- 1School of Marine Science and Engineering, Qingdao Agricultural University, Qingdao, China
- 2Key Laboratory of Sustainable Development of Marine Fisheries, Ministry of Agriculture and Rural Affairs, Yellow Sea Fisheries Research Institute, Chinese Academy of Fishery Sciences, Qingdao, China
- 3Laboratory for Marine Fisheries Science and Food Production Processes, Qingdao National Laboratory for Marine Science and Technology, Qingdao, China
- 4Key Laboratory of Mariculture, Ministry of Education, Ocean University of China, Qingdao, China
- 5Key Laboratory of Aquatic Genomics, Ministry of Agriculture and Rural Affairs, Yellow Sea Fisheries Research Institute, Chinese Academy of Fishery Sciences, Qingdao, China
Ammonia is the most common contaminant in aquaculture systems. Due to the unexpected deterioration of natural seawater quality caused by harmful alga blooms in summer, the water exchange cycle for the culture ponds of the swimming crab Portunus trituberculatus, an important aquaculture species in China, is often much longer, and the crabs may be exposed to long-term ammonia stress. However, less information is available regarding the long-term effects of ammonia in marine decapod crustaceans. Therefore, it is of great significance to understand the toxic effects of prolonged ammonia in decapods. In this study, alteration of histology, cellular stress responses (CSRs), and apoptosis in the hepatopancreas of P. trituberculatus, an important aquaculture species, during ammonia stress (5, 15, and 45 mg⋅L–1) for 1, 7, and 15 days and recovery at the normal condition for 7 days, were analyzed. Our results demonstrated a serious impact of long-term (15 days) ammonia stress by depressing crab CSR especially when ammonia concentration exceeds 15 mg⋅L–1. Overall, short- (1 day) and medium-term (7 days) ammonia stress induced CSR, evidenced by upregulated expression of the genes involved in antioxidant defense (SOD, CAT, and GPX), apoptosis (p53, Bax, and Caspase-3), heat shock response (HSR) (Hsp70 and Hsp90), unfolded protein response (UPR) (IRE1, ATF6, and XBP1), and DNA damage response (DDR) (ATR and DNA-PKcs). However, long-term (15 days) ammonia stress, especially when exposed to the ammonia of 15 and 45 mg⋅L–1, resulted in a higher level of apoptosis and severe damage of hepatopancreas, which may be related to the depressed CSR including antioxidant defense, HSR, UPR, and DDR. Notably, after recovery, the expressions of many genes involved in apoptosis, antioxidant response, HSR, UPR, and DDR in the groups exposed to ammonia at 15 and 45 mg⋅L–1 were still significantly different to that of the control group. In summary, care should be taken when P. trituberculatus is exposed to ammonia over 15 mg⋅L–1 especially when exposure duration is longer than 15 days, as the CSR could be compromised. This study provides a reference for a comprehensive understanding of CSR in decapod crustaceans under ammonia stress and will be beneficial for management in the intensive culture of the swimming crab.
Introduction
Ammonia is the most common contaminant in aquaculture systems and, in fact, the major limiting factor during aquaculture that causes massive mortality of culture animals and results in huge economic losses to the industry (Romano and Zeng, 2013; Wang et al., 2017; Zhao et al., 2020). It typically presents in ionized (NH4+) and unionized (NH3) forms in water. Ammonia, in particular the unionized form, not only disrupts various physiological responses including the antioxidant defense but also amplifies the effect of other environmental factors (Li et al., 2008; Barbieri, 2010; Kim et al., 2020). In recent years, the water exchange cycle of marine ponds along coastal areas in northern China is usually prolonged in summer to avoid the potential threat of harmful alga blooms in natural seawater. This undoubtedly increased the risk for exposure of aquaculture animals to accumulated ammonia for a long period. Therefore, it is essential to investigate the long-term effect of ammonia on aquaculture animals.
Environmental stresses including ammonia exposure can inflict damages on cellular macromolecules and induce cellular stress responses (CSR), the defense reactions to the threat of macromolecular damages, which is critical for enabling organisms to cope with stressful stimuli (Kültz, 2005). It has been reported that ammonia can cause overproduction of reactive oxygen species (ROS) and oxidative damage to lipid, protein, and DNA in aquatic organisms (Şahin and Gümüşlü, 2004; Zhao et al., 2019; Bandara et al., 2021). To prevent cellular oxidative damage, the Keap1-Nrf2 pathway senses oxidative stress and coordinates downstream cytoprotective proteins including superoxide dismutase (SOD), catalase (CAT), and glutathione peroxidase (GPX; Kopacz et al., 2020; Sies and Jones, 2020). However, ammonia stress exceeding a threshold often depresses the antioxidant capability of aquatic animals to scavenge excessive ROS (Zhang et al., 2015; Liang et al., 2016; Yu et al., 2020; Cao et al., 2021). Oxidative stress is usually coupled with and the accumulation of misfolded protein, also known as endoplasmic reticulum (ER) stress. To cope with ER stress, the unfolded protein response (UPR) is triggered to maintain protein homeostasis (Dandekar et al., 2015; Liang et al., 2016; Di Conza and Ho, 2020; Zhang et al., 2021). Furthermore, the UPR has also been reported to interact with the DNA damage response (DDR), a pathway involved in detecting and repairing DNA lesions, to maintain genome integrity (Burger et al., 2019; González-Quiroz et al., 2020). Hence, multiple cytoprotective CSR may be activated to counteract ammonia-induced damage in aquatic animals.
When CSR capacity is impaired and not sufficient for alleviation of cellular stress, apoptosis will be activated to eliminate the damaged cells (Kültz, 2005; Somero, 2020). Apoptosis is usually initiated and transduced via the intrinsic (mitochondrial) or the extrinsic (receptor-mediated) pathway (Elmore, 2007; Eimon and Ashkenazi, 2010). It has been reported that acute high environment ammonia (HEA) can induce apoptosis in aquatic animals via the p53-Bax mitochondrial signaling pathway (Cheng et al., 2015, 2019; Liang et al., 2016; Zhang et al., 2020; Meng et al., 2021; Yan et al., 2021). However, the relation of CSR and apoptosis in aquatic animals during long-term ammonia stress is still largely unknown.
The swimming crab Portunus trituberculatus is an important aquaculture species in China. In recent years, a few studies have been conducted on the effect of acute or short-term ammonia stress in intensive culture of P. trituberculatus (Yue et al., 2010; Pan et al., 2018; Si et al., 2020), while scarce information is available in terms of long-term culture conditions. In this study, we hypothesize that long-term ammonia may impair CSR capability and result in tissue damage in the swimming crab. To investigate the CSR and tissue damage during long-term ammonia stress, we analyzed changes in the activities of antioxidant enzymes, expression of the genes involved in antioxidant defense, UPR, DDR, the heat shock response (HSR), and apoptosis pathways, as well as histology of hepatopancreatic tissue of the swimming crabs exposed to ammonia (5, 15, and 45 mg⋅L–1) for short- (1 day), medium- (7 days), and long-term (15 days) period, respectively. Our study may provide a reference for the comprehensive understanding of CSR in decapod crustaceans under ammonia stress and will be beneficial for management in intensive culture.
Materials and Methods
Crab Collection and Maintenance
This experiment was conducted in Blue Valley Campus of Qingdao Agricultural University from August to September 2020. To avoid gender differences in physiology, subadults of male P. trituberculatus (body weight: 115.4 ± 22.1 g; carapace width: 96.3 ± 6.3 mm) were obtained from a local farm in Jiaonan district (Qingdao, Shandong, China) and were acclimated for 2 weeks before experiment initiation. During acclimation, seawater (temperature: 23 ± 1°C; salinity: 30‰; pH: 7.5; NO2–: <0.03 mg⋅L–1; NO3–: <0.46 mg⋅L–1), which meets the standard “water quality standard for fisheries” (GB 11607-89) and a photoperiod of 14:10 h light: dark, were provided. Crabs were fed once every day with fresh Ruditapes philippinarum, and feces were removed after 3 h of feeding. The seawater of 1/2 in each tank was exchanged daily. Each tank was continuously aerated with air stones. As P. trituberculatus is a non-protected species, no special permission is needed in this experiment.
Experimental Protocol
After acclimation, healthy individuals in the intermolt stage of legal size (∼100 g wet weight) were chosen and randomly divided into four groups (80 crabs in each group). For each group, 80 crabs were randomly allocated into four tanks (20 individuals in each tank) as four replicates. One group in natural seawater was used as the control group. The other three groups were exposed to low (5 mg⋅L–1 TAmm, LA), medium (15 mg⋅L–1 TAmm, MA), and high concentration (45 mg⋅L–1 TAmm, HA) of ammonia for at most 15 days, respectively. After ammonia exposure, treatment groups recovered in normal seawater for 7 days. The ammonia concentrations were chosen based on our preliminary experiment, which showed that the mortality of the crabs was ∼50% at 15 mg⋅L–1 after 15 days of exposure, while it was ∼90% when exposed to 45 mg⋅L–1.
