- 1Institute for Zoology and Didactics in Biology, University of Wuppertal, Wuppertal, Germany
- 2Department of Biology and CESAM, Centre for Environmental and Marine Studies, University of Aveiro, Aveiro, Portugal
- 3Centre for Molecular Biodiversity Research, Leibniz Institute for the Analysis of Biodiversity Change/ZFMK, Museum Koenig, Bonn, Germany
- 4LOEWE Centre for Translational Biodiversity Genomics (LOEWE-TBG), Frankfurt, Germany
Symbiosis with photoautotrophic organisms has evolved in various species and even whole animal lineages, which allowed them to directly benefit from photosynthesis. This so-called photosymbiosis is best studied in cnidarians, which primarily establish symbioses with dinoflagellates from the family Symbiodiniaceae. In most other animals the mechanisms of establishing photosymbiosis, the physiological basis, and the evolution of a photosymbiotic life history remain poorly understood. Sea slugs belonging to the Cladobranchia (Gastropoda, Nudibranchia) are no exception, and are a rather neglected animal lineage in the research field of photosymbiosis. Yet, studying these sea slugs holds great potential to establish a unique photosymbiosis model, as they are the only known taxon that has evolved two different strategies to acquire their symbiont: either from cnidarian prey (thus becoming a secondary host) or directly out of the water column. The mechanisms for photobiont uptake and maintenance are unknown for these sea slugs, but might be similar to those of cnidarians. However, in terms of the evolution of photosymbiosis, Cladobranchia seem to share many commonalities with more closely related sea slugs belonging to the Sacoglossa, which only maintain the chloroplasts of the algae they feed on. Hence, Cladobranchia have the potential to shed light on the evolution of photosymbiosis in taxonomically divergent animals that also harbor photobionts of different evolutionary lineages.
Introduction
Symbiotic relationships shape genomic and morphological plasticity, which is a driving force of evolution within prokaryotes and eukaryotes (Margulis, 1981; Burki et al., 2020). Most common are symbioses between heterotrophic organisms, but some protists (Decelle, 2013; Decelle et al., 2015; Foster and Zehr, 2019) and a few animal lineages (Melo Clavijo et al., 2018) engage in symbioses with photoautotrophic organisms (photobionts) – the so-called photosymbiosis (Cowen, 1988; Stanley, 2006). Among animals, stony corals (Scleractinia) are probably the best-known example of a successful photosymbiosis. Corals primarily form a mutualistic symbiosis with dinoflagellates of the family Symbiodiniaceae (Fensome, 1993), that are referred to zooxanthellae when in hospite. Yet, more recently the mutualism of the photosymbiosis has been questioned and some authors refer to a host-controlled parasitism of the photobiont (Wooldridge, 2010; Blackstone and Golladay, 2018). Independent on the exact nature of the symbiosis, the photosymbiosis of corals and Symbiodiniaceae is based on an interdependent nutrient exchange cycle between the host and the photobiont (Stanley and Helmle, 2010; Tornabene et al., 2017). It has been shown that the coral host obtains nutritional support by the photobiont in the form of sugars, amino acids and some other essential nutrients, sometimes even superseding its nutritional requirements, allowing the coral host to thrive in oligotrophic waters (Muscatine and Porter, 1977; Falkowski et al., 1984; Lin et al., 2015) and enhances their ability to form skeletons and build up the coral reef structure (Stanley and Lipps, 2011; Tambutté et al., 2011; Roth, 2014). In return, the coral host provides carbon dioxide and ammonium, which are key limiting compounds for the photobiont (e.g., Yellowlees et al., 2008). The coordination of this nutrient exchange cycle is complex. Its disruption, for instance caused by heat stress, can lead to the breakdown of the symbiosis and can result in the expulsion of the photobionts. This process is known as bleaching and often eventually results in the coral’s death leading in extreme cases to mass bleaching events, as seen in recent years, that are endangering entire reef communities across the globe (Suggett and Smith, 2020).
Aside from Scleractinia, photosymbiosis in metazoans is not well understood (Melo Clavijo et al., 2018). Considering its potential benefit for the heterotrophic host, it remains unclear why the symbiosis with a photobiont has evolved only in a few metazoan lineages. It is likely that photosymbiotic animals share components of a common genetic tool kit, which are essential for the initiation and maintenance of a photosymbiosis. This set of genes probably includes a large fraction related to the innate immune system (Gross et al., 2009; Davy et al., 2012; Mansfield and Gilmore, 2019), which is also highly relevant for other animal-microbe symbioses (e.g., McFall-Ngai et al., 2012; Schmittmann et al., 2021). Genomic data could help to understand these molecular mechanisms, but are still scarce for most photosymbiotic animal lineages (Melo Clavijo et al., 2018). Fortunately, genome-sequencing initiatives such as the recently launched Aquatic Symbiosis Genomics Project by the Welcome Sanger Institute and the Gordon and Betty Moore Foundation are addressing this lack of genomic data. Among others, this initative focuses on key photosymbiotic species in different lineages, which will provide a wealth of data and hence enable us to boost our understanding of photosymbioses. However, the acquisition of genomes of non-photosymbiotic congeners will be eminent to identify relevant genomic adaptations promoting photosymbiosis. Analyzing and comparing photosymbiotic and non-photosymbiotic animals within and between different lineages will have the potential to unravel their common genomic adaptations for photobiont recognition and maintenance. Such comparisons also hold the key to clarify at which point in the evolutionary history the animal host acquired distinct adaptations needed for photosymbiosis and if these adaptations evolved convergently or homologously.
We propose that a specific group of sea slugs, the Cladobranchia that belong to the Nudibranchia, should be studied in more detail. This lineage could considerably contribute to our understanding of photosymbiotic processes and the evolution of photosymbiosis in distantly related animals.
“Butterflies of the Sea”
Nudibranchia are a morphologically diverse and colorful group of non-shelled sea slugs, belonging to the Heterobranchia (Burmeister, 1837) and consisting of the suborder Doridina and the suborder Cladobranchia. Over 4,000 nudibranch species have been described and, due to their colorful appearance, they fascinate scientists and non-scientists alike and are often called “butterflies of the sea” (Anderson, 1995). Current research on Nudibranchia focuses on assessing their biodiversity (e.g., Eisenbarth et al., 2018; Fritts-Penniman et al., 2020; Korshunova et al., 2021), their developmental biology and life cycle (Page, 1993; Kristof and Klussmann-Kolb, 2010; Ahmadian et al., 2016; Togawa et al., 2019), and phylogenetic relationships within the different groups (e.g., Carmona et al., 2013; Goodheart et al., 2015a,b; Karmeinski et al., 2021Korshunova et al., 2021). Furthermore, because most Nudibranchia lost their protective shell, alternative defense strategies, such as mimicry of food sources (Gosliner and Behrens, 1990), calcareous needles (Cattaneo-Vietti et al., 1995), the synthesis of toxic metabolites (Bogdanov et al., 2017), and storing and using cnidocysts “stolen” from their cnidarian food source (Obermann et al., 2012; Goodheart et al., 2018) are investigated. Especially the potential pharmaceutical relevance of their secondary metabolites (reviewed by Cimino and Gavagnin, 2006; Putz et al., 2010; Fisch et al., 2017) makes them an interesting group for researchers. Photosymbiosis is only found in the Cladobranchia that comprise approximately 1,000 species and that can be identified by the lack of gills and their large dorsal appendices, the cerata, that also function as respiratory organ. However, photosymbiosis in Cladobranchia is not well understood.
