beaches with a comprehensive management of it in all its “legal states”; i.e., as a natural resource, as raw material, and as waste.
Concluding Remarks and Future Challenges
Current available scientific evidence points to massive sargasso accumulations being the new norm at present and in the future (Oxenford et al., 2021; UNEP-CEP, 2021). The trends show seasonal variability in Mexico, with the peak times for influx being between May and September (Chávez et al., 2020). Although seasonal influx patterns are to a large degree predictable, this does not apply to the enormous interannual variability in the sargasso biomass. Regarding these uncertainties, action plans must be in place to address the influxes in critical periods, as well as in intermediate periods. The many potential uses of sargasso should be considered as green solutions that also contribute to mitigating coastal squeeze (Chávez et al., 2021; Silva et al., 2021).
The results of satellite observations over recent years, strongly suggest that it is both inefficient and futile to collect sargasso in the open sea in the northern section of the Mexican Caribbean, in front of Tulum to Cabo Catoche. Although further studies are recommended as to the effectiveness of open sea collection of sargasso in the south east of the Mexican Caribbean, which could reduce the massive influxes further north.
Mexico has sufficient institutional capacity, as well as technical, scientific, industrial and marketing talent to generate effective and lasting solutions. In September 2018, an honorary Advisory Council (Consejo Técnico Asesor) was formed to comprehensively analyze the sargasso accumulation and define a scientific, technological and innovation agenda. This initiative seeks to advise the Federal and State Government, acting efficiently and promptly, with careful planning; however, a National Strategy for the use of sargasso must be developed as soon as possible, in order to manage its life cycle using a regenerative approach based on scientific evidence.
A decisive impulse toward the consolidation of a national industry for the sustainable and regulated use of this sargasso is needed now, so that Mexico can take advantage of this biotic resource, inducing a shift in the common perception of the massive influxes. Sargasso should not be seen as a threat to the economy, rather as an opportunity, a potential means of developing a greener economy alongside improving environmental preservation.
Author Contributions
RS, ME, VC, and BT contributed to conception of the study. JL and ME wrote the first draft of the manuscript. AU-M and EC performed the data analysis. LC, AU-M, EC, IR, LM, and CC-K wrote sections of the manuscript. VC, RS, and BT revised and edited the manuscript. All authors contributed to manuscript revision, read, and approved the submitted version.
Conflict of Interest
LM and CC-K are employed by Creamos Más S.A. de C.V.
The remaining authors declare that the research was conducted in the absence of any commercial or financial relationships that could be construed as a potential conflict of interest.
Publisher’s Note
All claims expressed in this article are solely those of the authors and do not necessarily represent those of their affiliated organizations, or those of the publisher, the editors and the reviewers. Any product that may be evaluated in this article, or claim that may be made by its manufacturer, is not guaranteed or endorsed by the publisher.
Acknowledgments
The authors are grateful to the Centro Mexicano de Innovación en Energía del Océano (CEMIE-Océano) funded by the CONACYT-SENER Sustentabilidad Energética project: FSE-2014-06-249795.
Supplementary Material
The Supplementary Material for this article can be found online at: https://www.frontiersin.org/articles/10.3389/fmars.2021.768470/full#supplementary-material
References
Anantharaj, M., and Venkatesalu, V. (2002). Studies on the effect of seaweed extracts on Dolichos biflorus. Seaweed Res. Utiln. 24, 129–137.
Angelidaki, I., Xie, L., Luo, G., Zhang, Y., Oechsner, H., Lemmer, A., et al. (2019). “Biogas upgrading: current and emerging technologies,” in Biofuels: Alternative Feedstocks and Conversion Processes for the Production of Liquid and Gaseous Biofuels (Second Edition), eds A. Pandey, C. Larroche, C.-G. Dussap, E. Gnansounou, S. K. Khanal, and S. Ricke (London: Elsevier), 817–843.
Balina, K., Romagnoli, F., and Blumberga, D. (2017). Seaweed biorefinery concept for sustainable use of marine resources. Energy Procedia 128, 504–511. doi: 10.1016/j.egypro.2017.09.067
Balmaceda, A. (2017). La Transición de la Unión Europea Hacia una Economía Circular. Available online at: https://www.zicla.com/blog/transicion-economia-circular-en-la-union-europea/ (accessed July 31, 2021).