During the experiment, 1/2 of seawater in each container was daily exchanged with fresh seawater at desired ammonia concentration, which was prepared with solid ammonium chloride (NH4Cl, CAS: 12125-02-9, purity ≥99.5%) (Sinopharm Chemical Reagent Co., Ltd., Shanghai, China). During the ammonia exposure, we monitored the ammonia levels in the water and adjusted it with freshly prepared stock solution daily to ensure consistency. On day 1 (short-term stress), day 7 (medium-term stress), and day 15 (long-term stress) following ammonia exposure and after recovery, five to six crabs in each group were randomly chosen for hepatopancreatic sampling. All samples were stored at −80°C for further analysis.
Histological Analysis
After 15 days of stress, the hepatopancreas was fixed in 4% paraformaldehyde with a volume of 10 times the sample. The fixed specimens were trimmed into processing cassettes, dehydrated in graded ethanol solutions (70, 80, 90, and 99% for 1–2 h in each step) (CAS: 64-17-5, purity ≥99.8%) (Sinopharm Chemical Reagent Co., Ltd., Shanghai, China), cleared in 100% xylene (CAS: 1330-20-7, purity ≥99%) (Sinopharm Chemical Reagent Co., Ltd., Shanghai, China), and embedded in pure paraffin wax (CAS: 8002-74-2). The sections (5–6 μm) were prepared with a microtome, stained with hematoxylin-eosin (HE), and observed under a light microscope (Olympus, Tokyo, Japan). After observation, the relative area of the tubule (%) and relative area of the lumen (%) (4–5 fields for each individual, and three individuals for each treatment) were quantified according to the previous study (Huang et al., 2020) and using the CaseViewer 2.0 software.
Terminal Deoxynucleotidyl Transferase-Mediated dUTP Nick End Labeling Assay
Terminal deoxynucleotidyl transferase-mediated dUTP nick end labeling (TUNEL) assay was carried out using a Fluorescein TUNEL Cell Apoptosis Detection Kit (Servicebio, Wuhan, China), according to the manufacturer’s instructions. Briefly, the fixed hepatopancreatic samples were dehydrated in graded concentrations of ethanol, embedded in paraffin wax, divided into sections, and mounted on glass slides. The tissue sections were deparaffinized, rehydrated, and treated with protease K solution at 37°C for 25 min, and then washed three times with phosphate buffer solution (PBS). After that, the slides were incubated with the TUNEL reagent in a humid chamber at 37°C for 2 h, washed three times with PBS, counterstained with 4′,6-diamidino-2-phenylindole (DAPI), and visualized on a Nikon Eclipse 80i (Nikon, Melville, NY, United States).
Determination of Antioxidant Enzymes
Hepatopancreatic tissue was powdered under liquid nitrogen, weighed, and homogenized in 0.85% ice-cold saline solution (w:v = 1:9). Homogenates were immediately centrifuged for 10 min at 4°C and 3,000 r/min. The collected supernatants were used for the determination of activities of SOD, CAT, and GPX. All preparation procedures were carried out at 4°C.
The activities of SOD and CAT were determined using commercial kits (Nanjing Jiancheng Bioengineering Institute, Nanjing, China) according to the method described in our previous study (Lu et al., 2016). One SOD unit was defined as the amount of SOD capable of inhibiting 50% of nitrite formation per milligram of protein. One CAT activity unit was defined as the decomposition of 1 mmol H2O2 per second per milligram of protein.
The GPX can catalyze the oxidation of reduced glutathione by H2O2. Thus, the GPX activity could be obtained by monitoring the depletion of reduced glutathione at 412 nm. According to the protocol of the commercial GPX assay kit, each tissue homogenate (0.2 ml) was mixed with 0.2 ml GSH solution (1 mmol L–1). After incubation at 37°C for 5 min, 0.1 ml work solution I was added and then incubated at 37°C for another 5 min. After mixing with 2 ml work solution II, the reaction system was centrifuged for 10 min at 3,500 r/min. The supernatant of 1 ml was then collected; mixed with work solution III (1.0 ml), IV (0.25 ml), and V (0.05 ml); and reacted for 15 min at room temperature. The absorbance of the final reaction system at 412 nm was recorded to calculate the GPX activity. One GPX unit was defined as the amount catalyzing the oxidation of 1 μmol glutathione at 37°C per min for per milligram protein. The final absorbances of all reaction systems in a transparent 96 well plate with flat bottom were read at 550 nm for SOD, 405 nm for CAT, and 412 nm for GPX using Synergy multifunctional enzyme plate analyzer. All enzyme activities were expressed as U⋅mg prot–1.
Quantitative Real-Time PCR
Total RNAs from hepatopancreas were extracted using TRIzol Reagent (Invitrogen, Carlsbad, CA, United States). After confirmation of RNA concentration and purity, 1 μg of total RNA was reversely transcribed into complementary DNA (cDNA) using Evo M-MLV RT Kit (Accurate Biology, Changsha, China). Real-time quantitative PCR (RT-qPCR) was then conducted to quantify the mRNA expression levels of Keap1 (kelch-like ECH associated protein 1), SOD, CAT, GPX, ATR (ataxia-telangiectasia mutated and Rad3 related), DNA-PKcs (DNA-dependent protein kinase catalytic subunit), IRE1 (inositol-requiring enzymes 1), XBP1 (X-box binding protein 1), ATF-6 (activating transcription factor 6), Hsp70 (heat shock protein 70), Hsp90 (heat shock protein 90), p53, Bax, and caspase-3. β-actin was used as the internal control. Specific primers were designed based on gene sequences from our previous transcriptome data or previous studies (Zhang et al., 2009; Cui et al., 2010; Meng et al., 2014, 2015, 2020; Ren et al., 2016; Table 1). The reactions were performed with the following program: 95°C for 30 s; 94°C for 5 s, and 60°C for 15 s (35–40 cycles). Relative mRNA expression levels were calculated using the 2–ΔΔCt method.
Statistical Analysis
Data in this study were expressed as mean ± SD. The SPSS 20.0 software was used to conduct the data analysis. All data were first tested for normality (Kolmogorov–Smirnov test) and homogeneity of variances (Levene’s test), and where necessary log-transformations were applied to meet these assumptions. One-way ANOVA analysis was conducted followed by Duncan’s multiple comparisons test. The detailed statistics are listed in the Supplementary Table 1. P < 0.05 was considered statistically significant.
Results
Histological Changes in Hepatopancreas
Significant histological changes in hepatopancreas under ammonia stress were noticed after day 15 [relative area of tubule, F(DFn = 3, DFd = 54) = 30.699, P = 0.000; relative area of lumen, F(DFn = 3, DFd = 54) = 38.289, P = 0.000] (Figure 1). The hepatopancreas of the control crab was kept in the normal stellate lumen, and R cells were dominant in hepatic tubules. Compared with the control group, the relative area of tubule in the three treatment groups became significantly smaller (P < 0.05). For the LA group, a less clear asterisk-like appearance and a significant dilation of the tubule lumen were observed (P < 0.05). The asterisk-like appearance of the tubule lumen disappeared in the MA and HA groups and their tubule lumen was significantly larger than the control group (P < 0.05) and the LA group (P < 0.05). The hepatopancreatic cells of MA and HA groups also showed various degrees of tumefaction, disintegration, and apoptosis characteristic changes such as nuclear condense. Notably, the most serious damage by HEA occurred in the HA groups, whose cell and nucleus integrities were severely disrupted.