Cladobranchia Evolved Different Strategies of Photobiont Acquisition
Like most photosymbiotic animals, Cladobranchia acquire their photobionts anew in each generation (i.e., horizontally, instead of vertically from their parents), which is the most common mechanism of photobiont acquisition in animals (reviewed in Melo Clavijo et al., 2018). However, only Cladobranchia evolved two different modes of horizontal photobiont acquisition – out of the water column or from photosymbiotic cnidarians (Figure 1). Within the Cladobranchia, members of the Dendronotoidea (Allmann, 1845), such as Melibe engeli Risbec, 1937 (Figure 1), experienced morphological modifications that resulted in a fan-like mouth opening (Gosliner, 1987), allowing them to effectively ingest the photobionts out of the water column (Bleidissel, 2010; Burghardt and Wägele, 2014). To the best of our knowledge, adults of the genus Melibe are the only sea slugs to obtain their photobionts this way. The vast majority of Cladobranchia, however, acquire the photobionts by feeding on cnidarian prey and “stealing” the cnidarians’ photobionts (Rudman, 1981; Kempf, 1984; Wägele, 2004). This is a unique photobiont acquisition strategy in animals that evolved in Cladobranchia probably multiple times in the superfamilies Arminoidea, Iredale and O’Donoghue (1923), Fionoidea Gray (1827), and Aeolidioidea Gray (1827) Figure 1).
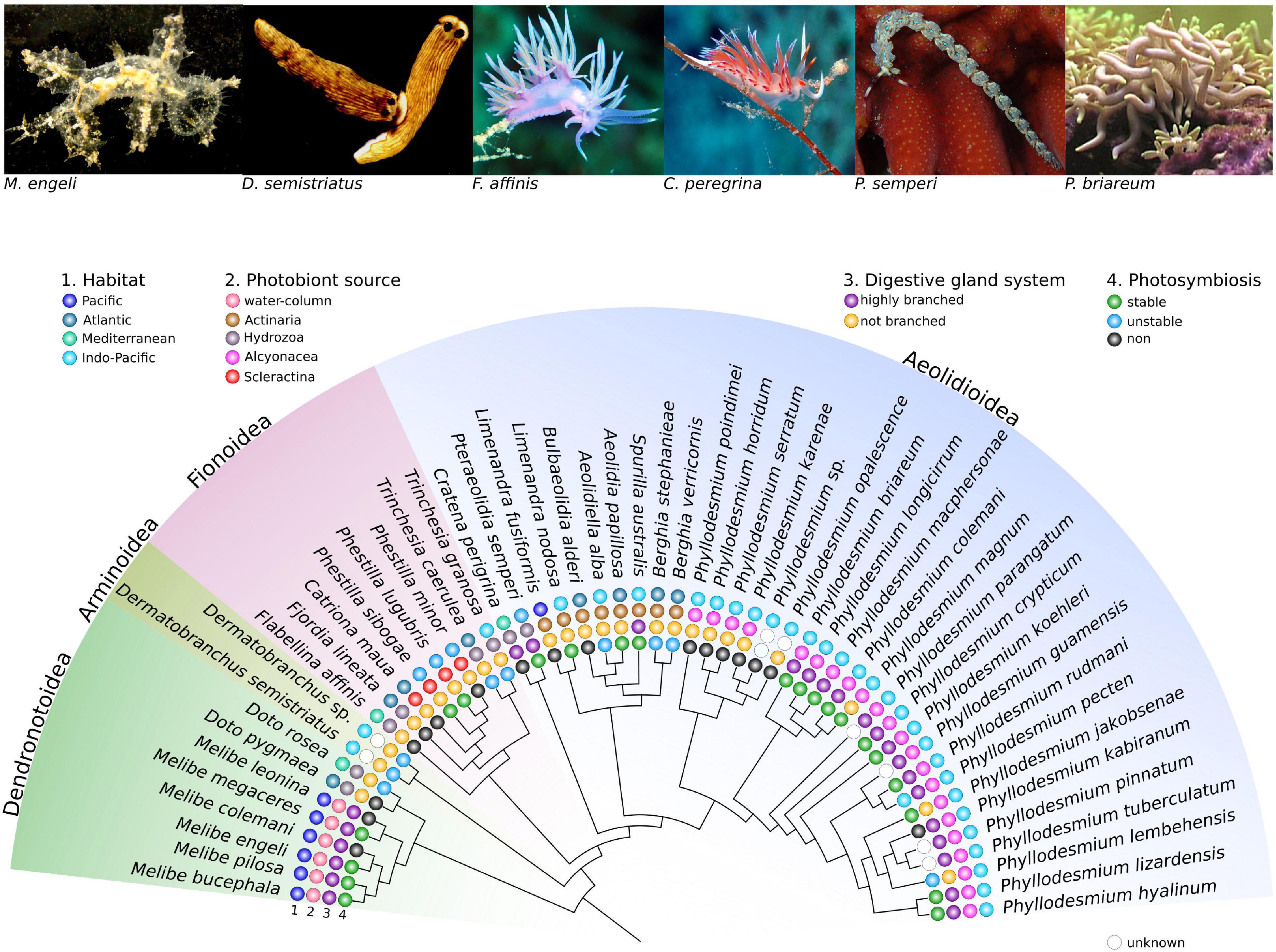
Figure 1. Cladogram of selected Cladobranchia species showing different levels of photosymbiosis. Members of Cladobranchia are widespread distributed (1. Habitat) and acquire their photobiont horizontally either from the water column or by feeding on photosymbiotic cnidarians (2. Photobiont source). Different degree of ramification of the digestive gland system is present in Cladobranchia (3. Digestive gland system) that might be correlated to the stability of the photosymbiosis (4. Photosymbiosis). The cladogramm and the respective information are based on published data (Wägele and Willan, 2000; Johnson and Gosliner, 2012; Moore and Gosliner, 2014; Goodheart et al., 2018; Karmeinski et al., 2021). The images were taken by Heike Waegele (Melibe engeli, Dermatobranchus semistriatus), Daniela Kupschus (Flabellina affinis, Cratena peregrina, Pteraeolidia ianthina), and Sabrina Bleidissel (Phyllodesmium briareum).
