- 1MOE Key Laboratory of Marine Genetics and Breeding, College of Marine Life Sciences, Ocean University of China, Qingdao, China
- 2Yantai Marine Economic Research Institute, Yantai, China
- 3Laboratory for Marine Fisheries Science and Food Production Processes, Qingdao National Laboratory for Marine Science and Technology, Qingdao, China
Zhikong scallop (Chlamys farreri) is a bivalve species with broad economic and biological value, and an essential species of aquaculture in North China. Recently, efforts have been made to improve knowledge of genome, genetics, and cytogenetics, which is devoted to develop the molecular breeding project for the scallop. In this study, we constructed a cytogenetic map and identified all chromosomes of C. farreri using fluorescence in situ hybridization (FISH). A total of 100 Bacterial Artificial Chromosome (BAC) clones and 27 fosmid clones, including 58 microsatellite marker-anchored BAC clones, 4 genes-anchored BAC clones, 38 random BAC clones, 22 repetitive sequences-anchored fosmid clones, and 5 gene-anchored fosmid clones, were tested as probes, and 69 of them produced specific and stable signal on one pair of chromosomes. Then, multiple co-hybridizations were conducted to distinguish all the submetacentric and subtelocentric chromosomes with similar morphology by the abovementioned chromosome-specific markers. On this basis, a cytogenetic map of C. farreri containing 69 clones was constructed by co-hybridization and karyotype analysis. The markers covered all 19 pairs of chromosomes, and the average number of markers on each chromosome was 3.6. The cytogenetic map provides a platform for genetic and genomic analysis of C. farreri, which facilitates the molecular breeding project of C. farreri and promotes the comparative studies of chromosome evolution in scallops and even bivalves.
Introduction
Chromosome identification is an important part of genome research, which provides a broad view of chromatin structure organization. Traditionally, chromosome morphological characteristics (e.g., chromosome relative lengths and arm ratios) and banding techniques (e.g., C-banding, G-banding, and NORs-banding) have shown to be a powerful utility for the chromosome identification in many animal and plant species (Xu and Shi, 2007; Ocalewicz et al., 2008). However, these procedures have proven to be difficult in scallops and other bivalve species (Insua et al., 1998; Gajardo et al., 2002; Huang et al., 2007a,b), which were mainly due to the lack of cultured cells for the preparation of high-quality elongated chromosomes.
The development of fluorescence in situ hybridization (FISH) is a significant step toward chromosome identification, and FISH is a well-established approach for determining the location and relative order of DNA sequences in chromosomes (Zhang et al., 2008a; Merlo et al., 2021). The initial probes used as landmarks for chromosome distinguish were repetitive sequences, mainly including satellite sequences (Wang et al., 2001; Zhang et al., 2008a), ribosomal DNA (Zhang et al., 1999; Xu et al., 2001; Huang et al., 2007a,b), and histone genes (Eirín-López et al., 2002, 2004; Zhang et al., 2007a). However, these repetitive sequence probes could identify only partial chromosomes. Mapping using large-insert DNA genomic clones [e.g., P1, fosmid, and bacterial artificial chromosome (BAC)] as probes for FISH provided an alternative approach that has been successfully used in some shellfish species. Wang et al. (2005) accomplished the identification of seven pairs of chromosomes of eastern oysters by using P1 clones as FISH probes. Zhang et al. (2008a) successfully distinguished eight pairs of chromosomes of Chlamys farreri using eight fosmid clones. Li et al. (2016) and Yang et al. (2016) identified six and five pairs of chromosomes of Patinopecten yessoensis by six and eight fosmid clones, respectively. In some plant species, especially in those species with relatively small chromosomes, BAC-FISH had played an important role in chromosome identification and cytogenetic researches (Wang et al., 2007; Wai et al., 2010; Lee et al., 2020; Mendoza et al., 2020). In white campion Silene latifolia, 12 pairs of chromosomes were distinguished by the simultaneous hybridization of five BAC clones and three repetitive sequences on mitotic chromosomes (Lengerova et al., 2004). Furthermore, based on FISH, the integration of genetic and cytogenetic maps was carried out in many organisms, such as Bombyx mori (Yoshido et al., 2005), C. farreri (Feng et al., 2014), Scophthalmus maximus (Taboada et al., 2014), P. yessoensis (Yang et al., 2019), and some plants (Wang et al., 2006; Xiong et al., 2010; Dong et al., 2018; Yurkevich et al., 2021), which assisted the chromosome identification and evolution researches in the abovementioned species.
Zhikong scallop (C. farreri, Jones et Preston 1904), distributing along the sea coast of China, Japan, Korea, and Sakhalin in Russia, is one of the main mariculture species in China. It possessed the typical chromosome number for Pectinidae (2n = 38), which was considered the closest representative of the ancestral karyotype of Pectinidae (Wang and Guo, 2004). Chromosome morphological characteristics revealed that the chromosomes of C. farreri were continuous in size. In addition to three pairs of typical metacentric chromosomes, C. farreri had 16 pairs of submetacentric and/or subtelocentric chromosomes appearing similar in morphology (Wang and Guo, 2004; Huang et al., 2006). In recent years, the genomic resources of C. farreri, such as BAC libraries (Zhang et al., 2008b; Cheng, 2010), fosmid libraries (Zhang et al., 2007b), and genome (Li et al., 2017), are available. Some chromosomes of C. farreri had been distinguished by FISH using repetitive sequences or large-insert DNA clones as probes (Huang et al., 2006, 2012; Zhang et al., 2007a, 2008b; Huan et al., 2009a,b; Feng et al., 2014). However, it is still difficult to identify all 19 chromosomes of C. farreri based on the present FISH results of these probes. Until now, a complete set of chromosome-specific DNA markers have not been developed for this species.
In this study, chromosome-specific BAC and fosmid clones were selected as probes to accomplish the chromosome identification and cytogenetic map construction of C. farreri by multiple FISH. Among them, each chromosome of C. farreri could be identified by co-hybridization and karyotype analysis. The integrated cytogenetic map will contribute to further characterization and application of the C. farreri genome.
Materials and Methods
Scallop Chromosome Preparation
Zhikong scallop (C. farreri) trochophore larvae were obtained from an aquatic hatchery in Rongcheng, Shandong Province, China. Metaphase was prepared according to the methods described by Huang et al. (2006). Briefly, larvae were treated with colchicines (0.01%) in seawater for 2 h at room temperature, then exposed to 0.075 M KCl solution for 20 min, and, finally, fixed three times (15 min each) in the fresh Carnoy's solution (ethanol:glacial acetic acid, 3:1 v/v). The fixed larvae were dissociated into a cell suspension using 50% acetic acid and then dropped onto hot-wet slides and air-dried.
Probe Selection and Labeling
The chosen clones for testing signal specificity in FISH studies included microsatellite marker-anchored BAC clones, genes-anchored BAC clones, random BAC clones, repetitive sequences-anchored fosmid clones, and genes-anchored fosmid clones. All BAC clones used in the FISH analysis were screened from the C. farreri HindIII-BAC (BH) and BamHI-BAC (BB) libraries (Cheng, 2010), and the microsatellite markers contained in the BAC clones were selected based on the SSR genetic map of C. farreri (Zhan et al., 2009). All fosmid clones used in the FISH analysis were selected according to the information from pair-end sequencing results of the fosmid library of C. farreri (Zhang et al., 2007b). BAC and fosmid DNA were isolated via the standard laboratory methods (Sambrook et al., 1989) and then labeled with digoxigenin (Dig)-11-2'-deoxyuridine 5'-triphosphate (dUTP) or biotin-16-dUTP by Dig- or biotin-nick translation mix (Roche, American) according to the instructions of the manufacturer.
