- 1Global Change Research Group, IMEDEA Consejo Superior de Investigaciones Científicas (CSIC) – Universitat de les Illes Balears (UIB), Institut Mediterrani d’Estudis Avançats, Esporles, Spain
- 2Centro do Ciencias do Mar (CCMAR), Centro Interdisciplinar de Investigação Marinha e Ambiental (CIMAR), Universidade do Algarve, Faro, Portugal
- 3Área de Biodiversidad y Conservación, Departamento de Biología, Geología, Física y Química Inorgánica, Escuela Superior de Ciencias y Tecnología (ESCET), Universidad Rey Juan Carlos, Móstoles, Spain
- 4Red Sea Research Centre (RSRC) and Computational Bioscience Research Center (CBRC), King Abdullah University of Science and Technology (KAUST), Thuwal, Saudi Arabia
The spread of exotic species to new areas can be magnified when favored by future climatic conditions. Forecasting future ranges using species distribution models (SDMs) could be improved by considering physiological thresholds, because models solely based on occurrence data cannot account for plasticity due to acclimation of individuals to local conditions over their life-time or to adaptation due to selection within local populations. This is particularly relevant for the exotic seagrass Halophila stipulacea, which colonized the Mediterranean Sea a century ago and shifted its thermal niche, coping with a colder regime. Here, we used two hybrid models combining correlative SDMs with the thermal limits for growth of native and exotic H. stipulacea populations to predict the distribution of the species in its native (Indian Ocean and Red Sea) and exotic ranges (Mediterranean Sea and Caribbean Sea) under two scenarios forecasting limited (RCP 2.6) and severe (RCP 8.5) future climate changes by 2050 and 2100. Then, we assessed the differences between hybrid models based on native Red Sea thermal limits (niche conservatism: 17–36°C) and on exotic Mediterranean thermal limits (local adaptation: 14–36°C). At the Mediterranean exotic range, the local adaptation hybrid model accurately agreed with the present distribution of the species while the niche conservatism-based hybrid model failed to predict 87% of the current occurrences of the species. By contrast, both hybrid models predicted similar species distributions for the native range and exotic Caribbean range at present and projected that H. stipulacea will maintain its current worldwide under all future greenhouse gas emission scenarios. The hybrid model based on Mediterranean thermal limits projected the expansion of H. stipulacea through the western Mediterranean basin (except the gulf of Leon) under the most severe scenario (RCP 8.5) by 2100, increasing its distribution by 50% in the Mediterranean. The future expansion of H. stipulacea is related to its capacity to cope with warm waters and it may become a relevant species in the future, particularly under the projected decline of native Mediterranean seagrasses, resulting in important shifts in seagrass communities and overall ecosystem functions.
Introduction
Temperature regimes, a determinant factor controlling the biogeographic distribution of species (Brown et al., 2004; Dell et al., 2011), are expected to continue to increase due to the rise in anthropogenic emissions of greenhouse gases (Intergovernmental Panel on Climate Change [IPCC], 2014), driving a global redistribution of species with economic and ecological consequences (Pecl et al., 2017). Changes in species phenology and distribution of climatic niches have been documented widely, both on land (e.g., Chen et al., 2011; Gottfried et al., 2012) and ocean (e.g., Sunday et al., 2015; Poloczanska et al., 2016; Wernberg et al., 2016), where marine range shifts tend to be greater and faster than terrestrial ones (Parmesan and Yohe, 2003; Sorte et al., 2010), because of high connectivity and continuity of the marine domain (Burrows et al., 2011).
Species distributions are also altered by human activities that facilitate the spread (Mack et al., 2000; Seebens et al., 2017) and impacts (Geraldi et al., 2020) of exotic species beyond their native biogeographical range, which are expected to increase in the future (Sardain et al., 2019). Climate change is likely to aggravate the degradation of ecosystem services and biodiversity by exotic species (Bennett et al., 2021), which are already substantial (Vilà et al., 2011; Maggi et al., 2015; Doherty et al., 2016; Gallardo et al., 2016; Anton et al., 2019). Similar climatic conditions between native and recipient locations are key for successful biological invasion and establishment (Duncan et al., 2003; Thuiller et al., 2005; Bennett et al., 2021) and, therefore, it is expected that warming might accelerate the spread of exotic tropical species into warm temperate areas and/or increase their abundance, exacerbating their impacts (Bates et al., 2013; Poloczanska et al., 2013; Sorte et al., 2013; Bennett et al., 2021). Besides, in marine systems the impact of exotic species on recipient ecosystems is maximized where the median temperature is slightly (2.2°C) cooler than the optimal average temperature at the original range of the species (Bennett et al., 2021).
Projections of exotic species distributions under climate change scenarios are critical to anticipate future retractions or expansions in their distributional ranges to guide management actions (Bates et al., 2013; Guisan et al., 2014). Potential future geographical ranges of exotic organisms have been forecasted with species distribution models (SDMs) (Chefaoui and Varela-Álvarez, 2018; Chefaoui et al., 2019; Wilson et al., 2019; Goldsmit et al., 2020; Poursanidis et al., 2020). Correlative SDMs (Guisan and Thuiller, 2005; Elith and Leathwick, 2009) are based on the species’ realized distribution, assuming that all relevant ecological processes are captured by the correlative analyses (Guisan and Thuiller, 2005; Buckley et al., 2010; Peterson and Soberón, 2012). However, SDMs do not take into account the mechanisms controlling species distributions and therefore have limitations when making projections for conditions not recorded before, such as new regions or future climates (Elith and Leathwick, 2009; Buckley et al., 2010), which are particularly relevant for the case of exotic species. As an alternative, the physiological tolerance of species has been used to integrate the species’ fundamental niche into correlative SDMs to deliver more robust projections (Kearney and Porter, 2009; Buckley et al., 2011). Thus, hybrid of correlative and mechanistic models are increasingly being used to improve marine species distribution projections by incorporating thermal thresholds for survival (e.g., Martínez et al., 2015), growth (Gamliel et al., 2020) or the reproductive phenology of invasive species (Chefaoui et al., 2019).
As temperatures are expected to continue to rise, local populations can also adapt to the changing climate (Matesanz et al., 2010; Nicotra et al., 2010; Hoffmann and Sgrò, 2011; Schlüter et al., 2014; Padfield et al., 2016). Furthermore, individuals may have sufficient plasticity to adjust during their life-time to withstand different conditions (acclimation). Therefore, future ranges may depend not only on the original species thermal niche (Dell et al., 2011), but also on the possibility of niche shifts by individual adjustments (local acclimation; Peck et al., 2014; Rohr et al., 2018) or, longer-term, adaptive evolution in populations (adaptation) (Conover et al., 2006; Sanford and Kelly, 2011; King et al., 2018) as opposed to niche conservatism across populations (Peterson et al., 1999; Khaliq et al., 2015). Thus, realized thermal niches (based on occurrence data) might vary due to individual modifications through acclimation and are typically smaller than a species fundamental niche based on physiological tolerances, which might be conserved or vary across populations through adaptation. In the case of biological invasions, there is evidence that rapid adaptation (niche shift) can be an important contributor for successful establishment of exotic species (Lee and Bell, 1999; Prentis et al., 2008; Davidson et al., 2011; Parravicini et al., 2015; Wesselmann et al., 2020b), although niche conservatism has also been documented in exotic species (Chefaoui and Varela-Álvarez, 2018; Liu et al., 2020). Therefore, species distribution models (SDMs) predicting the future expansion of exotic species should integrate not only the fundamental niche (physiological tolerance) into correlative SDMs (realized niche) but also include the variation of the fundamental niche across populations in order to consider the potential for niche shifts following invasions (Guisan et al., 2014; Chefaoui and Varela-Álvarez, 2018).
The small tropical seagrass Halophila stipulacea is native to the Red Sea and Indian Ocean (Forskal, 1775; Den Hartog, 1970) and entered the Mediterranean Sea through the Suez Canal over 100 years ago (Fritsch, 1895; Forti, 1927). Currently, H. stipulacea has spread across the eastern (Lipkin, 1975a) and central Mediterranean Sea basin (Sghaier et al., 2011; Gambi et al., 2018). In 2002, H. stipulacea was introduced in the eastern Caribbean Islands probably by anchors of recreational boats (Ruiz and Ballantine, 2004) and recently it reached the northern coast of South America (Vera et al., 2014). This tropical seagrass is able to expand through regions with cooler thermal regimes than those in the native range, which might confer an advantage to invade new environments: in the Mediterranean, H. stipulacea is exposed to much cooler temperatures (minimum SSTs; 14.96°C) than in the Red Sea (minimum SST: 21.82°C) (Wesselmann et al., 2020b). As a result, the fundamental thermal niche of H. stipulacea is not conserved within the species geographical range, with significantly lower thermal limits for growth and for metabolism (experimentally determined) for exotic Mediterranean populations than for a native Red Sea population (Wesselmann et al., 2020b). Plants from native and exotic populations were exposed to 12 temperature treatments from 8 to 40°C and specimens from exotic populations started to show rhizome growth at 14°C while specimens from a native population at 17°C (Wesselmann et al., 2020a). This change in the thermal limits occurred in response to the lower Mediterranean SST, as Red Sea populations are not under selective pressure to adapt to lower temperatures, suggesting a rapid adaptation to Mediterranean waters (Wesselmann et al., 2020b). This adaptation to cooler thermal regimes and the trajectories of increase of annual minimum and maximum sea surface temperature (SST) projected for the end of this century (Intergovernmental Panel on Climate Change [IPCC], 2014) suggest that H. stipulacea may be able to spread further and improve its performance in the future due to its high optimum temperature and upper thermal threshold (Wesselmann et al., 2020b).
Here we project the future global distribution of H. stipulacea under projected climatic scenarios using two hybrid models by integrating the experimentally determined thermal limits (fundamental niche), and their variation across native and exotic populations, into the realized species distributions (correlative SDM). Hybrid model 1 was constructed by filtering the correlative SDMs with the physiological thermal limits at the native range (17–36°C) and Hybrid model 2 by filtering the correlative SDMs with the physiological thermal limits at the exotic range (14–36°C). These hybrid models, considering niche conservatism (hybrid model 1) or local adaptation (hybrid model 2), were compared to assess differences in H. stipulacea distribution and corroborate or reject the thermal niche shift postulated by Wesselmann et al. (2020b). We hypothesize that the inclusion of the fundamental niche (thermal limits) considering both native and exotic physiological tolerances into the realized species’ distributions (correlative SDM) may provide a more robust projection of the spread of this marine exotic species compared to models based on native thermal niches alone.
Materials and Methods
Occurrence records of H. stipulacea from its native (Indian Ocean, Red Sea and Arabian Gulf) and exotic range (Mediterranean Sea and the Caribbean islands and northern coast of South America) were extracted from the scientific literature and from online data repositories: the Global Biodiversity Information Facility (GBIF) and the Ocean Biogeographic Information System (OBIF) (Figure 1 and Supplementary Appendix 1). We used the 30 arc-seconds resolution GEBCO’s gridded bathymetric data set1 to delimit the pixels within a 50 m depth, the reported H. stipulacea depth limit (Sharon et al., 2011), as the study coastal area. For georeferencing of H. stipulacea occurrence data and climatic variables, a resolution of 5 arc minutes (∼ 9.2 km) was used, obtaining 553 occurrence records of H. stipulacea entire global distribution, with observations in the exotic range not restricted to harbor, ports and marinas. Only one record per cell was used to reduce spatial autocorrelation (n = 278 cells).
