- 1College of Environment, Engineering and Information Technology, Charles Darwin University, Darwin, NT, Australia
- 2Australian Fisheries Management Authority, Darwin, NT, Australia
- 3Australian Institute of Marine Science, Darwin, NT, Australia
- 4Australian Bureau of Meteorology, Sydney, NSW, Australia
- 5Northern Institute, College of Indigenous Futures, Arts and Society, Charles Darwin University, Darwin, NT, Australia
Accessing the world's oceans is essential for monitoring and sustainable management of the maritime domain. Difficulty in reaching remote locations has resulted in sparse coverage, undermining our capacity to deter illegal activities and gather data for physical and biological processes. Uncrewed Surface Vessels (USVs) have existed for over two decades and offer the potential to overcome difficulties associated with monitoring and surveillance in remote regions. However, they are not yet an integral component of maritime infrastructure. We analyse 15 years of non-autonomous and semi-autonomous USV-related literature to determine the factors limiting technological diffusion into everyday maritime operations. We systematically categorised over 1,000 USV-related publications to determine how government, academia and industry sectors use USVs and what drives their uptake. We found a striking overlap between these sectors for 11 applications and nine drivers. Low cost was a consistent and central driver for USV uptake across the three sectors. Product ‘compatibility' and lack of ‘complexity' appear to be major factors limiting USV technological diffusion amongst early adopters. We found that the majority (21 of 27) of commercially available USVs lacked the complexity required for multiple applications in beyond the horizon operations. We argue that the best value for money to advance USV uptake is for designs that offer cross-disciplinary applications and the ability to operate in an unsheltered open ocean without an escort or mothership. The benefits from this technological advancement can excel under existing collaborative governance frameworks and are most significant for remote and developing maritime nations.
Introduction
Access to the world's oceans underpins the sustainable development and management of global maritime resources. Broader coverage of the maritime domain reduces the risk of natural resource degradation, exploitation and illegal activity (Lindstrom et al., 2012; Visbeck, 2018; Claudet et al., 2020), particularly in large ocean nations (United Nations, 2015; Dunn et al., 2018) and areas beyond national jurisdictions (Cremers et al., 2020; United Nations, 2021). High spatiotemporal coverage for metocean data collection is fundamental to the sustainable management of our oceans (Beal et al., 2019; Smith et al., 2019; Friedman et al., 2020). Increased ocean coverage is an internationally agreed goal, underpinning the United Nations Sustainable Development Goals (United Nations, 2015) and Decade of Ocean Science for Sustainable Development (Ryabinin et al., 2019). Conventional methods of ocean access such as crewed ships, lagrangian observatories and fixed-point moorings play a critical role in global ocean monitoring. However, annual running costs associated with one standard European fixed-point observatory is almost 1 M USD, including daily operational ship costs estimated at 25 K USD (Cristini et al., 2016). Lagrangian observatories also depend on deployable vessels to obtain ocean coverage, and their positions are unable to be controlled. These constraints and high expenditures limit the ability to operate at vast distances, long time-frames and flexible schedules in the open ocean. Severe weather and lack of infrastructure in remote areas compound these difficulties. A cost-effective solution is needed to improve ocean access.
Global investment in technology has transformed maritime domain awareness and is set to continue into the future (Friedman et al., 2020). Automatic Identification System (AIS), Vessel Monitoring Systems (VMS) and satellite technology are examples of strategic investments used to improve monitoring, control and surveillance, particularly in large ocean nations (Wood and Weigel, 2011; Dunn et al., 2018; McCauley et al., 2018). Recently, remotely operated and autonomous technologies such as gliders, uncrewed aerial vehicles, smart buoys and Argos profiling floats have filled spatial and temporal voids (Manley, 2016). However, these are limited by their design and implementation in their capacity to communicate and persist simultaneously.
Uncrewed and autonomous surface vessels are promising innovations due to their continuous access to communications, renewable surface energy, available propulsion sources, scalable payload and simultaneous access to the water-suface interface (Roberts and Sutton, 2006; Liu et al., 2016; Manley, 2016; Costanzi et al., 2020). Uncrewed Surface Vessels (USV), also referred to as Autonomous Surface Vessels (ASV), have varying levels of autonomy, and most rely on human decision making for the safety of crewed vessels at sea [the International Regulations for Avoiding Collisions at Sea (COLREGs, 1972)] and for maintaining operational environmental awareness. Definitions of autonomy vary worldwide, with eight different institutional standards of autonomy levels discussed in the Committee on Coast Guard Maritime Domain Awareness (2020).
USVs are relatively simple and low-cost. Continuous real-time communications analogous to an onboard environment allow operational flexibility and human decision-making for a multitude of applications, such as national security and surveillance, scientific data collection, and asset monitoring and protection (Ziegwied et al., 2016; Eleftherakis and Vicen-Bueno, 2020; Siddle et al., 2021; Sutton et al., 2021). USVs can increase precision for some tasks (Ferreira et al., 2009; Li et al., 2018; Raber and Schill, 2019) and be scaled in numbers to operate in bricks or service multiple locations concurrently for the price of a single crewed ship (Cole, 2020; Costanzi et al., 2020). Successful technological diffusion of innovations such as USVs depends on the gradual uptake of the technology by the community (Rogers, 2010). USV uptake is apparently constrained despite scores of commercial prototypes rapidly becoming available (Liu et al., 2016). Suggestions for the slow uptake include low consumer confidence (Costanzi et al., 2020), lagging legal and regulatory frameworks (Campbell et al., 2012; Negoro et al., 2020) and high capital costs of specialised new assets (Gu et al., 2020) where investements have already been made in multi-use flagships. However, there has been no overarching and systematic study to determine what might be limiting this hopeful technology's uptake.
This article is a synthesis of peer-reviewed studies reviewing 15 years of USV related literature. We undertake a qualitative and quantitative evaluation of the drivers, broadscale applications and operational environments of USV use. We discuss our results in response to the five successful innovation attributes required for diffusion of innovation: relative advantage, compatibility, complexity, trialability and observability (Rogers, 2010). Linking these attributes with our findings provides guidance for both USV developers and consumers towards decision-making to advance consumer uptake of this burgeoning technology.
Methods
Data Collation
We conducted a Web of Science literature search using the search terms: “unmanned surface vessel” and “unmanned surface vehicle,” not “aerial” and “airborne” from 2005 – August 2020 inclusive. There were no results for the search term “uncrewed surface vessels” prior to August 2020. Of the 1,690 articles retrieved and screened 1,106 articles were deemed to meet the eligibility criteria relevant to this review. The 1,106 articles were derived from 358 different journal titles and 448 conferences proceedings. We excluded the articles related to non-surface autonomous or uncrewed vehicles and those associated with autonomous cargo and transport (the shipping industry).
Our search strategy aimed to provide a representative sample of USV-related literature and did not attempt to identify an exhaustive list of USV articles or describe every available commercial model. We acknowledge that there are limitations to this search method, given not all the commercially available USV applications and developments are described in academic articles. We also note that there are many USV developments and applications with unpublished descriptions (grey literature or otherwise) because their designs are commercial-in-confidence or sensitive from a national security perspective. To ensure we had captured a critical quantity of academic literature, we conducted an extended search using the term: “Autonomous Surface Vessel.”
The first step was to manually categorise and count the 1,106 academic journal and conference proceedings articles, resulting in the following categories and counts; 1. Development (guidance, control, navigation, design and engineering) (848 articles) 2. Application (196), 3. Both categories 1 and 2 (49), 4. Other, not related to development or application (13). We recorded the publication year, hull type, and commercial USV make or model if provided. We included semi-submersible USVs but excluded hovercraft. Groups 2 and 3 above were merged to produce a total of 245 application articles which were assessed in further detail. Of the 245 application articles, 22 article titles referred to the described USV as autonomous and these articles were further evaluated to determine how each article defined autonomy. The extended search using the term “autonomous surface vessel” found 80 additional articles (31 journal, 49 conference proceedings). Of these, there were four application articles, representing 1.6% of the 245 application articles used in these analyses. We did not include any of the extended search results in the analyses.
The 245 application articles were manually assessed to determine the USV applications and drivers. USV applications refer to the application of the USVs in each study, and USV drivers refer to the reason for using a USV as an alternative to traditional methods, such as crewed ships. Driver categories were defined if they were listed in more than five articles. Multiple applications and drivers nominated by individual articles were counted individually towards the total, for example, one article could have multiple drivers and applications. The assessment resulted in 11 applications: hydrography, ecology, inshore surveillance, offshore surveillance, infrastructure protection/inspection, pollution control, environmental monitoring, search and rescue, multiple remotely operated vehicles, general military and mine countermeasures (see Supplementary Material 1 for long description), and nine drivers: cost, shallow water access, endurance, personnel protection, multi-USV spatial coverage, rapidity and spatiotemporal efficiency, support for other autonomous systems, precision, and low noise environment.
