- 1Faculty of Fisheries and Protection of Waters, South Bohemian Research Center of Aquaculture and Biodiversity of Hydrocenoses, Research Institute of Fish Culture and Hydrobiology, University of South Bohemia in České Budějovice, České Budějovice, Czechia
- 2Faculty of Science, Institute of Chemistry and Biochemistry, University of South Bohemia in České Budějovice, České Budějovice, Czechia
- 3BioCeV - Institute of Microbiology of the CAS, Vestec, Czechia
- 4Orekhovich Institute of Biomedical Chemistry, Moscow, Russia
- 5Department of Molecular Sciences, Swedish University of Agricultural Sciences, Uppsala, Sweden
Despite available information on the roles of osmotic pressure, potassium (K+), and calcium (Ca2+) in activation of Eurasian burbot spermatozoon motility, the changes in milt biochemical composition and mechanisms underlying their activation at temperatures above optimal spawning temperature is still unclear. We explored spermatozoon swelling, lipid composition and proteome in relation to osmolality and temperature of swimming medium. The result revealed that temperature increment from 4 to 30°C increases burbot spermatozoa vulnerability to osmotic pressure, decreasing motility in extremely hypotonic media, and the presence of Ca2+ decreases swelling of spermatozoa. No spermatozoon swelling was observed in non-ionic isotonic media at all studied temperatures. A role of swelling in activation of spermatozoa motility at 30°C was rejected. No differences were found in protein profile and lipid composition with respect to activation of burbot spermatozoa at 30°C. Burbot spermatozoon activation occurs at 30°C without modification of the spermatozoon membrane. Elucidation of the intrinsic signaling pathway of burbot spermatozoon spontaneous activation requires further study.
Introduction
The Eurasian burbot Lota lota (L.) is the only gadiform fish inhabiting fresh water (Scott and Crossman, 1973) and spawning in midwinter in a light-limited under-ice environment (McPhail, 1997). To prevent burbot extinction, restoration program and production of fry has been complemented (Babiak et al., 1998; Hardy et al., 2008). In this context, several studies have been conducted on different aspects of burbot reproduction (Kucharczyk et al., 1998; Lahnsteiner et al., 2004) and fry rearing (Wolnicki et al., 2002; Binner et al., 2008; Żarski et al., 2009). On the other hand, the large interest in restocking and market-sized production of burbot has led to controlled reproduction for conservation purposes and commercial aquaculture (Żarski et al., 2010; Wocher et al., 2012). In order to implement artificial insemination for this species, there is a need for greater understanding of its reproductive biology (Zuccarelli et al., 2007; Worthington et al., 2010).
Spermatozoa of freshwater fish are immotile in seminal fluid and in media with similar osmolality and ionic composition (Stoss, 1983; Lahnsteiner et al., 1995). Osmotic pressure (Morisawa et al., 1983a; Butts et al., 2013) and potassium (K+) ions (Morisawa et al., 1983b; Dziewulska and Pilarska, 2018) are key factors managing two main start-up pathways that activate spermatozoon motility in externally fertilizing fishes. The initiation of spermatozoon motility is also dependent on the presence of calcium (Ca2+) to overcome the inhibitory effect of the K+ ions (Baynes et al., 1981; Linhart et al., 2002; Cosson, 2010). Although transition of fish spermatozoa from an immotile to motile state occurs within a fraction of a second (Dzyuba and Cosson, 2014), it is associated with a cascade of cellular events related to changes in milt proteome (Dietrich et al., 2019), bioenergetics (Dzyuba and Cosson, 2014), and peroxidation processes (Dzyuba et al., 2015; Dadras et al., 2016).
Certain physical properties of the cell membrane are active in signal transduction processes (Krasznai et al., 2003). Lipid composition (Lenzi et al., 1996; Muller et al., 2008), particularly cholesterol (Gracia et al., 2010), regulates the reaction of spermatozoa to external cues. For example, lipid bilayers surrounding the blocking mechano-sensitive channels in common carp Cyprinus carpio spermatozoa are likely associated with motility activation (Krasznai et al., 2003). In rainbow trout Oncorhynchus mykiss, resistance of spermatozoa to hypo-osmotic stress is linearly positively correlated with membrane cholesterol, a process accompanied by decrease of membrane fluidity (Muller et al., 2008). This implies that the physiological reaction of spermatozoa to environmental conditions can be modified by aspects of spermatozoon plasma membrane lipid composition. Further, the changes of spermatozoon membrane composition in response to environmental cues at the time of fertilization involve subtle and intricate modifications of the spermatozoon membrane properties such as alterations of protein composition (Leahy and Gadella, 2011). Milt proteins, as targets of redox-dependent modifications depending on the level of ROS, are involved in activation/inactivation of signaling pathways with fundamental roles in spermatozoa vital functions such as motility (O’Flaherty and Matsushita-Fournier, 2017).
Previous studies of burbot have reported specific and unique properties of spermatozoa. Findings regarding triggering of spontaneous spermatozoon activation in seminal fluid by increased temperature have been contradictory, including no activation with temperature rise from 2 to 10°C (Zuccarelli et al., 2007), spontaneous activation at temperatures >5°C (Lahnsteiner et al., 1997), and spontaneous activation at 20°C with maximum motility rate at 30°C (Dadras et al., 2019). These reports show that burbot spermatozoon motility can be activated by increasing temperature, a unique trait among fishes. This type of spermatozoon motility activation is mediated by Ca2+ in the extracellular environment (Dadras et al., 2019). The relationship of motility activation to changes in biochemical and physiological properties of milt at higher temperatures is unclear, hindering understanding of the influence of temperature on burbot spermatozoa. Therefore, the goal of the present study was to determine European burbot spermatozoon motility rate, swelling, lipid composition, and proteome in relation to the osmolality and temperature of the swimming medium.
Materials and Methods
Three-year-old wild L. lota males [mean ± SD total length (TL) = 237 ± 27 mm, mass = 106 ± 24 g] were maintained in a flow-through system at the fish facility of the University of South Bohemia, Faculty of Fisheries and Protection of Waters. Conditions were ambient light and natural water temperature that gradually decreased from 18–22°C in summer to 4–5°C in winter. Milt was stripped from anesthetized males (100 mg l–1 MS 222; exposure time 7–10 min) (Svačina et al., 2016) by abdominal massage and stored in dry 5 ml vials on ice before and during the experiments. Spermatozoa samples with >90% motility were used to assess spermatozoon motility (n = 5), swelling (n = 5), lipid composition (n = 5), and protein profile (n = 5).
Media and Temperature Used for Spermatozoon Motility and Cell Volume Assessment
For evaluation of spermatozoon motility and cell volume changes, solutions with osmolality in range of 11–280 mOsm kg–1 were prepared including seminal fluid (SF) according to Dadras et al. (2019), pH 8.1, 280 mOsm kg–1; 10 mM Tris-HCl buffer, pH 8.5, 125 mM NaCl, 2 mM KCl, and 1 mM Ca2+, 297 mOsm kg–1 as ionic isotonic medium (AM); 10 mM Tris-HCl buffer, pH 8.5, 270 mM sucrose, 263 mOsm kg–1 as non-ionic isotonic medium (Suc 270); 10 mM Tris-HCl buffer, pH 8.5, 270 mM sucrose, and 1 mM CaCl2, 263 mOsm kg–1 as non-ionic isotonic medium (Suc 270 + Ca2+); 10 mM Tris-HCl buffer, pH 8.5,11 mOsm kg–1 as ionic hypotonic medium (Tris); 10 mM Tris-HCl buffer and 1 mM CaCl2, pH 8.5, 11 mOsm kg–1 as ionic hypotonic medium (Tris + Ca2+); 10 mM Tris-HCl buffer, pH 8.5, and 100 mM sucrose, 128 mOsm kg–1 as non-ionic hypotonic medium (Suc 100); 10 mM Tris-HCl buffer, pH 8.5, 100 mM sucrose, and 1 mM CaCl2, 128 mOsm kg–1 as non-ionic hypotonic medium (Suc 100 + Ca2).
The temperatures used in the present study ranged from 4°C (the temperature close to natural spawning temperature for burbot) to 30°C (the temperature that activation of spermatozoa in SF occurred with the highest motility), including 4, 10, 20, and 30°C.
Motility Analysis
Burbot spermatozoon motility was recorded for 60 s in all media except AM at 4°C (the temperature close to natural spawning temperature for burbot), 10, 20, and 30°C (the temperature at which spermatozoon in SF showed the highest motility rate). Sucrose as a non-ionic component was used to assess the influence of osmotic pressure at different temperatures regardless of ion concentration included in ionic media such as AM. Motility analysis was carried out following milt dilution in target media (∼1:10,000) mixed with the tip of an insulin syringe needle on 24 mm × 32 mm slides (ISO 8037/I, Thermo Scientific1). The spermatozoon motility was monitored for 60 s from 15 s post-activation using a CCD video camera (Sony, SSCDC50AP2) mounted on an inverted microscope equipped with a cooling stage (Olympus IX833) and a 20 × NIC contrast objective lens. Trials were conducted in triplicate.
Thermoblock (HLC BO50/154) and a copper-constantan thermocouple (Omega, L-044T, Taiwan) equipped with a data logger thermometer (Omega, HH1275) were used to adjust and record the temperature during motility, respectively. The recorded videos were analyzed using ImageJ software6 and a computer assisted sperm analyzer (CASA) plugin (Wilson-Leedy and Ingermann, 2007) upgraded according to Purchase and Earle (2012) to obtain individual spermatozoon motility parameters. The representative data of spermatozoon motility including curvilinear velocity (VCL, time-averaged velocity of the spermatozoon head along its actual curvilinear path, μm s–1) and linearity [LIN, defined as straight line velocity (VSL) per velocity average path (VAP), %] were collected from more than 40,000 spermatozoa. Spermatozoa with VCL < 10 μm s–1 were considered immotile and omitted from analysis.
Assessment of Spermatozoon Volume (Swelling)
Assessment of spermatozoon volume change was carried out according to Dzuba et al. (2001) and Bondarenko et al. (2013). Briefly, optical density of the milt suspension was measured using a spectrophotometer (SPECORD 210; Analytic Jena AG) equipped with a thermostat-controlled cell chamber in a 1 cm light path cuvette at wavelength of 500 nm. The milt:medium dilution rate ranged from 1:200 to 1:150 to obtain initial absorbance near 0.5. Seminal fluid was not included as a medium, since obtaining a volume sufficient for measurement was not feasible. Instead, ionic (AM) and non-ionic (Suc 270 and Suc 270 + Ca2+) media with osmolality close to that of SF were used. Immediately after adding milt to the target solutions, the cuvette was placed in the spectrophotometer and optical density every 0.5 s for 60 s was recorded. The measurements were conducted in triplicate for each milt sample.
To illustrate the dynamics of spermatozoon volume change, the spermatozoon swelling rate, a value representing the change of relative optical density (ROD), was calculated according to Dzuba and Kopeika (2002) and Bondarenko et al. (2013) based on the equation
where OD0 is the value of initial optical density immediately after adding milt to the target solutions, and ODn is the value of optical density at each time point. The ROD at 60 s post-activation (ROD60) was used for evaluation of spermatozoon swelling rate at the end of motility.
Analysis of Lipid Composition
To compare spermatozoon lipid composition of normally activated spermatozoa with that activated at high temperature, analysis of spermatozoon lipid composition was conducted at 4 and 30°C in milt diluted in SF and AM. A 150 μL milt sample from each of five males was diluted with 1,350 μL target media and incubated at 4 and 30°C for 2 min, the maximum time required for cessation of progressive movement. The milt samples were centrifuged at 1,000 × g for 5 min at 4°C, and pellets were used for spermatozoon lipid composition analysis.
