- 1Shandong Provincial Key Laboratory of Marine Ecological Restoration, Shandong Marine Resource and Environment Research Institute, Yantai, China
- 2Muping Coastal Environment Research Station, Yantai Institute of Coastal Zone Research, Chinese Academy of Sciences, Yantai, China
- 3Research and Development Center for Efficient Utilization of Coastal Bioresources, Yantai Institute of Coastal Zone Research, Chinese Academy of Sciences, Yantai, China
In the present study, Bacillus velezensis (BV007, CGMCC No. 20039) was isolated from the gut of Litopenaeus vannamei, and the effects of BV007 on the growth performance, immune responses, and intestine microbiota of the shrimp were investigated. A total of 1,200 healthy shrimp (3.0 ± 0.3 cm, 0.32 ± 0.8 g) were randomly divided into four groups, and fed diets supplemented with different levels of BV007 (C: 0; BV1: 1 × 105 CFU/g; BV2: 1 × 107 CFU/g; and BV3: 1 × 109 CFU/g) for 8 weeks. The results showed a significantly increased final body length (FBL), length gain rate (LGR), final body weight (FBW), weight gain rate (WGR), plumpness index (PI), and specific growth rate (SGR) in shrimp fed with BV007 for 42 days compared with shrimp fed with control diet. The activity of α-amylase in hepatopancreas was also significantly increased in the BV007-administered groups. After 42 days of growth trial, the challenge test with Vibrio parahaemolyticus was conducted for 2 weeks. The enhanced immune responses were exhibited by shrimp fed with BV007 after V. parahaemolyticus challenge, particularly in respiratory bursts and superoxide dismutase, catalase, and alkaline phosphatase activities. Moreover, the administration of BV007 could considerably increase the abundance of potential probiotics (Bacillus) and reduced the abundances of potential pathogenic bacteria (Vibrio) in shrimp intestines. In conclusion, the dietary supplementation with B. velezensis BV007 could promote the growth performance, enhance the immune responses, and modulate the intestine microbiota of shrimp, and 107 CFU/g feed was recommended to be used as a feed additive to enhance the growth and health status of shrimp.
Introduction
The Pacific white shrimp Litopenaeus vannamei is one of the major cultivated aquaculture crustacean species worldwide. In recent years, shrimp aquaculture has developed rapidly and intensively, but the low growth rates and disease have occurred more frequently, causing considerable economic losses (Neiland et al., 2001). The use of hormones and antibiotics as a traditional way to prevent and treat infectious diseases has led to the evolution of pathogenic bacteria and environmental pollution. Great effort has been invested to develop the potential alternatives to antibiotics to avoid the creation of bacterial resistance and high antibiotic residues. Currently, probiotics, prebiotics, and medicinal herbs have shown to be a vital alternative additive for the improvement of host health (Raman et al., 2019; Dawood et al., 2020; Zhang et al., 2021).
Probiotics are valuable in animal production due to their improvement of feed value, enzymatic contribution to digestion, and activation of the immune responses (Gao et al., 2018; Wang Y. et al., 2019; Qin et al., 2020). For example, oral administration of Bacillus PC465 enhanced the growth performance and survival rate of L. vannamei (Chai et al., 2016). Dietary supplementation with B. licheniformis at 105 CFU/ml for 8 weeks enhanced abalones growth and enhanced disease resistance to Vibrio parahemolyticus (Gao et al., 2018). Moreover, the activities of glutamic-pyruvic transaminase (GPT) and glutamic oxaloacetic transaminase (GOT) were significantly lower in shrimp fed with Enterococcus faecalis supplemented groups as compared with control shrimp (Wang Y. et al., 2019). In addition, dietary Bacillus amyloliquefaciens A23 at 108 CFU/g significantly enhanced the intestinal microbial diversity of Procambarus clarkii (Xu et al., 2021). Besides the promotion of Lac. pentosus, BD6 feeding can increase the relative abundance of beneficial bacteria and reduce the abundance of harmful pathogenic bacteria in the gut flora of shrimp, thus regulating the host immune system (Lin et al., 2017).
Although many studies suggest that B. subtilis possesses a number of beneficial traits when used as a supplement in the shrimp diets (Liu et al., 2010; Fu et al., 2011; Zokaeifar et al., 2012, 2014; Interaminense et al., 2018, 2019), few studies have investigated the appropriate dose of B. velezensis in L. vannamei farming. Therefore, the current study aimed to find the optimum supplementation level of B. velezensis and to investigate the potential effect on the growth performance, feed utilization, immune responses, and gut microbiota of L. vannamei.
Materials and Methods
Bacterial Strain and Diet Preparation
Bacillus velezensis (named as BV007) was previously isolated from healthy L. vannamei intestines. The intestines were immersed in sterile phosphate buffered saline (PBS) (0.05 M, pH 7.4), shook, and eluted at 30°C for 30 min. Spread the eluate on LB nutrient agar (Beijing Land Bridge Tech. Co. Ltd.) plates with gradient dilution and incubated at 30°C for 24 h. After picking a single colony and streaking for purification, the strain 007 with a wide range of enzyme-producing abilities was obtained. The genetic characterization of B. velezensis was accomplished by using 16S rDNA sequence analysis, and the strain was deposited by the China General Microbiological Culture Center (CGMCC no. 20039).
In the diet preparation, the bacterial strains were inoculated in nutrient broth, collected in sterilized water, and adjusted to 109 CFU/ml before feed production. The ingredients of the basal diet were measured to contain 41.35% crude protein and 7.67% crude lipid with fish meal, corn gluten meal, and soybean meal as the fundamental protein source whereas the main lipid sources were soybean oil and soy lecithin oil. Three experimental diets were supplemented with different levels of BV007: 105 CFU/g (BV1), 107 CFU/g (BV2), and 109 CFU/g (BV3). The group without BV007 was served as a control (C). The bacterial amounts of experimental diets were also confirmed by the plate count method, and the colonies that emerged on the plates were counted. The four formulated experimental diets were extruded through a 1.2-mm die. The resulting pellets were dried at 25°C with the aid of an air conditioner. After drying, all diets were stored at 4°C until usage.
Experimental Design and Daily Management
Healthy L. vannamei (body length: 3.0 ± 0.3 cm, body weight: 0.32 ± 0.8 g) were purchased from a local farm (Yantai, Shandong Province, China) and acclimated at 25–27°C and 30% of salinity for 2 weeks before processing. The shrimp were randomly dispersed into 12 fiberglass tanks (60 L), each containing 100 shrimp. The shrimp were fed four times daily (06:00, 12:00, 17:00, and 22:00) at 6% of body weight. The cumulative mortality of the shrimp was recorded daily. After 42 days of feeding, the total number of shrimp and the weight in each tank was quantified to calculate the survival rate (SR, in percentage), final body weight (FBW), final body length (FBL), plumpness index (PI), specific growth rate (SGR, %/d) according to the methods described previously (Velmurugan et al., 2015; Amoah et al., 2019b).
Sample Collection
Hemolymph samples (eight replicates were tested in each treatment, and three shrimps were tested in each replicate [n = 24]) were individually withdrawn from the pericardial cavity of the shrimp using a 1-ml sterile syringe containing an anticoagulant (30 mM trisodium citrate, 0.34 M sodium chloride, and 10 mM EDTA at pH 7.55 with an osmolality adjusted to 780 mOsm/kg with glucose). The volume ratio of anticoagulant to hemolymph was 1:1. The harvested hemocytes were centrifuged at 4°C, 4,000 rpm/min for 10 min, collected, and adjusted to 106 cells/ml with PBS (0.05 M, pH 7.4) for the phagocytic activity and respiratory burst assays. In addition, the hepatopancreas was collected for the assay of digestive, antioxidant, and immune-related enzyme activities, eight replicates were tested in each treatment, and three shrimps were tested in each replicate (n = 24). The middle intestines and hepatopancreas of each group were collected sterile into 4% paraformaldehyde and stored at 4°C for the analysis of microorganisms (three parallel assays).
Phagocytosis Assay
The phagocytosis assay was adapted from the method of Delaporte (Delaporte et al., 2003) with minor modifications. Briefly, the hemolymph was mixed with 2.3% yellow-green FluoSpheres (diameter 2.0 μm, Polyscience, Eppelheilm, Germany), and incubated in the dark at 18 °C for 1 h with rotation. After incubation, the hemocytes were analyzed by flow cytometry (BD Accuri C6 Plus, BD Biosciences, America) using the FL-1 tunnel to detect hemocytes containing fluorescent beads. The phagocytosis rates were expressed as the percentage of hemocytes that engulfed three or more beads.
