- 1Instituto de Ciencias Marinas y Pesquerías (ICIMAP), Universidad Veracruzana, Veracruz, Mexico
- 2Laboratorio de Mamíferos Marinos (LabMMar-IIB-ICIMAP), Universidad Veracruzana, Veracruz, Mexico
- 3Instituto de Investigaciones Biológicas, Universidad Veracruzana, Xalapa, Mexico
- 4Museu Oceanografico Prof. Eliézer de C. Rios, Universidade Federal do Rio Grande/FURG, Rio Grande, Brazil
- 5Kaosa, Rio Grande, Brazil
- 6Laboratório de Ecologia e Conservação da Megafauna Marinha (ECOMEGA), Instituto de Oceanografia, Universidade Federal do Rio Grande/FURG, Rio Grande, Brazil
The presence of transient and temporary individuals in capture-mark-recapture studies may violate the assumption on equal catchability, and thus yield biased estimates. We investigated the effects of residency patterns on population parameters of bottlenose dolphins inhabiting the coastal waters off the Alvarado Lagoon System (ALS), Veracruz, Mexico. We hypothesized that this population is open but there exists a “core community” that behaves as a closed population. Between 2006 and 2010, we conducted 75 photo-identification surveys and recorded 263 dolphin group encounters, in which 231 dolphins were identified. Individuals present during only one season, classified as transients (n = 85), were excluded from the study, and a standardized residency index (IH4) was computed for each dolphin that remained in the sample (n = 146). We used the K-means clustering method to split the sample into groups based on individual (seasonal, annual) IH4 values. These clusters were named as regular residents (RR, n = 55), occasional residents (OR, n = 45), and occasional visitors (OV, n = 46). The cumulative frequency of newly identified individuals displayed an asymptotic trend for the whole sample and all clusters, indicating that most of the individuals present in the study area during the study period were identified. The assumption of demographic closure was tested to define the core community, and was rejected for the whole sample and the OV cluster (p < 0.001 in both cases), indicating that the population is open. The closure assumption was not rejected for RR and OR clusters (χ2 = 6.88, DF = 13, p = 0.91, and χ2 = 17.8, DF = 16, p = 0.33, respectively), indicating that these clusters were demographically closed over the 5-year period. Thus, we defined this aggregation of individuals as the “core community”. The closed population model Mth indicated that the total abundance of this core community was 123 individuals (95% CI: 114–133). Our results provide quantitative evidence of the existence of a core community in open waters of the Gulf of Mexico, and points toward residency pattern as a main driver of population dynamics. These results highlight the importance of considering residency patterns when dealing with heterogeneity in the sample of a highly mobile species.
Introduction
In most wildlife populations, sex and age have profound effects on the chances of an individual dying, or producing offspring (Neal, 2004), thus these factors are routinely considered when studying population structure and dynamics (Ojasti and Dallmeier, 2000). Other factors with the potential to influence population structure are site fidelity (SF) and philopatry (Bose et al., 2017). SF has been defined as the “tendency to return to a previously occupied location” (Greenwood, 1980; Switzer, 1993), whereas philopatry is the tendency to stay in a familiar environment, mainly related to the natal habitat (Begon et al., 1995). Several environmental factors have been suggested to influence species’ site fidelity and/or philopatry, such as habitat stability, predictability of reproductive failure, variability in territory quality within a habitat, and population pressure; individual characteristics may also include an individual’s previous reproductive success, age, and knowledge of other sites (Switzer, 1993).
In cetacean studies, SF and residency patterns are evaluated using information on the number of recaptures, the duration of stay, and/or the average recurrence of individuals (see Ballance, 1990 and Morteo et al., 2012b). In this regard, Ballance (1990), stated that “a high number of resightings, a long period time between the first sighting and the last resighting, and a short time interval between adjacent sightings describes an animal with a high degree of residence.” Thus, individual residency patterns can be considered as a manifestation of SF and philopatry.
For cetacean social species such as delphinids, other factors that are thought to shape population and social structure are kinship and reproductive condition (Bigg, 1982; Wells et al., 1987). Sex and age also influence the way that delphinids use their habitats. In general, females try to ensure the survival of their offspring, thus their distribution is often closely related to the quality and/or accessibility of food resources and habitats that have a lower risk of predation; conversely, the distribution of males is often related more to female distribution than to the availability of food (Gowans et al., 2007). Delphinids routinely travel throughout the day and can be exposed to large areas, their entire home range, or even multiple habitats on a daily basis (Gowans et al., 2007). Consequently, individual home ranges -that may depend on the age and sex of the individual- result in structured societies that are usually stable over time (Wells et al., 1980, 1987), in which females tend to be more resident to specific areas than males (Gowans et al., 2007).
Since Ballance’s (1990) work, several methods have been used to evaluate cetacean residence and this lack of standardization hinders proper comparison among SF studies (Tschopp et al., 2018, see below). Tschopp et al. (2018) found that, in general, the methods to quantify site fidelity include three approaches: (1) proportions (a ratio between the number of sightings or resightings, and a measure of effort), (2) categories (e.g., high, moderate, low), and (3) models (maximum likelihood methods). Recently, Tschopp et al. (2018) developed and compared the performance of a series of standardized indexes -based on Ballance’s parameters- to be able to quantify the site fidelity degree and ensure accurate comparability across related investigations. Regarding delphinids, several authors (e.g., Gowans et al., 2007; Wells and Scott, 2018) have predicted that in offshore environments, where food availability is patchy and unpredictable and predation risk might be high, dolphins range more widely and form larger groups to forage on sparsely distributed prey schools and to reduce predation. In contrast, in complex inshore environments with predictable resource availability and potentially lower predation, dolphins are predicted to remain resident in relatively small areas and to form smaller groups to avoid feeding competition. This seems to be the case for the bottlenose dolphin (Tursiops truncatus) populations inhabiting semi-enclosed bays or sounds, or inshore habitats in the Gulf of Mexico (GoM). This species has been widely studied in the northern GoM on the basis of photo-ID methods and capture-mark-recapture (CMR) models (see review in Vollmer and Rosel, 2013). In the northern GoM, where long-term, year-round residents are best documented, there is also evidence for seasonal changes in abundance; most often these are thought to result from movements of seasonal, short-term residents and/or transients (very short-term visitors) to the area. It is generally thought that these shorter-term residents and visitors come from the adjacent coastal population, although comprehensive and directed studies to address this question have yet to be performed (Vollmer and Rosel, 2013).
The bottlenose dolphin has been widely documented in some localities in the southern GoM (Ortega Ortiz, 2002; Ortega Ortiz et al., 2004; Ramírez-León et al., 2020), including waters off the Tamiahua Lagoon System (Galindo et al., 2009; Martínez-Serrano et al., 2011; Valdés-Arellanes et al., 2011); Nautla (Morteo et al., 2019); and the Alvarado Lagoon System (ALS) in Veracruz state (Morteo, 2011; Morteo et al., 2012b, 2014, 2017, 2019; Morales-Rincón et al., 2019), and some coastal areas in the states of Tabasco, Campeche and Yucatán (Delgado-Estrella, 2015).
Off the ALS, Morteo et al. (2014) found that this population is sexually segregated, where females were more resident, had higher site fidelity, and had weaker associations with a higher number of partners than males. On the other side, males were assumed to be primarily responsible for gene flow among adjacent locations (Morteo et al., 2014).
Capture-mark-recapture (CMR) studies have been used as a general sampling and analysis method to assess population status and trends in many biological populations (White and Burnham, 1999). The photographic documentation of long-lasting natural marks on the dorsal fin or flukes (i.e., photo-identification, photo-ID) has been used since the early 1970’s to study aspects such as group structure, site fidelity, movement patterns, and abundance using CMR models in cetaceans (Würsig and Jefferson, 1990). Models usually require a set of restrictive assumptions about the properties of the population under study, such as individual homogeneity in capture and survival probabilities, among others (Krebs, 1972). Failure to comply with these assumptions implies that the model does not adequately fit the data, and thus may introduce severe bias in parameter estimates (Krebs, 1972). In structured delphinid populations, heterogeneity in capture or survival probabilities is often produced by age, sex, or size of the individuals (Williams et al., 1993), as well as inconspicuous marks, low photographic quality, and social bonds (Morteo et al., 2012a).
In recent studies, researchers started to include site fidelity and residency pattern as factors to account for heterogeneity in the data when studying demographics or population ecology of several cetacean species, including the southern Australian bottlenose dolphin (Tursiops cf australis, Zanardo et al., 2016; Passadore et al., 2017), the Australian humpback dolphin (Sousa sahulensis, Hunt et al., 2017), the Risso’s dolphin (Grampus griseus, Carlucci et al., 2020) and the fin whale (Balaenoptera physalus, Schleimer et al., 2019). These researchers calculated sighting rates and site fidelity indexes for each individual and then used agglomerative clustering methods (Legendre and Legendre, 1998) to identify clusters of individuals with similar degrees of site fidelity, before running CMR analysis. In particular, Haughey et al. (2020) used for the first time Tschopp et al. (2018) standardized indexes to stratify the sample and estimate population parameters of Indo-Pacific bottlenose dolphin (T. aduncus) off western Australia by residency pattern.
In this paper, we used the recently developed standardized site fidelity index (SSFI) IH4 (Tschopp et al., 2018) to study potential differences in population parameters between resident and non-resident common bottlenose dolphins (Tursiops truncatus) that use the open, coastal waters off Alvarado Lagoon System, south-western Gulf of Mexico. On the basis of our knowledge of the study area, we hypothesized that bottlenose dolphins using the marine coastal waters adjacent to the ALS are part of an open population within which exists a core community (sensu Wells et al., 1987), that behaves as a closed population, in the sense that it is composed of all the groups and individuals “… that share large portions of their ranges and interact with each other to a much greater extent than with members of similar units in adjacent waters, but genetics exchange occurrs between communities.”
Materials and Methods
For this study, we used the photo-ID catalog and database from the Marine Mammal Lab at Universidad Veracruzana (LabMMar-IIB-ICIMAP). This database includes the sighting histories of 231 bottlenose dolphins from 75 survey trips conducted between May 2006 and August 2010 (Morteo, 2011; Morteo et al., 2012b, 2014, 2017). Previously, Morteo et al. (2014, 2017) used a partial subset of these databases, covering the period May 2006–April 2008, to explore the abundance and social ecology of this dolphin population under open population modeling by considering the population as a homogeneous unit, and without considering temporal variations or population structure in the parameters. Detailed definitions of methodological terms are presented in Supplementary Material 1.
Study Area
The coastal waters of Alvarado, Veracruz, are shallow (less than 20 m), with an average temperature of 27°C (Morteo, 2011), and are strongly influenced by the discharge of the Alvarado Lagoon System (ALS, de la Lanza Espino and Lozano Montes, 1999; Cruz-Escalona et al., 2007). The ALS is a coastal wetland located in the center-south of the State of Veracruz, southwestern Gulf of Mexico, formed by the confluence of the Acula, Blanco, Limón, and -mainly- the Papaloapan rivers (Figure 1). The ALS has an elongated shape, parallel to the coastline, with an approximate length of 26 km, with a maximum width of 5 km and an average depth of 2.5 m, for a total area of about 80 km2 (de la Lanza Espino and Lozano Montes, 1999). The weather is tropical, with three marked climatic seasons; following Morteo (2011) and Morteo et al. (2014), we defined these seasons as: Dry, with a significant reduction in average precipitation, from March to June; Rainy, in which runoff causes high organic matter and nutrient input into coastal waters, from July to October, and, finally, the “Nortes” or “Northern Winds,” with strong winds associated to cold fronts which may last several days (de la Lanza Espino and Lozano Montes, 1999; Cruz-Escalona et al., 2007).