Fat Extraction
Lipid extraction was carried out according to Hara and Radin (1978) with a slight modification for fish spermatozoa. Solvents and chemicals used were obtained from Merck (Darmstadt, Germany). The sample was weighed and homogenized in hexane-isopropanol (3:2, v/v). The homogenized sample was placed in a centrifuge tube, and 6.67% of Na2SO4 was added and centrifuged at 2,737 × g for 5 min. The upper phase of the homogenate containing lipids was transferred into pre-weighed tubes and evaporated using nitrogen. The weighed samples were dissolved in hexane and stored at −80°C until further analysis.
Fatty Acid Analysis
To investigate fatty acid (FA) composition, methylation of total lipids was performed using a combination of NaOH and boron trifluoride-methanol complex according to Appelqvist (1968). Fatty acid analysis was performed using gas chromatography (Trace Ultra FID; Thermo Scientific, Milan, Italy) on a BPX-70 50 m fused silica capillary column (i.d. 0.22 mm, 0.25 μm film thickness, SGE, United States) as described by Sampels et al. (2005). The FAs were identified by comparison of sample retention times with those of the standard mixture GLC-68-A (Nu-Chek Prep, Elysian, United States) and other previously validated standards (Nu-Chek Prep, Elysian, United States; Larodan, Sweden).
Lipid Class Composition
The procedures of Olsen and Henderson (1989) were used for separation of major lipid classes using a mobile phase consisting of hexane, diethyl ether, and acetic acid (85:15:2, v/v/v). Separation and quantification of phospholipid classes were carried out based on protocol described by Olsen and Henderson (1989) using a mobile phase consisting of 10 mL 0.25% KCl, 10 mL methanol, 25 ml chloroform, 25 mL methyl-acetate, and 25 ml isopropanol. Samples in both cases were applied with a CAMAG TLC Sampler ATS4 (Camag Switzerland) 2 cm from the base edge of the TLC plates (20 cm × 10 cm; Silica gel 60; 0.20 mm layer, Merck, Darmstadt, Germany) in 2 mm bands with an application speed of 250 nL s–1. Nitrogen was used as spray gas. All samples were tested in duplicate, with distance between tracks of 9.8 mm. Separation of the lipid classes was executed with a CAMAG Automatic Developing Chamber 2 (ADC 2) (Camag, Switzerland). To identify major lipid and phospholipid classes, plates were dipped in a solution of 3% cupric acetate in 8% phosphoric acid and charred for 20 min at 160°C. For quantitative determination of the separated lipid classes, the plates were assessed by CAMAG TLC scanner 3 (Camag, Switzerland). Scanning was conducted at 20 mm/sec and data resolution of 100 μm step–1, with a slit dimension of 6.00 mm × 0.45 mm at wavelength of 350 nm. The Savitzky–Golay 7 mode was used for data filtering. The lipid classes were identified by comparison with a standard mix of all lipid classes (TLC 18-4A NuCheck, Prep, Elysian, United States) or single phospholipid standards (Avanti Polar Lipids, Inc., Alabama, United States). WinCats integration software was used for peak integration, and manual baseline and peak correction were applied as required.
Protein Analysis
Preparation of Samples
To prepare samples for protein analysis, separate milt samples from 5 burbot males were diluted in SF and AM (100 μL milt:500 μL medium), incubated for 2 min at 4 and 30°C, and centrifuged at 3,000 × g for 20 min at 4°C.
Protein samples were prepared as described by Xin et al. (2019) with minor modifications. Briefly, spermatozoa were suspended in 50 mM Tris/HCl pH 7.4 supplemented with 1% Triton X-100 and protease inhibitors (Thermo Fisher Scientific, Waltham, MA, United States). Samples were sonicated and vortexed continuously for 1 h, and subsequently centrifuged at 13,000 × g for 10 min at 4°C. The protein concentration was measured using a BCA Protein Assay Kit (Thermo Fisher Scientific, Waltham, MA, United States).
Two D-PAGE Separation
2D-PAGE electrophoresis was conducted according to Xin et al. (2019). The images of gels were captured and digitalized using G:BOX Chemi XX6 system (Syngene, Cambridge, United Kingdom) and analyzed using SameSpots software (TotalLab, Newcastle upon Tyne, United Kingdom). All samples were run at least in triplicate.
In-Gel Digestion and MALDI-MS and MS/MS Analysis
Protein spots of interest were excised from the corresponding SDS-PAGE gel and digested with trypsin according to the protocol by Shevchenko et al. (2006). Obtained peptides were separated using a microgradient device (Rehulka et al., 2018) with gradient of ACN as described (Loginov et al., 2019). MS and MS/MS spectra were acquired using an Autoflex Speed mass spectrometer controlled by flexControl 3.4 software (both Bruker Daltonics). WarpLC 1.3 (Bruker Daltonics, Billerica, MA, United States) was used for automatic measurement of microgradient MALDI fractions. Spectra were processed using flexAnalysis 3.4 and ProteinScape 3.1 software (Bruker Daltonics, Billerica, MA, United States).
Mascot Server 2.4 (Matrix Science, Boston, MA, United States) was used to conduct searches against in-house prepared databases containing protein sequences from the Gadus morhua National Center for Biotechnology Information (NCBI) database (accessed 2018-06-05; 533,418 sequences) supplemented with sequences of common contaminants (Max Planck Institute of Biochemistry, Martinsried, Germany). Parameters used for the database search were enzyme specificity, trypsin, two missed cleavages were allowed; fixed modifications, carbamidomethylation of cysteine; variable modifications, N-terminal protein acetylation and methionine oxidation. Precursor ion tolerance was set at 50 ppm, and the mass tolerance for MS/MS fragment ions was set at 0.5 Da.
Statistical Analysis
Descriptive statistics of motility parameters from more than 40,000 spermatozoa from five fish were assessed. VCL and LIN were calculated as a mean of average values obtained from each male. Data were tested for normality of distribution by the Kolmogorov–Smirnov test and checked for homogeneity of variance. The data of VCL did not meet criteria for homogeneity of variances and were transformed using square root transformation. One-way ANOVA with Tukey’s post hoc test was applied to analyze the effect of temperature and medium on VCL. The transformation failed to satisfy normality assumptions for LIN; thus, analysis of the effect of media and temperature on this parameter was conducted using non-parametric Kruskal–Wallis ANOVA with subsequent multiple comparisons of mean ranks for all groups. To compare lipid composition of spermatozoa diluted in SF and AM at 4 and 30°C, the non-parametric Mann–Whitney U-test was used. Statistical significance was considered at P < 0.05 in all tests.
A principal component analysis (PCA) for lipid composition (fatty acids and lipid classes) at 4 and 30°C in AM and SF was carried out using Unscrambler v. X 10.1 software (Camo Process A/S, Oslo, Norway). All values were weighted with 1/SD. The NIPALS algorithm was applied, and cross validation with three random segments was used as validation model. We identified two principal components (PC) with PC1 and PC2 describing 44 and 16% of data variability. These PCs were presented as scatterplot with 95% confidence ellipses. All analyses and plotting were conducted using STATISTICA [TIBCO Software Inc. (2018). v.13.7 ] and Microsoft Excel software.
Results and Discussion
Effect of Temperature on Spermatozoon Motility Parameters in Relation to Activation Medium Osmolality
General Trends
Motility duration, velocity, and fertilizing ability of fish spermatozoa may be strongly influenced by ambient temperature (Cosson et al., 1985; Billard et al., 1995; Bombardelli et al., 2013; Dadras et al., 2016) and osmolality (Billard et al., 1995; Wilson-Leedy et al., 2009). The osmolality of media used for activation of spermatozoa has a significant effect on ATP level (Boryshpolets et al., 2009). However, still no defined metabolic pathway responsible for these changes has been identified.
Burbot spermatozoon motility behavior at different osmolalities in relation to temperature was investigated in vitro. Trends of changes in spermatozoon VCL and LIN in the six studied media (Tris, Tris + Ca2+, Suc100, Suc 100 + Ca2+, Suc 270 and Suc 270 + Ca2+) are shown in Figures 1, 2, respectively. The trends of spermatozoon VCL and LIN in SF are presented in Supplementary Figures 1,2, respectively. The trend of VCL in spermatozoa diluted in SF (Supplementary Figure 1) was similar to that in non-ionic isotonic medium (Figure 1). The VCL showed nearly identical patterns in Tris and Tris + Ca2+ media (the media with lowest osmolality among those used) with the lowest VCL observed at 30°C and the highest VCL at 4°C during the course of motility (Figure 1). In contrast, in a study of sterlet Acipenser ruthenus spermatozoa, Dadras et al. (2016) observed the highest VCL at a high temperature (24°C) in hypotonic activation medium.
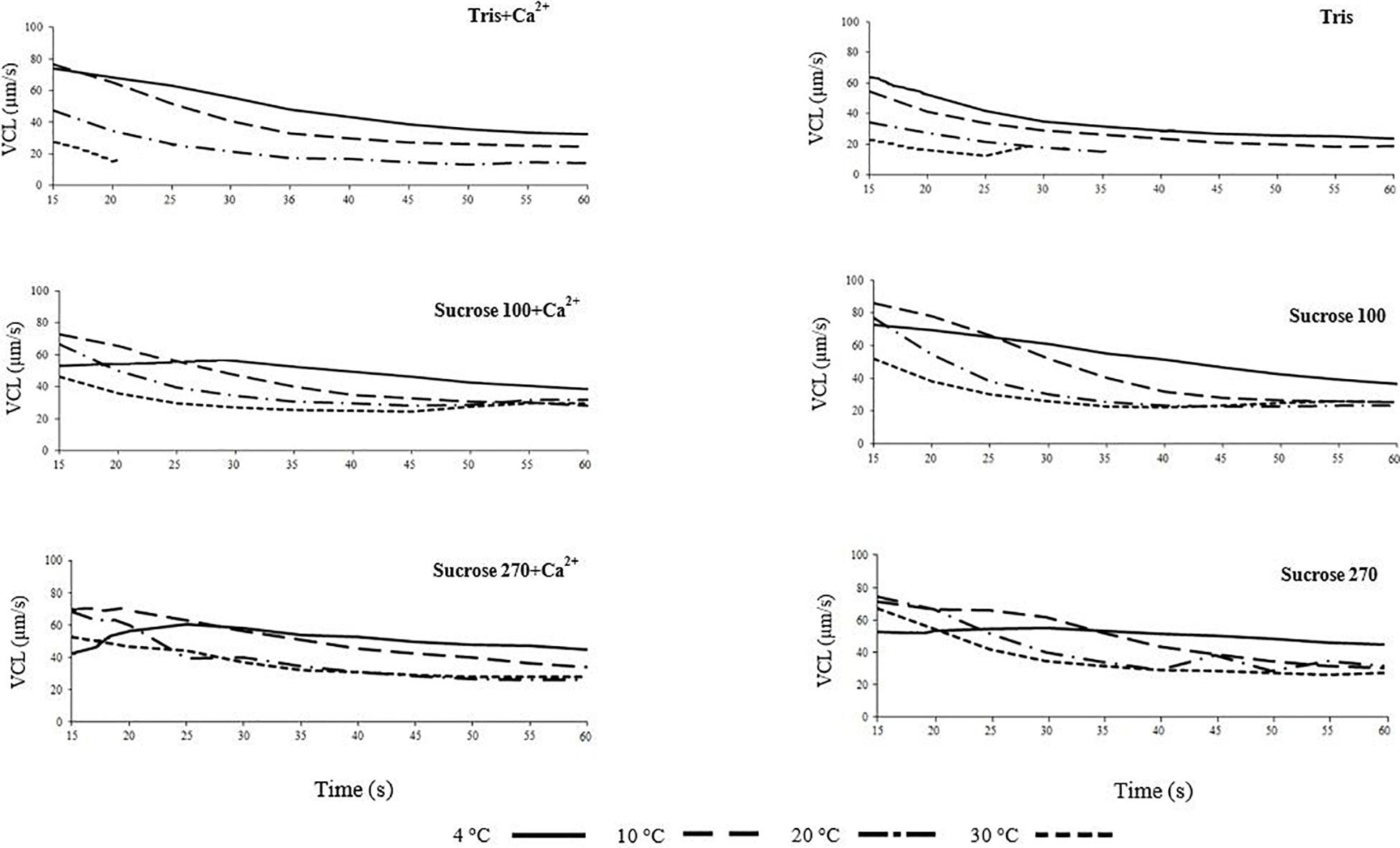
Figure 1. Curvilinear velocity (VCL) of Lota lota spermatozoa in relation to time post-activation (up to 60 s) at 4, 10, 20, and 30°C in Tris, Tris + Ca2+, Suc100, Suc100 + Ca2+, Suc270, and Suc270 + Ca2+.
