- 1School of Earth and Environmental Sciences, College of Natural Sciences, Seoul National University, Seoul, South Korea
- 2Research Institute of Oceanography, Seoul National University, Seoul, South Korea
The newly described dinoflagellate, Shimiella gracilenta, is known to survive for approximately 1 month on the plastids of ingested prey cells during starvation, indicating kleptoplastidy. To understand the population dynamics of this dinoflagellate in marine planktonic food webs, its growth and mortality rate due to predation should be assessed. Thus, we investigated the feeding occurrence of eight common heterotrophic protists on S. gracilenta. We also determined the growth and ingestion rates of Oxyrrhis marina and the naked ciliate, Rimostrombidium sp. on S. gracilenta as a function of the prey concentration. The common heterotrophic dinoflagellates (HTDs) Gyrodinium dominans, O. marina, and Pfiesteria piscicida and a naked ciliate Rimostrombidium sp. were able to feed on S. gracilenta; whereas the HTDs Aduncodinium glandula, Gyrodinium jinhaense, Oblea rotunda, and Polykrikos kofoidii were not. Shimiella gracilenta supported positive growth of O. marina and Rimostrombidium sp. but did not support that of G. dominans and P. piscicida. With increasing prey concentrations, the growth and ingestion rates of O. marina and Rimostrombidium sp. on S. gracilenta increased and became saturated. The maximum growth rates of O. marina and Rimostrombidium sp. on S. gracilenta were 0.645 and 0.903 day−1, respectively. Furthermore, the maximum ingestion rates of O. marina and Rimostrombidium sp. on S. gracilenta were 0.11 ng C predator day−1 (1.6 cells predator−1 day−1) and 35 ng C predator day−1 (500 cells predator−1 day−1), respectively. The maximum ingestion rate of O. marina on S. gracilenta was lower than that on any other algal prey reported to date, although its maximum growth rate was moderate. In conclusion, S. gracilenta had only a few common heterotrophic protist predators but could support moderate growth rates of the predators. Thus, S. gracilenta may not be a common prey species for diverse heterotrophic protists but may be a suitable prey for a few heterotrophic protists.
Introduction
Dinoflagellates are ubiquitous protists and one of the major components of marine ecosystems (Coats, 1999; Taylor et al., 2008; Jeong et al., 2010b). They play diverse ecological roles in marine food webs as primary producers, predators of diverse prey types (including bacteria, microalgae, and metazoans), prey for heterotrophic protists and metazoans (Shumway, 1990; Stoecker, 1999; Jeong et al., 2010b; Johnson, 2015; Mitra et al., 2016; Ward and Follows, 2016), symbiotic partners (Jeong et al., 2012; Lee et al., 2016; LaJeunesse et al., 2018), and parasites (Park et al., 2013b). Their diverse ecological roles in marine ecosystems may be related to their diverse trophic modes (i.e., autotrophy, heterotrophy, kleptoplastidy, and mixotrophy) (Jeong et al., 2010b; Lim et al., 2015). In particular, mixotrophic dinoflagellates perform photosynthesis using their own plastids, whereas kleptoplastidic dinoflagellates perform photosynthesis using plastids acquired from algal prey (Mitra et al., 2016; Ward and Follows, 2016; Jeong et al., 2021). Kleptoplastidic dinoflagellates can survive for certain long periods under starvation but eventually die if they do not subsequently feed on prey, unlike mixotrophic dinoflagellates (Gast et al., 2007; Mitra et al., 2016; Ok et al., 2021). Far less kleptoplastidic dinoflagellate species have been reported than mixotrophic dinoflagellate species; however, the number of studies describing new kleptoplastidic dinoflagellates is increasing (e.g., Jacobson and Anderson, 1986, 1996; Park et al., 2006, 2013a; Gast et al., 2007; Jeong et al., 2010a; Ok et al., 2021).
The dinoflagellate Shimiella gracilenta SGJH1904 that was isolated from the waters of Jinhae Bay, Korea, has been recently described (Ok et al., 2021). This dinoflagellate is known to survive without added prey for approximately 1 month on chloroplasts obtained from the prey, a cryptophyte called Teleaulax amphioxeia, indicating its “kleptoplastidy” (Ok et al., 2021). To understand the ecological roles of a newly described heterotrophic, kleptoplastic, or mixotrophic dinoflagellate, its prey and predators should be first identified (Potvin et al., 2013; Jeong et al., 2018a,b; Kang et al., 2019). Based on the results of the studies on the prey and predators, the transfer of elements from prey (i.e., the target dinoflagellate) to the predators in cycling of elements and food webs can be understood (Jeong et al., 2010b). To explore the ecological role of S. gracilenta as a predator, the kind of prey that S. gracilenta is able to feed on, growth and ingestion rates of S. gracilenta on prey, and grazing impact by S. gracilenta on prey populations should be assessed. On the other hand, to explore the ecological role of S. gracilenta as prey, the kind of predators that are able to feed on S. gracilenta, growth and ingestion rates of the predator on S. gracilenta, and predation impact by the predator on S. gracilenta populations should be assessed. Furthermore, to understand the population dynamics of the dinoflagellate, its growth rate (k) and mortality rate due to predation (g) under a given condition should be determined (Jeong et al., 2015). To determine the mortality rate due to predation, first, predators that are able to feed on the dinoflagellate prey should be identified (Berge et al., 2008; Lim et al., 2015). Second, the ingestion and growth rates of the predators on the dinoflagellate prey should be determined (Jeong et al., 2016; Anderson and Menden-Deuer, 2017). The ingestion rate of a predator on dinoflagellate prey is proportional to the clearance rate that, in turn, is proportional to g in bottle incubation experiments (Jeong et al., 2011; Kang et al., 2011). When data on the abundances of the target dinoflagellate and its co-occurring predator species in marine environments are available, the mortality rate due to predation by multiple predators can be estimated (Yoo et al., 2013a; Lim et al., 2017). Thus, revealing predators that are able to feed on S. gracilenta and determining the ingestion and growth rates of the predators on S. gracilenta are needed.
Typically, to identify predators of specific dinoflagellates, mixotrophic and/or heterotrophic organisms, which are abundant during or after the bloom of the target dinoflagellate (Yoo et al., 2013a; Lim et al., 2017; Eom et al., 2021), are selected as the potential predators. Alternatively, predators of the target dinoflagellate can be identified by observing the feeding behavior under a microscope after mixing the target dinoflagellate cells with potential predators commonly found in many marine environments (Yoo et al., 2010, 2015; Potvin et al., 2013; Ok et al., 2017; Kim et al., 2019). The heterotrophic dinoflagellates (HTDs) Gyrodinium dominans, Oblea rotunda, Oxyrrhis marina, Pfiesteria piscicida, and Polykrikos kofoidii and the naked ciliate Rimostrombidium sp. are commonly present in the waters of many countries (e.g., Costello et al., 2001; Jakobsen et al., 2002; Rhodes et al., 2006; Rublee et al., 2006; Sherr and Sherr, 2007; Felder and Camp, 2009; Agatha, 2011; Watts et al., 2011; Kilias et al., 2013; McCarthy, 2013). They have also been regarded as potential heterotrophic protist predators on many mixotrophic or kleptoplastidic dinoflagellates (Jacobson and Anderson, 1986, 1996; Jeong et al., 2001a, 2007a, 2017, 2018a,b; Ok et al., 2017). The newly described HTD, Gyrodinium jinhaense, is commonly found in Korean coastal waters (Lee et al., 2021). The HTD Aduncodinium glandula has been used as a potential predator in several studies (Jang et al., 2016; Jeong et al., 2018a). These heterotrophic protists have been reported to be present in the eastern South Sea of Korea, where S. gracilenta SGJH1904 was isolated (Jeong et al., 2006, 2011; Kang et al., 2015, 2019; Lim et al., 2017; Jung et al., 2021). Thus, S. gracilenta is likely to encounter these heterotrophic protists in natural environments. Moreover, these protists exhibit diverse sizes and shapes and adopt various feeding mechanisms, such as engulfment, peduncle, and pallium feeding.
In the present study, using the clonal cultures of S. gracilenta SGJH1904, we investigated the feeding occurrence of these potential heterotrophic protist predators on S. gracilenta and also determined the growth and ingestion rates of O. marina and Rimostrombidium sp. on S. gracilenta as a function of prey concentration. This was done because S. gracilenta supported the growth of only these two predators. Additionally, we compared the growth rate of O. marina feeding on S. gracilenta to that on other algal prey species. The results of this study can contribute to the understanding of interactions between S. gracilenta and common heterotrophic protists, as well as the understanding of ecological roles of S. gracilenta in marine planktonic food webs.
Materials and Methods
Preparation of Experimental Organisms
Shimiella gracilenta SGJH1904 cells were isolated from surface waters off the coast of Jinhae, Korea on April 6, 2019 (Ok et al., 2021). A clonal culture of S. gracilenta was established using two serial single cell isolations, and a culture of S. gracilenta was grown on T. amphioxeia (30,000–40,000 cells ml−1). Teleaulax amphioxeia was originally isolated from the coastal waters of Gomso Bay, Korea.
