- 1Key Laboratory of Microbial Technology for Industrial Pollution Control of Zhejiang Province, College of Environment, Zhejiang University of Technology, Hangzhou, China
- 2CAS Key Laboratory of Coastal Environmental Processes and Ecological Remediation, Yantai Institute of Coastal Zone Research, Chinese Academy of Sciences, Yantai, China
- 3Leibniz Institute for Baltic Sea Research, Rostock, Germany
- 4CAS Key Laboratory of Soil Environment and Pollution Remediation, Institute of Soil Science, Chinese Academy of Sciences, Nanjing, China
Microplastic pollution in semi-enclosed seas is gaining attention since microplastics are more likely to accumulate there. However, research on the vertical distribution of microplastics and impact factors is still limited. In this study, we focus on the Baltic Sea, which has distinguished salinity stratification, and we assume that the resulting strong density stratification (halocline) can influence the vertical distribution of microplastics in the water column. Therefore, we analyzed the vertical abundance distribution, the composition, and the sizes of microplastics (27.3–5,000.0 μm) in the Baltic Sea. The results showed that microplastics comprising fibers, fragments, and films occurred throughout the water column at an abundance of 1.1–27.7 items L−1. The abundance of microplastics (3.2–27.7 items L−1) at haloclines was significantly higher than those at other water depths except the near surfaces (p < 0.05), contributing 24.1–53.2% of the microplastics in the whole water column. Small microplastics (<100 μm) were more likely to accumulate in the water layers above halocline. Moreover, the current with high turbidity might be another carrier of microplastics in the near-bottom water layer due to its strong correlation with microplastics abundance. This study provides valuable evidence for the accumulation trend of microplastics in water columns and its influencing factors in the semi-enclosed marginal sea. Further research on the vertical distribution of microplastics under the control of multiple factors should be conducted in the future.
Introduction
The widespread occurrence of microplastics as an anthropogenic fingerprint in the environment has received global attention (Rochman, 2018). Microplastics can persist for a long time in the marine environment owing to their durable properties and are readily transported over long distances from sources by wind and currents (Barnes et al., 2009; Isobe et al., 2014; Zhang, 2017; Zhang et al., 2021). During this period, there is an increased possibility of microplastics compounding environmental chemicals (i.e., metals or persistent organic pollutants) and microorganisms (Frias et al., 2010; Reisser et al., 2014; Brennecke et al., 2016). This may lead to changes in their density and influence their fate by sinking, further damaging marine organisms, living at different depths of the water column, or even ecosystems (Kowalski et al., 2016; Jeong et al., 2018; Paul-Pont et al., 2018; Zhang et al., 2020a; Uurasjärvi et al., 2021).
Until recently, most studies focus on the amounts of marine microplastic pollution in the surface or subsurface layers (Lusher et al., 2015; Song et al., 2015; Zhang et al., 2017; Kanhai et al., 2018). However, it is difficult to determine the number of microplastics in the ocean without investigating their abundance at different depths. Polypropylene (PP, ρ = 0.85–0.92 g cm−3), polyethylene (PE, ρ = 0.89–0.98 g cm−3), expandable polystyrene (EPS, ρ = 0.01–0.05 g cm−3), polyethylene terephthalate (PET, ρ = 1.38 g cm−3), and rayon (ρ = 1.46–1.54 g cm−3) are considered common polymer types in the marine environment (Dai et al., 2018; Silvestrova and Stepanova, 2020; Zhang et al., 2020b). Although the densities of these plastics are lower or higher than the density of seawater, there is growing evidence that they can be transported up and down the water column by vertical mixing, and get to different layers of the water column (Kukulka et al., 2012; Gorokhova, 2015; Dai et al., 2018; Cincinelli et al., 2019; Rebeca et al., 2021; Uurasjärvi et al., 2021) and sediments (Van Cauwenberghe et al., 2013; Bergmann et al., 2017; Barrett et al., 2020; Reineccius et al., 2020) and do not just simply float on the surface. However, an understanding of the impact factors on the vertical distribution of microplastics is still limited.
The studies on microplastic vertical distribution are usually based on predictions and measurements made in laboratory experiments based on particle shape, size, and density, and also different environmental factors (Cole et al., 2016; Kowalski et al., 2016). For example, currents, aggregation with organic and inorganic particles, and biofouling can affect the transportation, sinking, and distribution of microplastics in the marine environment (Long et al., 2015; Suaria et al., 2016; Kaiser et al., 2017; Kooi et al., 2017; Wu et al., 2020). However, there is still not enough research based on the in situ data on microplastics to understand the vertical distribution in the water column and the factors determining it. More and more studies have indicated that the densities of virgin plastics cannot be considered as a decisive factor affecting the vertical distribution of microplastics in the seawater (Kaiser et al., 2017; Chen et al., 2021; Karkanorachaki et al., 2021). It seems that environmental factors play an important role in the vertical distribution of microplastics. Some studies show that microplastics accumulate mainly at the near-surface, the near-bottom, or specific layers due to environmental factors including storms, wind-driven mixing events, resuspension, and attachment (Lattin et al., 2004; Corcoran, 2015; Reisser et al., 2015; Katija et al., 2017; Martin et al., 2017; Dai et al., 2018; Song et al., 2018; Choy et al., 2019). For instance, Choy et al. (2019) found the highest abundance of microplastics at depths between 200 and 600 m (roughly 15 particles m−3) in Monterey Bay because of the contribution of the pelagic particle feeders. Dai et al. (2018) reported that the turbulence caused microplastics to accumulate mainly at 5–15 m depth with an abundance of 0.6–23.0 items L−1 in the Bohai Sea. The halocline, which was caused by a strong, vertical salinity gradient within a body of seawater, usually is a distinctive feature of the semi-enclosed sea (Ferentinos et al., 2010; Virtasalo et al., 2011). It was believed to affect the vertical distribution of the particles including plastics in the water column, but there is little unequivocal evidence for the small microplastics (Gorokhova, 2015; Bagaev et al., 2017). Moreover, turbidity currents prevail at the near-bottom layers in the ocean including semi-enclosed seas, but the ability of bottomed currents to transport and accumulate plastics is essentially unknown (Pohl et al., 2020).
The Baltic Sea is a typical semi-enclosed sea. Microplastic pollution in the Baltic Sea has been reported in recent years, and most investigations have focused on the (near) surface water (Setälä et al., 2016; Gewert et al., 2017; Schönlau et al., 2020; Hänninen et al., 2021), seafloor, and beach sediments (Stolte et al., 2015; Talvitie et al., 2015; Graca et al., 2017; Zobkov and Esiukova, 2017; Kammann et al., 2018; Urban-Malinga et al., 2018). There are only a few studies focused on the vertical distribution of microplastics, but research based on the multi-regions, multi-depths, and relevant impact factors of microplastics distribution are limited (Gorokhova, 2015; Bagaev et al., 2017, 2018; Zobkov et al., 2019; Uurasjärvi et al., 2021). Moreover, as a semi-enclosed sea, the density of the seawater in the Baltic Sea varies considerably with temperature and salinity such that a microplastic that floats may sink as soon as the salinity or temperature changes (Bagaev et al., 2017; Uurasjärvi et al., 2021). Previous studies indicate that the halocline may affect the vertical distribution of the plastic particles in the Baltic Sea, but small microplastics (especially < 100 μm) were ignored (Gorokhova, 2015; Bagaev et al., 2017; Uurasjärvi et al., 2021).
Here, we focused on the distribution characteristics of microplastics with a wide size range of 25.0–5,000.0 μm aiming to (1) clarify the differences of the regional and vertical distribution of microplastics. We tested the hypotheses that, (2) the strong salinity stratification can facilitate the accumulation of microplastics in the water column. Finally, (3) the turbidity can influence the abundance of microplastics at the near-bottom of the water column. This study provides valuable evidence for the accumulation trend of microplastics in water columns and impacts of halocline and turbidity on the vertical distribution of microplastics in the semi-enclosed Baltic Sea.
Materials and Methods
The Study Area
The Baltic Sea (53°-66°N, 10°-30°E), with average depth of 55 m and a maximum depth of 459 m, is surrounded by nine countries and is the largest inland brackish adjacent sea of the Atlantic Ocean. The water temperature of the Baltic Sea varies significantly depending on the exact location, season, and depth. The salinity in the Baltic Sea is much lower than that of ocean water, mainly as a result of abundant freshwater runoff from the surrounding rivers and streams. There are more than 250 streams that drain a basin of about a volume of 660 km3 year−1 to the Baltic Sea. Moreover, due to the inflow of the North Sea water with higher salinity from the west, the bottom of the Baltic Sea is saltier than the surface. It creates a vertical stratification named halocline of the water column, which acts as a barrier for the exchange of oxygen, nutrients, and particles (Maciejewska and Pempkowiak, 2014; Liblik and Lips, 2019).
