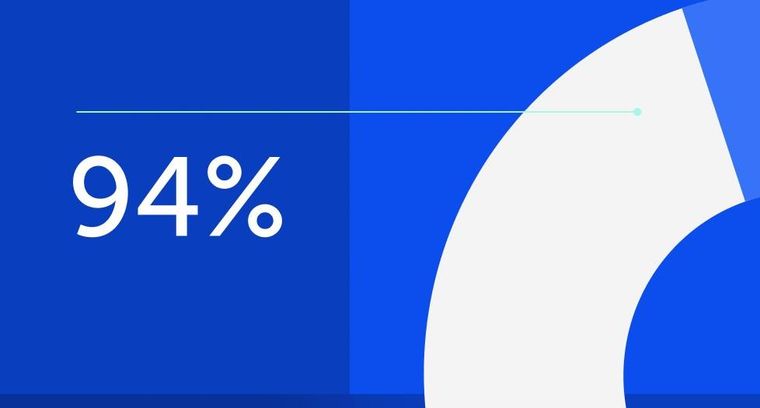
94% of researchers rate our articles as excellent or good
Learn more about the work of our research integrity team to safeguard the quality of each article we publish.
Find out more
EDITORIAL article
Front. Mar. Sci., 27 September 2021
Sec. Marine Ecosystem Ecology
Volume 8 - 2021 | https://doi.org/10.3389/fmars.2021.764035
This article is part of the Research TopicUnraveling Mechanisms Underlying Annual Plankton Blooms in the North Atlantic and their Implications for Biogenic Aerosol Properties and Cloud FormationView all 22 articles
Editorial on the Research Topic
Unraveling Mechanisms Underlying Annual Plankton Blooms in the North Atlantic and Their Implications for Biogenic Aerosol Properties and Cloud Formation
Ocean ecosystems play a central role in the vitality of our biosphere and both influence and are influenced by the overlying atmosphere. Plankton-based compounds released from the ocean through bubble bursting and wave breaking contribute to atmospheric aerosol load and influence the formation and properties of clouds (Meskhidze et al., 2013). In parallel, ocean ecosystems are recipients of atmospheric depositions, often transported from distant sources (van de Meent et al., 2011). Ocean-atmosphere feedbacks are particularly amplified when pristine atmospheres overlay strong annual plankton cycles. The western subarctic Atlantic is one such place. The North Atlantic Aerosol and Marine Ecosystem Study (NAAMES)1 was a National Aeronautics and Space Administration (NASA) Earth Venture Suborbital mission focused on plankton blooms, aerosols, and clouds in this subarctic region (~40–55°N) and conducted between 2015 and 2019. The mission entailed four field campaigns targeting specific events in the annual plankton cycle and included a diversity of ship-based, airborne, and autonomous measurements (Behrenfeld et al.). At the time of writing, nearly 80 publications stem from NAAMES (21 represented within this frontiers research topic) and more are in development. This Editorial provides a brief synopsis of many of the NAAMES scientific findings.
Analysis of mean dynamic topography (MDT) separated the NAAMES study region into four provinces [Della Penna and Gaube(a)] with hydrographic boundaries strongly influencing plankton community composition (Bolaños et al., 2020; Bolaños et al.), phenology (Yang et al.), and physiological state (Fox et al.). In general, amplicon sequence variant (ASV) analysis revealed phytoplankton communities to be more closely related between seasons within an MDT province than between provinces within a given season, although strong mesoscale features occasionally disrupted these ecological boundaries (Bolaños et al., 2020). The NAAMES study region featured an energetic field of mesoscale eddies and meanders (radial scale: 20–50 km) formed by instability in the Gulf Stream and baroclinic instability in the open ocean. These features were largest in the Gulf Stream region (amplitudes exceeding 40 cm), but most formed in the open ocean, trapping large water parcels for days to weeks [Della Penna and Gaube(a)]. The vertical (Schulien et al.) and horizontal (Bolaños et al., 2020; Mojica et al., 2020) structure of microbial communities were strongly influenced by these physical features, but accurate representation of this spatial variability remains a significant challenge in bio-physical simulations (Eveleth et al.).