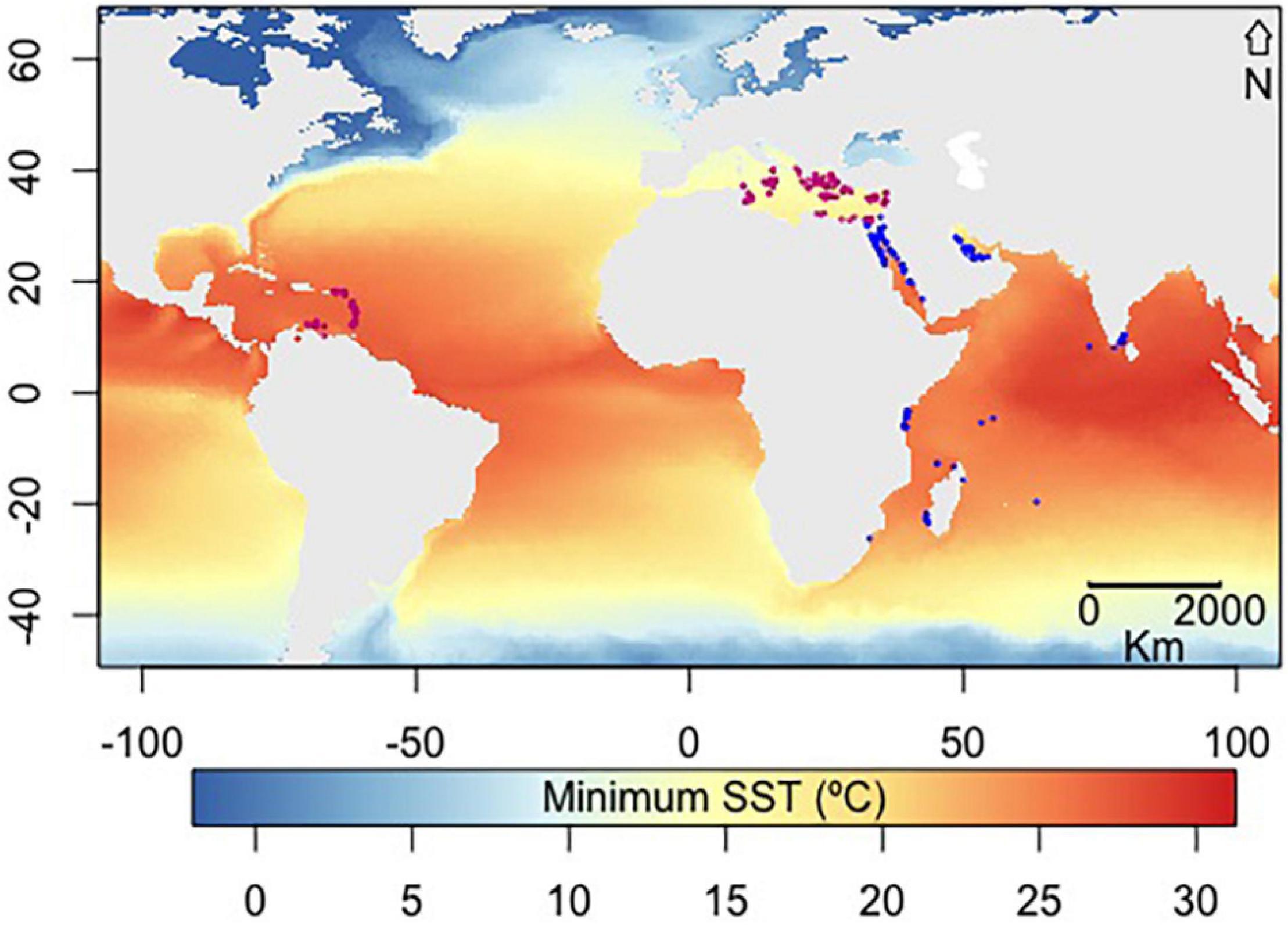
Figure 1. Minimum SST and records of Halophila stipulacea included in the current analysis showing its distribution in its native range in the Indian Ocean and Red Sea (blue points) and exotic Mediterranean and Caribbean ranges (pink points). Minimum SST was extracted from bio-oracle.
From those ocean variables available both for current and future conditions, we selected sea surface temperature as the most relevant for limiting the species geographic distribution, according to previous studies (Hemminga and Duarte, 2000; Wesselmann et al., 2020b). Salinity was not included as H. stipulacea is an euryhaline species (Den Hartog, 1970; Hemminga and Duarte, 2000; Oscar et al., 2018), implying that salinity regimes are not expected to limit its spread. Parameters such as fetch or current velocity were also not included in the model because H. stipulacea’s distribution is not restricted solely to sheltered areas (Supplementary Appendix 1). Maximum and minimum SST were obtained from Bio-ORACLE v2.0 dataset (Tyberghein et al., 2012; Assis et al., 2018) and tested by Pearson’s correlation coefficient < | 0.75| in our study area.
Projected SST values were ensembled from five ocean general circulation models (see Table 1 in Chefaoui et al., 2018) pertaining to the Coupled Model Intercomparison Project Phase 5 (CMIP52). We used two representative concentration pathways (RCP), the RCP 2.6 and the RCP 8.5, providing the lowest and highest greenhouse gas concentration scenarios, respectively (Moss et al., 2008; Meinshausen et al., 2011). The monthly averaged data by 2050 (from 2030 to 2050) and 2100 (from 2080 to 2100) were computed for each scenario.
Current and Future Species Distribution Models
We implemented an ensemble approach to obtain the realized current and projected distribution of the species by 2050 and 2100 under the RCP 2.6 and the RCP 8.5 scenarios. We used the “biomod2” package (Thuiller et al., 2020) to perform six presence-absence algorithms: flexible discriminant analysis (FDA), generalized additive model (GAM), generalized boosting model (GBM), generalized linear model (GLM), multiple adaptive regression splines (MARS), and randomForest (RF). We extracted at random the same number of pseudo-absences as presences for each of the 3 sets of pseudo-absences used. Data were split into a calibration (70%) and a validation (30%) set in each of the 5 iterations performed for each model and set of pseudo-absences. Thus, 90 models were computed (3 pseudo-absence sets × 6 modeling techniques × 5 iterations). We evaluated model performance using the true skill statistic (TSS; Allouche et al., 2006), the area under the receiver operating characteristic (ROC) curve (AUC), and ROC-derived sensitivity (presences correctly projected) and specificity (absences correctly projected) measures (Fielding and Bell, 1997), with the threshold which optimized ROC and TSS scores (Thuiller et al., 2020). To produce the ensemble, we just used the models which obtained TSS > 0.6. The consensus projection under current conditions was an ensemble computed through the average of binary projection (committee averaging ensemble). Ensembles were projected to the RCP 2.6 and 8.5 scenarios to obtain the projection for the future. To assess the uncertainty in future projections related to the different values for each variable between the training range and the novel scenarios we computed a clamping mask.
We estimated the importance of each variable following a procedure similar to “randomForest” (Liaw and Wiener, 2002). The correlation between the full model and a model rearranged without one variable using three iterations was calculated to obtain an importance value from 0 to 1 (highest importance) (Thuiller et al., 2020). Analyses were performed in R (R Core Team, 2020) using a computational cluster facility. We applied the true skill statistic (TSS) related threshold to transform projection into binary maps.
Hybrid Models
Halophila stipulacea is able to survive in regions with SST from 8 to 36°C but the species cannot grow properly and develop viable populations at the lower range of this thermal interval for survival (Wesselmann et al., 2020a). Thus, we used the observed thermal limit of rhizome growth for H. stipulacea reported in the dataset of Wesselmann et al. (2020a) for the Red Sea (SST = 17–36°C) and the Mediterranean Sea (SST = 14–36°C) to produce the hybrid models, where the lower thermal limit for rhizome growth in Mediterranean populations compared to those in the Red Sea provides evidence of adaptation when growing in cooler, exotic ranges.
We applied these thresholds to reclassify the ensembles of minimum and maximum SST pertaining to each RCP scenario by 2050 and 2100 using the “raster” package (Hijmans, 2020) in R. Finally, we filtered the projection obtained by the correlative SDM (realized niche) with the SST related thresholds of growth of native and exotic populations (fundamental niche) to obtain two hybrid models: i) Hybrid model 1 was obtained by filtering the correlative SDM with the thermal limits of growth reported in the native range (SST = 17–36°C), considering niche conservatism and ii) Hybrid model 2 by filtering the correlative SDM with the limits of growth reported for the exotic Mediterranean populations (SST = 14–36°C), allowing, therefore, for local adaptation based on experimental evidence on performance for Mediterranean populations (Wesselmann et al., 2020b).
For each hybrid model, we estimated the areas of agreement (area identified as suitable by the correlative model and the thermal limits, i.e., where the realized and fundamental distribution match), overestimation (area identified as suitable by the correlative model but with SST outside the thermal limits for growth of the species) and underestimation (area not identified as suitable by the correlative model but with SST inside the thermal limits for growth of H. stipulacea) of the correlative SDMs in relation to the thermal limits of growth as a percentage of cells.
Results
The highest validation scores of the models were produced by Random Forest (RF) with TSS (± SD) = 0.567 ± 0.074 and the lowest by Generalized Linear Model (GLM) with TSS = 0.39 ± 0.068 (Supplementary Table 1). The mean evaluation scores for the ensemble were: AUC = 0.957, sensitivity = 87.008, and specificity = 94.712 and TSS = 0.817. Minimum sea surface temperature was the variable with the highest relative importance (mean scores = 0.868) for the ensemble model, compared to the maximum sea surface temperature (0.66). This adds to previous evidence supporting minimum SST a limiting factor of the current distribution of H. stipulacea in the Mediterranean Sea (Wesselmann et al., 2020b).
Current and Future Correlative Species Distribution Models
The ensemble model for the present time approximated the actual distribution of the species, matching a high proportion of the reported species occurrences in the native range (78%) and in the exotic Caribbean (90%) and Mediterranean (82%) ranges (Supplementary Figure 1). Min SST extracted from the thermal occurrence envelopes used in the correlative model was lower for the exotic Mediterranean than for the exotic Caribbean and the native range (Figure 2 and Supplementary Table 2). The model found suitable the Indian Ocean, Red Sea, Arabian Gulf, eastern Caribbean Islands and eastern Mediterranean Sea, as well as most of the western Mediterranean, coast of Mexico, Florida, South Carolina and the western Caribbean Islands (Supplementary Figure 1), where the species has not been reported so far (Figure 1). The correlative model projected future contractions of H. stipulacea suitable area in the north of the Red Sea, Arabian Gulf, Caribbean Islands and eastern Mediterranean by 2050 (RCP 2.6 and 8.5) and 2100 (RCP 2.6) and the complete disappearance from the eastern Mediterranean under the most severe scenario by 2100 (under RCP 8.5; Supplementary Figure 1). Habitat increases were projected for the south of the Red Sea, Seychelles, western Mediterranean and Atlantic coast of Morocco by 2050 and 2100 (RCP 2.6). The ensemble also identified the western coast of Africa as presently suitable for H. stipulacea (Supplementary Figure 1). Hence, the currently realized niche is much smaller than the potential suitable niche.