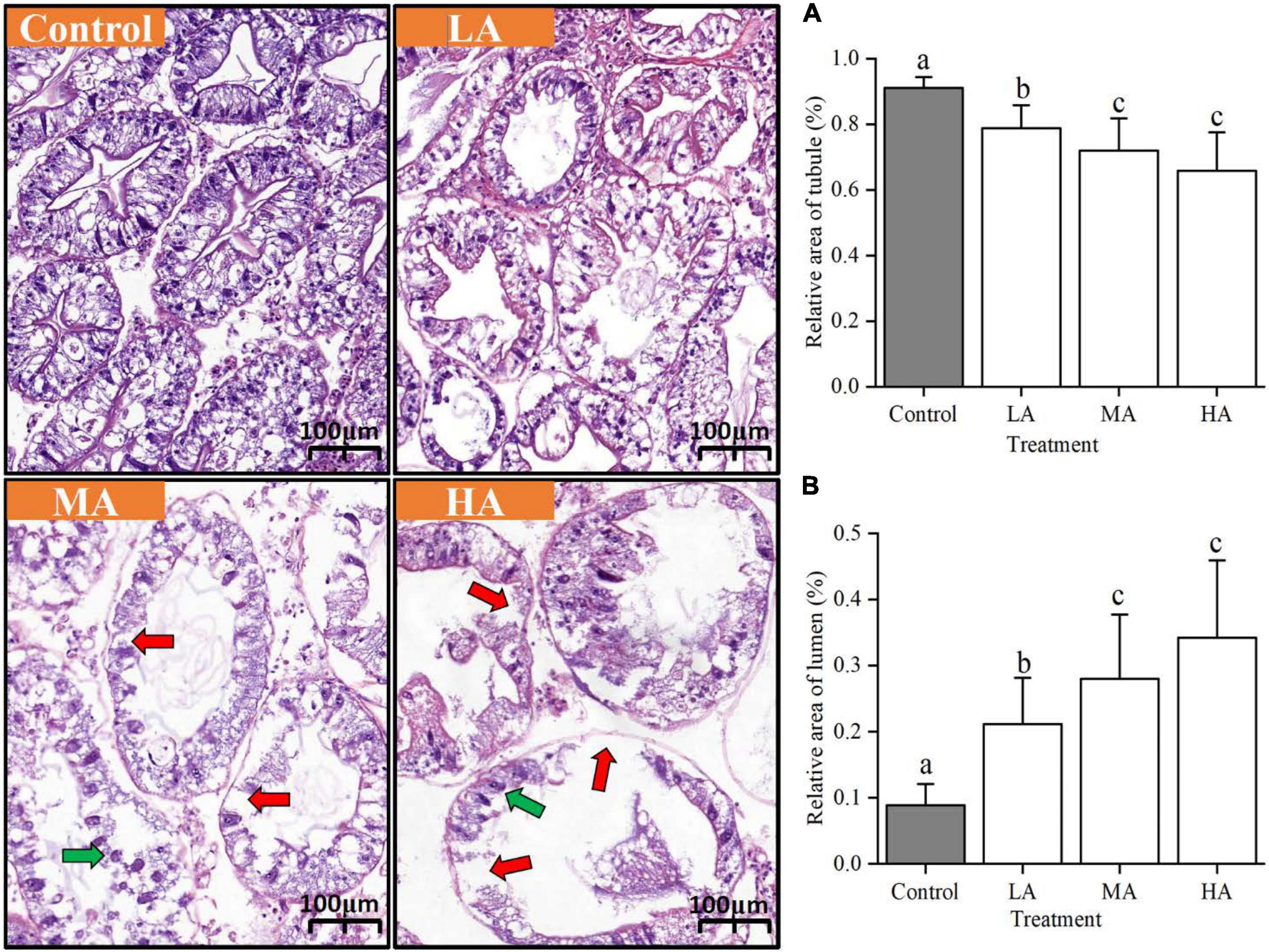
Figure 1. Histological changes in the hepatopancreas of ammonia-stressed P. trituberculatus. (A) Relative area of tubule, (B) relative area of lumen. Red arrow: damaged cellular structure. Green arrow: nuclear condense. Data are represented as mean ± S.D., with P < 0.05 considered significant. Data are represented as mean ± S.D., n = 13-15, with P < 0.05 considered significant.
Antioxidant Response
As shown in Figure 2, the SOD activity was only induced in the LA group on day 7 (P < 0.05). The CAT activity of the LA group was similar to that of the control group, while the CAT activity of the MA and HA groups was significantly decreased after 7 days of ammonia stress (P < 0.05). The GPX activity of the LA and MA groups showed no significant difference compared with the control group. For the HA group, the GPX activity significantly reduced after 15 days of exposure (P < 0.05). Notably, the activity of CAT and GPX in the MA group was significantly higher than that of the control level after recovery (P < 0.05).
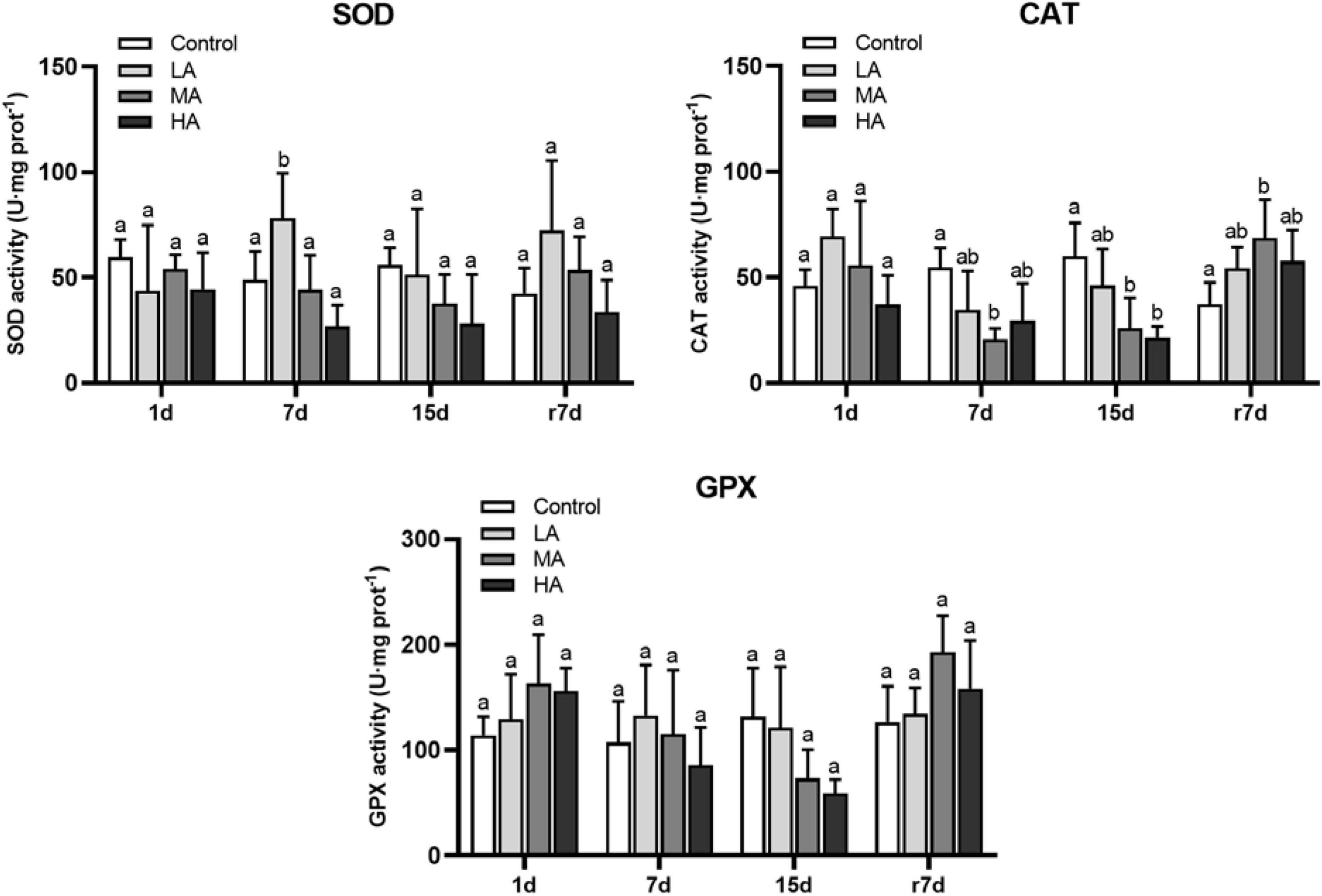
Figure 2. The enzymes activities in hepatopancreas ofP. trituberculatus after ammonia stress and recovery. Data are represented as mean ± S.D., n = 4-6, with P < 0.05 considered significant. Different letters represent significant differences among different groups.
Following ammonia exposure, mRNA expression of SOD was upregulated after short-term stress in the MA group (P < 0.05), while a significant downregulation was observed in the MA and HA groups after day 15 (P < 0.05) (Figure 3). Overall, the CAT expression was negatively related to ammonia stress. During stress, the CAT expression was significantly downregulated in the HA group at each time interval (P < 0.05). The mRNA expression of GPX was upregulated in the MA and HA groups on day 1, while it returned to the control level after day 7 for the HA group and after day 15 for the MA group. The ammonia stress resulted in a downregulation of Keap1 in all treatment groups, and a significant difference was observed in the MA and HA groups at each time interval (P < 0.05). Notably, after recovery, the MA and HA groups showed increased levels of CAT and GPX but decreased expression of Keap1.