The HTDs A. glandula, G. dominans, G. jinhaense, O. rotunda, O. marina, and P. kofoidii, isolated from plankton samples collected from the coastal waters of Masan, Jeongok, Jinhae, Kunsan, and Jangheung during 2001–2019, were used in this study (Table 1). The clonal culture of P. piscicida was obtained from the National Center for Marine Algae and Microbiota (NCMA) in the USA. To isolate and cultivate the naked ciliate Rimostrombidium sp., plankton samples were collected from the coastal waters of Saemankeum, Korea, in July 2020 (Table 1).
Determination of Carbon Content and Cell Volume
The carbon contents of the potential predators and S. gracilenta were estimated based on cell volume (Menden-Deuer and Lessard, 2000). The cell volumes of A. glandula, G. jinhaense, G. dominans, O. rotunda, O. marina, P. kofoidii, P. piscicida, and Rimostrombidium sp. were estimated following the method described by Jang et al. (2016), Kang et al. (2020), Jeong et al. (2001b, 2007a, 2008), Ok et al. (2017), and Eom et al. (2021). The cell volume of S. gracilenta was also measured in the present study.
Determination of Feeding Capability
Experiment 1 was designed to examine whether the selected potential heterotrophic protists could feed on S. gracilenta after mixing the two species. Shimiella gracilenta cells were added to 42-ml polycarbonate (PC) bottles at a concentration of 15,000 cells ml−1. Next, each of the potential predators was added. A predator control bottle (no prey) and a prey control bottle (no predator) were also set up for each experiment. The initial concentration of each target heterotrophic protist was set up, considering its carbon contents or the maximum cell abundances as described in Supplementary Table 1. The bottles were placed on a plankton wheel rotating at 0.9 rpm (0.00017 × g) and incubated at 20°C under 20-μE m−2 s−1 illumination with cool white fluorescent light and a 14:10-h light-and-dark cycle.
After 1, 2, 6, 24, and 48 h of incubation, 5-ml aliquots were collected from each bottle using an autopipette and transferred into six-well plate chambers. When prey cells were not eaten by a target predator, >40 of the cells of the predator were observed under a dissecting microscope at a magnification of × 10–63 to determine the feeding occurrence of the potential predators on S. gracilenta. However, when a prey cell was eaten by a target predator, >15 cells of the predator were observed. The attack ratio (i.e., number of attempted captures relative to the number of physical contacts between a predator and prey) and capture success (i.e., number of prey ingested relative to the number of attempted captures) were determined by monitoring the behavior of the potential dinoflagellate predators in the presence of S. gracilenta. The ratios for Rimostrombidium sp. were not measured because this predator quickly jumped.
To explore the process of feeding, wells containing both a predator and S. gracilenta were placed under a light microscope (Zeiss-Axiovert 200M; Carl Zeiss Ltd., Göttingen, Germany), equipped with a video-analyzing system (Sony DXC-C33; Sony Co., Tokyo, Japan). The feeding process of the target species was recorded at a magnification of × 200–630. Cells of each target predator species and S. gracilenta were photographed on a confocal dish with cover glasses using a digital camera (Zeiss AxioCam 506; Carl Zeiss Ltd.), attached to a light and an epifluorescence microscope at a magnification of × 400–1,000.
Measurement of Growth and Ingestion Rates as a Function of Prey Concentration
In the preliminary test, S. gracilenta supported the positive growth rate of only two of the investigated potential predators, namely O. marina and Rimostrombidium sp. Thus, Experiments 2 and 3 were designed to determine the growth and ingestion rates of O. marina and Rimostrombidium sp. on S. gracilenta.
In Experiment 2, when prey cells were undetected under 1-ml Sedgewick–Rafter chamber (SRC), a dense culture of O. marina was transferred into a round 1-L PC bottle (Triforest® PC Centrifuge/Wide-mouth bottles) containing S. gracilenta (approximately 2,000 S. gracilenta cells ml−1 and 2,000 O. marina cells ml−1). The bottle was filled to the capacity with freshly filtered seawater to decrease the damage to the organisms, capped and placed on a plankton wheel rotating at 0.9 rpm (0.00017 × g). It was then incubated at 20°C under an illumination of 20 μE m−2 s−1 and placed in a 14:10-h light-and-dark cycle. Triplicate 1-ml aliquots were removed from the bottle every day, and cells of S. gracilenta and O. marina were counted under a light microscope to determine complete consumption of S. gracilenta and cell concentration and residual growth of O. marina (i.e., no difference in the growth rates of O. marina with and without added prey). After subsampling, the bottle was refilled with freshly filtered seawater and placed on the plankton wheel once again. The predetermined volumes of O. marina and S. gracilenta were transferred to PC bottles using an autopipette (Supplementary Table 1). Triplicate 42-ml PC experimental bottles (containing mixtures of predators and prey) and triplicate control bottles (containing only prey) were set up for each predator–prey combination. Triplicate control bottles containing only predators were also set up at a single predator concentration. Cultures of predator cells were passed through a 0.2-μm disposable syringe filter (DISMIC-25CS type, 25 mm; Advantec, Toyo Roshi Kaisha Ltd., Chiba, Japan), and then the filtrates were added to the prey control bottles as the same volume as that of the predator culture added to the experimental bottles for each predator—prey combination to ensure similar water conditions. All the bottles were then filled to capacity with freshly filtered seawater and capped. At the start of the experiment, a 5-ml aliquot was collected from each bottle, fixed in 5% Lugol's solution, and enumerated under a light microscope to determine the actual predator and prey abundances. The bottles were refilled to the capacity with freshly filtered seawater and placed on rotating wheels under the conditions described above. Dilution of the cultures by refilling the bottles was considered when calculating the growth and ingestion rates. After 48 h of incubation, a 10-ml aliquot was collected from each bottle and fixed in 5% Lugol's solution. The predator and prey abundance sampled at 0 and 48 h after setting up the experiment was determined by counting all (or over 200) cells using 1-ml SRC in triplicate.
In Experiment 3, when the prey cells were completely consumed, a dense culture of Rimostrombidium sp. was transferred to a 500-ml PC bottle containing S. gracilenta (approximately 600 S. gracilenta cells ml−1 and 20 Rimostrombidium sp. cells ml−1), and the bottle was filled with filtered seawater. The bottles were incubated under the same conditions as described above. Every 12 h of incubation, triplicate 1-ml aliquots were collected from the bottle, and the cell concentration and residual growth of Rimostrombidium sp. were determined under a light microscope. The initial concentration of Rimostrombidium sp. and S. gracilenta was established using an autopipette to deliver predetermined volumes of known cell concentrations to the bottles (Supplementary Table 1). The experiment was conducted using the methodology described above. After 24 h of incubation, a 10-ml aliquot was collected from each bottle, fixed in 5% Lugol's solution, and enumerated under an inverted microscope (BX51, Olympus, Japan). Triplicate 10-ml aliquots were collected from the bottles with the three lowest concentrations and all predator control bottles and enumerated in six-well plate chambers under a dissecting microscope (SZX2-ILLB, Olympus, Japan) without fixation.
The specific growth rate of each heterotrophic protist predator, μ (day−1), was calculated as follows:
where P0 and Pt are the concentrations of predators at 0 and 24 h for Rimostrombidium sp. or at 0 and 48 h for O. marina, respectively. Rimostrombidium sp. depleted S. gracilenta cells in <48 h, and, thus, was incubated for 24 h.
The calculated growth rate was fitted to the Michaelis–Menten equation as follows:
where μmax is the maximum growth rate (day−1); x is the prey concentration (cells ml−1 or ng C ml−1); x′ is the threshold prey concentration (prey concentration where μ = 0); and KGR is the prey concentration sustaining 1/2 μmax.
Data were iteratively fitted to the model using Delta Graph (IBM-SPSS Inc., Armonk, NY, USA).
The ingestion and clearance rates were calculated using the modified equations described by Frost (1972) and Heinbokel (1978). The incubation time for calculating the ingestion and clearance rates was the same as that for estimating the growth rate. The calculated ingestion rate (IR; cells predator−1 day−1) of each heterotrophic protist was fitted to the Michaelis–Menten equation as follows:
where Imax is the maximum ingestion rate (cells predator−1 day−1 or ng C predator−1 day−1); x is the prey concentration (cells ml−1 or ng C ml−1); and KIR is the prey concentration sustaining 1/2 Imax.
Measurement of Swimming Speed
A culture of S. gracilenta with approximately 40,000 cells ml−1 growing on T. amphioxeia was transferred into a 1-L PC bottle. To determine the presence of any prey cells in the S. gracilenta culture, a 5 ml aliquot was removed from the bottle, fixed with 5% Lugol's solution, and examined under a light microscope. When no prey cells were detected, an aliquot of S. gracilenta culture was transferred to a 38-ml cell culture flask (BD Biosciences, USA) and allowed to acclimate for 30 min to avoid the interference of water flow. A video camera was focused on a single frame on the cell culture flask under a stereomicroscope (SZX2-ILLK, Olympus, Japan) at 20°C. Swimming of S. gracilenta cells was recorded at a magnification of × 25 using a video-analyzing system (SRD-1673 DN, Samsung, Korea) and a CCD camera (DXC-C33, SONY, Japan). The speed of all swimming cells viewed within 10–70 min was measured, and the average swimming speed (n = 30) was calculated based on the straight line of the cells during single-frame playback.