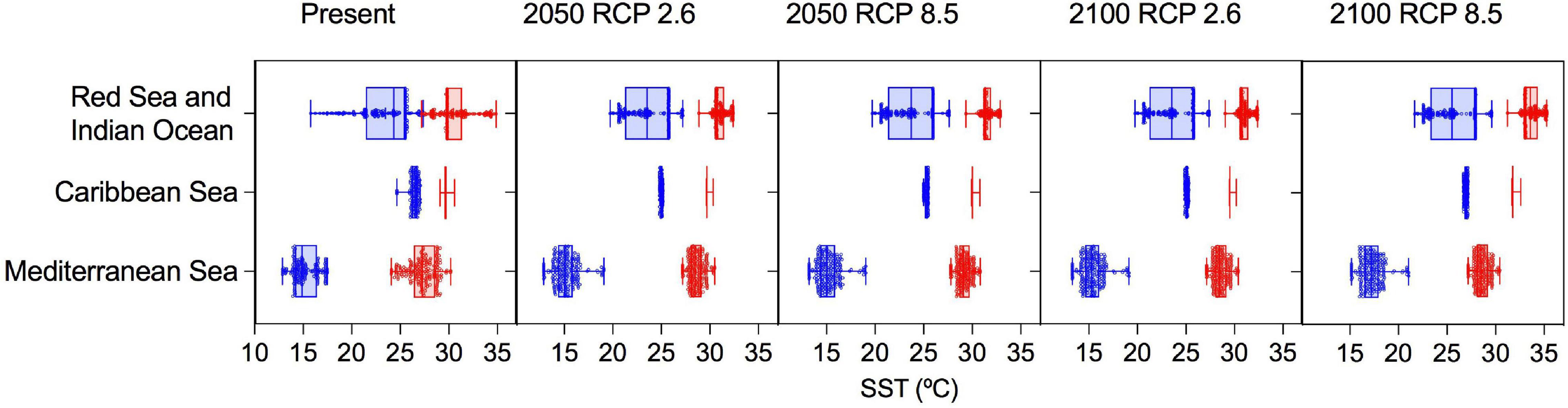
Figure 2. Box plots showing the minimum (blue) and maximum (red) SST corresponding to the occurrences of Halophila stipulacea used in the species distribution models within its native (Indian ocean and Red Sea) and invaded ranges (Mediterranean and Caribbean) under present conditions and 4 different climate change scenarios.
The results suggest loss from the Red Sea, which would become too warm to support the species. However, clamping masks revealed uncertainty in some areas of the Red Sea due to the different values of one variable between the training range and the worst greenhouse gas emission scenario (RCP 8.5 by 2100; see Supplementary Figure 2). Thus, future projections for this region and scenario should be taken with caution.
Spatial Distribution of Thermal Limits for Growth
The lower thermal limits would restrict H. stipulacea distribution, whereas upper thermal thresholds would not constrain the predicted distribution of the species in the future, as all coastal cells would present maximum SST below 36°C, even under the most severe scenario in 2100. The lower thermal limits for growth of H. stipulacea in its native range implied, under present conditions, suitable temperatures for growth in the western Pacific from 15°N to 23°S, in the western Atlantic from 35°N to 28°S and in the eastern Atlantic through the coast of Africa (Supplementary Figure 1). The lower thermal limits of H. stipulacea obtained from Mediterranean populations also identified as suitable the northern coast of Argentina and North Carolina (Supplementary Figure 1). This suitable area for growth would increase in the western Atlantic by 2100, from North Carolina to Virginia (United States) under RCP 2.6 and to Pennsylvania (United States) under RCP 8.5 by 2100 (Supplementary Figure 1).
In the Mediterranean Sea, according to the thermal limits for growth of the species in its native range (17–36°C; Wesselmann et al., 2020a), under present condition H. stipulacea would find suitable conditions for growth solely in the Mediterranean coast of Egypt, Israel and Lebanon, in 2050 RCP 2.6 the coast of Libya and in 2100 RCP 8.5 the coast of Tunisia, Syria, Turkey and the south of Greece. In contrast, the thermal limits for growth obtained for the Mediterranean region (14–36°C; Wesselmann et al., 2020a) expand H. stipulacea suitable habitat to include the entire eastern Mediterranean and Sicily (similar to the actual distribution of the species) and the northern coast of Africa in the western Mediterranean Sea. Under a scenario of no reduction in greenhouse gas emissions (RCP 8.5), H. stipulacea is projected to have suitable temperatures for spreading into other areas in the western Mediterranean Sea by 2100 (Supplementary Figure 1).
Hybrid Model
Overall, the output from our hybrid models revealed that the correlative model underestimated H. stipulacea potential areal extent in many regions, as maximum seawater temperatures will not exceed the species thermal limits (36°C) in any future scenario. Therefore, H. stipulacea would not lose habitat and current seagrass meadows would persist in the future, maintaining 99% of the cells occupied at present (Figure 3). The hybrid model based on native Red Sea thermal limits and on exotic Mediterranean thermal limits (Hybrid model 1 and 2, respectively, hereafter) provided identical results for the Indian Ocean, relatively similar distributions for the Caribbean Sea and projections diverged for the Mediterranean Sea (Figure 3). The area of agreement and underestimation of Hybrid model 1 and 2 in the present distribution identified most of the reported species occurrences within the native (87%) and Caribbean exotic ranges (97%), exceeding the outcome of the correlative SDMs by 10% (Figures 1, 4). In contrast, in the Mediterranean Sea, the hybrid model 1 (agreement and underestimation area) recognized solely 13% of the reported occurrences of H. stipulacea, while the hybrid model 2 identified 90% of the occurrences (Figures 1, 5).
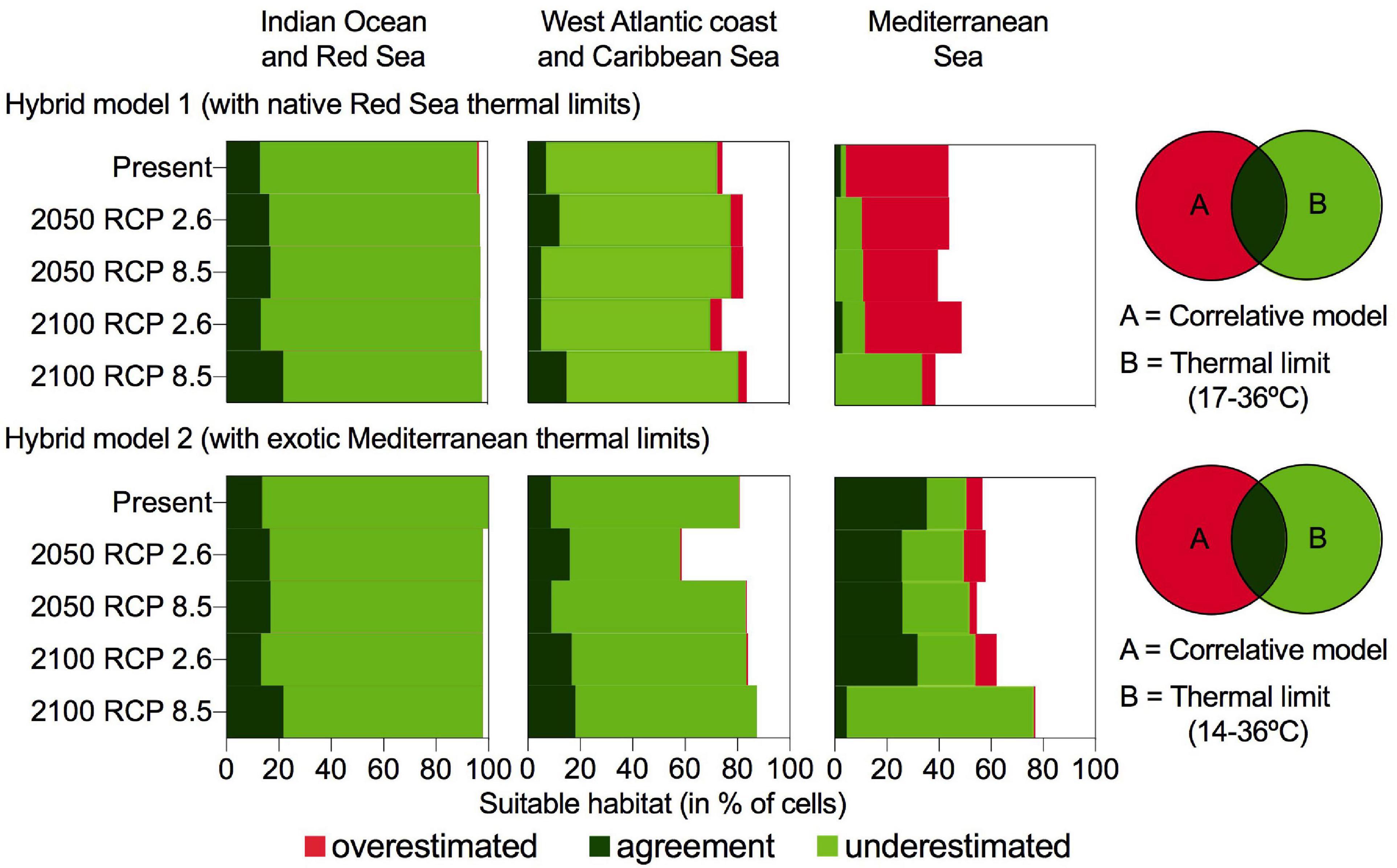
Figure 3. Cumulative barplots with the suitable habitat (in% of cells) for H. stipulacea according to hybrid model 1 (based on native Red Sea thermal limits; 17–36°C) and the hybrid model 2 (based on exotic Mediterranean thermal limits; 14–36°C) under present conditions and 4 different climate change scenarios. The hybrid models were obtained by combining the correlative SDMs (realized niche; A) and the species physiological growth limits (fundamental niche; B). Dark green bars represent the agreement region, light green bars the underestimated region and red bars the overestimated region of the correlative model in relation to the thermal limits of growth.
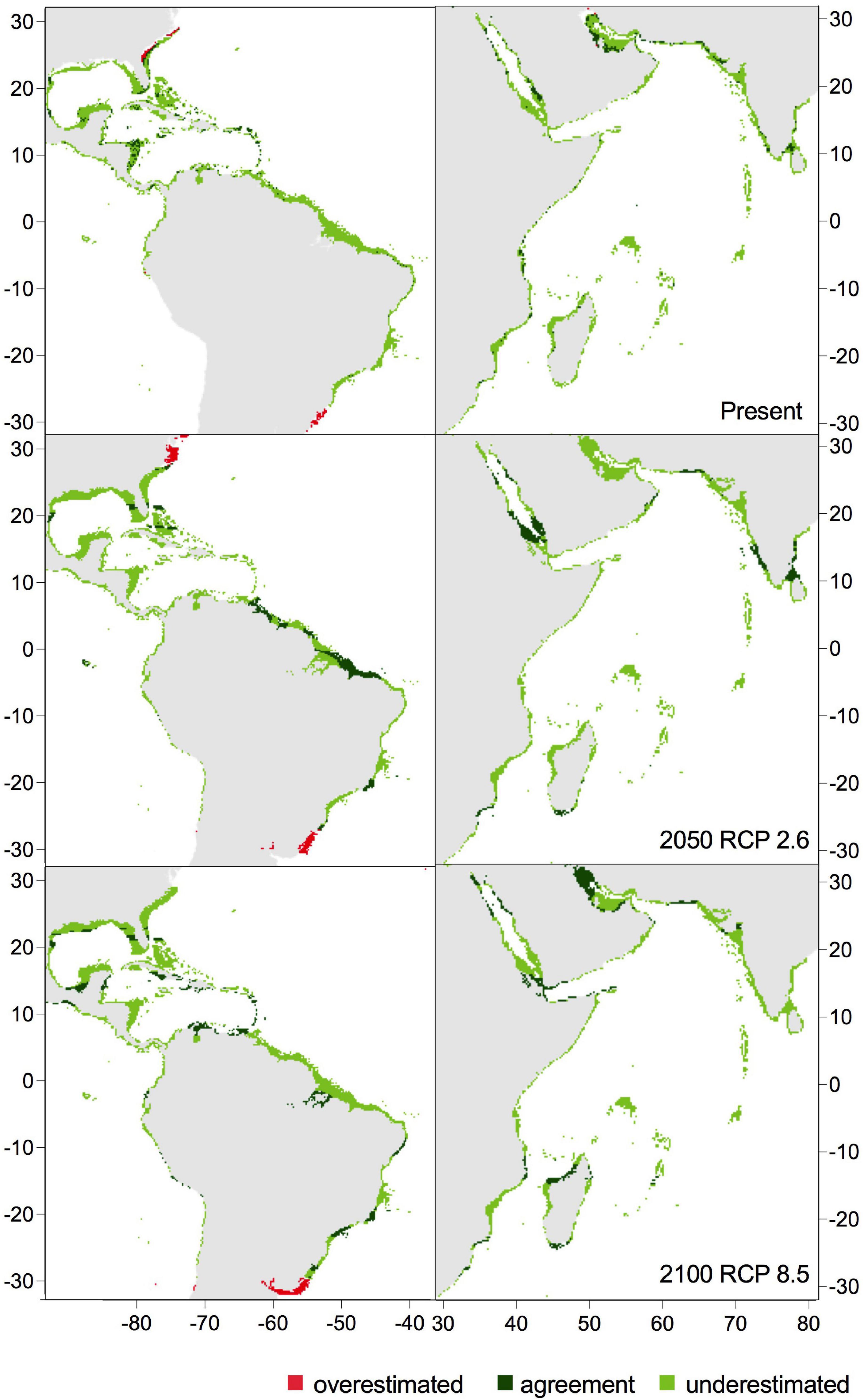
Figure 4. Predicted occurrence of Halophila stipulacea according to the hybrid model 1 (based on native Red Sea thermal limits considering niche conservatism; 17–36°C) under present conditions and 2 different climate change scenarios in the native range in the Indian Ocean and Red Sea (on the right) and in the exotic range in the Caribbean Sea (on the left).