FISH and Co-hybridization
The FISH experiments were performed following the methods published by Huang et al. (2007b). Chromosome slides were pretreated with RNase A (100 μg/ml) in 2× saline sodium citrate buffer (SSC) at 37°C for 1 h, followed by pepsin (0.005%) in 10 mM HCl at 37°C for 10 min. Chromosome slides were denatured in 70% formamide mixed with 2× SSC at 75°C for 2 min, immediately dehydrated in a chilled ethanol series (70, 90, and 100%) for 5 min each, and then air-dried. Hybridization mixture consisted of 10–15 ng/μl Dig-11-dUTP and/or biotin-16-dUTP labeled BAC or fosmid DNAs, 50% deionized formamide, 10% dextran sulfate, and 2× SSC. The hybridization mixture was denatured at 90°C for 5 min and immediately cooled on ice. A denatured probe was then applied onto the slide, and DNA-DNA in situ hybridization was carried out in a moist chamber at 37°C for 16–18 h. Then, slides were washed in 50% formamide in 2× SSC at 37°C for 10 min, in 1× SSC at 37°C three times (5 min each), and in 2× SSC at room temperature for 5 min. Dig-labeled and biotin-labeled probes were detected using anti-digoxigenin-rhodamine (Roche, American) and fluorescein isothiocyanate (FITC)-conjugated avidin D cell sorter (DOS) (Vector, American), respectively. Chromosomal DNAs were then counterstained with 4,6-diamidino-2-phenylindole (DAPI) or 1.5 μg/ml propidium iodide (PI) at 37°C for 10 min. Hybridization signals were visualized, and images were recorded using a (Leica, Germany) DM4000B microscope equipped with an epifluorescence system and a charge coupled device (CCD) camera. At least 10 metaphases were examined for each probe and karyotype analysis, and the chromosomal pairs marked by clones were classified based on the Levan's criteria (Levan et al., 1964). Then, clones within distinct signals and discernable chromosomal location were selected for co-hybridization with other clones.
For co-hybridization, probes labeled with Dig- and biotin- were pooled for hybridization, and other protocol was the same as that for regular hybridization mentioned earlier.
Results
Development of Chromosome-Specific Cytogenetic Markers
After screening the BAC libraries of C. farreri, 58 microsatellite marker-anchored BAC clones and 4 genes-anchored BAC clones were obtained. Meanwhile, according to the information of pair-end sequences of the fosmid clones, 22 repetitive sequences-anchored clones and 5 genes-anchored clones were selected. In addition, 38 random BAC clones were also tested as a probe for FISH. In total, 127 BAC and fosmid clones were isolated and hybridized on mitotic metaphase chromosomes. Among them, 72 clones could successfully map on the chromosomes, and a total of 69 clones, including 58 BACs and 11 fosmids, could produce specific FISH signals on one pair of chromosomes. These BAC and fosmid clones producing unambiguous and reproducible FISH signals were used as chromosome-specific markers. As shown in Figure 1A, BAC clone BH1060G11 containing microsatellite marker CFLD034 hybridized to the middle of the long arm of a big pair of subtelocentric chromosomes. BAC clone BH565D6 containing microsatellite marker CFMSM014 was mapped to the long arm of a pair of submetacentric chromosomes, about two-fifth of the long arm away from the centromere (Figure 1B). FISH with BAC clone BB240C9 containing Dmrt4 gene revealed one single locus on the telomeric region of the long arm of a pair of submetacentric chromosomes (Figure 1C). The random BAC clone BH60F12 was located on the long arm of a pair of subtelocentric chromosomes, about four-fifth of the long arm away from the centromere (Figure 1D). In addition, fosmid clone F421B9 containing tandemly repetitive DNAs showed distinct signals on the telomeric region of the short arm of a pair of metacentric chromosomes (Figure 1E). Hybridization with fosmid clone F541H2 containing heat shock protein (Hsp22) gene (Figure 1F) revealed intensive signals on the short arm of a pair of subtelocentric chromosomes. The FISH results of all the isolated chromosome-specific clones were summarized in Table 1.
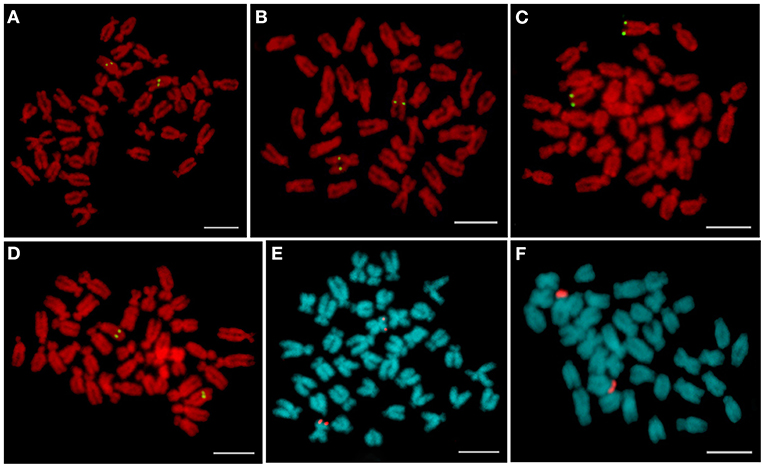
Figure 1. Representative fluorescence in situ hybridization (FISH) pattern of bacterial artificial chromosome (BAC) and fosmid clones on mitotic metaphase chromosomes of Chlamys farreri. (A) BH1060G11 containing microsatellite marker CFLD034; (B) BH565D6 containing microsatellite marker CFMSM014; (C) BB240C9 containing Dmrt4 gene; (D) random BAC clone BH60F12; (E) F421B9 containing repetitive sequences; and (F) F541H2 containing Hsp22 gene. Scale bars = 5 μm.