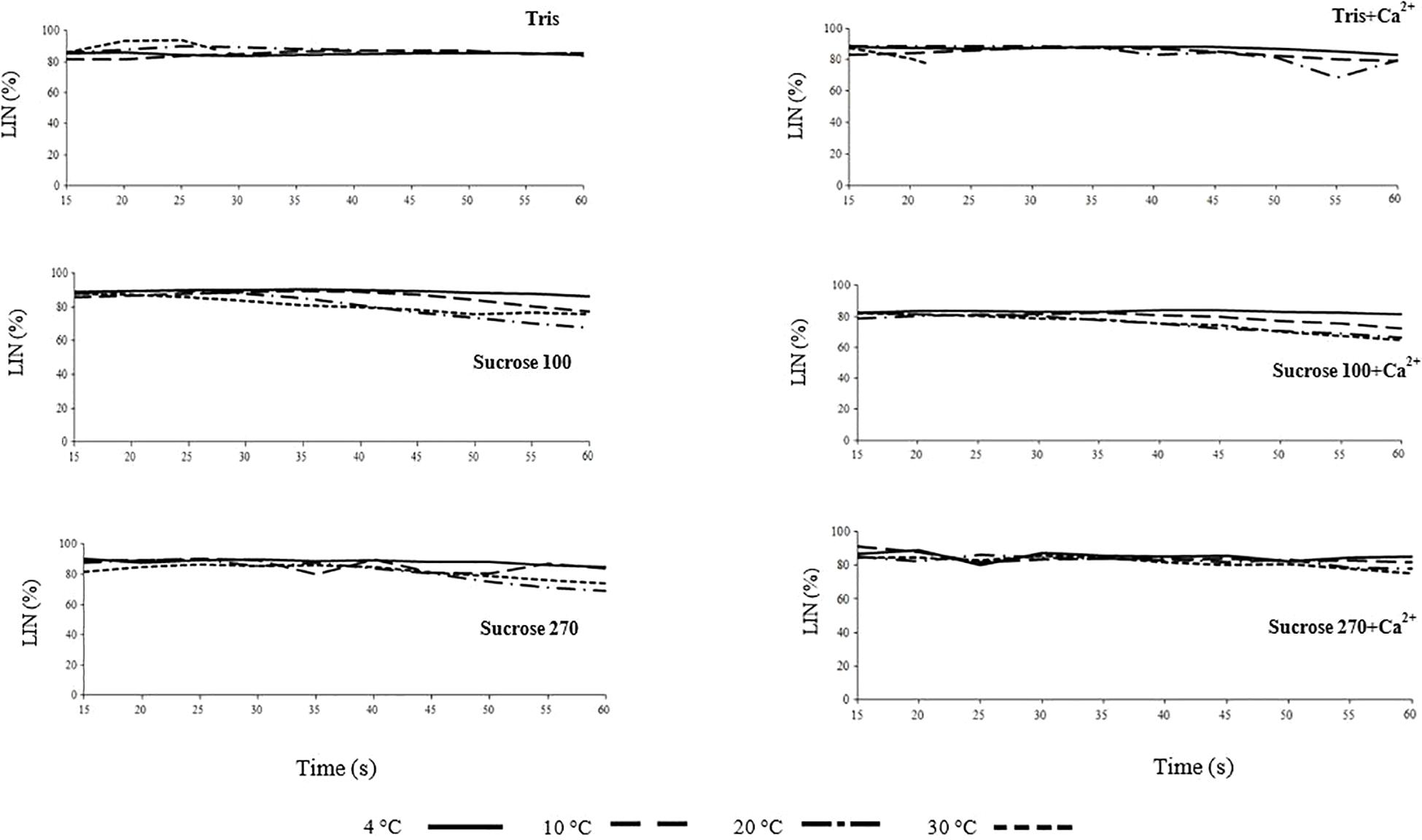
Figure 2. Linearity (LIN) of L. lota spermatozoa in relation to time post-activation (up to 60 s) at 4, 10, 20, and 30°C in Tris, Tris + Ca2+; Suc 100, Suc 100 + Ca2+, Suc 270, and Suc 270 + Ca2+.
The spermatozoa diluted in non-ionic isotonic media (Suc 270 and Suc 270 + Ca2+) showed similar initial VCL at 4 and 30°C, however, at 30°C, a sharp decrease of VCL in Suc 270 and a gradual decrease of VCL in Suc 270 + Ca2+ were observed (Figure 1). This may be due to higher sensitivity of burbot spermatozoa to Ca2+ at high temperature (Dadras et al., 2019).
The spermatozoa diluted in hypotonic media (Tris and Tris + Ca2+) exhibited shorter motility (∼20–30 s) and lower VCL at 30°C compared to media with higher osmolality, possibly due to osmotic shock and consequent hypotonic cell swelling (Perchec-Poupard et al., 1997; Cosson, 2008; Bondarenko et al., 2013). The short duration of spermatozoon motility and lower VCL in Tris and Tris + Ca2+ at 30°C compared to the other temperatures in the same media (Figure 1) might be attributed to greater vulnerability of spermatozoa to osmotic pressure at higher temperatures (Dadras et al., 2019).
The trend of LIN with time post-activation was similar in all studied conditions (Figure 2 and Supplementary Figure 2) and not affected by medium osmolality or temperature, similar to reports for osmolality by Dziewulska and Pilarska (2018).
The VCL of spermatozoa was significantly lower at higher temperatures in hypotonic media (Tris ± Ca2+), particularly at the initial phase of motility, with the maximum at 15 s post-activation at 30°C of 27 and 73 μm s–1 at 4°C. In activation media with higher osmolality, the initial VCL (15 s post-activation) showed smaller differences among the studied temperatures with activation by Sucrose 100 ± Ca2+ (maximum of 55 μm s–1 at 30°C and 73 μm s–1 at 4°C) and by Suc 270 ± Ca2+ (maximum of 68 μm s–1 at 30°C and 52 μm s–1 at 4°C). At 20–25 s post-activation in all studied media, VCL remained at the highest level at 4°C compared to the higher temperatures.
Comparative Effects
After comparison of general trend of VCL and LIN, the motility at 15 s post-activation at different temperatures was compared in each medium as well as at each temperature among target media (Figures 3A,B).
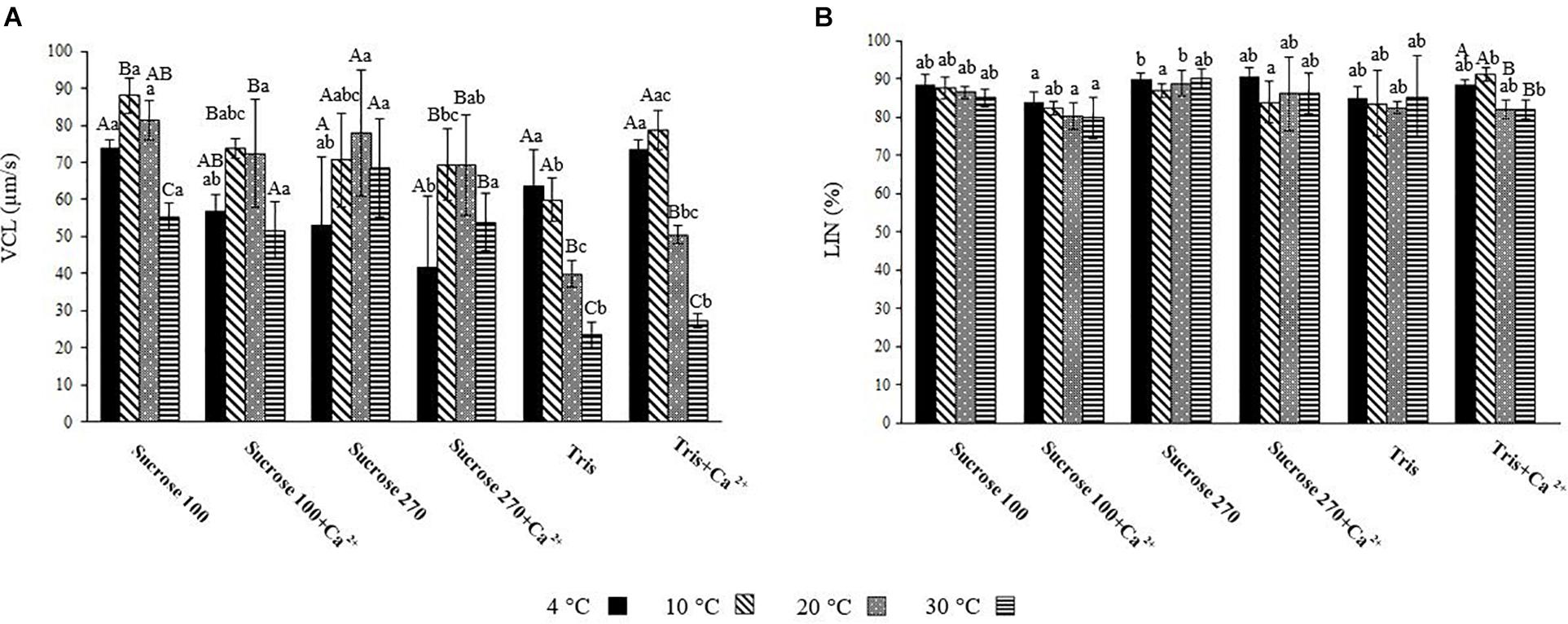
Figure 3. (A) Curvilinear velocity (VCL) of L. lota spermatozoa at 15 s post-activation at 4, 10, 20, and 30°C in Tris; Tris + Ca2+; Suc 100; Suc 100 + Ca2+; Suc 270; and Suc 270 + Ca2+. (B) Linearity (LIN) of L. lota spermatozoa at 15 s post-activation at 4, 10, 20, and 30°C in Tris, Tris + Ca2+; Suc 100, Suc 100 + Ca2+, Suc 270, and Suc 270 + Ca2+. Different uppercase letters indicate significant difference (P < 0.05) among studied temperatures in each solution. Different lowercase letters indicate significant difference (P < 0.05) among studied solutions at each temperature. Data are expressed as mean ± SD. Values with the same superscript do not differ significantly (P > 0.05).
The spermatozoon VCL in Tris medium showed significant differences between low (4°C) and high (20 and 30°C) temperatures (Figure 3A), with the lowest recorded at 30°C (23.33 ± 3.56 μ ms–1). A similar relationship was observed in Tris + Ca2+ (Figure 3A). Comparison of spermatozoon motility in Suc 100 at different temperatures showed VCL at 30°C (55.35 ± 3.82 μm s–1) to be significantly lower than at other temperatures (Figure 3A). Significant differences of VCL in Suc 100 + Ca2+ were revealed between 30 (51.65 ± 7.7 μm s–1) and 10°C (73.68 ± 2.6 μm s–1) as well as between 30 and 20°C (72.4 ± 14.49 μm s–1) (Figure 3A). The VCL in Suc 270 did not differ with temperature (Figure 3A).