Statistical Analysis
Pearson's correlation analysis was used to examine the association among the variables [i.e., equivalent spherical diameter (ESD) of prey species, μmax and Imax of O. marina feeding on dinoflagellate prey species, maximum swimming speed (MSS) of prey species, and μmax and Imax of O. marina feeding on dinoflagellate prey species]. Statistical analyses were performed using SPSS ver. 25.0 (IBM-SPSS Inc., Armonk, NY, USA).
Results
Feeding Occurrence by Heterotrophic Protists on Shimiella gracilenta
The HTDs O. marina, P. piscicida, and G. dominans, as well as the naked ciliate Rimostrombidium sp., were able to feed on S. gracilenta SGJH1904 (Table 2; Supplementary Figures 1, 2; Supplementary Video 1). Cells of O. marina, G. dominans, and Rimostrombidium sp. engulfed prey cells, while P. piscicida cells fed on prey cells using a peduncle (Supplementary Figures 1, 2). The attack ratios of G. dominans, P. piscicida, and O. marina to S. gracilenta were 6, 33, and 14%, respectively (Table 2). Once these predators attacked S. gracilenta, they successfully fed on S. gracilenta (i.e., 100%).
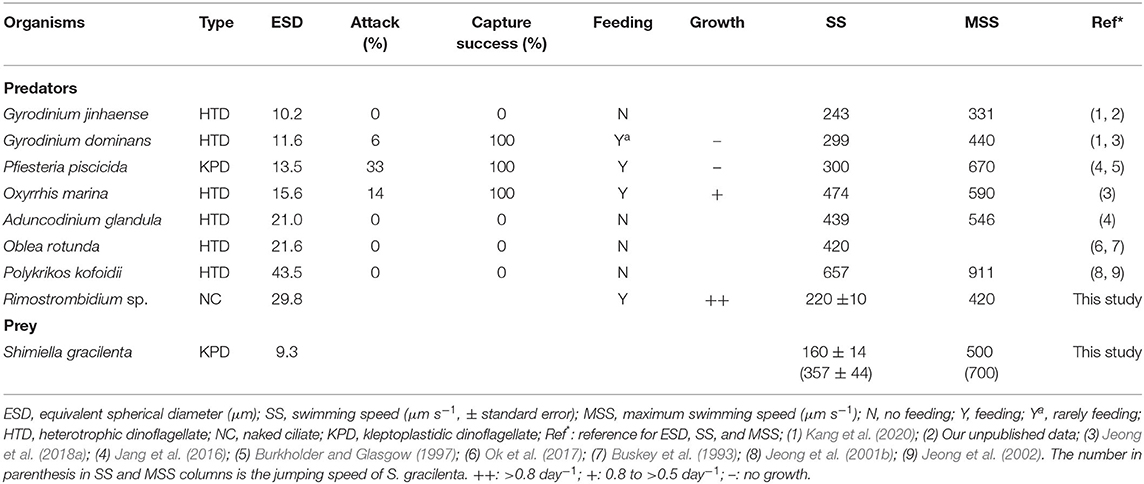
Table 2. Feeding capability and comparison of the swimming speeds of predator species and Shimiella gracilenta.
The HTDs A. glandula, G. jinhaense, O. rotunda, and P. kofoidii did not feed on S. gracilenta (Table 2; Supplementary Figure 3). Cells of A. glandula, G. jinhaense, O. rotunda, and P. kofoidii did not attack S. gracilenta cells. Furthermore, S. gracilenta cells were observed quickly jumping backward when A. glandula, G. jinhaense, O. rotunda, and P. kofoidii touched them. However, it was observed that O. marina spun very quickly around an S. gracilenta cell and engulfed the prey cell (Supplementary Video 1). Cells of Rimostrombidium sp. made feeding currents to draw prey cells and intercepted prey cells inside the feeding currents. Often, several cells of P. piscicida attacked an S. gracilenta cell together.
Growth Rates of Rimostrombidium sp. and Oxyrrhis marina Feeding on Shimiella gracilenta as a Function of Prey Concentration
With increasing mean prey concentrations, the specific growth rate of Rimostrombidium sp. feeding on S. gracilenta increased at mean prey concentrations ≤204 ng C ml−1 (2,919 cells ml−1), but this became saturated at higher mean prey concentrations (Figure 1A). When the data were fitted to Equation (2), the calculated maximum growth rate (μmax) of Rimostrombidium sp. feeding on S. gracilenta was 0.903 day−1.
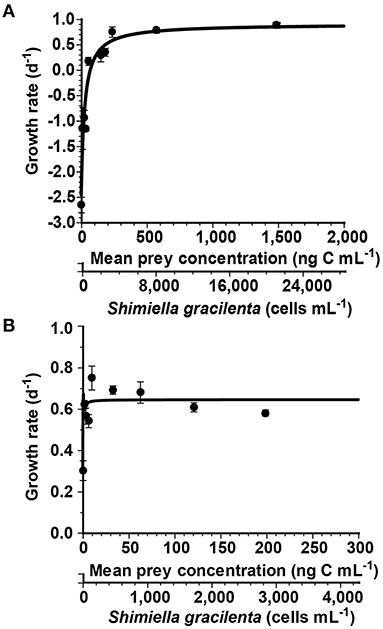
Figure 1. Growth rates of Rimostrombidium sp. (A) and Oxyrrhis marina (B) feeding on Shimiella gracilenta as a function of mean prey concentration (x). Symbols represent treatment means ± standard error (SE). The curves are fitted by the Michaelis–Menten equation (Equation 2), using all treatments in the experiment. (A) The growth rate (day−1) = 0.90 {(x – 69.1)/[94.5 + (x – 69.1)]}, r2 = 0.89. (B) The growth rate (day−1) = 0.65 {(x + 0.12)/[0.14 + (x + 0.12)]}, r2 = 0.63.
The specific growth rate of O. marina feeding on S. gracilenta rapidly increased with the increase of mean prey concentrations, with the highest growth rate at a mean prey concentration of 9.4 ng C ml−1 (134 cells ml−1; Figure 1B). However, this slightly decreased at higher mean prey concentrations. When the data were fitted to Equation (2), the calculated μmax of O. marina on S. gracilenta was 0.645 day−1.
Ingestion Rates of Rimostrombidium sp. and Oxyrrhis marina Feeding on Shimiella gracilenta as a Function of Prey Concentration
With increasing mean prey concentrations, the ingestion rate of Rimostrombidium sp. feeding on S. gracilenta rapidly increased at mean prey concentrations ≤204 ng C ml−1 (2,919 cells ml−1) but slowly increased at higher mean prey concentrations (Figure 2A). When the data were fitted to Equation (3), the calculated maximum ingestion rate (Imax) of Rimostrombidium sp. feeding on S. gracilenta was 35 ng C predator−1day−1 (500 cells predator−1 day−1).
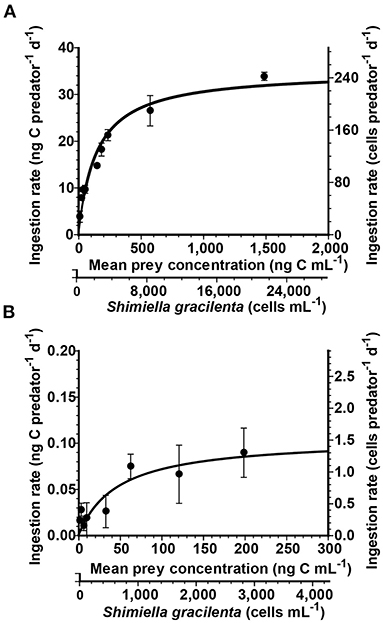
Figure 2. Ingestion rates of Rimostrombidium sp. (A) and Oxyrrhis marina (B) feeding on Shimiella gracilenta as a function of mean prey concentration (x). Symbols represent treatment means ± standard error (SE). The curves are fitted by the Michaelis–Menten equation (Equation 3), using all treatments in the experiment. (A) The ingestion rate (ng C predator−1 day−1) = 35 [x/(146 + x)], r2 = 0.92. (B) The ingestion rate (ng C predator−1 day−1) = 0.11 [x/(47.1 + x)], r2 = 0.46.
With increasing mean prey concentrations, the ingestion rate of O. marina feeding on S. gracilenta increased at mean prey concentrations ≤62.3 ng C ml−1 (890 cells ml−1) but became almost saturated at higher mean prey concentrations (Figure 2B). When the data were fitted to Equation (3), the calculated Imax of O. marina on S. gracilenta was 0.11 ng C predator−1 day−1 (1.6 cells predator−1 day−1).
Swimming Speed
The average (± SE, n = 30) and maximum swimming speeds of S. gracilenta under experiment conditions were 160 (± 14) and 500 μm s−1, respectively, with the maximum jumping speed of 700 μm s−1 (Table 2).