Anticyclonic (clockwise-rotating) eddies in the western North Atlantic have anomalously warm surface water, low chlorophyll concentrations (CHL) (Gaube et al., 2014), and often enhanced vertical mixing (Gaube et al., 2019b), presumably as a result of deep and sustained convection that homogenizes phytoplankton such that their vertically-integrating biomass can exceed that of stratified cyclonic eddies with higher near-surface CHL concentrations. The eddies sampled during NAAMES revealed that anticyclones contained anomalously high concentrations of mesopelagic organisms, including fish, squid, jellies, and crustaceans, when compared to neighboring cyclones [Della Penna and Gaube(b)]. Coupled with elevated temperatures, enhanced mesopelagic prey biomass may explain the systematic selection of anticyclonic cores by feeding white (Caracaius carcaridon) and blue (Prionace glauca) sharks (Gaube et al., 2018; Braun et al., 2019).
Features with spatial scales ranging from <1 km to > 100 km, referred to as the oceanic (sub)mesoscale, can modulate the stability and vertical extent of the marine atmospheric boundary layer (MABL) and create pressure gradients that elevate wind speeds down-wind of (sub)mesoscale fronts (Gaube et al., 2019a). Elevated wind speed at the air-sea interface can enhance primary aerosol (sea spray) and secondary aerosol (volatile compounds that nucleate into new particles) production (see below).
A surprising observation during NAAMES was the general paucity of large phytoplankton during the spring bloom (Bolaños et al., 2020; Menden-Deuer et al., 2020). Instead, ASV, particle count, size-fractionated CHL, flow cytometry (FCM), and image-based [e.g., Imaging FlowCytobot (IFCB), FlowCam] analyses generally all indicated that pico- and nano-sized cells dominated phytoplankton abundance and total bio-volume (Bolaños et al., 2020; Chase et al., 2020; Menden-Deuer et al., 2020), although pigment-based taxonomical classification suggested stronger diatom contributions (Kramer et al.). In cases where elevated diatom abundance was indicated by ASV, image analysis revealed these to be species in the nano- or smaller micro-phytoplankton size classes (Bolaños et al., 2020; Menden-Deuer et al., 2020). These findings have important biogeochemical implications, as the subarctic Atlantic bloom is broadly assumed to be dominated by large diatoms that efficiently export carbon to depth (Lochte et al., 1993; Sieracki et al., 1993; Hashioka et al., 2013; Rynearson et al., 2013). While an explanation for the scarcity of large diatoms during NAAMES has been proposed (Behrenfeld et al., 2021a,b), the observation is a reminder that diagnostic pigment approaches can notably overestimate microplankton contributions to phytoplankton biomass (Chase et al., 2020).
The Disturbance-Recovery Hypothesis (DRH) provides a framework for understanding temporal dynamics in phytoplankton biomass in the context of imbalances in phytoplankton division and loss rates (Behrenfeld and Boss, 2018; Behrenfeld et al.). One element of the DRH is the role of mixed layer dynamics on predator-prey coupling, and a unique opportunity presented itself during NAAMES to empirically test this idea. Specifically, during the 2016 May-June campaign, a cold-air event caused an ~10-fold deepening of the mixed layer (to >200 m) followed by rapid restratification (Graff and Behrenfeld). The deep mixing decoupled phytoplankton division and loss rates by dispersing both predators and prey. Phytoplankton rapidly photoacclimated (Graff and Behrenfeld) and their growth remained largely unchecked during the subsequent restratification period due to a temporal lag in the zooplankton response to accumulating prey biomass (Behrenfeld, 2014; Behrenfeld and Boss, 2018; Morison et al.). Mojica et al. used shipboard incubation experiments to emulate these results, quantitatively demonstrating that the rate of change in phytoplankton biomass varies with the specific rate of change in phytoplankton division rate (Δμ) (Behrenfeld et al., 2017; Behrenfeld and Boss, 2018). Episodic deep mixing events also appear to be an important mechanism “pumping” new biomass into communities deeper in the water column (Penta et al., 2021).
