- 1The Centre for Ornithology, School of Biosciences, College of Life and Environmental Sciences, University of Birmingham, Birmingham, United Kingdom
- 2The Army Ornithological Society (AOS), Aldershot, United Kingdom
- 3The Royal Air Force Ornithological Society (RAFOS), High Wycombe, United Kingdom
- 4Department of Animal and Agriculture, Hartpury University, Gloucester, United Kingdom
- 5Biotrack Ltd., Wareham, United Kingdom
- 6Functional Genomics, Proteomics and Metabolomics Facility, School of Biosciences, College of Life and Environmental Sciences, University of Birmingham, Birmingham, United Kingdom
- 7Ascension Island Government Conservation and Fisheries Department, Georgetown, South Atlantic
- 8Centre for Ecology and Conservation, Biosciences, College of Life and Environmental Sciences, University of Exeter, Penryn, United Kingdom
- 9Royal Society for the Protection of Birds (RSPB), Sandy, United Kingdom
- 10Saint Helena National Trust, St Helena Island, South Atlantic
- 11Department of Life Sciences, MARE—Marine and Environmental Sciences Centre, University of Coimbra, Coimbra, Portugal
Seabirds are among the most threatened birds as a result of acute exposure to many anthropogenic threats. Their effective conservation requires a detailed understanding of how seabirds use marine habitats. Recently, one of the largest no-take marine reserves in the Atlantic was designated in tropical waters surrounding Ascension Island, on which the largest Atlantic population of sooty terns (Onychoprion fuscatus) breeds. Although they are the most abundant tropical seabird, they appear to have suffered marked population declines on Ascension Island as they have elsewhere. Here, we describe year-round movements and habitat use of male and female sooty terns between 2011 and 2015. On average, birds traveled 47,000 km during their 8 months of migration, during which they remained within 2,900 km of the island. They spent most of the day and night in flight, only touching down briefly on the ocean most likely to feed. Habitat suitability models successfully predicted foraging ranges of birds and their at-sea distributions varied considerably between seasons, years and sexes. Considerable variation in range overlap between birds and the new marine protected area (MPA) suggests that similar such studies of other marine taxa are urgently needed. The range of sooty terns mainly falls in the high seas outside of the new MPA, highlighting the very large areas that many oceanic seabirds forage across and the challenges their conservation present.
Introduction
Effective animal conservation first requires detailed knowledge of an organism’s spatial and temporal distributions. Seabirds present conservationists with challenges in this regard in part because of their high mobility (Norris and Pain, 2002; Oppel et al., 2018) and extensive foraging ranges, particularly when not breeding (Flint, 1991; Catry et al., 2009). In light of their perilous conservation status compared to other avian taxa (Votier and Sherley, 2017; Dias et al., 2019), characterizing seabird movements to inform conservation strategies is paramount, especially since many have undergone steep recent declines in population size worldwide (Croxall et al., 2012; Paleczny et al., 2015).
Seabirds are excellent bio-indicators of anthropogenic pressures on the marine environment (Furness and Monaghan, 1987). Their movements may also reflect the distributions of other key elements of marine food webs (Phillips et al., 2009). This is especially true of tropical seabirds, many of which have near-obligate relationships with cetaceans and predatory fish such as tuna that drive prey species to the surface where seabirds feed on them (Veit and Harrison, 2017; Reynolds et al., 2019) in so called “facilitated foraging” (Maxwell and Morgan, 2013). Understanding seabird movements is therefore of high importance for planning and assessing the effectiveness of marine reserves (Lascelles et al., 2016; Anon 2018; Oppel et al., 2018).
While at-sea distributions of far-ranging seabirds are difficult to assess through direct observation, tagging allows their movements to be studied remotely and in great detail (Fehlmann and King, 2016; Bernard et al., 2021). Here, we deploy geolocator (GLS) tags on sooty terns (Onychoprion fuscatus) nesting within one of the world’s largest marine reserves centered on Ascension Island in the Atlantic Ocean. Sooty terns are long-lived and pan-tropically distributed (Schreiber et al., 2002), and they are near-obligate commensals of tuna, cetaceans and other sub-surface predators (Au and Pitman, 1986). With a global population of 21–25 million birds, their conservation status is of “Least Concern” but under the Ascension Island Government’s (AIG’s) Schedule of the Wildlife Protection Ordinance they are a “Protected Species.” Many sooty tern populations have declined significantly since the mid-1,800s (Hughes et al., 2017a), including our study population on Ascension Island (Reynolds et al., 2019) that is currently the largest breeding colony of this species in the Atlantic Ocean. In August 2019, the entirety of Ascension Island’s 200 nautical mile Exclusive Economic Zone (EEZ)—an area of approximately 445,000 km2—was designated as an MPA that is closed to all commercial fishing. Much remains to be investigated in assessing this MPA’s benefits to marine taxa. Here, we investigate year-round movements of this iconic Ascension seabird over 5 years. Multi-month and multi-year movements of both sexes are essential to evaluate the consistency in the usage of at-sea areas. Ascension Island is the only location where sooty terns breed sub-annually (i.e., every 9.6 months; Reynolds et al., 2014) resulting in birds over many years experiencing highly variable foraging conditions that are driven seasonally (Soanes et al., 2021). We relate their at-sea locations and behaviors predominantly outside of their breeding seasons to prevailing meteorological and oceanographic conditions, season and commercial fishing activities targeting the surface-schooling skipjack tuna (Katsuwonus pelamis) and yellowfin tuna (Thunnus albacares) with which they commonly associate (Reynolds et al., 2019). We use habitat modeling to define suitability of at-sea habitats and relate this to bird distributions. Finally, we examine the extent of overlap between terns and the MPA, and assess the latter’s potential to protect the wider ecological community.
Materials and Methods
Field Methods
Study Site and Population, and Conservation
The study was carried out at sooty tern breeding colonies at Mars Bay and Waterside on Ascension Island in the South Atlantic (07°57′S, 14°24′W). Ascension is a 97 km2 volcanic island that is one of three making up the UK Overseas Territory (UKOT) of St Helena, Ascension and Tristan da Cunha. Ascension Island is geographically isolated with St Helena being its nearest neighbor 1,300 km to the southeast. The island contains several Important Bird and Biodiversity Areas (IBAs), including the sooty tern nesting grounds at the “Wideawake Fairs” (IBA SH009). This population of sooty terns has been studied intensively over many decades, including a detailed account of their breeding biology (Ashmole, 1963).
As is typical of many seabird species (Croxall et al., 2012; Paleczny et al., 2015), many sooty tern populations have declined markedly in recent decades (reviewed in Hughes et al., 2017a), including that on Ascension Island. Hughes et al. (2017a) compared its breeding population on the island between 1942 and 2005, and estimated a decline of approximately 84% from 2.35 million to 370,000 birds. This decline is unprecedented on the island; an earlier estimate of the population in 1877 suggested that it contained ∼2.43 million birds. Seabirds on the island have been heavily impacted by predation by invasive species such as domestic cats (Felis silvestris catus) (Hughes et al., 2008), black rats (Rattus rattus) (Hughes et al., 2019) and common mynas (Acridotheres tristis) (Hughes et al., 2017b). As a result, the Royal Society for the Protection of Birds (RSPB) launched a seabird restoration project in 2002 to promote the recovery of these seabird populations (Pickup, 1999). The sooty tern population has remained stable since the eradication of cats (Reynolds et al., 2019), but does not appear to be increasing like Ascension’s other ground-nesting seabird species. While MPAs might meet the at-sea conservation needs of some seabird species, on-island conservation efforts need to persist if sooty tern numbers are not to decline further.
Geolocator Tag Deployment and Retrieval
We deployed tags on birds at the edges of breeding colonies to minimize disturbance of breeding birds. As a result, it is possible that our sample might have included more recent recruits or inexperienced birds that are often forced into nesting on the periphery. Birds on the margins are significantly more likely to have eggs depredated by common mynas than birds laying in colony centers that are inaccessible to mynas (Hughes et al., 2019). Sooty terns usually lay only one egg and, once lost to predation, they usually abandon the breeding colony and initiate migratory movements (Ashmole, 1963; Schreiber et al., 2002). While we did not monitor ongoing breeding attempts of birds after tag deployment to minimize their further disturbance, we assumed that few birds in our study would have raised chicks for approximately 66 days to the point of fledging, meaning that the geolocation data reflect mostly non-breeding movements and a fraction of the 29-day incubation period before tag retrieval (see Figure 3 of Reynolds et al., 2014 for further details of relative durations of different phases of their sub-annual cycles). Because the estimated spatial error of geolocator tags can be approximately 200 km (Phillips et al., 2004), we were unable to assess fine-scale breeding movements around the breeding colony. Therefore, geolocation data from our tagged birds reflect their year-round at-sea movements, and their use of the large MPA.
We captured adult sooty terns with long-handled landing nets while they were in the latter stages of incubation. Stage of incubation was assessed from embryonic stage of recently depredated eggs nearby. We attached a total of 40 LAT290 GLS tags supplied by Biotrack Ltd. (Wareham, United Kingdom) (hereafter “Biotrack tags”) in March 2011 (n = 20) and December 2012 (n = 20). We subsequently attached a further 50 Intigeo-C65 GLS tags supplied by Migrate Technology Ltd. (Cambridge, United Kingdom) (hereafter “Migrate tags”) in September 2013 (n = 10), May 2014 (n = 20) and April 2015 (n = 20). All GLS tags were attached to birds under British Trust for Ornithology (BTO) Unconventional Methods Permits (#s 2483 and 4395, respectively) issued to CWP who also holds a BTO ringing permit (A4318) under which all adults were ringed. Tags were secured on the left leg of each bird where they were held in place with one (for Migrate tags) or two (for Biotrack tags) cable tie(s) passed around the device in the former case and threaded through the metal cradle holding the device in the latter case. Cable ties held the tag securely in place against a blank metal ring covered in tesaTM self-amalgamating tape (tesa UK Ltd., Milton Keynes, United Kingdom). The combined weight of the blank metal ring, tesa tape, cable tie(s) and GLS tag was 1.7 g for Migrate tags and 1.9 g for Biotrack tags, amounting to 0.90 and 1.01%, respectively, of sooty tern body mass (mean: 191 g, range: 145–296 g, n = 748 birds; EHKL, unpubl. data) (see Supplementary Figure 1 showing each tag type in situ on birds). A color ring identifying the year of deployment (Supplementary Table 1) was placed between the ring carrying the tag and the foot of each bird to prevent the tag from abrading tissue of the foot. Each bird was also ringed with a uniquely numbered BTO or South African Ringing Scheme (SAFRING) metal ring (Supplementary Table 1) and a matching color ring on the right leg.