Discussion
Feeding Ability of the Predators
Among the eight common heterotrophic protists tested in the present study, four species were able to feed on S. gracilenta SGJH1904, whereas the other four species did not feed at all. The feeding mechanism, behavior, size, and/or shape of protist predators, as well as the biochemistry, behavior, size, and/or shape of the prey, have been known to affect the feeding abilities of predators (Scharf et al., 2000; Strom et al., 2003; Evans and Wilson, 2008; Jeong et al., 2010b; Roberts et al., 2011). The engulfment feeders G. dominans, O. marina, and Rimostrombidium sp. ingested S. gracilenta, whereas other engulfment feeders, G. jinhaense and P. kofoidii, did not feed on the prey species. Furthermore, the peduncle feeder P. piscicida fed on S. gracilenta, whereas another peduncle feeder, A. glandula, did not feed on the prey species. Therefore, feeding mechanisms are likely not to affect the feeding occurrence of these heterotrophic protists on S. gracilenta. Meanwhile, among the protists G. dominans, P. piscicida, O. marina, and Rimostrombidium sp., which were able to feed on S. gracilenta, the sizes were larger than those of G. jinhaense, which was not able to feed on S. gracilenta but smaller than that of A. glandula, O. rotunda, and P. kofoidii, which were not able to feed on the prey (Table 2). Therefore, the predator size may not affect the feeding occurrence of these heterotrophic protists on S. gracilenta.
Although G. jinhaense and G. dominans are included in the same genus and have similar ESD values, G. dominans is known to feed on Mesodinium rubrum, which has jumping behavior, whereas G. jinhaense does not (Lee et al., 2014b; Kang et al., 2020). Thus, the rapid jumping behavior of S. gracilenta may be an effective anti-predation tool against G. jinhaense, but not against G. dominans. Moreover, the maximum swimming speed of G. dominans (440 μm s−1) is considerably greater than that of G. jinhaense (331 μm s−1, Table 2). Therefore, the swimming speed of these two Gyrodinium species may affect this differential feeding occurrence on S. gracilenta.
Cells of P. kofoidii are known to deploy a nematocyst-taeniocyst complex to capture a prey cell (Matsuoka et al., 2000; Tillmann and Hoppenrath, 2013). However, cells of P. kofoidii were not observed deploying a nematocyst-taeniocyst complex on the surface of an S. gracilenta cell. The minimum prey size that P. kofoidii was able to feed on is known to be approximately 10 μm (Jeong et al., 2001b). Therefore, P. kofoidii may have difficulty deploying a nematocyst-taeniocyst complex on the surface of a small-sized S. gracilenta cell.
The pallium feeder, O. rotunda, was able to feed on smaller prey than S. gracilenta (Strom and Buskey, 1993). Ok et al. (2021) reported that many trichocysts were observed inside the cytoplasm of S. gracilenta. It is known that trichocysts of Fibrocapsa japonica may substantially complicate the capturing process involving the tow filament of O. rotunda (Tillmann and Reckermann, 2002). Thus, trichocysts in S. gracilenta may act mechanically as a grazer deterrent to O. rotunda.
Growth and Ingestion Rates of the Predators
Both the growth and ingestion rates of O. marina and Rimostrombidium sp. on S. gracilenta are affected by prey concentrations (Figures 1, 2). The half-saturation constant for the growth (KGR) of O. marina feeding on S. gracilenta (0.14 ng C ml−1; 2 cells ml−1) was lower than that of Rimostrombidium sp. (94.5 ng C ml−1; 1,350 cells ml−1). Additionally, the half-saturation constant for the ingestion (KIR) of O. marina (47.1 ng C ml−1; 673 cells ml−1) was also lower than that of Rimostrombidium sp. (146 ng C ml−1; 2,086 cells ml−1). Thus, at low prey concentrations, the growth and ingestion rates of O. marina would respond more readily to changes in prey concentrations than those of Rimostrombidium sp. Until now, the maximum cell abundance of S. gracilenta in natural environments has been reported as 29.9 cells ml−1 (Back et al., 2021). Without considering spatial dependence and other physical effects, O. marina may grow under this S. gracilenta concentration, while Rimostrombidium sp. may not.
The threshold concentration of S. gracilenta for the growth of Rimostrombidium sp. was 69.1 ng C ml−1 (987 cells ml−1). At this prey concentration, the ingestion rate of Rimostrombidium sp. on S. gracilenta was 11.2 ng C predator−1 day−1 (160 cells ml−1). Thus, this ingestion rate corresponds to the threshold prey concentration for basic maintenance in the abundance of Rimostrombidium sp. Meanwhile, the growth rate of O. marina without added S. gracilenta cells (i.e., predator control) was positive. Oxyrrhis marina is known to feed on bacteria and grow on dissolved nutrients (Jeong et al., 2008; Lowe et al., 2011). Thus, this physiological characteristic of O. marina may be the reason why it had a positive growth without added S. gracilenta cells in this study.
There have been three kleptoplastidic dinoflagellates whose predators were identified and on which the growth and ingestion rates of the predators were determined; Gymnodinium smaydae, P. piscicida, and S. gracilenta (Supplementary Table 2; Jeong et al., 2007a, 2018b). These three kleptoplastidic dinoflagellates were ingested by O. marina. The ranges of μmax and Imax of O. marina on the three dinoflagellates were 0.41–0.66 day−1 and 0.11–0.33 ng C predator−1 day−1, respectively (Supplementary Table 2). These ranges of μmax and Imax of O. marina on kleptoplastic dinoflagellates showed no distinct values compared with those of O. marina on phototrophic and heterotrophic dinoflagellates (0.16–1.20 day−1 and 0.07–6.36 ng C predator−1 day−1, respectively). Moreover, the KGR of O. marina feeding on G. smaydae and S. gracilenta (1.4 and 0.14 ng C ml−1, respectively) showed relatively low values, while that on P. piscicida (21 ng C ml−1) was much higher (Jeong et al., 2007a, 2018b). Thus, the nutritional properties of each kleptoplastidic dinoflagellate as prey for a predator species may vary from species to species. More studies are needed to understand the nutritional values of kleptoplastidic dinoflagellates as prey in marine ecosystems.
Neither the Imax nor the μmax of O. marina on target prey species was significantly correlated with prey size (>0.05, Pearson's correlation analysis; Figures 3A,B), and the μmax was also not significantly correlated with the Imax (>0.05; Figure 3C). However, the maximum swimming speed (MSS) of the prey species was significantly and negatively correlated with the μmax of O. marina on prey species (<0.05), but it was not correlated with the Imax (>0.05; Figure 4). The higher the MSS of prey species, the more the predator O. marina may spend energy in catching the prey cells. Thus, the growth rates of O. marina are likely to be affected by the MSS of prey species. Among the reported dinoflagellate prey species of O. marina having the size of 9–10 μm, the μmax of O. marina on S. gracilenta was higher than those on A. cf. poporum, B. adriatica, G. smaydae, and a toxic strain of K. veneficum CCMP2064. Therefore, O. marina may spend less energy in capturing, ingesting, and digesting S. gracilenta than A. cf. poporum, B. adriatica, and G. smaydae. Among the 16 dinoflagellate prey species of O. marina, the Imax of O. marina on S. gracilenta was lower than that on the other prey species, except for Yihiella yeosuensis and Luciella masanensis (Supplementary Table 2). The fast swimming speed and quick jumping behavior of Y. yeosuensis might be responsible for the lack of predation (Jeong et al., 2018a). Therefore, the quick jumping behavior of S. gracilenta may lower the Imax of O. marina on itself. However, the jumping speed of S. gracilenta is lower than that of Y. yeosuensis, and this may partially be responsible for the higher Imax of O. marina on S. gracilenta. Cells of L. masanensis do not have the quick jumping behavior, but it was suggested that they excrete chemicals to protect themselves from predation (Jeong et al., 2007a).
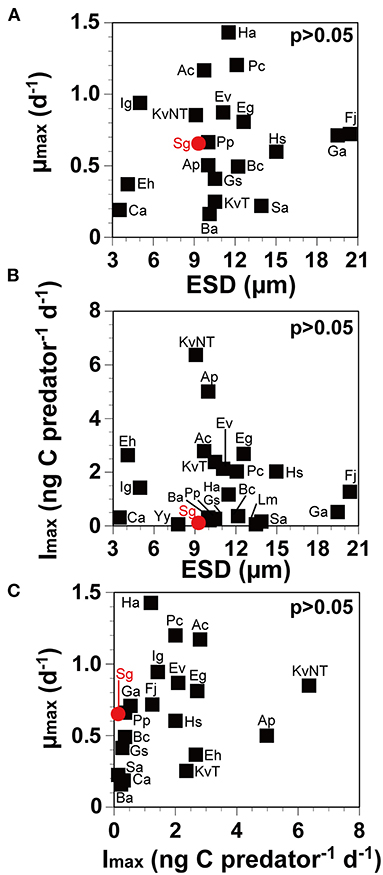
Figure 3. The maximum growth rate (μmax) and the ingestion rate (Imax) of Oxyrrhis marina on prey as a function of prey size (ESD, equivalent spherical diameter; μm) and μmax as a function of Imax, as in Supplementary Table 2, respectively. The μmax (A; r = 0.15, p = 0.53) and Imax (B; r = 0.13, p = 0.57) of Oxyrrhis marina on prey (black square) and Shimiella gracilenta (red circle), as a function of prey size (ESD). (C; r = 0.26, p = 0.27). The μmax as a function of Imax. Ac, Amphidinium carterae; Ap, Azadinium cf. poporum; Ba, Biecheleriopsis adriatica; Bc, Biecheleria cincta; Ca, Cafeteria sp.; Eh, Emiliania huxleyi; Eg, Eutreptiella gymnastica; Ev, Effrenium voratum; Fj, Fibrocapsa japonica; Ga, Gymnodinium aureolum; Gs, Gymnodinium smaydae; Ha, Heterosigma akashiwo; Hs, Heterocapsa steinii; Ig, Isochrysis galbana; KvNT, Karlodinium veneficum (non-toxic; MD5); KvT, K. veneficum (toxic; CCMP2064); Lm, Luciella masanensis; Pc, Prorocentrum cordatum; Pp, Pfiesteria piscicida; Sa, Stoeckeria algicida; Sg, Shimiella gracilenta; Yy, Yihiella yeosuensis. Data were obtained from Lee (1998), Jeong et al. (2001a, 2003, 2007a,b, 2011, 2014, 2018a,b), Tillmann and Reckermann (2002), Strom et al. (2003), Kimmance et al. (2006), Adolf et al. (2007), Yoo et al. (2010, 2013b,c), Potvin et al. (2013), Anderson and Menden-Deuer (2017), and Kang et al. (2019).