Biogeochemical (BGC)-Argo autonomous profiling floats provided a continuous long-term record of bio-optical measurements for monitoring plankton dynamics during NAAMES. These data (along with ship-based measurements) confirmed the DRH prediction of phytoplankton bloom initiation during early winter (Behrenfeld, 2010; Behrenfeld and Boss, 2018) when division rates are still decreasing and mixed layer growth conditions are deteriorating (Yang et al., 2021; Yang et al.). Similar results were found for the Southern Ocean (Arteaga et al., 2020). BCG-Argo data also yielded a new net primary production (NPP) model (Fox et al.) enabling high temporal-resolution comparisons of phytoplankton division (μ), loss (l), and biomass accumulation (r) rates. NAAMES data show that r is not directly proportional to μ (Fox et al.; Yang et al.), but rather governed by Δμ. Ship-based measurements provided additional insights on the physiological underpinnings of the phytoplankton annual cycle. Using biomarkers of programmed cell death, compromised membranes, viral infection, and nutrient limitation, Diaz et al. (2021) showed low levels of cellular stress during the main spring accumulation phase of the annual phytoplankton cycle, a pronounced increase in cell stress in late spring heralding bloom termination, and maximum cell stress and death during the autumn declining phase. Host physiological stress can be a critical factor triggering phytoplankton viruses to abandon a temperate life style and become lytic (Knowles et al., 2020), implying that low measured rates of lytic infection in spring (Morison et al.; Mojica et al.) may in part be attributable to a prominence of temperate viral behavior during this low-stress season. Enhanced cell stress was also evident during winter bloom initiation (Diaz et al., 2021), but is overridden by the greater impacts of mixed layer deepening on predator-prey relationships (Yang et al.; Yang et al., 2021).
Highest rates of net bacterial production (BP), bacterial carbon demand (BCD), and bacterial abundance (BA) were observed during NAAMES in the late spring, paralleled by the largest flux of labile dissolved organic matter (DOM) to bacterioplankton (Baetge Behrenfeld et al.). The BCD:NPP ratio was low in late spring, suggesting new organic matter was largely partitioned into particulate organic matter and export (Baetge Behrenfeld et al.). At this time, the net biogenic production of volatile organic compounds (VOCs) was also elevated (Davie-Martin et al.). Flavobacteriales and Oceanospirillales were relatively abundant in late spring, suggesting a response of specific copiotrophic members of these groups to fresh labile DOM (Bolaños et al.). The Flavobacteria taxa also included vitamin B1 auxotrophs that significantly depleted dissolved thiamin levels (Suffridge et al.). Between late spring and early autumn, net community production (NCP) accumulating as seasonal (semi-labile) surplus DOC increased (Baetge Graff et al.), along with the abundance of SAR202 genomes in the euphotic zone (Bolaños et al.). This period corresponded to enhanced physiological stress and viral mediated phytoplankton mortality, the latter enhancing substrate for heterotrophic prokaryote growth and potentially impacting community structuring (Mojica and Brussaard, 2020).
Sea spray aerosol (SSA) during winter represented approximately 50% of the total aerosol, with no evidence of aerosol transported from other sources (Quinn et al., 2019). Concentrations of this “SSA mode” were significant but < 20% during other seasons. Notably, these findings demonstrate the long-hypothesized link between phytoplankton and atmospheric aerosols and are a step forward in understanding processes producing aerosols in the marine environment (Brooks and Thornton, 2018). Even so, debate persists regarding quantitative budgets (Bates et al., 2020) and the direct flux of marine aerosol remains unquantified (Sanchez et al., 2018, 2021).
Dissolved organic constituents in seawater include VOCs emitted by phytoplankton (Halsey et al., 2017a,b; Moore et al., 2020; Davie-Martin et al.), but a majority of these compounds may be consumed by heterotrophic bacteria before reaching the air-sea interface. During NAAMES, biogenic VOC production increased with phytoplankton productivity and incident sunlight (Davie-Martin et al.). Dimethyl sulfide (DMS) and methanethiol were highest over phytoplankton blooms, while acetonitrile was high in both late spring and early autumn. The composition of submicron primary marine aerosol varied with season and latitude (Lewis et al.). Organic materials in aerosol were linked to ubiquitous recalcitrant dissolved organic matter and transient polysaccharides emitted under bloom conditions (Lawler et al., 2020).
Total aerosol mass was not correlated with surface layer dissolved organic carbon mass, indicating aerosol production through multiple processes with difference timescales (Sanchez et al., 2021). DMS and primary marine aerosol correlate on short air-mass timescales (i.e., local sources). In contrast, marine non-refractory aerosol correlates with primary production when air masses were weighted on long (5d) timescales. This finding is consistent with processes of biogenic VOC emissions and subsequent photolysis in the atmosphere allowing detection significantly distant from source regions, and also implies an important role of wind speed and trajectory (Saliba et al., 2019, 2020). Future composition and microphysical measurements are still needed to better quantify radiative impacts of the direct and indirect aerosol effects sourced from the ocean (Saliba et al., 2019, 2020; Wilbourn et al., 2020; Sanchez et al., 2021; Bell et al.; Hendrickson et al.).
