- 1Department of Geography, University of California, Los Angeles, Los Angeles, CA, United States
- 2Department of Applied Ocean Physics and Engineering, Woods Hole Oceanographic Institution, Woods Hole, MA, United States
- 3Department of Geography, University of Victoria, Victoria, BC, Canada
- 4The Nature Conservancy, Sacramento, CA, United States
- 5Hakai Institute, Heriot Bay, BC, Canada
- 6Institute of the Oceans and Fisheries, University of British Columbia, Vancouver, BC, Canada
- 7Ministry of Forests, Lands, Natural Resource Operations and Rural Development, Victoria, BC, Canada
- 8Department of Ocean Sciences, University of California, Santa Cruz, Santa Cruz, CA, United States
- 9Universidad Autónoma de Baja California, Facultad de Ciencias Marinas, Baja California, Mexico
- 10Department of Ecology and Evolutionary Biology, University of California, Santa Cruz, Santa Cruz, CA, United States
- 11Marine Science Institute, University of California, Santa Barbara, Santa Barbara, CA, United States
- 12California Department of Fish and Wildlife, Sacramento, CA, United States
- 13Department of Integrative Biology, Oregon State University, Corvallis, OR, United States
- 14Cooperative Institute for Marine and Atmospheric Studies, University of Miami, Miami, FL, United States
- 15National Oceanic and Atmospheric Administration (NOAA), Atlantic Oceanographic and Meteorological Laboratory, Ocean Chemistry and Ecosystem Division, Miami, FL, United States
- 16Kelp Recovery Program, Greater Farallones National Marine Sanctuary, Greater Farallones Association, San Francisco, CA, United States
- 17Gwaii Haanas National Park Reserve, National Marine Conservation Area Reserve, and Haida Heritage Site, Parks Canada, Skidegate, BC, Canada
- 18Tijuana River National Estuarine Research Reserve, Imperial Beach, CA, United States
- 19Facultad de Ciencias, Universidad Autónoma de Baja California, Baja California, Mexico
Surface-canopy forming kelps provide the foundation for ecosystems that are ecologically, culturally, and economically important. However, these kelp forests are naturally dynamic systems that are also threatened by a range of global and local pressures. As a result, there is a need for tools that enable managers to reliably track changes in their distribution, abundance, and health in a timely manner. Remote sensing data availability has increased dramatically in recent years and this data represents a valuable tool for monitoring surface-canopy forming kelps. However, the choice of remote sensing data and analytic approach must be properly matched to management objectives and tailored to the physical and biological characteristics of the region of interest. This review identifies remote sensing datasets and analyses best suited to address different management needs and environmental settings using case studies from the west coast of North America. We highlight the importance of integrating different datasets and approaches to facilitate comparisons across regions and promote coordination of management strategies.
Introduction
Kelp forests occur on shallow subtidal rocky reefs in temperate seas around the world and provide the foundation for some of the most productive and valuable coastal marine ecosystems. Some of the most iconic species of kelp, e.g., giant kelp, form large canopies that float on the surface of the ocean. Functionally, these large kelps act as “foundation species” (sensu Dayton, 1972) by providing both primary production and three-dimensional habitat to a diverse array of ecologically, culturally, and economically important species of algae, invertebrates, fish, birds, and marine mammals (Schiel and Foster, 2015; Carr and Reed, 2016). They also act as ecosystem engineers, altering environmental conditions such as light, currents, substrate availability, structural complexity, and seawater chemistry (Jones et al., 1994; Pfister et al., 2019; Hirsh et al., 2020). Kelp itself also has a great deal of cultural and economic value. It is harvested for a variety of purposes including food, cosmetics, and industrial uses (Schiel and Foster, 2015), and has an important role in traditional subsistence usage as well as symbolic and spiritual aspects of indigenous cultural systems (Turner, 2001; Calloway et al., 2020). Collectively, ecosystem services of kelp forests have been valued at $684 billion/year (Eger et al., 2021).
As fast-growing primary producers, kelp forests exhibit natural dynamics in abundance over space and time. Kelp forests are also particularly sensitive to changes in environmental conditions and face a variety of threats that span local to global scales (Beas-Luna et al., 2020). Increases in the frequency and intensity of marine heatwaves have been linked to kelp range contractions around the world (Arafeh-Dalmau et al., 2020; Smale, 2020; McPherson et al., 2021; Oliver et al., 2021). Overgrazing by consumers such as sea urchins has caused some forests to transition to barren states denuded of kelp (Estes and Palmisano, 1974; Ebeling et al., 1985; Ling et al., 2009; Rogers-Bennett and Catton, 2019; Smith et al., 2021; McPherson et al., 2021). Furthermore, because of their close proximity to shore, kelp forest ecosystems face local threats such as point-source pollution and sedimentation from coastal development (Foster and Schiel, 2010). Subsequent declines in kelp abundance have cascading negative impacts to the ecosystems and the communities they support (e.g., Dudley et al., 2021).
In the face of these threats, there is an increasing need to develop strategies to protect and restore kelp ecosystems (Eger et al., 2020b). For example, local pressures can be mitigated by strategies such as actively reducing grazing pressure, reintroducing kelp populations via seeding, transplanting, and artificial reefs, implementing marine protected areas, managing kelp harvest, and regulating coastal development (Eger et al., 2020a; Eisaguirre et al., 2020; Layton et al., 2020; Morris et al., 2020). To effectively implement these strategies, managers and conservation practitioners require data on how the distribution, abundance, and health of kelp populations are changing over a variety of temporal and spatial scales. These data can lead to a better understanding of the environmental factors and ecological processes that contribute to kelp forest persistence and resilience (e.g., Young et al., 2016), guide the timing and distribution of active interventions where kelp has been lost (Gleason et al., 2021), and evaluate the effectiveness of management actions.
Long-term data over large scales provide disproportionate value for management and policy (Hughes et al., 2017). Long-term data is particularly important for kelp monitoring because of their high levels of natural background variability in abundance (Bell et al., 2020a). However, subtidal in situ sampling traditionally used to monitor kelp forests is often impractical due to cost and time constraints. Remote sensing provides a valuable tool to meet these needs. Kelps that form large floating canopies are relatively easy to distinguish with airborne and satellite imagery, particularly with multispectral imagery, demonstrated by a long history of using remote sensing data to document changes in canopy area and biomass (Jensen et al., 1980; Cavanaugh et al., 2011; Schroeder et al., 2019; Bell et al., 2020a) and estimate harvestable amounts of kelp (Stekoll et al., 2006; Reed, 2014). Availability of both airborne and spaceborne remote sensing data has increased over the past decade, and scientists now have access to datasets that span a range of spatial, temporal, and spectral coverage and resolutions.
Here, we review the benefits and constraints associated with using remote sensing data to inform kelp forest management, using case studies from the west coast of North America. We describe the physical and biological characteristics that affect remote monitoring of surface canopy-forming kelps in this region and identify sensor characteristics that can help address challenges particular to certain parts of the region. Specific management applications are linked to the datasets best suited to address them. The west coast of North America provides an ideal area for examining these issues due to the wide range of kelp forest management needs faced by this region and the temporal and spatial variability in important physical and biological factors that influence remote sensing of kelp.
Processes That Influence Variability in Kelp Abundance
Giant kelp (Macrocystis pyrifera) and bull kelp (Nereocystis luetkeana), the two primary species of large surface canopy-forming kelps along west coast of North America, exhibit pronounced spatiotemporal variability driven by many interacting abiotic and biotic factors that influence kelp reproduction, growth, and survival. A full review of these factors is outside of the scope of this paper, but see Graham et al. (2007); Springer et al. (2010), Schiel and Foster (2015), and Carr and Reed (2016) for comprehensive reviews. Here, we focus on the anthropogenic activities and pressures that can lead to changes in kelp abundance across a range of scales (Supplementary Table 1). We follow definitions from the Driver-Activity-Pressure-State-Impact-Response (DAPSIR) framework, commonly used to develop management responses to observed changes in marine ecosystems that address human needs (Oesterwind et al., 2016; Elliott et al., 2017; Bryhn et al., 2020). Based on this framework, human activities and the resulting pressures cause changes in the distribution, abundance, and health of canopy-forming kelp, hereafter referred to as state changes. Note that the relative contributions of different pressures to kelp state changes is often unknown, and that interactions among different pressures may drive state changes (e.g., Rogers-Bennett and Catton, 2019). Data on spatiotemporal variability in kelp dynamics (e.g., from remote sensing data) can be used disentangle links between pressures and kelp state changes if the spatial and temporal resolution of the remote sensing data matches the spatial and temporal scales of the pressures and state changes.
Due to spatial variability in these pressures, trends in kelp abundance have varied substantially among regions along the west coast of North America over the last few decades, with declines in north-central California and the Aleutian Islands, small increases in the Southern California Bight, and no clear trends in other regions (Krumhansl et al., 2016; Beas-Luna et al., 2020). Major pressures include heatwaves linked to anthropogenic climate change, and processes that have resulted in an overabundance of kelp grazers such as sea urchins (e.g., sea star wasting disease, climatic variability in urchin recruitment, overfishing of other urchin predators, sea otter removals, etc., see bolded references in Supplementary Table 1).
Remote Sensing of Surface-Canopy Forming Kelps
Quantifying the area, density, and condition of surface canopy-forming kelps using optical remotely sensed imagery relies on reflectance characteristics of floating kelp. The most common methods for remote sensing of kelp canopy involve passive optical sensors with coverage in the visible and near infrared (NIR) portions of the electromagnetic spectrum (Jensen et al., 1980; Deysher, 1993; Schroeder et al., 2019). Like terrestrial vegetation, floating kelp tissue reflects a high proportion of the incident radiant flux in the NIR region of the electromagnetic spectrum, while seawater absorbs nearly all NIR light (Jensen et al., 1980). As a result, NIR imagery is useful for distinguishing emergent surface canopy, but is not well suited for detecting submerged kelp.
A variety of image analysis methods have been used to identify kelp canopy from visible and near infrared imagery, including manual classification, spectral indices, supervised and unsupervised classification, object based image analysis, and deep learning approaches (see Schroeder et al., 2019 for a review of these methods). For coarser satellite imagery, methods such as multiple endmember spectral unmixing can be used to estimate the fraction of each pixel covered by kelp canopy (Bell et al., 2020a; Hamilton et al., 2020), potentially leading to better agreement among estimates from imagery of different resolutions.
The reflectance of the kelp canopy is also affected by the concentration and ratios of photosynthetic pigments within the tissue. Pigments such as fucoxanthin and chlorophylls a and c absorb light in different regions of the visible spectrum and are associated with physiological processes such as primary production and senescence (Rodriguez et al., 2016; Bell et al., 2018). However, the spectral bands of most multispectral sensors are too wide to leverage these subtle changes in reflectance and assess variations in canopy pigment concentration. The narrow and contiguous spectral bands measured by hyperspectral sensors are better suited for estimating physiological properties such as Chl:C ratios (Bell et al., 2015) and tissue nitrogen content (Bell et al., 2020b).
Factors That Influence the Remote Sensing of Kelp Canopy
Numerous physical and biological factors influence the remote detection of kelp, and determine the sensor characteristics (i.e., spatial and spectral resolutions, temporal coverage, etc.) needed to accurately map kelp canopy cover in specific locations. Important factors for the west coast of North America include multiple species of surface-canopy forming kelps, complex bathymetry and coastline morphologies, sun glint, adjacency effects, shadowing (especially in areas of steep topography), tides, and oceanographic conditions such as currents, tides, wave exposure, and phytoplankton blooms (Table 1, Figure 1, and Supplementary Figures 1, 2).