Vertical Sampling of Water and Sediment
The water samples were taken at 12 locations during a routine winter monitoring cruise of the Leibniz Institute for Baltic Sea Research (Warnemünde, Germany) in February 2019, and two locations in the northern Baltic Sea were added to the data set in May 2019 covering different basins of the Baltic Sea to obtain the spatial diversity of sampling. The sampling stations were located in the nearshore and offshore zones, covering Arkona Basin, Bornholm Basin, Gotland Basin, Gulf of Bothnia, Northern Baltic Proper, Lübeck Bay, Kiel Bight, and Fehmarn Strait as shown in Figure 1 and Supplementary Table 1. About 10 L of seawater was collected at each selected water depth between the near surface (1–3 m from the seawater surface) and the bottom (1–5 m from the seabed surface with a maximum sampled depth of up to 437 m) using a 12-bottle rosette sampler system (SBE 9 Carousel Water Sampler) connected to a conductivity, temperature, and depth sensor (CTD) system (SBE911Plus, Sea-Bird, Bellevue, WA, USA) and equipped with 5-L free flow bottles. In addition to the water salinity, other water parameters including temperature, dissolved oxygen, chlorophyll a, and turbidity were also measured in situ by the CTD. Different water depths were chosen (the near surface and the depths of 10, 20, 50, 100, and 200 m until the near-bottom water layer) based on the station-specific depth and the depth of halocline for sampling (see Supplementary Table 1). About 5 L of water sample with two replicates collected at each depth and station was stored in a clean glass bottle for microplastic analysis.
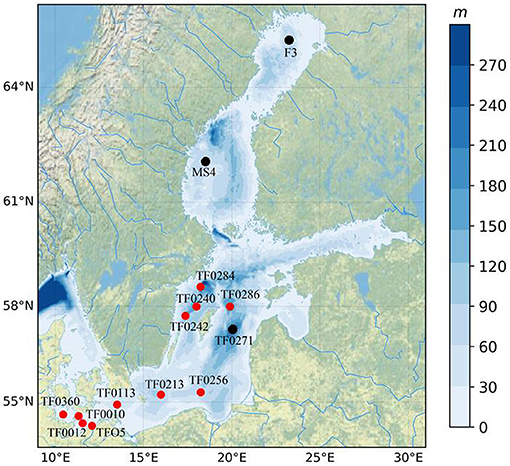
Figure 1. Regional distribution of sampling stations for water and sediment in the Baltic Sea. (Red dot, both water, and sediment samples were sampled; black dot, only water samples were sampled. Sediment samples at stations TF0271, MS4, and F3 were not obtained due to bad weather conditions and resulting time limitations).
The bottom sediments (top 3 cm) at each station were collected using a stainless multicore with tubes of 10 cm diameter. At each sampling site, three subsamples were collected and then bulked to form one composite sample. All the samples were put into the clean (pre-washed) aluminum containers respectively and stored at low temperature.
Microplastics Extraction From Water and Sediment
Extraction of the microplastics from the water samples was conducted using the method of Dai et al. (2018). Briefly, each 5-L water sample was filtered through a 47-mm diameter cellulose nitrate filter with 5 μm porosity (Whatman AE98, Cytiva, Marlborough, MA, USA) immediately by using the vacuum filtration device in the filtration laboratory on-board. The samples were not treated with hydrogen peroxide or enzyme-digested because they were needed for subsequent surface micromorphology analysis, and samples collected during the winter had low organic matter contents (Cole et al., 2014; Bagaev et al., 2018). Each filter was stored in cleaned glass Petri dishes with a diameter of 60 mm. The dishes with filters were sealed using glass lids, wrapped with aluminum foil, and stored at 4°C for further observation.
The microplastics were extracted from the sediment samples by density separation (Thompson et al., 2004; Vianello et al., 2013). All sediment samples collected were dried in a vacuum freeze dryer and filtered through a stainless-steel screen with a 2-mm aperture for removing large pieces of biological residues. Dried sediment (5.0 g) was placed in a pre-cleaned 250-ml tall glass beaker, mixed and dispersed thoroughly with 50-ml saturated sodium chloride solution (ρ = 1.2 g ml−1, filtered through a 5 μm cellulose nitrate filter), and then more saturated sodium chloride solution was continually added to the beaker until the maximum mark line. Two replicates were conducted simultaneously. The glass rod was used to stir thoroughly for 2 min to make the sediment evenly dispersed in the solution, and then the substance adhered to the glass rod was rinsed into the beaker with sodium chloride solution. The beaker was covered with aluminum foil and settled for 24 h for microplastic separation. Floating materials were collected by filtration using a cellulose nitrate filter with 5 μm pore diameter (Whatman AE 98, Cytiva, Marlborough, MA, USA).
Observation, Identification, and Quantification of Microplastics
Preliminary observation and quantification of the microplastics in the filtered residues were conducted by visual examination under a Zeiss Discovery V8 stereomicroscope with ×80 magnification (Zeiss AG, Oberkochen, Germany). All filters were observed under the eyepiece with the aid of stainless-steel tweezers and dissection needles. Putative microplastics were counted based on the colors and morphology types of particles previously published in photographs and references (Nor and Obbard, 2014; Bagaev et al., 2017; Zhou et al., 2018). They were then photographed under the stereomicroscope equipped with a charge-coupled device (CCD) camera, and the shape type and color of every suspected microplastic were recorded. The quantification and the size measurement, including the length and the width of all potential microplastics, were carried out using the ImageJ program (National Institutes of Health, Bethesda, MD, USA). A total of 2,557 potential microplastics comprising 2,268 fibers, 280 fragments, and nine films were recorded. Due to time constraints, only a limited number of microplastics have been analyzed using micro-Fourier transform infrared spectroscopy (μ-FTIR). For doing it, we classified them into different groups and subgroups based on their color, shape, and morphologies (Supplementary Table 3). Microplastics and suspected microplastics comprising 227 fibers, 20 fragments, and four films were selected based on the different colors and shape morphologies from each group (Supplementary Table 3). They were identified using μ-FTIR spectrometry (Spotlight 400, Perkin Elmer, Waltham, MA, USA) with spectral region 750–4,000 cm−1 and a resolution of 4 cm−1 at a rate of 16 scans per analysis. The spectra obtained were compared with the standard database provided by the OMNIC software (Thermo Scientific Inc., Waltham, MA, USA). The analysis provided us with a proportion of the real number of plastic particles and the non-plastic particles in the original group and allowed us to correct our original counts in each subgroup in the same proportion according to the identification results and morphology information. Rayon is an artificial semi-synthetic material, which is different from natural materials, such as cotton and wood (Obbard et al., 2014; Gago et al., 2017). Rayon makes up a significant proportion of synthetic microparticles found in the marine environment (Lusher et al., 2013; Suaria et al., 2020) and has a large proportion in this study. Therefore, we classified rayon into a microplastics group for further examination and discussion. Moreover, in this study, we also considered the poly (N-methyl acrylamide) as a plastic polymer based on its chemical structure and acrylic properties. Finally, a total of 88 particles were not plastics in the groups of selected particles. Based on the proportion of identification results and the information on the recorded particles, all non-plastics were removed, and a total of 1,449 particles comprising 1,265 fibers, 176 fragments, and eight films were regarded as microplastics and analyzed further.
The microplastics from the near-surface water (two particles), haloclines (four particles), the near-bottom water (four particles), and sediments (three particles) were selected randomly to observe the surface substances using a MERLIN VP compact (Carl Zeiss, Oberkochen, Germany) in a vacuum. Samples were dried at room temperature first, and then placed on pin stubs with adhesive carbon pads and sputter coated with iridium by argon plasma at high voltage before analysis. The scanning electron microscope (SEM) was operated at 15.0-kV electron accelerating voltage.
Quality Assurance and Quality Control
All containers and instruments were cleaned with MilliQ water and covered with aluminum foil before use. Sample processing and separation were carried out in a laboratory specified for marine-particle analysis at the IOW (Germany), whereas the filtration of water samples was carried out onboard. Non-textile jumpsuits were worn during the experimental work to prevent contamination from fibers and other microplastic particles. Procedural blanks using MilliQ water were run in parallel with water samples. Blanks using filtered NaCl solution were run parallel with the processing of the sediment samples in the laboratory at IOW. Only blue fibers were observed in the blanks with one to four particles in 5-L MilliQ. Only two to three blue and transparent fibers were counted in the blanks during the sediment analysis. The quantities of the microplastics in all samples were calibrated based on the blanks.
Statistical Analysis
Microplastic abundances in water or sediments are presented as the number of microplastic particles per liter or per gram dry weight (items L−1 or items g−1 d.w.). Data analysis was conducted using Microsoft Excel 2010 (Microsoft Corp., Redmond, WA, USA) and OriginPro 8.0 (OriginLab Corporation, Northampton, MA, USA). The Pearson correlation was used for the correlation analysis between the abundance of microplastics and temperature, salinity, turbidity, dissolved oxygen, or chlorophyll a. One-way ANOVA was conducted to compare the difference of microplastics abundance among different regions and sampling depths using the IBM SPSS 20.0 software package (IBM Corp., Armonk, NY, USA), and the mapping of the spatial distribution of microplastics was conducted using ArcGIS10.2 (ESRI, Redlands, CA, USA).
Results
Occurrence Characteristics of Microplastics in the Baltic Sea
The range of microplastic abundance was 1.1–27.7 items L−1 with the mean abundance of 5.8 ± 5.0 items L−1 in the water (for the original data, see Supplementary Table 4). The highest abundance of microplastics was found at station TF0286 in the Northern Baltic Proper and the lowest at station MS4 in the north of the Baltic Sea (Bothnia Sea). In the horizontal distribution, the microplastic abundance in the near-surface layers of the southern (8.8 ± 3.8 items L−1) and central (6.0 ± 7.8 items L−1) regions of the Baltic Sea was significantly higher than in the northern region (1.0 ± 0.8 items L−1), (p < 0.05). The vertical distribution of microplastics showed different patterns that changed with depth among the stations (Figure 2). The coefficients of variation of microplastics abundance at different depths within the water column were 0.2–0.9 (cv < 1, Table 1), showing small differences.