Bennett, N. J., Blythe, J., White, C. S., and Campero, C. (2021). Blue growth and blue justice: ten risks and solutions for the ocean economy. Mar. Policy 125:104387. doi: 10.1016/j.marpol.2020.104387
Bhatia, P., and Chugh, A. (2015). Role of marine bioprospecting contracts in developing access and benefit sharing mechanism for marine traditional knowledge holders in the pharmaceutical industry. Glob. Ecol. Conserv. 3, 176–187. doi: 10.1016/j.gecco.2014.11.015
Blue-Green (2018). Blue & Green - Servicios y Soluciones al Medio Ambiente. Available online at: http://blue-green.com.mx/ (accessed June 1, 2021).
Bolaños Guerra, B. (2018). Biopolíticas del cambio climático para Centroamérica. Trace Trav. Rech. Amériques Centre 74, 135–158.
Borines, M. G., de Leon, R. L., and Cuello, J. L. (2013). Bioethanol production from the macroalgae Sargassum spp. Bioresour. Technol. 138, 22–29. doi: 10.1016/j.biortech.2013.03.108
Calderón, O. A. R., Abdeldayem, O. M., Pugazhendhi, A., and Rene, E. R. J. (2020). Current updates and perspectives of biosorption technology: an alternative for the removal of heavy metals from wastewater. Curr. Pollut. Rep. 6, 8–27. doi: 10.1007/s40726-020-00135-7
Carrillo Domínguez, S., Casas Valdez, M., Ramos Ramos, F., Pérez-Gil, F., and Sánchez Rodríguez, I. J. A. L. D. N. (2002). Algas marinas de Baja California Sur, México: valor nutrimental. Arch. Latinoam. Nutr. 52, 400–405.
Carus, M., and Dammer, L. (2018). The circular bioeconomy—concepts, opportunities, and limitations. Ind. Biotechnol. 14, 83–91. doi: 10.1089/ind.2018.29121.mca
Casas-Valdez, M., Hernández-Contreras, H., Marín-Álvarez, A., Aguila-Ramírez, R. N., Hernández-Guerrero, C. J., Sánchez-Rodríguez, I., et al. (2006). El alga marina Sargassum (Sargassaceae): una alternativa tropical para la alimentación de ganado caprino. Rev. Biol. Trop. 54, 83–92.
C-Combinator (2021). C-Combinator. Available online at: https://www.c-combinator.com/ (accessed June 30, 2021).
Chávez, V., Lithgow, D., Losada, M., and Silva-Casarin, R. (2021). Coastal green infrastructure to mitigate coastal squeeze. J. Infrastruct. Preserv. Resil. 2:7. doi: 10.1186/s43065-021-00026-1
Chávez, V., Uribe-Martínez, A., Cuevas, E., Rodríguez-Martínez, R. E., van Tussenbroek, B. I., Francisco, V., et al. (2020). Massive influx of pelagic Sargassum spp. on the coasts of the Mexican Caribbean 2014–2020: challenges and opportunities. Water 12:2908.
Cherubini, F. (2010). The biorefinery concept: using biomass instead of oil for producing energy and chemicals. Energy Conv. Manage. 51, 1412–1421. doi: 10.1016/j.enconman.2010.01.015
Creamos_Más (2020). Creamos Más S.A. de C.V, Mexico. Available online at: https://www.creamosmas.com/ (accessed July 21, 2021).
Cuevas, E., Uribe-Martínez, A., and Liceaga-Correa, M. (2018). A satellite remote-sensing multi-index approach to discriminate pelagic Sargassum in the waters of the Yucatan Peninsula, Mexico. Int. J. Rem. Sens. 39, 3608–3627.
Dakatso (2020). Grupo Dakatso de México S. A. de C. V. Available online at: https://www.dakatso.com.mx/ (accesed June 1, 2021).
Dakatso (2021a). Proyecto “GIS” Gestión Integral del Sargazo. Available online at: https://www.dakatso.com.mx/proyecto-gis/ (accessed June 10, 2021).
Dakatso (2021b). Sargacreto Mexico. Available online at: https://sargacreto.com.mx/ (accessed August 10, 2021).