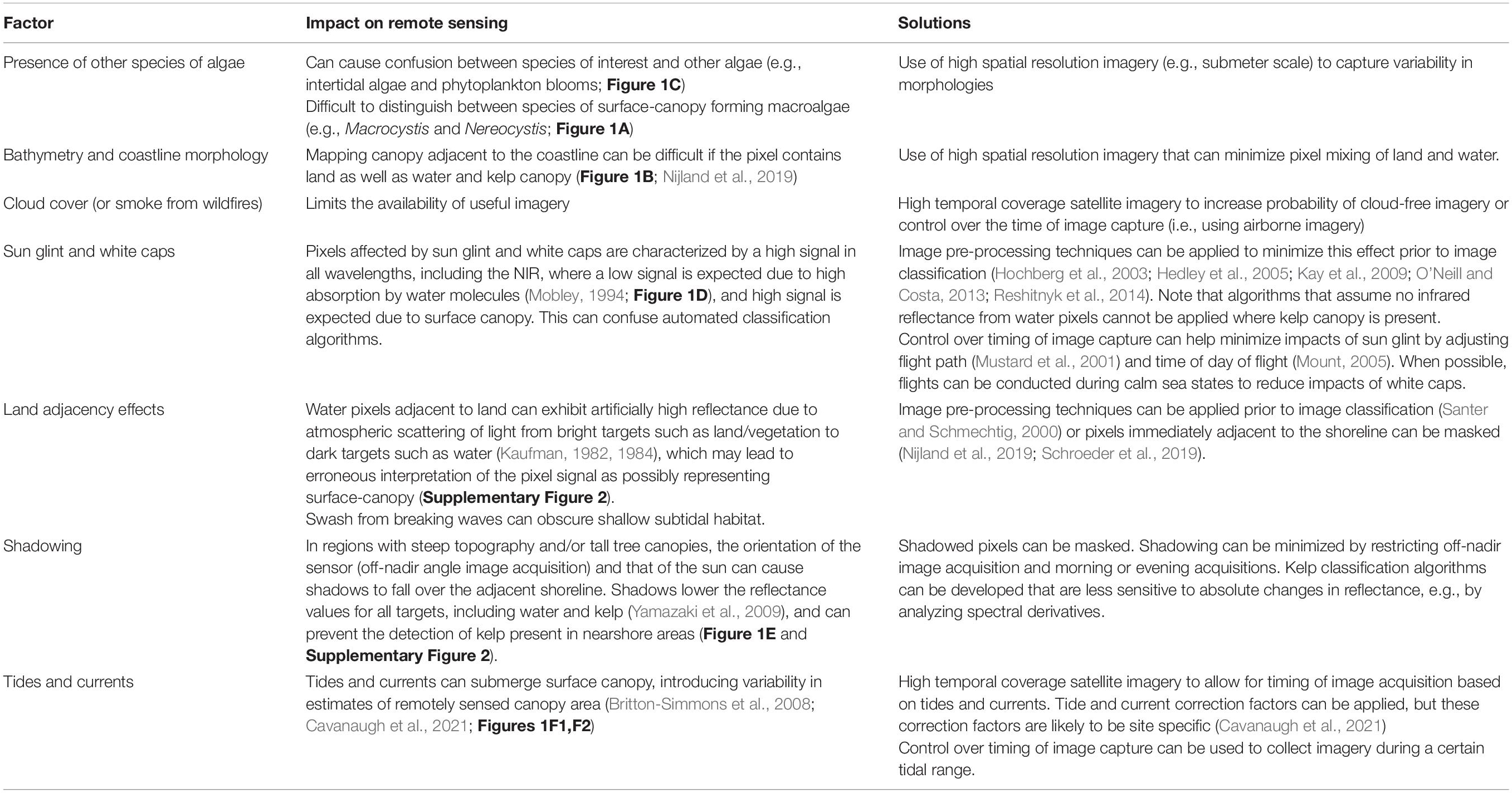
Table 1. Physical and biological factors that influence the ability to detect surface-canopy forming kelps using airborne and spaceborne imagery.
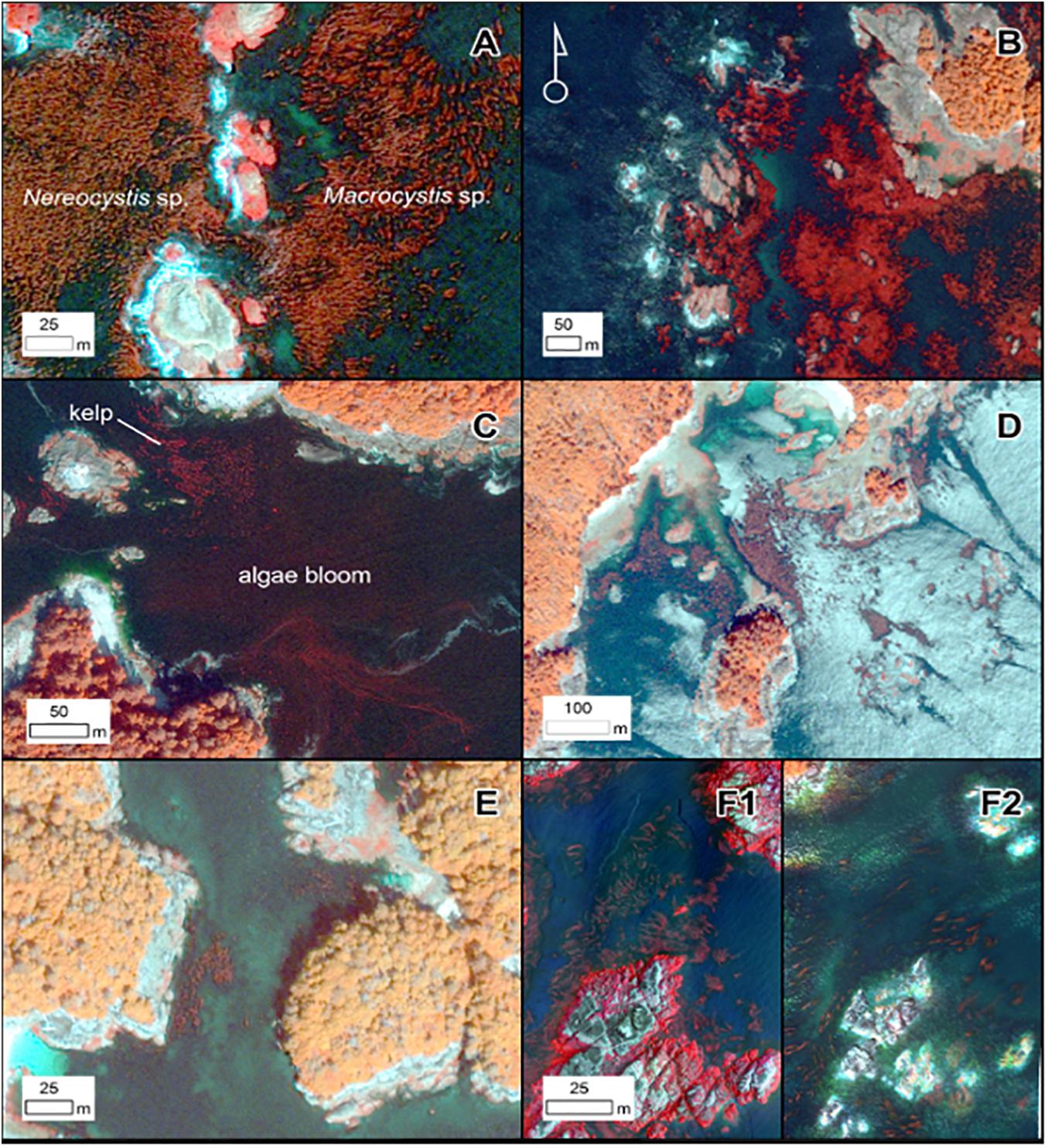
Figure 1. Pansharpened (0.5 m), false-color infrared World View-2 imagery depicting some of the factors described in Table 1. (A) Both Nereocystis luetkeana and Macrocystis pyrifera canopy are present. (B) Waves present in imagery (white areas on western side of island). (C) Algae bloom and kelp canopy present in the same regions. (D) Specular reflection present in imagery (white areas on water surface), obscuring the kelp bed. (E) Presence of shadow along the shoreline. (F) Drone imagery of the same site at low tide (F1) and high tide (F2). WorldView-2 and drone imagery provided by the Hakai Institute.
This review focuses on large surface canopy-forming kelps, as these are the most amenable to monitoring using a variety of remotely sensed datasets. Similar methods have been used to map subtidal kelps without a surface canopy and intertidal kelps, but these applications are limited by impacts of the water column and land. Absorption by the water column greatly reduces reflectance of submerged plants and this effect is exacerbated by optically active water components such as suspended sediment and chlorophyll (Mobley, 1994). In some cases band ratios from the visible portion of the electromagnetic spectrum (which has higher water penetration than the near infrared region) have been applied to multispectral imagery (e.g., Casal et al., 2011). Studies have also used hyperspectral imagery to estimate optical properties of the water column and isolate benthic reflectance (Garcia et al., 2018; Vahtmäe et al., 2020). Such methods have been used to map submerged kelp down to about 6 –10 m, but maximum depth will vary widely depending on water clarity. Furthermore, distinguishing species or even functional types of subsurface macroalgae is typically very difficult, although Uhl et al. (2016) demonstrated success separating Fucales from kelps in shallow water. For intertidal kelp, the main challenges are associated with the narrowness of the habitat and the influence of land and breaking waves. High reflectance from land and breaking waves can overwhelm the kelp reflectance signal, limiting the usefulness of methods that attempt to estimate fractional coverage of coarse pixels. As a result, very high resolution imagery is typically needed to map intertidal kelps (Tait et al., 2019; Rossiter et al., 2020).
Geography of Challenges
Many of the challenges to remote sensing of kelp canopy increase in severity from south to north along the west coast of North America, due to more cloud cover, higher amplitude tides and currents, more complex topography, steeper bathymetry, greater turbidity, and lower sun angles, which can exacerbate shadowing along the coast (Figure 2). In addition, mixed beds of giant and fringing bull kelp, which require higher spatial resolution to detect, are more common in central California and protected waters of Canada and Alaska (Figure 2). All regions experience issues related to sun glint, local waves and white caps during windy conditions, localized currents, algal blooms, and turbidity.