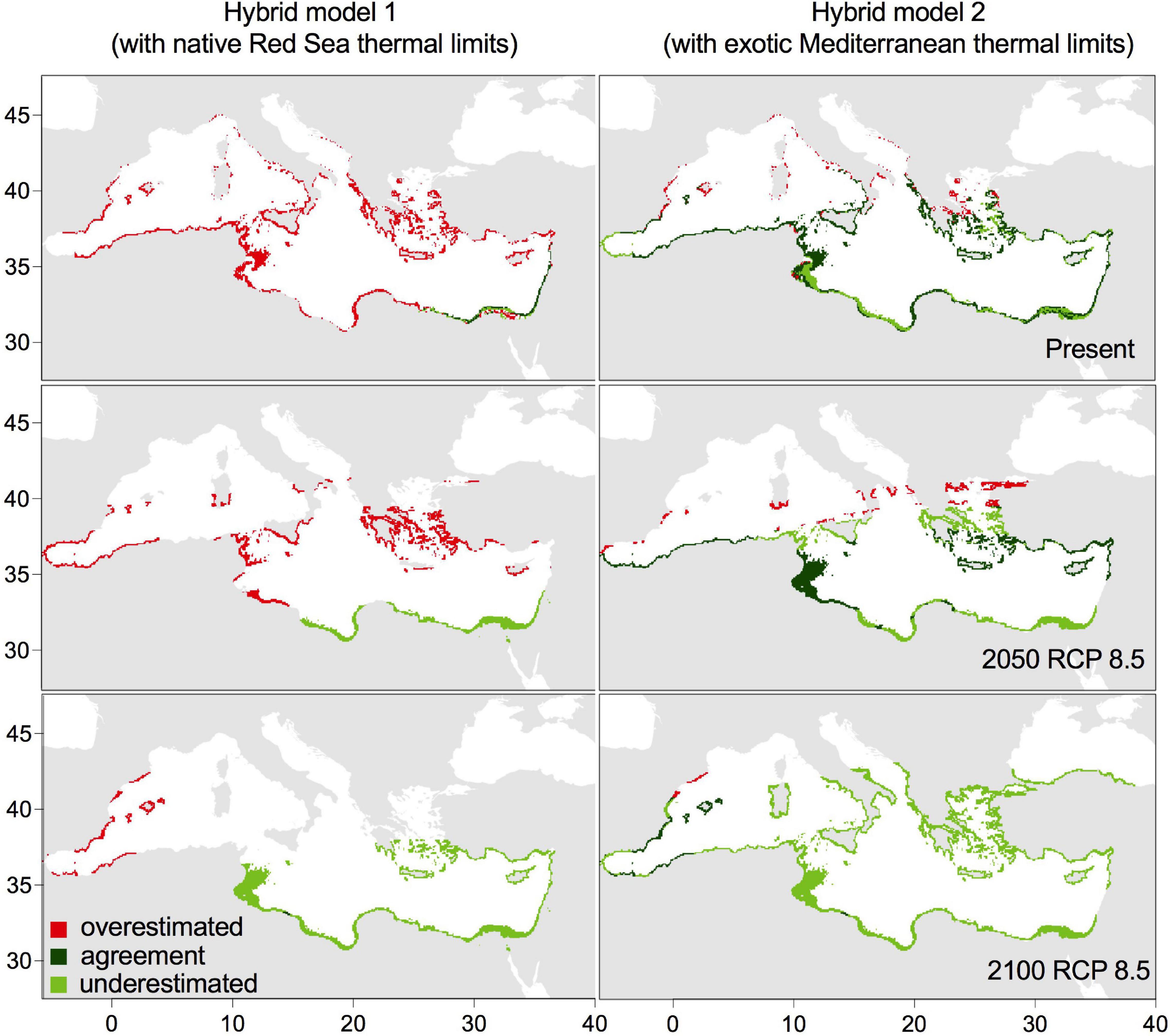
Figure 5. Predicted occurrence of Halophila stipulacea according to the hybrid model 1 (based on native Red Sea thermal limits; 17–36°C) and the hybrid model 2 (based on exotic Mediterranean thermal limits; 14–36°C) under present conditions and 2 different climate change scenarios in the Mediterranean Sea.
In the native range, both hybrid models projected an increase in the area of agreement from the present (13%) to 2050 (16–17%) and to 2100 under RCP 8.5 (22%) and identified an extensive area underestimated by the correlative model, accounting for 76–83% of the cells along the coast of the native range at present and future scenarios (Figure 3). Considering the area of agreement and underestimation, in all future warming scenarios, H. stipulacea could gain 1–2% of its habitat in the Arabian Gulf (Figures 3, 4 and Supplementary Figure 3).
In the Caribbean, the area of agreement between the physiological thermal limits and the correlative models in the hybrid model 1 increased from 7% in the present to 15% by 2100 under RCP 8.5 scenario, while the agreement area in the hybrid model 2 was wider (from 9 to 18%) because it included the coast of Argentina and the coast of North Carolina as suitable to support H. stipulacea’s growth (Figures 3, 4 and Supplementary Figure 3). The correlative model underestimated a huge potentially suitable area, corresponding to 65–74% of all total cells of the western Atlantic coast at the present and under future RCP scenarios, according to hybrid model 1 and 2 (Figures 3, 5 and Supplementary Figure 3). Taking into account the agreement and underestimated area, H. stipulacea could spread southwards and westwards, reaching the Dominican Republic, Cuba, the Gulf of Mexico, Venezuela and Brazil, gaining 7% of its habitat in 2050 and 11% in 2100 under the most severe scenario (RCP 8.5) according to hybrid model 1, whereas according to hybrid model 2, it could increase from 3 to 8% (Figures 3, 4 and Supplementary Figure 3).
In the Mediterranean Sea, the hybrid model 1 showed a reduced suitable area for H. stipulacea in comparison with the hybrid model 2. The area of agreement in Hybrid model 1 was negligible at present and under all future warming scenarios (0–3% of all the cells) (Figures 3, 5) and for most of the western Mediterranean Sea it was overestimated by the correlative model and identified as unsuitable for H. stipulacea’s growth, as minimum temperatures are below 17°C (Figures 3, 5). Even adding the area of agreement and underestimation, the suitable habitat would still be restricted to 4% of all the cells of the Mediterranean at present, 10–12% by 2050 and 2100 (RCP 2.6), and 33% by 2100 (RCP 8.5, Figure 3, 5). Conversely, the agreement area of hybrid model 2 at present, 2050 and 2100 (RCP 2.6) occupied most of the eastern Mediterranean basin and the south-western basin along the coast of Africa (25–35% of all the Mediterranean coastal cells) and disagreed with the correlative model prediction of the presence of the species at the southeastern Iberian Peninsula, southern Adriatic and northern Aegean Sea, as temperature at these regions would remain unsuitable for H. stipulacea growth (< 14°C). By 2100 under RCP 8.5, H. stipulacea could spread into the western Mediterranean Sea, extending from Valencia to Andalusia and the Balearic Islands, and into the eastern Atlantic Ocean through the Gibraltar strait, although the area of agreement of hybrid model 2 would decrease to 5% of all the cells in the Mediterranean Sea (Figures 3, 5). This reduction is due to an underestimation of the correlative model, as 15% at present, 22–24% in 2050 (RCP 2.6 and 8.5) and 2100 (RCP 2.6) and up to 72% by 2100 (RCP8.5) of all the cells in the Mediterranean Sea, mostly concentrated in the eastern basin, would be suitable for H. stipulacea according to the thermal limits of growth of the species (14–36°C, Figure 5). Therefore, adding together the area of agreement and underestimation of hybrid model 2, H. stipulacea could actually occupy 50% of all the cells of the Mediterranean coast at present and increase its habitat by 51% by 2100 under RCP 8.5, occupying 76% of all the cells of the Mediterranean spreading through the western basin (Figures 3, 5).
Discussion
In this study, we show that the global future spread of Halophila stipulacea in contrasting climate change scenarios is better predicted when accounting for local adaptation as compared to niche conservatism. This novel outcome was possible by combining correlative SDM with experimentally resolved estimates of the thermal limits for growth of Halophila stipulacea, obtained for the exotic Mediterranean range and for the native range in the Red Sea. The hybrid models derived from correlative models and thermal niches of populations at the native range (hybrid model 1: niche conservatism) and the Mediterranean range (local adaptation) were similar for the native range (Indian Ocean and Red Sea) and exotic Caribbean range, whereas in the Mediterranean Sea the hybrid model based on the thermal limits of the native range failed to predict most of the current occurrences of the species, which were however, correctly captured by the model based on the thermal limits of the exotic populations in the Mediterranean range that incorporated local adaptation. This study demonstrates the importance of including knowledge on the physiological thermal limits of the species and accounting for their variation across populations, and adaptation and acclimation capacity when predicting future distributions, particularly for biological invasions at regions where thermal conditions extend beyond the limits of the native range.
Hybrid models projected that H. stipulacea will maintain all its current habitat in the future and increase it slightly in the exotic Caribbean (7% by 2050 and 11% by 2100) and up to 50% in the Mediterranean ranges, expanding through the western Mediterranean basin (except the Gulf of Lion) under the most severe scenario (RCP 8.5) by 2100, as previously hypothesized for the species (Wesselmann et al., 2020b). Similar westward longitudinal expansions in response to climate-driven temperature increases have been projected for other Lessepsian migrants, such as fish (Pais et al., 2007; Azzurro et al., 2013, 2017; Coro et al., 2018; Amen and Azzurro, 2020), foraminifera (Langer et al., 2012) and bivalves (Sarà et al., 2018).
The current distribution of H. stipulacea in the Mediterranean Sea is related to the capacity of the Mediterranean populations to grow at 14°C (lower than the native populations; Wesselmann et al., 2020b), allowing its westward expansion. However, the low winter temperatures still prevent occurrence of H. stipulacea in most of the western Mediterranean basin at present. Local adaptation has been described as a mechanism likely employed by exotic species that establish successfully in new environments (Prentis et al., 2008). However, this process has been ignored by niche prediction models, largely because of a gap of experimental data on local adaptation of the thermal niche of exotic species (Valladares et al., 2014).