Not All Cladobranchia Can Maintain Photobionts
Subsequent to the uptake, Cladobranchia selectively phagocytize the photobionts into epithelial cells of their digestive gland system (DGS). The DGS branches throughout the entire body and, particularly, into the cerata (Figures 2A,B). Once the algae are phagocytized in the epithelial cells, they remain in their coccoid state and are surrounded by the phagosomal membrane (Figures 2C,D; Wägele and Johnsen, 2001; Wakefield and Kempf, 2001). In most cladobranchs, like Flabellina affinis (Gmelin, 1791) or Cratena peregrina (Gmelin, 1791) (Figure 1), the algae are then rapidly digested, while some species, like Berghia stephanieae (Valdés, 2005) (Figure 2A) are capable to maintain the algae photosynthetically active for a few days (Monteiro et al., 2019). Regarding the organismic interaction that we describe in this review we refer to the general symbiotic terminology. Within that terminology, the existing subcategories of photosymbiosis are defined based on the beneficial aspects and the time-span of interaction, following the definitions by Kempf (1991). Based on this, maintaining the photobiont for a short term is here referred to an unstable photosymbiosis, because the algae reside intracellularly, but the slugs, like B. stephanieae, tend to digest the algae within a couple of days, or expel them from the cells and secrete them in a viable state in the feces. Species that have evolved an unstable photosymbiosis do not appear to benefit from the photosynthesis performed by the acquired photobionts. They are neither able to maintain their symbionts nor their biomass when solely relying on the photobiont as source of nutrition (Kempf, 1991; McFarland and Muller-Parker, 1993; Bleidissel, 2010; Monteiro et al., 2019). Yet, some taxa have evolved the ability to establish a stable photosymbiosis, maintaining the photobionts for months. For a couple of these photosymbiotic species, like Melibe engeli and Phyllodesmium briareum (Bergh, 1896), it has been shown that the photobiont can fully support the host, enhancing growth and the ability of long-term reproduction without a reduction in the quantity and quality of egg-masses under regular light conditions (Kempf, 1984; Burghardt and Wägele, 2004, 2006, 2014; Burghardt et al., 2005, 2008a,b; Burghardt and Gosliner, 2006). These observations support the idea that at least these Cladobranchia species and Symbiodiniaceae are involved in a mutualistic symbiosis, which is unique in gastropods. More closely related Sacoglossa sea slugs, that are in a sort of photosymbiosis with chloroplasts of their algal prey, are not able to grow, or even maintain their biomass, if they are exclusively dependent on their ingested chloroplasts for more than a couple of weeks (Pelletreau et al., 2012; Christa et al., 2014). Further, they are not able to maintain the quantity and quality of egg-masses during periods of food depletion (Cartaxana et al., 2019). Hence, Cladobranchia provide a unique opportunity to understand which genomic adaptations are needed to evolve a mutualistic photosymbiosis in sea slugs. Comparative analyses of photosymbiotic and non-photosymbiotic Cladobranchia with Sacoglossa and Cnidaria might uncover if these genomic adaptations are based on convergent evolution or if, for instance, epigenetic modifications are involved in activating specific genes in photosymbiotic slugs.
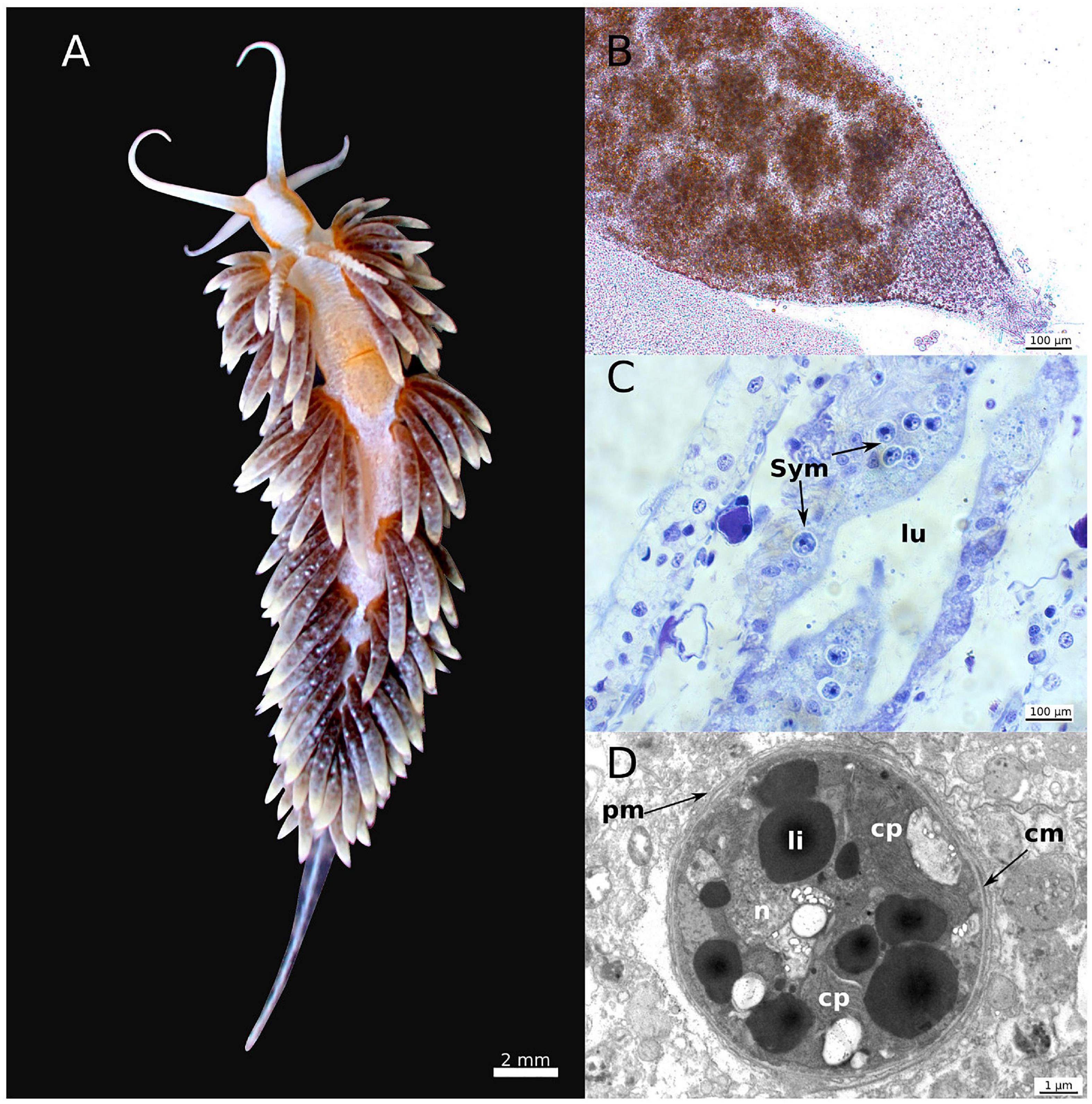
Figure 2. Morphology and microscopic details of Berghia stephanieae. Morphology of B. stephanieae (A). Detail of a cerata of B. stephanieae bearing the photobionts (B), histological cross-section through a cerata showing the host cells of the cerata bearing the photobiont (C), and detail of the coccoid (non-motile) stage of intracellular Symbiodiniaceae (D). cm: Symbiodiniaceae cell membrane, cp: chloroplast, li: lipid droplets, lu: lumen of the host digestive gland, n: nucleus, pm: host phagosomal membrane, Sym: Symbiodiniaceae. The images were taken by Gregor Christa (A,B), Jenny Melo (C) and Elise Laetz (D).