General hull design provided an indicator for each study's operational environments. Inherently, multi-hull, rigid hull inflatables or centre consol USVs require in situ monitoring and intervention if capsized and cannot be persistent in the open ocean. We categorised these as Sheltered and Escorted (SE) archetypes. Other USVs were explicitly designed to be self-righting and unattended in the open ocean, categorised as Unsheltered and Unescorted (UU) archetypes.
Quantitative Data Analysis
Correspondence analysis (CA) is a quantitative method of characterising data tables with multiple categorical variables by reducing the number of dimensions represented by the variables and graphing them. We used the python package ‘prince' to reduce the dimensionality for the 11 applications, nine drivers and their associated publication years extracted from the literature review. We present two CA analyses: Applications and drivers, and Drivers and publication years.
To determine how the sectors industry, government and academia are related, we weighted each USV application by relevance to the sector, 3 being the most relevant and 1 being the least, resulting in an 11 × 3 matrix. All three sectors are inherent in USV applications, so all sectors were represented in the weightings. Government weighted highest (weighting = 3) in 9 out of the 11 applications because even if used throughout industry, their presiding reason for use was for regulation or compliance, prescribed by the government. Offshore surveillance in the literature pertained to asset protection (e.g., offshore infrastructure patrols) and offshore energy, so government was weighted high, industry medium and academia low. Military related applications were weighted high to government and medium to industry because the USV products used in the military are commercialised, innovative robotics industry products. Academia weighted high in environmental and ecological surveys because the tools and methods used in these assessments stem from academic pursuits and methodologies. Industry weighted medium for ecological surveys because many of the articles were associated with commercial fisheries research. Each weighting matrix was multiplied with the CA coordinates and plotted as an ellipse representing one standard deviation of the weighted application coordinates.
We listed the essential features required to fulfil the 11 resultant applications beyond navigation, guidance and control. Our aim was to list features that simulate an onboard environment: real-time communications, passive perception, payload capacity, payload power, manoeuvrability, deployments at depth and precision stability. In addition, we included the features that make USVs cost-effective that are unavailable in crewed ships: endurance, easy deployment and with multiple-USV collaborative capability.
Results
Development vs. Application Articles
The total counts of category 1 (development) articles and the combined categories 2 and 3 (applications) articles were plotted over time as raw counts and as percentage totals (Figure 1). The total number of articles increased over time in both categories, but the proportion of articles referring directly to USV applications remained consistently between 10 and 20%. From 2014 to 2019, the number of application articles increased by 34 articles, whereas the number of development articles increased by 115.
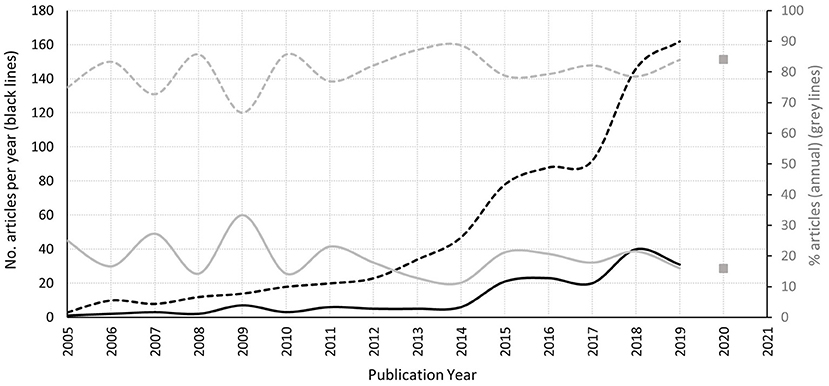
Figure 1. Publications about USV applications (solid) and developments (guidance, control, design, navigation and engineering) (dashed) raw counts (black) and proportion of annual total (grey) vs. publication year based on publications between January 2005 and August 2020.
USVs and Autonomy
While the search terms used in the analysis did not include “autonomous surface vessel,” articles referring to their prototypes as “autonomous” still appeared in 22 of the results used in the analyses. There were no apparent differences between prototypes described as “autonomous” as opposed to “unmanned,” nor did they display higher levels of autonomy. Most articles appeared to use the term “autonomous” to describe an autonomous feature, e.g., autonomous: navigation (Idris et al., 2016), detection and tracking specific environmental conditions (Zhang et al., 2019), or sailing (Frizzell-Makowski et al., 2011). Thus, “USV” and “ASV” were used interchangeably. The results from the extended search resulted in only 80 additional articles and four additional application articles (1.6% of the analysed data), indicating that “USV” is a more popular method than “ASV” for describing essentially the same technology, which is surface vessels, without any crew, and with varying levels of autonomy and automation.
Archetypes for (Un)sheltered and (Un)escorted USVs
Of the 186 articles that described the USV hull type, 65% were only capable of operating under Escort or in Sheltered (ES) environments, whilst 35% could operate in Unescorted and Unsheltered (UU) environments (Figure 2A). Of the 186 articles, 58 articles (31%) specified and described a commercial USV. Roughly equal numbers of the commercial UVS's described could operate in UU environments (47%) and ES environments (53%). The total number of unique commercial USV archetypes was 27 [UU =6 (22%), ES = 21 (78%)] (Figure 2A). In most cases, individual ES commercial archetypes were referred to in only one article, with a small number of ES archetypes referred to in two or three different articles, except for the Wave Adopted Modular Vessel (WAM-V) which was referred to in 5 articles. This was similar for UU archetypes, with the exception of Saildrone and Wave Glider, which were referenced in 10 and 13 articles, respectively. Despite the low number (6) of commercial UU archetypes, nearly half (47%) of the 58 articles referencing commercial archetypes were written about 22% of the commercially available archetypes (Figure 2A).
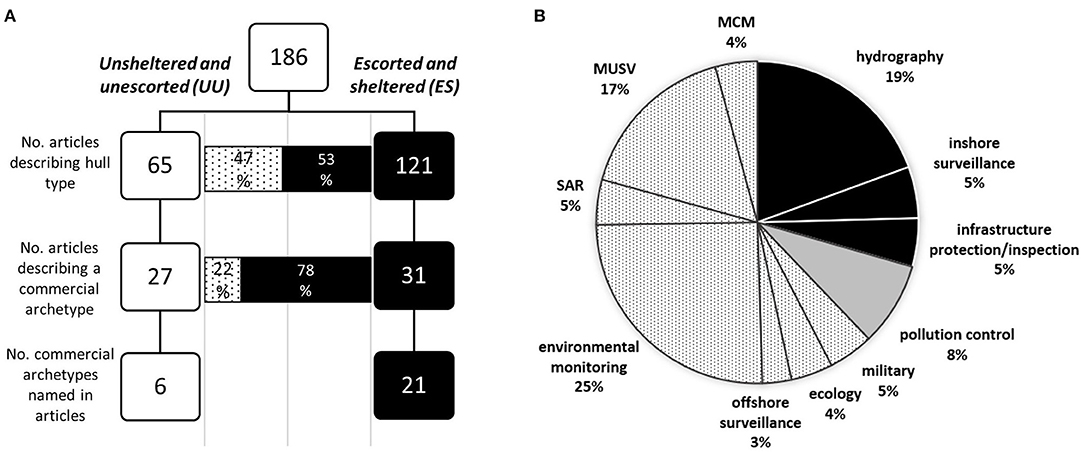
Figure 2. Proportion of literature describing unescorted and unsheltered (UU) and escorted and sheltered (ES) operations in the literature description of environmental conditions, commercial make and available commercial prototypes (A), and proportion of literature describing UU (dotted), ES (black) and UU or ES (grey) USV applications (B).
USV Applications and Drivers
Of the 245 application articles, 63% described in the literature could have been carried out on a single hull archetype and could therefore undertake applications in a UU environment (Figure 2B). ES applications specifically requiring precision stability and optimal manoeuvrability made up 29% of the total applications, including pollution control, which could be either ES or UU (Figure 2B). This contrasts with the low availability of commercial USVs for UU operations.
Cost was the most common driver of USV use (no. publications stating cost as a driver = 78) followed by personnel protection (60), rapidity and spatiotemporal efficiency (53), shallow water access (50), endurance (48), support for other autonomous systems (40), precision (18), multi-USV spatial coverage (17), and low noise environment (6). Other drivers not included in these analyses were: adaptability (4), launch efficiency (2), simple (1), renewable energy source (no fossil fuels) (1), structural stability (1), access to deep water (1).