Our results also show that the expansion of H. stipulacea beyond its native range is not only limited by its capacity to tolerate certain temperatures but is also dispersal limited. Our distribution models of H. stipulacea indicate the suitability of multiple regions beyond the range of current occurrences (Figures 1, 3), such as the coast of North Carolina, the Caribbean coast of Honduras and Nicaragua and the Algerian coast in the western Mediterranean. This suggests that the occupation of potential areas with suitable temperatures is limited by long distance dispersal, which could occur through rafting of rhizome fragments (Steiner and Willette, 2015; Smulders et al., 2017; Willette et al., 2020) or seeds (Chiquillo et al., 2018; Nguyen et al., 2018), despite possible constraints within short scales by locally acting factors (e.g., seawater turbidity and exposure). Long-range seed transport seems unlikely as the genera Halophila produces dormant seeds, neutral or negatively buoyant, and are therefore transported largely through bedload transport over short distances (up to 100 of meters; Kendrick et al., 2012), although longer ranges may be reached during storms or if ingested by highly mobile grazers (Heck et al., 2008; Tol et al., 2017). Successful long-term dispersal through rhizome fragments appears viable, as small (2 cm) fragments are able to grow floating in the seawater in the laboratory (Willette et al., 2020) and a field experiment demonstrated settlement of H. stipulacea fragments within 10 days of detachment (Smulders et al., 2017). Fragments could reach considerable distances over 10 days, either drifting or inadvertently transported by vessels or other vectors. Even a single fragment could create a new clonal population and disperse even further through a stepping-stone model, as hypothesized for other seagrasses (Arnaud-Haond et al., 2012). The prevailing Caribbean Current could facilitate the colonization of the western Caribbean, which is in agreement with a prediction made by Ruiz et al. (2017). However, the further colonization of the coast of Venezuela and the coast of Brazil through currents is less likely due the westward direction of the Caribbean Current (Richardson, 2005). Similarly, considering the water circulation in the Mediterranean Sea, H. stipulacea could colonize first the entire western Italian coast and Corsica than the Algerian coast because the oceanographic current flows counterclockwise in the western Mediterranean (Millot and Taupier-Letage, 2005). Despite this, human mediated transport would be required to fill the potential niche of H. stipulacea populations, as shown by its arrival to Martinique and the Lesser Antilles through recreational boats (Ruiz and Ballantine, 2004; Willette and Ambrose, 2009) or to other H. stipulacea populations in the Mediterranean dispersed either as fragments in fishing gear or attached to the hull of ships or boat anchors (Lipkin, 1975b; Gambi et al., 2018).
Although the current presence of H. stipulacea in the Mediterranean coast is limited by the lower sea temperatures (Wesselmann et al., 2020b), the present study supports the hypothesis that H. stipulacea will continue to expand into the western basin propelled by increasing SST (Wesselmann et al., 2020b). However, our results differ from previous studies predicting a future expansion of H. stipulacea along the northwestern Mediterranean coast already by 2050 (Beca-Carretero et al., 2020) or by 2100 (Gamliel et al., 2020). The capacity of H. stipulacea to both cope with very warm waters and to grow at temperatures several degrees below the minimum temperatures in its native range are key to successfully continue to spread. Exotic marine species often have a greater thermotolerance than related native species (Sorte et al., 2010; Zerebecki and Sorte, 2011; Magozzi and Calosi, 2015), and can profit from warming by expanding in their recipient range. Indeed, the upper thermal tolerance of H. stipulacea is higher than native Mediterranean seagrasses, especially for Posidonia oceanica (Savva et al., 2018). Future projections indicate temperature increases that would exceed the physiological upper thermal tolerance limit of P. oceanica and lead to a decline of its habitat (Jordà et al., 2012; Marbà et al., 2015). Recently, it has been projected that, under the most severe climate change scenario, C. nodosa could lose 46% of its habitat and P. oceanica’s habitat could be completely lost by 2100 (Chefaoui et al., 2018). If these projections are met and the native plants do not adapt, the Mediterranean will lose key foundation species, which may be replaced by H. stipulacea. The future expansion of H. stipulacea and the potential shift in the dominant flora in the Mediterranean (from P. oceanica to H. stipulacea and the native warm tolerant species C. nodosa) may have profound effects on seagrass ecosystem functioning. The ecological roles of P. oceanica and H. sipulacea are different as they have different evolutionary histories and H. stipulacea tends to colonize muddy estuarine waters and unstable environments (Wesselmann et al., 2021). The limited information available on the provision of ecosystem functions of H. stipulacea in the Mediterranean Sea indicates a similar carbon storage capacity of H. stipulacea and native seagrasses in the eastern Mediterranean (Wesselmann et al., 2021), although carbon stocks in that region ranks among the lowest across seagrass meadows (Fourqurean et al., 2012; Duarte et al., 2013; Mazarrasa et al., 2017). Besides, the dense and tall canopy of P. oceanica attenuates the waves and its meadows play a key role on coastal protection (Sánchez-González et al., 2011), whereas the provision of this ecosystem service by H. stipulacea, with a canopy few centimeters high (Den Hartog, 1970), is low (Christianen et al., 2013). Moreover, the three-dimensional structure of P. oceanica canopy provides food and more shelter for diverse faunal assemblages (Francour, 1997; Boudouresque et al., 2006) than the short one of H. stipulacea. Faunal assemblages in H. stipulacea meadows are mainly characterized by species from the phyla Mollusca, Amphipoda, Decapoda, Polychaeta, Crustacea (Cancemi et al., 1994; Acunto et al., 1997) and a fish community dominated by sparids, labrids, and gobiids in which planktivorous species (common in other Mediterranean seagrasses) are absent (Di Martino et al., 2007). Di Genio et al. (2021) found higher species richness of benthic fishes in H. stipulacea meadows than in the nearby dead matte of P. oceanica, however, it remains unclear if the fish abundance and species richness is similar in H. stipulacea and P. oceanica meadows. Finally, H. stipulacea may also drive populations shifts in species that utilize P. oceanica as a component of their diet (Tomas et al., 2005), although it has already been documented that the herbivorous fish Sarpa salpa, typically feeding on P. oceanica, grazes year-round on H. stipulacea leaves (Di Genio et al., 2021) and signs of grazing were recorded in more than half of all H. stipulacea leaves (Gambi et al., 2018).
On the contrary, in the exotic range in the Atlantic Ocean, H. stipulacea does not show higher thermal tolerance than native seagrasses. While in the Mediterranean H. stipulacea coexists with temperate seagrasses (although C. nodosa has a warm affinity; Savva et al., 2018), in the native range and in the Caribbean Sea it coexists with native, warm tolerant species (Den Hartog, 1970; Willette and Ambrose, 2012). As tropical seagrasses have similar thermal tolerances (McMillan, 1984; Koch et al., 2007), sustained elevated temperatures in the Caribbean Sea are unlikely to promote the replacement of the native seagrasses by H. stipulacea. In the Caribbean Sea, H. stipulacea mostly colonized bare “sand halos,” where it forms monospecific meadows, although it has also been observed in mixed seagrass beds with Thalassia testudinum, Syringodium filiforme, Halodule wrightii, and H. decipiens (Willette et al., 2014). Therefore, in this region the expansion of H. stipulacea might be driven by its fast growth rates (Wesselmann et al., 2020b), low light requirements (Sharon et al., 2011), capacity to resist siltation (Terrados et al., 1998) and fast recovery after perturbations (e.g., turtle grazing, storms, anchoring, or bioturbation: Steiner and Willette, 2015; Smulders et al., 2017; Christianen et al., 2019).
In general, the distribution of H. stipulacea based on thermal limits delimited a wider potential range for the species than the realized native and exotic niche. Our hybrid models, combining correlative parametrization with experimentally derived evidence of thermal performance in native and exotic ranges, clearly demonstrate that overlapping thermal limits with SDM is useful to provide an improved future projection on responses of exotic species to climate warming, as it is able to address regions where statistical approaches mismatch physiological information. Thermal limits have been combined before with SDM to improve projections (e.g., Woodin et al., 2013; Martínez et al., 2015; Talluto et al., 2016; Gamliel et al., 2020). However, the thermal tolerance of species over a wide thermal range and its potential variation due to local adaptation are usually ignored in these models (Valladares et al., 2014). Gamliel et al. (2020) combined SDMs with data on H. stipulacea‘s physiological thermal limits, obtained after exposing plants to a narrower temperature range (from 10 to 30°C; Georgiou et al., 2016) than those used here (from 8 to 40°C; Wesselmann et al., 2020a), to project H. stipulacea expansion in the northern Mediterranean coast. Our study takes the additional step of combining SDM with knowledge on thermal limits, considering niche conservatism and local adaptation, to project future trajectories of marine invasions. However, the thermal limits for rhizome growth used in the hybrid model do not consider the phenology of the species and that local populations could potentially adjust their rhizome growth to match the optimal conditions for growth, avoiding the lower thermal limit. Moreover, the integration of other relevant parameters, such as reproductive phenology (Chefaoui et al., 2019), oceanographic currents (Assis et al., 2015), or biotic interactions (Record et al., 2018) would certainly improve projections.
Conclusion
In conclusion, including the variation of thermal limits across native and exotic populations in SDMs improves the prediction of realized, and hence likely projected, distributions of exotic species. Our models support two important insights, (1) warming threatens the expansion of H. stipulacea habitat suitability through the western Mediterranean under the most severe scenario (RCP 8.5) by 2100, increasing its distribution by 50% in the Mediterranean; (2) the potential range of H. stipulacea is vastly larger than that presently occupied, so risks of contemporary expansions in subtropical and tropical waters are high. In the future, H. stipulacea may replace, but not displace, dominant temperate seagrasses in the Mediterranean Sea, as the thermal limits of the native species are exceeded with future climate warming, resulting in important changes in the ecosystem functioning of Mediterranean seagrass meadows (Chefaoui et al., 2018).
Data Availability Statement
The original contributions presented in the study are included in the article/Supplementary Material, further inquiries can be directed to the corresponding author/s.
Author Contributions
CD, NM, ES, and MW conceived the study design. RC developed the models. MW produced the figures of the models and analyzed the data. CD, NM, ES, RC, and MW interpreted the results. MW wrote the initial draft and all coauthors contributed to editing the manuscript and gave final approval for publication.
Funding
This study was funded by the Spanish Ministry of Science, Innovation and Universities (SuMaEco RTI2018-095441-B-C21), King Abdullah University for Science and Technology (3834 KAUST-CSIC Research Collaboration), the Foundation for Science and Technology (UIDB/04326/2020), and the Operational Programmes CRESC Algarve 2020 and COMPETE 2020 (EMBRC.PT ALG-01-0145-FEDER-022121 and BIODATA.PT ALG-01-0145-FEDER-022231). MW was supported by a Ph.D. contract (BES-2016-078241) of the Spanish Ministry of Science, Innovation and Universities. RC obtained additional support from the European Union’s Horizon.
Conflict of Interest
The authors declare that the research was conducted in the absence of any commercial or financial relationships that could be construed as a potential conflict of interest.
Publisher’s Note
All claims expressed in this article are solely those of the authors and do not necessarily represent those of their affiliated organizations, or those of the publisher, the editors and the reviewers. Any product that may be evaluated in this article, or claim that may be made by its manufacturer, is not guaranteed or endorsed by the publisher.
Acknowledgments
We thank Cymon Cox for his help with the CCMAR high-performance computing infrastructure (CETA).
Supplementary Material
The Supplementary Material for this article can be found online at: https://www.frontiersin.org/articles/10.3389/fmars.2021.759676/full#supplementary-material
Footnotes
References
Acunto, S., Maltagliati, F., Rindi, F., Rossi, F., Cinelli, F., and Lardicci, C. (1997). “Indagine su una prateria di Halophila stipulacea (Forssk.) Aschers.(Hydrocharitaceae) dell’Isola di Vulcano,” in Atti 12 Congresso dell’Associazione Italiana di Oceanologia e Limnologia, ed. M. Piccazzo (Genoa: AIOL), 51–60.
Allouche, O., Tsoar, A., and Kadmon, R. (2006). Assessing the accuracy of species distribution models: prevalence, kappa and the true skill statistic (TSS). J. Appl. Ecol. 43, 1223–1232. doi: 10.1111/j.1365-2664.2006.01214.x
Amen, D. M., and Azzurro, E. (2020). Lessepsian fish invasion in Mediterranean marine protected areas: a risk assessment under climate change scenarios. ICES J. Mar. Sci. 77, 388–397.