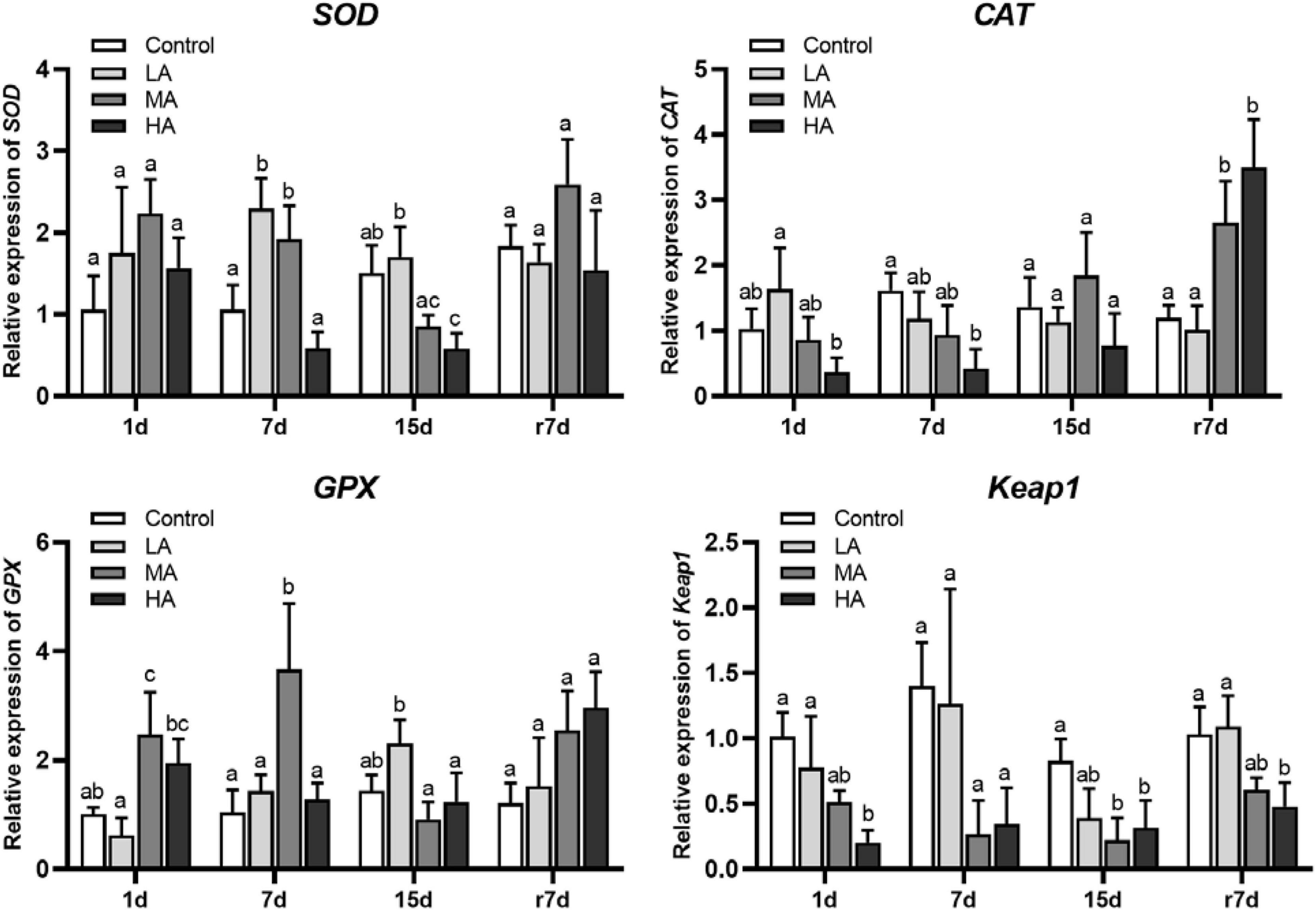
Figure 3. mRNA expression of SOD, CAT, GPX, and Keap1 in hepatopancreas of P. trituberculatus after ammonia stress and recovery. Data are represented as mean ± S.D., n = 3, with P < 0.05 considered significant. Different letters represent significant differences among different groups.
Expression of the Heat Shock Proteins
The expressions of Hsp70 and Hsp90 in the MA and HA groups were significantly upregulated at day 1 (P < 0.05), while they were downregulated at day 15 (Figure 4). During recovery, except for the Hsp90 expression of the LA group, all treatment groups showed a significant upregulation of Hsp70 and Hsp90 in comparison with the control group (P < 0.05).
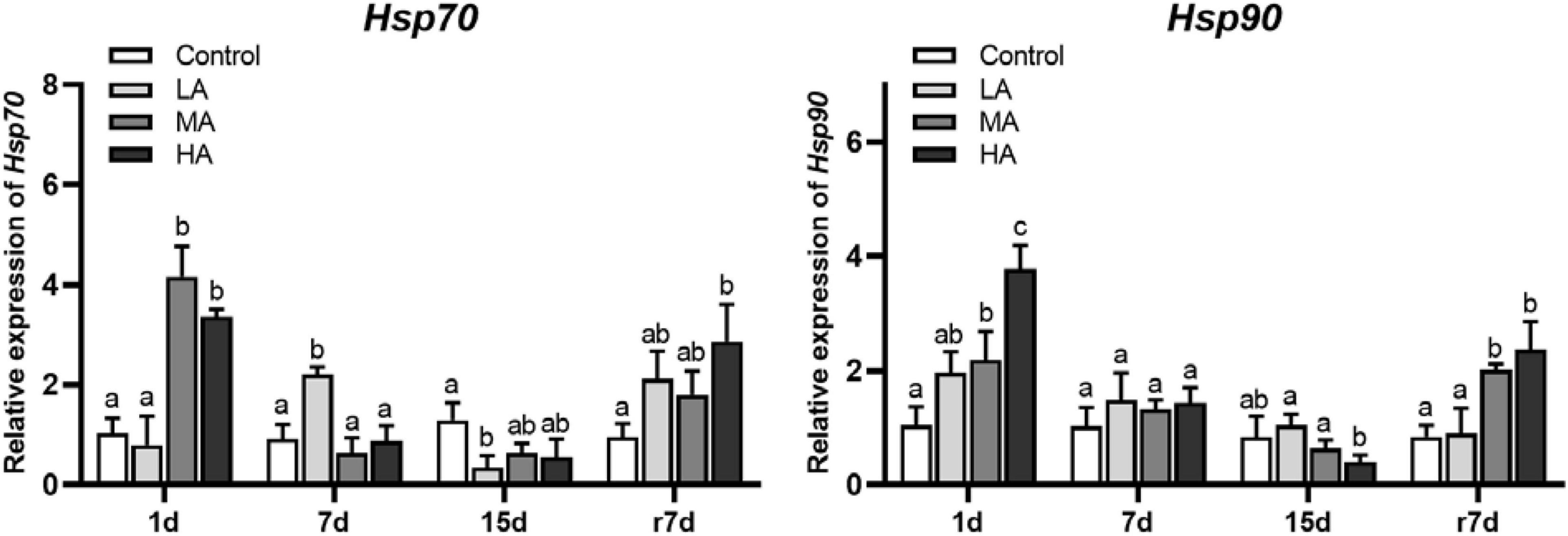
Figure 4. The mRNA expression of heat shock proteins in hepatopancreas of P. trituberculatus after ammonia exposure and recovery. Data are represented as mean ± S.D., n = 3, with P < 0.05 considered significant. Different letters represent significant differences among different groups.
Expression of the Genes Involved in Unfolded Protein Response
The short- and medium-term stress significantly upregulated the mRNA expression of ATF6 and XBP1 (P < 0.05), which returned to the control level at day 15 (Figure 5). During stress, the mRNA expression of IRE1 kept stable in all treatments except for the HA group at day 7. After recovery, an upregulation of IRE1, ATF6, and XBP1 was observed in the MA and HA groups, while a significant difference was only recorded in the XBP1 expression of the HA group (P < 0.05).
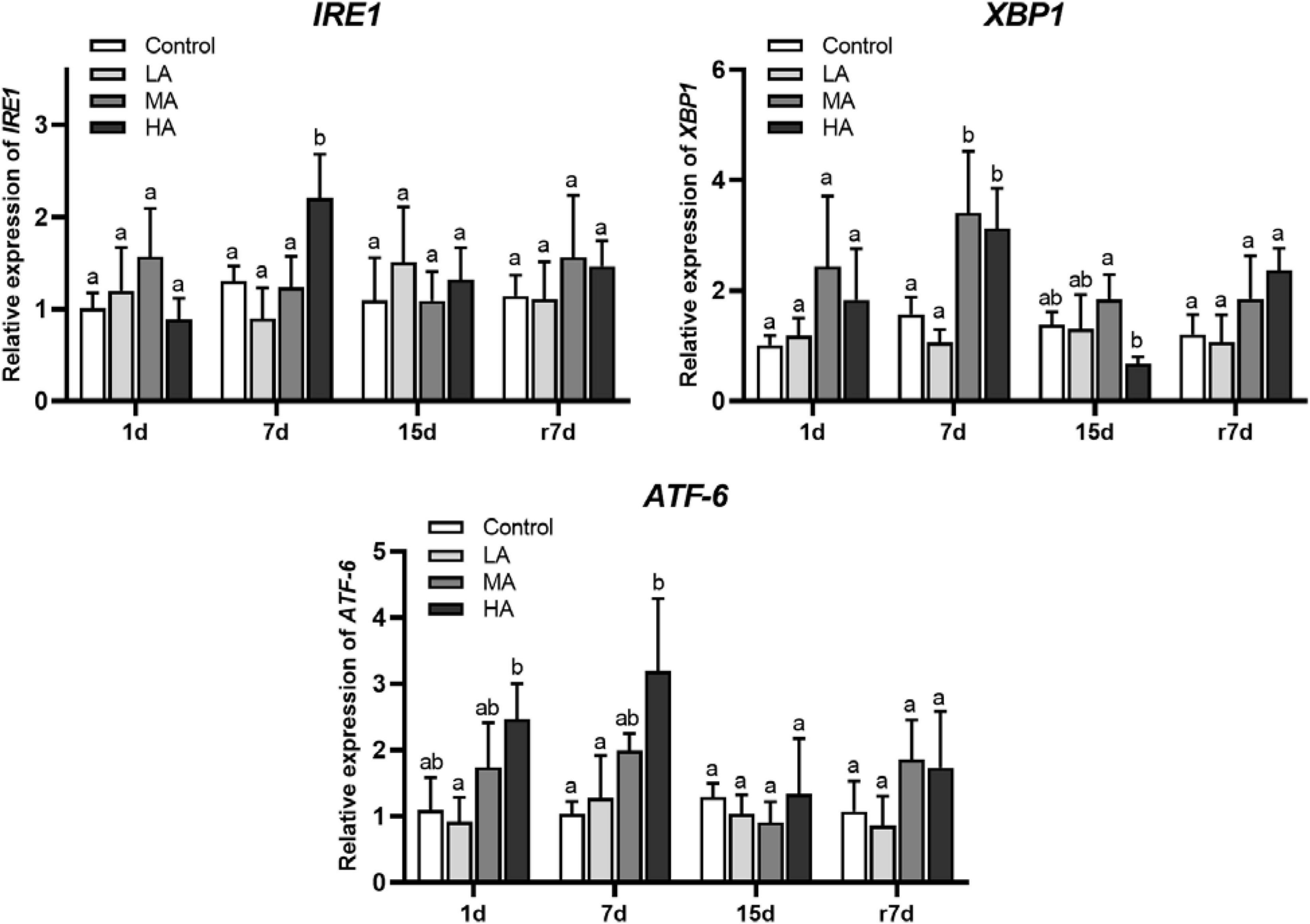
Figure 5. The mRNA expression genes of UPR signaling in hepatopancreas of P. trituberculatus after ammonia exposure and recovery. Data are represented as mean ± S.D., n = 3, with P < 0.05 considered significant. Different letters represent significant differences among different groups.