Davis, D., Simister, R., Campbell, S., Marston, M., Bose, S., McQueen-Mason, S. J., et al. (2021). Biomass composition of the golden tide pelagic seaweeds Sargassum fluitans and S. natans (morphotypes I and VIII) to inform valorisation pathways. Sci. Total Environ. 762:143134. doi: 10.1016/j.scitotenv.2020.143134
Davis, D., Simister, R., Campbell, S., Marston, M., Bose, S., McQueen-Mason, S. J., et al. (2020). Biomass composition of the golden tide pelagic seaweeds Sargassum fluitans and S. natans (morphotypes I and VIII) to inform valorisation pathways. Sci. Total Environ. 762:143134.
de Farias Silva, C. E., and Bertucco, A. (2018). Severity factor as an efficient control parameter to predict biomass solubilization and saccharification during acidic hydrolysis of microalgal biomass. BioEnergy Res. 11, 491–504. doi: 10.1007/s12155-018-9913-4
de Sousa, A. P. A., Torres, M. R., Pessoa, C., de Moraes, M. O., Rocha Filho, F. D., Alves, A. P. N. N., et al. (2007). In vivo growth-inhibition of Sarcoma 180 tumor by alginates from brown seaweed Sargassum vulgare. Carbohydr. Polym. 69, 7–13.
Desrochers, A., Cox, S., Oxenford, H., and Van Tussenbroek, B. (2020). Sargassum Uses Guide: A Resource for Caribbean Researchers, Entrepreneurs and Policy Makers. Bridgetown: Centre for Resource Management and Environmental Studies (CERMES), University of the West Indies, Cave Hill Campus.
Devault, D. A., Massat, F., Baylet, A., Dolique, F., and Lopez, P.-J. (2021a). Arsenic and chlordecone contamination and decontamination toxicokinetics in Sargassum sp. Environ. Sci. Pollut. Res. doi: 10.1007/s11356-020-12127-7
Devault, D. A., Pierre, R., Marfaing, H., Dolique, F., and Lopez, P.-J. (2021b). Sargassum contamination and consequences for downstream uses: a review. J. Appl. Phycol. 33, 567–602. doi: 10.1007/s10811-020-02250-w
Dianco (2021). Dianco México. Available online at: http://gpomd.com/dianco (accessed June 17, 2021).
DOF (2021a). Congreso de la Unión Ley General para la Prevención y Gestión Integral de los Residuos, Artículo 19. Available online at: http://www.diputados.gob.mx/LeyesBiblio/pdf/263_180121.pdf (accessed July 10, 2021).
DOF (2021b). Congreso de la Unión, Ley Federal del. Mar, Artículo 6, fe de Erratas 9.01.1986. Available online at: http://www.diputados.gob.mx/LeyesBiblio/ref/lfm.htm (accessed June 16, 2021).
DOF (2021c). Congreso de la Unión, Ley General de Bienes Nacionales, Artículo 6, Última Reforma 20.05.21. Available online at: http://www.diputados.gob.mx/LeyesBiblio/ref/lgbn.htm [Accessed June 16, 2021]
DOF (2021d). Congreso de la Unión, Ley Orgánica de la Administración Pública Federal, Artículo 30, Última Reforma 11.01.21. Available online at: http://www.diputados.gob.mx/LeyesBiblio/pdf/153_110121.pdf (accessed June 16, 2021).
Elgarahy, A. M., Elwakeel, K. Z., Mohammad, S. H., and Elshoubaky, G. A. (2021). A critical review of biosorption of dyes, heavy metals and metalloids from wastewater as an efficient and green process. Clean. Eng. Technol. 4:100209. doi: 10.1016/j.clet.2021.100209
Eluvakkal, T., Sivakumar, S., and Arunkumar, K. (2010). Fucoidan in some Indian brown seaweeds found along the coast Gulf of Mannar. Int. J. Bot. 6, 176–181. doi: 10.3923/ijb.2010.176.181
EMF (2021). Ellen MacArthur Foundation. What is Circular Economy?. Available online at: https://www.ellenmacarthurfoundation.org/circular-economy/what-is-the-circular-economy (accessed July 1, 2021).
Erpel, F., Mateos, R., Pérez-Jiménez, J., and Pérez-Correa, J. R. (2020). Phlorotannins: from isolation and structural characterization, to the evaluation of their antidiabetic and anticancer potential. Food Res. Int. 137:109589. doi: 10.1016/j.foodres.2020.109589
Fertah, M. (2017). “Isolation and characterization of Alginate from seaweed,” in Seaweed Polysaccharides, eds J. Venkatesan, S. Anil, and S.-K. Kim (Amsterdam: Elsevier), 11–26. doi: 10.1016/b978-0-12-809816-5.00002-5
Flórez-Fernández, N., Illera, M., Sánchez, M., Lodeiro, P., Torres, M. D., López-Mosquera, M. E., et al. (2021). Integrated valorization of Sargassum muticum in biorefineries. Chem. Eng. J. 404:125635. doi: 10.1016/j.cej.2020.125635
García-Sánchez, M., Graham, C., Vera, E., Escalante-Mancera, E., Álvarez-Filip, L., and van Tussenbroek, B. I. (2020). Temporal changes in the composition and biomass of beached pelagic Sargassum species in the Mexican Caribbean. Aquat. Bot. 167:103275. doi: 10.1016/j.aquabot.2020.103275
Godínez-Ortega, J. L., Cuatlán-Cortés, J. V., Lopez-bautista, J., and Tussenbroek, B. I. (2021). “A natural history of floating Sargassum species (Sargasso) from Mexico,” in Natural History and Ecology of Mexico and Central America, ed. L. Hufnagel (London: IntechOpen).