Respiratory Burst Activity
Respiratory burst activity was analyzed according to the protocols described by Kalgraff et al., 2011. Briefly, hemocytes (1 × 106 cells/ml) were challenged by phorbol 12-myristate 13-acetate (PMA; final concentration of 0.1 μg/ml) for 10 min. Subsequently, dihydrorhodamine 123 (DHR 123) was added to a final concentration of 2 μg/ml and then incubated for 30 min prior to flow cytometry analysis. The flow cytometry analyses were done on a BD FACSCalibur flow cytometer equipped with a 15 mW 488 nm argon ion laser. Further data analyses were done using FCS Express 3 software (De Novo Software, CA, USA).
Assay of Digestive and Immune-Related Enzymes
The digestive enzymes and immune enzymes were measured as described by Cao et al. (2012). Briefly, the digestive enzymes included α-amylase (AMS), trypsin (TRP), lipase (LPS), gltamic-pyruvic transaminase (GPT), glutamic oxaloacetic transaminase (GOT), and the immune enzymes included catalase (CAT), superoxide dismutase (SOD), acid phosphatase (ACP), and alkaline phosphatase (AKP). The enzymatic activities were quantified with corresponding commercial detection kits (C016-1-1, A080-2-2, A054-1-1, C009-2-1, C010-2-1, A007-1-1, A001-3-2, A001-3-1, and A060-2-1, Nanjing Jiancheng Bioengineering Institute, P.R. China) according to the instructions of the manufacturer.
Real-Time PCR Analysis
Total RNA was isolated from hepatopancreas of different treatments using RNAiso (TaKaRa, Japan) reagent. cDNA synthesis was performed according to Promega M-MLV RT Usage information. The temporal expression profiles were carried out with an Applied Biosystem 7500 fast real-time PCR system (Applied Biosystems, MA, USA) using SYBR Green I (Biotek, VT, USA). The PCR amplification program was 95°C for 10 min, then 40 cycles of 95°C for 15 s, 60°C for 1 min, followed by 95°C for 15 s, 60°C for 1 min, and 95°C for 15 s. At the end of each PCR, a dissociation curve analysis of amplification products was performed to confirm the purity of the PCR product. The 2−ΔΔCT method was used to analyze the expression levels (Livak and Schmittgen, 2001). All data were given in terms of relative mRNA expressed as mean ± SD (N = 6). The primers for qRT-PCR of genes for antioxidant-related genes (cat and sod), antibacterial peptides genes (crustin and penaiedin 3a), pattern recognition receptors (lgbp and lec), along with the housekeeping gene β-actin, are shown in Table 1.
Histopathological Examination of the Intestine and Hepatopancreas
Three similar-sized shrimp from each tank were sampled and dissected to obtain the midgut and the hepatopancreas (5 mm3). The fixed intestinal and hepatopancreas samples were dehydrated in a graded series of ethyl alcohol and embedded in paraffin. Then, the paraffin blocks were sliced transversely into 5-μm thick sections and stained with H&E. The villus length of intestinal slice and the cell type of hepatopancreas slice were measured by a light microscope with a computerized image system (Olympus, DP73, Tokyo, Japan).
Intestinal Microbial Composition
The intestinal community of the micro-organisms was performed according to Suo (Suo et al., 2017) with slight changes. The total DNA of microbes in the intestine was extracted directly with the E.Z.N.A. Stool DNA Kit (Omega Bio-tek, Inc., GA, USA) (Xin et al., 2015). The amplification and sequencing of the hypervariable region (V4 + V5) of the bacterial 16S DNA gene was performed for the sequencing analysis and species identification. High-throughput sequencing was performed on the IonS5TMXL platform (Novogene, China). The sequencing reads were assigned to each sample according to the individual unique barcode. The sequences were analyzed with the QIIME software package (Quantitative Insights Into Microbial Ecology) and the UPARSE pipeline (Caporaso et al., 2011). The reads were first filtered and clustered into operational taxonomic units (OTUs) at an identity threshold of 97%. The alpha and beta diversity analyses were then performed as described by Amoah et al. (2019b).
Vibrio Parahaemolyticus Challenge
Vibrio parahaemolyticus was used for the challenge experiment (Amoah et al., 2019b). At the end of the culture experiment, 30 shrimps from each tank were injected with V. parahaemolyticus (final concentration 2 × 107 CFU). In each tank, 20 shrimps were used for cumulative mortality assay, which was recorded daily for 14 days. The other 10 shrimps were cultured for immunoassays and 1 week after bacterial challenge, both the hemocytes and hepatopancreas were sampled and analyzed as described above. In addition, each treatment group was continuously fed with the experimental diets. The cumulative mortality rate was calculated following the formula of Liu et al. (2017).
Statistical Analysis
The experimental data are presented as the mean ± SE. The data were statistically analyzed for significance using a one-way ANOVA, and then the differences among the means at P < 0.05 were tested with Duncan's multiple range test using SPSS 23.0 (SPSS Inc., 2005, IL, USA).
Result
Growth Performance and Survival
The growth performance and survival of L. vannamei fed experimental diets are shown in Table 2. The results showed that FBL, FBW, PI, and SGR were significantly improved by the different levels of probiotics supplementation, whereas no significant differences were observed in SR among the control and probiotic diets.
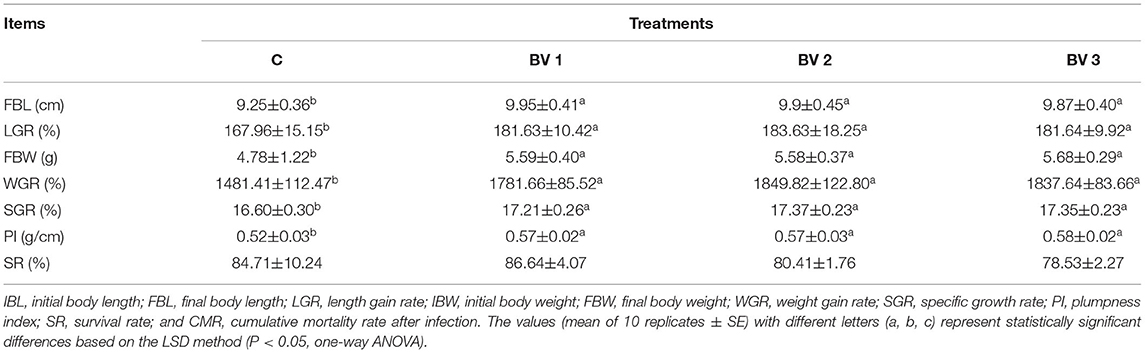
Table 2. Effects of different supplementation levels of B. velezensis BV007 on the growth performance and survival of L. vannamei.
Phagocytic and Respiratory Burst Activity
After 42 days of feeding, there was a significant increase in the phagocytic activity of shrimp fed diets supplemented with B. velezensis as compared to the control group (Figure 1A). However, no significant increase in the rates of phagocytosis was found in either the probiotics-supplemented or non-supplemented shrimps after the V. parahaemolyticus challenge (Figure 1B). Compared with the control group, significantly reduced reactive oxygen species (ROS) production was observed in the BV3 group, but not in the BV1 or BV2 groups (Figure 1C). In contrast, the production of ROS by hemocytes decreased statistically in the BV1, BV2, and BV3 groups when shrimp were challenged with V. parahaemolyticus (P < 0.01; Figure 1D).
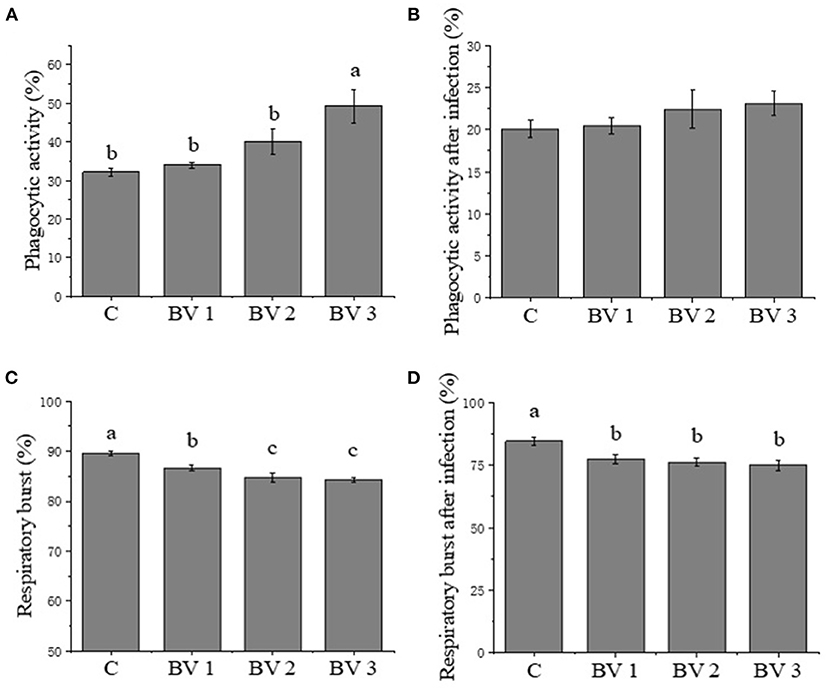
Figure 1. (A-D) Phagocytic activity and respiratory burst activity of hemocytes in Litopenaeus vannamei fed diets containing Bacillus velezensis concentration of 0 CFU/g (C), 105 CFU/g (BV1), 107 CFU/g (BV2), and 109 CFU/g (BV3) for 6 weeks and then infected with Vibrio parahaemolyticus for 1 week. Error bars with different letters (a, b, c) represent statistically significant differences based on the least significant difference (LSD) method (P < 0.05, one-way ANOVA). The results were shown as mean ± SE (N = 12).