Correspondence Analysis
Correspondence analysis (CA) dimension reduction of 11 applications and 9 drivers to two dimensions described 40.5% (component 1) and 26.2% (component 2) of variance. The resultant plot (Figure 3A) can be roughly divided into quadrants where x and y intersect at zero. In the intersection, cost and spatiotemporal efficiency are key drivers to all applications. In the bottom left quadrant, nearshore and sheltered water environments appear to drive USV applications in hydrographic surveys, infrastructure protection/inspection and inshore surveillance (Figure 3A). This cluster represents ES USV applications. The literature strongly represents hydrographic surveys in water too shallow or dangerous to be accessed by crewed vessels (Figure 3A). In the bottom right quadrant, military applications, mine countermeasures, and to some degree, search and rescue (Figure 3A) appears to be driven by personnel safety. In the top-right quadrant, interoperability between other autonomous systems appears to be driven by the need for autonomous support (Figure 3A). In the top left quadrant, cost, precision and endurance drive environmental and ecological surveys, and although in another quadrant, are clustered with offshore surveillance (Figure 3B).
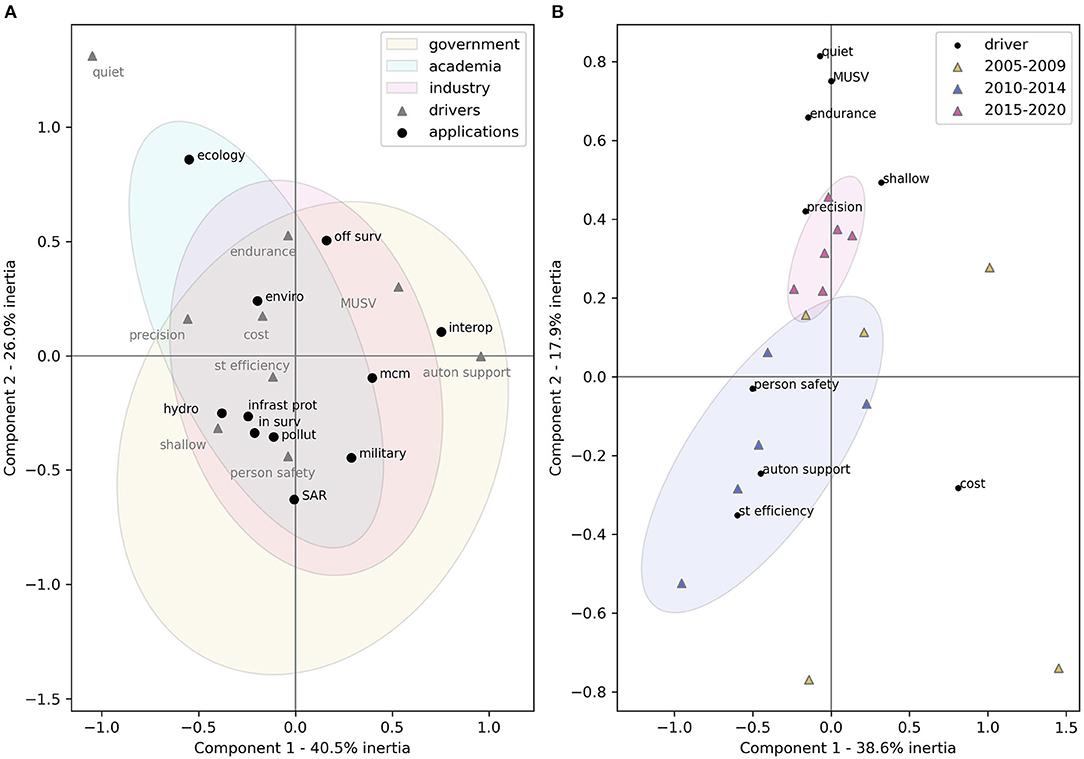
Figure 3. Correlation analysis of USV applications and their drivers (A), and drivers and publication year (B). The ellipse for years 2005–2009 was excluded as there was no clear clustering.
All sectors associated with USV applications overlap completely except for ecology and interoperability. Offshore surveillance overlaps with government and industry, but not academia. Ecological surveys (weighted in order academia-industry-government) were associated with wildlife detection, particularly cetaceans, hence their correlation with “quiet” as a driver. Interoperability was represented in the literature by military applications and did not overlap with industry or academia.
CA reduction of drivers and years to two dimensions described 38.6% (component 1) and 17.9% (component 2) of variance (Figure 3B). Application trends are visible in 5 yearly groups: 2005–2009 no clustering, 2010–2014 clusters for personnel safety, spatiotemporal efficiency, autonomous support vessel, and 2015–2020 strong clustering for endurance, multiple USV, quiet, precise and shallow water (Figure 3B). Cost is not clustered with any temporal grouping, suggesting it is a consistent factor through time.
USV Features
USV field success for particular applications relies on custom integrated USV features. Multiple custom features involve increased complexity in design and engineering. In addition, some features were more important than others across applications. We ranked the importance and complexity of custom features required for the 11 applications (Figure 4). We assume that guidance, control and navigation already exist in modern, commercially viable USVs so were excluded from Figure 4. Environmental monitoring requires the most features for mission success. The most important feature across all applications is real-time communications. The number of features required in UU operations generally exceeds ES, suggesting that there is a higher level of complexity required for UU applications.
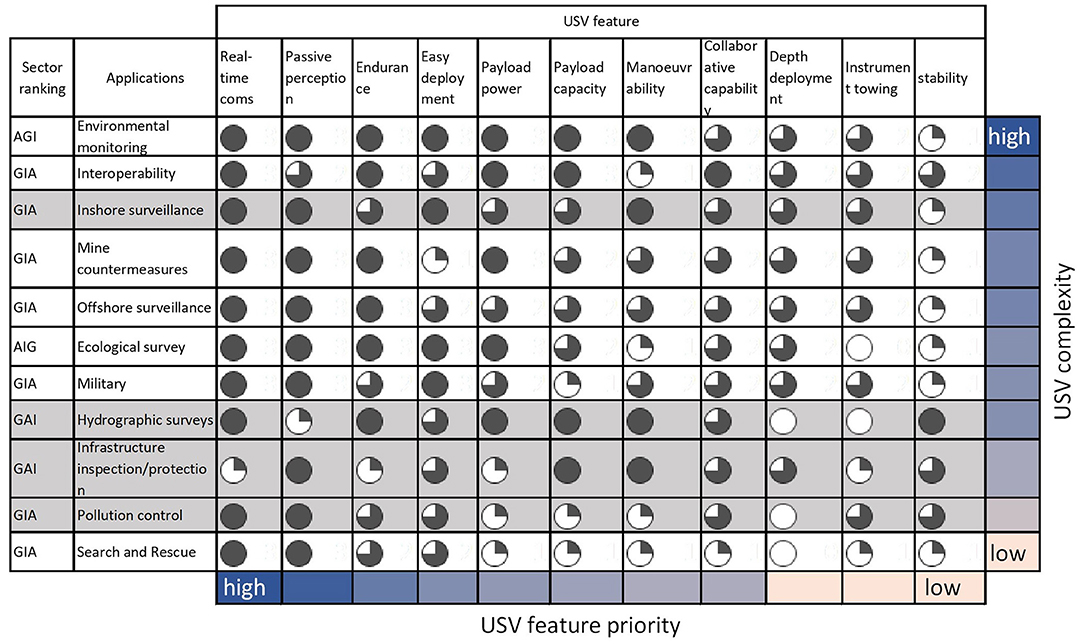
Figure 4. USV features are ranked using symbols: essential for application success,
highly enhances application success,
beneficial to application,
application does not benefit from this feature. Columns and rows depict feature importance (column) to the application (row). Total USV complexity and feature priority are ranked from blue (high) to beige (low). Grey and white rows are ES and UU archetype applications, respectively. Sector ranking is described in the Quantitative Data Analysis section of the Methods.
Discussion
USV developers require a better understanding of their potential consumer market. Similarly, future consumers without deep technical awareness require a straightforward template for choosing a USV to suit their needs. In this review of 1,106 peer-reviewed articles spanning the last 15 years, we analysed the current state of USV technological diffusion. Our focus on quantifying the applications and drivers amongst existing consumers led to our recommendation that USV developers focus their products on those in need of affordable access to the open ocean. In addition, we suggest multiple pathways to achieve widespread consumer uptake under existing collaborative governance frameworks.