Gojon-Báez, H., Siqueiros-Beltrones, D. A., and Hernández-Contreras, H. J. (1998). In situ ruminal digestibility and degradability of Macrocystis pyrifera and Sargassum spp. in bovine livestock. Cienc. Marinas 24, 463–481. doi: 10.7773/cm.v24i4.762
Golberg, A., Zollmann, M., Prabhu, M., and Palatnik, R. R. (2020). “Enabling bioeconomy with offshore macroalgae biorefineries,” in Bioeconomy for Sustainable Development, ed. C. Keswani (Singapore: Springer), 173–200. doi: 10.1016/j.biortech.2017.06.061
Hannachi, Y., and Hafidh, A. (2020). Biosorption potential of Sargassum muticum algal biomass for methylene blue and lead removal from aqueous medium. Int. J. Environ. Sci. Technol. 17, 3875–3890. doi: 10.1007/s13762-020-02742-9
Harun, R., Yip, J., Thiruvenkadam, S., Ghani, W., Cherrington, T., and Danquah, M. (2013). Algal biomass conversion to bioethanol—A step-by-step assessment. Biotechnol. J. 9, 73–86. doi: 10.1002/biot.201200353
Hernández-Carmona, G., Rodríguez-Montesinos, Y., Arvizu-Higuera, D., Reyes-Tisnado, R., Murillo-Álvarez, J., and Muñoz-Ochoa, M. (2012). Avances tecnológicos en la producción de alginatos en México. Ingeniería Investig. Tecnol. 13, 155–168.
Hifney, A. F., Fawzy, M. A., Abdel-Gawad, K. M., and Gomaa, M. (2016). Industrial optimization of fucoidan extraction from Sargassum sp. and its potential antioxidant and emulsifying activities. Food Hydrocolloids 54, 77–88. doi: 10.1016/j.foodhyd.2015.09.022
Human, L. R. D., Snow, G. C., Adams, J. B., Bate, G. C., and Yang, S.-C. (2015). The role of submerged macrophytes and macroalgae in nutrient cycling: a budget approach. Estuar. Coast. Shelf Sci. 154, 169–178. doi: 10.1016/j.ecss.2015.01.001
IEA-Bioenergy (2017). International Energy Agency Bioenergy Inter-Task Strategic Project. State of Technology Review-Algae Bioenergy. Paris: IEA.
Jesumani, V., Du, H., Aslam, M., Pei, P., and Huang, N. J. M. D. (2019). Potential use of seaweed bioactive compounds in skincare—a review. Mar. Drugs 17:688. doi: 10.3390/md17120688
Joshi, S. M. (2008). The sick building syndrome. Indian J. Occup. Environ. Med. 12, 61–64. doi: 10.4103/0019-5278.43262
Kalaivanan, C., and Venkatesalu, V. J. (2012). Utilization of seaweed Sargassum myriocystum extracts as a stimulant of seedlings of Vigna mungo (L.) Hepper. Spanish J. Agric. Res. 10, 466–470. doi: 10.5424/sjar/2012102-507-10
Kanamarlapudi, S. L. R. K., Chintalpudi, V. K., and Muddada, S. (2018). Application of biosorption for removal of heavy metals from wastewater. Biosorption 18:69.