Digestive Enzyme Activities
As shown in Table 3, the specific activity of the α-amylase enzyme was enhanced in B. velezensis-supplemented groups. As compared with the control group, the AMS activity was enhanced significantly in shrimp fed BV1, BV2, and BV3 diets with the highest activity observed in the BV2 group (P < 0.01). However, no significant differences were observed in TRP or LPS activities among any of the groups. In addition, the GOT and GPT activities were significantly reduced in the BV3 and BV1 groups as compared with the control group, respectively (P < 0.05).
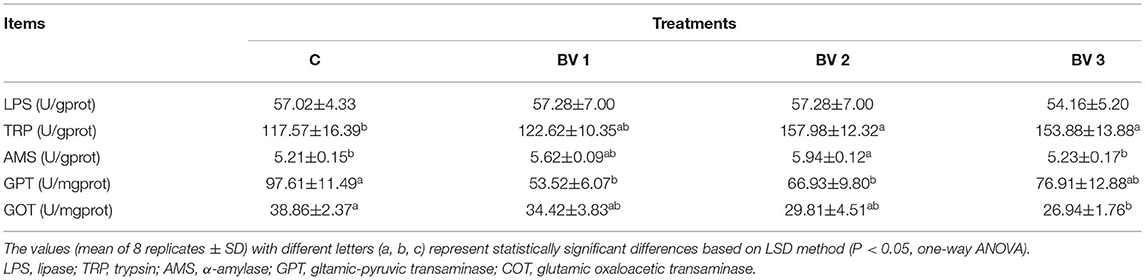
Table 3. Digestive enzyme activities in the hepatopancreas of L. vannamei fed diets containing 0 CFU/g (C), 105 CFU/g (BV1), 107 CFU/g (BV2), 109 CFU/g (BV3) B. velezensis for 6 weeks.
Immune-Related Enzyme Activities
The activities of ACP showed no differences among the tested groups after being challenged with V. parahaemolyticus (Table 4). Similarly, no significant difference in AKP activity was detected among the BV1, BV2, and BV3 groups. However, the AKP activity was enhanced significantly after V. parahaemolyticus challenge among the tested groups (P < 0.01; Table 4). Both the CAT and SOD activities were enhanced in B. velezensis-supplemented groups and in the groups challenged with pathogenic bacteria (Table 4). The highest SOD and CAT activities were observed in the BV2 (P < 0.01; Table 4) and BV3 (P < 0.01; Table 4) groups.
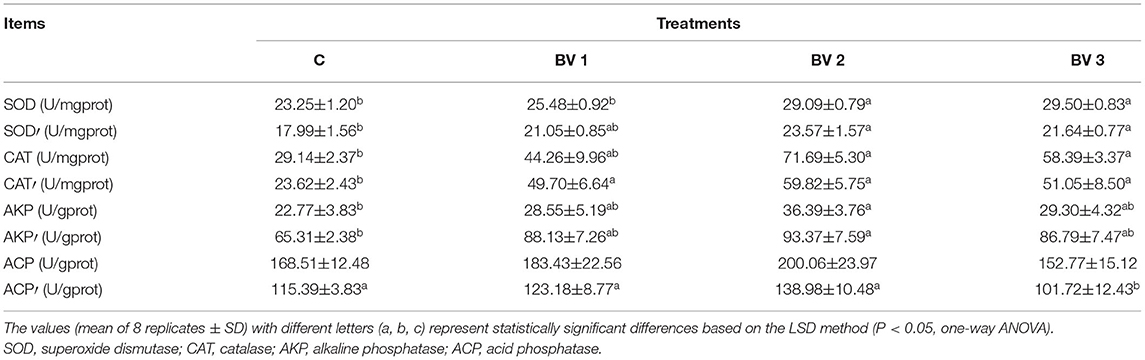
Table 4. Immune-related enzyme activities in the hepatopancreas of L. vannamei fed diets containing 0 CFU/g (C), 105 CFU/g (BV1), 107 CFU/g (BV2), or 109 CFU/g (BV3) B. velezensis for 6 weeks and then, infected with 107 CFU/ml V. parahaemolyticus for one week (use words with a superscript comma).
Expression of Immune-Related Genes
As revealed in Figure 2, the feeding dosage of B. velezensis had a significant effect on the expression levels of immune-related genes. The expression levels of cat were significantly induced in BVs groups (P < 0.05, Figure 2A). After V. parahaemolyticus challenge, the cat transcripts in BV1 group were significantly higher than other groups (P < 0.05, Figure 2A). As concerned to sod expression, the higher expression levels were observed in BV2 and BV3 groups compared with C and BV1 groups, while the levels were significantly upregulated in BV1 and BV2 groups after the bacterial challenge (P < 0.05, Figure 2B). No significant changes in the expression levels of antibacterial peptides (crustin and penaeidin 3α) were observed among C, BV1, BV2, and BV3 groups. After the bacterial challenge, the expression levels of crustin and penaeidin 3α in BV2 were significantly higher than the other groups (P < 0.05, Figures 2C,D). In addition, the expression levels of lgbp and lec were highly enhanced regardless of whether the shrimp were bacterial challenged, especially in BV1 and BV2 groups (P < 0.05, Figures 2E,F).
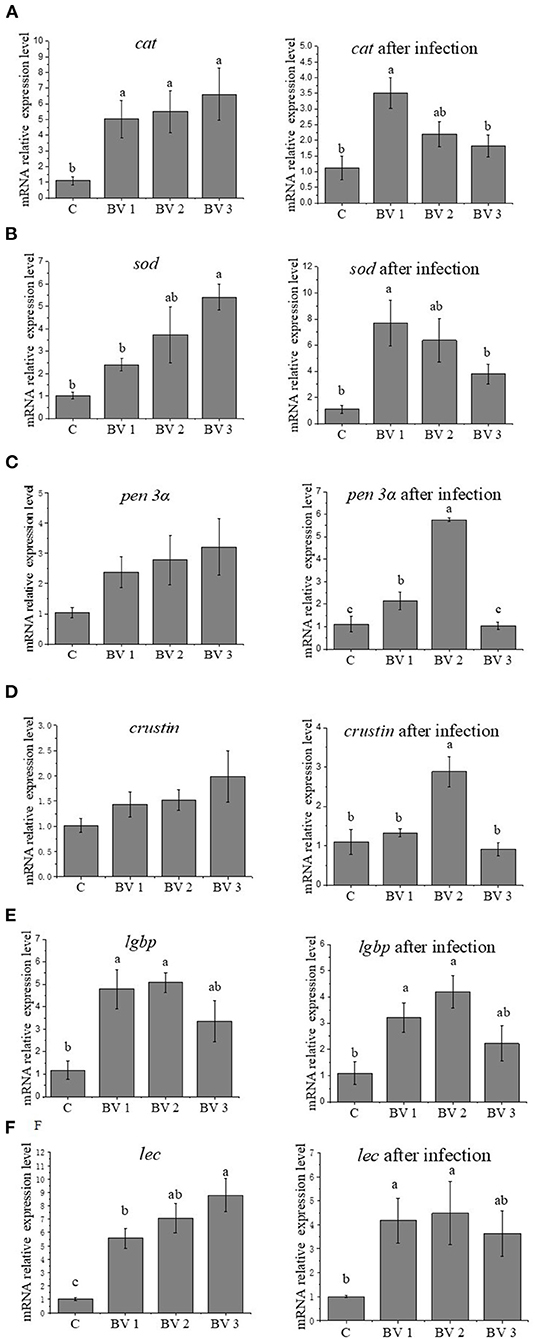
Figure 2. Effects of different levels of dietary B. velezensis on the relative expression of cat (A), sod (B), pen 3α (C), crustin (D), lgbp (E), and lec (F) in hepatopancreas. Error bars with different letters (a, b, c) represent statistically significant differences based on the LSD method (P < 0.05, one-way ANOVA). The results were shown as mean ± SE (N = 6).