USV Developers and Consumer Applications
Our review found a distinct mismatch between the number of USVs developed for Escorted or Sheltered (ES) applications and the apparent consumer interest in Unsheltered and Unescorted (UU) applications. ES-specific USVs made up more than three quarters (78%, n = 27) of commercial USV archetypes. However, UU archetypes were more popularly published, with an average of 4.5 articles per individual UU USV vs. 1.5 articles per individual ES USV. Developers tend to make ES USVs using retrofitted boats originally designed for crews, e.g., (Norgren et al., 2015; Weaver and Dunlap, 2016; Kitowski, 2019), or using elements such as canoes to form the hull structure - reasonable and cheap ways to develop and test USV systems. Thus, ES USVs tend to be the status quo, with nominally few available commercial UU USVs. We argue that UU USVs should be the status quo because of their greater potential for diversity and robustness, and ES USVs should be specialised for the 3 (out of 11) applications that specifically require their stability and manoeuvrability, e.g., hydrographic surveys, inshore surveillance and inshore infrastructure protection and inspection. Possible reasons for the developer-consumer dichotomy are: (1) A robust, ocean-faring multi-use USV is more complex and expensive to make than ES USVs, and (2) developers may be unaware of their multifaceted market potential. However, robust, ocean-faring, multi-use USVs in unsheltered waters and without an escort or mothership cost a fraction of crewed vessels, and are disruptive for ocean surveillance and monitoring across sectors.
Feature Complexity in Disruptive USV Design
Cost was a central, timeless and most common driver for USV uptake. Here, we discuss feature complexity with respect to cost and consumer value. Complex, multi-tasking USVs with multiple integrated features offer better value than bespoke designs. While high complexity (e.g., numerous integrated features) and development costs are mutually implicated, a single “one-size-fits-most” USV offers better value to coorperative multi-sectoral investors.
This study found real-time communications and passive perception were the most important USV features across applications and UU and ES archetypes. Real-time communications are essential for maritime surveillance constabulary response times, and significantly improve weather and climate model forecasts via oceanographic model data assimilation (Toyoda et al., 2015). Real-time communications and passive perception go hand-in-hand, where perception is required for operating the vessel in the vicinity of other traffic, and real-time communications are needed to transfer the information. A major problem for USV real-time communications in remote locations is expensive high bandwidth satellite data. USV developers have overcome this by implementing machine learning to reduce non-essential data sent over satellite. Implicit (data driven) or explicit (model driven) machine learning isolates relevant data snippets for real-time relay, such as objects or acoustics (Martin et al., 2019), reducing the amount of data sent and received. Consumers choosing USVs with this integrated feature may reduce the significant data overheads required in over-the-horizon surveillance.
Major cost savings can be gained by USV endurance. Endurance requires not only a physically robust platform but one or several renewable power sources and multiple redundancy fail-safe systems. Renewable power for perpetual USVs typically consists of solar, whereas propulsion sources tended to be wind and wave for UU and electric motor for ES USVs. Risks associated with solar power reliance are the uncertainty of persistent overcast days, and night-time operations. Therefore, ample battery storage must be available—but the requirements vary significantly between models due to size and power payload, which relates to the system complexity, e.g., number of devices needing power. Another factor related to endurance and cost was the ease of launch and recovery. Some USVs require deep-water launch and recovery such as from a mothership or crane. The high cost of these methods may be offset by endurance, however, even the most durable craft will require land-based maintenance, which may increase cost and complexity in very remote locations. Simple launch and recovery from boat ramps or beaches will be of particular appeal in large ocean states and remote maritime locations.
Stability is a feature requirement mainly for hydrographic surveys, which tend to be ES USVs and inherently require calm water. Precision manoeuvrability is also crucial for ES operations in restricted space, such as inshore asset protection (Pastore and Djapic, 2010), infrastructure inspection (Steimle et al., 2009; Shimono et al., 2015) and hydrographic surveys (Ferreira et al., 2009; Han et al., 2015). However, manoeuvrability is also a requirement in UU operations. For example, station-holding for gateway communications, depth measurements and instrument or array towing. Adjustable length cables for varying depth deployment is a significant investment in a USV design, with broad potential in subsurface profiling, such as Conductivity Temperature and Depth (CTD) data collection, ecological acoustic monitoring and anti-submarine warfare or mine countermeasures.
Economic value for collaborative capabilities is arguably the highest, offering the most versatile for extending the range of other autonomous or uncrewed marine vehicles. USV surface position makes an ideal collaborative robot capable of recharging power, networking, and providing gateway data streams (Brown et al., 2010; Zwolak et al., 2017; Li et al., 2019) to and from other autonomous or uncrewed vehicles.
Funding Complex USVs via Cross-Sectoral Cooperation
Our results show a striking overlap between government, academia and industry in the past 15 years of USV uptake. Remarkable collaboration opportunities exist for USVs, particularly in the maritime domain where governance models for multi-use operations, shared assets, knowledge sharing and technology transfer already exist (Ardron et al., 2014; Weller et al., 2019; Friedman et al., 2020). For example, cooperative governance between the 17 member states of the Pacific Islands Forum Fisheries Agency (FFA) has a significant positive impact on strengthening national capacity and regional solidarity towards maritime domain governance for fisheries (Sokimi and Aqorau, 2019). Under various agreements, the FFA member states, comprising mainly of large ocean nations, share assets such as aircraft, vessels, knowledge and intel on fisheries activities in the region, maximising the effectiveness of fisheries management and surveillance operations over the large collective maritime domain. USVs and their real-time data could also be shared under existing governance frameworks.
Similarly, cross-disciplinary models of maritime security forces, which share vessels, aircraft, data and information, are demonstrated by the Australian Maritime Border Command (MBC), a multi-agency organisation covering maritime security threats to defence, customs, fisheries, environment, law enforcement, immigration and biosecurity (Noonan and Williams, 2016). Shared asset use and knowledge transfer improve maritime awareness, promote best practises sharing, eliminate redundancy, and foster collaboration (Tikanmäki and Ruoslahti, 2017). The economic benefits of these co-investments are already prompting public investment in USV technology (Meinig et al., 2019). Given the low (n = 6) number of published commercial USV prototypes in UU environments, we argue that UU USVs will be of most value to international collaborations in remote and populated areas such as the Pacific Islands. Strong governance and collaborative partnerships will be the cornerstone of seeing this promising technology being adopted more widely.
Niche Opportunities for USVs
Niche applications exist for USVs that conventional methods currently cannot undertake. We provide a summary of notable USV applications that surpass conventional methods for ocean surveillance and monitoring based on the literature in this review (Table 1). We challenge the perspective that USVs are replacements for crewed ships and other existing ocean observing technologies. The contents of Table 1 emphasises USVs ability to hold a unique functionality that complements popular methods of ocean observing.
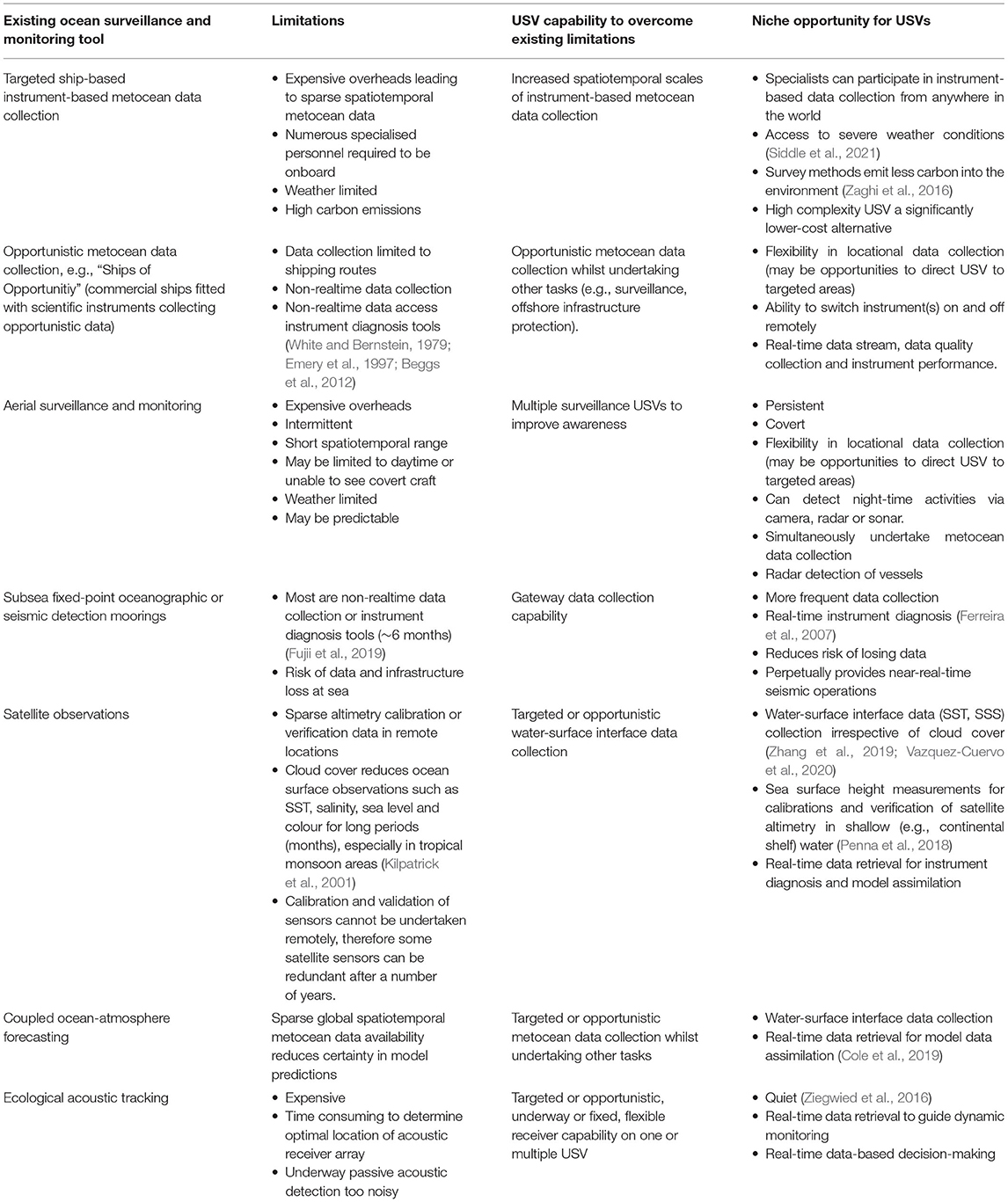
Table 1. Niche opportunities for USVs to support existing methods for ocean surveillance and monitoring.