Expression of the Genes Involved in DNA Damage Response
As shown in Figure 6, ATR was only upregulated in the HA group on day 7. For DNA-PKcs, an upregulation was observed in the HA group at days 1 and 7, while a significant downregulation in the HA group was noticed at day 15 (P < 0.05).
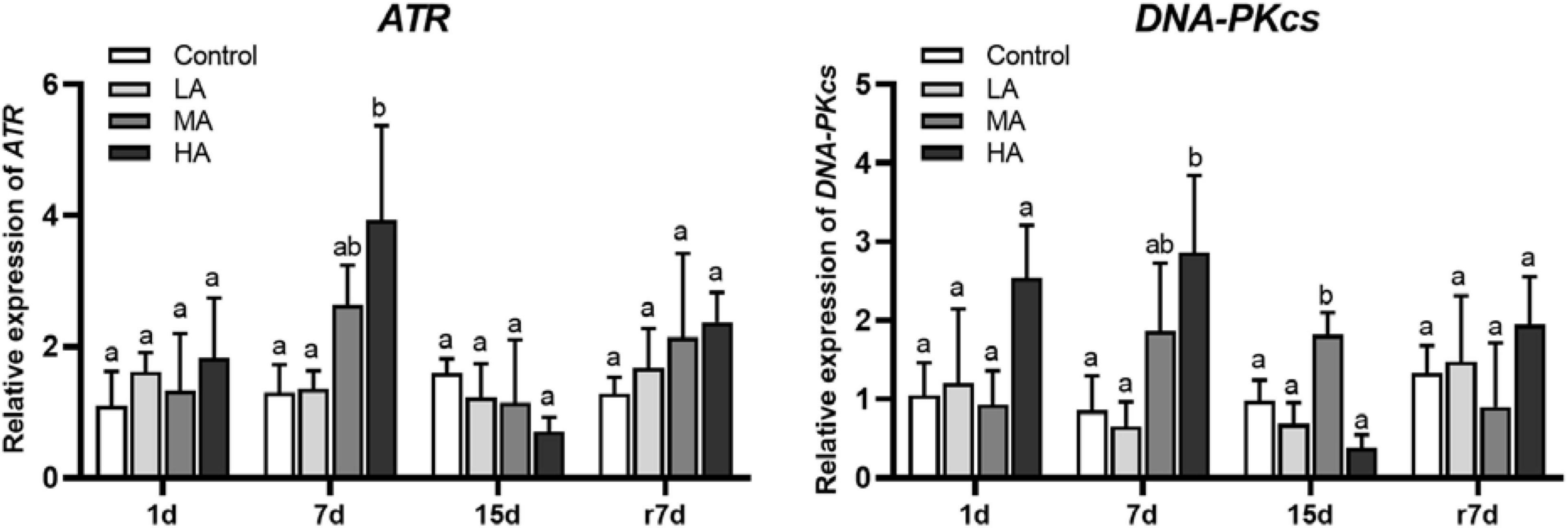
Figure 6. The mRNA expression of the genes involved in DDR signaling in hepatopancreas of P. trituberculatus after ammonia exposure and recovery. Data are represented as mean ± S.D., n = 3, with P < 0.05 considered significant. Different letters represent significant differences among different groups.
Apoptosis and Related Gene Expression
The mRNA expression of p53, Bax, and caspase-3 in the MA and HA groups increased significantly (P < 0.05) after ammonia exposure (Figure 7). It was noticed that only the p53 expression returned to the control level on day 15. After recovery, the Bax expression in the MA and HA groups and caspase-3 expression in the HA group remained at a higher level than those in the control group, and a statistical significance was recorded in the Bax expression of the MA group (P < 0.05). As shown in Figure 8, an increase in the TUNEL-positive cells was observed in all three treatment groups after day 15.
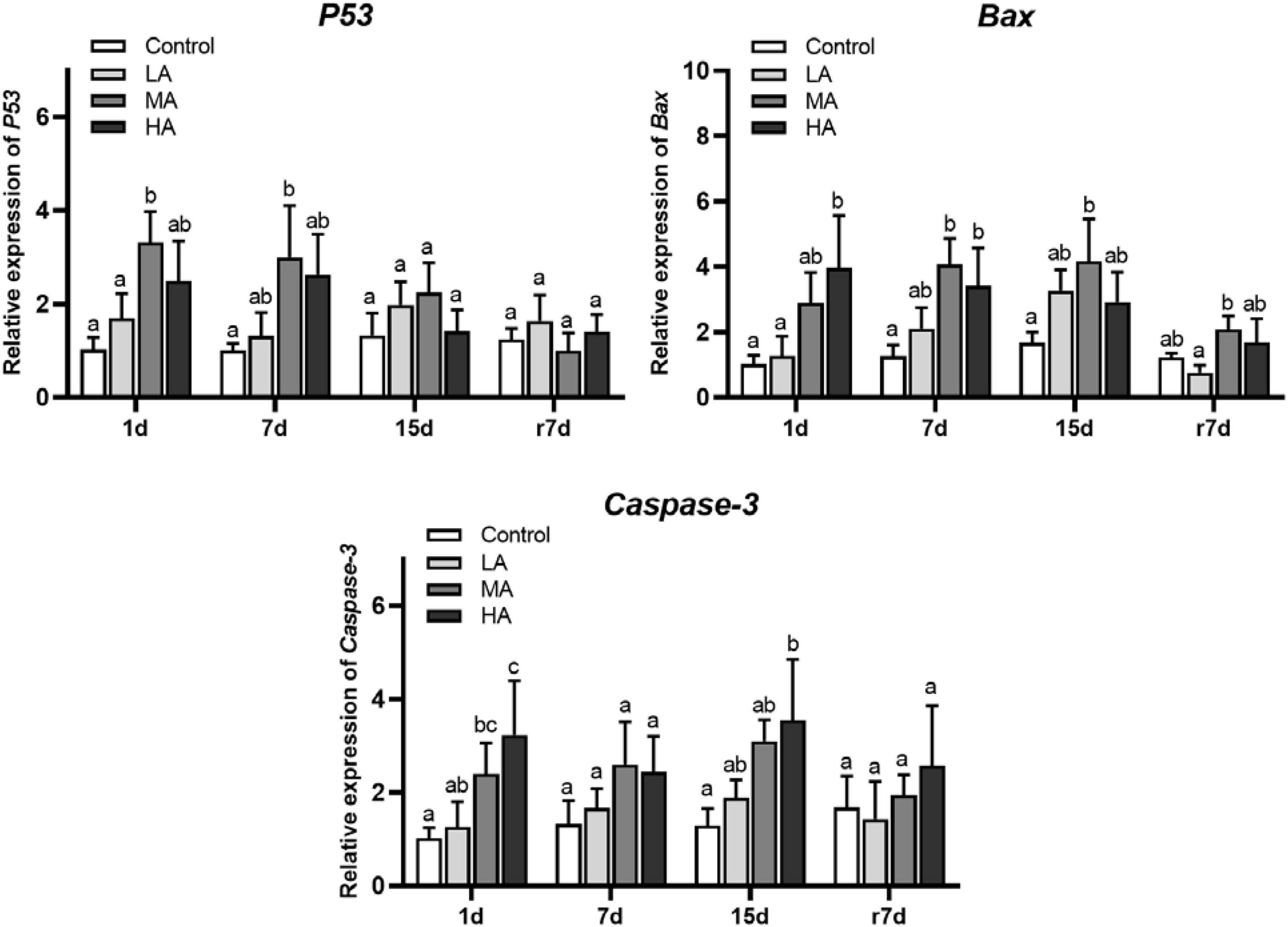
Figure 7. The mRNA expression of apoptotic pathway in hepatopancreas of P. trituberculatus after ammonia exposure and recovery. Data are represented as mean ± S.D., n = 3, with P < 0.05 considered significant. Different letters represent significant differences among different groups.