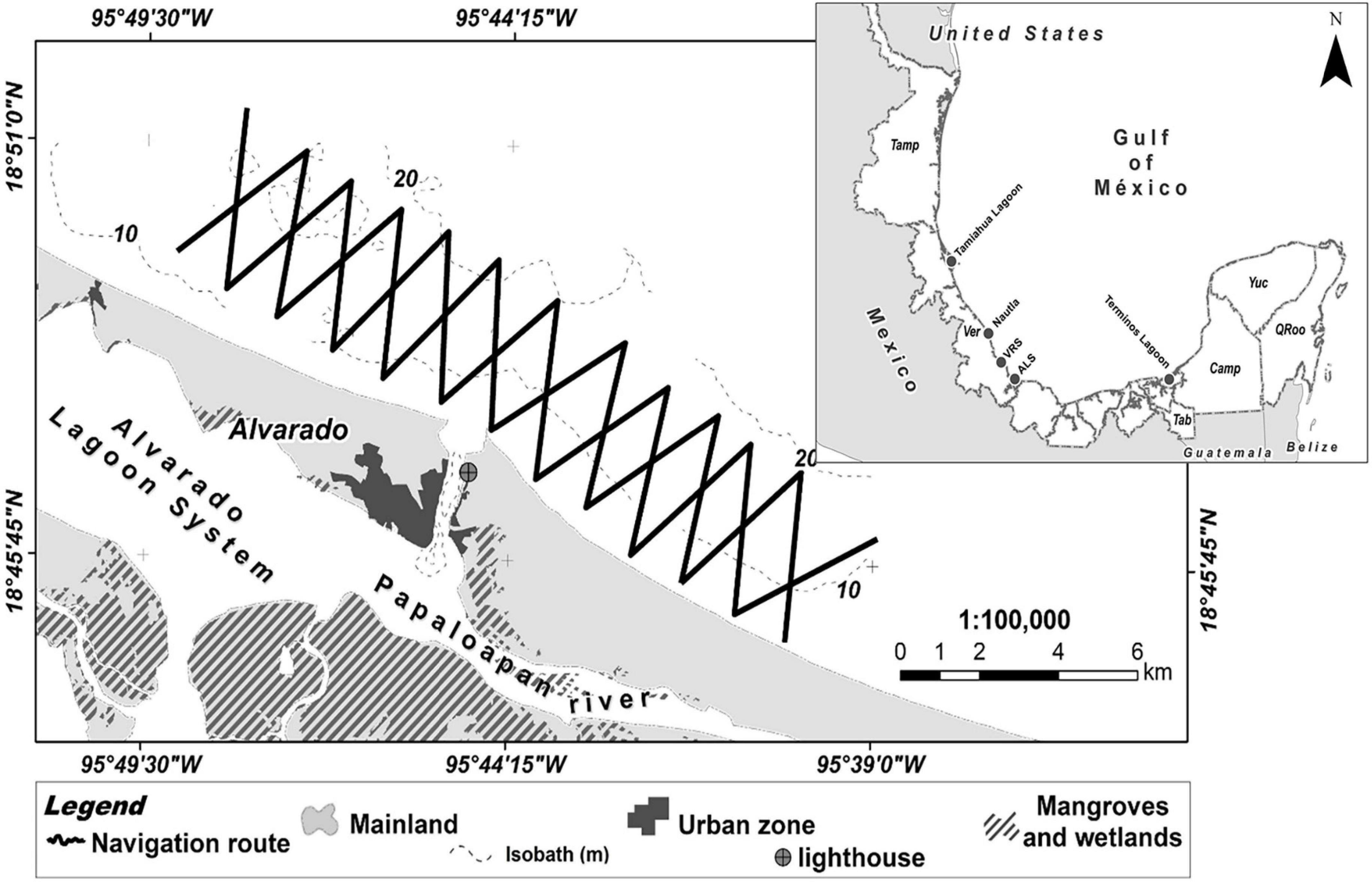
Figure 1. Location of the study area. ALS = Alvarado Lagoon System, Tam = Tamaulipas, Ver = Veracruz, Tab = Tabasco, Cam = Campeche, Yuc = Yucat n, QRoo = Quintana Roo. Nearby locations with studies on bottlenose dolphins are also shown (e.g., Tamiahua Lagoon, Nautla, VRS = Veracrus Reef System National Park). Zigzag lines indicate line transect surveys.
Survey Protocol
The survey protocol represents an extension of the procedures described in Morteo (2011), and Morteo et al. (2014, 2017). All sampling surveys were conducted following a zigzag pattern that started at the mouth of the ALS and extended 4 km offshore, and 9 km on each side of the estuary mouth (Morteo, 2011; Morteo et al., 2014, 2017, see Figure 1). Typically, all surveys covered the whole study area, and no surveys were conducted inside the lagoon system, based on previous data showing virtually no presence of dolphins (Morteo, pers. obs.).
Dolphin Photo-ID
Standard photo-ID techniques were used to individually identify and catalog adult dolphins based on long-lasting marks in their dorsal fins (Würsig and Jefferson, 1990; Morteo et al., 2014, 2017; Urian et al., 2015). All photographs were graded for quality (PQ) and dolphin distinctiveness (D). Following Urian et al. (2013, 2015), only excellent (PQ1) and good quality (PQ2) pictures of very (D1) and average (D2) distinctive dolphins were used for analysis, to minimize misidentification. We then plotted discovery curves for new identified individuals across time (Fisher et al., 1943; Colwell et al., 2004).
Mark Rate
A mark rate (θ), representing the proportion of marked animals in the groups, was calculated for each sighting to adjust abundance estimates by accounting for the unmarked fraction of the population, and thus produce estimates of seasonal abundances for the full population (Wilson et al., 1999; Wickman et al., 2020). Following Morteo et al. (2014, 2017), we used a combination of field and analytical approaches to accurately estimate group size and θ for each encounter. In the field, minimum, maximum, and best group size estimates were recorded, and the best estimate was used to calculate a mark rate for each sighting. By using the full dataset [N = 263 groups], we found that the average difference between the minimum and maximum group size estimates was 0.92 dolphins (± 3.70 SD) and this difference increased with larger groups. As the average group size for the full study period was fairly small (9.0 ± 11.2 SD) and the standard deviation of the difference between extreme field estimates was less than one individual, we considered that our group size estimates are highly accurate, and thus representative of the total number of animals in the group. Additionally, we used the empirical criteria developed independently by Würsig (1978) and Ballance (1990) to double-check dolphin counts on the field; this approach states that the probability of having photographed all the dolphins in a group is higher than 95% if all marked individuals in the sighting were correctly photographed at least four times (Ballance, 1990; Würsig and Jefferson, 1990).
Residency Pattern Assessment
Morteo et al. (2012b) reviewed the parameters proposed by Ballance (1990), suggested modifications, and established “Occurrence,” “Permanence” and “Periodicity,” as their names. The occurrence was redefined as the number of times the animal was recaptured (that is, eliminating the first sighting from the calculation); the permanence was defined as “the time over which an individual was recorded, determined by the difference between its first and last sighting,” whereas the periodicity was redefined as “the recurrence of the individual, determined by the inverse of the average time (in days) between consecutive recaptures.” Tschopp et al. (2018) used these definitions to evaluate the performance of their standardized indexes. For the purpose of this study, the periodicity was calculated by season and year.
We used the IH4 index of Tschopp et al. (2018) to split the sample into clusters according to individual’s site fidelity. We chose this index because it consistently had the best performance in all of the scenarios, and the authors proposed it as a standardized measure of site fidelity (SSFI). The IH4 is based on the harmonic mean of parameters permanence (IT) and periodicity (It), as expressed in equation 1:
Where: IT = Permanence
It = Periodicity
For each dolphin, we calculated the IH4 per season and year, to account for their presence in the study area across the studied period under different temporal stratifications. We then constructed a matrix (IH4-matrix) in which each line corresponded to an individual dolphin and columns included the two IH4 measures. We used the IH4–matrix to split the sample into groups by using the K-means clustering method (Legendre and Legendre, 1998). With the K-means method, a set of n objects (= dolphins) in a p-dimensional space (= IH4-matrix) can be partitioned into K groups -or clusters- such that the objects within each cluster are more similar to one another than to objects in other clusters; the number of groups (K) is often determined by the user based on expert knowledge (Legendre and Legendre, 1998), but there exist some numeric criteria to select the best grouping strategy (see below). The function cascade KM of the “vegan” package (Oksanen et al., 2019) of the R environment (R Core Team, 2020) was used to determine the groupings with the K-means method. The best-fitting K was selected by means of the Calinski-Harabasz index (ICH, Calinski and Harabasz, 1974; Legendre and Legendre, 1998) available in the vegan package. According to the ICH, the grouping with the highest index value corresponds to the best grouping given the data (Calinski and Harabasz, 1974; Oksanen et al., 2019). As we were interested in population analysis by the residency pattern, we excluded the transient individuals from the sample. We defined as transients those individuals that were present in the study area only during one season, independently of the number of recaptures during that season. Through the cluster analysis, we then compared the performance of 2 and 3 clusters (K), respectively.
Population Parameters
Estimates were made under standard CMR models in the program MARK (White and Burnham, 1999). Parameters can be established as time-variant or time-invariant, as well as variant or invariant among groups. Following White and Burnham (1999), the notation (.) was used to indicate time-invariant parameters and (t) to indicate those time-variant parameters. The notation (g) was used to indicate time-invariant parameters, different for each residency group, and (g*t) to indicate parameters different for each group and time-variant. The model(s) that best fitted the data were selected by using the lowest value of the Akaike Information Criterion, corrected for small samples (AICc, Akaike, 1973; White and Burnham, 1999). Before analyses, sighting histories were collapsed by season and stratified by residency pattern. We used two steps to estimate population parameters. In the first step, we used the POPAN superpopulation approach of the Jolly-Seber model (Schwarz and Arnason, 1996; White and Burnham, 1999) to determine survival, recruitment, and seasonal abundance of the dolphins according to their residency pattern under the assumption of open population. In the second step, we used closed capture-recapture methods available in the CAPTURE routine of the MARK program to determine the seasonal abundances of the core community only. Finally, total abundances were estimated as:
where Ntotal = total abundance, Nm = abundance of marked individuals, and θ = mark rate. The variance was calculated by using the Delta method (Wilson et al., 1999) as:
where n is the total number of individuals on which θ was estimated. The standard error was calculated as:
and then, log-normal 95 % confidence intervals (Burnham et al., 1987; Tezanos-Pinto et al., 2013) were calculated as:
where Nlower and Nupper are the lower and upper bound, respectively, of the confidence interval, and
where z0.025 is the normal deviate (1.96), and CV is the coefficient of variation.
Assumption Compliance and Parameter Estimations
Valid inference in CMR studies requires compliance with several assumptions (Pollock et al., 1990; White and Burnham, 1999). The U-CARE software (version 2.3.2, Choquet et al., 2009, 2005) was used to assess the goodness of fit of the model(s) to the data.
We used the POPAN parameterization of the Jolly-Seber model (Schwarz and Arnason, 1996) to get estimates for each group of apparent survival (Phi, hereafter survival), catchability (p), and probability of entrance of individuals from the superpopulation (hereafter recruitment) to the study area. Survival is termed “apparent” as the algorithm cannot discriminate between mortality and permanent emigration (White and Burnham, 1999). We used the Closed Population approach (Otis et al., 1978; Chao et al., 1992; Stanley and Burnham, 1999) to get estimates of the population size for the more resident fraction of the population. The closure assumption was confirmed with the closure test by Stanley and Burnham (1999), as provided by the Close Test software (Stanley and Burnham, 1999: Stanley and Richards, 2005). We applied these tests to the whole sample (n = 231 individuals), as well as to the clusters obtained through the k-means method. In all cases, the probability of first capture was set equal to the probability of recapture (p = c), as the process of taking photographs does not involve the physical capture of individuals so no behavioral effect is expected (Tezanos-Pinto et al., 2013).