While NAAMES measurements confirmed that marine secondary organic aerosols were of the size and solubility to be cloud forming aerosol (Sanchez et al., 2018), the relative contribution of organic compounds in marine cloud condensation nuclei (CCN) is small (Hendrickson et al.). CCN activation by marine soluble organic compounds is similar to that of salts (Hendrickson et al.) and the strongest link between marine biology and CCN may be non-sea-salt sulfate (Saliba et al., 2020). For cold cloud development, however, microorganisms from the sea surface do have ice-nucleating properties and facilitate the freezing of ice atmospheric droplets despite antifreeze properties of associated salts (Wilbourn et al., 2020).
NAAMES produced substantial evidence of new particle formation in the marine boundary layer, with significant links to ocean biology (Croft et al., 2021; Zheng et al., 2021). Integration of these observations into the GEOS-Chem-TOMAS model indicated that aerosols are significantly undercounted if marine new particle formation is not accounted for (Croft et al., 2021). NAAMES also provided a platform for instrument and technique development, including cloud droplet number (CDN) and collection of liquid cloud water for detailed offline analysis (Crosbie et al., 2018). Sinclair et al. (2019) evaluated CDN concentrations by coupling airborne and ship-based measurements, Alexandrov et al. (2018) linked remote scanning polarimeter (RSP) retrievals of CDN to direct in-cloud measurements, and Stamnes et al. (2018) used high spectral resolution lidar (HSRL) measurements to derive detailed cloud microphysical properties.
With respect to remote sensing, Painemal et al. (2021) used in-situ cloud droplet probe measurements to validate satellite retrieved cloud properties, while Allen et al. used in-water optical measurements to evaluate seasonal and spatial patterns of inherent and apparent optical properties and tied their spectral dependence to colored dissolved organic matter, phytoplankton pigment absorption, and particulate backscatter. The NAAMES mission also contributed significantly to a growing body of literature regarding satellite lidar as an invaluable tool for ocean remote sensing (Hostetler et al., 2018; Jamet et al., 2019), including the characterization of polar plankton cycles (Behrenfeld et al., 2017), testing of heritage satellite ocean color products (Bisson et al., 2021a,b), identification of phytoplankton taxonomic groups within and outside eddies (Schulien et al.), and detection of global ocean diel animal vertical migrations (DVM) (Behrenfeld et al., 2019), noting here that vertical carbon transport by DVM activities is now an explicit element in ocean biogeochemical models (Archibald et al., 2019).
The NAAMES experience also yielded a variety of higher-level syntheses regarding phytoplankton bloom hypotheses (Behrenfeld and Boss, 2018), diatom ecology and evolution (Behrenfeld et al., 2021a), phytoplankton size distributions and succession (Behrenfeld et al., 2021c), and a new interpretation of Hutchinson's (1961) “Paradox of the Plankton” regarding the unexpected diversity of phytoplankton species in water columns with few readily identifiable niches (Behrenfeld et al., 2021b).
MB, SB, PG, and KM jointly wrote and edited the manuscript. All authors contributed to the article and approved the submitted version.
NAAMES was supported by the NASA Earth Venture Suborbital program. Analysis of NAAMES samples by SB was provided by NSF ATM (grant # 1539881). PG was supported by NASA grants 80NSSC20M0202 and 80NSSC118k0757 during the writing of the manuscript. MJB was supported by NASA grant 80NSSC17K0568 during the writing of the manuscript.
The authors declare that the research was conducted in the absence of any commercial or financial relationships that could be construed as a potential conflict of interest.
All claims expressed in this article are solely those of the authors and do not necessarily represent those of their affiliated organizations, or those of the publisher, the editors and the reviewers. Any product that may be evaluated in this article, or claim that may be made by its manufacturer, is not guaranteed or endorsed by the publisher.
We thank the tremendous contributions of the more than 270 scientists and support personnel involved in the NAAMES project and the outstanding support of the R/V Atlantis and St. John's International Airport.