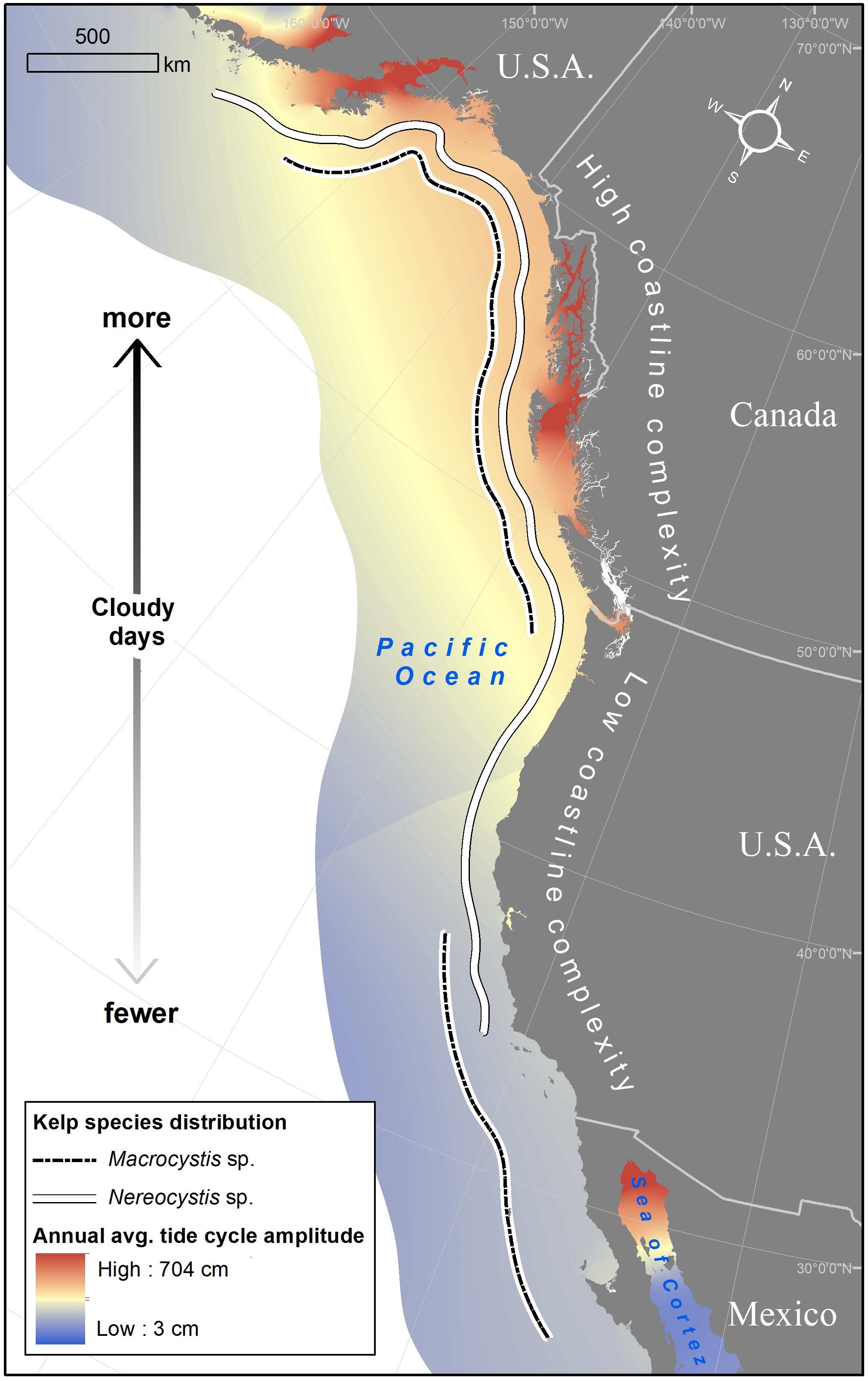
Figure 2. The spatial distribution of factors which contribute to challenging conditions for the remote sensing of canopy-forming kelp on the west coast of North America. Tidal data from Vestbo et al., 2018.
Remote Sensing Platforms for Kelp Monitoring
Remote sensing platforms can broadly be separated into three categories: satellite, occupied airborne, and unoccupied airborne systems (UAS) (Table 2, see Schroeder et al., 2019 for a comprehensive review of imagery and data processing methods for mapping kelp). Satellite multispectral sensors can monitor kelp canopy dynamics across extensive areas but vary in their temporal coverage and pixel resolution. Pixel resolution of imagery used to map kelp canopy varies from sub-meter to ∼100 m. Revisit time for satellite systems varies from days to weeks and temporal coverage ranges from years to decades (Table 2). Note that sensor degradation can occur with time, and so radiometric calibration in needed to improve long-term consistency (Chander et al., 2007). Also, calibration among multiple sensors is necessary for programs that use multiple sensors to improve temporal coverage (e.g., Landsat and Planet; Table 2; Chander et al., 2009). Some government satellite programs such as Landsat (NASA/USGS) and Sentinel-2 (European Space Agency) make their imagery freely available, while higher resolution commercial imagery (e.g., WorldView-2 and -3, Planet) requires licensing. The price and availability of commercial data can vary due to a range of market forces, potentially impacting long-term monitoring. Occupied airborne platforms can provide high resolution images, ∼0.25–3.0 m, depending on the sensor and flight altitude, and give the user control over the timing of data acquisition, which can address many of the challenges associated with remote sensing of kelp canopy (e.g., sun glint, tides, cloud cover, sea conditions; Table 1). However, aerial imagery can have relatively high operational costs. The ultimate cost of satellite vs. airborne imagery will depend on several factors including the area, spatial resolution, and temporal coverage required. Small UAS are becoming more accessible and affordable, providing scientists and managers with the flexibility to collect their own cm-scale imagery. These smaller, relatively inexpensive systems are also more amenable to crowd sourced citizen science programs. A variety of sensors can be mounted on UAS; visible and NIR multispectral cameras are most commonly used for kelp and macroalgal mapping purposes (Murfitt et al., 2017; Tait et al., 2019; Cavanaugh et al., 2021). The area covered in a single flight is dependent on the platform, altitude, and flight conditions, but is typically on the scale of 0.1–1 km2 (10s–100s of hectares). The time required to conduct UAS surveys and process resulting imagery limits the spatial coverage of these data. As with occupied aerial imagery, one benefit of UAS is the ability to determine the timing of flights, which allows users to plan around weather, tides (Cavanaugh et al., 2021), and ocean conditions, and respond quickly to events such as large wave disturbances and sewage and oil spills.
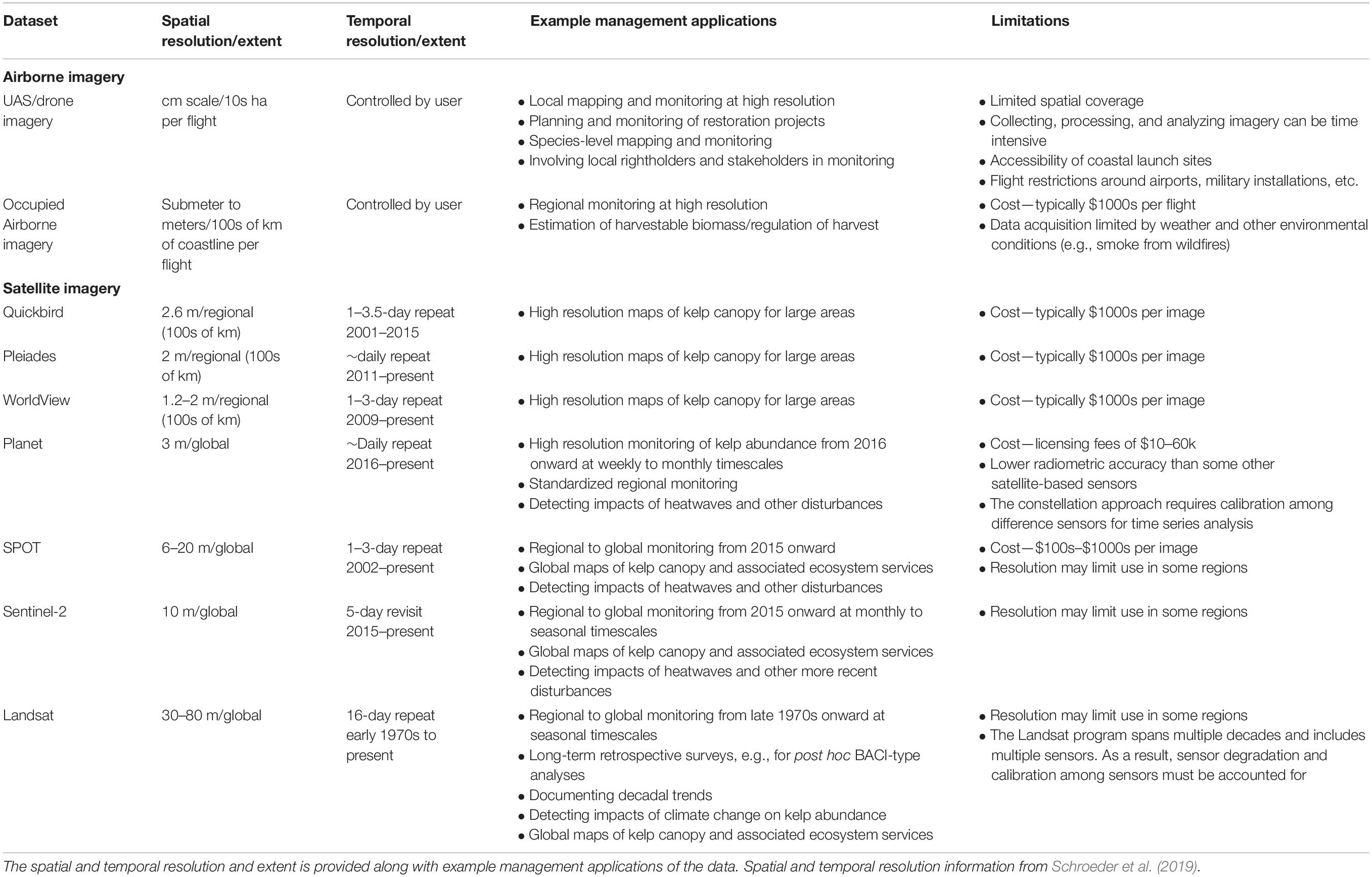
Table 2. List of remote sensing data that has been used for monitoring surface canopy-forming kelps.
Validation and Uncertainty Estimation
As with any application of remote sensing, rigorous ground truthing is required at multiple locations to validate kelp canopy features estimated over large areas from satellite or aerial imagery. Kelp canopy presence, areal extent, or species composition can be assessed using information gathered from a boat or kayak (Schroeder et al., 2019) or small UAS (Burt et al., 2018; Mora-Soto et al., 2020; Cavanaugh et al., 2021). Canopy biomass can be assessed by determining the relationship between the remote estimates of canopy density and field estimates of canopy biomass (Stekoll et al., 2006; Cavanaugh et al., 2011). Remotely sensed estimates of canopy physiology require the development of spectral algorithms established through the comparison of laboratory measured pigment concentration of kelp tissue with field or laboratory measured spectral reflectance (Bell et al., 2015, 2020b). These spectral algorithms are then validated by comparing physiological estimates from the imagery to measurements in the field or by direct comparison of image and field measured reflectance spectra. An additional consideration for pairing field measurements with imagery is that the kelp canopy is a “moving target,” constantly shifting its position and distribution with changing tides and currents. Some studies have attempted to account for these variations using spatial smoothing (e.g., two dimensional gaussian filter) to account for the shifting canopy in validation imagery (Hamilton et al., 2020). This is an important consideration for all ground truthing activities since field validation is rarely achieved simultaneous to image acquisition.
Management Needs
The choice of remote sensing data for informing resource management depends on the bio-physical characteristics described above, and the spatial and temporal scales of key pressures on kelp abundance (Supplementary Table 1) and associated management actions. For example, local and state governments and municipalities may manage kelp harvest or restoration activities at individual sites or along small stretches of coastline. National, state, and provincial governments may be responsible for monitoring and management over hundreds to thousands of km, and international organizations (e.g., IUCN) may be interested in tracking global trends in kelp abundance. In some cases, focus will be on contemporary data (e.g., monitoring restoration projects) while for others, a longer historical perspective will be necessary (e.g., detecting impacts of climate change and invasive species on kelp abundance). Spatiotemporal context is key to guiding investments in data collection and processing to address trade-offs between spatial and temporal coverage and resolution. For example, ephemeral perturbations (e.g., oil spills, nutrient discharges) might require greater investment in the speed of data processing relative to longer lasting disturbances (e.g., coastal development, persistent cooling water discharges). Often, the physical and biological factors (Table 1) will determine the minimum spatial resolution needed to accurately measure kelp, while the management application will determine the required spatial and temporal coverage and rate of data processing (Table 2).