At 4°C, the VCL in Suc 270 + Ca2+ (41.58 ± 19.5 μm s–1) was significantly lower than that recorded in Sucr 100, Tris, and Tris + Ca2+ (Figure 3A). At 10°C, spermatozoon VCL in Tris (59.94 ± 5.87 μm s–1) was significantly lower than in Suc100 (88.04 ± 4 μ ms–1) (Figure 3A). In addition, the mean spermatozoon VCL in Tris at 20°C (39.91 ± 3.53 μm s–1) was significantly lower than was recorded in Suc 100, Suc 100 + Ca2+, Suc 270 and Suc 270 + Ca2+ (Figure 3A). The spermatozoa activated in Tris (23.33 ± 3.56 μm s–1) and Tris + Ca2+ (27.16 ± 1.96 μm s–1) showed significantly lower VCL compared to all other groups at 30°C (Figure 3A). Comparison of the LIN in Tris, Tris + Ca2, Suc 100, Suc 100 + Ca2, Sucrose 270, and Suc 270 + Ca2 did not differ with temperature (Figure 3B).
In line with reported threshold of osmolality of sucrose (>450–480 mOsm kg–1) for burbot spermatozoa motility (Dziewulska and Pilarska, 2018), the spermatozoa were motile in non-ionic solutions (Suc 100 and Suc 270 ± Ca2+) in the present study. The results showed that higher temperatures increase burbot spermatozoa vulnerability to osmotic pressure, affecting negatively motility of burbot spermatozoa in extremely hypotonic media (Tris and Tris + Ca2+). This hypotonic signal can induce swelling and efflux of K+, resulting in membrane hyperpolarization (Morisawa et al., 1983a; Takai and Morisawa, 1995; Krasznai et al., 2000).
Effect of Temperature on Spermatozoon Volume in Relation to Osmolality
General Trends
Information about the role of swelling in motility activation of freshwater fish spermatozoa is scarce. A significant correlation of hypo-osmotic swelling with progressive motility of spermatozoon has been reported in mammals (Padrik et al., 2012). Trends of spermatozoon volume changes illustrated by relative optical density in the six studied media (Tris, Tris + Ca2+, Suc 100, Suc 100 + Ca2+, Suc 270, and Suc 270 + Ca2+) are shown in Figure 4. The trend of spermatozoa volume change in AM is presented in Supplementary Figure 3.
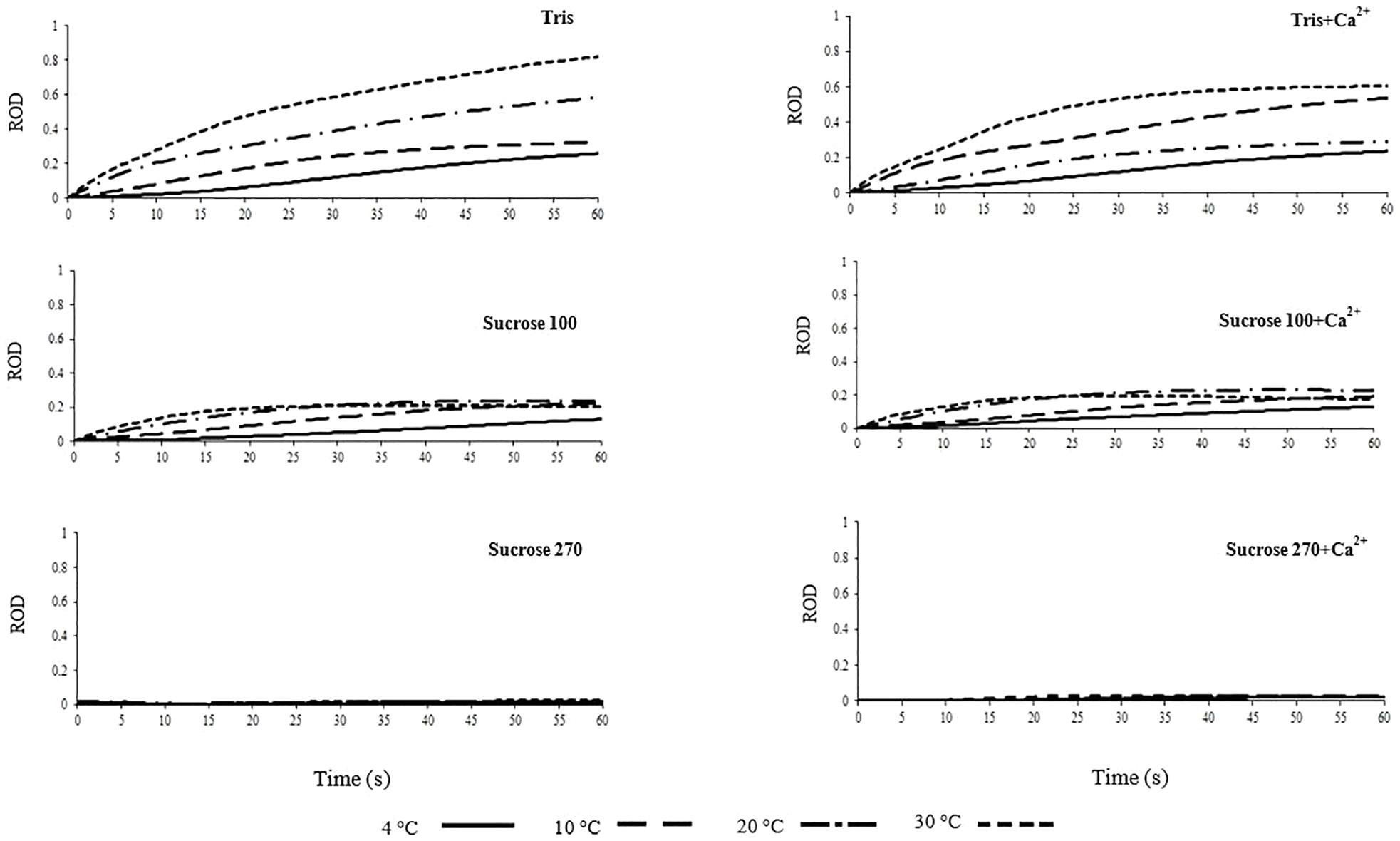
Figure 4. Relative optical density (ROD) of L. lota spermatozoa in relation to time post-activation (up to 60 s) at 4, 10, 20, and 30°C in Tris, Tris + Ca2+, Suc 100, Suc 100 + Ca2+, Suc 270, and Suc 270 + Ca2+.
Spermatozoon swelling in hypotonic conditions might play different roles depending on the spermatozoon motility signaling mode of fish species (Bondarenko et al., 2013). A considerable increase of ROD, representing spermatozoon swelling, in spermatozoa diluted in Tris was observed at 20 and 30°C compared to 4°C (Figure 4). In spermatozoa diluted in Tris + Ca2+, higher ROD was observed at 10 and 30°C (Figure 4), showing temperature dependency of spermatozoon swelling in burbot. The trend of change in ROD in Tris at 30°C was steeper compared to Tris + Ca2+ at the same temperature, suggesting that spermatozoon swelling at high temperature is inhibited by external Ca2+ in burbot.
In Suc100 and Suc 100 + Ca2+, extremely low levels of swelling were observed with slight increase of ROD at all temperatures over the course of 60 s, with no difference among the studied temperatures (Figure 4). No changes of ROD were observed in Suc 270, Suc 270 + Ca2+ (Figure 4) and AM (Supplementary Figure 3) during the 60 s period. This lack of swelling in artificial isotonic media can be generalized to spermatozoa diluted in SF. The swelling of burbot spermatozoa at 4°C, a temperature close to the natural spawning temperature, was not observed at a high level, while higher spermatozoon swelling was detected at 30°C. Swelling of burbot spermatozoa was higher in extremely hypotonic media (Tris and Tris + Ca2+) and increased at high temperatures (20 and 30°C). However, in species with an osmotic-dependent activation mode of spermatozoon motility such as carp, a significant spermatozoon volume change is involved in the motility activation process at natural spawning temperature (Bondarenko et al., 2013).
Comparative Effects
To better visualize differences in spermatozoon swelling, the ROD60 was assessed in each medium at different temperatures as well as at each temperature in different tested media (Figure 5). The trends of ROD in Suc 270, Suc 270 + Ca2+, and AM did not differ with temperature (Figure 4). The results in media in which ROD changed over time included Suc 100, Suc 100 + Ca2+, and Tris and Tris + Ca2+ are presented in Figure 5.
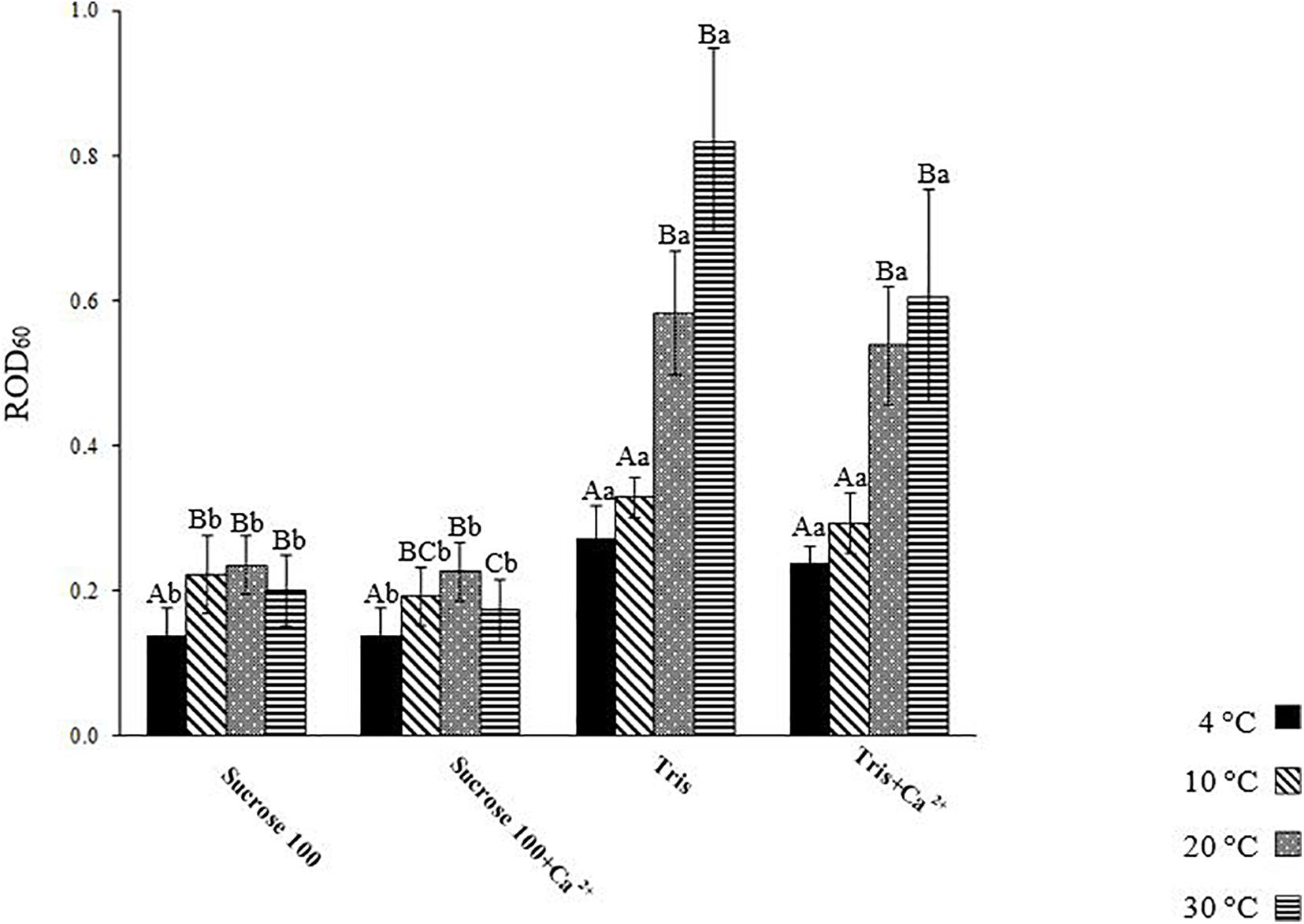
Figure 5. Relative optical density of L. lota spermatozoa at 60 s post-activation (ROD60) at 4, 10, 20, and 30°C in Tris, Tris + Ca2+, Suc 100, and Suc 100 + Ca2+. Different uppercase letters indicate significant difference (P < 0.05) among studied temperatures in each solution. Different lowercase letters indicate significant difference (P < 0.05) among studied solutions at each temperature. Data are expressed as mean ± SD. Values with the same superscript do not differ significantly (P > 0.05).