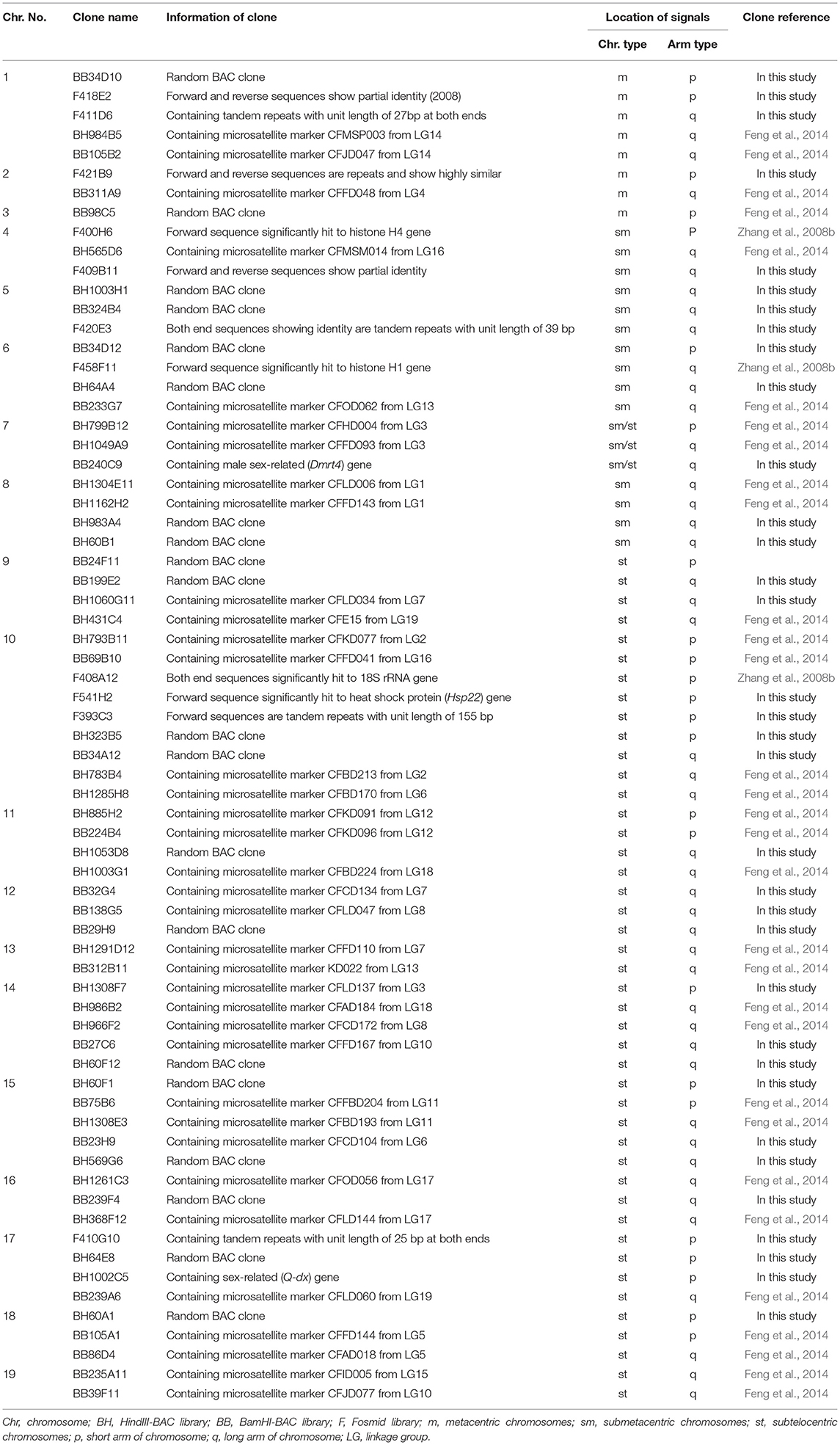
Table 1. Sequence information and hybridization results of 69 chromosome-specific markers in the cytogenetic map of Chlamys farreri.
Identification of All Chromosomes
Three pairs of typical metacentric chromosomes of C. farreri could be identified by their distinct size, as shown in Figure 2. The largest one was named as Chr. 1, then the middle one as Chr. 2, and the smallest one as Chr. 3.
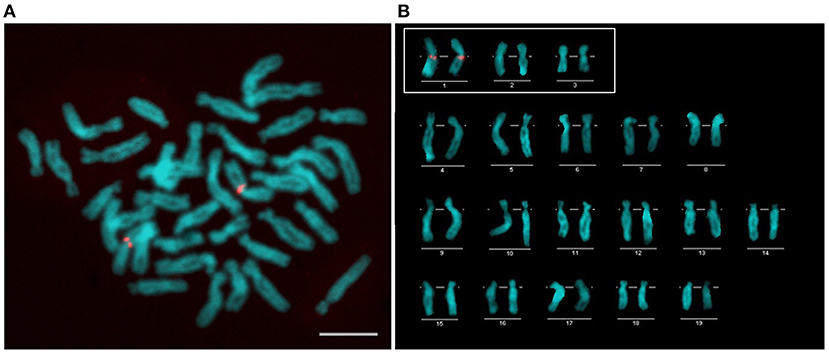
Figure 2. FISH mapping of F411D6 containing tandem repeats (A) and karyotype (B) of C. farreri after 4,6-diamidino-2-phenylindole (DAPI) staining. The chromosomes in the white box are three pairs of metacentric chromosomes. Scale bars = 5 μm.
Due to the other 16 pairs of submetacentric and subtelocentric chromosomes appeared similar in morphology and continuous in size, at least 15 chromosome-specific markers are required to distinguish them. Among the 69 clones showing unambiguous unique FISH signals in the earlier results, 15 most reliable representations from the 15 pairs of submetacentric and subtelocentric chromosomes, which were shown to be located on the same type or similar size of chromosomes (Figure 3), were selected for multiple two-color FISH. As shown in Supplementary Figure 1-1a, three clones of F409B11, F420E3, and F458F11 were hybridized to three different pairs of submetacentric chromosomes, and we named the three chromosomes as Chr. 4 (Figure 3A), Chr. 5 (Figure 3B), and Chr. 6 (Figure 3C), respectively. The co-hybridization with the mixed probes containing F409B11, F420E3, F458F11, and BH1049A9 (Supplementary Figure 1-1b) revealed that BAC clone BH1049A9 located another submetacentric/subtelocentric chromosomes, which were different from Chr. 4–6, and we named it as Chr. 7 (Figure 3D). Supplementary Figures 1-1c,d show that BH1304E11 was mapped on one small pair of submetacentric chromosomes differing from Chr. 4–7, and this chromosome was named for Chr. 8 (Figure 3E).
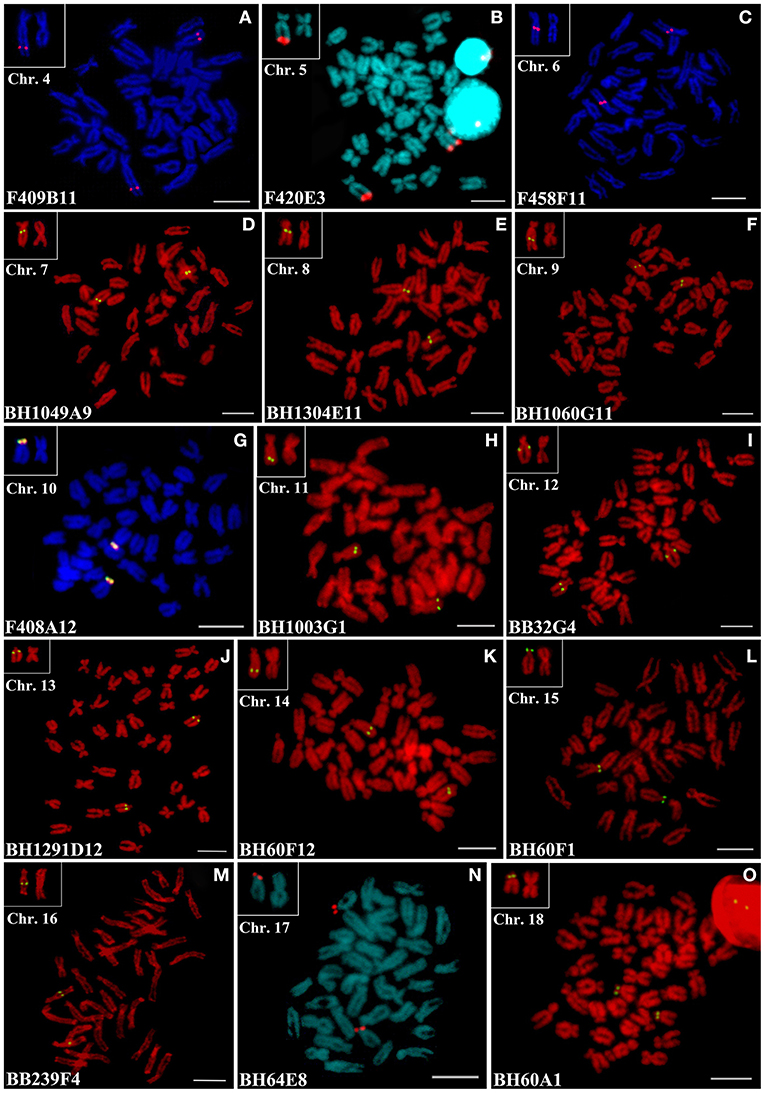
Figure 3. FISH results of 15 reliable representations from the 15 pairs of submetacentric and subtelocentric chromosomes of C. farreri. The chromosomes in the white box are the chromosome with specific signal and the largest metacentric chromosome. Scale bars = 5 μm.