Results
Between May 2006 and August 2010, we conducted 75 surveys, totaling 6,328.8 km surveyed, 288.1 h of search effort, and 110.4 h photographing dolphin groups (Table 1). Search effort ranged from 3.8 to 8.15 h day−1 (x̄ = 3.6 ± 1.1 SD) and the full study area was covered in each and all survey trips. In general, the effort was greater during the first two seasons, but we found no evidence of a difference in search effort among seasons (Kruskal-Wallis, H = 0.91, p = 0.63). Photo-ID effort (h day–1) ranged from 0.0 to 3.6 (mean 1.4 ± 0.8 SD), and no seasonal differences were found (Kruskal-Wallis, H = 10.55, p = 0.10).
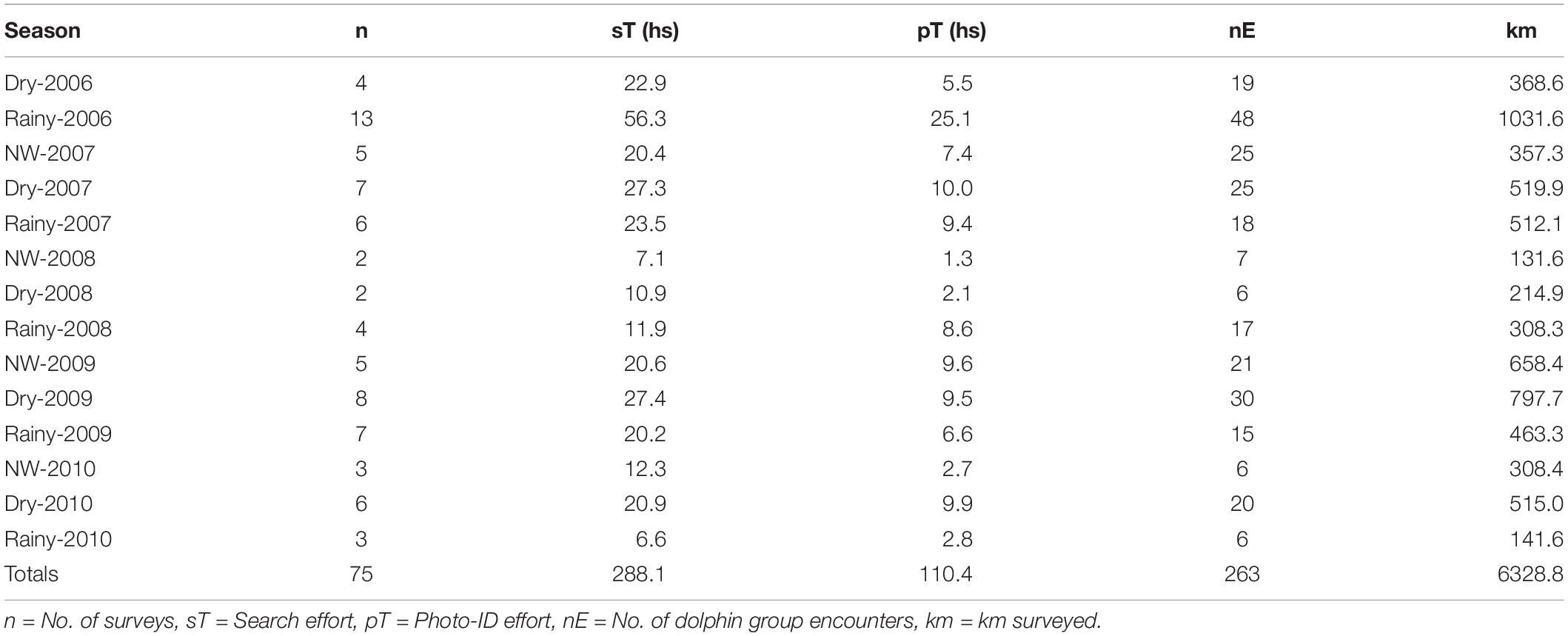
Table 1. Summary of survey and photo-identification effort of bottlenose dolphins off Alvarado Veracruz System, south-western Gulf of Mexico.
Two hundred and sixty-three groups were encountered totaling 2,320 dolphins sampled, of which 231 distinct individuals were identified. We processed 30,402 pictures of which 10,958 (36%) were useful for photo-ID. Group size averaged 9.0 individuals (± 11.2 SD, range: 1–100), and remained similar among seasons (Kruskal-Wallis, H = 2.62, p = 0.27). The number of recaptures for marked dolphins ranged from 0 to 12 occasions, where 36.8 % (n = 85) were transients (Figure 2).
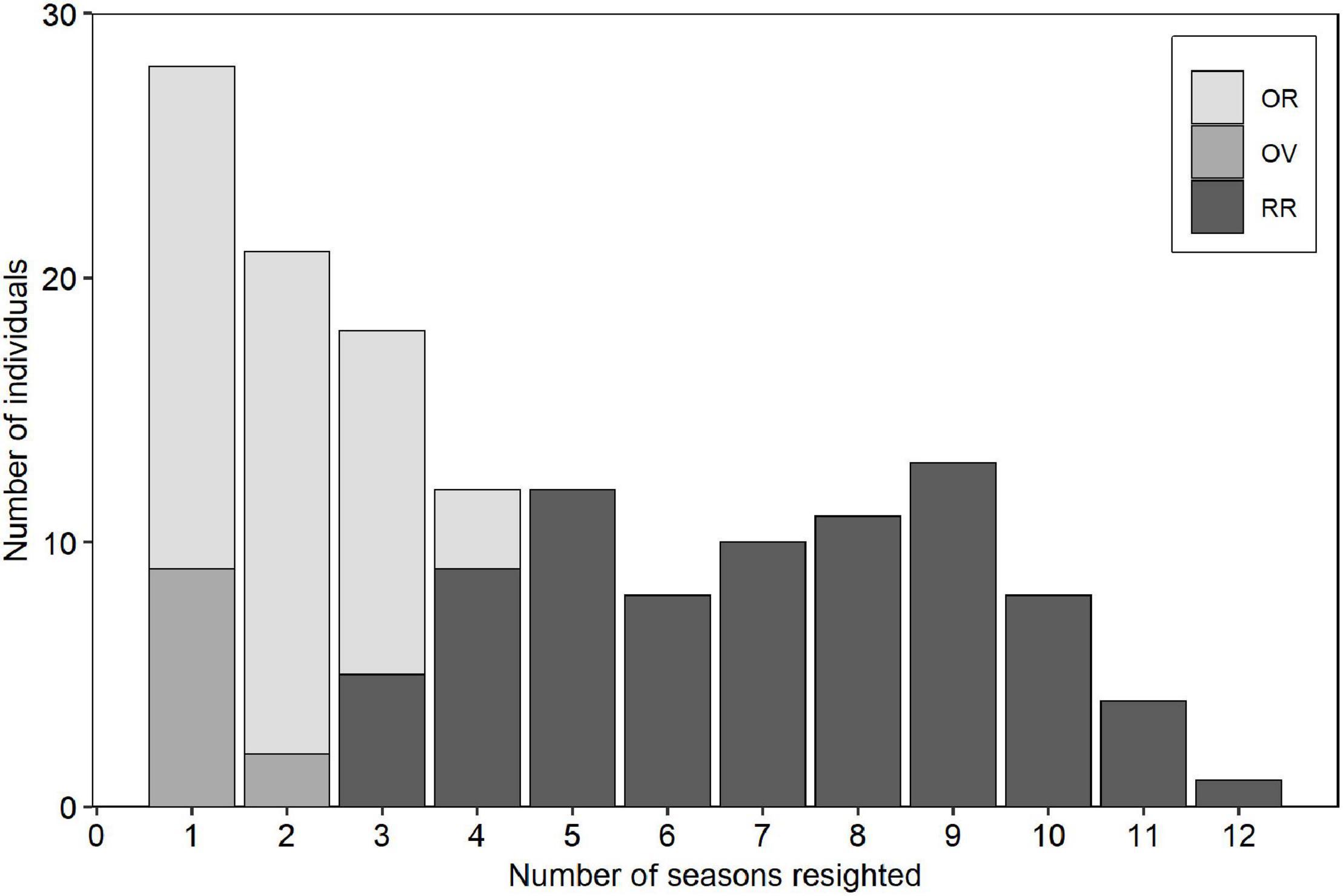
Figure 2. Frequency of individual resightings of bottlenose dolphins off the Alvarado Lagoon between May 2006 through August 2010 (n = 146 distinct adult dolphins). RR = regular residents, OR = occasional residents, OV = occasional visitors.
Clustering of Individuals
The ICH (Calinski and Harabasz, 1974) indicated that K = 3 (ICH = 389.4) was the best grouping strategy according to the data. Cluster 1 consisted of 46 individuals with low seasonal (mean = 0.27 ± 0.08 SD) and annual (mean = 0.31 ± 0.18 SD) site fidelity indexes. These individuals were resighted between 1 and 4 seasons. Cluster 2 comprised 45 individuals with medium seasonal (mean = 0.49 ± 0.10 SD) and annual (mean = 0.76 ± 0.10 SD) site fidelity indexes; these individuals were resighted between 2 and 8 seasons. Cluster 3 consisted of 55 individuals with high seasonal (mean = 0.79 ± 0.09 SD) and annual (mean = 0.96 ± 0.06 SD) site fidelity indexes; these individuals were recaptured between 5 and 12 seasons. Following Zanardo et al. (2016) and Passadore et al. (2017) these clusters were defined as “occasional visitors” (OV), “occasional residents” (OR), and “regular residents” (RR), respectively (Figures 3, see definitions in Supplementary Material 1).
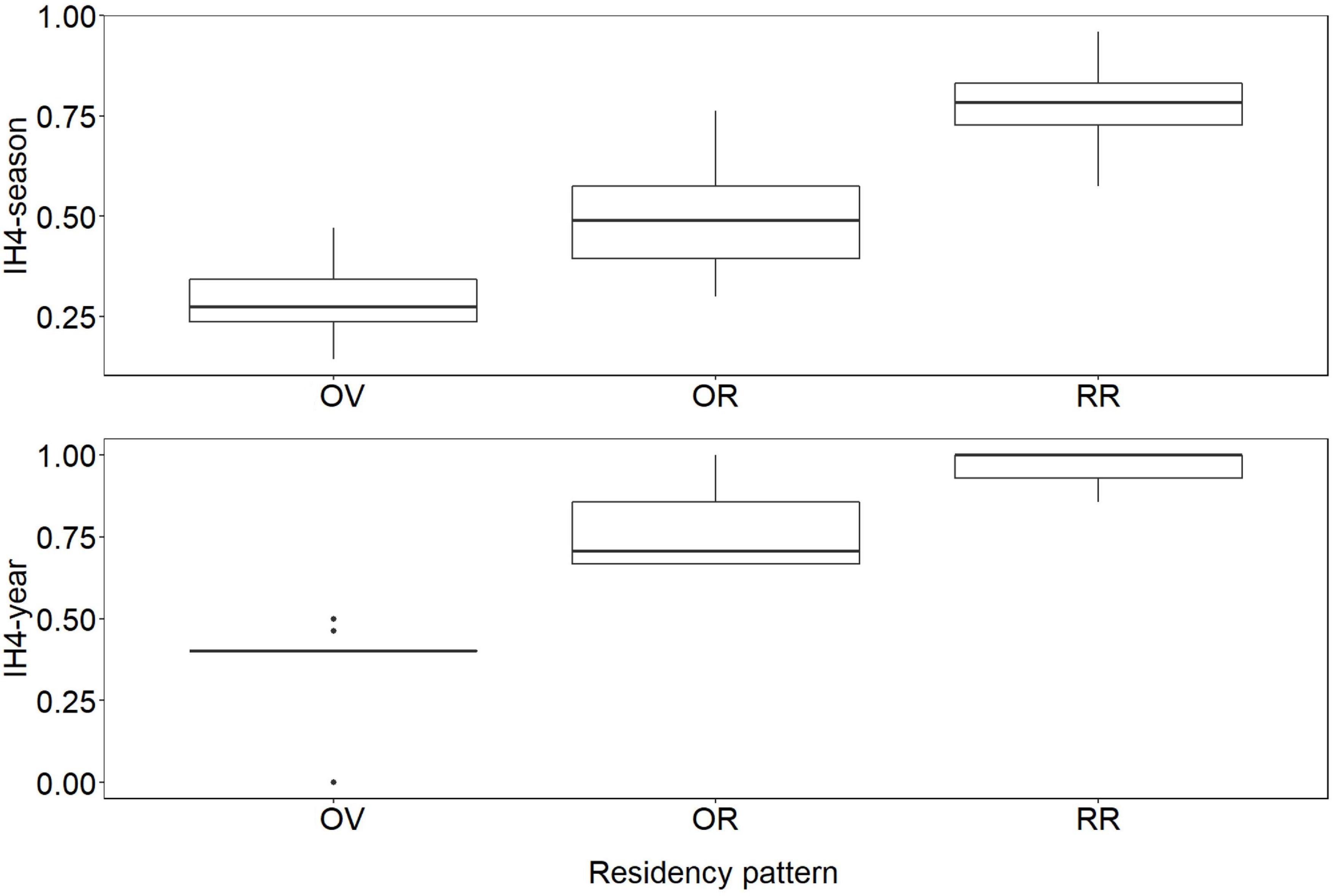
Figure 3. Boxplot of IH4 values, by season and year, according to the residency pattern. OV = occasional visitors (n = 46), OR = occasional residents (n = 45), RR = regular residents (n = 55).