Alexandrov, M. D., Cairns, B., Sinclair, K., Wasilewski, A. P., Ziemba, L., Crosbie, E., et al. (2018). Retrievals of cloud droplet size from the research scanning polarimeter data: validation using in situ measurements. Remote Sens. Environ. 210, 76–95. doi: 10.1016/j.rse.2018.03.005
Archibald, K. M., Siegel, D. A., and Doney, S. C. (2019). Modeling the impact of zooplankton diel vertical migration on the carbon export flux of the biological pump. Global Biogeochem. Cycles 33, 181–199. doi: 10.1029/2018GB005983
Arteaga, L. A., Boss, E., Behrenfeld, M. J., Westberry, T. K., and Sarmiento, J. L. (2020). Seasonal modulation of phytoplankton biomass in the Southern Ocean. Nat. Commun. 11:5364. doi: 10.1038/s41467-020-19157-2
Bates, T. S., Quinn, P. K., Coffman, D. J., Johnson, J. E., Upchurch, L., Saliba, G., et al. (2020). Variability in marine plankton ecosystems are not observed in freshly emitted sea spray aerosol over the North Atlantic Ocean. Geophys. Res. Lett. 47:e2019GL085938. doi: 10.1029/2019GL085938
Behrenfeld, M., Boss, E. S., and Halsey, K. H. (2021c). Phytoplankton community structuring and succession in a competition-neutral resource landscape. ISME Commun. 1, 1–8. doi: 10.1038/s43705-021-00011-5
Behrenfeld, M. J. (2010). Abandoning sverdrup's critical depth hypothesis on phytoplankton blooms. Ecology 91, 977–989. doi: 10.1890/09-1207.1
Behrenfeld, M. J. (2014). Climate-mediated dance of the plankton. Nat. Clim. Chang. 4, 880–887. doi: 10.1038/nclimate2349
Behrenfeld, M. J., and Boss, E. S. (2018). Student's tutorial on bloom hypotheses in the context of phytoplankton annual cycles. Glob. Chang. Biol. 24, 55–77. doi: 10.1111/gcb.13858
Behrenfeld, M. J., Gaube, P., Della Penna, A., O'malley, R. T., Burt, W. J., Hu, Y. X., et al. (2019). Global satellite-observed daily vertical migrations of ocean animals. Nature 576, 257–261. doi: 10.1038/s41586-019-1796-9
Behrenfeld, M. J., Halsey, K. H., Boss, E., Karp-Boss, L., Milligan, A. J., and Peers, G. (2021a). Thoughts on the evolution and ecological niche of diatoms. Ecol. Monographs 91:e01457. doi: 10.1002/ecm.1457
Behrenfeld, M. J., Hu, Y. X., O'malley, R. T., Boss, E. S., Hostetler, C. A., Siegel, D. A., et al. (2017). Annual boom-bust cycles of polar phytoplankton biomass revealed by space-based lidar. Nat. Geosci. 10, 118–122. doi: 10.1038/ngeo2861
Behrenfeld, M. J., O'malley, R., Boss, E., Karp-Boss, L., and Mundt, C. (2021b). Phytoplankton biodiversity and the Inverted Paradox. ISME Comm.