Mechanisms for Photobiont Recognition and Maintenance Are Unknown in Cladobranchia
The selective incorporation of photobionts in Cladobranchia is a complex process. It remains unknown how the slugs are able to distinguish between photobionts and plankton and further digest all plankton (e.g., in Melibe) or tissues of the cnidarian prey while maintaining the photobionts intact. The mechanisms of photobiont recognition by the epithelial cells of the DGS have not yet been addressed, while in cnidarians the photobiont recognition is based on a set of animal host pattern recognition receptors (PRRs). Specific microbe associated molecular patterns (MAMPs) of the photobiont (Neubauer et al., 2016, 2017; Mansfield and Gilmore, 2019) bind to the PRRs, which triggers downstream signaling cascades to maintain the photobiont (Davy et al., 2012). The PRRs of Cladobranchia might be similar to those of their cnidarian host, as they incorporate the same photobiont and hence need to recognize the same MAMPs. However, Cladobranchia could also just use different PRRs to recognize other MAMPs to identify the equivalent photobiont. The incorporation process might even be more similar to the selective uptake of chloroplasts by more closely related members of sea slugs belonging to the Sacoglossa (Chan et al., 2018; Melo Clavijo et al., 2020). Using phylogenetic and domain specific analyses of the respective receptors will help to understand if the relationship of the respective PRRs is matching the taxonomy of the host. In combination with subsequent functional analyses, for instance by gene expression and manipulation, a list of candidate genes can be generated that could be involved in photobiont recognition. Therefore, Cladobranchia are the only animals that could permit a direct comparison of photobiont recognition mechanisms, in particular PRRs, between a primary (Cnidaria) and secondary (Cladobranchia) host of the same photobiont. At the same time, Cladobranchia allow comparisons with mechanisms of chloroplast recognition in the more closely related Sacoglossa. These analyses may provide insights into the relevance of PRRs in photosymbiosis across taxonomically divergent animal taxa. Furthermore, they may elucidate how conserved the signaling pathways for initiating photosymbiosis are – independent from the taxonomic lineage of the photobiont.
Evolution of Stable Photosymbiosis in Cladobranchia
The evolution of a stable photosymbiosis in Cladobranchia is still not well understood. It appears that photosymbiosis evolved several times independently in different Cladobranchia superfamilies (Figure 1). For instance, it might have evolved independently in the Dendronotoidea and a monophyletic group including Arminoidea, Fionoidea, and Aeolidioidea. This would explain the evolution of the two different acquisition modes. Alternatively, photosymbiosis could also have evolved in each of the superfamilies independently. It is furthermore unknown whether unstable and stable photosymbiosis evolved separately, or if stable photosymbiosis evolved from unstable photosymbiosis.
Commonly, photosymbiotic Cladobranchia have a highly branched digestive gland system and specialized digestive structures, such as large circular chambers, fine tubules and cisternae that are located at the tips and harbor the photobionts (Burghardt and Wägele, 2014). These structures are considered to enlarge colonizable space and optimize light attenuation, increasing photobiont density and photosynthesis, respectively (Rudman, 1991; Burghardt et al., 2008a,b; Moore and Gosliner, 2011). Yet, despite of having a highly branched digestive gland system, species of Phestilla or Limenandra (Figure 1), and species like Melibe leonina (Gould, 1852) or Phyllodesmium kabiranum Baba, 1991, are non-photosymbiotic (Figure 1). Aside of this exception the genus Phyllodesmium shows a correlation between photosymbiosis and a highly branched digestive gland system (Rudman, 1991; Burghardt et al., 2008a,b; Moore and Gosliner, 2011). A highly branched DGS might not be a prerequisite to evolve a stable photosymbiosis, but it appears to be advantageous to harbor larger numbers of photobionts and to optimize photosynthesis.
The cnidarian prey is considered important for the stability of the photosymbiosis in Cladobranchia (Wägele et al., 2010). For instance, members of the genus Phyllodesmium, in which most species have a stable photosymbiosis, feed exclusively on xeneid cnidarians belonging to the Alcyonacea. However, Pteraeolidia semperi (Bergh, 1970), also in a stable photosymbiosis, obtains the photobionts from Hydrozoa, so that the food source is not strictly connected to photosymbiosis (Figure 1). Instead, it seems to be based on obtaining specific secondary metabolites for defense purposes (Bogdanov et al., 2017) and the feeding preference is rather taxon-specific. Independent of the source of the photobiont, the efficiency of the photosymbiosis may be further influenced by the specific algal taxon. For instance, in other stable photosymbiotic systems, such as Cnidaria-Symbiodiniaceae and Bivalvia-Symbiodiniaceae associations, the animal hosts could benefit from more physiologically resilient photobionts during increased ambient temperature (Hume et al., 2016; Cziesielski et al., 2018; Mies, 2019; Cunning and Baker, 2020). When corals are in symbiosis with multiple symbiodiniacean genera, the active removal of less resilient strains results in an adaptive bleaching, which might increase the animal’s fitness and improve the stability of the symbiosis considerably (Ziegler et al., 2014; Bayliss et al., 2019; Chen et al., 2019). So far, only a few studies have investigated the diversity and composition of Symbiodiniaceae in Cladobranchia (Loh et al., 2006; FitzPatrick et al., 2012; Ziegler et al., 2014; Wecker et al., 2015; Yorifuji et al., 2015). Nevertheless, these studies have not uncovered any correlation between specific Symbiodiniaceae taxa and the ability to establish a photosymbiosis with Nudibranchia. It rather seems that symbionts are taken up from the cnidarian prey indiscriminately. Future analyses of photobiont abundance in cladobranchs in comparison to their cnidarian prey will help to understand if the slugs are able to distinguish between Symbiodiniaceae taxa and selectively expel less beneficial photobionts. Comparative metabarcoding analyses, as available for some cnidarians and their Symbiodiniaceae composition (Fujise et al., 2021), is still lacking for sea slugs, but are needed to reveal the relevance for a stable photosymbiosis in Cladobranchia. It might be further worth to investigate, if the slugs play a role in genotype dispersion of symbiodiniaceans in marine habitats. The fact that symbionts are transferred from a sessile to a motile host could potentially enhance the dispersion of symbiodiniaceans and could change the composition of clades and strains in environmental populations (Parker, 1984). This could be beneficial for other sessile photosymbiotic animals and their symbiont uptake, facing environmental changes with different adapted symbionts (Umeki et al., 2020).
Regardless of the stability of the photosymbiosis, little is known on how nutrients are exchanged between the slugs and the algae. The phagosomal membrane surrounding photobionts in cnidarians is known as symbiosome (Hill and Hill, 2012). The symbiosome plays a crucial role in the successful establishment of the symbiosis. Transporters relevant for nutrient exchange, i.e., sugars from the algae to the animal and dissolved inorganic compounds from the animal to the algae, are situated in the symbiosomal membrane (Sproles et al., 2018). In cladobranchs it is unknown if and which transporters are present on the phagosomal membrane and how comparable it is to the symbiosome in terms of functionality. Future immuno-histochemical studies could give valuable insights into the localization of photosymbiosis-relevant receptors and their role in the nutritional exchange between the two partners, while metabolomics could provide important information on the interdependent nutrient exchange between both partners. Further, it remains to be shown whether in species with a stable photosymbiosis the symbiosis is beneficial for the host and whether the symbiosis is mutualistic or even some sort of parasitism as proposed for other photosymbiotic animals by some authors (Lesser et al., 2013; Blackstone and Golladay, 2018; Androuin et al., 2020).
Cladobranchia Can Shed Light on Photosymbiosis
Cladobranchia are a promising model to deepen the knowledge on fundamental processes that lead towards the evolution of photosymbiosis in animals as they resemble a connecting link between the well studied photosymbiosis in cnidarians and the less understood animal lineages such as sea slugs. Future research combining genomics, metabolomics, physiological, and immuno-histochemical studies, as well as phylogenetic analyzes of key receptors or proteins involved in photosymbiosis, will highlight if photosymbiosis evolved convergently or homologously in the different animal lineages.