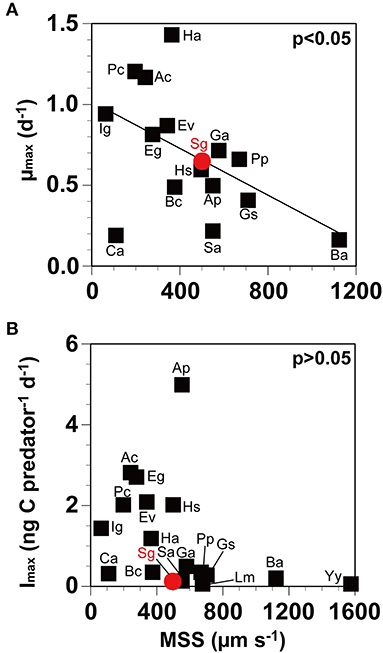
Figure 4. The maximum growth rate (μmax) and the ingestion rate (Imax) of Oxyrrhis marina on prey as a function of maximum swimming speed (MSS), as described in Supplementary Table 2. The μmax (A; r = −0.51, p = 0.04) and the Imax (B; r = −0.37, p = 0.13) of Oxyrrhis marina on prey (black square) and Shimiella gracilenta (red circle), as a function of MSS. Ac, Amphidinium carterae; Ap, Azadinium cf. poporum; Ba, Biecheleriopsis adriatica; Bc, Biecheleria cincta; Ca, Cafeteria sp.; Eg, Eutreptiella gymnastica; Ev, Effrenium voratum; Ga, Gymnodinium aureolum; Gs, Gymnodinium smaydae; Ha, Heterosigma akashiwo; Hs, Heterocapsa steinii; Ig, Isochrysis galbana; Lm, Luciella masanensis; Pc, Prorocentrum cordatum; Pp, Pfiesteria piscicida; Sa, Stoeckeria algicida; Sg, Shimiella gracilenta; Yy, Yihiella yeosuensis. Data were obtained from Throndsen (1973), Kamykowski and McCollum (1986), Lee (1998), Jeong et al. (1999, 2001a, 2002, 2003, 2007a,b, 2010a, 2011, 2014, 2018a,b), Kimmance et al. (2006), Yoo et al. (2010, 2013b,c), Kang et al. (2011, 2019), Potvin et al. (2013), Lee et al. (2014a), Jang et al. (2015, 2016, 2017), Anderson and Menden-Deuer (2017), and Lim et al. (2018).
This study clearly showed that S. gracilenta supported the positive growth of only O. marina and Rimostrombidium sp. among the potential predator species tested. Notably, these two predators have cosmopolitan distributions (Agatha, 2011; Watts et al., 2011). Shimiella gracilenta has been found in the regions where O. marina and Rimostrombidium sp. have been found: the east coast of the United States (Campbell, 1973; Johnson et al., 2003; Tucker et al., 2017), the Baltic Sea (Pedersen and Hansen, 2003; Hällfors, 2004; Watts et al., 2011), and Jinhae Bay, Korea (Choi et al., 2016; Yoon, 2018; Ok et al., 2021). Therefore, O. marina and Rimostrombidium sp. may grow on S. gracilenta if both predators and prey coexist in the regions.
To investigate the impact of predation by O. marina or Rimostrombidium sp. on the populations of S. gracilenta, ingestion rates of O. marina or Rimostrombidium sp. on S. gracilenta and their abundances should be quantified. The results of the present study provided the ingestion rates of O. marina and Rimostrombidium sp. on S. gracilenta as a function of the prey concentration. Thus, if the abundances of S. gracilenta and co-occurring O. marina or Rimostrombidium sp. are available, the predation impact by O. marina or Rimostrombidium sp. on the populations of S. gracilenta can be estimated at any prey concentration. Depending on the degree of predation impact by O. marina or Rimostrombidium sp. on the populations of S. gracilenta, the ecological roles of S. gracilenta as prey for the predator can be evaluated. Moreover, the results of the present study provide the threshold S. gracilenta concentration for the growth of O. marina or Rimostrombidium sp. and also, growth rates of the predators as a function of the prey concentration. Thus, the ecological roles of S. gracilenta as prey for O. marina or Rimostrombidium sp. can be determined. This study suggested that S. gracilenta may have an advantage for survival due to only a few common heterotrophic protist predators and low ingestion rates of the predators in marine ecosystems.
Data Availability Statement
The original contributions presented in the study are included in the article/Supplementary Material, further inquiries can be directed to the corresponding author/s.
Author Contributions
SP and HJ designed the study conception and drafted the manuscript. SP, HJ, JO, HK, JY, SE, and EP obtained the data and conducted the experiments. SP, HJ, and JO performed data analyses. All authors contributed to the article and approved the submitted version.
Funding
This research was supported by the Useful Dinoflagellate program of Korea Institute of Marine Science and Technology Promotion (KIMST) funded by the Ministry of Oceans and Fisheries (MOF) and the National Research Foundation (NRF) funded by the Ministry of Science and ICT (NRF-2020M3F6A1110582 and NRF-2021M3I6A1091272) awarded to HJ.
Conflict of Interest
The authors declare that the research was conducted in the absence of any commercial or financial relationships that could be construed as a potential conflict of interest.
Publisher's Note
All claims expressed in this article are solely those of the authors and do not necessarily represent those of their affiliated organizations, or those of the publisher, the editors and the reviewers. Any product that may be evaluated in this article, or claim that may be made by its manufacturer, is not guaranteed or endorsed by the publisher.
Supplementary Material
The Supplementary Material for this article can be found online at: https://www.frontiersin.org/articles/10.3389/fmars.2021.738547/full#supplementary-material
References
Adolf, J. E., Krupatkina, D., Bachvaroff, T., and Place, A. R. (2007). Karlotoxin mediates grazing by Oxyrrhis marina on strains of Karlodinium veneficum. Harmful Algae 6, 400–412. doi: 10.1016/j.hal.2006.12.003
Agatha, S. (2011). Global diversity of aloricate Oligotrichea (Protista, Ciliophora, Spirotricha) in marine and brackish sea water. PLoS ONE 6:e22466. doi: 10.1371/journal.pone.0022466
Anderson, S. R., and Menden-Deuer, S. (2017). Growth, grazing, and starvation survival in three heterotrophic dinoflagellate species. J. Eukaryot. Microbiol. 64, 213–225. doi: 10.1111/jeu.12353
Back, D. Y., Ha, S. Y., Else, B., Hanson, M., Jones, S. F., Shin, K. H., et al. (2021). On the impact of wastewater effluent on phytoplankton in the Arctic coastal zone: a case study in the Kitikmeot Sea of the Canadian Arctic. Sci. Total Environ. 764:143861. doi: 10.1016/j.scitotenv.2020.143861
Berge, T., Hansen, P. J., and Moestrup, Ø. (2008). Feeding mechanism, prey specificity and growth in light and dark of the plastidic dinoflagellate Karlodinium armiger. Aquat. Microb. Ecol. 50, 279–288. doi: 10.3354/ame01165
Burkholder, J. M., and Glasgow, H. B. Jr. (1997). Pfiesteria piscicida and other Pfiesreria-like dinoflagellates: behavior, impacts, and environmental controls. Limnol. Oceanogr. 42, 1052–1075. doi: 10.4319/lo.1997.42.5_part_2.1052
Buskey, E. J., Coulter, C., and Strom, S. (1993). Locomotory patterns of microzooplankton: potential effects on food selectivity of larval fish. Bull. Mar. Sci. 53, 29–43.
Campbell, P. H. (1973). The Phytoplankton of Gales Creek With Emphasis on the Taxonomy and Ecology of Estuarine Phytoflagellates. Thesis, University of North Carolina, Chapel Hill, NC. p. 354.
Choi, J. K., Xu, K., Lei, Y., Gong, J., Kim, S. Y., and Cho, B. C. (2016). 24. “Other marine ciliates,” in Protists of Korea Vol. 3, ed Korean Society of Protistologists (Seoul). p. 77.