Anton, A., Geraldi, N. R., Lovelock, C. E., Apostolaki, E. T., Bennett, S., Cebrian, J., et al. (2019). Global ecological impacts of marine exotic species. Nat. Ecol. Evol. 3, 787–800.
Arnaud-Haond, S., Duarte, C. M., Diaz-Almela, E., Marbà, N., Sintes, T., and Serrão, E. A. (2012). Implications of extreme life span in clonal organisms: millenary clones in meadows of the threatened seagrass Posidonia oceanica. PLoS One 7:e30454. doi: 10.1371/journal.pone.0030454
Assis, J., Tyberghein, L., Bosch, S., Verbruggen, H., Serrão, E. A., and De Clerck, O. (2018). Bio-ORACLE v2. 0: extending marine data layers for bioclimatic modelling. Glob. Ecol. Biogeogr. 27, 277–284.
Assis, J., Zupan, M., Nicastro, K. R., Zardi, G. I., McQuaid, C. D., and Serrao, E. A. (2015). Oceanographic conditions limit the spread of a marine invader along southern African shores. PLoS One 10:e0128124. doi: 10.1371/journal.pone.0128124
Azzurro, E., Franzitta, G., Milazzo, M., Bariche, M., and Fanelli, E. (2017). Abundance patterns at the invasion front: the case of Siganus luridus in Linosa (Strait of Sicily, Central Mediterranean Sea). Mar. Freshw. Res. 68, 697–702. doi: 10.1071/mf16024
Azzurro, E., Soto, S., Garofalo, G., and Maynou, F. (2013). Fistularia commersonii in the Mediterranean Sea: invasion history and distribution modeling based on presence-only records. Biol. Invasions 15, 977–990. doi: 10.1007/s10530-012-0344-4
Bates, A. E., McKelvie, C. M., Sorte, C. J., Morley, S. A., Jones, N. A., Mondon, J. A., et al. (2013). Geographical range, heat tolerance and invasion success in aquatic species. Proc. R. Soc. B Biol. Sci. 280:20131958. doi: 10.1098/rspb.2013.1958
Beca-Carretero, P., Teichberg, M., Winters, G., Procaccini, G., and Reuter, H. (2020). Projected Rapid Habitat Expansion of Tropical Seagrass Species in the Mediterranean Sea as Climate Change Progresses. Front. Plant Sci. 11:555376. doi: 10.3389/fpls.2020.555376
Bennett, S., Santana-Garcon, J., Marbà, N., Jordà, G., Anton, A., Apostolaki, E. T., et al. (2021). Climate-driven impacts of exotic species on marine ecosystems. Glob. Ecol. Biogeogr. 30, 1043–1055. doi: 10.1016/j.marpolbul.2014.08.021
Boudouresque, C. F., Bernard, G., Bonhomme, P., Charbonnel, E., Diviacco, G., Meinesz, A., et al. (2006). Préservation et conservation des herbiers à Posidonia oceanica. Monaco: Ramoge.
Brown, J. H., Gillooly, J. F., Allen, A. P., Savage, V. M., and West, G. B. (2004). Toward a metabolic theory of ecology. Ecology 85, 1771–1789. doi: 10.1890/03-9000
Buckley, L. B., Urban, M. C., Angilletta, M. J., Crozier, L. G., Rissler, L. J., and Sears, M. W. (2010). Can mechanism inform species’ distribution models?. Ecol. Lett. 13, 1041–1054.
Buckley, L. B., Waaser, S. A., MacLean, H. J., and Fox, R. (2011). Does including physiology improve species distribution model predictions of responses to recent climate change?. Ecology 92, 2214–2221. doi: 10.1890/11-0066.1
Burrows, M. T., Schoeman, D. S., Buckley, L. B., Moore, P., Poloczanska, E. S., Brander, K. M., et al. (2011). The pace of shifting climate in marine and terrestrial ecosystems. Science 334, 652–655.
Cancemi, G., Terlizzi, A., Scipione, M., and Mazzella, L. (1994). Il prato ad Halophila stipulacea (Forssk.) aschers. di G. Naxos (Sicilia): caratteristiche della planta e del popolamento a fauna vagile. Biol. Mar. Mediter. 1, 401–402.
Chefaoui, R. M., Duarte, C. M., and Serrão, E. A. (2018). Dramatic loss of seagrass habitat under projected climate change in the Mediterranean Sea. Glob. Chang. Biol. 24, 4919–4928. doi: 10.1111/gcb.14401
Chefaoui, R. M., Serebryakova, A., Engelen, A. H., Viard, F., and Serrão, E. A. (2019). Integrating reproductive phenology in ecological niche models changed the predicted future ranges of a marine invader. Divers. Distrib. 25, 688–700. doi: 10.1111/ddi.12910
Chefaoui, R. M., and Varela-Álvarez, E. (2018). Niche conservatism and spread of seaweed invasive lineages with different residence time in the Mediterranean Sea. Biol. Invasions 20, 423–435. doi: 10.1007/s10530-017-1544-8
Chen, I. C., Hill, J. K., Ohlemüller, R., Roy, D. B., and Thomas, C. D. (2011). Rapid range shifts of species associated with high levels of climate warming. Science 333, 1024–1026. doi: 10.1126/science.1206432
Chiquillo, K. L., Barber, P. H., and Willette, D. A. (2018). Fruits and flowers of the invasive seagrass Halophila stipulacea in the Caribbean Sea. Botanica Marina 62, 109–112. doi: 10.1515/bot-2018-0052
Christianen, M. J., Smulders, F. O., Engel, M. S., Nava, M. I., Willis, S., Debrot, A. O., et al. (2019). Megaherbivores may impact expansion of invasive seagrass in the Caribbean. J. Ecol. 107, 45–57. doi: 10.1111/1365-2745.13021
Christianen, M. J., van Belzen, J., Herman, P. M., van Katwijk, M. M., Lamers, L. P., van Leent, P. J., et al. (2013). Low-canopy seagrass beds still provide important coastal protection services. PLoS One 8:e62413. doi: 10.1371/journal.pone.0062413
Conover, D. O., Clarke, L. M., Munch, S. B., and Wagner, G. N. (2006). Spatial and temporal scales of adaptive divergence in marine fishes and the implications for conservation. J. Fish Biol. 69, 21–47. doi: 10.1111/j.1095-8649.2006.01274.x
Coro, G., Vilas, L. G., Magliozzi, C., Ellenbroek, A., Scarponi, P., and Pagano, P. (2018). Forecasting the ongoing invasion of Lagocephalus sceleratus in the Mediterranean Sea. Ecol. Modell. 371, 37–49. doi: 10.1016/j.ecolmodel.2018.01.007
Davidson, A. M., Jennions, M., and Nicotra, A. B. (2011). Do invasive species show higher phenotypic plasticity than native species and, if so, is it adaptive? A meta-analysis. Ecol. Lett. 14, 419–431. doi: 10.1111/j.1461-0248.2011.01596.x
Dell, A. I., Pawar, S., and Savage, V. M. (2011). Systematic variation in the temperature dependence of physiological and ecological traits. Proc. Natl. Acad. Sci. U. S. A. 108, 10591–10596. doi: 10.1073/pnas.1015178108
Den Hartog, C. (1970). The seagrasses of the world. Verhandelingen der Koninklijke Nederlandsche Akademie van Wetenshappen Afdeeling Natuurkunde. Amsterdam: North-Holland Publishing Company, 275.
Di Genio, S., Gaglioti, M., Meneghesso, C., Barbieri, F., Cerrano, C., and Gambi, M. C. (2021). Phenology and ecology of the alien seagrass Halophila stipulacea in its northern range limit in the Mediterranean Sea. Aquat. Bot. 168:103304. doi: 10.1016/j.aquabot.2020.103304
Di Martino, V., Stancanelli, B., and Molinari, A. (2007). Fish community associated with Halophila stipulacea meadow in the Mediterranean Sea. Cybium Int. J. Ichthyol. 31, 451–458.
Doherty, T. S., Glen, A. S., Nimmo, D. G., Ritchie, E. G., and Dickman, C. R. (2016). Invasive predators and global biodiversity loss. Proc. Natl. Acad. Sci. U.S.A. 113, 11261–11265.
Duarte, C. M., Kennedy, H., Marbà, N., and Hendriks, I. (2013). Assessing the capacity for seagrass meadows for carbon burial: current limitations and future strategies. Ocean Coast. Manage. 51, 671–688.
Duncan, R. P., Blackburn, T. M., and Sol, D. (2003). The ecology of bird introductions. Annu. Rev. Ecol. Evol. Syst. 34, 71–98. doi: 10.1146/annurev.ecolsys.34.011802.132353
Elith, J., and Leathwick, J. R. (2009). Species distribution models: ecological explanation and prediction across space and time. Annu. Rev. Ecol. Evol. Syst. 40, 677–697. doi: 10.1146/annurev.ecolsys.110308.120159
Fielding, A. H., and Bell, J. F. (1997). A review of methods for the assessment of prediction errors in conservation presence/absence models. Environ. Conserv. 24, 38–49. doi: 10.1017/s0376892997000088
Forskal, P. (1775). Flora Aegyptiaco-Arabica: Sive Descriptiones Plantarum, Quas per Aegyptum Inferiorem et Arabiam Felicem Detexit. Hanniae: Ex Officina Mölleri.
Forti, A. (1927). La propagazione dell’Halophila stipulacea (Forsk) Asch. Anche nel Mediterraneo. Nuovo Giorno Botanica 34, 714–716.
Fourqurean, J. W., Duarte, C. M., Kennedy, H., Marbà, N., Holmer, M., Mateo, M. A., et al. (2012). Seagrass ecosystems as a globally significant carbon stock. Nat. Geosci. 5, 505–509. doi: 10.1002/eap.1489
Francour, P. (1997). Fish assemblages of Posidonia oceanica beds at Port-Cros (France, NW Mediterranean): assessment of composition and long-term fluctuations by visual census. Mar. Ecol. 18, 157–173.
Fritsch, C. (1895). Über die Auffindung einer marinen Hydrocharidee im Mittelmeer. Verhandlungen der Zoologish-Botanischen Gesellshaft in Wien 45, 104–106.
Gallardo, B., Clavero, M., Sánchez, M. I., and Vilà, M. (2016). Global ecological impacts of invasive species in aquatic ecosystems. Glob. Chang. Biol. 22, 151–163. doi: 10.1111/gcb.13004
Gambi, M. C., Gaglioti, M., and Barbieri, F. (2018). Sometimes they come back: the re-colonization of the alien seagrass Halophila stipulacea (Forsskål and Niebuhr) Ascherson, 1867 (Hydrocharitaceae) in the Palinuro Harbor (Tyrrhenian Sea, Italy). Bioinvasions Rec. 7, 215–221. doi: 10.3391/bir.2018.7.3.01
Gamliel, I., Buba, Y., Guy-Haim, T., Garval, T., Willette, D., Rilov, G., et al. (2020). Incorporating physiology into species distribution models moderates the projected impact of warming on selected Mediterranean marine species. Ecography 43, 1090–1106.
Georgiou, D., Alexandre, A., Luis, J., and Santos, R. (2016). Temperature is not a limiting factor for the expansion of Halophila stipulacea throughout the Mediterranean sea. Mar. Ecol. Prog. Ser. 544, 159–167.
Geraldi, N. R., Anton, A., Santana-Garcon, J., Bennett, S., Marbà, N., Lovelock, C. E., et al. (2020). Ecological effects of non-native species in marine ecosystems relate to co-occurring anthropogenic pressures. Glob. Chang. Biol. 26, 1248–1258. doi: 10.1111/gcb.14930
Goldsmit, J., McKindsey, C. W., Schlegel, R. W., Stewart, D. B., Archambault, P., and Howland, K. L. (2020). What and where? Predicting invasion hotspots in the Arctic marine realm. Glob. Chang. Biol. 26, 4752–4771. doi: 10.1111/gcb.15159
Gottfried, M., Pauli, H., Futschik, A., Akhalkatsi, M., Barančok, P., Alonso, J. L. B., et al. (2012). Continent-wide response of mountain vegetation to climate change. Nat. Clim. Chang. 2, 111–115.