Fluorescence in situ hybridization with BH1060G11, F409B11 (Chr. 4), and F420E3 (Chr. 5) (Supplementary Figure 1-1e) revealed that BH1060G11 was mapped on a big pair of a subtelocentric chromosome instead of submetacentric Chr. 4 and Chr. 5, although these three pairs of chromosomes showed similar size. The subtelocentric chromosome mapped with BH1060G11 was named as Chr. 9 (Figure 3F). Similarly, Supplementary Figures 1-1f,g show that clone F408A12 was assigned to another big pair of subtelocentric chromosomes named as Chr. 10 (Figure 3G) differing from Chr. 9 and Chr. 8. Clone BH1003G1 was located on a pair of submetacentric/subtelocentric chromosomes, and the co-hybridizations of this clone and F458F11 (Chr. 6) (Supplementary Figure 1-1j), this clone and BH1049A9 (Chr. 7) (Supplementary Figure 1-1h), as well as this clone and BH1060G11 (Chr. 9) and F408A12 (Chr. 10) (Supplementary Figure 1-1i) confirmed the different chromosome assignments of BH1003G1, and we named this chromosome as Chr. 11 (Figure 3H). BB32G4, BH60F1, BH1003G1 (Chr. 11), and F408A12 (Chr. 10) were hybridized to four different pairs of chromosomes (Supplementary Figures 1-1k,l), and the chromosome hybridized by BB32G4 was named as Chr. 12 (Figure 3I). BH1291D12 was located on another pair of subtelocentric chromosomes differing from Chr. 9–12 by the co-hybridization of BH1291D12, BH1060G11 (Chr. 9), and BB32G4 (Chr. 12) (Supplementary Figure 1-1m), BH1291D12 and F408A12 (Chr. 10) (Supplementary Figure 1-1n), as well as BH1291D12 and BH1003G1 (Chr. 11) (Supplementary Figure 1-1o), and this pair of chromosomes was named as Chr. 13 (Figure 3J). Supplementary Figures 1-2(a–c) revealed that clones BH60F12, BH1291D12 (Chr. 13), BB32G4 (Chr. 12), BH1003G1 (Chr. 11), and BH60F1 were assigned to different subtelocentric chromosomes, and the BH60F12-locating chromosome was slightly smaller than Chr. 13, and it was named as Chr. 14 (Figure 3K). Clone BH60F1 confirmed its location at another subtelocentric chromosome [named as Chr. 15 (Figure 3L)] by the co-hybridization results of this clone with the other clones mapped to the identified subtelocentric chromosomes, as shown in Supplementary Figures 1-1(k,l), 1-2(c,d). The clone BB239F4 had an assignment of the subtelocentric chromosome, named as Chr. 16 (Figure 3M), which were different from Chr. 9–15, which were revealed by the co-hybridization results of BB239F4 and BH60F1 (Chr. 15) (Supplementary Figure 1-2e), BB239F4 and BH60F12 (Chr. 14) (Supplementary Figure 1-2f), BB239F4, BH1291D12 (Chr. 13), and BH1060G11 (Chr. 11) (Supplementary Figure 1-2g), BB239F4 and BB32G4 (Chr. 12) (Supplementary Figure 1-2h), as well as BB239F4, F408A12 (Chr. 10), and BH1060G11 (Chr. 9) (Supplementary Figure 1-2i). BH64E8 and BH60A1 were readily mapped on two pairs of small subtelocentric chromosomes (Supplementary Figure 1-2o), and the co-hybridization analysis revealed that they could separate these two pairs of chromosomes from the other small subtelocentric chromosomes involving Chr. 16, 15, 14, and 13, as shown in Supplementary Figures 1-2(j–n). These two small subtelocentric chromosomes were named as Chr. 17 (Figure 3N) and Chr. 18 (Figure 3O), respectively. Since 15 of the 16 submetacentric and subtelocentric chromosomes with similar morphology and size had been identified, the remaining one would be automatically distinguished, and it was named as Chr. 19.
Based on the abovementioned data, all the 19 chromosomes of C. farreri were successfully distinguished and named for the first time.
Construction of a Cytogenetic Map
The establishment of representative chromosome-specific cytogenetic markers allowed to construct a cytogenetic map of C. farreri. The main strategy was to do the co-localization analysis of the newly developed chromosome-specific markers and the representative chromosome-specific markers on the same chromosome.
By using this strategy, fosmid clone F418E2, BAC clones BB34D10, BH984B5, and BB105B2 were all hybridized to Chr. 1, and BB34D10 was located on the telomeric region of the short arm of Chr. 1, F418E2 was on the middle of one arm of this chromosome, while BH984B5 and BB105B2 were mapped to about middle and telomeric region of the other arm of Chr. 1, respectively (Figures 4A–C). The co-hybridizations of F458F11, BB34D12, and BB233G7 (Figure 4D), as well as BB34D12 and BH64A4 (Figure 4E), revealed that these clones were all assigned to Chr. 6. Among them, BB34D12 was located near the telomeric region of the short arm, the other three clones were all mapped to the long arm, which were close to the centromere (F458F11 and BH64A4), and about telomeric region of Chr. 6 (BB233G7). As shown in Figure 4F, BH1049A9 and BB240C9 were observed as located near the centromere and at the telomeric region of the long arm of Chr. 7, respectively. Clone BH799B12 was also mapped to Chr. 7, and it was located about midway on the short arm of this chromosome. The co-hybridization of BH1304E11 and BH60B1 confirmed their locations on Chr. 8, near the centromere and about four-fifth of the long arm away from the centromere, respectively (Figure 4G). BH1162H2 was mapped to the location that was near the BH1304E11 on the same chromosome. The chromosomal locations of F541H2, F393C3, BB69B10, BH323B5, and BH1285H8 were analyzed by simultaneously hybridizing with the representative clone of Chr. 10 (F408A12). Five clones of F541H2, F393C3, BB69B10, BH323B5, and F408A12 were all located on the short arm of Chr. 10, and their relative order on the chromosome was not unambiguous on the highly condensed metaphase chromosomes (Figures 4H–J). Clone BH1285H8 produced bright signals on the long arm of Chr. 10, about one-fourth from the centromere (BH1285H8, Figure 4K). BH60F12 and BH 966F2 were hybridized on the same chromosome with BH986B2, and they were all located on the long arm of Chr. 14 (Figures 4L,M). Of which, BH986B2 was mapped to near the centromeric region, BH 966F2 was on the middle of the long arm, and BH60F12 was about three-fourth from the centromere. BAC clones BH60F1 and BB75B6 were both located at the end of the short arm of Chr. 15 (Figure 4N). BH1308E3 was also mapped to Chr. 15 by co-hybridization with BB75B6, and it was hybridized near the centromeric region of the long arm. BH64E8 and BH1002C5 were simultaneously located on the short arm of Chr. 17, and it was difficult to distinguish their chromosomal locations (Figure 4O).