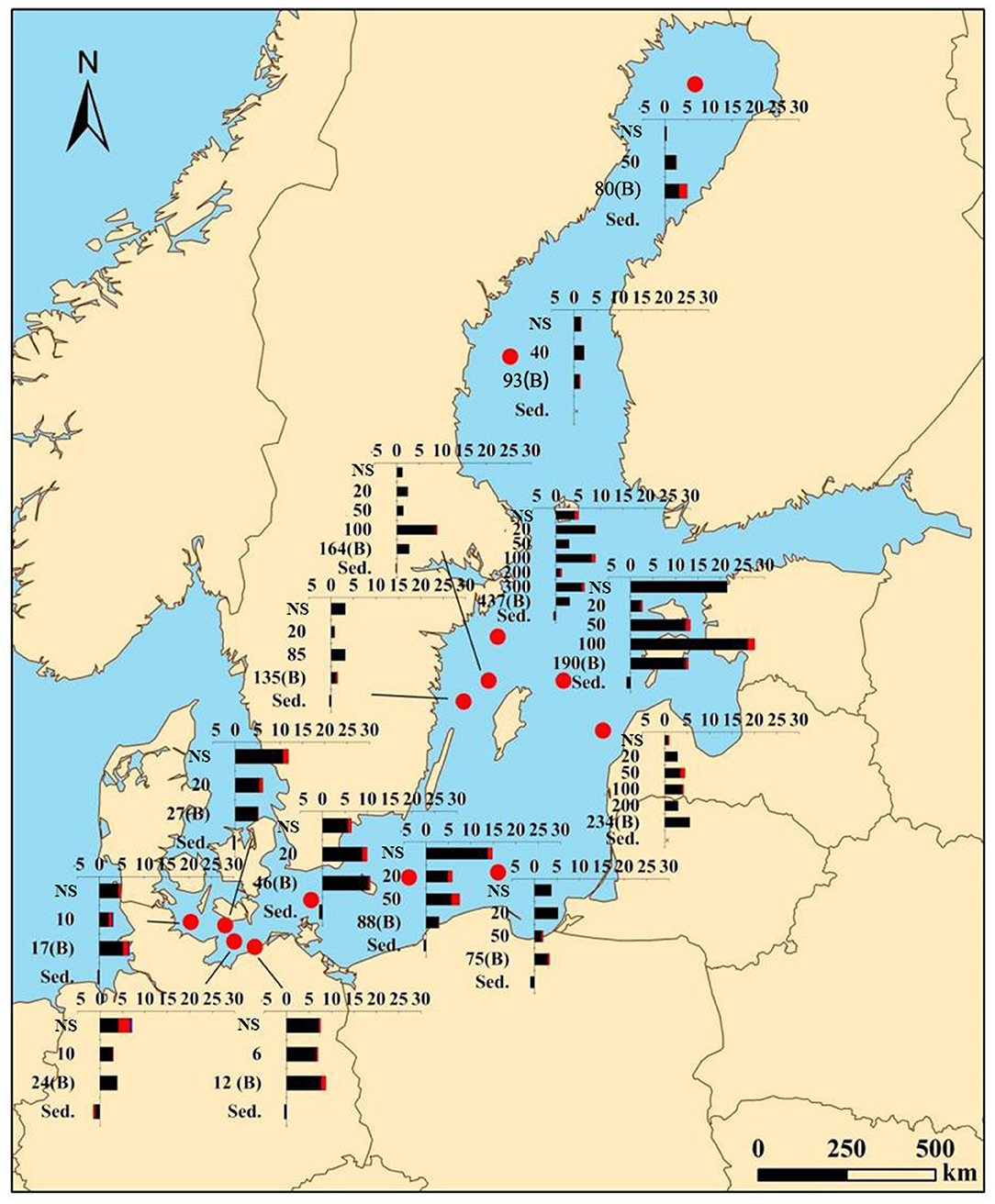
Figure 2. Distribution of different microplastic types in the water and sediments in the Baltic Sea. The unit of the abundance of microplastics in the water column and sediments are items L−1 and items g−1 d.w.; “NS,” near the surface; “B,” near-bottom layer; “Sed.,” sediment; the Y-axis represents sampling depth (m); the X-axis represents the abundance of microplastics.
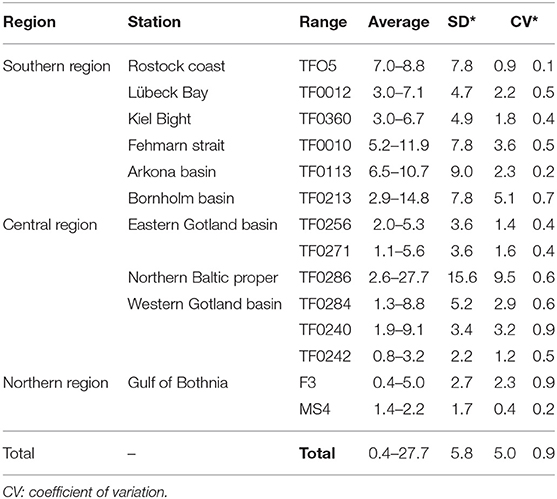
Table 1. Variation in the vertical distribution of microplastics (items L−1) in the water column in different regions of the Baltic Sea.
Three shape types of microplastics comprising fibers, fragments, and films were found in the Baltic Sea (Supplementary Figure 1). The fibers were the most popular type with a mean abundance of 5.2 ± 4.8 items L−1 (~90.4% of all microplastics) in the Baltic Sea water (Figure 3A and Supplementary Figure 6). Fragments were the second most abundant type with a mean abundance in the water of 0.5 ± 0.6 items L−1 (~9.4% of all microplastics), (Figure 3A). Films showed the lowest abundance of only 0.01 ± 0.07 items L−1 (~0.3% of all microplastics), (Figure 3A). Films were found only at two sampling stations in the southern area (TF0012 and TF0360) close to the coast. The microplastic shape types were less diverse in the sediments than in the water column. Here, only fibers (0.6 ± 0.4 items g−1 d.w.) and fragments (0.1 ± 0.2 items g−1 d.w.) were found (Supplementary Figure 7). Black and blue microplastic particles were dominant in both water and sediments (Supplementary Figure 2). The microplastic polymers consisted of rayon (81.0%), PET (4.9%), PP (4.3%), polyamide 6 (PA6), (3.7%), polybutene (PB), (2.5%), polytetrafluoroethylene (PTFE), (1.8%), and poly (N-methyl acrylamide), (1.8%) based on all identified polymer particles (Supplementary Figures 3, 4). The histogram presents the size distribution of microplastics in waters and sediments (Figure 3B and Supplementary Figure 8). Microplastics with size range < 1 mm dominated both in water (77.5%) and sediments (91.6%) sampled. However, the size distributions of microplastics showed one peak at 300–1,000 μm in water but 100–300 μm in sediments.
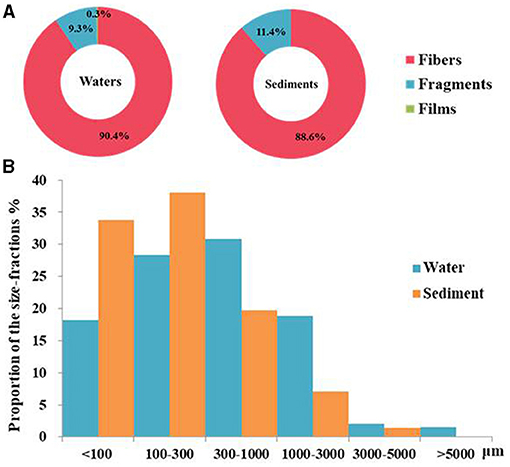
Figure 3. The shape types (%) (A) and the size fractions (%) (B) of the microplastics in water and the sediments of the Baltic Sea.
Microplastics at the Haloclines
The six sampling stations TF0360, TF0213, TF0286, TF0284, TF0240, and TF0242 (Figure 1) with strong haloclines (Supplementary Table 1) showed significant accumulation of microplastics in this distinct layer (Figure 4). By contrast, other sampling stations (e.g., TF0010 and TF0012) without evident haloclines showed microplastic accumulation at the near-surface layer. The microplastic abundance in the halocline at ~16 m depth (a salinity difference of 3.2) of station TF0360 was 6.7 items L−1, accounting for 45.9% of them in the entire water column. This was 1.4–2.2 times higher than at the other two water depths with microplastic abundances of 3.0 and 4.9 items L−1, respectively (Figure 4A). At station TF0213, the abundance of microplastics at the halocline (7.5 items L−1) was lower than at the near-surface layer (14.8 items L−1) but significantly higher than at other layers (2.9–5.8 items L−1), (Figure 4B). In the deeper basins at stations TF0286, TF0284, TF0240, and TF0242, the abundances of microplastics in the halocline at a depth of ~100 m were all higher than at the other depths (Figures 4C–F). Among them, the highest microplastic abundance in the water columns of the Baltic Sea occurred in the halocline of station TF0286 with up to 27.7 items L−1. In general, the microplastic abundance in the halocline at the above six stations ranged from 3.2 to 27.7 items L−1 (mean 10.5 ± 8.7 items L−1), contributing 24.1–53.2% of the microplastics in the whole-water column and in total 33.8% at all sampling depths of the six stations.