At the time of tag deployment we sexed each bird by taking a small (i.e., approximately 250 μL) blood sample by venepuncture from the left brachial vein under local ethical approval from the AIG and also from the Animal Welfare Ethical Review Board (AWERB) of the University of Birmingham, Birmingham, United Kingdom. Molecular sexing of each bird from its blood sample was carried out by FTH at the University of Birmingham following each breeding season (see Reynolds et al., 2008 for further details).
We returned to the island every 9.6 months to coincide with the sub-annual breeding schedule of birds (Reynolds et al., 2014), to monitor the breeding ecology of the population and to capture adults to deploy/recover GLS tags. We targeted areas of sub-colonies of birds where they were attending eggs because this was when birds were most reluctant to leave nests when disturbed. Birds carrying tags were detected relatively easily because they were the only ones in the breeding population that carried matching color rings on left and right legs. They were caught as per earlier capture for tag deployment and immediately the GLS tag was removed by severing the cable tie(s) holding it to the blank metal ring on the bird’s leg. The tesa tape was removed from the ring and the bird’s leg was checked for any tissue trauma before release. Recovered tags were immediately placed into a black film canister where they remained until data were downloaded.
Data Processing and Analysis
At-Sea Locations and Behaviors
Geolocations of each bird were estimated using the tripEstimation package (Sumner and Wotherspoon, 2016) in R v3.5.1 (R Core Team, 2018) and following the method of Thiebot and Pinaud (2010). Raw geolocation data were filtered by removing positions (1) 15–20 days before and after equinoxes, (2) with obvious interference at dawn or dusk, and (3) when flight speeds were higher than 30 km per h applying backward/forward speed filtering following approaches in previous research dealing with geolocation estimations from light level data (e.g., McConnell et al., 1992; Zajkova et al., 2017). Retained geolocations of each bird were examined using the adehabitatHR package in R (Calenge, 2006) by generating kernel utilization distribution (UD) estimates with a smoothing parameter (h) chosen via Least Squares Cross Validation (LSCV) and a cell size of 1° (Calenge, 2006). We considered the 50 and 95% kernel UD contours to represent the core foraging range (CFR) and the home range (HR), respectively. To investigate spatial segregation within and among tracked cohorts, the overlaps between kernel CFRs of different (1) sexes and (2) study years were calculated using the kerneloverlap function and Bhattacharyya’s affinity (BA) method of the adehabitatHR package (Calenge, 2006).
Diel activity patterns of sooty terns were characterized using both immersion and light-level data recorded by GLS tags on each bird. Tags were set up prior to deployment to test for saltwater immersion every 3 s using two electrodes and they stored the number of positive tests from 0 (when continuously dry) to 200 (when continuously wet) at the end of each 10-min recording period. Tags also measured light level every minute and they stored the maximum (truncated at a value of 64) at the end of each 10-min recording period. Each of these 10-min blocks was categorized as either daylight or darkness based upon the timing of nautical twilight (after Mackley et al., 2010). Immersion data were categorized as having been collected during either the day or the night based upon light-level data. Immersion data allowed us to estimate the proportion of time during the day and night that birds spent on the ocean surface as opposed to flying or on land. Ashmole (1963) described in great detail the foraging behavior of Ascension sooty terns when they associate with cetaceans and large predatory fish that drive small fish prey to the surface where they, as well as many other tropical seabirds, seize them from, or just above, the ocean surface. Periods that birds spent on the ocean surface were identified as any continuous sequence of 10-min blocks when birds spent at least 3 s sitting on the water, while a continuous sequence of dry (0) values was interpreted as a period of sustained flight. We were then able to calculate for each bird the (1) percentage of time it spent on the ocean surface, and (2) average duration of flying bouts when the device was continuously dry for at least one 10-min period (after Phalan et al., 2007; McKnight et al., 2011, 2013).
Habitat Suitability Models
Many environmental variables have been examined in modeling and analyzing the distribution of seabirds. We selected those most relevant to the movement ecology of sooty terns as listed in Table 1 of Tremblay et al. (2009). They reviewed 218 such studies and we selected variables that were considered in many of them. Therefore, to characterize the oceanographic conditions in areas used by the tracked terns, we extracted: (1) ocean floor depth (DEP), (2) sea surface temperature (SST), (3) sea surface chlorophyll a concentration (CHL), (4) wind speed (WSP), and (5) distance to colony (DCOL) (see Supplementary Table 2 for further details). Monthly averages were extracted for the dynamic variables (i.e., SST, CHL, WSP). Anomalies of variables 2 and 3 (i.e., CHLA and SSTA, respectively) were computed by calculating the difference between the mean value of the variable for a given month and the observed average for that month over a 12-year period (2002–2013). Variable 5 was computed as the minimum Euclidean distance to the breeding colony on Ascension Island. All environmental predictors were re-sampled onto a standard 1° × 1° grid to match the spatial resolution of the tags and composites of the environmental predictors corresponding to the tracking period were computed on the dynamic variables for habitat modeling purposes.
Model construction, training and testing were performed with Maximum Entropy (MaxEnt) modeling (version 3.3.3) based on presence-only data (after Phillips et al., 2006).1 The MaxEnt method does not require absence data for the species being modeled but, instead, it uses background environmental data from the entire study area (i.e., a colony buffer with a radius equal to the maximum foraging range of all tracked birds; 3,500 km). This method performs well compared with alternatives (Elith et al., 2006) and when modeling habitat use from tracking data (Louzao et al., 2012; Quillfeldt et al., 2013; Afán et al., 2014). Locations of individual sooty terns within the CFR (i.e., 50% kernel UDs) were divided into training and test data by setting aside approximately 30% of the dataset for evaluation of the predictive performance of the fitted models (Araújo and Guisan, 2006). We ran MaxEnt on the presence-only positions 50 times using random (bootstrap) subsamples with duplicates removed (i.e., only one presence per cell was retained for analysis) and returning habitat suitability values for each grid cell on a logistic scale (i.e., between 0 and 1). We then calculated the mean of the 50 MaxEnt predictions for each cell to obtain an average prediction and coefficient of variation (Édren et al., 2010). The MaxEnt program was run separately for each sex and study year (2011–2015), yielding 10 habitat models in total. From the results of MaxEnt program the Jackknife chart was used to evaluate the contribution of each environmental layer to the final result, thereby providing the explanatory power of each variable when used in isolation. Model performance was assessed using the area under the receiver operator curve (AUC). The AUC estimates the likelihood that a randomly selected point of presence is located in a raster cell with a higher probability value for species’ occurrence than a randomly generated point. Fitted models are generally interpreted from AUC values as follows:>90%—excellent; 80–90%—good; 70–80%—adequate; 60–70%—poor; and 50–60%—invalid. All model evaluation statistics and optimal thresholds were calculated using the package PresenceAbsence in R (Freeman and Moisen, 2008).
Overlap With Fishing Effort and the Marine Protected Area
Foraging sooty terns commonly associate with surface-schooling predatory fish such as yellowfin and skipjack tuna that are predominantly caught by purse-seiners and drifting long-lines in the central and eastern tropical Atlantic Ocean (Miyake and Kebe, 1996). To assess whether the foraging distributions of tagged birds overlapped with areas of high fishing effort, we accessed yearly gridded fishing effort statistics for these fisheries in the tropical Atlantic within the maximum migration range and tracking period of sooty terns between 2011 and 2015 (Dureuil et al., 2018).2 Global Fishing Watch (GFW) provides 0.1° gridded information on daily fishing effort (h) of vessels transmitting their location by automatic identification system (AIS).
The kerneloverlap function and BA method of the adehabitatHR package (Calenge, 2006) was used to compute the overlap between the CFRs and the fishing efforts of (1) purse-seiners and (2) long-liners that target surface-schooling tuna species with which sooty terns associate when foraging, and (3) the MPA. To test if birds’ foraging distributions overlapped more than by chance with fisheries’ distributions, correlated random walks within the individual home ranges were simulated using the simm.crw function from the adehabitatLT R package (Calenge, 2006). To generate each random track, we split the real track into two parts: one from the colony to the most distant location and one back to the colony. We generated 50 simulations per real track (after Hindell et al., 2020) which were then combined to estimate the simulated kernel UDs. We then extracted the 50% kernel UDs (i.e., the CFR) for those random tracks (simulated UDs) and measured the overlap with the distributions of (1) purse-seiners and (2) long-liners.
Statistical Analysis
The following parameters (response variables) were calculated for the yearly distribution of each individual:% time on ocean surface (1) at night and (2) during the day, time in (3) nocturnal, and (4) diurnal flight (h), proportion of overlap in CFR with (5) other individuals within the same sex-year cohort (i.e., mean overlap of each individual with others of the same sex), (6) in the opposing sex cohort for the same year (i.e., mean overlap of each individual with others of the opposite sex), (7) purse-seiners, (8) long-liners, and (9) the MPA. Distance flown (km) (10), range (i.e., maximum distance from the colony; km) (11) and trip duration (months) (12) were also computed yearly for the non-breeding distribution of each individual. Generalized linear mixed models (GLMMs) were run using the lme4 package in R (Bates et al., 2015) to test the effect of (1) sex and (2) study year (2011–2015) as independent variables on these 12 dependent variables. The GLMMs with proportions as the response variable were fitted using a beta binomial error family and others using a Gaussian error distribution. Individual bird identity (ID) was included as a random effect to control for any pseudo-replication issues (Zuur et al., 2007). General linear models (GLMs) were used to test the effect of study year (the independent variable) on the dependent variables (1) SSTA and (2) CHLA within 200 nautical miles of Ascension Island. They were also used to test the effect of observed vs. random UDs on the overall mean overlap with (1) purse-seiners and (2) long-liners. Post hoc analyses were performed using Estimated Marginal Means (EMMs) and Tukey’s p-value adjustment under the emmeans package in R (Lenth, 2020).