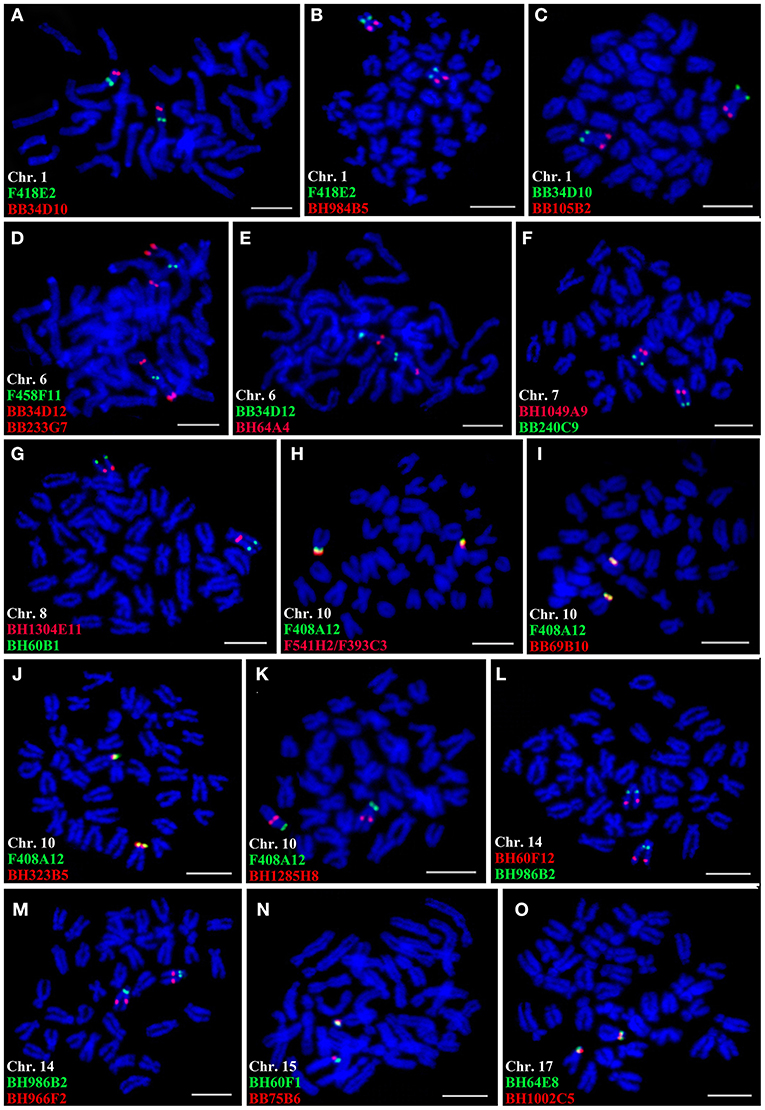
Figure 4. Co-hybridization of the clones on the same chromosomes. Red and green signals correspond to digoxigenin (Dig)- and biotin-labeled probes, respectively. Scale bars = 5 μm.
Referring to the FISH results of a single specific probe, 69 clones could produce positive signals on a single chromosome. However, for some of them, especially those producing high background, it was difficult to achieve the co-hybridization of multiple clones because the FISH condition of these clones was different. We determined the chromosomal locations of these clones by the karyotype analysis based on their one-color FISH. In summary, all the available data were used for the construction of the karyotype ideogram of C. farreri, which indicated the FISH mapping of 69 cytogenetic markers (Figure 5). All the information on these 69 markers is provided in Table 1. The cytogenetic markers on different chromosomes were different, with 3.6 as an average number of markers. Of which, Chr. 10 has the most number of markers which was nine, and Chr. 3 has the least number of markers which was one.
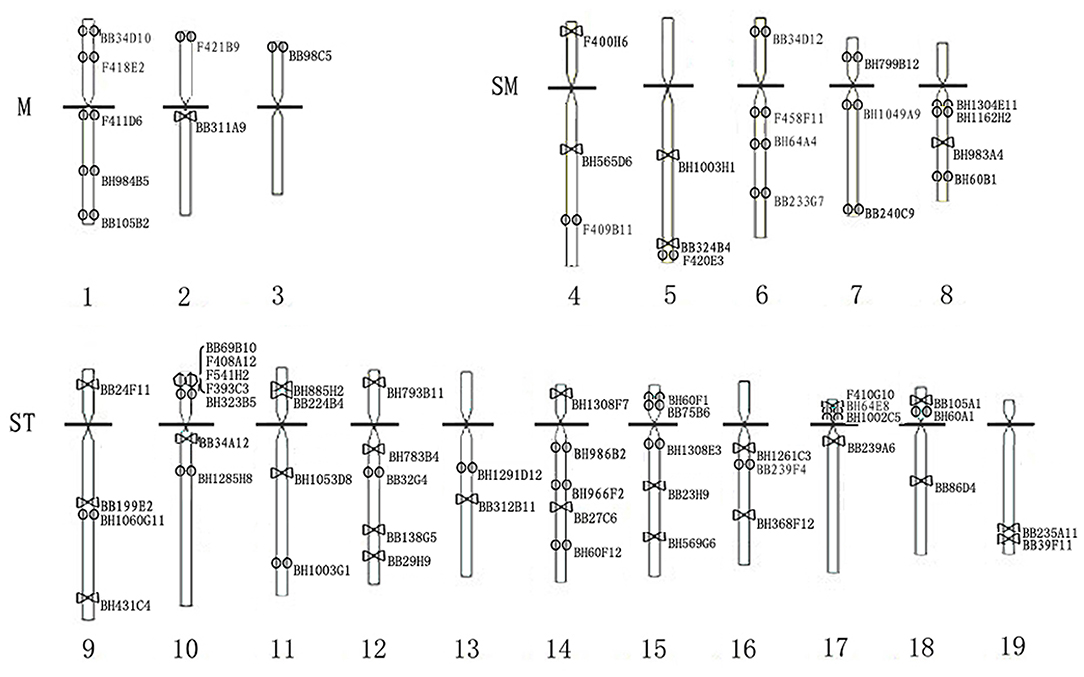
Figure 5. Karyotype ideogram of C. farreri showing chromosome assignment of 69 markers. The circles represent the loci of the markers that have been confirmed by co-hybridization. The triangles represent the loci of the markers determined by the karyotype analysis.
Discussion
Cytogenetic researches rely on accurate chromosome identification, which is also the first step in understanding the genome organization of a species. Unfortunately, it is still a challenge to identify all the individual chromosomes of C. farreri. The studies of karyotype, silver staining, and fluorescent staining (PI and DAPI) offered limited information for the reliably distinguishing C. farreri chromosomes (Huang et al., 2006; Xu et al., 2011). Based on the large-insert genomic DNA libraries (e.g., fosmid and BAC), FISH is an efficient method to accomplish the chromosome-specific identification. Zhang et al. (2008a) have successfully identified eight chromosomes of C. farreri by using eight fosmid clones as FISH probes, among which five clones were simultaneously located on different chromosomes by two-color co-hybridization. Combining these results, in this study, we isolated some chromosome-specific fosmid clones from the C. farreri fosmid library (Zhang et al., 2007b). In addition, a large number of chromosome-specific BAC clones were also isolated from the C. farreri BH and BB libraries (Cheng, 2010). These were the basis for identifying individual chromosome and constructing the cytogenetic map of C. farreri.