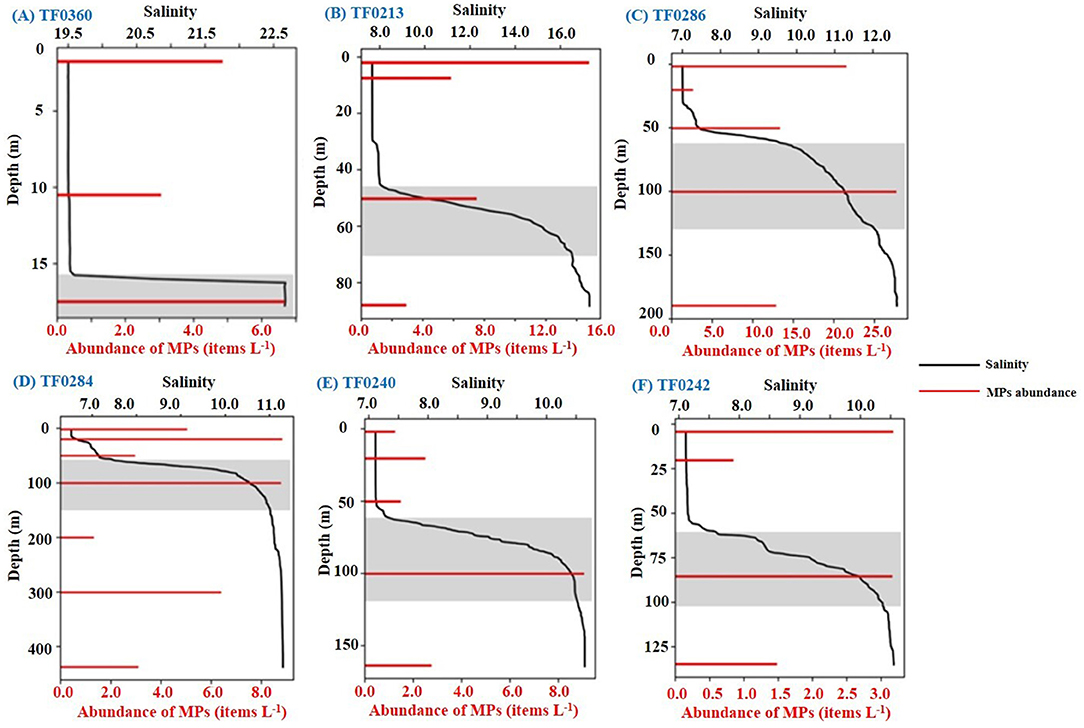
Figure 4. Changes in microplastic abundance with salinity over depth in the water column at different stations (A–F). Shadows in the figure indicate the locations of the halocline in the corresponding water column and their microplastics abundance. “MPs” refers to microplastics. The Y-axis represents sampling depth (m), the X-axis of the top (in black) represents the salinity, and the X-axis of the bottom (in red) represents microplastics abundance (items L−1).
In terms of types, fibers pre-dominated in the halocline, accounting for 90.3%, whereas fragments and films contributed only 9.4 and 0.3%, respectively, consistent with the overall distribution of microplastic types in the Baltic Sea. Heavy polymers (PET, ρ = 1.38 g cm−3) with densities higher than seawater and light polymers (PP, ρ = 0.85–0.92 g cm−3), together with the semisynthetic polymers (rayon), were detected in the halocline. The size of microplastics in the halocline showed a wide range of 30.0–4,972.0 μm, with a mean size of 694.0 ± 860.6 μm. The proportion of microplastics with size < 300 μm accounted for nearly 50% in the halocline and almost 40% of microplastics at all water depths. Moreover, the percentage of microplastics with size < 100 μm in the layers above the haloclines (including haloclines, 18.1%) was higher than those in the layers below the halocline (11.9%), but there were no discernible differences (p > 0.05), (Supplementary Figure 5).
Microplastics at the Near-Bottom Turbid Water Layer
The results here showed that microplastics tend to accumulate in the near-bottom layer in addition to the halocline in the Baltic Sea (Figure 2). The mean abundance of microplastics was 5.3 ± 3.5 items L−1 at the near-bottom layer, lower than at the near-surface layer (6.0 ± 6.5 items L−1) and the halocline (10.5 ± 8.7 items L−1), but higher than at the other water depths (4.4 ± 3.1 items L−1), (Supplementary Table 4). Fibers accounted for 90.4% (4.8 ± 4.3 items L−1), but fragments and films accounted for only 9.3% (0.5 ± 0.5 items L−1) and 0.3% (0.01 ± 0.05 items L−1), respectively, consistent with the distribution of the microplastic types at the haloclines. The most abundant polymer was semisynthetic rayon, accounting for 81.2%, whereas the polymers PET, PA6, and PB accounted for only 3.1–6.3%. The mean size of the microplastics at the near-bottom layer was 591.0 ± 840.0 μm with a high percentage (53.6%) of short particles (< 300 μm).
Discussions
High Levels of Microplastic Pollution in the Semi-enclosed Baltic Sea
Surprisingly, the abundance of microplastics in the Baltic Sea is one order of magnitude higher than that in the open sea such as the North Sea (23.0 items m−3), (Roscher et al., 2021), (Supplementary Table 2). Similar microplastic abundances in the Baltic Sea were also reported by Bagaev et al. (2018) and Tamminga et al. (2018). Previous studies on the semi-enclosed seas, including the Bohai Sea and the Maowei Sea, showed high levels of microplastic pollution, with microplastic abundance up to 7,000 items m−3 (Dai et al., 2018; Zhu et al., 2019). They indicated that semi-enclosed seas are particularly susceptible to trapping microplastics, due to the emission of micropollutants from industrial and domestic uses, slow flushing, and restricted water exchange (Korpinen et al., 2012; Li et al., 2018; Schmidt et al., 2020). The microplastic abundance in the near-surface layers of the southern and central regions of the Baltic Sea was significantly higher than in the northern region in this study. This is because there are more intense anthropogenic activities including harbors, shipping, wastewater treatment plants, and the heavier load of pollutants in rivers caused by urban areas in the south than those in central and northern areas (Stankiewicz, 2010; Talvitie et al., 2015; Gewert et al., 2017; Rotander and Kärrman, 2019).
Fiber was the major contributor of types of microplastic pollution in the Baltic Sea. It shows clear domination of fibers at all depths of the water column and in the surface sediments, consistent with other surveys of microplastics in the Baltic Sea (e.g., Bagaev et al., 2017; 2018; Tamminga et al., 2018; Zobkov et al., 2019). Rayon fibers were the most prevalent type and distributed throughout the water columns and sediments in the Baltic Sea, which is consistent with the study on microplastics in the Coral Reef Systems (Ding et al., 2019), Maowei Sea (Zhu et al., 2019), Arctic Sea ice (Obbard et al., 2014), and in Arctic polar waters (Lusher et al., 2015). Numerous studies have indicated the widespread occurrence of rayon fibers throughout marine environments (Lusher et al., 2013; Remy et al., 2015; Taylor et al., 2016; Sanchez-Vidal et al., 2018; Suaria et al., 2020). However, it is controversial to classify rayon as a microplastic worldwide (Peeken et al., 2018). Clothing made from synthetic and semisynthetic fibers, such as rayon, PET, and PA6 is common and therefore potential source of contamination in the Baltic Sea.
Haloclines Facilitate the Trapping of Microplastics
Interestingly, a distinct accumulation of microplastics at the specific sampling depth (the haloclines, most of them roughly at 50 or 100 m) at the sampling stations TF0360, TF0213, TF0286, TF0284, TF0240, and TF0242 was revealed. It indicated that the halocline is a key layer for the accumulation of microplastics in the Baltic Sea. The size distributions of microplastic particles at the halocline, the water layers above halocline, and the water layers below halocline were presented in Supplementary Figure 5. The proportion of microplastics with the size of < 100 μm at halocline was 44.9%, which was higher than those at the water layers above (41.7%) or below (35.1%) halocline. This leads to the presumption that the halocline may act as a barrier to small microplastics (< 100 μm) and prevent them from sinking. Although previous studies have given valuable evidence that microplastics can accumulate at the halocline, most of them have been based on the large microplastics (>175 μm) and overlooked the difference between the size of the microplastics in the haloclines and other water layers (Gorokhova, 2015; Bagaev et al., 2017; Uurasjärvi et al., 2021). In this study, the vertical distribution (including the halocline) of microplastics with a wider size range was analyzed and has confirmed that the halocline tends to accumulate small particles with size <100 μm. We suspect that this probably is related to the larger specific surface area of the small-sized microplastic particles by comparing with the larger ones. Because the small-sized particles with the larger specific surface area would lose buoyancy much more rapidly than larger ones, causing them easy to sink (Fazey and Ryan, 2016; Dai et al., 2018).