Below we review four general categories of kelp forest management where remote sensing data may be particularly valuable: (1) managing wild kelp harvest, (2) designing, monitoring, and managing marine protected areas (MPAs), (3) assessing the need and scale of kelp forest restoration, and (4) detecting climate change impacts to kelp forests. For each category, we provide examples from the west coast of North America where remote sensing data has been or will be used to inform management action and describe the geographic challenges associated with kelp remote sensing in these locations. Note that these case studies do not involve new data collection or analyses. We identified these examples through a review of scientific literature and government reports. Based on our review, we provide suggestions for how the use of remote sensing data could be further developed for each use case.
Managing Wild Kelp Harvest
There is an increasing interest and demand for harvested macroalgae for food, biofuel and other products, highlighting the importance of ensuring these resources are managed sustainably (Krumhansl et al., 2017; Mac Monagail et al., 2017; Ulaski et al., 2020). Data on kelp species, area, density, and biomass can help inform spatial management of harvesting, harvest limits, and adaptive management (e.g., temporary closures during periods of low kelp abundance). This data could also be used to inform permitting and zonation of aquaculture activities, i.e., where aquaculture should or should not occur based on presence and persistence of wild populations, potential for delivering certain ecosystem services, etc.
In British Columbia (BC), Canada, the Provincial Ministry of Forests, Lands, Natural Resource Operations and Rural Development (FLNRORD) currently manages wild aquatic plant harvest under the Fish and Seafood Act and the Fish and Seafood Licensing Regulation (2017). Licensing decisions are informed by the geographic area where harvesting aquatic plants may occur, the type of aquatic plants, and the quantity of each aquatic plant species that may be harvested. To inform kelp harvest management, the Province of BC surveyed canopy kelp forest distribution and abundance between 1976 and 2007 using Foreman’s (1975) Kelp Inventory Method (KIM-1) occupied airborne surveys, to quantify standing crop of canopy kelps and inform licensing decisions (Sutherland et al., 2008; Costa et al., 2020). Due to the logistical challenges and prohibitive cost of collecting this information across a coastline of roughly 25,000 km, these surveys do not cover the full extent of kelp forest distribution and most of the areas have only been surveyed once. As such, these data are of limited use for informing current licensing, identifying areas of persistent kelp, and determining trends in kelp abundance and condition.
To address these data gaps and in response to stakeholder concerns about perceived declines in kelp, the Marine Plan Partnership (MaPP), a partnership between the Province of BC and 16 coastal First Nations (Diggon et al., 2020), initiated a collaborative regional kelp monitoring program in 2018 with many partners that is intended to improve understanding of the distribution, abundance, biomass, and condition of kelp, document changes over time, and identify pressures on these systems across the BC coast. Data are collected using a variety of remote sensing methods, including WorldView and Landsat satellite imagery, multispectral data from plane-based surveys, and localized UAS imagery coupled with in situ data, including kayak/skiff and SCUBA surveys. This multi-scale approach allows the data to be used for multiple management questions related to kelp harvest (Table 2 and Figure 3). For example, the Landsat imagery can be used to make large-scale (i.e., BC-wide) kelp inventories and detect trends in aerial extent to inform licensing decisions at local and provincial levels for large kelp forests. WorldView (and other high spatial resolution satellite platforms) can be used for detecting trends in aerial extent of narrow fringing kelp forests. Plane-based surveys provide higher resolution data that can be useful for inventories of smaller, nearshore beds for specific regions, and to delineate areas for species-specific harvest or protection. In situ data and UAS imagery are useful for monitoring individual kelp forests and making more detailed measurements of kelp species composition, condition, density, and biomass. These measurements can also be used to calibrate and validate remote sensing data.
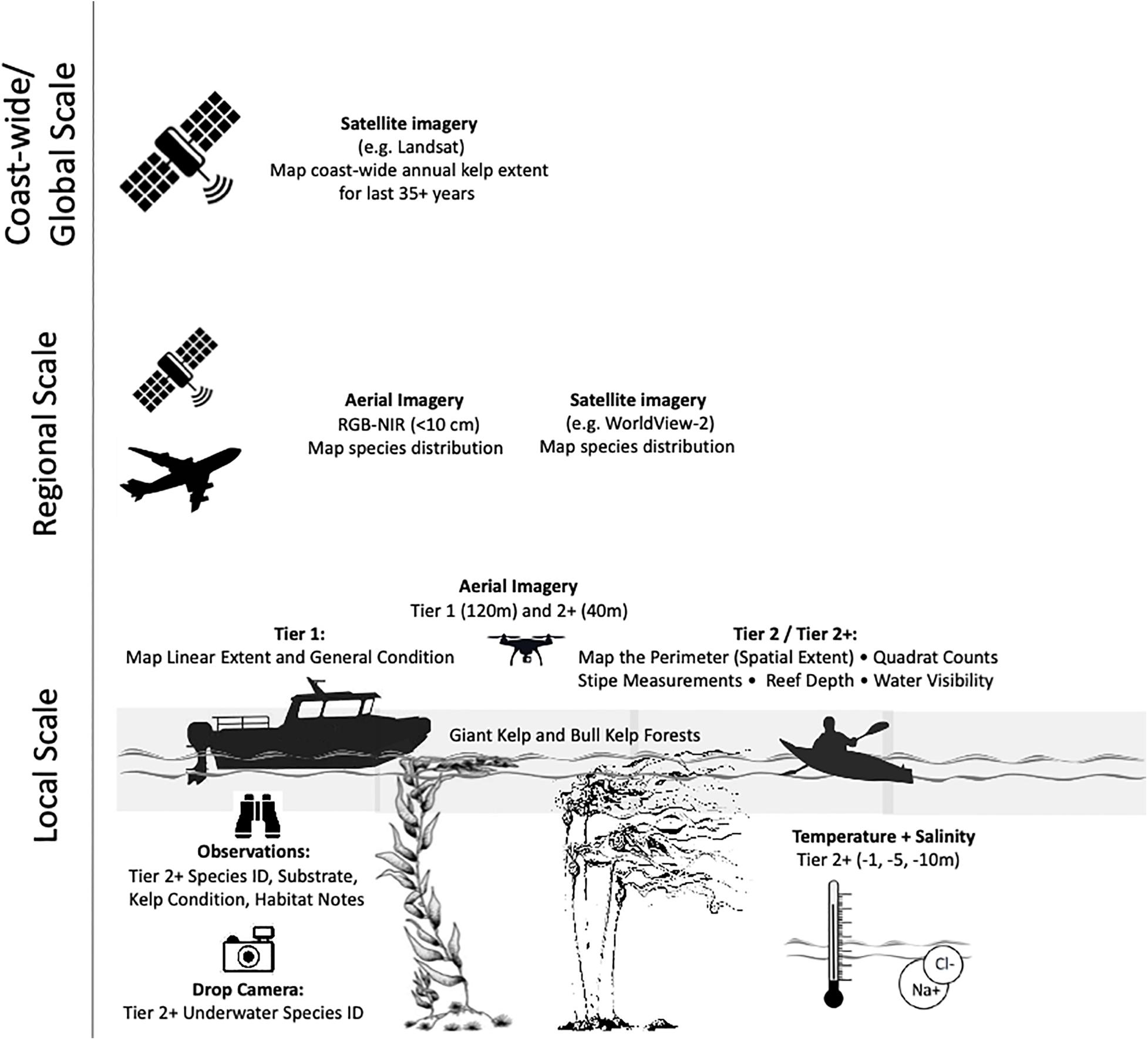
Figure 3. Multi-scale canopy kelp monitoring approach taken by the Marine Plan Partnership. Local scale observations are separated into Tiers which represent increasing complexity of measurements.
Designing, Monitoring, and Managing Marine Protected Areas
State and federal marine protected areas (MPAs) have become key tools for ocean conservation and ecosystem-based management (UNEP, 2010; O’Leary et al., 2016). Effectively designing MPAs and MPA networks to protect and/or restore biodiversity and ecological functioning requires data on the location of representative, as well as and rare or vulnerable, species and habitat types (Margules and Pressey, 2000; Saarman et al., 2013; Green et al., 2014). MPA networks should include replicates of habitats or species that are distributed across sites that vary in environmental characteristics to capture the full suite of biodiversity and to promote resilience in the face of climate change (Gaines et al., 2010; Green et al., 2014; Magris et al., 2014; Carr et al., 2017). Remote sensing approaches are particularly well-suited to create large-scale kelp habitat maps that can inform marine planning by identifying areas of higher kelp extent and density and locations of temporally persistent kelp forests indicating resilience to environmental change (Cogan et al., 2009; Kachelriess et al., 2014).
Long term monitoring can provide insight into MPA management effectiveness (Carr et al., 2019). MPA monitoring is rarely initiated prior to MPA implementation (one exception to this is the Channel Islands National Park), which limits the ability to conduct before-after-control-impact (BACI) statistical designs, common for ecological assessments of management interventions (Pomeroy et al., 2005; Rassweiler et al., 2021). Further, ecological monitoring of MPA networks is rarely adequately funded and often relies on costly in situ techniques, making relevant monitoring data challenging to collect (Grorud-Colvert et al., 2014). Historical remote sensing data can provide a way to conduct BACI type analyses retroactively, and contemporary imagery can provide a cost-effective method for monitoring kelp abundance inside and outside of MPAs.
In 1999, the state of California passed the Marine Life Protection Act (MLPA) requiring the re-evaluation of all existing state MPAs and the design of new MPAs that together would function as a statewide MPA network focused on goals of protecting marine ecosystems (including kelp) and rebuilding depleted marine life populations (Botsford et al., 2014). This statewide MPA network followed federal efforts such as the National Marine Sanctuaries (NMS) Act passed in 1972. Four regional science-based and stakeholder-led MPA planning processes were conducted between 2006 and 2012, ultimately leading to the design and designation of a network of 124 MPAs covering 16% of state waters (0–3 nmi; Gleason et al., 2013). Kelp, and especially areas of persistent kelp, were identified by stakeholders and scientists as an important ecosystem to represent and replicate in MPAs (Saarman et al., 2013). The California Department of Fish and Wildlife (CDFW) conducted airborne kelp surveys during peak growth periods (late summer-fall) at irregular intervals since 1989 (Veisze et al., 2001). This high spatial resolution (2 m) multi-spectral imagery was used to develop maps of canopy kelp and to identify areas of persistent kelp (Figure 4). These kelp mapping data, as well as data for other key habitats, were made available to stakeholders, scientists, and policy-makers in an online decision-support tool used during MPA planning that provided analyses of how much of each habitat would be protected by proposed MPAs (Merrifield et al., 2013). Ultimately, about 22% of persistent kelp was protected in the statewide MPA network (Gleason et al., 2013). To monitor kelp habitat across the network, the State of California performed additional airborne kelp surveys through 2016; however, high costs have limited the ability to conduct airborne surveys annually across the State. Landsat-based time series of canopy area and biomass (Bell et al., 2020a) are currently being used to document trends inside and outside of MPAs across the network. This moderate resolution data can be supplemented with analysis of high resolution satellite imagery (e.g., WorldView and Planet) and UAS surveys (Cavanaugh et al., 2021).