Guisan, A., Petitpierre, B., Broennimann, O., Daehler, C., and Kueffer, C. (2014). Unifying niche shift studies: insights from biological invasions. Trends Ecol. Evol. 29, 260–269. doi: 10.1016/j.tree.2014.02.009
Guisan, A., and Thuiller, W. (2005). Predicting species distribution: offering more thn simple habitat models. Ecol. Lett. 8, 993–1009. doi: 10.1111/j.1461-0248.2005.00792.x
Heck, K. L., Carruthers, T. J., Duarte, C. M., Hughes, A. R., Kendrick, G., Orth, R. J., et al. (2008). Trophic transfers from seagrass meadows subsidize diverse marine and terrestrial consumers. Ecosystems 11, 1198–1210. doi: 10.1007/s10021-008-9155-y
Hijmans, R. J. (2020). raster: Geographic Data Analysis and Modeling. R package version 3.0-12. Available online at: https://CRAN.R-project.org/package=raster (accessed September, 2020).
Hoffmann, A. A., and Sgrò, C. M. (2011). Climate change and evolutionary adaptation. Nature 470, 479–485.
Intergovernmental Panel on Climate Change [IPCC] (2014). “Climate Change 2014: Synthesis Report,” in Contribution of Working Groups I, II and III to the Fifth Assessment Report of the Intergovernmental Panel on Climate Change, eds R. K. Pachauri and L. A. Meyer (Geneva: IPCC), 151.
Jordà, G., Marbà, N., and Duarte, C. M. (2012). Mediterranean seagrass vulnerable to regional climate warming. Nat. Clim. Chang. 2, 821–824. doi: 10.1038/nclimate1533
Kearney, M., and Porter, W. (2009). Mechanistic niche modelling: combining physiological and spatial data to predict species’ ranges. Ecol. Lett. 12, 334–350. doi: 10.1111/j.1461-0248.2008.01277.x
Kendrick, G. A., Waycott, M., Carruthers, T. J., Cambridge, M. L., Hovey, R., Krauss, S. L., et al. (2012). The central role of dispersal in the maintenance and persistence of seagrass populations. Bioscience 62, 56–65. doi: 10.1525/bio.2012.62.1.10
Khaliq, I., Fritz, S. A., Prinzinger, R., Pfenninger, M., Böhning-Gaese, K., and Hof, C. (2015). Global variation in thermal physiology of birds and mammals: evidence for phylogenetic niche conservatism only in the tropics. J. Biogeogr. 42, 2187–2196.
King, N. G., McKeown, N. J., Smale, D. A., and Moore, P. J. (2018). The importance of phenotypic plasticity and local adaptation in driving intraspecific variability in thermal niches of marine macrophytes. Ecography 41, 1469–1484.
Koch, M. S., Schopmeyer, S., Kyhn-Hansen, C., and Madden, C. J. (2007). Synergistic effects of high temperature and sulfide on tropical seagrass. J. Exp. Mar. Biol. Ecol. 341, 91–101.
Langer, M. R., Weinmann, A. E., Lötters, S., and Rödder, D. (2012). “Strangers” in Paradise: modeling the biogeographic range expansion of the foraminifera Amphistegina in the Mediterranean Sea. J. Foraminiferal Res. 42, 234–244.
Lee, C. E., and Bell, M. A. (1999). Causes and consequences of recent freshwater invasions by saltwater animals. Trends Ecol. Evol. 14, 284–288. doi: 10.1016/S0169-5347(99)01596-7
Lipkin, Y. (1975a). Halophila stipulacea in Cyprus and Rhodes. 1967–1970. Aquat. Bot. 1, 309–318. doi: 10.1016/0304-3770(75)90029-7
Lipkin, Y. (1975b). Halophila stipulacea, a review of a successful immigration. Aquat. Bot. 1, 203–215. doi: 10.1016/0304-3770(75)90023-6
Liu, C., Wolter, C., Xian, W., and Jeschke, J. M. (2020). Most invasive species largely conserve their climatic niche. Proc. Natl. Acad. Sci. U. S. A. 117, 23643–23651. doi: 10.1073/pnas.2004289117
Mack, R. N., Simberloff, D., Lonsdale, W. M., Evans, H., Clout, M., and Bazzae, F. A. (2000). Biotic invasions: causes, epidemiology, global consequences, and control. Ecol. Appl. 10, 689–710. doi: 10.1890/1051-0761(2000)010[0689:bicegc]2.0.co;2
Maggi, E., Benedetti-Cecchi, L., Castelli, A., Chatzinikolaou, E., Crowe, T. P., Ghedini, G., et al. (2015). Ecological impacts of invading seaweeds: a meta-analysis of their effects at different trophic levels. Divers. Distrib. 21, 1–12. doi: 10.1111/ddi.12264
Magozzi, S., and Calosi, P. (2015). Integrating metabolic performance, thermal tolerance, and plasticity enables for more accurate predictions on species vulnerability to acute and chronic effects of global warming. Glob. Chang. Biol. 21, 181–194. doi: 10.1111/gcb.12695
Marbà, N., Jorda, G., Agustí, S., Girard, C., and Duarte, C. M. (2015). Footprints of climate change on Mediterranean Sea biota. Front. Mar. Sci. 2:56. doi: 10.3389/fmars.2015.00056
Martínez, B., Arenas, F., Trilla, A., Viejo, R. M., and Carreño, F. (2015). Combining physiological threshold knowledge to species distribution models is key to improving forecasts of the future niche for macroalgae. Glob. Chang. Biol. 21, 1422–1433. doi: 10.1111/gcb.12655
Matesanz, S., Gianoli, E., and Valladares, F. (2010). Global change and the evolution of phenotypic plasticity in plants. Ann. N. Y. Acad. Sci. 2, 35–55. doi: 10.1111/j.1749-6632.2010.05704.x
Mazarrasa, I., Marbà, N., Garcia-Orellana, J., Masqué, P., Arias-Ortiz, A., and Duarte, C. M. (2017). Effect of environmental factors (wave exposure and depth) and anthropogenic pressure in the C sink capacity of Posidonia oceanica meadows. Limnol. Oceanogr. 62, 1436–1450.
McMillan, C. (1984). The distribution of tropical seagrasses with relation to their tolerance of high temperatures. Aquat. Bot. 19, 369–379.
Meinshausen, M., Smith, S. J., Calvin, K., Daniel, J. S., Kainuma, M. L., and Lamarque, J. F. (2011). The RCP greenhouse gas concentrations and their extensions from 1765 to 2300. Clim. Chang. 109, 213–241. doi: 10.1007/s10584-011-0156-z
Millot, C., and Taupier-Letage, I. (2005). “Circulation in the Mediterranean Sea,” in The Mediterranean Sea, ed. A. Saliot (Berlin: Springer), 29–66.
Moss, R. H., Babiker, M., Brinkman, S., Calvo, E., Carter, T., Edmonds, J. A., et al. (2008). Towards new scenarios for the analysis of emissions: Climate change, impacts and response strategies. Geneva: Intergovernmental Panel on Climate Change.
Nguyen, H. M., Kleitou, P., Kletou, D., Sapir, Y., and Winters, G. (2018). Differences in flowering sex ratios between native and invasive populations of the seagrass Halophila stipulacea. Botanica Marina 61, 337–342. doi: 10.1515/bot-2018-0015
Nicotra, A. B., Atkin, O. K., Bonser, S. P., Davidson, A. M., Finnegan, E. J., Mathesius, U., et al. (2010). Plant phenotypic plasticity in a changing climate. Trends Plant Sci. 15, 684–692. doi: 10.1016/j.tplants.2010.09.008
Oscar, M. A., Barak, S., and Winters, G. (2018). The tropical invasive seagrass, Halophila stipulacea, has a superior ability to tolerate dynamic changes in salinity levels compared to its freshwater relative, Vallisneria americana. Front. Plant Sci. 9:950. doi: 10.3389/fpls.2018.00950
Padfield, D., Yvon-Durocher, G., Buckling, A., Jennings, S., and Yvon-Durocher, G. (2016). Rapid evolution of metabolic traits explains thermal adaptation in phytoplankton. Ecol. Lett. 19, 133–142. doi: 10.1111/ele.12545
Pais, A., Merella, P., Follesa, M. C., and Garippa, G. (2007). Westward range expansion of the Lessepsian migrant Fistularia commersonii (Fistulariidae) in the Mediterranean Sea, with notes on its parasites. J. Fish Biol. 70, 269–277. doi: 10.1111/j.1095-8649.2006.01302.x
Parmesan, C., and Yohe, G. (2003). A globally coherent fingerprint of climate change impacts across natural systems. Nature 421, 37–42. doi: 10.1038/nature01286
Parravicini, V., Azzurro, E., Kulbicki, M., and Belmaker, J. (2015). Niche shift can impair the ability to predict invasion risk in the marine realm: an illustration using Mediterranean fish invaders. Ecol. Lett. 18, 246–253. doi: 10.1111/ele.12401
Peck, L. S., Morley, S. A., Richard, J., and Clark, M. S. (2014). Acclimation and thermal tolerance in Antarctic marine ectotherms. J. Exp. Biol. 217, 16–22. doi: 10.1242/jeb.089946
Pecl, G. T., Araújo, M. B., Bell, J. D., Blanchard, J., Bonebrake, T. C., Chen, I. C., et al. (2017). Biodiversity redistribution under climate change: impacts on ecosystems and human well-being. Science 355:eaai9214. doi: 10.1126/science.aai9214
Peterson, A. T., and Soberón, J. (2012). Species distribution modeling and ecological niche modeling: getting the concepts right. Nat. Conserv. 10, 102–107. doi: 10.4322/natcon.2012.019
Peterson, A. T., Soberón, J., and Sánchez-Cordero, V. (1999). Conservatism of ecological niches in evolutionary time. Science 285, 1265–1267. doi: 10.1126/science.285.5431.1265
Poloczanska, E. S., Brown, C. J., Sydeman, W. J., Kiessling, W., Schoeman, D. S., Moore, P. J., et al. (2013). Global imprint of climate change on marine life. Nat. Clim. Chang. 3, 919–925. doi: 10.1038/nclimate1958
Poloczanska, E. S., Burrows, M. T., Brown, C. J., GarcíaMolinos, J., Halpern, B. S., Hoegh-Guldberg, O., et al. (2016). Responses of marine organisms to climate change across oceans. Front. Mar. Sci. 3:62. doi: 10.3389/fmars.2016.00062
Poursanidis, D., Kalogirou, S., Azzurro, E., Parravicini, V., Bariche, M., and zu Dohna, H. (2020). Habitat suitability, niche unfilling and the potential spread of Pterois miles in the Mediterranean Sea. Mar. Pollut. Bull. 154:111054. doi: 10.1016/j.marpolbul.2020.111054
Prentis, P. J., Wilson, J. R. U., Dormontt, E. E., Richardson, D. M., and Lowe, A. J. (2008). Adaptive evolution in invasive species. Trends Plant Sci. 13, 288–294. doi: 10.1016/j.tplants.2008.03.004
R Core Team. (2020). R: A language and environment for statistical computing. Vienna: R Foundation for Statistical Computing.