Opportunities for Large Ocean Nations and Very Remote Locations
Nations with remote maritime areas, particularly large ocean nations, face an orchestra of unique geopolitical pressures due to their high sea to land ratios and the high cost of accessing those sovereign maritime jurisdictions. Here, we discuss the challenges of maritime crime, sustainability, and environmental monitoring, focusing on large ocean nations and remote maritime environments' potential uptake of multi-use USVs.
Illegal, Unreported and Unregulated (IUU) fisheries disproportionately affect developing countries, affecting livelihoods and contributing significantly to economic losses and environmental degradation (Agnew et al., 2009). In addition, IUU fisheries are synonymous with other organised and transnational crime involving drug trafficking, people trafficking and money laundering (Witbooi et al., 2020; United Nations, 2021). The impact of organised crime (Bruwer, 2019) and sanctioned fisheries in low-income nations (McCauley et al., 2018) threatens the global blue economy, sustainability and regional stability (Brashares et al., 2014; Pinsky et al., 2018; UN Security Council, 2019). Heightened surveillance and monitoring is needed to cover vast areas of ocean in remote sovereign areas and on the high seas. Covert USVs can, in real-time, identify, locate and monitor illegal activities using radar, cameras, infrared, LiDAR and acoustics. Multiple covert USVs transmitting real-time situational awareness have the potential to enhance significantly existing maritime security enforcement measures (Patterson et al., 2013; Real-Arce et al., 2015; Johnston and Poole, 2017).
Fewer and less robust metocean observations in large ocean nations and remote locations are the result of ocean access difficulties (Sem, 2007; World Meteorological Organisation, 2016; Powers et al., 2019). The tropics are especially difficult for ocean-surface data collection because they are subject to extended periods of cloud cover, reducing satellite coverage for substantial periods of the year (Kilpatrick et al., 2001). In addition, the risk of monsoon troughs and tropical cyclones reduces in situ observations extensively during these events. This results in a reduction of weather and climate model certainty (Flannaghan et al., 2014) and reduces the prediction capability of severe weather events, some of which can lead to environmental and humanitarian disasters (Alcántara-Ayala, 2002). Autonomous or remotely operated technology can transform ocean observations (Malone et al., 2014). For large ocean developing nations, “frugal innovation” (i.e., survival through technological innovation), is a necessity (Bhatti et al., 2018; Harris et al., 2020), and stand to benefit from a triple-helix (academia-industry-government) approach (Etzkowitz et al., 2000) to broadscale USV uptake.
The Current State of USV Technological Diffusion
This study indicates that the technological diffusion of USVs is in its infancy. USV engineering and development articles dominate the literature (71% of articles in this study), whereas uptake in applications, including UU and ES operations, is relatively few (22%). Compare this to aerial drones—a common household item and considered mature in their technological diffusion—where engineering-related literature declined and applications literature increased from 2013 to 2017 (Chabot, 2018).
To further explain the infancy we see in USV technological diffusion, we discuss the diffusion of innovation success attributes derived by Rogers (2010). We use evidence from this study to discuss the success attributes; relative advantage, observability, trialability, compatibility and complexity in Table 2. We argue that relative advantage is currently a non-limiting success attribute; however, due to the relatively early stages of USV technological diffusion, the perceived advantage is likely to grow. Observability, trialability, compatibility, and complexity currently limit the diffusion of USV technology amongst existing and potential consumers.
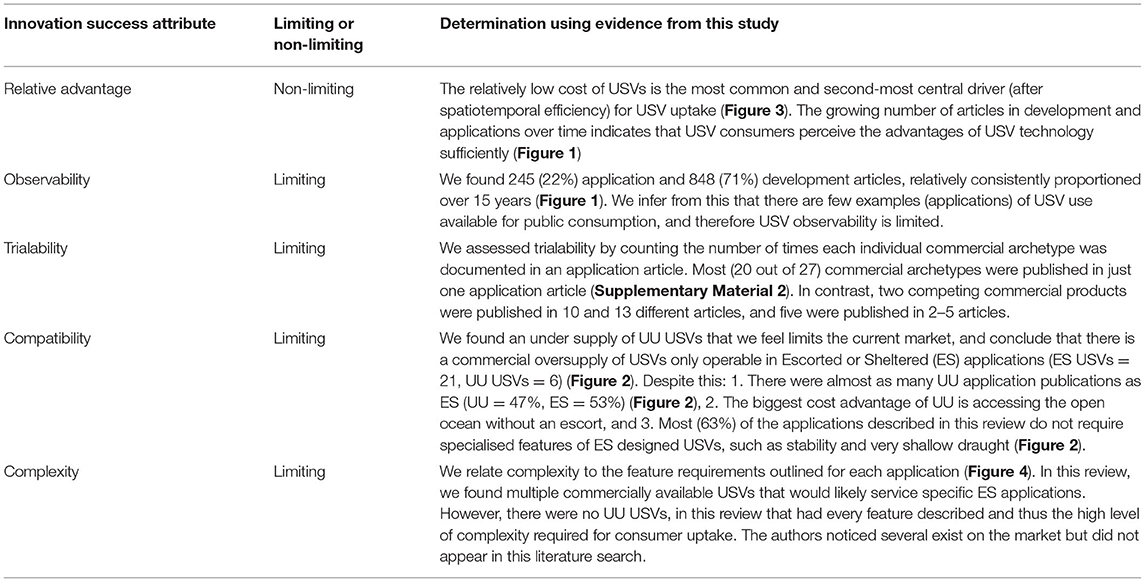
Table 2. Technology diffusion innovation success attributes that are limiting or non-limiting to the diffusion of USV technology.
Finding Our Way to Complex Multi-Use USVs
Global demand for USVs has increased substantially since crewed offshore operations worldwide were plagued by COVID-19 lockdowns (Depellegrin et al., 2020). In response, USVs were rapidly adopted to temporarily fill the void of crewed operations [National Oceanic and Atmospheric Administration (NOAA), 2020]. COVID-19 made the risk of investment (Gu et al., 2020) and confidence (Costanzi et al., 2020) in the new technology permissible, in exchange for personnel proximity, a dichotomy that is accelerating an already anticipated expansion and commercialisation of USVs (Manley, 2016).
System interoperability between different types of remotely operated vehicles must be realised for cross-disciplinary collaborations, for example, standard control interfaces, software and protocols (Costanzi et al., 2020). Cybersecurity, spoofing and jamming pose significant threats to the normalisation of USVs (Dobryakova et al., 2016; Hogg and Ghosh, 2016). The lagging development of legal, regulatory frameworks must also be overcome for the small to medium commercial USV market to thrive (Ferreira et al., 2009).
A notable limitation to this study is the few military and defence related applications captured in the literature search. Classified and detailed descriptions of military and defence operations are unlikely to be described in peer-reviewed articles. There is an opportunity to explore further the patterns of consumer applications and drivers based on grey literature encompassing military USV uptake.
Autonomy and USVs
Most institutional definitions of full autonomy agree that the platform should operate without human intervention and automatically adapt to environmental and operational conditions (Committee on Coast Guard Maritime Domain Awareness, 2020). This includes adhering to COLREGs (1972) as outlined in Campbell et al. (2012) for a surface vessel. While there were no fully autonomous surface vessels described amongst the 245 application articles, it is possible that the drivers for use, and the factors limiting their uptake, are different to the USVs described in this article. We suggest policy/regulations and technology are fundamental development limitations for fully autonomous surface vessels. Policy and regulations might prevent developers from testing fully autonomous surface vessel prototypes in the open ocean, limiting trialability and observability (pers. comm. Melanie Olsen, Australian Institute of Marine Science).