All results are reported as means ± 1 standard deviation (SD), unless otherwise stated. All statistical analyses were carried out in R (R Core Team, 2018). Response variables were tested for normality (Q-Q plots) and homogeneity (Cleveland dotplots) before each statistical test and transformed when needed (Zuur et al., 2010). All statistical tests were performed using an alpha threshold of 0.05.
Results
At-Sea Locations and Behaviors
Of 90 tags deployed on sooty terns between 2011 and 2015, 30 were recovered from 17 males and 13 females (Supplementary Table 1). An additional two tagged birds were observed at Mars Bay but could not be approached closely enough to allow recapture. At tag removal no bird was found to have tissue trauma to their legs as a result of tag deployment. Birds traveled 46984.4 ± 5834.2 km to a maximum distance of 2903.7 ± 856.9 km from the island during 8.1 ± 0.6 months on post-breeding migration (Supplementary Figure 2). Data from integrated saltwater immersion sensors in GLS tags indicated that birds spent the majority of the day (97.3%) and night (99.9%) in flight and little time on the ocean surface (Table 1). This was particularly apparent in 2013 and 2014, being most marked in males in 2013 (Tables 1, 2).
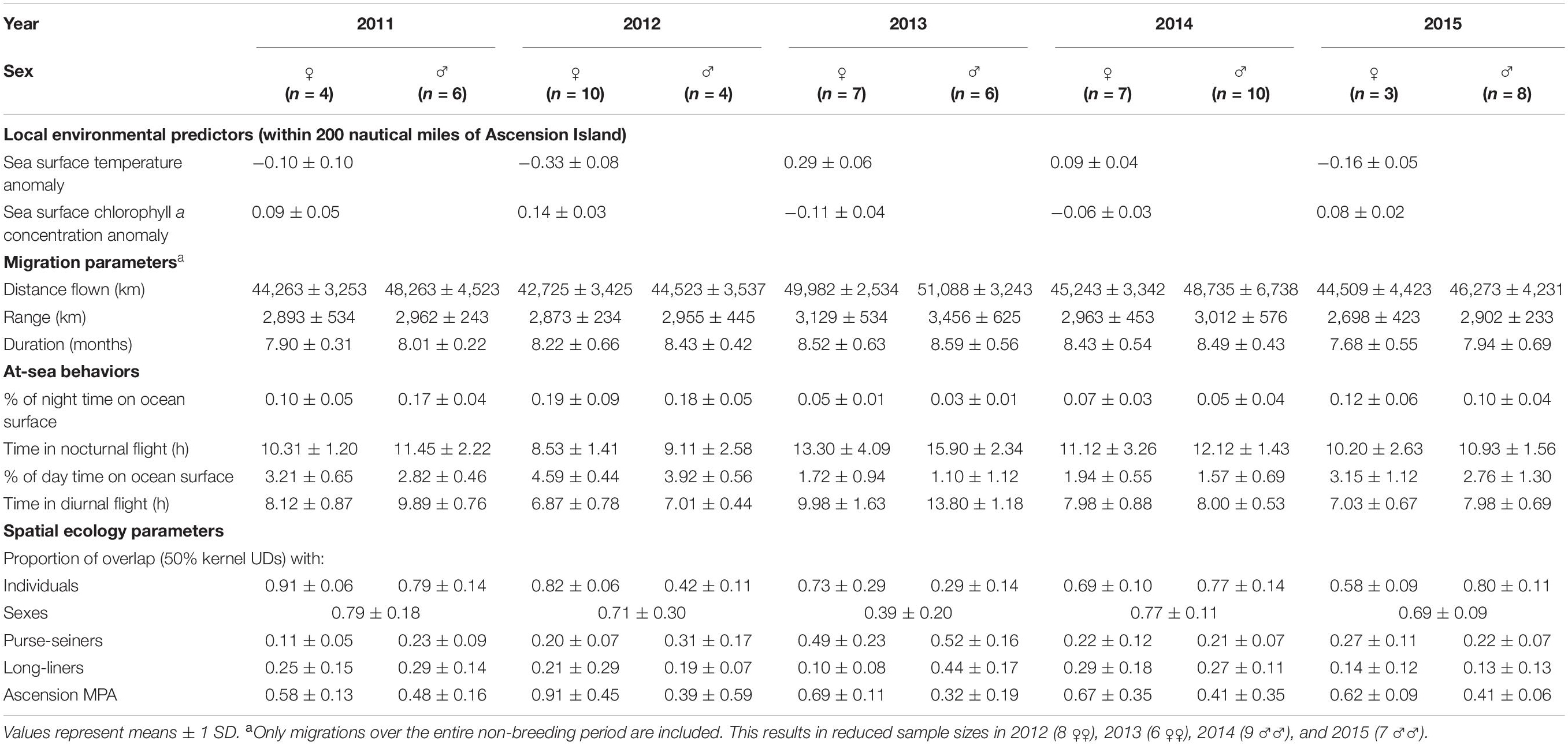
Table 1. Local environmental predictors in the Ascension Island MPA, migration parameters, at-sea behaviors and spatial ecology parameters related to movements of female (♀) and male (♂) sooty terns that had bred on Ascension Island between 2011 and 2015.
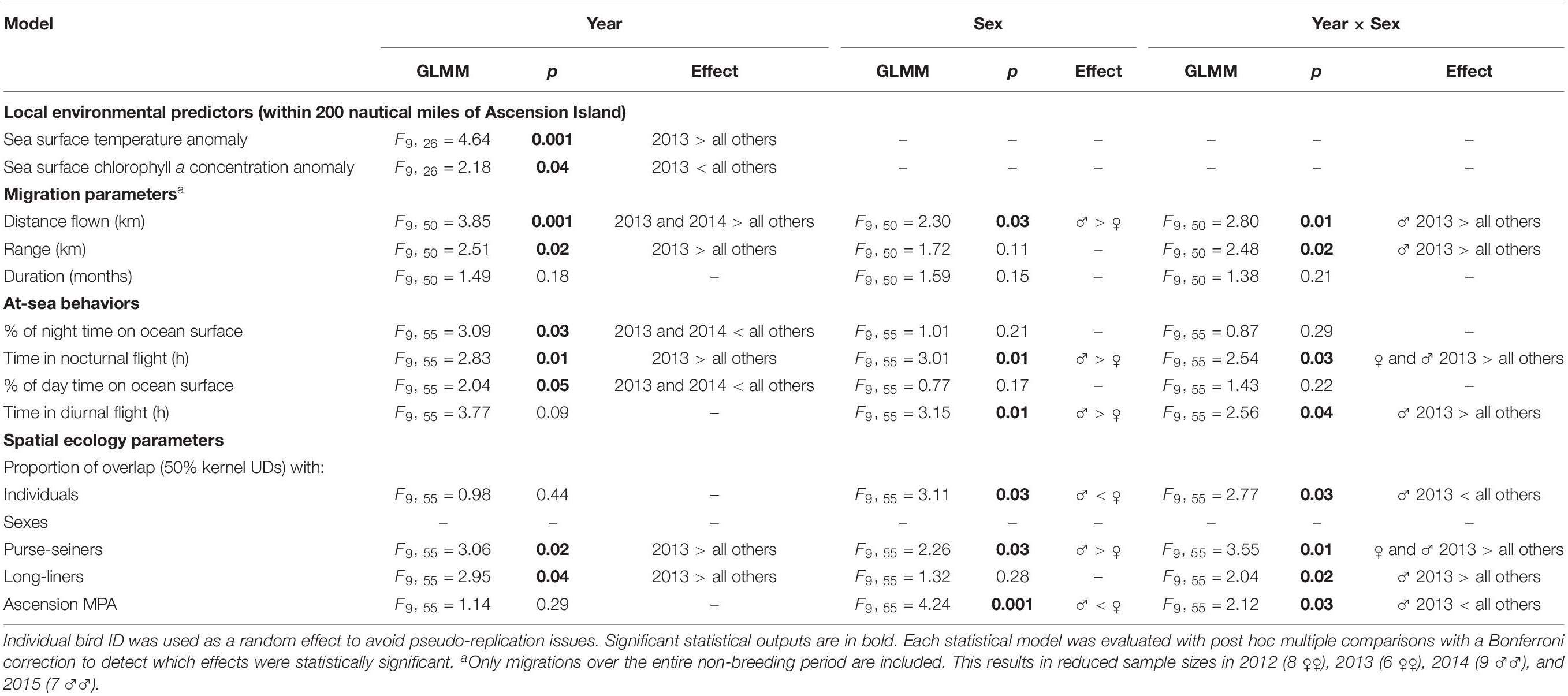
Table 2. Generalized linear mixed models (GLMMs) testing the effect of year (2011–2015), sex and the year × sex interaction on the local environmental predictors within the Ascension MPA, migration parameters, at-sea behaviors and spatial ecology parameters of sooty terns, as detailed in Table 1.
There were noticeable differences between sexes and years in the at-sea distributions of sooty terns (Figure 1). For example, males in 2013 undertook significantly longer migrations and traveled further from the island than birds in other sex-year combinations (Tables 1, 2). In 2011 both sexes migrated north-west from Ascension Island to foraging areas over the Mid-Atlantic Ridge north of the equator (Figure 1). In contrast, both sexes appeared to disperse even more widely in 2013 and 2014 with females concentrated to the north and south of the island along the Mid-Atlantic Ridge, but with males favoring the Gulf of Guinea, particularly in 2013. Both sexes remained closer to the Ascension Island MPA in 2012 (Figure 1). Between-individual overlap in the CFRs of tracked birds was generally higher in females than in males, especially in 2013 (Table 1). From a monthly perspective, between-sex overlap in the CFRs varied between 46.7% in September and 81.2% in February (Figure 2).