The Kruskal-Wallist test showed that there were differences in the medians of the IH4 values among groups (IH4-season: p < 0.001, IH4-year: p < 0.001). The Mann-Whitney pairwise post-hoc test showed that the medians of the groups were different (IH4-season: p < < 0.001; IH4-year: p < < 0.001, in all cases), indicating differences in the time spent in the study area for individuals classified in different clusters.
Discovery Curves
The cumulative frequency of newly identified individuals by residency pattern displayed an asymptotic trend in all cases (Figure 4), indicating that most of the individuals present in the study area for the duration of the study were identified. For RR and OR individuals, the curve reached a plateau within the first seasons of our study. Additionally, for OV, transients (TR), and the whole sample, the curves presented two plateaus, indicating at least one occasional pulse of incorporation of individuals to the study area (Figure 4).
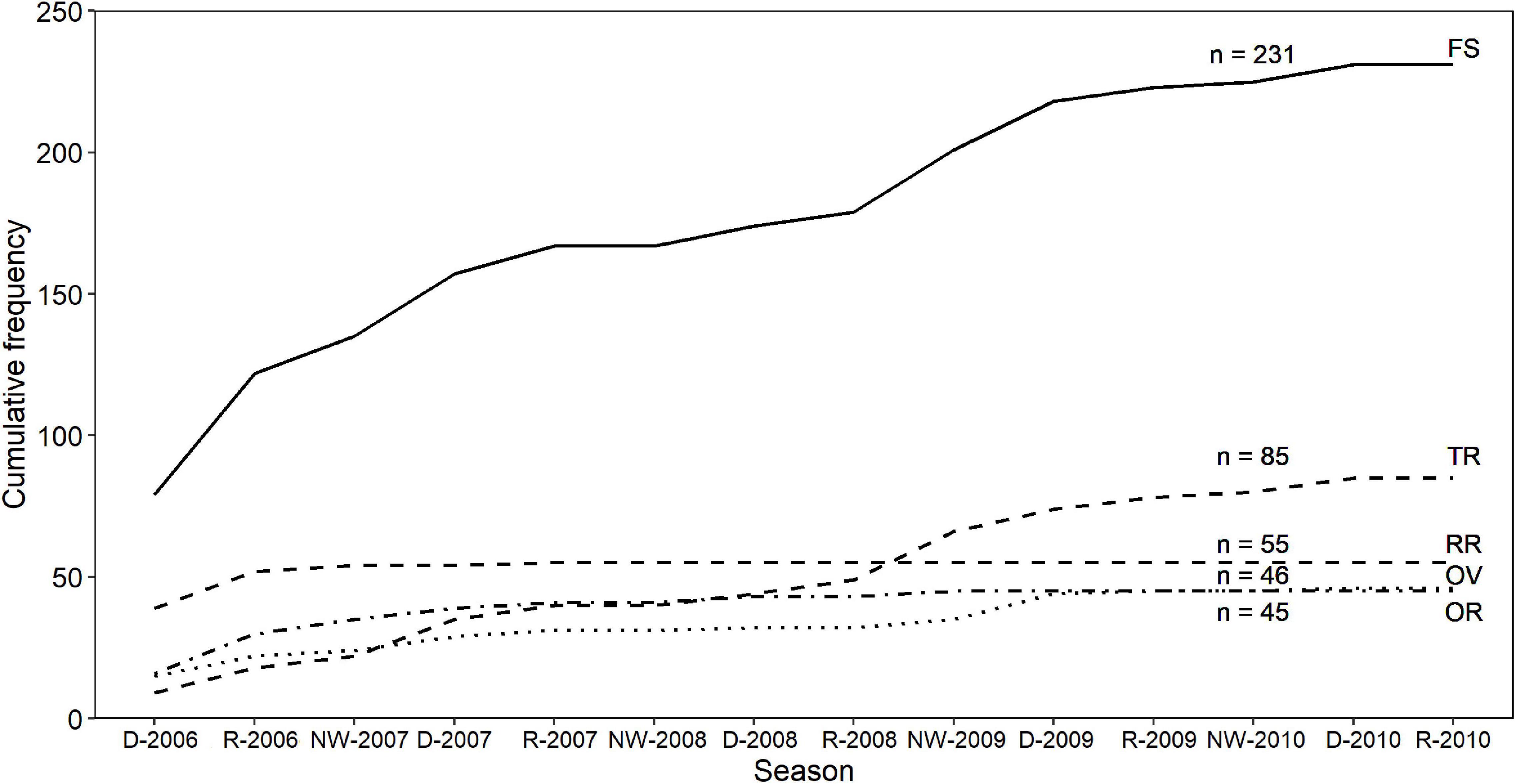
Figure 4. Discovery curve of the cumulative frequency of newly identified bottlenose dolphins off the Alvarado Lagoon System, between 2006 and 2010, by residency pattern. FS = full sample, TR = transients, RR = regular residents, OV = occasional visitors, OR = occasional residents, NW = Northern Winds. Number of individuals belonging to each cluster are indicated.
Goodness of Fit Tests and Model Selection
The global test for the sample stratified by residency pattern (excluding transients) was non-significant (χ2 = 70.1, D.F. = 78, P-value = 0.73), indicating compliance with the model assumptions.
The most parsimonious model (AICc = 1810.9; AICc weight = 0.83) indicated that survival was time-invariant, different among groups, and that both catchability and recruitment were time-variant and group-variant [Phi(g), p(g*t) pent(g*t), model 1 in Table 2]. Formulations with invariant recruitment [pent(g), pent(.) or equal for all groups pent(t)] failed to converge or presented unrealistic values or null standard error, thus they were not included in the analyses.
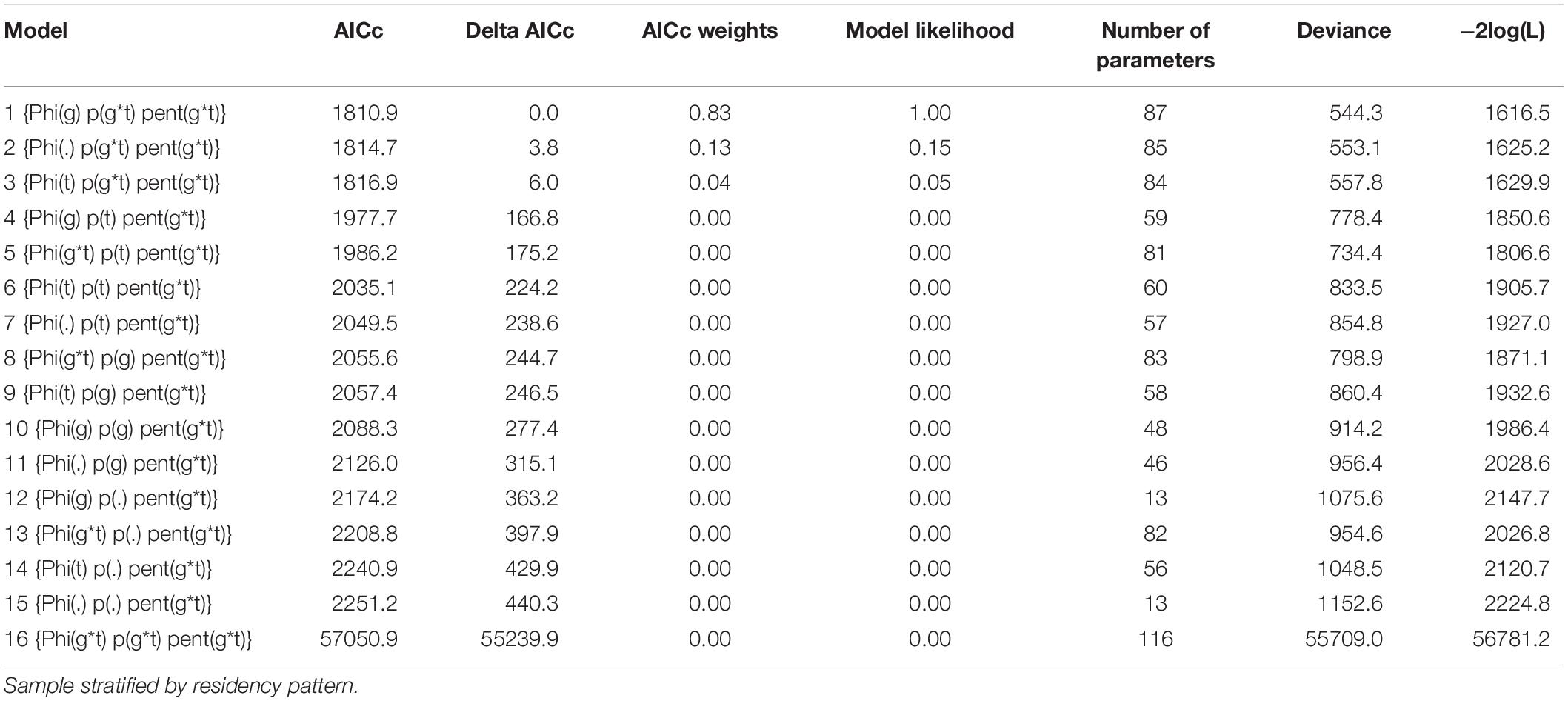
Table 2. Set of candidate models arranged in ascending order by AICc for population analysis of the bottlenose dolphin off Alvarado Veracruz System, southwestern Gulf of Mexico, between 2006 and 2010.
Population Parameters
Catchability (Figure 5) for RR (mean 0.67 ± 0.25 SD) was higher than for OR (0.35 ± 0.20 SD) and OV (mean 0.33 ± 0.27 SD) (Anova, F = 8.64, p = 0.0008).