We identified that the desire for full autonomy differed amongst end-users. Most of the UU USVs did not appear to strive for full autonomy. On the contrary, human intervention was seen as a necessary step in fulfilling many of the applications, such as search and rescue and offshore surveillance. In all cases, a “human-on-the-loop” was still far cheaper than deploying a crewed vessel for the UU applications alone.
Throughout the application literature, “ASV” and “USV” were the principal terms used interchangeably. We also observed the shift from “unmanned” to “unscrewed” at the end of 2020 for political correctness. Our analysis and extended search using the term “autonomous surface vessels” confirmed that the term “unmanned surface vessel” was a more popular term to describe this technology than “autonomous surface vessel.” Both USVs and ASVs essentially described the same technology, all with varying degrees of autonomy. This finding prompts questions on how the definitions between end-users (industry, academia and government) and manufacturers may influence the uptake of this technology. We believe universally accepted industry-standard definitions for the levels of autonomy will be crucial to help elucidate end-users' needs and provide appropriate direction for developers. We suggest further analysis of the past and current terminology used amongst end-users and manufacturers to quantify this.
In the shipping industry, levels of autonomy are defined by the International Maritime Organisation (outlined in Manley, 2019). Shipping autonomy appears to be motivated by several factors, including overall cost savings and a suggested improvement in safety that comes with eliminating human error (Li and Fung, 2019). Fully autonomous guidance, navigation and control systems appear to be a realistic goal for maritime industries such as shipping and transport, but the technology is not ready for implementation at this stage (Li and Fung, 2019).
Conclusion
The uptake of USVs, built for robust, multidisciplinary offshore surveillance and monitoring, will significantly improve ocean management and protection by significantly reducing access costs. There are few USV archetypes suited to these needs on the current market, despite the apparent interest found in this study. Remote and large ocean nations will benefit substantially from multi-USV collaborations under existing governance frameworks and multinational organisations. Collaborative investment in USV technology will underpin the advancement and diffusion of this innovation, improving our ability to monitor and manage the ocean and improve the livelihoods of many of the most vulnerable coastal communities in the world.
Data Availability Statement
The original contributions presented in the study are included in the article/Supplementary Material, further inquiries can be directed to the corresponding author/s.
Author Contributions
RP undertook conception and design of the study and wrote the first draft of the manuscript. RP, HC, and VU performed the data and statistical analysis. GB, EL, and RG contributed significantly to the development of ideas in the manuscript. RP, EL, VU, GB, RG, and HC wrote and significantly revised sections of the manuscript. All authors contributed to the article and approved the submitted version.
Funding
This study was partly funded by the APRIntern Internship.
Conflict of Interest
RP was employed by a small start-up company developing USVs for military demonstrations. RP omitted any reference to the company or the companies' products in this publication, except in the acknowledgements section.
The remaining authors declare that the research was conducted in the absence of any commercial or financial relationships that could be construed as a potential conflict of interest.
Publisher's Note
All claims expressed in this article are solely those of the authors and do not necessarily represent those of their affiliated organizations, or those of the publisher, the editors and the reviewers. Any product that may be evaluated in this article, or claim that may be made by its manufacturer, is not guaranteed or endorsed by the publisher.
Acknowledgments
The authors thank the two reviewers for critically reading the manuscript and whose comments lead to substantial improvements. RP acknowledges the support of the APRIntern program under which this publication was made possible and the engineering team at Ocius Technology Limited who inspired rich discussions on the future of USVs in the world today.
Supplementary Material
The Supplementary Material for this article can be found online at: https://www.frontiersin.org/articles/10.3389/fmars.2021.736984/full#supplementary-material
References
Agnew, D. J., Pearce, J., Pramod, G., Peatman, T., Watson, R., Beddington, J. R, et al. (2009). Estimating the worldwide extent of illegal fishing. PLoS ONE 4:e4570. doi: 10.1371/journal.pone.0004570
Alcántara-Ayala, I. (2002). Geomorphology, natural hazards, vulnerability and prevention of natural disasters in developing countries. Geomorphology 47, 107–124. doi: 10.1016/S0169-555X(02)00083-1
Ardron, J. A., Rayfuse, R., Gjerde, K., and Warner, R. (2014). The sustainable use and conservation of biodiversity in ABNJ: what can be achieved using existing international agreements? Marine Policy 49, 98–108. doi: 10.1016/j.marpol.2014.02.011
Beal, L. M., Vialard, J., and Roxy, M. K. (2019). IndOOS-2: A roadmap to sustained observations of the Indian Ocean for 2020-2030. p. 206. doi: 10.36071/clivar.rp.4.2019
Beggs, H., Verein, R., Paltoglou, G., Kippo, H., and Underwood, M. (2012). Enhancing ship of opportunity sea surface temperature observations in the Australian region. J. Operat. Oceanogr. 5, 59–73. doi: 10.1080/1755876X.2012.11020132
Bhatti, Y., Basu, R. R., Barron, D., and Ventresca, M. J. (2018). Frugal Innovation: Models, Means, Methods. Cambridge: Cambridge University Press.
Brashares, J. S., Abrahms, B., Fiorella, K. J., Golden, C. D., Hojnowski, C. E., Marsh, R. A., et al. (2014). Wildlife decline and social conflict. Science 345:376. doi: 10.1126/science.1256734
Brown, H. C., Jenkins, L. K., Meadows, G. A., and Shuchman, R. A. (2010). BathyBoat: an autonomous surface vessel for stand-alone survey and underwater vehicle network supervision. Marine Technol. Soc. J. 44, 20–29. doi: 10.4031/MTSJ.44.4.5
Bruwer, C. (2019). Transnational organised crime at sea as a threat to the sustainable development goals: taking direction from piracy and counter-piracy in the Western Indian Ocean. Afr. Sec. Rev. 28, 172–188. doi: 10.1080/10246029.2020.1728352
Campbell, S., Naeem, W., and Irwin, G. W. (2012). A review on improving the autonomy of unmanned surface vehicles through intelligent collision avoidance manoeuvres. Ann. Rev. Control 36, 267–283. doi: 10.1016/j.arcontrol.2012.09.008
Chabot, D. (2018). Trends in drone research and applications as the Journal of Unmanned Vehicle Systems turns five. J. Unmanned Vehicle Syst. 6, vi–xv. doi: 10.1139/juvs-2018-0005
Claudet, J., Bopp, L., Cheung, W. W. L., Devillers, R., Escobar-Briones, E., Haugan, P., et al. (2020). A roadmap for using the UN decade of ocean science for sustainable development in support of science, policy, and action. One Earth 2, 34–42. doi: 10.1016/j.oneear.2019.10.012
Cole, R., Kinder, J., Yu, W., Ning, C. L., Wang, F., and Chao, Y. (2019). Ocean climate monitoring. Front. Marine Sci. 6:503. doi: 10.3389/fmars.2019.00503
Cole, W. (2020). Coast Guard Completes Drone Ship Exercise in Hawaii as Navy Prepares Unmanned Asset Test. Available online at: https://www.staradvertiser.com/2020/11/30/breaking-news/coast-guard-completes-drone-ship-exercise-in-hawaii-as-navy-prepares-unmanned-asset-test/ (accessed Febuary 16, 2021).
COLREGs (1972). Convention on the International Regulations for Preventing Collisions at Sea. London: International Maritime Organization.
Committee on Coast Guard Maritime Domain Awareness (2020). Appendix D of Leveraging Unmanned Systems for Coast Guard Missions: A Strategic Imperative. Washington, DC: Transportation Research Board Special Report, 335.