Author Contributions
MR, JM, and GC designed the figures. All authors searched and analyzed the literature, wrote the draft, and reviewed and accepted the final version of the manuscript.
Funding
Financial support for this work was made possible by the EvoSym project (grant no. POCI-01-0145-FEDER-028751), funded by the Fundo Europeu de Desenvolvimento Regional (FEDER) through the “Programa Operacional Competitividade e Internacionalização” and by national funds from the Fundação para a Ciência e a Tecnologia (FCT)/Ministério da Ciência, Tecnologia e Ensino Superior (MCTES) via the Centre for Environmental and Marine Studies (CESAM), (grants numbers UIDP/50017/2020 and UIDB/50017/2020) and the German Academic Exchange Service. Additional funding was provided by the Central Research Funding Budget (ZEFFT) of the University of Wuppertal, Germany. We acknowledge support from the Open Access Publication Fund of the University of Wuppertal.
Conflict of Interest
The authors declare that the research was conducted in the absence of any commercial or financial relationships that could be construed as a potential conflict of interest.
Publisher’s Note
All claims expressed in this article are solely those of the authors and do not necessarily represent those of their affiliated organizations, or those of the publisher, the editors and the reviewers. Any product that may be evaluated in this article, or claim that may be made by its manufacturer, is not guaranteed or endorsed by the publisher.
Acknowledgments
We very much appreciate the help of Horst Bennemann, Heike Waegele, and Daniela Kupschus for providing images of the slugs and Elise Laetz for providing the TEM image of the Symbiodiniaceae.
References
Ahmadian, R., Burghardt, I., and Shepherd, U. L. (2016). Embryonic development of the solar-powered nudibranch Phyllodesmium lizardensis (Gastropoda: nudibranchia). Molluscan Res. 36, 285–289. doi: 10.1080/13235818.2016.1150770
Allmann, G. J. (1845). On the anatomy of Actaeon, with remarks on the order Phlebenterata of M. de Quatrefages. Ann. Mag. Nat. Hist. 16, 145–162.
Anderson, R. (1995). Nudibranchs: butterflies of the sea. Int. Zoo. Yearb. 34, 65–70. doi: 10.1111/j.1748-1090.1995.tb00659.x
Androuin, T., Six, C., Bordeyne, F., de Bettignies, F., Noisette, F., and Davoult, D. (2020). Better off alone? New insights in the symbiotic relationship between the flatworm Symsagittifera roscoffensis and the microalgae Tetraselmis convolutae. Symbiosis 81, 161–171. doi: 10.1007/s13199-020-00691-y
Bayliss, S. L. J., Scott, Z. R., Coffroth, M. A., and TerHorst, C. P. (2019). Genetic variation in Breviolum antillogorgium, a coral reef symbiont, in response to temperature and nutrients. Ecol. Evol. 9, 2803–2813. doi: 10.1002/ece3.4959
Blackstone, N. W., and Golladay, J. M. (2018). Why do corals bleach? Conflict and conflict mediation in a host/symbiont community. Bioessays 40:e1800021. doi: 10.1002/bies.201800021
Bleidissel, S. (2010). Molekulare Untersuchungen zur Evolution der Aeolidida (Mollusca, Gastropoda, Nudibranchia, Cladobranchia) und zur Evolution einer sekundären Symbiose mit Symbiodinium (Dinoflagellata) in den Aeolidida. Wuppertal: Bergische University Wuppertal.
Bogdanov, A., Hertzer, C., Kehraus, S., Nietzer, S., Rohde, S., Schupp, P. J., et al. (2017). Secondary metabolome and its defensive role in the aeolidoidean Phyllodesmium longicirrum, (Gastropoda, Heterobranchia, Nudibranchia). Beilstein J. Org. Chem. 13, 502–519. doi: 10.3762/bjoc.13.50
Burghardt, I., Evertsen, J., Johnsen, G., and Wägele, H. (2005). Solar powered seaslugs - Mutualistic symbiosis of aeolid nudibranchia (Mollusca, Gastropoda, Opisthobranchia) with Symbiodinium. Symbiosis 38, 227–250.
Burghardt, I., and Gosliner, T. M. (2006). Phyllodesmium rudmani (Mollusca: nudibranchia: aeolidoidea), a new solar powered species from the Indo-West Pacific with data on its symbiosis with zooxanthellae. Zootaxa 1308:31.
Burghardt, I., Schrödl, M., and Wägele, H. (2008a). Three new solar-powered species of the genus Phyllodesmium Ehrenberg, 1831 (Mollusca: nudibranchia: aeolidioidea) from the tropical Indo-Pacific, with analysis of their photosynthetic activity and notes on biology. J. Molluscan Stud. 74, 277–292. doi: 10.1093/mollus/eyn016
Burghardt, I., Stemmer, K., and Wägele, H. (2008b). Symbiosis between Symbiodinium (Dinophyceae) and various taxa of Nudibranchia (Mollusca: gastropoda), with analyses of long-term retention. Org. Divers. Evol. 8, 66–76. doi: 10.1016/j.ode.2007.01.001
Burghardt, I., and Wägele, H. (2004). A new solar powered species of the genus Phyllodesmium Ehrenberg, 1831 (Mollusca: nudibranchia: aeolidoidea) from Indonesia with analysis of its photosynthetic activity and notes on biology. Zootaxa 596:1. doi: 10.11646/zootaxa.596.1.1
Burghardt, I., and Wägele, H. (2006). Interspecific differences in the efficiency and photosynthetic characteristics of the symbiosis of “solarpowered” Nudibranchia (Mollusca: gastropoda) with zooxanthellae. Rec. West. Aust. Museum, Suppl. 69, 1–9. doi: 10.18195/issn.0313-122x.69.2006.001-009
Burghardt, I., and Wägele, H. (2014). The symbiosis between the “solar-powered” nudibranch Melibe engeli Risbec, 1937 (Dendronotoidea) and Symbiodinium sp. (Dinophyceae). J. Molluscan Stud. 80, 508–517. doi: 10.1093/mollus/eyu043
Burmeister, H. (1837). Handbuch der Naturgeschichte. Zum Gebrauch bei Vorlesungen. Berlin: Theodor Christian Friedrich Enslin.
Burki, F., Roger, A. J., Brown, M. W., and Simpson, A. G. B. (2020). The new tree of Eukaryotes. Trends Ecol. Evol. 35, 43–55. doi: 10.1016/j.tree.2019.08.008
Carmona, L., Pola, M., Gosliner, T. M., and Cervera, J. L. (2013). A tale that morphology fails to tell: a molecular phylogeny of Aeolidiidae (aeolidida, nudibranchia, Gastropoda). PLoS One 8:e63000. doi: 10.1371/journal.pone.0063000
Cartaxana, P., Morelli, L., Jesus, B., Calado, G., Calado, R., and Cruz, S. (2019). The photon menace: kleptoplast protection in the photosynthetic sea slug Elysia timida. J. Exp. Biol. 222:jeb202580. doi: 10.1242/jeb.202580
Cattaneo-Vietti, R., Angelini, S., Gaggero, L., and Lucchetti, G. (1995). Mineral composition of Nudibranch spicules. J. Molluscan Stud. 61, 331–337. doi: 10.1093/mollus/61.3.331
Chan, C. X., Vaysberg, P., Price, D. C., Pelletreau, K. N., Rumpho, M. E., and Bhattacharya, D. (2018). Active host response to algal symbionts in the sea slug Elysia chlorotica. Mol. Biol. Evol. 35, 1706–1711. doi: 10.1093/molbev/msy061
Chen, B., Yu, K., Liang, J., Huang, W., Wang, G., Su, H., et al. (2019). Latitudinal variation in the molecular diversity and community composition of Symbiodiniaceae in coral from the South China Sea. Front. Microbiol. 10:1278. doi: 10.3389/fmicb.2019.01278
Christa, G., Händeler, K., Kück, P., Vleugels, M., Franken, J., Karmeinski, D., et al. (2014). Phylogenetic evidence for multiple independent origins of functional kleptoplasty in Sacoglossa (Heterobranchia, Gastropoda). Org. Divers. Evol. 15, 23–36. doi: 10.1007/s13127-014-0189-z
Cimino, G., and Gavagnin, M. (2006). Molluscs: From Chemo-Ecological Study to Biotechnological Application. Berlin: Springer.