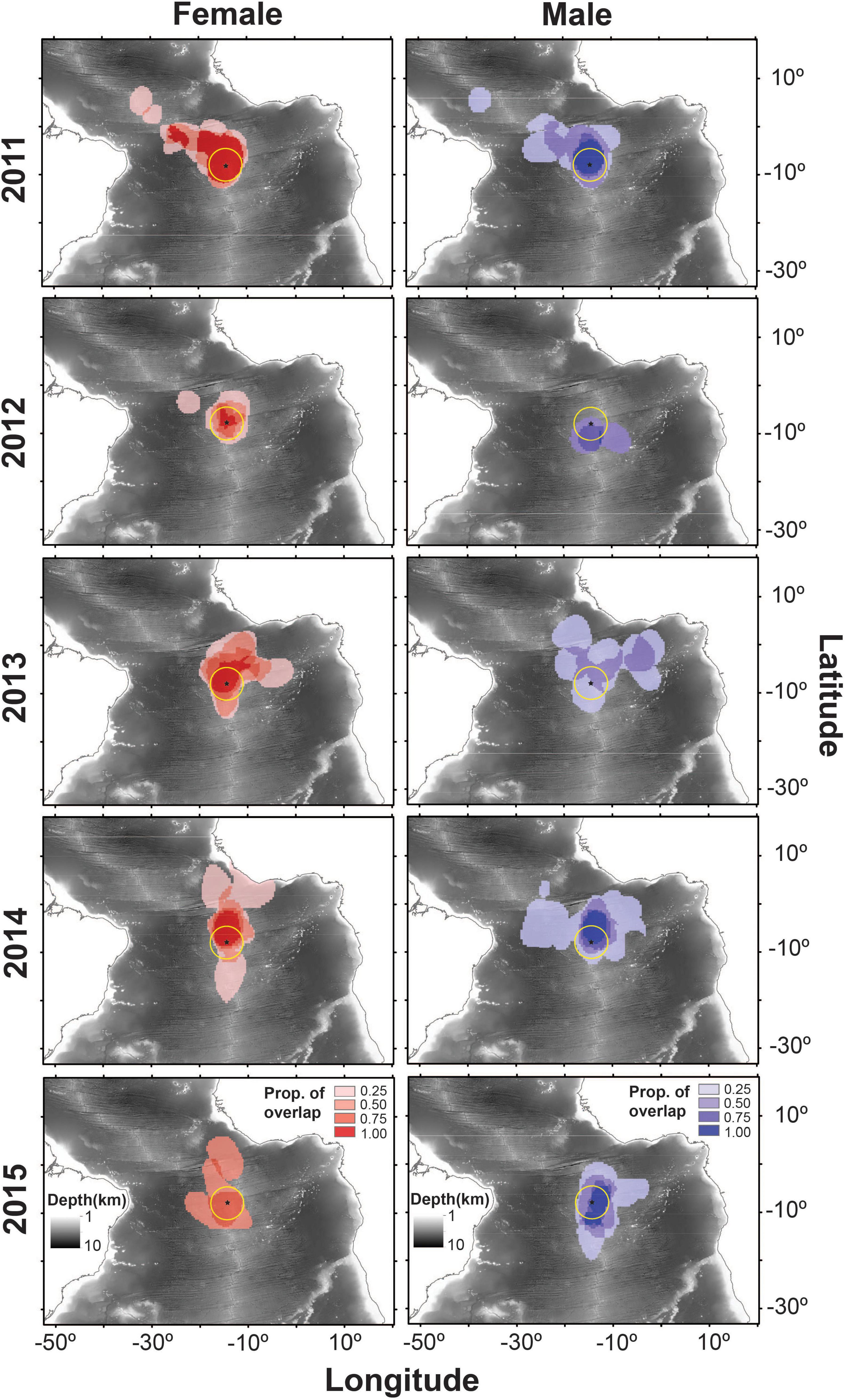
Figure 1. Overlap of the individual 50% kernel utilization distributions (UDs) of female (red) and male (blue) sooty terns breeding on Ascension Island and then tracked during migration between 2011 and 2015. The yellow circle centered on the island is the MPA that extends 200 nautical miles around Ascension Island. Ocean depth is represented in the background.
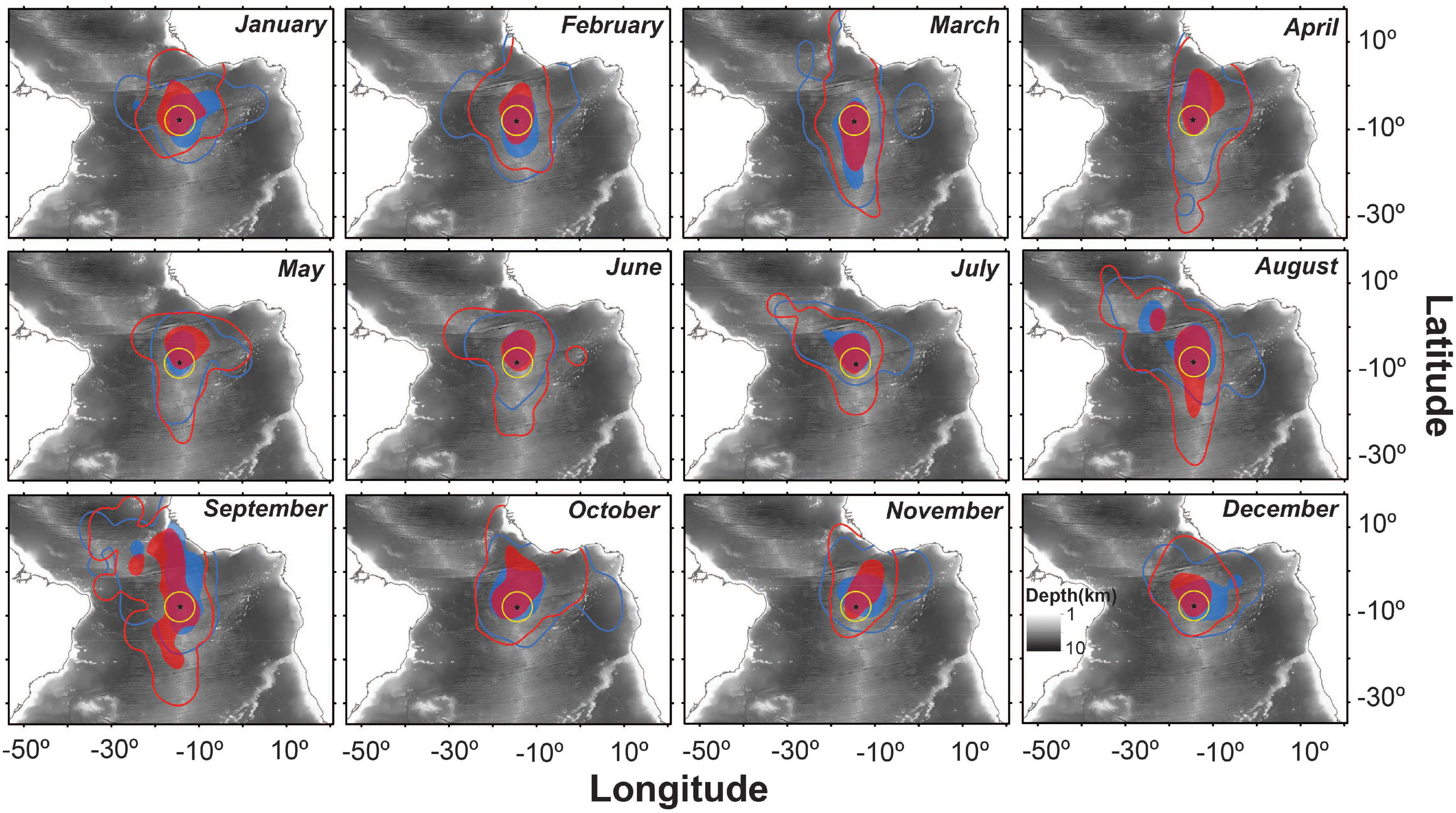
Figure 2. Monthly 50% (filled polygons) and 95% (line) kernel utilization distributions (UDs) of female (red) and male (blue) sooty terns from Ascension Island carrying GLS tags between 2011 and 2015. The yellow circle represents the MPA that extends 200 nautical miles around Ascension Island. Ocean depth is represented in the background.
Habitat Suitability Models
All habitat suitability models showed a good to excellent ability to predict the observed CFRs (i.e., 50% kernel UDs) of female and male sooty terns. Reduced DCOL and SSTA were the best predictors of the at-sea distribution in both cases, especially in 2012 when DCOL explained approximately 50% of the foraging distribution of individuals (Figure 3). In contrast, the distribution of males in 2013 was explained more by areas of higher WSP and lower SSTA but less by shorter DCOL when compared to other study years and the distributions of females (Figure 3).
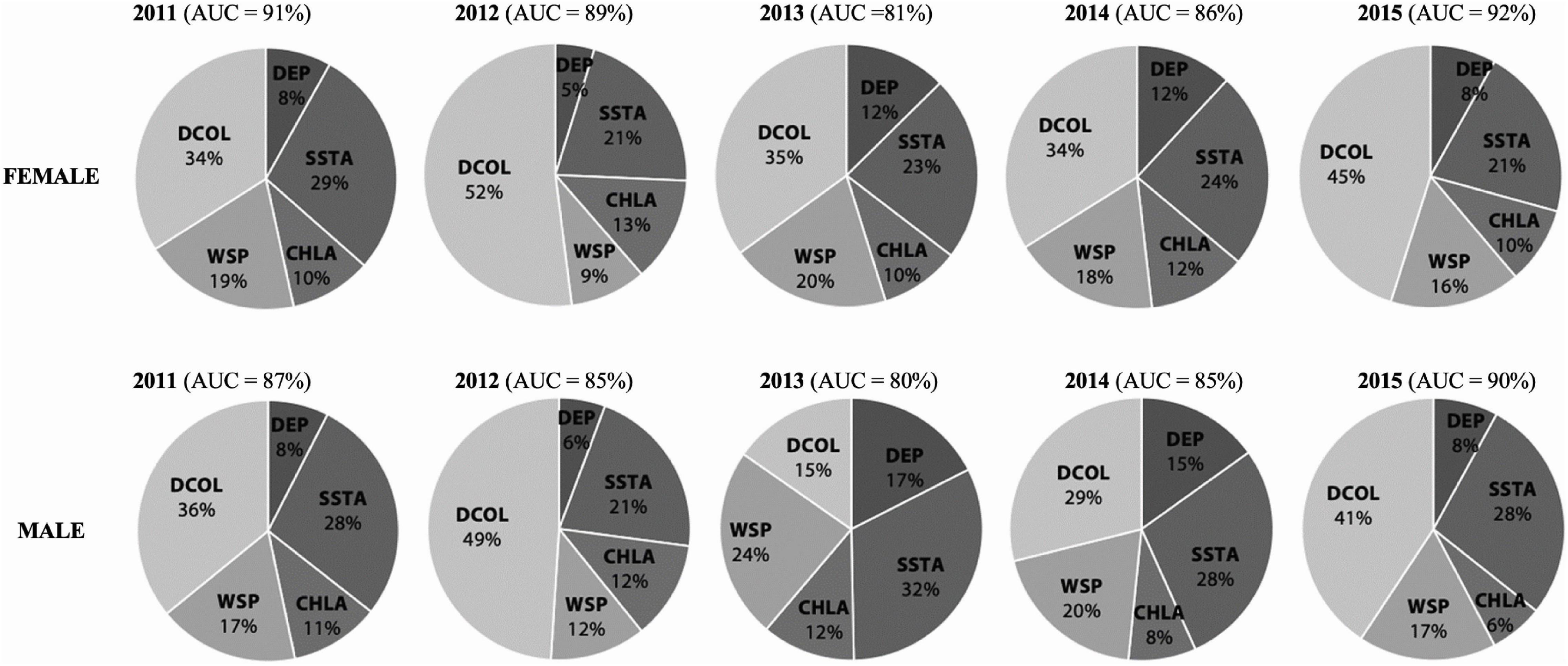
Figure 3. Percentage contributions of environmental predictors from the MaxEnt habitat models to the foraging distributions (locations inside 50% kernel utilization distribution [UD]) of female and male sooty terns from Ascension Island between 2011 and 2015. DCOL—distance to colony, DEP—ocean floor depth, SSTA—sea surface temperature anomaly, CHLA—sea surface chlorophyll a concentration anomaly, WSP—wind speed, AUC—area under receiver operating characteristic curve (i.e., an evaluation of a model’s performance).