Costanzi, R., Fenucci, D., Manzari, V., Micheli, M., Morlando, L., Terracciano, D., et al. (2020). Interoperability among unmanned maritime vehicles: review and first in-field experimentation. Front. Robot. AI. 7, 1–15. doi: 10.3389/frobt.2020.00091
Cremers, K., Wright, G., and Rochette, J. (2020). Strengthening monitoring, control and surveillance of human activities in marine areas beyond national jurisdiction: challenges and opportunities for an international legally binding instrument. Marine Policy 122:103976. doi: 10.1016/j.marpol.2020.103976
Cristini, L., Lampitt, R. S., Cardin, V., Delory, E., Haugan, P., O'Neill, N., et al. (2016). Cost and value of multidisciplinary fixed-point ocean observatories. Marine Policy 71, 138–146. doi: 10.1016/j.marpol.2016.05.029
Depellegrin, D., Bastianini, M., Fadini, A., and Menegon, S. (2020). The effects of COVID-19 induced lockdown measures on maritime settings of a coastal region. Sci. Total Environ. 740:140123. doi: 10.1016/j.scitotenv.2020.140123
Dobryakova, L., Lemieszewski, L., and Ochin, E. (2016). The vulnerability of unmanned vehicles to terrorist attacks such as Global Navigation Satellite System spoofing. Sci. J. Maritime Univ. 46, 181–188. doi: 10.17402/135
Dunn, D. C., Jablonicky, C., Crespo, G. O., McCauley, D. J., Kroodsma, D. A., Boerder, K., et al. (2018). Empowering high seas governance with satellite vessel tracking data. Fish Fish. 19, 729–739. doi: 10.1111/faf.12285
Eleftherakis, D., and Vicen-Bueno, R. (2020). Sensors to increase the security of underwater communication cables: a review of underwater monitoring sensors. Sensors 20:737. doi: 10.3390/s20030737
Emery, W. J., Cherkauer, K., Shannon, B., and Reynolds, R. W. (1997). Hull-mounted sea surface temperatures from ships of opportunity. J. Atmos. Oceanic Technol. 14, 1237–1251. doi: 10.1175/1520-0426(1997)014<1237:HMSSTF>2.0.CO;2
Etzkowitz, H., Webster, A., Gebhardt, C., and Terra, B. R.C. (2000). The future of the university and the university of the future: evolution of ivory tower to entrepreneurial paradigm. Res. Policy 29, 313–330. doi: 10.1016/S0048-7333(99)00069-4
Ferreira, H., Almeida, C., Martins, A., Almeida, J., Dias, N., Dias, A., et al. (2009). “Autonomous bathymetry for risk assessment with ROAZ robotic surface vehicle” in Oceans 2009-Europe (Bremen), 1–6.
Ferreira, H., Martins, R., Marques, E., Pinto, J., Martins, A., Almeida, J., et al. (2007). “Swordfish: an autonomous surface vehicle for network centric operations,” in OCEANS 2007 - Europe (Aberdeen), 1–6.
Flannaghan, T., Fueglistaler, S., Held, I. M., Po-Chedley, S., Wyman, B., and Zhao, M. (2014). Tropical temperature trends in atmospheric general circulation model simulations and the impact of uncertainties in observed SSTs. J. Geophys. Res. Atmos. 119, 13–327. doi: 10.1002/2014JD022365
Friedman, W. R., Halpern, B. S., McLeod, E., Beck, M. W., Duarte, C. M., Kappel, C. V., et al. (2020). Research priorities for achieving healthy marine ecosystems and human communities in a changing climate. Front. Mar. Sci. 7:5. doi: 10.3389/fmars.2020.00005
Frizzell-Makowski, L. J., Shelsby, R. A., Mann, J., and Scheidt, D. (2011). “An autonomous energy harvesting station-keeping vehicle for persistent ocean surveillance,” in OCEANS'11 MTS/IEEE (Kona), 1–4.
Fujii, Y., Rémy, E., Zuo, H., Oke, P., Halliwell, G., Gasparin, F., et al. (2019). Observing system evaluation based on ocean data assimilation and prediction systems: on-going challenges and a future vision for designing and supporting ocean observational networks. Front. Mar. Sci. 6:417. doi: 10.3389/fmars.2019.00417
Gu, Y., Goez, J. C., Guajardo, M., and Wallace, S. W. (2020). Autonomous vessels: state of the art and potential opportunities in logistics. Int. Transact. Operat. Res. 1–34. doi: 10.1111/itor.12785
Han, J., Park, J., Kim, T., and Kim, J. (2015). Precision navigation and mapping under bridges with an unmanned surface vehicle. Autonomous Robots 38, 349–362. doi: 10.1007/s10514-015-9419-2
Harris, M., Bhatti, Y., Buckley, J., and Sharma, D. (2020). Fast and frugal innovations in response to the COVID-19 pandemic. Nat. Med. 26, 814–817. doi: 10.1038/s41591-020-0889-1
Hogg, T., and Ghosh, S. (2016). Autonomous merchant vessels: examination of factors that impact the effective implementation of unmanned ships. Aust. J. Maritime Ocean Affairs 8, 206–222. doi: 10.1080/18366503.2016.1229244
Idris, M. H. B. M., Kamarudin, M. A. A. B. C., Sahalan, M. I., Abidin, Z. B. Z., and Rashid, M. M. (2016). “Design and development of an autonomous surface vessel for inland water depth monitoring,” in 2016 International Conference on Computer and Communication Engineering (ICCCE). Kuala Lumpur: IEEE, 177–182.
Johnston, P., and Poole, M. (2017). “Marine surveillance capabilities of the autonaut wave-propelled unmanned surface vessel (USV),” in OCEANS 2017 (Aberdeen), 1–46.
Kilpatrick, K., Podesta, G., and Evans, R. (2001). Overview of the NOAA/NASA advanced very high resolution radiometer Pathfinder algorithm for sea surface temperature and associated matchup database. J. Geophys. Res. Oceans 106, 9179–9197. doi: 10.1029/1999JC000065
Kitowski, Z. (2019). Selection of UUV type ROV equipment and cooperation system with USV “Edredon” in protection tasks of ports and critical objects. Transact. Maritime Sci. 8, 198–204. doi: 10.7225/toms.v08n02.004
Li, B., Page, B. R., Hoffman, J., Moridian, B., and Mahmoudian, N. (2019). Rendezvous planning for multiple AUVs with mobile charging stations in dynamic currents. IEEE Robot. Automat. Lett. 4, 1653–1660. doi: 10.1109/LRA.2019.2896899
Li, C., Weeks, E., Huang, W., Milan, B., and Wu, R. (2018). Weather-induced transport through a tidal channel calibrated by an unmanned boat. J. Atmos. Oceanic Technol. 35, 261–279. doi: 10.1175/JTECH-D-17-0130.1
Li, S., and Fung, K. S. (2019). Maritime autonomous surface ships (MASS): implementation and legal issues. Maritime Business Rev. 4, 330–339. doi: 10.1108/MABR-01-2019-0006
Lindstrom, E., Gunn, J., Fischer, A., McCurdy, A., and Glover, L. K. (2012). A Framework for Ocean Observing. By the Task Team for an Integrated Framework for Sustained Ocean Observing. Paris: UNESCO.
Liu, Z., Zhang, Y., Yu, X., and Yuan, C. (2016). Unmanned surface vehicles: an overview of developments and challenges. Ann. Rev. Control 41, 71–93. doi: 10.1016/j.arcontrol.2016.04.018
Malone, T. C., DiGiacomo, P. M., Gonçalves, E., Knap, A. H., Talaue-McManus, L., and de Mora, S. (2014). A global ocean observing system framework for sustainable development. Marine Policy 43, 262–272. doi: 10.1016/j.marpol.2013.06.008
Manley, J. E. (2016). “Unmanned maritime vehicles, 20 years of commercial and technical evolution,” in OCEANS 2016 MTS/IEEE (Monterey), 1–6.
Manley, J. E. (2019). “Waypoints on the voyage to autonomous ships,” in OCEANS 2019 MTS/IEEE (Seattle), 1–5.
Martin, B., Tarraf, D. C., Whitmore, T. C., DeWeese, J., Kenney, C., Schmid, J., et al. (2019). Advancing Autonomous Systems: an Analysis of Current and Future Technology for Unmanned Maritime Vehicles. Fort Belvoir: RAND Corporation.
McCauley, D. J., Jablonicky, C., Allison, E. H., Golden, C. D., Joyce, F. H., Mayorga, J., et al. (2018). Wealthy countries dominate industrial fishing. Sci. Adv. 4:eaau2161. doi: 10.1126/sciadv.aau2161
Meinig, C., Burger, E. F., Cohen, N., Cokelet, E. D., Cronin, M. F., Cross, J. N., et al. (2019). Public–private partnerships to advance regional ocean-observing capabilities: a saildrone and NOAA-PMEL case study and future considerations to expand to global scale observing. Front. Marine Sci. 6. doi: 10.3389/fmars.2019.00448
National Oceanic and Atmospheric Administration (NOAA) (2020). NOAA, US Navy Will Increase Nation's Unmanned Maritime Systems Operations. Available online : https://www.noaa.gov/media-release/noaa-us-navy-will-increase-nation-s-unmanned-maritime-systems-operations (accessed August 17, 2020).