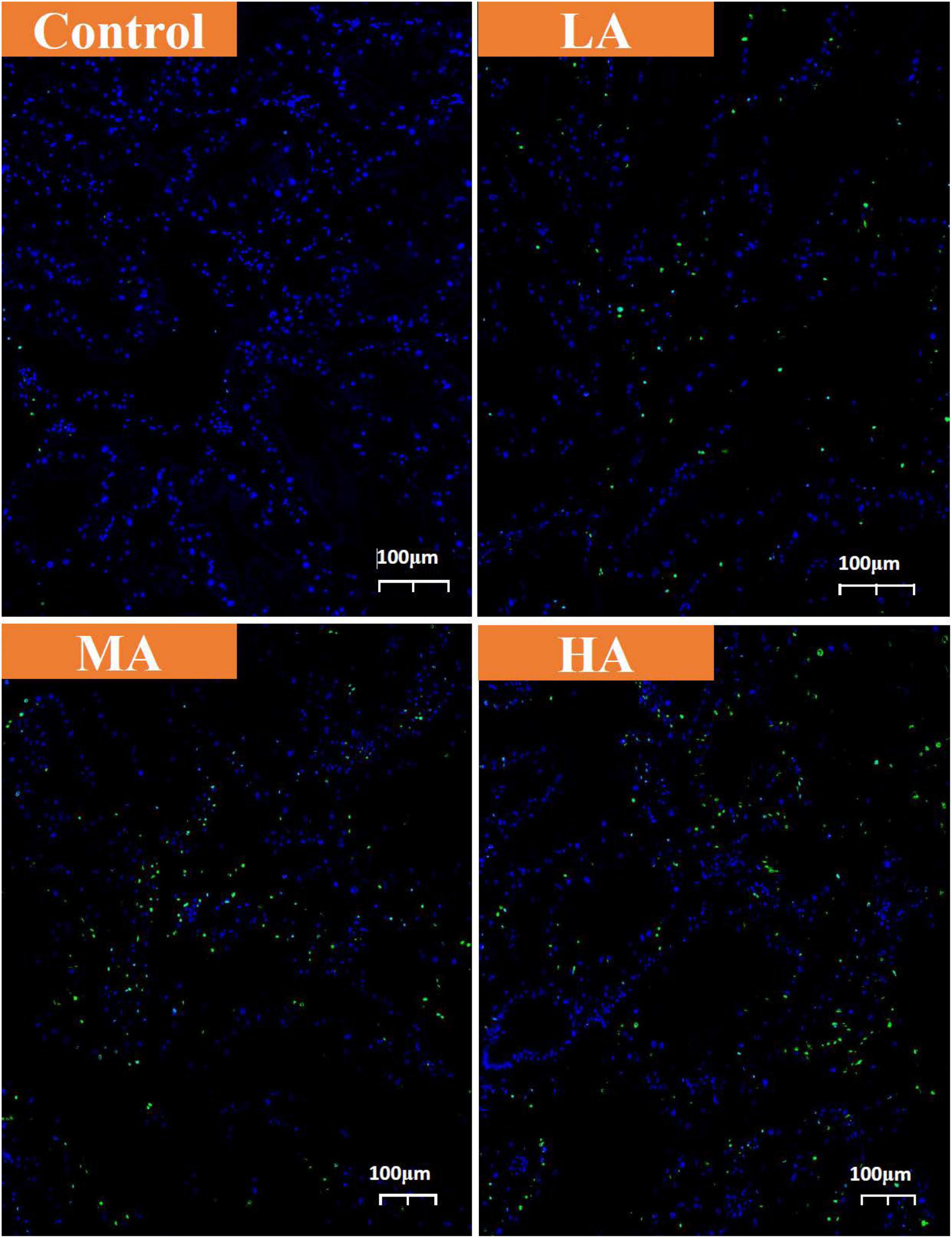
Figure 8. TUNEL assay of hepatopancreas from control, LA, MA, and HA groups. The apoptotic cells were detected by TUNEL (green) and the nuclei were detected by DAPI (blue).
Discussion
Long-term elevation of ammonia levels in aquaculture systems can often be experienced by crustaceans, which can result in massive mortality. However, there has been limited information regarding the toxic effects in crustaceans under prolonged ammonia stress. In this study, histological analysis showed that the relative area of tubule and lumen in hepatopancreas in all treatment groups were significantly decreased and increased, respectively, after 15 days of ammonia exposure. In addition, the hepatopancreatic cells in the MA (15 mg⋅L–1) and HA (45 mg⋅L–1) groups also exhibited disintegration, tumefaction, and apoptotic characteristics. These results clearly demonstrated that long-term HEA could cause hepatopancreatic damage in the swimming crab.
Cellular stress responses are crucial for organisms to maintain cellular homeostasis under environmental stress, including ammonia exposure (Swan and Sistonen, 2015; Kültz, 2020). Previous studies showed that HEA can cause over-generation of ROS and result in oxidative stress in aquatic animals (Cheng et al., 2019; Zhang et al., 2020; Zhao et al., 2020). The Keap1-Nrf2 pathway plays a critical role in alleviating oxidative stress via upregulating its downstream antioxidant enzymes. The activity of the Nrf2 transcription factor is primarily controlled by Keap1 which acts as a negative regulator of Nrf2 (Gañán-Gómez et al., 2013). In this study, the Keap1 expression was significantly downregulated in the MA and HA groups, accompanied by the upregulation of SOD and GPX observed after ammonia exposure for days 1 and 7, as well as after recovery. These results indicated that HEA at 15–45 mg⋅L–1 can induce oxidative stress and activate the Keap1-Nrf2 pathway in the hepatopancreas of the swimming crab. On day 15 after exposure, the expression of Keap1 remained low in the MA and HA groups, but the expression of antioxidant enzymes also exhibited downregulation, which suggested that long-term HEA at 15–45 mg⋅L–1 could influence the Keap1-Nrf2 signaling and impair antioxidant defense in P. trituberculatus. Interestingly, there was a discrepancy in changes in the activity and mRNA expression of GPX after ammonia exposure. The GPX activity in the treatment groups at most sampling points showed no significant difference with the control group, while the GPX expression was upregulated in the treatment groups after different exposure times. This discrepancy was also observed in a previous study, which may be attributed to decreased contents of glutathione, the substrate of GPX, after ammonia stress (Li and Qi, 2019).
Oxidative stress is usually coupled with ER stress, leading to the induction of HSR and UPR, which can protect proteins by facilitating protein folding or degrading unfolded and misfolded protein (Kalmar and Greensmith, 2009; Dandekar et al., 2015; Di Conza and Ho, 2020). Activation of UPR is mediated by several master regulators, such as IRE1, XBP1, and ATF6 (Dandekar et al., 2015; Di Conza and Ho, 2020). In this study, upregulation of IRE1, XBP1, and ATF6 was observed in the MA and HA groups at 1 and 7 days of post-exposure. Similarly, Hsp70 and Hsp90 were also upregulated after 1-day ammonia exposure. These results indicated that UPR and HSR were activated to relieve ammonia-induced ER stress and restore ER homeostasis after short-term exposure. Interestingly, the expression of the genes involved in HSR and UPR returned to the control level or was even lower than that of the control after the long-term stress, which implies that long-term ammonia stress could inhibit UPR and HSR, and may impact protein homeostasis in the swimming crab.
Environmental stress, including ammonia, can pose a considerable threat to genome integrity and lead to diverse DNA lesions, among which DNA double-strand breaks (DSB) are considered one of the most cytotoxic lesions (Khan and Ali, 2017; Zhang et al., 2020). After sensing DSB, ATM, ATR, and DNA-PKcs recruitment to DSBs initiate the DSB signaling to repair DNA, which is known as DDR (Blackford and Jackson, 2017; Burger et al., 2019). The result of this study indicated that short-term ammonia exposure can activate DDR, as evidenced by the upregulation of ATR and DNA-PKcs. Similar to the genes involved in antioxidant defense, UPR, and HSR, the expression of ATR and DNA-PKcs was also reduced to the control level or lower than that of the control level. Taking those results together, we may speculate that long-term ammonia exposure over 15 mg⋅L–1 can compromise CSR, including antioxidant defense, UPR, HSR, and DDR, in the hepatopancreas of P. trituberculatus.