Overlap With Fishing Effort and the Marine Protected Area
Mean overlap of sooty tern CFRs with fisheries was significantly higher than that of simulated UDs, both for purse-seiners [34.2 ± 1.2% vs. 22.1 ± 0.9%; GLMM, F(1, 130) = 5.69, p = 0.02] and long-liners [26.9 ± 1.6% vs. 19.3 ± 1.1%; GLMM, F(1, 130) = 4.82, p = 0.03]. Birds were occupying foraging habitat non-randomly and were sharing areas also occupied by fishing vessels that target surface-schooling tuna. This was particularly true of both females and males in 2013 whose foraging ranges overlapped with 0.49 and 0.52, respectively, of the UDs of purse-seiners (Table 1 and Figure 4). Males in 2013 had CFRs that overlapped more significantly with purse-seiners and long-liners than did CFRs of females in the same year and of birds from other years (Tables 1, 2 and Figure 4).
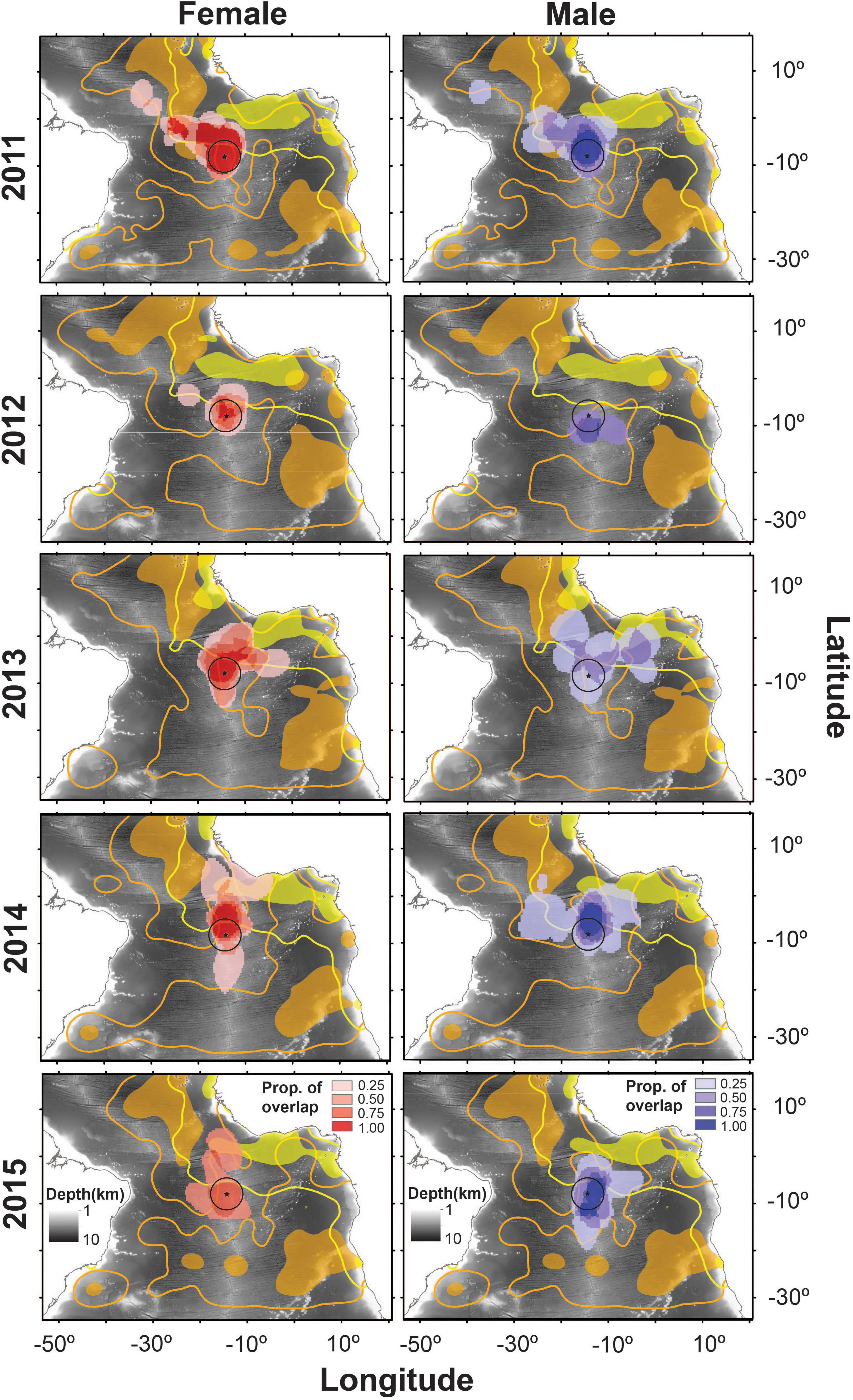
Figure 4. Overlap of the individual 50% kernel utilization distributions (UDs) of female (red) and male (blue) sooty terns from Ascension Island (tracked between 2011 and 2015) with the 50% (filled area) and 95% (contour line) kernel UDs of purse-seiners (yellow) and drifting long-liners (orange). Fisheries distribution data were extracted from https://globalfishingwatch.org/map-and-data/. The black circle represents the MPA that extends 200 nautical miles around Ascension Island. Ocean depth is represented in the background.
The overlap of the CFRs of birds with the MPA generally did not differ between years but in 2013 those of males overlapped significantly less than for all others (Table 2). Birds did appear to migrate widely in search of food (Supplementary Figure 2) with between 0.32 and 0.91 of their CFRs overlapping with the MPA (Table 1).
Discussion
Pelagic seabirds undergo some of the most extensive migrations of any vertebrate taxa (e.g., Shaffer et al., 2006; Egevang et al., 2010; Weimerskirch et al., 2015). Likewise, sooty terns from the Ascension Island colony undergo extensive post-breeding migration that justifies their characterization as ocean “wanderers” (Feare, 2017). Their estimated total migration distances are similar to those of post-breeding sooty terns tracked from Bird Island in the Seychelles in the western Indian Ocean (i.e., 46,984 ± 5,834 km cf. 52,503 ± 6,546 km; Jaeger et al., 2017). However, migrating Ascension birds ranged closer to their breeding location than did Seychelles’ birds (i.e., 2,904 ± 857 km cf. 4,423 ± 1,190 km; Jaeger et al., 2017). Whether this is attributable to differences in productivity and thus prey availability in foraging areas (Weimerskirch, 2007), or to intensity of interference competition from conspecifics (Oppel et al., 2015) and/or heterospecifics (Weimerskirch et al., 2005) is unclear. Duration of migration of our study birds appeared to be relatively fixed (i.e., 7.9–8.6 months; Table 1), perhaps a consequence of their sub-annual breeding (Chapin, 1954) compared with annually breeding sooty terns that may have more temporal flexibility (Reynolds et al., 2014).
Data from the geolocator (GLS) tags indicated that birds spent the vast majority of the day and night in flight, and little time, as revealed by immersion data, on the ocean surface where they likely feed on small fish driven by cetaceans and large predatory (Maxwell and Morgan, 2013; Table 1). Therefore, they may be among the most aerial of all bird species. Unusually for a seabird, sooty terns appear to have poorly waterproofed feathers because of the reduced lipid content of their preen oil compared with other tern species that land on the water (Johnston, 1979). An inability to repel water from plumage effectively can have lethal consequences (Dinsmore, 1972) as well as non-lethal ones such as negatively impacting their flight capabilities as they expend much energy attempting to take-off (Mahoney, 1984).
There were distinct differences between at-sea distributions of sooty terns according to their sex and year (Figure 1). Compared with polar and temperate waters, tropical oceanic waters are less productive and food availability is often patchy and less predictable (Weimerskirch, 2007). The distribution of avian and mammalian marine consumers therefore often reflects spatially dynamic patches of high prey availability (Moore and Kuletz, 2019). Oceanographic features such as eddies, water mass boundaries and currents can influence the at-sea distributions of seabirds (Schneider, 1991). Processes such as up- and downwelling can also result in the accumulation of planktonic organisms, providing predictable sources of prey for fish that in turn attract seabird and mammalian consumers (Catry et al., 2009; Weber et al., 2021). The highly variable at-sea distributions of our birds between years suggest complex relationships with ocean productivity and circulation. In 2013, for example, terns dispersed more widely during the significant elevation in sea surface temperature (i.e., high SSTA) and subsequent low productivity (i.e., low chlorophyll α concentration or CHLA) inside the Exclusive Economic Zone (EEZ) (Table 2 and Figure 1). Further research should therefore assess the degree of reliance of sooty terns on ocean productivity mediated through their near-obligate foraging associations with cetaceans and large predatory fish (Maxwell and Morgan, 2013). This will require detailed monitoring of movements of sub-surface predators in parallel with avian movements. Such research is especially pertinent in the face of ocean warming. Our findings in 2013 suggest that as the SSTA increases, birds appeared to migrate longer distances and thus to overlap less with the designated Ascension marine protected area (MPA). Conversely, the global abundances of yellowfin and skipjack tuna are predicted to increase in tropical waters as oceans warm (Erauskin-Extramiana et al., 2019).