Coats, D. W. (1999). Parasitic life styles of marine dinoflagellates 1. J. Eukaryot. Microbiol. 46, 402–409. doi: 10.1111/j.1550-7408.1999.tb04620.x
Costello, M. J., Emblow, C. S., and White, R., (eds.). (2001). European Register of Marine Species. A Check-list of the Marine Species in Europe and a Bibliography of Guides to their Identification. Patrimoines naturels, Vol. 50 Paris:. Publications Scientifiques du Muséum National d'Histoire Naturelle. p. 55.
Eom, S. H., Jeong, H. J., Ok, J. H., Park, S. A., Kang, H. C., You, J. H., et al. (2021). Interactions between common heterotrophic protists and the dinoflagellate Tripos furca: implication on the long duration of its red tides in the South Sea of Korea in 2020. Algae 36, 25–36. doi: 10.4490/algae.2021.36.2.22
Evans, C., and Wilson, W. H. (2008). Preferential grazing of Oxyrrhis marina on virus infected Emiliania huxleyi. Limnol. Oceanogr. 53, 2035–2040. doi: 10.4319/lo.2008.53.5.2035
Felder, D. L., and Camp, D. K. (2009). “Gulf of Mexico–origins, waters, and biota,” in Biodiversity, Vol. 1, eds J. W. Tunnell Jr, D. L. Felder, and S. A. Earle (College Station, TX: Texas A&M Press), 140.
Frost, B. W. (1972). Effects of size and concentration of food particles on the feeding behavior of the marine planktonic copepod Calanus pacificus. Limnol. Oceanogr. 17, 805–815. doi: 10.4319/lo.1972.17.6.0805
Gast, R. J., Moran, D. M., Dennett, M. R., and Caron, D. A. (2007). Kleptoplasty in an Antarctic dinoflagellate: caught in evolutionary transition? Environ. Microbiol. 9, 39–45. doi: 10.1111/j.1462-2920.2006.01109.x
Hällfors, G. (2004). Checklist of Baltic Sea Phytoplankton Species. Baltic Sea Environment Proceedings No. 95. Helsinki Commission, Baltic Marine Protection Commission, p. 208.
Heinbokel, J. F. (1978). Studies on the functional role of tintinnids in the Southern California Bight. I. Grazing and growth rates in laboratory cultures. Mar. Biol. 47, 177–189. doi: 10.1007/BF00395638
Jacobson, D. M., and Anderson, D. M. (1986). Thecate heterophic dinoflagellates: feeding behavior and mechanisms. J. Phycol., 22, 249–258. doi: 10.1111/j.1529-8817.1986.tb00021.x
Jacobson, D. M., and Anderson, D. M. (1996). Widespread phagocytosis of ciliates and other protists by marine mixotrophic and heterotrophic thecate dinoflagellates. J. Phycol. 32, 279–285. doi: 10.1111/j.0022-3646.1996.00279.x
Jakobsen, K. S., Tengs, T., Vatne, A., Bowers, H. A., Oldach, D., Burkholder, J. M., et al. (2002). Discovery of the toxic dinoflagellate Pfiesteria in northern European waters. Proc. R. Soc. Lond. B Biol. Sci. 269, 211–214. doi: 10.1098/rspb.2001.1852
Jang, S. H., Jeong, H. J., Kwon, J. E., and Lee, K. H. (2017). Mixotrophy in the newly described dinoflagellate Yihiella yeosuensis: a small, fast dinoflagellate predator that grows mixotrophically, but not autotrophically. Harmful Algae 62, 94–103. doi: 10.1016/j.hal.2016.12.007
Jang, S. H., Jeong, H. J., Lim, A. S., Kwon, J. E., and Kang, N. S. (2016). Feeding by the newly described heterotrophic dinoflagellate Aduncodinium glandula: having the most diverse prey species in the family Pfiesteriaceae. Algae 31, 17–31. doi: 10.4490/algae.2016.31.2.2
Jang, S. H., Jeong, H. J., Moestrup, Ø., Kang, N. S., Lee, S. Y., Lee, K. H., et al. (2015). Morphological, molecular and ecophysiological characterization of the phototrophic dinoflagellate Biecheleriopsis adriatica from Korean coastal waters. Eur. J. Phycol. 50, 301–317. doi: 10.1080/09670262.2015.1054892
Jeong, H. J., Ha, J. H., Park, J. Y., Kim, J. H., Kang, N. S., Kim, S., et al. (2006). Distribution of the heterotrophic dinoflagellate Pfiesteria piscicida in Korean waters and its consumption of mixotrophic dinoflagellates, raphidophytes and fish blood cells. Aquat. Microb. Ecol. 44, 263–278. doi: 10.3354/ame044263
Jeong, H. J., Kang, H., Shim, J. H., Park, J. K., Kim, J. S., and Song, J. Y. (2001a). Interactions among the toxic dinoflagellate Amphidinium carterae, the heterotrophic dinoflagellate Oxyrrhis marina, and the calanoid copepods Acartia spp. Mar. Ecol. Prog. Ser. 218, 77–86. doi: 10.3354/meps218077
Jeong, H. J., Kang, H. C., Lim, A. S., Jang, S. H., Lee, K., Lee, S. Y., et al. (2021). Feeding diverse prey as an excellent strategy of mixotrophic dinoflagellates for global dominance. Sci. Adv. 72, eabe4214. doi: 10.1126/sciadv.abe4214
Jeong, H. J., Kang, H. C., You, J. H., and Jang, S. H. (2018a). Interactions between the newly described small-and fast-swimming mixotrophic dinoflagellate Yihiella yeosuensis and common heterotrophic protists. J. Eukaryot. Microbiol. 65, 612–626. doi: 10.1111/jeu.12506
Jeong, H. J., Kim, J. S., Lee, K. H., Seong, K. A., Yoo, Y. D., Kang, N. S., et al. (2017). Differential interactions between the nematocyst-bearing mixotrophic dinoflagellate Paragymnodinium shiwhaense and common heterotrophic protists and copepods: killer or prey. Harmful Algae 62, 37–51. doi: 10.1016/j.hal.2016.12.005
Jeong, H. J., Kim, J. S., Song, J. Y., Kim, J. H., Kim, T. H., Kim, S. K., et al. (2007a). Feeding by protists and copepods on the heterotrophic dinoflagellates Pfiesteria piscicida, Stoeckeria algicida, and Luciella masanensis. Mar. Ecol. Prog. Ser. 349, 199–211. doi: 10.3354/meps07094
Jeong, H. J., Kim, J. S., Yoo, Y. D., Kim, S. T., Kim, T. H., Park, M. G., et al. (2003). Feeding by the heterotrophic dinoflagellate Oxyrrhis marina on the red-tide raphidophyte Heterosigma akashiwo: a potential biological method to control red tides using mass-cultured grazers. J. Eukaryot. Microbiol. 50, 274–282. doi: 10.1111/j.1550-7408.2003.tb00134.x
Jeong, H. J., Kim, S. K., Kim, J. S., Kim, S. T., Yoo, Y. D., and Yoon, J. Y. (2001b). Growth and grazing rates of the heterotrophic dinoflagellate Polykrikos kofoidii on red-tide and toxic dinoflagellates. J. Eukaryot. Microbiol. 48, 298–308. doi: 10.1111/j.1550-7408.2001.tb00318.x
Jeong, H. J., Kim, T. H., Yoo, Y. D., Yoon, E. Y., Kim, J. S., Seong, K. A., et al. (2011). Grazing impact of heterotrophic dinoflagellates and ciliates on common red-tide euglenophyte Eutreptiella gymnastica in Masan Bay, Korea. Harmful Algae 10, 576–588. doi: 10.1016/j.hal.2011.04.008
Jeong, H. J., Lim, A. S., Franks, P. J., Lee, K. H., Kim, J. H., Kang, N. S., et al. (2015). A hierarchy of conceptual models of red-tide generation: nutrition, behavior, and biological interactions. Harmful Algae 47, 97–115. doi: 10.1016/j.hal.2015.06.004
Jeong, H. J., Lim, A. S., Yoo, Y. D., Lee, M. J., Lee, K. H., Jang, T. Y., et al. (2014). Feeding by heterotrophic dinoflagellates and ciliates on the free-living dinoflagellate Symbiodinium sp. (Clade E). J. Eukaryot. Microbiol. 61, 27–41. doi: 10.1111/jeu.12083
Jeong, H. J., Ok, J. H., Lim, A. S., Kwon, J. E., Kim, S. J., and Lee, S. Y. (2016). Mixotrophy in the phototrophic dinoflagellate Takayama helix (family Kareniaceae): predator of diverse toxic and harmful dinoflagellates. Harmful Algae 60, 92–106. doi: 10.1016/j.hal.2016.10.008
Jeong, H. J., Seong, K. A., Yoo, Y. D., Kim, T. H., Kang, N. S., Kim, S., et al. (2008). Feeding and grazing impact by small marine heterotrophic dinoflagellates on heterotrophic bacteria. J. Eukaryot. Microbiol. 55, 271–288. doi: 10.1111/j.1550-7408.2008.00336.x
Jeong, H. J., Shim, J. H., Kim, J. S., Park, J. Y., Lee, C. W., and Lee, Y. (1999). Feeding by the mixotrophic thecate dinoflagellate Fragilidium cf. mexicanum on red-tide and toxic dinoflagellates. Mar. Ecol. Prog. Ser. 176, 263–277. doi: 10.3354/meps176263