It has been demonstrated that oxidative stress, ER stress, and DNA damage can interfere with cell homeostasis and activate proapoptotic signaling (Chandra et al., 2000; Tabas and Ron, 2011). Previous studies have shown ammonia-induced apoptosis in aquatic animals (Cheng et al., 2015, 2019; Liang et al., 2016; Zhang et al., 2020). This was also observed in our study when the crabs were exposed to HEA of 15–45 mg⋅L–1, as indicated by the TUNEL assay. Recent studies in aquatic animals reported that ammonia induced apoptosis in the hepatopancreas via the p53-Bax signaling pathway (Cheng et al., 2015; Jia et al., 2015). In the pathway, activation of p53 upregulates proapoptotic genes, including the Bax, which causes the activation of caspases and eventually apoptosis (Schuler and Green, 2001). For the MA and HA groups, the expression of p53, Bax, and caspase-3, the executioner of apoptosis, were significantly upregulated. We, thus, inferred that the p53-Bax-mediated caspase-dependent apoptotic pathway was involved in the HEA-induced apoptosis in the swimming crab.
In summary, the ammonia level exceeding 15 mg⋅L–1 is harmful to the swimming crab P. trituberculatus. Long-term ammonia exposure compromised CSR and induced apoptosis and tissue damage in the hepatopancreas. Even recovery of 7 days could not eliminate the detrimental effects of the long-term ammonia exposure at 15–45 mg⋅L–1. This might be an important reason why crabs cannot withstand HEA for a long time.
Data Availability Statement
The original contributions presented in the study are included in the article/Supplementary Material, further inquiries can be directed to the corresponding author.
Author Contributions
YL and XM contributed to the conceptualization of the manuscript and funding acquisition. JZ and JC contributed to the methodology and investigation. YL, XM, JZ, and JC contributed to the software, validation, formal analysis, data curation, and original draft. YL, JZ, and XM contributed to the writing, review, and editing. PL and JL contributed to the resources, supervision, and project administration. All authors contributed to the article and approved the submitted version.
Funding
This research was funded by the National Natural Science Foundation of China (41976106 and 31972784), the National Key Research and Development Plan (2019YFD0900402), High-Level Talents Research Fund of Qingdao Agricultural University (No. 663/1119032), China Agriculture Research System of MOF and MARA, and “First Class Fishery Discipline Programme” in Shandong Province, China, Tianjin Natural Science Foundation Project (18JCQNJC14800).
Conflict of Interest
The authors declare that the research was conducted in the absence of any commercial or financial relationships that could be construed as a potential conflict of interest.
Publisher’s Note
All claims expressed in this article are solely those of the authors and do not necessarily represent those of their affiliated organizations, or those of the publisher, the editors and the reviewers. Any product that may be evaluated in this article, or claim that may be made by its manufacturer, is not guaranteed or endorsed by the publisher.
Acknowledgments
The authors thank Xinyuan Cheng, Jiyan He, Youyan Wang, Jinghao Xu, Xitao Guan, and Heng Tang for their excellent assistance during the experiment.
Supplementary Material
The Supplementary Material for this article can be found online at: https://www.frontiersin.org/articles/10.3389/fmars.2021.757602/full#supplementary-material
References
Bandara, A. B., Drake, J. C., James, C. C., Smyth, J. W., and Brown, D. A. (2021). Complex I protein NDUFS2 is vital for growth, ROS generation, membrane integrity, apoptosis, and mitochondrial energetics. Mitochondrion 58, 160–168. doi: 10.1016/j.mito.2021.03.003
Barbieri, E. (2010). Acute toxicity of ammonia in white shrimp (litopenaeus schmitti) (burkenroad, 1936, crustacea) at different salinity levels. Aquaculture 306, 329–333. doi: 10.1016/j.aquaculture.2010.06.009
Blackford, A. N., and Jackson, S. P. (2017). ATM, ATR, and DNA-PK: the trinity at the heart of the DNA damage response. Mol. Cell 66, 801–817. doi: 10.1016/j.molcel.2017.05.015
Burger, K., Ketley, R. F., and Gullerova, M. (2019). Beyond the trinity of ATM, ATR, and DNA-PK: multiple kinases shape the DNA damage response in concert with RNA metabolism. Front. Mol. Biosci. 6:61. doi: 10.3389/fmolb.2019.00061
Cao, S., Zhao, D., Huang, R., Xiao, Y., Xu, W., Liu, X., et al. (2021). The influence of acute ammonia stress on intestinal oxidative stress, histology, digestive enzymatic activities and PepT1 activity of grass carp (ctenopharyngodon idella). Aquac. Rep. 20:100722. doi: 10.1016/j.aqrep.2021.100722
Chandra, J., Samali, A., and Orrenius, S. (2000). Triggering and modulation of apoptosis by oxidative stress. Free Radical Biol. Med. 29, 323–333.
Cheng, C. H., Ma, H. L., Su, Y. L., Deng, Y. Q., Feng, J., Xie, J. W., et al. (2019). Ammonia toxicity in the mud crab (scylla paramamosain): the mechanistic insight from physiology to transcriptome analysis. Ecotoxicol. Environ. Safety 179, 9–16. doi: 10.1016/j.ecoenv.2019.04.033
Cheng, C. H., Yang, F. F., Ling, R. Z., Liao, S. A., Miao, Y. T., Ye, C. X., et al. (2015). Effects of ammonia exposure on apoptosis, oxidative stress and immune response in pufferfish (takifugu obscurus). Aquatic Toxicol. 164, 61–71. doi: 10.1016/j.aquatox.2015.04.004
Cui, Z., Liu, Y., Luan, W., Li, Q., Wu, D., and Wang, S. J. F. (2010). Molecular cloning and characterization of a heat shock protein 70 gene in swimming crab (portunus trituberculatus). Fish Shellfish Immunol. 28, 56–64. doi: 10.1016/j.fsi.2009.09.018
Dandekar, A., Mendez, R., and Zhang, K. (2015). “Cross talk between er stress, oxidative stress, and inflammation in health and disease,” in Stress Responses: Methods and Protocols, ed. C. M. Oslowski (New York: Springer), 205–214. doi: 10.1007/978-1-4939-2522-3_15
Di Conza, G., and Ho, P. C. (2020). ER stress responses: an emerging modulator for innate immunity. Cells 9:695. doi: 10.3390/cells9030695
Eimon, P. M., and Ashkenazi, A. (2010). The zebrafish as a model organism for the study of apoptosis. Apoptosis 15, 331–349. doi: 10.1007/s10495-009-0432-9
González-Quiroz, M., Blondel, A., Sagredo, A., Hetz, C., Chevet, E., and Pedeux, R. (2020). When endoplasmic reticulum proteostasis meets the dna damage response. Trends Cell Biol. 30, 881–891. doi: 10.1016/j.tcb.2020.09.002
Huang, X., Feng, Y., Duan, J., Xiong, G., Fan, W., Liu, S., et al. (2020). Antistarvation strategies of E. sinensis: regulatory networks under hepatopancreas consumption. Oxidative Med. Cell. Long. 2020:6085343. doi: 10.1155/2020/6085343
Jia, R., Han, C., Lei, J. L., Liu, B. L., Huang, B., Huo, H. H., et al. (2015). Effects of nitrite exposure on haematological parameters, oxidative stress and apoptosis in juvenile turbot (scophthalmus maximus). Aquatic Toxicol. 169, 1–9. doi: 10.1016/j.aquatox.2015.09.016
Kalmar, B., and Greensmith, L. (2009). Induction of heat shock proteins for protection against oxidative stress. Adv. Drug Delivery Rev. 61, 310–318. doi: 10.1016/j.addr.2009.02.003
Khan, F. A., and Ali, S. O. (2017). Physiological roles of DNA double-strand breaks. J. Nucleic. Acid. 2017:6439169.