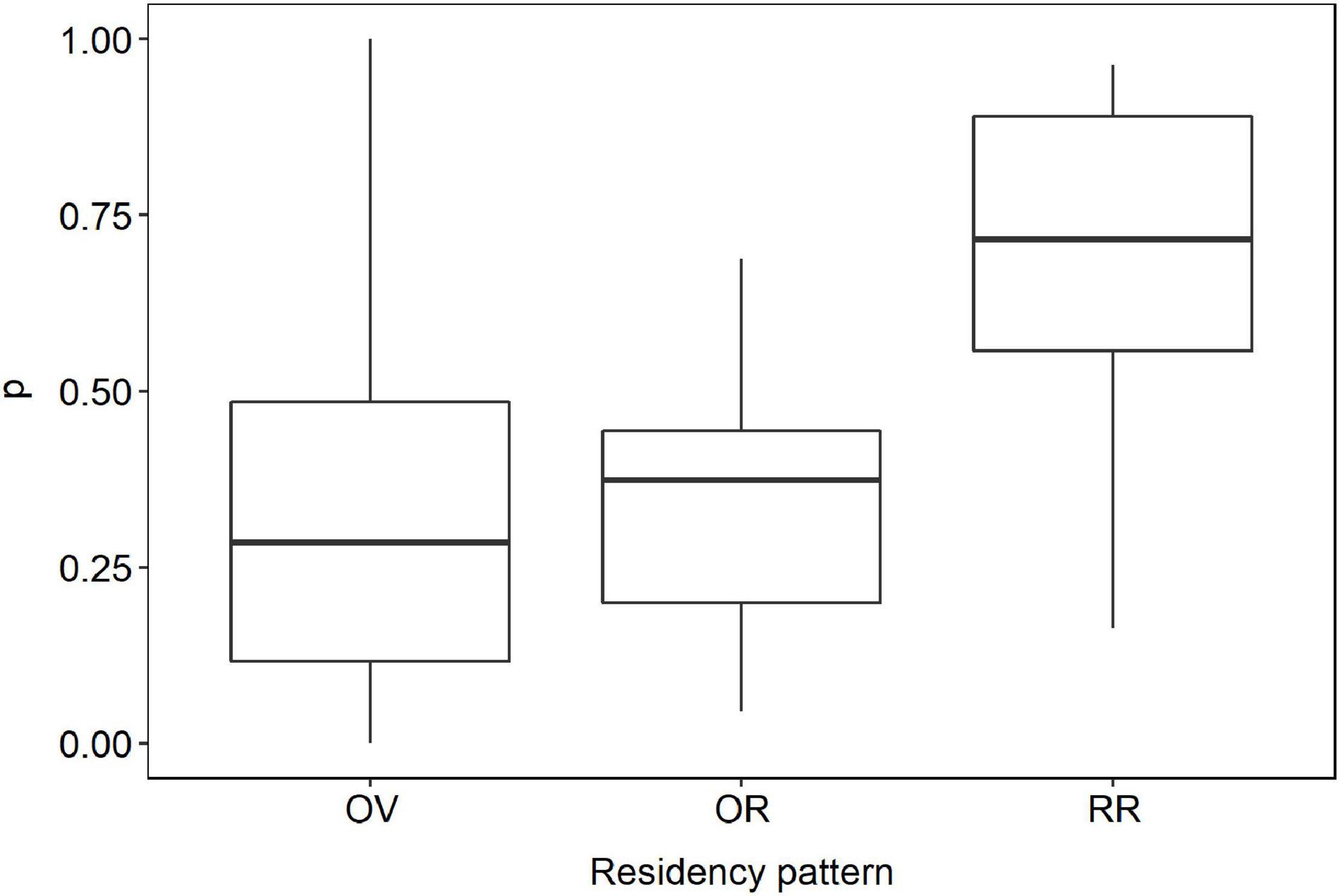
Figure 5. Catchability of the bottlenose dolphin (T. truncatus) in the coastal waters off Alvarado Lagoon System according to their residency pattern. OV = occasional visitors (n = 46), OR = occasional residents (n = 45), RR = regular residents (n = 55).
Apparent Survival and Recruitment
According to the most parsimonious model, seasonal survival was time-invariant and resulted in 1.00 (95% CI: 1.00–1.00) for RR and OR, and 0.91 (95% CI: 0.85–0.95) for OV individuals. When scaled to represent annual rates (see Cooch and White, 2019, p. 4.27), survival was 1.00 (95% CI: 1.00–1.00) for RR and OR, and 0.75 (95% CI: 0.61–0.86) for OV. Recruitment for the duration of the study was 0.72, 0.20, and 0.02 for OV, OR, and RR clusters, respectively, such that 28%, 80%, and 98% of OV, OR, and RR individuals, respectively, were already present in the population just before the beginning of the study (Table 3).
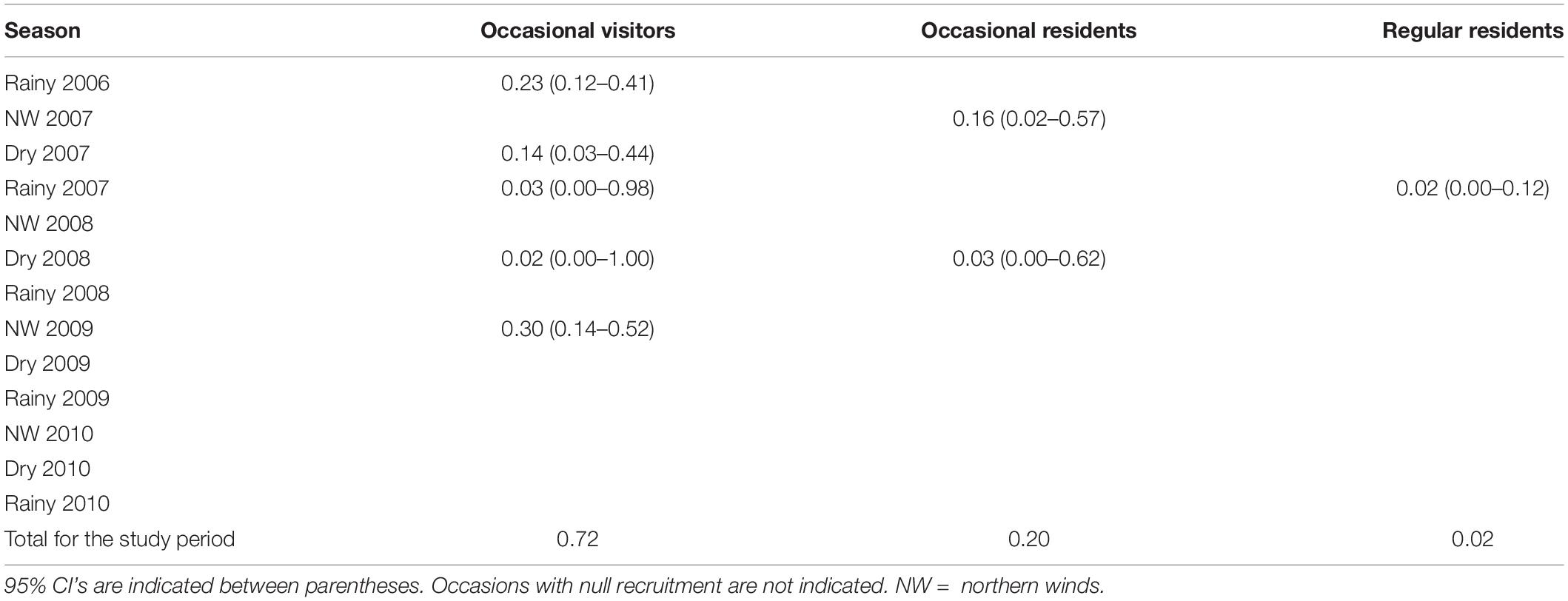
Table 3. Recruitment from the superpopulation of the bottlenose dolphin off the Alvarado Lagoon System, southwestern Gulf of Mexico, 2006–2010.
Occasional visitors were recruited in up to five out of 14 sampling occasions, with peaks in the rainy season of 2006 (23%), and the northern winds season of 2009 (30%). Occasional residents were recruited only during the NW 2007 and Dry 2008 seasons, with the highest percentage in the dry season of 2007 (16 %). Regular residents were recruited only in the Rainy season of 2007, but in a low proportion (2%).
The high survival values of the RR and OR clusters, as well as the null and low recruitment rates of RR and OR individuals, respectively, were consistent with the assumption of demographic or geographical closure for these clusters. As stated earlier, additional analyses were conducted to determine abundance for the core community, under the assumption of closed populations (see below).
Abundance
All abundances were adjusted by θ to include the unmarked fraction of the population. The total abundance of occasional visitors averaged 34 individuals (± 6.0 D.E., range: 18–42). The abundance of occasional residents averaged 57 (± 7.3 D.E., range: 44–76), and regular residents averaged 72 (± 7.2 D.E., range: 65–93) dolphins. A regression analysis indicated a low, positive, non-significant trend in the seasonal abundance of OV individuals (p = 0.09, R2 = 0.21). The residual plot of abundances for all clusters showed a non-random pattern (see Supplementary Material 2).
Closed Population Approach
The closure assumption was rejected for the whole sample and the OV cluster (p < 0.001 in both cases), however, this was not the case for the RR and OR clusters (χ2 = 6.88, DF = 13, p = 0.91, and χ2 = 17.8, DF = 16, p = 0.33, respectively). Thus, we defined the aggregation of these clusters as the “core community” (Wells et al., 1987), and then used closed models in MARK to determine its abundance by means of the Mth formulation (Chao et al., 1992), which includes heterogeneity (Chao et al., 1992; White and Burnham, 1999; Cooch and White, 2019). We chose the Mth estimator (Chao et al., 1992) because the sampling protocol does not require the animals to be physically captured, and because of the heterogeneity in the capture probabilities of the OR an RR clusters (see section “Population parameters”). According to the Mth formulation, the abundance of marked adult individuals (RR+OR) was 100 individuals (SE = 0.21). When corrected for the average mark rate (0.81 ± 0.17 SD), total abundance of the core community was 123 individuals (95% CI: 114–133). Interestingly, the closure test for the “core community” (OR+RR) was rejected (χ2 = 49.5, DF = 16, p = 0.00003), indicating a potential violation of this assumption; nevertheless, as both OR and RR clusters separately complied with the closure assumption, we believe that the significant result is an artifact of the different capture probabilities of each cluster (0.35 and 0.67, respectively). In this regard, it is well-known that heterogeneity in capture probabilities can cause both the tests of immigration and emigration to reject the null hypothesis of closure (Cooch and White, 2019, p. 14.6).
Discussion
Population structure of highly mobile marine organisms can be complex and difficult to study, but it is important to understand how individuals within a population partition their environment, in order to better address conservation challenges (Vollmer et al., 2021). In this regard, recent advancements in the implementation of standardized site fidelity indexes (Tschopp et al., 2018) and considerations on residency patterns when studying cetacean population dynamics (e.g., Zanardo et al., 2016; Hunt et al., 2017; Passadore et al., 2017; Schleimer et al., 2019; Carlucci et al., 2020; Haughey et al., 2020) provide improved methodological tools for dealing with heterogeneity in capture or survival probabilities inherent to the dynamics of social species with fission-fusion societies such as bottlenose dolphins (Connor et al., 2000). This is the first study to explore the population dynamics of the common bottlenose dolphin (T. truncatus) in the Gulf of Mexico based on its residency patterns, and to provide quantitative evidence of the existence of a “core community” (sensu Wells et al., 1987) in an open, coastal habitat. As this study is an extension of previous work by Morteo (2011) and Morteo et al. (2014, 2017), we included new data on previously unknown parameters, improving the temporal coverage as well as a more comprehensive analytical approach that yielded more refined estimates, and a more robust assessment of the population structure.
Due to the longevity of bottlenose dolphins, we acknowledge that short-term studies such as this one may not encapsulate the population processes related to factors such as mortality or emigration (Haughey et al., 2020). Despite the high number of transient individuals in the sample (Figure 2), the asymptotic trend in the discovery curves (Figure 4) indicated that most of the marked individuals in the population had been photographed by the end of the study period. For the full sample, the existence of two plateaus and decrease in the discovery rate of new individuals after the rainy season of 2009 suggest that the addition of new members is low, with sporadic immigration pulses as occurs in this, and other areas within the southern Gulf of Mexico (e.g., Martínez-Serrano et al., 2011; Valdés-Arellanes et al., 2011; Morteo et al., 2014, 2017, 2019; Delgado-Estrella, 2015). On the other side, the similarity between the discovery curves of the whole sample, occasional visitors, and transients showed that the dynamics of this population is mainly regulated by the influx of non-resident individuals.
Catchability of RR (mean 0.67 ± 0.27 SD) individuals was greater than for OR (0.35 ± 0.20) and OV (0.32 ± 0.27 SD) individuals, and thus abundance estimates for this cluster will be more precise (Williams et al., 2002). In general, catchability should be directly related to research effort alone but, in this case, it probably reflects that regular residents are more catchable by unit of effort than individuals in any other clusters (Williams et al., 2002).