Kim, M. S., Oh, G. H., Kim, M. J., and Hwang, J. K. (2013). Fucosterol inhibits matrix metalloproteinase expression and promotes type-1 procollagen production in UVB-induced HaCaT cells. Photochem. Photobiol. 89, 911–918. doi: 10.1111/php.12061
Konda, N. V. S. N. M., Singh, S., Simmons, B. A., and Klein-Marcuschamer, D. (2015). An investigation on the economic feasibility of macroalgae as a potential feedstock for biorefineries. BioEnergy Res. 8, 1046–1056. doi: 10.1007/s12155-015-9594-1
Kostas, E. T., White, D. A., and Cook, D. J. (2017). Development of a bio-refinery process for the production of speciality chemical, biofuel and bioactive compounds from Laminaria digitata. Algal Res. 28, 211–219. doi: 10.1016/j.algal.2017.10.022
Kumar, G., Shobana, S., Nagarajan, D., Lee, D.-J., Lee, K.-S., Lin, C.-Y., et al. (2018). Biomass based hydrogen production by dark fermentation—recent trends and opportunities for greener processes. Curr. Opin. Biotechnol. 50, 136–145. doi: 10.1016/j.copbio.2017.12.024
Kumar, N. A., Vanlalzarzova, B., Sridhar, S., and Baluswami, M. (2012). Effect of liquid seaweed fertilizer of Sargassum wightii Grev. on the growth and biochemical content of green gram (Vigna radiata (L.) R. Wilczek). Recent Res. Sci. Technol. 4, 40–45.
Lange, L., Bak, U. G., Hansen, S. C. B., Gregersen, O., Harmsen, P., Karlsson, E. N., et al. (2020). “Opportunities for seaweed biorefinery,” in Sustainable Seaweed Technologies, eds M. D. Torres, S. Kraan, and H. Dominguez (Salt Lake City, UT: Elsevier Inc.), 3–31.
Li, Y.-X., Wijesekara, I., Li, Y., and Kim, S.-K. (2011). Phlorotannins as bioactive agents from brown algae. Process Biochem. 46, 2219–2224. doi: 10.1016/j.procbio.2011.09.015
Liu, L., Heinrich, M., Myers, S., and Dworjanyn, S. A. (2012). Towards a better understanding of medicinal uses of the brown seaweed Sargassum in Traditional Chinese Medicine: a phytochemical and pharmacological review. J. Ethnopharmacol. 142, 591–619. doi: 10.1016/j.jep.2012.05.046
López-Miranda, J. L., Silva, R., Molina, G. A., Esparza, R., Hernandez-Martinez, A., Hernández-Carteño, J., et al. (2020). Evaluation of a dynamic bioremediation system for the removal of metal ions and toxic dyes using Sargassum Spp. J. Mar. Sci. Eng. 8:899.
Mahmood, Z., Zahra, S., Iqbal, M., Raza, M. A., and Nasir, S. (2017). Comparative study of natural and modified biomass of Sargassum sp. for removal of Cd2+ and Zn2+ from wastewater. Appl. Water Sci. 7, 3469–3481. doi: 10.1007/s13201-017-0624-3
Mazumder, A., Holdt, S. L., De Francisci, D., Alvarado-Morales, M., Mishra, H. N., and Angelidaki, I. (2016). Extraction of alginate from Sargassum muticum: process optimization and study of its functional activities. J. Appl. Phycol. 28, 3625–3634. doi: 10.1007/s10811-016-0872-x
Mendoza-Becerril, M. A., Serviere-Zaragoza, E., Mazariegos-Villarreal, A., Rivera-Perez, C., Calder, D. R., Vázquez-Delfín, E. F., et al. (2020). Epibiont hydroids on beachcast Sargassum in the Mexican Caribbean. PeerJ 8:e9795. doi: 10.7717/peerj.9795
Metco (2021). Metco. Available online at: https://www.metco.com.mx/language/en/ (accessed June 20, 2021).
Meza, A. (1998). Impacto Sobre la Calidad del Huevo al Incluir Algas Marinas en Raciones Para Gallinas ponedoras. Tesis de Maestría. Facultad de Medicina Veterinaria y Zootecnia. Mexico: Universidad Nacional Autónoma de México.
Milledge, J. J., Nielsen, B. V., Maneein, S., and Harvey, P. J. (2019). A brief review of anaerobic digestion of algae for bioenergy. Energies 12:1166.
Mohammed, A., Bissoon, R., Bajnath, E., Mohammed, K., Lee, T., Bissram, M., et al. (2018). Multistage extraction and purification of waste Sargassum natans to produce sodium alginate: an optimization approach. Carbohydr. Polym. 198, 109–118. doi: 10.1016/j.carbpol.2018.06.067
Mohammed, A., Rivers, A., Stuckey, D. C., and Ward, K. (2020). Alginate extraction from Sargassum seaweed in the Caribbean region: optimization using response surface methodology. Carbohydr. Polym. 245:116419. doi: 10.1016/j.carbpol.2020.116419
Mohanty, D., Adhikary, S., Chattopadhyay, G., Prakasam, V., Gopamma, D., Ramoji, G., et al. (2013). Seaweed liquid fertilizer (SLF) and its role in agriculture productivity. The Ecoscan III 23–26, 1–10.