The level of spermatozoon swelling in Suc 100 and Suc 100 + Ca2+ was significantly lower at 4°C (0.14 ± 0.04) compared to other temperatures (Figure 5). The spermatozoa activated in Tris and Tris + Ca2+ exhibited similar levels of swelling at 4 (Tris: 0.27 ± 0.05 and Tris + Ca2+: 0.24 ± 0.02) as well as at 10°C (Tris: 0.33 ± 0.03 and Tris + Ca2+: 0.29 ± 0.04) (Figure 5). A similar trend in Tris and Tris + Ca2+ was observed at both 20 and 30°C (Figure 5). However, the ROD60 at 4 and 10°C was significantly lower than that observed at 20 and 30°C in both mentioned media, showing a higher swelling rate at high temperatures.
Lower ROD60 was revealed in sperm diluted in Suc 100 and Suc 100 + Ca2+ compared to that diluted in Tris and Tris + Ca2+ at all studied temperatures, showing an increase of spermatozoon volume with exposure to low environmental osmolality (Perchec-Poupard et al., 1997).
Spermatozoon swelling at 60 s was greater than at 30 s in Tris (Figure 4). A short motility duration (∼20–30 s) was observed in hypotonic media at 30°C and the results of changes of ROD showed that swelling continued even after cessation of motility. It is assumed that the decrease of motility duration in hypotonic media might be accompanied by high degree of cell swelling and probably structural changes. However, species-specific modes of spermatozoon motility signaling might affect the role of spermatozoon swelling in hypotonic media.
Swelling of burbot spermatozoa during motility in hypotonic conditions appears to be a temperature-dependent mechanism. No swelling was observed during 60 s motility in ionic and non-ionic isotonic media (Suc 270, Suc 270 + Ca2+, and AM) at any studied temperature, suggesting that spermatozoon swelling is not involved in signaling of spermatozoon motility activation and spontaneous activation of burbot.
Effect of Temperature on Lipid Composition of Spermatozoa in Relation to Osmolality
The physicochemical state of the plasma membrane determines reactions of fish spermatozoa to environmental changes (Engel et al., 2019, 2020). Fluidity of the spermatozoon membrane is influenced by lipid composition and ambient temperature, which can affect the state of the membrane lipids (Pustowka et al., 2000).
Analysis of FA composition of burbot spermatozoa (Table 1) showed no difference in spermatozoa PUFA content among studied conditions. The proportions of mono-unsaturated fatty acids (MUFA) and saturated fatty acids (SFA) in spermatozoa were not affected by temperature or medium (Table 1).
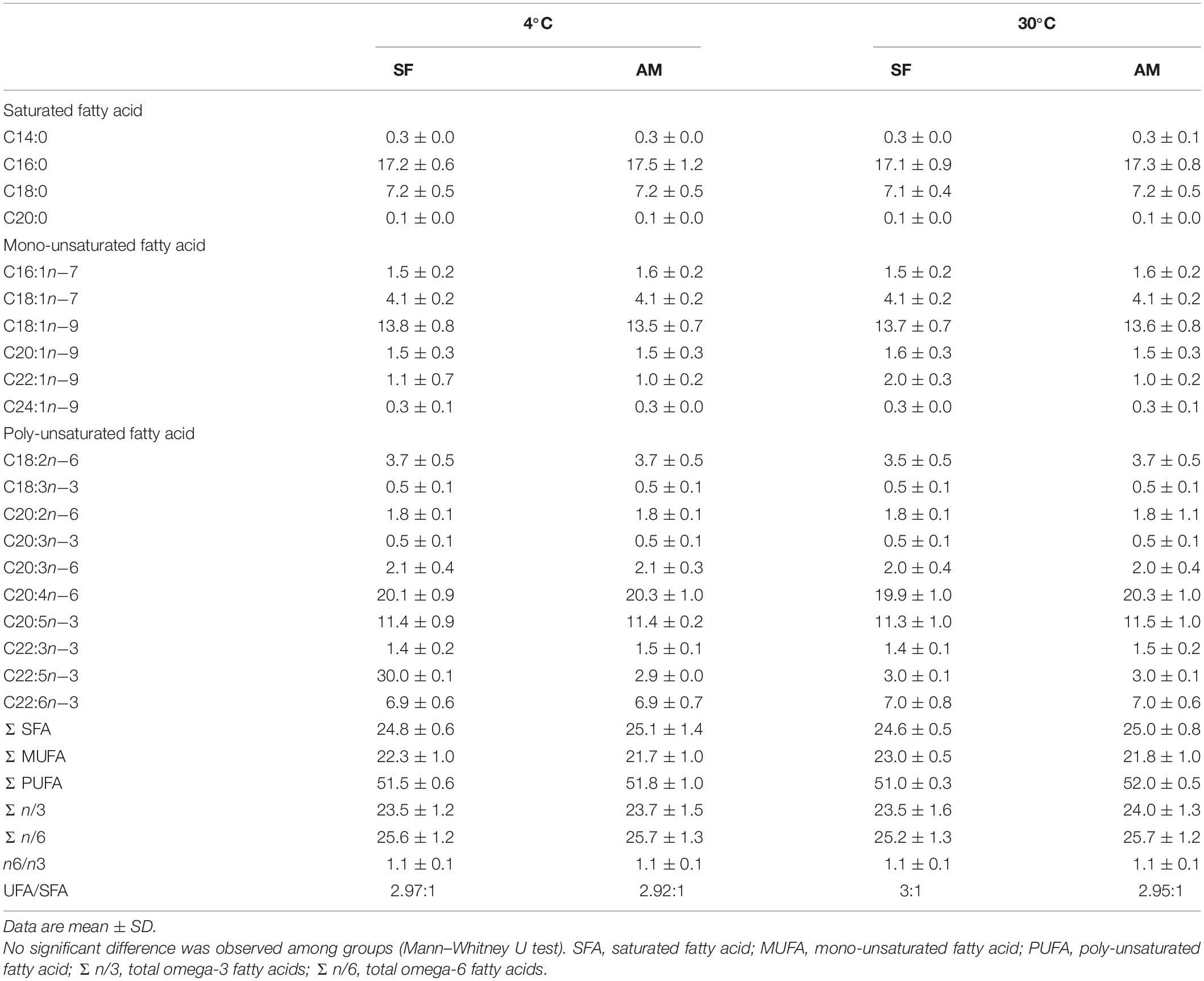
Table 1. Fatty acid composition (%) of Lota lota spermatozoa diluted in seminal fluid (SF) and activation medium (AM) at different in vitro temperatures.
Burbot spermatozoa was characterized by a high PUFA concentration (51.0 ± 0.3–52.0 ± 0.5%), similar to that reported in sterlet and ocellate river stingray Potamotrygon motoro, fishes with external and internal modes of fertilization, respectively (Engel et al., 2020). We identified three PUFAs in wild burbot: C20:3n−3, C20:3n−6, and C22:3n−3. C22:3n−3 has not been previously reported in either wild or cultured burbot. This study also represents the first report of the MUFAs C20:1n−9 (1.5 ± 0.3–1.6 ± 0.3%), C22:1n−9 (1.0 ± 0.2–2.0 ± 0.3%), and C24:1n−9 (0.3 ± 0.0–0.3 ± 0.1%) in burbot spermatozoa.
The identified classes of PLs in burbot spermatozoa is presented Table 2. No differences were observed in phospholipids (PL) in the studied conditions (Table 2).
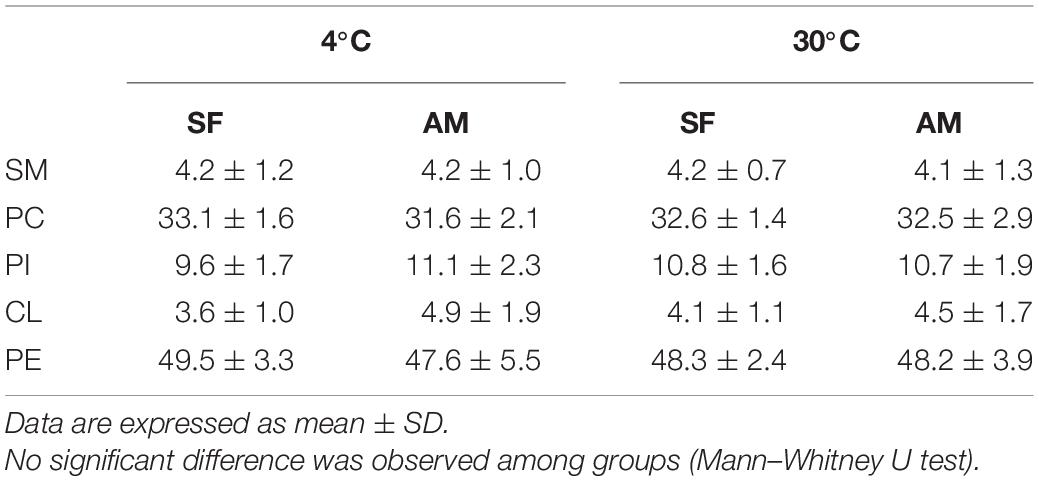
Table 2. Proportions (%) of phospholipid classes including SM, sphingomyelin; PC, phosphatidylcholine; PI, phosphatidylinositol; CL, cardiolipin; and PE, phosphatidylethanolamine in Lota lota spermatozoa diluted in seminal fluid (SF) and activation medium (AM) at different temperatures.
No significant differences were observed in the proportion of total PL, total cholesterol (Chol), and Chol:PL in activated spermatozoa at the studied temperatures (Table 3). The observed proportion of PL (70.4 ± 2.0–71.6 ± 1.4%) was considerably higher than previously reported (58.27 ± 2.38%) in wild burbot (Blecha et al., 2018). The proportion of spermatozoa Chol reported by Blecha et al. (2018) in wild burbot (41.73 ± 2.31%) was higher than Chol measured in the present study (28.4 ± 1.4–29.6 ± 2.0). To summarize the relationship of spermatozoa lipid composition and motility in burbot, scatterplots of principal component scores based on lipid composition of spermatozoa diluted in SF and AM after incubation at 4 and 30°C is presented in Figure 6. No difference was observed among studied conditions in the relationship of spermatozoa lipid composition to motility in burbot. Lipid composition does not appear to play a role in spontaneous activation of burbot spermatozoa.
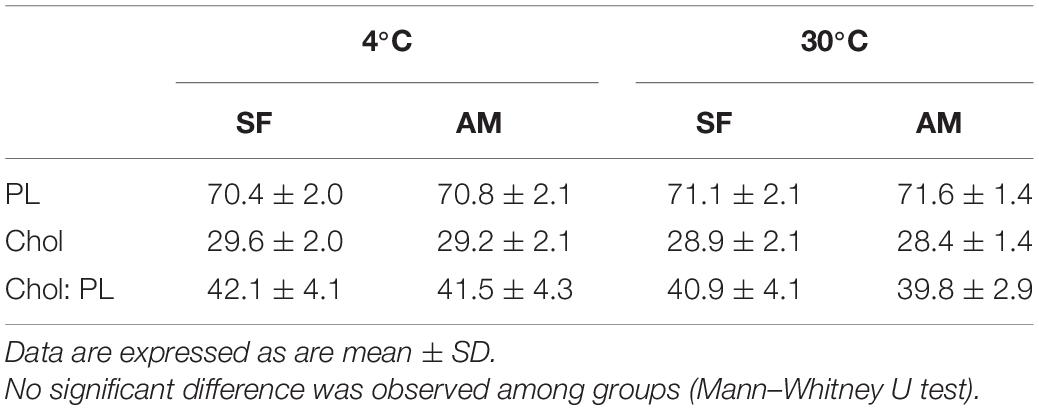
Table 3. Proportions (%) of total phospholipid (PL), total cholesterol (Chol), and Chol: PL in Lota lota spermatozoa diluted in seminal fluid (SF) and activation medium (AM) at different temperatures.