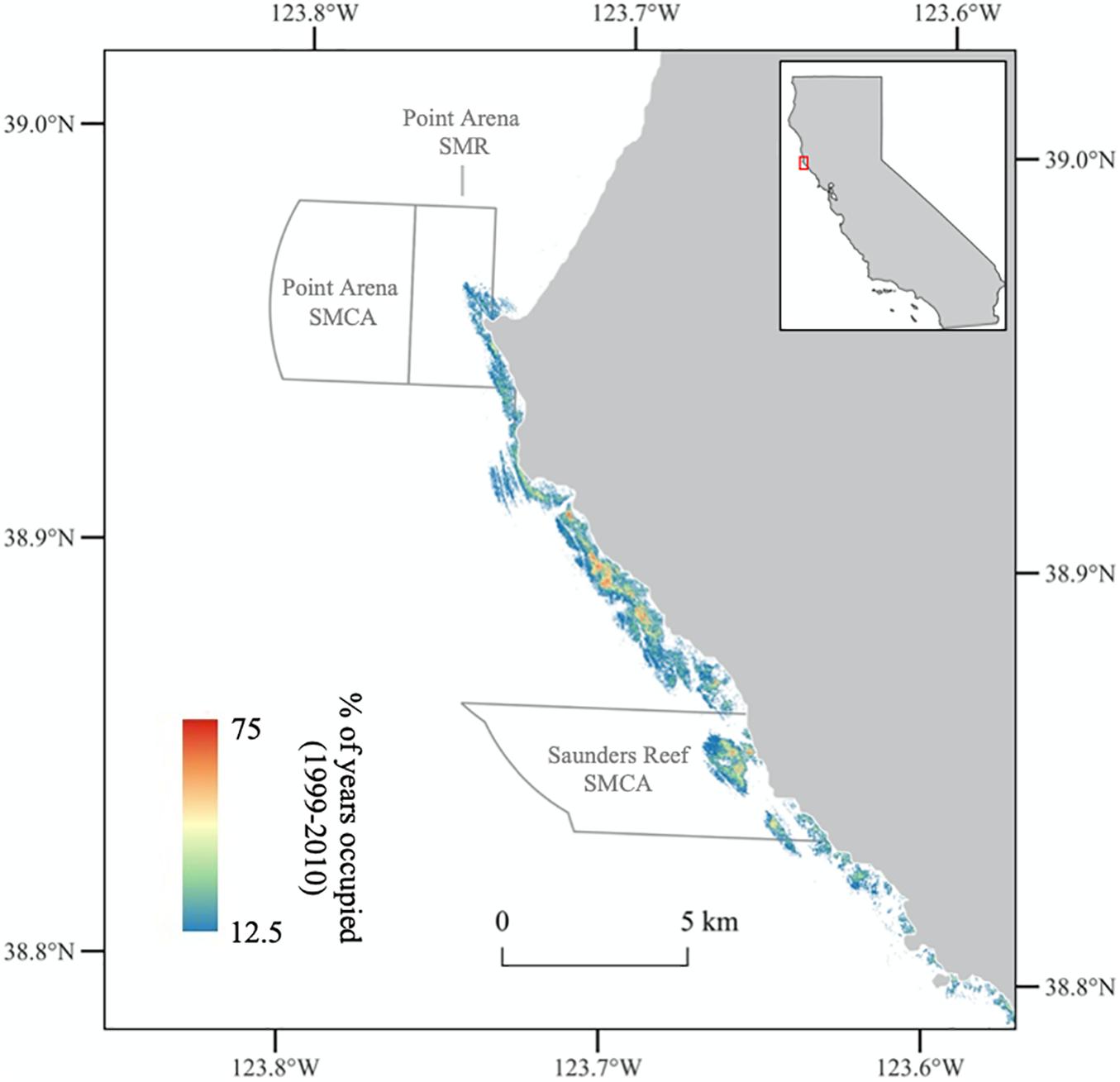
Figure 4. Example kelp canopy persistence map created from the California Department of Fish and Wildlife annual aerial surveys. Persistence is calculated as the percentage of years between 1999 and 2010 with kelp canopy. State Marine Reserves (SMR) and State Marine Conservation Areas (SMCA) are outlined in gray. Kelp persistence data can be accessed from California Department of Fish and Wildlife at https://map.dfg.ca.gov/metadata/ds1058.html.
Similar data will be critical for informing the development and management of MPAs associated with kelp forests in Baja California. Protected areas have been historically managed by fishers under territorial rights called “cooperativas,” with a high degree of variability in protection level and extent (McCay, 2017; Villaseñor-Derbez et al., 2019). In 2017, the Mexican government decreed all islands in Baja California as a Biosphere Reserve. However, protection to marine habitats is variable as fishing and aquaculture use is still permitted in many reserves. Future efforts to expand the extent and protection levels of marine reserves will require data on the distribution of habitat such as kelp forests (Arafeh-Dalmau et al., 2021).
Kelp Forest Restoration
Across the globe, kelp forests have become increasingly threatened by multiple stressors that are exacerbated by climate change (Krumhansl et al., 2016; Wernberg et al., 2016; Arafeh-Dalmau et al., 2020). Documented kelp forest declines around the world, including several dramatic examples of local or regional extinctions of kelp (California, United States: Rogers-Bennett and Catton, 2019; Australia: reviewed in Layton et al., 2020, Atlantic Canada and Europe: reviewed in Filbee-Dexter and Wernberg, 2018) have driven increasing interest in kelp forest restoration as a way to conserve and rebuild populations (Eger et al., 2020a; Layton et al., 2020). On portions of the west coast of North America, heatwaves, sea star wasting disease, and increases in grazer populations have caused local- to regional-scale declines in kelp abundance. Resource managers have expressed interest in restoring kelp forests in areas where declines have been most severe and enduring to recover declining and lost ecosystem functions. Pilot projects including herbivore removal and kelp outplanting are beginning and interest for more effort is high but identifying locations for restoration efforts has been challenging across such a large geography. Remote sensing offers an efficient option for mapping areas where kelp has been lost (including urchin barrens; Carnell and Keough, 2019; Glasby and Gibson, 2020), locating remnant kelp patches (for spore production), selecting sites that may be suitable for restoration based on the history of kelp presence and persistence, and monitoring the impacts of restoration efforts over time (Gleason et al., 2021).
For example, resource managers, scientists and policy advocates convened to outline strategies for restoring populations of bull kelp following its widespread demise along the coast of Northern California and in the Greater Farallones National Marine Sanctuary (Hohman et al., 2019). Recommended actions included the harvest of purple sea urchins, reintroduction of sea stars, and seeding of kelp. A key planning step in all these actions involves potential site selection; historical canopy persistence was identified as an important variable for this process. Hohman et al. (2019) suggested calculating persistence using the time series of CDFW airborne surveys described in the previous section (Veisze et al., 2001; Figure 4). This imagery has high enough spatial resolution to map the nearshore, fringing forests of bull kelp found along this region. However, these annual surveys ended in 2016 (with additional airborne surveys attempted in 2019 and 2020 but incomplete due to historic wildfire smoke occluding imagery), and the Greater Farallones Sanctuary Advisory Council emphasized the importance of developing a cost-effective long-term monitoring program to document future changes in bull kelp abundance in restored and non-restored areas. A combination of UAS and high resolution satellite imagery were recommended for these monitoring efforts because (1) high spatial resolution (∼1–10 m) imagery is needed to capture small nearshore forests in this region, (2) control over the timing of image acquisition or imagery with a high revisit frequency is needed to account for the relatively high cloud cover in the region, and (3) the spatial extent of the decline is relatively large (100s of km) and may be difficult to cover with UAS imagery alone. To this end, a collaborative UAS monitoring network was started in 2019 to monitor key sites in the hardest hit parts of the region. High-resolution satellite, UAS, and in situ monitoring are also being used to assess the effectiveness of pilot restoration efforts.
Detecting Climate Change Impacts to Kelp
Distinguishing the impacts of climate change on kelp abundance is an important management challenge that requires cost-effective time series data at large spatial scales. This type of application requires long-term (>30 years) monitoring of kelp dynamics in order to separate climate related trends from other sources of variability (Bell et al., 2020a). As a result, long-term satellite time series such as the Landsat program, which has been operating continuously since 1972 (imagery freely available), and the SPOT program, which has been operating since 1984 (imagery not freely available), are likely to be the most useful tools for these applications. The multidecadal Landsat time series has been used to examine trends in kelp abundance in Baja California (Arafeh-Dalmau et al., 2019; Cavanaugh et al., 2019), southern California (Bell et al., 2020a), central California (Young et al., 2016), northern California (Finger et al., 2021; McPherson et al., 2021), and Oregon (Hamilton et al., 2020). But 30 m resolution Landsat imagery is not suitable for monitoring kelp in certain regions (see “Geography of Challenges”). In these locations, time series from higher resolution satellite and airborne sensors should be combined with other data such as historical airborne and in situ surveys to characterize long-term trends and drivers (e.g., Pfister et al., 2018; Schroeder et al., 2020; Berry et al., 2021).
Such data can be used to inform approaches aimed at mitigating the widespread effects of climate change on kelp across a spectrum of more localized management actions discussed above (e.g., kelp harvesting, MPA design, and kelp forest restoration). For example, near real-time kelp monitoring can facilitate adaptive management of kelp forest harvesting and kelp dependent fisheries. Harvest limits and closures can be implemented during disturbances such as marine heatwaves (e.g., the closure of the recreational abalone fishery following collapse of kelp forests in northern California after 2014; Rogers-Bennett and Catton, 2019), while harvest could be expanded when conditions promote high productivity. The identification of remnant forests might identify sources of resistant genotypes that can be used to restore forests more likely to persist in future environmental conditions. Data on long-term changes to kelp distributions can be used to predict range shifts of kelp and associated species and support proactive management.
Conclusion
Geographic variability in the physical environment, the highly dynamic nature of kelp forests, and a wide variety of management issues combine to create challenges for remote monitoring of kelp canopy. Fortunately, the availability of remote sensing tools for monitoring and management of surface-canopy forming kelp forests is increasing due to new satellite datasets and advances in UAS technology. These approaches can be used to conduct monitoring of kelp species and abundance over much larger areas than is possible with in situ methods.
Our four case studies demonstrate how a variety of datasets are required to address different management issues across a large geographic area with a wide range of kelp habitat types. Calibrated regional to global satellite-based datasets on kelp distributions and dynamics (Bell et al., 2020a; Mora-Soto et al., 2020) are useful for applications like detecting the impacts of climate change at large spatial scales. However, these large-scale data are not suitable for mapping kelp in some locations, including areas where kelp forms small, fringing, nearshore forests. These large-scale datasets can be complemented with mapping efforts that utilize other remote sensing data such as high-resolution satellite/airborne and UAS imagery, and with targeted in situ surveys of kelp abundance and size structure. Scientists and managers must also calibrate and standardize data from different sensors and/or different processing methods, and in situ data can be valuable for this purpose. This standardization is critical for creating time series of kelp dynamics from different data sources.
More work is needed to characterize and account for the uncertainties of remote estimates of kelp abundance due to sensor characteristics, processing methods, and environmental factors. This uncertainty will determine the usefulness of different remote sensing approaches for a particular management action or objective. For example, if a review of kelp harvesting is triggered by a certain level of decline in kelp abundance in a region (e.g., 20%), then the sampling method must be sufficient to distinguish this level of change. It is particularly important to characterize detection limits and sensitivity for coarser resolution satellite imagery (e.g., Hamilton et al., 2020; Finger et al., 2021). Characterizing uncertainty in datasets is especially important and challenging for interpretation of historical imagery where contemporaneous validation data is missing.
While our case studies are focused on the west coast of North America, the principles discussed here are relevant for remote sensing of canopy-forming kelp forests in other parts of the world. For example, remote sensing data have been used to document surface-canopy forming kelp dynamics in Tasmania (Johnson et al., 2011; Butler et al., 2020), New Zealand (Fyfe et al., 1999), Chile (Huovinen et al., 2020), and Argentina (Friedlander et al., 2020). Recently a global map of giant kelp distribution was created from Sentinel-2 satellite imagery (Mora-Soto et al., 2020). Next steps for scientists are to map changes in kelp distributions at global scales with increasing spatial and temporal resolution to better inform management and increase understanding of key drivers of kelp loss. The characteristics that affect remote sensing of kelp that we identified here (Table 1) can help guide the development of future datasets that expand the spatial and temporal coverage of canopy kelp forest dynamics.