Cowen, R. (1988). The role of algal symbiosis in reefs through time. Palaios 3, 221–227. doi: 10.2307/3514532
Cunning, R., and Baker, A. C. (2020). Thermotolerant coral symbionts modulate heat stress-responsive genes in their hosts. Mol. Ecol. 29, 2940–2950. doi: 10.1111/mec.15526
Cziesielski, M. J., Liew, Y. J., Cui, G., Schmidt-Roach, S., Campana, S., Marondedze, C., et al. (2018). Multi-omics analysis of thermal stress response in a zooxanthellate cnidarian reveals the importance of associating with thermotolerant symbionts. Proc. R. Soc. B Biol. Sci. 285:20172654. doi: 10.1098/rspb.2017.2654
Davy, S. K., Allemand, D., and Weis, V. M. (2012). Cell biology of cnidarian-dinoflagellate symbiosis. Microbiol. Mol. Biol. Rev. 76, 229–261. doi: 10.1128/mmbr.05014-11
Decelle, J. (2013). New perspectives on the functioning and evolution of photosymbiosis in plankton: mutualism or parasitism? Commun. Integr. Biol. 6:e24560. doi: 10.4161/cib.24560
Decelle, J., Colin, S., and Foster, R. A. (2015). “Photosymbiosis in marine planktonic protists,” in Marine Protists, eds S. Ohtsuka, T. Suzaki, T. Horiguchi, N. Suzuki, and F. Not (Tokyo: Springer), 465–500. doi: 10.1007/978-4-431-55130-0_19
Eisenbarth, J. H., Undap, N., Papu, A., Schillo, D., Dialao, J., Reumschüssel, S., et al. (2018). Marine Heterobranchia (Gastropoda, Mollusca) in Bunaken National Park, North Sulawesi, Indonesia-A follow-up diversity study. Diversity 10:127. doi: 10.3390/d10040127
Falkowski, P. G., Dubinsky, Z., Muscatine, L., and Porter, J. W. (1984). Bioenergetics symbiotic coral. Bioscience 34, 705–709.
Fensome, R. A. (1993). A Classification of Living and Fossil Dinoflagellates. New York, NY: American Museum of Natural History.
Fisch, K. M., Hertzer, C., Hringer, N. B., Wuisan, Z. G., Schillo, D., Bara, R., et al. (2017). The potential of Indonesian heterobranchs found around Bunaken island for the production of bioactive compounds. Mar. Drugs 15:384. doi: 10.3390/md15120384
FitzPatrick, S. K., Liberatore, K. L., Garcia, J. R., Burghardt, I., Colman, D. R., Moquin, S. A., et al. (2012). Symbiodinium diversity in the soft coral Heteroxenia sp. and its nudibranch predator Phyllodesmium lizardensis. Coral Reefs 31, 895–905. doi: 10.1007/s00338-012-0913-0
Foster, R. A., and Zehr, J. P. (2019). Diversity, genomics, and distribution of phytoplankton-cyanobacterium single-cell symbiotic associations. Annu. Rev. Microbiol. 73, 435–456. doi: 10.1146/annurev-micro-090817-062650
Fritts-Penniman, A. L., Gosliner, T. M., Mahardika, G. N., and Barber, P. H. (2020). Cryptic ecological and geographic diversification in coral-associated nudibranchs. Mol. Phylogenet. Evol. 144:106698. doi: 10.1016/j.ympev.2019.106698
Fujise, L., Suggett, D. J., Stat, M., Kahlke, T., Bunce, M., Gardner, S. G., et al. (2021). Unlocking the phylogenetic diversity, primary habitats, and abundances of free-living Symbiodiniaceae on a coral reef. Mol. Ecol. 30, 343–360. doi: 10.1111/mec.15719
Goodheart, J. A., Bazinet, A. L., Collins, A. G., and Cummings, M. P. (2015a). Phylogeny of Cladobranchia (Gastropoda: nudibranchia): a total evidence analysis using DNA sequence data from public databases. Digit. Repos. Univ. Maryl. 2:150196.
Goodheart, J. A., Bazinet, A. L., Collins, A. G., and Cummings, M. P. (2015b). Relationships within cladobranchia (Gastropoda: nudibranchia) based on RNA-seq data: an initial investigation. R. Soc. Open Sci. 2:150196. doi: 10.1098/rsos.150196
Goodheart, J. A., Bleidißel, S., Schillo, D., Strong, E. E., Ayres, D. L., Preisfeld, A., et al. (2018). Comparative morphology and evolution of the cnidosac in Cladobranchia (Gastropoda: heterobranchia: nudibranchia). Front. Zool. 15:43. doi: 10.1186/s12983-018-0289-2
Gosliner, T. M. (1987). Review of the nudibranch genus Melibe (Opisthobranchia: Dendronotacea) with Descriptions of Two New Species. Berkeley: Calif. Malacozoological Society.
Gosliner, T. M., and Behrens, D. W. (1990). “Special resemblance, aposematic coloration and mimicry in Opisthobranch gastropods,” in Adaptive Coloration in Invertebrates, ed. M. Wicksten (College Station: Texas A. & M. University Press), 127–138.
Gray, J. E. (1827). “Plate mollusca [= plate 3], plate mollusca III [= plate 4], plate mollusca IV [= plate 6],” in Encyclopaedia Metropolitana, Vol. 7, eds E. Smedley, H. J. Rose, and H. J. Rose plates to zoology.
Gross, R., Vavre, F., Heddi, A., Hurst, G. D. D., Zchori-Fein, E., and Bourtzis, K. (2009). Immunity and symbiosis. Mol. Microbiol. 73, 751–759. doi: 10.1111/j.1365-2958.2009.06820.x
Hill, M., and Hill, A. (2012). The magnesium inhibition and arrested phagosome hypotheses: new perspectives on the evolution and ecology of Symbiodinium symbioses. Biol. Rev. 87, 804–821. doi: 10.1111/j.1469-185X.2012.00223.x
Hume, B. C. C., Voolstra, C. R., Arif, C., D’Angelo, C., Burt, J. A., Eyal, G., et al. (2016). Ancestral genetic diversity associated with the rapid spread of stress-tolerant coral symbionts in response to Holocene climate change. Proc. Natl. Acad. Sci. U. S. A. 113, 4416–4421. doi: 10.1073/pnas.1601910113
Iredale, T.O’Donoghue, C. H. (1923). List of British nudibranchiate Mollusca. Proc. Malacol. Soc. London 15, 195–233.