Intestinal and Hepatopancreas Morphology
The intestinal and hepatopancreas morphometric parameters were presented in Figure 3 and Table 5. The liver tubule of hepatopancreas in the BV addition groups are arranged relatively tightly and significantly generally star-shaped or polygonal (P < 0.05), while the lumen of the control group is mostly circular. Besides, the control group distributed many differentiated E cells (embryonic cells), while the BV addition group distributed more B cells (secreting cells), F cells (fibrocyte), and R cells (absorbing cells). The shrimps from all dietary treatments had an intact epithelial barrier with extensive mucosal folds and abundant Microvilli. The mucosal structure of the control group was slightly loose, the length of intestinal villi was significantly shorter (P < 0.05), and the number of mucosal epithelial cells was less. The intestinal tissue structures of the BV addition groups were relatively complete, and the length of the intestinal villi was increased and closely connected with the intestinal wall.
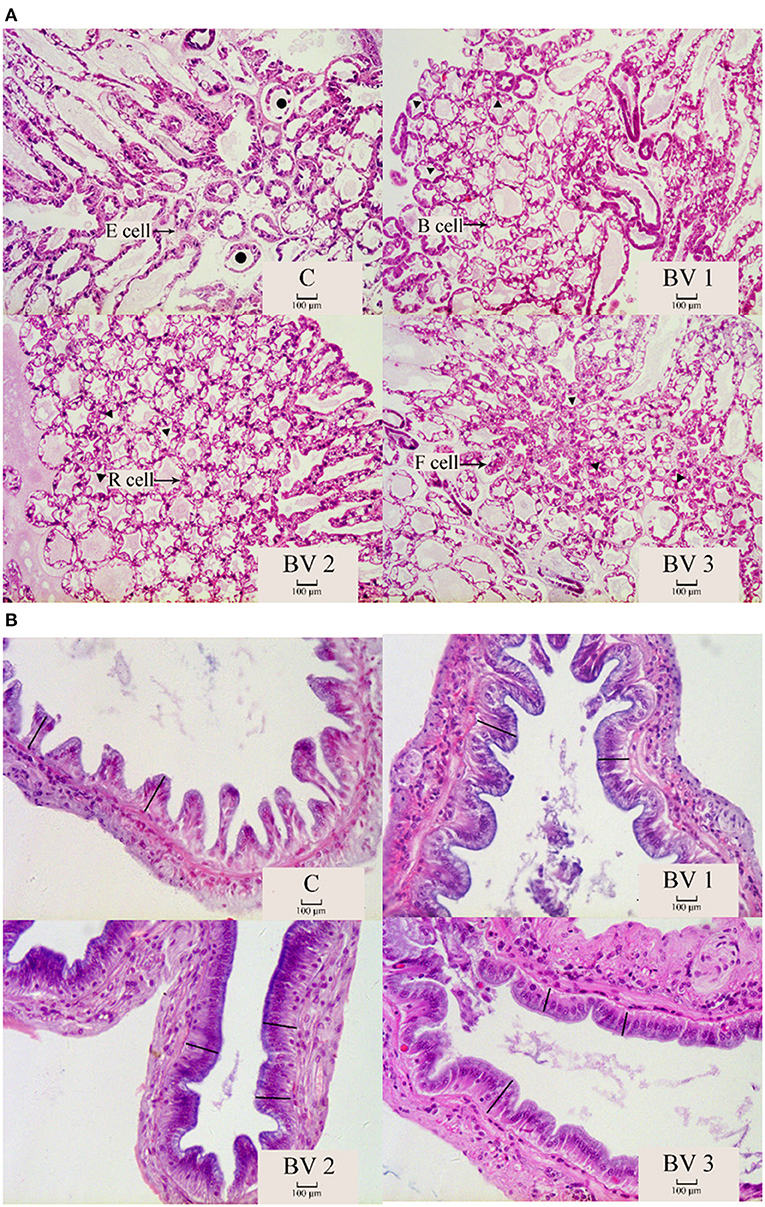
Figure 3. The morphological structure of hepatopancreas (A) and intestine (B). C, 0 CFU/g; BV1, 105 CFU/g group; BV2, 107 CFU/g group; BV3, 109 CFU/g group. •: liver tubules are round; ▴: the liver tubules are star-like or polygonal. -: intestinal villus.

Table 5. Statistics of the morphology and structure of hepatopancreas and midgut of L. vannamei fed diets containing 0 CFU/g (C), 105 CFU/g (BV1), 107 CFU/g (BV2), and 109 CFU/g (BV3) B. velezensis for 6 weeks.
Microbiota
Richness and Diversity of Intestinal Microflora
The alpha diversity was calculated using the Shannon and Simpson indices and the Chao1, ACE, and PD whole tree estimators (Table 6). The nonparametric species-richness estimator Chao1 showed a minimum OTUs count of 287 in the BV1 group and a maximum count of 730 in the BV2 group (P < 0.05). The highest level of supplemented probiotics (109 CFU/g) also had a higher OTU count than the control group (P < 0.05). The number of observed species, Shannon index, and ACE showed similar trends with BV3 exhibiting significantly higher values than the other groups. Phylogenetic diversity was measured using the PD whole tree estimator, which ranged from 26.50 to 52.64. All these indices suggested that the BV2 and BV3 groups had a higher microbial diversity in their intestines, whether measured via richness or evenness. A Venn diagram was constructed to identify the OTUs held in common or unique to shrimp under different diets. In this regard, 961 OTUs were shared among all the shrimp gut samples (Figure 4). In contrast, 43, 49, 197, and 245 OTUs were unique to the C, BV1, BV2, and BV3 diets, respectively. This observation suggests that the exposure of shrimp to different graded probiotics led to the selection of unique microbial populations.
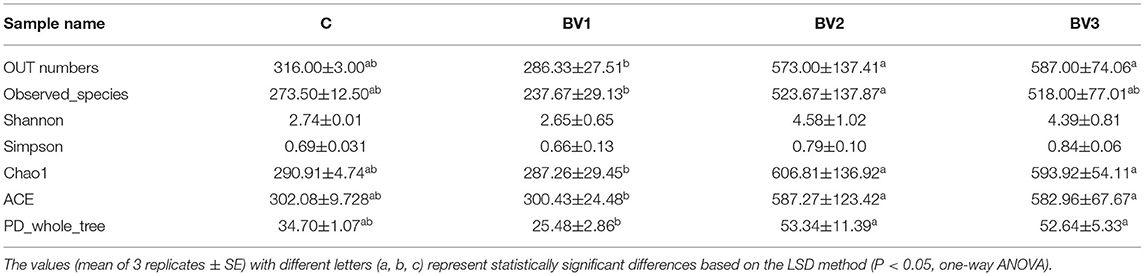
Table 6. Operational taxonomic unit (OTU) numbers, species richness, and diversity indices for the intestinal microbial community diversity analysis of L. vannamei fed different levels of B. velezensis BV007.
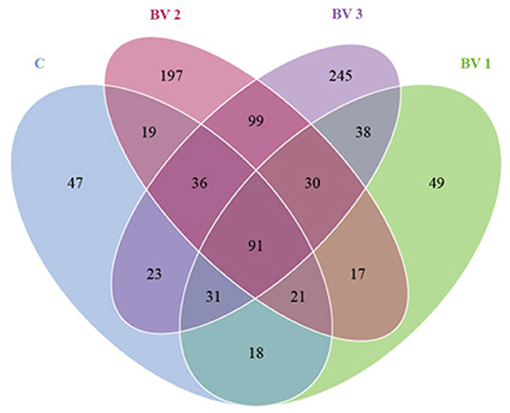
Figure 4. A Venn diagram showing the unique and shared operational taxonomic units (OTUs) among groups of L. vannamei fed diets containing 0 CFU/g (C), 105 CFU/g (BV1), 107 CFU/g (BV2), or 109 CFU/g (BV3) B. velezensis.
Bacterial Composition of Intestinal Microflora
The bacterial composition at the genus level in the intestine of L. vannamei is represented in Figure 5. The most abundant genera in the shrimp intestine were Candidatus Bacilloplasma, Vibrio, Pseudoalteromonas, Bacillus, and Tenacibaculum. After 42 days of feeding, the relative abundance of the predominant genera differed between the control and the groups fed with the BV2 and BV3 diets. In particular, the proportions of Vibrio and Pseudoalteromonas in the BV2 group (11.7 and 5.7%) were decreased compared with the C group (30.2 and 10.9%), BV1 group (15.2 and 10.7%), and BV3 group (17.9 and 8.8%). In addition, the proportion of Bacillus in the BV2 group (7.7%) was significantly higher than that in the C group (0.2%, P < 0.05).