Kim, J. H., Park, H. J., Hwang, I. K., Han, J. M., Kim, D. H., Oh, C. W., et al. (2020). Toxic effects of juvenile sablefish, anoplopoma fimbria by ammonia exposure at different water temperature. Environ. Toxicol. Pharmacol. 54, 169–176. doi: 10.1016/j.etap.2017.07.008
Kopacz, A., Kloska, D., Forman, H. J., Jozkowicz, A., and Grochot-Przeczek, A. (2020). Beyond repression of Nrf2: an update on Keap1. Free Radical Biol. Med. 157, 63–74. doi: 10.1016/j.freeradbiomed.2020.03.023
Kültz, D. (2005). Molecular and evolutionary basis of the cellular stress response. Ann. Rev. Physiol. 67, 225–257. doi: 10.1146/annurev.physiol.67.040403.103635
Kültz, D. (2020). Evolution of cellular stress response mechanisms. J. Exp. Zool. Part A Ecol. Integrat. Physiol. 333, 359–378. doi: 10.1002/jez.2347
Li, E., Chen, L., Zeng, C., Yu, N., Xiong, Z., Chen, X., et al. (2008). Comparison of digestive and antioxidant enzymes activities, haemolymph oxyhemocyanin contents and hepatopancreas histology of white shrimp, litopenaeus vannamei, at various salinities. Aquaculture 274, 80–86. doi: 10.1016/j.aquaculture.2007.11.001
Li, L., and Qi, H. (2019). Effect of acute ammonia exposure on the glutathione redox system in FFRC strain common carp (cyprinus carpio L.). Environ. Sci. Pollut. Res. 26, 27023–27031. doi: 10.1007/s11356-019-05895-4
Liang, Z., Liu, R., Zhao, D., Wang, L., Sun, M., Wang, M., et al. (2016). Ammonia exposure induces oxidative stress, endoplasmic reticulum stress and apoptosis in hepatopancreas of pacific white shrimp (litopenaeus vannamei). Fish Shellfish Immunol. 54, 523–528. doi: 10.1016/j.fsi.2016.05.009
Lu, Y., Wu, Z., Song, Z., Xiao, P., Liu, Y., Zhang, P., et al. (2016). Insight into the heat resistance of fish via blood: effects of heat stress on metabolism, oxidative stress and antioxidant response of olive flounder paralichthys olivaceus and turbot scophthalmus maximus. Fish Shellfish Immunol. 58, 125–135. doi: 10.1016/j.fsi.2016.09.008
Meng, X. L., Liu, P., Li, J., Gao, B. Q., and Chen, P. J. A. (2014). Physiological responses of swimming crab portunus trituberculatus under cold acclimation: antioxidant defense and heat shock proteins. Aquaculture 434, 11–17. doi: 10.1016/j.aquaculture.2014.07.021
Meng, X., Liu, P., Jia, F., Jian, L., and Gao, B. Q. (2015). De novo transcriptome analysis of Portunus trituberculatus ovary and testis by rna-seq: identification of genes involved in gonadal development. PLoS One 10:e0133659. doi: 10.1371/journal.pone.0133659
Meng, X., Jayasundara, N., Zhang, J., Ren, X., Gao, B., Li, J., et al. (2021). Integrated physiological, transcriptome and metabolome analyses of the hepatopancreas of the female swimming crab Portunus trituberculatus under ammonia exposure. Ecotoxicol. Environ. Safety. 228:113026. doi: 10.1016/j.ecoenv.2021.113026
Meng, X., Zhang, M., Gao, B., Lv, J., and Liu, P. (2020). Integrative proteomic and microrna analysis: insights into mechanisms of eyestalk ablation-induced ovarian maturation in the swimming crab Portunus trituberculatus. Front. Endocrinol. 11:533. doi: 10.3389/fendo.2020.00533
Pan, L., Si, L., Liu, S., Liu, M., and Wang, G. (2018). Levels of metabolic enzymes and nitrogenous compounds in the swimming crab Portunus trituberculatus exposed to elevated ambient ammonia-N. J. Ocean Univ. China 17, 957–966. doi: 10.1007/s11802-018-3574-y
Ren, X., Yu, X., Gao, B., Li, J., and Liu, P. (2016). The immune responses and antioxidant status of portunus trituberculatus individuals with different body weights. Fish Shellfish Immunol. 51, 337–345. doi: 10.1016/j.fsi.2016.03.004
Romano, N., and Zeng, C. (2013). Toxic effects of ammonia, nitrite, and nitrate to decapod crustaceans: a review on factors influencing their toxicity, physiological consequences, and coping mechanisms. Rev. Fisher. Sci. 21, 1–21. doi: 10.1080/10641262.2012.753404
Şahin, E., and Gümüşlü, S. (2004). Alterations in brain antioxidant status, protein oxidation and lipid peroxidation in response to different stress models. Behav. Brain Res. 155, 241–248. doi: 10.1016/j.bbr.2004.04.022
Schuler, M., and Green, D. R. (2001). Mechanisms of p53-dependent apoptosis. Biochem. Soc. Trans. 29, 684–688.
Si, L., Pan, L., Wang, H., and Zhang, X. (2020). Transcriptomic response to ammonia-N stress in the hepatopancreas of swimming crab Portunus trituberculatus. Mar. Life Sci. Technol. 2, 135–145. doi: 10.1007/s42995-020-00033-3
Sies, H., and Jones, D. P. (2020). Reactive oxygen species (ROS) as pleiotropic physiological signalling agents. Nat. Rev. Mol. Cell Biol. 21, 363–383. doi: 10.1038/s41580-020-0230-3
Somero, G. N. (2020). The cellular stress response and temperature: function, regulation, and evolution. J. Exp. Zool. Part A Ecol. Integrat. Physiol. 333, 379–397. doi: 10.1002/jez.2344
Swan, C. L., and Sistonen, L. (2015). Cellular stress response cross talk maintains protein and energy homeostasis. EMBO. J. 34, 267–269. doi: 10.15252/embj.201490757
Tabas, I., and Ron, D. (2011). Integrating the mechanisms of apoptosis induced by endoplasmic reticulum stress. Nat. Cell Biol. 13, 184–190. doi: 10.1038/ncb0311-184
Wang, H. J., Xiao, X. C., Wang, H. Z., Li, Y., Yu, Q., Liang, X. M., et al. (2017). Effects of high ammonia concentrations on three cyprinid fish: acute and whole-ecosystem chronic tests. Sci. Total Environ. 598, 900–909. doi: 10.1016/j.scitotenv.2017.04.070
Yan, X., Chen, Y., Dong, X., Tan, B., Liu, H., Zhang, S., et al. (2021). Ammonia toxicity induces oxidative stress, inflammatory response and apoptosis in hybrid grouper (♀ epinephelus fuscoguttatus× ♂ E. lanceolatu). Front. Mar. Sci. 8:390.
Yu, Z., Quan, Y. N., Huang, Z. Q., Wang, H. H., and Wu, L. F. (2020). Monitoring oxidative stress, immune response, Nrf2/NF-κB signaling molecules of rhynchocypris lagowski living in BFT system and exposed to waterborne ammonia. Ecotoxicol. Environ. Safety 205:111161. doi: 10.1016/j.ecoenv.2020.111161
Yue, F., Pan, L., Xie, P., Zheng, D., and Li, J. (2010). Immune responses and expression of immune-related genes in swimming crab portunus trituberculatus exposed to elevated ambient ammonia-N stress. Comparative Biochem. Physiol. Part A Mol. Integrat. Physiol. 157, 246–251. doi: 10.1016/j.cbpa.2010.07.013
Zhang, J., Zhang, M., Jayasundara, N., Ren, X., Gao, B., Liu, P., et al. (2021). Physiological and molecular responses in the gill of the swimming crab portunus trituberculatus during long-term ammonia stress. Front. Mar. Sci. 8:797241. doi: 10.3389/fmars.2021.797241
Zhang, T., Yan, Z., Zheng, X., Wang, S., Fan, J., and Liu, Z. (2020). Effects of acute ammonia toxicity on oxidative stress, DNA damage and apoptosis in digestive gland and gill of Asian clam (corbicula fluminea). Fish Shellfish Immunol. 99, 514–525. doi: 10.1016/j.fsi.2020.02.046
Zhang, W., Jiang, Q., Liu, X., Pan, D., Yang, Y., and Yang, J. (2015). The effects of acute ammonia exposure on the immune response of juvenile freshwater prawn, macrobrachium nipponense. J. Crustacean Biol. 35, 76–80. doi: 10.1163/1937240x-00002292
Zhang, X. Y., Zhang, M. Z., Zheng, C. J., Liu, J., and Hu, H. (2009). Identification of two hsp90 genes from the marine crab, portunus trituberculatus and their specific expression profiles under different environmental conditions. Comparative Biochem. Physiol. Part C Toxicol. Pharmacol. 150, 465–473. doi: 10.1016/j.cbpc.2009.07.002
Zhao, M., Yao, D., Li, S., Zhang, Y., and Aweya, J. J. (2020). Effects of ammonia on shrimp physiology and immunity: a review. Rev. Aquac. 12, 2194–2211. doi: 10.1111/raq.12429
Keywords: crab, prolonged ammonia, unfolded protein response, DNA damage response, apoptosis, antioxidant response
Citation: Lu Y, Zhang J, Cao J, Liu P, Li J and Meng X (2022) Long-Term Ammonia Toxicity in the Hepatopancreas of Swimming Crab Portunus trituberculatus: Cellular Stress Response and Tissue Damage. Front. Mar. Sci. 8:757602. doi: 10.3389/fmars.2021.757602
Received: 13 August 2021; Accepted: 27 December 2021;
Published: 28 January 2022.
Edited by:
Jiang-Shiou Hwang, National Taiwan Ocean University, TaiwanReviewed by:
Edison Barbieri, Instituto de Pesca, Agência de Agronegócio e Tecnologia de São Paulo (APTA), BrazilGabriela Verónica Aguirre-Martínez, Arturo Prat University, Chile
Copyright © 2022 Lu, Zhang, Cao, Liu, Li and Meng. This is an open-access article distributed under the terms of the Creative Commons Attribution License (CC BY). The use, distribution or reproduction in other forums is permitted, provided the original author(s) and the copyright owner(s) are credited and that the original publication in this journal is cited, in accordance with accepted academic practice. No use, distribution or reproduction is permitted which does not comply with these terms.
*Correspondence: Xianliang Meng, eGxtZW5nQHlzZnJpLmFjLmNu