High values of annual survival for the core community (cluster RR + OR) (1.00, 95% CI: 1.00–1.00) contrast sharply to those of occasional visitors: (0.75, 95% CI: 0.59–0.87). As transients were not included in the analysis, and RR and OR clusters behave as a closed population, these highest values for the core community are most probably the result of both a high survival rate and a very low emigration rate.
For long-lived species with complex life history processes, such as cetaceans, adult survival is expected to be high and variable with age, sex, and individual fitness (Ralls et al., 1980). Higher survival rates for resident individuals were indirectly assumed by Morteo et al. (2012b), who showed that these animals interact less frequently with fisheries, when compared to non-residents, thus facing lower exposure to entanglements in fishing gear and retaliation measures by fishers. Therefore, although much lower survival estimates for the visitor fraction is largely based on a high proportion of dolphins permanently leaving the study area shortly after the sampling, it is likely that higher mortality for non-residents is also due to a greater risk of predation by sharks and higher risk of entanglement in fishing gear (Morteo et al., 2012b, 2014, 2017; Rechimont et al., 2018; Morales-Rincón et al., 2019). The latter is supported by reports from recently stranded animals – recorded between 2002 and 2019 (decomposition code 1–3, according to Geraci and Lounsbury, 1993) – showing that fisheries-related injuries only occurred in individuals that had not been previously photographed in the study area (Fuentes Del Muro and Morteo, unpublished data, LabMMar-UV).
Survival values recorded for regular and occasional residents in this study are higher than those reported elsewhere (e.g., Wells and Scott, 1990; Speakman et al., 2010; Daura-Jorge et al., 2013; Tezanos-Pinto et al., 2013; Fruet et al., 2015; Vermeulen and Bräger, 2015; Vermeulen et al., 2017; Methion and Díaz López, 2018), probably because of differences in the way we stratified the sample by residency pattern. It is important to notice that the null standard error in the point estimate of survival for both RR and OR is commonly attributed to problems with the data (Cooch and White, 2019, p. 6–24); nevertheless, because of the stratification by residency type, it probably reflects true high values and precision of the estimates due to effective population closure within the study timeframe (i.e., 5-y).
In general, coastal bottlenose dolphins are known to exhibit a wide spectrum of residency patterns, which include transients, seasonal migrants, year-round residents, and a combination of occasional long-range movements, and repeated local residency (Morteo et al., 2014; Wells and Scott, 2018). This seems to be the case for the population in our study area, where Ruíz-Hernández (2014) and Morteo et al. (2019) recorded a limited exchange of individuals between coastal waters off ALS and two northern locations (the Veracruz Reef System and Nautla, see Figure 1), for which dolphins need to travel at least 100 and 230 km, respectively. Our results are similar to other contributions made to the south of our study area, where it was documented that some individuals traveled 270 km on average in a few months, including a dolphin that traveled more than 800 km between the states of Quintana Roo and Tabasco (Delgado-Estrella, 2015, see Figure 1).
Differences in recruitment rate and timing between the core community (RR + OR) and visitors, as found in this study, could correspond to sex-related movement patterns, as reported previously in the Gulf of Mexico, the Caribbean (e.g., Urian et al., 2009; Caballero et al., 2012; Wells, 2014) and also in the study area, through greater residency in females and larger dispersion by males (Morteo et al., 2017, 2019). In this sense, natal site philopatry of both sexes is common and associated to age, as it occurs in the Sarasota population (Wells, 2003, 2014; Sellas et al., 2005). In the northern Gulf of Mexico, molecular data also suggest that some females may move and breed among different communities (Duffield and Wells, 2002), but implications at a local level remain to be investigated.
The existence of two plateaus in the discovery curves of the full sample, OV and OR individuals, indicates at least one occasional pulse of individuals entering from the superpopulation to the study area. These results are consistent with previous findings (Morteo, 2011; Morteo et al., 2014, 2017), suggesting that it is an open population, but the rate of incorporation of new individuals is low in the medium-long term.
Comparable absolute abundances of several estuarine bottlenose dolphin communities around the world are often estimated between 60 and 150 individuals (e.g., Williams et al., 1993; Wilson et al., 1999; Wells, 2003; Balmer et al., 2008; Vermeulen and Cammareri, 2009; Fruet et al., 2011, 2015; Félix et al., 2017). Monthly averages of daily abundances by Morteo et al. (2017) in the study area were about 125 dolphins under the Jolly-Seber model. This number looks consistent with our estimates both within and between years, but results are not directly comparable because of differences in the treatment of the samples.
Our results support the assumption that the dolphin population that uses the coastal waters off ALS is open (Morteo et al., 2017), but emphasizes the existence of a core community of resident individuals that, occasionally, receives an influx of individuals from neighboring waters, with no apparent seasonal trend. It is noteworthy that, unlike other study areas, this core community -living in an open habitat- largely behaves as a closed population. This situation may be more common than previously thought for the species across the Gulf of Mexico, where many individuals remain in relatively small but well provisioned areas, whereas short-term residents and visitors come from the adjacent coastal populations, or have nomadic habits in their constant pursuit of food and mates (Shane, 1980; Irvine et al., 1981; Wells et al., 1987; Ruíz-Hernández, 2014; Delgado-Estrella, 2015; Morteo et al., 2019, among others). The existence of such core communities in the northern Gulf of Mexico has been generally established based on qualitative criteria, mainly the overlap in the presence of transients with year-round and seasonal residents (i.e., Shane, 1980, 1990; Wells et al., 1987; Balmer et al., 2008; Tyson et al., 2011, among others) and, in most cases, refer to dolphin populations living in inshore, estuarine habitats. However, in a coastal habitat adjacent to the Sarasota Bay, Fazioli et al. (2006) found that the dolphin community that prefer the Gulf of Mexico is primarily composed of transients, seasonal residents an individuals with a home range greater than the study area, with fewer year-round residents; nevertheless, details on the process to compute individual residency, and quantitative approaches on the classification of such individuals based on the dynamics of the population is generally lacking.
The permanent presence of dolphin groups and the existence of a core community in the coastal waters off ALS are probably related to a predictable supply of prey and a sheltered environment. Interestingly the dynamic flux of individuals, abundance at a local level (i.e., the core community) seems stable over time, which suggests that a sort of carrying capacity effect is in place. High site fidelity and/or restricted ranging patterns are likely driving population parameters for the core community of bottlenose dolphins in the coastal waters of Alvarado; as this could increase their chances of living in a provisioned habitat, it could also make them more prone to detrimental effects by both documented and currently unknown local threats. Future work should focus on exploring at a finer scale the relationship between these population parameters, the structure of the population (sex/age), and relevant environmental variables for the species.
Differences in residency patterns for the dolphins in our study area could be explained by the socioecological model of Gowans et al. (2007) such that, as resources are spatially and temporally predictable, dolphins remain resident in relatively small areas (Morteo et al., 2014, 2017). Conversely, competition with local dolphins may lead non-resident individuals (mostly males) to range widely to find sufficient resources and mating opportunities (Gowans et al., 2007). This strategy helps to prevent inbreeding (Caballero et al., 2012), and would cause male dolphins of this population to prey on other species that may be available off river mouths and estuaries along coastal waters off the southwestern Gulf of Mexico (Martínez-Serrano et al., 2011; Morteo et al., 2014, 2017, 2019). As the existence of many resident communities has evidenced limited genetic exchange among adjacent sites all along the Gulf of Mexico (Sellas et al., 2005; Caballero et al., 2012; Vollmer and Rosel, 2013), this raises the question of a probable structure at metapopulation level, which should also be investigated.
Data Availability Statement
The raw data supporting the conclusions of this article will be made available by the authors, without undue reservation. The reduced m-array produced by the MARK output, that contains summary information on the numbers of individuals released at each occasion, and is the basis for the estimation of parameters, is presented in Supplementary Material 3 for reproducibility and replicability.
Ethics Statement
This animal study was accomplished through federal permits SGPA/DGVS/00351/06, 01407/08 (EM) and SGPA/DGVS/00870/07, 02788/07, 01344/08, and 01649/08 (M. C. Bazúa) issued by the Subsecretaria de Gestion para la Proteccion Ambiental.
Author Contributions
JB-J: conceptualization, formal analysis, investigation, methodology, validation, visualization, and writing – original draft. EM: conceptualization, data curation, funding acquiring, methodology, project administration, resources, supervision, validation, and writing – review and editing. CD-A and JB-P: methodology, supervision, validation, and writing – review and editing. PF and ES: conceptualization, methodology, supervision, validation, and writing – review and editing. All authors contributed to the article and approved the submitted version.
Conflict of Interest
The authors declare that the research was conducted in the absence of any commercial or financial relationships that could be construed as a potential conflict of interest.
Publisher’s Note
All claims expressed in this article are solely those of the authors and do not necessarily represent those of their affiliated organizations, or those of the publisher, the editors and the reviewers. Any product that may be evaluated in this article, or claim that may be made by its manufacturer, is not guaranteed or endorsed by the publisher.
Acknowledgments
This research was part of the first author’s doctoral thesis at Universidad Veracruzana, supported through doctoral fellowship Number 291197/CVU636908 by the Mexican National Council for Science and Technology (CONACyT). This work was also part of the CONACyT project 221750 on the” Trophic ecology of bottlenose dolphins (Tursiops truncatus) and artisanal fisheries interactions in coastal waters off Veracruz State”. Fieldwork was accomplished through federal permits SGPA/DGVS/00351/06, 01407/08 (EM) and SGPA/DGVS/00870/07, 02788/07, 01344/08, and 01649/08 (M. C. Bazúa). Surveys were always conducted by at least one of the authors and many undergraduate students at LabMMar, which were also involved in data collection, photographic analyses and data processing. Israel Huesca helped produce the figures. Local fishers R. Tiburcio, J. Tiburcio and E. Tiburcio always returned the crew safely back to shore. Dagmar Fertl and Nathalie Ward reviewed earlier versions of this manuscript. Paul Conn, Manuel Cach, Alberto Delgado Estrella, Tim Gerrodette, Carlos Lira, Manuel Mendoza Carranza, Cecilia Passadore, Grgur Pleslić, Pedro Sánchez Palomino, Kate Sprogis, Gabriela Tezanos Pinto, Fernando Trujillo, and Randy Wells provided valuable information and/or references. This manuscript was greatly improved by comments from MD and AL. This study was part of a collaboration between LabMMar-ICIMAP-IIB, Universidad Veracruzana, and ECOMEGA, Universidade Federal do Rio Grande-FURG. All the fieldwork was conducted in compliance with the “Guidelines for the Treatment of Marine Mammals in Field Research” of the Society for Marine Mammalogy (available at https://marinemammalscience.org/about-us/ethics/marine-mammal-treatment-guidelines).
Supplementary Material
The Supplementary Material for this article can be found online at: https://www.frontiersin.org/articles/10.3389/fmars.2021.753484/full#supplementary-material
References
Akaike, H. (1973). “Information theory and an extension of the maximum likelihood principle,” in Proceedings of the Second International Symposium on Information Theory, eds B. N. Petrov and F. Caski (Budapest: Akademiai Kiado), 267–281.
Ballance, L. T. (1990). “Residence patterns, group organization and surface association of bottlenose dolphins in Kino Bay, Gulf of California, Mexico,” in The Bottlenose Dolphin, eds S. Leatherwood and R. R. Reeves (San Diego: Academic Press), 267–284. doi: 10.1016/B978-0-12-440280-5.50017-2
Balmer, B. C., Wells, R. S., Nowacek, S. M., Nowacek, D. P., Schwake, L. H., McLellan, W. A., et al. (2008). Seasonal abundance and distribution patterns of common bottlenose dolphins (Tursiops truncatus) near St. Joseph Bay, Florida, USA. Cetacean Res. Manag. 10, 157–167.
Begon, M., Townsend, C. R., and Harper, J. L. (1995). Ecología: individuos, poblaciones y comunidades. Barcelona: Ediciones Omega.