Furthermore, we selected the water column and sediment sampled at stations TF0286 and TF0284 as representative examples to observe the attachments on the microplastic surfaces at different depths, aiming for exploring the vertical migration of microplastics (Figure 5). The elements, including silicon and aluminum in addition to organisms, were detected on the surfaces of the micro-fibers (PP) found at the halocline (Figures 5A,B). However, fewer attachments were observed on the surface of particles at near-surface water layers by comparing with those at haloclines (Supplementary Figure 9). We assume that attachments may change the buoyancy of microplastics with low density and cause them to sink from upper layers and to access the halocline (Lattin et al., 2004; Katija et al., 2017; Uurasjärvi et al., 2021). However, they may not have enough negative buoyancy to escape from the halocline and continue sinking and ultimately accumulate in the halocline. It was assumed that microplastics first accumulate to halocline, when present, and sink slowly toward the bottom ultimately (Uurasjärvi et al., 2021). In this study, we detected metals, such as silver accumulating on the surface of the light fibers in the near-bottom layer (Figures 5C,D) and sulfur–iron mineral aggregates attached to the surface of fibers in sediments (Figures 5E,F), but they were not detected on the particle surfaces in the halocline. This indicates that microplastics with high-density elements (such as silver) attached might have enough negative buoyancy to overcome the halocline and sink continuously into the near-bottom water layer. However, this study did not consider the water hydrodynamic influence, which is also important to affect the microplastic transportation and accumulation (Zalesny et al., 2014; Zhang, 2017). Further studies are required to prove the hypothesis.
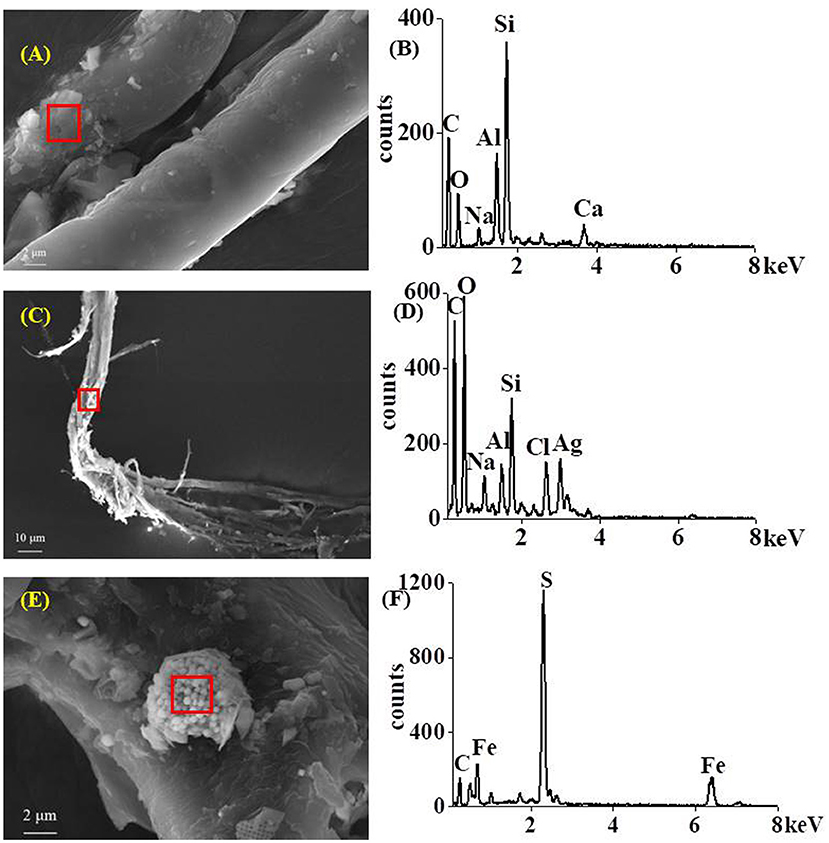
Figure 5. Scanning electron microscope (SEM) images and energy spectra showing the substances on the surfaces of micro-fibers in the halocline [PP, (A,B)] and near-bottom layer [PB, (C,D)] and sediment [PP, (E,F)] in the Baltic Sea.
Turbidity Affects the Accumulation of Microplastics at the Near-Bottom Water Layer
The microplastic abundance and size distribution in sediments were analyzed for discussing microplastic accumulation at near-bottom layers. In sediments, the range of microplastic abundance was 0.2–1.5 items g−1 d.w. with the mean abundance of 0.6 ± 0.4 items g−1 d.w. Moreover, the higher proportion (53.6%) of short microplastics (<300 μm) at the near-bottom water is consistent with those in sediments (<300 μm, 71.8%), which indicated that the short microplastics at the near-bottom layers may come from bottom sediments in addition to upper water layers. We assumed that it is related to the water turbidity (e.g., particles resuspension from sediments). We further used the correlation analysis between the microplastic abundance and the salinity, dissolved oxygen, turbidity, and chlorophyll in the near-bottom water layers to explain the potential impact factor on the accumulation of microplastics (data refer to Supplementary Table 1). A strong correlation (r = 0.70) between the abundance of microplastics and the turbidity of the near-bottom water layer was found (Table 2, Figure 6). The near-bottom currents carrying turbid water usually occur when sediment particles are suspended above the seafloor by waves or incoming river plumes, and the turbid and relatively dense seawater cascades down due to the pull of gravity (Azpiroz-Zabala et al., 2017; Paull et al., 2018). The bottom current can transport sand, clay, organic carbon, and microplastics (Galy et al., 2007; Gwiazda et al., 2015). Therefore, bottom currents with high turbidity play a distinct role in the dispersal or accumulation of microplastics in the bottom water and sediments (Ballent et al., 2013; Pohl et al., 2020).
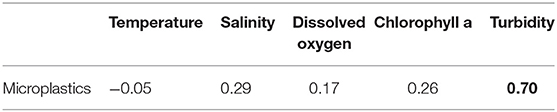
Table 2. Pearson correlation coefficients among different water parameters and microplastics in the near-bottom layer of the Baltic sea (significant correlation is given in bold).
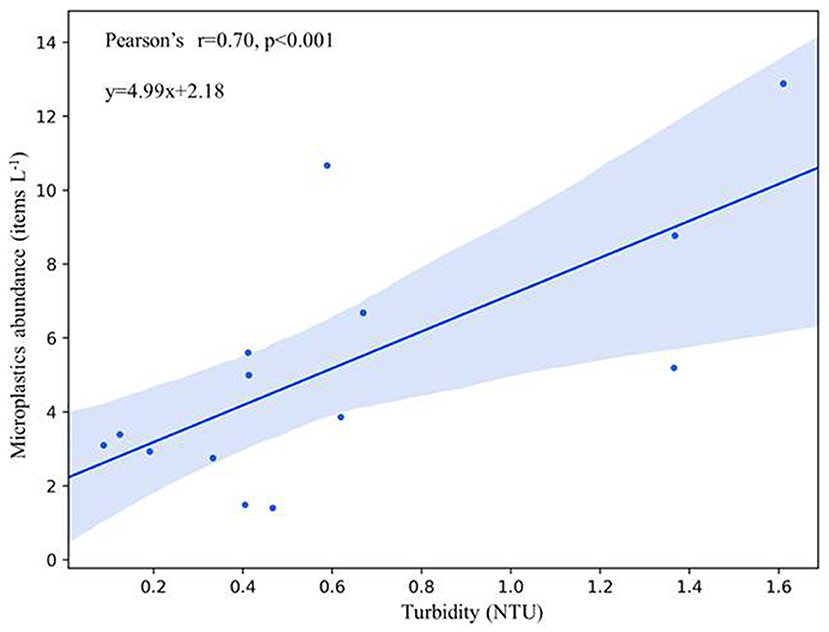
Figure 6. Correlations between the microplastics abundance and turbidity at the near-bottom water layer. Linear least squares regression relationships were estimated.
Moreover, we found heavy metals (silver and iron) attached on the surface of light microplastics in the near-bottom water layer and sediments (Figures 5C–F), which indicated that it is difficult to keep these microplastics retain in water even though there is high salinity in the near-bottom water. However, near-bottom currents can retain microplastics in resuspension, and stronger and sheared turbulent pulses on the surface of the seabed can prevent the microplastics from sinking (Lemckert et al., 2004; Bagaev et al., 2017). The process of resuspension can affect the turbidity of the near-bottom water by causing the particles, including microplastics, organic particles, and minerals, to reenter the water body. These suspended organic or inorganic particles can also hinder the microplastics from sinking. Therefore, the turbidity of the near-bottom water probably can indicate microplastic abundance at the near-bottom water in the Baltic Sea.
Conclusions
The vertical distribution of microplastics in the water column shows spatial heterogeneity in the Baltic Sea. Fibers and fragments were found throughout the water column, whereas films occurred only at the (near) surface water layer. The abundance of the microplastics at the near-surface, the halocline, and the near-bottom layer were considerably higher than those in other water layers. Strong halocline stratification and turbidity affected the vertical distribution of the microplastics and facilitated the accumulation of microplastics at those layers. The occurrence of microplastics in the surface sediments provides a potential source of particles to the near-bottom water. Studies on the sinking behavior of microplastics under multiple impact factors, especially material fouling in the marine environment, are needed.
Data Availability Statement
The original contributions presented in the study are included in the article/Supplementary Material, further inquiries can be directed to the corresponding author.
Author Contributions
QZ collected samples, conducted the laboratory analysis of processing the samples, isolating and counting microplastics, the statistical analyses, and wrote the manuscript. JY and CF conducted spectral analysis on microplastics identification and generated the figures and maps. YL assisted with method development and contributed to editing. CT and JW conceptualized the study, contributed to editing, and acquired funding. All authors contributed to the article and approved the submitted version.