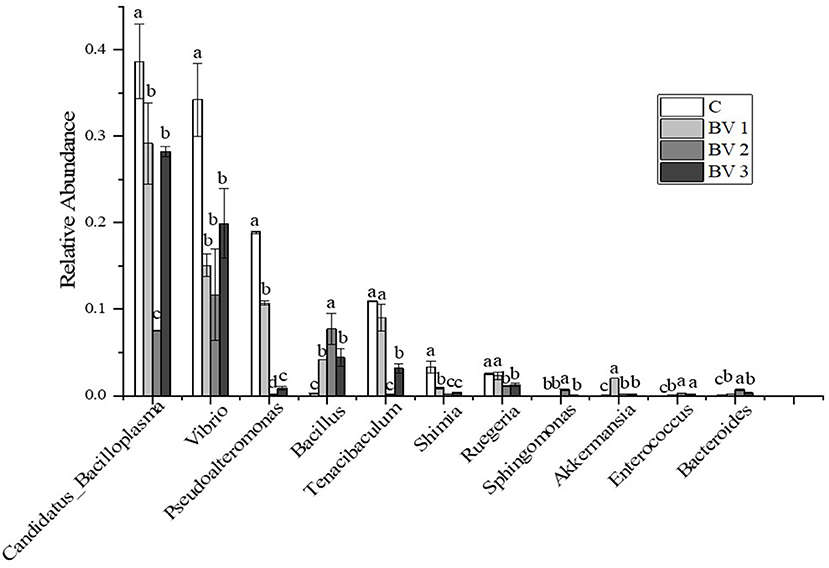
Figure 5. Relative abundance of the top 10 classes at the genus level of intestinal microflora of L. vannamei fed diets containing 0 CFU/g (C), 105 CFU/g (BV1), 107 CFU/g (BV2), or 109 CFU/g (BV3) B. velezensis. Error bars with different letters (a, b, c) represent statistically significant differences based on the LSD method (P < 0.05, one-way ANOVA). The results were shown as mean ± SE (N = 3).
Challenge Test
After the 2-weeks challenge with V. parahaemolyticus, the cumulative mortality rates of L. vannamei were shown in Figure 6. It was observed that the cumulative mortality was significantly lower in the treated groups than in the untreated ones, that is, 97.2, 61.1, 30.6, and 51.4% for shrimps fed with the C, BV1, BV2, and BV3, respectively.
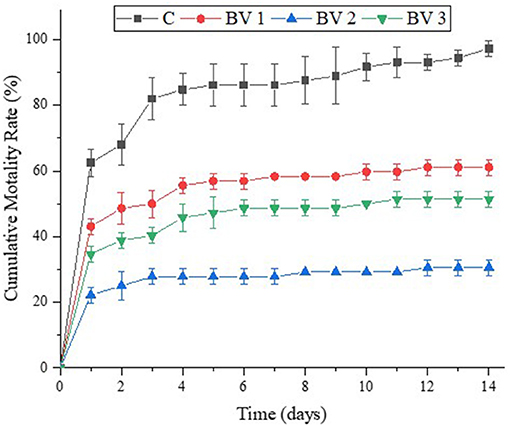
Figure 6. Effects of dietary administration of B. velezensis on the cumulative mortality percentage of L. vannamei after infection with V. parahaemolyticus.
Discussion
Many strains of some Bacillus sp. are currently used as probiotic dietary supplements in aquatic animal feeds (Foysal and Lisa, 2018; Kewcharoen and Srisapoome, 2019; Kuebutornye et al., 2019; Zhai et al., 2019; Zhou et al., 2019; Liu et al., 2020). These probiotics not only produce certain essential micronutrients that promote better growth and feed utilization of the hosts (Xie et al., 2019) but also participate in the digestion processes that break down nutrients, such as carbohydrates, proteins, and lipids by producing extracellular enzymes (e.g., amylase, trypsin, lipase) (Wang et al., 2007; Zhang et al., 2020). In this study, a basal diet with continuous supplementation of B. velezensis was shown to increase the growth performance of shrimp, which is consistent with the results of previous studies in tilapia (Galagarza et al., 2018; Hassaan et al., 2018), carp (Fan et al., 2018; Jiang et al., 2019), and grouper (Li et al., 2019b). The observed improvement in growth performance could be ascribed to the enhanced intestinal digestive enzyme activities (Bokkenheuser et al., 1988; Xu et al., 2003; Fu et al., 2018). In the present study, the digestive enzymes, such as α-amylase (known to catalyze the hydrolysis of starch into sugars), lipase (known to catalyze the hydrolysis of fats and lipids), and trypsin (catalyzing the hydrolysis of proteins into smaller peptides) significantly increased in the treated groups compared with the untreated shrimp (Rawlings and Barrett, 1994; Svendsen, 2000). Similar observations were recorded in Apostichopus japonicus and Salmo salar L. that were fed diets supplemented with B. velezensis (Wang et al., 2018; Wang J. et al., 2019). It was also noted that Bacillus genus might secrete a wide range of exoenzymes that aid in the nutritional enhancement of the host. As a result, the increase in digestive enzyme activities can be linked to the elevated beneficial bacteria in the Bacillus-treated groups since they secrete chemical compounds, such as protease, amylases, and β-galactosidases. Notably, the GPT and GOT activities were reduced in the B. velezensis-supplemented groups, indicating that no hepatopancreas tissue damage or dysfunction was induced by the addition of probiotics (Cao et al., 2012).
The modulation of immune responses and disease resistance is a major function induced by probiotics. As a result, both cellular and humoral immunity are stimulated in response to a pathogenic challenge (Goulart et al., 2019; Ng et al., 2019; Wang G. et al., 2019). In this study, the phagocytosis of L. vannamei hemocytes was increased with the addition of B. velezensis. Similarly, Rengpipat et al. (2000) found that the use of Bacillus S11 could activate cellular immune defenses of Penaeus monodon. During phagocytosis, respiratory bursts were used to kill bacterial pathogens, relying in particular on ROS, but their overproduction can cause damage to biomolecules, such as lipids, proteins, and nucleic acids, and generate oxidative stress (Spencer et al., 2019). After infection, BVs groups significantly reduced the damage of ROS to the body, possibly by modulation of the internal antioxidants and antioxidative systems (Chen et al., 2015). The SOD and CAT are considered to be molecular biomarkers for evaluating the oxidative stress status of aquatic organisms (Valavanidis et al., 2006; Yang et al., 2015). After the bacterial challenge, the activities of SOD and CAT showed similar trends, increasing slightly at first and then decreasing, which suggest that dietary supplemented probiotics improved the hepatopancreas antioxidant capacity of shrimp. ACP and AKP are necessary for phosphorylation and dephosphorylation, which are very important to the crustacean immune system (Yang et al., 2007). In the present study, the AKP activities in B. velezensis-supplemented groups were significantly higher than that in the control group. Similar results have also been found by Amoah and Cai (Amoah et al., 2019b; Cai et al., 2019). The results suggested that dietary supplementation of Paenibacillus polymyxa, B. licheniformis, and Bacillus flexus enhanced the growth, hepatopancreas immune, and antioxidant activities of shrimps. In addition, the expressions of immune-related genes were highly induced in BVs groups. Among them, crustin and penaeidin 3α are vital antibacterial peptides in shrimps, powerful for the elimination of Vibrio (Cuthbertson et al., 2002, 2005; Muñoz et al., 2002; Arockiaraj et al., 2013). The enhanced expression of these genes contributes to resist invading bacteria and improve non-specific immunity (Muñoz et al., 2002; Arockiaraj et al., 2013; Cuthbertson et al., 2005). Overall, the dietary supplemented B. velezensis BV007 could boost the innate immunity of shrimp at an appropriate level.