As the remote sensing community integrates various datasets and compares processing methodologies, the management community should work to share and coordinate management strategies across regions and organizations. Kelp forests span local, regional, and national borders, and coordination among management organizations could help to address the ongoing multi-scale pressures on kelp forest ecosystems (Aburto-Oropeza et al., 2018; Supplementary Table 1).
Author Contributions
KyC, TB, MG, and NE conceived the idea for the manuscript. KyC, TB, MCo, LG, MG, MH-L, RF, MM, and LR led the writing of the manuscript. KyC, MCo, LG, MH-L, RF, OP, and LR led creation of the figures and tables. KyC developed the first draft. All authors contributed critically to the drafts and gave final approval for publication.
Funding
Funding was provided by the Nature Conservancy (Grant No. 02042019-5719), the U.S. National Science Foundation (Grant No. OCE 1831937), and the U.S. Department of Energy ARPA-E (Grant No. DE-AR0000922).
Conflict of Interest
The authors declare that the research was conducted in the absence of any commercial or financial relationships that could be construed as a potential conflict of interest.
Publisher’s Note
All claims expressed in this article are solely those of the authors and do not necessarily represent those of their affiliated organizations, or those of the publisher, the editors and the reviewers. Any product that may be evaluated in this article, or claim that may be made by its manufacturer, is not guaranteed or endorsed by the publisher.
Acknowledgments
This paper was the result of a kelp remote sensing workshop hosted by the Nature Conservancy.
Supplementary Material
The Supplementary Material for this article can be found online at: https://www.frontiersin.org/articles/10.3389/fmars.2021.753531/full#supplementary-material
References
Aburto-Oropeza, O., Johnson, A. F., Agha, M., Allen, E. B., Allen, M. F., González, J. A., et al. (2018). Harnessing cross-border resources to confront climate change. Environ. Sci. Policy 87, 128–132. doi: 10.1016/j.envsci.2018.01.001
Arafeh-Dalmau, N., Cavanaugh, K. C., Possingham, H. P., Munguia-Vega, A., Montaño-Moctezuma, G., Bell, T. W., et al. (2021). Southward decrease in the protection of persistent giant kelp forests in the northeast Pacific. Commun. Earth Environ. 2:119. doi: 10.1038/s43247-021-00177-9
Arafeh-Dalmau, N., Montaño-Moctezuma, G., Martínez, J. A., Beas-Luna, R., Schoeman, D. S., and Torres-Moye, G. (2019). Extreme marine heatwaves alter kelp forest community near its equatorward distribution limit. Front. Mar. Sci. 6:499. doi: 10.3389/fmars.2019.00499
Arafeh-Dalmau, N., Schoeman, D. S., Montaño-Moctezuma, G., Micheli, F., Rogers-Bennett, L., Olguin-Jacobson, C., et al. (2020). Marine heat waves threaten kelp forests. Science 367:635. doi: 10.1126/science.aba5244
Beas-Luna, R., Micheli, F., Woodson, C. B., Carr, M., Malone, D., Torre, J., et al. (2020). Geographic variation in responses of kelp forest communities of the California Current to recent climatic changes. Glob. Change Biol. 26, 6457–6473. doi: 10.1111/gcb.15273
Bell, T. W., Cavanaugh, K. C., and Siegel, D. A. (2015). Remote monitoring of giant kelp biomass and physiological condition: an evaluation of the potential for the Hyperspectral Infrared Imager (HyspIRI) mission. Remote Sens. Environ. 167, 218–228. doi: 10.1016/j.rse.2015.05.003
Bell, T. W., Allen, J. G., Cavanaugh, K. C., and Siegel, D. A. (2020a). Three decades of variability in California’s giant kelp forests from the Landsat satellites. Remote Sens. Environ. 238:110811. doi: 10.1016/j.rse.2018.06.039
Bell, T. W., Nidzieko, N. J., Siegel, D. A., Miller, R. J., Cavanaugh, K. C., Nelson, N. B., et al. (2020b). The utility of satellites and autonomous remote sensing platforms for monitoring offshore aquaculture farms: a case study for canopy forming kelps. Front. Mar. Sci. 7:1083. doi: 10.3389/fmars.2020.520223
Bell, T. W., Reed, D. C., Nelson, N. B., and Siegel, D. A. (2018). Regional patterns of physiological condition determine giant kelp net primary production dynamics. Limnol. Oceanogr. 63, 472–483. doi: 10.1002/lno.10753
Berry, H. D., Mumford, T. F., Christiaen, B., Dowty, P., Calloway, M., Ferrier, L., et al. (2021). Long-term changes in kelp forests in an inner basin of the Salish Sea. PLoS One 16:e0229703. doi: 10.1371/journal.pone.0229703
Botsford, L. W., White, J. W., Carr, M. H., and Caselle, J. E. (2014). Marine protected area networks in California, USA. Adv. Mar. Biol. 69, 205–251. doi: 10.1016/B978-0-12-800214-8.00006-2
Britton-Simmons, K., Eckman, J. E., and Duggins, D. O. (2008). Effect of tidal currents and tidal stage on estimates of bed size in the kelp Nereocystis luetkeana. Mar. Ecol. Prog. Ser. 355, 95–105. doi: 10.3354/meps07209
Bryhn, A., Kraufvelin, P., Bergström, U., Vretborn, M., and Bergström, L. (2020). A model for disentangling dependencies and impacts among human activities and marine ecosystem services. Environ. Manag. 65, 575–586. doi: 10.1007/s00267-020-01260-1
Burt, J. M., Tim Tinker, M., Okamoto, D. K., Demes, K. W., Holmes, K., and Salomon, A. K. (2018). Sudden collapse of a mesopredator reveals its complementary role in mediating rocky reef regime shifts. Proc. R. Soc. B Biol. Sci. 285:20180553. doi: 10.1098/rspb.2018.0553
Butler, C., Lucieer, V., Wotherspoon, S., and Johnson, C. (2020). Multi-decadal decline in cover of giant kelp Macrocystis pyrifera at the southern limit of its Australian range. Mar. Ecol. Prog. Ser. 653, 1–18. doi: 10.3354/meps13510
Calloway, M., Oster, D., Berry, H., Mumford, T., Naar, N., Peabody, B., et al. (2020). Puget Sound Kelp Conservation and Recovery Plan. (Seattle, WA: NOAA-NMFS), 52. Available online at: https://nwstraits.org/our-work/kelp/
Carnell, P. E., and Keough, M. J. (2019). Reconstructing historical marine populations reveals major decline of a kelp forest ecosystem in Australia. Estuar. Coast. 42, 765–778. doi: 10.1007/s12237-019-00525-1
Carr, M. H., and Reed, D. C. (2016). “Shallow Rocky Reefs and Kelp Forests,” in Ecosystems of California, eds H. Mooney and E. Zavaleta (Berkeley, CA:University of California Press), 311–336.
Carr, M. H., Robinson, S. P., Wahle, C., Davis, G., Kroll, S., Murray, S., et al. (2017). The central importance of ecological spatial connectivity to effective coastal marine protected areas and to meeting the challenges of climate change in the marine environment. Aquat. Conserv. 27, 6–29. doi: 10.1002/aqc.2800
Carr, M. H., White, J. W., Saarman, E., Lubchenco, J., Milligan, K., and Caselle, J. E. (2019). Marine protected areas exemplify the evolution of science and policy. Oceanography 32, 94–103. doi: 10.5670/oceanog.2019.315
Casal, G., Sánchez-Carnero, N., ánchez-Rodríguez, E. S., and Freire, J. (2011). Remote sensing with SPOT-4 for mapping kelp forests in turbid waters on the south European Atlantic shelf. Estuar. Coast. Shelf Sci. 91, 371–378. doi: 10.1016/j.ecss.2010.10.024
Cavanaugh, K. C., Cavanaugh, K. C., Bell, T. W., and Hockridge, E. G. (2021). An automated method for mapping giant kelp canopy dynamics from UAV. Front. Environ. Sci. 8:587354. doi: 10.3389/fenvs.2020.587354
Cavanaugh, K. C., Reed, D. C., Bell, T. W., Castorani, M. C. N., and Beas-Luna, R. (2019). Spatial variability in the resistance and resilience of giant kelp in southern and baja California to a multiyear heatwave. Front. Mar. Sci. 6:413. doi: 10.3389/fmars.2019.00413
Cavanaugh, K. C., Siegel, D. A., Reed, D. C., and Dennison, P. E. (2011). Environmental controls of giant-kelp biomass in the Santa Barbara Channel, California. Mar. Ecol. Prog. Ser. 429, 1–17. doi: 10.3354/meps09141
Chander, G., Markham, B. L., and Barsi, J. A. (2007). Revised Landsat-5 thematic mapper radiometric calibration. IEEE Geosci. Remote Sens. Lett. 4, 490–494. doi: 10.1109/LGRS.2007.898285
Chander, G., Markham, B. L., and Helder, D. L. (2009). Summary of current radiometric calibration coefficients for Landsat MSS. TM, ETM+, and EO-1 ALI sensors. Remote Sens. Environ. 113, 893–903. doi: 10.1016/j.rse.2009.01.007
Cogan, C. B., Todd, B. J., Lawton, P., and Noji, T. T. (2009). The role of marine habitat mapping in ecosystem-based management. ICES J. Mar. Sci. 66, 2033–2042. doi: 10.1093/icesjms/fsp214
Costa, M., Le Baron, N., Tenhunen, K., Nephin, J., Willis, P., Mortimor, J. P., et al. (2020). Historical distribution of kelp forests on the coast of British Columbia: 1858–1956. Appl. Geogr. 120:102230. doi: 10.1016/j.apgeog.2020.102230
Dayton, P. K. (1972). “Toward an understanding of community resilience and the potential effects of enrichments to the benthos at McMurdo Sound, Antarctica,” in Proceedings of The Colloquium On Conservation Problems In Antarctica, (Lawrence, KS: Allen Press), 81–95.
Deysher, L. E. (1993). Evaluation of remote sensing techniques for monitoring giant kelp populations. Hydrobiologia 261, 307–312. doi: 10.1007/BF00049033
Diggon, S., Bones, J., Short, C. J., Smith, J. L., Dickinson, M., Wozniak, K., et al. (2020). The marine plan partnership for the North Pacific Coast-MaPP: a collaborative and co-led marine planning process in British Columbia. Mar. Policy. doi: 10.1016/j.marpol.2020.104065 [Epub ahead of print].