Seasonal variation in the CFRs of males and females most likely reflects fluctuations in the seasonal availability of fish prey. Sooty terns in the tropical Atlantic predominantly feed on small fish such as halfbeaks (Oxyporhamphus micropterus), blue flying fishes (Exocoetus volitans), redlip blennies (Ophioblennius atlanticus), Simony’s frostfishes (Benthodesmus simonyi) and hairtails (Trichiurus spp.) (Ashmole, 1963), but also squid (Teuthida) (Reynolds et al., 2019). Significant overlap of CFRs between the sexes across years suggests that they are employing similar migratory strategies in response to food availability. There is no evidence of sex differences in their diet but future research might usefully examine such foraging strategies in greater detail such as by month (Figure 2).
In habitat suitability models explaining their core foraging ranges (CFRs), distance to colony (DCOL) featured as a major driver of sooty tern distribution in both sexes across all years of the study (Figure 3). This is unsurprising given the geometry of dispersal following breeding on the island, migration and return to the same point to breed in subsequent seasons. Fewer cells at shorter distances from the island that all birds need to pass through and a much larger potential foraging area as distance from the island increases represent the “null model” of foraging distribution of seabirds (Oppel et al., 2017). This null model reflects the random dispersal of birds away from, and return to, the island under which their core utilization distributions (UDs) will always be centered on the island. Besides DCOL, SSTA was the other main predictor of non-breeding distributions (Figure 3), with tracked birds being less likely to occur in areas of higher SSTA. A number of seabird species have been shown to respond with high sensitivity to changes in SST (Hunt et al., 1992) with foraging success of sooty terns impacted heavily by its variation (Erwin and Congdon, 2007). In the Pacific Ocean, SSTAs are precursors to intense El Niño events that can have negative impacts on temperate and tropical seabirds manifested by declines in their breeding productivity (Devney et al., 2009). These climate anomalies deflate upwelling phenomena and hence cause seasonal-scale crashes in productivity at lower trophic levels and resultant reductions in the abundance and hence availability of fish prey (Spear et al., 2001; Stenseth et al., 2002).
We found that birds occupied foraging habitat non-randomly and shared areas also occupied by fishing vessels that target surface-schooling tuna. Tropical seabird species are surface foragers and therefore they do not interact directly with long-line fishing that targets mature tuna that rarely feed at the ocean surface (Danckwerts et al., 2014). In contrast, purse-seiners target more juvenile tuna with which sooty terns may interact far more often when foraging. Their non-random association with areas of high purse-seine fishing effort in our study highlights a need for more research elaborating their foraging relationship with these predatory fish.
Given low reported direct mortality of sooty terns from fishery interactions (e.g., Gales et al., 1998; Danckwerts et al., 2014), if the Ascension MPA is to benefit this species substantially, it must protect the large predatory fish with which they commonly associate. Limited tracking data are available for such species in Ascension Island waters but satellite-tagged yellowfin tuna spent 100–200 days resident in the Ascension EEZ, much of it within 20 km of the island (Richardson et al., 2018). By alleviating the fishing pressures on semi-resident fish stocks, it is possible that the MPA, which prohibits all forms of commercial fishing, may increase the foraging success of sooty terns while they are located within the Ascension EEZ such as during breeding periods when adults appear to range only approximately 256 km from the island on average (Anon, 2018). However, it is doubtful that the Ascension MPA will enhance the regional population status of highly mobile pelagic fish stocks without being far larger (Grüss, 2014; Curnick et al., 2020), and sooty terns spend considerable periods foraging in the high seas outside the MPA boundaries during their non-breeding migrations. As for other members of the seabird community that have been tracked to date, breeding masked boobies (Sula dactylatra) appear to spend all of their time within the MPA (Oppel et al., 2015) while Ascension frigatebirds (Fregata aquila) frequently range far beyond it (Oppel et al., 2017).
We think that the population of sooty terns on Ascension Island is now stable in size, albeit markedly reduced from 2.35 million birds in 1942 (Hughes et al., 2017a). A cause of their decline was undoubtedly heavy predation pressure on adults from invasive cats (now absent due to successful cat eradication), and that from rats and mynas on chicks and eggs (ongoing) (Hughes et al., 2019). Beyond on-island predator-driven population dynamics of sooty terns, we have a greater understanding of potential at-sea drivers of their breeding population size as a result of a long-term dietary study of terns spanning 110 years that included the mid-twentieth century decline and stabilization of their population size (Reynolds et al., 2019). This indicated that birds had shifted diet from predominantly small fish prey to squid, indicating that prey availability to birds had changed partly as a result of overfishing of tuna (Reynolds et al., 2019). However, whether the shift was due to reduced fish prey availability, resulting from either overexploitation of tuna stocks in the tropical Atlantic or increased SST in recent decades, remains unclear (discussed in Reynolds et al., 2019 and references therein). Furthermore, it is not simple to estimate the relevant impacts of predation and food availability in explaining the steep decline in the sooty tern population. If we are to evaluate the conservation benefits of the MPA to sooty terns, further at-sea research must focus on movements of large predatory fish species, the extent to which their ranges are protected by the MPA, the consequences for small fish prey availability to birds, and the comparative foraging successes of birds within and outside of the MPA at critical times of their sub-annual cycle. Therefore, our study provides baseline information about post-breeding sooty tern movements that can be used to inform future studies assessing the effectiveness of the MPA. In conclusion, we argue that key foraging habitat for some species of the seabird community will be protected by the Ascension Island MPA but benefits to sooty terns outside of the breeding period will likely be more limited.
Data Availability Statement
The raw data supporting the conclusions of this article will be made available by the authors upon request via Movebank (https://www.movebank.org).
Ethics Statement
The animal study was reviewed and approved by (1) the Animal Welfare and Ethical Review Body (AWERB), University of Birmingham, Edgbaston, Birmingham, United Kingdom, (2) the Ascension Island Government (AIG), Georgetown, Ascension Island, (3) the South African Bird Ringing Unit (SAFRING), Percy FitzPatrick Institute of African Ornithology, University of Cape Town, Cape Town, South Africa, and (4) the British Trust for Ornithology (BTO), The Nunnery, Thetford, United Kingdom.
Author Contributions
SR: conceptualization, methodology, investigation, and writing-original draft preparation. CW: methodology and investigation. BH: conceptualization, methodology, and investigation. RD: investigation. LG: investigation and writing-original draft preparation. SW and FH: methodology. NW and JR: writing-original draft preparation. SBW: methodology and writing-original draft preparation. EL and KA: investigation. VP: methodology, formal analysis, investigation, and writing-original draft preparation. All authors contributed to the article and approved the submitted version.
Funding
The Ascension Island Government (AIG) was supportive of our “Adopt a Sooty Tern” scheme that attracted funding for GLS tags from various sources including the AOS, Helen Dickey, Pat Hughes, Robert King, King Edward’s School Birmingham, Pocklington School, the RNBWS, Julie-Ann Wearn and the University of Exeter (Cornwall Campus). The RSPB provided some funding for on-island vehicle hire for some of the expeditions.
Conflict of Interest
SW was employed by Biotrack Ltd.
The remaining authors declare that the research was conducted in the absence of any commercial or financial relationships that could be construed as a potential conflict of interest.
Publisher’s Note
All claims expressed in this article are solely those of the authors and do not necessarily represent those of their affiliated organizations, or those of the publisher, the editors and the reviewers. Any product that may be evaluated in this article, or claim that may be made by its manufacturer, is not guaranteed or endorsed by the publisher.
Acknowledgments
We are grateful to the many members of the Army Ornithological Society (AOS), the Royal Air Force Ornithological Society (RAFOS) and the Royal Navy Birdwatching Society (RNBWS) who spent painstaking hours on AOS expeditions attempting to relocate birds carrying GLS tags often in colonies containing many tens of thousands of birds. We thank staff at Biotrack, Lotek and Migrate Technology for their patience in fielding numerous technical questions and their willingness, on occasion, to retrieve data from GLS tags with which we struggled to connect. The staff at the AIG Conservation and Fisheries Department (AIGCFD) spent many hours during routine fieldwork scanning seabird colonies for birds carrying GLS tags and we thank them for their dedication to the tracking project. We also thank them for overall permission to conduct fieldwork on many expeditions to the island, the Animal Welfare and Ethical Review Body (AWERB) at the University of Birmingham for permission to blood sample sooty terns, the BTO and SAFRING for licenses to ring birds and, again, the BTO for a special methods permit to deploy GLS tags. The manuscript was improved by constructive comments from an editor and two reviewers.
Supplementary Material
The Supplementary Material for this article can be found online at: https://www.frontiersin.org/articles/10.3389/fmars.2021.744506/full#supplementary-material
Footnotes
- ^ https://biodiversityinformatics.amnh.org/open_source/maxent/
- ^ https://globalfishingwatch.org/map-and-data/
References
Afán, I., Navarro, J., Cardador, L., Ramírez, F., Kato, A., Rodríguez, B., et al. (2014). Foraging movements and habitat niche of two closely related seabirds breeding in sympatry. Mar. Biol. 161, 657–668. doi: 10.1007/s00227-013-2368-4
Anon (2018). Ascension Island Marine Protected Area Evidence and Options Document. Washington, DC: Ascension Island Government.