Funding
This work was funded by the Sino-German Cooperation Project, BMBF (No. 03F0786A), the Key Research Program of Frontier Sciences, CAS (No. QYZDJ-SSW-DQC015), and the National Key Research and Development Program of China (2016YFC1402202).
Conflict of Interest
The authors declare that the research was conducted in the absence of any commercial or financial relationships that could be construed as a potential conflict of interest.
Publisher's Note
All claims expressed in this article are solely those of the authors and do not necessarily represent those of their affiliated organizations, or those of the publisher, the editors and the reviewers. Any product that may be evaluated in this article, or claim that may be made by its manufacturer, is not guaranteed or endorsed by the publisher.
Acknowledgments
We acknowledge the support by the crew of the RV Elisabeth Mann Borgese and the scientists involved in the regular monitoring efforts at IOW for their support during sample collection. We thank Professor Li Xu at Beijing Research Center for Agricultural Standards and Testing, Beijing Academy of Agriculture and Forestry Sciences, for support in microplastic sample identification. We also thank Dr. Peter Christie at the Institute of Soil Science, Chinese Academy of Sciences, for the revision of the English language.
Supplementary Material
The Supplementary Material for this article can be found online at: https://www.frontiersin.org/articles/10.3389/fmars.2021.761566/full#supplementary-material
References
Azpiroz-Zabala, M., Cartigny, M. J. B., Talling, P. J., Parsons, D. R., Sumner, E. J., and Clare, M. A. (2017). Newly recognized turbidity current structure can explain prolonged flushing of submarine canyons. Sci. Adv. 3, 1–12. doi: 10.1126/sciadv.1700200
Bagaev, A., Khatmullina, L., and Chubarenko, I. (2018). Anthropogenic microlitter in the Baltic sea water column. Mar. Pollut. Bull. 129, 918–923. doi: 10.1016/j.marpolbul.2017.10.049
Bagaev, A., Mizyuk, A., Khatmullina, L., Isachenko, I., and Chubarenko, I. (2017). Anthropogenic fibres in the Baltic sea water column: field data, laboratory and numerical testing of their motion. Sci. Total Environ. 599, 560–571. doi: 10.1016/j.scitotenv.2017.04.185
Ballent, A., Pando, S., Purser, A., Juliano, M. F., and Thomsen, L. (2013). Modelled transport of benthic marine microplastic pollution in the nazaré canyon. Biogeosciences 10, 7957–7970. doi: 10.5194/bg-10-7957-2013
Barnes, D. K., Galgani, F., Thompson, R. C., and Barlaz, M. (2009). Accumulation and fragmentation of plastic debris in global environments. Phil. Trans. R. Soc. B. 364, 1985–1998. doi: 10.1098/rstb.2008.0205
Barrett, J., Chase, Z., Zhang, J., Holl, M. M. B., Willis, K., Williams, A., et al. (2020). Microplastic pollution in deep-sea sediments from the Great Australian Bight. Front. Mar. Sci. 7:808. doi: 10.3389/fmars.2020.576170
Bergmann, M., Wirzberger, V., Krumpen, T., Lorenz, C., Primpke, S., Tekman, M. B., et al. (2017). High quantities of microplastic in Arctic deep-sea sediments from the Hausgarten observatory. Environ. Sci. Tech. 51, 11000–11010. doi: 10.1021/acs.est.7b03331
Brennecke, D., Duarte, B., Paiva, F., Caçador, I., and Canning-Clode, J. (2016). Microplastics as vector for heavy metal contamination from the marine environment. Estuar. Coast. Shelf Sci. 178, 189–195. doi: 10.1016/j.ecss.2015.12.003
Chen, Y., Liu, R., Wu, X., Liu, Y., Fu, J., and Ou, H. (2021). Surface characteristic and sinking behavior modifications of microplastics during potassium permanganate pre-oxidation. J. Hazard. Mater. 422:126855. doi: 10.1016/j.jhazmat.2021.126855
Choy, C. A., Robison, B. H., Gagne, T. O., Erwin, B., Firl, E., Halden, R. U., et al. (2019). The vertical distribution and biological transport of marine microplastics across the epipelagic and mesopelagic water column. Sci. Rep. 9:7843. doi: 10.1038/s41598-019-44117-2
Cincinelli, A., Martellini, T., Guerranti, C., Scopetani, C., Chelazzi, D., and Giarrizzo, T. (2019). A potpourri of microplastics in the sea surface and water column of the Mediterranean sea. TrAC Trend. Anal. Chem. 110, 321–326. doi: 10.1016/j.trac.2018.10.026
Cole, M., Lindeque, P. K., Fileman, E., Clark, J., Lewis, C., Halsband, C., et al. (2016). Microplastics alter the properties and sinking rates of zooplankton faecal pellets. Environ. Sci. Tech. 50, 3239–3246. doi: 10.1021/acs.est.5b05905
Cole, M., Webb, H., Lindeque, P. K., Fileman, E. S., Halsband, C., and Galloway, T. S. (2014). Isolation of microplastics in biota-rich seawater samples and marine organisms. Sci. Rep. 4:4528. doi: 10.1038/srep04528
Corcoran, P. L. (2015). Benthic plastic debris in marine and fresh water environments. Environ. Sci. Proc. Imp. 17, 1363–1369. doi: 10.1039/C5EM00188A
Dai, Z., Zhang, H., Zhou, Q., Tian, Y., Chen, T., Tu, C., et al. (2018). Occurrence of microplastics in the water column and sediment in an inland sea affected by intensive anthropogenic activities. Environ. Pollut. 242, 1557–1565. doi: 10.1016/j.envpol.2018.07.131
Ding, J., Jiang, F., Li, J., Wang, Z., Sun, C., Wang, Z., et al. (2019). Microplastics in the Coral Reef Systems from Xisha Islands of South China sea. Environ. Sci. Tech. 53, 8036–8046. doi: 10.1021/acs.est.9b01452
Fazey, F. M. C., and Ryan, P. G. (2016). Biofouling on buoyant marine plastics: an experimental study into the effect of size on surface longevity. Environ. Pollut. 210, 354–360. doi: 10.1016/j.envpol.2016.01.026
Ferentinos, G., Papatheodorou, G., Geraga, M., Iatrou, M., Fakiris, E., Christodoulou, D., et al. (2010). Fjord water circulation patterns and dysoxic/anoxic conditions in a Mediterranean semi-enclosed embayment in the Amvrakikos Gulf, Greece. Estuar. Coast. Shelf. S. 88, 473–481. doi: 10.1016/j.ecss.2010.05.006
Frias, J. P. G. L., Sobral, P., and Ferreira, A. M. (2010). Organic pollutants in microplastics from two beaches of the Portuguese coast. Mar. Pollut. Bull. 60, 1988–1992. doi: 10.1016/j.marpolbul.2010.07.030
Gago, J., Carretero, O., Filgueiras, A. V., and Viñas, L. (2017). Synthetic microfibers in the marine environment: a review on their occurrence in seawater and sediments. Mar. Pollut. Bull. 127, 365–376. doi: 10.1016/j.marpolbul.2017.11.070
Galy, V., France-Lanord, C., Beyssac, O., Faure, P., Kudrass, H., and Palhol, F. (2007). Efficient organic carbon burial in the Bengal fan sustained by the Himalayan erosional system. Nature 450, 407–410. doi: 10.1038/nature06273
Gewert, B., Ogonowski, M., Barth, A., and MacLeod, M. (2017). Abundance and composition of near surface microplastics and plastic debris in the Stockholm Archipelago, Baltic sea. Mar. Pollut. Bull. 120, 292–302. doi: 10.1016/j.marpolbul.2017.04.062
Gorokhova, E. (2015). Screening for microplastic particles in plankton samples: how to integrate marine litter assessment into existing monitoring programs? Mar. Pollut. Bull. 99, 271–275. doi: 10.1016/j.marpolbul.2015.07.056
Graca, B., Szewc, K., Zakrzewska, D., Dołega, A., and Szczerbowska-Boruchowska, M. (2017). Sources and fate of microplastics in marine and beach sediments of the Southern Baltic sea-a preliminary study. Environ. Sci. Pollut. Res. 24, 7650–7661. doi: 10.1007/s11356-017-8419-5
Gwiazda, R., Paull, C. K., Ussler, W., and Alexander, C. R. (2015). Evidence of modern fine-grained sediment accumulation in the monterey fan from measurements of the pesticide DDT and Its metabolites. Mar. Geol. 363, 125–133. doi: 10.1016/j.margeo.2015.02.006
Hänninen, J., Weckstrm, M., Pawowska, J., Szymańska, N., Uurasjärvi, E., Zajaczkowski, M., et al. (2021). Plastic debris composition and concentration in the Arctic Ocean, the North sea and the Baltic sea. Mar. Pollut. Bull. 165:112150. doi: 10.1016/j.marpolbul.2021.112150