Dudley, P. N., Rogers, T. L., Morales, M. M., Stoltz, A. D., Sheridan, C. J., Beulke, A. K., et al. (2021). A more comprehensive climate vulnerability assessment framework for fisheries social-ecological systems. Front. Mar. Sci. 8:674. doi: 10.3389/fmars.2021.678099
Ebeling, A. W., Laur, D. R., and Rowley, R. J. (1985). Severe storm disturbances and reversal of community structure in a southern California kelp forest. Mar. Biol. 84, 287–294. doi: 10.1007/BF00392498
Eger, A. M., Vergés, A., Choi, C. G., Christie, H., Coleman, M. A., Fagerli, C. W., et al. (2020b). Financial and institutional support are important for large-scale kelp forest restoration. Front. Mar. Sci. 7:811. doi: 10.3389/fmars.2020.535277
Eger, A. M., Marzinelli, E., Gribben, P., Johnson, C. R., Layton, C., Steinberg, P. D., et al. (2020a). Playing to the positives: using synergies to enhance kelp forest restoration. Front. Mar. Sci. 7:544. doi: 10.3389/fmars.2020.00544
Eger, A., Marzinelli, E., Baes, R., Blain, C., Blamey, L., Carnell, P., et al. (2021). The economic value of fisheries, blue carbon, and nutrient cycling in global marine forests. EcoEvoRxiv [Preprint]. doi: 10.32942/osf.io/n7kjs
Eisaguirre, J. H., Eisaguirre, J. M., Davis, K., Carlson, P. M., Gaines, S. D., and Caselle, J. E. (2020). Trophic redundancy and predator size class structure drive differences in kelp forest ecosystem dynamics. Ecology 101:e02993. doi: 10.1002/ecy.2993
Elliott, M., Burdon, D., Atkins, J. P., Borja, A., Cormier, R., de Jonge, V. N., et al. (2017). “And DPSIR begat DAPSI(W)R(M)!”-A unifying framework for marine environmental management. Mar. Pollut. Bull. 118, 27–40. doi: 10.1016/j.marpolbul.2017.03.049
Estes, J. A., and Palmisano, J. F. (1974). Sea otters: their role in structuring nearshore communities. Science 185, 1058–1060. doi: 10.1126/science.185.4156.1058
Filbee-Dexter, K., and Wernberg, T. (2018). Rise of turfs: a new battlefront for globally declining kelp forests. BioScience 68, 64–76. doi: 10.1093/biosci/bix147
Finger, D. J. I., McPherson, M. L., Houskeeper, H. F., and Kudela, R. M. (2021). Mapping bull kelp canopy in northern California using Landsat to enable long-term monitoring. Remote Sens. Environ. 254:112243. doi: 10.1016/j.rse.2020.112243
Foreman, R. E. (1975). KIM-1, A Method For Inventory Of Floating Kelps And Its Application To Selected Areas Of Kelp License Area 12. BERP Reports. 75-1. Submitted Federal Fisheries and Marine Service and BC Marine Resources Branch. British Columbia, BC: BC Marine Resources Branch, 1–81.
Foster, M. S., and Schiel, D. R. (2010). Loss of predators and the collapse of southern California kelp forests (?): alternatives, explanations and generalizations. J. Exp. Mar. Biol. Ecol. 393, 59–70. doi: 10.1016/j.jembe.2010.07.002
Friedlander, A. M., Ballesteros, E., Bell, T. W., Caselle, J. E., Campagna, C., Goodell, W., et al. (2020). Kelp forests at the end of the earth: 45 years later. PLoS One 15:e0229259. doi: 10.1371/journal.pone.0229259
Fyfe, J., Israel, S. A., Chong, A., Ismail, N., Hurd, C. L., and Probert, K. (1999). Mapping marine habitats in Otago, Southern New Zealand. Geocarto Int. 14, 17–28. doi: 10.1080/10106049908542113
Gaines, S. D., White, C., Carr, M. H., and Palumbi, S. R. (2010). Designing marine reserve networks for both conservation and fisheries management. Proc. Natl. Acad. Sci. U.S.A. 107, 18286–18293. doi: 10.1073/pnas.0906473107
Garcia, R. A., Lee, Z., and Hochberg, E. J. (2018). Hyperspectral shallow-water remote sensing with an enhanced benthic classifier. Remote Sens. 10:147. doi: 10.3390/rs10010147
Glasby, T. M., and Gibson, P. T. (2020). Decadal dynamics of subtidal barrens habitat. Mar. Environ. Res. 154:104869. doi: 10.1016/j.marenvres.2019.104869
Gleason, M. G., Caselle, J. E., Heady, W. N., Saccomanno, V. R., Zimmerman, J., McHugh, T. A., et al. (2021). A Structured Approach for Kelp Restoration and Management Decisions in California. Arlington, VA: The Nature Conservancy.
Gleason, M., Fox, E., Ashcraft, S., Vasques, J., Whiteman, E., Serpa, P., et al. (2013). Designing a network of marine protected areas in California: achievements, costs, lessons learned, and challenges ahead. Ocean Coast. Manag. 74, 90–101. doi: 10.1016/j.ocecoaman.2012.08.013
Graham, M. H., Vásquez, J. A., and Buschmann, A. H. (2007). Global ecology of the giant kelp Macrocystis: from ecotypes to ecosystems. Oceanogr. Mar. Biol. 45, 39–88. doi: 10.1201/9781420050943.ch2
Green, A. L., Fernandes, L., Almany, G., Abesamis, R., McLeod, E., Aliño, P. M., et al. (2014). Designing marine reserves for fisheries management. biodiversity conservation, and climate change adaptation. Coast. Manag. 42, 143–159. doi: 10.1080/08920753.2014.877763
Grorud-Colvert, K., Claudet, J., Tissot, B. N., Caselle, J. E., Carr, M. H., Day, J. C., et al. (2014). Marine protected area networks: assessing whether the whole is greater than the sum of its parts. PLoS One 9:e102298. doi: 10.1371/journal.pone.0102298
Hamilton, S. L., Bell, T. W., Watson, J. R., Grorud-Colvert, K. A., and Menge, B. A. (2020). Remote sensing: generation of long-term kelp bed data sets for evaluation of impacts of climatic variation. Ecology 101:e03031. doi: 10.1002/ecy.3031
Hedley, J. D., Harborne, A. R., and Mumby, P. J. (2005). Technical note: simple and robust removal of sun glint for mapping shallow-water benthos. Int. J. Remote Sens. 26, 2107–2112. doi: 10.1080/01431160500034086
Hirsh, H. K., Nickols, K. J., Takeshita, Y., Traiger, S. B., Mucciarone, D. A., Monismith, S., et al. (2020). Drivers of biogeochemical variability in a central California kelp forest: implications for local amelioration of ocean acidification. J. Geophys. Res. Oceans 125:e2020JC016320. doi: 10.1029/2020JC016320
Hochberg, E. J., Andréfouët, S., and Tyler, M. R. (2003). Sea surface correction of high spatial resolution ikonos images to improve bottom mapping in near-shore environments. IEEE Geosci. Remote Sens. 41, 1724–1729. doi: 10.1109/TGRS.2003.815408
Hohman, R., Hutto, S. V., Catton, C. A., and Koe, F. (2019). Sonoma-Mendocino bull kelp recovery plan. Page Sonoma-Mendocino Bull Kelp Recovery Plan. Plan for the Greater Farallones National Marine Sanctuary and the California Department of Fish and Wildlife. San Francisco, CA: Department of Fish and Wildlife.
Hughes, B. B., Beas-Luna, R., Barner, A. K., Brewitt, K., Brumbaugh, D. R., Cerny-Chipman, E. B., et al. (2017). Long-Term studies contribute disproportionately to ecology and policy. BioScience 67, 271–278. doi: 10.1093/biosci/biw185
Huovinen, P., Ramírez, J., Palacios, M., and Gómez, I. (2020). Satellite-derived mapping of kelp distribution and water optics in the glacier impacted Yendegaia Fjord (Beagle Channel, Southern Chilean Patagonia). Sci. Total Environ. 703:135531. doi: 10.1016/j.scitotenv.2019.135531
Jensen, J. R., Estes, J. E., and Tinney, L. (1980). Remote sensing techniques for kelp surveys. Photogramm. Eng. Remote Sensing 46, 743–755.
Johnson, C. R., Banks, S. C., Barrett, N. S., Cazassus, F., Dunstan, P. K., Edgar, G. J., et al. (2011). Climate change cascades: shifts in oceanography, species’ ranges and subtidal marine community dynamics in eastern Tasmania. J. Exp. Mar. Biol. Ecol. 400, 17–32. doi: 10.1016/j.jembe.2011.02.032
Jones, C. G., Lawton, J. H., and Shachak, M. (1994). Organisms as ecosystem engineers. Oikos 69, 373–386. doi: 10.2307/3545850
Kachelriess, D., Wegmann, M., Gollock, M., and Pettorelli, N. (2014). The application of remote sensing for marine protected area management. Ecol. Indic. 36, 169–177. doi: 10.1016/j.ecolind.2013.07.003
Kaufman, Y. J. (1982). Solution of the equation of radiative transfer for remote sensing over nonuniform surface reflectivity. J. Geophys. Res. 87:4137. doi: 10.1029/JC087iC06p04137
Kaufman, Y. J. (1984). “Atmospheric effects on remote sensing of surface reflectance,” in Proceedings Of The Remote Sensing: Critical Review Of Technology, Arlington, United States. doi: 10.1117/12.966238
Kay, S., Hedley, J. D., and Lavender, S. (2009). Sun glint correction of high and low spatial resolution images of aquatic scenes: a review of methods for visible and near-infrared wavelengths. Remote Sens. 1, 697–730. doi: 10.3390/rs1040697
Krumhansl, K. A., Bergman, J. N., and Salomon, A. K. (2017). Assessing the ecosystem-level consequences of a small-scale artisanal kelp fishery within the context of climate-change. Ecol. Appl. 27, 799–813. doi: 10.1002/eap.1484
Krumhansl, K. A., Okamoto, D. K., Rassweiler, A., Novak, M., Bolton, J. J., Cavanaugh, K. C., et al. (2016). Global patterns of kelp forest change over the past half-century. Proc. Natl. Acad. Sci. U.S.A. 113, 13785–13790. doi: 10.1073/pnas.1606102113
Layton, C., Coleman, M. A., Marzinelli, E. M., Steinberg, P. D., Swearer, S. E., Vergés, A., et al. (2020). Kelp forest restoration in Australia. Front.Mar. Sci. 7:74. doi: 10.3389/fmars.2020.00074
Ling, S. D., Johnson, C. R., Frusher, S. D., and Ridgway, K. R. (2009). Overfishing reduces resilience of kelp beds to climate-driven catastrophic phase shift. Proc. Natl. Acad. Sci. 106, 22341–22345. doi: 10.1073/pnas.0907529106
Mac Monagail, M. L., Cornish, L., Morrison, Araújo, R., and Critchley, A. T. (2017). Sustainable harvesting of wild seaweed resources. Eur. J. Phycol. 52, 371–390. doi: 10.1080/09670262.2017.1365273
Magris, R. A., Pressey, R. L., Weeks, R., and Ban, N. C. (2014). Integrating connectivity and climate change into marine conservation planning. Biol. Conserv. 170, 207–221. doi: 10.1016/j.biocon.2013.12.032
Margules, C. R., and Pressey, R. L. (2000). Systematic conservation planning. Nature 405, 243–253. doi: 10.1038/35012251
McCay, B. J. (2017). Territorial use rights in fisheries of the northern Pacific coast of Mexico. Bull. Mar. Sci. 93, 69–81. doi: 10.5343/bms.2015.1091
McPherson, M. L., Finger, D. J. I., Houskeeper, H. F., Bell, T. W., Carr, M. H., Rogers-Bennett, L., et al. (2021). Large-scale shift in the structure of a kelp forest ecosystem co-occurs with an epizootic and marine heatwave. Commun. Biol. 4, 1–9. doi: 10.1038/s42003-021-01993-7
Merrifield, M. S., McClintock, W., Burt, C., Fox, E., Serpa, P., Steinback, C., et al. (2013). MarineMap: a web-based platform for collaborative marine protected area planning. Ocean Coast. Manag. 74, 67–76. doi: 10.1016/j.ocecoaman.2012.06.011
Mobley, C. D. (1994). Light And Water: Radiative Transfer In Natural Waters. Cambridge, MA: Academic press.