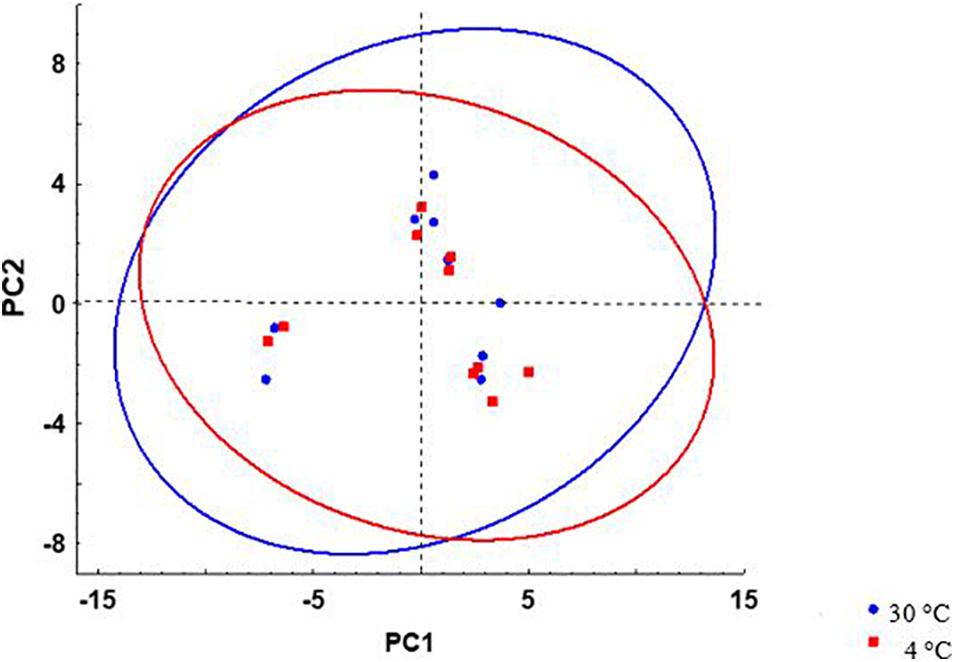
Figure 6. Scatterplots of principal component scores based on lipid composition of L. lota spermatozoa after incubation at 4 and 30°C. 95% prediction interval ellipses for different temperatures are shown by different colors. Data of samples incubated in AM or SF were included in analysis.
Effect of Temperature on Protein Profile of Spermatozoa in Relation to Osmolality
Dispersal of fish species from salt to freshwater involved reproductive adaptation resulting in high variation of genetic information in gametes and consequently high variation in spermatozoa characteristics (Pearse, 1950; Alberts et al., 2002). Proteins of seminal plasma and spermatozoa play a prominent role in adaptation of teleostean and chondrostean fishes to the reproductive environment, being involved in the protection of spermatozoa in the quiescent state (Loir et al., 1990; Zhelenova et al., 2000; Wojtczak et al., 2005, 2007) and the regulation of motility and molecular functions of spermatozoa following impaired spermatozoon function (Li et al., 2010; Zilli et al., 2014; Dietrich et al., 2015).
We did not find differences in the protein profiles of samples incubated at 4 and 30°C using comparison of obtained 2D gels. Comparison of gels from milt samples incubated in AM and SF, regardless of temperature, revealed 15 protein spots with significantly different expression (Figure 7). These spots were excised from the gels and subjected to MS identification. In this first report of burbot spermatozoa, six protein spots were identified (Table 4). Differentially expressed proteins in spermatozoa diluted in AM were radial spoke head protein 6 homolog A-like (spot 1), T-complex protein 1 subunit alpha (spot 7), T-complex protein 1 subunit gamma (spot 8), and ATP synthase subunit alpha mitochondrial (spot 10) (Table 4). Serotransferrin-like proteins (spots 4 and 5) were detected in spermatozoa diluted in SF (Table 4).
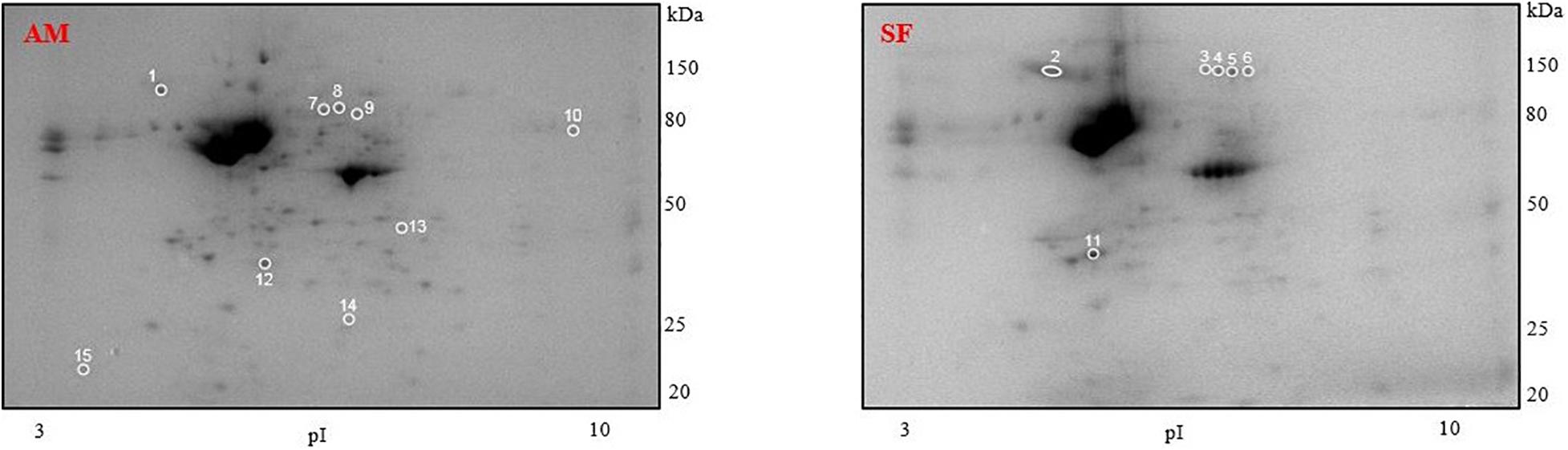
Figure 7. Representative protein profiles of L. lota spermatozoa after dilution in AM and SF. Each specimen was analyzed five times by two-dimensional gel electrophoresis. Circles show differentially expressed protein spots. Molecular weight marker is on the right. pI, isoelectric points.
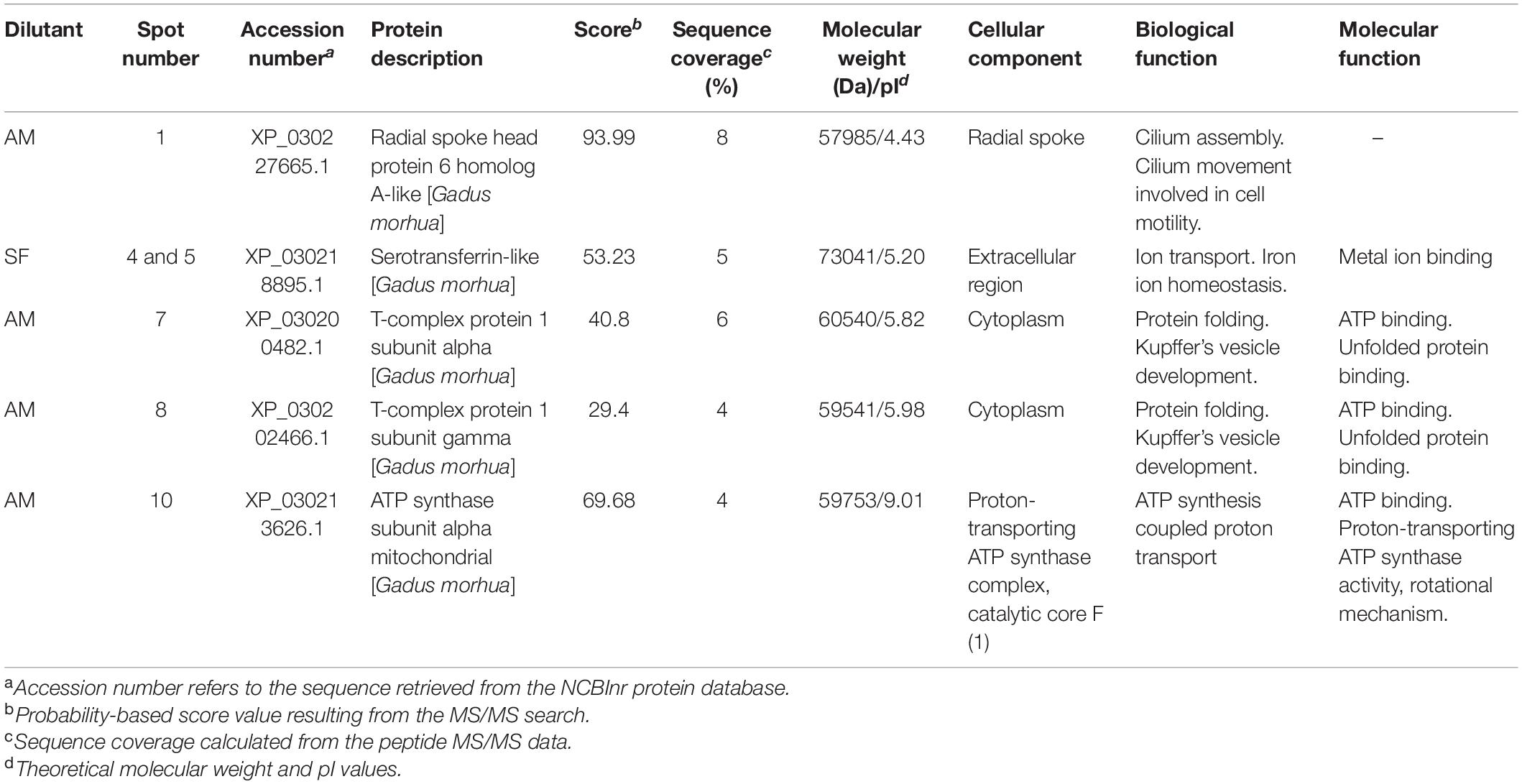
Table 4. Differentially expressed proteins in burbot spermatozoa diluted in seminal fluid (SF) and activation medium (AM).
The increased number of expressed proteins in spermatozoa diluted in AM could be attributed to leakage of intracellular proteins from spermatozoa into the surrounding medium, resulting in dynamic changes in the spermatozoa proteome (Dietrich et al., 2019). These alterations might be involved in the mechanism of the motility pathway and account for movement trajectory disturbances after dilution of spermatozoa (Inaba, 2011). Other mechanisms may be involved in the observed differences in protein profile and require further investigation.
Most reported information on the proteins detected in the present study is derived from studies of mammalian sperm. To our knowledge, this is the first report of radial spoke head protein 6 homolog A-like, T-complex protein 1 subunit alpha, T-complex protein 1 subunit gamma, ATP synthase subunit alpha mitochondrial, and serotransferrin-like in burbot spermatozoa.