Johnson, R. F., and Gosliner, T. M. (2012). Traditional taxonomic groupings mask evolutionary history: a molecular phylogeny and new classification of the chromodorid nudibranchs. PLoS One 7:e33479. doi: 10.1371/journal.pone.0033479
Karmeinski, D., Meusemann, K., Goodheart, J. A., Schroedi, M., Martynov, A., Korshunova, T., et al. (2021). Transcriptomics provides a robust framework for the relationships of the major clades of cladobranch sea slugs (Mollusca, Gastropoda, Heterobranchia), but fails to resolve the position of the enigmatic genus Embletonia. bioRxiv [Preprint]. doi: 10.1101/2020.09.22.307728
Kempf, S. C. (1984). Symbiosis between the Zooxanthella Symbiodinium (= Gymnodinium) microadriaticum (Freudenthal) and four species of Nudibranchs. Biol. Bull. 166, 110–126.
Kempf, S. C. (1991). A ‘primitive’ symbiosis between the aeolid nudibranch Berghia verrucicornis (A. costa, 1867) and a zooxanthella. J. Molluscan Stud. 57, 75–85. doi: 10.1093/mollus/57.Supplement_Part_4.75
Korshunova, T., Bakken, T., Grøtan, V. V., Johnson, K. B., Lundin, K., and Martynov, A. (2021). A synoptic review of the family Dendronotidae (Mollusca: nudibranchia): a multilevel organismal diversity approach. Contrib. Zool. 90, 93–153. doi: 10.1163/18759866-BJA10014
Kristof, A., and Klussmann-Kolb, A. (2010). Neuromuscular development of Aeolidiella stephanieae Valdéz, 2005 (Mollusca, Gastropoda, Nudibranchia). Front. Zool. 7:5. doi: 10.1186/1742-9994-7-5
Lesser, M. P., Stat, M., and Gates, R. D. (2013). The endosymbiotic dinoflagellates (Symbiodinium sp.) of corals are parasites and mutualists. Coral Reefs 32, 603–611. doi: 10.1007/s00338-013-1051-z
Lin, S., Cheng, S., Song, B., Zhong, X., Lin, X., Li, W., et al. (2015). The Symbiodinium kawagutii genome illuminates dinoflagellate gene expression and coral symbiosis. Science 350, 691–694. doi: 10.1126/science.aad0408
Loh, W. K. W., Cowlishaw, M., and Wilson, N. G. (2006). Diversity of Symbiodinium dinoflagellate symbionts from the Indo-Pacific sea slug Pteraeolidia ianthina (Gastropoda: mollusca). Mar. Ecol. Prog. Ser. 320, 177–184. doi: 10.3354/meps320177
Mansfield, K. M., and Gilmore, T. D. (2019). Innate immunity and cnidarian-Symbiodiniaceae mutualism. Dev. Comp. Immunol. 90, 199–209. doi: 10.1016/j.dci.2018.09.020
Margulis, L. (1981). Symbiosis in Cell Evolution. New York: W.H. Freeman, doi: 10.1016/0305-1978(83)90070-4
McFall-Ngai, M., Heath-Heckman, E. A. C., Gillette, A. A., Peyer, S. M., and Harvie, E. A. (2012). The secret languages of coevolved symbioses: insights from the Euprymna scolopes-Vibrio fischeri symbiosis. Semin. Immunol. 24, 3–8. doi: 10.1016/j.smim.2011.11.006
McFarland, F. K., and Muller-Parker, G. (1993). Photosynthesis and retention of zooxanthellae and zoochlorellae within the aeolid nudibranch Aeolidia papillosa. Biol. Bull. 184, 223–229. doi: 10.2307/1542230
Melo Clavijo, J., Donath, A., Serôdio, J., and Christa, G. (2018). Polymorphic adaptations in metazoans to establish and maintain photosymbioses. Biol. Rev. 93, 2006–2020. doi: 10.1111/brv.12430
Melo Clavijo, J., Frankenbach, S., Fidalgo, C., Serôdio, J., Donath, A., Preisfeld, A., et al. (2020). Identification of scavenger receptors and thrombospondin-type-1 repeat proteins potentially relevant for plastid recognition in Sacoglossa. Ecol. Evol. 10, 12348–12363. doi: 10.1002/ece3.6865
Mies, M. (2019). Evolution, diversity, distribution and the endangered future of the giant clam–Symbiodiniaceae association. Coral Reefs 38, 1067–1084. doi: 10.1007/s00338-019-01857-x
Monteiro, E. A., Güth, A. Z., Banha, T. N. S., Sumida, P. Y. G., and Mies, M. (2019). Evidence against mutualism in an aeolid nudibranch associated with Symbiodiniaceae dinoflagellates. Symbiosis 79, 183–189. doi: 10.1007/s13199-019-00632-4
Moore, E., and Gosliner, T. (2014). Additions to the genus Phyllodesmium, with a phylogenetic analysis and its implications to the evolution of symbiosis. Veliger 51, 237–251. doi: 10.5281/zenodo.20947
Moore, E. J., and Gosliner, T. M. (2011). Molecular phylogeny and evolution of symbiosis in a clade of Indopacific nudibranchs. Mol. Phylogenet. Evol. 58, 116–123. doi: 10.1016/j.ympev.2010.11.008
Muscatine, L., and Porter, J. W. (1977). Reef corals: mutualistic symbioses adapted to nutrient-poor environments. Bioscience 27, 454–460. doi: 10.2307/1297526
Neubauer, E. F., Poole, A. Z., Neubauer, P., Detournay, O., Tan, K., Davy, S. K., et al. (2017). A diverse host thrombospondin-type-1 repeat protein repertoire promotes symbiont colonization during establishment of cnidarian-dinoflagellate symbiosis. Elife 6:e24494. doi: 10.7554/eLife.24494
Neubauer, E. F., Poole, A. Z., Weis, V. M., and Davy, S. K. (2016). The scavenger receptor repertoire in six cnidarian species and its putative role in cnidarian-dinoflagellate symbiosis. PeerJ 4:e2692. doi: 10.7717/peerj.2692
Obermann, D., Bickmeyer, U., and Wägele, H. (2012). Incorporated nematocysts in Aeolidiella stephanieae (Gastropoda, Opisthobranchia, Aeolidoidea) mature by acidification shown by the pH sensitive fluorescing alkaloid Ageladine A. Toxicon 60, 1108–1116. doi: 10.1016/j.toxicon.2012.08.003
Page, L. R. (1993). Development of behaviour in juveniles of Melibe leonina (Gastropoda; Nudibranchia). Mar. Behav. Physiol. 22, 141–161. doi: 10.1080/10236249309378843
Parker, G. M. (1984). Dispersal of zooxanthellae on coral reefs by predators on cnidarians. Biol. Bull. 167, 159–167.