Record, S., Strecker, A., Tuanmu, M. N., Beaudrot, L., Zarnetske, P., Belmaker, J., et al. (2018). Does scale matter? a systematic review of incorporating biological realism when predicting changes in species distributions. PLoS One 13:e0194650. doi: 10.1371/journal.pone.0194650
Richardson, P. L. (2005). Caribbean Current and eddies as observed by surface drifters. Deep Sea Res. II Top. Stud. Oceanogr. 52, 429–463. doi: 10.1016/j.dsr2.2004.11.001
Rohr, J. R., Civitello, D. J., Cohen, J. M., Roznik, E. A., Sinervo, B., and Dell, A. I. (2018). The complex drivers of thermal acclimation and breadth in ectotherms. Ecol. Lett. 21, 1425–1439. doi: 10.1111/ele.13107
Ruiz, H., and Ballantine, D. L. (2004). Occurrence of the seagrass Halophila stipulacea in the tropical West Atlantic. Bull. Mar. Sci. 75, 131–135.
Ruiz, H., Ballantine, D. L., and Sabater, J. (2017). Continued spread of the seagrass Halophila stipulacea in the Caribbean: documentation in Puerto Rico and the British Virgin Islands. Gulf Caribb. Res 28, SC5–SC7.
Sánchez-González, J. F., Sánchez-Rojas, V., and Memos, C. D. (2011). Wave attenuation due to Posidonia oceanica meadows. J. Hydraul. Res. 49, 503–514.
Sanford, E., and Kelly, M. W. (2011). Local adaptation in marine invertebrates. Annu. Rev. Mar. Sci. 3, 509–535. doi: 10.1146/annurev-marine-120709-142756
Sarà, G., Porporato, E. M., Mangano, M. C., and Mieszkowska, N. (2018). Multiple stressors facilitate the spread of a non-indigenous bivalve in the Mediterranean Sea. J. Biogeogr. 45, 1090–1103. doi: 10.1111/jbi.13184
Sardain, A., Sardain, E., and Leung, B. (2019). Global forecasts of shipping traffic and biological invasions to 2050. Nat. Sustain. 2, 274–282.
Savva, I., Bennett, S., Roca, G., Jordà, G., and Marbà, N. (2018). Thermal tolerance of Mediterranean marine macrophytes: vulnerability to global warming. Ecol. Evol. 8, 12032–12043. doi: 10.1002/ece3.4663
Schlüter, L., Lohbeck, K. T., Gutowska, M. A., Gröger, J. P., Riebesell, U., and Reusch, T. B. H. (2014). Adaptation of a globally important coccolithophore to ocean warming and acidification. Nat. Clim. Chang. 4, 1024–1030. doi: 10.1126/sciadv.1501660
Seebens, H., Blackburn, T. M., Dyer, E. E., Genovesi, P., Hulme, P. E., Jeschke, J. M., et al. (2017). No saturation in the accumulation of alien species worldwide. Nat. Commun. 8:14435. doi: 10.1038/ncomms14435
Sghaier, Y. R., Zakhama-Sraieb, R., Benamer, I., and Charfi-Cheikhrouha, F. (2011). Occurrence of the seagrass Halophila stipulacea (Hydrocharitaceae) in the southern Mediterranean. Botanica Marina 54, 575–582. doi: 10.1007/s00248-019-01450-3
Sharon, Y., Levitan, O., Spungin, D., Berman-Frank, I., and Beer, S. (2011). Photoacclimation of the seagrass Halophila stipulacea to the dim irradiance at its 48-meter depth limit. Limnol. Oceanogr. 56, 357–362. doi: 10.4319/lo.2011.56.1.0357
Smulders, F. O., Vonk, J. A., Engel, M. S., and Christianen, M. J. (2017). Expansion and fragment settlement of the non-native seagrass Halophila stipulacea in a Caribbean bay. Mar. Biol. Res. 13, 967–974. doi: 10.1080/17451000.2017.1333620
Sorte, C. J., Ibáñez, I., Blumenthal, D. M., Molinari, N. A., Miller, L. P., Grosholz, E. D., et al. (2013). Poised to prosper? A cross-system comparison of climate change effects on native and non-native species performance. Ecol. Lett. 16, 261–270. doi: 10.1111/ele.12017
Sorte, C. J. B., Williams, S. L., and Carlton, J. T. (2010). Marine range shifts and species introductions: comparative spread rates and community impacts. Glob. Ecol. Biogeogr. 19, 303–316. doi: 10.1111/j.1466-8238.2009.00519.x
Steiner, S. C. C., and Willette, D. A. (2015). The expansion of Halophila stipulacea (Hydrocharitaceae, Angiospermae) is changing the seagrass landscape in the commonwealth of Dominica, Lesser Antilles. Caribb. Nat. 22, 1–19.
Sunday, J. M., Pecl, G. T., Frusher, S., Hobday, A. J., Hill, N., Holbrook, N. J., et al. (2015). Species traits and climate velocity explain geographic range shifts in an ocean-warming hotspot. Ecol. Lett. 18, 944–953. doi: 10.1111/ele.12474
Talluto, M. V., Boulangeat, I., Ameztegui, A., Aubin, I., Berteaux, D., Butler, A., et al. (2016). Cross-scale integration of knowledge for predicting species ranges: a metamodelling framework. Glob. Ecol. Biogeogr. 25, 238–249. doi: 10.1111/geb.12395
Terrados, J., Duarte, C. M., Fortes, M. D., Borum, J., Agawin, N. S. R., Bach, S., et al. (1998). Changes in community structure and biomass of seagrass communities along gradients of siltation in SE Asia. Estuar. Coast. Shelf Sci. 46, 757–768. doi: 10.1006/ecss.1997.0304
Thuiller, W., Georges, D., Engler, R., and Breiner, F. (2020). biomod2: Ensemble Platform for Species Distribution Modeling. R package version 3.4.10.
Thuiller, W., Richardson, D. M., Pysek, P., Midgley, G. F., Hughes, G. O., and Rouget, M. (2005). Niche-based modelling as a tool for predicting the risk of alien plant invasions at a global scale. Glob. Chang. Biol. 11, 2234–2250. doi: 10.1111/j.1365-2486.2005.001018.x
Tol, S. J., Jarvis, J. C., York, P. H., Grech, A., Congdon, B. C., and Coles, R. G. (2017). Long distance biotic dispersal of tropical seagrass seeds by marine mega-herbivores. Sci. Rep. 7:4458. doi: 10.1038/s41598-017-04421-1
Tomas, F., Turon, X., and Romero, J. (2005). Seasonal and small-scale spatial variability of herbivory pressure on the temperate seagrass Posidonia oceanica. Mar. Ecol. Prog. Ser. 301, 95–107. doi: 10.3354/meps301095
Tyberghein, L., Verbruggen, H., Pauly, K., Troupin, C., Mineur, F., and De Clerck, O. (2012). Bio-ORACLE: a global environmental dataset for marine species distribution modelling. Glob. Ecol. Biogeogr. 21, 272–281. doi: 10.1111/j.1466-8238.2011.00656.x
Valladares, F., Matesanz, S., Guilhaumon, F., Araújo, M. B., Balaguer, L., Benito-Garzón, M., et al. (2014). The effects of phenotypic plasticity and local adaptation on forecasts of species range shifts under climate change. Ecol. Lett. 17, 1351–1364. doi: 10.1111/ele.12348
Vera, B., Collado-Vides, L., Moreno, C., and van Tussenbroek, B. I. (2014). Halophila stipulacea (Hydrocharitaceae): a recent introduction to the continental waters of Venezuela. Caribb. J. Sci. 48, 66–70. doi: 10.18475/cjos.v48i1.a11
Vilà, M., Espinar, J. L., Hejda, M., Hulme, P. E., Jarošík, V., Maron, J. L., et al. (2011). Ecological impacts of invasive alien plants: a meta-analysis of their effects on species, communities and ecosystems. Ecol. Lett. 14, 702–708. doi: 10.1111/j.1461-0248.2011.01628.x
Wernberg, T., Bennett, S., Babcock, R. C., De Bettignies, T., Cure, K., Depczynski, M., et al. (2016). Climate-driven regime shift of a temperate marine ecosystem. Science 353, 169–172. doi: 10.1126/science.aad8745
Wesselmann, M., Anton, A., Duarte, C. M., Hendriks, I. E., Agustí, S., Savva, I., et al. (2020b). Tropical seagrass Halophila stipulacea shifts thermal tolerance during Mediterranean invasion. Proc. R. Soc. B 287:20193001. doi: 10.1098/rspb.2019.3001
Wesselmann, M., Anton, A., Duarte, C. M., Hendriks, I. E., Agustí, S., Savva, I., et al. (2020a). Thermal tolerance of Halophila stipulacea in its native and exotic distributional range [Dataset]. Madrid: DIGITAL.CSIC.
Wesselmann, M., Geraldi, N. R., Duarte, C. M., Garcia-Orellana, J., Díaz-Rúa, R., Arias- Ortiz, A., et al. (2021). Seagrass (Halophila stipulacea) invasion enhances carbon sequestration in the Mediterranean Sea. Glob. Chang. Biol. 27, 2592–2607. doi: 10.1111/gcb.15589
Willette, D. A., and Ambrose, R. F. (2009). The distribution and expansion of the invasive seagrass Halophila stipulacea in Dominica, West Indies, with a preliminary report from St. Lucia. Aquat. Bot. 91, 137–142.
Willette, D. A., and Ambrose, R. F. (2012). Effects of the invasive seagrass Halophila stipulacea on the native seagrass, Syringodium filiforme, and associated fish and epibiota communities in the Eastern Caribbean. Aquat. Bot. 103, 74–82.
Willette, D. A., Chalifour, J., Debrot, A. D., Engel, M. S., Miller, J., Oxenford, H. A., et al. (2014). Continued expansion of the trans-Atlantic invasive marine Halophila stipulacea—Past, Present, and Future angiosperm Halophila stipulacea in the Eastern Caribbean. Aquat. Bot. 112, 98–102. doi: 10.1016/j.aquabot.2013.10.001
Willette, D. A., Chiquillo, K. L., Cross, C., Fong, P., Kelley, T., Toline, C. A., et al. (2020). Growth and recovery after small-scale disturbance of a rapidly expanding invasive seagrass in St. John, U.S. Virgin islands. J. Exp. Mar. Biol. Ecol. 523:151265.
Wilson, K. L., Skinner, M. A., and Lotze, H. K. (2019). Projected 21st-century distribution of canopy-forming seaweeds in the Northwest Atlantic with climate change. Divers. Distrib. 25, 582–602.
Woodin, S. A., Hilbish, T. J., Helmuth, B., Jones, S. J., and Wethey, D. S. (2013). Climate change, species distribution models, and physiological performance metrics: predicting when biogeographic models are likely to fail. Ecol. Evol. 3, 3334–3346. doi: 10.1002/ece3.680
Keywords: non-native, seagrass, species distribution model (SDM), physiological limits, temperature, Mediterranean Sea, Red Sea, Caribbean Sea
Citation: Wesselmann M, Chefaoui RM, Marbà N, Serrao EA and Duarte CM (2021) Warming Threatens to Propel the Expansion of the Exotic Seagrass Halophila stipulacea. Front. Mar. Sci. 8:759676. doi: 10.3389/fmars.2021.759676
Received: 16 August 2021; Accepted: 10 November 2021;
Published: 01 December 2021.
Edited by:
Glenn Hyndes, Edith Cowan University, AustraliaReviewed by:
Leigh W. Tait, National Institute of Water and Atmospheric Research (NIWA), New ZealandJosé M. Rico, University of Oviedo, Spain
Copyright © 2021 Wesselmann, Chefaoui, Marbà, Serrao and Duarte. This is an open-access article distributed under the terms of the Creative Commons Attribution License (CC BY). The use, distribution or reproduction in other forums is permitted, provided the original author(s) and the copyright owner(s) are credited and that the original publication in this journal is cited, in accordance with accepted academic practice. No use, distribution or reproduction is permitted which does not comply with these terms.
*Correspondence: Marlene Wesselmann, bS53ZXNzZWxtYW5uQGltZWRlYS51aWItY3NpYy5lcw==
†These authors have contributed equally to this work