Bisson, K. M., Boss, E., Werdell, P. J., Ibrahim, A., and Behrenfeld, M. J. (2021a). Particulate backscattering in the global ocean: a comparison of independent assessments. Geophys. Res. Lett. 48:e2020GL090909. doi: 10.1029/2020GL090909
Bisson, K. M., Boss, E. S., Werdell, P. J., Ibrahim, A., Frouin, R., and Behrenfeld, M. J. (2021b). Seasonal bias in global ocean color observations. Appl. Opt. 60, 6978–6988. doi: 10.1364/AO.426137
Bolaños, L. M., Karp-Boss, L., Choi, C. J., Worden, A. Z., Graff, J. R., Haentjens, N., et al. (2020). Small phytoplankton dominate western North Atlantic biomass. ISME J. 14, 1663–1674. doi: 10.1038/s41396-020-0636-0
Braun, C. D., Gaube, P., Sinclair-Taylor, T. H., Skomal, G. B., and Thorrold, S. R. (2019). Mesoscale eddies release pelagic sharks from thermal constraints to foraging in the ocean twilight zone. Proc. Natl. Acad. Sci. U.S.A. 116, 17187–17192. doi: 10.1073/pnas.1903067116
Brooks, S. D., and Thornton, D. C. O. (2018). Marine aerosols and clouds. Ann. Rev. Mar. Sci. 10, 289–313. doi: 10.1146/annurev-marine-121916-063148
Chase, A. P., Kramer, S. J., Haentjens, N., Boss, E. S., Karp-Boss, L., Edmondson, M., et al. (2020). Evaluation of diagnostic pigments to estimate phytoplankton size classes. Limnol. Oceanography-Methods 18, 570–584. doi: 10.1002/lom3.10385
Croft, B., Martin, R. V., Moore, R. H., Ziemba, L. D., Crosbie, E. C., Liu, H. Y., et al. (2021). Factors controlling marine aerosol size distributions and their climate effects over the northwest Atlantic Ocean region. Atmosph. Chem. Phys. 21, 1889–1916. doi: 10.5194/acp-21-1889-2021
Crosbie, E., Brown, M. D., Shook, M., Ziemba, L., Moore, R. H., Shingler, T., et al. (2018). Development and characterization of a high-efficiency, aircraft-based axial cyclone cloud water collector. Atmosph. Measure. Techniq. 11, 5025–5048. doi: 10.5194/amt-11-5025-2018
Diaz, B., Knowles, B., Johns, C. T., Laber, C. P., Bondoc, K. G. V., Harvey, E. L., et al. (2021). Seasonal mixed layer stratification contextualizes phytoplankton physiology, viral infection, and accumulation in the North Atlantic. Nat. Commun.
Gaube, P., Braun, C. D., Lawson, G. L., Mcgillicuddy, D. J., Della Penna, A., Skomal, G. B., et al. (2018). Mesoscale eddies influence the movements of mature female white sharks in the Gulf Stream and Sargasso Sea. Sci. Rep. 8:7363. doi: 10.1038/s41598-018-25565-8
Gaube, P., Chickadel, C. C., Branch, R., and Jessup, A. (2019a). Satellite observations of SST-induced wind speed perturbation at the oceanic submesoscale. Geophys. Res. Lett. 46, 2690–2695. doi: 10.1029/2018GL080807
Gaube, P., Mcgillicuddy, D. J., Chelton, D. B., Behrenfeld, M. J., and Strutton, P. G. (2014). Regional variations in the influence of mesoscale eddies on near-surface chlorophyll. J. Geophys. Res. -Oceans 119, 8195–8220. doi: 10.1002/2014JC010111
Gaube, P., Mcgillicuddy, D. J., and Moulin, A. J. (2019b). Mesoscale eddies modulate mixed layer depth globally. Geophys. Res. Lett. 46, 1505–1512. doi: 10.1029/2018GL080006
Halsey, K. H., Davie-Martin, C., Giovannoni, S. J., and Ocel, D. (2017a). The planktonic VOC metabolome. Phycologia 56, 72–73. doi: 10.2216/0031-8884-56.sp4.1
Halsey, K. H., Giovannoni, S. J., Graus, M., Zhao, Y. L., Landry, Z., Thrash, J. C., et al. (2017b). Biological cycling of volatile organic carbon by phytoplankton and bacterioplankton. Limnol. Oceanogr. 62, 2650–2661. doi: 10.1002/lno.10596
Hashioka, T., Vogt, M., Yamanaka, Y., Le Quere, C., Buitenhuis, E. T., Aita, M. N., et al. (2013). Phytoplankton competition during the spring bloom in four plankton functional type models. Biogeosciences 10, 6833–6850. doi: 10.5194/bg-10-6833-2013
Hostetler, C. A., Behrenfeld, M. J., Hu, Y. X., Hair, J. W., and Schulien, J. A. (2018). Spaceborne lidar in the study of marine systems. Ann. Rev. Mar. Sci. 10, 121–147. doi: 10.1146/annurev-marine-121916-063335