Jeong, H. J., Song, J. E., Kang, N. S., Kim, S., Yoo, Y. D., and Park, J. Y. (2007b). Feeding by heterotrophic dinoflagellates on the common marine heterotrophic nanoflagellate Cafeteria sp. Mar. Ecol. Prog. Ser. 333, 151–160. doi: 10.3354/meps333151
Jeong, H. J., Yoo, Y. D., Kang, N. S., Lim, A. S., Seong, K. A., Lee, S. Y., et al. (2012). Heterotrophic feeding as a newly identified survival strategy of the dinoflagellate Symbiodinium. Proc. Natl. Acad. Sci. U.S.A. 109, 12604–12609. doi: 10.1073/pnas.1204302109
Jeong, H. J., Yoo, Y. D., Kang, N. S., Rho, J. R., Seong, K. A., Park, J. W., et al. (2010a). Ecology of Gymnodinium aureolum. I. Feeding in western Korean waters. Aquat. Microb. Ecol. 59, 239–255. doi: 10.3354/ame01394
Jeong, H. J., Yoo, Y. D., Kim, J. S., Seong, K. A., Kang, N. S., and Kim, T. H. (2010b). Growth, feeding and ecological roles of the mixotrophic and heterotrophic dinoflagellates in marine planktonic food webs. Ocean Sci. J. 45, 65–91. doi: 10.1007/s12601-010-0007-2
Jeong, H. J., Yoon, J. Y., Kim, J. S., Yoo, Y. D., and Seong, K. A. (2002). Growth and grazing rates of the prostomatid ciliate Tiarina fusus on red-tide and toxic algae. Aquat. Microb. Ecol. 28, 289–297. doi: 10.3354/ame028289
Jeong, H. J., You, J. H., Lee, K. H., Kim, S. J., and Lee, S. Y. (2018b). Feeding by common heterotrophic protists on the mixotrophic alga Gymnodinium smaydae (Dinophyceae), one of the fastest growing dinoflagellates. J. Phycol. 54, 734–743. doi: 10.1111/jpy.12775
Johnson, M. D. (2015). Inducible mixotrophy in the dinoflagellate Prorocentrum minimum. J. Eukaryot. Microbiol. 62, 431–443. doi: 10.1111/jeu.12198
Johnson, M. D., Rome, M., and Stoecker, D. K. (2003). Microzooplankton grazing on Prorocentrum minimum and Karlodinium micrum in Chesapeake Bay. Limnol. Oceanogr. 48, 238–248. doi: 10.4319/lo.2003.48.1.0238
Jung, M. K., Yin, T. Y., Moon, S. J., Park, J., and Yoon, E. Y. (2021). Taxonomy and physiology of Oxyrrhis marina and Oxyrrhis maritima in Korean waters. Water 13:2057. doi: 10.3390/w13152057
Kamykowski, D., and McCollum, S. A. (1986). The temperature acclimatized swimming speed of selected marine dinoflagellates. J. Plankton Res. 8, 275–287. doi: 10.1093/plankt/8.2.275
Kang, H. C., Jeong, H. J., Jang, S. H., and Lee, K. H. (2019). Feeding by common heterotrophic protists on the phototrophic dinoflagellate Biecheleriopsis adriatica (Suessiaceae) compared to that of other suessioid dinoflagellates. Algae 34, 127–140. doi: 10.4490/algae.2019.34.5.29
Kang, H. C., Jeong, H. J., Park, S. A., Eom, S. H., Ok, J. H., You, J. H., et al. (2020). Feeding by the newly described heterotrophic dinoflagellate Gyrodinium jinhaense: comparison with G. dominans and G. moestrupii. Mar. Biol. 167, 1–16. doi: 10.1007/s00227-020-03769-9
Kang, N. S., Jeong, H. J., Moestrup, Ø., Jang, T. Y., Lee, S. Y., and Lee, M. J. (2015). Aduncodinium gen. nov. and A. glandula comb. nov. (Dinophyceae, Pfiesteriaceae), from coastal waters off Korea: morphology and molecular characterization. Harmful Algae 41, 25–37. doi: 10.1016/j.hal.2014.11.002
Kang, N. S., Jeong, H. J., Yoo, Y. D., Yoon, E. Y., Lee, K. H., Lee, K., et al. (2011). Mixotrophy in the newly described phototrophic dinoflagellate Woloszynskia cincta from western Korean waters: feeding mechanism, prey species and effect of prey concentration. J. Eukaryot. Microbiol. 58, 152–170. doi: 10.1111/j.1550-7408.2011.00531.x
Kilias, E., Wolf, C., Nöthig, E. M., Peeken, I., and Metfies, K. (2013). Protist distribution in the Western Fram Strait in summer 2010 based on 454-pyrosequencing of 18S rDNA. J. Phycol. 49, 996–1010. doi: 10.1111/jpy.12109
Kim, S. J., Jeong, H. J., Kang, H. C., You, J. H., and Ok, J. H. (2019). Differential feeding by common heterotrophic protists on four Scrippsiella species of similar size. J. Phycol. 55, 868–881. doi: 10.1111/jpy.12864
Kimmance, S. A., Atkinson, D., and Montagnes, D. J. (2006). Do temperature–food interactions matter? Responses of production and its components in the model heterotrophic flagellate Oxyrrhis marina. Aquat. Microb. Ecol. 42, 63–73. doi: 10.3354/ame042063
LaJeunesse, T. C., Parkinson, J. E., Gabrielson, P. W., Jeong, H. J., Reimer, J. D., Voolstra, C. R., et al. (2018). Systematic revision of Symbiodiniaceae highlights the antiquity and diversity of coral endosymbionts. Curr. Biol. 28, 2570–2580. doi: 10.1016/j.cub.2018.07.008
Lee, C. W. (1998). Growth and Grazing Rates of the Heterotrophic Dinoflagellate Oxyrrhis marina and the Ciliate Stormbidinopsis sp. on Prorocentrum spp. Thesis, Kunsan National University, Kunsan. p. 36.
Lee, K. H., Jeong, H. J., Jang, T. Y., Lim, A. S., Kang, N. S., Kim, J. H., et al. (2014a). Feeding by the newly described mixotrophic dinoflagellate Gymnodinium smaydae: feeding mechanism, prey species, and effect of prey concentration. J. Exp. Mar. Biol. Ecol. 459, 114–125. doi: 10.1016/j.jembe.2014.05.011
Lee, K. H., Jeong, H. J., Yoon, E. Y., Jang, S. H., Kim, H. S., and Yih, W. (2014b). Feeding by common heterotrophic dinoflagellates and a ciliate on the red-tide ciliate Mesodinium rubrum. Algae 29, 153–163. doi: 10.4490/algae.2014.29.2.153
Lee, M. J., Jeong, H. J., Jang, S. H., Lee, S. Y., Kang, N. S., Lee, K. H., et al. (2016). Most low-abundance “background” Symbiodinium spp. are transitory and have minimal functional significance for symbiotic corals. Microb. Ecol. 71, 771–783. doi: 10.1007/s00248-015-0724-2
Lee, S. Y., Jeong, H. J., Kang, H. C., Ok, J. H., You, J. H., Park, S. A., et al. (2021). Comparison of the spatial-temporal distributions of the heterotrophic dinoflagellates Gyrodinium dominans, G. jinhaense, and G. moestrupii in Korean coastal waters. Algae 36, 37–50. doi: 10.4490/algae.2021.36.3.4
Lim, A. S., Jeong, H. J., Kim, J. H., Jang, S. H., Lee, M. J., and Lee, K. (2015). Mixotrophy in the newly described dinoflagellate Alexandrium pohangense: a specialist for feeding on the fast-swimming ichthyotoxic dinoflagellate Cochlodinium polykrikoides. Harmful Algae 49, 10–18. doi: 10.1016/j.hal.2015.07.010
Lim, A. S., Jeong, H. J., Ok, J. H., and Kim, S. J. (2018). Feeding by the harmful phototrophic dinoflagellate Takayama tasmanica (Family Kareniaceae). Harmful Algae 74, 19–29. doi: 10.1016/j.hal.2018.03.009
Lim, A. S., Jeong, H. J., Seong, K. A., Lee, M. J., Kang, N. S., Jang, S. H., et al. (2017). Ichthyotoxic Cochlodinium polykrikoides red tides offshore in the South Sea, Korea in 2014: II. Heterotrophic protists and their grazing impacts on red-tide organisms. Algae 32, 199–222. doi: 10.4490/algae.2017.32.8.25
Lowe, C. D., Martin, L. E., Roberts, E. C., Watts, P. C., Wootton, E. C., and Montagnes, D. J. (2011). Collection, isolation and culturing strategies for Oxyrrhis marina. J. Plankton Res. 33, 569–578. doi: 10.1093/plankt/fbq161
Matsuoka, K., Cho, H. J., and Jacobson, D. M. (2000). Observations of the feeding behavior and growth rates of the heterotrophic dinoflagellate Polykrikos kofoidii (Polykrikaceae, Dinophyceae). Phycologia 39, 82–86. doi: 10.2216/i0031-8884-39-1-82.1
McCarthy, P. M. (2013). Census of Australian Marine Dinoflagellates. Available online at: http://www.anbg.gov.au/abrs/Dinoflagellates/index_Dino.html