Based on the present results, no difference of protein profile was observed in activated spermatozoa at high temperature (30°C), and further studies are required to elucidate the role of proteins in the spontaneous activation of burbot spermatozoa at elevated temperature without involvement of an external medium, a unique trait among fishes. The obtained results include information of several biomolecules in spermatozoa of burbot, the only gadiform freshwater fish, which represents a potential area for future research.
Conclusion
Burbot spermatozoa show increased vulnerability to osmotic pressure in extremely hypotonic media at 20 and 30°C. Osmotic shock results in spermatozoon swelling, particularly at high temperature (20 and 30°C), and a decreased swelling rate in the presence of Ca2+. Swelling, protein profile, and lipid composition do not seem to be involved in, or affected by, activation of spermatozoon motility at high temperatures. Burbot spermatozoon activation by high temperature (30°C) occurs without modification of the spermatozoon membrane, and elucidation of intrinsic signaling pathway of this phenomenon requires further study.
Data Availability Statement
The datasets presented in this study can be found in online repositories. The names of the repository/repositories and accession number(s) can be found below: The mass spectrometry proteomics data have been deposited to the ProteomeXchange Consortium via the PRIDE partner repository with the dataset identifier PXD027402.
Ethics Statement
Treatment of animals was carried out according to the authorization for the breeding and delivery of experimental animals (Reference number: 44218/2015-MZE-1721417OZ14202/2015-17214) and the authorization for the use of experimental animals (Reference number: 2293/2015-MZE-1721416OZ22302/2014-17214) issued to the Faculty of Fisheries and Protection of Waters, University of South Bohemia, by the Ministry of Agriculture of the Czech Republic.
Author Contributions
BD, HD, and SB contributed to conception and design of the study. HD, BD, SS, DL, JS, YL, VK, MX, and AS participated in the sample analysis and data curation. HD wrote the first draft of the manuscript. BD, TP, SS, DL, JS, and VK contributed to manuscript revision, read, and approved the submitted version. All authors contributed to the article and approved the submitted version.
Funding
This study was financially supported by the Ministry of Agriculture of the Czech Republic, project NAZV QK1920326, and by the Ministry of Education, Youth and Sports of the Czech Republic, projects: CENAKVA (LM2018099) and Biodiversity (CZ.02.1.01./0.0/0.0/16_025/0007370).
Conflict of Interest
The authors declare that the research was conducted in the absence of any commercial or financial relationships that could be construed as a potential conflict of interest.
Publisher’s Note
All claims expressed in this article are solely those of the authors and do not necessarily represent those of their affiliated organizations, or those of the publisher, the editors and the reviewers. Any product that may be evaluated in this article, or claim that may be made by its manufacturer, is not guaranteed or endorsed by the publisher.
Supplementary Material
The Supplementary Material for this article can be found online at: https://www.frontiersin.org/articles/10.3389/fmars.2021.736087/full#supplementary-material
Footnotes
- ^ www.thermoscientific.com
- ^ www.sony.com
- ^ https://www.olympus-lifescience.com/en/microscopes/inverted/ix83/
- ^ www.ditabis.com
- ^ www.omega-cana.com
- ^ https://imagej.nih.gov/ij/
- ^ http://tibco.com
References
Alberts, B., Johnson, A., Lewis, J., Raff, M., Roberts, P., and Walter, P. (2002). Molecular Biology of the Cell, 4th Edn. New York, NY: Garland Science, 1400.
Appelqvist, L. Å (1968). Rapid methods of lipid extraction and fatty acid methyl ester preparation for seed and leaf tissue with special remarks on preventing the accumulation of lipid contaminants. Arkiv för kemi 28, 551–570.
Babiak, I., Glogowski, J., Kujawa, R., Kucharczyk, D., and Mamcarz, A. (1998). Cryopreservation of sperm from asp Aspius aspius. Progr. Fish Cult. 60, 146–148.
Baynes, S. M., Scott, A. P., and Dawson, A. P. (1981). Rainbow trout, Salmo gairdnerii Richardson, spermatozoa: effects of cations and pH on motility. J. Fish Biol. 19, 259–267. doi: 10.1111/j.1095-8649.1981.tb05830.x
Billard, R., Cosson, J., Perchec, G., and Linhart, O. (1995). Biology of sperm and artificial reproduction in carp. Aquaculture 124, 95–112. doi: 10.1016/0044-8486(94)00231-C
Binner, M., Kloas, W., and Herdewig, I. (2008). Energy allocation in juvenile roach and burbot under different temperature and feeding regimes. Fish Physiol. Biochem. 34, 103–116. doi: 10.1007/s10695-007-9151-8
Blecha, M., Dzyuba, B., Boryshpolets, S., Horokhovatskyi, Y., Dadras, D., Malinovskyi, M., et al. (2018). Spermatozoa quality and sperm lipid composition in intensively cultured and wild burbot (Lota lota). Anim. Reprod. Sci. 198, 129–136. doi: 10.1016/j.anireprosci.2018.09.011
Bombardelli, R. A., Sanches, E. A., Baggio, D. M., Sykora, R. M., Souza, B. E., Tessaro, L., et al. (2013). Effects of the spermatozoa: oocyte ratio, water volume and water temperature on artificial fertilisation and sperm activation of cascudo-preto. Rev. Bras. de Zootec. 42, 1–6. doi: 10.1590/S1516-35982013000100001
Bondarenko, O., Dzyuba, B., Cosson, J., Yamaner, G., Prokopchuk, G., Psenicka, M., et al. (2013). Volume changes during the motility period of fish spermatozoa: interspecies differences. Theriogenology 79, 872–881. doi: 10.1016/j.theriogenology.2013.01.005
Boryshpolets, S., Dzyuba, B., Stejskal, V., and Linhart, O. (2009). Dynamics of ATP and movement in Eurasian perch (Perca fluviatilis L.) sperm in conditions of decreasing osmolality. Theriogenology 72, 851–859. doi: 10.1016/j.theriogenology.2009.06.005
Butts, I. A. E., Alavi, S. H. A., Mokdad, A., and Pitcher, T. E. (2013). Physiological functions of osmolality and calcium ions on the initiation of sperm motility and swimming performance in redside dace, Clinostomus elongatus. Comp. Biochem. Physiol. A Mol. Integr. Physiol. 166, 147–157. doi: 10.1016/j.cbpa.2013.05.011
Cosson, J. (2008). “The motility apparatus of fish spermatozoa,” in Fish Spermatology, eds S. M. H. Alavi, J. Cosson, and K. Coward (Oxford: Alfa Science), 281–316.
Cosson, J. (2010). Frenetic activation of fish spermatozoa flagella entails short-term motility, portending their precocious decadence. J. Fish Biol. 76, 240–279. doi: 10.1111/j.1095-8649.2009.02504.x
Cosson, M. P., Billard, R., Gatti, J. L., and Christen, R. (1985). Rapid and quantitative assessment of trout spermatozoa motility using stroboscopy. Aquaculture 46, 71–75. doi: 10.1016/0044-8486(85)90178-4
Dadras, H., Boryshpolets, S., Golpour, A., Policar, T., Blecha, M., and Dzyuba, M. (2019). Effects of temperature on sperm motility of burbot Lota lota: spontaneous activation and calcium dependency. J. Fish Biol. 95, 1137–1144. doi: 10.1111/jfb.14110
Dadras, H., Dzyuba, V., Cosson, J., Golpour, A., and Dzyuba, B. (2016). The in vitro effect of temperature on motility and antioxidant response of common carp Cyprinus carpio spermatozoa. J. Therm. Biol. 59, 64–68. doi: 10.1016/j.jtherbio.2016.05.003
Dietrich, M. A., Arnold, G. J., Frohlich, T., Otte, K. A., Dietrich, G. J., and Ciereszko, A. (2015). Proteomic analysis of extracellular medium of cryopreserved carp (Cyprinus carpio L.) semen. Comp. Biochem. Physiol. Part D Genomics Proteomics 15, 49–57. doi: 10.1016/j.cbd.2015.05.003
Dietrich, M. A., Nynca, J., and Ciereszko, A. (2019). Proteomic and metabolomic insights into the functions of the male reproductive system in fishes. Theriogenology 132, 182–200. doi: 10.1016/j.theriogenology.2019.04.018
Dziewulska, K., and Pilarska, M. (2018). Inhibitory effect of K+ ions and influence of other ions and osmolality on the spermatozoa motility of European burbot (Lota lota L.). PLoS One 13:e0196415. doi: 10.1371/journal.pone.0196415
Dzuba, B., and Kopeika, E. (2002). Relationship between the changes in cellular volume of fish spermatozoa and their cryoresistance. Cryo Letters 23, 353–360.
Dzuba, B. B., Bozhok, G. A., and Rudenko, S. V. (2001). A study of the dynamics of volume changes during the period of activation motility in carp. Cyprinus carpio L., spermatozoa. Aquac. Res. 32, 51–56. doi: 10.1111/j.1365-2109.2001.00527.x
Dzyuba, V., and Cosson, J. (2014). Motility of fish spermatozoa: from external signaling to flagella response. Reprod. Biol. 14, 165–175. doi: 10.1016/j.repbio.2013.12.005
Dzyuba, V., Cosson, J., Dzyuba, B., and Rodina, M. (2015). Oxidative stress and motility in tench Tinca tinca spermatozoa. Czech J. Anim. Sci. 60, 250–262. doi: 10.17221/8238-CJAS
Engel, K. M., Dzyuba, V., Ninhaus-Silveira, A., Veríssimo-Silveira, R., Dannenberger, D., Schiller, J., et al. (2020). Sperm lipid composition in early diverged fish species: internal vs. external mode of fertilization. Biomolecules 10:172. doi: 10.3390/biom10020172
Engel, K. M., Sampels, S., Dzyuba, B., Podhorec, P., Policar, T., Dannenberger, D., et al. (2019). Swimming at different temperatures: the lipid composition of sperm from three freshwater fish species determined by mass spectrometry and nuclear magnetic resonance spectroscopy. Chem. Phys. Lipids 221, 65–72. doi: 10.1016/j.chemphyslip.2019.03.014
Gracia, R. S., Bezlyepkina, N., Knorr, R. L., Lipowsky, R., and Dimova, R. (2010). Effect of cholesterol on the rigidity of saturated and unsaturated membranes: fluctuation and electrodeformation analysis of giant vesicles. Soft Matter 6:1472e82. doi: 10.1039/B920629a
Hara, A., and Radin, N. S. (1978). Lipid extraction of tissues with a low toxicity solvent. Anal. Biochem. 90, 420–426. doi: 10.1016/0003-2697(78)90046-5
Hardy, R., Paragamian, V. L., and Neufeld, M. D. (2008). Zooplankton communities and burbot relative abundance of some oligotrophic lakes of Idaho, USA and British Columbia, Canada. Am. Fish. Soc. Symp. 59, 79–89.
Inaba, K. (2011). Sperm flagella: comparative and phylogenetic perspectives of protein components. Mol. Hum. Reprod. 17:524e38. doi: 10.1093/molehr/gar034
Krasznai, Z., Marian, T., Izumi, H., Damjanovich, S., Balkay, L., Tron, L., et al. (2000). Membrane hyperpolarization removes inactivation of Ca2+ channels, leading to Ca2+ influx and subsequent initiation of sperm motility in the common carp. Proc. Natl. Acad. Sci. U.S.A. 97, 2052–2057. doi: 10.1073/pnas.040558097
Krasznai, Z., Morisawa, M., Krasznai, Z. T., Morisawa, S., Inaba, K., Bazsáné, Z. K., et al. (2003). Gadolinium, a mechano-sensitive channel blocker, inhibits osmosis-initiated motility of sea- and freshwater fish sperm, but does not affect human or ascidian sperm motility. Cell Motil. Cytoskelet. 55, 232–243. doi: 10.1002/cm.10125
Kucharczyk, D., Mamcarz, A., Skrzypczak, A., Kujawa, R., and Babiak, I. (1998). Artificial spawning of burbot (Lota lota L.) under controlled conditions. Eur. Aquac. Soc. Spec. Publ. 26, 149–150.