Negoro, A. H. S., Muhlisin, M. N., Kusumah, M., and Trihartono, A. (2020). Maritime policies in the era of regional autonomy: identifying the challenges. IOP Conf. Ser. Earth Environ. Sci. 485:012030. doi: 10.1088/1755-1315/485/1/012030
Noonan, M., and Williams, E. (2016). Combating maritime transnational crime: an Australian perspective. J. Indian Ocean Region. 12, 46–51. doi: 10.1080/19480881.2016.1138711
Norgren, P., Ludvigsen, M., Ingebretsen, T., and Hovstein, V. E. (2015). “Tracking and remote monitoring of an autonomous underwater vehicle using an unmanned surface vehicle in the Trondheim fjord,” in OCEANS 2015 - MTS/IEEE (Washington, DC), 1–6.
Pastore, T., and Djapic, V. (2010). Improving autonomy and control of autonomous surface vehicles in port protection and mine countermeasure scenarios. J. Field Robot. 27, 903–914. doi: 10.1002/rob.20353
Patterson, M. C. L., Mulligan, A., and Boiteux, F. (2013). “Safety and security applications for micro-unmanned surface vessels,” in 2013 OCEANS (San Diego), 1–6.
Penna, N. T., Morales Maqueda, M. A., Martin, I., Guo, J., and Foden, P. R. (2018). Sea surface height measurement using a GNSS wave glider. Geophys. Res. Lett. 45, 5609–5616. doi: 10.1029/2018GL077950
Pinsky, M. L., Reygondeau, G., Caddell, R., Palacios-Abrantes, J., Spijkers, J., and Cheung, W. W.L. (2018). Preparing ocean governance for species on the move. Science 360:1189. doi: 10.1126/science.aat2360
Powers, M., Begg, Z., Smith, G., and Miles, E. (2019). Lessons from the Pacific Ocean portal: Building Pacific Island capacity to interpret, apply, and communicate ocean information. Front. Mar. Sci. 6:476. doi: 10.3389/fmars.2019.00476
Raber, G. T., and Schill, S. R. (2019). A low-cost small unmanned surface vehicle (sUSV) for very high-resolution mapping and monitoring of shallow marine habitats. Remote Sens. Ocean Sea Ice Coast. Waters Large Water Regions 11150:1115004. doi: 10.1117/12.2531361
Real-Arce, D. A., Barrera, C., Hernández, J., and Llinás, O. (2015). “Ocean surface vehicles for maritime security applications (The PERSEUS project),” in OCEANS 2015 (Genova), 1–4.
Ryabinin, V., Barbière, J., Haugan, P., Kullenberg, G., Smith, N., McLean, C., et al. (2019). The UN decade of ocean science for sustainable development. Front. Mar. Sci. 6:470. doi: 10.3389/fmars.2019.00470
Sem, G. (2007). Vulnerability and Adaptation to Climate Change in Small Island Developing States. New York, NY: United Nations Development Programme.
Shimono, S., Matsubara, O., Toyama, S., Nishizawa, U., Kato, S., and Arisumi, H. (2015). “Development of underwater inspection system for dam inspection,” in OCEANS 2015 - MTS/IEEE (Washington, DC), 1–6.
Siddle, E., Heywood, K., and Webber, B. (2021). First measurements of ocean and atmosphere in the Tropical North Atlantic using Caravela, a novel AutoNaut uncrewed surface vessel. Weather 76, 200–204. doi: 10.1002/wea.4004
Smith, N., Kessler, W. S., Cravatte, S., Sprintall, J., Wijffels, S., Cronin, M. F., et al. (2019). Tropical pacific observing system. Front. Mar. Sci. 6:31. doi: 10.3389/fmars.2019.00031
Sokimi, W., and Aqorau, T. (2019). The Pacific Islands Forum Fisheries Agench: 40 Years of Successful Regional Cooperation. Department of Pacific Affairs, Canberra: Australian National University.
Steimle, E. T., Murphy, R. R., Lindemuth, M., and Hall, M. L. (2009). “Unmanned marine vehicle use at hurricanes Wilma and Ike” in OCEANS 2009 (Biloxi), 1–6.
Sutton, A. J., Williams, N. L., and Tilbrook, B. (2021). Constraining southern ocean CO2 flux uncertainty using uncrewed surface vehicle observations. Geophys. Res. Lett. 48:e2020GL091748. doi: 10.1029/2020GL091748
Tikanmäki, I., and Ruoslahti, H. (2017). Increasing cooperation between the European maritime domain authorities. Int. J. Environ. Sci. 2, 392–399.
Toyoda, T., Fujii, Y., Kuragano, T., Matthews, J. P., Abe, H., Ebuchi, N., et al. (2015). Improvements to a global ocean data assimilation system through the incorporation of Aquarius surface salinity data. Quar.J. R. Meteorol. Soc. 141, 2750–2759. doi: 10.1002/qj.2561
UN Security Council (2019). “Maintenance of international peace and security. transnational organised crime at sea as a threat to international peace and security.” in 8457th meeting of the United Nations Security Council Report No. S/PV.8457 (New York: UNSC).
United Nations (2015). Transforming Our World: The 2030 Agenda for Sustainable Development (New York).
United Nations (2021). Office on Drugs and Crime. Available online at: https://www.unodc.org/unodc/about-unodc/campaigns/fisheriescrime.html (accessed March 25, 2021).
Vazquez-Cuervo, J., Gomez-Valdes, J., and Bouali, M. (2020). Comparison of satellite-derived sea surface temperature and sea surface salinity gradients using the Saildrone California/Baja and North Atlantic Gulf stream deployments. Remote Sens. 12:1839. doi: 10.3390/rs12111839
Visbeck, M. (2018). Ocean science research is key for a sustainable future. Nat. Commun. 9:690. doi: 10.1038/s41467-018-03158-3
Weaver, J., and Dunlap, D. (2016). “Hell bay trials: a multinational collaborative marine autonomy field experimentation program,” in OCEANS 2016 MTS/IEEE (Monterey), 1–7.
Weller, R. A., Baker, D. J., Glackin, M. M., Roberts, S. J., Schmitt, R. W., Twigg, E. S., et al. (2019). The challenge of sustaining ocean observations. Front. Mar. Sci. 6:105. doi: 10.3389/fmars.2019.00105
White, W., and Bernstein, R. (1979). Design of an oceanographic network in the midlatitude North Pacific. J. Phys. Oceanogr. 9, 592–606. doi: 10.1175/1520-0485(1979)009<0592:DOAONI>2.0.CO;2
Witbooi, E., Ali, K.-D., Santosa, M. A., Hurley, G., Husein, Y., Maharaj, S., et al. (2020). Organised crime in the fisheries sector threatens a sustainable ocean economy. Nature 588, 48–56. doi: 10.1038/s41586-020-2913-5
Wood, D., and Weigel, A. (2011). Building technological capability within satellite programs in developing countries. Acta Astronautica 69, 1110–1122. doi: 10.1016/j.actaastro.2011.06.008
World Meteorological Organisation (2016). Climate Predictions for Small Island Nations: Managing Risks, Maximising Opportunities. Geneva: World Meteorological Organisation.
Zaghi, S., Dubbioso, G., Broglia, R., and Muscari, R. (2016). Hydrodynamic characterisation of USV vessels with innovative SWATH configuration for coastal monitoring and low environmental impact. Transport. Res. Proc. 14, 1562–1570. doi: 10.1016/j.trpro.2016.05.121
Zhang, Y., Rueda, C., Kieft, B., Ryan, J. P., Wahl, C., O'Reilly, T. C., et al. (2019). Autonomous tracking of an oceanic thermal front by a Wave Glider. J. Field Robot. 36, 940–954. doi: 10.1002/rob.21862
Keywords: USV, ROV, maritime governance, ocean monitoring, ocean surveillance, large ocean nations
Citation: Patterson RG, Lawson E, Udyawer V, Brassington GB, Groom RA and Campbell HA (2022) Uncrewed Surface Vessel Technological Diffusion Depends on Cross-Sectoral Investment in Open-Ocean Archetypes: A Systematic Review of USV Applications and Drivers. Front. Mar. Sci. 8:736984. doi: 10.3389/fmars.2021.736984
Received: 06 July 2021; Accepted: 13 December 2021;
Published: 06 January 2022.
Edited by:
Justin Manley, Independent Researcher, Boston, MA, United StatesReviewed by:
Matthew Dunbabin, Queensland University of Technology, AustraliaJulie Angus, Open Ocean Robotics, Canada
Copyright © 2022 Patterson, Lawson, Udyawer, Brassington, Groom and Campbell. This is an open-access article distributed under the terms of the Creative Commons Attribution License (CC BY). The use, distribution or reproduction in other forums is permitted, provided the original author(s) and the copyright owner(s) are credited and that the original publication in this journal is cited, in accordance with accepted academic practice. No use, distribution or reproduction is permitted which does not comply with these terms.
*Correspondence: Ruth G. Patterson, cnV0aC5wYXR0ZXJzb25AY2R1LmVkdS5hdQ==