In many plant species, chromosomes were arranged based on their lengths in conventional karyotyping, with the longest one as the first chromosome and the shortest one as the last chromosome, although the chromosome numbering systems were not unified. In the potato karyotyping, the chromosomes distinguished based on the Giemsa-banding patterns were named either numerically, from chromosome 1 to chromosome 12 (Pijnacker and Ferwerda, 1984), or alphabetically, from chromosome A to chromosome L (Wagenvoort et al., 1994). Moreover, the pachytene chromosomes of potatoes were named using a Roman number system (Yeh and Peloquin, 1965). Unfortunately, there has been no unambiguous numbering and naming for all the chromosomes of C. farreri due to the lack of accurate chromosomal markers. In some species, such as sorghum (Kim et al., 2002, 2005), silkworm (Yoshido et al., 2005), alpaca (Mendoza et al., 2020), and Senegalese sole (Merlo et al., 2021), all chromosomes could be identified by simultaneous FISH of a cocktail of landed large-insert DNA clones and/or repetitive DNA probes. In this study, we successfully distinguished all the 19 chromosomes of C. farreri through multiple two-color FISH and numbered them based on the chromosome-specific markers aided by the chromosomal morphology and lengths. They were arranged with the three metacentric chromosomes being chromosomes 1–3 from the longest one to the shortest one, following the five submetacentric chromosomes being chromosomes 4–8 from the longest one to the shortest one, and then all the subtelocentric chromosomes being chromosomes 9–19. In addition, our studies confirmed that this naming system could apply to the species with a large number of chromosomes as well as having difficulty in identifying individual chromosome using traditional karyotype and banding methods.
The chromosome assignment of large-insert DNA clones containing genetic markers provided bases not only for the chromosome identification but also for the integration of cytological and genetic linkage maps. Some species have been conducted multiple linkage maps, but it is still difficult to compare or integrate the different linkage groups due to the lack of a common nomenclature system for linkage groups. Actually, the problem could be solved by unifying the linkage groups and chromosomes, which could be accomplished by the chromosomal location of the large-insert DNA clones containing genetic markers (Mendes et al., 2020). In many plant species, the FISH mapping of BAC clones containing amplified fragment length polymorphism (AFLP) or microsatellite genetic markers accomplished the recognition of chromosomes as well as the integration of linkage groups and chromosomes (Kim et al., 2002, 2005; Tang et al., 2009; Yurkevich et al., 2021). Similar research was also reported in the scallop species of P. yessoensis, and the 19 LGs were successfully assigned to 19 pairs of chromosomes by SNP-anchored fosmid clones, which achieving chromosome recognition and integration of genetic and cytogenetic map (Yang et al., 2019). In addition, the distributions of microsatellite markers in some plant species were observed that they were specific and correlated with certain chromosomal organizations (e.g., euchromatin, heterochromatin, and centromeres) (Cuadrado et al., 2008). With the aid of chromosome-specific markers, we could locate more molecular markers on the chromosomes to understand chromosomal structures.
The integration of cytogenetic and genomic data had advanced our understanding of the genomic structure and evolution of the organism. In mandarin Citrus reticulata, the BAC-FISH of 18 BAC clones established correlations between mandarin scaffolds and chromosomes, allowing further structural genomic and comparative study with the sweet orange genome (Mendes et al., 2020). In Siberian sturgeon Acipenser baerii with polyploidy genome, a detailed chromosomal map consisted of starlet-specific repeats and chromosomal probes reconstructed the evolutionary reorganizations of 11 ancestral chromosomes after Ac1R and Ac2R (Biltueva et al., 2020). A cytogenetic map of C. farreri was constructed for the first time by FISH with probes of clones, such as microsatellite-anchored BAC clones, random BAC clones, repetitive DNA fosmid clones, genes-anchored BAC, and fosmid clones. All the mapped BAC and fosmid clones could be developed as sequence-tagged sites for further unification of the cytogenetic, physical, and genetic maps. The use of these markers is highly reproducible and does not require any specific stages of chromosome preparation which is required for traditional bandings. FISH with the chromosome-specific cytogenetic markers is the certain method for chromosome identification and construction of a cytogenetic map of C. farreri. In addition, the C. farreri genome contained numerous repetitive sequences which led to difficulties in genome assembly. The ability to identify the position of the repetitive sequence on the chromosomes of C. farreri would enable us to fill those gaps in the genome.
Conclusion
The cytogenetic mapping will facilitate accurate assembly of the genome and understanding the genome organization of C. farreri, and enable comparative cytogenetic research of the bivalve.
Data Availability Statement
The original contributions presented in the study are included in the article/Supplementary Material, further inquiries can be directed to the corresponding authors.
Author Contributions
LH, XH, and ZB conceived and designed the experiments. QX, QZ, and LW collected the samples. LH, LJ, and QX performed the experiments. LH, ZY, and XH analyzed the data. LH wrote the manuscript. All authors have read and approved the final manuscript.
Funding
This study was supported by the grants of the Chinese Ministry of Science and Technology through the National Key Research and Development Program of China (2018YFD0901402 and 2018YFD0900304), the Yantai Science and Technology Project (2020MSGY063), the China Agriculture Research System of MOF and MARA, and the Earmarked Fund for Agriculture Seed Improvement Project of the Shandong Province (2020LZGC016).
Conflict of Interest
The authors declare that the research was conducted in the absence of any commercial or financial relationships that could be construed as a potential conflict of interest.
Publisher's Note
All claims expressed in this article are solely those of the authors and do not necessarily represent those of their affiliated organizations, or those of the publisher, the editors and the reviewers. Any product that may be evaluated in this article, or claim that may be made by its manufacturer, is not guaranteed or endorsed by the publisher.
Supplementary Material
The Supplementary Material for this article can be found online at: https://www.frontiersin.org/articles/10.3389/fmars.2021.741230/full#supplementary-material
Supplementary Figures 1-1 and 1-2. Co-hybridization of the markers on different chromosomes. Red and green signals correspond to Dig- and biotin-labeled probes, respectively. Scale bars = 5 μm.
References
Biltueva, L. S., Prokopov, D. Y., Romanenko, S. A., Interesova, E. A., Schartl, M., and Trifonov, V. A. (2020). Chromosome distribution of highly conserved tandemly arranged repetitive DNAs in the Siberian Sturgeon (Acipenser baerii). Genes 11:1375. doi: 10.3390/genes11111375
Cheng, J. (2010). Construction and Application Analysis of Large Fragment Genomic Libraries of Zhikong Scallop (Chlamys farreri). Diss. Ocean University of China.