Lahnsteiner, F., Bergcr, R., Weismand, T., and Patzner, R. (1997). Sperm motility and seminal fluid composition in the burbot, Lota lota. J. Appl. Ichthyol. 13, 113–119. doi: 10.1111/j.1439-0426.1997.tb00110.x
Lahnsteiner, F., Berger, B., Weismann, T., and Patzner, R. A. (1995). Fine structure and motility of spermatozoa and composition of the seminal plasma in the perch. J. Fish Biol. 47, 492–508. doi: 10.1111/j.1095-8649.1995.tb01917.x
Lahnsteiner, F., Mansour, N., and Berger, B. (2004). The effect of inorganic and organic pollutants on sperm motility of some freshwater teleosts. J. Fish Biol. 65, 1283–1297. doi: 10.1111/j.0022-1112.2004.00528.x
Leahy, T., and Gadella, B. (2011). Sperm surface changes and physiological consequences induced by sperm handling and storage. Reproduction 142, 759–778. doi: 10.1530/REP-11-0310
Lenzi, A., Picardo, M., Gandini, L., and Dondero, F. (1996). Lipids of the sperm plasma membrane: from polyunsaturated fatty acids considered as markers of sperm function to possible scavenger therapy. Hum. Reprod. Update 2, 246–256. doi: 10.1093/humupd/2.3.246
Li, P., Hulak, M., Koubek, P., Sulc, M., Dzyuba, B., Boryshpolets, S., et al. (2010). Ice-age endurance: the effects of cryopreservation on proteins of sperm of common carp, Cyprinus carpio L. Theriogenology 74, 413–423. doi: 10.1016/j.theriogenology.2010.02.024
Linhart, O., Cosson, J., Mims, S. D., Shelton, W. L., and Rodina, M. (2002). Effects of ions on the motility of fresh and demembranated paddlefish (Polyodon spathula) spermatozoa. Reproduction 124, 713–719. doi: 10.1530/rep.0.1240713
Loginov, D. S., Loginova, Y. F., Dycka, F., Böttinger, K., Vechtova, P., and Sterba, J. (2019). Tissue-specific signatures in tick cell line MS profiles. Parasit. Vectors 12:212. doi: 10.1186/s13071-019-3460-5
Loir, M., Labbe, C., Maisse, G., Pinson, A., Boulard, G., Mourot, B., et al. (1990). Proteins of seminal fluid and spermatozoa in the trout (Oncorhynchus mykiss): partial characterization and variations. Fish Physiol. Biochem. 8, 485–495. doi: 10.1007/BF00003405
McPhail, J. D. A. (1997). Review of burbot (Lota lota) life history and habitat use in relation to compensation and improvement opportunities. Can. Manuscr. Rep. Fish. Aquat. Sci. 2397:37.
Morisawa, M., Suzuki, K., and Morisawa, S. (1983a). Effects of potassium and osmolality on spermatozoa motility of salmonid fishes. J. Exp. Biol. 107, 105–113.
Morisawa, M., Suzuki, K., Shimizu, H., Morisawa, S., and Yasuda, K. (1983b). Effects of osmolality and potassium on motility of spermatozoa from freshwater cyprinid fishes. J. Exp. Biol. 107, 95–103. doi: 10.1242/jeb.107.1.95
Muller, K., Muller, P., Pincemy, G., Kurz, A., and Labbe, C. (2008). Characterization of sperm plasma membrane properties after cholesterol modification: consequences for cryopreservation of rainbow trout spermatozoa. Biol. Reprod. 78, 390–399. doi: 10.1095/biolreprod.107.064253
O’Flaherty, C., and Matsushita-Fournier, D. (2017). Reactive oxygen species and protein modifications in spermatozoa. Biol. Reprod. 97, 577–585. doi: 10.1093/biolre/iox104
Olsen, R. E., and Henderson, R. J. (1989). The rapid analysis of neutral and polar marine lipids using double-development HPTLC and scanning densitometry. J. Exp. Mar. Biol. Ecol. 129, 189–197. doi: 10.1016/0022-0981(89)90056-7
Padrik, P., Hallap, T., Kaart, T., Bulitko, T., and Jaakma, Ü (2012). Relationships between the results of hypo-osmotic swelling tests, sperm motility, and fertility in Estonian Holstein dairy bulls. Czech J. Anim. Sci. 57, 490–497. doi: 10.17221/6349-CJAS
Perchec-Poupard, G., Gatti, J. L., Cosson, J., Jeulin, C., Fierville, F., and Billard, R. (1997). Effects of extracellular environment on the osmotic signal transduction involved in activation of motility of carp spermatozoa. J. Reprod. Fertil. 110, 315–327. doi: 10.1530/jrf.0.1100315
Purchase, C. F., and Earle, P. T. (2012). Modifications to the IMAGEJ computer assisted sperm analysis plugin greatly improve efficiency and fundamentally alter the scope of attainable data. J. Appl. Ichthyol. 28, 1013–1016. doi: 10.1111/jai.12070
Pustowka, C., McNiven, M. A., Richardson, G. F., and Lall, S. P. (2000). Source of dietary lipid affects sperm plasma membrane integrity and fertility in rainbow trout Oncorhynchus mykiss (Walbaum) after cryopreservation. Aquac. Res. 31, 297–305.
Rehulka, P., Zahradnikova, M., Rehulkova, H., Dvorakova, P., Nenutil, R., Valik, D., et al. (2018). Microgradient separation technique for purification and fractionation of permethylated N-glycans before mass spectrometric analyses. J. Sep. Sci. 41, 1973–1982. doi: 10.1002/jssc.201701339
Sampels, S., Pickova, J., and Wiklund, E. (2005). Influence of production system, age and sex on carcass parameters and some biochemical meat quality characteristics of reindeer (Rangifer tarandus tarandus L.). Rangifer 25, 85–96. doi: 10.7557/2.25.2.253
Scott, W. B., and Crossman, E. J. (1973). Freshwater fishes of Canada. Ottawa: Fisheries Research Board of Canada.
Shevchenko, A., Tomas, H., Havli, J., Olsen, J. V., and Mann, M. (2006). In-gel digestion for mass spectrometric characterization of proteins and proteomes. Nat. Protoc. 1, 2856–2860. doi: 10.1038/nprot.2006.468
Stoss, J. (1983). “Fish gamete preservation and spermatozoan physiology,” in Fish Physiology, eds W. S. Hoar, D. J. Randall, and E. M. Donaldson (New York, NY: Academic Press), 305–350.
Svačina, P., Příborský, J., Blecha, M., Policar, T., and Velíšek, J. (2016). Haematological and biochemical response of burbot (Lota lota L.) exposed to four different anaesthetics. Czech J. Anim. Sci. 61, 414–420. doi: 10.17221/14/2016-CJAS
Takai, H., and Morisawa, M. (1995). Change in intracellular K+ concentration caused by external osmolality change regulates sperm motility of marine and freshwater teleosts. J. Cell Sci. 108, 1175–1181. doi: 10.1242/jcs.108.3.1175
Wilson-Leedy, J. G., and Ingermann, R. L. (2007). Development of a novel CASA system based on open source software for characterization of zebrafish sperm motility parameters. Theriogenology 67, 661–672. doi: 10.1016/j.theriogenology.2006.10.003
Wilson-Leedy, J. G., Kanuga, M. K., and Ingermann, R. L. (2009). Influence of osmolality and ions on the activation and characteristics of zebrafish sperm motility. Theriogenology 71, 1054–1062. doi: 10.1016/j.theriogenology.2008.11.006
Wocher, H., Harsányi, A., and Schwarz, F. J. (2012). Larviculture of burbot (Lota lota L.): larval rearing using artemia and weaning onto dry feed. Aquac. Res. 44, 106–113. doi: 10.1111/j.1365-2109.2011.03015.x
Wojtczak, M., Calka, J., and Glogowski, J. (2007). Isolation and characterization of alpha1-proteinase inhibitor from common carp (Cyprinus carpio) seminal plasma. Comp. Biochem. Physiol. B Biochem. Mol. Biol. 148, 264–276. doi: 10.1016/j.cbpb.2007.06.004
Wojtczak, M., Dietrich, G. J., and Ciereszko, A. (2005). Transferrin and antiproteases are major proteins of common carp seminal plasma. Fish Shellfish Immunol. 19, 387–391. doi: 10.1016/j.fsi.2005.01.009
Wolnicki, J., Kamiński, R., and Myszkowski, L. (2002). Temperature-influenced growth and survival of burbot Lota lota (L.) larvae fed live food under controlled conditions. Arch. Pol. Fish. 10, 109–113.
Worthington, T., Kemp, P., Osborne, P. E., Howes, C., and Easton, K. (2010). Former distribution and decline of the burbot (Lota lota) in the UK. Aquat. Conserv. 20, 371–377. doi: 10.1002/aqc.1113
Xin, M., Vechtova, P., Shaliutina-Kolesova, A., Fussy, Z., Loginov, D., Dzyuba, B., et al. (2019). Transferrin identification in sterlet (Acipenser ruthenus) reproductive system. Animals 9:753. doi: 10.3390/ani9100753
Żarski, D., Kucharczyk, D., Sasinowski, W., Targońska, K., and Mamcarz, A. (2010). The influence of temperature on successful reproductions of burbot, Lota lota (L.) under hatchery conditions. Pol. J. Nat. Sci. 25, 93–105. doi: 10.2478/v10020-010-0007-9
Żarski, D., Sasinowski, W., Kucharczyk, D., Kwiatkowski, M., Krejszeff, S., and Targońska, K. (2009). Mass initial rearing of burbot Lota Lota (L.) larvae under controlled conditions. Pol. J. Nat. Sci. 24, 76–84. doi: 10.2478/v10020-009-0007-9
Zhelenova, E. E., Markham, P., Edgar, R., Bibi, E., Neyfakh, A. A., and Brennan, R. G. (2000). A structure-based mechanism for drug binding by multidrug transporters. Trends Biochem. Sci. 25, 39–43. doi: 10.1016/s0968-0004(99)01514-5
Zilli, L., Beirao, J., Schiavone, R., Herraez, M. P., Gnoni, A., and Vilella, S. (2014). Comparative proteome analysis of cryopreserved flagella and head plasma membrane proteins from sea bream spermatozoa: effect of antifreeze proteins. PLoS One 9:e99992. doi: 10.1371/journal.pone.0099992
Keywords: biomolecule, fish, lipid, osmolality, protein, sperm activation
Citation: Dadras H, Policar T, Loginov D, Boryshpolets S, Loginova Y, Sampels S, Sotnikov A, Kholodnyy V, Sterba J, Xin M and Dzyuba B (2021) Relationship of Motility Activation to Lipid Composition, Protein Profile, and Swelling Rate of Burbot Lota lota Spermatozoon Following Change of Temperature and Osmolality. Front. Mar. Sci. 8:736087. doi: 10.3389/fmars.2021.736087
Received: 04 July 2021; Accepted: 30 September 2021;
Published: 21 October 2021.
Edited by:
Jorge G. Farias, University of La Frontera, ChileReviewed by:
Neeraj Kumar, National Institute of Abiotic Stress Management (ICAR), IndiaBrad Buckley, Portland State University, United States
Copyright © 2021 Dadras, Policar, Loginov, Boryshpolets, Loginova, Sampels, Sotnikov, Kholodnyy, Sterba, Xin and Dzyuba. This is an open-access article distributed under the terms of the Creative Commons Attribution License (CC BY). The use, distribution or reproduction in other forums is permitted, provided the original author(s) and the copyright owner(s) are credited and that the original publication in this journal is cited, in accordance with accepted academic practice. No use, distribution or reproduction is permitted which does not comply with these terms.
*Correspondence: Hadiseh Dadras, dadras@frov.jcu.cz; fh.dadras@gmail.com