Jamet, C., Ibrahim, A., Ahmad, Z., Angelini, F., Babin, M., Behrenfeld, M. J., et al. (2019). Going beyond standard ocean color observations: Lidar and polarimetry. Front. Mar. Sci. 6:251. doi: 10.3389/fmars.2019.00251
Knowles, B., Bonachela, J. A., Behrenfeld, M. J., Bondoc, K. G., Cael, B. B., Carlson, C. A., et al. (2020). Temperate infection in a virus-host system previously known for virulent dynamics. Nat. Commun. 11:4626. doi: 10.1038/s41467-020-18078-4
Lawler, M. J., Lewis, S. L., Russell, L. M., Quinn, P. K., Bates, T. S., Coffman, D. J., et al. (2020). North Atlantic marine organic aerosol characterized by novel offline thermal desorption mass spectrometry: polysaccharides, recalcitrant material, and secondary organics. Atmosph. Chem. Phys. 20, 16007–16022. doi: 10.5194/acp-20-16007-2020
Lochte, K., Ducklow, H. W., Fasham, M. J. R., and Stienen, C. (1993). Plankton succession and carbon cycling at 47°N 20°W during the JGOFS North Atlantic Bloom Experiment. Deep-Sea Res. II 40, 91–114. doi: 10.1016/0967-0645(93)90008-B
Menden-Deuer, S., Morison, F., Montalbano, A. L., Franze, G., Strock, J., Rubin, E., et al. (2020). Multi-instrument assessment of phytoplankton abundance and cell sizes in mono-specific laboratory cultures and whole plankton community composition in the North Atlantic. Front. Mar. Sci. 7:254. doi: 10.3389/fmars.2020.00254
Meskhidze, N., Petters, M. D., Tsigaridis, K., Bates, T., O'dowd, C., Reid, J., et al. (2013). Production mechanisms, number concentration, size distribution, chemical composition, and optical properties of sea spray aerosols. Atmosph. Sci. Lett. 14, 207–213. doi: 10.1002/asl2.441
Mojica, K. D. A., and Brussaard, C. P. D. (2020). Significance of viral activity for regulating hetertrophic prokaryote community dynamics along a meridional gradient of stratificaiton in the Northeast Atlantic Ocean. Viruses 12:1293. doi: 10.3390/v12111293
Mojica, K. D. A., Carlson, C. A., and Behrenfeld, M. J. (2020). Regulation of low and high nucleic acid fluorescent heterotrophic prokaryote subpopulations and links to viral-induced mortality within natural prokaryote-virus communities. Microb. Ecol. 79, 213–230. doi: 10.1007/s00248-019-01393-9
Moore, E. R., Davie-Martin, C. L., Giovannoni, S. J., and Halsey, K. H. (2020). Pelagibacter metabolism of diatom-derived volatile organic compounds imposes an energetic tax on photosynthetic carbon fixation. Environ. Microbiol. 22, 1720–1733. doi: 10.1111/1462-2920.14861
Painemal, D., Spangenberg, D., Smith, J.r,., W. L., Minnis, P., Cairns, B., et al. (2021). Evaluation of satellite retrievals of liquid clouds from the GOES-13 Imager and MODIS over the midlatitude North Atlantic during NAAMES campaign. Atmosph. Measure. Techniq. Discussions. doi: 10.5194/amt-2021-7
Penta, W. B., Fox, J., and Halsey, K. H. (2021). Rapid photoacclimation during episodic deep mixing augments the biological carbon pump. Limnol. Oceanogr. 66, 1850–1866. doi: 10.1002/lno.11728
Quinn, P. K., Bates, T. S., Coffman, D. J., Upchurch, L., Johnson, J. E., Moore, R., et al. (2019). Seasonal variations in western North Atlantic remote marine aerosol properties. J. Geophys. Res. Atmosph. 124, 14240–14261. doi: 10.1029/2019JD031740
Rynearson, T. A., Richardson, K., Lampitt, R. S., Sieracki, M. E., Poulton, A. J., Lyngsgaard, M. M., et al. (2013). Major contribution of diatom resting spores to vertical flux in the sub-polar North Atlantic. Deep-Sea Res. I-Oceanographic Res. Papers 82, 60–71. doi: 10.1016/j.dsr.2013.07.013
Saliba, G., Chen, C. L., Lewis, S., Russell, L. M., Quinn, P. K., Bates, T. S., et al. (2020). Seasonal differences and variability of concentrations, chemical composition, and cloud condensation nuclei of marine aerosol over the North Atlantic. J. Geophys. Res. Atmosph. 125:e2020JD033145. doi: 10.1029/2020JD033145