The intestinal microbiota of shrimp plays an essential role in mediating immunity, nutrient metabolism, and energy homeostasis (Zhang et al., 2020). The previous studies have shown that probiotics in the shrimp diet could effectively modulate the intestinal microbiota, improve growth performance, and enhance disease resistance (Yang et al., 2015; Suo et al., 2017; Amoah et al., 2019a; Xie et al., 2019). In the present study, a greater diversity of bacterial species was found in the BV007 supplemented groups than that in the control group. The reason may lie in the changed metabolic activities or beneficial effects on gut microbiota regulated by B. velezensis (He et al., 2019; Li et al., 2019a). Notably, the Vibrio species are severe pathogens in aquatic organisms (Hsu and Chen, 2007). In 2013, severe acute hepatopancreatic necrosis disease (AHPND) was induced by V. parahaemolyticus (Tran et al., 2013) causing mass mortality of shrimps specifically in the affected Southeast Asian countries (Hsu and Chen, 2007). In this study, cumulative mortality of the probiotics treated groups was significantly lower than the untreated group after V. parahaemolyticus challenge. It suggested that B. velezensis 007 could improve the disease resistance of L. vannamei. Similar results were also proved by Zokaeifar et al. (2012) and Amoah et al. (2019b). They suggested that Bacillus subtilis and Bacillus coagulans ATCC 7050 enhanced the anti-disease ability in shrimp. The results might be attributed to the increased abundances of beneficial genus bacteria Bacillus and enhanced immune responses in the treated groups (Amoah et al., 2019b).
The present study showed that diet supplementation with the potential probiotic B. velezensis BV007 could significantly promote growth performance, enhance immune response, and disease resistance in L. vannamei. Based on these findings, we conclude that a dose of 107 CFU/g feed provides health and growth benefits by improving the digestive enzyme activities, immune responses, intestinal development, and gut microflora.
Data Availability Statement
The datasets presented in this study can be found in online repositories. The names of the repository/repositories and accession number(s) can be found in the article/supplementary material.
Author Contributions
SH conceived and designed the experiments. LC and CL conducted the experiments. BL, HZ, and QZ analyzed the data. LR, XZ, CS, and JG contributed reagents, materials, and analytical tools. LC, CL, and SH wrote the manuscript. All authors contributed to the article and approved the submitted version.
Funding
This research was supported by grants from the Key Research and Development Project of Shandong (No. 2020CXGC011404), the Natural Science Foundation of Shandong Province (ZR2020QD097), and the Key Research and Development Project of Yantai (2019XDHZ105).
Conflict of Interest
The authors declare that the research was conducted in the absence of any commercial or financial relationships that could be construed as a potential conflict of interest.
Publisher's Note
All claims expressed in this article are solely those of the authors and do not necessarily represent those of their affiliated organizations, or those of the publisher, the editors and the reviewers. Any product that may be evaluated in this article, or claim that may be made by its manufacturer, is not guaranteed or endorsed by the publisher.
References
Amoah, K., Huang, Q.-C., Dong, X.-H., Tan, B.-P., Zhang, S., Chi, S.-Y., et al. (2019a). Paenibacillus polymyxa, improves the growth, immune and antioxidant activity, intestinal health, and disease resistance in Litopenaeus vannamei challenged with Vibrio parahaemolyticus. Aquaculture 518:734563. doi: 10.1016/j.aquaculture.2019.734563
Amoah, K., Huang, Q.-C., Tan, B.-P., Zhang, S., Chi, S.-Y., Yang, Q.-H., et al. (2019b). Dietary supplementation of probiotic Bacillus coagulans ATCC 7050, improves the growth performance, intestinal morphology, microflora, immune response, and disease confrontation of Pacific white shrimp, Litopenaeus vannamei. Fish Shellfish Immunol. 87, 796–808. doi: 10.1016/j.fsi.2019.02.029
Arockiaraj, J., Gnanam, A. J., Muthukrishnan, D., Gudimella, R., Milton, J., Singh, A., et al. (2013). Crustin, a WAP domain containing antimicrobial peptide from freshwater prawn Macrobrachium rosenbergii: immune characterization. Fish Shellfish Immunol. 34, 109–118. doi: 10.1016/j.fsi.2012.10.009
Bokkenheuser, V. D., Shackleton, C., and Winter, J. (1988). Hydrolysis of dietary flavonoid glycosides by strains of intestinal Bacteroides from humans. Biochem. J. 248, 953–956. doi: 10.1042/bj2480953
Cai, Y., Yuan, W., Wang, S., Guo, W., Li, A., Wu, Y., et al. (2019). In vitro screening of putative probiotics and their dual beneficial effects: to white shrimp (Litopenaeus vannamei) postlarvae and to the rearing water. Aquaculture 498, 61–71. doi: 10.1016/j.aquaculture.2018.08.024
Cao, J. M., Yan, J., Wang, G. X., Huang, Y. H., Zhang, R. B., Zhou, T. L., et al. (2012). Effects of replacement of fish meal with housefly maggot meal on digestive enzymes, transaminases activities and hepatopancreas histological structure of Litopenaeus vannamei. South China Fish. Sci. 8, 72–79. doi: 10.3969/j.issn.2095-0780.2012.05.011
Caporaso, J., Lauber, C., Walters, W., Berg-Lyons, D., Lozupone, C., Turnbaugh, P., et al. (2011). Global patterns of 16S rRNA diversity at a depth of millions of sequences per sample. Proc. Natl. Acad. Sci. USA. 108 Suppl 1, 4516–4522. doi: 10.1073/pnas.1000080107
Chai, P.-C., Song, X.-L., Chen, G.-F., Xu, H., and Huang, J. (2016). Dietary supplementation of probiotic Bacillus PC465 isolated from the gut of Fenneropenaeus chinensis improves the health status and resistance of Litopenaeus vannamei against white spot syndrome virus. Fish Shellfish Immunol. 54, 602–611. doi: 10.1016/j.fsi.2016.05.011
Chen, Y. Y., Lee, P.-C., Wu, Y.-L., and Liu, L.-Y. (2015). In vivo effects of free form astaxanthin powder on anti-oxidation and lipid metabolism with high-cholesterol diet. PLoS ONE 10:e0134733. doi: 10.1371/journal.pone.0134733
Cuthbertson, B. J., Shepard, E. F., Chapman, R. W., and Gross, P. S. (2002). Diversity of the penaeidin antimicrobial peptides in two shrimp species. Immunogenetics 54, 442–445. doi: 10.1007/s00251-002-0487-z
Cuthbertson, B. J., Yang, Y., Bachère, E., Büllesbach, E. E., and Aumelas, A. J. J. o. B. C. (2005). Solution structure of synthetic penaeidin-4 with structural and functional comparisons with penaeidin-3. J. Biol. Chem. 280, 16009–16018. doi: 10.1074/jbc.M412420200
Dawood, M., Abo-Al-Ela, H. G., and Hasan, M. T. (2020). Modulation of transcriptomic profile in aquatic animals: probiotics, prebiotics and synbiotics scenarios. Fish Shellfish Immunol. 97, 268–282. doi: 10.1016/j.fsi.2019.12.054
Delaporte, M., Soudant, P., Moal, J., Lambert, C., Quéré, C., Miner, P., et al. (2003). Effect of a mono-specific algal diet on immune functions in two bivalve species—Crassostrea gigas and Ruditapes philippinarum. J. Exp. Biol. 206, 3053–3064. doi: 10.1242/jeb.00518
Fan, Y., Liu, L., Zhao, L., Wang, X., Wang, D., Huang, C., et al. (2018). Influence of Bacillus subtilis ANSB060 on growth, digestive enzyme and aflatoxin residue in Yellow River carp fed diets contaminated with aflatoxin B1. Food Chem. Toxicol. 113, 108–114. doi: 10.1016/j.fct.2018.01.033
Foysal, M. J., and Lisa, A. K. (2018). Isolation and characterization of Bacillus sp. strain BC01 from soil displaying potent antagonistic activity against plant and fish pathogenic fungi and bacteria. J. Genet. Eng. Biotechnol. 16, 387–392. doi: 10.1016/j.jgeb.2018.01.005