Isobe, A., Kubo, K., Tamura, Y., Nakashima, E., and Fujii, N. (2014). Selective transport of microplastics and mesoplastics by drifting in coastal waters. Mar. Pollut. Bull. 89, 324–330. doi: 10.1016/j.marpolbul.2014.09.041
Jeong, C. B., Kang, H. M., Lee, Y. H., Kim, M. S., Lee, J. S., Seo, J. S., et al. (2018). Nanoplastic ingestion enhances toxicity of persistent organic pollutants (POPs) in the monogonont rotifer Brachionus koreanus via multixenobiotic resistance (MXR) disruption. Environ. Sci. Tech. 52, 11411–11418. doi: 10.1021/acs.est.8b03211
Kaiser, D., Kowalski, N., and Waniek, J. J. (2017). Effects of biofouling on the sinking behavior of microplastics. Environ. Res. Lett. 12:124003. doi: 10.1088/1748-9326/aa8e8b
Kammann, U., Aust, M. O., Bahl, H., and Lang, T. (2018). Marine litter at the seafloor–Abundance and composition in the North sea and the Baltic sea. Mar. Pollut. Bull. 127, 774–780. doi: 10.1016/j.marpolbul.2017.09.051
Kanhai, L. D. K, Gårdfeldt, K., Lyashevska, O., Hassellöv, M., et al. (2018). Microplastics in sub-surface waters of the arctic central basin. Mar. Pollut. Bull. 130, 8–18. doi: 10.1016/j.marpolbul.2018.03.011
Karkanorachaki, K., Syranidou, E., and Kalogerakis, N. (2021). Sinking characteristics of microplastics in the marine environment. Sci. Total Environ. 793:148526. doi: 10.1016/j.scitotenv.2021.148526
Katija, K., Choy, C. A., Sherlock, R. E., Sherman, A. D., and Robison, B. H. (2017). From the surface to the seafloor: how giant larvaceans transport microplastics into the deep sea. Sci. Adv. 3:1700715. doi: 10.1126/sciadv.1700715
Kooi, M., Van Nes, E. H., Scheffer, M., and Koelmans, A. A. (2017). Ups and downs in the ocean: effects of biofouling on vertical transport of microplastics. Environ. Sci. Tech. 51, 7963–7971. doi: 10.1021/acs.est.6b04702
Korpinen, S., Meski, L., Andersen, J. H., and Laamanen, M. (2012). Human pressures and their potential impact on the Baltic sea ecosystem. Ecol. Indic. 15, 105–114. doi: 10.1016/j.ecolind.2011.09.023
Kowalski, N., Reichardt, A. M., and Waniek, J. J. (2016). Sinking rates of microplastics and potential implications of their alteration by physical, biological, and chemical factors. Mar. Pollut. Bull. 109, 310–319. doi: 10.1016/j.marpolbul.2016.05.064
Kukulka, T., Proskurowski, G., Moret-Ferguson, S., Meyer, D. W., and Law, K. L. (2012). The effect of wind mixing on the vertical distribution of buoyant plastic debris. Geophys. Res. Lett. 39:L07601. doi: 10.1029/2012GL051116
Lattin, G. L., Moore, C. J., Zellers, A. F., Moore, S. L., and Weisberg, S. B. (2004). A comparison of neustonic plastic and zooplankton at different depths near the southern California shore. Mar. Pollut. Bull. 49, 291–294. doi: 10.1016/j.marpolbul.2004.01.020
Lemckert, C. J., Antenucci, J. P., Saggio, A., and Imberger, J. (2004). Physical properties of turbulent benthic boundary layers generated by internal waves. J. Hydraul. Eng. 130, 58–69. doi: 10.1061/(ASCE)0733-9429(2004)130:1(58)
Li, Y., Wolanski, E., Dai, Z., Lambrechts, J., Tang, C., and Zhang, H. (2018). Trapping of plastics in semi-enclosed seas: insights from the bohai sea, china. Mar. Pollut. Bull. 137, 509–517. doi: 10.1016/j.marpolbul.2018.10.038
Liblik, T., and Lips, U. (2019). Stratification has strengthened in the Baltic sea-an analysis of 35 years of observational data. Front. Ear. Sci. 7:174. doi: 10.3389/feart.2019.00174
Long, M., Moriceau, B., Gallinari, M., Lambert, C., Huvet, A., Raffray, J., et al. (2015). Interactions between microplastics and phytoplankton aggregates: impact on their respective fates. Mar. Chem. 175, 39–46. doi: 10.1016/j.marchem.2015.04.003
Lusher, A. L., McHugh, M., and Thompson, R. C. (2013). Occurrence of microplastics in the gastrointestinal tract of pelagic and demersal fish from the English channel. Mar. Pollut. Bull. 67, 94–99. doi: 10.1016/j.marpolbul.2012.11.028
Lusher, A. L., Tirelli, V., O'Connor, I., and Officer, R. (2015). Microplastics in Arctic polar waters: the first reported values of particles in surface and sub-surface samples. Sci. Rep. 5:14947. doi: 10.1038/srep14947
Maciejewska, A., and Pempkowiak, J. (2014). DOC and POC in the water column of the southern Baltic. Part I. Evaluation of factors influencing sources, distribution and concentration dynamics of organic matter. Oceanologia 56, 523–548. doi: 10.5697/oc.56-3.523
Martin, J., Lusher, A., Thompson, R. C., and Morley, A. (2017). The deposition and accumulation of microplastics in marine sediments and bottom water from the Irish continental shelf. Sci. Rep. 7:10772. doi: 10.1038/s41598-017-11079-2
Nor, N. H. M., and Obbard, J. P. (2014). Microplastics in Singapore's coastal mangrove ecosystems. Mar. Pollut. Bull. 79, 278–283. doi: 10.1016/j.marpolbul.2013.11.025
Obbard, R. W., Sadri, S., Wong, Y. Q., Khitun, A. A., Baker, I., and Thompson, R. C. (2014). Global warming releases microplastic legacy frozen in Arctic sea ice. Earth's Future 2, 315–320. doi: 10.1002/2014EF000240
Paull, C. K., Talling, P. J., Maier, K. L., Parsons, D., Xu, J., and Caress, D. W. (2018). Powerful turbidity currents driven by densebasal layers. Nat. Commun. 9:4114. doi: 10.1038/s41467-018-06254-6
Paul-Pont, I., Tallec, K., Gonzalez-Fernandez, C., Lambert, C., Vincent, D., Mazurais, D., et al. (2018). Constraints and priorities for conducting experimental exposures of marine organisms to microplastics. Front. Mar. Sci. 5:252. doi: 10.3389/fmars.2018.00252
Peeken, I., Primpke, S., Beyer, B., Gütermann, J., Katlein, C., Krumpen, T., et al. (2018). Arctic sea ice is an important temporal sink and means of transport for microplastic. Nat. Commun. 9:1505. doi: 10.1038/s41467-018-03825-5
Pohl, F., Eggenhuisen, J. T., Kane, I. A., and Clare, M. A. (2020). Transport and burial of microplastics in deep-marine sediments by turbidity currents. Environ. Sci. Tech. 54, 4180–4189. doi: 10.1021/acs.est.9b07527
Rebeca, D., Drótos, G., Hernández-García, E., López, C., and van Sebille, E. (2021). Sinking microplastics in the water column: simulations in the Mediterranean sea. Ocean. Sci. 17, 431–453. doi: 10.5194/os-17-431-2021
Reineccius, J., Appelt, J., Hinrichs, T., Kaiser, D., Stern, J., Prien, R. D., et al. (2020). Abundance and characteristics of microfibers detected in sediment trap material from the deep subtropical North Atlantic Ocean. Sci. Total. Environ. 738:140354. doi: 10.1016/j.scitotenv.2020.140354
Reisser, J., Shaw, J., Hallegraeff, G., Proietti, M., Barnes, D. K., Thums, M., et al. (2014). Millimeter-sized marine plastics: a new pelagic habitat for microorganisms and invertebrates. PLoS ONE 9:100289. doi: 10.1371/journal.pone.0100289
Reisser, J., Slat, B., Noble, K., du Plessis, K., Epp, M., Proietti, M., et al. (2015). The vertical distribution of buoyant plastics at sea: an observational study in the North Atlantic Gyre. Biogeosciences 12, 1249–1256. doi: 10.5194/bg-12-1249-2015
Remy, F., Collard, F., Gilbert, B., Compère, P., Eppe, G., and Lepoint, G. (2015). When microplastic is not plastic: the ingestion of artificial cellulose fibers by macrofauna living in seagrass macrophytodetritus. Environ. Sci. Tech. 49, 11158–11166. doi: 10.1021/acs.est.5b02005
Rochman, C. M. (2018). Microplastics research—from sink to source. Science, 360:28–29. doi: 10.1126/science.aar7734
Roscher, L., Fehres, A., Reisel, L., Halbach, M., and Gerdts, G. (2021). Microplastic pollution in the weser estuary and the german north sea. Environ Pollut. 288:117681. doi: 10.1016/j.envpol.2021.117681
Rotander, A., and Kärrman, A. (2019). Microplastics in Södertälje: From Lake Mälaren to the Baltic sea. Örebro University.