Mora-Soto, A., Palacios, M., Macaya, E., Gómez, I., Huovinen, P., Pérez-Matus, A., et al. (2020). A high-resolution global map of giant kelp (Macrocystis pyrifera) forests and intertidal green algae (Ulvophyceae) with sentinel-2 imagery. Remote Sens. 12:694. doi: 10.3390/rs12040694
Morris, R. L., Hale, R., Strain, E. M. A., Reeves, S. E., Vergés, A., Marzinelli, E. M., et al. (2020). Key principles for managing recovery of kelp forests through restoration. BioScience 70, 688–698. doi: 10.1093/biosci/biaa058
Mount, R. (2005). Acquisition of through-water aerial survey images. Photogramm. Eng. Remote Sensing 71, 1407–1415. doi: 10.14358/PERS.71.12.1407
Murfitt, S. L., Allan, B. M., Bellgrove, A., Rattray, A., Young, M. A., and Ierodiaconou, D. (2017). Applications of unmanned aerial vehicles in intertidal reef monitoring. Sci. Rep. 7, 1–11. doi: 10.1038/s41598-017-10818-9
Mustard, J. F., Staid, M. I., and Fripp, W. J. (2001). A semianalytical approach to the calibration of AVIRIS data to reflectance over water: application in a temperate estuary. Remote Sens. Environ. 75, 335–349. doi: 10.1016/S0034-4257(00)00177-2
Nijland, W., Reshitnyk, L., and Rubidge, E. (2019). Satellite remote sensing of canopy-forming kelp on a complex coastline: a novel procedure using the Landsat image archive. Remote Sens. Environ. 220, 41–50. doi: 10.1016/j.rse.2018.10.032
O’Leary, B. C., Winther-Janson, M., Bainbridge, J. M., Aitken, J., Hawkins, J. P., and Roberts, C. M. (2016). Effective coverage targets for ocean protection. Conserv. Lett. 9, 398–404. doi: 10.1111/conl.12247
O’Neill, J. D., and Costa, M. (2013). Mapping eelgrass (Zostera marina) in the Gulf Islands National Park Reserve of Canada using high spatial resolution satellite and airborne imagery. Remote Sens. Environ. 133, 152–167. doi: 10.1016/j.rse.2013.02.010
Oesterwind, D., Rau, A., and Zaiko, A. (2016). Drivers and pressures - Untangling the terms commonly used in marine science and policy. J. Environ. Manag. 181, 8–15. doi: 10.1016/j.jenvman.2016.05.058
Oliver, E. C. J., Benthuysen, J. A., Darmaraki, S., Donat, M. G., Hobday, A. J., Holbrook, N. J., et al. (2021). Marine Heatwaves. Ann. Rev. Mar. Sci. 13, 313–342. doi: 10.1146/annurev-marine-032720-095144
Pfister, C. A., Altabet, M. A., and Weigel, B. L. (2019). Kelp beds and their local effects on seawater chemistry, productivity, and microbial communities. Ecology 100:e02798. doi: 10.1002/ecy.2798
Pfister, C. A., Berry, H. D., and Mumford, T. (2018). The dynamics of Kelp Forests in the Northeast Pacific Ocean and the relationship with environmental drivers. J. Ecol. 106, 1520–1533. doi: 10.1111/1365-2745.12908
Pomeroy, R. S., Watson, L. M., Parks, J. E., and Cid, G. A. (2005). How is your MPA doing? A methodology for evaluating the management effectiveness of marine protected areas. Ocean Coast. Manag. 48, 485–502. doi: 10.1016/j.ocecoaman.2005.05.004
Rassweiler, A., Okamoto, D. K., Reed, D. C., Kushner, D. J., Schroeder, D. M., and Lafferty, K. D. (2021). Improving the ability of a BACI design to detect impacts within a kelp-forest community. Ecol. Appl. 31:e02304. doi: 10.1002/eap.2304
Reed, D. (2014). SBCLTER: Reef: Historical Kelp Database for giant kelp (Macrocystis pyrifera) Biomass In California And Mexico Ver 13. Environmental Data Initiative.
Reshitnyk, L., Costa, M., Robinson, C., and Dearden, P. (2014). Evaluation of WorldView-2 and acoustic remote sensing for mapping benthic habitats in temperate coastal Pacific waters. Remote Sens. Environ. 153, 7–23. doi: 10.1016/j.rse.2014.07.016
Rodriguez, G. E., Reed, D. C., and Holbrook, S. J. (2016). Blade life span, structural investment, and nutrient allocation in giant kelp. Oecologia 182, 397–404. doi: 10.1007/s00442-016-3674-6
Rogers-Bennett, L., and Catton, C. (2019). Marine heat wave and multiple stressors tip bull kelp forest to sea urchin barrens. Sci. Rep. 9:15050. doi: 10.1038/s41598-019-51114-y
Rossiter, T., Furey, T., McCarthy, T., and Stengel, D. B. (2020). UAV-mounted hyperspectral mapping of intertidal macroalgae. Estuar. Coast. Shelf Sci. 242:106789. doi: 10.1016/j.ecss.2020.106789
Saarman, E., Gleason, M., Ugoretz, J., Airamé, S., Carr, M., Fox, E., et al. (2013). The role of science in supporting marine protected area network planning and design in California. Ocean Coast. Manag. 74, 45–56. doi: 10.1016/j.ocecoaman.2012.08.021
Santer, R., and Schmechtig, C. (2000). Adjacency effects on water surfaces: primary scattering approximation and sensitivity study. Appl. Opt. 39:361–375. doi: 10.1364/AO.39.000361
Schiel, D. R., and Foster, M. S. (2015). The Biology And Ecology Of Giant Kelp Forests. Berkeley, CA: University of California Press. doi: 10.1525/california/9780520278868.001.0001
Schroeder, S. B., Boyer, L., Juanes, F., and Costa, M. (2020). Spatial and temporal persistence of nearshore kelp beds on the west coast of British Columbia, Canada using satellite remote sensing. Remote Sens. Ecol. Conserv. 6, 327–343. doi: 10.1002/rse2.142
Schroeder, S. B., Dupont, C., Boyer, L., Juanes, F., and Costa, M. (2019). Passive remote sensing technology for mapping bull kelp (Nereocystis luetkeana): a review of techniques and regional case study. Glob. Ecol. Conserv. 19:e00683. doi: 10.1016/j.gecco.2019.e00683
Smale, D. A. (2020). Impacts of ocean warming on kelp forest ecosystems. New Phytologist 225, 1447–1454. doi: 10.1111/nph.16107
Smith, J. G., Tomoleoni, J., Staedler, M., Lyon, S., Fujii, J., and Tinker, M. T. (2021). Behavioral responses across a mosaic of ecosystem states restructure a sea otter-urchin trophic cascade. Proc. Natl. Acad. Sci. U.S.A. 118:e2012493118. doi: 10.1073/pnas.2012493118
Springer, Y. P., Hays, C. G., Carr, M. H., and Mackey, M. R. (2010). Toward ecosystem-based management of marine macroalgae—the bull kelp Nereocystis luetkeana. Oceanogr. Mar. Biol. Annu. Rev. 48, 1–42. doi: 10.1201/ebk1439821169-1
Stekoll, M. S., Deysher, L. E., and Hess, M. (2006). A remote sensing approach to estimating harvestable kelp biomass. J. Appl. Phycol. 18, 323–334. doi: 10.1007/s10811-006-9029-7
Sutherland, I. R., Karpouzi, V., Mamoser, M., and Carswell, B. (2008). Kelp inventory, 2007: Areas of the British Columbia Central Coast From Hakai Passage To The Bardswell Group. British Columbia, BC: Ministry of Environment, Oceans and Marine Fisheries Branch.
Tait, L., Bind, J., Charan-Dixon, H., Hawes, I., Pirker, J., and Schiel, D. (2019). Unmanned aerial vehicles (uavs) for monitoring macroalgal biodiversity: comparison of rgb and multispectral imaging sensors for biodiversity assessments. Remote Sens. 11:2332. doi: 10.3390/rs11192332
Turner, N. J. (2001). “Coastal peoples and marine plants on the Northwest Coast,” in Proceedings of the International Association of Aquatic and Marine Science Libraries and Information Centers. Victoria, British Columbia.
Uhl, F., Bartsch, I., and Oppelt, N. (2016). Submerged kelp detection with hyperspectral data. Remote Sens. 8:487. doi: 10.3390/rs8060487
Ulaski, B. P., Konar, B., and Otis, E. O. (2020). Seaweed reproduction and harvest rebound in southcentral alaska: implications for wild stock management. Estuar. Coast. 43, 2046–2062. doi: 10.1007/s12237-020-00740-1
UNEP (2010). Report of the Tenth Meeting of the Conference of the Parties to the Convention on Biological Diversity. Nagoya: UNEP.
Vahtmäe, E., Paavel, B., and Kutser, T. (2020). How much benthic information can be retrieved with hyperspectral sensor from the optically complex coastal waters? J. Appl. Remote Sens. 14:16504. doi: 10.1117/1.JRS.14.016504
Veisze, P., Kilgore, A., and Lampinen, M. (2001). “Building a California kelp knowledge database using GIS,” in Page Proceedings of the 2001 ESRI users, San Diego.
Vestbo, S., Obst, M., Quevedo Fernandez, F. J., Intanai, I., and Funch, P. (2018). Present and potential future distributions of Asian horseshoe crabs determine areas for conservation. Front. Mar. Sci. 5:164. doi: 10.3389/fmars.2018.00164
Villaseñor-Derbez, J. C., Aceves-Bueno, E., Fulton, S., Suarez, A., Hernández-Velasco, A., Torre, J., et al. (2019). An interdisciplinary evaluation of community-based TURF-reserves. PLoS One 14:e0221660. doi: 10.1371/journal.pone.0221660
Wernberg, T., Bennett, S., Babcock, R. C., De Bettignies, T., Cure, K., Depczynski, M., et al. (2016). Climate-driven regime shift of a temperate marine ecosystem. Science 353, 169–172. doi: 10.1126/science.aad8745
Yamazaki, F., Liu, W., and Takasaki, M. (2009). “Characteristics of shadow and removal of its effects for remote sensing imagery,” in Proceedings of the Page International Geoscience and Remote Sensing Symposium (IGARSS). (Cape Town: IEEE). doi: 10.1109/IGARSS.2009.5417404
Keywords: kelp forest, remote sensing, North America, coastal management, kelp management, bull kelp, giant kelp
Citation: Cavanaugh KC, Bell T, Costa M, Eddy NE, Gendall L, Gleason MG, Hessing-Lewis M, Martone R, McPherson M, Pontier O, Reshitnyk L, Beas-Luna R, Carr M, Caselle JE, Cavanaugh KC, Flores Miller R, Hamilton S, Heady WN, Hirsh HK, Hohman R, Lee LC, Lorda J, Ray J, Reed DC, Saccomanno VR and Schroeder SB (2021) A Review of the Opportunities and Challenges for Using Remote Sensing for Management of Surface-Canopy Forming Kelps. Front. Mar. Sci. 8:753531. doi: 10.3389/fmars.2021.753531
Received: 05 August 2021; Accepted: 28 September 2021;
Published: 20 October 2021.
Edited by:
Caren Barceló, Office of Science and Technology, NOAA Fisheries, United StatesReviewed by:
Tim Glasby, New South Wales Department of Primary Industries, AustraliaSu Yin Chee, Universiti Sains Malaysia, Malaysia
Copyright © 2021 Cavanaugh, Bell, Costa, Eddy, Gendall, Gleason, Hessing-Lewis, Martone, McPherson, Pontier, Reshitnyk, Beas-Luna, Carr, Caselle, Cavanaugh, Flores Miller, Hamilton, Heady, Hirsh, Hohman, Lee, Lorda, Ray, Reed, Saccomanno and Schroeder. This is an open-access article distributed under the terms of the Creative Commons Attribution License (CC BY). The use, distribution or reproduction in other forums is permitted, provided the original author(s) and the copyright owner(s) are credited and that the original publication in this journal is cited, in accordance with accepted academic practice. No use, distribution or reproduction is permitted which does not comply with these terms.
*Correspondence: Kyle C. Cavanaugh, a2NhdmFuYXVnaEBnZW9nLnVjbGEuZWR1