Fu, L.-L., Wang, Y., Wu, Z.-C., and Li, W.-F. (2011). In vivo assessment for oral delivery of Bacillus subtilis harboring a viral protein (VP28) against white spot syndrome virus in Litopenaeus vannamei. Aquaculture 322–323, 33–38. doi: 10.1016/j.aquaculture.2011.09.036
Fu, S., Wang, L., Tian, H., Wei, D., and Liu, Y. (2018). Pathogenicity and genomic characterization of Vibrio parahaemolyticus strain PB1937 causing shrimp acute hepatopancreatic necrosis disease in China. Ann. Microbiol. 68, 175–184. doi: 10.1007/s13213-018-1328-0
Galagarza, O. A., Smith, S. A., Drahos, D. J., Eifert, J. D., Williams, R. C., and Kuhn, D. D. (2018). Modulation of innate immunity in Nile tilapia (Oreochromis niloticus) by dietary supplementation of Bacillus subtilis endospores. Fish Shellfish Immunol. 83, 171–179. doi: 10.1016/j.fsi.2018.08.062
Gao, X., Zhang, M., Li, X., Han, Y., Wu, F., and Liu, Y. (2018). Effects of a probiotic (Bacillus licheniformis) on the growth, immunity, and disease resistance of Haliotis discus hannai Ino. Fish Shellfish Immunol. 76, 143–152. doi: 10.1016/j.fsi.2018.02.028
Goulart, C., Rodriguez, D., Kanno, A. I., Silva, J. L. S. C., and Leite, L. C. C. (2019). Early pneumococcal clearance in mice induced by systemic immunization with recombinant BCG PspA-PdT prime and protein boost correlates with cellular and humoral immune response in bronchoalveolar fluids (BALF). Vaccine: X 4:100049. doi: 10.1016/j.jvacx.2019.100049
Hassaan, M. S., Soltan, M. A., Mohammady, E. Y., Elashry, M. A., El-Haroun, E. R., and Davies, S. J. (2018). Growth and physiological responses of Nile tilapia, Oreochromis niloticus fed dietary fermented sunflower meal inoculated with Saccharomyces cerevisiae and Bacillus subtilis. Aquaculture 495, 592–601. doi: 10.1016/j.aquaculture.2018.06.018
He, G., Dong, Y., Huang, J., Wang, X., Zhang, S., Wu, C., et al. (2019). Alteration of microbial community for improving flavor character of Daqu by inoculation with Bacillus velezensis and Bacillus subtilis. LWT 111, 1–8. doi: 10.1016/j.lwt.2019.04.098
Hsu, S.-W., and Chen, J.-C. (2007). The immune response of white shrimp Penaeus vannamei and its susceptibility to Vibrio alginolyticus under sulfide stress. Aquaculture 271, 61–69. doi: 10.1016/j.aquaculture.2007.05.028
Interaminense, J., Vogeley, J., Gouveia, C., Portela, R., Oliveira, J., Andrade, H., et al. (2018). In vitro and in vivo potential probiotic activity of Bacillus subtilis and Shewanella algae for use in Litopenaeus vannamei rearing. Aquaculture 488, 114–122. doi: 10.1016/j.aquaculture.2018.01.027
Interaminense, J. A., Vogeley, J. L., Gouveia, C. K., Portela, R. S., Oliveira, J. P., Silva, S. M. B. C., et al. (2019). Effects of dietary Bacillus subtilis and Shewanella algae in expression profile of immune-related genes from hemolymph of Litopenaeus vannamei challenged with Vibrio parahaemolyticus. Fish Shellfish Immunol. 86, 253–259. doi: 10.1016/j.fsi.2018.11.051
Jiang, Y., Zhou, S., and Chu, W. (2019). The effects of dietary Bacillus cereus QSI-1 on skin mucus proteins profile and immune response in Crucian Carp (Carassius auratus gibelio). Fish Shellfish Immunol. 89, 319–325. doi: 10.1016/j.fsi.2019.04.014
Kalgraff, C. A. K., Wergeland, H. I., and Pettersen, E. F. (2011). Flow cytometry assays of respiratory burst in Atlantic salmon (Salmo salar L.) and in Atlantic cod (Gadus morhua L.) leucocytes. Fish Shellfish Immunol. 31, 381–388. doi: 10.1016/j.fsi.2011.05.028
Kewcharoen, W., and Srisapoome, P. (2019). Probiotic effects of Bacillus spp. from Pacific white shrimp (Litopenaeus vannamei) on water quality and shrimp growth, immune responses, and resistance to Vibrio parahaemolyticus (AHPND strains). Fish Shellfish Immunol. 94, 175–189. doi: 10.1016/j.fsi.2019.09.013
Kuebutornye, F. K. A., Abarike, E. D., and Lu, Y. (2019). A review on the application of Bacillus as probiotics in aquaculture. Fish Shellfish Immunol. 87, 820–828. doi: 10.1016/j.fsi.2019.02.010
Li, A., Wang, Y., Pei, L., Mehmood, K., Li, K., Qamar, H., et al. (2019a). Influence of dietary supplementation with Bacillus velezensis on intestinal microbial diversity of mice. Microb. Pathog. 136:103671. doi: 10.1016/j.micpath.2019.103671
Li, J., Wu, Z.-B., Zhang, Z., Zha, J.-W., Qu, S.-Y., Qi, X.-Z., et al. (2019b). Effects of potential probiotic Bacillus velezensis K2 on growth, immunity and resistance to Vibrio harveyi infection of hybrid grouper (Epinephelus lanceolatus♂ × E. fuscoguttatus♀). Fish Shellfish Immunol. 93, 1047–1055. doi: 10.1016/j.fsi.2019.08.047
Lin, H. L., Shiu, Y. L., Chiu, C. S., Huang, S. L., and Liu, C. H. (2017). Screening probiotic candidates for a mixture of probiotics to enhance the growth performance, immunity, and disease resistance of asian seabass, lates calcarifer (bloch), against aeromonas hydrophila. Fish Shellfish Immunol. 60, 474–482. doi: 10.1016/j.fsi.2016.11.026
Liu, H., Wang, S., Cai, Y., Guo, X., Cao, Z., Zhang, Y., et al. (2017). Dietary administration of Bacillus subtilis HAINUP40 enhances growth, digestive enzyme activities, innate immune responses and disease resistance of tilapia, Oreochromis niloticus. Fish Shellfish Immunol. 60, 326–333. doi: 10.1016/j.fsi.2016.12.003
Liu, K.-F., Chiu, C.-H., Shiu, Y.-L., Cheng, W., and Liu, C.-H. (2010). Effects of the probiotic, Bacillus subtilis E20, on the survival, development, stress tolerance, and immune status of white shrimp, Litopenaeus vannamei larvae. Fish Shellfish Immunol. 28, 837–844. doi: 10.1016/j.fsi.2010.01.012
Liu, S., Wang, S., Cai, Y., Li, E., Ren, Z., Wu, Y., et al. (2020). Beneficial effects of a host gut-derived probiotic, Bacillus pumilus, on the growth, non-specific immune response and disease resistance of juvenile golden pompano, Trachinotus ovatus. Aquaculture 514:734446. doi: 10.1016/j.aquaculture.2019.734446
Livak, K. J., and Schmittgen, T. (2001). Analysis of relative gene expression data using real-time quantitative PCR and the 2−ΔΔCT method. Methods 25, 402–408. doi: 10.1006/meth.2001.1262
Muñoz, M., Vandenbulcke, F., Saulnier, D., and Bachère, E. J. E. J. (2002). Expression and distribution of penaeidin antimicrobial peptides are regulated by haemocyte reactions in microbial challenged shrimpandnbsp. 269, 2678–2689. doi: 10.1046/j.1432-1033.2002.02934.x
Neiland, A. E., Soley, N., Varley, J. B., and Whitmarsh, D. J. (2001). Shrimp aquaculture: economic perspectives for policy development. Mar. Policy 25, 265–279. doi: 10.1016/S0308-597X(01)00017-3
Ng, K.-H., Zhang, S. L., Tan, H. C., Kwek, S. S., Sessions, O. M., Chan, C.-Y., et al. (2019). Persistent dengue infection in an immunosuppressed patient reveals the roles of humoral and cellular immune responses in virus clearance. Cell Host Microbe 26, 601–605.e603. doi: 10.1016/j.chom.2019.10.005
Qin, L., Xiang, J., Xiong, F., Wang, G., Zou, H., Li, W., et al. (2020). Effects of Bacillus licheniformis on the growth, antioxidant capacity, intestinal barrier and disease resistance of grass carp (Ctenopharyngodon idella). Fish Shellfish Immunol. 97, 344–350. doi: 10.1016/j.fsi.2019.12.040
Raman, M., Ambalam, P., and Doble, M. (2019). Probiotics, prebiotics, and fibers in nutritive and functional beverages. Nutr. Beverag. 2019, 315–367. doi: 10.1016/B978-0-12-816842-4.00009-5
Rawlings, N. D., and Barrett, A. J. (1994). “Families of serine peptidases,” in Methods in Enzymology, vol. 244 (New York, NY: Academic Press), 19–61. doi: 10.1016/0076-6879(94)44004-2