Sanchez-Vidal, A., Thompson, R. C., Canals, M., Haan, W. D., and Chin, W. C. (2018). The imprint of microfibres in Southern European deep seas. PLoS ONE 13:0207033. doi: 10.1371/journal.pone.0207033
Schmidt, C., Kumar, R., Yang, S., and Büttner, O. (2020). Microplastic particle emission from wastewater treatment plant effluents into river networks in Germany: loads, spatial patterns of concentrations and potential toxicity. Sci. Total. Environ. 737:139544. doi: 10.1016/j.scitotenv.2020.139544
Schönlau, C., Karlsson, T. T., Rotander, A., Nilsson, H., Engwall, M., Van Bavel, B., et al. (2020). Microplastics in sea-surface waters surrounding Sweden sampled by manta trawl and in-situ pump. Mar. Pollut. Bull. 153:111019. doi: 10.1016/j.marpolbul.2020.111019
Setälä, O., Magnusson, K., Lehtiniemi, M., and Norén, F. (2016). Distribution and abundance of surface water microlitter in the Baltic sea: a comparison of two sampling methods. Mar. Pollut. Bull. 110, 177–183. doi: 10.1016/j.marpolbul.2016.06.065
Silvestrova, K., and Stepanova, N. (2020). The distribution of microplastics in the surface layer of the atlantic ocean from the subtropics to the equator according to visual analysis. Mar. Pollut. Bull. 162:111836. doi: 10.1016/j.marpolbul.2020.111836
Song, Y. K., Hong, S. H., Eo, S., Jang, M., Han, G. M., Isobe, A., et al. (2018). Horizontal and vertical distribution of microplastics in Korean coastal waters. Environ. Sci. Tech. 52, 12188–12197. doi: 10.1021/acs.est.8b04032
Song, Y. K., Hong, S. H., Jang, M., Han, G. M., and Shim, W. J. (2015). Occurrence and distribution of microplastics in the sea surface microlayer in Jinhae Bay, South Korea. Arch. Environ. Con. Tox. 69, 279–287. doi: 10.1007/s00244-015-0209-9
Stankiewicz, M. (Ed.). (2010). Maritime Activities in the Baltic Sea: An Integrated Thematic Assessment on Maritime Activities and Response to Pollution at Sea in the Baltic Sea Region. Helsinki Commission-Baltic Marine Environment Protection Commission.
Stolte, A., Forster, S., Gerdts, G., and Schubert, H. (2015). Microplastic concentrations in beach sediments along the German Baltic coast. Mar. Pollut. Bull. 99, 216–229. doi: 10.1016/j.marpolbul.2015.07.022
Suaria, G., Achtypi, A., Perold, V., Lee, J. R., Pierucci, A., Bornman, T. G., et al. (2020). Microfibers in oceanic surface waters: a global characterization. Sci. Adv. 6:8493. doi: 10.1126/sciadv.aay8493
Suaria, G., Avio, C. G., Mineo, A., Lattin, G. L., Magaldi, M. G., Belmonte, G., et al. (2016). The Mediterranean plastic soup: synthetic polymers in Mediterranean surface waters. Sci. Rep. 6:37551. doi: 10.1038/srep37551
Talvitie, J., Heinonen, M., Pääkkönen, J. P., Vahtera, E., Mikola, A., Setälä, O., et al. (2015). Do wastewater treatment plants act as a potential point source of microplastics? Preliminary study in the coastal Gulf of Finland, Baltic sea. Water. Sci. Tech. 72, 1495–1504. doi: 10.2166/wst.2015.360
Tamminga, M., Hengstmann, E., and Fischer, E. K. (2018). Microplastic analysis in the South Funen Archipelago, Baltic Sea, implementing manta trawling and bulk sampling. Mar. Pollut. Bull. 128, 601–608. doi: 10.1016/j.marpolbul.2018.01.066
Taylor, M. L., Gwinnett, C., Robinson, L. F., and Woodall, L. C. (2016). Plastic microfibre ingestion by deep-sea organisms. Sci. Rep. 6:33997. doi: 10.1038/srep33997
Thompson, R. C., Olsen, Y. S., Mitchell, R. P., Davis, A., Rowland, S. J., John, A. W., et al. (2004). Lost at sea: where is all the plastic? Science 304, 838–838. doi: 10.1126/science.1094559
Urban-Malinga, B., Wodzinowski, T., Witalis, B., Zalewski, M., Radtke, K., and Grygiel, W. (2018). Marine litter on the seafloor of the southern Baltic. Mar. Pollut. Bull. 127, 612–617. doi: 10.1016/j.marpolbul.2017.12.052
Uurasjärvi, E., Pkknen, M., Setl, O., Koistinen, A., and Lehtiniemi, M. (2021). Microplastics accumulate to thin layers in the stratified Baltic sea. Environ. Pollut. 268:115700. doi: 10.1016/j.envpol.2020.115700
Van Cauwenberghe, L., Vanreusel, A., Mees, J., and Janssen, C. R. (2013). Microplastic pollution in deep-sea sediments. Environ. Pollut. 182, 495–499. doi: 10.1016/j.envpol.2013.08.013
Vianello, A., Boldrin, A., Guerriero, P., Moschino, V., Rella, R., Sturaro, A., et al. (2013). Microplastic particles in sediments of Lagoon of Venice, Italy: first observations on occurrence, spatial patterns and identification. Estuar. Coast. Shelf. S. 130, 54–61. doi: 10.1016/j.ecss.2013.03.022
Virtasalo, J. J., Leipe, T., Moros, M., and Kotilainen, A. T. (2011). Physicochemical and biological influences on sedimentary-fabric formation in a salinity and oxygen-restricted semi-enclosed sea: Gotland Deep, Baltic sea. Sedimentology 58, 352–375. doi: 10.1111/j.1365-3091.2010.01166.x
Wu, N., Zhang, Y., Li, W., Wang, J., Zhang, X., He, J., et al. (2020). Co-effects of biofouling and inorganic matters increased the density of environmental microplastics in the sediments of Bohai Bay coast. Sci. Total. Environ. 717:134431. doi: 10.1016/j.scitotenv.2019.134431
Zalesny, V., Gusev, A., Chernobay, S., Aps, R., Tamsalu, R., Kujala, P., et al. (2014). The Baltic Sea circulation modelling and assessment of marine pollution. Russ. J. Numer. Anal. M. 29, 129–138. doi: 10.1515/rnam-2014-0010
Zhang, D., Cui, Y., Zhou, H., Jin, C., Yu, X., Xu, Y., et al. (2020a). Microplastic pollution in water, sediment, and fish from artificial reefs around the Ma'an Archipelago, Shengsi, China. Sci. Total Environ. 703:134768. doi: 10.1016/j.scitotenv.2019.134768
Zhang, D., Liu, X., Huang, W., Li, J., Wang, C., Zhang, D., et al. (2020b). Microplastic pollution in deep-sea sediments and organisms of the Western Pacific Ocean. Environ. Pollut. 259:113948. doi: 10.1016/j.envpol.2020.113948
Zhang, H. (2017). Transport of microplastics in coastal seas. Estuar. Coast. Shelf. S. 199, 74–86. doi: 10.1016/j.ecss.2017.09.032
Zhang, K., Hamidian, A. H., Tubić, A., Zhang, Y., Fang, J. K., Wu, C., et al. (2021). Understanding plastic degradation and microplastic formation in the environment: a review. Environ. Pollut. 274:116554. doi: 10.1016/j.envpol.2021.116554
Zhang, W., Zhang, S., Wang, J., Wang, Y., Mu, J., Wang, P., et al. (2017). Microplastic pollution in the surface waters of the Bohai sea, China. Environ. Pollut. 231, 541–548. doi: 10.1016/j.envpol.2017.08.058
Zhou, Q., Zhang, H., Fu, C., Zhou, Y., Dai, Z., Li, Y., et al. (2018). The distribution and morphology of microplastics in coastal soils adjacent to the Bohai sea and the Yellow sea. Geoderma 322, 201–208. doi: 10.1016/j.geoderma.2018.02.015
Zhu, J., Zhang, Q., Li, Y., Tan, S., Kang, Z., Yu, X., et al. (2019). Microplastic pollution in the Maowei sea, a typical mariculture bay of China. Sci. Total Environ. 658, 62–68. doi: 10.1016/j.scitotenv.2018.12.192
Zobkov, M., and Esiukova, E. (2017). Microplastics in Baltic bottom sediments: quantification procedures and first results. Mar. Pollut. Bull. 114, 724–732. doi: 10.1016/j.marpolbul.2016.10.060
Keywords: Baltic Sea, halocline, microplastics, turbidity, vertical distribution
Citation: Zhou Q, Tu C, Yang J, Fu C, Li Y and Waniek JJ (2021) Trapping of Microplastics in Halocline and Turbidity Layers of the Semi-enclosed Baltic Sea. Front. Mar. Sci. 8:761566. doi: 10.3389/fmars.2021.761566
Received: 20 August 2021; Accepted: 30 September 2021;
Published: 04 November 2021.
Edited by:
Xuetao Guo, Northwest A&F University, ChinaReviewed by:
Dongdong Zhang, Zhejiang University, ChinaChenxi Wu, Institute of Hydrobiology, Chinese Academy of Sciences (CAS), China
Pin Gao, Donghua University, China
Copyright © 2021 Zhou, Tu, Yang, Fu, Li and Waniek. This is an open-access article distributed under the terms of the Creative Commons Attribution License (CC BY). The use, distribution or reproduction in other forums is permitted, provided the original author(s) and the copyright owner(s) are credited and that the original publication in this journal is cited, in accordance with accepted academic practice. No use, distribution or reproduction is permitted which does not comply with these terms.
*Correspondence: Chen Tu, ctu@yic.ac.cn