Cuadrado, A., Cardoso, M., and Jouve, N. (2008). Physical organisation of simple sequence repeats (SSRs) in Triticeae: structural, functional and evolutionary implications. Cytogenet. Genome Res. 120, 210–219. doi: 10.1159/000121069
Dong, G., Shen, J., Zhang, Q., Wang, J., Yu, Q., Ming, R., et al. (2018). Development and applications of chromosome-specific cytogenetic BAC-FISH probes in S. spontaneum. Front. Plant Sci. 9:218. doi: 10.3389/fpls.2018.00218
Eirín-López, J. M., González-Tizón, A. M., Martínez, A., and Méndez, J. (2002). Molecular and evolutionary analysis of mussel histone genes (mytilus spp): possible evidence of an “orphon origin” for H1 histone genes. J. Mol. Evol. 55, 272–283. doi: 10.1007/s00239-002-2325-1
Eirín-López, J. M., Ruiz, M. F., González-Tizón, A. M., Martínez, A., Sánchez, L., and Méndez, J. (2004). Molecular evolutionary characterization of the mussel mytilus histone multigene family: first record of a tandemly repeated unit of five histone genes containing an h1 subtype with “orphon” features. J. Mol. Evol. 58, 131–144. doi: 10.1007/s00239-003-2531-5
Feng, L., Hu, L., Fu, X., Liao, H., Li, X., Zhan, A., et al. (2014). An integrated genetic and cytogenetic map for Zhikong scallop, Chlamys farreri, based on microsatellite markers. PLoS ONE 9:e92567. doi: 10.1371/journal.pone.0092567
Gajardo, G., Parraguez, M., and Colihueque, N. (2002). Karyotype analysis and chromosome banding of the Chilean-Peruvian scallop Argopecten purpuratus (Lamarck, 1819). J. Shellfish Res. 21, 585–590.
Huan, P., Zhang, X., Li, F., Zhang, Y., Zhao, C., Liu, B., et al. (2009a). Chromosomal localization of heat shock protein 70 (HSP70) gene in Zhikong scallop Chlamys farreri using BAC-FISH. Mar. Sci. 33, 10–15. doi: 10.1016/S1874-8651(10)60079-8
Huan, P., Zhang, X., Li, F., Zhang, Y., Zhao, C., Liu, B., et al. (2009b). Chromosomal localization and development of SNP markers of a serine protease gene in Farrer's scallop (Chlamys farreri). Hereditas 31, 1241–1247. doi: 10.3724/SP.J.1005.2009.01241
Huang, C., Huan, P., Zhang, X., and Xiang, J. (2012). Chromosomal localization of NDPK gene in Zhikong scallop Chlamys farreri using BAC-FISH. Mar. Sci. 36, 1–5. Available online at: https://xueshu.baidu.com/usercenter/paper/show?paperid=9bd6e080843f2fdea7474c7bfb505fab
Huang, X., Bao, Z., Bi, K., Hu, J., Zhang, C., Zhang, Q., et al. (2006). Chromosomal localization of the major ribosomal RNA genes in scallop Chlamys farreri. Acta Oceanol. Sin. 25, 108–115. doi: 10.1016/j.marchem.2005.09.003
Huang, X., Hu, J., Hu, X., Zhang, C., Zhang, L., Wang, S., et al. (2007a). Cytogenetic characterization of the bay scallop, Argopecten irradians irradians, by multiple staining techniques and fluorescence in situ hybridization. Genes Genet. Syst. 82, 257–263. doi: 10.1266/ggs.82.257
Huang, X., Hu, X., Hu, J., Zhang, L., Wang, S., Lu, W., et al. (2007b). Mapping of ribosomal DNA and (TTAGGG)n telomeric sequence by FISH in the bivalve Patinopecten yessoensis. J. Mollus Stud. 73, 393–398. doi: 10.1093/mollus/eym036
Insua, A., López-Piñón, M. J., and Méndez, J. (1998). Characterization of Aequipecten opercularis (Bivalvia: Pectinidae) chromosomes by different staining techniques and fluorescent in situ hybridization. Genes Genet. Syst. 73, 193–200. doi: 10.1266/ggs.73.193
Kim, J. S., Childs, K. L., Islam-Faridi, M. N., Menz, M. A., Klein, R. R., Klein, P. E., et al. (2002). Integrated karyotyping of sorghum by in situ hybridization of landed BACs. Genome 45, 402–412. doi: 10.1139/g01-141
Kim, J. S., Klein, P. E., Klein, R. R., Price, H. J., Mullet, J. E., and Stelly, D. M. (2005). Chromosome identification and nomenclature of Sorghum bicolor. Genetics 169, 1169–1173. doi: 10.1534/genetics.104.035980
Lee, L. S., Navarro-Domínguez, B. M., Wu, Z., Montiel, E. E., Badenhorst, D., Bista, B., et al. (2020). Karyotypic evolution of sauropsid vertebrates illuminated by optical and physical mapping of the painted turtle and slider turtle genomes. Genes 11:928. doi: 10.3390/genes11080928
Lengerova, M., Kejnovsky, E., Hobza, R., Macas, J., Grant, S. R., and Vyskot, B. (2004). Multicolor FISH mapping of the dioecious model plant, Silene latifolia. Theor. Appl. Genet. 108, 1193–1199. doi: 10.1007/s00122-003-1568-6
Levan, A., Fredga, K., and Sandberg, A. A. (1964). Nomenclature for centromeric position on chromosomes. Hereditas 52, 201–220. doi: 10.1111/j.1601-5223.1964.tb01953.x
Li, X., Yang, Z., Liao, H., Zhang, Z., Huang, X., and Bao, Z. (2016). Chromosomal mapping of tandem repeats in the Yesso scallop, Patinopecten yessoensis (Jay, 1857), utilizing fluorescence in situ hybridization. Comp. Cytogenet. 10, 157–169. doi: 10.3897/CompCytogen.v10i1.7391
Li, Y., Sun, X., Hu, X., Xun, X., Zhang, J., Guo, X., et al. (2017). Scallop genome reveals molecular adaptations to semi-sessile life and neurotoxins. Nat. Commun. 8:1721. doi: 10.1038/s41467-017-01927-0
Mendes, S., Régis, T., Terol, J., Soares Filho, W. D. S., Talon, M., and Pedrosa-Harand, A. (2020). Integration of mandarin (Citrus reticulata) cytogenetic map with its genome sequence. Genome 63, 437–444. doi: 10.1139/gen-2020-0046
Mendoza, M. N., Raudsepp, T., More, M. J., Gutiérrez, G. A., and Ponce de León, F. A. (2020). Cytogenetic mapping of 35 new markers in the Alpaca (Vicugna pacos). Genes 11:522. doi: 10.3390/genes11050522
Merlo, M. A., Portela-Bens, S., Rodríguez, M. E., García-Angulo, A., Cross, I., Arias-Pérez, A., et al. (2021). A comprehensive integrated genetic map of the complete karyotype of Solea senegalensis (Kaup 1858). Genes 12:49. doi: 10.3390/genes12010049
Ocalewicz, K., Penman, D. J., and Babiak, I. (2008). Variation in size and location of the Ag-NOR in the Atlantic halibut (Hippoglossus hippoglossus). Genetica 133, 261–267. doi: 10.1007/s10709-007-9209-7
Pijnacker, L. P., and Ferwerda, M. A. (1984). Giemsa C-banding of potato chromosomes. Genome 26, 415–419. doi: 10.1139/g84-067
Sambrook, J., Fritsch, E. F., and Maniatis, T. (1989). Molecular Cloning: A Laboratory Manual. Cold Spring Harbor, NY: Cold Spring Harbor Laboratory Press.