Araújo, M. B., and Guisan, A. (2006). Five (or so) challenges for species distribution modelling. J. Biogeogr. 33, 1677–1688. doi: 10.1111/j.1365-2699.2006.01584.x
Ashmole, N. P. (1963). The biology of the Wideawake or Sooty Tern Sterna fuscata on Ascension Island. IBIS 103b, 297–351. doi: 10.1111/j.1474-919X.1963.tb06757.x
Au, D. W., and Pitman, R. L. (1986). Seabird interactions with dolphins and tuna in eastern tropical Pacific. Condor 88, 304–317. doi: 10.2307/1368877
Bates, D., Mächler, M., Bolker, B. M., and Walker, S. C. (2015). Fitting linear mixed-effects models using lme4. J. Stat. Softw. 67, 1–48. doi: 10.18637/jss.v067.i01
Bernard, A., Rodrigues, A. S. L., Cazalis, V., and Grémillet, D. (2021). Toward a global strategy for seabird tracking. Conserv. Lett. 14:e12804. doi: 10.1111/conl.12804
Calenge, C. (2006). The package “adehabitat” for the R software: a tool for the analysis of space and habitat use by animals. Ecol. Modell. 197, 516–519. doi: 10.1016/j.ecolmodel.2006.03.017
Catry, T., Ramos, J. A., Jaquemet, S., Faulquier, L., Berlincourt, M., Hauselmann, A., et al. (2009). Comparative foraging ecology of a tropical seabird community of the Seychelles, western Indian Ocean. Mar. Ecol. Prog. Ser. 374, 259–272. doi: 10.3354/meps07713
Croxall, J. P., Butchart, S. H. M., Lascelles, B., Stattersfield, A. J., Sullivan, B., Symes, A., et al. (2012). Seabird conservation status, threats and priority actions: a global assessment. Bird Conserv. Int. 22, 1–34. doi: 10.1017/S0959270912000020
Curnick, D. J., Collen, B., Koldewey, H. J., Jones, K. E., Kemp, K. M., and Ferretti, F. (2020). Interactions between a large marine protected area, pelagic tuna and associated fisheries. Front. Mar. Sci. 7:318. doi: 10.3389/fmars.2020.00318
Danckwerts, D. K., McQuaid, C. D., Jaeger, A., McGregor, G. K., Dwight, R., Le Corre, M., et al. (2014). Biomass consumption by breeding seabirds in the western Indian Ocean: indirect interactions with fisheries and implications for management. ICES J. Mar. Science 71, 2589–2598. doi: 10.1093/icesjms/fsu093
Devney, C. A., Short, M., and Congdon, B. C. (2009). Sensitivity of tropical seabirds to El Niño precursors. Ecology 90, 1175–1183. doi: 10.1890/08-0634.1
Dias, M. P., Martin, R., Pearmain, E. J., Burfield, I. J., Small, C., Phillips, R. A., et al. (2019). Threats to seabirds: a global assessment. Biol. Conserv. 237, 525–537. doi: 10.1016/j.biocon.2019.06.033
Dureuil, M., Boerder, K., Burnett, K. A., Froese, R., and Worm, B. (2018). Elevated trawling inside protected areas undermines conservation outcomes in a global fishing hot spot. Science 362, 1403–1407. doi: 10.1126/science.aau0561
Édren, S. M. C., Wisz, M. S., Teilmann, J., Dietz, R., and Söderkvist, J. (2010). Modelling spatial patterns in harbour porpoise satellite telemetry data using maximum entropy. Ecography 33, 698–708. doi: 10.1111/j.1600-0587.2009.05901.x
Egevang, C., Stenhouse, I. J., Phillips, R. A., Petersen, A., Fox, J. W., and Silk, J. R. D. (2010). Tracking of Arctic terns Sterna paradisaea reveals longest animal migration. Proc. Natl. Acad. Sci. U.S.A. 107, 2078–2081. doi: 10.1073/pnas.0909493107
Elith, J., Graham, C. H., Anderson, R. P., Dudík, M., Ferrier, S., Guisan, A., et al. (2006). Novel methods improve prediction of species’ distributions from occurrence data. Ecography 29, 129–151. doi: 10.1111/j.2006.0906-7590.04596.x
Erauskin-Extramiana, M., Arrizabalaga, H., Hobday, A. J., Cabré, A., Ibaibarriaga, L., Arregui, I., et al. (2019). Large-scale distribution of tuna species in a warming ocean. Glob. Change Biol. 25, 2043–2060. doi: 10.1111/gcb.14630
Erwin, C. A., and Congdon, B. C. (2007). Day-to-day variation in sea-surface temperature reduces sooty tern Sterna fuscata foraging success on the Great Barrier Reef, Australia. Mar. Ecol. Prog. Ser. 331, 255–266. doi: 10.3354/meps331255
Feare, C. J. (2017). Orange Omelettes & Dusky Wanderers: Studies and Travels in Seychelles over Four Decades. Mahé: Calusa Bay Publications.
Fehlmann, G., and King, A. J. (2016). Bio-logging. Curr. Biol. 26, R830–R831. doi: 10.1016/j.cub.2016.05.033
Flint, E. N. (1991). Time and energy limits to the foraging radius of sooty terns Sterna fuscata. IBIS 133, 43–46. doi: 10.1111/j.1474-919X.1991.tb04808.x
Freeman, E. A., and Moisen, G. (2008). PresenceAbsence: an R package for presence absence analysis. J. Stat. Softw. 23, 1–31. doi: 10.18637/jss.v023.i11
Gales, R., Brothers, N., and Reid, T. (1998). Seabird mortality in the Japanese tuna longline fishery around Australia, 1988–1995. Biol. Conserv. 86, 37–56. doi: 10.1016/S0006-3207(98)00011-1
Grüss, A. (2014). Modelling the impacts of marine protected areas for mobile exploited fish populations and their fisheries: What we recently learnt and where we should be going. Aquat. Living Resour. 27, 107–133. doi: 10.1051/alr/2014013
Hijmans, R. J. (2019). geosphere: Spherical Trigonometry. R Package Version 1.5-10. Available online at: https://CRAN.R-project.org/package=geosphere (accessed April 4, 2020).
Hindell, M. A., Reisinger, R. R., Ropert-Coudert, Y., Hückstädt, L. A., Trathan, P. N., Bornemann, H., et al. (2020). Tracking of marine predators to protect Southern Ocean ecosystems. Nature 580, 87–92. doi: 10.1038/s41586-020-2126-y
Hughes, B. J., Dickey, R. C., and Reynolds, S. J. (2019). “Predation pressures on sooty terns by cats, rats and common mynas on Ascension Island in the South Atlantic,” in Island Invasives: Scaling Up to Meet the Challenge, eds C. R. Veitch, M. N. Clout, A. R. Martin, J. C. Russell, and C. J. West (Gland: IUCN), 295–301.
Hughes, B. J., Martin, G. R., Giles, A. D., and Reynolds, S. J. (2017a). Long-term population trends of Sooty Terns (Onychoprion fuscatus): implications for conservation status. Popul. Ecol. 59, 213–224. doi: 10.1007/s10144-017-0588-z
Hughes, B. J., Martin, G. R., and Reynolds, S. J. (2017b). Estimating the extent of seabird egg depredation by introduced Common Mynas on Ascension Island in the South Atlantic. Biol. Invasions 19, 843–857. doi: 10.1007/s10530-016-1294-z
Hughes, B. J., Martin, G. R., and Reynolds, S. J. (2008). Cats and seabirds: Effects of feral Domestic Cat Felis silvestris catus eradication on the population of Sooty Terns Onychoprion fuscata on Ascension Island, South Atlantic. IBIS 150, (Suppl. 1), 122–131. doi: 10.1111/j.1474-919X.2008.00838.x
Hunt, G. L., Priddle, J., Whitehouse, M. J., Veit, R. R., and Heywood, R. B. (1992). Changes in seabird species abundance near South Georgia during a period of rapid change in sea surface temperature. Antarct. Sci. 4, 15–22. doi: 10.1017/S0954102092000051
Jaeger, A., Feare, C. J., Summers, R. W., Lebarbenchon, C., Larose, C. S., and Le Corre, M. (2017). Geolocation reveals year-round at-sea distribution and activity of a superabundant tropical seabird, the Sooty Tern Onychoprion fuscatus. Front. Mar. Sci. 4:394. doi: 10.3389/fmars.2017.00394
Johnston, D. W. (1979). The uropygial gland of the sooty tern. Condor 81, 430–432. doi: 10.2307/1366977
Lascelles, B. G., Taylor, P. R., Miller, M. G. R., Dias, M. P., Oppel, S., Torres, L., et al. (2016). Applying global criteria to tracking data to define important areas for marine conservation. Divers. Distrib. 22, 422–431. doi: 10.1111/ddi.12411
Lenth, R. (2020). emmeans: Estimated Marginal Means, aka Least-Squares Means. R Package Version 1.4.7. Available online at: https://CRAN.R-project.org/package=emmeans (accessed March 4, 2020).
Louzao, M., Delord, K., García, D., Boué, A., and Weimerskirch, H. (2012). Protecting persistent dynamic oceanographic features: transboundary conservation efforts are needed for the critically endangered Balearic Shearwater. PLoS One 7:e35728. doi: 10.1371/journal.pone.0035728
Mackley, E. K., Phillips, R. A., Silk, J. R. D., Wakefield, E. D., Afanasyev, V., Fox, J. W., et al. (2010). Free as a bird? Activity patterns of albatrosses during the nonbreeding period. Mar. Ecol. Prog. Ser. 406, 291–303. doi: 10.3354/meps08532
Maxwell, S. M., and Morgan, L. E. (2013). Foraging of seabirds on pelagic fishes: implications for management of pelagic marine protected areas. Mar. Ecol. Prog. Ser. 481, 289–303. doi: 10.3354/meps10255
McConnell, B. J., Chambers, C., and Fedak, M. A. (1992). Foraging ecology of southern elephant seals in relation to the bathymetry and productivity of the Southern Ocean. Antarct. Sci. 4, 393–398. doi: 10.1017/S0954102092000580
McKnight, A., Allyn, A. J., Duffy, D. C., and Irons, D. B. (2013). ‘Stepping stone’ pattern in Pacific Arctic tern migration reveals the importance of upwelling areas. Mar. Ecol. Prog. Ser. 491, 253–264. doi: 10.3354/meps10469
McKnight, A., Irons, D. B., Allyn, A. J., Sullivan, K. M., and Suryan, R. M. (2011). Winter dispersal and activity patterns of post-breeding black-legged kittiwakes Rissa tridactyla from Prince William Sound, Alaska. Mar. Ecol. Prog. Ser. 442, 241–253. doi: 10.3354/meps09373
Miyake, P. M., and Kebe, P. (1996). “Multi-species and multi-gear tuna fisheries in the Atlantic and possible interactions between gears and species,” in Proceedings of the Second FAO Expert Consultation on Interactions of Pacific Tuna Fisheries, Shimizu, Japan, eds R. S. Shomura, J. Majkowski, and R. F. Harman (Rome: FAO), 48–66.