Nicolucci, C., and Monegato, A. (1996). Process for Manufacturing Paper from Seaweed. U.S. Patent No. 5,567,275. Washington, DC: U.S. Patent and Trademark Office.
Ortega, M. M., Godínez, J. L., and Solórzano, G. G. (2001). Catálogo de Algas Bénticas de las Costas Mexicanas del Golfo de México y Mar Caribe. Tlalnepantla: UNAM.
Oxenford, H. A., Cox, S.-A., van Tussenbroek, B. I., and Desrochers, A. (2021). Challenges of turning the Sargassum crisis into gold: current constraints and implications for the Caribbean. Phycology 1, 27–48. doi: 10.3390/phycology1010003
Pantoja, I. R. (2020). Inconstitucionalidad del Modelo Económico Nacional: 500 Años del Municipio. Mexico: Ediciones Navarra, 228–230.
Pantoja, I. R. (2021). Políticas Públicas Ambientales. Un Enfoque Ambiental, Jurídico y Social. México: Ediciones Gedisa, 79–104.
Priyan Shanura Fernando, I., Kim, K.-N., Kim, D., and Jeon, Y.-J. (2019). Algal polysaccharides: potential bioactive substances for cosmeceutical applications. Crit. Rev. Biotechnol. 39, 99–113. doi: 10.1080/07388551.2018.1503995
Puspita, M., Déniel, M., Widowati, I., Radjasa, O. K., Douzenel, P., Marty, C., et al. (2017). Total phenolic content and biological activities of enzymatic extracts from Sargassum muticum (Yendo) Fensholt. J. Appl. Phycol. 29, 2521–2537. doi: 10.1007/s10811-017-1086-6
Puspita, M., Setyawidati, N. A. R., Stiger-Pouvreau, V., Vandanjon, L., Widowati, I., Radjasa, O. K., et al. (2020). Indonesian Sargassum species bioprospecting: potential applications of bioactive compounds and challenge for sustainable development. Adv. Bot. Res. 95, 113–161.
Putman, N. F., and He, R. (2013). Tracking the long-distance dispersal of marine organisms: sensitivity to ocean model resolution. J. R. Soc. Interface 10:20120979. doi: 10.1098/rsif.2012.0979
Rajak, R. C., Jacob, S., and Kim, B. S. (2020). A holistic zero waste biorefinery approach for macroalgal biomass utilization: a review. Sci. Total Environ. 716, 137067. doi: 10.1016/j.scitotenv.2020.137067
Renovare (2021). Notas Renovare. Available online at: https://renovareco.com/#!/-notas-renovare/ (accessed June 30, 2021).
Robalds, A., Naja, G. M., and Klavins, M. J. (2016). Highlighting inconsistencies regarding metal biosorption. J. Hazard. Mater. 304, 553–556. doi: 10.1016/j.jhazmat.2015.10.042
Robledo, D., Vázquez-Delfín, E., Freile-Pelegrín, Y., Vásquez-Elizondo, R. M., Qui-Minet, Z. N., and Salazar-Garibay, A. (2021). Challenges and opportunities in relation to Sargassum events along the Caribbean Sea. Front. Mar. Sci. 8:699664. doi: 10.3389/fmars.2021.699664
Rodríguez-Martínez, R. E., Roy, P. D., Torrescano-Valle, N., Cabanillas-Terán, N., Carrillo-Domínguez, S., Collado-Vides, L., et al. (2020). Element concentrations in pelagic Sargassum along the Mexican Caribbean coast in 2018-2019. PeerJ 8:e8667. doi: 10.7717/peerj.8667
Saldarriaga-Hernandez, S., Nájera-Martínez, E. F., Martínez-Prado, M. A., and Melchor-Martínez, E. M. J. (2020). Sargassum-based potential biosorbent to tackle pollution in aqueous ecosystems–An overview. Case Stud. Chem. Environ. Eng. 2:100032. doi: 10.1016/j.cscee.2020.100032
Salgax (2019). SALGAX Biotecnología Marina Aplicada. Available online at: https://www.salgax.com/ (accessed 30 June, 2021).