Pelletreau, K. N., Worful, J. M., Sarver, K. E., and Rumpho, M. E. (2012). Laboratory culturing of Elysia chlorotica reveals a shift from transient to permanent kleptoplasty. Symbiosis 58, 221–232. doi: 10.1007/s13199-012-0192-0
Putz, A., König, G. M., and Wägele, H. (2010). Defensive strategies of Cladobranchia (Gastropoda, Opisthobranchia). Nat. Prod. Rep. 27, 1386–1402. doi: 10.1039/b923849m
Roth, M. S. (2014). The engine of the reef: photobiology of the coral-algal symbiosis. Front. Microbiol. 5:422. doi: 10.3389/fmicb.2014.00422
Rudman, W. B. (1981). The anatomy and biology of alcyonarian-feeding aeolid opisthobranch molluscs and their development of symbiosis with zooxanthellae. Zool. J. Linn. Soc. 72, 219–262. doi: 10.1111/j.1096-3642.1981.tb01571.x
Rudman, W. B. (1991). Further studies on the taxonomy and biology of the octocoral-feeding genus Phyllodesmium Ehrenberg, 1831 (Nudibranchia: aeolidoidea). J. Molluscan Stud. 57, 167–203. doi: 10.1093/mollus/57.2.167
Schmittmann, L., Franzenburg, S., and Pita, L. (2021). Individuality in the immune repertoire and induced response of the sponge Halichondria panicea. Front. Immunol. 12:689051. doi: 10.3389/fimmu.2021.689051
Sproles, A. E., Kirk, N. L., Kitchen, S. A., Oakley, C. A., Grossman, A. R., Weis, V. M., et al. (2018). Phylogenetic characterization of transporter proteins in the cnidarian-dinoflagellate symbiosis. Mol. Phylogenet. Evol. 120, 307–320. doi: 10.1016/j.ympev.2017.12.007
Stanley, G. D. (2006). Photosymbiosis and the evolution of modern coral reefs. Science 312, 857–858. doi: 10.1126/science.1123701
Stanley, G. D., and Helmle, K. P. (2010). Middle triassic coral growth bands and their implication for photosymbiosis. Palaios 25, 754–763. doi: 10.2110/palo.2010.p10-039r
Stanley, G. D., and Lipps, J. H. (2011). Photosymbiosis: the driving force for reef success and failure. Paleontol. Soc. Pap. 17, 33–59. doi: 10.1017/s1089332600002436
Suggett, D. J., and Smith, D. J. (2020). Coral bleaching patterns are the outcome of complex biological and environmental networking. Glob. Change Biol. 26, 68–79. doi: 10.1111/gcb.14871
Tambutté, S., Holcomb, M., Ferrier-Pagès, C., Reynaud, S., Tambutté, É, Zoccola, D., et al. (2011). Coral biomineralization: from the gene to the environment. J. Exp. Mar. Bio. Ecol. 408, 58–78. doi: 10.1016/j.jembe.2011.07.026
Togawa, Y., Junpei, S., Fukatsu, T., and Miura, T. (2019). Development of cerata in the cladobranchian sea slug Pteraeolidia semperi (Mollusca: gastropoda: nudibranchia). Zoolog. Sci. 36, 387–394. doi: 10.2108/zs190057
Tornabene, C., Martindale, R. C., Wang, X. T., and Schaller, M. F. (2017). Detecting photosymbiosis in fossil scleractinian corals. Sci. Rep. 7:9465. doi: 10.1038/s41598-017-09008-4
Umeki, M., Yamashita, H., Suzuki, G., Sato, T., Ohara, S., and Koike, K. (2020). Fecal pellets of giant clams as a route for transporting Symbiodiniaceae to corals. PLoS One 15:e0243087. doi: 10.1371/journal.pone.0243087
Wägele, H. (2004). Potential key characters in Opisthobranchia (Gastropoda, Mollusca) enhancing adaptive radiation. Org. Divers. Evol. 4, 175–188. doi: 10.1016/j.ode.2004.03.002
Wägele, H., and Johnsen, G. (2001). Observations on the histology and photosynthetic performance of “solar-powered” opisthobranchs (Mollusca, Gastropoda, Opisthobranchia) containing symbiotic chloroplasts or zooxanthellae. Org. Divers. Evol. 1, 193–210. doi: 10.1078/1439-6092-00016
Wägele, H., Raupach, M. J., Burghardt, I., Grzymbowski, Y., and Händeler, K. (2010). “Solar powered seaslugs (Opisthobranchia, Gastropoda, Mollusca): incorporation of photosynthetic units: a key character enhancing radiation?,” in Evolution in Action, ed. M. Glaubrecht (Berlin: Springer), 263–282. doi: 10.1007/978-3-642-12425-9_13
Wägele, H., and Willan, R. C. (2000). Phylogeny of the Nudibranchia. Zool. J. Linn. Soc. 130, 83–181. doi: 10.1111/j.1096-3642.2000.tb02196.x
Wakefield, T. S., and Kempf, S. C. (2001). Development of host- and symbiont-specific monoclonal antibodies and confirmation of the origin of the symbiosome membrane in a cnidarian-dinoflagellate symbiosis. Biol. Bull. 200, 127–143. doi: 10.2307/1543306
Wecker, P., Fournier, A., Bosserelle, P., Debitus, C., Lecellier, G., and Berteaux-Lecellier, V. (2015). Dinoflagellate diversity among nudibranchs and sponges from French Polynesia: insights into associations and transfer. Comptes Rendus Biol. 338, 278–283. doi: 10.1016/j.crvi.2015.01.005
Wooldridge, S. A. (2010). Is the coral-algae symbiosis really ‘mutually beneficial’ for the partners? Bioessays 32, 615–625. doi: 10.1002/bies.200900182
Yellowlees, D., Rees, T. A. V., and Leggat, W. (2008). Metabolic interactions between algal symbionts and invertebrate hosts. Plant Cell Environ. 31, 679–694. doi: 10.1111/j.1365-3040.2008.01802.x
Yorifuji, M., Takeshima, H., Mabuchi, K., Watanabe, T., and Nishida, M. (2015). Comparison of Symbiodinium dinoflagellate flora in sea slug populations of the Pteraeolidia ianthina complex. Mar. Ecol. Prog. Ser. 521, 91–104. doi: 10.3354/meps11155
Keywords: Nudibranchia, photosynthetic symbiosis, sea slugs, Symbiodiniaceae, symbiont recognition
Citation: Rola M, Frankenbach S, Bleidissel S, Sickinger C, Donath A, Frommlet JC, Greve C, Serôdio J, Preisfeld A, Melo Clavijo J and Christa G (2022) Cladobranchia (Gastropoda, Nudibranchia) as a Promising Model to Understand the Molecular Evolution of Photosymbiosis in Animals. Front. Mar. Sci. 8:745644. doi: 10.3389/fmars.2021.745644
Received: 22 July 2021; Accepted: 29 November 2021;
Published: 04 January 2022.
Edited by:
Marco Munari, Anton Dohrn Zoological Station, ItalyReviewed by:
Haoya Tong, Hong Kong University of Science and Technology, Hong Kong SAR, ChinaWong Yue Him, Shenzhen University, China
Copyright © 2022 Rola, Frankenbach, Bleidissel, Sickinger, Donath, Frommlet, Greve, Serôdio, Preisfeld, Melo Clavijo and Christa. This is an open-access article distributed under the terms of the Creative Commons Attribution License (CC BY). The use, distribution or reproduction in other forums is permitted, provided the original author(s) and the copyright owner(s) are credited and that the original publication in this journal is cited, in accordance with accepted academic practice. No use, distribution or reproduction is permitted which does not comply with these terms.
*Correspondence: Jenny Melo Clavijo, bWVsb2NsYXZpam9AdW5pLXd1cHBlcnRhbC5kZQ==; Gregor Christa, Y2hyaXN0YUB1bmktd3VwcGVydGFsLmRl
†These authors have contributed equally to this work