Rengpipat, S., Rukpratanporn, S., Piyatiratitivorakul, S., and Menasaveta, P. (2000). Immunity enhancement in black tiger shrimp (Penaeus monodon) by a probiont bacterium (Bacillus S11). Aquaculture 191, 271–288. doi: 10.1016/S0044-8486(00)00440-3
Spencer, S. P., Fragiadakis, G. K., and Sonnenburg, J. L. (2019). Pursuing Human-Relevant Gut Microbiota-Immune Interactions. Immunity 51, 225–239. doi: 10.1016/j.immuni.2019.08.002
Suo, Y., Li, E., Li, T., Jia, Y., Qin, J. G., Gu, Z., et al. (2017). Response of gut health and microbiota to sulfide exposure in Pacific white shrimp Litopenaeus vannamei. Fish Shellfish Immunol. 63, 87–96. doi: 10.1016/j.fsi.2017.02.008
Svendsen, A. (2000). Lipase protein engineering. Biochim. Biophys. Acta Protein Struct. Mol. Enzymol. 1543, 223–238. doi: 10.1016/S0167-4838(00)00239-9
Tran, L., Nunan, L., Redman, R. M., Mohney, L. L., Pantoja, C. R., Fitzsimmons, K., et al. (2013). Determination of the infectious nature of the agent of acute hepatopancreatic necrosis syndrome affecting penaeid shrimp. Dis. Aquat. Org. 105, 45–55. doi: 10.3354/dao02621
Valavanidis, A., Vlachogianni, T., Dassenakis, M., and Scoullos, M. (2006). Molecular biomarkers of oxidative stress in aquatic organisms in relation to toxic environmental pollutants. Ecotoxicol. Environ. Saf. 64, 178–189. doi: 10.1016/j.ecoenv.2005.03.013
Velmurugan, S., Palanikumar, P., Velayuthani, P., Donio, M. B. S., Babu, M. M., Lelin, C., et al. (2015). Bacterial white patch disease caused by Bacillus cereus, a new emerging disease in semi-intensive culture of Litopenaeus vannamei. Aquaculture 444, 49–54. doi: 10.1016/j.aquaculture.2015.03.017
Wang, C., Liu, Y., Sun, G., Li, X., and Liu, Z. (2018). Growth, immune response, antioxidant capability, and disease resistance of juvenile Atlantic salmon (Salmo salar L.) fed Bacillus velezensis V4 and Rhodotorula mucilaginosa compound. Aquaculture 500, 65–74. doi: 10.1016/j.aquaculture.2018.09.052
Wang, G., Na, S., and Qin, L. (2019). Uncovering the cellular and humoral immune responses of Antheraea pernyi hemolymph to Antheraea pernyi nucleopolyhedrovirus infection by transcriptome analysis. J. Invertebr. Pathol. 166:107205. doi: 10.1016/j.jip.2019.107205
Wang, J., Li, B., Wang, Y., Liao, M., Rong, X., and Zhang, Z. (2019). Influences of immersion bathing in Bacillus velezensis DY-6 on growth performance, non-specific immune enzyme activities and gut microbiota of Apostichopus japonicus. J. Oceanol. Limnol. 37, 1449–1459. doi: 10.1007/s00343-019-8119-8
Wang, Q., Garrity, G., Tiedje, J. R., and Cole's, J. (2007). Naïve Bayesian classifier for rapid assignment of rRNA sequences into the new bacterial taxonomy. Appl. Environ. Microbiol. 73, 5261–5267. doi: 10.1128/AEM.00062-07
Wang, Y.-C., Hu, S.-Y., Chiu, C.-S., and Liu, C.-H. (2019). Multiple-strain probiotics appear to be more effective in improving the growth performance and health status of white shrimp, Litopenaeus vannamei, than single probiotic strains. Fish Shellfish Immunol. 84, 1050–1058. doi: 10.1016/j.fsi.2018.11.017
Xie, J., Liu, Q., Liao, S., Fang, H., Yin, P., Xie, S., et al. (2019). Effects of dietary mixed probiotics on growth, non-specific immunity, intestinal morphology and microbiota of juvenile pacific white shrimp, Litopenaeus vannamei. Fish Shellfish Immunol. 90, 456–465. doi: 10.1016/j.fsi.2019.04.301
Xin, Y., Xiaohe, G., Tingting, Y., Hongyu, W., Xiali, G., and Xi, Z. (2015). Study of enteropathogenic bacteria in children with acute diarrhoea aged from 7 to 10 years in Xuzhou, China. Microb. Pathog. 91, 41–45. doi: 10.1016/j.micpath.2015.11.027
Xu, J., Bjursell, M., Himrod, J., Deng, S., Carmichael, L., Chiang, H., et al. (2003). A genomic view of the Human–Bacteroides thetaiotaomicron symbiosis. Science 299, 2074–2076. doi: 10.1126/science.1080029
Xu, L., Yuan, J., Chen, X., Zhang, S., and Wu, Z. (2021). Screening of intestinal probiotics and the effects of feeding probiotics on the digestive enzyme activity, immune, intestinal flora and wssv resistance of procambarus clarkii. Aquaculture 540:736748. doi: 10.1016/j.aquaculture.2021.736748
Yang, C., Kong, J., Wang, Q., Liu, Q., Tian, y., and Luo, K. (2007). Heterosis of haemolymph analytes of two geographic populations in Chinese shrimp Fenneropenaeus chinensis. Fish Shellfish Immunol. 23, 62–70. doi: 10.1016/j.fsi.2006.09.005
Yang, H., Yang, M., Sun, J.-J., Guo, F., Lan, J., Wang, X., et al. (2015). Catalase eliminates reactive oxygen species and influences the intestinal microbiota of shrimp. Fish Shellfish Immunol. 47, 63–73. doi: 10.1016/j.fsi.2015.08.021
Zhai, J., Jiumu, L., Cong, L., Wu, Y., Dai, L., Zhang, Z., et al. (2019). Reed decomposition under Bacillus subtilis addition conditions and the influence on water quality. Ecohydrol. Hydrobiol. 20, 504–512. doi: 10.1016/j.ecohyd.2019.11.003
Zhang, J., Chen, H., Luo, L., Zhou, Z., and Wu, M. (2021). Structures of fructan and galactan from polygonatum cyrtonema and their utilization by probiotic bacteria. Carbohydr. Polym. 267:118219. doi: 10.1016/j.carbpol.2021.118219
Zhang, M., Shan, C., Tan, F., Limbu, S. M., Chen, L., and Du, Z.-Y. (2020). Gnotobiotic models: powerful tools for deeply understanding intestinal microbiota–host interactions in aquaculture. Aquaculture 517:734800. doi: 10.1016/j.aquaculture.2019.734800
Zhou, S., Song, D., Zhou, X., Mao, X., Zhou, X., Wang, S., et al. (2019). Characterization of Bacillus subtilis from gastrointestinal tract of hybrid Hulong grouper (Epinephelus fuscoguttatus × E. lanceolatus) and its effects as probiotic additives. Fish Shellfish Immunol. 84, 1115–1124. doi: 10.1016/j.fsi.2018.10.058
Zokaeifar, H., Babaei, N., Saad, C. R., Kamarudin, M. S., Sijam, K., and Balcazar, J. L. (2014). Administration of Bacillus subtilis strains in the rearing water enhances the water quality, growth performance, immune response, and resistance against Vibrio harveyi infection in juvenile white shrimp, Litopenaeus vannamei. Fish Shellfish Immunol. 36, 68–74. doi: 10.1016/j.fsi.2013.10.007
Zokaeifar, H., Balcazar, J., Saad, C., Kamarudin, M. S., Sijam, K., Arshad, A. B., et al. (2012). Effects of Bacillus subtilis on the growth performance, digestive enzymes, immune gene expression and disease resistance of white shrimp, Litopenaeus vannamei. Fish Shellfish Immunol. 33, 683–689. doi: 10.1016/j.fsi.2012.05.027
Keywords: Litopenaeus vannamei, Bacillus velezensis, growth performance, innate immunity, intestinal health
Citation: Chen L, Lv C, Li B, Zhang H, Ren L, Zhang Q, Zhang X, Gao J, Sun C and Hu S (2021) Effects of Bacillus velezensis Supplementation on the Growth Performance, Immune Responses, and Intestine Microbiota of Litopenaeus vannamei. Front. Mar. Sci. 8:744281. doi: 10.3389/fmars.2021.744281
Received: 20 July 2021; Accepted: 23 August 2021;
Published: 23 September 2021.
Edited by:
Yangfang Ye, Ningbo University, ChinaReviewed by:
Bin Xia, Qingdao Agricultural University, ChinaYanjiao Zhang, Ocean University of China, China
Yancui Zhao, Ludong University, China
Copyright © 2021 Chen, Lv, Li, Zhang, Ren, Zhang, Zhang, Gao, Sun and Hu. This is an open-access article distributed under the terms of the Creative Commons Attribution License (CC BY). The use, distribution or reproduction in other forums is permitted, provided the original author(s) and the copyright owner(s) are credited and that the original publication in this journal is cited, in accordance with accepted academic practice. No use, distribution or reproduction is permitted which does not comply with these terms.
*Correspondence: Shunxin Hu, hushunxin001@163.com
†These authors have contributed equally to this work