Salter, M. A., Rodríguez-Martínez, R. E., Álvarez-Filip, L., Jordán-Dahlgren, E., and Perry, C. T. (2020). Pelagic Sargassum as an emerging vector of high rate carbonate sediment import to tropical Atlantic coastlines. Glob. Planet. Change 195:103332. doi: 10.1016/j.gloplacha.2020.103332
Sanjeewa, K. A., Kang, N., Ahn, G., Jee, Y., Kim, Y.-T., and Jeon, Y.-J. J. F. H. (2018). Bioactive potentials of sulfated polysaccharides isolated from brown seaweed Sargassum spp in related to human health applications: a review. Food Hydrocoll. 81, 200–208. doi: 10.1016/j.foodhyd.2018.02.040
Sarganico (2021). Sargánico Productos Sustentables 2019, Mexico. Available online at: https://www.facebook.com/sarganico/ (accessed June 1, 2021).
Scott, P. (2001). Bioprospecting as a conservation tool: history and background: crossing boundaries in park management,” in Proceedings of the 11th Conference on Research and Resource Management in Parks and on Public Lands, (Hancock, MI: The George Washington Wright Society).
Silva, R., Mendoza, E., Mariño-Tapia, I., Martínez, M. L., and Escalante, E. (2016). An artificial reef improves coastal protection and provides a base for coral recovery. J. Coast. Res. 75, 467–471. doi: 10.2112/SI75-094.1
Silva, R., Oumeraci, H., Martínez, M. L., Chávez, V., Lithgow, D., van Tussenbroek, B. I., et al. (2021). Ten commandments for sustainable, safe, and W/Healthy Sandy Coasts Facing Global Change. Front. Mar. Sci. 8:616321. doi: 10.3389/fmars.2021.616321
Silva, R., Villatoro, M., Ramos, F., Pedroza, D., Ortiz, M., Mendoza, E., et al. (2014). Caracterización de la Zona Costera y Planteamiento de Elementos Técnicos Para la Elaboración de Criterios de Regulación y Manejo Sustentable. Mexico City: Instituto de Ingeniería, Universidad Nacional Autónoma de México (UNAM), 118.
Singh, S., Kumar, V., Datta, S., Dhanjal, D. S., Sharma, K., Samuel, J., et al. (2020). Current advancement and future prospect of biosorbents for bioremediation. Sci. Total Environ. 709:135895. doi: 10.1016/j.scitotenv.2019.135895
Sinha, S., Astani, A., Ghosh, T., Schnitzler, P., and Ray, B. J. P. (2010). Polysaccharides from Sargassum tenerrimum: structural features, chemical modification and anti-viral activity. Phytochemistry 71, 235–242. doi: 10.1016/j.phytochem.2009.10.014
Sinurat, E., Peranginangin, R., and Saepudin, E. (2016). Purification and characterization of fucoidan from the brown seaweed Sargassum binderi Sonder. Squalen Bull. Mar. Fish. Postharvest Biotechnol. 10, 79–87.
Smetacek, V., and Zingone, A. J. N. (2013). Green and golden seaweed tides on the rise. Nature 504, 84–88.
Song, M., Duc Pham, H., Seon, J., and Chul Woo, H. (2015). Marine brown algae: a conundrum answer for sustainable biofuels production. Renew. Sustain. Energy Rev. 50, 782–792. doi: 10.1016/j.rser.2015.05.021
Sutharsan, S., Nishanthi, S., and Srikrishnah, S. J. (2014). Effects of foliar application of seaweed (Sargassum crassifolium) liquid extract on the performance of Lycopersicon esculentum Mill. in sandy regosol of Batticaloa District Sri Lanka. Am. Eurasian J. Agric. Environ. Sci. 14, 1386–1396.