Menden-Deuer, S., and Lessard, E. J. (2000). Carbon to volume relationships for dinoflagellates, diatoms, and other protist plankton. Limnol. Oceanogr. 45, 569–579. doi: 10.4319/lo.2000.45.3.0569
Mitra, A., Flynn, K. J., Tillmann, U., Raven, J. A., Caron, D., Stoecker, D. K., et al. (2016). Defining planktonic protist functional groups on mechanisms for energy and nutrient acquisition: incorporation of diverse mixotrophic strategies. Protist 167, 106–120. doi: 10.1016/j.protis.2016.01.003
Ok, J. H., Jeong, H. J., Lee, S. Y., Park, S. A., and Noh, J. H. (2021). Shimiella gen. nov. and Shimiella gracilenta sp. nov. (Dinophyceae, Kareniaceae), a kleptoplastidic dinoflagellate from Korean waters and its survival under starvation. J. Phycol. 57, 70–91. doi: 10.1111/jpy.13067
Ok, J. H., Jeong, H. J., Lim, A. S., and Lee, K. H. (2017). Interactions between the mixotrophic dinoflagellate Takayama helix and common heterotrophic protists. Harmful Algae 68, 178–191. doi: 10.1016/j.hal.2017.08.006
Park, M. G., Kim, M. R., and Kang, M. S. (2013a). A dinoflagellate Amylax triacantha with plastids of the cryptophyte origin: phylogeny, feeding mechanism, and growth and grazing responses. J. Eukaryot. Microbiol. 60, 363–376. doi: 10.1111/jeu.12041
Park, M. G., Kim, S., Shin, E. Y., Yih, W., and Coats, D. W. (2013b). Parasitism of harmful dinoflagellates in Korean coastal waters. Harmful Algae 30, S62–S74. doi: 10.1016/j.hal.2013.10.007
Park, M. G., Kim, S. J., Kim, H. S., Myung, G., Kang, Y. G., and Yih, W. (2006). First successful culture of the marine dinoflagellate Dinophysis acuminata. Aquat. Microb. Ecol. 45, 101–106. doi: 10.3354/ame045101
Pedersen, M. F., and Hansen, P. J. (2003). Effects of high pH on the growth and survival of six marine heterotrophic protists. Mar. Ecol. Prog. Ser. 260, 33–41. doi: 10.3354/meps260033
Potvin, E., Hwang, Y. J., Yoo, Y. D., Kim, J. S., and Jeong, H. J. (2013). Feeding by heterotrophic protists and copepods on the photosynthetic dinoflagellate Azadinium cf. poporum from western Korean waters. Aquat. Microb. Ecol. 68, 143–158. doi: 10.3354/ame01603
Rhodes, L. L., Adamson, J. E., Rublee, P. A., and Schaefer, E. (2006). Geographic distribution of Pfiesteria spp. (Pfiesteriaceae) in Tasman Bay and Canterbury, New Zealand (2002–03). N. Z. J. Mar. Freshwater Res. 40, 211–220. doi: 10.1080/00288330.2006.9517414
Roberts, E. C., Wootton, E. C., Davidson, K., Jeong, H. J., Lowe, C. D., and Montagnes, D. J. (2011). Feeding in the dinoflagellate Oxyrrhis marina: linking behaviour with mechanisms. J. Plankton Res. 33, 603–614. doi: 10.1093/plankt/fbq118
Rublee, P. A., Nuzzi, R., Waters, R., Schaefer, E. F., and Burkholder, J. M. (2006). Pfiesteria piscicida and Pfiesteria shumwayae in coastal waters of Long Island, New York, USA. Harmful Algae 5, 374–379. doi: 10.1016/j.hal.2006.03.007
Scharf, F. S., Juanes, F., and Rountree, R. A. (2000). Predator size-prey size relationships of marine fish predators: interspecific variation and effects of ontogeny and body size on trophic-niche breadth. Mar. Ecol. Prog. Ser. 208, 229–248. doi: 10.3354/meps208229
Sherr, E. B., and Sherr, B. F. (2007). Heterotrophic dinoflagellates: a significant component of microzooplankton biomass and major grazers of diatoms in the sea. Mar. Ecol. Prog. Ser. 352, 187–197. doi: 10.3354/meps07161
Shumway, S. E. (1990). A review of the effects of algal blooms on shellfish and aquaculture. J. World Aquacult. Soc. 21, 65–104. doi: 10.1111/j.1749-7345.1990.tb00529.x
Stoecker, D. K. (1999). Mixotrophy among dinoflagellates. J. Eukaryot. Microbiol. 46, 397–401. doi: 10.1111/j.1550-7408.1999.tb04619.x
Strom, S., Wolfe, G., Holmes, J., Stecher, H., Shimeneck, C., and Sarah, L. (2003). Chemical defense in the microplankton I: Feeding and growth rates of heterotrophic protists on the DMS-producing phytoplankter Emiliania huxleyi. Limnol. Oceanogr. 48, 217–229. doi: 10.4319/lo.2003.48.1.0217
Strom, S. L., and Buskey, E. J. (1993). Feeding, growth, and behavior of the thecate heterotrophic dinoflagellate Oblea rotunda. Limnol. Oceanogr. 38, 965–977.
Taylor, F. J. R., Hoppenrath, M., and Saldarriaga, J. F. (2008). Dinoflagellate diversity and distribution. Biodivers. Conserv. 17, 407–418. doi: 10.1007/s10531-007-9258-3
Tillmann, U., and Hoppenrath, M. (2013). Life cycle of the pseudocolonial dinoflagellate Polykrikos kofoidii (Gymnodiniales, Dinoflagellata). J. Phycol. 49, 298–317. doi: 10.1111/jpy.12037
Tillmann, U., and Reckermann, M. (2002). Dinoflagellate grazing on the raphidophyte Fibrocapsa japonica. Aquat. Microb. Ecol. 26, 247–257. doi: 10.3354/ame026247
Tucker, S. J., McManus, G. B., Katz, L. A., and Grattepanche, J. D. (2017). Distribution of abundant and active planktonic ciliates in coastal and slope waters off New England. Front. Microbiol. 8:2178. doi: 10.3389/fmicb.2017.02178
Ward, B. A., and Follows, M. J. (2016). Marine mixotrophy increases trophic transfer efficiency, mean organism size, and vertical carbon flux. Proc. Natl. Acad. Sci. U.S.A. 113, 2958–2963. doi: 10.1073/pnas.1517118113
Watts, P. C., Martin, L. E., Kimmance, S. A., Montagnes, D. J., and Lowe, C. D. (2011). The distribution of Oxyrrhis marina: a global disperser or poorly characterized endemic? J. Plankton Res. 33, 579–589. doi: 10.1093/plankt/fbq148
Yoo, Y. D., Jeong, H. J., Kang, N. S., Kim, J. S., Kim, T. H., and Yoon, E. Y. (2010). Ecology of Gymnodinium aureolum. II. Predation by common heterotrophic dinoflagellates and a ciliate. Aquat. Microb. Ecol. 59, 257–272. doi: 10.3354/ame01401
Yoo, Y. D., Jeong, H. J., Kim, J. S., Kim, T. H., Kim, J. H., Seong, K. A., et al. (2013a). Red tides in Masan Bay, Korea in 2004–2005: II. Daily variations in the abundance of heterotrophic protists and their grazing impact on red-tide organisms. Harmful Algae 30, S89–S101. doi: 10.1016/j.hal.2013.10.009
Yoo, Y. D., Jeong, H. J., Lee, S. Y., Yoon, E. Y., Kang, N. S., Lim, A. S., et al. (2015). Feeding by heterotrophic protists on the toxic dinoflagellate Ostreopsis cf. ovata. Harmful Algae 49, 1–9. doi: 10.1016/j.hal.2015.08.001
Yoo, Y. D., Yoon, E. Y., Jeong, H. J., Lee, K. H., Hwang, Y. J., Seong, K. A., et al. (2013b). The newly described heterotrophic dinoflagellate Gyrodinium moestrupii, an effective protistan grazer of toxic dinoflagellates. J. Eukaryot. Microbiol. 60, 13–24. doi: 10.1111/jeu.12002
Keywords: ciliate, grazer, microbial food web, mixotrophy, predation
Citation: Park SA, Jeong HJ, Ok JH, Kang HC, You JH, Eom SH and Park EC (2021) Interactions Between the Kleptoplastidic Dinoflagellate Shimiella gracilenta and Several Common Heterotrophic Protists. Front. Mar. Sci. 8:738547. doi: 10.3389/fmars.2021.738547
Received: 09 July 2021; Accepted: 30 August 2021;
Published: 11 October 2021.
Edited by:
Gordon T. Taylor, Stony Brook University, United StatesCopyright © 2021 Park, Jeong, Ok, Kang, You, Eom and Park. This is an open-access article distributed under the terms of the Creative Commons Attribution License (CC BY). The use, distribution or reproduction in other forums is permitted, provided the original author(s) and the copyright owner(s) are credited and that the original publication in this journal is cited, in accordance with accepted academic practice. No use, distribution or reproduction is permitted which does not comply with these terms.
*Correspondence: Hae Jin Jeong, hjjeong@snu.ac.kr