Bigg, M. A. (1982). An assessment of killer whale (Orcinus orca) stocks off Vancouver Island, British Columbia. Rep. Int. Whaling Comm. 32, 655–666.
Bose, S., Forrester, T. D., Brazeal, J. L., Sacks, B. N., Casady, D. S., and Wittmer, H. U. (2017). Implications of fidelity and philopatry for the population structure of female black-tailed deer. Behav. Ecol. 28, 983–990. doi: 10.1093/beheco/arx047
Burnham, K. P., Anderson, D. R., White, G. C., Brownie, C., and Pollock, K. H. (1987). Design and Analysis of Fish Survival Experiments Based on Release–Recapture Data. Bethesda: American Fisheries Society Monograph.
Caballero, S., Islas-Villanueva, V., Tezanos-Pinto, G., Duchene, S., Delgado-Estrella, A., Sanchez-Okrucky, R., et al. (2012). Phylogeography, genetic diversity and population structure of common bottlenose dolphins in the Wider Caribbean inferred from analyses of mitochondrial DNA control region sequences and microsatellite loci: conservation and management implications. Anim. Conserv. 15, 95–112. doi: 10.1111/j.1469-1795.2011.00493.x
Calinski, T., and Harabasz, J. (1974). A dendrite method for cluster analysis. Commun. Stat. Simul. Comput. 3, 1–27. doi: 10.1080/03610917408548446
Carlucci, R., Baş, A. A., Liebig, P., Bellomo, S., Fanizza, C., Maglietta, R., et al. (2020). Residency patterns and site fidelity of Grampus griseus (Cuvier, 1812) in the Gulf of Taranto (Northern Ionian Sea, Central-Eastern Mediterranean Sea). Mamm. Res. 65, 445–455. doi: 10.1007/s13364-020-00485-z
Chao, A., Lee, S. M., and Jeng, S. L. (1992). Estimating population size for capture-recapture data when capture probabilities vary by time and individual animal. Biometrics 48, 201–216. doi: 10.2307/2532750
Choquet, R., Lebreton, J. D., Gimenez, O., Reboulet, A. M., and Pradel, R. (2009). U-CARE: utilities for performing goodness of fit tests and manipulating CApture-REcapture data. Ecography 32, 1071–1074. doi: 10.1111/j.1600-0587.2009.05968.x
Choquet, R., Reboulet, A. M., Lebreton, J. D., Gimenez, O., and Pradel, R. (2005). U-CARE 2.2 User’s Manual. Montpellier: CEFE.
Colwell, R. K., Mao, C. X., and Chang, J. (2004). Interpolating, extrapolating, and comparing incidence-based species accumulation curves. Ecology 85, 2717–2727. doi: 10.1890/03-0557
Connor, R. C., Wells, R. S., Mann, J., Read, A., Connor, R. C., Tyack, P. L., et al. (2000). “The bottlenose dolphin: social relationships in a fission-fusion society,” in Cetacean Societies: Field Studies of Whales and Dolphins, ed. J. Mann (Chicago: University of Chicago Press), 91–126.
Cooch, E. G., and White, G. C. (2019). Program MARK: A Gentle Introduction, 19th Edn. Fort Collins: Colorado State University.
Cruz-Escalona, V. H., Arreguín-Sánchez, F., and Zetina-Rejón, M. (2007). Analysis of the ecosystem structure of Laguna Alvarado, western Gulf of Mexico, by means of a mass balance model. Estuar. Coast. Shelf Sci. 72, 155–167. doi: 10.1016/j.ecss.2006.10.013
Daura-Jorge, F., Ingram, S. N., and Simões-Lopes, P. C. (2013). Seasonal abundance and adult survival of bottlenose dolphins (Tursiops truncatus) in a community that cooperatively forages with fishermen in southern Brazil. Mar. Mamm. Sci. 29, 2933–2911. doi: 10.1111/j.1748-7692.2012.00571.x
de la Lanza Espino, G., and Lozano Montes, L. (1999). Comparación fisicoquímica de las lagunas de Alvarado y Términos. Hidrobiológica 9, 15–30.
Delgado-Estrella, A. (2015). Patrones de residencia y movimientos a largo plazo de las toninas, Tursiops truncatus, en la región suroeste del Golfo de México. Therya 37, 379–392.
Duffield, D. A., and Wells, R. S. (2002). “The molecular profile of a resident community of bottlenose dolphins, Tursiops truncatus,” in Molecular and Cell Biology of Marine Mammals, ed. C. J. Pfeiffer (Melbourne: Krieger), 3–11.
Fazioli, K. L., Hofmann, S., and Wells, R. S. (2006). Use of Gulf of Mexico coastal waters by distinct assemblages of bottlenose dolphins (Tursiops truncatus). Aquat. Mamm. 32, 212–222. doi: 10.1578/AM.32.2.2006.212
Félix, F., Calderón, A., Vintimilla, M., and Bayas−Rea, R. A. (2017). Decreasing population trend in coastal bottlenose dolphin (Tursiops truncatus) from the Gulf of Guayaquil, Ecuador. Aquat. Conserv. 27, 856–866. doi: 10.1002/aqc.2763
Fisher, R. A., Corbet, A. S., and Williams, C. B. (1943). The relationship between the number of species and the number of individuals in a random sample of an animal population. J. Anim. Ecol. 12, 42–58. doi: 10.2307/1411
Fruet, P. F., Daura-Jorge, F. G., Möller, L. M., Genoves, R. C., and Secchi, E. R. (2015). Abundance and demography of bottlenose dolphins inhabiting a subtropical estuary in the Southwestern Atlantic Ocean. J. Mammal. 96, 332–343. doi: 10.1093/jmammal/gyv035
Fruet, P. F., Secchi, E. R., Di Tullio, J. C., and Kinas, P. G. (2011). Abundance of bottlenose dolphins, Tursiops truncatus (Cetacea: delphinidae), inhabiting the Patos Lagoon estuary, southern Brazil: implications for conservation. Zoologia (Curitiba) 28, 23–30. doi: 10.1590/S1984-46702011000100004
Galindo, J. A., Serrano, A., Vázquez-Castán, L., González-Gándara, C., and López-Ortega, M. (2009). Cetacean diversity, distribution, and abundance in northern Veracruz, Mexico. Aquat. Mamm. 35, 12–18. doi: 10.1578/AM.35.1.2009.12
Geraci, J. R., and Lounsbury, V. J. (1993). Marine Mammals Ashore: A Field Guide for Strandings. College Station: Texas A&M University Sea Grant Publication.
Gowans, S., Würsig, B., and Karczmarski, L. (2007). The social structure and strategies of Delphinids: predictions based on an ecological framework. Adv. Mar. Biol. 53, 195–294. doi: 10.1016/S0065-2881(07)53003-8
Greenwood, P. J. (1980). Mating systems, philopatry and dispersal in birds and mammals. Anim. Behav. 28, 1140–1162. doi: 10.1016/S0003-3472(80)80103-5
Haughey, R., Hunt, T., Hanf, D., Rankin, R. W., and Parra, G. J. (2020). Photographic capture-recapture analysis reveals a large population of Indo-Pacific bottlenose dolphins (Tursiops aduncus) with low site fidelity off the North West Cape, Western Australia. Front. Mar. Sci. 6:781. doi: 10.3389/fmars.2019.00781
Hunt, T., Bejder, L., Allen, S. J., Rankin, R. W., Hanf, D. M., and Parra, G. J. (2017). Demographic characteristics of Australian humpback dolphins reveal important habitat toward the southwestern limit of their range. Endang. Spec. Res. 32, 71–88. doi: 10.3354/esr00784
Irvine, A. B., Scott, M. D., Wells, R. S., and Kaufmann, J. H. (1981). Movements and activities of the atlantic bottlenose dolphin, Tursiops truncatus, near Sarasota, Florida. Fish. Bull. U. S. 79, 671–688.
Krebs, C. J. (1972). Ecology: The Experimental Analysis of Distribution and Abundance. New York: Harper and Row.
Martínez-Serrano, I., Serrano, O., Heckel, G., and Schramm, Y. (2011). Distribution and home range of bottlenose dolphins (Tursiops truncatus) off Veracruz. Mexico. Cien. Mar. 37, 379–392. doi: 10.7773/cm.v37i4A.1860
Methion, S., and Díaz López, B. (2018). Abundance and demographic parameters of bottlenose dolphins in a highly affected coastal ecosystem. Mar. Freshw. Res. 69, 1355–1364. doi: 10.1071/MF17346
Morales-Rincón, N., Morteo, E., and Delfín-Alfonso, C. A. (2019). Influence of artisanal fisheries on the behavior and social structure of Tursiops truncatus in the Southwestern Gulf of Mexico. J. Mar. Biol. Assoc. U. K. 99, 1841–1849. doi: 10.1017/S002531541900078X
Morteo, E. (2011). Ecología social de los delfines (Tursiops truncatus) en las aguas costeras de Alvarado, Veracruz, México. Ph. D. thesis. Veracruz: Universidad Veracruzana.
Morteo, E., Ramírez y Romero, T. E., Castillo-Olvera, V., Delfín-Alfonso, C. A., Bello-Pineda, J., and González-Christen, A. (2019). “Ecología poblacional de delfines (Tursiops truncatus) en el corredor arrecifal del suroeste del Golfo de México,” in Estudios Científicos en el Corredor Arrecifal del Suroeste del Golfo de México, eds A. Granados-Barba, L. Ortiz-Lozano, C. González-Gándara, and D. Salas-Monreal (Campeche: Universidad Autónoma de Campeche), 191–214.
Morteo, E., Rocha-Olivares, A., and Abarca-Arenas, L. (2014). Sexual segregation of coastal bottlenose dolphins (Tursiops truncatus) in the Southwestern Gulf of Mexico. Aquat. Mamm. 40, 375–385. doi: 10.1578/AM.40.4.2014.375
Morteo, E., Rocha-Olivares, A., and Abarca-Arenas, L. G. (2017). Abundance, residency and potential hazards for coastal bottlenose dolphins (Tursiops truncatus) off a productive lagoon in the Gulf of Mexico. Aquat. Mamm. 43, 308–319. doi: 10.1578/AM.43.3.2017.308
Morteo, E., Rocha-Olivares, A., and Morteo, R. (2012b). Sensitivity analysis of residency and site fidelity estimations to variations in sampling effort and individual catchability. Rev. Mex. Biodivers. 83, 487–495. doi: 10.22201/ib.20078706e.2012.2.972
Morteo, E., Rocha-Olivares, A., Arceo-Briseño, P., and Abarca-Arenas, L. G. (2012a). Spatial analyses of bottlenose dolphin-fisheries interactions reveal human avoidance off a productive lagoon in the western Gulf of Mexico. J. Mar. Biol. Assoc. U. K. 92, 1893–1900. doi: 10.1017/S0025315411000488
Ojasti, J., and Dallmeier, F. (eds) (2000). Manejo de Fauna Silvestre Neotropical, SI/MAB Series # 5. Washington: Smithsonian Institution.
Oksanen, F. J., Blanchet, G., Kindt, R., Legendre, P., Minchin, P. R., O’Hara, B., et al. (2019). Vegan: Community Ecology Package. R package Version 2.4-3.
Ortega Ortiz, J. G. (2002). Multiscale analysis of cetacean distribution in the Gulf of Mexico. Ph. D. thesis. College Station: Texas A & M University.
Ortega Ortiz, J., Delgado-Estrella, A., and Ortega-Argueta, E. (2004). “Mamíferos marinos del Golfo de México: estado actual del conocimiento y recomendaciones para su conservación,” in Diagnóstico Ambiental del Golfo de México, eds M. Caso, I. Pisanty, and E. Ezcurra (Mexico: Instituto Nacional de Ecología), 135–160.
Otis, D. L., Burnham, K. P., White, G. C., and Anderson, D. R. (1978). Statistical inference from capture data on closed animal populations. Wildl. Monogr. 62:135.