Sverko Grdic, Z., Nizic, M., and Rudan, E. (2020). Circular economy concept in the context of economic development in EU Countries. Sustainability 12:3060. doi: 10.3390/su12073060
Tapia-Tussell, R., Avila-Arias, J., Domínguez Maldonado, J., Valero, D., Olguin-Maciel, E., Pérez-Brito, D., et al. (2018). Biological pretreatment of Mexican Caribbean macroalgae consortiums using Bm-2 Strain (Trametes hirsuta) and its enzymatic broth to improve biomethane potential. Energies 11:494. doi: 10.3390/en11030494
Thinh, P. D., Menshova, R. V., Ermakova, S. P., Anastyuk, S. D., Ly, B. M., and Zvyagintseva, T. N. J. (2013). Structural characteristics and anticancer activity of fucoidan from the brown alga Sargassum mcclurei. Mar. Drugs 11, 1456–1476. doi: 10.3390/md11051456
Thompson, T. M., Ramin, P., Udugama, I., Young, B. R., Gernaey, K. V., and Baroutian, S. (2021). Techno-economic and environmental impact assessment of biogas production and fertiliser recovery from pelagic Sargassum: a biorefinery concept for Barbados. Energy Conv. Manage. 245:114605. doi: 10.1016/j.enconman.2021.114605
Thompson, T. M., Young, B. R., and Baroutian, S. (2019). Advances in the pretreatment of brown macroalgae for biogas production. Fuel Process. Technol. 195:106151. doi: 10.1016/j.biortech.2017.05.172
Thompson, T. M., Young, B. R., and Baroutian, S. (2020b). Pelagic Sargassum for energy and fertiliser production in the Caribbean: a case study on Barbados. Renew. Sustain. Energy Rev. 118:109564. doi: 10.1016/j.rser.2019.109564
Thompson, T. M., Young, B. R., and Baroutian, S. (2020a). Efficiency of hydrothermal pretreatment on the anaerobic digestion of pelagic Sargassum for biogas and fertiliser recovery. Fuel 279:118527. doi: 10.1016/j.fuel.2020.118527
Torres, M. D., Kraan, S., and Domínguez, H. (2019). Seaweed biorefinery. Rev. Environ. Sci. Bio Technol. 18, 1–54. doi: 10.1007/s11157-019-09496-y
Tyler, A. C., McGlathery, K. J., and Anderson, I. C. (2001). Macroalgae Mediation of Dissolved Organic nitrogen fluxes in a temperate coastal lagoon. Estuar. Coast. Shelf Sci. 53, 155–168. doi: 10.1006/ecss.2001.0801
Ubando, A. T., Felix, C. B., and Chen, W.-H. (2020). Biorefineries in circular bioeconomy: a comprehensive review. Bioresour. Technol. 299:122585. doi: 10.1016/j.biortech.2019.122585
UNEP-CEP (2021). “Sargassum white paper—turning the crisis into an opportunity,” in Proceedings of the Ninth Meeting of the Scientific and Technical Advisory Committee (STAC) to the Protocol Concerning Specially Protected Areas and Wildlife (SPAW) in the Wider Caribbean Region, (Kingston: UNEP-CEP), 56.
van Tussenbroek, B. I., Arana, H. A. H., Rodríguez-Martínez, R. E., Espinoza-Avalos, J., Canizales-Flores, H. M., González-Godoy, C. E., et al. (2017). Severe impacts of brown tides caused by Sargassum spp. on near-shore Caribbean seagrass communities. Mar. Pollut. Bull. 122, 272–281. doi: 10.1016/j.marpolbul.2017.06.057
Wang, M., Hu, C., Barnes, B. B., Mitchum, G., Lapointe, B., and Montoya, J. P. (2019). The great Atlantic Sargassum belt. Science 365, 83–87.
Yanagisawa, M., Kawai, S., and Murata, K. (2013). Strategies for the production of high concentrations of bioethanol from seaweeds: production of high concentrations of bioethanol from seaweeds. Bioengineered 4, 224–235. doi: 10.4161/bioe.23396
Zayed, A., and Ulber, R. (2019). Fucoidan production: approval key challenges and opportunities. Carbohydr. Polym. 211, 289–297. doi: 10.1016/j.carbpol.2019.01.105
Keywords: Mexican Caribbean, Quintana Roo, sargasso, collection, legal framework, SWOT, circular economy
Citation: López Miranda JL, Celis LB, Estévez M, Chávez V, van Tussenbroek BI, Uribe-Martínez A, Cuevas E, Rosillo Pantoja I, Masia L, Cauich-Kantun C and Silva R (2021) Commercial Potential of Pelagic Sargassum spp. in Mexico. Front. Mar. Sci. 8:768470. doi: 10.3389/fmars.2021.768470
Received: 31 August 2021; Accepted: 25 October 2021;
Published: 12 November 2021.
Edited by:
Wei-Bo Chen, National Science and Technology Center for Disaster Reduction (NCDR), TaiwanReviewed by:
Danay Carrillo Nieves, Tecnologico de Monterrey, MexicoJosé Agüero, Medusozoa México, Mexico
Copyright © 2021 López Miranda, Celis, Estévez, Chávez, van Tussenbroek, Uribe-Martínez, Cuevas, Rosillo Pantoja, Masia, Cauich-Kantun and Silva. This is an open-access article distributed under the terms of the Creative Commons Attribution License (CC BY). The use, distribution or reproduction in other forums is permitted, provided the original author(s) and the copyright owner(s) are credited and that the original publication in this journal is cited, in accordance with accepted academic practice. No use, distribution or reproduction is permitted which does not comply with these terms.
*Correspondence: Valeria Chávez, dmNoYXZlemNAaWluZ2VuLnVuYW0ubXg=