Saliba, G., Chen, C. L., Lewis, S., Russell, L. M., Rivellini, L. H., Lee, A. K. Y., et al. (2019). Factors driving the seasonal and hourly variability of sea-spray aerosol number in the North Atlantic. Proc. Natl. Acad. Sci. U.S.A. 116, 20309–20314. doi: 10.1073/pnas.1907574116
Sanchez, K. J., Chen, C. L., Russell, L. M., Betha, R., Liu, J., Price, D. J., et al. (2018). Substantial seasonal contribution of observed biogenic sulfate particles to cloud condensation nuclei. Sci. Rep. 8:3235. doi: 10.1038/s41598-018-21590-9
Sanchez, K. J., Zhang, B., Liu, H. Y., Saliba, G., Chen, C. L., Lewis, S. L., et al. (2021). Linking marine phytoplankton emissions, meteorological processes, and downwind particle properties with FLEXPART. Atmosph. Chem. Phys. 21, 831–851. doi: 10.5194/acp-21-831-2021
Sieracki, M. E., Verity, P. G., and Stoecker, D. K. (1993). Plankton community response to sequential silicate and nitrate depletion during the 1989 North Atlantic spring bloom. Deep-Sea Res. II 40, 213–225. doi: 10.1016/0967-0645(93)90014-E
Sinclair, K., Van Diedenhoven, B., Cairns, B., Alexandrov, M., Moore, R., Crosbie, E., et al. (2019). Polarimetric retrievals of cloud droplet number concentrations. Remote Sens. Environ. 228, 227–240. doi: 10.1016/j.rse.2019.04.008
Stamnes, S., Hostetler, C., Ferrare, R., Burton, S., Liu, X., Hair, J., et al. (2018). Simultaneous polarimeter retrievals of microphysical aerosol and ocean color parameters from the “MAPP” algorithm with comparison to high-spectral-resolution lidar aerosol and ocean products. Appl. Opt. 57, 2394–2413. doi: 10.1364/AO.57.002394
van de Meent, D., Hollander, A., Peijnenburg, W., and Breure, T. (2011). “Fate and transport of contaminants,” in Ecological Impacts of Toxic Chemicals, eds. F. Sánchez-Bayo, P. Van Den Brink and R. M. Mann (Bentham Science Publishers Ltd.), 13.42. doi: 10.2174/978160805121211101010013
Wilbourn, E. K., Thornton, D. C. O., Ott, C., Graff, J., Quinn, P. K., Bates, T. S., et al. (2020). Ice nucleation by marine aerosols over the North Atlantic Ocean in late spring. J. Geophys. Res. Atmosph. 125:e2019JD030913. doi: 10.1029/2019JD030913
Yang, B., Fox, J., Behrenfeld, M. J., Boss, E. S., Haentjens, N., Halsey, K. H., et al. (2021). In situ estimates of net primary production in the western North Atlantic with argo profiling floats. J. Geophys. Res. Biogeosci. 126:e2020JG006116. doi: 10.1029/2020JG006116
Keywords: NAAMES, phytoplankton, blooms, marine aerosols, clouds, remote sensing
Citation: Behrenfeld MJ, Brooks SD, Gaube P and Mojica KDA (2021) Editorial: Unraveling Mechanisms Underlying Annual Plankton Blooms in the North Atlantic and Their Implications for Biogenic Aerosol Properties and Cloud Formation. Front. Mar. Sci. 8:764035. doi: 10.3389/fmars.2021.764035
Received: 24 August 2021; Accepted: 27 August 2021;
Published: 27 September 2021.
Edited and reviewed by: Angel Borja, Technological Center Expert in Marine and Food Innovation (AZTI), Spain
Copyright © 2021 Behrenfeld, Brooks, Gaube and Mojica. This is an open-access article distributed under the terms of the Creative Commons Attribution License (CC BY). The use, distribution or reproduction in other forums is permitted, provided the original author(s) and the copyright owner(s) are credited and that the original publication in this journal is cited, in accordance with accepted academic practice. No use, distribution or reproduction is permitted which does not comply with these terms.
*Correspondence: Michael J. Behrenfeld, bWljaGFlbC5iZWhyZW5mZWxkQG9yZWdvbnN0YXRlLmVkdQ==
Disclaimer: All claims expressed in this article are solely those of the authors and do not necessarily represent those of their affiliated organizations, or those of the publisher, the editors and the reviewers. Any product that may be evaluated in this article or claim that may be made by its manufacturer is not guaranteed or endorsed by the publisher.
Research integrity at Frontiers
Learn more about the work of our research integrity team to safeguard the quality of each article we publish.