Taboada, X., Pansonato-Alves, J. C., Foresti, F., Martínez, P., Viñas, A., Pardo, B. G., et al. (2014). Consolidation of the genetic and cytogenetic maps of turbot (Scophthalmus maximus) using FISH with BAC clones. Chromosoma 123, 281–291. doi: 10.1007/s00412-014-0452-2
Tang, X., de Boer, J. M., van Eck, H. J., Bachem, C., Visser, R. G., and de Jong, H. (2009). Assignment of genetic linkage maps to diploid Solanum tuberosum pachytene chromosomes by BAC-FISH technology. Chromosome Res. 17, 899–915. doi: 10.1007/s10577-009-9077-3
Wagenvoort, M., Rouwendal, G. J. A., and Kuiper-Groenwold, G., and Vries-van, Hulten. (1994). Chromosome identification in potato trisomics (2n=2x+1=25) by conventional staining, Giemsa C-banding and non-radioactive in situ hybridization. Cytologia 59, 405–417. doi: 10.1508/cytologia.59.405
Wai, C. M., Ming, R., Moore, P. H., Paull, R. E., and Yu, Q. (2010). Development of chromosome-specific cytogenetic markers and merging of linkage fragments in Papaya. Trop. Plant Biol. 3, 171–181. doi: 10.1007/s12042-010-9054-1
Wang, C. J. R., Harper, L., and Cande, W. Z. (2006). High-resolution single-copy gene fluorescence in situ hybridization and its use in the construction of a cytogenetic map of maize chromosome 9. Plant Cell 18, 529–544. doi: 10.2307/20076617
Wang, K., Guo, W., and Zhang, T. (2007). Development of one set of chromosome-specific microsatellite-containing BACs and their physical mapping in Gossypium hirsutum L. Theor. Appl. Genet. 115, 675–682. doi: 10.1007/s00122-007-0598-x
Wang, Y., and Guo, X. (2004). Chromosomal rearrangement in Pectinidae revealed by rRNA loci and implications for bivalve evolution. Biol. Bull. 207, 247–256. doi: 10.2307/1543213
Wang, Y., Xu, Z., and Guo, X. (2001). A centromeric satellite sequence in the Pacific oyster (Crassostrea gigas Thunberg) identified by fluorescence in situ hybridization. Mar. Biotechnol. 3, 486–492. doi: 10.1007/s10126-001-0063-3
Wang, Y., Xu, Z., Pierce, J. C., and Guo, X. (2005). Characterization of eastern oyster (Crassostrea virginica Gmelin) chromosomes by fluorescence in situ hybridization with bacteriophage P1 clones. Mar. Biotechnol. 7, 207–214. doi: 10.1007/s10126-004-0051-y
Xiong, Z., Kim, J. S., and Pires, J. C. (2010). Integration of genetic, physical, and cytogenetic maps for Brassica rapa chromosome A7. Cytogenet. Genome Res. 129, 190–198. doi: 10.1159/000314640
Xu, J., Ren, X., Bao, Z., Wang, S., Hu, L., and Huang, X. (2011). DAPI-banding and PI-banding of Zhikong scallop (Chlamys farreri). Periodical of Ocean University of China 4, 77–80. doi: 10.3724/SP.J.1011.2011.00415
Xu, J., and Shi, J. (2007). C banding and fluorescent banding pattern of the chromosomes of Cunninghamia lanceolate. Mol. Plant Breed. 4, 515–520.
Xu, Z., Guo, X., Gaffney, P. M., and Pierce, J. C. (2001). Chromosomal location of themajor ribosomal RNA genes in Crassostrea virginica and Crassostrea gigas. Veliger 44, 79–83. doi: 10.3901/JME.2001.02.079
Yang, Z., Li, X., Liao, H., Hu, L., Peng, C., Zhang, Z., et al. (2019). A molecular cytogenetic map of scallop (Patinopecten yessoensis). Mar. Biotechnol. 21, 731–742. doi: 10.1007/s10126-019-09918-6
Yang, Z., Li, X., Liao, H., Hu, L., Zhang, Z., Zhao, B., et al. (2016). Physical mapping of immune-related genes in Yesso scallop (Patinopecten yessoensis) using fluorescent in situ hybridization. Comp. Cytogenet. 10, 529–541. doi: 10.3897/CompCytogen.v10i4.10047
Yeh, B. P., and Peloquin, S. J. (1965). Pachytene chromosomes of the potato (Solanum tuberosum, Group Andigena). Am. J. Bot. 52, 1014–1020.
Yoshido, A., Bando, H., Yasukochi, Y., and Sahara, K. (2005). The Bombyx mori karyotype and the assignment of linkage groups. Genetics 170, 675–685. doi: 10.1534/genetics.104.040352
Yurkevich, O. Y., Samatadze, T. E., Selyutina, I. Y., Romashkina, S. I., Zoshchuk, S. A., Amosova, A. V., et al. (2021). Molecular cytogenetics of eurasian species of the genus Hedysarum L.(Fabaceae). Plants 10, 89. doi: 10.3390/plants10010089
Zhan, A., Hu, J., Hu, X., Hui, M., Wang, M., Peng, W., et al. (2009). Construction of microsatellite-based linkage maps and identification of size-related quantitative trait loci for Zhikong scallop (Chlamys farreri). Anim. Genet. 40, 821–831. doi: 10.1111/j.1365-2052.2009.01920.x
Zhang, L., Bao, Z., Cheng, J., Li, H., Huang, X., Wang, S., et al. (2007b). Fosmid library construction and initial analysis of end sequences in Zhikong scallop (Chlamys farreri). Mar. Biotechnol. 9, 606–612. doi: 10.1007/s10126-007-9014-4
Zhang, L., Bao, Z., Wang, S., Hu, X., and Hu, J. (2008a). FISH mapping and identification of Zhikong scallop (Chlamys farreri) chromosomes. Mar. Biotechnol. 10, 151–157. doi: 10.1007/s10126-007-9045-x
Zhang, L., Bao, Z., Wang, S., Huang, X., and Hu, J. (2007a). Chromosome rearrangements in Pectinidae (Bivalvia: Pteriomorphia) implied based on chromosomal localization of histone H3 gene in four scallops. Genetica 130, 193–198. doi: 10.1007/s10709-006-9006-8
Zhang, Q., Yu, G., Cooper, R. K., and Tiersch, T. R. (1999). Chromosomal location by fluorescence in situ hybridization of the 28S ribosomal RNA gene of the eastern oyster. J. Shellfish Res. 18, 431–435.
Keywords: Chlamys farreri, chromosome identification, cytogenetic map, FISH, BAC, fosmid
Citation: Hu L, Jiang L, Xing Q, Yang Z, Zhao Q, Wang L, Huang X and Bao Z (2021) Chromosome Identification and Cytogenetic Map Construction of Zhikong Scallop (Chlamys farreri) Based on Fluorescence in situ Hybridization. Front. Mar. Sci. 8:741230. doi: 10.3389/fmars.2021.741230
Received: 14 July 2021; Accepted: 09 August 2021;
Published: 09 September 2021.
Edited by:
Yuehuan Zhang, South China Sea Institute of Oceanology, Chinese Academy of Sciences (CAS), ChinaReviewed by:
Bin Xia, Qingdao Agricultural University, ChinaZhenming Lv, Zhejiang Ocean University, China
Copyright © 2021 Hu, Jiang, Xing, Yang, Zhao, Wang, Huang and Bao. This is an open-access article distributed under the terms of the Creative Commons Attribution License (CC BY). The use, distribution or reproduction in other forums is permitted, provided the original author(s) and the copyright owner(s) are credited and that the original publication in this journal is cited, in accordance with accepted academic practice. No use, distribution or reproduction is permitted which does not comply with these terms.
*Correspondence: Zujing Yang, yzj@ouc.edu.cn; Xiaoting Huang, xthuang@ouc.edu.cn