Passadore, C., Möller, L., Diaz-Aguirre, F., and Parra, G. J. (2017). High site fidelity and restricted ranging patterns in southern Australian bottlenose dolphins. Ecol. Evol. 8, 242–256. doi: 10.1002/ece3.3674
Pollock, K. H., Nichols, J. D., Brownie, C., and Hines, J. E. (1990). Statistical inference for capture-recapture experiments. Wildl. Monogr. 107, 3–97.
R Core Team (2020). R: A language and environment for statistical computing. Vienna: R Foundation for Statistical Computing.
Ralls, K., Brownell, R. L. Jr., and Ballou, J. (1980). Differential mortality by sex and age in mammals, with specific reference to the sperm whale. Rep. Int. Whaling Comm. 2, 233–243.
Ramírez-León, M. R., García-Aguilar, M. C., Aguayo-Lobo, A., Fuentes-Allen, I., and Sosa-Nishizaki, O. (2020). What do we know about cetaceans in the Mexican waters of the Gulf of Mexico? A review. Aquat. Mamm. 46, 623–632. doi: 10.1578/AM.46.6.2020.623
Rechimont, M. E., Lara-Domínguez, A. L., Morteo, E., Martínez-Serrano, I., and Equihua, M. (2018). Depredation by coastal bottlenose dolphins (Tursiops truncatus) in the southwestern Gulf of Mexico in relation to fishing techniques. Aquat. Mamm. 44, 469–481. doi: 10.1578/AM.44.5.2018.469
Ruíz-Hernández, A. 2014. Desplazamientos de toninas (Tursiops truncatus) en la costa central de Veracruz, México. [Tesis de Licenciatura], Xalapa, Veracruz, México. Veracruz: Universidad Veracruzana.
Schleimer, A., Ramp, C., Delarue, J., Carpentier, A., Bérubé, M., and Palsbøll, P. J., et al. (2019). Decline in abundance and apparent survival rates of fin whales (Balaenoptera physalus) in the northern Gulf of St. Lawrence. Ecol. Evol. 9, 4231–4244. doi: 10.1002/ece3.5055
Schwarz, C. J., and Arnason, A. N. (1996). A general methodology for the analysis of capture-recapture experiments in open populations. Biometrics 52, 860–873. doi: 10.2307/2533048
Sellas, A., Wells, R., and Rosel, P. (2005). Mitochondrial and nuclear DNA analyses reveal fine scale geographic structure in bottlenose dolphins (Tursiops truncatus) in the Gulf of Mexico. Conserv. Genet. 6, 715–728. doi: 10.1007/s10592-005-9031-7
Shane, S. H. (1980). Occurrence, movements, and distribution of bottlenose dolphin, Tursiops truncatus, in southern Texas. Fish. Bull. 78, 593–601.
Shane, S. H. (1990). “Behavior and ecology of the bottlenose dolphin at Sanibel Island, Florida,” in The bottlenose dolphin, eds S. Leatherwood and R. R. Reeves (San Diego: Academic Press), 245–265. doi: 10.1016/B978-0-12-440280-5.50016-0
Speakman, T., Lane, S., Schwacke, L., Fair, P., and Zolman, E. (2010). Mark-recapture estimates of seasonal abundance and survivorship for bottlenose dolphins (Tursiops truncatus) near Charleston, South Carolina. J. Cetacean Res. Manag. 11, 53–62.
Stanley, T. R., and Burnham, K. P. (1999). A closure test for time-specific capture–recapture data. Environ. Ecol. Stat. 6, 197–209. doi: 10.1023/A:1009674322348
Stanley, T. R., and Richards, J. D. (2005). Software Review: a program for testing capture–recapture data for closure. Wildl. Soc. Bull. 33, 782–785. doi: 10.2193/0091-7648(2005)33[782:SRAPFT]2.0.CO;2
Switzer, P. V. (1993). Site fidelity in predictable and unpredictable habitats. Evol. Ecol. 7, 533–555. doi: 10.1007/BF01237820
Tezanos-Pinto, G., Constantine, R., Brooks, L., Jackson, J. A., Mourão, F., Wells, S., et al. (2013). Decline in local abundance of bottlenose dolphins (Tursiops truncatus) in the Bay of Islands, New Zealand. Mar. Mamm. Sci. 29, E390–E410. doi: 10.1111/mms.12008
Tschopp, A., Ferrari, M. A., Crespo, E., and Coscarella, M. (2018). Development of a site fidelity index based on population recapture data. PeerJ 6:e4782. doi: 10.7717/peerj.4782
Tyson, R. B., Nowacek, S. M., and Nowacek, D. P. (2011). Community structure and abundance of bottlenose dolphins Tursiops truncatus in coastal waters of the northeast Gulf of Mexico. Mar. Ecol. Prog. Ser. 438, 253–265. doi: 10.3354/meps09292
Urian, K. W., Hoffman, S., Wells, R. S., and Read, A. J. (2009). Fine-scale population structure of bottlenose dolphins (Tursiops truncatus) in Tampa Bay, Florida. Mar. Mamm. Sci. 25, 619–638. doi: 10.1111/j.1748-7692.2009.00284.x
Urian, K. W., Waples, D. M., Tyson, R. B., Hodge, L. E. W., and Read, A. J. (2013). Abundance of bottlenose dolphins (Tursiops truncatus) in estuarine and near-shore waters of North Carolina, USA. J. N. C. Acad. Sci. 129, 165–171. doi: 10.7572/2167-5880-129.4.165
Urian, K., Gorgone, A., Read, A., Balmer, B., Wells, R. S., Berggren, P., et al. (2015). Recommendations for photo-identification methods used in capture-recapture models with cetaceans. Mar. Mamm. Sci. 31, 298–321. doi: 10.1111/mms.12141
Valdés-Arellanes, M. P., Serrano, A., Heckel, G., Schramm, Y., and Martínez-Serrano, I. (2011). Abundancia de dos poblaciones de toninas (Tursiops truncatus) en el norte de Veracruz, México. Rev. Mex. Biodivers. 82, 227–235. doi: 10.22201/ib.20078706e.2011.1.367
Vermeulen, E., and Bräger, S. (2015). Demographics of the disappearing bottlenose dolphin in Argentina: a common species on its way out? PLoS One 10:e0119182. doi: 10.1371/journal.pone.0119182
Vermeulen, E., and Cammareri, A. (2009). Residency patterns, abundance, and social composition of bottlenose dolphins (Tursiops truncatus) in Bahía San Antonio, Patagonia, Argentina. Aquat. Mamm. 35, 379–386. doi: 10.1578/AM.35.3.2009.378
Vermeulen, E., Bastida, R., Berninsone, L. G., Bordino, P., Failla, M., Fruet, P., et al. (2017). A review on the distribution, abundance, residency, survival and population structure of coastal bottlenose dolphins in Argentina. Latin Am. J. Aquat. Mamm. 12, 2–16. doi: 10.5597/00233
Vollmer, N. L., and Rosel, P. E. (2013). A review of common bottlenose dolphins (Tursiops truncatus truncatus) in the northern Gulf of Mexico: population biology, potential threats, and management. Southeast. Nat. 12, 1–43.
Vollmer, N. L., Rosel, P. E., Mullin, K. D., Schwacke, L. H., Garrison, L. P., Balmer, B. C., et al. (2021). Assessing common bottlenose dolphin (Tursiops truncatus) population structure in Mississippi Sound and coastal waters of the north central Gulf of Mexico. Aquat. Conserv. Mar. Freshwater Ecosyst. 2021, 1–16. doi: 10.1002/aqc.3668
Wells, R. S. (2003). “Dolphin social complexity: lessons from long-term study and life history,” in Animal social complexity: intelligence, culture, and individualized societies, eds F. B. M. de Waal and P. L. Tyack (Cambridge: Harvard University Press), 32–56. doi: 10.4159/harvard.9780674419131.c4
Wells, R. S. (2014). “Social structure and life history of bottlenose Dolphins near Sarasota Bay, Florida: insights from four decades and five generations,” in Primates and Cetaceans: field Research and Conservation of Complex Mammalian Societies. Primatology Monographs, eds J. Yamagiwa and L. Karczmarski (Japan: Springer), 149–172. doi: 10.1007/978-4-431-54523-1_8
Wells, R. S., and Scott, M. D. (1990). Estimating bottlenose dolphin population parameters from individual identification and capture-release techniques. Rep. Int. Whaling Comm. 12, 407–415.
Wells, R. S., and Scott, M. D. (2018). “Bottlenose dolphin, Tursiops truncatus, common bottlenose dolphin,” in Encyclopedia of marine mammals, 3rd Edn, eds B. Würsig, J. G. M. Thewissen, and K. Kovacs (San Diego: Academic Press), 118–125. doi: 10.1016/B978-0-12-804327-1.00072-8
Wells, R. S., Irvine, A. B., and Scott, M. D. (1980). “The social ecology of inshore odontocetes,” in Cetacean Behavior: Mechanisms and Functions, ed. L. M. Herman (New York: Wiley), 263–317.
Wells, R. S., Scott, M. D., and Irvine, A. B. (1987). “The social structure of free ranging bottlenose dolphins,” in Current mammalogy, ed. H. H. Genoways (New York: Plenum Press), 247–305. doi: 10.1007/978-1-4757-9909-5_7
White, G. C., and Burnham, K. P. (1999). Program MARK: survival estimation from populations of marked animals. Bird Study 46, S120–S138. doi: 10.1080/00063659909477239
Wickman, L., Rayment, W., Slooten, E., and Dawson, S. M. (2020). Recommendations for estimating mark rate of cetaceans in photo-ID research: a critique of field sampling protocols and variance estimation. Mar. Mamm. Sci. 34, 1–16. doi: 10.1111/mms.12723
Williams, B. K., Nichols, D. J., and Conroy, M. J. (2002). Analysis and management of animal populations. London: Academic Press.
Williams, J. A., Dawson, S. M., and Slooten, E. (1993). The abundance and distribution of bottlenosed dolphins (Tursiops truncatus) in Doubtful Sound, New Zealand. Can. J. Zool. 71, 2080–2088. doi: 10.1139/z93-293
Wilson, B., Hammond, P. S., and Thompson, P. M. (1999). Estimating size and assessing trends in a coastal bottlenose dolphin population. Ecol. Appl. 9, 288–300. doi: 10.1890/1051-0761(1999)009[0288:ESAATI]2.0.CO;2
Würsig, B. (1978). Occurrence and group organization of Atlantic bottlenose porpoises (Tursiops truncatus) in an Argentine bay. Biol. Bull. 154, 348–359. doi: 10.2307/1541132
Würsig, B., and Jefferson, T. A. (1990). Methods of photo-identification for small cetaceans. Rep. Int. Whaling Comm. 12, 43–52.
Keywords: core community, residency pattern, transients, Alvarado Lagoon System, closed population, open population, population structure
Citation: Bolaños-Jiménez J, Morteo E, Delfín-Alfonso CA, Fruet PF, Secchi ER and Bello-Pineda J (2021) Population Dynamics Reveal a Core Community of the Common Bottlenose Dolphin (Tursiops truncatus) in Open Waters of the South-Western Gulf of Mexico. Front. Mar. Sci. 8:753484. doi: 10.3389/fmars.2021.753484
Received: 04 August 2021; Accepted: 11 October 2021;
Published: 29 November 2021.
Edited by:
Rob Harcourt, Macquarie University, AustraliaReviewed by:
Martina Duras, University of Zagreb, CroatiaAimee R. Lang, Southwest Fisheries Science Center (NOAA), United States
Copyright © 2021 Bolaños-Jiménez, Morteo, Delfín-Alfonso, Fruet, Secchi and Bello-Pineda. This is an open-access article distributed under the terms of the Creative Commons Attribution License (CC BY). The use, distribution or reproduction in other forums is permitted, provided the original author(s) and the copyright owner(s) are credited and that the original publication in this journal is cited, in accordance with accepted academic practice. No use, distribution or reproduction is permitted which does not comply with these terms.
*Correspondence: Eduardo Morteo, eduardo.morteo@gmail.com