Moore, S. E., and Kuletz, K. J. (2019). Marine birds and mammals as ecosystem sentinels in and near Distributed Biological Observatory regions: an abbreviated review of published accounts and recommendations for integration to ocean observatories. Deep Sea Res. II Top. Stud. Oceanogr. 162, 211–217. doi: 10.1016/j.dsr2.2018.09.004
Norris, K., and Pain, D. J. (2002). Conserving Bird Biodiversity: General Principles and their Application. Cambridge: Cambridge University Press.
Oppel, S., Beard, A., Fox, D., Mackley, E., Leat, E., Henry, L., et al. (2015). Foraging distribution of a tropical seabird supports Ashmole’s hypothesis of population regulation. Behav. Ecol. Sociobiol. 69, 915–926. doi: 10.1007/s00265-015-1903-3
Oppel, S., Bolton, M., Carneiro, A. P. B., Dias, M. P., Green, J. A., Masello, J. F., et al. (2018). Spatial scales of marine conservation management for breeding seabirds. Mar. Policy 98, 37–46. doi: 10.1016/j.marpol.2018.08.024
Oppel, S., Weber, S., Weber, N., Fox, D., Leat, E., Sim, J., et al. (2017). Seasonal shifts in foraging distribution due to individual flexibility in a tropical pelagic forager, the Ascension frigatebird. Mar. Ecol. Prog. Ser. 585, 199–212. doi: 10.3354/meps12377
Paleczny, M., Hammill, E., Karpouzi, V., and Pauly, D. (2015). Population trend of the world’s monitored seabirds, 1950-2010. PLoS One 10:e0129342. doi: 10.1371/journal.pone.0129342
Phalan, B., Phillips, R. A., Silk, J. R. D., Afanasyev, V., Fukuda, A., Fox, J., et al. (2007). Foraging behaviour of four albatross species by night and day. Mar. Ecol. Prog. Ser. 340, 271–286. doi: 10.3354/meps340271
Phillips, R. A., Bearhop, S., McGill, R. A. R., and Dawson, D. A. (2009). Stable isotopes reveal individual variation in migration strategies and habitat preferences in a suite of seabirds during the nonbreeding period. Oecologia 160, 795–806. doi: 10.1007/s00442-009-1342-9
Phillips, R. A., Silk, J. R. D., Croxall, J. P., Afanasyev, V., and Briggs, D. R. (2004). Accuracy of geolocation estimates for flying seabirds. Mar. Ecol. Prog. Ser. 266, 265–272. doi: 10.3354/meps266265
Phillips, S. J., Anderson, R. P., and Schapire, R. E. (2006). Maximum entropy modeling of species geographic distributions. Ecol. Model. 190, 231–259. doi: 10.1016/j.ecolmodel.2005.03.026
Quillfeldt, P., Masello, J. F., Navarro, J., and Phillips, R. A. (2013). Year-round distribution suggests spatial segregation of two small petrel species in the South Atlantic. J. Biogeogr. 40, 430–441. doi: 10.1111/jbi.12008
R Core Team (2018). R: A Language and Environment for Statistical Computing. Vienna: R Foundation for Statistical Computing.
Reynolds, S. J., Hughes, B. J., Wearn, C. P., Dickey, R. C., Brown, J., Weber, N., et al. (2019). Long-term dietary shift and population decline of a pelagic seabird–A health check on the tropical Atlantic? Global Change Biol. 25, 1383–1394. doi: 10.1111/gcb.14560
Reynolds, S. J., Martin, G. R., Dawson, A. S., Wearn, C. P., and Hughes, B. J. (2014). The sub-annual breeding cycle of a tropical seabird. PLoS One 9:e93582. doi: 10.1371/journal.pone.0093582
Reynolds, S. J., Martin, G. R., Wallace, L. L., Wearn, C. P., and Hughes, B. J. (2008). Sexing sooty terns (Onychoprion fuscata) on Ascension Island from morphometric measurements. J. Zool. 274, 2–8. doi: 10.1111/j.1469-7998.2007.00350.x
Richardson, A. J., Downes, K. J., Nolan, E. T., Brickle, P., Brown, J., Weber, N., et al. (2018). Residency and reproductive status of yellowfin tuna in a proposed large-scale pelagic marine protected area. Aquat. Conserv. 28, 1308–1316. doi: 10.1002/aqc.2936
Schneider, D. C. (1991). The role of fluid dynamics in the ecology of marine birds. Oceanogr. Mar. Biol. Annu. Rev. 29, 487–521.
Schreiber, E. A., Feare, C. J., Harrington, B. A., Murray, B. G. Jr., and Robertson, W. B. Jr., et al. (2002). “Sooty tern (Sterna fuscata),” in The Birds of North America, No. 665, eds A. Poole and F. Gill (Philadelphia, PA: The American Ornithologists’ Union).
Shaffer, S. A., Tremblay, Y., Weimerskirch, H., Scott, D., Thompson, D. R., Sagar, P. M., et al. (2006). Migratory shearwaters integrate oceanic resources across the Pacific Ocean in an endless summer. Proc. Natl. Acad. Sci. U.S.A. 103, 12799–12802. doi: 10.1073/pnas.0603715103
Soanes, L. M., Green, J. A., Bolton, M., Milligan, G., Mukhida, F., and Halsey, L. G. (2021). Linking foraging and breeding strategies in tropical seabirds. J. Avian Biol. 52:e02670. doi: 10.1111/jav.02670
Spear, L. B., Ballance, L. T., and Ainley, D. G. (2001). Response of seabirds to thermal boundaries in the tropical Pacific: the thermocline versus the Equatorial Front. Mar. Ecol. Prog. Ser. 219, 275–289. doi: 10.3354/meps219275
Stenseth, N. C., Mysterud, A., Ottersen, G., Hurrell, J. W., Chan, K. S., and Lima, M. (2002). Ecological effects of climate fluctuations. Science 297, 1292–1296. doi: 10.1126/science.1071281
Sumner, M. D., and Wotherspoon, S. (2016). tripEstimation: Metropolis Sampler and Supporting Functions for Estimating Animal Movement From Archival Tags and Satellite Fixes. Available online at: http://cran.r-project.org/web/packages/tripEstimation (accessed April 20, 2020).
Thiebot, J.-B., and Pinaud, D. (2010). Quantitative method to estimate species habitat use from light-based geolocation data. Endanger. Species Res. 10, 341–353. doi: 10.3354/esr00261
Tremblay, Y., Bertrand, S., Henry, R. W., Kappes, M. A., Costa, D. P., and Shaffer, S. A. (2009). Analytical approaches to investigating seabird-environment interactions: a review. Mar. Ecol. Prog. Ser. 391, 153–163. doi: 10.3354/meps08146
Veit, R. R., and Harrison, N. M. (2017). Positive interactions among foraging seabirds, marine mammals and fishes and implications for their conservation. Front. Ecol. Evol. 5:121. doi: 10.3389/fevo.2017.00121
Votier, S. C., and Sherley, R. B. (2017). Seabirds. Curr. Biol. 27, R448–R450. doi: 10.1016/j.cub.2017.01.042
Weber, S. B., Richardson, A. J., Brown, J., Bolton, M., Clark, B. L., Godley, B. J., et al. (2021). Direct evidence of a prey depletion “halo” surrounding a pelagic predator colony. Proc. Natl. Acad. Sci. U.S.A. 118:e2101325118. doi: 10.1073/pnas.2101325118
Weimerskirch, H. (2007). Are seabirds foraging for unpredictable resources? Deep Sea Res. II Top. Stud. Oceanogr. 54, 211–223. doi: 10.1016/j.dsr2.2006.11.013
Weimerskirch, H., Delord, K., Guitteaud, A., Phillips, R. A., and Pinet, P. (2015). Extreme variation in migration strategies between and within wandering albatross populations during their sabbatical year, and their fitness consequences. Sci. Rep. 5:8853. doi: 10.1038/srep08853
Weimerskirch, H., le Corre, M., Jaquemet, S., and Marsac, F. (2005). Foraging strategy of a tropical seabird, the red-footed booby, in a dynamic marine environment. Mar. Ecol. Prog. Ser. 288, 251–261. doi: 10.3354/meps288251
Zajkova, Z., Militão, T., and González-Solís, J. (2017). Year-round movements of a small seabird and oceanic isotopic gradient in the tropical Atlantic. Mar. Ecol. Prog. Ser. 579, 169–183. doi: 10.3354/meps12269
Zuur, A. F., Ieno, E. N., and Elphick, C. S. (2010). A protocol for data exploration to avoid common statistical problems. Methods Ecol. Evol. 1, 3–14. doi: 10.1111/j.2041-210X.2009.00001.x
Keywords: Ascension Island, at-sea behavior and distribution, geolocation, large-scale MPA, seabird conservation
Citation: Reynolds SJ, Wearn CP, Hughes BJ, Dickey RC, Garrett LJH, Walls S, Hughes FT, Weber N, Weber SB, Leat EHK, Andrews K, Ramos JA and Paiva VH (2021) Year-Round Movements of Sooty Terns (Onychoprion fuscatus) Nesting Within One of the Atlantic’s Largest Marine Protected Areas. Front. Mar. Sci. 8:744506. doi: 10.3389/fmars.2021.744506
Received: 20 July 2021; Accepted: 02 November 2021;
Published: 29 November 2021.
Edited by:
Carolyn J. Lundquist, National Institute of Water and Atmospheric Research (NIWA), New ZealandReviewed by:
Kees (C. J.) Camphuysen, Royal Netherlands Institute for Sea Research (NIOZ), NetherlandsChristy Wails, Virginia Tech, United States
Copyright © 2021 Reynolds, Wearn, Hughes, Dickey, Garrett, Walls, Hughes, Weber, Weber, Leat, Andrews, Ramos and Paiva. This is an open-access article distributed under the terms of the Creative Commons Attribution License (CC BY). The use, distribution or reproduction in other forums is permitted, provided the original author(s) and the copyright owner(s) are credited and that the original publication in this journal is cited, in accordance with accepted academic practice. No use, distribution or reproduction is permitted which does not comply with these terms.
*Correspondence: S. James Reynolds, Si5SZXlub2xkcy4yQGJoYW0uYWMudWs=